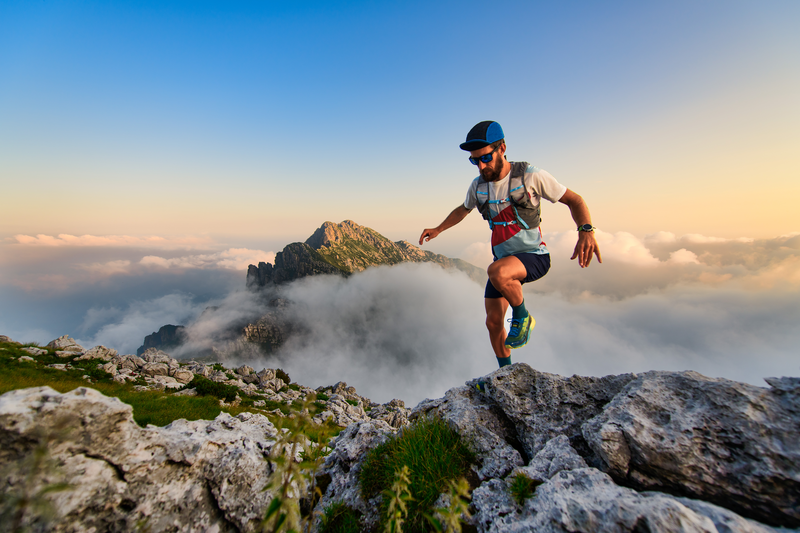
95% of researchers rate our articles as excellent or good
Learn more about the work of our research integrity team to safeguard the quality of each article we publish.
Find out more
ORIGINAL RESEARCH article
Front. Microbiol. , 12 January 2022
Sec. Antimicrobials, Resistance and Chemotherapy
Volume 12 - 2021 | https://doi.org/10.3389/fmicb.2021.782363
This article is part of the Research Topic Metal Resistance in Microorganisms View all 12 articles
Metallothioneins (MTs) are cysteine-rich, metal-sequestering cytosolic proteins that play a key role in maintaining metal homeostasis and detoxification. We had previously characterized NmtA, a MT from the heterocystous, nitrogen-fixing cyanobacterium Anabaena sp. strain PCC 7120 and demonstrated its role in providing protection against cadmium toxicity. In this study, we illustrate the regulation of Anabaena NmtA by AzuR (Alr0831) belonging to the SmtB/ArsR family of transcriptional repressors. There is currently no experimental evidence for any functional role of AzuR. It is observed that azuR is located within the znuABC operon but in the opposite orientation and remotely away from the nmtA locus. Sequence analysis of AzuR revealed a high degree of sequence identity with Synechococcus SmtB and a distinct α5 metal binding site similar to that of SmtB. In order to characterize AzuR, we overexpressed it in Escherichia coli and purified it by chitin affinity chromatography. Far-UV circular dichroism spectroscopy indicated that the recombinant AzuR protein possessed a properly folded structure. Glutaraldehyde cross-linking and size-exclusion chromatography revealed that AzuR exists as a dimer of ∼28 kDa in solution. Analysis of its putative promoter region [100 bp upstream of nmtA open reading frame (ORF)] identified the presence of a 12–2–12 imperfect inverted repeat as the cis-acting element important for repressor binding. Electrophoretic mobility shift assays (EMSAs) showed concentration-dependent binding of recombinant dimeric AzuR with the promoter indicating that NmtA is indeed a regulatory target of AzuR. Binding of AzuR to DNA was disrupted in the presence of metal ions like Zn2+, Cd2+, Cu2+, Co2+, Ni2+, Pb2+, and Mn2+. The metal-dependent dissociation of protein–DNA complexes suggested the negative regulation of metal-inducible nmtA expression by AzuR. Overexpression of azuR in its native strain Anabaena 7120 enhanced the susceptibility to cadmium stress significantly. Overall, we propose a negative regulation of Anabaena MT by an α5 SmtB/ArsR metalloregulator AzuR.
Trace metal ions are crucial for nearly all aspects of metabolism in the prokaryotic cells. These are involved in various biological processes like enzymatic reactions that require metal ions as cofactors, for folding and structural stabilization of the proteins or for the maintenance of the metal-sensing regulatory factors (Rees, 2002; Bertini et al., 2007; Chandrangsu et al., 2017). Although the essential metal ions are indispensable, these are toxic in excess amounts (Chandrangsu et al., 2017). As a result, the microorganisms have developed mechanisms to regulate the homeostasis of the essential metal ions. Metal homeostasis is mediated by balancing the uptake, storage, transfer, and efflux of the metals so that the cellular requirements are fulfilled and the right metal is introduced into the right macromolecule in the cells for various biological processes (Tottey et al., 2005; Waldron and Robinson, 2009).
Metallothioneins (MTs) are cysteine-rich, low-molecular-weight, metal-sequestering proteins that are known to bind metal ions via metal–thiolate clusters and are involved in maintaining homeostasis of physiologically important metals like zinc (Zn2+) and copper (Cu2+) (Klaassen et al., 1999; Blindauer, 2011). Apart from binding to the essential metals, MTs are implicated in the detoxification of toxic metals including cadmium (Cd2+) and mercury (Hg2+) from the cells (Klaassen et al., 1999). MTs are induced in the presence of ionic species of various metals like Cd, Zn, Cu, Hg, Au, Ag, Co, Bi, Pb, Ni, and Cr (Palmiter, 1987; Huckle et al., 1993) as well as oxidative stress (Andrews, 2000). MT expression is strictly regulated owing to its role in maintaining metal homeostasis. While eukaryotic MT gene expression has been shown to be under positive regulation (Klaassen et al., 1999), prokaryotic MT expression is proposed to be negatively regulated (Turner and Robinson, 1995). The first characterized prokaryotic MT is Synechococcus sp. SmtA (Blindauer and Leszczyszyn, 2010). The smtA gene expression is negatively regulated by a zinc responsive transcriptional repressor SmtB (Erbe et al., 1995; Turner et al., 1996) of the SmtB/ArsR family of transcriptional regulatory proteins. The SmtB/ArsR family of proteins bind to specific regulatory sequences present upstream of the gene. Derepression of transcription by such regulators results from direct binding of the metal to the repressor, which inhibits its binding to the operator/promoter (O/P) region of the gene under regulation (Busenlehner et al., 2003; Osman and Cavet, 2010).
Analysis of the genome sequence of Anabaena PCC 7120 (hereby referred as Anabaena 7120) revealed two SmtB-like repressors of the SmtB/ArsR family, namely, (a) AztR (All7621) and (b) AzuR (Alr0831) (Liu et al., 2005). AztR has been identified as a Zn2+/Pb2+/Cd2+-responsive metalloregulator constituting a Zn2+/Pb2+/Cd2+ efflux operon (aztAR operon) regulating AztA, a Zn2+-translocating CPx-ATPase (Liu et al., 2005, 2008). However, presently, there is no experimental evidence toward the functionality and regulation of the other repressor, AzuR in Anabaena 7120, that shares 60% identity with SmtB (Figure 1A). Previously, we had identified and characterized a MT from the heterocystous, filamentous cyanobacterium Anabaena 7120 (also belonging to the BmtA family) referred to as NmtA. Overexpression of NmtA in its native strain conferred tolerance to cadmium stress (Divya et al., 2018). We had observed increased abundance of the nmtA transcripts in the presence of elevated concentrations of metal ions like Zn2+, Cu2+, and Cd2+ (Divya et al., 2018), indicating transcriptional regulation of nmtA expression. It is proposed that the expression of the proteins associated with metal homeostasis is largely regulated at the transcriptional level in bacteria (Finney and O’Halloran, 2003). It is, therefore, worthwhile to explore whether AzuR, which is an SmtB-like repressor, has any role in the regulation of NmtA expression in Anabaena 7120.
