- 1Microbiology Department, Faculty of Veterinary Medicine, Zagazig University, Zagazig, Egypt
- 2Zoonoses Department, Faculty of Veterinary Medicine, Zagazig University, Zagazig, Egypt
- 3Animal Medicine Department (Infectious Diseases), Faculty of Veterinary Medicine, Zagazig University, Zagazig, Egypt
- 4Microbiology and Immunology Department, Faculty of Pharmacy, Suez Canal University, Ismailia, Egypt
- 5Bacteriology, Immunology and Mycology Department, Faculty of Veterinary Medicine, Benha University, Benha, Egypt
- 6Food Control Department, Faculty of Veterinary Medicine, Zagazig University, Zagazig, Egypt
- 7Veterinary Public Health Department, Faculty of Veterinary Medicine, Zagazig University, Zagazig, Egypt
- 8Veterinary Hospital, Faculty of Veterinary Medicine, Zagazig University, Zagazig, Egypt
- 9Animal Health Research Institute, Agriculture Research Center, Cairo, Egypt
- 10Institute of Veterinary Sciences, Department of Veterinary Medicine, College of Animal Sciences, Zhejiang University, Hangzhou, China
- 11Hygiene and Zoonoses Department, Faculty of Veterinary Medicine, Mansoura University, Mansoura, Egypt
- 12Bacterial Epidemiology and Antimicrobial Resistance Research Unit, US National Poultry Research Center, US Department of Agriculture, Agricultural Research Service (USDA-ARS), Athens, GA, United States
Antimicrobial resistance is a major concern in the dairy industry. This study investigated the prevalence, antimicrobial resistance phenotypes, and genome sequencing of Gram-negative bacteria isolated from clinical (n = 350) and subclinical (n = 95) bovine mastitis, and raw unpasteurized milk (n = 125). Klebsiella pneumoniae, Aeromonas hydrophila, Enterobacter cloacae (100% each), Escherichia coli (87.78%), and Proteus mirabilis (69.7%) were the most prevalent multidrug-resistant (MDR) species. Extensive drug-resistance (XDR) phenotype was found in P. mirabilis (30.30%) and E. coli (3.33%) isolates. Ten isolates (four E. coli, three Klebsiella species and three P. mirabilis) that displayed the highest multiple antibiotic resistance (MAR) indices (0.54–0.83), were exposed to whole-genome sequencing (WGS). Two multilocus sequence types (MLST): ST2165 and ST7624 were identified among the sequenced E. coli isolates. Three E. coli isolates (two from clinical mastitis and one from raw milk) belonging to ST2165 showed similar profile of plasmid replicon types: IncFIA, IncFIB, IncFII, and IncQ1 with an exception to an isolate that contained IncR, whereas E. coli ST7624 showed a different plasmid profile including IncHI2, IncHI2A, IncI1α, and IncFII replicon types. ResFinder findings revealed the presence of plasmid-mediated colistin mcr-10 and fosfomycin fosA5 resistance genes in a K. pneumoniae (K1) isolate from bovine milk. Sequence analysis of the reconstructed mcr-10 plasmid from WGS of K1 isolate, showed that mcr-10 gene was bracketed by xerC and insertion sequence IS26 on an IncFIB plasmid. Phylogenetic analysis revealed that K1 isolate existed in a clade including mcr-10-harboring isolates from human and environment with different STs and countries [United Kingdom (ST788), Australia (ST323), Malawi (ST2144), Myanmar (ST705), and Laos (ST2355)]. This study reports the first emergence of K. pneumoniae co-harboring mcr-10 and fosA5 genes from bovine milk in the Middle East, which constitutes a public health threat and heralds the penetration of the last-resort antibiotics. Hence, prudent use of antibiotics in both humans and animals and antimicrobial surveillance plans are urgently required.
Introduction
Mastitis is one of the most prevalent diseases in dairy cows, producing well-recognized detrimental effects on animal health and profitability of dairy farms (Ruegg, 2017; Abd El-Aziz et al., 2021a). Treatment of mastitis is often applied without prior knowledge of cause-related agents and mostly with broad-spectrum antimicrobials, which are known to enhance resistance evolution to a greater extent. This increases the selective pressure on potentially present pathogens and is considered a possible human health hazard (Neyra et al., 2014; Abd El-Aziz et al., 2021b).
Raw or processed milk is a good environment that promotes the existence of many pathogens, which could be either directly obtained via dairy cows, or indirectly from the environment of the farm (Oliver et al., 2005). Escherichia coli (E. coli) O157:H7 outbreaks associated with the consumption of raw milk have been described (Disassa et al., 2017; Ababu et al., 2020). The indiscriminate use of antimicrobials has led to the development of multidrug-resistant (MDR) Gram-negative bacteria in raw milk, in particular E. coli O157:H7, Klebsiella pneumoniae (K. pneumoniae), Aeromonas hydrophilia (A. hydrophilia), and Proteus mirabilis (P. mirabilis) (Zelalem and Bernard, 2006; Oliver et al., 2009; Garedew et al., 2012).
Resistance to most antimicrobial classes and the lack of new antimicrobial agents against Gram-negative bacteria potentiates the re-use of old antibiotics, particularly polymyxins such as colistin (Biswas et al., 2012). Colistin is currently known as the last-resort antimicrobial agent for the treatment of infections caused by MDR Gram-negative bacteria (Poirel et al., 2017). It binds to the negatively charged lipopolysaccharide (LPS) of the outer membrane of Gram-negative bacteria, leading to disruption of the membrane, induces the cytoplasmic material to fade away, and eventually cell death (Yahav et al., 2012).
Colistin has been greatly utilized in the agricultural system and veterinary medicine for decades, as a growth promoter and for the treatment of enterobacterial infections (Catry et al., 2015). Extensive colistin usages in livestock have facilitated the rapid spread of the mcr resistance genes (Shen et al., 2016). The mcr-1 plasmid-mediated colistin resistance gene has been first reported in Enterobacterales isolated from animals, food, and humans in China (Liu et al., 2016). Numerous studies have documented plasmid-borne mcr variants (mcr-1 to mcr-9) from this time onward (Xavier et al., 2016; Yin et al., 2017; Borowiak et al., 2019; Carroll et al., 2019; Gelbíčová et al., 2019; Wang et al., 2019). However, studies focused on mcr-resistant isolates from mastitis and raw unpasteurized milk have received little attention. Here, the genome sequences and genetic relatedness between MDR and extensive drug-resistant [XDR; resistance to at least one antibiotic in all tested antimicrobial groups except one or two as demonstrated in the worksheet of Magiorakos et al. (2012)]. Gram-negative bacteria isolated from bovine milk samples have been studied for the first time. This study aimed to: (1) investigate the occurrence and resistance phenotypes of the Gram-negative bacteria in mastitic cow’s milk as well as raw unpasteurized milk that consumed by the public; (2) identify the multilocus sequence types (MLST), resistance genes, and plasmid replicon types of MDR and XDR Gram-negative isolates; (3) determine the genetic environment of mcr-10 gene and epidemiological relatedness between the study isolate and the publicly available genomes of K. pneumoniae isolates harboring mcr genes.
Materials and Methods
Milk Samples
This study was carried out during the period between October 2018 and August 2020. A total of 445 quarter milk samples were collected from lactating cows in smallholder farms with clinical (n = 350) and subclinical (n = 95) mastitis. Moreover, 125 raw unpasteurized milk samples were collected from retail milk outlets located in Zagazig City, Sharkia Governorate, Egypt. Clinical mastitis was diagnosed based on the cardinal signs of inflammation and the changes in the milk of the infected mammary quarters. California mastitis test was performed on apparently normal milk samples for diagnosis of subclinical mastitis. The milk samples were aseptically collected from mastitic dairy cows before the antibiotic therapy following the standard procedures (National Mastitis Council, 1999). Collected milk samples were labeled, kept in an icebox, and transferred immediately to the Microbiology laboratory for further bacteriological analysis.
Isolation and Identification of Gram-Negative Bacteria
Ten milliliters of each milk sample were enriched in 90 mL of Buffered Peptone Water (BPW, Oxoid, United Kingdom) and incubated overnight at 37°C. A loopful from the enriched broth was cultivated on the agar media selective for each expected bacterium; coliforms (MacConkey Agar, CM0007; Oxoid, United Kingdom), E. coli (Eosin Methylene Blue Agar, CM0069; Oxoid, United Kingdom), K. pneumoniae (HiCrome Klebsiella Selective Agar, M1573; Himedia, India), and Proteus species [MacConkey Agar (without salt), CM0507; Oxoid, United Kingdom]. A suspected colony of each microorganism was purified on a slope agar, identified by Gram staining, and subjected to standard biochemical tests recommended for identification of each bacterial species according to Faddin (2000). Serotyping of all biochemically confirmed E. coli isolates was done by slide agglutination technique using diagnostic polyvalent and related monovalent E. coli O and H antisera (Denka Seiken Co., Japan). Polymerase chain reaction (PCR) was applied for confirmation of Gram-negative isolates using species-specific primer sets listed in Supplementary Table 1 (Cascón et al., 1996; Heijnen and Medema, 2006; Liu et al., 2008; Anbazhagan et al., 2010; Zhang et al., 2013). PCR amplifications were performed using a programmable 2720 thermal cycler (Applied Biosystem, Waltham, Massachusetts, United States) in a total reaction volume of 25 μL containing 12.5 μL of EmeraldAmp Max PCR Master Mix (Takara, Japan), 1 μL of each primer (20 pmoL) (Sigma-Aldrich, Co., St. Louis, United States), 5 μL of template DNA and 5.5 μL of nuclease-free water. The amplified products were visualized after 30 min of electrophoresis on a 2% agarose gel containing ethidium bromide.
