- 1Department of Aquaculture, Faculty of Fisheries and Environmental Sciences, Gorgan University of Agriculture Sciences and Natural Resources, Gorgan, Iran
- 2Laboratory Sciences Research Center, Golestan University of Medical Sciences, Gorgan, Iran
- 3Department of Laboratory Sciences, Faculty of Paramedicine, Golestan University of Medical Sciences, Gorgan, Iran
- 4Department of Animal Nutrition and Management, Aquaculture Nutraceuticals Research Group, Faculty of Veterinary Medicine and Animal Sciences, Swedish University of Agricultural Sciences, Uppsala, Sweden
In this study, we tested the compatibility of two extracts from the plant Jerusalem artichokes and button mushrooms with two different Lactobacillus probiotics (Lactobacillus acidophilus; La and Lactobacillus delbrueckii subsp. Bulgaricus; Lb) to develop a synbiotic formulation to improve the growth, survival, and reproductive performances of farmed fishes. Initially, we employed in vitro approach to monitor the growth of the probiotic lactobacilli in the presence of the different doses of the plant-based prebiotics, with the aim of selecting interesting combination(s) for further verification under in vivo conditions using zebrafish as a model. Results from the in vitro screening assay in the broth showed that both the probiotic species showed a preference for 50% mushroom extract as a source of prebiotic. A synbiotic formulation, developed with the selected combination of L. acidophilus, L. bulgaricus, and 50% mushroom extract, showed a positive influence on the growth and reproductive performances of the zebrafish. Our findings also imply that the improvement in the reproductive indices was associated with the upregulation of a cyp19a gene. Overall results suggest that a combination of L. acidophilus, L. bulgaricus, and mushroom extract can be considered as a potential synbiotic for the successful production of aquaculture species.
Introduction
The gut microbiota plays a central role in the health, well-being, growth, and disease prevention in fish (Xiong et al., 2019). The composition of the microbial communities in the fish gut is not constant and may change with nutritional status, age, rearing water, and other environmental conditions (Ringø et al., 2010; Zhang et al., 2018; Yukgehnaish et al., 2020). The microbial balance in the fish gut is crucial for its optimal metabolism and disease prevention. Over the past few years, there has been an increasing effort to steer the gut microbiota of fish toward beneficial communities as the dominance of such beneficial microbes in the fish gut has been linked to the improvement of the growth and reproductive performances, and the resistance of the fish toward pathogens (Allameh et al., 2015; Akbari Nargesi et al., 2020; Hasan and Banerjee, 2020; Kong et al., 2020; Ringø et al., 2020b; Montazeri-Parchikolaei et al., 2021). One approach to induce a healthy gut microbiome in fish has been through the administration of probiotics – live microorganisms that, when administered in adequate amounts, confer a health benefit on the host (Aydin and Şehriban, 2019; Cámara-Ruiz et al., 2020). Numerous probiotic studies have evaluated the effects of various genera, species, and strains of bacteria on the health status, disease resistance, and growth performance of farmed aquatic animals (Ashouri et al., 2018; Tan et al., 2019; Kuebutornye et al., 2020; Mohammadi Arani et al., 2021). These studies showed that the most common type of bacteria that are effective in improving the microbiota balance in the intestine of the host are the ones that belong to the Lactobacillus and Bifidobacterium genera (EFSA FEEDAP et al., 2018), although other bacteria and certain yeasts were also effective (Guluarte et al., 2019; Tarnecki et al., 2019; Banu et al., 2020). Bacteria belonging to Lactobacillus species have been used as probiotics in many economically important farmed fish, and it has been reported to improve the growth performance as well as to control infections caused by bacteria (such as Aeromonas salmonicida, Vibrio anguillarum, Flavobacterium psychrophilum, and Edwardsiella tarda) in fish species (Shabirah et al., 2019; Hasan and Banerjee, 2020; Kuebutornye et al., 2020; Mora-Sánchez et al., 2020; Ringø et al., 2020a). Despite all the health benefits of probiotics, a major limitation that remains for their uses as gut/health beneficial agents in farmed fish is related to the poor capacity of the administered probiotic strains to stably or even transiently colonize the host gastrointestinal mucosal surface (Suez et al., 2019; Amenyogbe et al., 2020). To overcome these limitations of probiotics, prebiotics are used together with probiotics to have a synergistic effect (an approach referred to as synbiotics) to markedly inhibit the growth of pathogenic microbes and improve the growth and/or activity of the beneficial/probiotic microorganisms in the host gut (FAO, 2007). Prebiotics are a non-viable food component that confers health benefit(s) on the host associated with modulation of the microbiota (Okolie et al., 2017; Carlson et al., 2018; Van Doan et al., 2020). The majority of studies dealing with synbiotics are conducted in humans, but over the past few years, increasing research has been focused on farmed aquatic animals (Hasan et al., 2019; Dawood et al., 2020). There is evidence to suggest that synbiotics influence the microbial ecology of the intestines of fish and play a role in causing beneficial effects on the health and growth traits of farmed fish, e.g., by preventing the negative effects imposed by infection as well as environmental stress, and by elevating the activities of the digestive enzymes, which eventually contribute to improved feed utilization and growth performances (Huynh et al., 2017; Kumar et al., 2018; Mohammadian et al., 2019; Hasyimi et al., 2020; Moustafa et al., 2020; Kong et al., 2021).
As prebiotics, the most studied in fish were inulin, mannan oligosaccharides, fructooligosaccharides, galactooligosaccharides, and nano-oligosaccharide (Cid García et al., 2020; Kishawy et al., 2020; Pietrzak et al., 2020; Zhou et al., 2020; Ghafarifarsani et al., 2021). These types of prebiotic molecules are present in a variety of plants, such as Jerusalem artichoke, cereals, leeks, asparagus, and garlic with varying degrees of polymerization, which is associated with their different functional features (Mensink et al., 2015; Bharathi et al., 2019; Harris et al., 2019; Khangwal and Shukla, 2019; Sribounoy et al., 2021). It is however important to mention that the use of food-grade prebiotic compounds derived from plant sources as a functional feed additive in the aquafeed industry is limited by the cost of the extraction process. In the interest of addressing the production cost, increasing attention has been paid to the direct use of raw plant extracts as potential sources of natural prebiotics. Jerusalem artichoke (Helianthus tuberosus), a natural prebiotic enriched with inulin and fructooligosaccharides, has become a focus for use as a functional feed ingredient in the diets of farmed (aquatic) animals (Tiengtam et al., 2017; Abedalhammed et al., 2020). An improvement in the growth performance, resistance toward bacterial disease, and immune responses in farmed (aquatic) animals like common carp diets (Cyprinus carpio L.), juvenile red tilapia (Oreochromis spp.), and sea cucumber as well as in poultry and swine in response to feeding Jerusalem artichoke-supplemented diet have been reported (Sewaka et al., 2019; Abedalhammed et al., 2020; Jia et al., 2020). Another plant of interest as a source of natural prebiotics is the edible mushroom owing to its richness in bioactive substances, such as functional polysaccharides, terpenes, peptides, glycoproteins, mineral elements, unsaturated fatty acids, phenolic substances, vitamin E, and vitamin C (Aida et al., 2009; Rathore et al., 2017; Safari et al., 2019; Ansari and Jadhav, 2021; Das et al., 2021). Mushrooms and their derivatives have been widely used in aquaculture for improvement of growth performance, hematological parameters, innate immunity, and diseases resistance in many cultured species (Ahmed et al., 2017; Amiri et al., 2018; Hoseinifar et al., 2019a,b; Safari et al., 2019; Rattanachan et al., 2020; Al-Maadhedy et al., 2021; Harikrishnan et al., 2021; Kumar et al., 2021). Among different cultured mushroom species, the white button mushroom, Agaricus bisporus, represents an interesting source of natural prebiotics because it is a safe food that is cultured worldwide (Hoseinifar et al., 2019b), and hence it is easily available for applications. Additionally, it contains a wide variety of nutraceutical substances, such as polyphenols, ergothioneine, vitamins, minerals, and polysaccharides (Tian et al., 2012; Ramos et al., 2019; Usman et al., 2021). Agaricus bisporus mushroom has been well-documented for its growth-promotion, immune-enhancing, and disease-resistance in some farmed fishes like grass carp (Ctenopharyngodon idella), common carp (Cyprinus carpio), catfish (Silurus asotus), and rainbow trout (Oncorhynchus mykiss) Nile tilapia (Oreochromis niloticus; Van Doan et al., 2017; Amiri et al., 2018; Harikrishnan et al., 2018, 2021; Hoseinifar et al., 2019b; Safari et al., 2019; Habib et al., 2021).
In this study, we tested two plant extracts (Jerusalem artichokes and button mushrooms) as potential sources of prebiotics using in vitro assay for their ability to facilitate the growth of two probiotic strains of interest [Lactobacillus acidophilus ATCC4356 (La) and L. delbrueckii subsp. bulgaricus ATCC11842 (Lb)]. Subsequently, the preferred prebiotic candidate, which showed the most prominent effect, was combined with each of the probiotic strains in an attempt to move toward identifying synbiotic combinations. The effectiveness of the synbiotic preparation was verified in vivo using zebrafish as a model organism by focusing on the following readouts: growth traits, survival, and reproductive performances.
Materials and Methods
Probiotic Bacterial Strains
Two probiotic bacterial strains, L. acidophilus ATCC4356 (La) and L. delbrueckii subsp. bulgaricus ATCC11842 (Lb), purchased from Persian Type Culture Collection, Iran, were used in this study. Stock cultures were prepared by mixing a pure culture of the strain, grown overnight at 37°C in De Man Rogosa Sharpe (MRS) broth medium (Merck, Darmstadt, Germany), with 25% (v/v) sterile glycerol as cryoprotectant and stored at −80°C (Haj-Mustafa et al., 2015).
Inoculum Preparation
Working inoculums were prepared by subculturing the stock culture in MRS broth overnight at 37°C. After that, a certain volume of the subculture was transferred (1% v/v) to a fresh volume of MRS broth and incubated with shaking at 150rpm for 18h at 37°C. The bacterial suspension was then centrifuged at 8,000 x g for 20min (4°C), the cell pellet washed twice with sterile phosphate buffer saline (PBS), and subsequently used for the inoculation of the culture medium (Haj-Mustafa et al., 2015).
Bacterial Growth Analysis
Growth of La and Lb was monitored by measuring the optical cell density at 600nm using a UV/Visible spectrophotometer (Shimidzo, Japan). The measured values were plotted on growth curves. The maximum specific growth rate during the exponential growth phase was calculated following the equation of Kask et al. (2003):
Where t=time, N=optical density at the end of the exponential growth phase (t), N0=optical density at the beginning of the exponential growth phase (t0), and μ=specific growth rate constant (h−1).
The doubling time was determined by the equation: Td=ln2/μmax, where, μmax – maximum specific growth rate, td- doubling time.
Preparation of Plant Extracts as Prebiotic Sources
Two plants, Jerusalem artichokes (H. tuberosus; hereafter referred to as artichoke) and white button mushrooms (A. bisporus; hereafter referred to as mushroom), procured from a private company in Iran, were used as sources of prebiotics. The plant materials were repeatedly washed with tap water to remove the presence of any dirt and then allowed to dry until the water droplets disappeared from their surface. Each one of the artichokes and the mushroom was cut into small slices. The slices were then dipped in a 0.5% (w/v) citric acid solution for 15min to avoid browning (Mahore and Shirolkar, 2018) and dried in an oven at 50°C for 48h. The dried samples were ground in a household grinder. The extraction process was performed by the soaking method as previously described (Harborne, 1980). Briefly, 100g of each dry powder was added in 1l of distilled water, and the mixture was kept in dark for 48h. After that, the concentrated liquid was centrifuged (10, 000 x g at 4°C, 15min; Sobye et al., 2018), and the resulting precipitate was discarded. Finally, the supernatant was then filtered using a 0.22μm syringe filter and used for preparing the desired prebiotic concentrations (2, 25, 50, 75, and 100%). In this assay, glucose was removed from all culture media (broth/agar) containing extracts as previously described (Hoseinifar et al., 2017).
In vitro Evaluation of Prebiotics Properties of the Extracts
Each of the extracts from artichoke and mushroom was added to the glucose-free MRS medium at different concentrations (2, 25, 50, 75, and 100%). The MRS medium supplemented with glucose as a carbon source was maintained as a positive control group. The pH of the media was adjusted by 0.1N HCl to 5.8. The glucose-free MRS and MRS media containing each of the extracts (50ml) were inoculated with a culture of the probiotic strain La or Lb at a concentration of 107 cells/ml. The solutions were incubated at 37°C for 48h under aerobic conditions after which the optical density (600nm) was measured as previously described (Hoseinifar et al., 2017).
Diet Preparation
A commercial diet for small fishes, kindly provided by the Blue Line Company (Italy), was used as a basal diet and for the preparation of the experimental diets. The proximate composition of the diets is mentioned in Table 1. Based on the outcome from in vitro studies, we designed seven experimental diets. Diet 1: the basal diet was used as the control diet, Diet 2: the basal diet supplemented with 1% mushroom extract prepared from 50% concentrated extract, Diets 3 and 4: the basal diet supplemented with La at 107 colony-forming units per gram of diet (CFUg−1) and Lb at 107CFUg−1, respectively, Diet 5: the basal diet supplemented with La at 107CFUg−1 and 1% of a mushroom extract prepared from 50% concentrated extract, Diet 6: the basal diet supplemented with Lb at 107CFUg−1 and 1% mushroom extract prepared from a 50% concentrated extract, and Diet 7: the basal diet supplemented with La and Lb, each at 107CFUg−1 and mushroom extract at 1% prepared from a 50% concentrated extract. The supplements were sprayed on the feed, mixed manually, and then each experimental diet was coated with 5% gelatin. To avoid the possible effects of gelatin, the control diet was also coated with 5% gelatin. All the diets were dried in a clean place at room temperature (25°C) and were then stored at 4°C until use. Diets were prepared weekly throughout the feeding trial and also analyzed for the viability of bacteria by culturing random samples of diets containing probiotics in MRS broth (Ashouri et al., 2018).