Figure 1. Sequence analysis, structural modeling, phylogeny, and genomic organization of AzuR. (A) Multiple sequence alignment of AzuR with representative SmtB/ArsR family transcriptional repressors, SmtB (P30340), AztR (Q8ZS91), ZiaR (P9WMI4), BxmR (Q55940), CzrA (O85142), and CadC (P20047). The metal-sensing amino acids present in the α5 site are highlighted in purple, and the residues present in the α3N site are indicated in orange. (B) Structure of AzuR. (i) The tertiary structure model of AzuR was generated by using SmtB as the template by I-TASSER with the overlap of SmtB (PDB ID: 1R23) shown as traces in purple. Ligand (Zn2+) binding residues predicted by I-TASSER with (ii) Staphylococcus aureus CadC (PDB ID: 1U2W) and (iii) Synechococcus PCC 7942 SmtB (PDB ID: 1R22). Zn2+ is shown in neon green and the metal-binding residues are indicated in blue. (C) Phylogenetic tree generated with representative sequences aligned by Clustal Omega using MEGA X software with 500 bootstrap replicates. The tree is drawn to scale, with branch lengths measured in the number of substitutions per site. (D) Schematic representation of the genetic locus of azuR ORF with respect to nmtA ORF in the Anabaena genome.
The present study provides a comprehensive characterization of Anabaena AzuR (Alr0831). We show here that AzuR indeed binds to the upstream region of the nmtA open reading frame (ORF). DNA binding was repressed in the presence of various divalent metal ions, indicating a negative regulation of nmtA expression by AzuR. Our results showed that overexpression of azuR in Anabaena enhanced the susceptibility of the recombinant strain to cadmium stress significantly. The present investigation advances our understanding of the mechanisms of metal-regulated gene expression in the nitrogen-fixing cyanobacterium Anabaena 7120.
Anabaena 7120 cultures were grown in BG-11 liquid medium, pH 7.2, with combined nitrogen (17 mM NaNO3) under continuous illumination (30 μEm–2 s–1) without or with shaking (100 rpm) at 27°C ± 2°C (Allen, 1968). Escherichia coli cultures were grown in Luria–Bertani (LB) medium at 37°C (DH5α, HB101) or 30°C (SHuffle) with shaking at 120 rpm. The neomycin antibiotic was used for recombinant Anabaena cultures in BG-11 liquid medium (15 μg ml–1) or BG-11 agar plates (25 μg ml–1), whereas chloramphenicol (34 μg ml–1) or carbenicillin (100 μg ml–1) was used for E. coli cultures. Primers, plasmids, E. coli, and Anabaena strains used in this study are listed in Table 1.
Alignment of DNA and protein sequences was determined using ClustalW (Thompson et al., 1994) and Clustal Omega (Madeira et al., 2019), respectively. Jalview was used to visualize and edit aligned protein sequences (Waterhouse et al., 2009). A phylogenetic tree was constructed using MEGA version X (Kumar et al., 2018) by the maximum likelihood method. The I-TASSER software was used to predict the tertiary structure of AzuR and metal-binding residues (Zhang, 2008; Roy et al., 2010; Yang et al., 2015). Pattern search analysis of conserved sequences was carried out using the online tool Pattern Locator (Mrázek and Xie, 2006). The −10 and −35 boxes of the upstream region of nmtA were predicted from BPROM (Salamov and Solovyevand, 2011).
The azuR ORF (363 bp) was PCR amplified from Anabaena 7120 genomic DNA and cloned into pTwin1 vector at NdeI–SapI sites. The resulting construct pTwinazuR was confirmed by sequencing and transformed into an E. coli SHuffle strain. Overexpression of chitin-binding domain (CBD)-tagged AzuR was induced by the addition of 0.5 mM IPTG. The protein purification was carried out by chitin affinity chromatography as per the manufacturer’s protocol (New England Biolabs). The protein was cleaved from its tag and eluted following incubation with 40 mM DTT at 4°C for 3 days. CBD was also eluted as the contaminating protein. This eluate was loaded onto the fresh chitin resin after DTT removal. The flow-through was collected, which contained purified Anabaena AzuR without CBD. The purified protein band following electrophoresis on 15% SDS-PAGE was excised and processed for LC-MS/MS analysis (Q Exactive Plus BioPharma High-Resolution Orbitrap MS system, Thermo Fischer Scientific) at the Sophisticated Analytical Instrument Facility (SAIF), IIT Bombay, India. Spectrum was acquired in positive ion mode in a mass range from 350 to 2,000 m/z. The resultant spectrum was used for peptide identification using the Anabaena 7120 protein database available at UniProt.
Determination of the oligomeric status of AzuR was done by glutaraldehyde cross-linking of protein in the native state. Purified AzuR was incubated with 10 mM glutaraldehyde at room temperature (RT) for 10–15 min in 10 mM Tris, pH 7.5. To this, a cracking buffer without or with DTT (50 mM) was added. The resulting cross-linked protein was analyzed by 15% SDS-PAGE. The native molecular mass of AzuR was determined by size-exclusion chromatography (AKTA FPLC system, GE Healthcare) using the GE Superdex 75 column equilibrated with 20 mM Tris, 100 mM NaCl, pH 7.5 at 25°C at a flow rate of 0.5 ml min–1. The column was previously calibrated using a set of gel filtration markers [bovine serum albumin (66 kDa), ovalbumin (44 kDa), carbonic anhydrase (44.3 kDa), and cytochrome c (29 kDa)] (GE Healthcare).
Analysis of the secondary structure of AzuR was performed by circular dichroism (CD) spectroscopy (MOS-500 Biologic CD spectrometer equipped with a Peltier-type thermostatic cell holder) at 25°C. The CD spectrum was recorded in the wavelength range of 200–260 nm using a cuvette with a path length cell of 0.1 mm. The samples were prepared in 10 mM Tris buffer, pH 7.5. The alpha helical content was calculated using the online tool K2D2 (Perez-Iratxeta and Andrade-Navarro, 2008). CD spectra were also recorded for titrations of AzuR with increasing concentrations of zinc (molar equivalents ranging from 1 to 10).
Total RNA was isolated from Anabaena 7120 treated with 10 μM cadmium for 1 h as described earlier (Divya et al., 2018). cDNA was synthesized with 0.5 μg of total RNA using ReadyScript cDNA Synthesis Mix (Sigma-Aldrich). Following dA tailing of cDNA by terminal transferase (Roche), PCR was performed with the oligo(dT)-anchor primer and nmtA primer as listed in Table 1. The PCR product was then sequenced.
The putative promoter region (100 bp DNA sequence upstream of nmtA ORF) was PCR amplified (primers listed in Table 1) and end-labeled with DIG-ddUTP as per manufacturer’s instructions (Roche). Two nanograms of a DIG-labeled probe (PnmtA) was incubated with various concentrations of AzuR protein in a total reaction volume of 20 μl containing 20 mM Tris–Cl (pH 7.5) and 1 mM EDTA at RT for 30 min. The DNA–protein complexes were resolved on 10% native PAGE in 0.5× TBE. Separated complexes were electroblotted onto a nylon membrane, cross-linked with UV, and stored at 4°C. It was probed with an anti-DIG antibody and developed using a colorimetric substrate, NBT-BCIP, according to the manufacturer’s protocol (DIG High Prime DNA Labeling and Detection Starter Kit I, Roche). The bands were quantified by the ImageJ software, and the data were fitted to Hill’s equation. Each experiment was repeated three times. In order to evaluate the specificity of interaction of the DNA-AzuR protein binding, electrophoretic mobility shift assay (EMSA) was performed with 100 ng of AzuR (360 nM) with either 20 ng of PnmtA or 20 ng of non-specific DNA (nmtA gene). For protein specificity, 20 ng of PnmtA with non-specific proteins like AnLexA, BSA, or NmtA, each at 360 nM concentration, was taken for EMSA. The DNA–protein complexes were resolved on 10% native PAGE and visualized by ethidium bromide staining. To evaluate whether different divalent metal ions affect the binding of AzuR to the target 100 bp DNA, EMSAs were carried out in the presence of 100 μM of various metals. The metal salts used in the study were ZnSO4.7H2O, CdCl2.1/2H2O, CuSO4.5H2O, Co(NO3)2.6H2O, NiSO4.7H2O, MnCl2.4H2O, and Pb(NO3)2. EMSAs were also carried out in the presence of 1 mM DTT (Erbe et al., 1995) for reactions containing all the aforesaid metals.
