- 1Institute of Comparative Medicine, College of Veterinary Medicine, Yangzhou University, Yangzhou, China
- 2Jiangsu Co-innovation Center for Prevention and Control of Important Animal Infectious Diseases and Zoonoses, Yangzhou University, Yangzhou, China
- 3Joint International Research Laboratory of Agriculture and Agri-Product Safety, The Ministry of Education of China, Yangzhou University, Yangzhou, China
- 4Nanjing Stomatological Hospital, Medical School of Nanjing University, Nanjing, China
Klebsiella oxytoca is an important nosocomial and community-acquired opportunistic pathogenic Klebsiella and has become the second most prevalent strain in the clinic after K. pneumoniae. However, there have been few reports of bacteriophages used for treating K. oxytoca. In this study, a novel bacteriophage, vB_Kox_ZX8, which specifically infects K. oxytoca AD3, was isolated for the first time from human fecal samples. The biological characteristics of vB_Kox_ZX8 showed an incubation period of 10 min, a burst size of 74 PFU/cell, and a stable pH range of 3–11. Genomic bioinformatics studies of vB_Kox_ZX8 showed that it belongs to the genus Przondovirus, subfamily Studiervirinae, family Autographiviridae. The genome of vB_Kox_ZX8 is 39,398 bp in length and contains 46 putative open reading frames encoding functional proteins, such as DNA degradation, packaging, structural, lysin-holin, and hypothetical proteins. We further investigated the efficacy of vB_Kox_ZX8 phage in the treatment of mice with bacteremia caused by K. oxytoca infection. The results showed that vB_Kox_ZX8 (5 × 109 PFU/mouse) injected intraperitoneally alone was metabolized rapidly in BALB/c mice, and no significant side effects were observed in the control and treatment groups. Importantly, intraperitoneal injection with a single dose of phage vB_Kox_ZX8 (5 × 107 PFU/mouse) for 1 h post-infection saved 100% of BALB/c mice from bacteremia induced by intraperitoneal challenge with a minimum lethal dose of K. oxytoca AD3. However, all negative control mice injected with PBS alone died. Owing to its good safety, narrow host infectivity, high lysis efficiency in vitro, and good in vivo therapeutic effect, phage vB_Kox_ZX8 has the potential to be an excellent antibacterial agent for clinical K. oxytoca-caused infections.
Introduction
The gram-negative bacterium Klebsiella, which exists in the normal flora of the oral cavity, skin, and intestine, is an opportunistic pathogen that can lead to nosocomial infection (Podschun and Ullmann, 1998). K. oxytoca is the second most prevalent Klebsiella in the clinic after K. pneumoniae (Neog et al., 2021). Opportunistic K. oxytoca in hospitals mainly infects infants, the elderly, and patients with low immune function, and is the main cause of colitis, endocarditis, sepsis, and urinary and respiratory tract infections (Beaugerie et al., 2003; Ménard et al., 2010; Egger et al., 2017; Tsubouchi et al., 2019; Soto-Hernández et al., 2020; Surani et al., 2020). The prevalence rate of K. oxytoca ranges from 2.3 to 24%, accounting for 13–24% of the total nosocomial bacteremia (Watanakunakorn and Jura, 1991; Hansen et al., 1998; Manohar et al., 2017; Ahmad et al., 2018). The high prevalence of K. oxytoca warrants extensive attention.
Bacteriophages (phages) are viruses that infect bacteria as their hosts and widely exist in nature. Phages have significant effects on bacteria-phage coevolution and microbial community ecology (Díaz-Muñoz and Koskella, 2014). In addition, there are a large number of phages in the human intestine, which are closely related to health and disease (Manrique et al., 2017; Seo and Kweon, 2019). Recently, owing to the emergence of multidrug-resistant bacteria worldwide, phages have been widely used as substitutes for traditional antibiotics (Kortright et al., 2019). Phage therapy has the advantage of rapid and highly selective bactericidal activity. In addition, phage therapy has a good therapeutic effect on mouse bacteremia, pneumonia, liver abscess, and burn infection caused by K. pneumoniae (Hung et al., 2011; Chadha et al., 2017; Kaabi and Musafer, 2019; Anand et al., 2020). However, only a small number of K. oxytoca phages have been reported, such as K. oxytoca phage ABG-IAUF-1, KLEB010, vB_Klox_2, and PKO111 (Karumidze et al., 2013; Brown et al., 2017; Park et al., 2017; Amiri Fahliyani et al., 2018). Therefore, more K. oxytoca phages and their therapeutic effects warrant further investigation. Based on transmission electron microscopy (TEM), there are approximately 1015 particles of phages in the human gastrointestinal tract, 1012 virus-like particles per gram in fecal samples, and the main families of phages are Myoviridae, Siphoviridae, and Podoviridae from the order Caudovirales (Dalmasso et al., 2014; Hoyles et al., 2014). With advances in sequencing technology, metagenomic analysis has become the main research method for analyzing intestinal phages. For instance, crAs-like and Microviridae phages were found to be the most stable colonizers of the human gut via metagenome sequencing (Shkoporov et al., 2019; Koonin and Yutin, 2020). In contrast, the isolation of gut-associated phages was confined to samples derived from sewage from human microbiota samples. Isolation of intestinal phages holds great promise for improving our knowledge of the gut virome, facilitating metagenomic studies, and aiding in vitro and in vivo studies to investigate the influence of phages on prokaryotic populations in the human gut (Manrique et al., 2017).
In this study, a lytic K. oxytoca phage, vB_Kox_ZX8, was isolated from a clinical fecal sample for the first time. The biological characteristics, genomic characteristics, and therapeutic effect of vB_Kox_ZX8 on mice with bacteremia caused by K. oxytoca AD3 (AD3) were investigated.
Materials and Methods
Animals
Male BABL/C mice (23–25 g) aged 6–8 weeks were purchased and cultured in the experimental animal center of Yangzhou University. All animal experiments were performed in strict accordance with the Regulations for the Administration of Affairs Concerning Experimental Animals approved by the State Council of the People’s Republic of China and the Animal Welfare and Research Ethics Committee at Yangzhou University.
Host and Culture Conditions
Klebsiella oxytoca AD3 was isolated from clinical samples provided by Nanjing Stomatological Hospital in January 2021 and cultured in lysogeny broth (LB) on an orbital shaker at 37°C and 220 rpm. The 16S rRNA gene was amplified using primers 27F and 1492R for identification. K1, K2, K5, K20, and K54 primers were used for serotyping. RmpA, allS, ybtA, iucB, iroNB, fimH, ureA, uge, wabG, and wcaG were determined for virulence factors (Supplementary Table 1). Multi-locus sequence typing (MLST) was performed using the primers provided by the Institut Pasteur MLST.1 Antibiotic resistance of bacteria was determined using the standard Kirby-Bauer disk diffusion method, and the interpretation of the data was performed using the standardized protocol of the National Committee for Clinical Laboratory Standards and designated as R (resistant), I (intermediate sensitive), and S (sensitive) (Supplementary Table 2).
Isolation and Purification of Phage vB_Kox_ZX8
Phage vB_Kox_ZX8 was isolated from fecal samples collected from the Nanjing Stomatological Hospital in January 2021. Briefly, 1 g of fecal sample was mixed with 1 mL of PBS, and the solution was mixed well, centrifuged at 8228 × g for 5 min, and passed through a 0.22-μm filter. The supernatant filtrate was added to 5 mL of K. oxytoca AD3 cultures at log phase (OD600 = 0.6), and the solution was cultured at 37°C and 220 rpm for 2–4 h. The lysate was centrifuged at 18,514 × g for 2 min, and the phage in the supernatant was purified using the double plate method. Briefly, 300 μL of bacterial and 100 μL of 10-fold dilution series of phage were mixed and added to 5 mL of top LB soft agar, followed by pouring onto an LB agar plate and culturing at 37°C overnight. The next day, a single plaque was picked from the plate, inoculated into 5 mL of a log phase host, and cultured at 37°C and 220 rpm for 2–4 h. The cultures were centrifuged at 18,514 × g for 2 min, and the lysates were passed through a 0.22-μm filter. The above steps were repeated at least three times until a single phage was obtained.
The phage (MOI = 0.01) was added to 200 mL of log phase host and cultured at 37°C and 220 rpm for 2–4 h. The culture was centrifuged at 8228 × g for 10 min, and DNase I and RNase A (1 μg/mL) were added to the lysates and incubated at 37°C for 30 min. NaCl (1 M) was added to the supernatants and incubated in an ice bath for 1 h. After centrifugation at 8228 × g for 10 min, 10% (w/v) polyethylene glycol 8000 (PEG 8000) was added to the lysates and precipitated overnight at 4°C. After centrifugation at 18,514 × g for 20 min, the precipitate was resuspended in PBS and dissolved completely. An equal volume of chloroform was added to the above solution, and centrifuged at 4629 × g for 15 min, followed by transferring the upper water phase to a new centrifuge tube, and the above steps were repeated three times. The collected phages were concentrated in 100 KDa ultrafiltration tubes (Millipore, United States). The phage suspension was then stored at 4°C.
Biological Characteristics of Phage vB_Kox_ZX8
The morphology of the phage was observed using a transmission electron microscope (TEM) HT7800 (Hitachi, Japan). Before observation, the phage suspension was incubated on carbon grids (200 mesh) for 10 min, stained with 2% phosphotungstic acid for 3 min, and dried for 30 min.
The host spectrum of the isolated phages was determined using the spot-test assay on 25 bacterial strains isolated from patient fecal samples. The antibacterial activity was assayed against 10 K. oxytoca, 10 K. pneumoniae, 5 Escherichia coli, and 5 Proteus mirabilis strains (Supplementary Table 3). Briefly, 5 μL of phage (108 PFU/mL) was spotted onto fresh bacterial lawns and incubated at 37°C for 6–8 h. The experiment was repeated three times.
The one-step growth curve of the phage was measured to calculate its incubation period, outbreak period, and platform period. The phage suspension was added to a fresh host culture (108 CFU/mL) at a multiplicity of infection (MOI) of 0.01. The mixture was incubated at 37°C for 10 min and centrifuged at 18,500 × g for 1 min to remove the non-absorbed phages. The precipitate was re-suspended in LB broth (time zero) and cultured at 37°C and 220 rpm. Two parallel samples were taken every 10 min and centrifuged at 18,500 × g for 1 min. The phage titer in the supernatant was determined using the double agar plate method. Burst size was computed as the ratio of the final count of released phage particles to the initial count of infected bacterial cells during the latent period (Ciacci et al., 2018). The experiment was repeated three times.
Thermal stability test of phage: Phage suspension (300 μL, 108 PFU/mL) was incubated at 4, 40, 50, 60, 70, and 80°C for 20–60 min. The phage titer was determined using the double-layer plate method.
pH stability test of phage: Phage suspension (300 μL, 108 PFU/mL) was incubated at 37°C for 60 min under different pH values (2–11). The phage titer was determined using the double-layer plate method. The experiment was repeated three times.
The bactericidal effect of phages in vitro was evaluated by measuring the effect of phage on the number of bacteria in the culture tube. Different doses of phage vB_Kox_ZX8 (MOI = 10, 1, 0.1, 0.01, and 0.001) were added to log phase K. oxytoca AD3 (5 × 108 CFU/mL) and cultured at 37°C and 220 rpm. The OD600 of the culture was determined every 10 min using a Smart Microplate Reader (Tecan infinite M200 Pro, Switzerland). The experiment was repeated three times.