Experimental Animals and Design
Before initiation of the feeding trial, zebrafish larvae, obtained from a private sector farm in Gorgan, Golestan province, Iran, were kept for 2weeks in a holding tank for acclimatization during which they were fed with basal diet. The larvae were regularly monitored for their health and performance. No mortality was recorded during this period. After 2weeks, a group of 840 larvae with a mean initial weight and length of 75.9±1.0mg and 16.0±1.0mm, respectively, were randomly distributed into seven experimental groups (diets 1–7) with three replicates per group. Each group was maintained in an aquarium of 60L capacity containing 30L of water at a density of 40 fish per aquarium. Each diet was fed thrice daily (8:00, 12:30, and 18:00h) at 5% of the body weight for 120days (Lin et al., 2012; Hoseinifar et al., 2015). Round-the-clock aeration was provided to all the aquaria. Siphoning of uneaten feed and faecal matter was done daily. An approximately 25% of the aquarium water was removed daily and replaced with well-aerated freshwater. The experiment was conducted in the 14:10h light–dark cycles (Mohammadi Arani et al., 2019). Water temperature, pH, and dissolved oxygen were monitored daily and maintained approximately at 25±2°C, 7±0.2, and 7.9±0.1mgL−1, respectively (Safari et al., 2018). No mortality was recorded during the feeding period. The zebrafish larvae were kept and handled following the ethical guidelines for in vivo experiments developed by the Gorgan University of Agriculture and Natural Resources, Iran.
Sample Collection and Growth Study
At the end of the feeding trial, fish in each tank fasted for 24h, and then 10 fish per aquarium (30 fish per treatment) were randomly sampled. They were immediately anesthetized using 200mgL−1 clove powder and their weight and length were measured at accuracy levels of 0.001g and 1mm, respectively (Mohammadi Arani et al., 2019). The growth and survival of the fish were monitored based on the following standard formula (Abedian-Amiri et al., 2017):
Where W2, W1, and t represent final weight (mg), initial weight (mg), and the trial period (day), respectively.
Analysis of the Reproductive Performance
Ten fish from each experimental group were randomly sampled and sacrificed using an overdose of 500mgL−1 clove solution. The ovaries were completely dissected out and each of them was weighed with 0.0001mg precision. The number of ovules in each ovary was counted using a microscope. The reproductive performance was calculated following standard formulae (Mohammadi Arani et al., 2019) as listed below:
Analysis of cyp19a Gene
Nine fish (three fishes/replicates) were randomly sampled from each treatment. They were anesthetized using clove solution (500mgL−1), and then the ovaries were dissected out. Total RNA from each sampled ovary (100mg) was extracted using RNAx-PLUS extraction kit following the manufacturer’s instructions (Sinaclon, Iran). The samples were treated with RNAase-free DNase to remove DNA contamination. The quality and quantity of the isolated RNA were measured as described previously (Hosseini et al., 2016). The first-strand cDNAs were synthesized from total RNA using the cDNA synthesis kit (Genet Bio-Synthesis, South Korea) according to the manufacturer’s instructions. The RT-PCR reaction was run in triplicate using a standard protocol (Hosseini et al., 2016). β-actin was used as an internal control for gene expression normalization. The qPCR primers for cyp19a and β-actin were designed using Oligo7 as previously described (Mohammadi Arani et al., 2021; Table 2). To validate primers, qPCR efficiency was also taken into account for choosing the best qPCR primers pair with specific and correct size. The PCR efficiency and relative mRNA expression of cyp19a were calculated based on standard curve analysis with serial dilution of cDNA (including five dilutions) as previously described by Miandare et al. (2019).
Amplification and detection of specific products were performed using StepOne Real-Time PCR System (Life Technologies, Carlsbad, CA, United States), qPCR Master Mix containing SYBR® Green (Life Technologies, Carlsbad, CA, United States), and cyp19a specific primers (GenBank accession number AF183906). The samples were amplified in 25μl reaction mixtures containing 5.5μl of nuclease-free water, 1μl of each primer, 12.5μl of Maxima SYBR Green qPCR Master mix, and 5μl of cDNA template. The thermal cycle protocol was denaturation, annealing, and extension at 94°C for 20s, 60°C for 30s, and 72°C for 40s, respectively. The present sizes of all PCR products were verified by inspection of the dissociation curve and gel electrophoresis. The relative quantification of gene expression was calculated using the following equation (Livak and Schmittgen, 2001):
Statistical Analysis
All the data were subjected to one-way ANOVA using statistical software Statistical Package for the Social Sciences (SPSS) version 16.0. Duncan’s multiple range tests were used to determine the differences among treatment means at p<0.05.
Results
Impact of Jerusalem Artichoke Extract on the Growth of L. acidophilus
The growth rate of L. acidophilus in the function of time, cultured in the different experimental media was different (Figure 1). No growth was recorded in the glucose-free and artichoke extract-free culture medium. However, in the glucose-free MRS medium with artichoke extract as a substrate, a marked increase in the growth of L. acidophilus was recorded. Maximum improvement in the growth of L. acidophilus was noted in the medium that contained 100% artichoke extract. This was followed by those that were grown in the medium that contained 75% extract. The growth of L. acidophilus cultured in the medium with 2, 25, or 50% artichoke extract as substrate was lower than that in the medium that contained a standard amount of glucose (i.e., positive control).
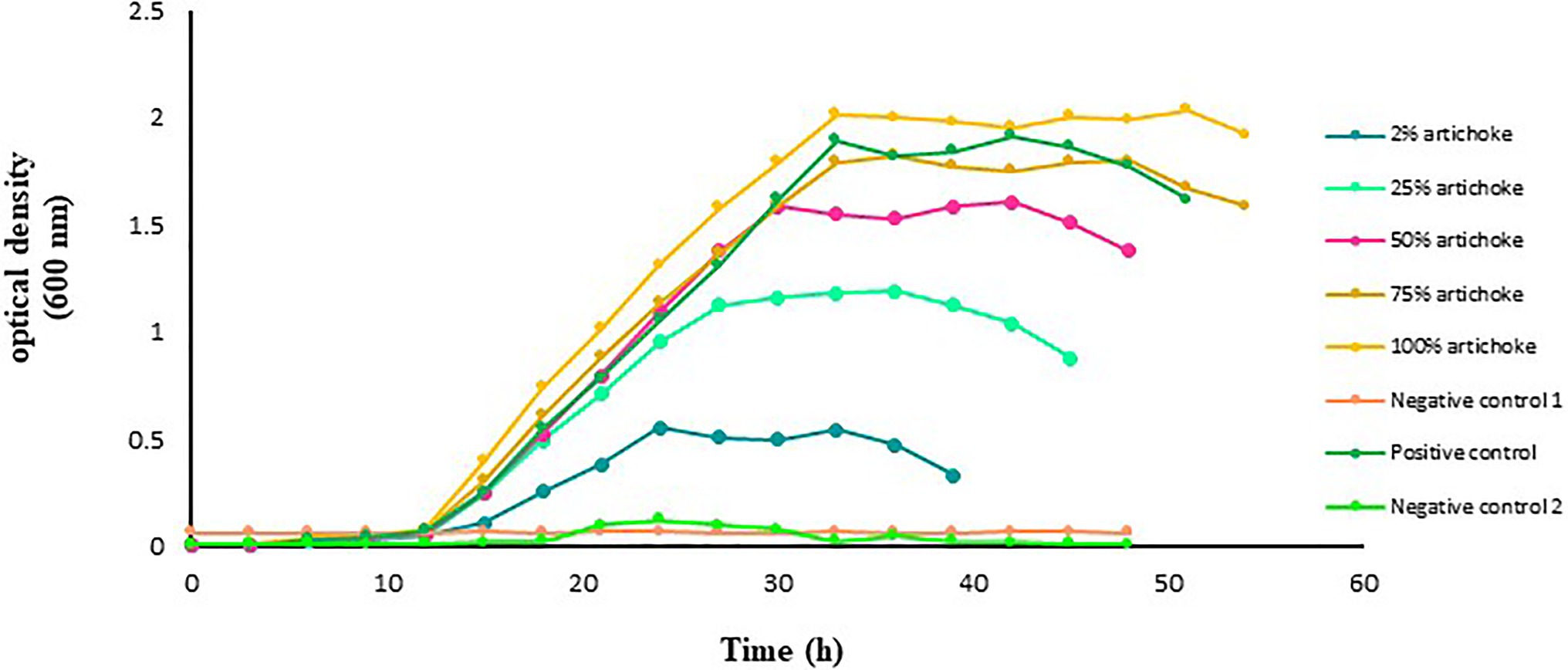
Figure 1. Growth of Lactobacillus acidophilus in De Man Rogosa Sharpe (MRS) broth medium with and without Jerusalem artichoke extract added at an increasing concentration of 2, 25, 50, 75, or 100%. Lactobacillus acidophilus grown in MRS broth medium with a standard amount of glucose served as a positive control. The glucose-free MRS medium with 100% of artichoke extract and without L. acidophilus served as negative control 1. The MRS medium free from both glucose and extract but with L. acidophilus served as negative control 2. The data points are the mean values of three replicates.
Impact of Mushroom Extract on the Growth of L. acidophilus
The growth of L. acidophilus was affected by the absence of glucose or mushroom extract in the medium (Figure 2). However, in the presence of mushroom extract, a marked improvement in the growth of L. acidophilus was observed, with the maximum being recorded in the medium that contained 50% extract. The growth of L. acidophilus cultured in the medium with 2, 25, or 100% mushroom extract as substrate was lower than that in the medium that contained a standard amount of glucose (i.e., positive control). Lactobacillus acidophilus cultured in the medium containing only glucose or 75% mushroom extract as substrate exhibited a similar growth rate.
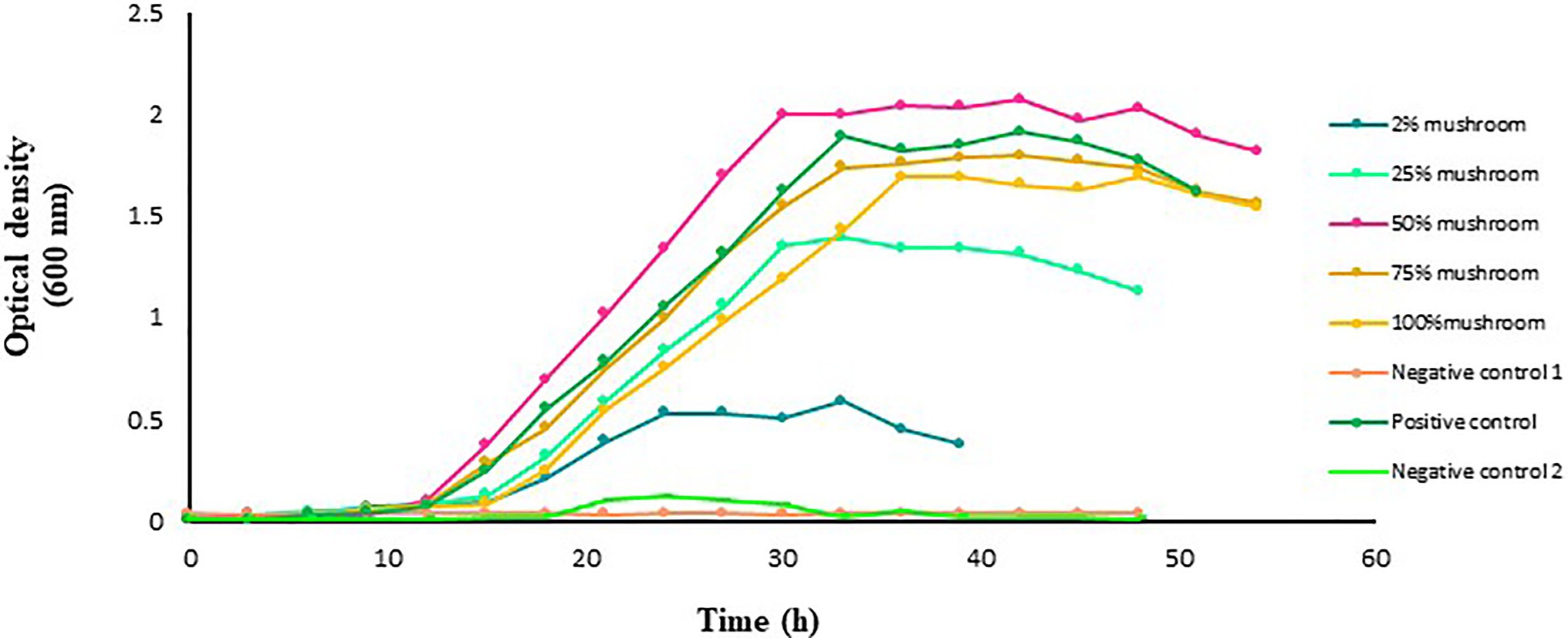
Figure 2. Growth of L. acidophilus in MRS broth medium with and without mushroom extract added at an increasing concentration of 2, 25, 50, 75, or 100%. Lactobacillus acidophilus grown in MRS broth medium with a standard amount of glucose served as a positive control. The glucose-free MRS medium with 100% of mushroom extract and without L. acidophilus served as negative control 1. The MRS medium free from both glucose and extract but with L. acidophilus served as negative control 2. The data points are the mean values of three replicates.
