- 1Department of Pharmacy and Biotechnology, Alma Mater Studiorum – University of Bologna, Bologna, Italy
- 2Department of Agricultural and Food Sciences, Centre of Foodomics, Alma Mater Studiorum – University of Bologna, Bologna, Italy
- 3National Institute of Geophysics and Volcanology, Bologna, Italy
- 4Centro de Referencia para Lactobacilos (CERELA)-CONICET, San Miguel de Tucumán, Argentina
- 5Department of Diagnostic and Specialty Medicine, Section of Microbiology, Alma Mater Studiorum – University of Bologna, Bologna, Italy
Lactobacilli are the dominant members of the healthy human vaginal microbiota and represent the first defense line from pathogen infection, including vulvovaginal candidiasis. Biofilm is the predominant microbial growth form in nature, and the formation of biofilms inside the human body has important implications in health and disease. In particular, the formation of biofilm by members of the human resident microbiota is desirable, as it can improve microbial persistence and influence functionality. In the present study, we investigated the capability of 16 vaginal Lactobacillus strains (belonging to Lactobacillus crispatus, Lactobacillus gasseri, Lactobacillus vaginalis, and Lactobacillus plantarum species) to form biofilms, and we correlated their mode of growth to anti-Candida activity. L. plantarum strains were the best biofilm producers, and high variability was registered in the level of biofilm formation among L. crispatus and L. gasseri strains. Culture supernatants derived from Lactobacillus biofilm and planktonic growth were tested toward a panel of Candida clinical isolates (Candida albicans, Candida glabrata, Candida lusitaniae, Candida tropicalis, Candida krusei, and Candida parapsilosis) and their metabolome assessed by 1H-NMR. L. crispatus and L. plantarum strains exhibited the best fungistatic profile, and biofilms enhanced their anti-Candida activity; on the contrary, L. gasseri strains were more effective when grown in a planktonic mode. Biofilm/planktonic mode of growth also affects Lactobacillus metabolism, mainly influencing nitrogen and amino acid pathways, and anti-Candida activity is instead strictly related to carbohydrate metabolism. The present study underlined the strict interdependence between microbial mode of growth, metabolism, and functional properties. Biofilm formation by members of the healthy human microbiota represents a crucial issue in the field of microbial physiology and host–microbiota interactions, beyond supporting the development of new antimycotic strategies based on probiotics grown in adherence.
Introduction
Members of the Lactobacillus genus are abundant and predominant in the vaginal niche of healthy women of reproductive age, reaching a concentration of 107 cfu/ml of vaginal sample, and 80% of the whole microbial content (Rönnqvist et al., 2006; Ceccarani et al., 2019). Most healthy women show the dominance of one species of Lactobacillus, and Lactobacillus crispatus, Lactobacillus iners, Lactobacillus jensenii, and Lactobacillus gasseri are the most frequent (Ravel et al., 2011). It is widely demonstrated that vaginal lactobacilli are involved in maintaining the state of vaginal eubiosis, preventing the colonization of genital, and sexually transmitted pathogens. Indeed, it has been reported that various vaginal Lactobacillus strains can interfere with Candida spp. overgrowth and reduce vulvovaginal candidiasis occurrence (Parolin et al., 2015; De Gregorio et al., 2019; Namarta et al., 2020). Anti-Candida activity of lactobacilli can be mediated through multiple mechanisms, including pH lowering, secretion of effective compounds, and impairment of fungal adhesion (Parolin et al., 2015, 2021; Calonghi et al., 2017; Abruzzo et al., 2018, 2021; De Gregorio et al., 2019).
Microorganisms’ growth can occur in two different forms: planktonic and biofilm, and the latter is the predominant form in nature (Rieu et al., 2014). The biofilm mode of growth is characterized by high cell density and secretion of a polymeric matrix, in which microorganisms are immersed (Flemming and Wingender, 2010). The formation of microbial biofilms inside the human body has important implications in health and disease: for example, pathogens’ biofilms generally show increased resistance to antibiotics, making their elimination challenging. On the other hand, the formation of biofilm by members of the human resident microbiota is desirable, as it can improve microbial persistence and favor functionality (Li et al., 2018). The planktonic growth or the alternative establishment of a sessile biofilm by a microbial species implies the remodeling of physiological and metabolic pathways, influencing in turn functional properties of probiotics. For some Lactobacillus species, it has been reported that biofilm mode of life can improve antagonistic effects toward bacterial pathogens and anti-inflammatory and immunomodulatory properties (Rieu et al., 2014; Aoudia et al., 2016; Liu et al., 2021), although few studies have investigated this issue till now.
In the present manuscript, 16 vaginal Lactobacillus strains, belonging to L. crispatus, L. gasseri, Lactobacillus vaginalis, and Lactobacillus plantarum species, were tested for their in vitro capacity to form biofilms. Culture supernatants derived from planktonic and biofilm growth were tested against a panel of Candida clinical isolates (Candida albicans, Candida glabrata, Candida lusitaniae, Candida tropicalis, Candida krusei, and Candida parapsilosis). Anti-Candida activity was then correlated with lactobacilli culture supernatants metabolome, assessed by 1H-NMR.
Materials and Methods
Microorganisms and Culture Conditions
All the 16 Lactobacillus strains included in this study were previously isolated from vaginal swabs of healthy premenopausal Caucasian women, following the protocol approved by the Ethics Committee of the University of Bologna, Bologna, Italy (52/2014/U/Tess) (Parolin et al., 2015). Lactobacillus strains are listed in Table 1. Lactobacilli were routinely cultured in de Man, Rogosa, and Sharpe (MRS) broth (Difco, Detroit, MI, United States) supplemented with 0.05% L-cysteine (Merck, Milan, Italy), at 37°C and in anaerobiosis. Anaerobic conditions were achieved by using jars containing GasPakTM (GasPakTM EZ Anaerobe Container System, Becton, Dickinson and Co., Sparks, MD, United States). Before the experiments, each strain was transferred from the frozen stock to MRS broth and allowed to grow for 24 h and then subcultured in the same medium and conditions for an additional 24 h.
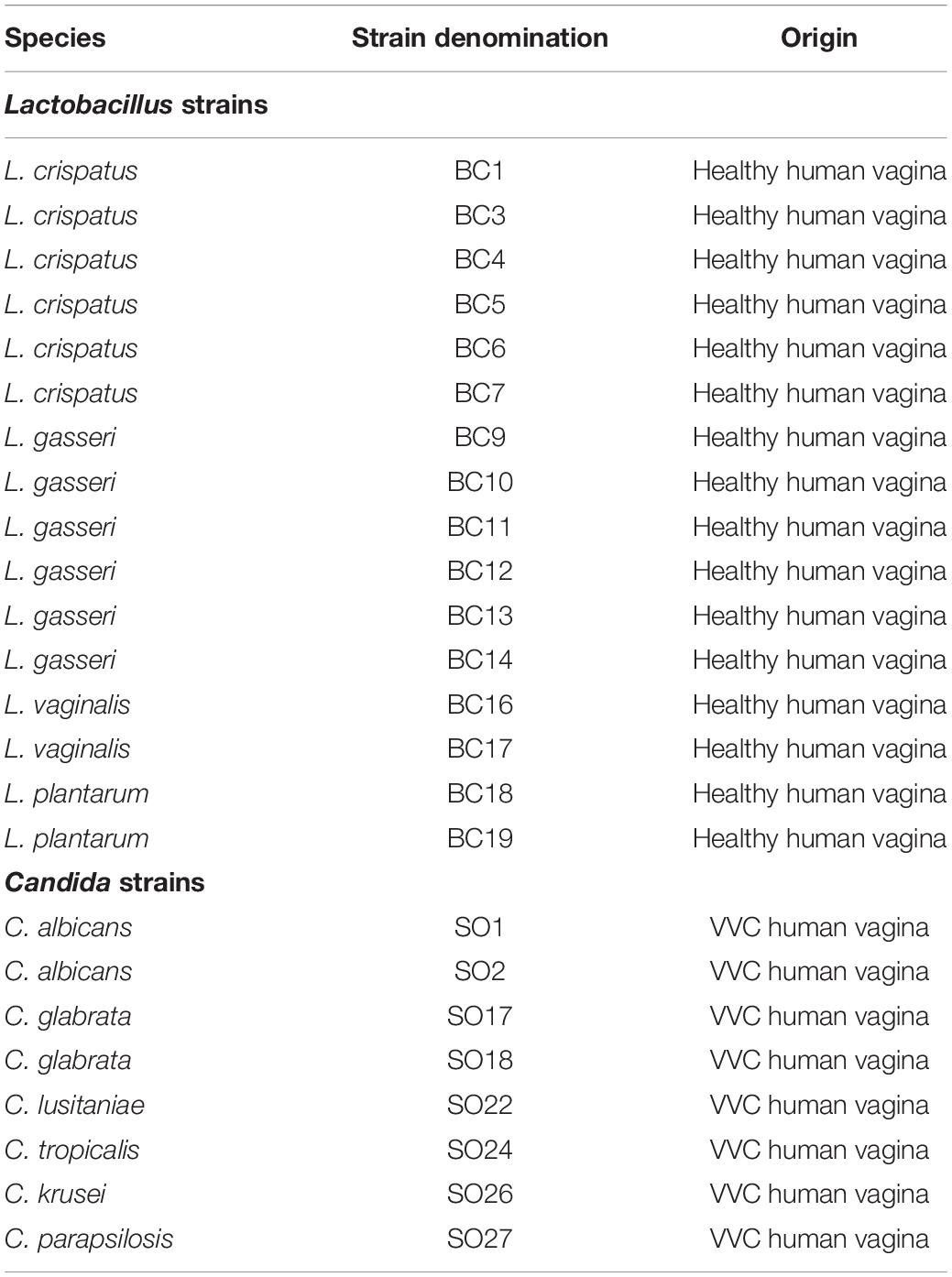
Table 1. List of microorganisms used in the present manuscript and origin (VVC: vulvovaginal candidiasis).
