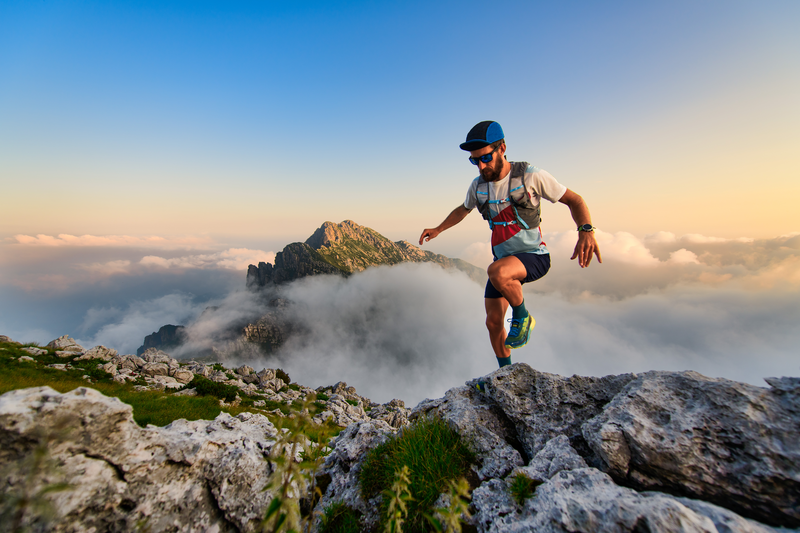
95% of researchers rate our articles as excellent or good
Learn more about the work of our research integrity team to safeguard the quality of each article we publish.
Find out more
ORIGINAL RESEARCH article
Front. Microbiol. , 18 November 2021
Sec. Virology
Volume 12 - 2021 | https://doi.org/10.3389/fmicb.2021.779460
We previously reported that a human immunodeficiency virus type 1 with a simian immunodeficiency virus vif substitution (HSIV-vifNL4-3) could replicate in pigtailed macaques (PTMs), demonstrating that Vif is a species-specific tropism factor of primate lentiviruses. However, infections did not result in high-peak viremia or setpoint plasma viral loads, as observed during simian immunodeficiency virus (SIV) infection of PTMs. Here, we characterized variants isolated from one of the original infected animals with CD4 depletion after nearly 4years of infection to identify determinants of increased replication fitness. In our studies, we found that the HSIV-vif clones did not express the HIV-1 Vpr protein due to interference from the vpx open reading frame (ORF) in singly spliced vpr mRNA. To examine whether these viral genes contribute to persistent viral replication, we generated infectious HSIV-vif clones expressing either the HIV-1 Vpr or SIV Vpx protein. And then to determine viral fitness determinants of HSIV-vif, we conducted three rounds of serial in vivo passaging in PTMs, starting with an initial inoculum containing a mixture of CXCR4-tropic [Vpr-HSIV-vifNL4-3 isolated at 196 (C/196) and 200 (C/200) weeks post-infection from a PTM with depressed CD4 counts] and CCR5-tropic HSIV (Vpr+ HSIV-vif derivatives based NL-AD8 and Bru-Yu2 and a Vpx expressing HSIV-vifYu2). Interestingly, all infected PTMs showed peak plasma viremia close to or above 105 copies/ml and persistent viral replication for more than 20weeks. Infectious molecular clones (IMCs) recovered from the passage 3 PTM (HSIV-P3 IMCs) included mutations required for HIV-1 Vpr expression and those mutations encoded by the CXCR4-tropic HSIV-vifNL4-3 isolate C/196. The data indicate that the viruses selected during long-term infection acquired HIV-1 Vpr expression, suggesting the importance of Vpr for in vivo pathogenesis. Further passaging of HSIV-P3 IMCs in vivo may generate pathogenic variants with higher replication capacity, which will be a valuable resource as challenge virus in vaccine and cure studies.
Several alternate animal models such as infection of macaques with simian immunodeficiency viruses (SIVs) or chimeric simian–human immunodeficiency viruses (SHIVs) have been developed to understand HIV pathogenesis and disease progression and determine the efficacy of vaccines and drugs. However, the genetic difference between HIV-1 and SIV, and the absence of other HIV-1 genes, such as gag, vif, vpr, and nef in SHIV limit the utility of these models. Therefore, there is a need to rationally and minimally modify HIV-1 such that it can replicate and cause AIDS in macaques. Such an animal model will be a valuable tool for preclinical evaluation of vaccines and the development of novel therapeutic strategies targeting HIV-1 proteins, and for understanding viral immunopathogenesis.
The important lentiviral restriction factors in macaque species such as rhesus macaques (RMs) are the apolipoprotein B mRNA editing enzyme catalytic polypeptide 3 (APOBEC3 or A3) family of proteins, tripartite motif containing (TRIM) family of proteins, BST2/CD317/Tetherin, and sterile alpha motif (SAM) and histidine/aspartic acid (HD) domain containing protein 1 (SAMHD1; reviewed in Thippeshappa et al., 2012; Saito and Akari, 2013). However, SIV can overcome RM TRIM5α and the APOBEC3 family of restriction factors and simian-tropic HIV-1 (stHIV-1) or macaque-tropic HIV-1 (mtHIV-1) have been developed by incorporating capsid and vif sequences from SIVmac239 (Hatziioannou et al., 2006; Saito et al., 2011; Doi et al., 2013, 2018; Nomaguchi et al., 2013; Otsuki et al., 2014). Instead of a full-length capsid substitution, an HIV-1 derivative carrying only a short 21 nucleotide segment from the SIV capsid sequence corresponding to the HIV-1 cylophilin A binding loop has also been constructed (Kamada et al., 2006). Additionally, variants with CCR5-tropic HIV-1 have also been developed (Otsuki et al., 2014; Doi et al., 2017). These variants of stHIV-1 or mtHIV-1 establish infection in vivo in different species of nonhuman primates (NHPs; Igarashi et al., 2007; Saito et al., 2011, 2013; Otsuki et al., 2014; Doi et al., 2018). However, none of the variants result in CD4 depletion, and there remains a need to develop a pathogenic macaque-tropic HIV-1 (reviewed in Thippeshappa et al., 2020).
Compared to other NHPs used in AIDS research, PTMs are relatively more susceptible to HIV-1 infection (Agy et al., 1992, 1997; Frumkin et al., 1993; Gartner et al., 1994a,b; Bosch et al., 1997, 2000). While PTMs can be infected with HIV-1, viral loads diminished rapidly (Agy et al., 1992). Attempts to in vivo passage HIV-1 in PTMs failed to select variants capable of persistent replication.
An explanation for the susceptibility of PTM CD4+ T cells to HIV-1 is that PTMs do not express restriction factor TRIM5α. Instead, they express novel isoforms of TRIM5 (TRIM5θ and TRIM5η) and TRIM5-cyclophilin A fusion protein (TRIMcyp) that do not interfere with HIV-1 infection (Liao et al., 2007; Brennan et al., 2008; Newman et al., 2008; Virgen et al., 2008). The absence of TRIM5α suggests that other retroviral restriction factors in PTMs, such as APOBEC3 family of proteins, BST2, and SAMHD1 may limit replication of HIV-1. Since APOBEC3 family proteins can be degraded by SIVmac and HIV-2 vif, Hatziioannou et al. constructed minimally modified HIV-1 derivatives carrying either SIVmac vif or HIV-2 vif (Hatziioannou et al., 2009). PTMs infected intravenously (IV) with a mixture of these two viruses exhibited acute infection and persistent viremia for up to 25weeks post-infection (wpi). However, CD4+ T cell depletion was not observed in the animals. To select a variant with increased fitness, serial in vivo passaging of a mixture of four clonal HIV-1NL4-3–derived viruses, each encoding CCR5-tropic gp120 env from YU2, BaL, AD8, and KB9, was conducted in PTMs transiently depleted of CD8 T cells. Viral swarm or infectious molecular clone (IMC) generated following passaging caused CD4 depletion only in macaques that were transiently depleted of CD8 T cells. However, they were controlled in immunocompetent PTMs (Hatziioannou et al., 2014; Schmidt et al., 2019). Inability of passaged viruses to cause AIDS in non-CD8-depleted macaques suggests partial adaptation to the PTM host. Despite these studies, the key characteristics necessary for enhanced replication of macaque-tropic HIV-1 clones remain poorly understood.
We have constructed PTM-tropic HIV-1 viruses (HSIV-vif) by replacing the vif genes with vif from highly pathogenic PTM-adapted SIVmne027 (Kimata et al., 1998, 1999). These cloned viruses (CXCR4-tropic HSIV-vifNL4-3 and CCR5-tropic HSIV-vifAD8 and HSIV-vifYu2) replicated better than their respective parental clones in PTM peripheral blood mononuclear cells (PBMCs; Thippeshappa et al., 2011). Intravenous (IV) inoculation of PTMs with HSIV-vifNL4-3 showed low viral replication during the post-acute stages of infection through 44wpi and small rebounds in viral titer at 64 and 72wpi in juvenile PTMs (Thippeshappa et al., 2011). Furthermore, we observed that unlike pathogenic SIVmne, HSIV-vifNL4-3 replication is suppressed by type I interferon (IFN) treatment in PTM CD4+ T cells, perhaps suggesting that the IFN response during acute infection may limit virus replication in PTMs. Interestingly, we found that HSIV-vifYu2 was resistant to interferon alpha (IFNα)-treatment in PTM CD4+ T cells in vitro, which may be due to envelope-mediated counteraction of IFNα-induced restrictions at the entry step of the viral life cycle (Thippeshappa et al., 2013). To further define important mutations in HSIV-vif that confer increased viral fitness in PTMs, we isolated and characterized variant virus isolates from peripheral blood CD4+ T cells after 196–200wpi with HSIV-vifNL4-3 infection when there was CD4+ T cell depletion, and then performed a serial in vivo passaging experiment using a mixture of these virus isolates and different clones of HSIV-vif to define genetic characteristics that could contribute to persistent viral replication in vivo.
