- 1Division of Acute Viral Diseases, Center for Emerging Virus Research, National Institute of Infectious Diseases, National Institute of Health, Korea Disease Control and Prevention Agency, Cheongju-si, South Korea
- 2Division of Emerging Virus and Vector Research, Center for Emerging Virus Research, National Institute of Infectious Diseases, National Institute of Health, Korea Disease Control and Prevention Agency, Cheongju-si, South Korea
- 3Center for Emerging Virus Research, National Institute of Infectious Diseases, National Institute of Health, Korea Disease Control and Prevention Agency, Cheongju-si, South Korea
Severe fever with thrombocytopenia syndrome virus (SFTSV) is an emerging, tick-borne Bandavirus that causes lethal disease in humans. As there are no licensed vaccines and therapeutics for SFTSV, there is an urgent need to develop countermeasures against it. In this respect, a reverse genetics (RG) system is a powerful tool to help achieve this goal. Herein, we established a T7 RNA polymerase-driven RG system to rescue infectious clones of a Korean SFTSV human isolate entirely from complementary DNA (cDNA). To establish this system, we cloned cDNAs encoding the three antigenomic segments into transcription vectors, with each segment transcribed under the control of the T7 promoter and the hepatitis delta virus ribozyme (HdvRz) sequences. We also constructed two helper plasmids expressing the nucleoprotein (NP) or viral RNA-dependent RNA polymerase (RdRp) under the control of the T7 promoter and the encephalomyocarditis virus (EMCV) internal ribosome entry site (IRES). After co-transfection into BHK/T7-9 cells with three transcription and two helper plasmids, then passaging in Vero E6 or Huh-7 cells, we confirmed efficient rescue of the recombinant SFTSV. By evaluating the in vitro and in vivo virological properties of the parental and rescued SFTSVs, we show that the rescued virus exhibited biological properties similar to those of the parental virus. This system will be useful for identifying molecular viral determinants of SFTSV infection and pathogenesis and for facilitating the development of vaccine and antiviral approaches.
Introduction
Severe fever with thrombocytopenia syndrome (SFTS), an emerging tick-borne viral infectious disease with a high fatality rate and symptoms including high fever, gastrointestinal symptoms, thrombocytopenia, and leukopenia, was first reported in China in 2009 (Yu et al., 2011) and was subsequently identified in South Korea and Japan in 2013 (Kim et al., 2013; Takahashi et al., 2014). Following the first report of SFTS in humans (Yu et al., 2011), the number of human cases has rapidly increased each year in East Asian countries. Unfortunately, currently there are no licensed vaccines or therapeutics for the prevention or treatment of severe fever with thrombocytopenia syndrome virus (SFTSV) infection.
Severe fever with thrombocytopenia syndrome virus, which has been renamed Dabie bandavirus belongs to the genus Bandavirus in the family Phenuiviridae of the order Bunyavirales (Kuhn et al., 2020). It has a tripartite RNA genome consisting of three negative-stranded RNA, designated large (L), medium (M), and small (S), which encode the RNA-dependent RNA polymerase (RdRp); the viral envelope glycoproteins (Gn and Gc); and a nucleocapsid protein (N) and a non-structural protein (NSs), respectively, in an ambisense orientation (Yu et al., 2011; Liu et al., 2014). The N and L proteins, together with the viral RNA, constitute a ribonucleoprotein (RNP) complex that is essential for genome replication and transcription (Zhou et al., 2013). It has been reported that SFTSV is transmitted via tick bites (Xu et al., 2011; Yu et al., 2011; Ding et al., 2014) and human-to-human transmission through close contact with the blood or body secretions of the infected patients (Bao et al., 2011; Gai et al., 2012; Liu et al., 2012; Chen et al., 2013; Tang et al., 2013; Kim et al., 2015).
The absence of vaccines and antiviral therapies against SFTSV emphasizes the need to focus on the molecular biology of SFTSV and its interaction with the host. In this respect, the reverse genetics (RG) system, which enables the generation of infectious virus from cloned complementary DNA (cDNA), is an effective tool for understanding the mechanisms of pathogenesis and the development of vaccines and therapeutics. To date, several groups have reported RG systems for the recovery of viruses belonging to the family Phenuiviridae, including Rift Valley fever virus (Ikegami et al., 2006; Gerrard et al., 2007; Billecocq et al., 2008; Habjan et al., 2008) and SFTSV (Brennan et al., 2015) using the T7 RNA polymerase or RNA polymerase I.
In the present study, we developed an RG system for the clinical SFTSV isolate KASJH from a Korean patient identified in 2014. We have characterized it using infectivity and pathogenicity tests in vitro and in vivo, as well as by genome sequencing. This system provides a platform for elucidating the molecular mechanisms involved in host tropism and pathogenesis of SFTSV infection.
Materials and Methods
Ethics Statement
All animal experiments were conducted in an enhanced biosafety level 3 (BSL3) laboratory in conformance with the guidelines of the Institutional Animal Care and Use Committee (IACUC) of the Korea Centers for Disease Control and Prevention (approval number, KCDC-073- 19-2A).
Cell Lines and Virus
BHK/T7-9 (RIKEN BRC cell bank, RCB4942, Ibaraki, Japan), a cell line derived from BHK-21 that stably expresses T7 RNA polymerase (Ito et al., 2003), was cultured in Dulbecco’s modified Eagle’s Medium (DMEM; Gibco) supplemented with 10% fetal bovine serum (FBS; Gibco), 10% tryptose phosphate broth (TPB; BD), and 1% penicillin/streptomycin at 37°C with 5% CO2. Vero E6 and Huh-7 cells were maintained in DMEM supplemented with 10% FBS and 1% antibiotics. The SFTSV strain KASJH was isolated from a Korean SFTS patient’s serum in 2014 (Yun et al., 2015).
Construction of Plasmids
Plasmids required for the rescue of SFTSV have been described previously (Brennan et al., 2015). We were kindly provided pTVT7-HB29L and pTM1-HB29L plasmids by Benjamin Brennan from MRC-University of Glasgow Centre for Virus Research. Using these plasmids as templates, we constructed plasmids containing the sequences of the Korean KASJH strain (GenBank accession nos. KP663731 to KP663733) on behalf of the Chinese HB29 strain using synthetic gene constructs and In-fusion cloning strategy. Plasmids containing full-length S, M, and L segments of KASJH strain in antigenomic sense flanked by the T7 promoter and hepatitis delta virus ribozyme (HdvRz) sequence were designated as pTVT7_KASJH_L(+), pTVT7_KASJH_M(+), and pTVT7_KASJH_S(+), respectively. Helper plasmids pTM1_KASJH_NP and pTM1_KASJH_RdRp contain the L and N open reading frames (ORFs) of KASJH under the control of the T7 promoter and encephalomyocarditis virus (EMCV) internal ribosome entry site (IRES) sequence. All plasmids were sequenced to ensure no undesired mutations. Primers used for the construction of these plasmids are available upon request.