The eight Candida strains used in the present study belong to a broad collection of yeasts isolated from vaginal swabs of premenopausal, VVC-affected women during routine diagnostic procedures at the “Microbiology Laboratory” in Sant’Orsola-Malpighi University Hospital of Bologna, Bologna, Italy. All the clinical isolates were coded to assure full anonymousness and are listed in Table 1 (Oliver et al., 2020; Parolin et al., 2021). Candida strains were grown aerobically in Sabouraud dextrose (SD) medium (Difco) at 35°C in aerobiosis.
Lactobacillus Biofilm Formation Assay
To assess Lactobacillus strains’ capability to form biofilms, bacterial pellets from the second subculture were washed in sterile saline and resuspended in MRS broth to a final concentration of 107 colony-forming units (cfu) per milliliter. The bacterial suspension was inoculated in polystyrene, U-bottom 96-well plates (0.2 ml per well) and allowed to grow for 72 h at 37°C, in anaerobiosis. At the end of the incubation, biofilm formation was evaluated by crystal violet staining, following the protocol described by Leccese Terraf et al. (2014). Briefly, culture supernatant was discarded and wells were gently washed with phosphate buffer, pH 7.4. Adherent biofilm was stained with 0.1% (w/v) crystal violet (CV) aqueous solution for 30 min and then washed with distilled water. Plates were dried overnight at room temperature, and the CV bound to the adherent biofilm was solubilized in 30% (v/v) acetic acid. CV absorbance was measured at 595 nm with an Enspire multiplate reader (Perkin Elmer, United States). Each strain was assayed in at least three independent experiments, each with four replicates. Additionally, a sterile culture medium was always included as negative control.
Preparation of Cell-Free Supernatants From Lactobacillus Planktonic and Biofilm Cultures
Lactobacillus strains were grown in planktonic and biofilm forms and culture supernatants collected. 107 cfu/ml bacterial suspensions were prepared as described above and inoculated in sterile glass tubes (10 ml, planktonic form) or polystyrene six-well plates (4 ml per well, biofilm form). Planktonic (pk) and biofilm (bf) samples were incubated for 72 h at 37°C, in anaerobiosis. Afterward, culture supernatants were recovered, centrifuged (2,750 × g, 10 min) and filtered through a 0.22-μm membrane filter to obtain cell-free supernatants (CFS). CFS obtained from planktonic (pk-CFS) and biofilm (bf-CFS) cultures were stored at −20°C until their use.
Metabolomics of Lactobacillus Cell-Free Supernatants
After centrifugation at 18,630 × g for 10 min at 4°C, 0.7 ml of pk-CFS and bf-CFS samples were added to 0.1 ml of a D2O solution of 3-(trimethylsilyl)-propionic-2,2,3,3-d4 acid sodium salt (TSP) 10 mM, set to pH 7.0 by means of a 1 M phosphate buffer. The solution contained also NaN3 2 mM, to avoid microorganisms’ proliferation. 1H-NMR spectra were recorded with an AVANCE III spectrometer (Bruker, Milan, IT, United States) at 298 K operating at a frequency of 600.13 MHz. To reduce broad signals caused by slowly tumbling molecules, a CPMG filter of 400 echoes, separated by an echo time of 400 μs, was applied. Water residual signal was reduced by presaturation. The signals were assigned by comparing their chemical shift and multiplicity with Chenomx software data bank (Chenomx Inc., Canada, ver 11.05) (Laghi et al., 2014). Metabolite concentrations were calculated and reported as differences with respect to MRS medium.
Anti-Candida Activity of Lactobacillus Supernatants
Planktonic and biofilm supernatants anti-Candida activity was tested by microdilution assay, following EUCAST guidelines (EUCAST Committee, 2020). Briefly, stock Candida suspensions prepared in sterile water at an absorbance (measured at 600 nm) of 0.008–0.137 were diluted 1:10 in RPMI 1640 medium buffered to pH 7.0 with 0.165 M MOPS (morpholinepropanesulfonic acid buffer) and added with 2% glucose. Final yeast suspensions, corresponding to 1–5 × 105 cfu/ml, were inoculated in flat-bottomed 96-well plates (0.1 ml per well) and added with the same volume of each Lactobacillus pk-CFS and bf-CFS. Positive growth control wells contained 0.1 ml of Candida suspension added with the same volume of sterile MRS medium. The plates were incubated at 35°C for 24 h; afterward, Candida growth was evaluated by reading the absorbance at 530 nm with an Enspire multiplate reader. Candida growth inhibition was calculated relative to the absorbance of the corresponding positive controls. A subset of Lactobacillus pk-CFS and bf-CFS was tested for anti-Candida activity in simulated vaginal fluid (SVF), pH 4.2, by microdilution assay, following the method described above. In brief, Candida suspensions were diluted 1:10 in SVF, composed of 3.51 g/L NaCl, 1.40 g/L KOH, 0.222 g/L Ca(OH)2, 0.018 g/L bovine serum albumin, 2 g/L lactic acid, 1 g/L acetic acid, 0.16 g/L glycerol, 0.4 g/L urea, and 5 g/L glucose. The pH was adjusted to 4.2 with HCl 0.1 N and the solution was filtered through a 0.22-μm membrane filter. CFS obtained from L. crispatus BC1, L. crispatus BC6, L. gasseri BC11, L. gasseri BC12, L. vaginalis BC17, and L. plantarum BC19 were tested.
Cell-free supernatants fungicidal activity was assessed by spotting 20 μl of active samples onto SD agar plates, then incubated at 35°C for 24/48 h. Candida viability was determined by the ability of forming colonies.
Data Analysis and Statistics
Data were analyzed by MatLab software (R2020b version 9.9.0.1524771, The MathWorks Inc., Natick, MA, United States) with the Statistics and Machine Learning Toolbox. Cluster analysis was performed by means of t-Distributed Stochastic Neighbor Embedding method (Van der Maaten and Hinton, 2008). Differences in biofilm formation were assessed by Kruskal–Wallis test followed by Tukey-Kramer test, and differences in metabolite concentrations were assessed by Wilcoxon rank test. Enrichment analysis was performed on significantly different metabolites, by using MetaboAnalyst web resource1. Differences were deemed significant for p < 0.05.
Results
Ability of Vaginal Lactobacilli to Form Biofilm
Sixteen Lactobacillus strains isolated from vaginal swabs were tested for their capability to form biofilm on an abiotic polystyrene surface (Figure 1). In general, all vaginal Lactobacillus used in this study can form biofilm, although at different amounts (p < 0.05). The strongest biofilm producers were L. plantarum BC18 and BC19 strains (p < 0.05), while L. vaginalis BC16 and BC17 strains were the weakest (p < 0.05). Within L. crispatus and L. gasseri species, very high variability was observed. In particular, among L. crispatus, BC3 and BC7 exhibited the highest biofilm production; for L. gasseri, BC12 was the best producer.