TZM-bl cells were obtained from the NIH HIV Reagent Program (Derdeyn et al., 2000; Wei et al., 2002). TZM-bl and 293T cells were cultured in Dulbecco’s modified Eagle’s medium (DMEM) supplemented with 10% heat-inactivated fetal bovine serum (HI-FBS), 2mM glutamine, 100U of penicillin per ml, and 100μg of streptomycin per ml (p/s; DMEM complete). The immortalized PTM CD4+ T cells, obtained from Dr. Hans-Peter Kiem (Fred Hutchinson Cancer Research Center), were maintained in Iscove’s Modified Dulbecco’s Medium (IMDM) containing 10% HI-FBS, 2mM glutamine, p/s, and 100U/ml human interleukin 2 (IL-2; Roche; Munoz et al., 2009). CEM×174 were obtained from the American Type Culture Collection and cultured in Roswell Park Memorial Institute (RPMI) media with 10% HI-FBS, 2mM glutamine, 100U of penicillin per ml, and 100μg of streptomycin per ml (RPMI complete).
Total CD4+ T cells were isolated from 1×107 PBMCs recovered at 196 and 200wpi of pigtailed macaque M08009 by negative selection using the Miltenyi nonhuman primate CD4+ T cell isolation kit (Miltenyi Biotech). The cells were isolated according to the manufacturer’s protocol. The M08009 CD4+ T cells were cocultured with the human T cell-B cell hybrid cell line, CEMx174 for up to 16days. Supernatants after 7 and 16days were assayed for HIV-1 p24 antigen by ELISA (Advanced Bioscience Laboratories). If positive, supernatants were passed through 0.45-μm syringe filters, aliquoted, and frozen at −80°C. The infectious titers of the stocks were determined by limiting dilution infection analysis using TZM-bl reporter cells and luciferase assay as described (Misra et al., 2018). Infectious virus recovered from CD4+ T cells at 196 and 200wpi was named C/196 and C/200, respectively.
TZM-bl cells (1×104 cells per well) were plated in wells of a 96-well plate in DMEM complete with 30μg/ml DEAE-dextran. In triplicate cultures, cells were treated with either the CXCR4 inhibitor, AMD3100, or CCR5 inhibitor, Maraviroc, such that after adding 250 infectious units of C/196, C/200, or control viruses HSIV-vifNL4-3 or HSIV-vifAD8 the final concentrations of inhibitors were 1μM, 500nM, or 250nM, and the final concentration of DEAE-dextran was 20μg/ml. After 2days of infection, the cells were washed once with PBS and lysed with Promega Glo Lysis buffer. Lysates were assayed for luciferase activity using the Promega luciferase assay system and tube luminometer according to the manufacturer’s instructions (Promega).
Neutralizing antibody titers in serum specimens from PTMs infected with HSIV-vifNL4-3 were determined using a TZMbl-based neutralization assay as described previously (Wu et al., 2006). Serum samples were heat-inactivated at 56°C for 30min prior to use.
Construction of the HSIV-vif clones based on NL4-3, NL-AD8, and Bru-Yu2 has been reported before (Thippeshappa et al., 2011). To generate Vpr+ HSIV-vifNL4-3 and HSIV-vifAD8 clones, SphI to SalI fragment of HSIV-vifNL4-3 encompassing HIV gag, pol, SIV vif, and HIV-1 vpr genes was cloned into pCR2.1 TOPO vector (Thermofisher). SIV vpx start codon and two additional ATG codons upstream of the HIV-1 vpr start codon were mutated by Quickchange mutagenesis (Stratagene) and the sequence between the stop codon of vif and start codon of vpr were deleted. After mutagenesis, SphI and SalI fragment was cloned back into HSIV-vifNL4-3 and HSIV-vifAD8. Similarly, SphI to SalI fragment of HSIV-vifYu2 was cloned into SacI site removed pUC19 vector (Thermofisher), ATG codons upstream of vpr were mutated, and cloned back into HSIV-vifYu2 (Supplementary Figure 1). HSIV-vif-VpxYu2 clone was generated by cloning SacI to NcoI fragment of SIVmne027 vpx gene into pUC19 vector containing SphI to SalI fragment of HSIV-vifYu2. SphI to SalI fragment containing full length vpx was confirmed by sequencing and cloned back into HSIV-vifYu2 (Supplementary Figure 2).
Virus stocks were generated by transfection of 293T cells with each plasmid clone of HSIV-vif using Fugene 6 or X-tremeGENE 9 DNA transfection reagent according to the manufacturer’s protocol (Roche). Infectious titers were determined by limiting dilution infection analysis using TZM-bl indicator cells, and the amount of virus in supernatants was measured by HIV-1 p24gag antigen ELISA (Advanced Bioscience Laboratories).
293T cells, seeded the day before, in 6-well plates were transfected with HSIV-vif plasmids using Fugene 6 or X-tremeGENE 9 DNA transfection reagent (Roche/Sigma). At 48h post-transfection, cell culture supernatants were used to concentrate viral particles by centrifugation at 23,600×g for 1h at 4°C. Viral particles were mixed with 2X SDS samples buffer and separated by SDS-PAGE using Tris-HCl ready gels (Bio-Rad). Cell lysates were prepared as previously described (Thippeshappa et al., 2011). Proteins were transferred to either nitrocellulose or PVDF membrane and probed with rabbit antiserum to Vpr, Nef, or Vpx. Goat anti-rabbit IgG HRP (Promega) was used as secondary antibody. Antiserum to Vpr (Catalog # ARP-11836), Nef (Catalog # ARP-2949), Vpx (Catalog # ARP-2609), and anti-HIV-1 p24 Gag monoclonal (ARP-6458) were obtained from NIH HIV reagent program.
For viral replication assays, PTM PBMCs and immortalized PTM CD4+ T cells were infected as previously described (Thippeshappa et al., 2011, 2013). PTM PBMCs were isolated using Ficoll hypaque gradient method. PBMCs were activated with concanavalin A (7μg/ml) for 3days. Cells were then washed twice and resuspended in RPMI complete media containing 40U/ml IL-2 (Sigma) and cultured for 2days. Approximately 1×106 activated PBMCs were infected in duplicate at a multiplicity of infection (MOI) of 0.01. To compare viral replication in PTM CD4+ T cells, approximately 2.5×105 or 5×105 cells in 100U/ml IL-2 containing IMDM were infected in duplicate with viruses at a MOI of 0.01 or 0.05. Human monocyte-derived macrophages (MDMs) were generated from PBMCs using previously described methods (Kimata et al., 1998, 2004; Biesinger et al., 2010). PBMCs of anonymous donors were isolated from leukopacks purchased from the Gulf Coast Blood Center, Houston, TX, Unites States. Briefly, human monocytes were isolated from PBMCs by plate adherence methods. Approximately 4×106 PBMCs were plated into each well of a 24-well plate and monocytes were allowed to adhere to the plate for 1h. Monocytes were then stimulated with RPMI complete containing 10U/ml GM-CSF (Invitrogen) for 7–10days to generate MDMs. Human MDMs were infected with the Vpr+ and Vpr-HSIV-vif in duplicate. Infection experiments were conducted at least 2–3 times. PBMCs from different donors were also used. After 3h of incubation, the cells were washed twice with phosphate buffered saline (PBS) or complete medium to remove unbound virus. Infected cells were then resuspended in RPMI complete media containing IL-2. To study the effect of IFNα, 200U/ml of Interferon-αA/D (IFN-αA/D or IFNα, Sigma) was added to the culture media. To monitor replication of HSIV-vif clones, supernatants were harvested every 2–4days for measurement of HIV-1 p24gag antigen using ELISA kit (Advanced Bioscience Laboratories or ExpressBio). Statistical analysis in GraphPad Prism was performed to compare groups using the Mann-Whitney test.
Four PTMs specific pathogen free for simian T lymphotropic virus type 1, SIV, simian retrovirus type D, and herpes B virus were enrolled for the study. All animals were housed and cared for in accordance with the guidelines of the American Association for Accreditation of Laboratory Animal Care and the Animal Care and Use Committee of the University of Washington. In passage 1: two PTMs were inoculated IV with a mixture of CXCR4 and CCR5-tropic viruses. In passage 2: pooled peripheral blood from passage 1 PTMs collected at 14wpi was used for inoculation of 1 PTM. In passage 3, peripheral blood from passage 2 PTM at 8wpi was used for transfusion into an additional PTM. At several time points post-inoculation, peripheral blood was drawn for CD4+ T cell count determinations and isolation of plasma, sera, and PBMC.
Plasma viral load measurements were determined by using the Roche Amplicor HIV-1 monitor test, version 1.5 according to the manufacturer’s protocol. CD4+ T cell counts were determined as previously described (Polacino et al., 2007). HIV-1-specific antibodies were measured by ELISA as previously described, using gradient-purified and disrupted whole HIV-1 virions as the capture antigen (Hu et al., 1989; Polacino et al., 2007).
The following steps were performed to generate IMCs (Supplementary Figure 3).
Proviral DNA was isolated from 1×106 PBMCs using Quick-DNA miniprep kit (Zymo Research). In the first round PCR, 1 to 2μl of proviral DNA (approximately 50–100ng) in a 25μl reaction was amplified using the following primers: FWD-1: AAATCTCTAGCAGTGGCGCCCGAACAG and REV-1: TGAGGGATCTCTAGTTACCAGAGTC. Reaction mix contained 1× High Fidelity Buffer, 2mM MgSO4, 0.2mM dNTPs, and 0.025U/μl Platinum Taq High Fidelity (Invitrogen). PCR conditions for the first round were 94°C for 2min, then 94°C for 30s, 64°C for 30s, and 68°C for 10min for 3cycles; 94°C for 30s, 61°C for 30s, and 68°C for 10min for 3cycles; 94°C for 30s, 59°C for 30s, and 68°C for 10min for 3cycles; 94°C for 30s, 57°C for 30s, and 68°C for 10min for 21cycles; and then 68°C for 10min. About 1 μl of first-round PCR reaction product was amplified using following primers FWD-2: ACAGGGACTTGAAAGCGAAAG and REV-2: CTAGTTACCAGAGTCACACAACAGACG. Reaction mix and PCR conditions were identical to the first round PCR. PCR products were visualized on 1% agarose gel and gel eluted using QIAquick gel extraction kit (Qiagen).
