- 1Cell and Molecular Biology, The University of Southern Mississippi, Hattiesburg, MS, United States
- 2Institute for Genomics, Biocomputing & Biotechnology, Mississippi State University, Mississippi State, MS, United States
- 3Department of Biological Sciences, Mississippi State University, Mississippi State, MS, United States
Listeria monocytogenes is a gram-positive facultative anaerobic bacterium that causes the foodborne illness listeriosis. The pathogenesis of this bacterium depends on its survival in anaerobic, acidic, and bile conditions encountered throughout the gastrointestinal (GI) tract. This transcriptomics study was conducted to analyze the differences in transcript levels produced under conditions mimicking the GI tract. Changes in transcript levels were analyzed using RNA isolated from L. monocytogenes strain F2365 at both aerobic and anaerobic conditions, upon exposure to 0 and 1% bile at acidic and neutral pH. Transcripts corresponding to genes responsible for pathogenesis, cell wall associated proteins, DNA repair, transcription factors, and stress responses had variations in levels under the conditions tested. Upon exposure to anaerobiosis in acidic conditions, there were variations in the transcript levels for the virulence factors internalins, listeriolysin O, etc., as well as many histidine sensory kinases. These data indicate that the response to anaerobiosis differentially influences the transcription of several genes related to the survival of L. monocytogenes under acidic and bile conditions. Though further research is needed to decipher the role of oxygen in pathogenesis of L. monocytogenes, these data provide comprehensive information on how this pathogen responds to the GI tract.
Introduction
Listeria monocytogenes is a gram-positive foodborne pathogen that is responsible for the disease listeriosis (Scallan et al., 2011). Pregnant women, infants, elderly, and immunocompromised individuals are more susceptible to listeriosis, with meningitis, septicemia, and spontaneous abortions being possible manifestations of the disease (Thigpen et al., 2011). Being a foodborne pathogen, this bacterium must be able to respond to the stressors encountered following ingestion of contaminated food. Low pH, bile, and hypoxic/anoxic environments are some of the key stressors that are encountered by L. monocytogenes within the gastrointestinal (GI) tract (Davis et al., 1996).
Low pH of the stomach is one of the initial stressors encountered by L. monocytogenes upon ingestion (White et al., 2015). The low pH of the gastric secretion is a roadblock to invasion by the bacteria. Listeria’s acid response involves the SOS response, LisRK (a two-component regulatory system that regulates listerial osmotolerance), components of sigma B regulon, ATPase proton pump, and enzymatic systems that regulate internal hydrogen ion concentration (Sleator and Hill, 2005). A transcriptomic study that was performed on Listeria grown in the presence of organic acids revealed an increase in the transcript levels of sigma B and prfA regulated genes, which included internalins, phospholipases, and other virulence genes. This previous study also indicated an up-regulation of oxidative stress defenses, DNA repair, intermediary metabolism, cell wall modification, and cofactor and fatty acid biosynthesis (Tessema et al., 2012). A proteomic study performed on Listeria grown in the presence of organic salts demonstrated an up-regulation of oxidoreductases and lipoproteins. Upon exposure to hydrochloric acid, it was also observed that proteins involved in respiration (enzyme dehydrogenases and reductases), osmolyte transport, protein folding and repair, general stress resistance, flagella synthesis and metabolism were expressed in the response to the acidic conditions (Bowman et al., 2012).
Listeria is also exposed to bile within the GI tract (White et al., 2015). Bile is synthesized by the liver and stored in the gall bladder. It is released into the duodenum during digestion (Monte et al., 2009). The bile acids are the antibacterial component of bile; bile acids induce damage to the cell wall and DNA (Coleman et al., 1979; Bernstein et al., 1999; Prieto et al., 2004, 2006). Within the gall bladder, bile is found at a nearly neutral pH (7.5), while in the duodenum it is more acidic (pH 5.5) (White et al., 2015). Bile is more bactericidal at acidic pH than at a neutral pH, as indicated in a study that showed a decrease in survival in bile under pH 5.5 in comparison to a pH of 7.5 (Dowd et al., 2011). Many studies have been conducted to determine the global response of L. monocytogenes to bile encountered within the GI tract. For instance, the transcription factor brtA, which senses cholic acid and regulates efflux pumps (MdrM and MdrT) is involved in bile tolerance (Quillin et al., 2011). Bile salt hydrolases neutralize conjugated bile acids, thereby providing protection against the bactericidal properties of bile (Dowd et al., 2011). The bilE gene is also involved in detoxifying bile acids (Dowd et al., 2011).
In addition to changes in pH and bile, L. monocytogenes is also exposed to changes in oxygen concentrations. The duodenum is considered microaerophilic in nature, while the gall bladder is anaerobic (Zheng et al., 2015). Oxygen availability has been found to influence bile resistance. A proteomics study performed under anaerobic conditions in the presence of bile observed notable alterations in cell wall associated proteins, DNA repair proteins and oxidative stress response proteins. Under anaerobic conditions the Listeria adhesion protein has been observed to have a significant role in intestinal infection (Burkholder et al., 2009). Additionally, oxygen deprivation has been found to affect the survival of L. monocytogenes in vitro (Payne et al., 2013; Wright et al., 2016), as well as in cell cultures, guinea pigs (Bo Andersen et al., 2007), and gerbils (Harris et al., 2019). These studies highlight the importance of oxygen in regulation of virulence. However, it is not known what the transcriptomic response of L. monocytogenes is to conditions that mimic the GI tract under physiologically relevant anaerobic conditions. Therefore, the goal of this study was to determine the impact of oxygen on the transcriptomic response of L. monocytogenes to bile in conditions that mimic the duodenum (pH 5.5) and the gall bladder (pH 7.5).
Results
Survival of L. monocytogenes in Conditions Mimicking Gastrointestinal Tract
Listeria monocytogenes exhibits slightly slower growth rates under anaerobic conditions (Figures 1A vs. 1B). Bile also impacted the viability of L. monocytogenes strain F2365 differently under anaerobic conditions. Under neutral pH, bile did not have a significant impact on survival of L. monocytogenes strain F2365 under either aerobic (Figure 1A) or anaerobic conditions (Figure 1B).
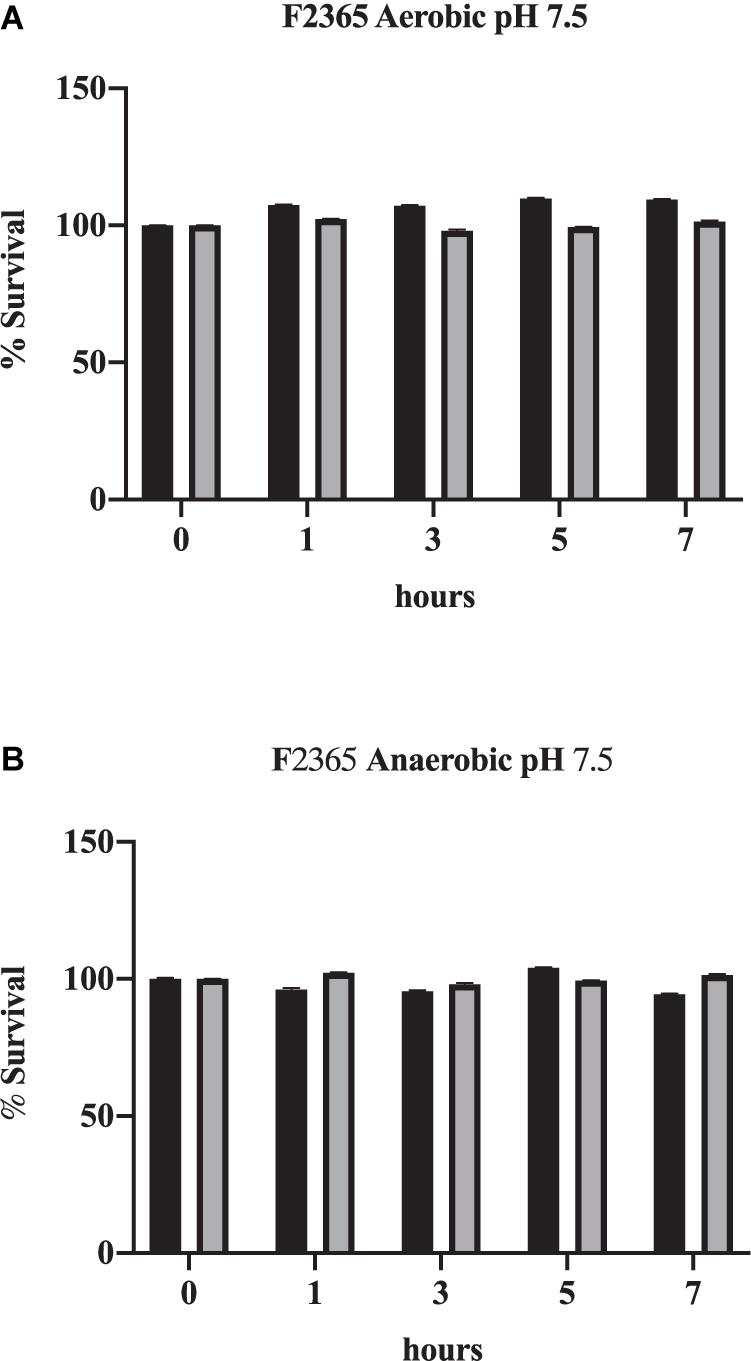
Figure 1. Impact of oxygen on the survival of L. monocytogenes in conditions mimicking the gall bladder. F2365 was exposed to either aerobic (A) or anaerobic (B) conditions with 0% bile (black bars) or 1% bile (gray bars) at a pH of 7.5 and survival was measured by viable plate counts over 7 h. Data represent averages of three independent replicates. Error bars represent standard deviation from biological replicates.