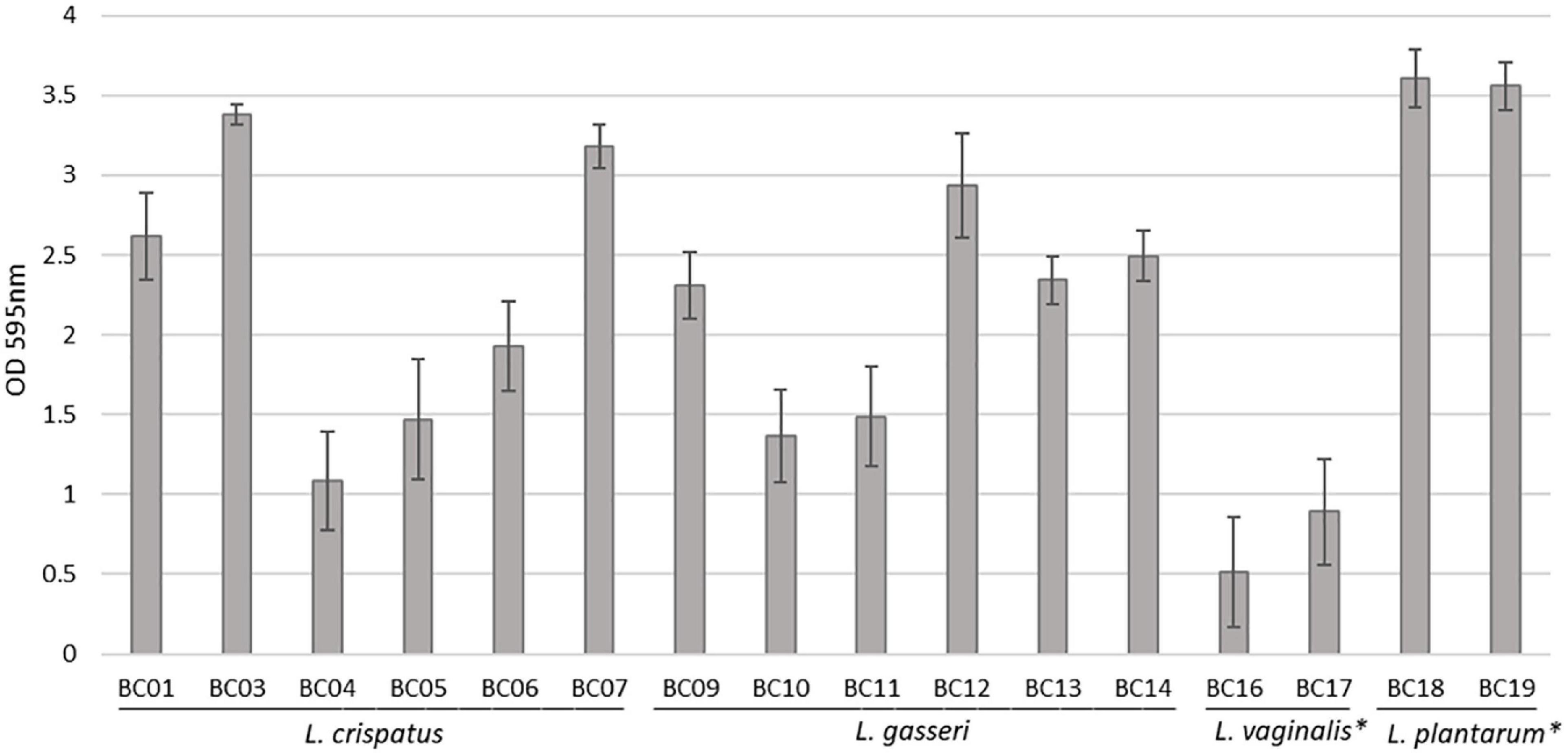
Figure 1. Biofilm formation by Lactobacillus strains, evaluated by crystal violet staining (average ± st. dev.). *p < 0.05 selected species vs. other Lactobacillus species.
Anti-Candida Activity of Lactobacilli in Planktonic/Biofilm Cultures
The fungistatic activity of bf-CFS and pk-CFS of vaginal lactobacilli was evaluated toward eight Candida clinical isolates, belonging to C. albicans and non-albicans species (Table 1). The anti-Candida activity was firstly assessed following EUCAST guidelines for antifungal susceptibility testing (EUCAST Committee, 2020), which imply RPMI supplemented with glucose and MOPS, pH 7. Overall, the most sensitive Candida isolates were C. albicans SO2, C. lusitaniae SO22, and C. parapsilosis SO27, whereas C. krusei SO26 was the most resistant to Lactobacillus CFS (Figure 2). bf-CFS from L. crispatus and L. plantarum strains showed the highest fungistatic activity toward all Candida isolates, exhibiting the best anti-Candida profile. In detail, L. crispatus and L. plantarum bf-CFS showed higher values of Candida growth inhibition than the respective pk-CFS: bf-CFS almost completely inhibited (over 95% growth inhibition) C. albicans SO2, C. glabrata SO17, C. glabrata SO18, C. lusitaniae SO22, and C. parapsilosis SO27 growth, and strongly inhibited (over 65% inhibition) C. albicans SO1 and C. tropicalis SO24. The corresponding pk-CFS showed inhibition rates ranging from 50 to 90% toward the same Candida strains. On the contrary, most L. gasseri strains exerted higher anti-Candida activity when they were cultured in planktonic mode rather than in biofilm, and great variability among Candida isolates’ inhibition was registered. Overall, L. gasseri pk-CFS were mostly active toward C. lusitaniae SO22 and C. parapsilosis SO27, and to a lesser extent on C. albicans SO2. Intermediate anti-Candida activity was shown on C. glabrata SO17, C. glabrata SO18, and C. tropicalis SO24. L. vaginalis BC16 bf-CFS and pk-CFS showed the lowest fungistatic activity against all Candida isolates, exhibiting the worst anti-Candida profile. Overall, CFS were not fungicidal, with the only exception of L. crispatus bf-CFS toward C. parapsilosis SO27 and L. plantarum bf-CFS toward C. lusitaniae SO22.
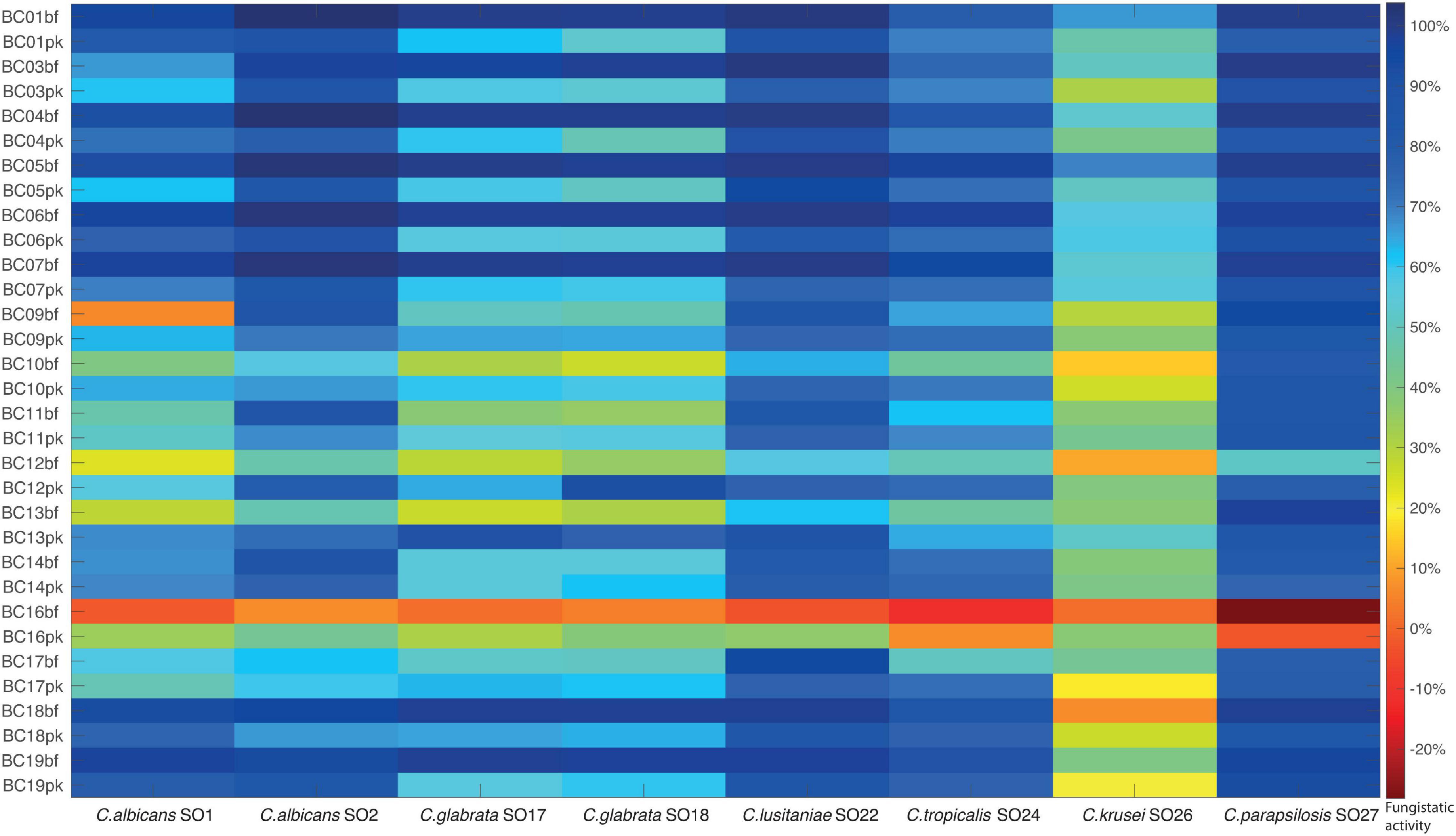
Figure 2. Fungistatic activity of Lactobacillus bf-CFS and pk-CFS toward clinical Candida isolates, tested following EUCAST guidelines (pH 7). OD 530 nm values were normalized for positive controls and data were expressed as inhibition (%) of Candida growth. Fungistatic activity color scale was reported (10% activity variation).