About 10ng of HSIV-vifNL4-3 was amplified with following primers: 3LTR-V-F90: TGTGTGACTCTGGTAA CTAGAGATCCCTCAGACCCTTTTAGTCAGTGTGGAAAAT CTC TAGCA CCCAGGA GGTAGAGGTTGCAGTGAGC and 5HIV-R2: CTTTCGCTTTCAAGTCCCTGTTCGGGCGCCA in a 50μl reaction volume. Platinum superfi II high-fidelity DNA polymerase (Thermofisher) was used for amplification of vector PCR product. Vector PCR product was visualized on 1% agarose gel and gel eluted using QIAqucik gel extraction kit.
Nested PCR amplification of near full length genome (NFLG) PCR product and vector PCR product were mixed with a minimum of 1:5 ratio in a 20μl reaction volume containing 10μl of HiFi assembly mix. After 1h of incubation at 50°C, 5μl of reaction mix was used for transformation of NEB STABLE cells (New England BioLabs). Miniprep plasmids were isolated using QIAprep spin miniprep kit (Qiagen). Plasmids containing full-length genomes were screened by restriction enzyme digestion with SalI and BamHI enzymes.
To determine whether the plasmids containing full length clones generate infectious virus, transfection supernatants were generated by transfecting 293T cells with proviral clones. Infectious nature of the supernatants was determined by infecting TZM-bl cells. Sequences of IMCs were determined by using primers targeting different regions of the genome that support coverage of the entire length of the genome.
Parental sequences for possible recombinant sequences were identified using Spits tree v4.17.1 (Huson and Bryant, 2006), and regions with evidence of recombination were confirmed by RAPR.1 Because indels are unlikely to emerge independently in the exact same position and with the same length, gaps in the alignment were also considered informative.
Sequences of HSIV-P3-114, HSIV-P3-161, and HSIV-P3-284 are deposited under accession numbers MZ146778, MZ146779, and MZ146780, respectively.
We previously reported HSIV-vifNL4-3 could persistently infect PTMs (Thippeshappa et al., 2011). We continued to monitor the viral loads in two of those HSIV-vifNL4-3-infected PTMs for nearly 4years (Figure 1A). Although viral RNA was below the detection limit at most of the late time points measured, viral DNA could be detected in PBMCs through 200wpi. Additionally, we recovered infectious virus from PBMCs at 196 and 200wpi, suggesting that the virus had been replicating in the animals for nearly 4years. Interestingly, one of the animals (M08009), despite low or undetectable viral loads, showed a gradual decline and then stably depressed CD4+ T cell counts, suggesting disease progression (Figure 1B).
Figure 1. Long-term monitoring of HSIV-vifNL4-3 infected pigtailed macaques (PTMs). Two juvenile PTMs (F08003 and M08009) were inoculated intravenously with HSIV-vifNL4-3. Plasma viral RNA loads (A) and CD4+ T cell counts (B) were measured at various time points post-infection. Data up to 90weeks post-infection (wpi) have been published previously (Thippeshappa et al., 2011). PTM M08009 shows gradual decline in CD4+ T cells at late-stage of infection.
We recovered infectious virus by coculturing peripheral blood CD4+ T cells from infected PTM (M08009, Figure 1) at 196 and 200wpi with CEMx174 cells (biological isolates C/196 and C/200). C/196 and C/200 were susceptible to inhibition by AMD3100 but not Maraviroc, suggesting that viral isolates were only CXCR4-tropic (Supplementary Figure 4). We next determined the replication capacity of C/196 and C/200 in PTM CD4+ T cells. Although the differences were not statistically significant, C/196 replicated to higher levels in PTM CD4+ T cells in both the presence (22-fold) and absence (17-fold) of IFNα relative to the parental clone, HSIV-vifNL4-3 (Figure 2). While C/200 displayed more limited replication, it was not affected by the addition of IFNα (Supplementary Figure 5). Partial genome sequencing analysis of PCR amplified fragments of C/196 and C/200 indicated that both isolates were clonal (Supplementary Figure 6). We also observed that both C/196 and C/200 were neutralization resistant to sera from M08009, the animal in which it evolved, as well as sera from the other PTM, F08003 that had been infected with HSIV-vifNL4-3 (Table 1). The emergence of immune escape variants of HSIV-vifNL4-3 suggest that it had persistently replicated in PTMs.
Figure 2. Replication kinetics of biological isolates of HSIV-vifNL4-3 (C/196 and C/200). PTM CD4+ T cells were infected in duplicate at a MOI of 0.01 with the parental HSIV-vifNL4-3 or variant isolates C/196 in the presence or absence of IFNα (200U/ml) in the culture media. Virus supernatants were collected every 3–4dpi and p24 was quantified by ELISA.
An explanation for the low but persistent replication of HSIV-vifNL4-3 in vivo is that the virus is attenuated because it does not express accessory proteins necessary for more robust viral replication. Two possible proteins that could enhance replication of HSIV-vifNL4-3 are the HIV-1 Vpr or SIV Vpx. Since we introduced SIV vif, which includes a partial open reading frame (ORF) for vpx into HIV-1 backbone, we determined whether it had affected the expression of HIV-1 vpr. Indeed, HIV-1 Vpr protein was not observed in virions of HSIV-vif clones, HSIV-vifNL4-3 or HSIV-vifAD8 (Figure 3). We determined that this is because singly spiced HIV-1 vpr RNA from HSIV-vif is generated using the splice acceptor site within the SIV vif gene (Supplementary Figure 1). The transcript, therefore, includes the partial ORF for the SIV Vpx protein upstream of the translational initiation site for HIV-1 Vpr, which may interfere with its expression (Supplementary Figure 1).
Figure 3. Vpr expression from HSIV clones. 293T cells were transfected with HSIV clones. At 48h post-transfection, virus supernatants were collected and concentrated by centrifugation. Virion lysates were analyzed by western blot using antibody to HIV-1 Vpr and Nef.
Vpr is a small 96 amino acid (14kDa) protein that is not required for HIV-1 replication in vitro. However, it is conserved among all primate lentiviruses, indicating its importance for pathogenesis (Tristem et al., 1992, 1998). Therefore, we modified HSIV-vif clones to express Vpr. We deleted the sequence between the stop codon of vif and start codon of vpr and disrupted the vpx translational start codon and other ATG codons upstream of the vpr initiation site by site directed mutagenesis (Supplementary Figures 1 and 8). Mutation of these three ATG codons, one of which results in M181L in SIV Vif, resulted in expression of Vpr, which is incorporated into progeny virions (Vpr+ HSIV-vifNL4-3 and Vpr+ HSIV-vifAD8, Figure 3). Antibody to Nef was used as a control for incorporation of a virion-associated protein in HSIV-vif clones. Additionally, since Vpx counteracts the function of SAMHD1 (Hrecka et al., 2011; Laguette et al., 2011) and is essential for replication of SIV in macaques (Hirsch et al., 1998; Belshan et al., 2012; Shingai et al., 2015) and because HSIV-vif already has a partial ORF for vpx, we also generated an HSIV-vif derivative carrying the full-length vpx gene (Supplementary Figure 2). We used HSIV-vifYu2, which is IFN-resistant (Thippeshappa et al., 2013), to generate HSIV-vif-vpx carrying the full-length vpx gene (named HSIV-vif-vpxYu2). By Western blot using rabbit anti-serum to HIV-2Rod Vpx protein, Vpx could be detected in the cell lysates of SIV, HIV-2, and HSIV-vif-vpxYu2, but not SIVΔVpx. However, it was not detected in virion lysates of HSIV-vif-vpxYu2 (Supplementary Figure 2) as HIV-1 does not have determinants in p6 Gag required for virion incorporation of Vpx (Sunseri et al., 2011).
We tested the effect of Vpr and Vpx expression on HSIV-vif replication in an immortalized PTM CD4+ T cell line (Munoz et al., 2009). PTM CD4+ T cells were infected with Vpr- (HSIV-vifNL4-3, HSIV-vifAD8, and HSIV-vifYu2), Vpr+ (Vpr+HSIV-vifNL4-3, Vpr+HSIV-vifAD8, and Vpr+HSIV-vifYu2), HSIV-vif-vpxYu2, or wild type HIV-1 (NL4-3, NL-AD8, and Bru-Yu2) viruses at an MOI of 0.01. Vpr+ HSIV-vif viruses, and HSIV-vif-vpxYu2 replicated in PTM CD4+ T cells to similar levels as Vpr- viruses (Figure 4). Expectedly, wild type HIV-1 (NL-AD8 and Bru-Yu2) failed to replicate (Figure 4).
Figure 4. Vpr and Vpx expressing HSIV replicate in PTM CD4 T cells. Cells were infected in duplicate with HSIV-vifNL4-3 (A), HSIV-vifAD8 (B), and HSIV-vifYu2 (C) variants at an MOI of 0.01. Virus supernatants were collected every 3–4dpi and assayed for p24 levels.
We also determined the replication capacity of vpr and vpx carrying HSIV clones in human MDMs. Human MDMs were generated using previously described methods (Biesinger et al., 2010) and infected with the Vpx+, Vpr+, and Vpr- HSIV-vif clones at an MOI of 0.01. Vpr+ HSIV- vifAD8 (Figure 5A) and Vpr+ HSIV-vifYu2 (Figure 5B) replicated to similar levels as Vpr- HSIV-vifAD8 and Vpr+ HSIV-vifYu2, but slightly less than wild type HIV-1 NL-AD8 and Bru-Yu2 (Figures 5A,B), although it was not statistically significant. Additionally, HSIV-vif-vpxYu2 replicated as well as the parental HIV-1 Bru-Yu2 (Figure 5B).
Figure 5. Vpr and Vpx expressing HSIV replicate in monocyte-derived macrophages (MDMs). Cells were infected in duplicate with HSIV-vifAD8 (A) and HSIV-vifYu2 (B) clones expressing Vpr or Vpx or neither at an MOI of 0.01. Virus supernatants were collected every 3–4dpi and assayed for p24 levels.