At acidic pH in the presence of bile, which mimics the exposure to bile in the duodenum, the percentage of L. monocytogenes that survived significantly declined (Figure 2A; p < 0.05). This further demonstrates the increase in toxicity exhibited by bile when in acidic conditions. Survival also declined under anaerobic conditions in comparison to time 0 h (Figure 2B, p < 0.05). However, the decrease in viability was not as severe under anaerobic conditions (Figure 2B) in comparison to aerobic conditions (Figure 2A; p < 0.05). This indicates that anaerobic conditions improve the survival of L. monocytogenes to the toxic effects of bile.
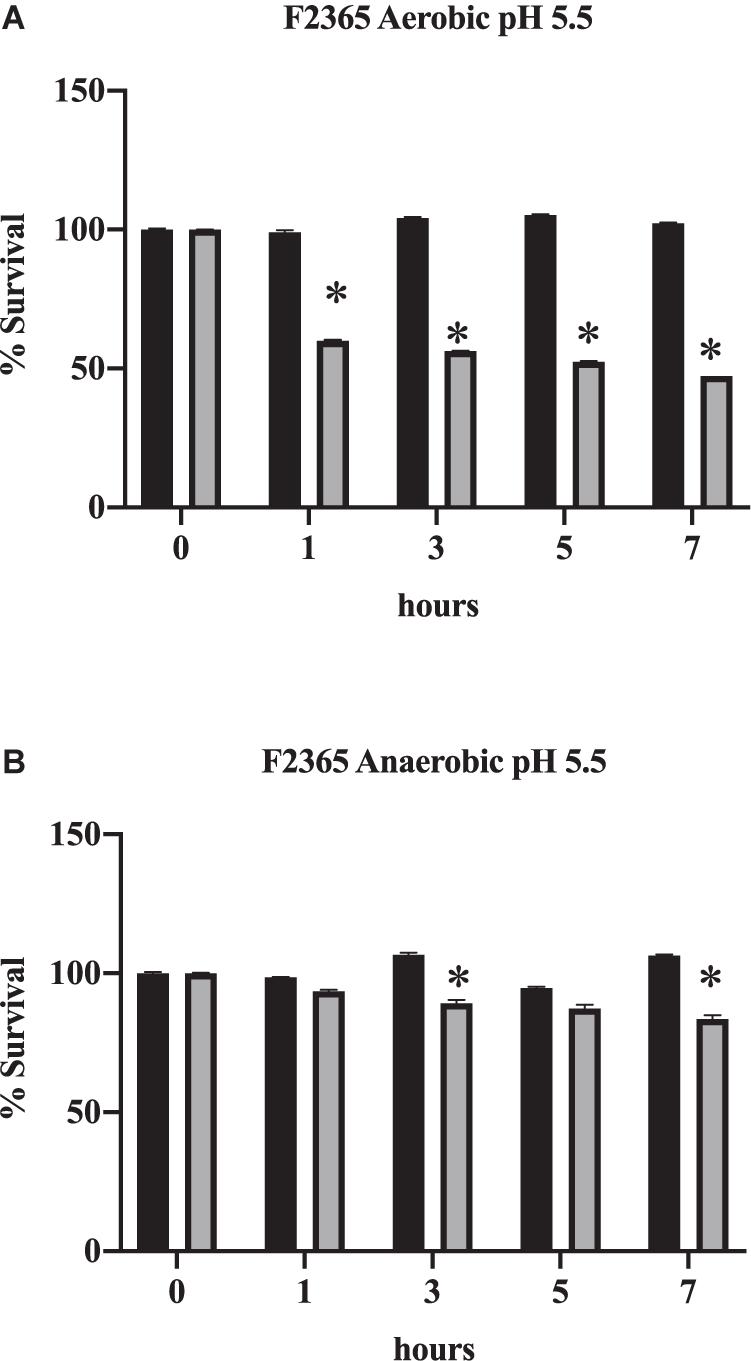
Figure 2. Impact of oxygen on the survival of L. monocytogenes in conditions mimicking the duodenum. F2365 was exposed to either aerobic (A) or anaerobic conditions (B) with 0% bile (black bars) or 1% bile (gray bars) at a pH of 5.5 and survival was measured by viable plate counts over 7 h. Data represent averages of three independent replicates. Error bars represent standard deviation from biological replicates. * Indicates p < 0.05 in comparison to time 0.
Overall Changes in Transcript Levels in Response to Conditions Mimicking the Gastrointestinal Tract
As significant alterations in survival were observed following 1 h of bile exposure under acidic conditions, this time point was selected to compare the impact that oxygen had on the transcriptome. Table 1 shows the overall changes in transcripts detected. Under anaerobic conditions, a total of 190 transcripts in media at pH 7.5 and 268 at pH of 5.5 were identified to be differentially expressed in comparison to aerobic conditions. In the presence of bile and absence of oxygen, 304 and 434 transcripts were differentially produced at pH 7.5 and 5.5, respectively. Under anaerobic conditions, upon exposure to bile, variations in the transcript levels of 200 genes were identified at pH 7.5 and 419 at pH 5.5. For all conditions tested, there were globally more transcripts identified to be up-regulated than down-regulated, except for acidic bile conditions under anaerobic growth.

Table 1. Total changes in transcript levels following exposure to bile at pH of 7.5 or 5.5 under either aerobic or anaerobic conditions.
Changes in Transcript Levels in Response to Anaerobic Conditions
Transcripts representative of five genes were found to be increased in expression levels under exposure to anaerobic conditions regardless of whether the cultivation was conducted under either neutral or acidic pH (Table 2 and Supplementary Figure 1). These included genes involved in membrane transport, protein folding, and stress response. Of these transcripts the amino acid transporter (LMOf2365_2333) had nearly a 9-fold increase in levels at neutral pH in comparison to acidic pH. Transcripts representative of the dnaJ (LMOf2365_1491) and dnaK (LMOf2365_1492) genes, which encode for molecular chaperones and have roles in phagocytosis and protein homeostasis, were also increased under anaerobic conditions at both pH conditions tested. The transcript representative of the cadA (LMOf2365_0672) gene, which encodes for a heavy metal translocating P-type ATPase and is a component of the CadAC efflux cassette, was also increased 6.1-fold at pH 7.5 and 3.8 at pH 5.5 under oxygen depleted conditions (Table 2).
The transcript levels of 18 genes were decreased under anaerobic conditions regardless of the pH condition tested (Table 3 and Supplementary Figure 2). Out of these eighteen transcripts, six were representative of uncharacterized hypothetical proteins; all of these had lower transcript levels under neutral conditions in comparison to acidic conditions. This could suggest that these hypothetical genes are regulated similarly. The remaining transcripts identified encoded for stress response, membrane associated protein, and metabolism protein (Table 3).
Changes in Transcript Levels in Response to Anaerobic Acidic Conditions
In acidic conditions, transcript levels of 140 genes were increased (Table 4 and Supplementary Figure 1) and 104 were decreased under anaerobiosis (Table 5). Analyzing these transcripts up-regulated in response to acidic conditions under anaerobiosis revealed that several biological pathways related to pathogenesis, stress response, membrane associated proteins, transcription factors and DNA repair mechanisms influenced the survival of L. monocytogenes (Table 4). Transcripts representative of genes involved in metabolism, transcription factor and pathogenesis were down-regulated (Table 5). Certain transcripts encoding for glycolytic enzymes increased under acidic anaerobic conditions as well (Table 4). These included the glyceraldehyde-3-phosphate dehydrogenase (5.4-fold increase), phosphoglycerate mutase (4.7-fold increase), and pyruvate kinase (6.7-fold increase).
Changes in Transcript Levels in Response to Bile Under Anaerobic Conditions
Transcripts representative of 53 genes were found to be up-regulated in response to exposure to bile under anaerobic conditions (Table 6 and Supplementary Figure 3). Transcripts encoding for transcription regulators of virulence, antibiotic resistance, metabolism, and membrane associated proteins were also observed to increase in their levels of expression (Table 6). Transcripts representative of nine genes were down-regulated under anaerobic conditions in presence of bile at both pH 7.5 and 5.5 (Table 7 and Supplementary Figure 4). Fold changes of the transcript levels of genes associated with metabolism, translation, pathogenesis, and transcription were down-regulated (Table 7).