DNA Isolation, Genome Sequencing, and Analysis
Phage DNA was extracted using a Virus Genomic DNA/RNA Extraction Kit (Tiangen Biotechnology Co., Ltd., China). Whole-genome sequencing of the phage was completed by Shanghai Bioengineering Co., Ltd. The phage DNA fragments with a length of approximately 500 bp were randomly interrupted by a Covaris ultrasonic crusher (Covaris, United States), and then purified using hieff NGS DNA selection beads (Yeasen Biotechnology Co., Ltd. China). The sequencing library was constructed using the NEB Next Ultra DNA Library Prep Kit for Illumina (NEB, United States), including terminal repair, adaptor ligation, DNA purification, and library amplification. The DNA library was sequenced on the Illumina hiseqpe150 sequencing platform after passing the quality test. The original sequencing data were filtered first and assembled using new blew 3.0 software.
tRNAs were predicted using tRNAscan-SE2.02 (Lowe and Chan, 2016). The virulence factors and drug resistance of phage genome were compared with Virulence Factors of Pathogenic Bacteria3 and The Comprehensive Antibiotic Resistance Database.4
The open reading frame was annotated using the RAST annotation server web (Aziz et al., 2008). Automatic annotation was manually reviewed using the BLASTp algorithm against RefSeq proteins deposited in the GenBank database. Trans-membrane helical domains and signal sequences were analyzed using Phobius (Käll et al., 2007). Visualization of the phage genome was performed using Easyfig (Sullivan et al., 2011). Phylogenetic analysis was performed using the phage terminase large subunit and major capsid protein of the subfamily Studiervirinae reported by the International Committee on Taxonomy of Viruses classification. The protein alignments were obtained using ClustalW, and the phylogenetic trees were generated using the maximum likelihood method with a bootstrap of 1000 in MERGA 6.0.
Safety of Treatment of Mice With Phage vB_Kox_ZX8
The Toxin Eraser Endotoxin Removal Kit (Genscript Biotechnology Co., Ltd., China) was used to remove endotoxins from the phage. The purified phage suspension was quantified using the ToxinSensor Single Test Kit with Standard (Genscript Biotechnology Co., Ltd, China). Endotoxin concentrations below 0.005–0.01 endotoxin units (EU)/PFU were deemed safe for injection in accordance with published data (Gangwar et al., 2021).
Each mouse in the experimental group was intraperitoneally (IP) injected with 100 μL of purified phage vB_Kox_ZX8 (5 × 109 and 5 × 107 PFU), and 100 μL of PBS was injected as a control, with five mice in each group. The weight and survival rates of the mice in each group were observed. The titers of phages in blood and tissues were determined using the double-layer plate method. The levels of tumor necrosis factor-α (TNF-α), interleukin 6 (IL-6), and interleukin 10 (IL-10) in organs were determined using ELISA.
Mouse Model of Bacteremia
Klebsiella oxytoca AD3 bacteria were cultured overnight and then centrifuged at 8228 × g for 2 min, followed by washing twice with PBS. Mice (six mice in each group) in the experimental group were IP injected with 100 μL of different doses of bacterial suspension (5 × 108, 108, 5 × 107, 107, 5 × 106, and 106 CFU) to determine the minimum lethal dose (MLD). MLD is the dose of bacteria that could cause all mice to die within 7 days. PBS (100 μL) was injected into the negative control group. The weight and survival rate of the mice in each group were recorded. Each mouse was IP injected with 100 μL of bacteria at the MLD to prepare the bacteremia mouse.
Phage vB_Kox_ZX8 Treatment Rescues K. oxytoca AD3-Infected Mice
Each mouse was IP injected with 100 μL of K. oxytoca AD3 at the MLD. After 1 h, different doses of phage vB_Kox_ZX8 (5 × 107, 5 × 106, 5 × 105 PFU) were IP injected into the experimental group, and 100 μL of PBS was injected as a control, with six mice in each group. The weight and survival rates of the mice in each group were recorded. The titers of K. oxytoca AD3 and phage vB_Kox_ZX8 in blood and organs were determined using bacterial monoclonal plate culture and double plate experiments, respectively.
After 48 h, the animals were euthanized, and tissues were collected. The organs were fixed with 4% paraformaldehyde for 24 h, dehydrated in alcohol, paraffin-embedded, sectioned, xylene dewaxed, hematoxylin and eosin stained, dehydrated in alcohol, and covered with glass. Pathological changes in the organs of the mice were observed.
Quantitation of Bacteremia and Phage in Mouse Blood and Tissue
Blood and various organs, including the eyeball, blood, heart, lungs, thymus, right upper liver, spleen, right kidney, and small intestine (2–4 cm downward from the pylorus) were collected immediately after the mice were euthanized. Blood was diluted with a PBS gradient immediately after collection. Each organ, including the small intestine, was homogenized with 1 mL of PBS and then diluted with a PBS gradient. The titers of bacteria and phage in blood and organ homogenates were determined using plate counting and the double plate method, respectively.
Statistical Analysis
All data were analyzed using GraphPad Prism 6. Statistical analysis of significance was also undertaken using the GraphPad Prism program via unpaired t test with a p < 0.05 considered significant.
Results
The Characteristics of K. oxytoca AD3 and Phage vB_Kox_ZX8
Klebsiella oxytoca AD3 was typed as ST367 and K1 serotype and encodes virulence factor genes rmpA, kfuBC, ybtA, fimH, uge, wabG, and wcaG. The strain is sensitive to most antibiotics but resistant to erythromycin, vancomycin, and tobramycin (Supplementary Table 2).
In this study, a phage named vB_Kox_ZX8 was isolated using K. oxytoca AD3. The phage formed small circular translucent plaques (diameter < 1 mm) on the lawns of the host (Figure 1A). Examination of phage morphology using TEM analysis showed that phage vB_Kox_ZX8 had an icosahedral head with a dimension of 53 ± 3.0 nm and a very short non-contractile tail (Figure 1B).
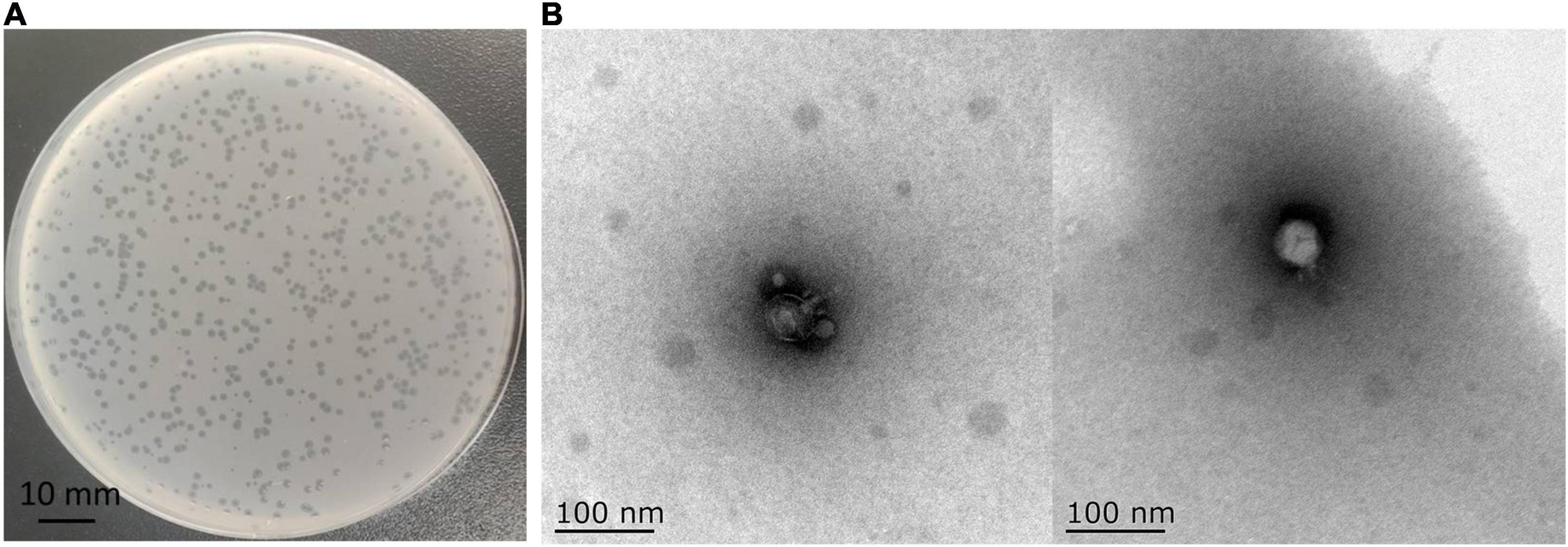
Figure 1. (A) Plaques formed by phage vB_Kox_ZX8 on a lawn of the host K. oxytoca AD3; (B) Transmission electron microscopy of phage vB_Kox_ZX8.
A total of 25 clinical isolates were used to evaluate the host range of vB_Kox_ZX8 (Supplementary Table 3). The results showed that phage vB_Kox_ZX8 only had lytic activity specific to K. oxytoca AD3. The narrow host spectrum of phages may be due to the limitations of the tested strains or the specificity of phage recognition sites.
One-Step Growth Curve and Stability Studies of Phage vB_Kox_ZX8
A one-step growth curve was used to analyze the adsorption velocity, latency period, and burst size of the phage (Figure 2A). The results showed that phage vB_Kox_ZX8 had a latency period of 10 min, followed by a rise period of 60 min and a growth plateau of approximately 50 min. The burst size of vB_Kox_ZX8 was computed as 74 phage particles per infected bacterium.
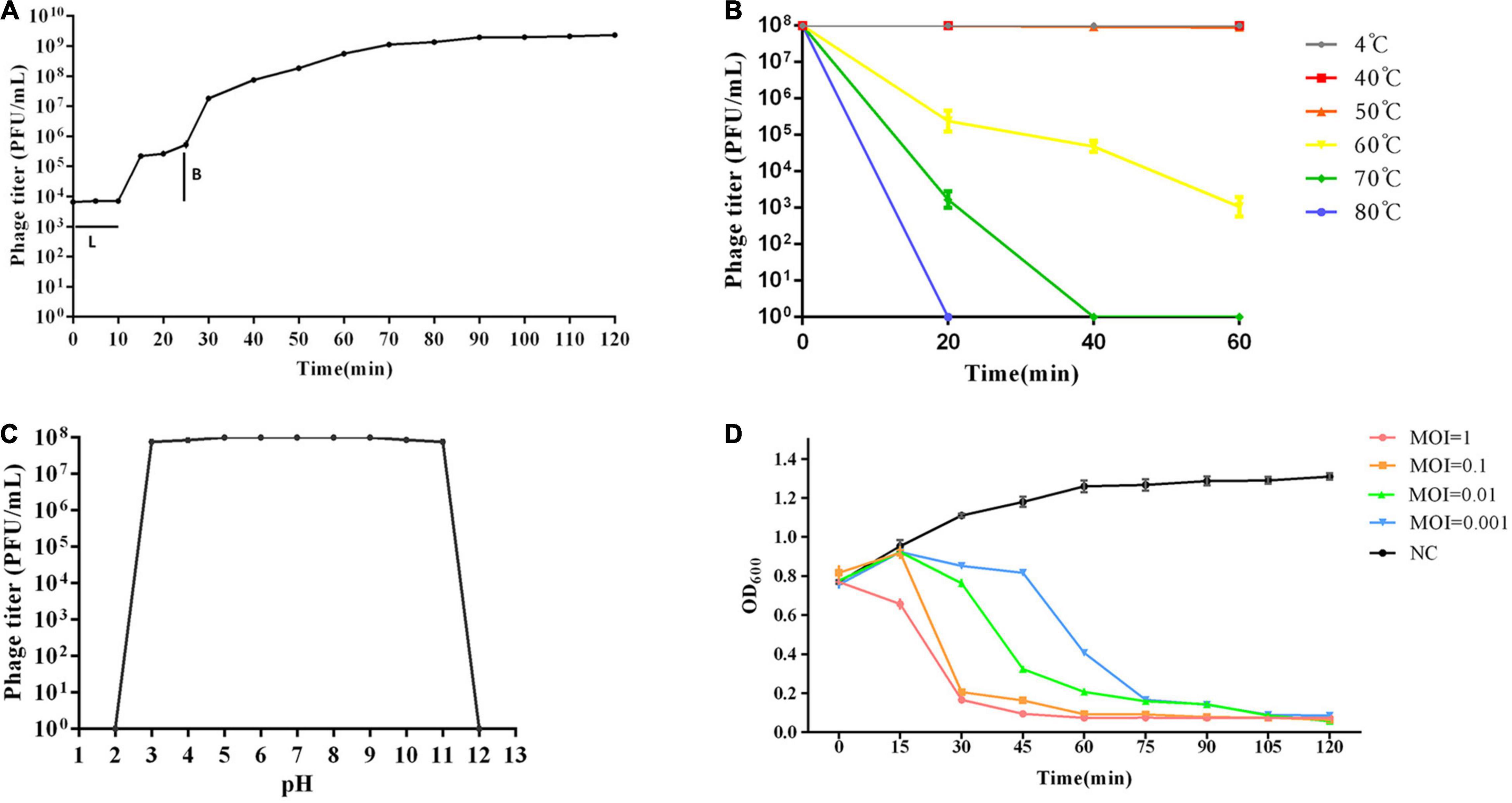
Figure 2. Biological characteristics of phage vB_Kox_ZX8. (A) One-step growth curve of phage vB_Kox_ZX8. L: latency period; B: burst size; (B) Thermal stability of phage vB_Kox_ZX8; (C) pH stability of phage vB_Kox_ZX8; (D) Killing dynamic of phage vB_Kox_ZX8 against K. oxytoca AD3. Viable cell count (OD600) of cultures infected with vB_Kox_ZX8 at MOI of 1, 0.1, 0.01, and 0.001, and the uninfected control (NC) are shown.