Because HSIV-vifNL4-3 replication in the initial PTM experiment was low with peak viremia <105 copies/ml (Figure 1; Thippeshappa et al., 2011), we conducted animal to animal transfer of infected PTM peripheral blood to adapt HSIV-vif to PTMs. For this experiment, the initial inoculum contained a mixture of CXCR4- (C/196 and C/200) and CCR5-tropic viruses (Vpr+ HSIV-vifAD8, Vpr+ HSIV-vifYu2, and HSIV-vif-vpxYu2). At 14wpi, pooled blood from infected PTMs (Z09080 and Z09067) was used to inoculate a naïve macaque (Z13086). At 8wpi, peripheral blood from Z13086 was transferred into an additional PTM (Z13098). Interestingly, all the macaques showed a peak viremia close to or above 105 copies/ml and the viral loads persisted for at least 20wpi (Figure 6A). Furthermore, increases in antibody titer over time suggest that all PTMs were persistently infected with HSIV-vif (Figure 6B). However, CD4+ T cell decline was not observed in the infected PTMs (Figure 6C).
Figure 6. Passaging of HSIV to generate pathogenic variants. Passage 1 macaques were infected with a mixture of viruses. At 14wpi, pooled blood from infected PTMs (Z09080 and Z09067) was used to inoculate a naïve macaque (Z13086) and then blood from Z13086 at 8wpi was passaged through an additional PTM (Z13098). At several time points post-inoculation, peripheral blood was drawn for measuring plasma viral loads (A), antibody titer (B), and CD4 T cell counts (C).
We generated IMCs from proviral DNA isolated from PBMCs of the passage 3 macaque (Z13098). Near full-length proviral genomes (NFLG) were amplified using nested PCR as described by Hiener et al. (2017) with slight modifications in PCR conditions. NFLGs was cloned into a vector PCR product containing 5′ LTR sequences (amplified from HSIV-vifNL4-3 plasmid) using NEBuilder HiFi assembly mix (Supplementary Figure 3). Briefly, ends of NFLG PCR product and vector PCR product containing 5′LTR sequences overlap with each other, which can be assembled using NEBuilder HiFi assembly mix. We screened nearly 300 colonies to identify 54 plasmids with full length genomes. To determine whether they could produce infectious virus, full length clone plasmids were transfected into 293T cells to generate virus. Infectious nature of the supernatants was determined by infecting TZM-bl cells. Three of the 54 plasmid clones tested (HSIV-P3-114, HSIV-P3-161, and HSIV-P3-284) produced measurable infectious virus. DNA sequencing showed that the three IMCs were closely related to the C/196 and C/200 biological clones of HSIV-vifNL4-3 (Table 2; Supplementary Figures 5, 6). Importantly, the three IMCs had more nonsynonymous mutations (Table 2) than synonymous mutations (Supplementary Table 1) throughout the genome suggestive of adaptation to PTMs. Most of the mutations in Env and Nef were seen in the biological clones of HSIV-vifNL4-3 (C/196 and C/200) recovered from PTM M08009 (Table 2), suggesting that these mutations have persisted through three additional in vivo passages. In the Vpr+ HSIV-vif clones, we had mutated SIV vpx ATG codon to ACG (silent mutation) and ATG codon at amino acid position 181 in SIV vif to TTG, which codes for leucine (M181L substitution; Supplementary Figure 1). However, SIV vpx start codon was present in the recovered IMCs. Additionally, ATA codon was present at amino acid position 181, which codes for isoleucine (M181I substitution). Further, all the recovered IMCs had the deletion of bases between SIV vif stop codon and vpr start codon (Supplementary Figure 8), suggesting that these clones could express Vpr protein. Third, recombination analysis using RAPR program suggested that the recovered IMCs are recombinants that consist of biological clone C/196 with an insertion of 737bp region spanning the 3′ end of vif to 5′ end of vpu (nucleotides 5,447–6,171 according to HXB2 reference sequence) from Vpr+HSIV-vifAD8. We confirmed the expression of Vpr from HSIV-P3 IMCs by western blot using rabbit anti-sera against Vpr protein (Figure 7). Interestingly, virion-associated Vpr was higher than that for the VPR+ HSIV-vifNL4-3 clone.
Figure 7. Vpr expression from HSIV-P3 IMCs clones. 293T cells were transfected with HSIV-P3 IMCs. At 48h post-transfection, virus supernatants were collected and concentrated by centrifugation. Virion lysates were analyzed by western blot using antibody to HIV-1 Vpr and p24.
We determined whether HSIV-P3 IMCs (HSIV-P3-114, HSIV-P3-161, and HSIV-P3-284) replicate in PTM PBMCs. PBMCs isolated from different donor PTMs were activated with concanavalin A for 3days and maintained in IL-2 containing media for 2days. Activated PBMCs were infected with HSIV-P3 IMCs and Vpr+ HSIV-vifNL4-3 at an MOI of 0.01. Viral supernatants were collected at various days post-infection to assay for p24 levels. We observed that HSIV-P3 IMCs replicated with different efficiency in PBMCs from different donor PTMs (Figure 8). Among the three HSIV-P3 IMCs, HSIV-P3-284 replicated to similar levels as Vpr+HSIV-vifNL4-3 in activated PBMCs.
Figure 8. HSIV-P3 IMCs replicate in PTM peripheral blood mononuclear cells (PBMCs). PBMCs from different donor PTMs were activated with concanavalin A for 3days and maintained in media containing IL-2 (40U/ml) for 2days. Cells were then infected in duplicate with HSIV-P3 IMCs or parental Vpr+ HSIV-vifNL4-3 virus at an MOI of 0.01, and supernatants were assayed for p24 by ELISA every 3–4dpi.
Since first-generation SHIV constructs replicated poorly in macaques, serial in vivo passages were conducted to enhance their infectivity or replicative capacity (Luciw et al., 1995; Joag et al., 1996; Reimann et al., 1996; Igarashi et al., 1999; Chen et al., 2000; Song et al., 2006). We previously reported the construction of HSIV-vifNL4-3 and replication trend in juvenile and newborn PTMs (Thippeshappa et al., 2011). Although HSIVvifNL4-3 persisted for nearly 4years, the peak viremia was below 105 copies/ml, rapidly declined, and was intermittently detectable thereafter in juvenile PTMs. Therefore, we conducted serial in vivo passaging of HSIV-vif in PTMs using a mixture of different molecular clones and variants as an initial inoculum. We report consistent replication of HSIV with peak plasma viral RNA levels close to or greater than 1×105 viral RNA copies/ml and continuously detectable for 20–30wpi in the passaged macaques. Additionally, we performed passaging at 14 (passage 2) and 8wpi (passage 3) when the viral loads were low in the donor monkey. Therefore, peak viremia of 105 copies/ml in the recipient monkey suggests consistent replication of HSIV in PTMs. To further characterize HSIV-vif selected in PTMs, we PCR cloned and generated IMCs from the passage 3 macaque using DNA isolated from PBMCs from passage 3 PTM at 4wpi. Our characterization indicated that the selected variants appear to be Vpr expressing recombinants of the HSIV-vifNL4-3 biological isolates from the long-term infected PTM with depressed CD4+ T cell counts. These data suggest that neutralization resistant variants which had evolved in association with CD4+ T cell decline and acquired the ability to express the HIV-1 Vpr had a fitness advantage for replication in PTMs (Overview of the experiments and data is provided in Supplementary Figure 9).
We have previously reported potential reasons for the attenuated replication of HSIV-vifNL4-3 in PTMs. In those studies, we noticed that HSIV-vifNL4-3 was inefficient in degrading PTM APOBEC3 family restriction factors compared to highly pathogenic SIVmne027 (Thippeshappa et al., 2011). We also observed that replication of HSIV-vifNL4-3 is inhibited in the presence of IFNα in PTM CD4 T cells (Thippeshappa et al., 2013). This perhaps suggests that HSIV-vifNL4-3 may not be able to overcome type I IFN response induced during acute stage of infection. Here, we also show that HSIV-vif clones do not express Vpr due to interference by the partial vpx ORF in the singly spliced vpr mRNA. We speculate that the absence of Vpr expression may have affected viral replication of HSIV-vifNL4-3 in the initial inoculation of PTMs (Figure 1).
HIV-1 Vpr incorporates into virions through an interaction with p6 of Gag (Bachand et al., 1999; Selig et al., 1999). In vitro studies have attributed several biological function to Vpr, which include: (i) cell cycle arrest and apoptosis (Di Marzio et al., 1995; He et al., 1995; Jowett et al., 1995; Planelles et al., 1995; Re et al., 1995; Bartz et al., 1996; Goh et al., 1998; Stewart et al., 1999, 2000; Zhang and Bieniasz, 2020); (ii) nuclear import of viral DNA (Popov et al., 1998a,b; Le Rouzic et al., 2002; Riviere et al., 2010); (iii) regulation of viral gene expression (Forget et al., 1998; Subbramanian et al., 1998; Vanitharani et al., 2001; Yurkovetskiy et al., 2018); (iv) infection of nondividing cells (Balliet et al., 1994; Connor et al., 1995; Campbell and Hirsch, 1997; Miller et al., 2017); (v) modulation of immune responses (Ayyavoo et al., 2002; Muthumani et al., 2004, 2005; Majumder et al., 2005, 2008; Okumura et al., 2008; Doehle et al., 2009; Khan et al., 2020); and (vi) interaction with uracil DNA glycosylase (UNG2), a DNA repair enzyme that specifically removes uracil from DNA, and reduction of G to A mutations during reverse transcription (Mansky et al., 2000; Chen et al., 2004; Ahn et al., 2010). Since Vpr performs multiple functions during HIV replication, we hypothesized that absence of HIV-1 Vpr expression in HSIV-vifNL4-3 may affect persistent viral replication in PTMs.