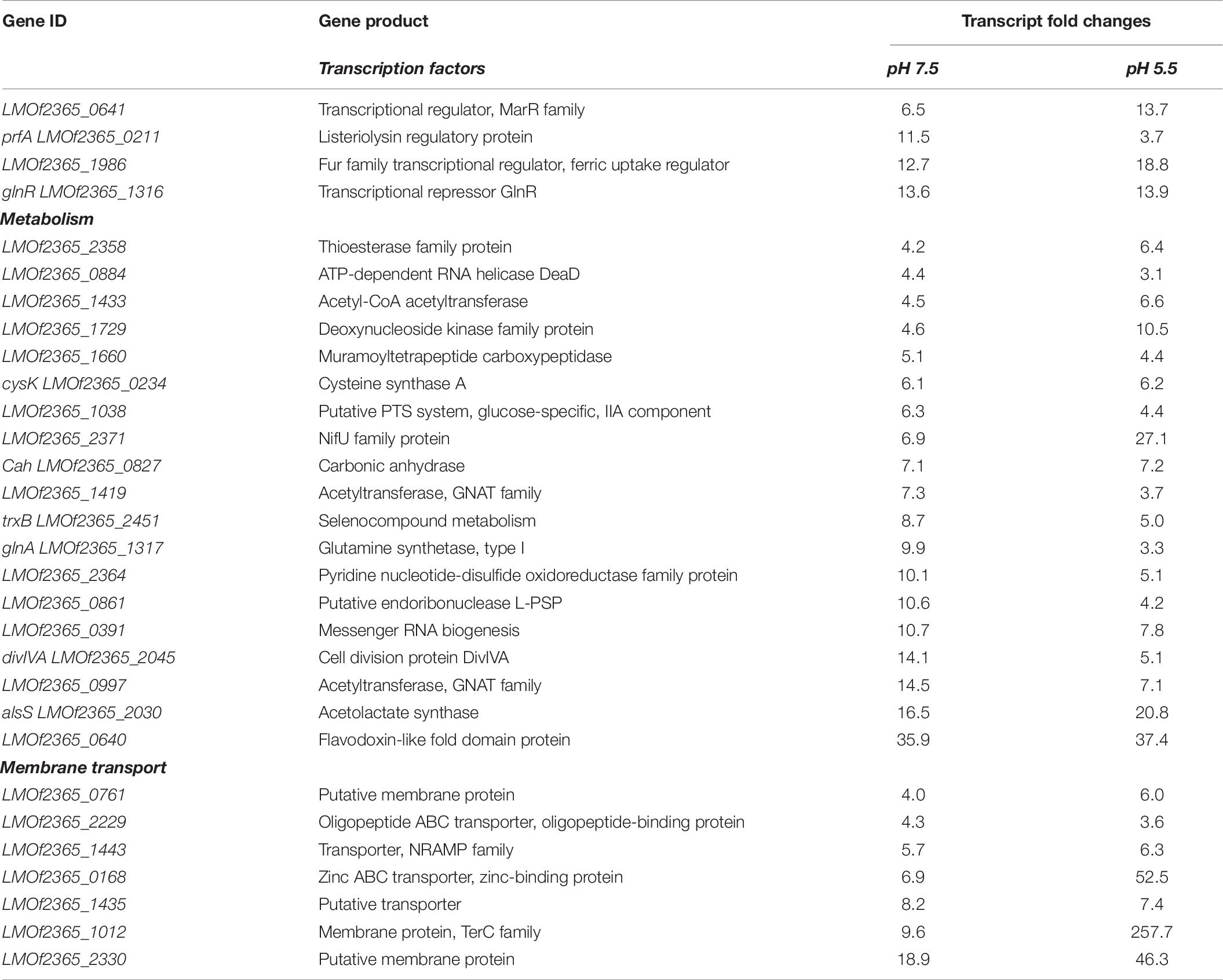
Table 6. Transcript levels increased for select genes in response to anaerobiosis at pH of 7.5 and 5.5.
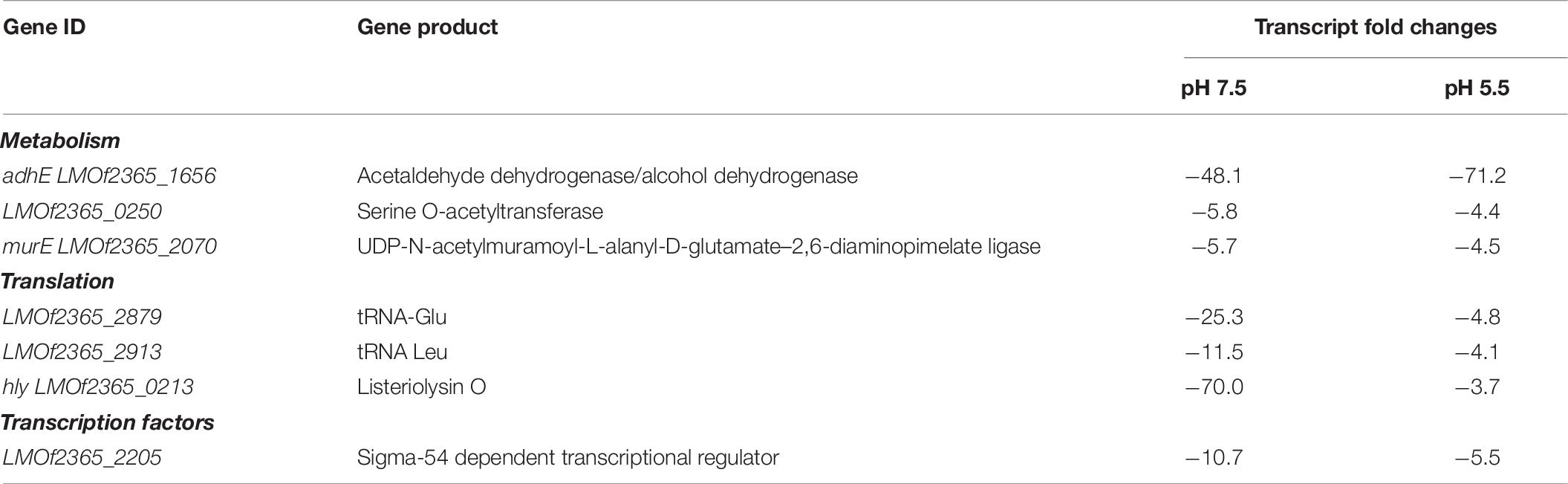
Table 7. Transcript levels decreased for select genes in response to anaerobiosis at pH 7.5 and 5.5.
Changes in Transcript Levels in Response to Bile Under Acidic and Anaerobic Conditions
Transcript levels of 210 genes were up-regulated in response to bile at acidic pH under anaerobic conditions (Table 8 and Supplementary Figure 3). Transcripts encoding for transcription factors, metabolism, replication and repair, cell signaling, protein folding, and pathogenesis were also found to be up-regulated. Additionally, transcripts representing 146 genes were down-regulated under anaerobic conditions with acidic bile (Table 9 and Supplementary Figure 4), with these being primarily associated with metabolism, membrane transport, replication and repair, pathogenesis, and transcription factors.

Table 8. Transcript levels increased for select genes in response to bile in anaerobic conditions at pH 5.5.

Table 9. Transcript levels decreased for select genes in response to bile in anaerobic conditions at pH 5.5.
Discussion
Anaerobiosis Improves Survival of L. monocytogenes in Conditions Mimicking the Gastrointestinal Tract
Survival of L. monocytogenes strain F2365 was analyzed under conditions mimicking the GI tract. This strain was chosen as it is a serotype 4b strain, which represents the serotype of a large portion of outbreak strains. F2365 was isolated from one of the deadliest outbreaks of L. monocytogenes (Linnan et al., 1988). F2365 has been sequenced (Nelson et al., 2004) and has been extensively studied for genomic analyses (Chatterjee et al., 2006; Liu and Ream, 2008; Payne et al., 2013), making it an ideal strain to analyze transcriptomic responses.
Bile is made in the liver, stored in the gall bladder, and released to the duodenum upon ingestion. The environment in the gall bladder is anaerobic and neutral pH, while the duodenum is acidic and microaerophilic (Zheng et al., 2015). The alterations in oxygen availability within the GI tract are essential to developing the redox relationship between microbes and host (He et al., 1999; Espey, 2013). Therefore, we tested how oxygen influenced the survival of L. monocytogenes under either acidic (mimicking the duodenum) or neutral (mimicking the gall bladder) bile conditions.