The thermal stability test showed that the phage was relatively stable between 4 and 50°C. After incubation at 60°C for 20, 40, and 60 min, the phage titer (108 PFU/mL) decreased to 2 × 105, 5 × 104, and 103 PFU/mL, respectively. After incubation at 70°C, phage activity decreased to 2 × 103 PFU/mL at 20 min and was completely lost at 40 min (Figure 2B). The pH stability test showed that phage activity was very stable at pH 3–11, and sharply lost at pH = 2 and pH = 12 (Figure 2C).
Killing Dynamic of Phage vB_Kox_ZX8 Against the K. oxytoca AD3 Strain
Different doses of phages (MOI = 10, 1, 0.1, 0.01, 0.001, respectively) were used to infect the host K. oxytoca AD3 in the log phase, and the OD600 of the culture was determined using a Smart Microplate Reader to evaluate the killing effect of phages on the host. The results showed that the number of bacteria decreased gradually after the addition of phages, and the rate of decrease was proportional to the number of phages. Even if the MOI of phages was 0.001, the bacteria were almost cleared after 90 min (Figure 2D). This result showed that phage vB_Kox_ZX8 had a good in vivo anti-K. oxytoca AD3 effect.
Genome Analysis of Phage vB_Kox_ZX8
The genome of phage vB_Kox_ZX8 (GenBank accession no. MZ424865) is a dsDNA that comprises 39,398 bp with a G+C content of 53.1%. tRNA was not predicted using tRNAscan-SE2.0. Virulence, toxin proteins, and drug-resistance genes were not detected. Whole-genome comparative analysis of phages showed that phage vB_Kox_ZX8 was most closely related to Klebsiella phage 2044-307w (95.05%), which is a member of the genus Przondovirus, subfamily Studiervirinae, family Autographiviridae.
The genome contains 46 coding sequences or open reading frames (ORFs) (Table 1). The ORFs of phage vB_Kox_ZX8 were divided into five modules: hypothetical protein, DNA metabolism, DNA packaging, phage structure, and phage lysis (Figure 3A). The main functional ORFs of phages are involved in DNA replication and degradation (ORF10, ORF11, ORF15, ORF17, ORF20, ORF21, ORF24, ORF26, ORF45, and ORF35); transcriptional factors (ORF13, ORF22, ORF30, and ORF31); phage capsid and scaffold (ORF3, ORF4, ORF5, and ORF6); phage tail (ORF1, ORF2, ORF7, ORF8, ORF41, ORF42, ORF43, and ORF44), phage assembly (ORF37 and ORF39), and phage lysis (ORF19, ORF38, and ORF40). ATG was proposed as a start codon for 41 ORFs, four ORFs used GTG, and one ORF used TTG. Signal peptides and transmembrane domains were found in seven ORFs: hypothetical protein ORF18, ORF29, ORF34, terminase large subunit ORF37, Rz-like lysis protein ORF38, holin class II ORF40, and internal virion protein B ORF44. The terminal large subunit and internal virion protein B are involved in the assembly of phage DNA. Holin forms pores on the inner membrane, and Rz-like lysis protein assists the lysozyme to enter the outer membrane from the inner membrane and cause complete cell lysis.
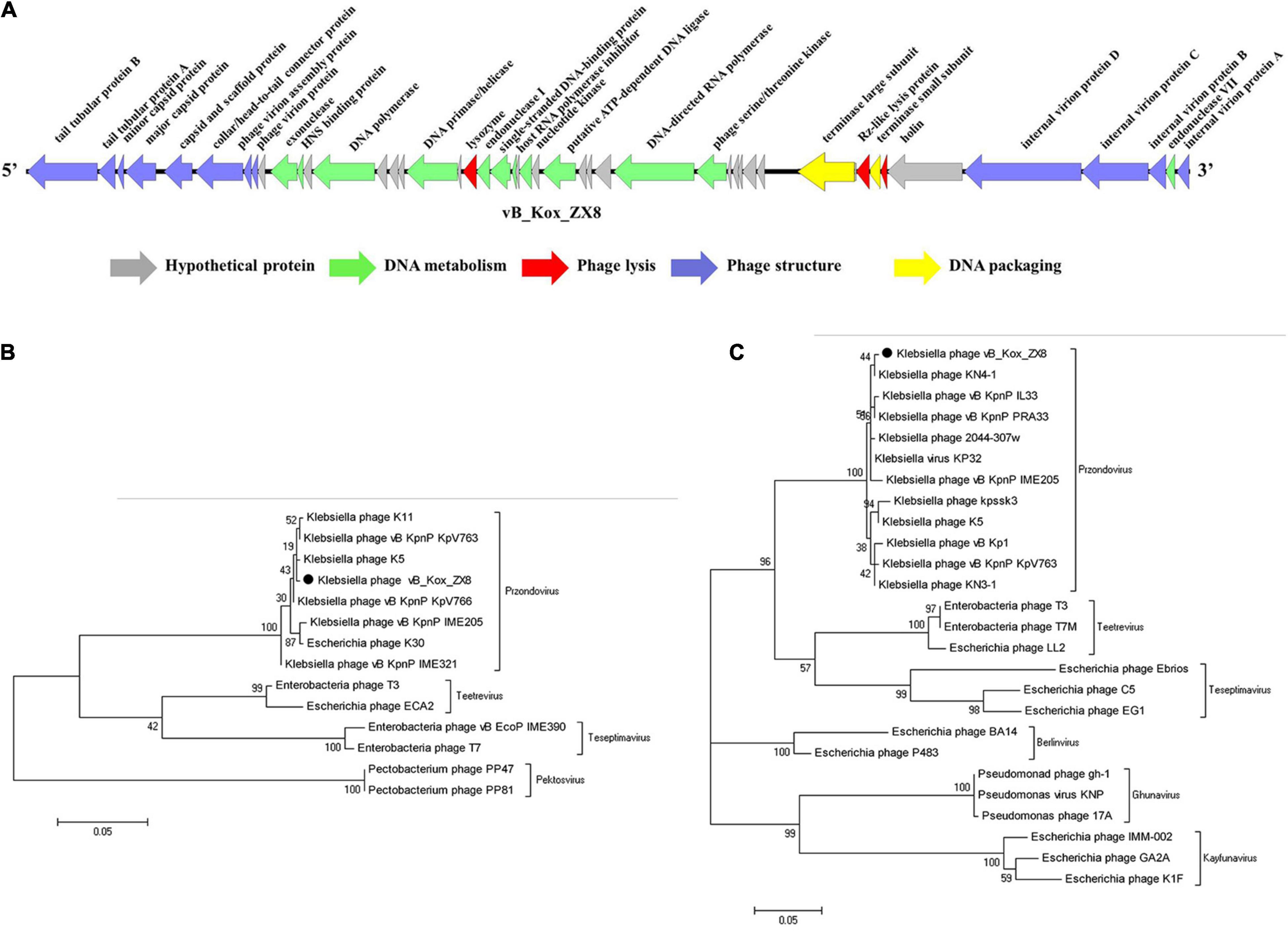
Figure 3. Genome analysis of phage vB_Kox_ZX8. (A) Linear genome map of phage vB_Kox_ZX8. Phylogenetic trees of the (B) terminase large subunit, and the (C) major capsid protein of phage vB_Kox_ZX8.
Phylogenetic analysis of the phage terminase large subunit and major capsid proteins showed that vB_Kox_ZX8 belonged to the genus Przondovirus, subfamily Studiervirinae, and family Autographiviridae (Figures 3B,C).
Effect of Treatment With Phage vB_Kox_ZX8 on Mice
Compared with the control group, phage vB_Kox_ZX8 had no significant effect on the weight of the mice (Figure 4A). In the inflammatory response, phage caused an increase in pro-inflammatory factors (IL-6 and TNF-α) and a decrease in anti-inflammatory factors (IL-10) (Figures 4B–D). The levels of IL-6, TNF-α, and IL-10 in the serum, liver, and spleen began to change after 12 h or 24 h of phage injection, and the changes caused by the high dose of phage (5 × 109 PFU) were more significant. The changes in inflammatory factors induced by phages showed a gradual upward trend within 48 h. At 48 h after administration of 5 × 109 PFU phage to healthy mice, the IL-6 levels in the serum, liver, and spleen of mice were 1.5, 1.6, and 2.0 times that of the control group, respectively; the levels of TNF-α in the serum, liver, and spleen of mice were 1.8, 1.7, and 1.7 times that of the control group, respectively; the IL-10 content in the serum, liver, and spleen of mice was 0.8, 0.8, and 0.9 times that of the control group, respectively. The fluctuation of IL-6, TNF-α, and IL-10 levels in mice caused by phage were slight, which is not enough to cause obvious adverse reactions.