In order to mimic the physiological condition typically found in the vaginal environment, the fungistatic activity of CFS was also tested in SVF, pH 4.2. A subset of active CFS was selected (i.e., CFS obtained from L. crispatus BC1, L. crispatus BC6, L. gasseri BC11, L. gasseri BC12, L. vaginalis BC17, and L. plantarum BC19) and tested toward the panel of Candida isolates (Figure 3). Also in these experimental conditions, CFS showed a remarkable anti-Candida activity; interestingly, Lactobacillus CFS were highly active also toward C. glabrata SO17, C. glabrata SO18, and C. krusei SO26 (over 60% inhibition). Overall, acidic pH of SVF enhanced Candida growth inhibition by CFS, especially for pk-CFS.
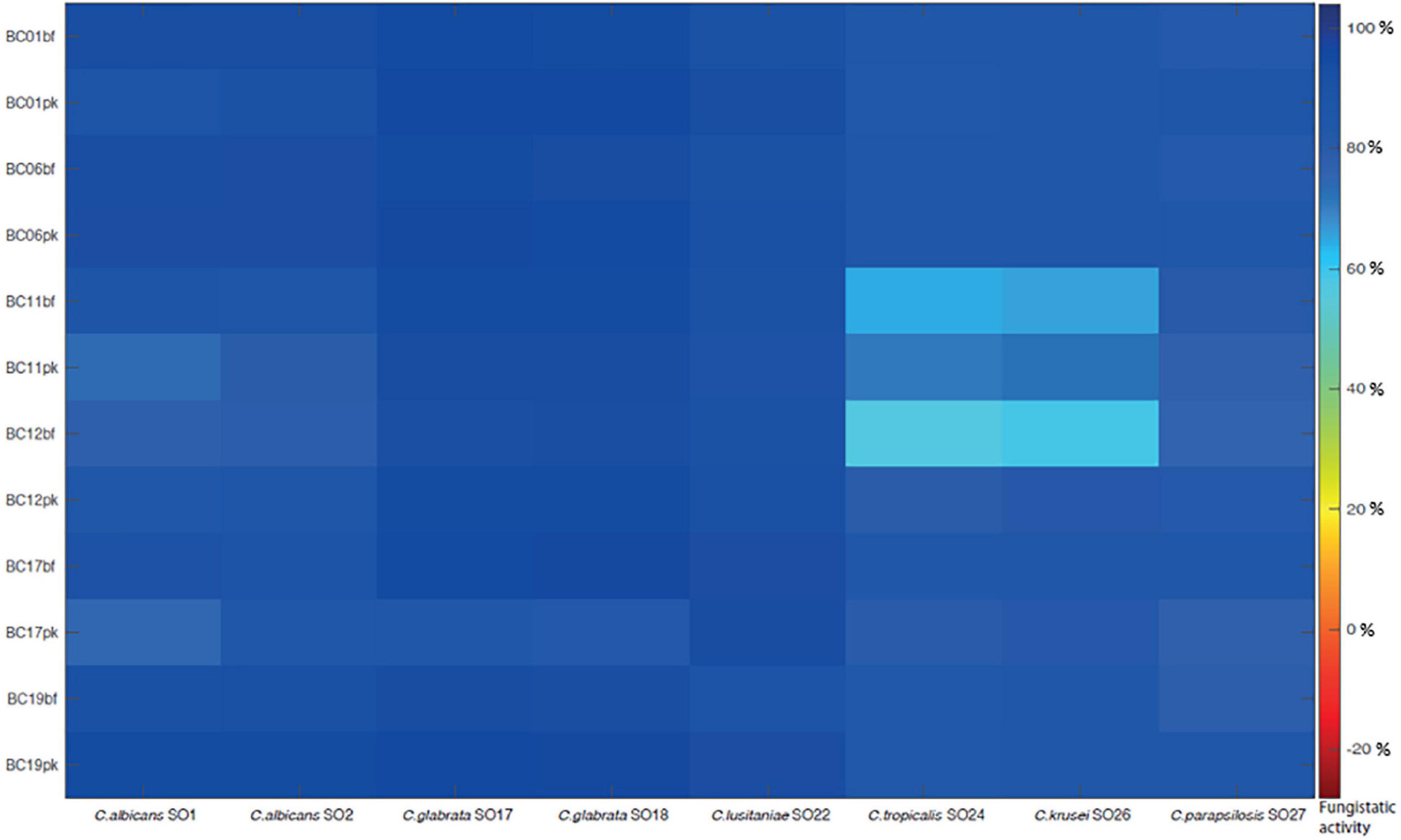
Figure 3. Fungistatic activity of L. crispatus BC1, L. crispatus BC6, L. gasseri BC11, L. gasseri BC12, L. vaginalis BC17, and L. plantarum BC19 bf-CFS and pk-CFS toward clinical Candida isolates, tested in simulated vaginal fluid, pH 4.2. OD 530 nm values were normalized for positive controls and data were expressed as inhibition (%) of Candida growth. Fungistatic activity color scale was reported.
To better understand the analogies of CFS activities toward Candida isolates, a cluster analysis was performed using the t-SNE method on data obtained following EUCAST guidelines (Figure 4). Considering the position of samples in the 3D map, we identified three groups of CFS defined as low, medium, and high activity. All L. crispatus and L. plantarum bf-CFS are grouped in the high-activity cluster, while pk-CFS from the same species and L. gasseri pk-CFS are grouped in the medium one. L. gasseri bf-CFS, except for L. gasseri BC11 and BC14 bf-CFS, are grouped with L. vaginalis BC16 CFS and demonstrated a low fungistatic activity.