Generation of Recombinant Severe Fever With Thrombocytopenia Syndrome Virus From Complementary DNA and Sequence Analysis
Subconfluent BHK/T7-9 cells (1 × 106 per 100-mm dish) were transfected using 3 μL Lipofectamine-3000 (Invitrogen) per ug of DNA in 500 μL Opti-MEM (Gibco), with 2 μg each of pTVT7_KASJH_L(+), pTVT7_KASJH_M(+), pTVT7_KASJH_S(+), 1 μg pTM1_KASJH_NP, and 0.2 μg pTM1_KASJH_RdRp. To distinguish between recombinant and parental viruses, we used an empty vector as a helper plasmid instead of one containing the KASJH RdRp gene. After 6 days incubation at 37°C, supernatants were collected, clarified, and passaged three times in Vero E6 or Huh-7 cells. Supernatants were harvested at 15 days post-infection (dpi). The rescued virus was designated rKASJH to distinguish it from the parental KASJH (wtKASJH) (Figure 1). To identify sequence differences between parental and rescued viruses, we sequenced the ORFs of these viruses using the Sanger method and DNAStar software (version 5.0.6) as previously described (Yun et al., 2017).
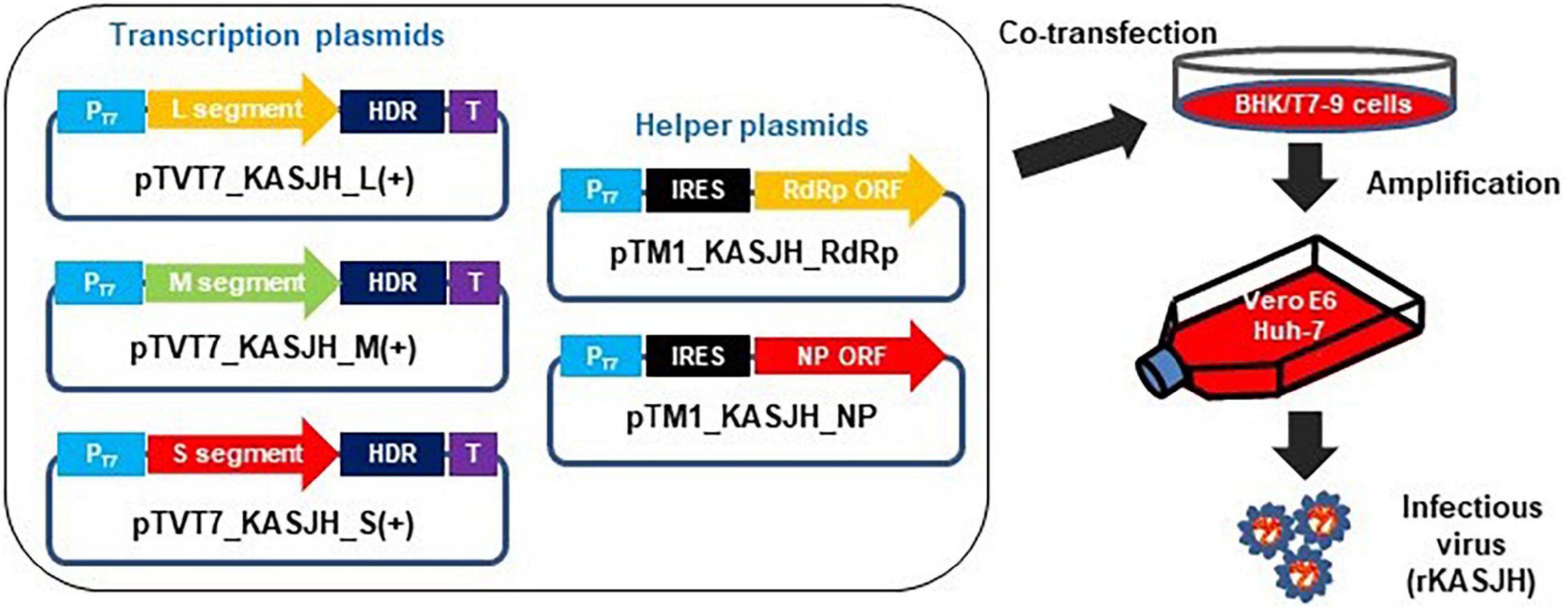
Figure 1. Schematic describing the generation of recombinant SFTSV using a reverse genetics system. BHK/T7-9 cells were co-transfected with three transcription and two helper plasmids. rKASJH was rescued from the culture supernatants of transfected BHK/T7-9 cells, followed by passages on Vero E6 or Huh-7 cells.
Growth Kinetics and Viral Protein Synthesis in Cell Culture
Subconfluent monolayer cultures of Vero E6 and Huh-7 cells growing in 24-well plates were infected with parental and rescued viruses at a multiplicity of infection (MOI) of 0.01 or 1. Cells were incubated at 37°C in 5% CO2. Supernatants were harvested daily for 7 days and stored in aliquots at −70°C prior to titration. The titers of SFTSV in daily samples were determined by focus formation on Vero E6 cells. Cells were also harvested from the daily samples described above for growth kinetics. Cell lysates were prepared and subjected to western blotting at the same time points to quantify the expression of viral N and NSs proteins.
Virus Titration
Vero E6 cells (3 × 105 per well) were seeded in 6-well plates and infected with 10-fold serial dilutions of virus for 1 h at 37°C, followed by the addition of 5 mL/well of overlay medium containing 2× DMEM (Welgene, Daegu, South Korea), 10% FBS, 1% penicillin/streptomycin, and 0.5% SeaKem LE Agarose (Lonza). Cells were incubated at 37°C for 7 days and then fixed with 10% formaldehyde in PBS. After washing with PBS, cells were permeabilized in 10% Triton X-100 in PBS for 10 min. Anti-SFTSV nucleoprotein (NP) monoclonal antibody (6B3, in-house) and HRP-conjugated anti-mouse IgG antibody were used for immunostaining the infected cells. Foci were detected using 3,3′-diaminobenzidine substrate (Vector Laboratories). Visible foci were counted and used to calculate viral titers.
Western Blotting
At different time points after infection, cell lysates were prepared by the addition of NP40 lysis buffer (Invitrogen) and protease inhibitor (Roche). Equal aliquots of lysates were heat denatured and separated on a 4–12% SDS-PAGE gel (Invitrogen). Separated proteins were transferred to a methanol-activated polyvinylidine difluoride (PVDF) membrane (Bio-Rad) and blocked in PBS containing 5% skim milk and 0.1% Tween 20. The blot was incubated with mouse anti-N (6B3, in-house), anti-NSs (8G4, in-house), and rabbit anti-GAPDH (Santa Cruz Biotechnology) monoclonal antibodies. The antigen-primary antibody complexes were recognized by alkaline phosphatase (AP)-conjugated goat anti-mouse or anti-rabbit IgG (Jackson ImmunoResearch) antibodies, as appropriate. Visualization of detected proteins was performed by leveraging the colorimetric detection of AP activity using 5-bromo-4-chloro-3-indolyl phosphate (BCIP) and nitro blue tetrazolium (NBT) substrate.