Antimicrobial Susceptibility Testing of Gram-Negative Bacteria
The antibiogram of all recovered Gram-negative bacteria was determined by the disc diffusion method (Bauer et al., 1966). The antimicrobial discs (Oxoid, Cambridge, United Kingdom) included ampicillin (AM; 10 μg), amoxicillin-clavulanic acid (AMC; 30 μg), piperacillin-tazobactam (TPZ; 40 μg), cefazolin (CFZ; 30 μg), cephalothin (CEF; 30 μg), cefoxitin (FOX; 30 μg), ceftriaxone (CRO; 30 μg), ceftazidime (CAZ; 30 μg), cefotaxime (CTX; 30 μg), cefepime (FEP; 30 μg), imipenem (IPM; 10 μg), gentamicin (CN; 10 μg), tobramycin (TOB; 10 μg), amikacin (AK; 30 μg), nalidixic acid (NA; 30 μg), ciprofloxacin (CIP; 5 μg), levofloxacin (LVX; 5 μg), sulfamethoxazole-trimethoprim (SXT; 25 μg), tigecycline (TGC; 15 μg), aztreonam (ATM; 30 μg), chloramphenicol (CHL; 30 μg), fosfomycin (FOF; 50 μg), colistin (CST; 25 μg), and tetracycline (TET; 30 μg). Selected antimicrobial agents were synchronized with the veterinary guidelines (Plumb, 2015). The justification for the antimicrobials chosen is to monitor antimicrobial resistance and for public health concerns about certain agents such as cephalosporins, carbapenems, aminoglycosides, fluoroquinolones, tigecycline, and fosfomycin. E. coli ATCC 25922, K. pneumoniae ATCC 700603, P. mirabilis ATCC 29906, and A. hydrophila ATCC 7966 were included as quality control strains. The minimum inhibitory concentration (MIC) for colistin against Gram-negative isolates was performed using broth microdilution method as documented previously (Rankin, 2005). The results for antimicrobial susceptibility testing were interpreted following the guidelines of the Clinical and Laboratory Standards Institute (CLSI, 2017) and the European Committee on Antimicrobial Susceptibility Testing guidelines (Eucast: The European Committee on Antimicrobial Susceptibility Testing, 2018). Colistin resistance breakpoint was considered at MIC > 2 mg/L. The fully colistin-susceptible E. coli ATCC 25922 and the mcr-1-positive E. coli NCTC 13846 with a colistin MIC of 4 mg/L were used as quality controls. The multiple antibiotic resistance (MAR) indices were estimated as documented by Tambekar et al. (2006), whereas the MDR and XDR isolates were determined according to Magiorakos et al. (2012).
Whole-Genome Sequencing and Bioinformatics Analyses
Whole-genome sequencing (WGS) was performed for 10 representative MDR and XDR isolates that exhibited unique antibiogram and the highest MAR indices. DNA libraries were constructed using Nextra DNA Flex kit (Illumina Inc., San Diego, CA, United States), and libraries were then sequenced at a 250 bp paired-end-read format using Illumina NovaSeq 6000 kit (Illumina Inc., San Diego, CA, United States), according to the manufacturer’s instructions.
The obtained raw fastq reads for each isolate were deposited into sequence read archive (SRA) database under the bioproject PRJNA713498. Quality check of the fastq reads was performed using fastQC,1 and low-quality reads were exposed to trimmomatic2 (Bolger et al., 2014). The reads were then de novo assembled using SPAdes3 (Bankevich et al., 2012) and the assembled fasta files were uploaded into the Center for Genomic Epidemiology (CGE)4 to identify the MLST, resistance genes, and plasmid replicon types via MLST 2.0, ResFinder 4.1 and PlasmidFinder 2.1 tools, respectively. For genotyping and source tracking, MLST for mcr-10-producing K. pneumoniae isolate (K1) was determined using bacterial whole-genome sequence typing database (BacWGSTdb)5 (Ruan and Feng, 2016). The genetic environment of mcr-10 gene was determined using a nucleotide Basic Local Alignment Search Tool (BLASTn) search6 in combination with ISFinder.7 The contig carrying the mcr-10 was annotated using prokka, and the gbk file was imported into Easyfig software8 for genetic comparison (Sullivan et al., 2011). The mcr-10-carrying plasmid of the study isolate (K1) was reconstructed from WGS using Plasmid SPAdes (Antipov et al., 2016) and PLACNETw (Vielva et al., 2017). BLASTn search of the obtained plasmid sequences was performed to determine the best plasmid hits. Reconstructed plasmid sequences of K1 isolate were aligned to the retrieved National Center for Biotechnology Information (NCBI) plasmid sequences using BLAST Ring Image Generator (BRIG) tool9 for genetic comparison (Alikhan et al., 2011).
Phylogenetic analysis based on single nucleotide polymorphisms (SNPs) was performed for the K. pneumoniae isolate (K1) carrying mcr-10 gene. Our K1 isolate was compared to the publicly available genomes of K. pneumoniae in the NCBI database, updated March 21st, 2021, that carried any variant of mcr gene (n = 241) or those carrying mcr-10 variant (n = 20). Isolates were mapped to the reference K. pneumoniae complete genome ATCC43816 (CP064352.1) for all the enrolled mcr-carrying isolates and 30684/NJST258_2 (CP006918.1) for mcr-10-producing K. pneumoniae isolates. Metadata for the selected K. pneumoniae sequences from NCBI are listed in Supplementary Tables 2A,B. SNPs variant calling were determined using Snippy v4.4.4,10 and the output files were combined into a core SNPs alignment using Snippy core. Maximum likelihood phylogenetic trees were then generated from SNPs alignment using RAxML, and the trees were visualized with iTOL (Letunic and Bork, 2016).
Results
Prevalence of Gram-Negative Bacteria Isolated From Cattle Mastitis and Raw Milk
As depicted in Table 1, a total of 184 isolates were recovered from cases of clinical (120/350; 34.29%) and subclinical (19/95; 20%) cattle mastitis, and raw milk samples (45/125; 36%). PCR of the amplified products of bacterial species could identify the isolates as E. coli (48.91%), K. pneumoniae (13.59%), P. mirabilis (17.93%), A. hydrophila (9.24%), E. cloacae (8.15%), and C. freundii (2.17%). According to the serotyping results, a variety of E. coli serotypes (n = 10) was identified. The predominant serotypes were O111:H4 from clinical (8%) and subclinical (2.11%) mastitis and O157:H7 from raw milk (6.4%).
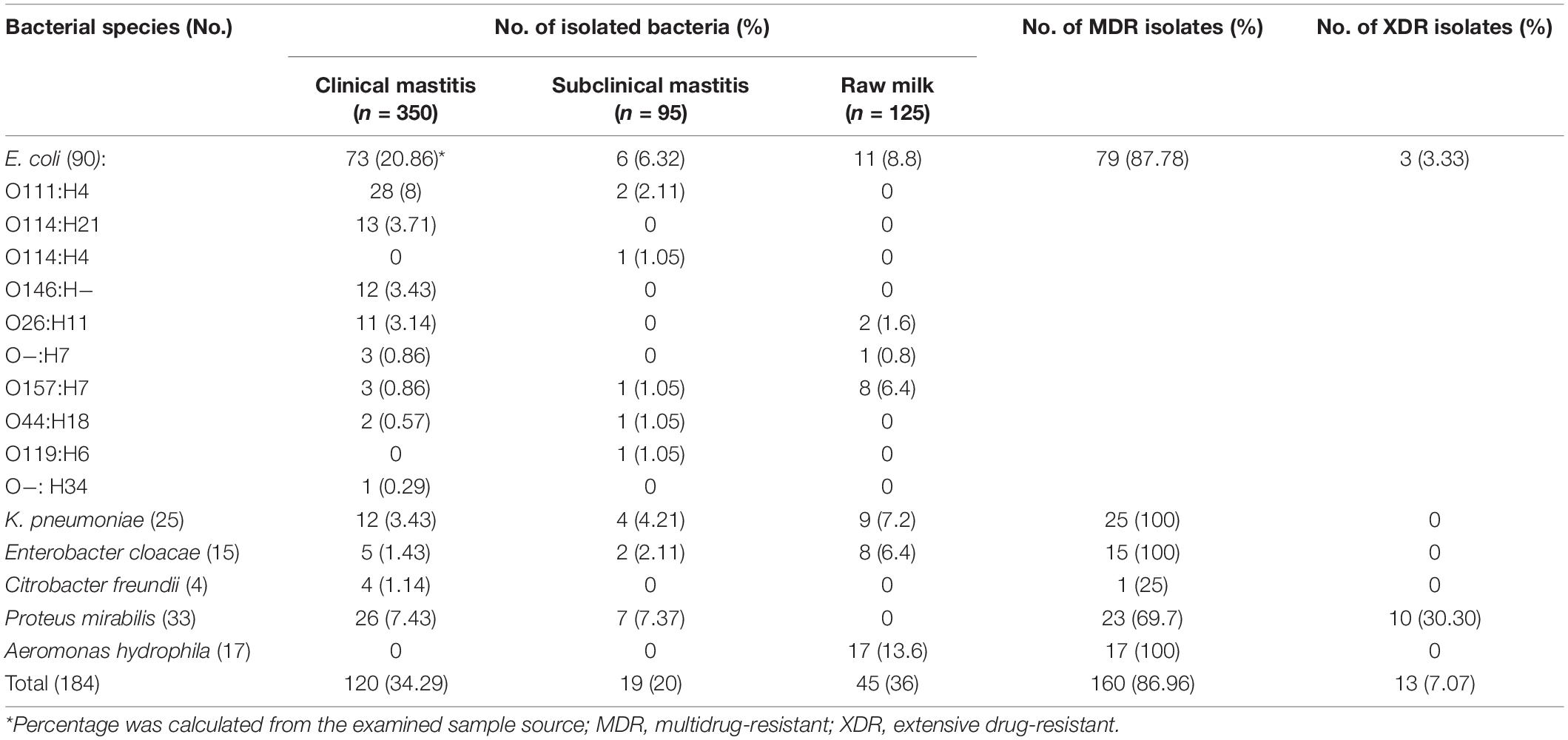
Table 1. Prevalence of Gram-negative bacteria isolated from clinical, subclinical bovine mastitis, and raw milk in Egypt.
Frequency of Multidrug-Resistant and Extensive Drug-Resistant Gram-Negative Bacteria From Milk Samples
The MDR and XDR phenotypes were determined in 86.96 and 7.07% of the isolates, respectively (Table 1). K. pneumoniae, A. hydrophila, E. cloacae (100%, each), E. coli (87.78%), and P. mirabilis (69.7%) were the most prevalent MDR species. XDR phenotype was found in P. mirabilis (30.30%) and E. coli (3.33%).
The most observed resistance phenotypes for E. coli isolates were against cephalothin, cefazolin, ceftazidime, ampicillin, amoxicillin-clavulanic acid (100% each), cefoxitin (98. 89%), cefotaxime (94.44%), piperacillin-tazobactam (75.56%), fosfomycin (63.33%), and colistin (26.67%). K. pneumoniae isolates were resistant to cephalothin, cefazolin, cefoxitin, ampicillin, amoxicillin-clavulanic acid, fosfomycin (100% each), ceftazidime, piperacillin-tazobactam (96% each), tigecycline (88%), cefotaxime and ceftriaxone (76% each), sulfamethoxazole-trimethoprim and colistin (56% each).
All A. hydrophila isolates were resistant to cephalothin, cefoxitin, ceftazidime, cefotaxime, ceftriaxone, and tetracycline. Meanwhile, 82.35, 76.47, and 70.59% of the isolates were resistant to colistin, nalidixic acid, and sulfamethoxazole-trimethoprim, respectively.