Overexpression of azuR gene in its native strain was achieved by triparental conjugation (Divya et al., 2018). The azuR gene was cloned downstream to the light-inducible psbA1 promoter in the pFPN vector at NdeI and BamHI sites. A SalI–XmaI fragment from pFPNazuR was excised and cloned into the E. coli/Anabaena shuttle vector pAM1956 upstream of the promoterless gfpmut2 gene. pAMazuR was then transferred into Anabaena 7120. The recombinant Anabaena strain was designated as AnazuR+. In a similar way, AnnmtA+ (Anabaena strain overexpressing NmtA) was also generated. AnpsbA+ (Anabaena harboring pAM1956 with constitutive expression of GFP) was generated by excising the PpsbA1 fragment from the pFPN vector and cloning it into the vector pAM1956 upstream of the promoterless gfpmut2 gene and transferred conjugally into Anabaena 7120. The recombinant Anabaena strains were repeatedly subcultured and maintained under the selective pressure of neomycin (Nm15). Visualization of GFP fluorescence in the recombinant cells confirmed the expression of the azuR gene placed upstream of the gfpmut2 gene.
For RT-PCR, 1 μg RNA was used for cDNA synthesis (ReadyScript cDNA Synthesis Mix, Sigma-Aldrich). RT-PCR was carried out with azuR-specific primers (Table 1) with 16S rRNA serving as the internal control. RT-PCR products were resolved by electrophoresis on 1% agarose gel and detected by staining with ethidium bromide. For quantification of nmtA transcripts, real-time PCR was performed with nmtA-specific primers in Qiagen rotor-Gene Q real-time PCR cycler. 16S rRNA was used as the internal control.
Exponential phase cultures (3-day-old cultures) of AnpsbA+, AnnmtA+, and AnazuR+ were inoculated in BG-11 N+ (Nm15) liquid medium at a chlorophyll a (Chla) density of ∼4 μg ml–1 and incubated for 10 days under illumination without or with cadmium at 10 and 20 μM concentrations. Growth was assessed by measuring Chla content at regular intervals. For spot assays, exponentially growing cultures of AnpsbA+, AnnmtA+, and AnazuR+ were spotted onto BG-11 N+ (Nm25) agar plates without or with cadmium (10, 20, and 40 μM) at the chlorophyll density mentioned in the figure and incubated under continuous illumination for 7 days.
Bright-light and fluorescence microscopy (FM) images were taken at ×600/×1,500 magnification on a Carl Zeiss Axioscope 40 microscope with a charge coupled device (CCD) AxioCam MRc camera (Zeiss). Green fluorescence of GFP was visualized using a Hg-arc lamp (excitation BP: 450–490 nm, emission LP: 515 nm). Chla fluorescence of Anabaena was visualized with green light excitation (excitation BP: 546/12, emission LP: 590 nm). It should be noted here that the microscopic settings for GFP fluorescence used the emission filter (λemission: 515 nm) that could detect both GFP and Chla fluorescence. For scanning electron microscopy (SEM), exponential-phase cells of WT, AnpsbA+, AnnmtA+, and AnazuR+ were harvested by centrifugation, and the resulting cell pellets were washed with 0.9% NaCl and fixed with 2.5% glutaraldehyde at 4°C for 1–2 h. Post fixation, the cells were serially dehydrated in 20, 30, 50, 70, 90, and 100% ethanol. The dehydrated sample was then gold coated with a sputtering device (Q 150R ES, Quorum) and visualized using SEM (EVO 18 Research, Carl Zeiss, United Kingdom).
Growth experiments were repeated three times. Average values with standard deviations are shown for a representative experiment. For determination of cell size, data are represented as average values ± standard deviation. One-way ANOVA was employed for calculating the significance of the difference in cell size between WT, AnpsbA+, AnnmtA+, and AnazuR+ cultures.
The genome of Anabaena PCC 7120 harbors two proteins belonging to the ArsR-SmtB family of proteins, All7621 and Alr0831. The ArsR-SmtB family of transcriptional metalloregulators represses the expression of genes/operons involved in maintaining metal homeostasis or toxic metal detoxification (Osman and Cavet, 2010). Among the 15 characterized metal binding motifs (Saha et al., 2017), the metal-sensing members of the regulators include two structurally diverse metal-binding sites, namely, α3N, and α5 (Busenlehner et al., 2003). All7621 in Anabaena 7120 encodes for AztR, a regulator of AztA [Zn(II)/Pb(II) CPx-ATPase efflux pump] (Liu et al., 2005), and belongs to the α3N group of proteins. The α3N site consists of cysteine thiolate ligands—two from the α3 helix with signature motifs Cx1–2C or Cx2CD and one or two cysteine ligands derived from the amino-terminus (Saha et al., 2017). The sequence analysis of the yet-uncharacterized Alr0831 (AzuR) revealed the absence of a functional α3N site in AzuR as it contained only one cysteine residue each in the α3 helix and at the amino-terminus (Figure 1A). Protein sequence alignment of AzuR with the Synechococcus transcriptional repressor SmtB showed 60% sequence identity, and the key amino acids in the α5 site important for metal sensing, i.e., His, Glu, and Asp in SmtB (VanZile et al., 2000, 2002), were found to be conserved in AzuR (Figure 1A). It is likely that the function of AzuR is similar to that of SmtB owing to the high degree of sequence identity. Tertiary structure prediction of AzuR using the software I-TASSER showed the presence of all the secondary structural folds (α1–α5, β1, and β2) similar to that of SmtB (Figure 1B, i). Structural modeling predicted zinc-binding residues Asp102 and His104 (Figure 1B, ii) of AzuR comparable to that of Staphylococcus aureus CadC as well as His115 and Glu118 (Figure 1B, iii) similar to that of Synechococcus SmtB. Hence, AzuR could possibly be grouped into α5 SmtB/ArsR metalloregulators with the signature motif DxHx10Hx2E present in the α5 helix (Figure 1A). Phylogenetic analysis of representative sequences from SmtB/ArsR family members showed that AzuR shared maximum identity to BxmR (67%), which contain both α3N and α5 sites (Figure 1C). It also showed that SmtB (α5) and proteins belonging to different groups—ZiaR (α3N, α5), AztR (α3N), BxmR (α3N, α5), and AzuR (α5)—evolved independently but were linked to a common ancestor (Figure 1C).
Several metal-responsive proteins and their repressors of SmtB/ArsR family members have been shown to exist as operons. For example, BmtA (MT of Oscillatoria brevis) and its repressor BxmR (Liu et al., 2004), ZiaA (Zn efflux protein of Synechocystis PCC 6803) and its repressor ZiaR (Thelwell et al., 1998), and AztA (Zn2+-translocating CPx-ATPase) and its repressor AztR (Liu et al., 2005) are organized in operons. In Synechococcus PCC 7942, the smtB gene and smtA gene are separated by 100 bp, forming a divergon (Huckle et al., 1993). However, there is a deviation in the genetic organization of Anabaena MT, which is not organized in an operon. The nmtA ORF (located between positions 3938083 and 3937925) is present within a larger ORF of an unknown protein, asr3266, but in the opposite orientation (Bose et al., 2006). Similarly, the putative regulator azuR is not placed adjacent to the nmtA locus but is present within the ZnuABC operon (Figure 1D). Alr0831 is positioned at 956795→957157 between alr0830 (ZnuC, ABC transporter permease protein) and alr0832 (ZnuA, ABC transporter ATP binding protein) in the opposite orientation. Similar to AzuR, the SmtB ortholog has been identified within an operon with an ABC-type transporter system in other cyanobacteria like Nodularia and Anabaena variabilis (Blindauer, 2008). Analysis of the genomic organization of other prokaryotic MTs like Pseudomonas MT also revealed an absence of regulatory protein adjacent to the Pseudomonas fluorescens Q2-87 MT locus. Also, the genes adjacent to the Pseudomonas MT gene code for proteins of unknown function (Habjanič et al., 2020). Genomic arrangement of MT and its regulator as operons apparently is not mandatory as such regulators function as trans-acting factors on cis-regulatory elements.