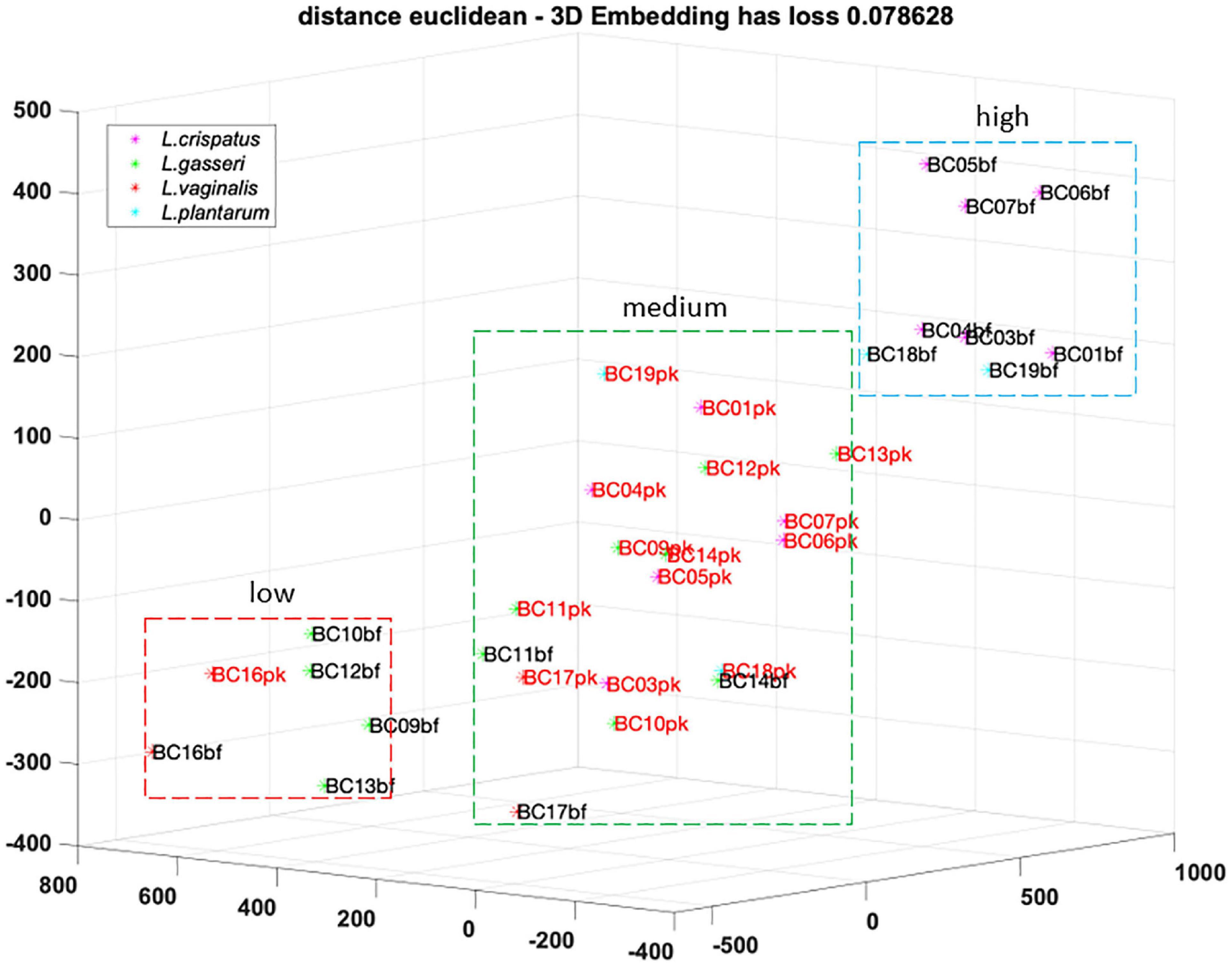
Figure 4. t-SNE cluster analysis on CFS fungistatic activity dataset. Three groups were identified, corresponding to low, medium, and high activity. Samples derived from biofilm and planktonic cultures were signed in black and red, respectively.
Lactobacillus Metabolome Analysis
In order to better characterize vaginal Lactobacillus CFS and bf-CFS and pk-CFS peculiarities, their metabolomic profiles were obtained by 1H-NMR. Fifty-one molecules belonging to the families of amino acids, organic acids, monosaccharides/disaccharides, ketones, and alcohols were identified (Figure 5), and differences were calculated with respect to MRS medium. All metabolites showed similar concentration median values, except for acetate and glucose. The same molecules also showed high variability among samples. We thus investigated the metabolites that mainly differentiate bf-CFS and pk-CFS samples, and we found out that the quantities of 17 molecules were significantly different between the two groups (Table 2). Among those, 10 amino acids (or derivatives) were identified, suggesting a deep involvement of this molecule category. Data were then subjected to overrepresentation analysis (Supplementary Figure 1), which highlighted that the growth form (bf vs. pk) mainly affects the nitrogen metabolism, and specifically several amino acidic pathways (serine, alanine, and lysine).
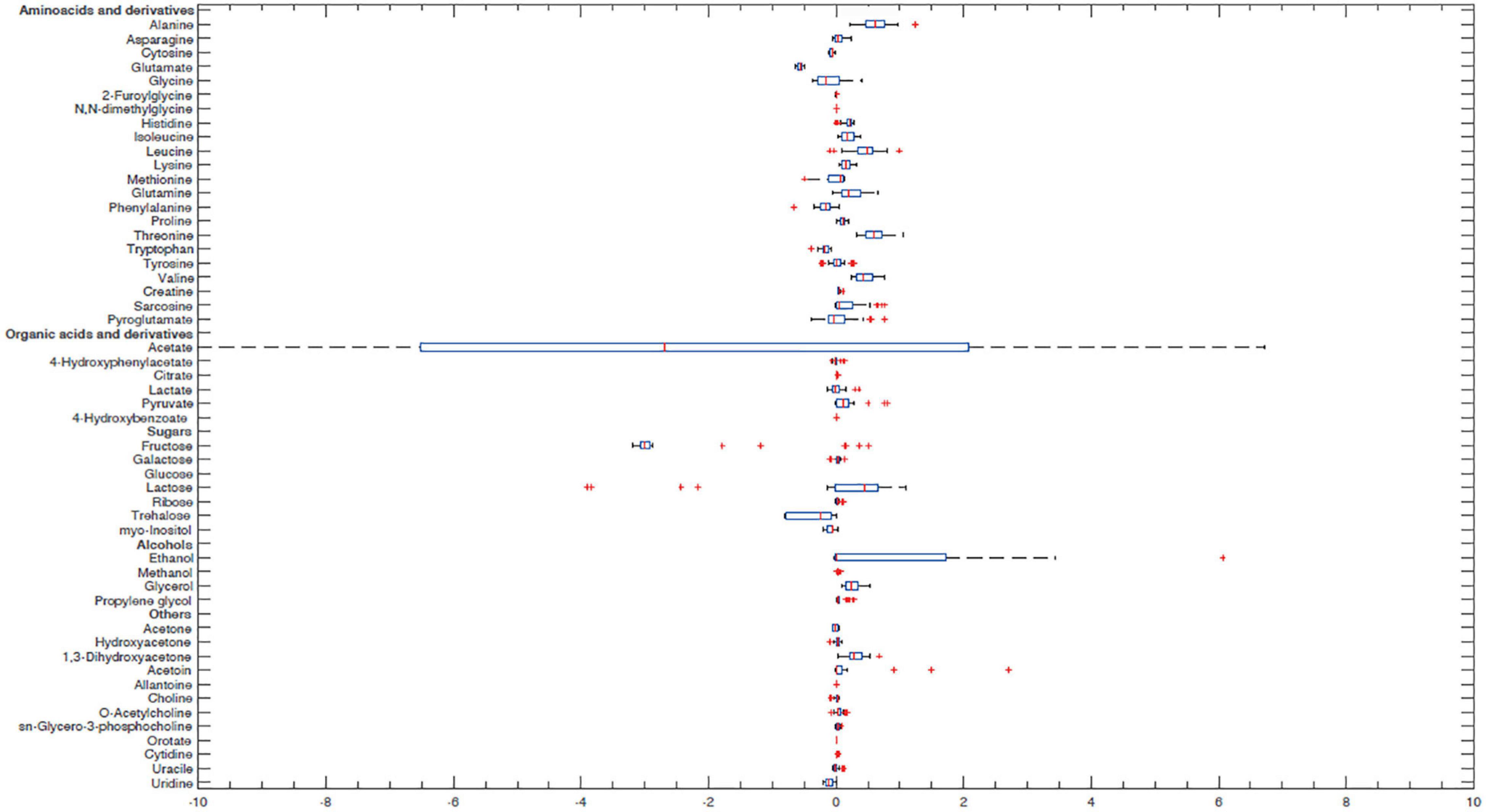
Figure 5. Box plot of Lactobacillus CFS metabolite concentrations. Concentrations were calculated as differences from MRS broth (mmol/L). Lines within the boxes indicate the median values of the samples. Each box represents the interquartile range (25–75th percentile). The extremes of the bars indicate the 10th and 90th percentiles, respectively. Outlier values are indicated (+). Glucose median and acetate interquartile range are not represented because they were out of the range considered.