Impact of Jerusalem Artichoke Extract on the Growth of L. delbrueckii Subsp. bulgaricus
The growth of L. delbrueckii in the MRS culture medium was directly associated with the concentration of artichoke extract, which increased with the increase in the extract concentration (Figure 3). Maximum optical density reached by L. delbrueckii culture was in the medium that contained 100% artichoke extract. In the glucose-free MRS medium that contained artichoke extract at a concentration lower than 75%, the growth of L. delbrueckii in the function of time was relatively lower than that in the positive control.
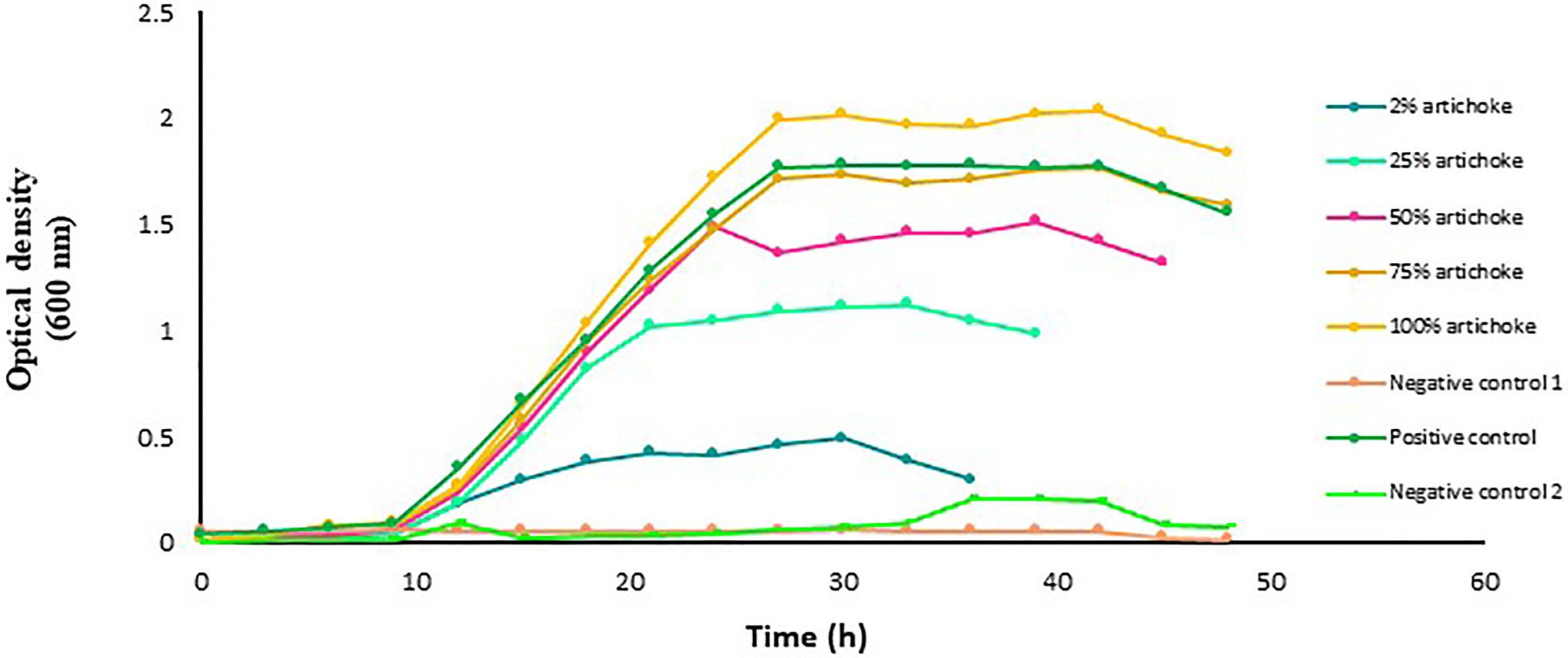
Figure 3. Growth of Lactobacillus delbrueckii subsp. bulgaricus in MRS broth medium with and without artichoke extract added at an increasing concentration of 2, 25, 50, 75, or 100%. Lactobacillus acidophilus grown in MRS broth medium with a standard amount of glucose served as a positive control. The glucose-free MRS medium with 100% of artichoke extract and without L. acidophilus served as negative control 1. The MRS medium free from both glucose and extract but with L. acidophilus served as negative control 2. The data points are the mean values of three replicates.
Impact of Mushroom Extract on the Growth of L. delbrueckii Subsp. bulgaricus
The mushroom extract added at concentration of 50% in the culture medium markedly promotes the growth of L. delbrueckii in comparison to the controls (Figure 4). Increasing the extract concentration to 75% and higher did not further improve the growth of L. delbrueckii. In contrast, the growth exhibited a pattern similar to that in the positive control. The growth of L. delbrueckii cultured in the medium with 2 or 25% mushroom extract as substrate was lower than that in the positive control.
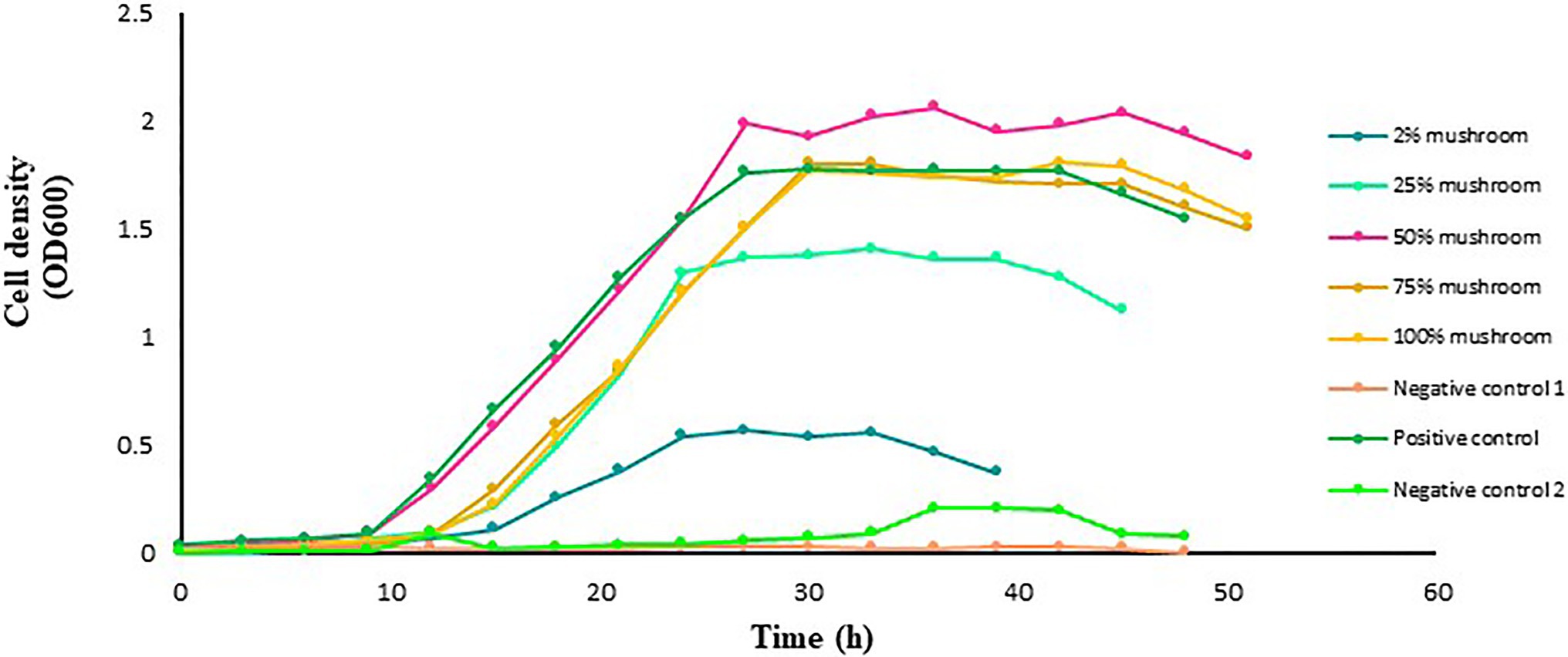
Figure 4. Growth of L. delbrueckii subsp. bulgaricus in MRS broth medium with and without mushroom extract added at an increasing concentration of 2, 25, 50, 75, or 100%. Lactobacillus acidophilus grown in MRS broth medium with a standard amount of glucose served as a positive control. The glucose-free MRS medium with 100% of mushroom extract and without L. acidophilus served as negative control 1. The MRS medium free from both glucose and extract but with L. acidophilus served as negative control 2. The data points are the mean values of three replicates.
Impact of the Plant Extracts on the Specific Growth Rate, Doubling Time of the Lactobacillus Probionts, and the pH of the Culture Media
A comparative analysis was carried out to determine the extracts that supported the best in promoting the growth indices, in terms of specific growth rate and doubling time, of the tested probiotic strains. The doses (50% of mushroom extract and 100% of artichoke extract) that showed the best performances in the bacterial growth studies were used for comparison purposes. As shown in Figure 5A, the specific growth rate of both the Lactobacillus probionts cultured in the medium with 50% mushroom extract was significantly (p<0.05) higher than those that were cultured with artichoke extract (100%) as substrate. No significant difference was observed in the doubling time between L. acidophilus and L. delbrueckii cultured on mushroom extract as substrate (p>0.05).
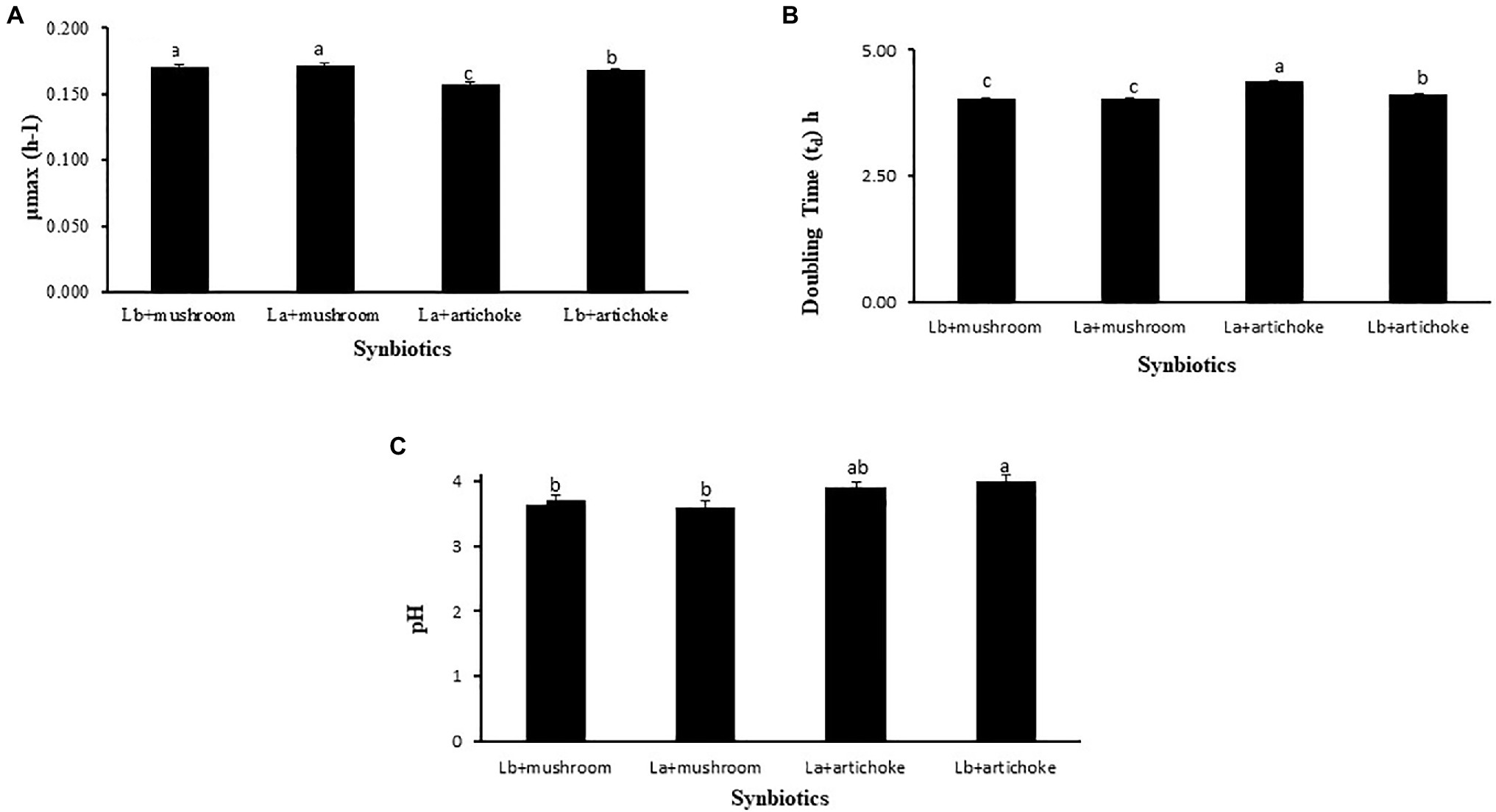
Figure 5. Comparison of the (A) specific growth rate (μmax; h−1), (B) doubling time (Td; h), and (C) pH of the medium of L. acidophillus with that of L. delbrueckii subsp. bulgaricus grown in MRS medium containing 50% mushroom or 100% artichoke extract. Bars with different letters indicate a significant difference (p<0.05). Data are presented as mean±SE.