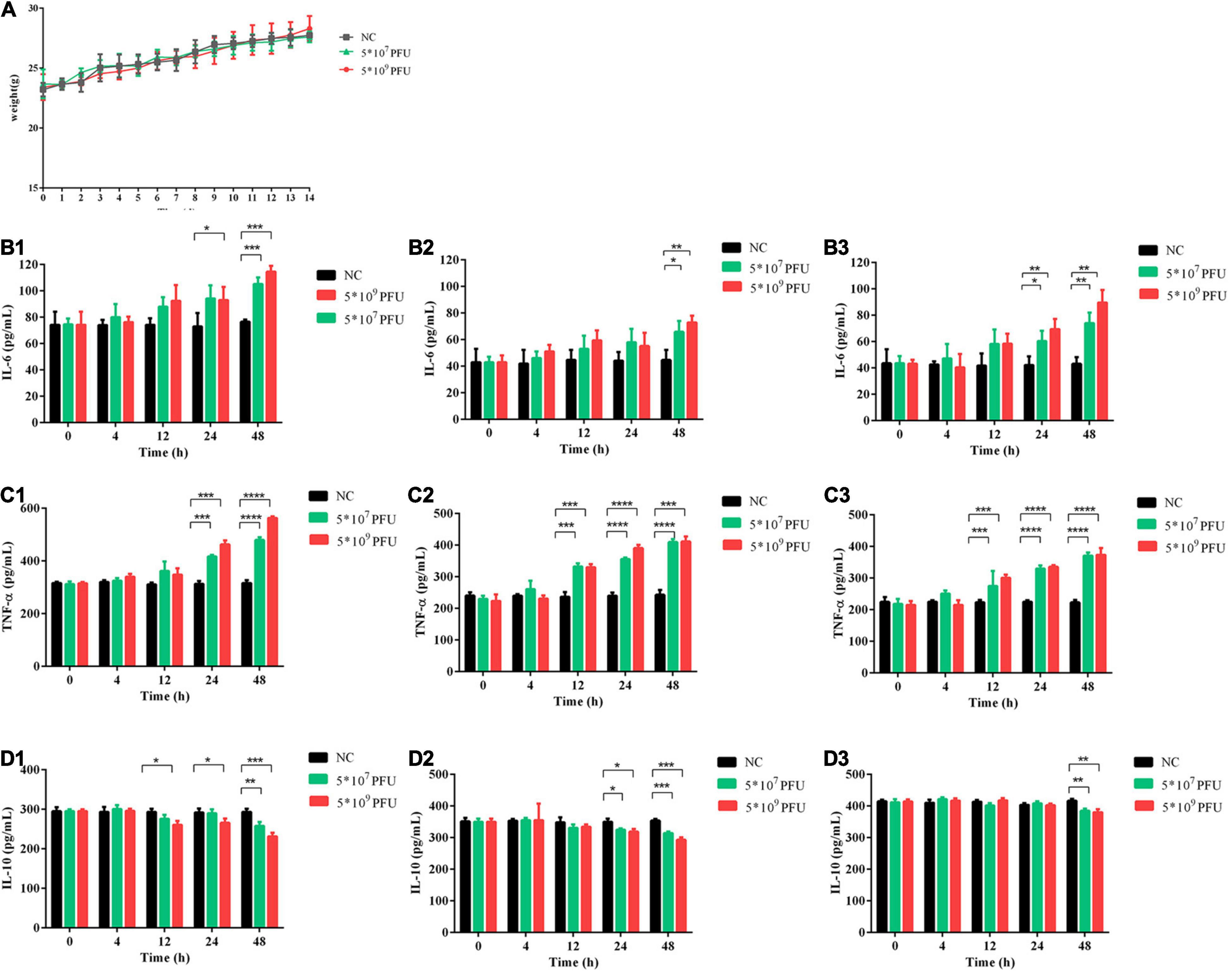
Figure 4. Safety evaluation of phage vB_Kox_ZX8 in mice. (A) The weight of mice after injection with phage vB_Kox_ZX8; IL-6 levels in the (B1) serum, (B2) liver, and (B3) spleen of mice after injection with phage vB_Kox_ZX8; TNF-α levels in the (C1) serum, (C2) liver, and (C3) spleen of mice after injection with phage vB_Kox_ZX8; IL-10 levels in the (D1) serum, (D2) liver, and (D3) spleen of mice after injection with phage vB_Kox_ZX8. Each mouse was injected with 5 × 107 PFU and 5 × 109 PFU of phage vB_Kox_ZX8, and the uninjected control (NC).
After intraperitoneal injection of 5 × 107 PFU and 5 × 109 PFU doses of phage vB_Kox_ZX8 to healthy mice, the phages disappeared in the blood after 3 and 5 h, respectively (Figure 5A). In mice injected IP with 5 × 109 PFU, the phage titer in the blood reached the highest (6 × 105 PFU/mL) at 30 min and lasted for 60 min, then dropped rapidly until it was completely undetectable. In mice injected IP with 5 × 107 PFU, the phage titer in the blood reached the highest (2 × 104 PFU/mL) at 15 min and lasted for 30 min, then dropped rapidly until it was completely undetectable. Phage vB_Kox_ZX8 had a higher titer and longer residence time in mouse organs because phages at 5 × 107 PFU and 5 × 109 PFU disappeared in organs within 24 and 48 h, respectively (Figures 5B,C). The titer of vB_Kox_ZX8 in the heart, thymus, lung, liver, spleen, kidney, and small intestine reached the highest levels within 1 h, and then gradually decreased. However, the titers of phages in the liver, spleen, kidney, and small intestine were significantly higher than those in the heart and lung after injection, which may be related to the injection method. At 12 h after 5 × 107 PFU phage injection, the phage titer of the thymus was the highest, followed by the spleen. At 24 h after 5 × 109 PFU phage injection, the phage titer of the spleen was the highest, followed by that of the thymus.

Figure 5. Metabolism in mice after injection with phage vB_Kox_ZX8. (A) Phage titer in the blood after mice were injected with 5 × 107 PFU and 5 × 109 PFU of phage vB_Kox_ZX8; phage titer in different organs after phage vB_Kox_ZX8 injection at (B) 5 × 107 PFU, (C) 5 × 109 PFU.
Mouse Model of Bacteremia
After the injection of K. oxytoca AD3, the mice were dispirited, and showed inverted hair, shivering, drowsiness, hunched back, weight loss, and death. Injection with a dose of 5 × 106 CFU, which is regarded as the MLD, caused the death of all six mice (Figure 6A).
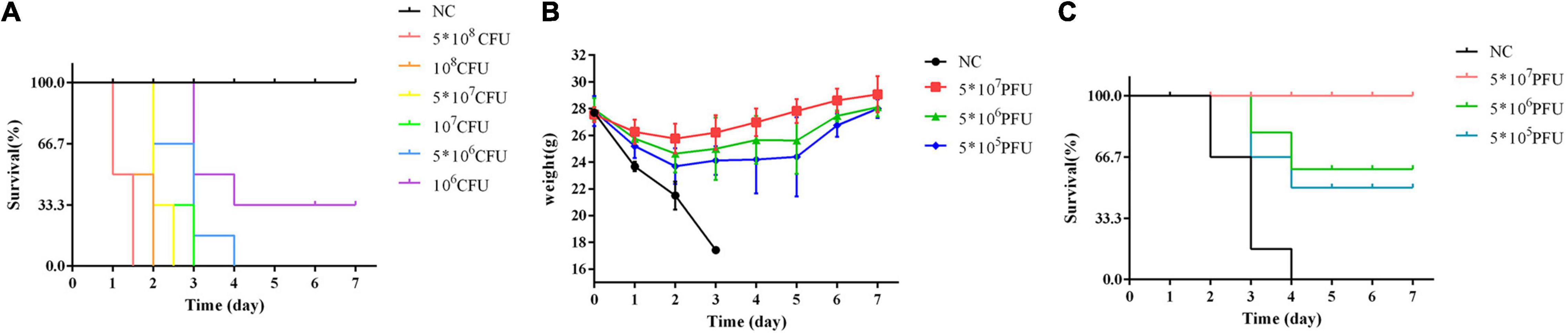
Figure 6. Therapy with phage vB_Kox_ZX8 rescues bacteremia mice. (A) The survival rate of mice infected with K. oxytoca AD3 and uninfected control (NC); (B) The weight and (C) Survival rate of bacteremia mice rescued by phage vB_Kox_ZX8 and unrescued control (NC).
Therapy With Single Phage Rescues Bacteremia Mice
The weight of bacteremic mice rescued by phage vB_Kox_ZX8 increased gradually after 2 days (Figure 6B). The survival rate of mice rescued with 5 × 107 PFU of phage reached 100%, and mice rescued with 5 × 106 PFU and 5 × 105 PFU of phage were 66% and 50% of survival rate, respectively (Figure 6C).
Therefore, 5 × 107 PFU phages were selected to rescue bacteremia mice, and the therapeutic effect of the phage was evaluated by measuring the titer of bacteria and phage in mice. The results showed that the bacterial titers in the blood and organs of mice treated with phage decreased gradually and were cleared after 48 h, while the bacterial titers in the blood and organs of mice in the negative control group increased and killed the mice (Figures 7A,B). After the phage entered the body of the mouse, it was first reproduced through K. oxytoca AD3, then gradually decreased, and was completely metabolized after 48 h in the blood (Figure 7C). The changes in the number of phages in the organs seem to be related to the bacteria count, and they were cleared after 48 h (Figure 7D). In organs, the bacteria count was decreased by the phages in a short time (<6 h), followed a slight upward trend, and finally cleared after 48 h. Bacteria isolated from the organs and blood were still sensitive to phage vB_Kox_ZX8 at 12, 24, and 36 h post-infection, indicating that bacteria and phages co-exist between 12 and 36 h in mice. However, the phage-resistant strains were isolated after 12 h of coculture in vitro. The reason for this phenomenon may be that phages in mice have less selective pressure on bacteria, and a higher phage therapeutic dose should be considered.
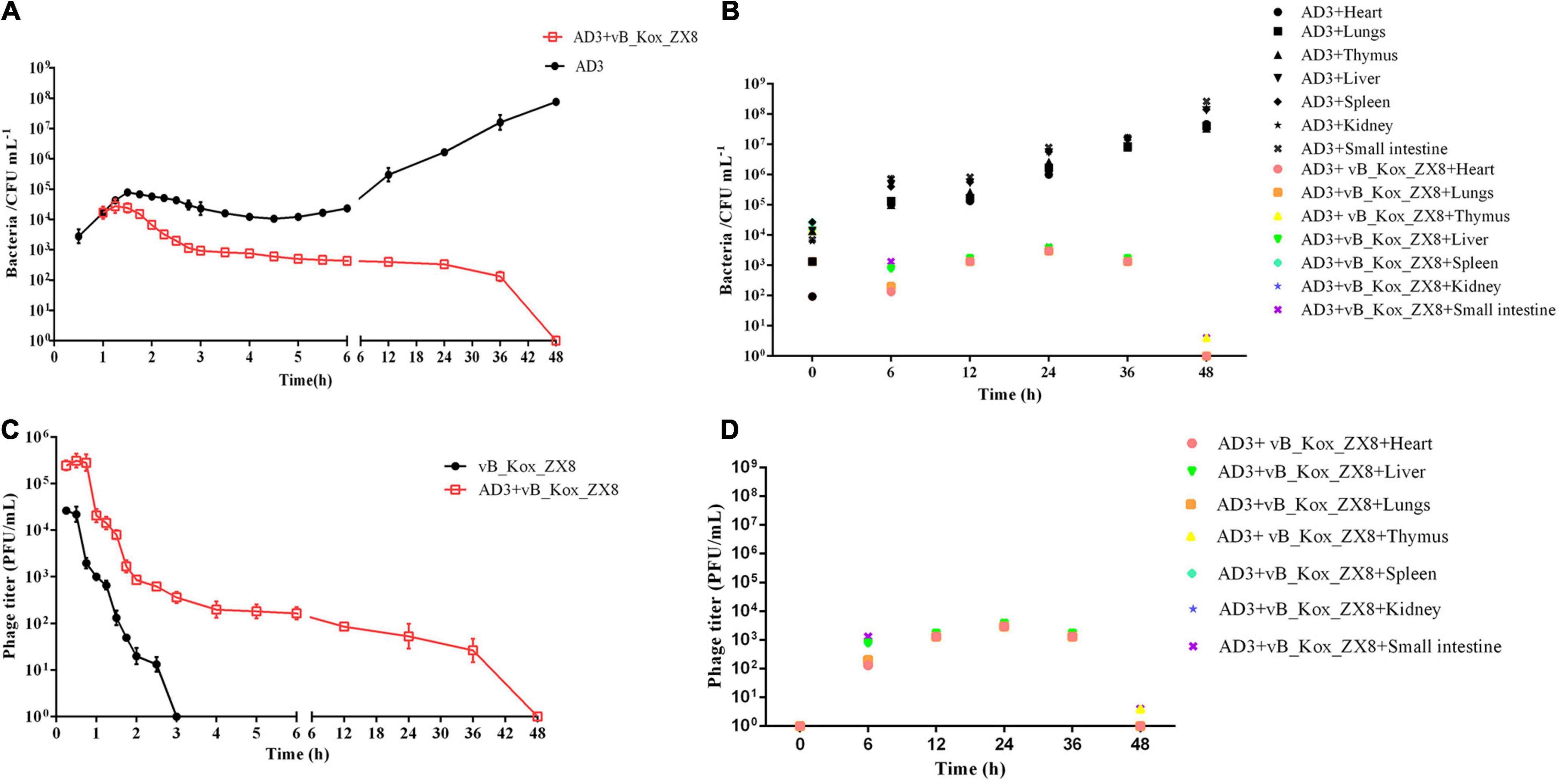
Figure 7. Changes in bacterial and phage titers in bacteremia mice after phage vB_Kox_ZX8 rescue. Bacteria titer in the (A) blood and (B) different organs of bacteremia mice rescued by phage vB_Kox_ZX8; phage titer in the (C) blood and (D) different organs of bacteremia mice rescued by phage vB_Kox_ZX8.