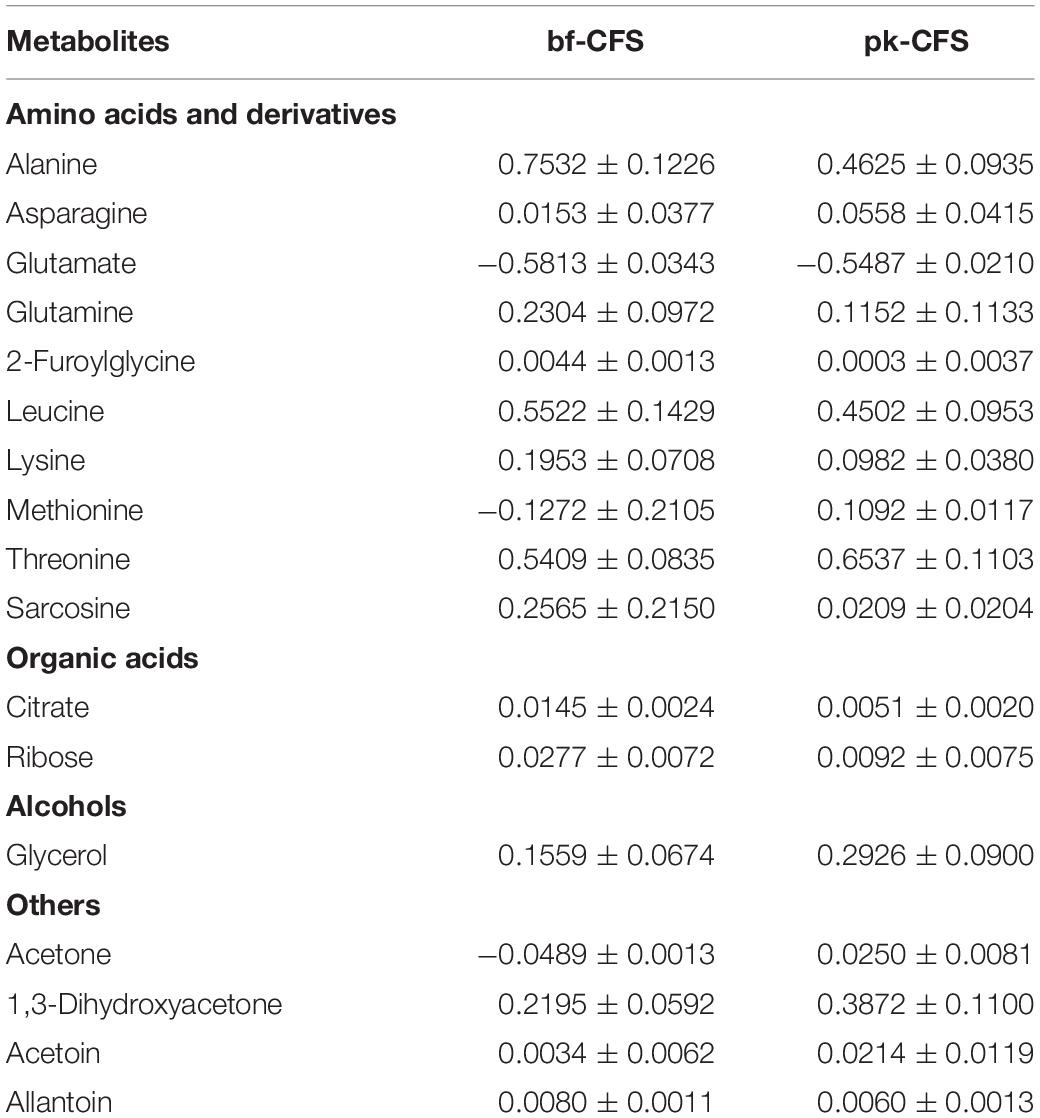
Table 2. List of metabolites significantly different between Lactobacillus bf-CFS and pk-CFS (p < 0.05) and concentrations, calculated as differences with respect to MRS medium [median value ± MAD (mmol/L)].
Correlation of Anti-Candida Activity With Supernatant Metabolomes
A principal component analysis (PCA) was performed for Lactobacillus bf-CFS and pk-CFS considering metabolomic and activity against Candida (Figure 6A). In the biplot, PC1 and PC2 represented the 44.3% of the total variance of the investigated samples (PC1 26.2%; PC2 18.1%). Lactobacillus CFS with different anti-Candida activity separated on PC1. In particular, L. crispatus and L. plantarum bf-CFS, which belong to the high anti-Candida activity group, were characterized by low values of PC1, while the low activity CFS samples showed high values of PC1 (Figure 6B). In order to identify the metabolites that mainly contribute to the anti-Candida activity of Lactobacillus CFS, we compared the metabolome of the active CFS to that of the less active ones. We found out that 18 molecule amounts were significantly different in the two groups (Table 3); most of them are represented by carbohydrates and amino acids. Enrichment analysis suggested a significant difference between the two CFS groups in the metabolism of sugars, mainly involving lactose and galactose pathways.
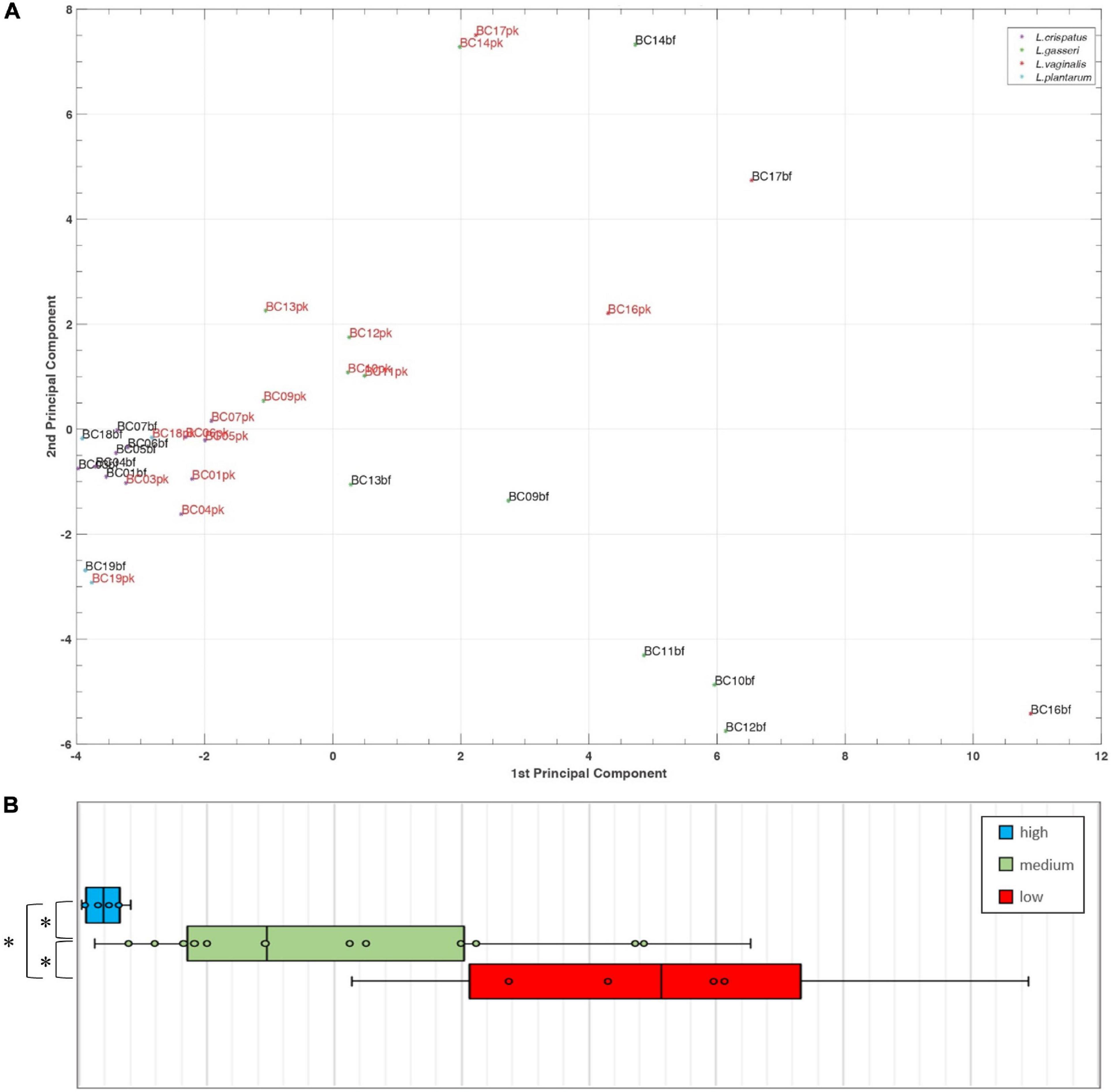
Figure 6. (A) Biplot of a PCA performed on Lactobacillus bf-CFS and pk-CFS metabolome and anti-Candida activity data. (B) Boxplot of CFS distribution on PC1 based on anti-Candida activity score. Lines within the boxes indicate the median values of the samples. Each box represents the interquartile range (25–75th percentile). The extremes of the bars indicate the 10th and 90th percentiles, respectively.