Pathogenicity in Mice
Mice lacking the type 1 interferon receptor (C57BL/6 IFNAR–/–) were purchased from the Jackson Laboratory. Briefly, 8 to 10-week-old male C57BL/6 IFNAR–/– mice (n = 5 per group) were infected with high and low (1 × 105 and 1 × 102 FFU, respectively) doses of SFTSV (100 μL) via the intramuscular (IM) route. DMEM was used to dilute the virus to the desired concentration. Control mock-infected mice were inoculated with DMEM by the same route. Body weight and clinical symptoms were observed for 14 days after inoculation; mice with a weight loss of more than 20% were euthanized. Spleen, liver, kidney, and serum samples were collected at 1, 3, and 5 dpi. Organs were individually weighed, homogenized, and prepared as 10% suspensions (w/v) in PBS containing 10% FBS. Suspensions were clarified by centrifugation (2,000 rpm for 5 min, 4°C) and stored at −70°C prior to titration. For virus titration in sera and the organ suspensions, focus-forming assays on Vero E6 cells were performed as described above. Viral RNA copy number was measured as described below.
Real-Time Quantitative Reverse Transcription Polymerase Chain Reaction
Total RNA was extracted using the QIAamp viral RNA Mini kit (Qiagen) according to the manufacturer’s protocol. Viral copy numbers were determined by real-time Reverse transcription polymerase chain reaction (qRT-PCR) with an M segment-based SFTSV-specific primer set. The forward primer was 5′-GTCATCATCATATTTTGTTCCTGATGC-3′ and the reverse primer was 5′-GGGCACCCAGACTGGCAG TC-3′. The probes were JOE/5′-TCCAGGTGTACATCAGTGAG GAGATGTCG-3′/BHQ and JOE/5′-TCCAGGTGTACATCTGT GAGGAGATGTCG-3′/BHQ. Copy numbers were calculated as ratios normalized to the control by a standard curve method. Real-time RT-PCRs were performed using a PowerChek SFTSV Real-time PCR kit (Kogenebiotech, Seoul, South Korea) on a 7500 Real-time RT-PCR system (Applied Biosystems).
Histopathological Analysis
Eight-to-ten-week-old male C57BL/6 IFNAR–/– mice in groups of three were inoculated IM with 100 μL of 102 FFU of either parental or rescued SFTSVs, whereas three control mice were mock inoculated with DMEM. All mice were observed daily and euthanized on day 1, 3, and 5 post-infection. Spleen, liver, and kidney tissues were separately harvested and fixed in 4% paraformaldehyde for 1 day at room temperature. For histopathology, fixed tissues were bisected, embedded in paraffin, sectioned at 3 μm, and stained with hematoxylin and eosin (H&E).
Statistical Analyses
All analyses were performed using GraphPad Prism version 7.0 (GraphPad Software Inc.). All data are presented as the mean ± s.d. (standard deviation). The in vitro growth kinetics were evaluated using an unpaired, two-tailed t-test. Survival was estimated using the Kaplan-Meier method and analyzed by log-rank analysis. For the analyses of viral load in serum and tissues, two-way analysis of variance (ANOVA) with Tukey’s test was used. P-values less than 0.05 were considered statistically significant.
Results
Recovery of Recombinant Severe Fever With Thrombocytopenia Syndrome Virus From Full-Length Complementary DNA Clones
We used a human-derived SFTSV isolate to construct the cDNA clone. This strain (KASJH) was isolated from a 69-year-old South Korean patient in 2014. As mentioned above, three transcription and two helper plasmids containing the viral sequences (GenBank accession Nos. KP667331-KP667333) were constructed using synthetic gene constructs and an In-Fusion cloning strategy. Sequences of the inserted viral genome were confirmed by sequencing. To recover rKASJH from full-length cDNA clones, three transcription (pTVT7_KASJH_L(+), pTVT7_KASJH_M(+), and pTVT7_KASJH_S(+)) and two helper plasmids (pTM1_KASJH_NP and pTM1_KASJH_RdRp) were co-transfected into BHK/T7-9 cells and passaged in Vero E6 or Huh-7 cells. We confirmed that the recombinant SFTSV was efficiently rescued by real-time qRT-PCR and focus-forming assays. We confirmed that the recombinant SFTSV was efficiently rescued by real-time qRT-PCR and focus-forming assays. The rescued viruses from passages in Huh-7 and Vero E6 cells reached a titer of 5.2 × 106 and 4.5 × 105 FFU/mL, respectively. The results revealed that the rescued virus from passages in Huh-7 cells replicated more effectively than in Vero E6 cells. No virus was detected in controls in which the helper plasmid encoding the RdRp sequence of KASJH was excluded. These results demonstrate that the recombinant virus was produced from cloned cDNAs following co-transfection and passage. As summarized in Table 1, comparison of the recombinant and parental virus sequences revealed two single-base mutations in the L segment, one of which was translationally silent.
In vitro Growth Properties of Parental Isolate and Rescued Virus
To analyze the in vitro properties of the recombinant SFTSV, we first examined plaque phenotypes and growth kinetics of the rKASJH compared with the parental KASJH strain in susceptible Vero E6 and Huh-7 cells over 7 dpi at MOIs of 0.01 and 1. As shown in Figure 2A, there was no obvious difference in the morphology of foci formed by the parental and recombinant SFTSVs. Comparing replication kinetics of parental and recombinant viruses in Vero E6 and Huh-7 cells, both cells infected with parental and rescued SFTSVs at MOIs of 0.01 and 1 exhibited cytopathic effects (CPE) on 3 to 7 dpi and rKASJH grew nearly as efficiently as wtKASJH, with minor differences in kinetics and maximum viral titers varying by cell type and MOI (Figure 2B). In Huh-7 cells infected with SFTSVs at an MOI of 1, the titer of rKASJH was >10-fold lower than that of wtKASJH at 1 dpi, comparable to that of wtKASJH at 2–7 dpi. Similar growth kinetics were also observed in Vero E6 cells. The peak titers in Huh-7 cells infected with wtKASJH and rKASJH at both MOIs were higher than those in Vero E6 cells.