Proteus mirabilis isolates exhibited a resistance rate of 100% to cephalothin, cefazolin, cefoxitin, sulfamethoxazole-trimethoprim, amoxicillin-clavulanic acid, and fosfomycin followed by ampicillin, tetracycline, and chloramphenicol (90.91% each), nalidixic acid (78.79%), ciprofloxacin, and levofloxacin (60.61% each), whereas a lower resistance rate was reported for colistin (4/33; 12.12%). All E. cloacae isolates were resistant to cephalothin, cefazolin, cefoxitin, ceftazidime, cefotaxime, cefepime, sulfamethoxazole-trimethoprim, ampicillin, amoxicillin-clavulanic acid, and tetracycline. While, C. freundii isolates were resistant to cephalothin, cefazolin, cefoxitin, ceftazidime, cefotaxime, ampicillin, and amoxicillin-clavulanic acid (100%). The latter two bacterial species showed no colistin resistance.
Whole-Genome Sequencing and Characteristics of Multidrug-Resistant and Extensive Drug-Resistant Gram-Negative Bacteria
We sequenced the genome of 10 representative Gram-negative bacterial isolates with the highest MAR indices (0.54–0.83) and determined the resistance genes, plasmid replicon types, and MLST types (Table 2). In silico analysis of E. coli sequences using MLST revealed that four isolates were assigned to two sequence types: ST2165 (n = 3) and ST7624 (n = 1). The three isolates belonging to ST2165 showed a markedly similar profile of plasmid replicon types including IncFIA, IncFIB, IncFII, and IncQ1 with an exception to isolate E2 that also contained IncR replicon type. The other E. coli isolate (E4) that was assigned to ST7624 showed the existence of IncHI2, IncHI2A, and IncI1α replicon types beside IncFII. Moreover, isolate E4 harbored the blaCTX–M–14b gene that has not been identified in any of the other three E. coli ST2165 isolates. MLST analysis of the three-klebsiella isolates (two K. pneumoniae and one K. aerogenes as finally identified by WGS) assigned K. aerogenes (K2) and K. pneumoniae (K3) into ST11 and ST48, respectively, and K. pneumoniae (K1) into a novel ST, closely matches ST1224. Both K. pneumoniae isolates showed multiple replicon types, whereas K. aerogenes only carried IncR replicon type. The K. pneumoniae isolate (K1) that carried IncFIA(HI1), IncFIB(K), IncFII(K), IncHI1B(pNDM-MAR), IncQ1, Col(pHAD28), Col440I, and IncR replicon types, was found harboring multiple resistance genes to ß-lactamases, aminoglycosides, quinolones, tetracyclines, macrolides, folate pathway inhibitors as well as the last therapeutic choices such as fosfomycin (fosA5) and colistin (mcr-10). PlasmidFinder results displayed the existence of both IncQ1 and pSL483 replicon types in the three examined P. mirabilis isolates.
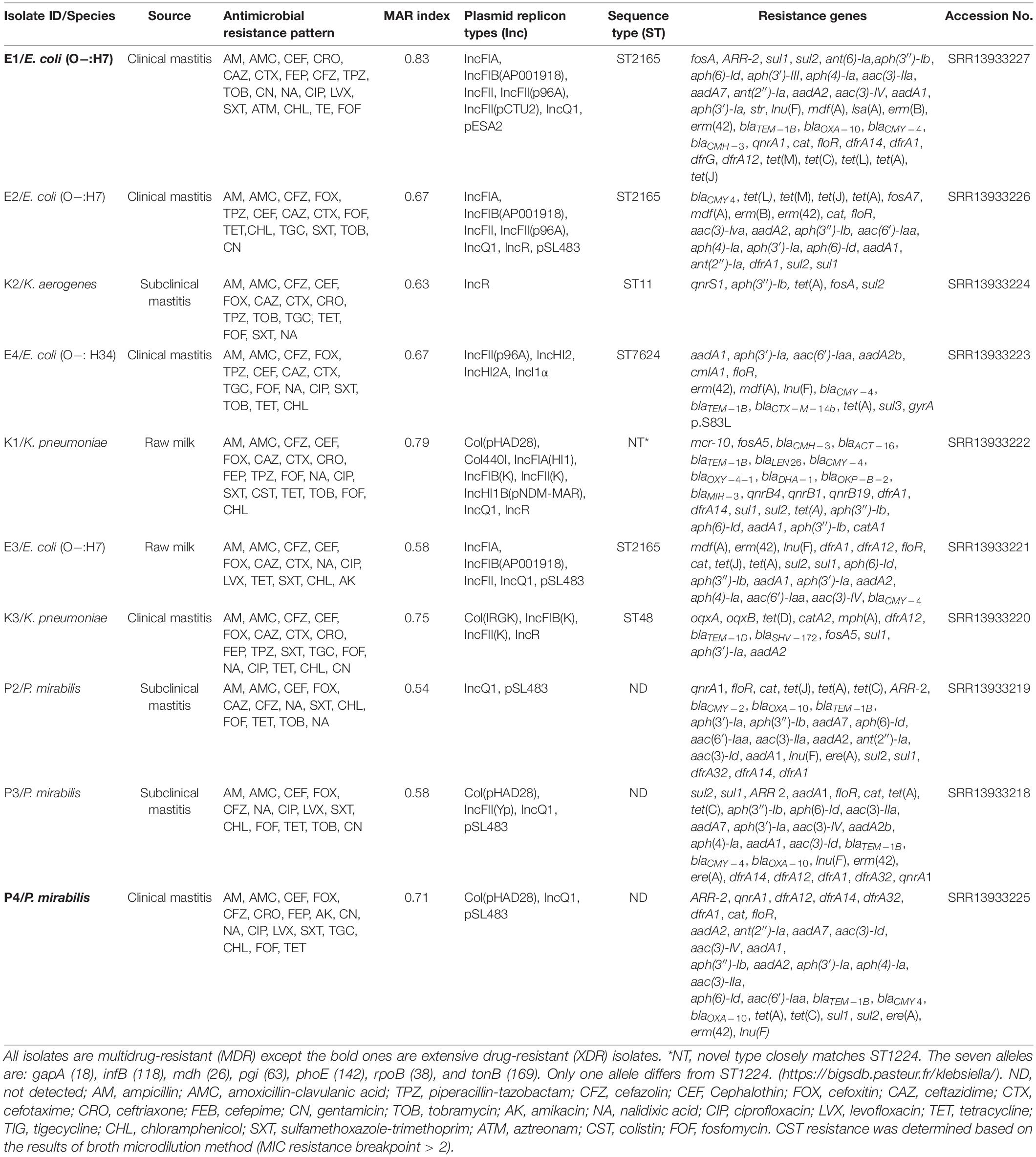
Table 2. Molecular characteristics of MDR and XDR Gram-negative bacteria recovered from milk samples.
Plasmid Characterization and Genetic Context of mcr-10 Gene
Plasmid reconstruction from the WGS of K. pneumoniae isolate (K1) using Plasmid SPAdes and PLACNETw revealed the existence of mcr-10 gene on an IncFIB plasmid (pK1). BLASTn of the reconstructed pK1 plasmid showed sequence similarities with the backbone of other IncF plasmids like Enterobacter kobei STW0522-51 (AP022432.1) plasmid pSTW0522-51-1 and Enterobacter roggenkampii Ecl_20_981 (CP048651.1) plasmid pEcl_20_981 (Figure 1). Our pK1 plasmid and the two NCBI retrieved plasmids, pSTW0522-51-1 and pEcl_20_981, possessed transfer (tra) genes, which are responsible for the plasmid conjugation. Genetic mapping of mcr-10 gene as depicted in Figure 2 revealed the existence of mcr-10 flanked by xerC and IS26 in our K1 isolate, xerC and IS2 in isolate Ecl_20_981 (CP048651.1) and xerC and IS21 in isolate STW0522-51 (AP022432.1).
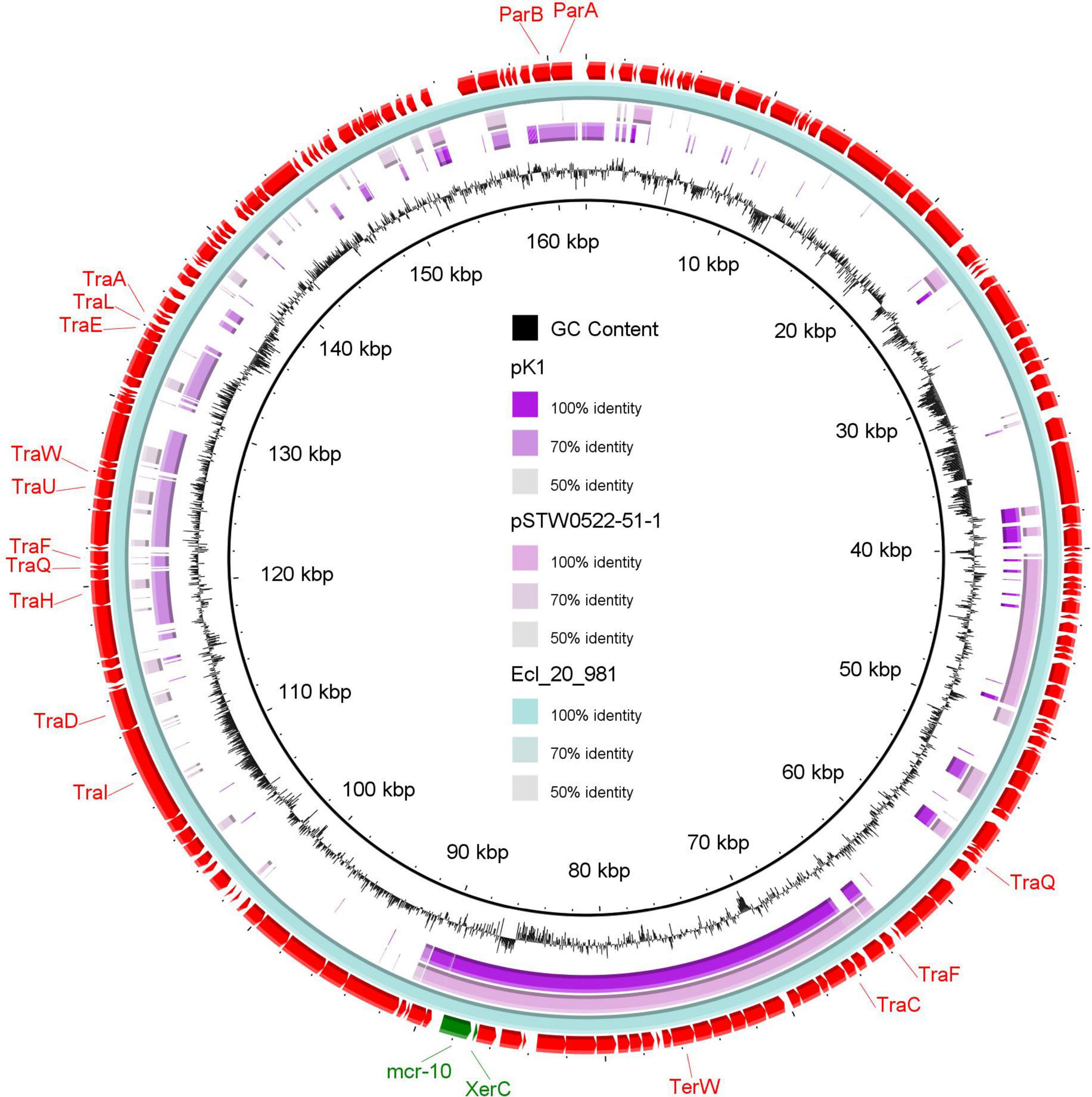
Figure 1. A circular map of the reconstructed IncFIB plasmid (pK1) from the draft genome sequence of K. pneumoniae (study isolate) compared to two NCBI retrieved plasmids, pEcl_20_981 of Enterobacter roggenkampii strain Ecl_20_981 (CP048651.1) and pSTW0522-51-1 of Enterobacter kobei strain STW0522-51 (AP022432.1). The out-layer circle (red color) represents plasmid pEcl_20_981, which used as the reference plasmid for sequence comparisons. The figure was generated using BLAST Ring Image Generator (BRIG) tool (http://sourceforge.net/projects/brig).