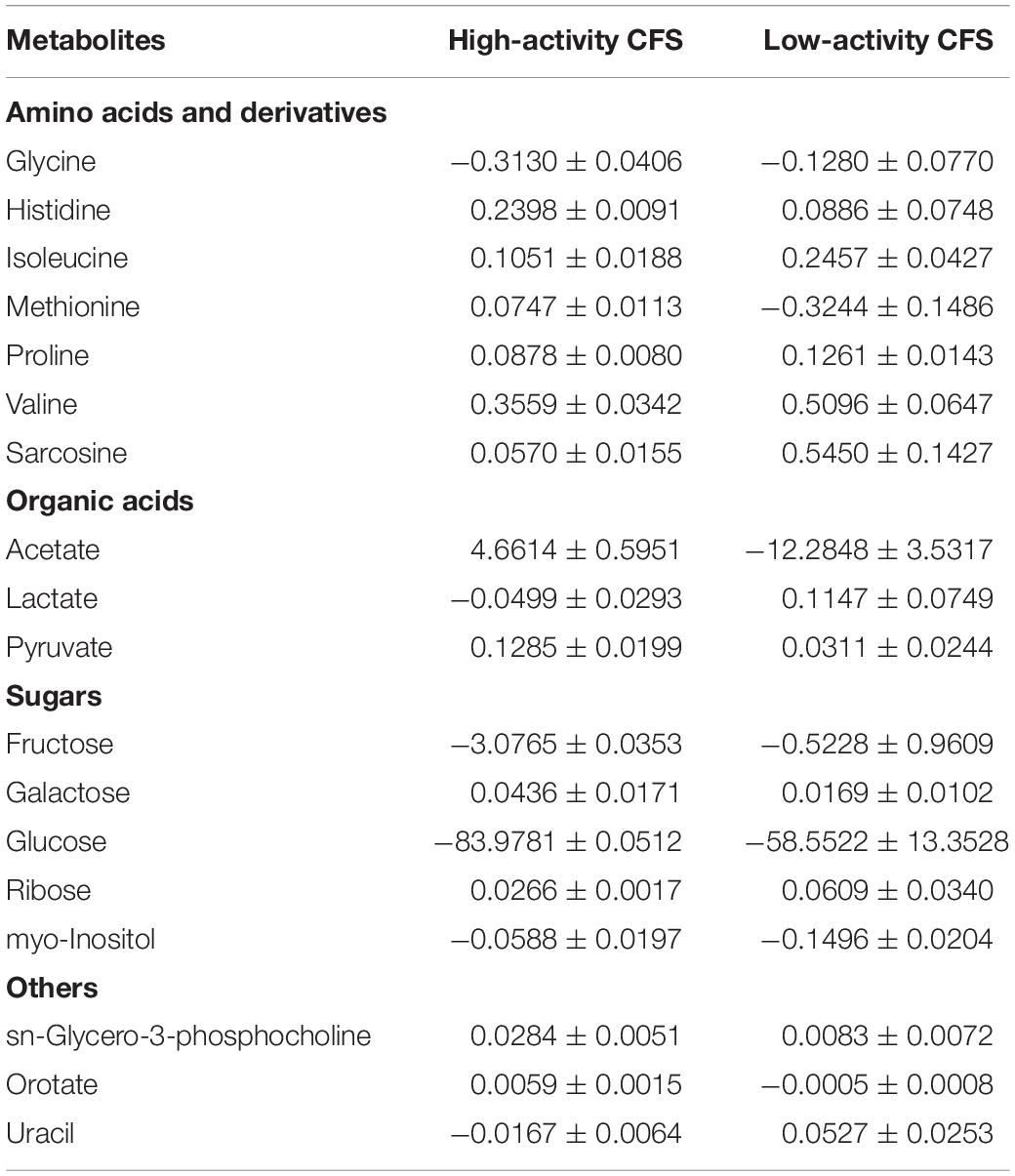
Table 3. List of metabolites significantly different between Lactobacillus CFS belonging to high anti-Candida activity group and low anti-Candida activity group (p < 0.05) and concentrations, calculated as differences with respect to MRS medium [median value ± MAD (mmol/L)].
Discussion
The two different forms of microbial growth, i.e., planktonic and biofilm, differ for physiological and metabolic features; indeed, the establishment of a sessile culture by a microbial strain requires the remodeling of several molecular pathways involved in adhesion, secretion, and other cellular functions. Such modifications of the global metabolism can, in turn, influence the functional properties of probiotics, beyond favoring the permanence of probiotics inside the human body (Donlan and Costerton, 2002; Ventolini, 2015).
In this context, we first assessed the ability of Lactobacillus strains of vaginal origin, belonging to L. crispatus, L. gasseri, L. vaginalis, and L. plantarum species, to form biofilm. We found out that L. plantarum species was the best biofilm producer, probably because of its adaptive features to niches (van den Nieuwboer et al., 2016). Indeed, L. plantarum is a versatile species able to adapt to different environmental niches and its genome, considered as one of the largest genome in lactic acid bacteria, encodes a huge repertoire of surface sugar, extracellular proteins, and regulatory peptide involved in the regulation of adherence (Kleerebezem et al., 2003; Sturme et al., 2005). Other authors previously reported that L. plantarum was able to produce a robust biofilm in vitro, resistant to environmental stresses (Kubota et al., 2008). Considering the most peculiar species of the vaginal microbiota, high variability was registered in the level of biofilm formation among L. crispatus and L. gasseri strains. Notably, L. crispatus BC3 biofilm was comparable to L. plantarum strains. Biofilm formation by L. crispatus and L. gasseri has been poorly investigated in the literature, and it has been reported that some L. crispatus strains of vaginal origin were not able to form biofilm and weakly autoaggregated (van der Veer et al., 2018), while Stivala et al. (2021) reported about vaginal L. crispatus and L. gasseri (and L. rhamnosus) strains as weak to moderate biofilm producers, with great variability.
From our results, the shift from a Lactobacillus planktonic culture to an adherent form implied major differences in the metabolism of nitrogen and amino acids, suggesting a key role of this molecule category in the formation of an adherent biomass, as already proposed by Liu et al. for Lactobacillus paraplantarum (Liu et al., 2021). In particular, alanine and lysine were produced/released at higher amounts by lactobacilli in the biofilm form compared to the planktonic one, whereas methionine was highly consumed by Lactobacillus in biofilm growth. These data support the finding that the methionine pathway is involved in the biofilm formation process, as proposed by Liu et al. (2018) in L. paraplantarum.
Although the anti-Candida activity of planktonic-derived Lactobacillus CFS has been reported and explored by several authors (Parolin et al., 2015; Wang et al., 2017; De Gregorio et al., 2019; Jang et al., 2019; Tortelli et al., 2020), no data are available on bf-CFS anti-Candida potential. In the present manuscript, both planktonic- and biofilm-derived CFS were tested against a broad spectrum of Candida clinical isolates, including C. albicans and C. non-albicans species. Overall, CFS did not exert fungistatic activity, with few exceptions. Fungistatic activity was tested in standard conditions following EUCAST protocol and validated in SVF. In accordance with results reported by Parolin et al. (2015), C. krusei resulted in being the most resistant isolate, although acidic conditions enhanced CFS fungistatic effect. C. glabrata isolates were susceptible to Lactobacillus CFS and notably their growth was almost abrogated by L. crispatus bf-CFS, although this species is often reported as resistant to antifungal substances (Rodrigues et al., 2014). Unlike previous results (Parolin et al., 2015) that were obtained with CFS from lactobacilli in exponential growth phase, Lactobacillus CFS from the stationary phase were active toward C. parapsilosis, pointing out the importance of growth phase and resulting metabolites on the anti-Candida activity. In addition, bf-CFS were more effective against C. parapsilosis than the respective pk-CFS.
Lactobacillus CFS that demonstrated high anti-Candida activity all derived from biofilm mode of growth and were produced by L. crispatus and L. plantarum strains; on the contrary, low-activity CFS were derived from L. gasseri (BC11–BC14) biofilm cultures and L. vaginalis BC16. This observation suggested that the anti-Candida activity of L. crispatus strains was enhanced when they were able to establish an adherent biofilm, while L. gasseri strains behaved in the opposite manner. This result, in turn, can offer new hints in the perspective of using L. crispatus or L. gasseri as probiotics to prevent vulvovaginal candidiasis or to restore the vaginal eubiosis status, also in the light of developing fourth-generation probiotics, based on biofilm-forming strains and encapsulation techniques (Salas-Jara et al., 2016). Peculiarly, L. vaginalis BC16, especially when grown in the biofilm mode, slightly stimulated Candida proliferation. This behavior correlated with L. vaginalis BC16 weak attitude to form an adherent biofilm, suggesting that its preferred mode of growth (i.e., planktonic) assured the best conditions to prevent from Candida infection, sustaining in turn the healthy status of the vaginal ecological niche.