To characterize the regulatory role of AzuR, the corresponding gene (alr0831) was cloned in the pTwin1 vector. The resulting construct pTwinazuR was expressed in the E. coli SHuffle strain. Induction with IPTG expressed a ∼42 kDa protein corresponding to CBD-tagged AzuR (Figure 2A). The cloning at NdeI–SapI sites ensured that no extra amino acids were incorporated in the purified protein following removal of the tag. AzuR was purified by chitin affinity chromatography, and the removal of the CBD tag was achieved by thiol-induced cleavage with 40 mM DTT at 4°C. The purified AzuR was visualized on SDS-PAGE as a monomer under reducing conditions with a molecular weight of ∼13.9 kDa (Figure 2B), which was further confirmed with LC-MS/MS analysis. The MS analysis identified six unique peptides, showing 77% coverage of the Anabaena AzuR protein sequence.
Figure 2. Overexpression and purification of AzuR. (A) Overexpression of AzuR. Whole-cell protein extracts (30 μg) of uninduced (UI) and induced (I) with 0.5 mM IPTG from E. coli SHuffle (pTwinazuR) cells were resolved on 15% SDS-PAGE, followed by visualization with Coomassie Brilliant Blue (CBB) staining. The lane marked as M is the protein molecular weight marker (NEB P7712). (B) Purification of AzuR (Alr0831). AzuR was purified by chitin affinity chromatography followed by thiol-mediated removal of the CBD tag. The purified AzuR protein corresponding to the monomer under reducing conditions on 15% SDS-PAGE is indicated by the arrow. The molecular mass in kDa is indicated on the left-hand side. The lane marked as M is the protein molecular weight marker (NEB P7712). (C) Cross-linking of AzuR with glutaraldehyde. The purified AzuR (5 μg) was cross-linked with glutaraldehyde (Glh) without or with the addition of DTT (50 mM) in the Laemmli buffer. The proteins were separated on 15% SDS-PAGE followed by staining with Coomassie Brilliant Blue. (D) Size-exclusion chromatography profile of the purified AzuR protein using Superdex 75. The calibration curve of standard proteins is shown in the inset. The calibration equation, y = –0.195x + 7.0463 (R2 = 0.992), was used for the molecular weight calculation of AzuR. (E) CD spectrum of purified AzuR showing 67.5% α helical content. Gray circles represent elution volume corresponding to different fractions and blue circles represent the standard molecular weight markers used.
The SmtB/ArsR family of proteins binds to the regulatory DNA sequences as homodimers (Osman and Cavet, 2010). To ascertain the native form of AzuR, the oligomeric status of AzuR was evaluated by glutaraldehyde cross-linking. The protein was predominantly found to be present in the dimeric state as observed by glutaraldehyde cross-linking (Figure 2C). The dimeric state was also confirmed with size-exclusion chromatography (Figure 2D). This is in agreement with the previously characterized SmtB/ArsR family of prokaryotic metalloregulatory transcriptional repressors that existed as stable dimers in solution (Busenlehner et al., 2001; Liu et al., 2005, 2008). It was observed that AzuR existed as a monomer under reducing conditions and dimer under non-reducing conditions (Figure 2C). These observations suggested the involvement of cysteine residues in AzuR dimerization. Secondary structure analysis by CD showed that AzuR is composed of 67.5% α helical content (Figure 2E), suggesting that the purified recombinant AzuR protein was properly folded. This is in agreement with the theoretical secondary structure prediction of AzuR using the SOPMA software, which projected 67% α helical content followed by 17% random coil and 11% extended strand.
The SmtB/ArsR family of transcriptional regulators binds to 12–2–12 inverted repeats present upstream or within the genes that they regulate (Erbe et al., 1995; Turner et al., 1996). RACE analysis with total RNA isolated from the cadmium-treated (IC50 10 μM) Anabaena 7120 showed an ∼200 bp cDNA product (Figure 3A). Sequence analysis of the product identified the transcriptional start site (TSS) to be at 23 nt upstream of the translational start of the nmtA ORF (Figure 3B). The palindromic sequence (12–2–12 imperfect inverted repeat), corresponding to the consensus of the α3N and α5 groups of SmtB/ArsR-binding sites (Saha et al., 2017), was found to be located 36 nt upstream of the nmtA translation start site (Figure 3B). Its position overlaps with the theoretical prediction of the −35 element of the promoter. It is shown that the cis-regulatory element of metal-inducible operons is composed of one or two inverted 12–2–12 repeats present in the vicinity or overlapping the transcriptional start site of the gene under regulation. For example, one of the two such inverted 12–2–12 repeats found in Synechococcus 7942 was essential for the regulation of smtA expression by its repressor, SmtB (Turner et al., 1996). Similarly, the Synechocystis zia O/P region has a single 12–2–12 inverted repeat between the −10 box and the translational start site of ziaA, which is regulated by a divergently transcribed repressor, ziaR (Thelwell et al., 1998). Pattern search analysis was performed with the conserved bases in the 12–2–12 imperfect repeat along the entire Anabaena 7120 genome. Similar repeats were found at sites upstream and within other genes that include all1178, which codes for a two-component hybrid sensor and regulator, alr7622 (also designated as aztA), encoding for cation-transporting ATPase and other hypothetical proteins (Table 2). The conserved 12–2–12 inverted repeat of SmtB/ArsR-regulated O/Ps are shown in Figure 3C. Although nmtA and its putative regulator azuR do not constitute an operon in Anabaena 7120, the inverted 12–2–12 imperfect repeat could be located at the appropriate upstream distance from the nmtA translation start site. Although the azuR ORF is present within the znuABC operon, a detailed search for conserved bases in the 12–2–12 imperfect repeat following global search analysis by PATLOC in the close vicinity of the znuABC operon (corresponding to the 500 bp upstream region to 500 bp downstream of the operon) and within the operon did not show any such repeat sequence in the entire analyzed region. The regulation of the znuABC operon by the zur (all2473)/furB regulator has been demonstrated previously in Anabaena 7120 (Napolitano et al., 2012). Zur (zinc uptake regulator), known to be the master regulator for zinc homeostasis in Anabaena 7120, regulated the expression of genes involved in zinc homeostasis like alr0830 (ZnuC), alr0833 (ZnuA), and all7621 (AztR). On analysis, we did not find zur-binding sequences upstream of the azuR ORF, indicating that the global regulator of zinc homeostasis, Zur, did not regulate azuR expression.
Figure 3. Mapping of transcriptional start site and inverted repeats. (A) Mapping of transcriptional start site by RACE was performed with RNA isolated from Anabaena cells treated with cadmium. The RACE product is indicated by an arrow. M, 100 bp DNA ladder (NEB). (B) Sequence analysis of the nmtA ORF and upstream region. The inverted repeat sequence is highlighted in green; the red A is the transcriptional start site (TSS); the sequences highlighted in pink and blue represent the –10-like box and –35 box, respectively; and the start codon ATG is highlighted in brown. (C) Sequence alignment of inverted repeat present upstream of the nmtA ORF with other characterized repeats essential for repressor binding by ClustalW. An asterisk (∗) indicates the conserved bases across all sequences.
Table 2. Anabaena genes possessing conserved sequences in the 12–2–12 inverted repeat identified by PATLOC.