It is interesting that even without Vpr expression, HSIV-vifNL4-3 persisted for nearly 4years (Figure 1). While the functions of Vpr have not been clearly defined in vivo, the potential importance of Vpr for HSIV-vif replication in pigtails is supported by pathogenesis studies of SIVmac, which demonstrate deletion of either Vpr or Vpx alone or together attenuates viral replication and ability to cause disease (Lang et al., 1993; Gibbs et al., 1995), and Vpx is necessary for SIV infection of CD4+ T cells in vivo (Belshan et al., 2012). Interestingly, Vpr null viruses reverted to Vpr expressing virus in SIV-infected macaques (Lang et al., 1993; Hoch et al., 1995), suggesting the importance of Vpr for in vivo pathogenesis. We speculated that the HIV-1 Vpr expressing HSIV may replicate better than the parental Vpr- HSIV-vifNL4-3 in vivo. Therefore, we generated HSIV derivatives expressing HIV-1 Vpr by introducing mutations in ATG codons upstream of the Vpr start codon. Since Vpx performs similar roles as Vpr and SIV vif gene already has partial ORF for vpx, we also generated an HSIV-vif derivative expressing the full length vpx gene. We cloned full-length vpx gene into HSIV-vifYu2 backbone, as we have previously shown that this clone resists IFN treatment in PTM CD4 T cells. HSIV-vif clones expressing either the HIV-1 vpr or SIV vpx were replication competent in vitro. However, accessory proteins such as Vpr and Vpx are not necessary for HIV-1 or SIV replication in vitro. Therefore, it is difficult to show the impact of Vpr or Vpx expression for HSIV-vif replication using in vitro studies. Infecting PTMs with different clones would be a better method to define the significance of the HIV-1 vpr for HSIV-vif replication. Indeed, in our passage studies, we used both HIV-1 Vpr- and Vpr+ HSIV to determine the importance of HIV-1 Vpr for in vivo pathogenesis in PTMs. Recovery of Vpr+ HSIV IMCs from the passage 3 macaque again suggests a role for Vpr in pathogenesis in vivo.
In our studies to characterize persistent HSIV-vif variants, we have developed and standardized a rapid and robust approach to generate IMCs from proviral DNA. We screened 54 plasmids for their ability to generate infectious virus. Out of which, only three generated infectious virus, which roughly correspond to 5% of total clones. This is not surprising as 90–95% of the proviral DNA is noninfectious (Ho et al., 2013; Bruner et al., 2016; Hiener et al., 2017). Interestingly, recovered IMCs from passage 3 macaque were Vpr+ HSIV-vifNL4-3. Although PCR-mediated recombination event is possible (Liu et al., 2014), this suggested a possible recombination between Vpr+ HSIV-vif clones (either HSIV-vifAD8 or HSIV-vifYu2) with biological isolates C/196 and C/200 recovered from M08009. Three observations suggest a recombination event: First, the three recovered IMCs had deletion of bases between SIV vif stop codon and HIV-1 vpr start codon. Second, most mutations observed in env and nef were already present in the HSIV-vifNL4-3 biological clones (C/196 and C/200) from M08009. Third, an analysis using RAPR program indicated that recovered IMCs may have resulted from recombination between biological clone C/196 and Vpr+HSIV-vifAD8, with a segment spanning from within vif to vpu of Vpr+ HSIV-vifAD8 that includes the mutation necessary for Vpr expression inserted into the biological clone C/196. Therefore, the recombination event to generate Vpr+ HSIV-P3 IMCs demonstrates the importance of HIV-1 Vpr for HSIV-vif pathogenesis in vivo.
Although we used mixture of CXCR4- and CCR5-tropic viruses for inoculation into passage 1 PTMs, it is interesting that CXCR4-tropic HSIV-vifNL4-3 persisted through three passages. We have previously reported that SIV variants emerging during late-stage disease have a higher replicative capacity and increased pathogenicity (Kimata et al., 1999). Similar observations have also been made with SHIV-1157ipd (Song et al., 2006). Therefore, recovered virus (C/196 or C/200) isolated during the late stage in our study may have greater fitness for replication in PTMs. Since recovered virus was also neutralization resistant, it may have helped the virus overcome antibody responses during additional passages. We also observed several nonsynonymous mutations throughout the genome of HSIV-P3-IMCs. Most of the mutations were shared among the three HSIV-P3 IMCs. However, HSIV-P3 IMCs also had mutations unique to each of the clones. We speculate that these mutations could help the virus overcome restriction factors, better utilize host dependency factors, or they could help the virus escape adaptive immune responses.
In conclusions, our results suggest that serial in vivo passaging improves HSIV replication and persistence in PTMs. Identification of several nonsynonymous mutations in IMCs recovered from passage 3 macaque also indicated that serial in vivo passaging helps in acquisition of mutations. Since these mutations are in the context of replication competent virus, they may play a significant role in the replication and pathogenesis in vivo. However, a shortcoming of the studies is the limited duration of the passage experiment and limited number of animals used for the study. While variants of HSIV-vifNL4-3 that acquired the ability to express the HIV-1 Vpr appear to have a selective advantage, the short duration of the experiments was insufficient to determine if the selected variants had increased pathogenicity. However, the in vivo data show consistent replication of HSIV-vif to 105 viral RNA copies/ml in four PTMs. Further in vivo passaging of HSIV-P3 IMCs with longer follow-up periods will be necessary to verify their increased replication fitness, and to generate pathogenic variants with enhanced replication capacity. Development of such pathogenic variants will be valuable as challenge viruses for preclinical evaluation of novel vaccines and therapeutics, as these HSIV clones have all the HIV immunologic and vaccine targets, such as Gag, Pol, Env, Tat, Rev., and Nef. Furthermore, establishment of HIV reservoirs in this model also provides an avenue for developing therapeutic vaccination approaches targeting HIV Gag, Pol, and Env, apart from testing latency reversal agents and cure strategies.
Sequences of HSIV-P3-114, HSIV-P3-161, and HSIV-P3-284 are deposited under accession numbers MZ146778, MZ146779, and MZ146780, respectively.
The animal study was reviewed and approved by University of Washington.
RT designed studies, performed experiments, and wrote manuscript. PP coordinated and analyzed data from animal studies and edited manuscript. SSC designed and performed experiments and edited manuscript. KT and AM performed experiments and edited manuscript. PCA performed experiments and edited manuscript. S-LH obtained funding, coordinated animal studies, and edited manuscript. DK obtained funding, coordinated studies, and edited manuscript. JTK obtained funding, designed studies, performed experiments, and wrote manuscript. All authors contributed to the article and approved the submitted version.
Funding support to PCA (Fulbright-CONICET Scholar award), S-LH (P51 ODO10425), DK (P51 OD011133 and U42OD010442), and JTK [AI108467, AI116167, AI08467, and the Texas D-CFAR (AI161943)].
The authors declare that the research was conducted in the absence of any commercial or financial relationships that could be construed as a potential conflict of interest.
All claims expressed in this article are solely those of the authors and do not necessarily represent those of their affiliated organizations, or those of the publisher, the editors and the reviewers. Any product that may be evaluated in this article, or claim that may be made by its manufacturer, is not guaranteed or endorsed by the publisher.
The following reagents were obtained through the NIH HIV reagent program, Division of AIDS, NIAID, NIH: Polyclonal Anti-Human Immunodeficiency Virus Type 2ROD Vpx Protein (antiserum, Rabbit), ARP-2609, contributed by Lee Ratner, Washington University; Polyclonal Anti-Human Immunodeficiency Virus Type 1 Vpr Protein, Residues 1–50 (antiserum, Rabbit), ARP-11836, contributed by Jeffrey Kopp; Polyclonal Anti-Human Immunodeficiency Virus Type 1 Nef Protein (antiserum, Rabbit), ARP-2949, contributed by Ronald Swanstrom; Anti-Human Immunodeficiency Virus 1 (HIV-1) p24 Gag Monoclonal (#24-3), ARP-6458, contributed by Michael Malim; Human Immunodeficiency Virus 1 (HIV-1), Strain NL4-3 Infectious Molecular Clone (pNL4-3), ARP-2852, contributed by M. Martin; Human Immunodeficiency Virus 1 (HIV-1) AD8 Infectious Molecular Clone [pNL(AD8)], ARP-11346, contributed by Eric O. Freed; Clone, ARP-1350, contributed by Beatrice Hahn and George M. Shaw; and TZM-bl Cells, ARP-8129, contributed by John C. Kappes and Xiaoyun Wu.