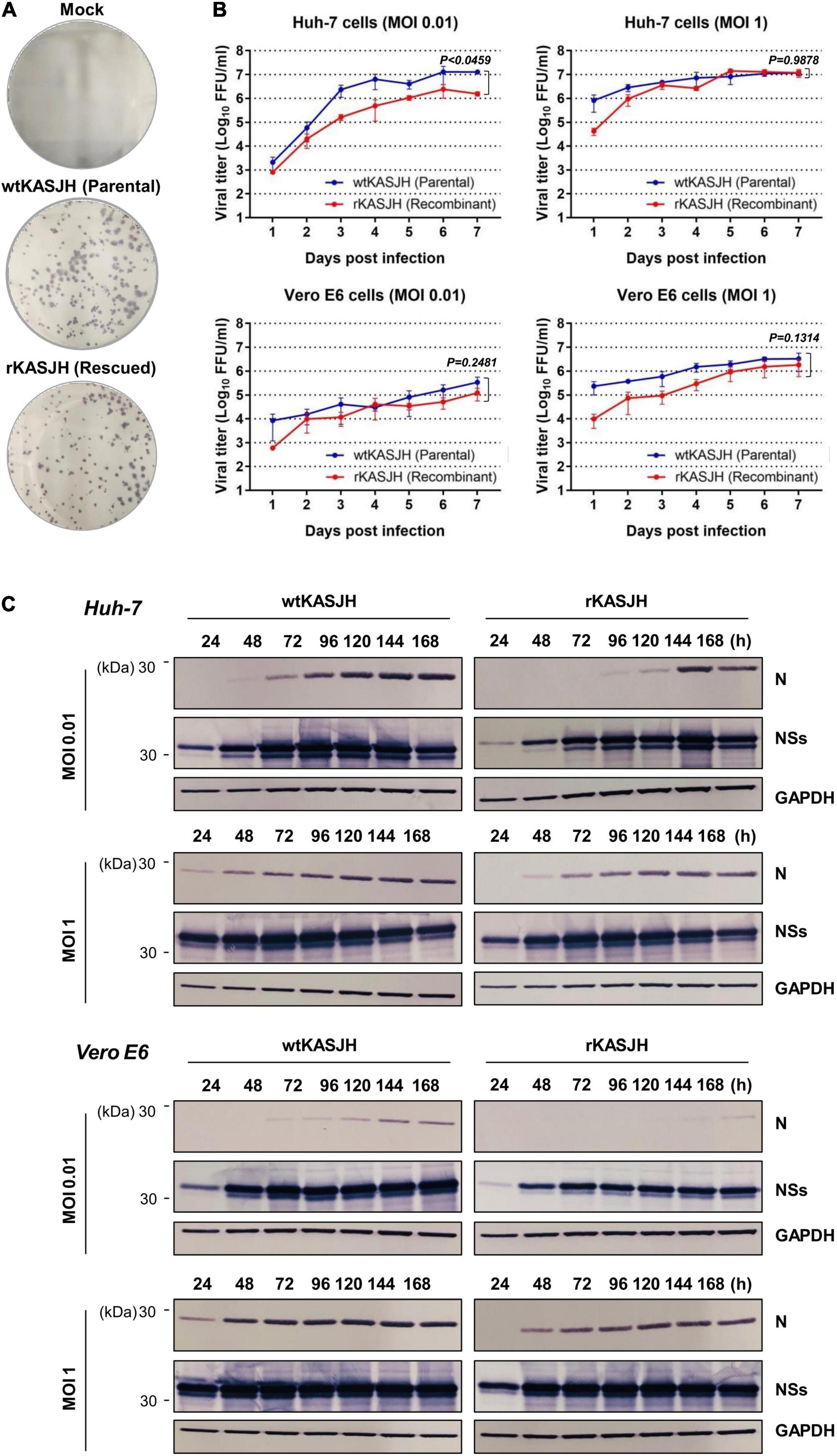
Figure 2. Characterization of parental and rescued SFTSVs in cell culture. (A) Representative focus morphology in Vero E6 cells immunostained with anti-SFTSV N antibody 7 dpi. (B) Growth kinetics of Vero E6 and Huh-7 cells infected with wtKASJH or rKASJH at MOIs of 0.01 and 1 FFU/cell and infections were performed in triplicate. Culture supernatants were collected from the infected cells at the indicated days after infection and virus titers were determined by focus-forming assays. Error bars indicate standard deviations of the mean. Statistical significance between wtKASJH and rKASJH infected groups was determined by an unpaired, two-tailed t-test. (C) Viral protein synthesis from parental and recombinant viruses in Vero E6 and Huh-7 cell lysates detected by immunoblotting with anti-SFTSV N, anti-SFTSV NSs, and anti-GAPDH antibodies.
To compare the viral protein expression profiles of wtKASJH and rKASJH, we measured the expression levels of viral N and NSs proteins produced in Vero E6 and Huh-7 cells at 1–7 dpi at MOIs of 0.01 and 1. Immunoblotting with mouse mAbs specific for SFTSV N and NSs revealed similar expression levels between viruses (Figure 2C).
Collectively, these results demonstrate that the recombinant SFTSV exhibited similar biological properties in vitro to those of the parental virus.
Pathogenesis of Parental Isolate and Rescued Virus in Mice
To compare the in vivo virulence of the parental and recombinant SFTSVs, we used 8 to 10-week-old C57BL/6 IFNAR–/– mice, a well-established immunodeficient mouse model for SFTSV infection. Groups of five mice were inoculated intramuscularly with high (105 FFU) and low (102 FFU) doses of wtKASJH and rKASJH. Compared with mock-infected mice, mice infected with the high dose of both the parental and rescued SFTSVs lost weight starting at 2–3 dpi. Mortality was observed starting on 4 dpi for both wtKASJH and rKASJH, with all mice dead at 5 dpi (Figures 3A,B). In mice infected with the low dose, both groups lost weight starting at 4–5 dpi. Except for one mouse infected with the low dose of wtKASJH, mortality was observed starting on 6 dpi for both the two viruses, with all mice dead at 7 dpi (Figures 3A,B). The log-rank test indicated that there was no significant difference in survival between groups (Figure 3B).