Anabaena 7120 AztA is transcriptionally regulated by AztR (belonging to the SmtB/ArsR family) by recognizing and binding to the inverted 12–2–12 imperfect repeat region. EMSA studies done with AztR and the nmtA/bmtA upstream region showed its binding in vitro (Tottey et al., 2007). Similar inverted repeat sequences identified by AztR and AzuR indicate that AztR and AzuR might be sharing the function of regulating AztA and NmtA. As described above, AztR belongs to the α3N group and AzuR to the α5 group of the SmtB/ArsR family. The α5 group members sense physiologically important metals like Zn2+, Cu2+, Co2+, and Ni2+, while the α3N group prefers larger, more thiophilic metal ions like Cd2+ or Pb2+ (Busenlehner et al., 2003). It is possible that AzuR and AztR preferred different groups of metal ions but could regulate both MT and efflux proteins, thus enabling the cell to respond to a wide range of metal ions.
Electrophoretic mobility shift assays (EMSAs) were done in order to identify the AzuR-DNA binding site using a 100 bp fragment (PnmtA) upstream of the nmtA gene (probe) containing the 12–2–12 inverted repeat sequence. The results showed that AzuR could bind and form complexes with PnmtA in a concentration-dependent manner (Figure 4A). The Hill coefficient of AzuR binding to DNA was calculated to be 2.48 ± 1.14 (>1) (Figure 4B), which indicated positive cooperative binding (Hill, 1910). SmtB has been shown to bind to the smt O/P in a multimeric state (Erbe et al., 1995). The positive cooperative binding suggested that AzuR bound to the target DNA as an oligomer similar to that of SmtB. The specificity of DNA–protein binding was confirmed by using the nmtA gene or DNA-binding protein LexA from Anabaena 7120 (AnLexA) or other proteins like BSA and NmtA. No retardation in the mobility of PnmtA was observed in the presence of AnLexA. Also, AzuR could not bind to the nmtA gene sequence, confirming that AzuR regulated nmtA expression by binding to the upstream sequence and not to its internal region (Figure 4C). Our results established the specific binding of PnmtA with the AzuR protein.
Figure 4. Binding of AzuR to the upstream region of nmtA. (A) Electrophoretic mobility shift assay (EMSA) of DIG-labeled 100 bp DNA sequence upstream of nmtA (2 ng) with purified AzuR. Different concentrations of AzuR protein were incubated with DIG-labeled DNA, and the assay mixtures were resolved on 10% native PAGE in 0.5× TBE. Detection with the DIG-labeled probe was carried out as per manufacturer’s protocol (Roche) using NBT-BCIP. Lane 1 contains 2 ng of PnmtA. Lanes 2–8 contain increasing concentrations of AzuR as indicated. Representative data from three independent experiments are shown. (B) Representative plot showing the percentage of bound complex against the concentration of AzuR protein fitted to the Hill equation. (C) EMSA for evaluation of non-specific interaction of DNA–protein binding. Lane 1: 100 bp DNA ladder. Lane 2: 20 ng of 100 bp PnmtA only or with 360 nM (100 ng) AzuR (lane 3) or 360 nM (478 ng) BSA (lane 4) or 360 nM (41 ng) NmtA (lane 5) or 360 nM (158 ng) AnLexA (lane 6). Lane 7 contains 20 ng of the nmtA gene only or with 360 nM (100 ng) AzuR (lane 8). The DNA–protein complexes were resolved on 10% native PAGE and visualized by ethidium bromide staining. (D) The CD spectrum of AzuR was recorded with increasing molar equivalents of zinc, which was an average of three scans. (E) EMSA of PnmtA with AzuR in the presence of Zn2+. Lane 1 contains 2 ng of PnmtA. Lanes 2–7 contain DNA with 100 ng of AzuR in the presence of increasing concentrations of Zn2+ as indicated. EMSA of PnmtA with AzuR protein with Zn2+ and Cd2+ in the absence of DTT (F) or in the presence of 1 mM DTT (G). Lane 1 contains 2 ng of probe only. Lanes 2–8 contain PnmtA with 100 ng of AzuR, lanes 3–5 with increasing concentrations of Zn2+, and lanes 6–8 with increasing concentrations of Cd2+ as indicated in (F,G). (H) EMSA of DNA probe with 70 ng of AzuR with 100 μM of divalent cations as indicated in the figure. All the reactions were performed in the absence of DTT.
SmtB senses metal ions through the α5 site. Zinc binding to residues present in this site allosterically regulates the DNA binding activity of SmtB to the smtA O/P region (VanZile et al., 2002) similar to other reported SmtB/ArsR repressors (Busenlehner et al., 2003). The bound Zn2+ changes the conformation of the protein, which inhibits the DNA binding. Since AzuR contains a similar α5 site, the conformational changes in AzuR as a result of metal binding was assessed by CD spectra of the protein in the presence of various concentrations of zinc (Figure 4D). The degree of the alpha helical region progressively decreased with increasing concentrations of zinc, indicating the changes in the secondary structure of AzuR in the presence of zinc. To further confirm whether zinc or other metal ions interfered with the AzuR DNA binding ability, EMSA was carried out in the presence of various metal ions. Dissociation of the DNA–AzuR complex was clearly evident with increasing concentrations of Zn2+ (Figures 4E,F). The interaction of Cd2+ with AzuR also disrupted the binding with PnmtA (Figure 4F); however, the disruption was more prominent in the presence of DTT (Figure 4G), emphasizing the requirement of free sulfhydryls for Cd2+ binding to AzuR in vitro. It was interesting to see the reversal of AzuR binding to PnmtA in the presence of other divalent metal ions like Cu2+, Co2+, Ni2+, Pb2+, and Mn2+ (Figure 4H), suggesting that AzuR not only senses toxic metal ions like Cd2+ and Pb2+ but also is capable of sensing essential metal ions like Zn2+, Cu2+, Co2+, Ni2+, and Mn2+. EMSAs attempted with metals other than Zn2+ and Cd2+ in the presence of DTT showed visible precipitates in the binding reaction and hence were not included here.
We have previously observed the induction of nmtA in the presence of Cd2+, Zn2+, and Cu2+ (Divya et al., 2018). AzuR, therefore, can be proposed as a negative regulator of nmtA as it binds to regulatory DNA sequence in the absence of the metals and the repression is relieved in the presence of metal ions. In view of our results, it can be suggested that AzuR might have a larger role in the metal resistance system of Anabaena 7120.
Overexpression of transcriptional regulators has been previously studied in Anabaena sp. (Wu et al., 2007). To gain insights into the effect of AzuR on various characteristics or phenotype of Anabaena 7120, we constructed a recombinant strain of Anabaena 7120 that overexpressed AzuR. The azuR gene was cloned and overexpressed constitutively in Anabaena 7120 from a strong light-inducible promoter, PpsbA. GFP fluorescence of the downstream reporter gene was the first indication of successful azuR gene expression (Figure 5A). GFP fluorescence was visualized in Anabaena harboring an empty vector with PpsbA upstream of the gfpmut2 gene, AnpsbA+ and Anabaena overexpressing nmtA, and AnnmtA+ (Figure 5A). WT cells did not show any such GFP fluorescence (Figure 5A). The observation of few cells appearing red in the filaments of recombinant cells under FM and blue-light excitation (BLE) conditions could be due to partial or reduced GFP expression (Figure 5A). The filament length in AnazuR+, AnpsbA+, and AnnmtA+ was comparable to that of WT Anabaena cells. The uniformity of the cell stacking in AnazuR+ filaments appeared to be compromised as compared to those in the filaments of WT, AnpsbA+, and AnnmtA+. However, the Chla fluorescence in AnazuR+ cells was intact and equivalent to that observed for WT, AnpsbA+, or AnnmtA+ cells (Figure 5A). A substantial increase in azuR transcript level was seen in RT-PCR performed with RNA isolated from AnazuR+ as compared to AnpsbA+ and AnnmtA+, thus confirming the overexpression of the regulator in vivo (Figure 5B).