Since variations in transcript levels were observed due to alterations in oxygen availability, we wanted to determine which genes were commonly expressed under anaerobiosis. Transcript levels of five genes were found to be up-regulated under exposure to anaerobic conditions regardless of whether the cultivation was conducted under either neutral or acidic pH (Table 2), though there were differential expressions between the two conditions. Transcripts common to both conditions included two membrane transporters LMOf2365_2333 and cadA (LMOf2365_0672), two chaperones, and the stress response related gene gadG (LMOf2365_2405). CadA has been previously shown to be involved in formation of biofilms at 25°C by L. monocytogenes (Parsons et al., 2017). CadA also has been implicated in having roles in virulence and pathogenesis (Parsons et al., 2017). Therefore, it is possible that CadA is involved in stress response mechanisms related to anaerobic survival and that the formation of biofilms may be a critical component to survival. Previous studies have also shown that various stressors (i.e., heat shock, nutrient limitation, acidic condition, etc.) cause an increase in the expression of chaperones (Wright et al., 2016). Indeed, the data showed an increase in the transcript levels of two chaperones (dnaK and dnaJ) under anaerobic conditions at both pH 7.5 and 5.5. Therefore, it is possible that L. monocytogenes uses molecular chaperones to combat anaerobic stress, which in turn assists with phagocytosis. The gadG encodes for an amino acid antiporter that is part of the glutamate decarboxylase system, which is a defense mechanism up-regulated by L. monocytogenes under acid stress and anaerobiosis. This system alleviates the acidification of the cytoplasm by consuming a proton (Cotter et al., 2001; Jydegaard-Axelsen et al., 2004; Paudyal et al., 2020). The fact that this transcript was up-regulated in response to anaerobic conditions suggests that there may be overlapping functions of the GAD system in both acid resistance and anaerobiosis. The transcript level of the LMOf2365_2333 gene was increased by nearly 9-fold in comparison to acidic pH. There is a possibility that this amino acid anti-transporter may function with gadG in response to bile. This should be further explored in future studies.
Transcript levels of eighteen genes were down-regulated under anaerobic conditions regardless of the pH, including histidine kinase, metabolic genes, a universal stress response gene, and genes coding for hypothetical proteins. As histidine kinases are involved in two-component systems, it is possible that suppression of this sensor is responsible for the response to oxygen availability. One of the metabolic genes, the phosphoglycerate mutase, has been shown in Bacillus subtilis to be responsible for the control of the two-component system required for sensing and responding to aerobic and anaerobic respiration (Nakano et al., 1999). The fact that the transcript level of this gene was down-regulated suggests that the accumulation of the product 1,3-bisphosphoglycerate, which is the intermediate in the reaction catalyzed by phosphoglycerate mutase, might impact the regulation of the histidine kinase LMOf2365_2554. The impact of this precursor on regulation of two-component systems needs to be explored in further detail. The transcript level of the gene acpP was also down-regulated. This gene product is involved in biosynthesis of fatty acids as a lipid transporter. This gene has been found to be differentially regulated under anaerobic conditions in many other bacteria, including Escherichia coli and Neisseria gonorrhoeae (Isabella and Clark, 2011). This indicates that the regulation of the fatty acid synthesis is necessary for the adaptability to anaerobiosis.
Differential Transcript Levels in Response to Anaerobic Acidic Conditions
An increase in the transcript levels of nrdD (LMOf2365_0299), which is an anaerobic ribonucleoside-triphosphate reductase that catalyzes the synthesis of dNTPs required for DNA replication, was observed under anaerobic conditions at acidic pH. NrdD is an essential enzyme required by L. monocytogenes and other GI pathogens, such as E. coli, to survive under anaerobic conditions (Garriga et al., 1996; Ofer et al., 2011). Since our study showed acidic conditions influence the up-regulation of this gene under anaerobic conditions, there is a possibility that this enzyme is involved in growth under acidic conditions. This may be required to stabilize the redox potential of the cell under acidic conditions. Ribonucleotide reductases have been explored as potential biomedical targets for bacterial infections (Torrents, 2014). Since the ribonucleotide reductase was up-regulated under anaerobic acidic conditions, it will be necessary for future studies to analyze the activity of antibacterial compounds under these conditions to effectively target the protein expressed.
Transcript levels of genes coding for a glycosyl hydrolases, which are involved in hydrolyzing the glycosidic linkages in sugars, were also up-regulated. Certain glycosyl hydrolases have been previously identified as virulence factors in gram positive pathogenic bacteria, including Streptococcus pneumoniae (Niu et al., 2013). Glycosyl hydrolase PssZ has been observed to degrade extracellular polymeric substance, thereby disrupting biofilm formation by L. monocytogenes (Wu et al., 2019). L. monocytogenes, which is an intracellular bacterium, may synthesize glycosyl hydrolases upon exposure to acidic pH under anaerobic conditions, which thereby hinders formation of biofilms and facilitates the bacterium’s entry into the host cells.
One of the virulence factors of L. monocytogenes is metalloproteases. Few such proteases were identified to have an increase in transcript levels at pH 5.5 in anaerobic conditions, including the aminopeptidase (LMOf2365_2308) (Table 4). It has been shown that the bacterial burden of L. monocytogenes EGDe strain in host cells decreased significantly when the aminopeptidase T of family M29 was deleted (Cheng et al., 2015). Thus, at anaerobic conditions under acidic pH, aminopeptidases may be up-regulated and function as virulence factors.
GalU (LMOf2365_1099), UTP-glucose-1-phosphate uridyltransferase, which catalyzes cell wall teichoic acid glycosylation, had an increase in transcript levels under anaerobic conditions at pH 5.5 (Table 4; Kuenemann et al., 2018). In silico design of GalU inhibitors attenuated virulence of L. monocytogenes, proving GalU to be an instrumental part in virulence pathways (Kuenemann et al., 2018). Various transcription factors were up-regulated under anaerobic conditions at pH 5.5 (Table 4), including the fur regulator that controls virulence of various pathogenic bacteria. We also observed that transcripts coding for virulence genes, such as listeriolysin O and internalin family proteins, were also up-regulated under these conditions. The transcript level of a methyl accepting chemotaxis protein was also increased. In L. monocytogenes chemotaxis genes cheA and cheY have been shown to facilitate to adhesion and thereby invasion into the host epithelial cells. As L. monocytogenes is an intracellular pathogen, it may be possible that along with the CheA and CheY system, it is using the methyl accepting chemotaxis proteins to attach to epithelial cells under anaerobic conditions at pH 5.5 (Dons et al., 2004).
Internalins A and B are required by L. monocytogenes for facilitating entry inside host cells. Transcript levels for genes encoding internalin proteins were found to be up-regulated under the acidic environment in absence of oxygen. Interestingly, the transcript level of inlE (LMOf2365_0283), which is a gene coding for the secreted protein Internalin E, was decreased. Internalins A and B are involved in adhesion and invasion by Listeria, but Internalin E is not involved in invasion (Dramsi et al., 1997). This indicates anaerobiosis influences the invasive potential of L. monocytogenes. The impact of anaerobiosis on invasion has been shown in vitro and in vivo, but the exact mechanism of such interplay has not been well characterized (Bo Andersen et al., 2007; Harris et al., 2019).
Differential Transcript Levels in Response to Bile Under Anaerobic Conditions
Previous studies have shown that following ingestion of L. monocytogenes into host systems, the prfA regulon is up-regulated (Scortti et al., 2007). prfA, the positive regulatory factor A, is a transcription factor that regulates major virulence factors of L. monocytogenes. prfA regulates listeriolysin O, phospholipase C and metalloproteases, all of which were up-regulated in anaerobiosis in presence of bile (Table 6). Following bile exposure, the transcript levels of the virulence regulator prfA were decreased (Boonmee et al., 2019); however these data show that under anaerobic conditions in presence of bile, prfA is up-regulated independent of pH. We have also observed that L. monocytogenes survives bile better under anaerobic conditions (Figure 2).
Previous transcriptomics studies in L. monocytogenes 10403S (Boonmee et al., 2019) have found that following exposure to bile, the house keeping sigma factor σA has a significant role in survival. marR [multiple antibiotic resistance regulator (LMOf2365_0641)] is a transcriptional regulator that was up-regulated in response to bile in anaerobic conditions regardless of the pH tested (Table 6). In pathogens such as Salmonella and Staphylococcus, marR homologs slyA and sarZ regulate virulence gene expression. marR homologs have also been found to regulate genes involved in stress response, degradation or efflux of harmful substances and metabolic pathways (Grove, 2013). Bile exposure under anaerobic environments may trigger the up-regulation of marR to export bile out of the bacterial cell, thereby contributing to the bile resistance of L. monocytogenes along with other factors. The role of marR in bile resistance needs to be further explored.
Glutamine synthetase catalyzes the condensation of ammonia and glutamate to form glutamine. The transcript level of the glutamine synthetase repressor, glnR (LMOf2365_1316) was increased following exposure to bile in anaerobic conditions. It is a central nitrogen metabolism regulator which is activated in presence of glutamine. When glutamine is in excess, GlnR represses the synthesis of glutamine synthetase (Kaspar et al., 2014). Another probable transcriptional regulator (tnrA or codY) represses glutamine synthetase and its activation have been found to be essential in replication Listeria intracellularly (Kaspar et al., 2014). Interestingly glutamine synthetase was also up-regulated under the same conditions, which indicates the possibility of a feedback loop.