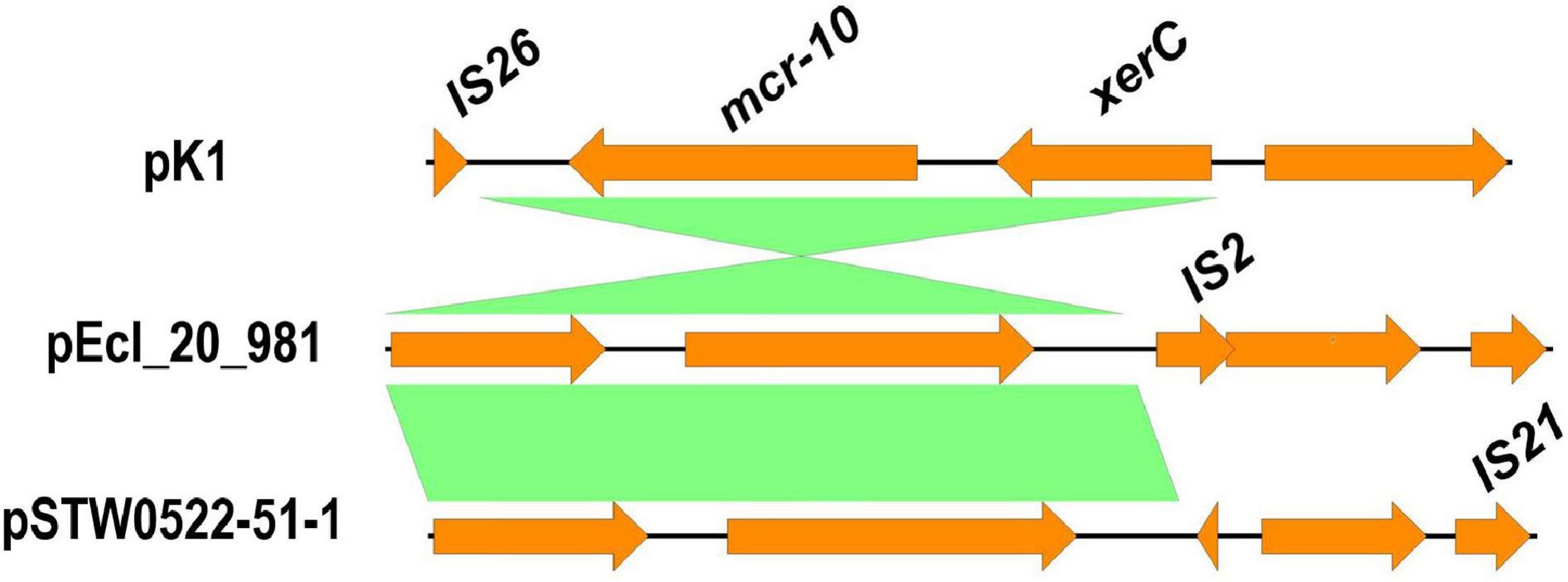
Figure 2. Schematic representation of the genetic environments of mcr-10 identified from the draft genome sequence of K. pneumoniae isolate (K1). The figure was drawn using the EasyFig tool (http://mjsull.github.io/Easyfig/).
Epidemiological Relatedness Between mcr-10-Harboring Isolate and the Publicly Available Klebsiella pneumoniae Isolates
The SNPs-based phylogenetic analysis was performed to determine the epidemiological relatedness between the study isolate (K1) and the publicly available K. pneumoniae isolates (n = 241) harboring mcr genes in the NCBI database. The findings showed clustering of K1 isolate in a clade containing isolates (n = 25) from humans (clinical), environment, and animals from different countries, including China, Singapore, Thailand, United States, Laos, and Myanmar (Figure 3 and Supplementary Table 2A). Likewise, when comparing our isolate to mcr-10-producing K. pneumoniae isolates from NCBI, the findings revealed the existence of our K1 isolate in a clade encompassing isolates belonging to various STs and sourced from humans and environment from different countries [United Kingdom (ST788), Australia (ST323), Malawi (ST2144), Myanmar (ST705), and Laos (ST2355)] (Figure 4 and Supplementary Table 2B).
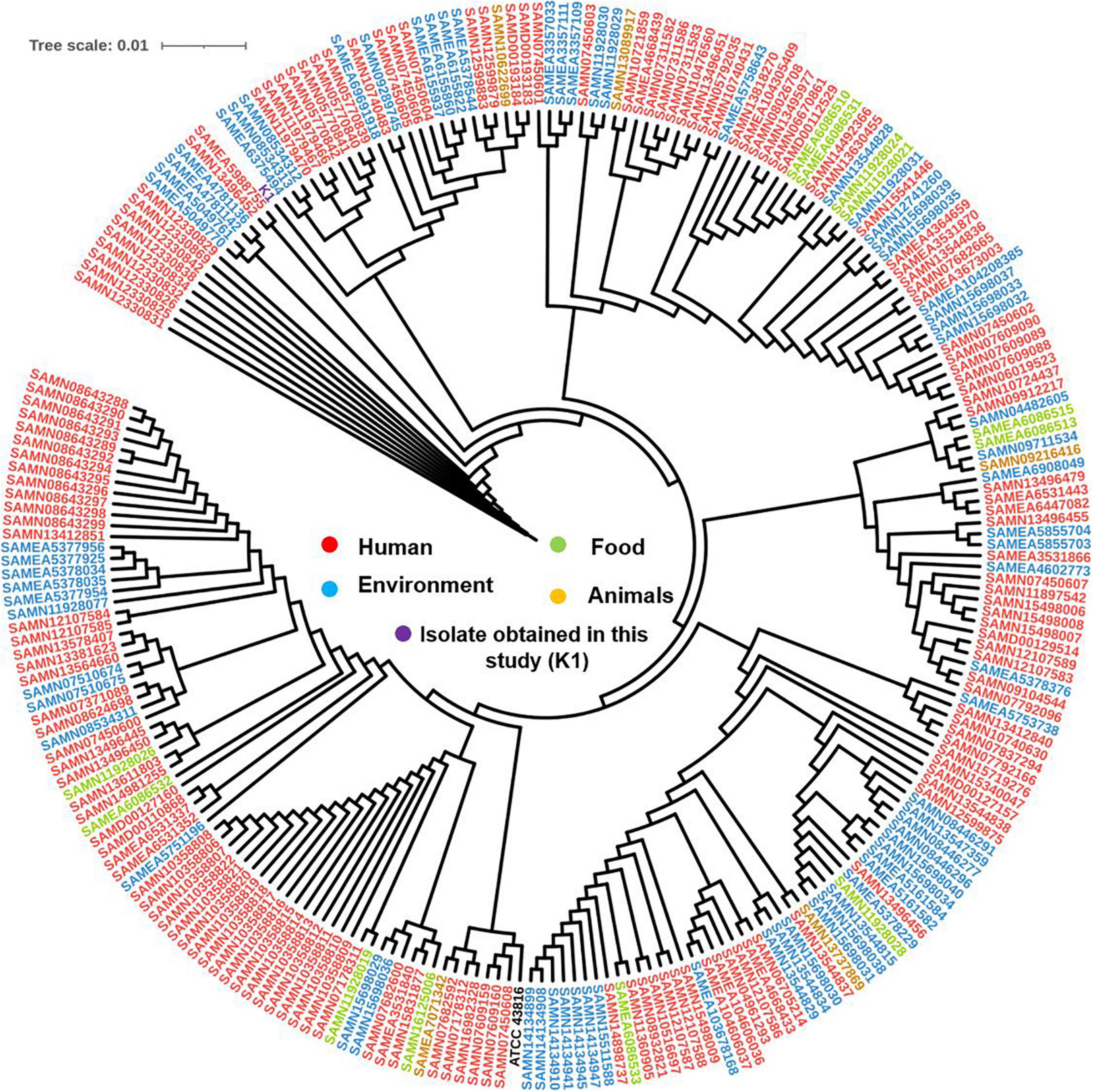
Figure 3. Global phylogenomic analysis of the present study K. pneumoniae isolate (K1) and all the publicly available genomes of K. pneumoniae (n = 241) in the NCBI database that carried any variant of mcr gene. Isolates were mapped to a reference K. pneumoniae complete genome ATCC 43816 (CP064352.1).