Figure 5. Overexpression of AzuR in Anabaena 7120. (A) Effect of AzuR overexpression on the morphology of Anabaena 7120. Bright-field (BF) and FM photomicrographs under blue light excitation (BLE) (excitation 470 nm, emission 508 nm) and green light excitation (GLE) (excitation 520 nm, emission 680 nm) at ×1,500 magnification and scanning electron micrographs (SEMs) at ×100,000 magnification of WT, AnpsbA+, AnnmtA+, and AnazuR+. Non-uniformity of cell stacking in AnazuR+ filament is indicated by red arrows in BF micrographs. (B) Confirmation of overexpression of azuR transcripts by RT-PCR. Total RNA of 1 μg was used for cDNA synthesis, which served as a template for PCR performed with azuR-specific primers. The amplified products were resolved on 1% agarose gel and visualized by ethidium bromide staining. The lower panel represents the products of 16S rRNA used as control. (C) Plot of average cell size of WT, AnpsbA+, AnnmtA+, and AnazuR+ as analyzed by SEM is presented. One-way ANOVA was employed for calculating significance of the difference. Data shown here represent mean ± standard deviation (n = 13), ns, non-significant.
Scanning electron microscopy (SEM) analysis of exponential-phase cells of AnazuR+ revealed a significant decrease in cell size with the cells showing spherical and globular morphology in contrast to AnpsbA+, AnnmtA+, and WT cells (Figure 5A). The average cell size of AnazuR+ cells was found to be 2.70 ± 0.26 μm as compared to 3.92 ± 0.50 μm for AnpsbA+ and 3.67 ± 0.34 μm for AnnmtA+. The cell size of AnazuR+ was lesser than the WT cells (3.214 ± 0.34 μm) (Figure 5C). Similar morphological changes regarding cell stacking and cell size were observed following overexpression of the global transcriptional regulator FurA in Anabaena 7120 (González et al., 2010). The elongated cell phenotype seen in AnpsbA+ and AnnmtA+ cells could be because of stress owing to neomycin and heterologous GFP overexpression. The gross morphological changes in AnazuR+ as compared to the empty vector AnpsbA+ indicate the possible involvement of AzuR in the regulation of genes involved in functions other than metal homeostasis. Chromatin immunoprecipitation (ChIP) studies need to be done in the future to identify direct binding of targets of AzuR in the Anabaena genome.
DNA binding studies by EMSA showed that AzuR bound to the upstream region of the nmtA ORF in vitro. Evaluation of nmtA expression levels in AnazuR+ by qRT-PCR with 16S rRNA as internal control showed the downregulation of nmtA expression in AnazuR+ by ∼32-fold as compared to its empty vector AnpsbA+. These results are in agreement with the negative regulation of nmtA transcription by AzuR in vivo.
Previously, overexpression of NmtA in Anabaena 7120 had conferred tolerance to cadmium stress (Divya et al., 2018). Since the negative regulation of nmtA transcription by AzuR was observed here, we were interested to see the effect of the overexpression of AzuR on the cadmium tolerance ability of Anabaena 7120. We compared the response of cadmium stress in AnazuR+, AnpsbA+, and AnnmtA+ cultures. Spot assays showed increased sensitivity of AnazuR+ cells to cadmium stress following 7 days of exposure (Figure 6A). Growth of AnazuR+ assessed in terms of Chla content showed a substantial decrease even at concentrations of 10 μM cadmium as compared to AnpsbA+ (Figure 6B). Growth kinetics studies in the presence of 20 μM cadmium resulted in almost complete bleaching of cultures of both AnpsbA+ and AnazuR+ after 10 days of exposure to the stress (Figure 6C) including extensive cell lysis in AnazuR+ culture (Figure 6D). In contrast, filaments of AnnmtA+ appeared intact, long, and healthy on exposure to cadmium (Figure 6D). The spot assays and growth studies assessed in terms of Chla contents (Figures 6A–C) of AnnmtA+ also supported the microscopy observations, which are in agreement with our previous results showing superior tolerance of AnnmtA+ against cadmium stress (Divya et al., 2018). The GFP and Chla fluorescence were found to be unaffected in AnnmtA+ similar to AnazuR+ and AnpsbA+ in the presence of cadmium (Figure 6D). The toxic effects of cadmium on the photosynthetic machinery have been studied extensively in Synechocystis PCC 6803 (Tóth et al., 2012). The major proteins involved in photosynthetic machinery include zinc-containing enzymes like carbonic anhydrase and sulfhydryl groups in ribulose-5-phosphate kinase among others that lose their activity by replacement with cadmium (Tóth et al., 2012). MTs play a key role in metal detoxification by directly binding to the toxic metal, which results in lesser bioavailability (Klaassen et al., 1999). This protects the essential metalloproteins from the toxic metal. Since AzuR overexpression leads to a decrease in basal nmtA expression, the protective role of NmtA in imparting cadmium tolerance could be obliterated, resulting in the susceptibility of AnazuR+ to cadmium stress, which was evident from its decreased growth and increased cell lysis. The susceptibility of AnazuR+ to cadmium stress confirms the negative regulation of nmtA expression at the physiological level in Anabaena.
Figure 6. Effect of AzuR overexpression on cadmium exposure. (A) Spot assays of AnpsbA+, AnnmtA+, and AnazuR+ following exposure to cadmium stress for 7 days. The cell densities are indicated in terms of Chla content (μg). (B) Growth kinetics of AnpsbA+, AnnmtA+, and AnazuR+ as assessed by contents of Chla. (C) The recombinant cultures were exposed to 10 or 20 μM cadmium for 10 days, and subsequently, the cultures were transferred to 12-well microtiter plate and photographed. (D) BF and FM microphotographs under BLE (excitation 470 nm, emission 508 nm) and GLE (excitation 520 nm, emission 680 nm) at ×600 magnification of AnpsbA+, AnnmtA+, and AnazuR+ cells after 10 days of cadmium exposure.
We have characterized the role of AzuR belonging to the SmtB/ArsR family of metalloregulators in the regulation of Anabaena MT NmtA. The sequence analysis of AzuR (Alr0831) identified a distinct α5 metal binding site similar to that of SmtB. Although the azuR gene locus was found to be situated remotely away from the nmtA locus, analysis of the region upstream of the nmtA ORF identified the presence of 12–2–12 imperfect inverted repeats, which are reportedly important for binding of metalloregulators belonging to the SmtB/ArsR family of proteins. EMSAs showed AzuR binding with putative PnmtA, indicating that NmtA is a regulatory target of AzuR. Dissociation of the protein–DNA complex was observed not only in the presence of toxic metal ions like Cd2+ and Pb2+ but also in the presence of essential metal ions like Zn2+, Cu2+, Co2+, Ni2+, and Mn2+, which suggested negative regulation of metal-inducible nmtA expression by AzuR. On the basis of our findings, we propose a model for Anabaena NmtA regulation by AzuR (Figure 7). In the absence of metals or basal conditions, the binding of AzuR to the upstream region of the nmtA ORF blocks the binding site for the RNA polymerase transcription initiation complex, resulting in the repression of nmtA. At elevated concentrations of the metals, the binding of AzuR to DNA is disrupted as a result of conformational changes in the protein resulting from metal binding. This leads to the induction of nmtA transcription in the presence of metals as seen earlier in our studies (Divya et al., 2018). The sensing of a large number of metal ions implies a greater role of AzuR in the modulation of metal ions in the intracellular environment in Anabaena 7120.
Figure 7. Schematic representation of Anabaena NmtA regulation by AzuR. In the absence of metals, binding of AzuR to the upstream region of the nmtA ORF blocks the binding site for the RNA polymerase transcription initiation complex, resulting in repression of nmtA. At elevated concentrations of the metals, the binding of AzuR to DNA is disrupted as a result of structural changes in the protein due to metal binding. This leads to the induction of nmtA transcription in the presence of metals.
Although we have largely focused on the role of AzuR in MT regulation, the presence of cis-regulatory elements important for repressor binding at several locations in the Anabaena 7120 genome indicates that AzuR might act as a global transcriptional regulator. It will be interesting to study the role of AzuR beyond metal homeostasis. The similar inverted repeats recognized by AztR (repressor of CPx-ATPase) and AzuR (repressor of MT) suggest that these two repressors could share regulation of their respective effector genes in vivo. The direct interaction between the two regulators and possibly the cross-talk between the two processes of metal sequestration and efflux would help us to understand the regulation of the metal homeostasis system in Anabaena.