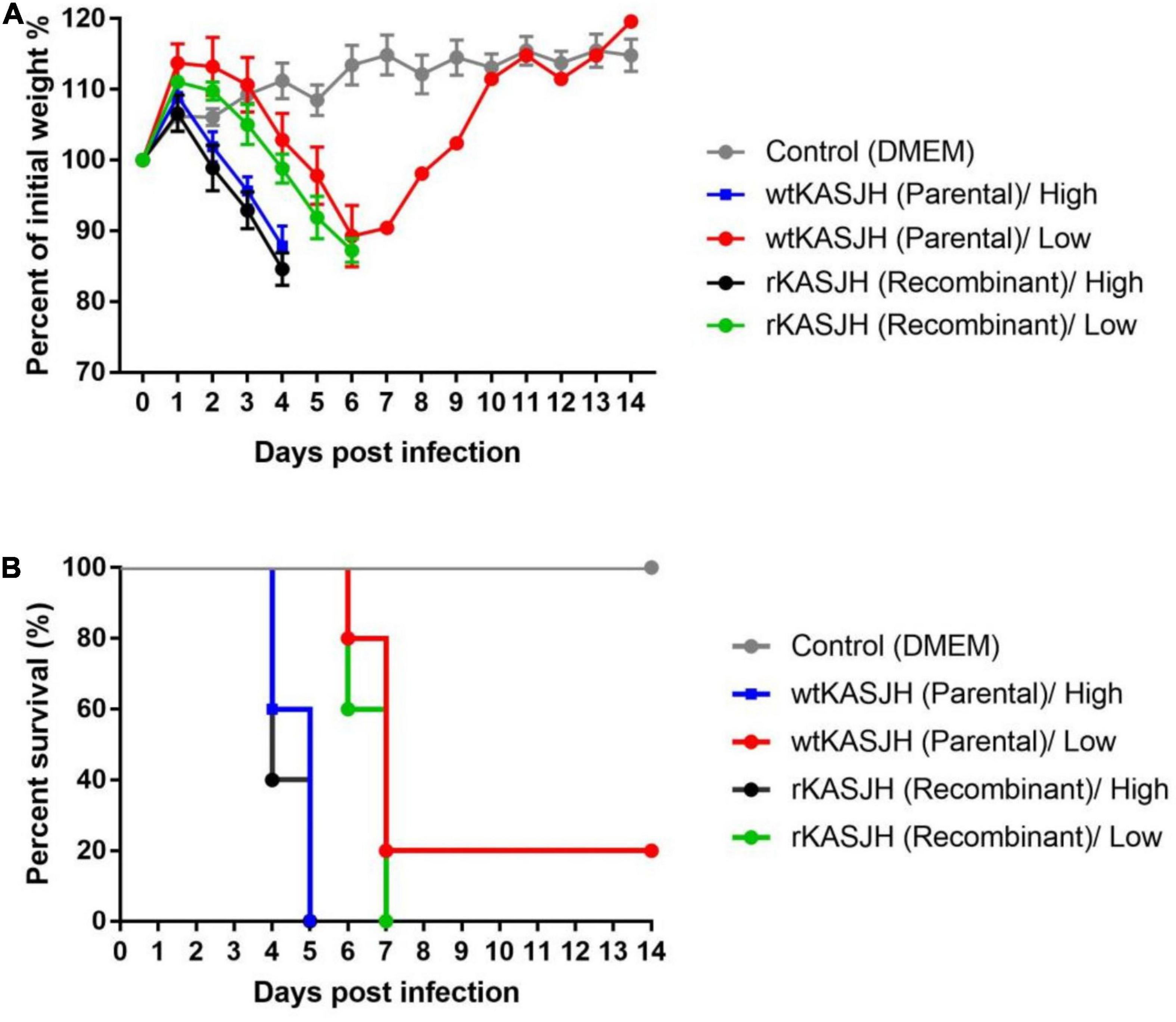
Figure 3. Virulence of parental and rescued SFTSVs in C57BL/6 IFNAR–/– mice. Groups of five mice were inoculated intramuscularly with high (1 × 105 FFU) and low (1 × 102 FFU) doses of the indicated viruses and then monitored for weight loss (A) and mortality rate (B). Error bars indicate standard deviations of the mean. Survival was quantified using the Kaplan-Meier method and tested for significance by log-rank analysis. ns, not significant.
To examine the viral tissue distribution and serum, tissue and serum samples were collected from 8 to 10-week-old male C57BL/6 IFNAR–/– mice in groups of three on 1, 3, and 5 dpi. Using the real-time qRT-PCR method, both wtKASJH and rKASJH were detected at 3 and 5 dpi in organs (spleen, liver, and kidney) and serum samples of the infected C57BL/6 IFNAR–/– mice (Figures 4A,B). Serum viral RNA copy number did not differ between wtKASJH and rKASJH-infected groups (Figure 4A). There was, however, a significant difference in copy number in tissues (spleen, liver, and kidney) at 5 dpi (Figure 4B). Remarkably, copy numbers were the highest in the serum, spleen, and kidney at 5 dpi. The viral titers in serum samples and tissues quantified by focus-forming assays revealed that viral load peaked at 5 dpi in the serum, spleen, liver, and kidney of both wtKASJH- and rKASJH-infected groups (Figures 4C,D). Among the organs used in this study, the spleen was the most susceptible organ, as it showed the highest viral titer (5.2log10FFU/g) 5 dpi after rKASJH infection.
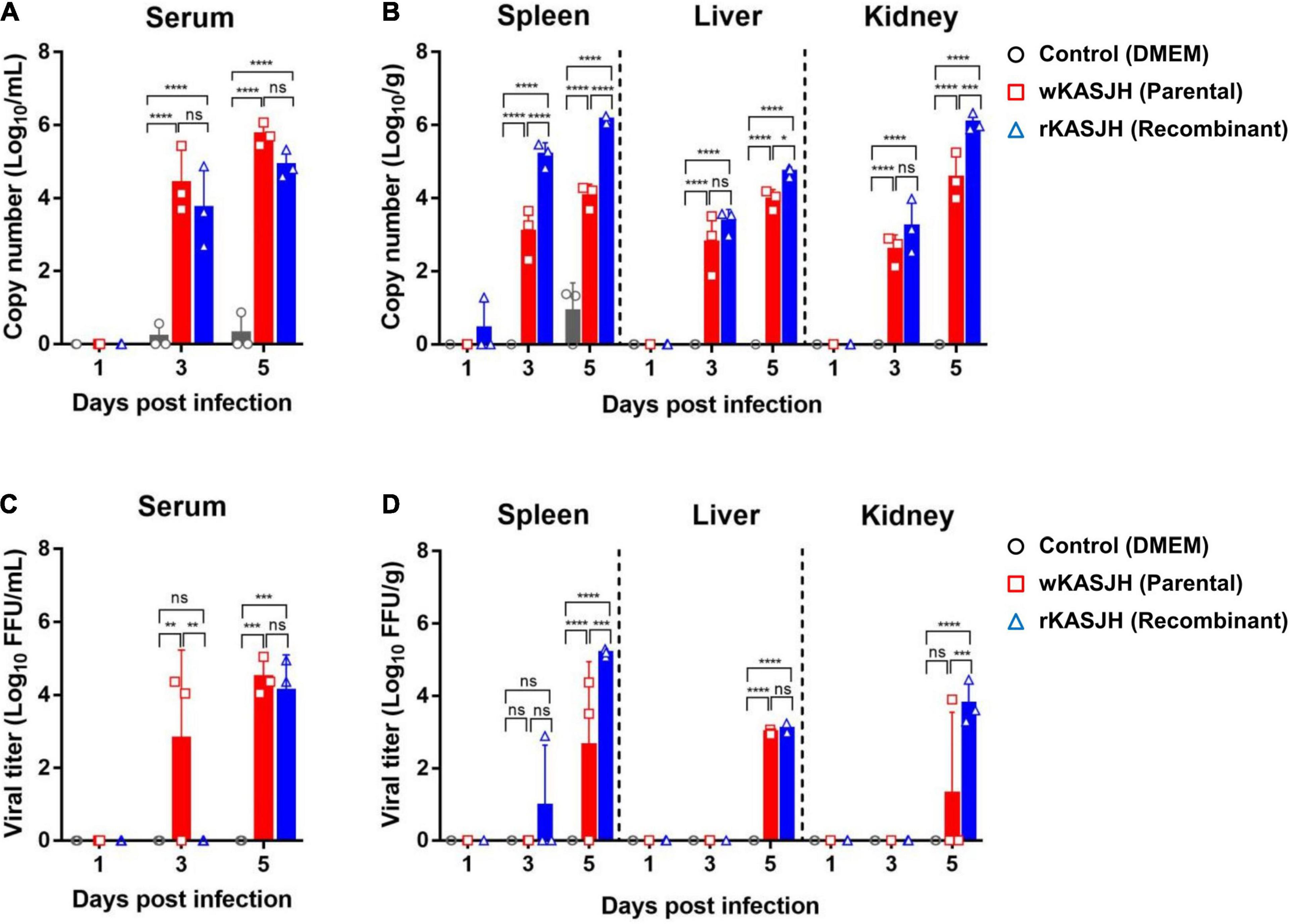
Figure 4. Viral RNA copy numbers and titers in serum (A,C) and tissues (B,D) from C57BL/6 IFNAR–/– mice infected with parental and recombinant SFTSVs. Samples were collected at 1, 3, and 5 dpi. Copy numbers and titers were determined by real-time qRT-PCR and focus-forming assays, respectively. Data are represented as the mean ± s.d. Significance was determined by 2-way ANOVA with Tukey’s multiple comparisons test. *p < 0.05; **p < 0.01; ***p < 0.001; ****p < 0.0001.