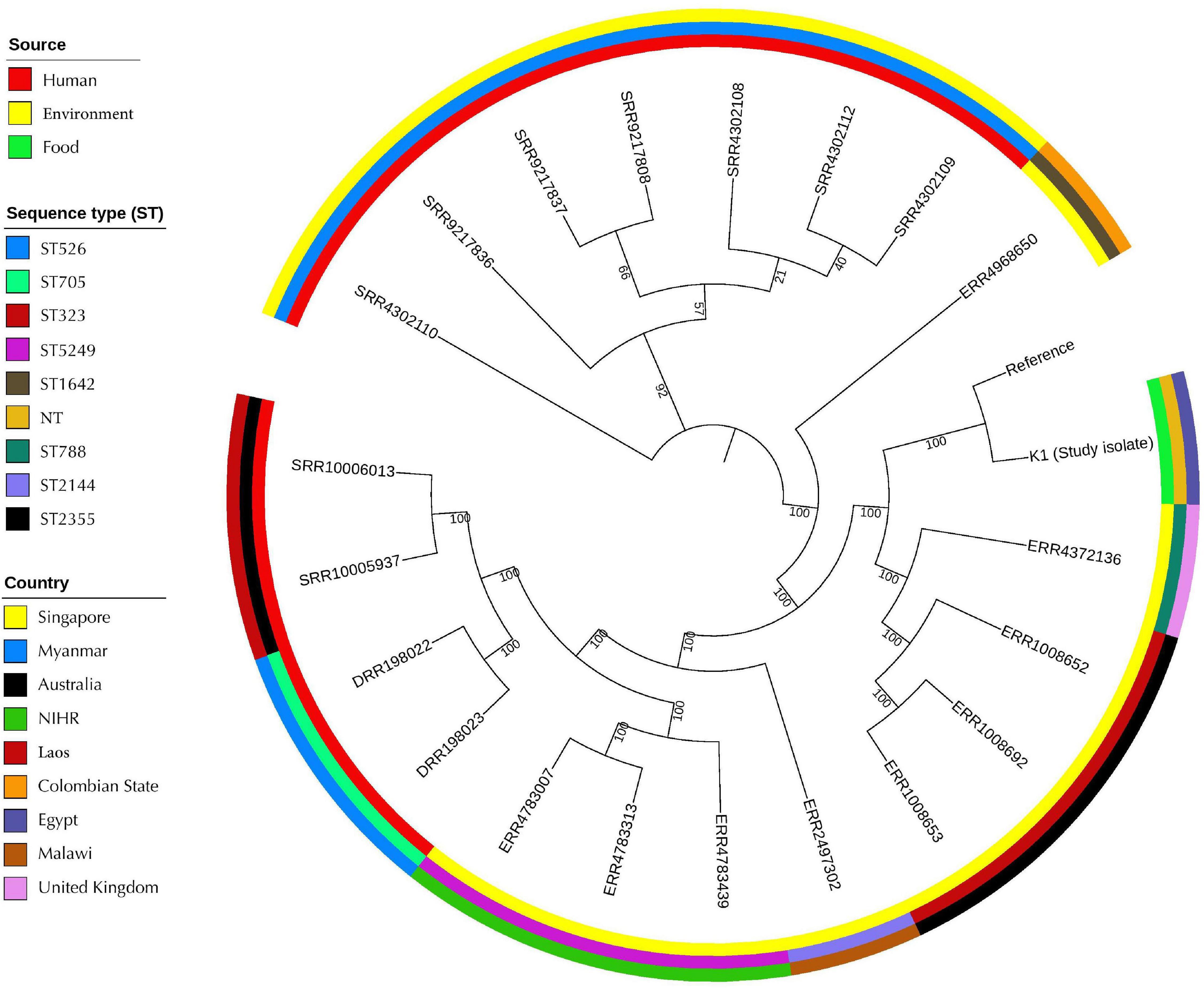
Figure 4. Global phylogenomic analysis of the present study K. pneumoniae isolate (K1) and the publicly available genomes of mcr-10-producing K. pneumoniae (n = 20) in the NCBI database. Isolates were mapped to a reference K. pneumoniae complete genome 30684/NJST258_2 (CP006918.1).
Discussion
The development of resistance to numerous antimicrobial agents in pathogenic microbes has become a critical threat, as there are fewer or indeed no effective antimicrobial agents beneficial for treating these organisms. The recent global dissemination of MDR and XDR Gram-negative bacteria has raised the alarm of antimicrobial resistance as a serious and an urgent threat to public health. Consequently, studies have been conducted to explore the distribution of several resistance genes in different reservoirs (Tartor and El-Naenaeey, 2016; Elmowalid et al., 2018; Abd El-Aziz et al., 2021c).
Mastitis is considered one of the most frequent diseases in dairy cows in Egypt. Here, we studied MDR and XDR Gram-negative bacteria isolated from mastitic and raw unpasteurized milk. Many studies have confirmed the presence of Gram-negative bacteria in mastitic milk (Hogan and Larry Smith, 2003; Malinowski et al., 2006; Nam et al., 2009). Similarly, we identified a variety of Gram-negative bacteria from milk samples. The most frequent was E. coli (48.91%), followed by P. mirabilis (17.9%), while the least isolated one was C. freundii (2.1%). Comparably, Nam et al. (2009) declared that E. coli predominated the Gram-negative pathogens from milk samples, while C. freundii was the least frequent one. Our prevalence of Gram-negative bacteria in subclinical mastitis was lower than those reported earlier (Mahlangu et al., 2018). These variations in the prevalence could be attributed to the changes in the host, the management factors, and the environmental differences that impact the infection (Amin et al., 2013).
Interestingly, we reported 24.46% (45/184) of the isolated bacteria from raw unpasteurized milk, which directs our attention to the human being, especially when talking later about MDR and XDR isolates as well as colistin resistance. A recent study revealed the presence of Gram-negative bacteria in raw milk and other dairy products in Egypt and imputed this contamination to the evidence that most of Gram-negative bacteria constitute a part of the flora of the mouth and intestinal tract of humans and animals as well as their presence in soil, water, and sewage (Sobeih et al., 2020).
Multidrug-resistant isolates were known to be common in mastitis milk samples. Herein, A. hydrophila, K. pneumoniae, E. cloacae (100% each), E. coli (87.78%), and P. mirabilis (69.7%) were MDR. All MDR A. hydrophila isolates were isolated from raw milk. Previous reports declared that MDR A. hydrophila isolates were commonly isolated from water and fish samples (Igbinosa et al., 2013; Tartor et al., 2021a), which affirm the role of human beings and environment in transferring MDR A. hydrophila to the raw milk.
Similarly, although we identified all E. cloacae as MDR isolates, they were previously reported with a low frequency (7.1%) in milk samples from Egypt in 2011 (Ahmed and Shimamoto, 2011). However, they were recently detected in human samples (Huang et al., 2021), which in turn emphasizes again the concept of transferring between animal/animal products including milk from/to human and animal during these 10 years. Alternatively, MDR K. pneumoniae isolates were widely detected in milk samples (Yang et al., 2020) and this is concordant with what was reported in our study.
It is known that E. coli is the major pathogen causing environmental mastitis and the spreading of MDR E. coli is considered a key challenge in mastitis treatment, especially, when combined with an XDR phenotype (Basak et al., 2016). We identified 7.07% XDR E. coli and P. mirabilis isolates. XDR is considered a pop-up threat; the emergence of commonly XDR, carbapenem-resistant, and ESBL producing E. coli reviewing the abundant usage of the broad-spectrum antimicrobials. A former report on MDR and XDR P. mirabilis infections has revealed that aminoglycosides, penicillins, cephalosporins, carbapenems, and fluoroquinolones have become ineffective as first-line agents (Magiorakos et al., 2012). Serotyping of E. coli isolates showed 10 different serotypes. As expected from the previous reports, O111:H4 was the predominant type in clinical and subclinical mastitis, whereas O157:H7 was prevalent in raw milk (Denny et al., 2008; Sayed, 2014; Ababu et al., 2020).
Since the first colistin-resistant mutant that was reported in 1981 (Vaara et al., 1981), colistin resistance has been evolving in several microorganisms like A. baumannii (Potron et al., 2019), K. pneumoniae (Shankar et al., 2019; Tartor et al., 2021b), and E. cloacae (Huang et al., 2019). A recent study has reported mcr-harboring isolates from animals and humans (Luo et al., 2020) and another group has isolated mcr-positive E. coli and K. pneumoniae from cancerous patients in Egypt (Zafer et al., 2019). Concordant with previous studies, we reported mcr-10 harboring K. pneumoniae.
To go deeper in the genetic and molecular characterization of MDR and XDR Gram-negative isolates, we selected the isolates with the highest MAR indices for WGS analysis. E. coli were found to have two-allele sequence types; ST2165 and ST7624. Although ST2165 was previously detected in colistin-resistant E. coli carrying mcr-1 from residents of long-term-care facilities (Giufrè et al., 2016), it was isolated elsewhere from chickens and store-bought produce (Reid et al., 2020; Foster-Nyarko et al., 2021). To our knowledge, this is the first isolation of this sequence type from milk. Similarly, E. coli ST7624 was not identified before from milk samples, although it was reported in wildlife (Arribas, 2019). In the view of plasmid replicon types, we found that the three E. coli isolates belonging to ST2165 have plasmid replicon types; IncFIA, IncFIB, IncFII, and IncQ1. Likewise, Foster-Nyarko et al. (2021) reported that the MDR isolates often co-carried large IncF plasmids like IncFIA, IncFIB, or IncFIC.
Herein, we found that E. coli isolates belonging to ST2165 are carrying several antibiotic resistance genes (Table 2), which confirms that IncF plasmids can play a potential role in disseminating antimicrobial resistance (Adenipekun et al., 2019). Comparably, the E. coli isolate belonging to ST7624 possessed multiple resistance genes to B-lactams (blaCMY–4, blaTEM–1B and blaCTX–M–14b), aminoglycosides [aadA1, aph(3′)-Ia, aac(6′)-Iaa and aadA2b], tetracyclines [tet(A)], sulfonamides (sul3), macrolides (erm), lincosamide (lnuF), and phenicols (floR, cmlA1), that might be attributed to the presence of IncFII, IncHI2, IncHI2A, and IncI1α replicon types. In a previous study, 27 acquired antimicrobial resistance genes were located on the IncHI2-IncHI2A-type plasmid, including blaCTX–M–65, fosA3, mphA, qepA, and rmtB (Zhang et al., 2021).
On the other hand, WGS analysis of Klebsiella species showed that K. pneumoniae had a novel ST, that was closely matched to ST1224, and ST48 alleles. In earlier studies, K. pneumoniae ST1224 was isolated from neonates (Chen et al., 2019), while K. pneumoniae ST48 was reported in chicken meat in Western Algeria, one of the Middle East region (Chaalal et al., 2021) indicating that these STs are not host specific and could be easily transmitted to humans from food animals and their products.
On contrary, although we identified ST11 in K. aerogenes, a previous study found ST11 in K. pneumoniae (Hao et al., 2019).
Interestingly, this study is the first isolation of mcr-10-carrying K. pneumoniae isolate from a raw milk sample. The mcr-10 gene flanked by xerC and IS26 in K1 isolate, xerC and IS2 in isolate Ecl_20_981 (CP048651.1) and xerC and IS21 in isolate STW0522-51 (AP022432.1). This possibly indicates the role of xerC gene, that encodes a tyrosine recombinase, in the mobilization of mcr-10 gene (Wang et al., 2020; Xu et al., 2021). Although the mcr-10 gene was detected recently in clinical strains of Enterobacter roggenkampii from different sources such as chickens (Lei et al., 2020), a patient (Wang et al., 2020), and sewage water (Xu et al., 2021), it is foremost found here in the raw milk sample that sequentially implicating the human in the transmission. Numerous isolates carrying mcr-1 gene obtained from hard cheese made from raw milk were reported in Egypt (Hammad et al., 2019; Ombarak et al., 2021), which in turn highlights the importance of raw milk quality control check in Egypt focusing on farm workers as well as the animal itself.