The Supplementary Material for this article can be found online at: https://www.frontiersin.org/articles/10.3389/fmicb.2021.779460/full#supplementary-material
Agy, M. B., Frumkin, L. R., Corey, L., Coombs, R. W., Wolinsky, S. M., Koehler, J., et al. (1992). Infection of Macaca nemestrina by human immunodeficiency virus type-1. Science 257, 103–106. doi: 10.1126/science.1621083
Agy, M. B., Schmidt, A., Florey, M. J., Kennedy, B. J., Schaefer, G., Katze, M. G., et al. (1997). Serial in vivo passage of HIV-1 infection in Macaca nemestrina. Virology 238, 336–343. doi: 10.1006/viro.1997.8832
Ahn, J., Vu, T., Novince, Z., Guerrero-Santoro, J., Rapic-Otrin, V., and Gronenborn, A. M. (2010). HIV-1 Vpr loads uracil DNA glycosylase-2 onto DCAF1, a substrate recognition subunit of a cullin 4A-ring E3 ubiquitin ligase for proteasome-dependent degradation. J. Biol. Chem. 285, 37333–37341. doi: 10.1074/jbc.M110.133181
Ayyavoo, V., Muthumani, K., Kudchodkar, S., Zhang, D., Ramanathan, P., Dayes, N. S., et al. (2002). HIV-1 viral protein R compromises cellular immune function in vivo. Int. Immunol. 14, 13–22. doi: 10.1093/intimm/14.1.13
Bachand, F., Yao, X. J., Hrimech, M., Rougeau, N., and Cohen, E. A. (1999). Incorporation of Vpr into human immunodeficiency virus type 1 requires a direct interaction with the p6 domain of the p55 gag precursor. J. Biol. Chem. 274, 9083–9091. doi: 10.1074/jbc.274.13.9083
Balliet, J. W., Kolson, D. L., Eiger, G., Kim, F. M., McGann, K. A., Srinivasan, A., et al. (1994). Distinct effects in primary macrophages and lymphocytes of the human immunodeficiency virus type 1 accessory genes vpr, vpu, and nef: mutational analysis of a primary HIV-1 isolate. Virology 200, 623–631. doi: 10.1006/viro.1994.1225
Bartz, S. R., Rogel, M. E., and Emerman, M. (1996). Human immunodeficiency virus type 1 cell cycle control: Vpr is cytostatic and mediates G2 accumulation by a mechanism which differs from DNA damage checkpoint control. J. Virol. 70, 2324–2331. doi: 10.1128/jvi.70.4.2324-2331.1996
Belshan, M., Kimata, J. T., Brown, C., Cheng, X., McCulley, A., Larsen, A., et al. (2012). Vpx is critical for SIVmne infection of pigtail macaques. Retrovirology 9:32. doi: 10.1186/1742-4690-9-32
Biesinger, T., White, R., Yu Kimata, M. T., Wilson, B. K., Allan, J. S., and Kimata, J. T. (2010). Relative replication capacity of phenotypic SIV variants during primary infections differs with route of inoculation. Retrovirology 7:88. doi: 10.1186/1742-4690-7-88
Bosch, M. L., Schmidt, A., Agy, M. B., Kimball, L. E., and Morton, W. R. (1997). Infection of Macaca nemestrina neonates with HIV-1 via different routes of inoculation. AIDS 11, 1555–1563. doi: 10.1097/00002030-199713000-00003
Bosch, M. L., Schmidt, A., Chen, J., Florey, M. J., Agy, M., and Morton, W. R. (2000). Enhanced replication of HIV-1 in vivo in pigtailed macaques (Macaca nemestrina). J. Med. Primatol. 29, 107–113. doi: 10.1034/j.1600-0684.2000.290303.x
Brennan, G., Kozyrev, Y., and Hu, S. L. (2008). TRIMCyp expression in Old World primates Macaca nemestrina and Macaca fascicularis. Proc. Natl. Acad. Sci. U. S. A. 105, 3569–3574. doi: 10.1073/pnas.0709511105
Bruner, K. M., Murray, A. J., Pollack, R. A., Soliman, M. G., Laskey, S. B., Capoferri, A. A., et al. (2016). Defective proviruses rapidly accumulate during acute HIV-1 infection. Nat. Med. 22, 1043–1049. doi: 10.1038/nm.4156
Campbell, B. J., and Hirsch, V. M. (1997). Vpr of simian immunodeficiency virus of African green monkeys is required for replication in macaque macrophages and lymphocytes. J. Virol. 71, 5593–5602. doi: 10.1128/jvi.71.7.5593-5602.1997
Chen, Z., Huang, Y., Zhao, X., Skulsky, E., Lin, D., Ip, J., et al. (2000). Enhanced infectivity of an R5-tropic simian/human immunodeficiency virus carrying human immunodeficiency virus type 1 subtype C envelope after serial passages in pig-tailed macaques (Macaca nemestrina). J. Virol. 74, 6501–6510. doi: 10.1128/JVI.74.14.6501-6510.2000
Chen, R., Le Rouzic, E., Kearney, J. A., Mansky, L. M., and Benichou, S. (2004). Vpr-mediated incorporation of UNG2 into HIV-1 particles is required to modulate the virus mutation rate and for replication in macrophages. J. Biol. Chem. 279, 28419–28425. doi: 10.1074/jbc.M403875200
Connor, R. I., Chen, B. K., Choe, S., and Landau, N. R. (1995). Vpr is required for efficient replication of human immunodeficiency virus type-1 in mononuclear phagocytes. Virology 206, 935–944. doi: 10.1006/viro.1995.1016
Derdeyn, C. A., Decker, J. M., Sfakianos, J. N., Wu, X., O’Brien, W. A., Ratner, L., et al. (2000). Sensitivity of human immunodeficiency virus type 1 to the fusion inhibitor T-20 is modulated by coreceptor specificity defined by the V3 loop of gp120. J. Virol. 74, 8358–8367. doi: 10.1128/JVI.74.18.8358-8367.2000
Di Marzio, P., Choe, S., Ebright, M., Knoblauch, R., and Landau, N. R. (1995). Mutational analysis of cell cycle arrest, nuclear localization and virion packaging of human immunodeficiency virus type 1 Vpr. J. Virol. 69, 7909–7916. doi: 10.1128/jvi.69.12.7909-7916.1995
Doehle, B. P., Hladik, F., McNevin, J. P., McElrath, M. J., and Gale, M. Jr. (2009). Human immunodeficiency virus type 1 mediates global disruption of innate antiviral signaling and immune defenses within infected cells. J. Virol. 83, 10395–10405. doi: 10.1128/JVI.00849-09
Doi, N., Miura, T., Mori, H., Sakawaki, H., Koma, T., Adachi, A., et al. (2018). CXCR4- and CCR5-tropic HIV-1 clones are Both tractable to grow in rhesus macaques. Front. Microbiol. 9:2510. doi: 10.3389/fmicb.2018.02510
Doi, N., Okubo, A., Yamane, M., Sakai, Y., Adachi, A., and Nomaguchi, M. (2013). Growth potentials of CCR5-tropic/CXCR4-tropic HIV-1mt clones in macaque cells. Front. Microbiol. 4:218. doi: 10.3389/fmicb.2013.00218
Doi, N., Sakai, Y., Adachi, A., and Nomaguchi, M. (2017). Generation and characterization of new CCR5-tropic HIV-1rmt clones. J. Med. Investig. 64, 272–279. doi: 10.2152/jmi.64.272
Forget, J., Yao, X. J., Mercier, J., and Cohen, E. A. (1998). Human immunodeficiency virus type 1 vpr protein transactivation function: mechanism and identification of domains involved. J. Mol. Biol. 284, 915–923. doi: 10.1006/jmbi.1998.2206
Frumkin, L. R., Agy, M. B., Coombs, R. W., Panther, L., Morton, W. R., Koehler, J., et al. (1993). Acute infection of Macaca nemestrina by human immunodeficiency virus type 1. Virology 195, 422–431. doi: 10.1006/viro.1993.1392
Gartner, S., Liu, Y., Lewis, M. G., Polonis, V., Elkins, W. R., Zack, P. M., et al. (1994a). HIV-1 infection in pigtailed macaques. AIDS Res. Hum. Retrovir. 10, S129–S133.
Gartner, S., Liu, Y., Polonis, V., Lewis, M. G., Elkins, W. R., Hunter, E. A., et al. (1994b). Adaptation of HIV-1 to pigtailed macaques. J. Med. Primatol. 23, 155–163.
Gibbs, J. S., Lackner, A. A., Lang, S. M., Simon, M. A., Sehgal, P. K., Daniel, M. D., et al. (1995). Progression to AIDS in the absence of a gene for vpr or vpx. J. Virol. 69, 2378–2383. doi: 10.1128/jvi.69.4.2378-2383.1995
Goh, W. C., Rogel, M. E., Kinsey, C. M., Michael, S. F., Fultz, P. N., Nowak, M. A., et al. (1998). HIV-1 Vpr increases viral expression by manipulation of the cell cycle: a mechanism for selection of Vpr in vivo. Nat. Med. 4, 65–71. doi: 10.1038/nm0198-065
Hatziioannou, T., Ambrose, Z., Chung, N. P., Piatak, M. Jr., Yuan, F., Trubey, C. M., et al. (2009). A macaque model of HIV-1 infection. Proc. Natl. Acad. Sci. U. S. A. 106, 4425–4429. doi: 10.1073/pnas.0812587106
Hatziioannou, T., Del Prete, G. Q., Keele, B. F., Estes, J. D., McNatt, M. W., Bitzegeio, J., et al. (2014). HIV-1-induced AIDS in monkeys. Science 344, 1401–1405. doi: 10.1126/science.1250761
Hatziioannou, T., Princiotta, M., Piatak, M. Jr., Yuan, F., Zhang, F., Lifson, J. D., et al. (2006). Generation of simian-tropic HIV-1 by restriction factor evasion. Science 314:95. doi: 10.1126/science.1130994
He, J., Choe, S., Walker, R., Di Marzio, P., Morgan, D. O., and Landau, N. R. (1995). Human immunodeficiency virus type 1 viral protein R (Vpr) arrests cells in the G2 phase of the cell cycle by inhibiting p34cdc2 activity. J. Virol. 69, 6705–6711. doi: 10.1128/jvi.69.11.6705-6711.1995
Hiener, B., Horsburgh, B. A., Eden, J. S., Barton, K., Schlub, T. E., Lee, E., et al. (2017). Identification of genetically intact HIV-1 proviruses in specific CD4(+) T cells from effectively treated participants. Cell Rep. 21, 813–822. doi: 10.1016/j.celrep.2017.09.081
Hirsch, V. M., Sharkey, M. E., Brown, C. R., Brichacek, B., Goldstein, S., Wakefield, J., et al. (1998). Vpx is required for dissemination and pathogenesis of SIV(SM) PBj: evidence of macrophage-dependent viral amplification. Nat. Med. 4, 1401–1408. doi: 10.1038/3992
Ho, Y. C., Shan, L., Hosmane, N. N., Wang, J., Laskey, S. B., Rosenbloom, D. I., et al. (2013). Replication-competent noninduced proviruses in the latent reservoir increase barrier to HIV-1 cure. Cell 155, 540–551. doi: 10.1016/j.cell.2013.09.020