Metalloenzyme carbonic acid catalyzes hydration of carbon dioxide into bicarbonate and proton (Supuran, 2016). The infection cycle of Legionella has similarities with that of L. monocytogenes, such as invasion and escaping the phagosome. Legionella has been shown to evade the destruction by maintaining neutral pH (Supuran, 2016). One of the enzymes involved in regulating the pH is carbonic anhydrase; the transcript level of carbonic anhydrase increased under anaerobic conditions in the presence of bile in L. monocytogenes (Table 6). This could indicate that environmental conditions mimicking parts of intestine can contribute to Listeria’s pathogenic potential. Interestingly, the transcript level of this gene was down-regulated under acidic conditions (Table 5), suggesting that the influence of bile is important to the expression of this gene.
Transcript levels representative of an uncharacterized membrane protein LMOf2365_1012 that belongs to the TerC family was up-regulated following exposure to bile in anaerobic conditions (Table 6). In B. subtilis, TerC has been found to confer manganese resistance (Paruthiyil et al., 2020). In Streptococcus, manganese homeostasis is linked to oxidative stress as well as virulence (Turner et al., 2015). It is possible that TerC is linked with manganese homeostasis and therefore virulence in the presence of bile under anaerobic conditions. Transcripts coding for several other membrane transporters were also increased in their levels under the anaerobic environment in response to bile. The zinc ABC transporter has been shown to have a role in virulence of L. monocytogenes in a mouse infection model (Corbett et al., 2012). Thus, bile exposure in absence of oxygen probably impacts uptake of zinc by the bacteria thereby impacting the virulence. NRAMP, which functions as a metal ion transporter on membranes, was up-regulated (Nevo and Nelson, 2006).
The transcript level of the oligopeptide ABC transporter, which is an oligopeptide binding protein that helps the bacteria survive intracellularly, was increased (Slamti and Lereclus, 2019). It is the substrate binding component or receptor of an ABC type oligopeptide transport system that binds extracellular peptides, relays it to the membrane component of the system and inside the bacterial cell afterward. Gram positive bacteria such as Listeria, Streptococcus, and Enterococcus, use peptides to sense and respond to environmental changes. The gene oppA, which encodes for an oligopeptide binding protein, has been found to be required for invasion (Borezee et al., 2000). Thus, the oligopeptide ABC transporter observed in our study could be responsible for intracellular survival of bacteria in presence of bile under anaerobic conditions.
Interestingly, there was a decrease in the transcript levels of hly (LMOf2365_0213), which encodes for listeriolysin O, at both pH 7.5 and 5.5 following exposure to bile under anaerobic conditions. This was different than what was observed under anaerobiosis at pH 5.5 alone, as hly (LMOf2365_0213) was up-regulated in these conditions (Table 4). This suggests that bile has an important role in regulating the invasiveness of L. monocytogenes. This correlates well with previous studies that have shown that L. monocytogenes remains extracellular in the gall bladder, which has high concentrations of bile (Hardy et al., 2004; Dowd et al., 2011).
Differential Transcript Levels in Response to Bile Under Acidic and Anaerobic Conditions
There was an increase in transcript levels for the myosin cross reactive antigen (McrA) (LMOF2365_0508; Table 8). Although its function in L. monocytogenes is yet unknown, in Streptococcus pyogenes McrA is a fatty acid double bond hydratase that adds water to double bonds of fatty acids. Upon deletion of this gene, decreased oleic acid resistance and reduced adherence and internalization in the host cell was observed in S. pyogenes (Volkov et al., 2010). Conditions encountered within the duodenum may directly or indirectly contribute to up-regulation of mcrA, which may regulate the pathogen’s resistance to bile.
Internalin E and hemolysin III are both virulence factors responsible for internalization and invasion for L. monocytogenes. Both had an increase in transcript levels, indicating that bile exposure at acidic and anaerobic conditions, which mimics the duodenum, is conducive to the pathogenesis of the bacteria.
The transcript level of the LPXTG-motif cell wall anchor domain (LMOF2365_1144) was also up-regulated. In the L. monocytogenes EGDe strain, it has been shown that a LPXTG protein encoded by the Listeria mucin binding invasion A gene, or lmiA, has roles in promoting bacterial adhesion and entry into the host cell (Mariscotti et al., 2014). MucBP domain present in LPXTG was observed to bind to mucin. Thus, up-regulation of LPXTG gene under conditions mimicking the duodenum indicates that these conditions may facilitate invasion of host cells by the bacteria.
The level of transcripts representing flagellin also increased. It has been shown that flagellin helps in motility soon after ingestion in vivo (O’Neil and Marquis, 2006) and invasion (Dons et al., 2004). A previous study has also observed up-regulation of motility under exposure to bile at pH 5.5 (Guariglia-Oropeza et al., 2018). The fact that expression increased in conditions that would be encountered soon after ingestion suggests that the flagellin are important for the motility of the bacteria to the location in the GI tract where they will invade the intestinal lining.
The transcript level of the histidine kinase LMOf2365_2554 was also up-regulated under conditions mimicking the duodenum. Histidine kinase is the signal receiver a two-component regulatory system. Its counterpart in the system is the response regulator (Chang and Stewart, 1998; Stock et al., 2000; West and Stock, 2001; Krell et al., 2010). Response regulators in L. monocytogenes have been proven to have roles in virulence and pathogenesis. Sensor histidine kinase, ChiS, regulates the chitin utilization pathway required by Vibrio cholerae, which is needed to survive in aquatic environments. Chourashi et al. (2016) observed that ChiS has an important role in adherence and intracellular survival of V. cholerae in HT-29 cell cultures. They also showed that the sensor histidine kinase ChiS was activated in the presence of intestinal mucin (Chourashi et al., 2016). In the case of L. monocytogenes, it could be possible that the conditions in the duodenum are favorable for activation of the sensor histidine kinase, which could in turn relay information that would result in the activation of transcription factors responsible for adhesion and invasion.
Transcript levels representative of replication and repair genes were also up-regulated. In L. monocytogenes strain EGDe, RecA has been shown to have roles in bile and acid resistance, as well as in adhesion and invasion to Caco-2 cell cultures (van der Veen and Abee, 2011). Our data indicate that in the pathogenic strain F2365, RecA has the similar role of bile and acid resistance. In our study, we have also found that under anaerobic conditions (along with bile and acidic) the transcript level of recA changed, indicating absence of oxygen may have impact on activation of RecA.
The transcript level for a gene encoding for the transcriptional regulator padR was up-regulated (Table 8). In L. monocytogenes EGDe, LftR, which is a PadR like transcriptional regulator, has been shown to influence invasion of human host cells (Kaval et al., 2015). It is already known that Listeria uses internalin proteins for adhering and internalizing into the cell. Kaval et al. (2015) found that LftR, which is an uncharacterized protein, is required for invasion.
Transcript level of the gene encoding for ctsR, (LMOf2365_0241) a class III stress gene repressor that negatively regulates clp, was up-regulated under these conditions (Table 8). CtsR has been shown to be required for virulence in mice. PrfA which regulates many virulence genes of L. monocytogenes has been shown to down-regulate ClpC production (Karatzas et al., 2003). Although Karatzas et al. (2003) could not find any relationship between clp and prfA, there is still a possibility that there is a connection between the regulation of Clp by CtsR under anaerobic conditions in exposure to bile at acidic pH (Cui et al., 2018).
The transcript level of the transcription elongation factor greA (LMOf2365_1515) also increased under anaerobic conditions with acidic bile. GreA has been found to have roles in affecting functions of virulence gene expression in the pathogen Francisella tularensis subsp. Novicida (Cui et al., 2018). In F. tularensis, GreA was found to be required for invasion and intracellular growth of bacteria. Cui et al. (2018) also observed suppression of virulence of the greA mutant in mouse model. Transcriptomics analysis of the greA mutant revealed down-regulation of various genes responsible for virulence. Thus, with respect to our work, conditions in the duodenum are favorable for induction of the transcription elongation factor greA, which may in turn regulate genes responsible for invasion and multiplication of L. monocytogenes.
This study indicates that not only one stressor, but combinations of different stressors impact the transcription of various virulence genes. Transcriptomic and phenotypic studies in absence of these genes under mimicking physiological condition could give us an insight into this mechanism. A better understanding of how these biological processes help the survival of L. monocytogenes will lead us to understand how the physiological conditions contribute to the pathogenesis.