The doubling time of L. acidophilus and L. delbrueckii cultured in the medium containing 50% mushroom extract was significantly shorter in comparison to that of the probionts cultured on artichoke extract as substrate (p<0.05; Figure 5B). No significant difference was observed in the specific growth rate between L. acidophilus and L. delbrueckii cultured on mushroom extract as substrate (p>0.05).
The pH of the MRS medium containing a culture of L. acidophilus or L. delbrueckii in the presence of 50% mushroom extract was significantly lower than that of the medium containing L. acidophilus or L. delbrueckii culture in combination with 100% artichoke extract (Figure 5C).
Growth Performances and Survival
The final weight of the fish after 4weeks of feeding and the resulting average fish weight gain during this experimental period were determined for all the treatments. At the start of the experiment, the fish in all the experimental groups weighed between 75 and 77mg and were not significantly different (Table 3). The weight of the fish in the experiment groups, irrespective of the treatment increased by 3–5-fold over the 4-week experimental period (Table 3). When calculating the growth variables, such as average fish weight gain and specific growth rate (SGR), no significant difference could be observed between the groups of fish fed a control diet, diet 3 supplemented with only La, and diet 5 supplemented with both La and mushroom extract. However, the group fed diet 6 supplemented with Lb and mushroom extract exhibited the highest weight gain and SGR values, and was significantly different from that of the control. Fish fed diet 7 supplemented with La, Lb, and mushroom extract in combination, their weight gain and SGR values did not differ significantly from that of the fish fed diet 6, however, was significantly higher than that of the control. The weight gain and SGR values in the groups fed diet 2 with mushroom extract as a supplement and diet 4 with Lb as a supplement were significantly higher than the control. However, the values were still significantly lower than the highest values that were observed for the diet 6 fed group. The average food conversion ratio (FCR) was 2.4 in the case of the control group. A similar FCR of 2.3 was found for zebrafish fed with diet 3 or diet 5. The feeding of diet 7 with Lb, La, and mushroom extract as a supplement resulted in a significant decrease in the FCR value. This was translated in a value of 1.8 for the diet 7 fed group, while the largest effect, although not significantly different from the latter, was noted for the fish fed diet 6 with an FCR value of 1.7. No mortality was recorded during the feeding period and the condition factor of the fish remained the same among the different experimental groups.
Reproduction Performances
The results obtained for reproductive indices are shown in Table 4. As a general trend, the Gonadosomatic Index (GSI), absolute fecundity, and relative fecundity were influenced by the dietary supplements. No significant difference of the three indicated indices could be observed between the control groups, the group fed diet 3 supplemented with La, and the group fed diet 5 supplemented with both La and mushroom extract. However, the group fed diet 7 supplemented with a mixture of La, Lb, and mushroom extract exhibited the highest GSI, absolute fecundity, and relative fecundity values, and was significantly different from that of the control. The working fecundity of the fish fed different experimental diets did not differ significantly; neither did the hatching percentage of the fish in different experimental groups. The survival percentage of the fry after 4weeks of hatching in the different experimental groups was between 71 and 77% and was not significantly different.
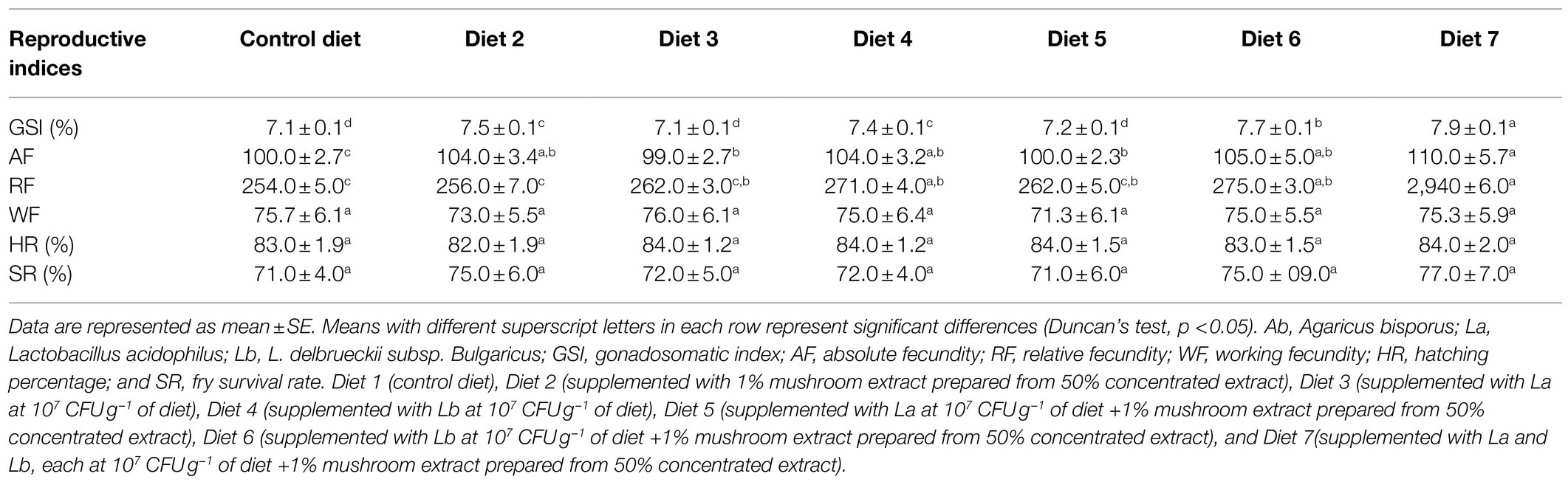
Table 4. Reproduction performance and fry survival of zebrafish fed different experimental diets for 120days.
Expression of cyp19a Gene
The relative abundance of mRNA encoding for the cyp19a gene was determined by qPCR in the ovaries of female zebrafish fed different experimental diets for 120days. At the end of the feeling trial, it could be observed that in the group fed with diet 3 supplemented with La, and in the group fed diet 5 supplemented with both La and mushroom extract, no significant upregulation of the cyp19a gene was recorded relative to the control. However, the groups fed diet 2, diet 3, diet 6, or diet 7; all these groups exhibited a significant increase in the expression level of cyp19a gene. Among all the experimental groups, those that were fed diet 7 supplemented with a mixture of La, Lb and mushroom extract recorded the highest expression level of cyp19a gene (p<0.05; Figure 6).
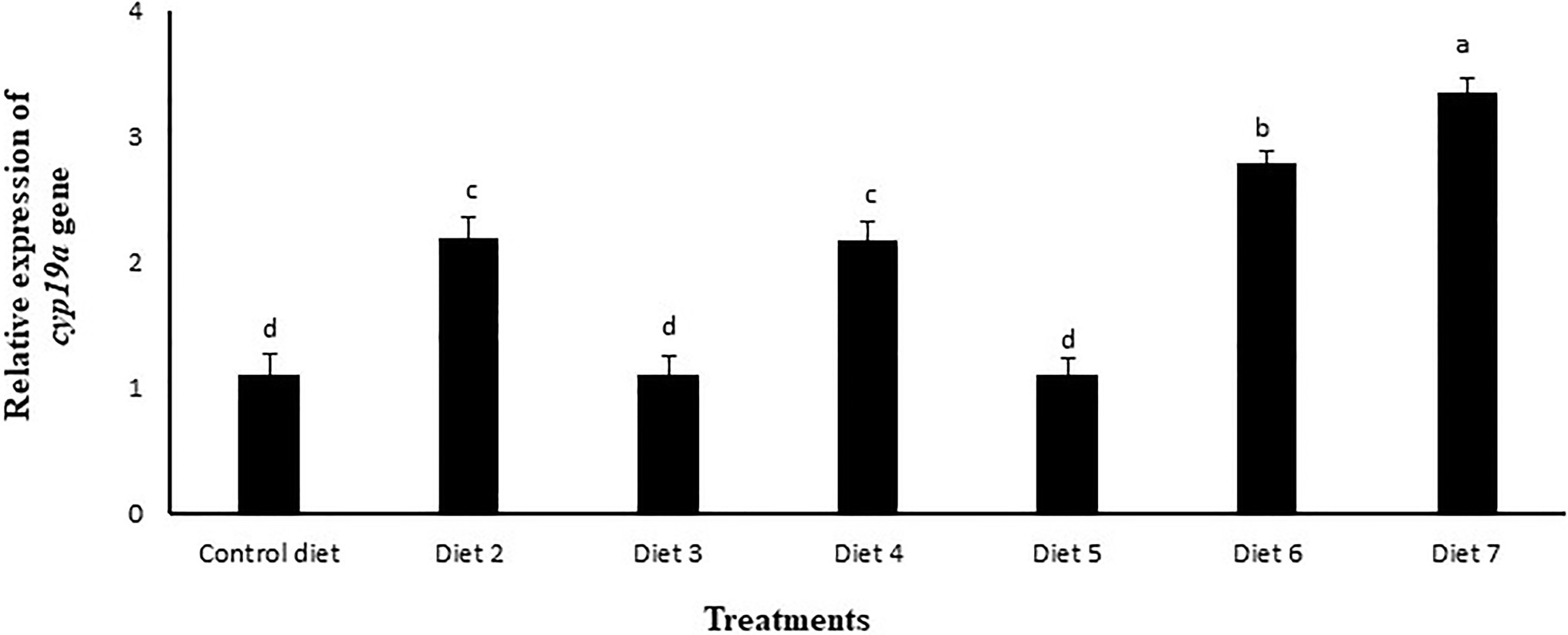
Figure 6. Effects of feeding various experimental diets for 120days on the relative expression of cyp19a gene in the ovary of zebrafish, normalized against β-actin factor as reference gene. Bars with different letters indicate significant differences (p<0.05). Data are presented as mean±SE of three replicates. Diet 1 (control diet), Diet 2 (supplemented with 1% mushroom extract prepared from 50% concentrated extract), Diet 3 (supplemented with La at 107CFUg−1 of diet), Diet 4 (supplemented with Lb at 107CFUg−1 of diet), Diet 5 (supplemented with La at 107CFUg−1 of diet +1% mushroom extract prepared from 50% concentrated extract), Diet 6 (supplemented with Lb at 107CFUg−1 of diet +1% mushroom extract prepared from 50% concentrated extract), and Diet 7(supplemented with La and Lb, each at 107CFUg−1 of diet +1% mushroom extract prepared from 50% concentrated extract).
Discussion
Here, we tested the compatibility of two extracts from the plant Jerusalem artichokes and button mushrooms with two different Lactobacillus probiotics to develop a synbiotic formulation with the aim to improve the growth and reproductive performances of farmed fishes. The two plant extracts were selected in this study because of their prebiotic potential as demonstrated in previous studies on farmed fishes (for details, see, Amiri et al., 2018; Boonanuntanasarn et al., 2018; Hoseinifar et al., 2019b; Sewaka et al., 2019; Abedalhammed et al., 2020). It is well-established that prebiotics can selectively promote the growth of probiotics. The underlying mechanism behind this growth promotion is the enzymatic hydrolysis of the non-digestible polysaccharides, followed by the uptake of the hydrolysis products, or even the direct uptake of small oligomers (Huynh et al., 2017; Nunpan et al., 2019; Massa et al., 2020). Thus, one of the prerequisites of a synbiotic should be the ability of the probiotic bacteria to ferment the prebiotic components. This ability of the probiotic bacteria is highly specific and is not only species and strain-dependent but also is markedly influenced by the dose, composition, and origin of the prebiotic (de Melo Pereira et al., 2018; Zoumpopoulou et al., 2018). Initially, we employed in vitro approach to monitor the growth of the probiotic lactobacilli in the presence of the different doses of the plant-based prebiotics, with the aim to select interesting combination(s) for further verification under in vivo conditions. Our results showed that when the two probiotic strains were tested in the presence of the two different plant extracts, a clear increase in the growth rate was achieved by both the strains, however, the growth promotion effect of the plant extracts on the tested probiotic strains was dose-dependent. In the case of the artichoke extract, it was the dose of 100% that resulted in higher growth rates of the L. acidophilus and L. delbrueckii (see, Figures 1, 3) but in the case of the mushroom extract, it was at the dose of 50% where the probiotic strain exhibited maximum growth rate (Figures 2, 4). The observed increase in the growth rate of the probionts in response to the addition of artichoke extract in the medium could be attributed to the presence of inulin-type fructans, such as inulin, fructooligosaccharide, in the artichoke extract (Yaseen, 2018; Rattanakiat et al., 2020; Zhu et al., 2020). Fructans are one of the most known prebiotics that were shown to stimulate probiotics growth in several organisms, including fish (Li et al., 2019; Manikandan et al., 2020; Ghafarifarsani et al., 2021; Zimmermann et al., 2021). In an in vitro study conducted by Van Doan et al. (2015), it was noted that Jerusalem artichoke extract at 5% concentration caused maximum growth in the bacterial population of Lactobacillus plantarum, finding that is in line with that of ours. Similarly, the observed increase in the growth of each of the two probiotics cultured together with mushroom extract could be attributed to the prebiotic components, such as chitin, hemicellulose, β- and α-glucans, mannans, xylans, and galatians, which are widely available in mushrooms (Mirończuk-Chodakowska and Witkowska, 2020; Atila et al., 2021). The findings from our study are supported by several other studies. For instance, Sawangwan et al. (2018) showed that the ability of edible mushroom extract as a prebiotic had a positive effect on the growth of probiotics L. acidophilus. This finding was complemented by other studies that showed that extract of oyster mushroom, Pleurotus eryngii, could promote the growth of not only Lactobacillus spp. but also other important probiotics species, such as Bifidobacterium spp., and Enterococcus faecium (Synytsya et al., 2009; Van Doan et al., 2016). It is interesting to mention that the artichoke and mushroom extracts at a concentration lower than 75% or mushroom extract at a higher concentration of 100% did not result in improvement in the growth-promotion of the probiotic species under study with respect to the corresponding species cultured in the presence of glucose. Reduced bacterial growth at lower and higher concentrations may be related to unavailability of suitable nutrients and unoptimized pH or high levels of beta-glucan in the culture medium, which has a negative effect on the bacterial growth; therefore, the optimization of nutrient concentration is very important (Reischke et al., 2015; Meshram et al., 2016; Rojo-Cebreros et al., 2017). As beta-glucan is made up of glucose monomers, when taken with probiotics, the bonds between them are broken down by beta-gluconase enzymes. Also, on the other hand, high levels of glucose have inhibitory properties for bacterial growth; the reduction in bacterial growth at a concentration of 100% of the mushroom extract can be justified (Reischke et al., 2015).