The original contributions presented in the study are included in the article/supplementary material, further inquiries can be directed to the corresponding author.
CA conceived, designed, and supervised the research. TVD performed the experiments. CA and TVD analyzed the data, wrote the draft of the manuscript, and revised the manuscript. Both authors approved the submitted version.
The authors declare that the research was conducted in the absence of any commercial or financial relationships that could be construed as a potential conflict of interest.
All claims expressed in this article are solely those of the authors and do not necessarily represent those of their affiliated organizations, or those of the publisher, the editors and the reviewers. Any product that may be evaluated in this article, or claim that may be made by its manufacturer, is not guaranteed or endorsed by the publisher.
We wish to acknowledge SAIF, IIT Bombay, for protein identification by O-HRLC-MS. We are grateful to Arvind Kumar, MBD, BARC, for Anabaena LexA protein. We thank Manisha Banerjee, MBD, BARC, for extending help in CD spectroscopy and Gagan Deep Gupta and Hiral Mistry, RB & HSD, BARC, for help in size-exclusion chromatography. We gratefully acknowledge H. S. Misra, MBD, BARC, for the support and encouragement during the course of this study.
Allen, M. M. (1968). Simple conditions for growth of unicellular blue-green algae on plates. J. Phycol. 4, 1–4. doi: 10.1111/j.1529-8817.1968.tb04667.x
Andrews, G. K. (2000). Regulation of metallothionein gene expression by oxidative stress and metal ions. Biochem. Pharmacol. 59, 95–104. doi: 10.1016/S0006-2952(99)00301-9
Bertini, G., Gray, H. B., Gray, H., Valentine, J. S., Stiefel, E. I., and Stiefel, E. (2007). Biological Inorganic Chemistry: Structure and Reactivity. Melville, NY: University Science Books.
Blindauer, C. A. (2008). Zinc-handling in cyanobacteria: an update. Chem. Biodivers. 5, 1990–2013. doi: 10.1002/cbdv.200890183
Blindauer, C. A. (2011). Bacterial metallothioneins: past, present, and questions for the future. J. Biol. Inorg. Chem. 16, 1011–1024. doi: 10.1007/s00775-011-0790-y
Blindauer, C. A., and Leszczyszyn, O. I. (2010). Metallothioneins: unparalleled diversity in structures and functions for metal ion homeostasis and more. Nat. Prod. Rep. 27, 720–741. doi: 10.1039/B906685N
Bose, M., Slick, D., Sarto, M. J., Murphy, P., Roberts, D., Roberts, J., et al. (2006). Identification of SmtB/ArsR cis elements and proteins in archaea using the prokaryotic intergenic exploration database (PIGED). Archaea 2, 39–49. doi: 10.1155/2006/837139
Busenlehner, L. S., Cosper, N. J., Scott, R. A., Rosen, B. P., Wong, M. D., and Giedroc, D. P. (2001). Spectroscopic properties of the metalloregulatory Cd (II) and Pb (II) sites of S. aureus pI258 CadC. Biochemistry 40, 4426–4436. doi: 10.1021/bi010006g
Busenlehner, L. S., Pennella, M. A., and Giedroc, D. P. (2003). The SmtB/ArsR family of metalloregulatory transcriptional repressors: structural insights into prokaryotic metal resistance. FEMS Microbiol. Rev. 27, 131–143. doi: 10.1016/S0168-6445(03)00054-8
Chandrangsu, P., Rensing, C., and Helmann, J. D. (2017). Metal homeostasis and resistance in bacteria. Nat. Rev. Microbiol. 15, 338–350. doi: 10.1038/nrmicro.2017.15
Chaurasia, A. K., Parasnis, A., and Apte, S. K. (2008). An integrative expression vector for strain improvement and environmental applications of the nitrogen fixing cyanobacterium, Anabaena sp. strain PCC7120. J. Microbiol. Methods 73, 133–141. doi: 10.1016/j.mimet.2008.01.013
Divya, T. V., Chandwadkar, P., and Acharya, C. (2018). NmtA, a novel metallothionein of Anabaena sp. strain PCC 7120 imparts protection against cadmium stress but not oxidative stress. Aquat. Toxicol. 199, 152–161. doi: 10.1016/j.aquatox.2018.03.035
Elhai, J., Vepritskiy, A., Muro-Pastor, A. M., Flores, E., and Wolk, C. P. (1997). Reduction of conjugal transfer efficiency by three restriction activities of Anabaena sp. strain PCC 7120. J. Bacteriol. 179, 1998–2005. doi: 10.1128/jb.179.6.1998-2005.1997
Erbe, J. L., Taylor, K. B., and Hall, L. M. (1995). Metalloregulation of the cyanobacterial smt locus: indentification of SmtB binding sites and direct interaction with metals. Nucleic Acids Res. 23, 2472–2478. doi: 10.1093/nar/23.13.2472
Finney, L. A., and O’Halloran, T. V. (2003). Transition metal speciation in the cell: insights from the chemistry of metal ion receptors. Science 300, 931–936. doi: 10.1126/science.1085049
González, A., Bes, M. T., Barja, F., Peleato, M. L., and Fillat, M. F. (2010). Overexpression of FurA in Anabaena sp. PCC 7120 reveals new targets for this regulator involved in photosynthesis, iron uptake and cellular morphology. Plant Cell Physiol. 51, 1900–1914. doi: 10.1093/pcp/pcq148
Habjanič, J., Mathew, A., Eberl, L., and Freisinger, E. (2020). Deciphering the enigmatic function of Pseudomonas metallothioneins. Front. Microbiol. 11:1709. doi: 10.3389/fmicb.2020.01709
Hill, A. V. (1910). The possible effects of the aggregation of the molecules of haemoglobin on its dissociation curves. J. Physiol. 40, 4–7.