After 48 h of infection with K. oxytoca AD3, the levels of IL-6 and TNF-α in the thymus, lung, spleen, kidney, and liver of mice increased significantly, while IL-10 decreased significantly, which was effectively alleviated via phage therapy (Figures 8A–C). K. oxytoca AD3 had the strongest pathogenicity in the small intestine, with obvious pathological changes, hyperemia, cell necrosis, and fuzzy structure (Figure 9A). The number of inflammatory cells in the thymus and spleen decreased significantly and inflammatory cell infiltration was observed in the liver (Figures 9B–D). No obvious pathological changes were observed in the kidneys and lungs (Figures 9E,F). Notably, the pathological changes in various organs in mice rescued by phage showed significant improvement.

Figure 8. Changes in immune factors in mice after phage vB_Kox_ZX8 rescue. (A) IL-6, (B) TNF-α, and (C) IL-10 levels in mice serum after injection with 5 × 106 CFU of K. oxytoca AD3 and 5 × 107 PFU of phage vB_Kox_ZX8, and the uninjected control (NC) are shown.
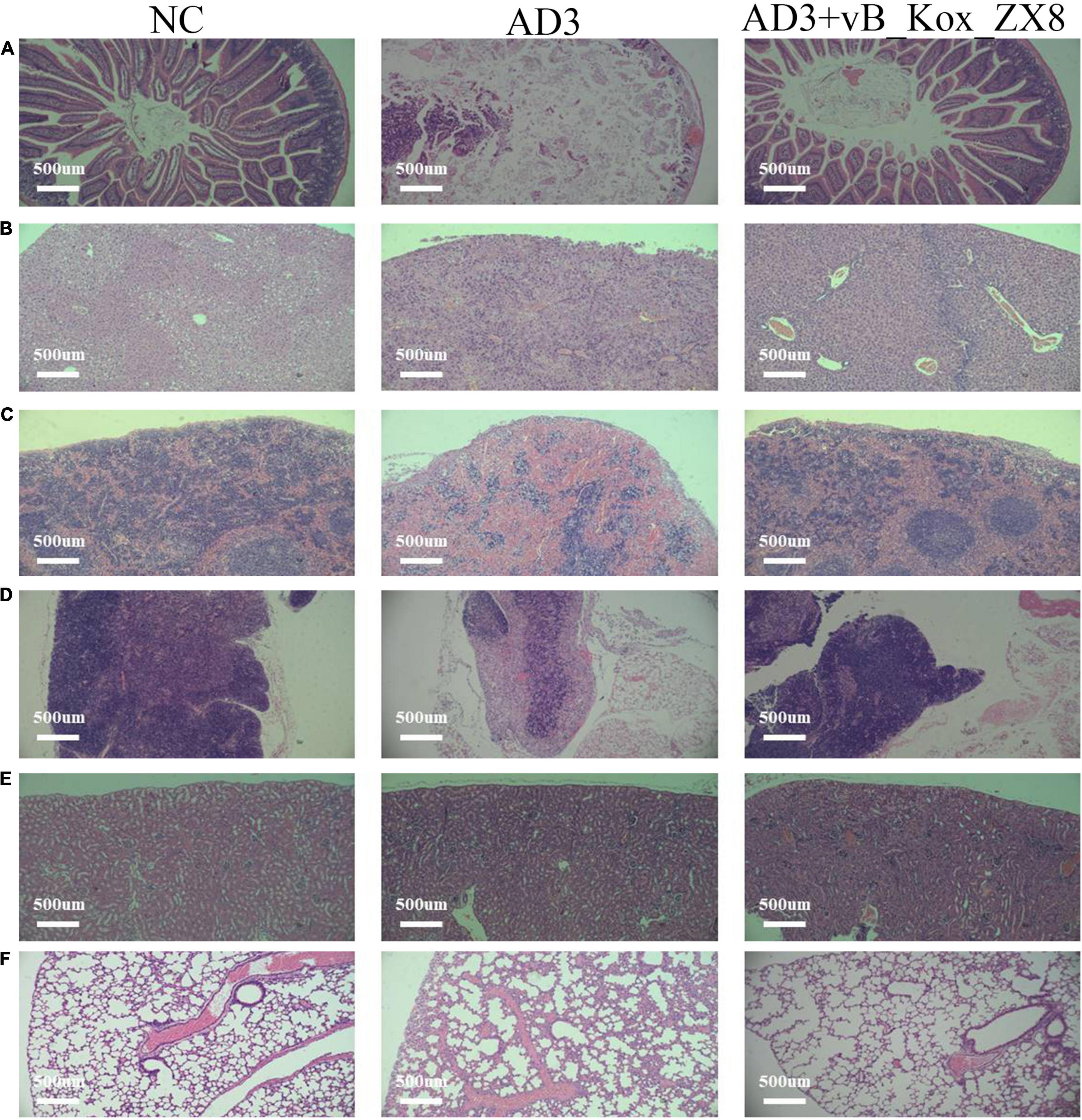
Figure 9. Pathological sections of the (A) small intestine, (B) liver, (C) spleen, (D) thymus, (E) kidney, and (F) lung in mice after 48 h rescued by phage vB_Kox_ZX8. Each mouse was injected with 5 × 106 CFU of K. oxytoca AD3 and 5 × 107 PFU of phage vB_Kox_ZX8, and the uninjected control (NC).
Discussion
The global trend of antibiotic resistance is an urgent problem that needs to be addressed. Based on horizontal gene transfer, some infections caused by pandrug-resistant bacteria cannot be cured with all available antibiotics (Magiorakos et al., 2012). Owing to the high pathogenicity and mortality of multidrug-resistant bacteria, new and effective treatments need to be developed. Phage is a good alternative to antibiotics because of its fast bactericidal effect, high specificity, and low cost. The rapid bactericidal effect of phages has been widely reported in animal models, such as mice, chickens, and cattle. For example, phages are used to treat pneumonia caused by K. pneumoniae, enteritis caused by Salmonella enteritidis and bovine mastitis caused by Staphylococcus aureus (Lim et al., 2012; Anand et al., 2020; Titze and Krömker, 2020). Successful cases of phage therapy in the clinic also show its rapidity and effectiveness, such as phages for prosthetic joint infections caused by Pseudomonas aeruginosa or K. pneumoniae (Cano et al., 2021; Ferry et al., 2021). At present, phages can be used for treatment only when patients are infected with multidrug-resistant bacteria or those in the antibiotic crisis of incurable infection in clinical practice (Lin et al., 2017). Moreover, some studies have shown that phages are beneficial for reducing the occurrence of antibiotic resistance. For example, the introduction of phages drives bacteria to expel multidrug resistance clusters or decrease antibiotic susceptibilities in antibiotic resistance-related gene expression (Uddin et al., 2019; Majkowska-Skrobek et al., 2021). Phage therapy has great potential applications, but phage resistance and phage pharmacodynamic and pharmacokinetic obstacles remain important challenges (Dąbrowska, 2019; Dąbrowska and Abedon, 2019; Pires et al., 2020).
In this study, Klebsiella phage vB_Kox_ZX8 was isolated from human feces, which has a narrow host spectrum. Generally, the tail fiber protein of phages is involved in the adsorption of phages and determines the host range (Bertozzi Silva et al., 2016). BLASTx analysis of phage tail fiber protein showed that two putative conserved domains were detected. The N-terminal of the tail fiber protein (1–154 amino acids) has high homology with T7-like phage tail fiber proteins, such as Klebsiella phage K5-4, Klebsiella phage SH-KP152226, and Klebsiella virus KP32, which have wide host ranges because they encode depolymerase (Hsieh et al., 2017; Pyra et al., 2017; Wu et al., 2019). The C-terminal of the tail fiber protein (amino acids 373–538) had high homology with the lysophospholipase L1-like subgroup of SGNH-hydrolases, which had low homology with other phage proteins. The characteristics of tail fiber proteins suggest that phage vB_Kox_ZX8 may have special recognition sites on the host. Phage vB_Kox_ZX8 has a latent period of less than 10 min, a medium-sized burst of 74 pfu/cell and is stable at pH (3–11) and temperature (4–50 °C). In LB medium, the phage with MOI = 1 could reduce the OD600 of K. oxytoca AD3 from 0.8 to 0.1 within 45 min. Among the previously reported Podoviridae phages, the incubation period was 10–40 min, the burst size was 120–200 pfu/cell, and pH stability was 4–11 (Manohar et al., 2019; Shi et al., 2020; Sofy et al., 2021). In this study, the short incubation period, wide pH stability, and special host indicate that phage vB_Kox_ZX8 can be considered as a component of the phage cocktail for treatment.
The current consensus is that phage therapy is safe on the premise that phage preparations are fully purified to ensure low endotoxin levels and remove other bacterial impurities (Speck and Smithyman, 2016; Wienhold et al., 2019). Many studies have reported that phage preparation does not cause changes in inflammatory factors in animals. For example, phage D29 administered via the endotracheal route did not cause significant changes in leukocytes, neutrophils, lymphocytes, and TNF-α levels in the lungs of healthy mice 24 h after treatment (Liu et al., 2016); phage BcepIL02 administered via IP injection did not cause significant changes in TNF-α levels in the lungs of healthy mice 24 h after treatment (Carmody et al., 2010); and phage Kp_Pokalde_002 administered via IP injection did not cause significant changes in TNF-α and IL-6 levels in the plasma of healthy mice 24 h after treatment (Dhungana et al., 2021). In this study, administration of high-dose phage vB_Kox_ZX8 caused slight changes in TNF-α, IL-6, and IL-10 in the serum, liver, and spleen of mice after 24 h of treatment, but did not induce discomfort in mice. This means that the purified phage preparation may contain a small amount of endotoxin and bacterial protein or nucleic acid, which can induce pro-inflammatory reactions in mice. After administration of high-dose PEV31 phage, TNF-α in mice was transiently upregulated at 4 h, and returned to the baseline level after 24 h (Chow et al., 2020). The level of phage preparation-induced inflammation may be temporary and will decrease over time. In addition, it has been reported that some phages can trigger endotoxin-independent inflammatory and anti-inflammatory responses, thereby reducing bacterial clearance to promote phage reproduction (Van Belleghem et al., 2017).