Our results showed that both the probiotic species showed a preference for 50% mushroom extract as a source of prebiotics since their growth with mushroom extract resulted in higher growth rates than those obtained in the presence of 100% artichoke extract (Figure 5). There is evidence suggesting that different prebiotic compounds display a varying degree of fermentability and short-chain fatty production in the presence of a probiotic strain (Carlson et al., 2018; Wang et al., 2019). It has been described that prebiotic compounds with higher polymerization degree are relatively more difficult to ferment than similar prebiotics with lower one (Mueller et al., 2016; Langa et al., 2019; Méndez et al., 2019). The preference of the two probiotics under study for the mushroom extract, over the artichoke extract, could be explained by the variation in the composition of the prebiotic compounds between artichoke and mushroom extracts, with the latter being more fermentable. A significant decline of pH in the medium that contained mushroom extract and each of the two probiotics relative to that in the medium that contained artichoke extract and the probiotics supports our above explanation that mushroom extract was better fermented by the tested probiotics.
Having observed that the mushroom extract at a concentration of 50% caused the most prominent growth-promoting effect, when cultured with each of the tested probiotic species response, we, therefore, selected the indicated dose of the mushroom extract and combined it with La and/or Lb to develop synbiotics. We then validate their beneficial effects using the in vivo model organism, zebrafish focusing on the survival, growth, and reproductive performances of zebrafish. Feeding of a diet supplemented with Lb and mushroom extract (diet 6) in combination caused a positive influence on the weight gain percentage and SGR of zebrafish. During the rearing periods of 120days, the average fish weight gain percentage increased in the group fed diet 6 increased to 342 and 335%, respectively, relative to 243% for the control group. Accordingly, the group fed diet 6 and diet 7; both showed an increase in the SGR by a fold of 1.2. This resulted in an FCR that was significantly lower for the group fed a diet supplemented with Lb and mushroom extract (diet 6) or with La, Lb, and mushroom extract (diet 7) in combination. Our results also showed that the condition factor (K), which is an indicator of the fish general condition, remained within the optimum range during the entire experimental period, and this was reflected in the survival results as no significant mortality was observed due to feeding experimental diets. This result suggests that the selected Lactobacillus species and the mushroom extract (50%) either alone or in combination caused no harmful effect on the fish, at least in our described experimental condition, and hence can be considered safe for use in fish feed. The underlying rationale for the observed improvement in the growth of zebrafish in response to feeding the developed synbiotic-supplemented diet could be multifactorial. For instance, (i) improvement in the gut microbial flora, (ii) inhibition of the attachment and cloning of pathogenic microorganisms to the intestinal wall of the fish, (iii) and maintenance of beneficial microbial population in the gut, (iv) improvement in the feed intake and digestion, (v) production of enzymes, vitamins, and other beneficial substances for the host, and (vi) improvement in the immune status of the fish (Aftabgard et al., 2018; Ashouri et al., 2018; Mirghaed et al., 2018; Dawood et al., 2020; Kazuń et al., 2020; Yang et al., 2020; El-Nobi et al., 2021; Mohammadi Arani et al., 2021; Zhandalgarova et al., 2021). However, at present, this is mere speculation and warrants further research. To our knowledge, no report is available on the use of probiotics La or Lb in combination with mushroom extract as a synbiotic formulation compound in farmed fishes. However, the beneficial effects of the synbiotic formulation on zebrafish performance parameters including weight gain percentage, SGR FCR, and survival are in agreement with previous studies (Safari et al., 2018; Mohammadian et al., 2019; Priya et al., 2021). For example, a similar improvement in the growth performances was observed in a study with a commercial synbiotic that comprised of a probiotic Entercoccus faecium and fructooligosaccharide as a prebiotic in zebrafish (Nekoubin et al., 2012b).
The reproductive performances of organisms, including fish, are gated by the state of energy reserves in the organism, and that there is a balance between energy homeostasis and fertility (Gioacchini et al., 2010; Mohammadi Arani et al., 2021). The impact of energy status on the reproductive axis is conveyed through several neuroendocrine hormones and metabolic cues, such as kiss1, kiss2, leptin, and Cyp19a, whose nature and mechanisms of action have begun to be deciphered in fish (Carnevali et al., 2016; Doering et al., 2019; Mohammadi Arani et al., 2021; Shan et al., 2021). Cyp19a is the key steroidogenic enzyme that plays an important role in the conversion of androgens to estrogens in the sexual differentiation and reproductive cycles of vertebrates (Zheng et al., 2019; Doering et al., 2021). Over the past few years, evidence has been put forward to demonstrate that microbial supplements can improve the reproductive performances of fishes (for details see, Ahmadifard et al., 2019; Gabriel, 2019; Mohammadi Arani et al., 2021). For instance, in a study carried out on zebrafish, feeding of the probiotic Lactobacillus rhamnosus caused a significant effect on follicle development, production of ovulated eggs, and hatching rate (Gioacchini et al., 2010). In another study, zebrafish fed a diet supplemented with probiotic Pediococcus acidilactici exhibited a significant improvement in the gonado somatic index, fecundity, and hatching percentage. Interestingly, this increase in the reproductive performances of the fish was associated with a significant increase in the expression of the cyp19a gene (Mohammadi Arani et al., 2021). Similar results have also been obtained with a synbiotic product Biomin imbo, which improved several reproductive indices like fecundity, hatching rate, hatching time, and the rate of germinal vesicle breakdown in zebrafish (Nekoubin et al., 2012b) and fecundity in angelfish Pterophyllum scalare (Nekoubin et al., 2012a). In agreement with the above findings, our results also demonstrated a stimulatory role of the synbiotic comprising of La, Lb, and mushroom extract in combination (diet 7) on a set of key reproductive indices of zebrafish. It is worth highlighting that despite the improvement in the reproductive performances, the hatching percentage, and the survival rate of the fry were not influenced by the feeding of synbiotic. A possible explanation for the observed response could be attributed to the culture conditions that were maintained at the optimum level for the culture of the fish. Consistent with our findings, Rodriguez-Estrada et al. (2009) observed no significant effect of feeding rainbow trout for 12weeks with Enterococcus faecalis and MOS/PHB on the survival rate of the fish. Also, similar results were observed in Japanese flounder (Paralichthys olivaceus) when fed with Bacillus clausii and MOS/FOS (Ye et al., 2011).
The molecular mechanisms behind the stimulating effects of prebiotics, probiotics, and/or synbiotics on the reproductive performances in organisms are not yet fully known. However, in our study, we found a significant upregulation of the cyp19a gene in the ovaries of the fish fed synbiotic-supplemented diet, and this coincided well with the improvement of the reproductive indices. The stimulatory role of synbiotic on female zebrafish reproductive performances may be due to both the activation of the endocrine control described above and to the direct action of synbiotic-mediated cyp19a on the ovary. In this study, although the cyp19a gene induced by synbiotic appeared to improve the reproductive factors, the induction of other metabolic signals, such as kiss1, kiss2, and leptin by this product and their collective involvement in governing the reproduction process cannot be excluded. Further studies are required to substantiate this assumption by developing or using primers specific for these genes of interest.
In essence, the in vitro screening assay in the broth showed a preference of L. acidophilus and L. bulgaricus for mushroom extract as a prebiotic. A synbiotic formulation, developed with the selected combination of L. acidophilus, L. bulgaricus, and 50% mushroom extract, showed a positive influence on the growth and reproductive performances of the in vivo model organism zebrafish. Our findings also imply that the improvement in the reproductive indices was associated with the upregulation of a cyp19a gene. Overall results suggest that a combination of L. acidophilus, L. bulgaricus, and mushroom extract can be considered as a potential synbiotic for aquaculture species. Further research is warranted to unravel the specific mechanisms involved in this synbiotic effect and the validation of this formulation following nutritional trials in aquaculture species of economic importance.
Data Availability Statement
The raw data supporting the conclusions of this article will be made available by the authors, without undue reservation.
Ethics Statement
The animal study was conducted following the ethical guidelines set by the Gorgan University of Agriculture Sciences and Natural Resources, Iran.
Author Contributions
HZ conducted the experiment and executed most of the data processing and analysis. MS, SH, and HP participated in the designing of the experiments and data analysis and guided and supervised the work. HZ and KB wrote the bulk of the manuscript and critically edited by MS, SH, and HP. All authors contributed to the article and approved the submitted version.
Funding
This work was supported by the institutional PhD grant provided by the Gorgan University of Agriculture and Natural Resources, Iran.
Conflict of Interest
The authors declare that the research was conducted in the absence of any commercial or financial relationships that could be construed as a potential conflict of interest.
Publisher’s Note
All claims expressed in this article are solely those of the authors and do not necessarily represent those of their affiliated organizations, or those of the publisher, the editors and the reviewers. Any product that may be evaluated in this article, or claim that may be made by its manufacturer, is not guaranteed or endorsed by the publisher.
Acknowledgments
The authors are grateful to the technical staff of the Gorgan University of Agriculture and Natural Resources, Iran for their excellent technical assistance, and Baruah’s lab members, especially Parisa Norouzitallab, for their helpful discussions.
References
Abedalhammed, H. S., Hassan, S. M., Naser, A. S., Al-Maathedy, M. H., Abdulateef, S. M., and Mohammed, T. T. (2020). Effect of using Jerusalem artichoke (Helianthus tuberosus L.) and sprout germinated barley as a prebiotics to the diets of common carp (Cyprinus Carpio L.) fingerling on the rates of apparent digestible coefficient and apparent protein digestible. EurAsian J. BioSci. 14, 3741–3745.
Abedian-Amiri, A., Azari Takami, G. H., Afsharnasab, M., and Razavilar, V. (2017). The comparative effects of dietary supplementation with Pediococcus acidilactici and enterococcus faecium on feed utilization, various health-related characteristics and yersiniosis in rainbow trout (Oncorhynchus mykiss walbaum, 1972). Iran. J. Fish. Sci. 16, 753–773. doi: 10.22092/ijfs.2018.114695
Aftabgard, M., Salarzadeh, A., Mohseni, M., Shabanipour, A. H. B., and Zorriehzahra, M. E. J. (2018). A survey of the functional, gut digestive, and serum antioxidant factors in Salmo trutta caspius (Kessler) fingerlings with the application of a dietary synbiotic. Fish. Aquat. Life 26, 31–38. doi: 10.2478/aopf-2018-0004
Ahmadifard, N., Aminlooi, V. R., Tukmechi, A., and Agh, N. (2019). Evaluation of the impacts of long-term enriched Artemia with Bacillus subtilis on growth performance, reproduction, intestinal microflora, and resistance to Aeromonas hydrophila of ornamental fish Poecilia latipinna. Probiotics Antimicrob. Proteins 11, 957–965. doi: 10.1007/s12602-018-9453-4
Ahmed, M., Abdullah, N., Shuib, A. S., Razak, S. A., and February, I. (2017). Influence of rawpolysaccharide extract from mushroom stalk waste on growth and pH perturbationinduced-stress in Nile tilapia, Oreochromis niloticus. Aquaculture 468, 60–70. doi: 10.1016/j.aquaculture.2016.09.043
Aida, F. M. N. A., Shuhaimi, M., Yazid, M., and Maaruf, A. G. (2009). Mushroom as a potential source of prebiotics: a review. Trends Food Sci. Technol. 20, 567–575. doi: 10.1016/j.tifs.2009.07.007
Akbari Nargesi, E., Falahatkar, B., and Sajjadi, M. M. (2020). Dietary supplementation of probiotics and influence on feed efficiency, growth parameters and reproductive performance in female rainbow trout (Oncorhynchus mykiss) broodstock. Aquac. Nutr. 26, 98–108. doi: 10.1111/anu.12970
Allameh, S. K., Ringø, E., Yusoff, F. M., Daud, H. M., and Ideris, A. (2015). Dietary supplement of enterococcus faecalis on digestive enzyme activities, short-chain fatty acid production, immune system response and disease resistance of Javanese carp (Puntinus gonionotus, Bleeker 1850). Aquac. Nutr. 23, 331–338. doi: 10.1111/anu.12397
Al-Maadhedy, A. T. A., Nafea, H. H., and Alubaydi, T. S. M. (2021). Effect of partial replacement of palm kernel powder, oyster mushrooms and commercial enzyme instead of corn in the diets on the blood traits of common carp fingerling fish (Cyprinus carpio L.). IOP Conf. Ser.: Earth Environ. Sci. 761:012091. doi: 10.1088/1755-1315/761/1/012091
Amenyogbe, E., Chen, G., Wang, Z., Huang, J., Huang, B., and Li, H. (2020). The exploitation of probiotics, prebiotics and synbiotics in aquaculture: present study, limitations and future directions: a review. Aquac. Int. 28, 1017–1041. doi: 10.1007/s10499-020-00509-0
Amiri, O., Miandare, H. K., Hoseinifar, S. H., Shabni, A., and Safari, R. (2018). Skin mucus protein profile, immune parameters, immune-related gene expression, and growth performance of rainbow trout (Oncorhynchus mykiss) fed white button mushroom (Agaricus bisporus) powder. Int. J. Med. Mushrooms 20, 337–347. doi: 10.1615/IntJMedMushrooms.2018025825
Ansari, T., and Jadhav, R. (2021). Assessment of dry weight protein content in fruit body and stipe of Agaricus bisporus using it as a fish feed to assess growth of. Uttar Pradesh J. Zool. 42, 31–37.