Next, to investigate viral pathology, multiple tissues of C57BL/6 IFNAR–/– mice infected with mock, wtKASJH, and rKASJH were examined by staining organ sections with H&E. As shown in Figure 5, we found that tissues from mice infected with both viruses exhibited cell infiltration, necrotizing hepatitis, and lymphoid depletion that was not observed in the mock-infected group. Moreover, more severe histopathological changes were observed at 5 dpi than at 3 dpi. In agreement with the viral load data, the most severe lesions were identified at 5 dpi in the spleens of rKASJH-infected mice.
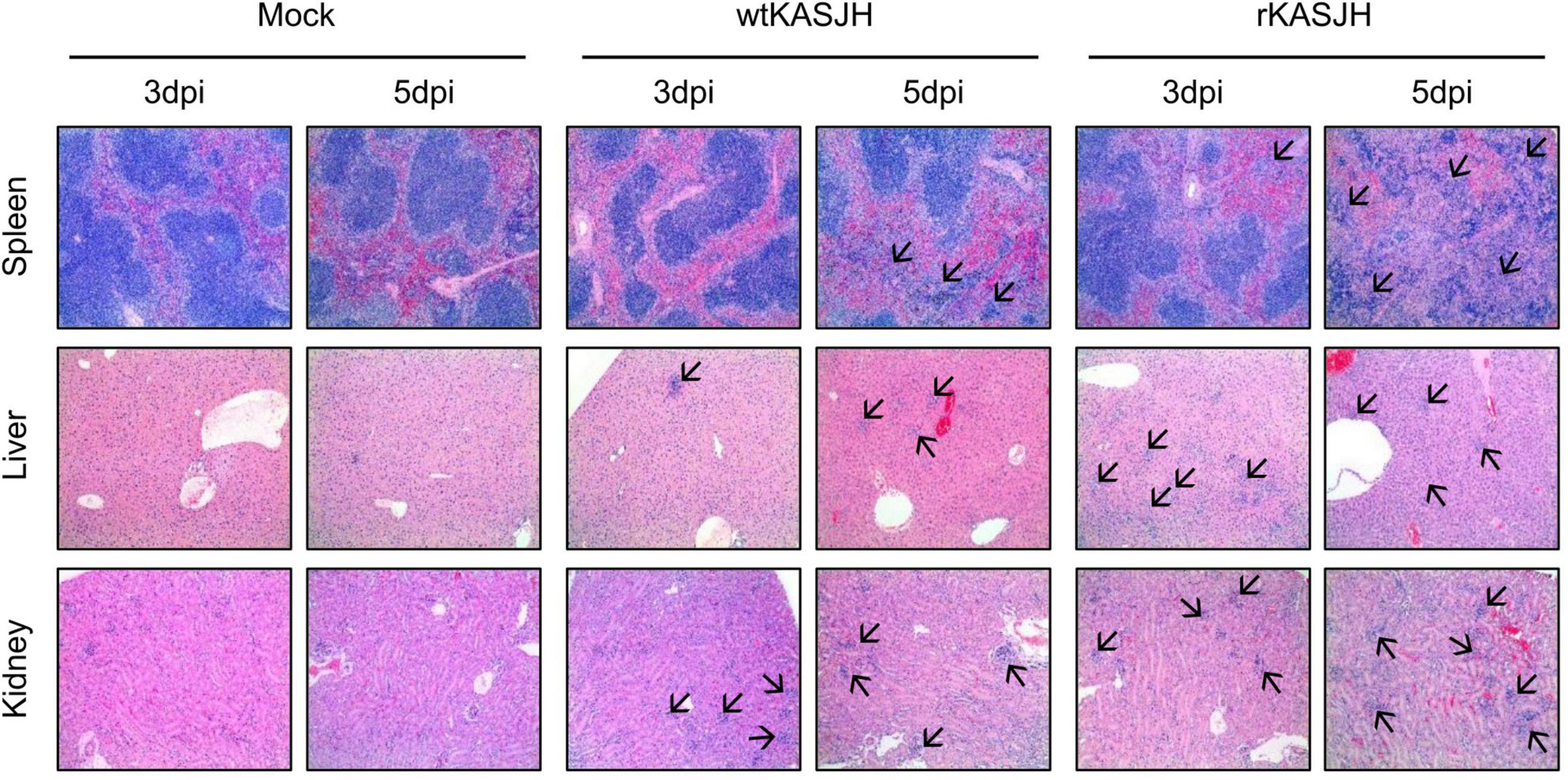
Figure 5. Histopathological analysis of mice infected with parental and rescued SFTSVs. Tissues were harvested at 3 and 5 dpi and processed for H&E staining. Both viruses caused severe lesions that were absent in tissues from mock-infected control mice. Arrows indicate inflamed regions. Magnification, 100×.
Together, our results showed that the rescued virus has similar in vivo characteristics as the parental virus on pathogenicity in C57BL/6 IFNAR–/– mice.
Discussion
Severe fever with thrombocytopenia syndrome is an emerging infectious disease caused by SFTSV infection and has high mortality and increasing prevalence in East Asia. However, there are currently no licensed vaccines or antivirals for controlling this virus. An RG system is a powerful tool for studying the virus life cycle and pathogenesis, with additional utility for development of vaccines and therapeutics against negative-strand RNA viruses (Bridgen and Elliott, 1996; Palese et al., 1996; Bouloy and Flick, 2009; Hoenen et al., 2011). Among them, development of an RG system for SFTSV was first reported for the Chinese HB29 strain in 2015 (Brennan et al., 2015). This system utilized BSR-T7/5 cells stably expressing T7 RNA polymerase to rescue the recombinant SFTSVs.