After 5 × 109 PFU of phage vB_Kox_ZX8 was IP injected into healthy mice, the phage titer in the blood was detected within 10 min, maintained at a high level at 30–90 min, gradually decreased, and disappeared after 6 h. In a study of Podoviridae phage kpssk3, which has 81% sequence similarity to vB_Kox_ZX8, Klebsiella phage kpssk3 showed a similar short residence time in mouse blood. After intraperitoneal injection of 108 PFU phage kpssk3 for 15 min, the blood titer of phages reached 105 PFU/mL, gradually decreasing after maintaining the high titer state for 4 h, and disappeared completely after 8 h (Shi et al., 2021). In other studies of the Podoviridae phage, Klebsiella phage Kp_Pokalde_002 and Pseudomonas phage PEV20 had a longer residence time in mouse blood. The titer of Kp_Pokalde_002 in the blood measured via IP injection of 108 PFU reached the maximum at the fourth hour, gradually decreased, and cleared at 48 h (Dhungana et al., 2021). The titer of PEV20 in rat blood measured via intravenous injection of 108 PFU reached the maximum within 1 h, gradually decreased, and cleared at 48 h (Lin et al., 2020). In previous reports, active phages were detected in the circulation within the first hour (even less than 5 min) (Bogovazova et al., 1991, 1992; Chhibber et al., 2008). The clearance time of phages in the blood seems to depend on the dose and size of phages, but there is still a knowledge gap between similar phages. In clinical practice, the short life span of phages in the blood is considered an unfavorable factor for phage therapy (Barr, 2017). Some studies have shown that the encapsulation of phages in microparticles and nanoparticles is not only conducive to the storage of phage preparations, but also prolongs the action time of phages in vivo (Singla et al., 2016; Malik et al., 2017).
Phages vB_Kox_ZX8 have a longer residence time in the organs than in the blood, and the residence time of phages injected IP at 5 × 109 PFU into the blood (6 h) and organs (48 h) was significantly different. It has been reported that the phage can enter the organ from the blood within a few minutes after administration, and the phage titer in the organ is higher than that in the blood within the first 3 h (Cerveny et al., 2002; Tiwari et al., 2011; Trigo et al., 2013). The acquisition of phages by these organs is usually regarded as a form of phage clearance from the blood, rather than phage distribution. The highest phage titer in mice was lower than the actual injection dose (including vB_Kox_ZX8), indicating that the phage was rapidly captured and neutralized after entering mice; for example, by the mononuclear phagocyte system. The liver and spleen contain many phagocyte precipitates, which are considered to be the main organs for phage clearance from animals and humans, and actively participate in phage neutralization (Lin et al., 2017; Dąbrowska and Abedon, 2019). During the metabolism of phage vB_Kox_ZX8, the spleen accumulated more phages than the liver, which may be because the spleen is more effective in filtering phage vB_Kox_ZX8. A circulating study of intravenous T4 phage in a mouse model showed that the liver has a higher accumulation concentration and faster elimination time for phage, which proves that Kupffer cells in the liver are very effective for the rapid removal of phage particles (Inchley, 1969; Kaźmierczak et al., 2021). The thymus also has high aggregation of phage vB_Kox_ZX8, which has rarely been mentioned in previous studies. The thymus, an important immune organ, may play an important role in phage clearance. According to the literature, some phages can enter most organs in the body including the bones, bladder, skin, salivary glands, and brain, from the blood (Nishikawa et al., 2008; Pouillot et al., 2012; Dąbrowska and Abedon, 2019). The high titer of phage vB_Kox_ZX8 in the small intestine indicates that vB_Kox_ZX8 can easily cross the intestinal barrier and enter the intestine from the blood. Reverse osmosis of phages from the blood to the gastrointestinal tract does not exist in all phages. In previous reports, phages were detected in the feces of calves and mice, the intestines of mice, rabbits, and chickens, and the stomachs of mice (Dąbrowska, 2019).
Phages administered via the IP route have the characteristics of higher dose delivery, earlier delivery time, and longer maintenance time, which is correlated with more effective protection of experimental animals from lethal septicemia (McVay et al., 2007). In mice bacteremia induced with K. oxytoca AD3, phage vB_Kox_ZX8 showed good therapeutic potential via IP injection. The bacterial titers in the blood and organs of mice in the phage-treated group decreased gradually and were cleared after 48 h. The survival rate of the treated group was 100%, while the untreated mice all died, which undoubtedly proved to have a good therapeutic effect. Some studies have shown that a single phage preparation can have a good therapeutic effect in the treatment of mouse bacteremia (Wang et al., 2006, 2018; Vinodkumar et al., 2008; Hung et al., 2011; Alvi et al., 2020). Some of these phages have better therapeutic effects than antibiotics (Sunagar et al., 2010). Recent studies have demonstrated that the combination of phage and antibiotics is more effective than monotherapy in the treatment of bacterial infections (Oechslin et al., 2017; Wang et al., 2021). In the synergistic therapy of phages and antibiotics, phage selection pressure can make bacteria sensitive to antibiotics (Segall et al., 2019). The determination of the type and dose of antibiotics, optimization of combination therapy, and prevention of side effects are very important. Generally, a single phage can effectively reduce the number of bacteria in a short time; however, it is easy to produce phage-resistant bacteria in the later stages of treatment (Hung et al., 2011; Hesse et al., 2021). Phage cocktail has a wider bactericidal range and a lower probability of phage resistant strains, and seems to better protect mice from death caused by bacteremia (Forti et al., 2018; Kaabi and Musafer, 2019). However, the competitive interference between different phages may affect the effectiveness of the phage mixture; therefore, it is necessary to confirm the effectiveness of the phage mixture on a single phage (Geng et al., 2020).
Although most of the phages that have presented good therapeutic effects were isolated from sewage, some lytic phages isolated from the intestine showed high bactericidal ability. Myoviridae phage ΦAPCEc01, ΦAPCEc02, and Siphoviridae ΦAPCEc03 were isolated from human feces samples, which can inhibit the growth of E. coli and reduce the formation of biofilm (Dalmasso et al., 2016). The Siphoviridae phage KLPN1 was isolated from a cecal effluent sample, which can lyse K. pneumoniae and has depolymerase activity (Hoyles et al., 2015). The Podoviridae Proteus phage PM16 was isolated from human feces and has characteristics of high stability, a short latency period, large burst size, and low phage resistance (Morozova et al., 2016).
In summary, a novel phage named vB_Kox_ZX8 that specifically infects K. oxytoca AD3 was isolated, and this is the first report of K. oxytoca phage obtained from a clinical fecal sample. The biological characteristics and rescue experiments in bacteremic mice showed that phage vB_Kox_ZX8 has the potential to be an excellent reagent for infection caused by K. oxytoca in the clinic.
Data Availability Statement
The datasets presented in this study can be found in online repositories. The names of the repository/repositories and accession number(s) can be found in the article/Supplementary Material.
Ethics Statement
The studies involving human participants were reviewed and approved by Nanjing Stomatological Hospital Medical School of Nanjing University. Written informed consent for participation was not required for this study in accordance with the national legislation and the institutional requirements. The animal study was reviewed and approved by Experimental Animal Ethics Committee of Yangzhou University.
Author Contributions
XZ and FY supervised the project, analyzed the data, and revised the manuscript. PL and YZ performed the experiments, drew the figures, and wrote the draft manuscript. All authors contributed to the final version of this manuscript.
Funding
This study was supported by the National Natural Science Foundation of China (No. 31870989), Nanjing Clinical Research Center for Oral Disease (No. 2019060009), the Natural Science Foundation of Jiangsu Province (No. BK20190133), the start-up fund provided by Yangzhou University (Nos. 5020/137011016 and 5020/137011618), the Jiangsu Graduate Research and Practice Innovation Program (No. KYCX21_3280), and the Priority Academic Program Development of Jiangsu Higher Education Institutions (PAPD).
Conflict of Interest
The authors declare that the research was conducted in the absence of any commercial or financial relationships that could be construed as a potential conflict of interest.
Publisher’s Note
All claims expressed in this article are solely those of the authors and do not necessarily represent those of their affiliated organizations, or those of the publisher, the editors and the reviewers. Any product that may be evaluated in this article, or claim that may be made by its manufacturer, is not guaranteed or endorsed by the publisher.
Supplementary Material
The Supplementary Material for this article can be found online at: https://www.frontiersin.org/articles/10.3389/fmicb.2021.763136/full#supplementary-material
Footnotes
- ^ https://bigsdb.pasteur.fr/klebsiella/klebsiella.html
- ^ http://lowelab.ucsc.edu/tRNAscan-SE/
- ^ http://www.mgc.ac.cn/VFs/main.htm
- ^ https://card.mcmaster.ca/
References
Ahmad, N., Khalid, S., Ali, S. M., and Khan, A. U. (2018). Occurrence of bla(NDM) variants among Enterobacteriaceae from a neonatal intensive care unit in a Northern India Hospital. Front. microbiol. 9:407. doi: 10.3389/fmicb.2018.00407
Alvi, I. A., Asif, M., Tabassum, R., Aslam, R., Abbas, Z., and Rehman, S. U. (2020). RLP, a bacteriophage of the family Podoviridae, rescues mice from bacteremia caused by multi-drug-resistant Pseudomonas aeruginosa. Arch. Virol. 165, 1289–1297. doi: 10.1007/s00705-020-04601-x
Amiri Fahliyani, S., Beheshti-Maal, K., and Ghandehari, F. (2018). Novel lytic bacteriophages of Klebsiella oxytoca ABG-IAUF-1 as the potential agents for mastitis phage therapy. FEMS Microbiol. Lett. 365:fny223. doi: 10.1093/femsle/fny223
Anand, T., Virmani, N., Kumar, S., Mohanty, A. K., Pavulraj, S., Bera, B. C., et al. (2020). Phage therapy for treatment of virulent Klebsiella pneumoniae infection in a mouse model. J. Glob. Antimicrob. Resist. 21, 34–41. doi: 10.1016/j.jgar.2019.09.018
Aziz, R. K., Bartels, D., Best, A. A., DeJongh, M., Disz, T., Edwards, R. A., et al. (2008). The RAST Server: rapid annotations using subsystems technology. BMC Genomics 9:75. doi: 10.1186/1471-2164-9-75
Barr, J. J. (2017). A bacteriophages journey through the human body. Immunol. Rev. 279, 106–122. doi: 10.1111/imr.12565
Beaugerie, L., Metz, M., Barbut, F., Bellaiche, G., Bouhnik, Y., Raskine, L., et al. (2003). Klebsiella oxytoca as an agent of antibiotic-associated hemorrhagic colitis. Clin. Gastroenterol. Hepatol. 1, 370–376. doi: 10.1053/s1542-3565(03)00183-6
Bertozzi Silva, J., Storms, Z., and Sauvageau, D. (2016). Host receptors for bacteriophage adsorption. FEMS Microbiol. Lett. 363:fnw002. doi: 10.1093/femsle/fnw002
Bogovazova, G. G., Voroshilova, N. N., and Bondarenko, V. M. (1991). [The efficacy of Klebsiella pneumoniae bacteriophage in the therapy of experimental Klebsiella infection]. Zh. Mikrobiol. Epidemiol. Immunobiol. 4, 5–8.
Bogovazova, G. G., Voroshilova, N. N., Bondarenko, V. M., Gorbatkova, G. A., Afanas’eva, E. V., Kazakova, T. B., et al. (1992). [Immunobiological properties and therapeutic effectiveness of preparations from Klebsiella bacteriophages]. Zh. Mikrobiol. Epidemiol. Immunobiol. 3, 30–33.