Ashouri, G., Soofiani, N. M., Hoseinifar, S. H., Jalali, S. A. H., Morshedi, V., Van Doane, H., et al. (2018). Combined effects of dietary low molecular weight sodium alginate and Pediococcus acidilactici MA18/5M on growth performance, haematological and innate immune responses of Asian sea bass (Lates calcalifer) juveniles. Fish Shellfish Immunol. 79, 34–41. doi: 10.1016/j.fsi.2018.05.009
Atila, F., Owaid, M. N., and Shariati, M. A. (2021). The nutritional and medical benefits of Agaricus bisporus: a review. J. Microbiol. Biotechnol. Food Sci. 7, 281–286. doi: 10.15414/jmbfs.2017/18.7.3.281-286
Aydin, F., and Şehriban, Ç. Y. (2019). Effect of probiotics on reproductive performance of fish. NESciences 4, 153–162. doi: 10.28978/nesciences.567113
Banu, M. R., Akter, S., Islam, M. R., Mondol, M. N., and Hossain, M. A. (2020). Probiotic yeast enhanced growth performance and disease resistance in freshwater catfish gulsa tengra, Mystus cavasius. Aquac. Rep. 16:100237. doi: 10.1016/j.aqrep.2019.100237
Bharathi, S., Antony, C., Cbt, R., Arumugam, U., Ahilan, B., and Aanand, S. (2019). Functional feed additives used in fish feeds. Int. J. Fish. Aquat. Stud. 7, 44–52.
Boonanuntanasarn, S., Tiengtam, N., Pitaksong, T., Piromyou, P., and Teaumroong, N. (2018). Effects of dietary inulin and Jerusalem artichoke (Helianthus tuberosus) on intestinal microbiota community and morphology of Nile tilapia (Oreochromis niloticus) fingerlings. Aquac. Nutr. 24, 712–722. doi: 10.1111/anu.12600
Cámara-Ruiz, M., Balebona, M. C., Moriñigo, M. Á., and Esteban, M. Á. (2020). Probiotic Shewanella putrefaciens (SpPdp11) as a fish health modulator: a review. Microorganisms 8:1990. doi: 10.3390/microorganisms8121990
Carlson, J. L., Erickson, J. M., Lloyd, B. B., and Slavin, J. L. (2018). Health effects and sources of prebiotic dietary fiber. Curr. Dev. Nutr. 2, 1–8. doi: 10.1093/cdn/nzy005
Carnevali, O., Maradona, F., and Gioacchini, G. (2016). Integrated control of fish metabolism, wellbeing and reproduction: the role of probiotic. Aquaculture 472, 144–155. doi: 10.1016/j.aquaculture.2016.03.037
Cid García, R. A., Hernández Hernández, L. H., Carrillo Longoria, J. A., and Fernández Araiza, M. A. (2020). Inclusion of yeast and/or fructooligosaccharides in diets with plant-origin protein concentrates for rainbow trout (Oncorhynchus mykiss) fingerlings. J. World Aquac. Soc. 51, 970–981. doi: 10.1111/jwas.12661
Das, A. K., Nanda, P. K., Dandapat, P., Bandyopadhyay, S., Gullón, P., Sivaraman, G. K., et al. (2021). Edible mushrooms as functional ingredients for development of healthier and more sustainable muscle foods: a flexitarian approach. Molecules 26:2463. doi: 10.3390/molecules26092463
Dawood, M. A., Eweedah, N. M., El-Sharawy, M. E., Awad, S. S., Van Doan, H., and Paray, B. A. (2020). Dietary white button mushroom improved the growth, immunity, antioxidative status and resistance against heat stress in Nile tilapia (Oreochromis niloticus). Aquaculture 523:735229. doi: 10.1016/j.aquaculture.2020.735229
de Melo Pereira, G. V., de Oliveira Coelho, B., Júnior, A. I. M., Thomaz-Soccol, V., and Soccol, C. R. (2018). How to select a probiotic? A review and update of methods and criteria. Biotechnol. Adv. 36, 2060–2076. doi: 10.1016/j.biotechadv.2018.09.003
Doering, J. A., Villeneuve, D. L., Fay, K. A., Randolph, E. C., Jensen, K. M., Kahl, M. D., et al. (2019). Differential sensitivity to in vitro inhibition of cytochrome P450 aromatase (CYP19) activity among 18 freshwater fishes. Toxicol. Sci. 170, 394–403. doi: 10.1093/toxsci/kfz115
Doering, J. A., Villeneuve, D. L., Tilton, C. B., Kittelson, A. R., Blackwell, B. R., Kahl, M. D., et al. (2021). Assessing effects of aromatase inhibition on fishes with group-synchronous oocyte development using western mosquitofish (Gambusia affinis) as a model. Aquat. Toxicol. 232:105741. doi: 10.1016/j.aquatox.2020.105741
EFSA FEEDAP Rychen, G., Aquilina, G., Azimonti, G., Bampidis, V., Bastos, M. L., et al. (2018). Guidance on the characterisation of microorganisms used as feed additives or as production organisms. EFSA J. 16:5206. doi: 10.2903/j.efsa.2018.5206
El-Nobi, G., Hassanin, M., Khalil, A. A., Mohammed, A. Y., Amer, S. A., Montaser, M. M., et al. (2021). Synbiotic effects of Saccharomyces cerevisiae, mannan oligosaccharides, and β-glucan on innate immunity, antioxidant status, and disease resistance of Nile tilapia, Oreochromis niloticus. Antibiotics 10:567. doi: 10.3390/antibiotics10050567
FAO (2007). Technical Meeting on Prebiotics: Food Quality and Standards Service (AGNS). FAO Technical Meeting Report, FAO. Rome, Italy, 15–16 September 2007.
Gabriel, N. N. (2019). Review on the progress in the role of herbal extracts in tilapia culture. Cogent Food Agric. 5:1619651. doi: 10.1080/23311932.2019.1619651
Ghafarifarsani, H., Rashidian, G., Bagheri, T., Hoseinifar, S. H., and Van Doan, H. (2021). Study on growth enhancement and the protective effects of dietary prebiotic inulin on immunity responses of rainbow trout (Oncorhynchus mykiss) fry infected with Aeromonas hydrophila. Ann. Anim. Sci. 21, 543–559. doi: 10.2478/aoas-2020-0074
Gioacchini, G., Maradonna, F., Lombardo, F., Bizzaro, D., Olivotto, I., and Carnevali, O. (2010). Increase of fecundity by probiotic administration in zebrafish (Danio rerio). Reproduction 140, 953–959. doi: 10.1530/REP-10-0145
Guluarte, C., Reyes-Becerril, M., Gonzalez-Silvera, D., Cuesta, A., Angulo, C., and Esteban, M. Á. (2019). Probiotic properties and fatty acid composition of the yeast Kluyveromyces lactis M3. In vivo immunomodulatory activities in gilthead seabream (Sparus aurata). Fish Shellfish Immunol. 94, 389–397. doi: 10.1016/j.fsi.2019.09.024
Habib, S. S., Naz, S., Khalid, S., Kanwal, R., Ameer, I., Khan, S. N. A., et al. (2021). Effect of white button mushroom (Agaricus bisporus) on immunity and haematological parameters of Oreochromis niloticus. Pakistan J. Zool. 7, 1–4. doi: 10.3390/jof7060427
Haj-Mustafa, M., Abdi, R., Sheikh-Zeinoddin, M., and Soleimanian-Zad, S. (2015). Statistical study on fermentation conditions in the optimization of exopolysaccharide production by lactobacillus rhamnosus 519 in skimmed milk base media. Biocatal. Agric. Biotechnol. 4, 521–527. doi: 10.1016/j.bcab.2015.08.013
Harborne, J. B. (1980). “Plant phenolics,” in Secondary Plant Products: Encyclopedia of Plant Physiology, New Series. Vol. 8. eds. E. A. Bell and B. V. Charlwood (New York: Springer–Verlag), 329–402.