Furthermore, our mcr-10-producing K. pneumoniae isolate was also carrying fosA5 gene, which is responsible for fosfomycin resistance. Fosfomycin was known to be always effective in the treatment of MDR Enterobacterales (Falagas et al., 2010). However, such identification of the fosA5 gene in one of Enterobacterales species; K. pneumoniae (K1), isolated mainly from raw milk, is considered a terrifying situation. Recently, some studies reported the fosfomycin resistance in isolates from beef samples (Sadek et al., 2020), human sputum (Soliman et al., 2020), and chicken feces (Soliman et al., 2021) in Egypt. This might be attributed to the habitual utilization of fosfomycin in combination with aminoglycosides widely in Egypt for the treatment of numerous diseases. Due to the misuse of different antimicrobial agents in Egypt, we found K. pneumoniae isolate was carrying not only the mcr-10 and fosA5 genes, but also multiple resistance genes to ß-lactamases, aminoglycosides, quinolones, tetracyclines, macrolides, and folate pathway inhibitors; unfortunately, these antimicrobials are used at both human and veterinary levels.
To correlate the genetic character of our K1 isolate with similar K. pneumoniae isolates worldwide, we performed a phylogenetic analysis to find out their epidemiological relationship.
Probably, we found that our isolate was clustered with 25 K. pneumoniae isolates from different sources including human, environment, and animal (Figure 3) supporting the direct/indirect transmission between human and animals and this in tune with the recent global concept of dissemination of mobilized colistin resistance genes from animals to humans (Luo et al., 2020). Followingly, this result emphasizing “the One Health” concept; human health is connected to the health of animals and the environment (Luo et al., 2020). Remarkably, this result affirms that mcr genes debilitate human wellbeing and contaminate the environment due to the plausibility of their worldwide spread brought from the raising, generation, worldwide exchange, and food utilization of animals (Anyanwu et al., 2020).
Similarly, when comparing the study isolate (K1) with different mcr-10 harboring K. pneumoniae, our isolate presented in a clade consisting of isolates from numerous sources such as human and environment. Strikingly, this is the first identification of colistin mcr-10 resistance gene in K. pneumoniae in the Middle East. Entirely, all formerly identified mcr-10 carrying K. pneumoniae were detected in China, Europe, and Canada from clinical or environmental sources only. Given this close genetic distance between K1 isolate and the other mcr-10 isolates (Figure 4), we investigated the sequence type of each isolate and they showed different sequence types. This agrees with what was reported by Luo et al. (2020), who documented that the sequence type diversity of the mcr-positive E. coli isolates showed a scattered and non-clonal prevalence.
Conclusion
The emergence of mcr-10 and fosA5 harboring K. pneumoniae from raw milk is alarming and poses a public health threat. The existence of multiple resistance genes in MDR and XDR Gram-negative bacteria from dairy cattle provides evidence for its spread from the veterinary sector to humans and heralds the penetration of the last-resort antibiotics. Hence, prudent use of antibiotics in both humans and animals and antimicrobial surveillance plans are urgently required to fight XDR and MDR bacteria.
Data Availability Statement
The data that support the findings of this study are available in the SRA database under the bioproject PRJNA713498.
Ethics Statement
Ethical review and approval was not required for the animal study because this study did not include any experimental animals. Written informed consent was obtained from the owners for the participation of their animals in this study.
Author Contributions
YHT and NKA contributed equally in the conception and design of the study and participated with RMAG and HME in the application of classical microbiological and molecular techniques. ME participated with HR in whole-genome sequence analysis. HR performed the bioinformatics. RMAG, HME, SE, EAS, ASAA, SSA, MMB, YAE-S, and ME conceived the study and participated in the design. RMAG, HME, SE, EAS, ASAA, SSA, MMB, YAE-S, and ME participated in interpretation of data. YHT, NKA, RMAG, HME, SE, and HR wrote the initial draft of the manuscript. All authors revised the manuscript critically for important intellectual content and gave the final approval of the version to be published.
Conflict of Interest
The authors declare that the research was conducted in the absence of any commercial or financial relationships that could be construed as a potential conflict of interest.
Publisher’s Note
All claims expressed in this article are solely those of the authors and do not necessarily represent those of their affiliated organizations, or those of the publisher, the editors and the reviewers. Any product that may be evaluated in this article, or claim that may be made by its manufacturer, is not guaranteed or endorsed by the publisher.
Supplementary Material
The Supplementary Material for this article can be found online at: https://www.frontiersin.org/articles/10.3389/fmicb.2021.770813/full#supplementary-material
Footnotes
- ^ https://www.bioinformatics.babraham.ac.uk/projects/fastqc/
- ^ http://www.usadellab.org/cms/index.php?page=trimmomatic
- ^ https://cab.spbu.ru/software/spades/
- ^ http://www.genomicepidemiology.org/services/
- ^ http://bacdb.cn/BacWGSTdb/
- ^ https://blast.ncbi.nlm.nih.gov/Blast.cgi
- ^ https://www-is.biotoul.fr/blast.php
- ^ http://mjsull.github.io/Easyfig/
- ^ http://sourceforge.net/projects/brig
- ^ https://github.com/tseemann/snippy
References
Ababu, A., Endashaw, D., and Fesseha, H. (2020). Isolation and antimicrobial susceptibility profile of Escherichia coli O157: H7 from raw milk of dairy cattle in Holeta district, central Ethiopia. Int. J. Microbiol. 2020, 1–8. doi: 10.1155/2020/6626488
Abd El-Aziz, N. K., Ammar, A. M., El Damaty, H. M., Abd Elkader, R. A., Saad, H. A., El-Kazzaz, W., et al. (2021a). Environmental Streptococcus uberis associated with clinical mastitis in dairy cows: virulence traits, antimicrobial and biocide resistance, and epidemiological typing. Animals 11:1849.
Abd El-Aziz, N. K., Ammar, A. M., El-Naenaeey, E. Y. M., El Damaty, H. M., Elazazy, A. A., Hefny, A. A., et al. (2021b). Antimicrobial and antibiofilm potentials of cinnamon oil and silver nanoparticles against Streptococcus agalactiae isolated from bovine mastitis: new avenues for countering resistance. BMC Vet. Res. 17:136. doi: 10.1186/s12917-021-02842-9
Abd El-Aziz, N. K., Tartor, Y. H., Gharieb, R. M. A., Erfan, A. M., Khalifa, E., Said, M. A., et al. (2021c). Extensive drug-resistant Salmonella enterica isolated from poultry and humans: prevalence and molecular determinants behind the co-resistance to ciprofloxacin and tigecycline. Front. Microbiol. 12:759170. doi: 10.3389/fmicb.2021.738784
Adenipekun, E. O., Jackson, C. R., Ramadan, H., Iwalokun, B. A., Frye, J. G., Barrett, J. B., et al. (2019). Plasmid replicons and β-Lactamase-encoding genes of multidrug-resistant Escherichia coli isolated from humans and food animals in Lagos Southwest Nigeria. Microb. Drug Resist. 25, 1410–1423. doi: 10.1089/mdr.2018.0305
Ahmed, A. M., and Shimamoto, T. (2011). Molecular characterization of antimicrobial resistance in gram-negative bacteria isolated from bovine mastitis in Egypt. Microbiol. Immunol. 55, 318–327. doi: 10.1111/j.1348-0421.2011.00323.x
Alikhan, N. F., Petty, N. K., Ben Zakour, N. L., and Beatson, S. A. (2011). BLAST ring image generator (BRIG): simple prokaryote genome comparisons. BMC Genom. 12:402. doi: 10.1186/1471-2164-12-402
Amin, M., Samad, M., and Rahman, A. A. (2013). Bacterial pathogens and risk factors associated with mastitis in black Bengal goats in Bangladesh. Bangladesh J. Vet. Med. 9, 155–159. doi: 10.3329/bjvm.v9i2.13458
Anbazhagan, D., Kathirvalu, G. G., Mansor, M., Yan, G. O. S., Yusof, M. Y., and Sekaran, S. D. (2010). Multiplex polymerase chain reaction (PCR) assays for the detection of Enterobacteriaceae in clinical samples. Afr. J. Microbiol. Res. 4, 1186–1191.
Antipov, D., Hartwick, N., Shen, M., Raiko, M., Lapidus, A., and Pevzner, P. A. (2016). PlasmidSPAdes: assembling plasmids from whole genome sequencing data. Bioinformatics 32, 3380–3387. doi: 10.1093/bioinformatics/btw493
Anyanwu, M. U., Jaja, I. F., and Nwobi, O. C. (2020). Occurrence and characteristics of mobile colistin resistance (mcr) gene-containing isolates from the environment: a review. Int. J. Environ. Res. Public Health 17:1028. doi: 10.3390/ijerph17031028
Arribas, C. A. A. (2019). Molecular Epidemiology of Escherichia Coli from Wildlife: Antimicrobial Resistance, Virulence and Genetic Diversity, Epidemiología Molecular en Escherichia coli Procedente de Fauna Salvaje: Resistencia Antimicrobiana, Virulencia y Diversidad y Diversidad Genética. Spain: UNIR.
Bankevich, A., Nurk, S., Antipov, D., Gurevich, A. A., Dvorkin, M., Kulikov, A. S., et al. (2012). SPAdes: a new genome assembly algorithm and its applications to single-cell sequencing. J. Comput. Biol. 19, 455–477. doi: 10.1089/cmb.2012.0021
Basak, S., Singh, P., and Rajurkar, M. (2016). Multidrug resistant and extensively drug resistant bacteria: a study. J. Pathog. 2016:4065603. doi: 10.1155/2016/4065603
Bauer, A. W., Kirby, W. M., Sherris, J. C., and Turck, M. (1966). Antibiotic susceptibility testing by a standardized single disk method. Am. J. Clin. Pathol. 45, 493–496.