Materials and Methods
Bacterial Strain and Culture Conditions
Listeria monocytogenes str. 4b F2365 was used for this study. Overnight cultures of L. monocytogenes str. 4b F2365 were grown at 37°C aerobically in Brain Heart Infusion (BHI) media at pH 7.5. Next day, inoculum (1:100) from the overnight culture was used to grow the cells to mid exponential phase in fresh BHI media (OD600 = 0.3 to 0.5) under either aerobic or anaerobic conditions in 5 mL aliquots. Anaerobic culture conditions were obtained using an incubator shaker set at 37°C inside a Coy Anaerobic Chamber with a gas mixture of 95% N2 and 5% H2 (Coy Laboratory Products, United States). Cells were then pelleted at 8000 × g at 23°C and resuspended in fresh BHI at a pH of either 7.5 or 5.5; pH was adjusted with either HCl or NaOH. For bile treated cells, mid exponential phase cells were resuspended in BHI at a pH of either 7.5 or 5.5 supplemented with 1% porcine bile extract (Sigma Aldrich, United States). Cells were then grown under either aerobic or anaerobic conditions at 37°C. This study had eight different conditions that mimicked parts of the GI tract. The conditions tested were: (1) aerobic at pH 5.5; (2) anaerobic at pH 5.5; (3) aerobic at pH 7.5; (4) anaerobic at pH 7.5; (5) aerobic at pH 5.5 with 1% porcine bile; (6) anaerobic at pH 5.5 with 1% porcine bile; (7) aerobic at pH 7.5 with 1% porcine bile; and (8) anaerobic at pH 7.5 with 1% porcine bile. For each time point during a 7 h incubation period, aliquots were serially diluted in phosphate buffered saline (PBS) and plated onto BHI agar plates. Plates were incubated overnight at 37°C prior to enumeration. Three independent replicates were performed in parallel for each individual condition tested.
RNA Extraction, Library Preparation and RNA Sequencing
To isolate the RNA for analysis of the transcript level expression, cells were collected after 1 h of incubation in the eight culture conditions described above. Three biological replicates were assayed. Briefly, 5 mL of culture was pelleted by centrifugation at 8,000 × g for 5 min at room temperature. Cell pellets were then treated with RNA Protect Bacterial Reagent (Qiagen, Germany). Total RNA was isolated using the RNeasy® Mini Kit (Qiagen, Germany) per manufacturer’s instructions. The extracted RNA was quantitated using Qubit 3 Fluorometer (Invitrogen, United States) using the Qubit RNA BR assay kit (Thermo Fisher, United States). Extracted samples with values of A260/280 ∼ 2.0 were selected for sequencing. Illumina HiSeqTM 2000 paired-end 50 bp sequencer (PE50) was used. Ribosomal RNA was reduced with Epicentre RiboMinus kit (Illumina, United States) coupled with Directional RNA-Seq library prep with TruSeq indexes (Illumina, United States) per manufacturer’s instructions.
Data Analysis
Differences in survival were determined using a student’s t-test (Prism 8). Tophat-2.0.8.b (Trapnell et al., 2009) was used to align the RNA-Seq data to the reference genome, AE017262.2 L. monocytogenes str. 4b F2365. Transcript level calculation and FPKM normalization were performed using Cufflinks-2.1.1 (Trapnell et al., 2010). FPKM filtering cutoff of 1.0 was maintained to determine expressed transcripts. Differential transcript levels of the genes were determined using Cuffdiff (Trapnell et al., 2013). Differential transcript levels which had a greater than 3-fold expression and were statistically significant (p < 0.01 and q < 0.01) were subjected to Gene Ontology (GO) enrichment analysis using Blast2GO (Conesa et al., 2005). In this software, the up- and down-regulated transcripts were selected, and BLAST was performed against the L. monocytogenes nucleotide database in NCBI. The BLAST results were then mapped and annotated.
Data Availability Statement
SRA IDs of the submitted data: SRR13859772, SRR13859774, and SRR13859773: F2365 pH 5.5 Aerobic, SRR13859144, SRR13859143, and SRR13859142: F2365 pH 5.5 Anaerobic, SRR13859527, SRR13859526, and SRR13859525: F2365 pH 5.5+ Bile Anaerobic, SRR13859600, SRR13859599, and SRR13859598: F2365 pH 7.5+ Bile aerobic, SRR13858938, SRR13858937, and SRR13858936: F2365 pH 7.5+ Bile Anaerobic, SRR13858765, SRR13858767, and SRR13858766: F2365 pH 7.5 Anaerobic, SRR13853432, SRR13853433, and SRR13853431: F2365 pH 5.5+ Bile Aerobic, SRR13849951, SRR13849952, and SRR13849950: F2365 pH 7.5 aerobic.
Author Contributions
JD: conceptualization, supervision, and project administration. MA, MD, JD, GS, and DC: methodology. GS and DC: software. GS, DC, and JD: validation and visualization. DC and JD: investigation and writing–review and editing. MA and GS: resources. DC: data curation and writing–original draft preparation. All authors have read and agreed to the published version of the manuscript.
Funding
This research was funded by the National Institutes of Health, Mississippi INBRE grant number P20GM103476 and MSU-COBRE grant number P20GM103646.
Conflict of Interest
The authors declare that the research was conducted in the absence of any commercial or financial relationships that could be construed as a potential conflict of interest.
Publisher’s Note
All claims expressed in this article are solely those of the authors and do not necessarily represent those of their affiliated organizations, or those of the publisher, the editors and the reviewers. Any product that may be evaluated in this article, or claim that may be made by its manufacturer, is not guaranteed or endorsed by the publisher.
Acknowledgments
We would like to extend our acknowledgment to Christopher Bryson and Trevor Perry for helping us with analyzing the data set.
Supplementary Material
The Supplementary Material for this article can be found online at: https://www.frontiersin.org/articles/10.3389/fmicb.2021.754748/full#supplementary-material
References
Bernstein, C., Bernstein, H., Payne, C. M., Beard, S. E., and Schneider, J. (1999). Bile salt activation of stress response promoters in Escherichia coli. Curr. Microbiol. 39, 68–72. doi: 10.1007/s002849900420
Bo Andersen, J., Roldgaard, B. B., Christensen, B. B., and Licht, T. R. (2007). Oxygen restriction increases the infective potential of Listeria monocytogenes in vitro in Caco-2 cells and in vivo in guinea pigs. BMC Microbiol. 7:55. doi: 10.1186/1471-2180-7-55
Boonmee, A., Oliver, H. F., and Chaturongakul, S. (2019). Listeria monocytogenes sigma(A) is sufficient to survive gallbladder bile exposure. Front. Microbiol. 10:2070. doi: 10.3389/fmicb.2019.02070
Borezee, E., Pellegrini, E., and Berche, P. (2000). OppA of Listeria monocytogenes, an oligopeptide-binding protein required for bacterial growth at low temperature and involved in intracellular survival. Infect. Immun. 68, 7069–7077. doi: 10.1128/IAI.68.12.7069-7077.2000
Bowman, J. P., Hages, E., Nilsson, R. E., Kocharunchitt, C., and Ross, T. (2012). Investigation of the Listeria monocytogenes Scott A acid tolerance response and associated physiological and phenotypic features via whole proteome analysis. J. Proteome Res. 11, 2409–2426. doi: 10.1021/pr201137c
Burkholder, K. M., Kim, K. P., Mishra, K. K., Medina, S., Hahm, B. K., Kim, H., et al. (2009). Expression of LAP, a SecA2-dependent secretory protein, is induced under anaerobic environment. Microbes Infect. 11, 859–867. doi: 10.1016/j.micinf.2009.05.006
Chang, C., and Stewart, R. C. (1998). The two-component system. Regulation of diverse signaling pathways in prokaryotes and eukaryotes. Plant Physiol. 117, 723–731. doi: 10.1104/pp.117.3.723
Chatterjee, S. S., Hossain, H., Otten, S., Kuenne, C., Kuchmina, K., Machata, S., et al. (2006). Intracellular gene expression profile of Listeria monocytogenes. Infect. Immun. 74, 1323–1338. doi: 10.1128/IAI.74.2.1323-1338.2006