Huckle, J. W., Morby, A. P., Turner, J. S., and Robinson, N. J. (1993). Isolation of a prokaryotic metallothionein locus and analysis of transcriptional control by trace metal ions. Mol. Microbiol. 7, 177–187. doi: 10.1111/j.1365-2958.1993.tb01109.x
Klaassen, C. D., Liu, J., and Choudhuri, S. (1999). Metallothionein: an intracellular protein to protect against cadmium toxicity. Annu. Rev. Pharmacool. Toxicol. 39, 267–294. doi: 10.1146/annurev.pharmtox.39.1.267
Kumar, S., Stecher, G., Li, M., Knyaz, C., and Tamura, K. (2018). MEGA X: molecular evolutionary genetics analysis across computing platforms. Mol. Biol. Evol. 35:1547. doi: 10.1093/molbev/msy096
Liu, T., Chen, X., Ma, Z., Shokes, J., Hemmingsen, L., Scott, R. A., et al. (2008). A Cu(I)-sensing ArsR family metal sensor protein with a relaxed metal selectivity profile. Biochemistry 47, 10564–10575. doi: 10.1021/bi801313y
Liu, T., Golden, J. W., and Giedroc, D. P. (2005). A zinc (II)/lead (II)/cadmium (II)-inducible operon from the cyanobacterium Anabaena is regulated by AztR, an α3N SmtB/ArsR metalloregulator. Biochemistry 44, 8673–8683. doi: 10.1021/bi050450+
Liu, T., Nakashima, S., Hirose, K., Shibasaka, M., Katsuhara, M., Ezaki, B., et al. (2004). A novel cyanobacterial SmtB/ArsR family repressor regulates the expression of a CPx-ATPase and a metallothionein in response to both Cu (I)/Ag (I) and Zn (II)/Cd (II). J. Biol. Chem. 279, 17810–17818. doi: 10.1074/jbc.M310560200
Madeira, F., Park, Y. M., Lee, J., Buso, N., Gur, T., Madhusoodanan, N., et al. (2019). The EMBL-EBI search and sequence analysis tools APIs in 2019. Nucleic Acids Res. 47, W636–W641. doi: 10.1093/nar/gkz268
Mrázek, J., and Xie, S. (2006). Pattern locator: a new tool for finding local sequence patterns in genomic DNA sequences. Bioinformatics 22, 3099–3100. doi: 10.1093/bioinformatics/btl551
Napolitano, M., Rubio, M. Á., Santamaria-Gomez, J., Olmedo-Verd, E., Robinson, N. J., and Luque, I. (2012). Characterization of the response to zinc deficiency in the cyanobacterium Anabaena sp. strain PCC 7120. J. Bacteriol. 194, 2426–2436. doi: 10.1128/JB.00090-12
Osman, D., and Cavet, J. S. (2010). Bacterial metal-sensing proteins exemplified by ArsR-SmtB family repressors. Nat. Prod. Rep. 27, 668–680. doi: 10.1039/B906682A
Palmiter, R. D. (1987). “Molecular biology of metallothionein gene expression,” in Metallothionein II. Experientia Supplementum, Vol. 52, eds J. H. R. Kägi and Y. Kojima (Basel: Birkhäuser), doi: 10.1007/978-3-0348-6784-9_4
Perez-Iratxeta, C., and Andrade-Navarro, M. A. (2008). K2D2: estimation of protein secondary structure from circular dichroism spectra. BMC Struct. Biol. 8:25. doi: 10.1186/1472-6807-8-25
Pinto, F., Pacheco, C. C., Ferreira, D., Moradas-Ferreira, P., and Tamagnini, P. (2012). Selection of suitable reference genes for RT-qPCR analyses in cyanobacteria. PLoS One 7:e34983. doi: 10.1371/journal.pone.0034983
Rees, D. C. (2002). Great metalloclusters in enzymology. Annu. Rev. Biochem. 71, 221–246. doi: 10.1146/annurev.biochem.71.110601.135406
Roy, A., Kucukural, A., and Zhang, Y. (2010). I-TASSER: a unified platform for automated protein structure and function prediction. Nat. Protoc. 5, 725–738. doi: 10.1038/nprot.2010.5
Saha, R. P., Samanta, S., Patra, S., Sarkar, D., Saha, A., and Singh, M. K. (2017). Metal homeostasis in bacteria: the role of ArsR–SmtB family of transcriptional repressors in combating varying metal concentrations in the environment. Biometals 30, 459–503. doi: 10.1007/s10534-017-0020-3
Salamov, V. S. A., and Solovyevand, A. (2011). “Automatic annotation of microbial genomes and metagenomic sequences,” in Metagenomics and Its Applications in Agriculture, Biomedicine and Environmental Studies, ed. R. W. Li (Hauppauge, NY: Nova Science Publishers), 61–78.
Thelwell, C., Robinson, N. J., and Turner-Cavet, J. S. (1998). An SmtB-like repressor from Synechocystis PCC 6803 regulates a zinc exporter. Proc. Natl. Acad. Sci. U.S.A. 95, 10728–10733.
Thompson, J. D., Higgins, D. G., and Gibson, T. J. (1994). CLUSTAL W: improving the sensitivity of progressive multiple sequence alignment through sequence weighting, position-specific gap penalties and weight matrix choice. Nucleic Acids Res. 22, 4673–4680. doi: 10.1093/nar/22.22.4673
Tóth, T., Zsiros, O., Kis, M., Garab, G., and Kovacs, L. (2012). Cadmium exerts its toxic effects on photosynthesis via a cascade mechanism in the cyanobacterium, Synechocystis PCC 6803. Plant Cell Environ. 35, 2075–2086. doi: 10.1111/j.1365-3040.2012.02537.x
Tottey, S., Harvie, D. R., and Robinson, N. J. (2005). Understanding how cells allocate metals using metal sensors and metallochaperones. Acc. Chem. Res. 38, 775–783. doi: 10.1021/ar0300118
Tottey, S., Harvie, D. R., and Robinson, N. J. (2007). “Understanding how cells allocate metals,” in Molecular Microbiology of Heavy Metals. Microbiology Monographs, Vol. 6, eds D. H. Nies and S. Silver (Berlin: Springer), doi: 10.1007/7171_2006_072
Turner, J. S., Glands, P. D., Samson, A. C., and Robinson, N. J. (1996). Zn 2+-sensing by the cyanobacterial metallothionein repressor SmtB: different motifs mediate metal-induced protein-DNA dissociation. Nucleic Acids Res. 24, 3714–3721. doi: 10.1093/nar/24.19.3714
Turner, J. S., and Robinson, N. J. (1995). Cyanobacterial metallothioneins: biochemistry and molecular genetics. J. Ind. Microbiol. 14, 119–125. doi: 10.1007/BF01569893
VanZile, M. L., Chen, X., and Giedroc, D. P. (2002). Allosteric negative regulation of smt O/P binding of the zinc sensor, SmtB, by metal ions: a coupled equilibrium analysis. Biochemistry 41, 9776–9786. doi: 10.1021/bi020178t
VanZile, M. L., Cosper, N. J., Scott, R. A., and Giedroc, D. P. (2000). The zinc metalloregulatory protein Synechococcus PCC7942 SmtB binds a single zinc ion per monomer with high affinity in a tetrahedral coordination geometry. Biochemistry 39, 11818–11829. doi: 10.1021/bi001140o
Waldron, K. J., and Robinson, N. J. (2009). How do bacterial cells ensure that metalloproteins get the correct metal? Nat. Rev. Microbiol. 7, 25–35. doi: 10.1038/nrmicro2057
Waterhouse, A. M., Procter, J. B., Martin, D. M., Clamp, M., and Barton, G. J. (2009). Jalview Version 2—a multiple sequence alignment editor and analysis workbench. Bioinformatics 25, 1189–1191. doi: 10.1093/bioinformatics/btp033
Wu, X., Lee, D. W., Mella, R. A., and Golden, J. W. (2007). The Anabaena sp. strain PCC 7120 asr1734 gene encodes a negative regulator of heterocyst development. Mol. Microbiol. 64, 782–794. doi: 10.1111/j.1365-2958.2007.05698.x
Yang, J., Yan, R., Roy, A., Xu, D., Poisson, J., and Zhang, Y. (2015). The I-TASSER Suite: protein structure and function prediction. Nat. Methods 12, 7–8. doi: 10.1038/nmeth.3213
Yoon, H. S., and Golden, J. W. (1998). Heterocyst pattern formation controlled by a diffusible peptide. Science 282, 935–938. doi: 10.1126/science.282.5390.935
Keywords: Anabaena 7120, AzuR, regulation, metallothionein, cadmium stress
Citation: Divya TV and Acharya C (2022) AzuR From the SmtB/ArsR Family of Transcriptional Repressors Regulates Metallothionein in Anabaena sp. Strain PCC 7120. Front. Microbiol. 12:782363. doi: 10.3389/fmicb.2021.782363
Received: 24 September 2021; Accepted: 30 November 2021;
Published: 12 January 2022.
Edited by:
Rob Van Houdt, Belgian Nuclear Research Centre, BelgiumReviewed by:
Vicente Mariscal, Institute of Plant Biochemistry and Photosynthesis, Spanish National Research Council (CSIC), SpainCopyright © 2022 Divya and Acharya. This is an open-access article distributed under the terms of the Creative Commons Attribution License (CC BY). The use, distribution or reproduction in other forums is permitted, provided the original author(s) and the copyright owner(s) are credited and that the original publication in this journal is cited, in accordance with accepted academic practice. No use, distribution or reproduction is permitted which does not comply with these terms.
*Correspondence: Celin Acharya, Y2VsaW5AYmFyYy5nb3YuaW4=
Disclaimer: All claims expressed in this article are solely those of the authors and do not necessarily represent those of their affiliated organizations, or those of the publisher, the editors and the reviewers. Any product that may be evaluated in this article or claim that may be made by its manufacturer is not guaranteed or endorsed by the publisher.
Research integrity at Frontiers
Learn more about the work of our research integrity team to safeguard the quality of each article we publish.