Biswas, S., Brunel, J. M., Dubus, J. C., Reynaud-Gaubert, M., and Rolain, J. M. (2012). Colistin: an update on the antibiotic of the 21st century. Expert Rev. Anti. Infect. Ther. 10, 917–934. doi: 10.1586/eri.12.78
Bolger, A. M., Lohse, M., and Usadel, B. (2014). Trimmomatic: a flexible trimmer for illumina sequence data. Bioinformatics 30, 2114–2120. doi: 10.1093/bioinformatics/btu170
Borowiak, M., Hammerl, J. A., Deneke, C., Fischer, J., Szabo, I., and Malorny, B. (2019). Characterization of mcr-5-harboring Salmonella enterica subsp. enterica Serovar Typhimurium isolates from animal and food origin in Germany. Antimicrob. Agents Chemother. 63, e00063–e00019. doi: 10.1128/AAC.00063-19
Carroll, L. M., Gaballa, A., Guldimann, C., Sullivan, G., Henderson, L. O., and Wiedmann, M. (2019). Identification of novel mobilized colistin resistance gene mcr-9 in a multidrug-resistant, colistin-susceptible Salmonella enterica serotype Typhimurium isolate. mBio 10, e00853–19. doi: 10.1128/mBio.00853-19
Cascón, A., Anguita, J., Hernanz, C., Sánchez, M., Fernández, M., and Naharro, G. (1996). Identification of Aeromonas hydrophila hybridization group 1 by PCR assays. Appl. Environ. Microbiol. 62, 1167–1170. doi: 10.1128/aem.62.4.1167-1170.1996
Catry, B., Cavaleri, M., Baptiste, K., Grave, K., Grein, K., Holm, A., et al. (2015). Use of colistin-containing products within the European Union and European Economic Area (EU/EEA): development of resistance in animals and possible impact on human and animal health. Int. J. Antimicrob. Agents 46, 297–306. doi: 10.1016/j.ijantimicag.2015.06.005
Chaalal, N., Touati, A., Bakour, S., Aissa, M. A., Sotto, A., Lavigne, J.-P., et al. (2021). Spread of OXA-48 and NDM-1-producing Klebsiella pneumoniae ST48 and ST101 in chicken meat in Western Algeria. Microb. Drug Resist. 27, 492–500. doi: 10.1089/mdr.2019.0419
Chen, D., Hu, X., Chen, F., Li, H., Wang, D., Li, X., et al. (2019). Co-outbreak of multidrug resistance and a novel ST3006 Klebsiella pneumoniae in a neonatal intensive care unit: a retrospective study. Medicine 98:e14285. doi: 10.1097/MD.0000000000014285
CLSI (2017). M100 Performance Standards for Antimicrobial Susceptibility Testing. Wayne, PA: Clinical and Laboratory Standards Institute.
Denny, J., Bhat, M., and Eckmann, K. (2008). Outbreak of Escherichia coli O157:H7 associated with raw milk consumption in the Pacific Northwest. Foodborne Pathog. Dis. 5, 321–328. doi: 10.1089/fpd.2007.0072
Disassa, N., Sibhat, B., Mengistu, S., Muktar, Y., and Belina, D. (2017). Prevalence and antimicrobial susceptibility pattern of E. coli O157:H7 isolated from traditionally marketed raw cow milk in and around Asosa town, Western Ethiopia. Vet. Med. Int. 2017:7581531. doi: 10.1155/2017/7581531
Elmowalid, G. A., Ahmad, A. A. M., Hassan, M. N., Abd El-Aziz, N. K., Abdelwahab, A. M., and Elwan, S. I. (2018). Molecular detection of new SHV β-lactamase variants in clinical Escherichia coli and Klebsiella pneumoniae isolates from Egypt. Comp. Immunol. Microbiol. Infect. Dis. 60, 35–41. doi: 10.1016/j.cimid.2018.09.013
Eucast: The European Committee on Antimicrobial Susceptibility Testing (2018). Breakpoint Tables for Interpretation of MICs and Zone Diameters. Version 8.1. Available online at: http://www.eucast.org. (Accessed date October 14, 2020).
Faddin, J. F. M. (2000). Biochemical Tests for Identification of Medical Bacteria. Philadelphia, PA: Lippincott Williams & Wilkins.
Falagas, M. E., Kastoris, A. C., Kapaskelis, A. M., and Karageorgopoulos, D. E. (2010). Fosfomycin for the treatment of multidrug-resistant, including extended-spectrum β-lactamase producing, Enterobacteriaceae infections: a systematic review. Lancet Infect. Dis. 10, 43–50. doi: 10.1016/S1473-3099(09)70325-1
Foster-Nyarko, E., Alikhan, N. F., Ravi, A., Thomson, N. M., Jarju, S., Kwambana-Adams, B. A., et al. (2021). Genomic diversity of Escherichia coli isolates from backyard chickens and guinea fowl in the Gambia. Microb. Genom. 7:mgen000484. doi: 10.1099/mgen.0.000484
Garedew, L., Berhanu, A., Mengesha, D., and Tsegay, G. (2012). Identification of Gram-negative bacteria from critical control points of raw and pasteurized cow milk consumed at Gondar town and its suburbs, Ethiopia. BMC Public Health 12:950. doi: 10.1186/1471-2458-12-950
Gelbíčová, T., Baráková, A., Florianová, M., and Karpíšková, R. (2019). Detection of colistin-resistant Acinetobacter baumannii with the mcr-4 gene. Klin. Mikrobiol. Infekc. Lek. 25, 4–6.
Giufrè, M., Monaco, M., Accogli, M., Pantosti, A., and Cerquetti, M. (2016). Emergence of the colistin resistance mcr-1 determinant in commensal Escherichia coli from residents of long-term-care facilities in Italy. J. Antimicrob. Chemother. 71, 2329–2331. doi: 10.1093/jac/dkw195
Hammad, A. M., Hoffmann, M., Gonzalez-Escalona, N., Abbas, N. H., Yao, K., Koenig, S., et al. (2019). Genomic features of colistin resistant Escherichia coli ST69 strain harboring mcr-1 on IncHI2 plasmid from raw milk cheese in Egypt. Infect. Genet. Evol. 73, 126–131. doi: 10.1016/j.meegid.2019.04.021
Hao, M., Shen, Z., Ye, M., Hu, F., Xu, X., Yang, Y., et al. (2019). Outbreak of Klebsiella pneumoniae carbapenemase-producing Klebsiella aerogenes strains in a tertiary hospital in China. Infect. Drug Resist. 12, 3283–3290. doi: 10.2147/IDR.S221279
Heijnen, L., and Medema, G. (2006). Quantitative detection of E. coli, E. coli O157 and other shiga toxin producing E. coli in water samples using a culture method combined with real-time PCR. J. Water Health 4, 487–498.
Hogan, J., and Larry Smith, K. (2003). Coliform mastitis. Vet. Res. 34, 507–519. doi: 10.1051/vetres:2003022
Huang, J., Xu, Q., Liu, F., Hao Xiong, H., and Yang, J. (2021). Enterobacter cloacae infection of the shoulder in a 52-year-old woman without apparent predisposing risk factor: a case report and literature review. BMC Infect. Dis. 21:13. doi: 10.1186/s12879-020-05699-9
Huang, L., Feng, Y., and Zong, Z. (2019). Heterogeneous resistance to colistin in Enterobacter cloacae complex due to a new small transmembrane protein. J. Antimicrob. Chemother. 74, 2551–2558. doi: 10.1093/jac/dkz236
Igbinosa, I. H., Chigor, V. N., Igbinosa, E. O., Obi, L. C., and Okoh, A. I. (2013). Antibiogram, adhesive characteristics, and incidence of class 1 integron in aeromonas species isolated from two South African rivers. Biomed. Res. Int. 2013:127570. doi: 10.1155/2013/127570
Lei, C. W., Zhang, Y., Wang, Y. T., and Wang, H. N. (2020). Detection of mobile colistin resistance gene mcr-10.1 in a conjugative plasmid from Enterobacter roggenkampii of chicken origin in China. Antimicrob. Agents Chemother. 64, e01191–e01120. doi: 10.1128/AAC.01191-20
Letunic, I., and Bork, P. (2016). Interactive tree of life (iTOL) v3: an online tool for the display and annotation of phylogenetic and other trees. Nucleic Acids Res. 44, W242–W245. doi: 10.1093/nar/gkw290
Liu, Y., Liu, C., Zheng, W., Zhang, X., Yu, J., Gao, Q., et al. (2008). PCR detection of Klebsiella pneumoniae in infant formula based on 16S-23S internal transcribed spacer. Int. J. Food Microbiol. 125, 230–235. doi: 10.1016/j.ijfoodmicro.2008.03.005
Liu, Y. Y., Wang, Y., Walsh, T. R., Yi, L. X., Zhang, R., Spencer, J., et al. (2016). Emergence of plasmid-mediated colistin resistance mechanism MCR-1 in animals and human beings in China: a microbiological and molecular biological study. Lancet Infect. Dis. 16, 161–168. doi: 10.1016/S1473-3099(15)00424-7
Luo, Q., Wang, Y., and Xiao, Y. (2020). Prevalence and transmission of mobilized colistin resistance (mcr) gene in bacteria common to animals and humans. Biosaf. Health 2, 71–78. doi: 10.1016/j.bsheal.2020.05.001
Magiorakos, A., Srinivasan, A., Carey, R. B., Carmeli, Y., Falagas, M. E., Giske, C. G., et al. (2012). Multidrug-resistant, extensively drug-resistant and pandrug-resistant bacteria: an international expert proposal for interim standard definitions for acquired resistance. Clin. Microbiol. Infect. 18, 268–281. doi: 10.1111/j.14690691.2011.03570.x
Mahlangu, P., Maina, N., and Kagira, J. (2018). Prevalence, risk factors, and antibiogram of bacteria isolated from milk of goats with subclinical mastitis in Thika East subcounty, Kenya. J. Vet. Med. 2018:3801479. doi: 10.1155/2018/3801479
Malinowski, E., Lassa, H., Kłossowska, A., Smulski, S., Markiewicz, H., and Kaczmarowski, M. (2006). Etiological agents of dairy cows’ mastitis in western part of Poland. Pol. J. Vet. Sci. 9, 191–194.
Nam, H. M., Lim, S. K., Kang, H. M., Kim, J. M., Moon, J. S., Jang, K. C., et al. (2009). Prevalence and antimicrobial susceptibility of gram-negative bacteria isolated from bovine mastitis between 2003 and 2008 in Korea. J. Dairy Sci. 92, 2020–2026. doi: 10.3168/jds.2008-1739
National Mastitis Council (1999). Laboratory Handbook on Bovine Mastitis. Madison, WI: National Mastitis Council.
Neyra, R. C., Frisancho, J. A., Rinsky, J. L., Resnick, C., Carroll, K. C., Rule, A. M., et al. (2014). Multidrug-resistant and methicillin-resistant Staphylococcus aureus (MRSA) in Hog slaughter and processing plant workers and their community in North Carolina (USA). Environ. Health Perspect. 122, 471–477. doi: 10.1289/ehp.1306741
Oliver, S. P., Boor, K. J., Murphy, S. C., and Murinda, S. E. (2009). Food safety hazards associated with consumption of raw milk. Foodborne Pathog. Dis. 6, 793–806. doi: 10.1089/fpd.2009.0302
Oliver, S. P., Jayarao, B. M., and Almeida, R. A. (2005). Foodborne pathogens in milk and the dairy farm environment: food safety and public health implications. Foodborne Pathog. Dis. 2, 115–129. doi: 10.1089/fpd.2005.2.115
Ombarak, R. A., Awasthi, S. P., Hatanaka, N., and Yamasaki, S. (2021). Detection of plasmid mediated colistin resistance mcr-1 gene in ESBL producing Escherichia coli isolated from raw milk hard cheese in Egypt. Int. Dairy J. 117:104986. doi: 10.1016/j.idairyj.2021.104986
Poirel, L., Jayol, A., and Nordmanna, P. (2017). Polymyxins: antibacterial activity, susceptibility testing, and resistance mechanisms encoded by plasmids or chromosomes. Clin. Microbiol. Rev. 30, 557–596. doi: 10.1128/CMR.00064-16
Potron, A., Bour, M., Triponney, P., Muller, J., Koebel, C., Bonnin, A., et al. (2019). Sequential emergence of colistin and rifampicin resistance in an OXA-72- producing outbreak strain of Acinetobacter baumannii. Int. J. Antimicrob. Agents 53, 669–673. doi: 10.1016/j.ijantimicag.2019.01.012
Rankin, D. I. (2005). “Test methods: MIC testing,” in Manual of Antimicrobial Susceptibility Testing, ed. B. M. Coyle (Washington, DC: American Society for Microbiology), 53–62.