Cheng, C., Wang, X., Dong, Z., Shao, C., Yang, Y., Fang, W., et al. (2015). Aminopeptidase T of M29 family acts as a novel intracellular virulence factor for Listeria monocytogenes infection. Sci. Rep. 5:17370. doi: 10.1038/srep17370
Chourashi, R., Mondal, M., Sinha, R., Debnath, A., Das, S., Koley, H., et al. (2016). Role of a sensor histidine kinase ChiS of Vibrio cholerae in pathogenesis. Int. J. Med. Microbiol. 306, 657–665. doi: 10.1016/j.ijmm.2016.09.003
Coleman, R., Iqbal, S., Godfrey, P. P., and Billington, D. (1979). Membranes and bile formation. Composition of several mammalian biles and their membrane-damaging properties. Biochem. J. 178, 201–208. doi: 10.1042/bj1780201
Conesa, A., Gotz, S., Garcia-Gomez, J. M., Terol, J., Talon, M., and Robles, M. (2005). Blast2GO: a universal tool for annotation, visualization and analysis in functional genomics research. Bioinformatics 21, 3674–3676. doi: 10.1093/bioinformatics/bti610
Corbett, D., Wang, J., Schuler, S., Lopez-Castejon, G., Glenn, S., Brough, D., et al. (2012). Two zinc uptake systems contribute to the full virulence of Listeria monocytogenes during growth in vitro and in vivo. Infect. Immun. 80, 14–21. doi: 10.1128/IAI.05904-11
Cotter, P. D., Gahan, C. G., and Hill, C. (2001). A glutamate decarboxylase system protects Listeria monocytogenes in gastric fluid. Mol. Microbiol. 40, 465–475. doi: 10.1046/j.1365-2958.2001.02398.x
Cui, G., Wang, J., Qi, X., and Su, J. (2018). Transcription elongation factor GreA plays a key role in cellular invasion and virulence of Francisella tularensis subsp. novicida. Sci. Rep. 8:6895. doi: 10.1038/s41598-018-25271-5
Davis, M. J., Coote, P. J., and O’Byrne, C. P. (1996). Acid tolerance in Listeria monocytogenes: the adaptive acid tolerance response (ATR) and growth-phase-dependent acid resistance. Microbiology 142(Pt 10), 2975–2982. doi: 10.1099/13500872-142-10-2975
Dons, L., Eriksson, E., Jin, Y., Rottenberg, M. E., Kristensson, K., Larsen, C. N., et al. (2004). Role of flagellin and the two-component CheA/CheY system of Listeria monocytogenes in host cell invasion and virulence. Infect. Immun. 72, 3237–3244. doi: 10.1128/IAI.72.6.3237-3244.2004
Dowd, G. C., Joyce, S. A., Hill, C., and Gahan, C. G. (2011). Investigation of the mechanisms by which Listeria monocytogenes grows in porcine gallbladder bile. Infect. Immun. 79, 369–379. doi: 10.1128/IAI.00330-10
Dramsi, S., Dehoux, P., Lebrun, M., Goossens, P. L., and Cossart, P. (1997). Identification of four new members of the internalin multigene family of Listeria monocytogenes EGD. Infect. Immun. 65, 1615–1625. doi: 10.1128/iai.65.5.1615-1625.1997
Espey, M. G. (2013). Role of oxygen gradients in shaping redox relationships between the human intestine and its microbiota. Free Radic. Biol. Med. 55, 130–140. doi: 10.1016/j.freeradbiomed.2012.10.554
Garriga, X., Eliasson, R., Torrents, E., Jordan, A., Barbe, J., Gibert, I., et al. (1996). nrdD and nrdG genes are essential for strict anaerobic growth of Escherichia coli. Biochem. Biophys. Res. Commun. 229, 189–192. doi: 10.1006/bbrc.1996.1778
Grove, A. (2013). MarR family transcription factors. Curr. Biol. 23, R142–R143. doi: 10.1016/j.cub.2013.01.013
Guariglia-Oropeza, V., Orsi, R. H., Guldimann, C., Wiedmann, M., and Boor, K. J. (2018). The Listeria monocytogenes bile stimulon under acidic conditions is characterized by strain-specific patterns and the upregulation of motility, cell wall modification functions, and the PrfA regulon. Front. Microbiol. 9:120. doi: 10.3389/fmicb.2018.00120
Hardy, J., Francis, K. P., DeBoer, M., Chu, P., Gibbs, K., and Contag, C. H. (2004). Extracellular replication of Listeria monocytogenes in the murine gall bladder. Science 303, 851–853. doi: 10.1126/science.1092712
Harris, J., Paul, O., Park, S. H., White, S. J., Budachetri, K., McClung, D. M., et al. (2019). Oxygen deprivation influences the survival of Listeria monocytogenes in gerbils. Transl. Anim. Sci. 3, 102–112. doi: 10.1093/tas/txy110
He, G., Shankar, R. A., Chzhan, M., Samouilov, A., Kuppusamy, P., and Zweier, J. L. (1999). Noninvasive measurement of anatomic structure and intraluminal oxygenation in the gastrointestinal tract of living mice with spatial and spectral EPR imaging. Proc. Natl. Acad. Sci. U.S.A. 96, 4586–4591. doi: 10.1073/pnas.96.8.4586
Isabella, V. M., and Clark, V. L. (2011). Identification of a conserved protein involved in anaerobic unsaturated fatty acid synthesis in Neiserria gonorrhoeae: implications for facultative and obligate anaerobes that lack FabA. Mol. Microbiol. 82, 489–501. doi: 10.1111/j.1365-2958.2011.07826.x
Jydegaard-Axelsen, A. M., Hoiby, P. E., Holmstrom, K., Russell, N., and Knochel, S. (2004). CO2- and anaerobiosis-induced changes in physiology and gene expression of different Listeria monocytogenes strains. Appl. Environ. Microbiol. 70, 4111–4117. doi: 10.1128/AEM.70.7.4111-4117.2004
Karatzas, K. A., Wouters, J. A., Gahan, C. G., Hill, C., Abee, T., and Bennik, M. H. (2003). The CtsR regulator of Listeria monocytogenes contains a variant glycine repeat region that affects piezotolerance, stress resistance, motility and virulence. Mol. Microbiol. 49, 1227–1238. doi: 10.1046/j.1365-2958.2003.03636.x
Kaspar, D., Auer, F., Schardt, J., Schindele, F., Ospina, A., Held, C., et al. (2014). Temperature- and nitrogen source-dependent regulation of GlnR target genes in Listeria monocytogenes. FEMS Microbiol. Lett. 355, 131–141. doi: 10.1111/1574-6968.12458
Kaval, K. G., Hahn, B., Tusamda, N., Albrecht, D., and Halbedel, S. (2015). The PadR-like transcriptional regulator LftR ensures efficient invasion of Listeria monocytogenes into human host cells. Front. Microbiol. 6:772. doi: 10.3389/fmicb.2015.00772
Krell, T., Lacal, J., Busch, A., Silva-Jimenez, H., Guazzaroni, M. E., and Ramos, J. L. (2010). Bacterial sensor kinases: diversity in the recognition of environmental signals. Annu. Rev. Microbiol. 64, 539–559. doi: 10.1146/annurev.micro.112408.134054
Kuenemann, M. A., Spears, P. A., Orndorff, P. E., and Fourches, D. (2018). In silico predicted glucose-1-phosphate uridylyltransferase (GalU) inhibitors block a key pathway required for Listeria virulence. Mol. Inform. 37:e1800004. doi: 10.1002/minf.201800004
Linnan, M. J., Mascola, L., Lou, X. D., Goulet, V., May, S., Salminen, C., et al. (1988). Epidemic listeriosis associated with Mexican-style cheese. N. Engl. J. Med. 319, 823–828. doi: 10.1056/NEJM198809293191303
Liu, Y., and Ream, A. (2008). Gene expression profiling of Listeria monocytogenes strain F2365 during growth in ultrahigh-temperature-processed skim milk. Appl. Environ. Microbiol. 74, 6859–6866. doi: 10.1128/AEM.00356-08
Mariscotti, J. F., Quereda, J. J., Garcia-Del Portillo, F., and Pucciarelli, M. G. (2014). The Listeria monocytogenes LPXTG surface protein Lmo1413 is an invasin with capacity to bind mucin. Int. J. Med. Microbiol. 304, 393–404. doi: 10.1016/j.ijmm.2014.01.003
Monte, M. J., Marin, J. J., Antelo, A., and Vazquez-Tato, J. (2009). Bile acids: chemistry, physiology, and pathophysiology. World J. Gastroenterol. 15, 804–816. doi: 10.3748/wjg.15.804
Nakano, M. M., Zhu, Y., Haga, K., Yoshikawa, H., Sonenshein, A. L., and Zuber, P. (1999). A mutation in the 3-phosphoglycerate kinase gene allows anaerobic growth of Bacillus subtilis in the absence of ResE kinase. J. Bacteriol. 181, 7087–7097. doi: 10.1128/JB.181.22.7087-7097.1999
Nelson, K. E., Fouts, D. E., Mongodin, E. F., Ravel, J., DeBoy, R. T., Kolonay, J. F., et al. (2004). Whole genome comparisons of serotype 4b and 1/2a strains of the food-borne pathogen Listeria monocytogenes reveal new insights into the core genome components of this species. Nucleic Acids Res. 32, 2386–2395. doi: 10.1093/nar/gkh562
Nevo, Y., and Nelson, N. (2006). The NRAMP family of metal-ion transporters. Biochim. Biophys. Acta 1763, 609–620. doi: 10.1016/j.bbamcr.2006.05.007