In this study, we report the development of a T7 RNA polymerase-driven plasmid-based RG system for a clinical Korean isolate, KASJH, based on the plasmid backbones used in the first reported RG system. We have used this system to rescue a recombinant SFTSV. This recombinant virus, rKASJH, was indistinguishable from the parental KASJH strain with respect to patterns of viral protein synthesis and growth kinetics in cultured mammalian cells, as well as virulence in mice.
We first investigated the in vitro phenotypic properties of the parental and rescued SFTSVs by examining focus formation and growth curves and viral protein expression profiles on Vero E6 and Huh-7 cells. Compared with the parental virus, we found that the foci phenotypes produced by the rescued virus on Vero E6 cells showed a similar morphology and the replication efficiency of the rescued virus was represented similar kinetics in Vero E6 and Huh-7 cells (Figures 2A,B). According to the dpi with each virus, the expression level of viral N and NSs proteins were detected by immunoblotting and there were no discernible differences in the patterns of expression profiles (Figure 2C). These results showed that the in vitro biological properties of the rescued virus resembled those of the parental virus.
Experimental assessment of the pathogenicity in animal models is an important aspect of the development of vaccine and antiviral therapies. We therefore evaluated the virulence of the parental and rescued SFTSVs in a previously described mouse model of SFTSV infection (Tani et al., 2016, 2018; Matsuno et al., 2017).
For in vivo experiments, although the tissue distributions and viremia following infection by the parental and rescued SFTSVs showed significant minor differences, there was no significant difference in survival between them. Additionally, histopathological analysis revealed lesions in the tissues of wtKASJH or rKASJH-infected groups (Figure 5). These data indicate that the rescued virus displayed a pathogenesis in C57BL/6 IFNAR–/– mice similar to that observed in case of the parental virus.
Sequencing revealed a single amino-acid substitution (Asn to Asp) at position 2 in the L segment of rescued virus that was not present in the parental virus (Table 1). As we observed no differences in in vitro and in vivo characteristics between the parental and rescued SFTSVs, this amino-acid residue identified in this study did not greatly affect viral replication and virulence.
In summary, we have established a T7 RNA polymerase-driven RG system for production and genetic manipulation of infectious SFTSV isolated from South Korea. The system reported here will be a valuable tool for studying the molecular biology of SFTSV, viral determinants of SFTSV pathogenesis, and the development of vaccines and antivirals against SFTSV infection. Further study is warranted using site-directed mutagenesis to identify pathogenic determinants of SFTSV and their underlying mechanisms.
Data Availability Statement
The original contributions presented in the study are included in the article/supplementary material, further inquiries can be directed to the corresponding author/s.
Ethics Statement
The animal study was reviewed and approved by Institutional Animal Care and Use Committee (IACUC) of the Korea Centers for Disease Control and Prevention (approval number, KCDC-073-19-2A).
Author Contributions
S-MY and Y-EK conceived the study, designed the experiments, analyzed and interpreted the data, and wrote the manuscript. S-MY, T-YL, and Y-EK performed the experiments and contributed to materials. H-YL, JR, and J-YL reviewed the manuscript. Y-EK supervised the study. All authors approved the final version of the manuscript.
Funding
This research was funded by Korea National Institute of Health Intramural Grants 2017-NI53002-02 and 2020-NI-030-00.
Conflict of Interest
The authors declare that the research was conducted in the absence of any commercial or financial relationships that could be construed as a potential conflict of interest.
Publisher’s Note
All claims expressed in this article are solely those of the authors and do not necessarily represent those of their affiliated organizations, or those of the publisher, the editors and the reviewers. Any product that may be evaluated in this article, or claim that may be made by its manufacturer, is not guaranteed or endorsed by the publisher.
Acknowledgments
We are grateful to Benjamin Brennan (MRC-University of Glasgow Centre for Virus Research, United Kingdom) for providing the pTVT7-HB29L and pTM1-HB29L plasmids.
References
Bao, C. J., Guo, X. L., Qi, X., Hu, J. L., Zhou, M. H., Varma, J. K., et al. (2011). A family cluster of infections by a newly recognized bunyavirus in eastern China, 2007: further evidence of person-to-person transmission. Clin. Infect. Dis. 53, 1208–1214. doi: 10.1093/cid/cir732
Billecocq, A., Gauliard, N., Le May, N., Elliott, R. M., Flick, R., and Bouloy, M. (2008). RNA polymerase I-mediated expression of viral RNA for the rescue of infectious virulent and avirulent Rift Valley fever viruses. Virology 378, 377–384. doi: 10.1016/j.virol.2008.05.033
Bouloy, M., and Flick, R. (2009). Reverse genetics technology for Rift Valley fever virus: current and future applications for the development of therapeutics and vaccines. Antiviral Res. 84, 101–118. doi: 10.1016/j.antiviral.2009.08.002
Brennan, B., Li, P., Zhang, S., Li, A., Liang, M., Li, D., et al. (2015). Reverse genetics system for severe fever with thrombocytopenia syndrome virus. J. Virol. 89, 3026–3037. doi: 10.1128/JVI.03432-14
Bridgen, A., and Elliott, R. M. (1996). Rescue of a segmented negative-strand RNA virus entirely from cloned complementary DNAs. Proc. Natl. Acad. Sci. U.S.A. 93, 15400–15404. doi: 10.1073/pnas.93.26.15400
Chen, H., Hu, K., Zou, J., and Xiao, J. (2013). A cluster of cases of human-to-human transmission caused by severe fever with thrombocytopenia syndrome bunyavirus. Int. J. Infect. Dis. 17, e206–e208. doi: 10.1016/j.ijid.2012.11.006
Ding, F., Guan, X. H., Kang, K., Ding, S. J., Huang, L. Y., Xing, X. S., et al. (2014). Risk factors for bunyavirus-associated severe Fever with thrombocytopenia syndrome, China. PLoS Negl. Trop. Dis. 8:e3267. doi: 10.1371/journal.pntd.0003267
Gai, Z., Liang, M., Zhang, Y., Zhang, S., Jin, C., Wang, S. W., et al. (2012). Person-to-person transmission of severe fever with thrombocytopenia syndrome bunyavirus through blood contact. Clin. Infect. Dis. 54, 249–252. doi: 10.1093/cid/cir776
Gerrard, S. R., Bird, B. H., Albarino, C. G., and Nichol, S. T. (2007). The NSm proteins of Rift Valley fever virus are dispensable for maturation, replication and infection. Virology 359, 459–465.