Harikrishnan, R., Devi, G., Van Doan, H., Balasundaram, C., Thamizharasan, S., Hoseinifar, S. H., et al. (2021). Effect of diet enriched with Agaricus bisporus polysaccharides (ABPs) on antioxidant property, innate-adaptive immune response and pro-anti inflammatory genes expression in Ctenopharyngodon idella against Aeromonas hydrophila. Fish Shellfish Immunol. 114, 238–252. doi: 10.1016/j.fsi.2021.04.025
Harikrishnan, R., Naafar, A., Musthafa, M. S., Ahamed, A., Arif, I. A., and Balasundaram, C. (2018). Effect of Agaricus bisporus enriched diet on growth, hematology, and immune protection in Clarias gariepinus against Flavobacterium columnare. Fish Shellfish Immunol. 73, 245–251. doi: 10.1016/j.fsi.2017.12.024
Harris, S., Monteagudo-Mera, A., Kosik, O., Charalampopoulos, D., Shewry, P., and Lovegrove, A. (2019). Comparative prebiotic activity of mixtures of cereal grain polysaccharides. AMB Express 9:203. doi: 10.1186/s13568-019-0925-z
Hasan, K. N., and Banerjee, G. (2020). Recent studies on probiotics as beneficial mediator in aquaculture: a review. J. Basic Appl. Zool. 81, 1–16. doi: 10.1186/s41936-020-00190-y
Hasan, M. T., Je Jang, W., Lee, J. M., Lee, B. J., Hur, S. W., Gu Lim, S., et al. (2019). Effects of immunostimulants, prebiotics, probiotics, synbiotics, and potentially immunoreactive feed additives on olive flounder (Paralichthys olivaceus): a review. Rev. Fish. Sci. Aquac. 27, 417–437. doi: 10.1080/23308249.2019.1622510
Hasyimi, W., Widanarni, W., and Yuhana, M. (2020). Growth performance and intestinal microbiota diversity in pacific white shrimp Litopenaeus vannamei fed with a probiotic bacterium, honey prebiotic, and synbiotic. Curr. Microbiol. 77, 2982–2990. doi: 10.1007/s00284-020-02117-w
Hoseinifar, S. H., Hosseini, M., Paknejad, H., Safari, R., Jafar, A., Yousefi, M., et al. (2019a). Enhanced mucosal immune responses, immune related genes and growth performance in common carp (Cyprinus carpio) juveniles fed dietary Pediococcus acidilactici MA18/5M and raffinose. Dev. Comp. Immunol. 94, 59–65. doi: 10.1016/j.dci.2019.01.009
Hoseinifar, S. H., Mirvaghefi, A., Amoozegar, A., Merrifield, D., and Ringo, E. (2017). In vitro selection of a synbiotic and in vivo evaluation on intestinal microbiota, performance and physiological response of rainbow trout (Oncorhynchus mykiss) fingerlings. Aquac. Nutr. 23, 111–118. doi: 10.1111/anu.12373
Hoseinifar, S. H., Roosta, Z., Hajimoradloo, A., and Vakili, F. (2015). The effects of lactobacillus acidophilus as feed supplement on skin mucosal immune parameters, intestinal microbiota, stress resistance and growth performance of black swordtail (Xiphophorus helleri). Fish Shellfish Immunol. 42, 533–538. doi: 10.1016/j.fsi.2014.12.003
Hoseinifar, S. H., Zou, H. K., Paknejad, H., Hajimoradloo, A., and Van Doan, H. (2019b). Effects of dietary white-button mushroom powder on mucosal immunity, antioxidant defence, and growth of common carp (Cyprinus carpio). Aquaculture 501, 448–454. doi: 10.1016/j.aquaculture.2018.12.007
Hosseini, M., Miandare, H. K., Hoseinifar, S. H., and Yarahmadi, P. (2016). Dietary lactobacillus acidophilus modulated skin mucus protein profile, immune and appetite genes expression in gold fish (Carassius auratus gibelio). Fish Shellfish Immunol. 59, 149–154. doi: 10.1016/j.fsi.2016.10.026
Huynh, T. G., Shiu, Y. L., Nguyen, T. P., Truong, Q. P., Chen, J. C., and Liu, C. H. (2017). Current applications, selection, and possible mechanisms of actions of synbiotics in improving the growth and health status in aquaculture: a review. Fish Shellfish Immunol. 64, 367–382. doi: 10.1016/j.fsi.2017.03.035
Jia, C., Zhu, X., Meng, X., and Yi, Y. (2020). Effects of Jerusalem artichoke powder on the growth performance, nonspecific immunity, and gastrointestinal microbiota of sea cucumber. J. World Aquac. Soc. 51, 200–213. doi: 10.1111/jwas.12633
Kask, S., Adamberg, K., Orłowski, A., Vogensen, F. K., Møller, P. L., Ardö, Y., et al. (2003). Physiological properties of lactobacillus paracasei, L. danicus and L. curvatus strains isolated from Estonian semi-hard cheese. Int. Food Res. J. 36, 1037–1046. doi: 10.1016/j.foodres.2003.08.002
Kazuń, B., Małaczewska, J., Kazuń, K., Kamiński, R., Adamek-Urbańska, D., and Żylińska-Urban, J. (2020). Dietary administration of β-1, 3/1, 6-glucan and lactobacillus plantarum improves innate immune response and increases the number of intestine immune cells in roach (Rutilus rutilus). BMC Vet. Res. 16:216. doi: 10.1186/s12917-020-02432-1
Khangwal, I., and Shukla, P. (2019). Potential prebiotics and their transmission mechanisms: recent approaches. J. Food Drug Anal. 27, 649–656. doi: 10.1016/j.jfda.2019.02.003
Kishawy, A. T., Sewid, A. H., Nada, H. S., Kamel, M. A., El-Mandrawy, S. A., Abdelhakim, T., et al. (2020). Mannanoligosaccharides as a carbon source in Biofloc boost dietary plant protein and water quality, growth, immunity and Aeromonas hydrophila resistance in Nile tilapia (Oreochromis niloticus). Animals 10:1724. doi: 10.3390/ani10101724
Kong, Y., Gao, C., Du, X., Zhao, J., Li, M., Shan, X., et al. (2020). Effects of single or conjoint administration of lactic acid bacteria as potential probiotics on growth, immune response and disease resistance of snakehead fish (Channa argus). Fish Shellfish Immunol. 102, 412–421. doi: 10.1016/j.fsi.2020.05.003
Kong, Y., Li, M., Chu, G., Liu, H., Shan, X., Wang, G., et al. (2021). The positive effects of single or conjoint administration of lactic acid bacteria on Channa argus: digestive enzyme activity, antioxidant capacity, intestinal microbiota and morphology. Aquaculture 531:735852. doi: 10.1016/j.aquaculture.2020.735852
Kuebutornye, F. K., Tang, J., Cai, J., Yu, H., Wang, Z., Abarike, E. D., et al. (2020). In vivo assessment of the probiotic potentials of three host-associated bacillus species on growth performance, health status and disease resistance of Oreochromis niloticus against Streptococcus agalactiae. Aquaculture 527:735440. doi: 10.1016/j.aquaculture.2020.735440
Kumar, R., Ali, N., Siddique, R. A., Sahu, D. S., Fahim, A., Singh, R., et al. (2021). Effect of different levels of mushroom powder (Agaricus bisporus) and probiotics (Saccharomyces cerevisiae) on carcass traits and hematological responses of broiler chickens. J. Entomol. Zool. Stud. 9, 244–248. doi: 10.22271/j.ento.2021.v9.i2d.8487
Kumar, P., Jain, K. K., Sardar, P., Jayant, M., and Tok, N. C. (2018). Effect of dietary synbiotic on growth performance, body composition, digestive enzyme activity and gut microbiota in Cirrhinus mrigala (ham.) fingerlings. Aquac. Nutr. 24, 921–929. doi: 10.1111/anu.12628
Langa, S., Van Den Bulck, E., Peirotén, A., Gaya, P., Schols, H. A., and Arqués, J. L. (2019). Application of lactobacilli and prebiotic oligosaccharides for the development of a synbiotic semi-hard cheese. LWT 114:108361. doi: 10.1016/j.lwt.2019.108361
Li, Z., Tran, N. T., Ji, P., Sun, Z., Wen, X., and Li, S. (2019). Effects of prebiotic mixtures on growth performance, intestinal microbiota and immune response in juvenile chu's croaker, Nibea coibor. Fish Shellfish Immunol. 89, 564–573. doi: 10.1016/j.fsi.2019.04.025
Lin, S., Mao, S., Guan, Y., Luo, L., Luo, L., and Pan, Y. (2012). Effects of dietary chitosan oligosaccharides and Bacillus coagulans on the growth, innate immunity and resistance of koi (Cyprinus carpio koi). Aquaculture 342, 36–41. doi: 10.1016/j.aquaculture.2012.02.009
Livak, K. J., and Schmittgen, T. D. (2001). Analysis of relative gene expression data using real-time quantitative PCR and the 2−ΔΔCt method. Methods 25, 402–408. doi: 10.1006/meth.2001.1262
Mahore, J. G., and Shirolkar, S. V. (2018). Investigation of effect of ripening and processing on prebiotic potential of banana. J. Young Pharm. 10, 409–413. doi: 10.5530/jyp.2018.10.90
Manikandan, K., Felix, N., Ahilan, B. S., and Anthony, C. (2020). The fructans: a valuable and functional additive as a prebiotic in promoting the disease prevention in modern aquaculture. J. Aquacult. Trop. 35, 67–79. doi: 10.32381/JAT.2020.35.1-4.7
Massa, N. M. L., Menezes, F. N. D. D., de Albuquerque, T. M. R., de Oliveira, S. P. A., dos Santos Lima, M., Magnani, M., et al. (2020). Effects of digested jabuticaba (Myrciaria Jaboticaba (Vell.) berg) by-product on growth and metabolism of lactobacillus and Bifidobacterium indicate prebiotic properties. LWT 131:109766. doi: 10.1016/j.lwt.2020.109766
Méndez, I., Rodríguez-Prado, M., Cuñé, J., Espadaler, J., and Farran-Codina, A. (2019). Effect of the degree of polymerization of fructans on ex vivo fermented human gut microbiome. Nutrients 11:1293. doi: 10.3390/nu11061293
Mensink, M. A., Frijlink, H. W., van der Voort Maarschalk, K., and Hinrichs, W. L. (2015). Inulin, a flexible oligosaccharide I: review of its physicochemical characteristics. Carbohydr. Polym. 130, 405–419. doi: 10.1016/j.carbpol.2015.05.026
Meshram, S. J., Murthy, H. S., Swain, H. S., Pai, R., and Ali, A. (2016). Preliminary study of the effect of dietary beta-glucan on the gut morphology of giant freshwater prawn, macrobrachium Rosenbergii. J. Aquac. Mar. Biol. 4:90. doi: 10.15406/jamb.2016.04.00090
Miandare, H. K., Mirghaed, A. T., Hosseini, M., Mazloumi, N., Zargar, A., and Nazari, S. (2019). Corrigendum to “dietary immunogen® modulated digestive enzyme activity and immune gene expression in litopenaeus vannamei post larvae” [fish and shellfish immunol. 70 (2017) 621-627]. Fish Shellfish Immunol. 93:1111. doi: 10.1016/j.fsi.2019.07.005
Mirghaed, A. T., Yarahmadi, P., Hosseinifar, S. H., Tahmasebi, D., Gheisvandi, N., and Ghaedi, A. (2018). The effects singular or combined administration of fermentable fiber and probiotic on mucosal immune parameters, digestive enzyme activity, gut microbiota and growth performance of Caspian white fish (Rutilus frisii kutum) fingerlings. Fish Shellfish Immunol. 77, 194–199. doi: 10.1016/j.fsi.2018.02.007
Mirończuk-Chodakowska, I., and Witkowska, A. M. (2020). Evaluation of polish wild mushrooms as beta-glucan sources. Int. J. Environ. Res. Public Health 17:7299. doi: 10.3390/ijerph17197299
Mohammadi Arani, M., Salati, A. P., Keyvanshokooh, S., and Safari, O. (2021). The effect of Pediococcus acidilactici on mucosal immune responses, growth, and reproductive performance in zebrafish (Danio rerio). Fish Physiol. Biochem. 47, 153–162. doi: 10.1007/s10695-020-00903-8
Mohammadi Arani, M., Salati, A. P., Safari, O., and Keyvanshokooh, S. (2019). Dietary supplementation effects of Pediococcus acidilactici as probiotic on growth performance, digestive enzyme activities and immunity response in zebra fish (Danio rerio). Aquac. Nutr. 25, 854–861. doi: 10.1111/anu.12904
Mohammadian, T., Nasirpour, M., Tabandeh, M. R., and Mesbah, M. (2019). Synbiotic effects of β-glucan, mannan oligosaccharide and lactobacillus casei on growth performance, intestine enzymes activities, immune-hematological parameters and immune-related gene expression in common carp, Cyprinus carpio: an experimental infection with Aeromonas hydrophila. Aquaculture 511:634197. doi: 10.1016/j.aquaculture.2019.06.011
Montazeri-Parchikolaei, H., Abedian-Kenari, A., and Esmaeili, M. (2021). Soya bean-based diets plus probiotics improve the profile of fatty acids, digestibility, intestinal microflora, growth performance and the innate immunity of beluga (Huso huso). Aquac. Res. 52, 152–166. doi: 10.1111/are.14877
Mora-Sánchez, B., Balcázar, J. L., and Pérez-Sánchez, T. (2020). Effect of a novel postbiotic containing lactic acid bacteria on the intestinal microbiota and disease resistance of rainbow trout (Oncorhynchus mykiss). Biotechnol. Lett. 42, 1957–1962. doi: 10.1007/s10529-020-02919-9
Moustafa, E. M., Saad, T. T., Khalil, R. H., Dawood, M. A. O., and Lolo, E. E. (2020). The ameliorative role of synbiotic culture techniques application in white shrimp (Litopenaeus vannamei) during nursery stage. Adv. Anim. Vet. Sci. 8, 260–277. doi: 10.17582/journal.aavs/2020/8.3.260.277
Mueller, M., Reiner, J., Fleischhacker, L., Viernstein, H., Loeppert, R., and Praznik, W. (2016). Growth of selected probiotic strains with fructans from different sources relating to degree of polymerization and structure. J. Funct. Foods 24, 264–275. doi: 10.1016/j.jff.2016.04.010
Nekoubin, H., Hatefi, S., Javahery, S., and Sudagar, M. (2012a). Effects of synbiotic (biomin Imbo) on growth performance, survival rate, reproductive parameters of angelfish (Pterophyllum scalare). Walailak J. Sci. Technol. 9, 327–332.
Nekoubin, H., Javaheri, S., and Imanpour, M. R. (2012b). Effects of synbiotic (biomin imbo) on fecundity and reproductive factors of zebrafish (Danio rerio). WJFMS 4, 65–67. doi: 10.1007/s00580-015-2071-6
Nunpan, S., Suwannachart, C., and Wayakanon, K. (2019). Effect of prebiotics-enhanced probiotics on the growth of Streptococcus mutans. Int. J. Microbiol. 2019:4623807. doi: 10.1155/2019/4623807
Okolie, C. L., Rajendran, S. R. C. K., Udenigwe, C. C., Aryee, A. N. A., and Mason, B. (2017). Prospects of brown seaweed polysaccharides (BSP) as prebiotics and potential immunomodulators. J. Food Biochem. 41:e12392. doi: 10.1111/jfbc.12392
Pietrzak, E., Mazurkiewicz, J., and Slawinska, A. (2020). Innate immune responses of skin mucosa in common carp (Cyprinus carpio) fed a diet supplemented with galactooligosaccharides. Animals 10:438. doi: 10.3390/ani10030438
Priya, P. S., Ashwitha, A., Thamizharasan, K., Harishkumar, M., Dinesh, S., Nithya, T. G., et al. (2021). Synergistic effect of durian fruit rind polysaccharide gel encapsulated prebiotic and probiotic dietary supplements on growth performance, immune-related gene expression, and disease resistance in Zebrafish (Danio rerio). Heliyon 7:e06669. doi: 10.1016/j.heliyon.2021.e06669
Ramos, M., Burgos, N., Barnard, A., Evans, G., Preece, J., Graz, M., et al. (2019). Agaricus bisporus and its by-products as a source of valuable extracts and bioactive compounds. Food Chem. 292, 176–187. doi: 10.1016/j.foodchem.2019.04.035
Rathore, H., Prasad, S., and Sharma, S. (2017). Mushroom nutraceuticals for improved nutrition and better human health: a review. PharmaNutrition 5, 35–46. doi: 10.1016/j.phanu.2017.02.001
Rattanachan, S., Bunnajirakul, S., and Punyadarsaniya, D. (2020). Effect of pre-supplementation with Pleurotus sajor-caju crude extracts on body weight and consequence responses of leukocytes and immune organs in fancy carp following inoculation with Aeromonas veronii. Vet. World 13:1010. doi: 10.14202/vetworld.2020.1010-1016
Rattanakiat, S., Pulbutr, P., Khunawattanakul, W., Sungthong, B., and Saramunee, K. (2020). Prebiotic activity of polysaccharides extracted from Jerusalem artichoke tuber and development of prebiotic granules. Pharmacogn. Mag. 12, 1402–1411. doi: 10.5530/pj.2020.12.194
Reischke, S., Kumar, M. G. K., and Bååth, E. (2015). Threshold concentration of glucose for bacterial growth in soil. Soil Biol. Biochem. 80, 218–223. doi: 10.1016/j.soilbio.2014.10.012
Ringø, E., Bendiksen, H. R., Wesmajervi, M. S., Olsen, R. E., Jansen, P. A., and Mikkelsen, H. (2020a). Lactic acid bacteria associated with the digestive tract of Atlantic salmon (Salmo salar L.). J. Appl. Microbiol. 89, 317–322. doi: 10.1046/j.1365-2672.2000.01116.x
Ringø, E., Olsen, R. E., Gifstad, T. Ø., Dalmo, R. A., Amlund, H., Hemre, G. I., et al. (2010). Prebiotics in aquaculture: a review. Aquac. Nutr. 16, 117–136. doi: 10.1111/j.1365-2095.2009.00731.x
Ringø, E., Van Doan, H., Lee, S. H., Soltani, M., Hoseinifar, S. H., Harikrishnan, R., et al. (2020b). Probiotics, lactic acid bacteria and bacilli: interesting supplementation for aquaculture. J. Appl. Microbiol. 129, 116–136. doi: 10.1111/jam.14628
Rodriguez-Estrada, U., Satoh, S., Haga, Y., Fushimi, H., and Sweetman, J. (2009). Effects of single and combined supplementation of enterococcus faecalis, mannanoligosaccharide and polyhydrobutyric acid on growth performance and immune response of rainbow trout Oncorhynchus mykiss. Aquac. Sci. 57, 609–617. doi: 10.11233/aquaculturesci.57.609
Rojo-Cebreros, A. H., Ibarra-Castro, L., Martínez-Brown, J. M., Velasco-Blanco, G., Martínez-Téllez, M. A., Medina-Jasso, M. A., et al. (2017). “Potential of nannochloropsis in beta glucan production,” in Nannochloropsis: Biology, Biotechnological Potential and Challenges eds. M. Jan and P. Kazik (New York, NY, USA: Nova Science Publishers, Inc.), 181–225.