Hoch, J., Lang, S. M., Weeger, M., Stahl-Hennig, C., Coulibaly, C., Dittmer, U., et al. (1995). Vpr deletion mutant of simian immunodeficiency virus induces AIDS in rhesus monkeys. J. Virol. 69, 4807–4813. doi: 10.1128/jvi.69.8.4807-4813.1995
Hrecka, K., Hao, C., Gierszewska, M., Swanson, S. K., Kesik-Brodacka, M., Srivastava, S., et al. (2011). Vpx relieves inhibition of HIV-1 infection of macrophages mediated by the SAMHD1 protein. Nature 474, 658–661. doi: 10.1038/nature10195
Hu, S. L., Zarling, J. M., Chinn, J., Travis, B. M., Moran, P. A., Sias, J., et al. (1989). Protection of macaques against simian AIDS by immunization with a recombinant vaccinia virus expressing the envelope glycoproteins of simian type D retrovirus. Proc. Natl. Acad. Sci. U. S. A. 86, 7213–7217. doi: 10.1073/pnas.86.18.7213
Huson, D. H., and Bryant, D. (2006). Application of phylogenetic networks in evolutionary studies. Mol. Biol. Evol. 23, 254–267. doi: 10.1093/molbev/msj030
Igarashi, T., Endo, Y., Englund, G., Sadjadpour, R., Matano, T., Buckler, C., et al. (1999). Emergence of a highly pathogenic simian/human immunodeficiency virus in a rhesus macaque treated with anti-CD8 mAb during a primary infection with a nonpathogenic virus. Proc. Natl. Acad. Sci. U. S. A. 96, 14049–14054. doi: 10.1073/pnas.96.24.14049
Igarashi, T., Iyengar, R., Byrum, R. A., Buckler-White, A., Dewar, R. L., Buckler, C. E., et al. (2007). Human immunodeficiency virus type 1 derivative with 7% simian immunodeficiency virus genetic content is able to establish infections in pig-tailed macaques. J. Virol. 81, 11549–11552. doi: 10.1128/JVI.00960-07
Joag, S. V., Li, Z., Foresman, L., Stephens, E. B., Zhao, L. J., Adany, I., et al. (1996). Chimeric simian/human immunodeficiency virus that causes progressive loss of CD4+ T cells and AIDS in pig-tailed macaques. J. Virol. 70, 3189–3197. doi: 10.1128/jvi.70.5.3189-3197.1996
Jowett, J. B., Planelles, V., Poon, B., Shah, N. P., Chen, M. L., and Chen, I. S. (1995). The human immunodeficiency virus type 1 vpr gene arrests infected T cells in the G2 + M phase of the cell cycle. J. Virol. 69, 6304–6313. doi: 10.1128/jvi.69.10.6304-6313.1995
Kamada, K., Igarashi, T., Martin, M. A., Khamsri, B., Hatcho, K., Yamashita, T., et al. (2006). Generation of HIV-1 derivatives that productively infect macaque monkey lymphoid cells. Proc. Natl. Acad. Sci. U. S. A. 103, 16959–16964. doi: 10.1073/pnas.0608289103
Khan, H., Sumner, R. P., Rasaiyaah, J., Tan, C. P., Rodriguez-Plata, M. T., Van Tulleken, C., et al. (2020). HIV-1 Vpr antagonizes innate immune activation by targeting karyopherin-mediated NF-kappaB/IRF3 nuclear transport. elife 9:e60821. doi: 10.7554/eLife.60821
Kimata, J. T., Kuller, L., Anderson, D. B., Dailey, P., and Overbaugh, J. (1999). Emerging cytopathic and antigenic simian immunodeficiency virus variants influence AIDS progression. Nat. Med. 5, 535–541. doi: 10.1038/8414
Kimata, J. T., Mozaffarian, A., and Overbaugh, J. (1998). A lymph node-derived cytopathic simian immunodeficiency virus Mne variant replicates in nonstimulated peripheral blood mononuclear cells. J. Virol. 72, 245–256. doi: 10.1128/JVI.72.1.245-256.1998
Kimata, J. T., Wilson, J. M., and Patel, P. G. (2004). The increased replicative capacity of a late-stage simian immunodeficiency virus mne variant is evident in macrophage- or dendritic cell-T-cell cocultures. Virology 327, 307–317. doi: 10.1016/j.virol.2004.07.010
Laguette, N., Sobhian, B., Casartelli, N., Ringeard, M., Chable-Bessia, C., Segeral, E., et al. (2011). SAMHD1 is the dendritic- and myeloid-cell-specific HIV-1 restriction factor counteracted by Vpx. Nature 474, 654–657. doi: 10.1038/nature10117
Lang, S. M., Weeger, M., Stahl-Hennig, C., Coulibaly, C., Hunsmann, G., Muller, J., et al. (1993). Importance of vpr for infection of rhesus monkeys with simian immunodeficiency virus. J. Virol. 67, 902–912. doi: 10.1128/jvi.67.2.902-912.1993
Le Rouzic, E., Mousnier, A., Rustum, C., Stutz, F., Hallberg, E., Dargemont, C., et al. (2002). Docking of HIV-1 Vpr to the nuclear envelope is mediated by the interaction with the nucleoporin hCG1. J. Biol. Chem. 277, 45091–45098. doi: 10.1074/jbc.M207439200
Liao, C. H., Kuang, Y. Q., Liu, H. L., Zheng, Y. T., and Su, B. (2007). A novel fusion gene, TRIM5-Cyclophilin A in the pig-tailed macaque determines its susceptibility to HIV-1 infection. AIDS 21, S19–S26. doi: 10.1097/01.aids.0000304692.09143.1b
Liu, J., Song, H., Liu, D., Zuo, T., Lu, F., Zhuang, H., et al. (2014). Extensive recombination due to heteroduplexes generates large amounts of artificial gene fragments during PCR. PLoS One 9:e106658. doi: 10.1371/journal.pone.0106658
Luciw, P. A., Pratt-Lowe, E., Shaw, K. E., Levy, J. A., and Cheng-Mayer, C. (1995). Persistent infection of rhesus macaques with T-cell-line-tropic and macrophage-tropic clones of simian/human immunodeficiency viruses (SHIV). Proc. Natl. Acad. Sci. U. S. A. 92, 7490–7494.
Majumder, B., Janket, M. L., Schafer, E. A., Schaubert, K., Huang, X. L., Kan-Mitchell, J., et al. (2005). Human immunodeficiency virus type 1 Vpr impairs dendritic cell maturation and T-cell activation: implications for viral immune escape. J. Virol. 79, 7990–8003. doi: 10.1128/JVI.79.13.7990-8003.2005
Majumder, B., Venkatachari, N. J., O’Leary, S., and Ayyavoo, V. (2008). Infection with Vpr-positive human immunodeficiency virus type 1 impairs NK cell function indirectly through cytokine dysregulation of infected target cells. J. Virol. 82, 7189–7200. doi: 10.1128/JVI.01979-07
Mansky, L. M., Preveral, S., Selig, L., Benarous, R., and Benichou, S. (2000). The interaction of vpr with uracil DNA glycosylase modulates the human immunodeficiency virus type 1 In vivo mutation rate. J. Virol. 74, 7039–7047. doi: 10.1128/JVI.74.15.7039-7047.2000
Miller, C. M., Akiyama, H., Agosto, L. M., Emery, A., Ettinger, C. R., Swanstrom, R. I., et al. (2017). Virion-associated Vpr alleviates a Postintegration block to HIV-1 infection of dendritic cells. J. Virol. 91, e00051–e00017. doi: 10.1128/JVI.00051-17
Misra, A., Gleeson, E., Wang, W., Ye, C., Zhou, P., and Kimata, J. T. (2018). Glycosyl-phosphatidylinositol-anchored anti-HIV Env single-chain variable fragments interfere with HIV-1 Env processing and viral infectivity. J. Virol. 92, e02080–e02017. doi: 10.1128/JVI.02080-17
Munoz, N. M., Trobridge, G. D., and Kiem, H. P. (2009). Ex vivo expansion and lentiviral transduction of Macaca nemestrina CD4+ T cells. J. Med. Primatol. 38, 438–443. doi: 10.1111/j.1600-0684.2009.00383.x
Muthumani, K., Desai, B. M., Hwang, D. S., Choo, A. Y., Laddy, D. J., Thieu, K. P., et al. (2004). HIV-1 Vpr and anti-inflammatory activity. DNA Cell Biol. 23, 239–247. doi: 10.1089/104454904773819824
Muthumani, K., Hwang, D. S., Choo, A. Y., Mayilvahanan, S., Dayes, N. S., Thieu, K. P., et al. (2005). HIV-1 Vpr inhibits the maturation and activation of macrophages and dendritic cells in vitro. Int. Immunol. 17, 103–116. doi: 10.1093/intimm/dxh190
Newman, R. M., Hall, L., Kirmaier, A., Pozzi, L. A., Pery, E., Farzan, M., et al. (2008). Evolution of a TRIM5-CypA splice isoform in old world monkeys. PLoS Pathog. 4:e1000003. doi: 10.1371/journal.ppat.1000003
Nomaguchi, M., Yokoyama, M., Kono, K., Nakayama, E. E., Shioda, T., Doi, N., et al. (2013). Generation of rhesus macaque-tropic HIV-1 clones that are resistant to major anti-HIV-1 restriction factors. J. Virol. 87, 11447–11461. doi: 10.1128/JVI.01549-13
Okumura, A., Alce, T., Lubyova, B., Ezelle, H., Strebel, K., and Pitha, P. M. (2008). HIV-1 accessory proteins VPR and Vif modulate antiviral response by targeting IRF-3 for degradation. Virology 373, 85–97. doi: 10.1016/j.virol.2007.10.042
Otsuki, H., Yoneda, M., Igarashi, T., and Miura, T. (2014). Generation of a monkey-tropic human immunodeficiency virus type 1 carrying env from a CCR5-tropic subtype C clinical isolate. Virology 460-461, 1–10. doi: 10.1016/j.virol.2014.04.037
Planelles, V., Bachelerie, F., Jowett, J. B., Haislip, A., Xie, Y., Banooni, P., et al. (1995). Fate of the human immunodeficiency virus type 1 provirus in infected cells: a role for vpr. J. Virol. 69, 5883–5889. doi: 10.1128/jvi.69.9.5883-5889.1995
Polacino, P., Cleveland, B., Zhu, Y., Kimata, J. T., Overbaugh, J., Anderson, D., et al. (2007). Immunogenicity and protective efficacy of gag/pol/Env vaccines derived from temporal isolates of SIVmne against cognate virus challenge. J. Med. Primatol. 36, 254–265. doi: 10.1111/j.1600-0684.2007.00243.x
Popov, S., Rexach, M., Ratner, L., Blobel, G., and Bukrinsky, M. (1998a). Viral protein R regulates docking of the HIV-1 preintegration complex to the nuclear pore complex. J. Biol. Chem. 273, 13347–13352.