Habjan, M., Penski, N., Spiegel, M., and Weber, F. (2008). T7 RNA polymerase-dependent and -independent systems for cDNA-based rescue of Rift Valley fever virus. J. Gen. Virol. 89(Pt 9) 2157–2166. doi: 10.1099/vir.0.2008/002097-0
Hoenen, T., Groseth, A., de Kok-Mercado, F., Kuhn, J. H., and Wahl-Jensen, V. (2011). Minigenomes, transcription and replication competent virus-like particles and beyond: reverse genetics systems for filoviruses and other negative stranded hemorrhagic fever viruses. Antiviral Res. 91, 195–208. doi: 10.1016/j.antiviral.2011.06.003
Ikegami, T., Won, S., Peters, C. J., and Makino, S. (2006). Rescue of infectious rift valley fever virus entirely from cDNA, analysis of virus lacking the NSs gene, and expression of a foreign gene. J. Virol. 80, 2933–2940. doi: 10.1128/JVI.80.6.2933-2940.2006
Ito, N., Takayama-Ito, M., Yamada, K., Hosokawa, J., Sugiyama, M., and Minamoto, N. (2003). Improved recovery of rabies virus from cloned cDNA using a vaccinia virus-free reverse genetics system. Microbiol. Immunol. 47, 613–617. doi: 10.1111/j.1348-0421.2003.tb03424.x
Kim, K. H., Yi, J., Kim, G., Choi, S. J., Jun, K. I., Kim, N. H., et al. (2013). Severe fever with thrombocytopenia syndrome, South Korea, 2012. Emerg. Infect. Dis. 19, 1892–1894. doi: 10.3201/eid1911.130792
Kim, W. Y., Choi, W., Park, S. W., Wang, E. B., Lee, W. J., Jee, Y., et al. (2015). Nosocomial transmission of severe fever with thrombocytopenia syndrome in Korea. Clin. Infect. Dis. 60, 1681–1683. doi: 10.1093/cid/civ128
Kuhn, J. H., Adkins, S., Alioto, D., Alkhovsky, S. V., Amarasinghe, G. K., Anthony, S. J., et al. (2020). 2020 taxonomic update for phylum Negarnaviricota (Riboviria:Orthornavirae), including the large orders Bunyavirales and Mononegavirales. Arch. Virol. 165, 3023–3072. doi: 10.1007/s00705-020-04731-2
Liu, Q., He, B., Huang, S. Y., Wei, F., and Zhu, X. Q. (2014). Severe fever with thrombocytopenia syndrome, an emerging tick-borne zoonosis. Lancet Infect. Dis. 14, 763–772. doi: 10.1016/S1473-3099(14)70718-2
Liu, Y., Li, Q., Hu, W., Wu, J., Wang, Y., Mei, L., et al. (2012). Person-to-person transmission of severe fever with thrombocytopenia syndrome virus. Vector Borne Zoonotic Dis. 12, 156–160. doi: 10.1089/vbz.2011.0758
Matsuno, K., Orba, Y., Maede-White, K., Scott, D., Feldmann, F., Liang, M., et al. (2017). Animal models of emerging tick-borne phleboviruses: determining target cells in a lethal model of SFTSV infection. Front. Microbiol. 8:104. doi: 10.3389/fmicb.2017.00104
Palese, P., Zheng, H., Engelhardt, O. G., Pleschka, S., and Garcia-Sastre, A. (1996). Negative-strand RNA viruses: genetic engineering and applications. Proc. Natl. Acad. Sci. U.S.A. 93, 11354–11358. doi: 10.1073/pnas.93.21.11354
Takahashi, T., Maeda, K., Suzuki, T., Ishido, A., Shigeoka, T., Tominaga, T., et al. (2014). The first identification and retrospective study of severe fever with Thrombocytopenia Syndrome in Japan. J. Infect. Dis. 209, 816–827. doi: 10.1093/infdis/jit603
Tang, X., Wu, W., Wang, H., Du, Y., Liu, L., Kang, K., et al. (2013). Human-to-human transmission of severe fever with thrombocytopenia syndrome bunyavirus through contact with infectious blood. J. Infect. Dis. 207, 736–739. doi: 10.1093/infdis/jis748
Tani, H., Fukuma, A., Fukushi, S., Taniguchi, S., Yoshikawa, T., Iwata-Yoshikawa, N., et al. (2016). Efficacy of T-705 (Favipiravir) in the treatment of infections with lethal severe fever with thrombocytopenia syndrome virus. mSphere 1, e00061–15. doi: 10.1128/mSphere.0006115
Tani, H., Komeno, T., Fukuma, A., Fukushi, S., Taniguchi, S., Shimojima, M., et al. (2018). Therapeutic effects of favipiravir against severe fever with thrombocytopenia syndrome virus infection in a lethal mouse model: dose-efficacy studies upon oral administration. PLoS One 13:e0206416. doi: 10.1371/journal.pone.0206416
Xu, B., Liu, L., Huang, X., Ma, H., Zhang, Y., Du, Y., et al. (2011). Metagenomic analysis of fever, thrombocytopenia and leukopenia syndrome (FTLS) in Henan Province, China: discovery of a new bunyavirus. PLoS Pathog. 7:e1002369. doi: 10.1371/journal.ppat.1002369
Yu, X. J., Liang, M. F., Zhang, S. Y., Liu, Y., Li, J. D., Sun, Y. L., et al. (2011). Fever with thrombocytopenia associated with a novel bunyavirus in China. N. Engl. J. Med. 364, 1523–1532. doi: 10.1056/NEJMoa1010095
Yun, M. R., Park, S. W., Kwon, T., Lee, S., Yoo, W. G., Choi, W., et al. (2015). Full-genome sequences of severe fever with thrombocytopenia syndrome virus isolated from South Korea in 2014. Genome Announc. 3, e00 181–15.
Yun, S. M., Park, S. J., Park, S. W., Choi, W., Jeong, H. W., Choi, Y. K., et al. (2017). Molecular genomic characterization of tick- and human-derived severe fever with thrombocytopenia syndrome virus isolates from South Korea. PLoS Negl. Trop. Dis. 11:e0005893. doi: 10.1371/journal.pntd.0005893
Keywords: severe fever with thrombocytopenia syndrome virus, reverse genetics system, SFTSV, animal model, recombinant virus
Citation: Yun S-M, Lee T-Y, Lim H-Y, Ryou J, Lee J-Y and Kim Y-E (2021) Development and Characterization of a Reverse Genetics System for a Human-Derived Severe Fever With Thrombocytopenia Syndrome Virus Isolate From South Korea. Front. Microbiol. 12:772802. doi: 10.3389/fmicb.2021.772802
Received: 08 September 2021; Accepted: 22 October 2021;
Published: 11 November 2021.
Edited by:
Denis Kainov, Norwegian University of Science and Technology, NorwayReviewed by:
Safder Ganaie, Washington University in St. Louis, United StatesYouichi Suzuki, Osaka Medical College, Japan
Copyright © 2021 Yun, Lee, Lim, Ryou, Lee and Kim. This is an open-access article distributed under the terms of the Creative Commons Attribution License (CC BY). The use, distribution or reproduction in other forums is permitted, provided the original author(s) and the copyright owner(s) are credited and that the original publication in this journal is cited, in accordance with accepted academic practice. No use, distribution or reproduction is permitted which does not comply with these terms.
*Correspondence: Young-Eui Kim, d2xzZG1sN0Brb3JlYS5rcg==