Safari, R., Hoseinifar, S. H., Dadar, M., and Khalili, M. (2018). Powder of the white bottom mushroom, Agaricus bisporus (Agaricomycetes), improved immunomodulatory and health-promoting effects of lactobacillus casei in Zebrafish (Danio rerio). Int. J. Med. Mushrooms 20, 695–704. doi: 10.1615/IntJMedMushrooms.2018026551
Safari, R., Hoseinifar, S. H., Nejadmoghaddam, S., and Dadar, M. (2019). Combined administration of the white button mushroom Agaricus bisporus (agaricomycetes) and lactobacillus casei modulated immune-related gene expression and mucosal and serum immune parameters in a goldfish (Carassius auratus) model. Int. J. Med. Mushrooms 21, 503–513. doi: 10.1615/IntJMedMushrooms.2019029551
Sawangwan, T., Wansanit, W., Pattani, L., and Noysang, C. (2018). Study of prebiotic properties from edible mushroom extraction. Agric. Nat. Resour. 52, 519–524. doi: 10.1016/j.anres.2018.11.020
Sewaka, M., Trullas, C., Chotiko, A., Rodkhum, C., Chansue, N., Boonanuntanasarn, S., et al. (2019). Efficacy of synbiotic Jerusalem artichoke and lactobacillus rhamnosus GG-supplemented diets on growth performance, serum biochemical parameters, intestinal morphology, immune parameters and protection against Aeromonas veronii in juvenile red tilapia (Oreochromis spp.). Fish Shellfish Immunol. 86, 260–268. doi: 10.1016/j.fsi.2018.11.026
Shabirah, A., Mulyani, Y., and Lili, W. (2019). Effect of types isolated lactic acid bacteria on hematocrit and differential leukocytes fingerling common carp (Cyprinus carpio L.) infected with Aeromonas hydrophila bacteria. World News Nat. Sci. 24, 22–35.
Shan, B., Liu, Y., Yang, C., Zhao, Y., and Sun, D. (2021). Comparative transcriptomic analysis for identification of candidate sex-related genes and pathways in crimson seabream (Parargyrops edita). Sci. Rep. 11, 1–12. doi: 10.1038/s41598-020-80282-5
Sobye, A., Knop Lund, M. L., and Fojan, P. (2018). Bioproduction of Natural Carotenoids by Dietzia Maris and Rhodococcus opacus. (Master Thesis). Denmark: Aalborg University, 85.
Sribounoy, U., Pirarat, N., Solval, K. M., Sathivel, S., and Chotiko, A. (2021). Development of pelleted feed containing probiotic lactobacillus rhamnosus GG and Jerusalem artichoke for Nile tilapia and its biocompatibility studies. 3 Biotech 11:279. doi: 10.1007/s13205-021-02829-1
Suez, J., Zmora, N., Segal, E., and Elinav, E. (2019). The pros, cons, and many unknowns of probiotics. Nat. Med. 25, 716–729. doi: 10.1038/s41591-019-0439-x
Synytsya, A., Míčková, K., Synytsya, A., Jablonský, I., Spěváček, J., Erban, V., et al. (2009). Glucans from fruit bodies of cultivated mushrooms Pleurotus ostreatus and Pleurotus eryngii: structure and potential prebiotic activity. Carbohydr. Polym. 76, 548–556. doi: 10.1016/j.carbpol.2008.11.021
Tan, H. Y., Chen, S. W., and Hu, S. Y. (2019). Improvements in the growth performance, immunity, disease resistance, and gut microbiota by the probiotic Rummeliibacillus stabekisii in Nile tilapia (Oreochromis niloticus). Fish Shellfish Immunol. 92, 265–275. doi: 10.1016/j.fsi.2019.06.027
Tarnecki, A. M., Wafapoor, M., Phillips, R. N., and Rhody, N. R. (2019). Benefits of a bacillus probiotic to larval fish survival and transport stress resistance. Sci. Rep. 9:4892. doi: 10.1038/s41598-019-39316-w
Tian, Y., Zeng, H., Xu, Z., Zheng, B., Lin, Y., Gan, C., et al. (2012). Ultrasonic-assisted extraction and antioxidant activity of polysaccharides recovered from white button mushroom (Agaricus bisporus). Carbohydr. Polym. 88, 522–529. doi: 10.1016/j.carbpol.2011.12.042
Tiengtam, N., Paengkoum, P., Sirivoharn, S., Phonsiri, K., and Boonanuntanasarn, S. (2017). The effects of dietary inulin and Jerusalem artichoke (Helianthus tuberosus) tuber on the growth performance, haematological, blood chemical and immune parameters of Nile tilapia (Oreochromis niloticus) fingerlings. Aquac. Res. 48, 5280–5288. doi: 10.1111/are.13341
Usman, M., Murtaza, G., and Ditta, A. (2021). Nutritional, medicinal, and cosmetic value of bioactive compounds in button mushroom (Agaricus bisporus): a review. Appl. Sci. 11:5943. doi: 10.3390/app11135943
Van Doan, H., Doolgindachbaporn, S., and Suksri, A. (2015). Effect of Lactobacillus plantarum and Jerusalem artichoke (Helianthus tuberosus) on growth performance, immunity and disease resistance of Pangasius catfish (Pangasius bocourti, Sauvage 1880). Aquac. Nutr. 22, 444–456. doi: 10.1111/anu.12263
Van Doan, H., Doolgindachbaporn, S., and Suksri, A. (2016). Effects of Eryngii mushroom (Pleurotus eryngii) and lactobacillus plantarum on growth performance, immunity and disease resistance of Pangasius catfish (Pangasius bocourti, Sauvage 1880). Fish Physiol. Biochem. 42, 1427–1440. doi: 10.1007/s10695-016-0230-6
Van Doan, H., Hoseinifar, S. H., Ringø, E., Ángeles Esteban, M., Dadar, M., Dawood, M. A., et al. (2020). Host-associated probiotics: a key factor in sustainable aquaculture. Rev. Fish. Sci. Aquac. 28, 16–42. doi: 10.1080/23308249.2019.1643288
Van Doan, H., Hoseinifar, S. H., Tapingkae, W., Chitmanat, C., and Mekchay, S. (2017). Effects of Cordyceps militaris spent mushroom substrate on mucosal and serum immune parameters, disease resistance and growth performance of Nile tilapia, (Oreochromis niloticus). Fish Shellfish Immunol. 67, 78–85. doi: 10.1016/j.fsi.2017.05.062
Wang, M., Wichienchot, S., He, X., Fu, X., Huang, Q., and Zhang, B. (2019). In vitro colonic fermentation of dietary fibers: fermentation rate, short-chain fatty acid production and changes in microbiota. Trends Food Sci. Technol. 88, 1–9. doi: 10.1016/j.tifs.2019.03.005
Xiong, J. B., Nie, L., and Chen, J. (2019). Current understanding on the roles of gut microbiota in fish disease and immunity. Zool. Res. 40, 70–76. doi: 10.24272/j.issn.2095-8137.2018.069
Yang, P., Yang, W., He, M., Li, X., and Leng, X. J. (2020). Dietary synbiotics improved the growth, feed utilization and intestinal structure of largemouth bass (Micropterus salmoides) juvenile. Aquac. Nutr. 26, 590–600. doi: 10.1111/anu.13020
Yaseen, A. A. (2018). Effect the addition of sweet whey on the survival of Lactobacıllus reuteri in the therapeutic ice cream. Adv. Environ. Biol. 12, 1–3. doi: 10.22587/aeb.2018.12.6.1
Ye, J. D., Wang, K., Li, F. D., and Sun, Y. Z. (2011). Single or combined effects of fructo- and mannan oligosaccharide supplements and Bacillus clausii on the growth, feed utilization, body composition, digestive enzyme activity, innate immune response and lipid metabolism of the Japanese flounder Paralichthys olivaceus. Aquac. Nutr. 17, 902–911. doi: 10.1111/j.1365-2095.2011.00863.x
Yukgehnaish, K., Kumar, P., Sivachandran, P., Marimuthu, K., Arshad, A., Paray, B. A., et al. (2020). Gut microbiota metagenomics in aquaculture: factors influencing gut microbiome and its physiological role in fish. Rev. Aquac. 12, 1903–1927. doi: 10.1111/raq.12416
Zhandalgarova, A. D., Bakhareva, A. A., Grozesku, Y. N., Fedorovykh, Y. N., and Sergeeva, Y. V. (2021). Normalization of the symbiotic intestinal microflora of sturgeon’s juvenile with the introduction of a new generation of probiotic preparations into their diet. IOP Conf. Ser.: Earth Environ. Sci. 723:022028. doi: 10.1088/1755-1315/723/2/022028
Zhang, Z., Li, D., Refaey, M. M., Xu, W., Tang, R., and Li, L. (2018). Host age affects the development of southern catfish gut bacterial community divergent from that in the food and rearing water. Front. Microbiol. 9:495. doi: 10.3389/fmicb.2018.00495
Zheng, J., Jia, Y., Li, F., Liu, S., Chi, M., Cheng, S., et al. (2019). Molecular characterization and expression analysis of cyp19a gene in culter alburnus. Fish. Sci. 85, 791–800. doi: 10.1007/s12562-019-01339-7
Zhou, L., Zhang, J., Yan, M., Tang, S., Wang, X., Qin, J. G., et al. (2020). Inulin alleviates hypersaline-stress induced oxidative stress and dysbiosis of gut microbiota in Nile tilapia (Oreochromis niloticus). Aquaculture 529:735681. doi: 10.1016/j.aquaculture.2020.735681
Zhu, Y., Liu, J., Lopez, J. M., and Mills, D. A. (2020). Inulin fermentation by lactobacilli and bifidobacteria from dairy calves. Appl. Environ. Microbiol. 87, e01738–e01820. doi: 10.1128/AEM.01738-20
Zimmermann, A., Visscher, C., and Kaltschmitt, M. (2021). Plant-based fructans for increased animal welfare: provision processes and remaining challenges. Biomass Convers. Biorefin. doi: 10.1007/s13399-021-01473-2 (in press).
Keywords: prebiotics, probiotics, zebrafish, mushroom, artichoke, reproduction, cyp19a gene
Citation: Zakariaee H, Sudagar M, Hosseini SS, Paknejad H and Baruah K (2021) In vitro Selection of Synbiotics and in vivo Investigation of Growth Indices, Reproduction Performance, Survival, and Ovarian Cyp19α Gene Expression in Zebrafish Danio rerio. Front. Microbiol. 12:758758. doi: 10.3389/fmicb.2021.758758
Edited by:
Mehdi Raissy, Islamic Azad University, IranReviewed by:
Pande Gde Sasmita Julyantoro, Udayana University, IndonesiaRishikesh Subhashrao Dalvi, Maharshi Dayanand College, India
Copyright © 2021 Zakariaee, Sudagar, Hosseini, Paknejad and Baruah. This is an open-access article distributed under the terms of the Creative Commons Attribution License (CC BY). The use, distribution or reproduction in other forums is permitted, provided the original author(s) and the copyright owner(s) are credited and that the original publication in this journal is cited, in accordance with accepted academic practice. No use, distribution or reproduction is permitted which does not comply with these terms.
*Correspondence: Mohammad Sudagar, c3VkYWdhcl9tQGdhdS5hYy5pcg==; Kartik Baruah, a2FydGlrLmJhcnVhaEBzbHUuc2U=