Brown, T. L., Petrovski, S., Hoyle, D., Chan, H. T., Lock, P., and Tucci, J. (2017). Characterization and formulation into solid dosage forms of a novel bacteriophage lytic against Klebsiella oxytoca. PLoS One 12:e0183510. doi: 10.1371/journal.pone.0183510
Cano, E. J., Caflisch, K. M., Bollyky, P. L., Van Belleghem, J. D., Patel, R., Fackler, J., et al. (2021). Phage therapy for limb-threatening prosthetic knee Klebsiella pneumoniae infection: case report and in vitro characterization of anti-biofilm activity. Clin. Infect. Dis. 73, e144–e151. doi: 10.1093/cid/ciaa705
Carmody, L. A., Gill, J. J., Summer, E. J., Sajjan, U. S., Gonzalez, C. F., Young, R. F., et al. (2010). Efficacy of bacteriophage therapy in a model of Burkholderia cenocepacia pulmonary infection. J. Infect. Dis. 201, 264–271. doi: 10.1086/649227
Cerveny, K. E., DePaola, A., Duckworth, D. H., and Gulig, P. A. (2002). Phage therapy of local and systemic disease caused by Vibrio vulnificus in iron-dextran-treated mice. Infect. Immun. 70, 6251–6262. doi: 10.1128/iai.70.11.6251-6262.2002
Chadha, P., Katare, O. P., and Chhibber, S. (2017). Liposome loaded phage cocktail: enhanced therapeutic potential in resolving Klebsiella pneumoniae mediated burn wound infections. Burns 43, 1532–1543. doi: 10.1016/j.burns.2017.03.029
Chhibber, S., Kaur, S., and Kumari, S. (2008). Therapeutic potential of bacteriophage in treating Klebsiella pneumoniae B5055-mediated lobar pneumonia in mice. J. Med. Microbiol. 57, 1508–1513. doi: 10.1099/jmm.0.2008/002873-0
Chow, M. Y. T., Chang, R. Y. K., Li, M., Wang, Y., Lin, Y., Morales, S., et al. (2020). Pharmacokinetics and time-kill study of inhaled antipseudomonal bacteriophage therapy in mice. Antimicrob. Agents Chemother. 65:e01470-20. doi: 10.1128/aac.01470-20
Ciacci, N., D’Andrea, M. M., Marmo, P., Demattè, E., Amisano, F., Di Pilato, V., et al. (2018). Characterization of vB_Kpn_F48, a newly discovered lytic bacteriophage for Klebsiella pneumoniae of sequence type 101. Viruses 10:482. doi: 10.3390/v10090482
Dąbrowska, K. (2019). Phage therapy: what factors shape phage pharmacokinetics and bioavailability? Systematic and critical review. Med. Res. Rev. 39, 2000–2025. doi: 10.1002/med.21572
Dąbrowska, K., and Abedon, S. T. (2019). Pharmacologically aware phage therapy: pharmacodynamic and pharmacokinetic obstacles to phage antibacterial action in animal and human bodies. Microbiol. Mol. Biol. Rev. 83:e00012-19. doi: 10.1128/mmbr.00012-19
Dalmasso, M., Hill, C., and Ross, R. P. (2014). Exploiting gut bacteriophages for human health. Trends Microbiol. 22, 399–405. doi: 10.1016/j.tim.2014.02.010
Dalmasso, M., Strain, R., Neve, H., Franz, C. M., Cousin, F. J., Ross, R. P., et al. (2016). Three new Escherichia coli phages from the human gut show promising potential for phage therapy. PLoS One 11:e0156773. doi: 10.1371/journal.pone.0156773
Dhungana, G., Nepal, R., Regmi, M., and Malla, R. (2021). Pharmacokinetics and pharmacodynamics of a novel virulent Klebsiella phage Kp_Pokalde_002 in a mouse model. Front. Cell. Infect. Microbiol. 11:684704. doi: 10.3389/fcimb.2021.684704
Díaz-Muñoz, S. L., and Koskella, B. (2014). Bacteria-phage interactions in natural environments. Adv. Appl. Microbiol. 89, 135–183. doi: 10.1016/b978-0-12-800259-9.00004-4
Egger, M., Kurath, S., Strenger, V., Grisold, A., Schlenke, P., Rosskopf, K., et al. (2017). Klebsiella oxytoca bacteremia induced septic shock following platelet transfusion. Klin. Padiatr. 229, 304–305. doi: 10.1055/s-0043-116850
Ferry, T., Kolenda, C., Batailler, C., Gaillard, R., Gustave, C. A., Lustig, S., et al. (2021). Case report: arthroscopic “debridement antibiotics and implant retention” with local injection of personalized phage therapy to salvage a relapsing Pseudomonas aeruginosa prosthetic knee infection. Front. Med. (Lausanne) 8:569159. doi: 10.3389/fmed.2021.569159
Forti, F., Roach, D. R., Cafora, M., Pasini, M. E., Horner, D. S., Fiscarelli, E. V., et al. (2018). Design of a broad-range bacteriophage cocktail that reduces Pseudomonas aeruginosa biofilms and treats acute infections in two animal models. Antimicrob. Agents Chemother. 62:e02573-17. doi: 10.1128/aac.02573-17
Gangwar, M., Rastogi, S., Singh, D., Shukla, A., Dhameja, N., Kumar, D., et al. (2021). Study on the effect of oral administration of bacteriophages in charles foster rats with special reference to immunological and adverse effects. Front. Pharmacol. 12:615445. doi: 10.3389/fphar.2021.615445
Geng, H., Zou, W., Zhang, M., Xu, L., Liu, F., Li, X., et al. (2020). Evaluation of phage therapy in the treatment of Staphylococcus aureus-induced mastitis in mice. Folia Microbiol. 65, 339–351. doi: 10.1007/s12223-019-00729-9
Hansen, D. S., Gottschau, A., and Kolmos, H. J. (1998). Epidemiology of Klebsiella bacteraemia: a case control study using Escherichia coli bacteraemia as control. J. Hosp. Infect. 38, 119–132. doi: 10.1016/s0195-6701(98)90065-2
Hesse, S., Malachowa, N., Porter, A. R., Freedman, B., Kobayashi, S. D., Gardner, D. J., et al. (2021). Bacteriophage treatment rescues mice infected with multidrug-resistant Klebsiella pneumoniae ST258. mBio 12:e00034-21. doi: 10.1128/mBio.00034-21
Hoyles, L., McCartney, A. L., Neve, H., Gibson, G. R., Sanderson, J. D., Heller, K. J., et al. (2014). Characterization of virus-like particles associated with the human faecal and caecal microbiota. Res. Microbiol. 165, 803–812. doi: 10.1016/j.resmic.2014.10.006
Hoyles, L., Murphy, J., Neve, H., Heller, K. J., Turton, J. F., Mahony, J., et al. (2015). Klebsiella pneumoniae subsp. pneumoniae-bacteriophage combination from the caecal effluent of a healthy woman. PeerJ 3:e1061. doi: 10.7717/peerj.1061
Hsieh, P.-F., Lin, H.-H., Lin, T.-L., Chen, Y.-Y., and Wang, J.-T. (2017). Two T7-like bacteriophages, K5-2 and K5-4, each encodes two capsule depolymerases: isolation and functional characterization. Sci. Rep. 7, 4624–4624. doi: 10.1038/s41598-017-04644-2
Hung, C. H., Kuo, C. F., Wang, C. H., Wu, C. M., and Tsao, N. (2011). Experimental phage therapy in treating Klebsiella pneumoniae-mediated liver abscesses and bacteremia in mice. Antimicrob. Agents Chemother. 55, 1358–1365. doi: 10.1128/aac.01123-10
Inchley, C. J. (1969). The actvity of mouse Kupffer cells following intravenous injection of T4 bacteriophage. Clin. Exp. Immunol. 5, 173–187.
Kaabi, S. A. G., and Musafer, H. K. (2019). An experimental mouse model for phage therapy of bacterial pathogens causing bacteremia. Microb. Pathog. 137:103770. doi: 10.1016/j.micpath.2019.103770
Käll, L., Krogh, A., and Sonnhammer, E. L. (2007). Advantages of combined transmembrane topology and signal peptide prediction–the Phobius web server. Nucleic Acids Res. 35, W429–W432. doi: 10.1093/nar/gkm256
Karumidze, N., Kusradze, I., Rigvava, S., Goderdzishvili, M., Rajakumar, K., and Alavidze, Z. (2013). Isolation and characterisation of lytic bacteriophages of Klebsiella pneumoniae and Klebsiella oxytoca. Curr. Microbiol. 66, 251–258. doi: 10.1007/s00284-012-0264-7
Kaźmierczak, Z., Majewska, J., Milczarek, M., Owczarek, B., and Dąbrowska, K. (2021). Circulation of fluorescently labelled phage in a murine model. Viruses 13:297. doi: 10.3390/v13020297
Koonin, E. V., and Yutin, N. (2020). The crAss-like phage group: how metagenomics reshaped the human virome. Trends Microbiol. 28, 349–359. doi: 10.1016/j.tim.2020.01.010
Kortright, K. E., Chan, B. K., Koff, J. L., and Turner, P. E. (2019). Phage therapy: a renewed approach to combat antibiotic-resistant bacteria. Cell Host Microbe 25, 219–232. doi: 10.1016/j.chom.2019.01.014
Lim, T. H., Kim, M. S., Lee, D. H., Lee, Y. N., Park, J. K., Youn, H. N., et al. (2012). Use of bacteriophage for biological control of Salmonella Enteritidis infection in chicken. Res. Vet. Sci. 93, 1173–1178. doi: 10.1016/j.rvsc.2012.06.004
Lin, D. M., Koskella, B., and Lin, H. C. (2017). Phage therapy: an alternative to antibiotics in the age of multi-drug resistance. World J. Gastrointest. Pharmacol. Ther. 8, 162–173. doi: 10.4292/wjgpt.v8.i3.162
Lin, Y. W., Chang, R. Y., Rao, G. G., Jermain, B., Han, M. L., Zhao, J. X., et al. (2020). Pharmacokinetics/pharmacodynamics of antipseudomonal bacteriophage therapy in rats: a proof-of-concept study. Clin. Microbiol. Infect. 26, 1229–1235. doi: 10.1016/j.cmi.2020.04.039
Liu, K. Y., Yang, W. H., Dong, X. K., Cong, L. M., Li, N., Li, Y., et al. (2016). Inhalation study of mycobacteriophage D29 aerosol for mice by endotracheal route and nose-only exposure. J. Aerosol Med. Pulm. Drug Deliv. 29, 393–405. doi: 10.1089/jamp.2015.1233
Lowe, T. M., and Chan, P. P. (2016). tRNAscan-SE On-line: integrating search and context for analysis of transfer RNA genes. Nucleic Acids Res. 44, W54–W57. doi: 10.1093/nar/gkw413
Magiorakos, A. P., Srinivasan, A., Carey, R. B., Carmeli, Y., Falagas, M. E., Giske, C. G., et al. (2012). Multidrug-resistant, extensively drug-resistant and pandrug-resistant bacteria: an international expert proposal for interim standard definitions for acquired resistance. Clin. Microbiol. Infect. 18, 268–281. doi: 10.1111/j.1469-0691.2011.03570.x
Majkowska-Skrobek, G., Markwitz, P., Sosnowska, E., Lood, C., Lavigne, R., and Drulis-Kawa, Z. (2021). The evolutionary trade-offs in phage-resistant Klebsiella pneumoniae entail cross-phage sensitization and loss of multidrug resistance. Environ. Microbiol. doi: 10.1111/1462-2920.15476 [Epub ahead of print].