Niu, S., Luo, M., Tang, J., Zhou, H., Zhang, Y., Min, X., et al. (2013). Structural basis of the novel S. pneumoniae virulence factor, GHIP, a glycosyl hydrolase 25 participating in host-cell invasion. PLoS One 8:e68647. doi: 10.1371/journal.pone.0068647
Ofer, A., Kreft, J., Logan, D. T., Cohen, G., Borovok, I., and Aharonowitz, Y. (2011). Implications of the inability of Listeria monocytogenes EGD-e to grow anaerobically due to a deletion in the class III NrdD ribonucleotide reductase for its use as a model laboratory strain. J. Bacteriol. 193, 2931–2940. doi: 10.1128/JB.01405-10
O’Neil, H. S., and Marquis, H. (2006). Listeria monocytogenes flagella are used for motility, not as adhesins, to increase host cell invasion. Infect. Immun. 74, 6675–6681. doi: 10.1128/IAI.00886-06
Parsons, C., Lee, S., Jayeola, V., and Kathariou, S. (2017). Novel cadmium resistance determinant in Listeria monocytogenes. Appl. Environ. Microbiol. 83:e02580-16. doi: 10.1128/AEM.02580-16
Paruthiyil, S., Pinochet-Barros, A., Huang, X., and Helmann, J. D. (2020). Bacillus subtilis TerC family proteins help prevent manganese intoxication. J. Bacteriol. 202:e00624-19. doi: 10.1128/JB.00624-19
Paudyal, R., O’Byrne, C. P., and Karatzas, K. A. (2020). Amino acids other than glutamate affect the expression of the GAD system in Listeria monocytogenes enhancing acid resistance. Food Microbiol. 90:103481. doi: 10.1016/j.fm.2020.103481
Payne, A., Schmidt, T. B., Nanduri, B., Pendarvis, K., Pittman, J. R., Thornton, J. A., et al. (2013). Proteomic analysis of the response of Listeria monocytogenes to bile salts under anaerobic conditions. J. Med. Microbiol. 62(Pt 1), 25–35. doi: 10.1099/jmm.0.049742-0
Prieto, A. I., Ramos-Morales, F., and Casadesus, J. (2004). Bile-induced DNA damage in Salmonella enterica. Genetics 168, 1787–1794. doi: 10.1534/genetics.104.031062
Prieto, A. I., Ramos-Morales, F., and Casadesus, J. (2006). Repair of DNA damage induced by bile salts in Salmonella enterica. Genetics 174, 575–584. doi: 10.1534/genetics.106.060889
Quillin, S. J., Schwartz, K. T., and Leber, J. H. (2011). The novel Listeria monocytogenes bile sensor BrtA controls expression of the cholic acid efflux pump MdrT. Mol. Microbiol. 81, 129–142. doi: 10.1111/j.1365-2958.2011.07683.x
Scallan, E., Hoekstra, R. M., Angulo, F. J., Tauxe, R. V., Widdowson, M. A., Roy, S. L., et al. (2011). Foodborne illness acquired in the United States–major pathogens. Emerg. Infect. Dis 17, 7–15. doi: 10.3201/eid1701.P11101
Scortti, M., Monzo, H. J., Lacharme-Lora, L., Lewis, D. A., and Vazquez-Boland, J. A. (2007). The PrfA virulence regulon. Microbes Infect. 9, 1196–1207. doi: 10.1016/j.micinf.2007.05.007
Slamti, L., and Lereclus, D. (2019). The oligopeptide ABC-importers are essential communication channels in Gram-positive bacteria. Res. Microbiol. 170, 338–344. doi: 10.1016/j.resmic.2019.07.004
Sleator, R. D., and Hill, C. (2005). A novel role for the LisRK two-component regulatory system in listerial osmotolerance. Clin. Microbiol. Infect. 11, 599–601. doi: 10.1111/j.1469-0691.2005.01176.x
Stock, A. M., Robinson, V. L., and Goudreau, P. N. (2000). Two-component signal transduction. Annu. Rev. Biochem. 69, 183–215. doi: 10.1146/annurev.biochem.69.1.183
Supuran, C. T. (2016). Legionella pneumophila carbonic anhydrases: underexplored antibacterial drug targets. Pathogens 5:44. doi: 10.3390/pathogens5020044
Tessema, G. T., Moretro, T., Snipen, L., Heir, E., Holck, A., Naterstad, K., et al. (2012). Microarray-based transcriptome of Listeria monocytogenes adapted to sublethal concentrations of acetic acid, lactic acid, and hydrochloric acid. Can. J. Microbiol. 58, 1112–1123. doi: 10.1139/w2012-091
Thigpen, M. C., Whitney, C. G., Messonnier, N. E., Zell, E. R., Lynfield, R., Hadler, J. L., et al. (2011). Bacterial meningitis in the United States, 1998-2007. N. Engl. J. Med. 364, 2016–2025. doi: 10.1056/NEJMoa1005384
Torrents, E. (2014). Ribonucleotide reductases: essential enzymes for bacterial life. Front. Cell Infect. Microbiol. 4:52. doi: 10.3389/fcimb.2014.00052
Trapnell, C., Hendrickson, D. G., Sauvageau, M., Goff, L., Rinn, J. L., and Pachter, L. (2013). Differential analysis of gene regulation at transcript resolution with RNA-seq. Nat. Biotechnol. 31, 46–53. doi: 10.1038/nbt.2450
Trapnell, C., Pachter, L., and Salzberg, S. L. (2009). TopHat: discovering splice junctions with RNA-Seq. Bioinformatics 25, 1105–1111. doi: 10.1093/bioinformatics/btp120
Trapnell, C., Williams, B. A., Pertea, G., Mortazavi, A., Kwan, G., van Baren, M. J., et al. (2010). Transcript assembly and quantification by RNA-Seq reveals unannotated transcripts and isoform switching during cell differentiation. Nat. Biotechnol. 28, 511–515. doi: 10.1038/nbt.1621
Turner, A. G., Ong, C. L., Gillen, C. M., Davies, M. R., West, N. P., McEwan, A. G., et al. (2015). Manganese homeostasis in group A Streptococcus is critical for resistance to oxidative stress and virulence. mBio 6:e00278-15. doi: 10.1128/mBio.00278-15
van der Veen, S., and Abee, T. (2011). Contribution of Listeria monocytogenes RecA to acid and bile survival and invasion of human intestinal Caco-2 cells. Int. J. Med. Microbiol. 301, 334–340. doi: 10.1016/j.ijmm.2010.11.006
Volkov, A., Liavonchanka, A., Kamneva, O., Fiedler, T., Goebel, C., Kreikemeyer, B., et al. (2010). Myosin cross-reactive antigen of Streptococcus pyogenes M49 encodes a fatty acid double bond hydratase that plays a role in oleic acid detoxification and bacterial virulence. J. Biol. Chem. 285, 10353–10361. doi: 10.1074/jbc.M109.081851
West, A. H., and Stock, A. M. (2001). Histidine kinases and response regulator proteins in two-component signaling systems. Trends Biochem. Sci. 26, 369–376. doi: 10.1016/s0968-0004(01)01852-7
White, S. J., McClung, D. M., Wilson, J. G., Roberts, B. N., and Donaldson, J. R. (2015). Influence of pH on bile sensitivity amongst various strains of Listeria monocytogenes under aerobic and anaerobic conditions. J. Med. Microbiol. 64, 1287–1296. doi: 10.1099/jmm.0.000160
Wright, M. L., Pendarvis, K., Nanduri, B., Edelmann, M. J., Jenkins, H. N., Reddy, J. S., et al. (2016). The effect of oxygen on bile resistance in Listeria monocytogenes. J. Proteomics Bioinform. 9, 107–119. doi: 10.4172/jpb.1000396
Wu, H., Qiao, S., Li, D., Guo, L., Zhu, M., and Ma, L. Z. (2019). Crystal structure of the glycoside hydrolase PssZ from Listeria monocytogenes. Acta Crystallogr. F Struct. Biol. Commun. 75(Pt 7), 501–506. doi: 10.1107/S2053230X19008100
Keywords: Listeria monocytogenes, transcriptomics, anaerobiosis, bile, stress response, anaerobic
Citation: Chakravarty D, Sahukhal G, Arick M II, Davis ML and Donaldson JR (2021) Transcriptomic Analysis of Listeria monocytogenes in Response to Bile Under Aerobic and Anaerobic Conditions. Front. Microbiol. 12:754748. doi: 10.3389/fmicb.2021.754748
Received: 06 August 2021; Accepted: 18 October 2021;
Published: 11 November 2021.
Edited by:
Aleksandra P. Djukic-Vukovic, University of Belgrade, SerbiaReviewed by:
Stephan Schmitz-Esser, Iowa State University, United StatesYvonne Sun, University of Dayton, United States
Copyright © 2021 Chakravarty, Sahukhal, Arick, Davis and Donaldson. This is an open-access article distributed under the terms of the Creative Commons Attribution License (CC BY). The use, distribution or reproduction in other forums is permitted, provided the original author(s) and the copyright owner(s) are credited and that the original publication in this journal is cited, in accordance with accepted academic practice. No use, distribution or reproduction is permitted which does not comply with these terms.
*Correspondence: Janet R. Donaldson, janet.donaldson@usm.edu