Reid, C. J., Blau, K., Jechalke, S., Smalla, K., and Djordjevic, S. P. (2020). Whole genome sequencing of Escherichia coli from store-bought produce. Front. Microbiol. 10:3050. doi: 10.3389/fmicb.2019.03050
Ruan, Z., and Feng, Y. (2016). BacWGSTdb, a database for genotyping and source tracking bacterial pathogens. Nucleic Acids Res. 44, D682–D687. doi: 10.1093/nar/gkv1004
Ruegg, P. L. (2017). A 100-year review: mastitis detection, management, and prevention. J. Dairy Sci. 100, 10381–10397. doi: 10.3168/jds.2017-13023
Sadek, M., Nariya, H., Shimamoto, T., Kayama, S., Yu, L., Hisatsune, J., et al. (2020). First genomic characterization of blavim–1 and mcr-9-coharbouring Enterobacter hormaechei isolated from food of animal origin. Pathogens 9:687. doi: 10.3390/pathogens9090687
Sayed, S. M. (2014). A contribution on coliforms causing mastitis in cows with reference to serotypes and virulence factors of E. coli isolates. Assiut Univ. Bull. Environ. Res. 17, 8393.
Shankar, C., Venkatesan, M., Rajan, R., Mani, D., Lal, B., Prakash, J. A. J., et al. (2019). Molecular characterization of colistin-resistant Klebsiella pneumoniae & its clonal relationship among Indian isolates. Indian J. Med. Res. 149, 199–207. doi: 10.4103/ijmr.IJMR_2087_17
Shen, Z., Wang, Y., Shen, Y., Shen, J., and Wu, C. (2016). Early emergence of mcr-1 in Escherichia coli from food-producing animals. Lancet Infect. Dis. 16:293. doi: 10.1016/S1473-3099(16)00061-X
Sobeih, A., Al-Hawary, I., Khalifa, E., and Ebied, N. (2020). Prevalence of Enterobacteriaceae in raw milk and some dairy products. Kafrelsheikh Vet. Med. J. 18, 9–13. doi: 10.21608/kvmj.2020.39992.1009
Soliman, A. M., Maruyama, F., Zarad, H. O., Ota, A., Nariya, H., Shimamoto, T., et al. (2020). Emergence of a multidrug-resistant Enterobacter hormaechei clinical isolate from Egypt co-harboring mcr-9 and blaVIM−4. Microorganisms 8:595. doi: 10.3390/microorganisms8040595
Soliman, A. M., Ramadan, H., Zarad, H., Sugawara, Y., Yu, L., and Sugai, M. (2021). Co-production of Tet(X7) conferring high-level Tigecycline resistance, Fosfomycin FosA4 and Colistin Mcr-1.1 in Escherichia coli strains from chickens in Egypt. Antimicrob. Agents Chemother. 65, e02084–e02020. doi: 10.1128/aac.02084-20
Sullivan, M. J., Petty, N. K., and Beatson, S. A. (2011). Easyfig: a genome comparison visualizer. Bioinformatics 27, 1009–1010. doi: 10.1093/bioinformatics/btr039
Tambekar, D., Dhanorkar, D., Gulhane, S., Khandelwal, V., and Dudhane, M. (2006). Antibacterial susceptibility of some urinary tract pathogens to commonly used antibiotics. African J. Biotechnol. 5, 1562–1565. doi: 10.4314/AJB.V5I17.43162
Tartor, Y. H., and El-Naenaeey, E.-S. Y. (2016). RT-PCR detection of exotoxin genes expression in multidrug resistant Pseudomonas aeruginosa. Cell. Mol. Biol. 62, 56–62.
Tartor, Y. H., El-Naenaeey, E.-S. Y., Abdallah, H. M., Samir, M., Yassen, M. M., and Abdelwahab, A. M. (2021a). Virulotyping and genetic diversity of Aeromonas hydrophila isolated from Nile tilapia (Oreochromis niloticus) in aquaculture farms in Egypt. Aquaculture 541:736781. doi: 10.1016/j.aquaculture.2021.736781
Tartor, Y. H., Gharieb, R. M. A., Abd El-Aziz, N. K., El Damaty, H. M., Enany, S., Khalifa, E., et al. (2021b). Virulence determinants and plasmid-mediated colistin resistance mcr genes in Gram-negative bacteria isolated from bovine milk. Front. Cell. Infect. Microbiol. 11:761417. doi: 10.3389/fcimb.2021.761417
Vaara, M., Vaara, T., Jensen, M., Helander, I., Nurminen, M., Rietschel, E. T., et al. (1981). Characterization of the lipopolysaccharide from the polymyxin-resistant pmrA mutants of Salmonella Typhimurium. FEBS Lett. 129, 145–149. doi: 10.1016/0014-5793(81)80777-6
Vielva, L., de Toro, M., Lanza, V. F., and de la Cruz, F. (2017). PLACNETw: a web-based tool for plasmid reconstruction from bacterial genomes. Bioinformatics 33, 3796–3798. doi: 10.1093/bioinformatics/btx462
Wang, C., Feng, Y., Liu, L., Wei, L., Kang, M., and Zong, Z. (2020). Identification of novel mobile colistin resistance gene mcr-10. Emerg. Microbes Infect. 9, 508–516. doi: 10.1080/22221751.2020.1732231
Wang, X., Wang, Y., Zhou, Y., Wang, Z., Wang, Y., Zhang, S., et al. (2019). Emergence of colistin resistance gene mcr-8 and its variant in Raoultella ornithinolytica. Front. Microbiol. 10:228. doi: 10.3389/fmicb.2019.00228
Xavier, B. B., Lammens, C., Ruhal, R., Malhotra-Kumar, S., Butaye, P., Goossens, H., et al. (2016). Identification of a novel plasmid-mediated colistin resistance gene, mcr-2, in Escherichia coli, Belgium, June 2016. Euro Surveill. 21, doi: 10.2807/1560-7917.ES.2016.21.27.30280
Xu, T., Zhang, C., Ji, Y., Song, J., Liu, Y., Guo, Y., et al. (2021). Identification of mcr-10 carried by self-transmissible plasmids and chromosome in Enterobacter roggenkampii strains isolated from hospital sewage water. Environ. Pollut. 268:115706. doi: 10.1016/j.envpol.2020.115706
Yahav, D., Farbman, L., Leibovici, L., and Paul, M. (2012). Colistin: new lessons on an old antibiotic. Clin. Microbiol. Infect. 18, 18–29. doi: 10.1111/j.1469-0691.2011.03734.x
Yang, Y., Peng, Y., Jiang, J., Gong, Z., Zhu, H., Wang, K., et al. (2020). Isolation and characterization of multidrug-resistant Klebsiella pneumoniae from raw cow milk in Jiangsu and Shandong provinces, China. Transbound. Emerg. Dis. 68:1033-1039. doi: 10.1111/tbed.13787
Yin, W., Li, H., Shen, Y., Liu, Z., Wang, S., Shen, Z., et al. (2017). Novel plasmid-mediated colistin resistance gene mcr-3 in Escherichia coli. mBio 8, 8:e00543-e0017. doi: 10.1128/mBio.00543-17
Zafer, M. M., El-Mahallawy, H. A., Abdulhak, A., Amin, M. A., Al-Agamy, M. H., and Radwan, H. H. (2019). Emergence of colistin resistance in multidrug-resistant Klebsiella pneumoniae and Escherichia coli strains isolated from cancer patients. Ann. Clin. Microbiol. Antimicrob. 18:40. doi: 10.1186/s12941-019-0339-4
Zelalem, Y., and Bernard, F. (2006). Handling and microbial load of cow’s milk and irgo - fermented milk collected from different shops and producers in central highlands of Ethiopia. Ethiopian. Ethiop. J. Anim. Prod. 6, 67–82.
Zhang, W., Niu, Z., Yin, K., Liu, P., and Chen, L. (2013). Quick identification and quantification of Proteus mirabilis by polymerase chain reaction (PCR) assays. Ann. Microbiol. 63, 683–689. doi: 10.1007/s13213-012-0520-x
Keywords: Gram-negative bacteria, mastitis, Klebsiella pneumoniae, mcr-10, fosA5, whole-genome sequencing
Citation: Tartor YH, Abd El-Aziz NK, Gharieb RMA, El Damaty HM, Enany S, Soliman EA, Abdellatif SS, Attia ASA, Bahnass MM, El-Shazly YA, Elbediwi M and Ramadan H (2021) Whole-Genome Sequencing of Gram-Negative Bacteria Isolated From Bovine Mastitis and Raw Milk: The First Emergence of Colistin mcr-10 and Fosfomycin fosA5 Resistance Genes in Klebsiella pneumoniae in Middle East. Front. Microbiol. 12:770813. doi: 10.3389/fmicb.2021.770813
Received: 05 September 2021; Accepted: 01 November 2021;
Published: 08 December 2021.
Edited by:
Gerson Nakazato, State University of Londrina, BrazilReviewed by:
Jaroslav Hrabak, Charles University, CzechiaXiaobin Zheng, The Fifth Affiliated Hospital of Sun Yat-sen University, China
Copyright © 2021 Tartor, Abd El-Aziz, Gharieb, El Damaty, Enany, Soliman, Abdellatif, Attia, Bahnass, El-Shazly, Elbediwi and Ramadan. This is an open-access article distributed under the terms of the Creative Commons Attribution License (CC BY). The use, distribution or reproduction in other forums is permitted, provided the original author(s) and the copyright owner(s) are credited and that the original publication in this journal is cited, in accordance with accepted academic practice. No use, distribution or reproduction is permitted which does not comply with these terms.
*Correspondence: Norhan K. Abd El-Aziz, bm9yaGFuX3ZldEBob3RtYWlsLmNvbQ==; bm91cmhhbl92ZXRAenUuZWR1LmVn; Hazem Ramadan, aGF6ZW1faGFzc2FuQG1hbnMuZWR1LmVn
†These authors have contributed equally to this work and share first authorship
‡ORCID: Yasmine H. Tartor, orcid.org/0000-0003-1246-6548; Norhan K. Abd El-Aziz, orcid.org/0000-0001-8309-9058; Hazem Ramadan, orcid.org/0000-0002-6687-3758