Popov, S., Rexach, M., Zybarth, G., Reiling, N., Lee, M. A., Ratner, L., et al. (1998b). Viral protein R regulates nuclear import of the HIV-1 pre-integration complex. EMBO J. 17, 909–917.
Re, F., Braaten, D., Franke, E. K., and Luban, J. (1995). Human immunodeficiency virus type 1 Vpr arrests the cell cycle in G2 by inhibiting the activation of p34cdc2-cyclin B. J. Virol. 69, 6859–6864. doi: 10.1128/jvi.69.11.6859-6864.1995
Reimann, K. A., Li, J. T., Veazey, R., Halloran, M., Park, I. W., Karlsson, G. B., et al. (1996). A chimeric simian/human immunodeficiency virus expressing a primary patient human immunodeficiency virus type 1 isolate env causes an AIDS-like disease after in vivo passage in rhesus monkeys. J. Virol. 70, 6922–6928. doi: 10.1128/jvi.70.10.6922-6928.1996
Riviere, L., Darlix, J. L., and Cimarelli, A. (2010). Analysis of the viral elements required in the nuclear import of HIV-1 DNA. J. Virol. 84, 729–739. doi: 10.1128/JVI.01952-09
Saito, A., and Akari, H. (2013). Macaque-tropic human immunodeficiency virus type 1: breaking out of the host restriction factors. Front. Microbiol. 4:187. doi: 10.3389/fmicb.2013.00187
Saito, A., Nomaguchi, M., Iijima, S., Kuroishi, A., Yoshida, T., Lee, Y.-J., et al. (2011). Improved capacity of a monkey-tropic HIV-1 derivative to replicate in cynomolgus monkeys with minimal modifications. Microbes Infect. 13, 58–64. doi: 10.1016/j.micinf.2010.10.001
Saito, A., Nomaguchi, M., Kono, K., Iwatani, Y., Yokoyama, M., Yasutomi, Y., et al. (2013). TRIM5 genotypes in cynomolgus monkeys primarily influence inter-individual diversity in susceptibility to monkey-tropic human immunodeficiency virus type 1. J. Gen. Virol. 94, 1318–1324. doi: 10.1099/vir.0.050252-0
Schmidt, F., Keele, B. F., Del Prete, G. Q., Voronin, D., Fennessey, C. M., Soll, S., et al. (2019). Derivation of simian tropic HIV-1 infectious clone reveals virus adaptation to a new host. Proc. Natl. Acad. Sci. U. S. A. 116, 10504–10509. doi: 10.1073/pnas.1818059116
Selig, L., Pages, J. C., Tanchou, V., Preveral, S., Berlioz-Torrent, C., Liu, L. X., et al. (1999). Interaction with the p6 domain of the gag precursor mediates incorporation into virions of Vpr and Vpx proteins from primate lentiviruses. J. Virol. 73, 592–600. doi: 10.1128/JVI.73.1.592-600.1999
Shingai, M., Welbourn, S., Brenchley, J. M., Acharya, P., Miyagi, E., Plishka, R. J., et al. (2015). The expression of functional Vpx during pathogenic SIVmac infections of rhesus macaques suppresses SAMHD1 in CD4+ memory T cells. PLoS Pathog. 11:e1004928. doi: 10.1371/journal.ppat.1004928
Song, R. J., Chenine, A. L., Rasmussen, R. A., Ruprecht, C. R., Mirshahidi, S., Grisson, R. D., et al. (2006). Molecularly cloned SHIV-1157ipd3N4: a highly replication-competent, mucosally transmissible R5 simian-human immunodeficiency virus encoding HIV clade C Env. J. Virol. 80, 8729–8738. doi: 10.1128/JVI.00558-06
Stewart, S. A., Poon, B., Jowett, J. B., Xie, Y., and Chen, I. S. (1999). Lentiviral delivery of HIV-1 Vpr protein induces apoptosis in transformed cells. Proc. Natl. Acad. Sci. U. S. A. 96, 12039–12043.
Stewart, S. A., Poon, B., Song, J. Y., and Chen, I. S. (2000). Human immunodeficiency virus type 1 vpr induces apoptosis through caspase activation. J. Virol. 74, 3105–3111. doi: 10.1128/JVI.74.7.3105-3111.2000
Subbramanian, R. A., Kessous-Elbaz, A., Lodge, R., Forget, J., Yao, X. J., Bergeron, D., et al. (1998). Human immunodeficiency virus type 1 Vpr is a positive regulator of viral transcription and infectivity in primary human macrophages. J. Exp. Med. 187, 1103–1111. doi: 10.1084/jem.187.7.1103
Sunseri, N., O'Brien, M., Bhardwaj, N., and Landau, N. R. (2011). Human immunodeficiency virus type 1 modified to package simian immunodeficiency virus Vpx efficiently infects macrophages and dendritic cells. J. Virol. 85, 6263–6274. doi: 10.1128/JVI.00346-11
Thippeshappa, R., Kimata, J. T., and Kaushal, D. (2020). Toward a macaque model of HIV-1 infection: roadblocks, Progress, and future strategies. Front. Microbiol. 11:882. doi: 10.3389/fmicb.2020.00882
Thippeshappa, R., Polacino, P., Yu Kimata, M. T., Siwak, E. B., Anderson, D., Wang, W., et al. (2011). Vif substitution enables persistent infection of pig-tailed macaques by human immunodeficiency virus type 1. J. Virol. 85, 3767–3779. doi: 10.1128/JVI.02438-10
Thippeshappa, R., Ruan, H., and Kimata, J. T. (2012). Breaking barriers to an AIDS model with macaque-tropic HIV-1 derivatives. Biology 1, 134–164. doi: 10.3390/biology1020134
Thippeshappa, R., Ruan, H., Wang, W., Zhou, P., and Kimata, J. T. (2013). A variant macaque-tropic human immunodeficiency virus type 1 is resistant to alpha interferon-induced restriction in pig-tailed macaque CD4+ T cells. J. Virol. 87, 6678–6692. doi: 10.1128/JVI.00338-13
Tristem, M., Marshall, C., Karpas, A., and Hill, F. (1992). Evolution of the primate lentiviruses: evidence from vpx and vpr. EMBO J. 11, 3405–3412. doi: 10.1002/j.1460-2075.1992.tb05419.x
Tristem, M., Purvis, A., and Quicke, D. L. (1998). Complex evolutionary history of primate lentiviral vpr genes. Virology 240, 232–237. doi: 10.1006/viro.1997.8929
Vanitharani, R., Mahalingam, S., Rafaeli, Y., Singh, S. P., Srinivasan, A., Weiner, D. B., et al. (2001). HIV-1 Vpr transactivates LTR-directed expression through sequences present within −278 to −176 and increases virus replication in vitro. Virology 289, 334–342. doi: 10.1006/viro.2001.1153
Virgen, C. A., Kratovac, Z., Bieniasz, P. D., and Hatziioannou, T. (2008). Independent genesis of chimeric TRIM5-cyclophilin proteins in two primate species. Proc. Natl. Acad. Sci. U. S. A. 105, 3563–3568. doi: 10.1073/pnas.0709258105
Wei, X., Decker, J. M., Liu, H., Zhang, Z., Arani, R. B., Kilby, J. M., et al. (2002). Emergence of resistant human immunodeficiency virus type 1 in patients receiving fusion inhibitor (T-20) monotherapy. Antimicrob. Agents Chemother. 46, 1896–1905. doi: 10.1128/AAC.46.6.1896-1905.2002
Wu, X., Parast, A. B., Richardson, B. A., Nduati, R., John-Stewart, G., Mbori-Ngacha, D., et al. (2006). Neutralization escape variants of human immunodeficiency virus type 1 are transmitted from mother to infant. J. Virol. 80, 835–844. doi: 10.1128/JVI.80.2.835-844.2006
Yurkovetskiy, L., Guney, M. H., Kim, K., Goh, S. L., McCauley, S., Dauphin, A., et al. (2018). Primate immunodeficiency virus proteins Vpx and Vpr counteract transcriptional repression of proviruses by the HUSH complex. Nat. Microbiol. 3, 1354–1361. doi: 10.1038/s41564-018-0256-x
Keywords: HIV-1, SIV, HSIV-vif, nonhuman primates, pigtailed macaques, animal model, in vivo passaging, infectious molecular clones
Citation: Thippeshappa R, Polacino P, Chandrasekar SS, Truong K, Misra A, Aulicino PC, Hu S-L, Kaushal D and Kimata JT (2021) In vivo Serial Passaging of Human–Simian Immunodeficiency Virus Clones Identifies Characteristics for Persistent Viral Replication. Front. Microbiol. 12:779460. doi: 10.3389/fmicb.2021.779460
Received: 18 September 2021; Accepted: 22 October 2021;
Published: 18 November 2021.
Edited by:
Hirofumi Akari, Kyoto University, JapanReviewed by:
Frank Kirchhoff, University of Ulm, GermanyCopyright © 2021 Thippeshappa, Polacino, Chandrasekar, Truong, Misra, Aulicino, Hu, Kaushal and Kimata. This is an open-access article distributed under the terms of the Creative Commons Attribution License (CC BY). The use, distribution or reproduction in other forums is permitted, provided the original author(s) and the copyright owner(s) are credited and that the original publication in this journal is cited, in accordance with accepted academic practice. No use, distribution or reproduction is permitted which does not comply with these terms.
*Correspondence: Rajesh Thippeshappa, cnRoaXBwZXNoYXBwYUB0eGJpb21lZC5vcmc=; Deepak Kaushal, ZGthdXNoYWxAdHhiaW9tZWQub3Jn; Jason T. Kimata, amtpbWF0YUBiY20uZWR1
Disclaimer: All claims expressed in this article are solely those of the authors and do not necessarily represent those of their affiliated organizations, or those of the publisher, the editors and the reviewers. Any product that may be evaluated in this article or claim that may be made by its manufacturer is not guaranteed or endorsed by the publisher.
Research integrity at Frontiers
Learn more about the work of our research integrity team to safeguard the quality of each article we publish.