Malik, D. J., Sokolov, I. J., Vinner, G. K., Mancuso, F., Cinquerrui, S., Vladisavljevic, G. T., et al. (2017). Formulation, stabilisation and encapsulation of bacteriophage for phage therapy. Adv. Colloid Interface Sci. 249, 100–133. doi: 10.1016/j.cis.2017.05.014
Manohar, P., Shanthini, T., Ayyanar, R., Bozdogan, B., Wilson, A., Tamhankar, A. J., et al. (2017). The distribution of carbapenem- and colistin-resistance in Gram-negative bacteria from the Tamil Nadu region in India. J. Med. Microbiol. 66, 874–883. doi: 10.1099/jmm.0.000508
Manohar, P., Tamhankar, A. J., Lundborg, C. S., and Nachimuthu, R. (2019). Therapeutic characterization and efficacy of bacteriophage cocktails infecting Escherichia coli, Klebsiella pneumoniae, and Enterobacter Species. Front. Microbiol. 10:574. doi: 10.3389/fmicb.2019.00574
Manrique, P., Dills, M., and Young, M. J. (2017). The human gut phage community and its implications for health and disease. Viruses 9:141. doi: 10.3390/v9060141
McVay, C. S., Velásquez, M., and Fralick, J. A. (2007). Phage therapy of Pseudomonas aeruginosa infection in a mouse burn wound model. Antimicrob. Agents Chemother. 51, 1934–1938. doi: 10.1128/aac.01028-06
Ménard, A., Harambat, J., Pereyre, S., Pontailler, J. R., Mégraud, F., and Richer, O. (2010). First report of septic arthritis caused by Klebsiella oxytoca. J. Clin. Microbiol. 48, 3021–3023. doi: 10.1128/jcm.00302-10
Morozova, V., Kozlova, Y., Shedko, E., Kurilshikov, A., Babkin, I., Tupikin, A., et al. (2016). Lytic bacteriophage PM16 specific for Proteus mirabilis: a novel member of the genus Phikmvvirus. Arch. Virol. 161, 2457–2472. doi: 10.1007/s00705-016-2944-2
Neog, N., Phukan, U., Puzari, M., Sharma, M., and Chetia, P. (2021). Klebsiella oxytoca and emerging nosocomial infections. Curr. Microbiol. 78, 1115–1123. doi: 10.1007/s00284-021-02402-2
Nishikawa, H., Yasuda, M., Uchiyama, J., Rashel, M., Maeda, Y., Takemura, I., et al. (2008). T-even-related bacteriophages as candidates for treatment of Escherichia coli urinary tract infections. Arch. Virol. 153, 507–515. doi: 10.1007/s00705-007-0031-4
Oechslin, F., Piccardi, P., Mancini, S., Gabard, J., Moreillon, P., Entenza, J. M., et al. (2017). Synergistic interaction between phage therapy and antibiotics clears Pseudomonas aeruginosa infection in endocarditis and reduces virulence. J. Infect. Dis. 215, 703–712. doi: 10.1093/infdis/jiw632
Park, E. A., Kim, Y. T., Cho, J. H., Ryu, S., and Lee, J. H. (2017). Characterization and genome analysis of novel bacteriophages infecting the opportunistic human pathogens Klebsiella oxytoca and K. pneumoniae. Arch. Virol. 162, 1129–1139. doi: 10.1007/s00705-016-3202-3
Pires, D. P., Costa, A. R., Pinto, G., Meneses, L., and Azeredo, J. (2020). Current challenges and future opportunities of phage therapy. FEMS Microbiol. Rev. 44, 684–700. doi: 10.1093/femsre/fuaa017
Podschun, R., and Ullmann, U. (1998). Klebsiella spp. as nosocomial pathogens: epidemiology, taxonomy, typing methods, and pathogenicity factors. Clin. Microbiol. Rev. 11, 589–603. doi: 10.1128/cmr.11.4.589
Pouillot, F., Chomton, M., Blois, H., Courroux, C., Noelig, J., Bidet, P., et al. (2012). Efficacy of bacteriophage therapy in experimental sepsis and meningitis caused by a clone O25b:H4-ST131 Escherichia coli strain producing CTX-M-15. Antimicrob. Agents Chemother. 56, 3568–3575. doi: 10.1128/aac.06330-11
Pyra, A., Brzozowska, E., Pawlik, K., Gamian, A., Dauter, M., and Dauter, Z. (2017). Tail tubular protein A: a dual-function tail protein of Klebsiella pneumoniae bacteriophage KP32. Sci. Rep. 7:2223. doi: 10.1038/s41598-017-02451-3
Segall, A. M., Roach, D. R., and Strathdee, S. A. (2019). Stronger together? Perspectives on phage-antibiotic synergy in clinical applications of phage therapy. Curr. Opin. Microbiol. 51, 46–50. doi: 10.1016/j.mib.2019.03.005
Seo, S. U., and Kweon, M. N. (2019). Virome-host interactions in intestinal health and disease. Curr. Opin. Virol. 37, 63–71. doi: 10.1016/j.coviro.2019.06.003
Shi, Y., Chen, Y., Yang, Z., Zhang, Y., You, B., Liu, X., et al. (2020). Characterization and genome sequencing of a novel T7-like lytic phage, kpssk3, infecting carbapenem-resistant Klebsiella pneumoniae. Arch. Virol. 165, 97–104. doi: 10.1007/s00705-019-04447-y
Shi, Y., Peng, Y., Zhang, Y., Chen, Y., Zhang, C., Luo, X., et al. (2021). Safety and efficacy of a phage, kpssk3, in an in vivo model of carbapenem-resistant hypermucoviscous Klebsiella pneumoniae bacteremia. Front. Microbiol. 12:613356. doi: 10.3389/fmicb.2021.613356
Shkoporov, A. N., Clooney, A. G., Sutton, T. D. S., Ryan, F. J., Daly, K. M., Nolan, J. A., et al. (2019). The human gut virome is highly diverse, stable, and individual specific. Cell Host Microbe 26, 527–541.e5. doi: 10.1016/j.chom.2019.09.009
Singla, S., Harjai, K., Raza, K., Wadhwa, S., Katare, O. P., and Chhibber, S. (2016). Phospholipid vesicles encapsulated bacteriophage: a novel approach to enhance phage biodistribution. J. Virol. Methods 236, 68–76. doi: 10.1016/j.jviromet.2016.07.002
Sofy, A. R., El-Dougdoug, N. K., Refaey, E. E., Dawoud, R. A., and Hmed, A. A. (2021). Characterization and full genome sequence of novel KPP-5 lytic phage against Klebsiella pneumoniae responsible for recalcitrant infection. Biomedicines 9:342. doi: 10.3390/biomedicines9040342
Soto-Hernández, J. L., Soto-Ramírez, A., Pérez-Neri, I., Angeles-Morales, V., Cárdenas, G., and Barradas, V. A. (2020). Multidrug-resistant Klebsiella oxytoca ventriculitis, successfully treated with intraventricular tigecycline: a case report. Clin. Neurol. Neurosurg. 188:105592. doi: 10.1016/j.clineuro.2019.105592
Speck, P., and Smithyman, A. (2016). Safety and efficacy of phage therapy via the intravenous route. FEMS Microbiol. Lett. 363:fnv242. doi: 10.1093/femsle/fnv242
Sullivan, M. J., Petty, N. K., and Beatson, S. A. (2011). Easyfig. Bioinformatics 27, 1009–1010. doi: 10.1093/bioinformatics/btr039
Sunagar, R., Patil, S. A., and Chandrakanth, R. K. (2010). Bacteriophage therapy for Staphylococcus aureus bacteremia in streptozotocin-induced diabetic mice. Res. Microbiol. 161, 854–860. doi: 10.1016/j.resmic.2010.09.011
Surani, A., Slama, E. M., Thomas, S., Ross, R. W., and Cunningham, S. C. (2020). Raoultella ornithinolytica and Klebsiella oxytoca pyogenic liver abscess presenting as chronic cough. IDCases 20:e00736. doi: 10.1016/j.idcr.2020.e00736
Titze, I., and Krömker, V. (2020). Antimicrobial activity of a phage mixture and a lactic acid bacterium against Staphylococcus aureus from bovine mastitis. Vet. Sci. 7:31. doi: 10.3390/vetsci7010031
Tiwari, B. R., Kim, S., Rahman, M., and Kim, J. (2011). Antibacterial efficacy of lytic Pseudomonas bacteriophage in normal and neutropenic mice models. J. Microbiol. 49, 994–999. doi: 10.1007/s12275-011-1512-4
Trigo, G., Martins, T. G., Fraga, A. G., Longatto-Filho, A., Castro, A. G., Azeredo, J., et al. (2013). Phage therapy is effective against infection by Mycobacterium ulcerans in a murine footpad model. PLoS Negl. Trop. Dis. 7:e2183. doi: 10.1371/journal.pntd.0002183
Tsubouchi, N., Tsurukiri, J., Numata, J., and Sano, H. (2019). Acute Infectious purpura fulminans caused by Klebsiella oxytoca. Intern. Med. 58, 1801–1802. doi: 10.2169/internalmedicine.2350-18
Uddin, M. J., Kim, B., Dawan, J., Ding, T., Kim, J. C., and Ahn, J. (2019). Assessment of antibiotic resistance in bacteriophage-insensitive Klebsiella pneumoniae. Microb. Pathog. 135:103625. doi: 10.1016/j.micpath.2019.103625
Van Belleghem, J. D., Clement, F., Merabishvili, M., Lavigne, R., and Vaneechoutte, M. (2017). Pro- and anti-inflammatory responses of peripheral blood mononuclear cells induced by Staphylococcus aureus and Pseudomonas aeruginosa phages. Sci. Rep. 7:8004. doi: 10.1038/s41598-017-08336-9
Vinodkumar, C. S., Kalsurmath, S., and Neelagund, Y. F. (2008). Utility of lytic bacteriophage in the treatment of multidrug-resistant Pseudomonas aeruginosa septicemia in mice. Indian J. Pathol. Microbiol. 51, 360–366. doi: 10.4103/0377-4929.42511
Wang, J., Hu, B., Xu, M., Yan, Q., Liu, S., Zhu, X., et al. (2006). Therapeutic effectiveness of bacteriophages in the rescue of mice with extended spectrum beta-lactamase-producing Escherichia coli bacteremia. Int. J. Mol. Med. 17, 347–355.
Wang, J. L., Kuo, C. F., Yeh, C. M., Chen, J. R., Cheng, M. F., and Hung, C. H. (2018). Efficacy of φkm18p phage therapy in a murine model of extensively drug-resistant Acinetobacter baumannii infection. Infect. Drug Resist. 11, 2301–2310. doi: 10.2147/idr.s179701
Wang, Z., Cai, R., Wang, G., Guo, Z., Liu, X., Guan, Y., et al. (2021). Combination therapy of phage vB_KpnM_P-KP2 and gentamicin combats acute pneumonia caused by K47 serotype Klebsiella pneumoniae. Front. Microbiol. 12:674068. doi: 10.3389/fmicb.2021.674068
Watanakunakorn, C., and Jura, J. (1991). Klebsiella bacteremia: a review of 196 episodes during a decade (1980-1989). Scand. J. Infect. Dis. 23, 399–405. doi: 10.3109/00365549109075086
Wienhold, S. M., Lienau, J., and Witzenrath, M. (2019). Towards inhaled phage therapy in Western Europe. Viruses 11:295. doi: 10.3390/v11030295
Keywords: Klebsiella oxytoca, biological characteristics, phage vB_Kox_ZX8, phage therapy, bacteremia
Citation: Li P, Zhang Y, Yan F and Zhou X (2021) Characteristics of a Bacteriophage, vB_Kox_ZX8, Isolated From Clinical Klebsiella oxytoca and Its Therapeutic Effect on Mice Bacteremia. Front. Microbiol. 12:763136. doi: 10.3389/fmicb.2021.763136
Received: 23 August 2021; Accepted: 04 November 2021;
Published: 03 December 2021.
Edited by:
Dwayne R. Roach, San Diego State University, United StatesCopyright © 2021 Li, Zhang, Yan and Zhou. This is an open-access article distributed under the terms of the Creative Commons Attribution License (CC BY). The use, distribution or reproduction in other forums is permitted, provided the original author(s) and the copyright owner(s) are credited and that the original publication in this journal is cited, in accordance with accepted academic practice. No use, distribution or reproduction is permitted which does not comply with these terms.
*Correspondence: Fuhua Yan, eWFuZmhAbmp1LmVkdS5jbg==; Xin Zhou, emhvdV94aW5AMTI2LmNvbQ==
†These authors have contributed equally to this work