In the present manuscript, we also sought for peculiarities in the metabolome of Lactobacillus CFS that demonstrated highly effective toward Candida clinical isolates. Highly active CFS differentiated from low active ones mostly for carbohydrates and amino acid quantification. Among carbohydrates, high anti-Candida activity was accompanied by increased consumption of glucose and fructose (corresponding to lower amounts), two well-known glycolytic fuel molecules. A marked consumption of glucose has already been correlated with Lactobacillus antimicrobial effect (Nardini et al., 2016), highlighting the mechanism of competition for nutrients between human beneficial microbes and pathogens as crucial. On the other hand, galactose concentration was higher in active CFS compared to non-active ones and lactose/galactose metabolism has been identified by overrepresentation analysis as a differential metabolic pathway between active and non-active CFS, denoting the preference of active Lactobacillus strains to utilize other carbon sources than galactose to sustain their growth.
Another recognized mechanism supporting the antimicrobial activity of lactobacilli is lactic acid production by fermentation and acidification (Boskey et al., 2001; O’Hanlon et al., 2013). In this regard, lower amount of lactate was quantified in active CFS compared to non-active ones, suggesting that the observed anti-Candida effect was not directly attributable to this organic acid. CFS endowed with high anti-Candida activity also showed higher amounts of pyruvate with respect to low-activity CFS, suggesting that the antifungal activity did not depend on a burst of the classical lactic fermentation pathway. In addition, active CFS contained high amounts of acetate, although L. crispatus, L. gasseri, and L. plantarum are recognized as homofermentative lactic acid bacteria. Lactobacillus CFS fungistatic activity was assessed in standard and acidic conditions, suggesting that the anti-Candida effect is not merely dependent on the acidic pH. Nevertheless, when CFS acted in an acidic environment, the anti-Candida activity was enhanced, pointing up the existence of a synergic activity among different components of the Lactobacillus supernatants and suggesting that the vaginal physiological environment could improve CFS anti-Candida potential.
To the best of our knowledge, the present study reports the anti-Candida activity of vaginal Lactobacillus grown in a sessile mode for the first time. Biofilm formation by lactobacilli represents a crucial aspect in the vaginal ecology, as this phenomenon can possibly influence endogenous microbiota persistence and functionality. Here, we demonstrated that Lactobacillus adherent growth strongly affects the anti-Candida potential and could in turn modulate Candida colonization of the vaginal niche. In particular, biofilm formation enhanced L. crispatus and L. plantarum fungistatic activity, while L. gasseri became more active when grown in a free form. Lactobacilli metabolism turned different between planktonic and biofilm forms, though the correlation between metabolism and functional properties demands more investigation. Further studies on lactobacilli biofilm formation in the vaginal niche will be approached to better understand its physiological role, also in the perspective to support the development of new antimycotic strategies based on probiotics grown in adherence.
Data Availability Statement
The original contributions presented in the study are included in the article/Supplementary Material, further inquiries can be directed to the corresponding author.
Author Contributions
CP and BV contributed to conception and design of the study. CP supervised the experiments. VC, LL, BG, and PD performed the experiments. CP, VC, LL, and MT analyzed the data. VC and MT produced the figures. CP and VC wrote the first draft of the manuscript. CF provided Candida strains. BV supervised the study. All authors contributed to manuscript revision, read, and approved the submitted version.
Funding
This research was funded by the Italian Ministry of University and Research (RFO free fund attributed to BV and CP).
Conflict of Interest
The authors declare that the research was conducted in the absence of any commercial or financial relationships that could be construed as a potential conflict of interest.
Publisher’s Note
All claims expressed in this article are solely those of the authors and do not necessarily represent those of their affiliated organizations, or those of the publisher, the editors and the reviewers. Any product that may be evaluated in this article, or claim that may be made by its manufacturer, is not guaranteed or endorsed by the publisher.
Supplementary Material
The Supplementary Material for this article can be found online at: https://www.frontiersin.org/articles/10.3389/fmicb.2021.750368/full#supplementary-material
Footnotes
References
Abruzzo, A., Giordani, B., Parolin, C., De Gregorio, P. R., Foschi, C., Cerchiara, T., et al. (2021). Lactobacillus crispatus BC1 biosurfactant delivered by hyalurosomes: an advanced strategy to counteract candida biofilm. Antibiotics 10:33. doi: 10.3390/antibiotics10010033
Abruzzo, A., Giordani, B., Parolin, C., Vitali, B., Protti, M., Mercolini, L., et al. (2018). Novel mixed vesicles containing lactobacilli biosurfactant for vaginal delivery of an anti- Candida agent. Eur. J. Pharm. Sci. 112, 95–101. doi: 10.1016/j.ejps.2017.11.012
Aoudia, N., Rieu, A., Briandet, R., Deschamps, J., Chluba, J., Jego, G., et al. (2016). Biofilms of Lactobacillus plantarum and Lactobacillus fermentum: effect on stress responses, antagonistic effects on pathogen growth and immunomodulatory properties. Food Microbiol. 53, 51–59. doi: 10.1016/j.fm.2015.04.009
Boskey, E. R., Cone, R. A., Whaley, K. J., and Moench, T. R. (2001). Origins of vaginal acidity: high D/L lactate ratio is consistent with bacteria being the primary source. Hum. Reprod. 16, 1809–1813. doi: 10.1093/humrep/16.9.1809
Calonghi, N., Parolin, C., Sartor, G., Verardi, L., Giordani, B., Frisco, G., et al. (2017). Interaction of vaginal Lactobacillus strains with HeLa cells plasma membrane. Benef. Microbes 8, 625–633. doi: 10.3920/BM2016.0212
Ceccarani, C., Foschi, C., Parolin, C., D’Antuono, A., Gaspari, V., Consolandi, C., et al. (2019). Diversity of vaginal microbiome and metabolome during genital infections. Sci. Rep. 9:14095. doi: 10.1038/s41598-019-50410-x
EUCAST Committee (2020). Method for the determination of broth dilution minimum inhibitory concentrations of antifungal agents for yeasts. E. Def. 7.3. 2, 1–21.
De Gregorio, P., Silva, J., Marchesi, A., and Nader-Macías, M. (2019). Anti-Candida activity of beneficial vaginal lactobacilli in in vitro assays and in a murine experimental model. FEMS Yeast Res. 19:foz008.
Donlan, R. M., and Costerton, J. W. (2002). Biofilms: survival mechanisms of clinically relevant microorganisms. Clin. Microbiol. Rev. 15, 167–193. doi: 10.1128/CMR.15.2.167-193.2002
Flemming, H. C., and Wingender, J. (2010). The biofilm matrix. Nat. Rev. Microbiol. 8, 623–633. doi: 10.1038/nrmicro2415
Jang, S. J., Lee, K., Kwon, B., You, H. J., and Ko, G. P. (2019). Vaginal lactobacilli inhibit growth and hyphae formation of Candida albicans. Sci. Rep. 9:8121. doi: 10.1038/s41598-019-44579-4
Kleerebezem, M., Boekhorst, J., Van Kranenburg, R., Molenaar, D., Kuipers, O. P., Leer, R., et al. (2003). Complete genome sequence of Lactobacillus plantarum WCFS1. Proc. Natl. Acad. Sci. U. S. A. 100, 1990–1995. doi: 10.1073/pnas.0337704100
Kubota, H., Senda, S., Nomura, N., Tokuda, H., and Uchiyama, H. (2008). Biofilm Formation by Lactic Acid Bacteria and Resistance to Environmental Stress. J. Biosci. Bioeng. 106, 381–386. doi: 10.1263/jbb.106.381
Laghi, L., Picone, G., Cruciani, F., Brigidi, P., Calanni, F., Donders, G., et al. (2014). Rifaximin modulates the vaginal microbiome and metabolome in women affected by bacterial vaginosis. Antimicrob. Agents Chemother. 58, 3411–3420. doi: 10.1128/AAC.02469-14
Leccese Terraf, M. C., Mendoza, L. M., Juárez Tomás, M. S., Silva, C., and Nader-Macías, M. E. F. (2014). Phenotypic surface properties (aggregation, adhesion and biofilm formation) and presence of related genes in beneficial vaginal lactobacilli. J. Appl. Microbiol. 117, 1761–1772. doi: 10.1111/jam.12642
Li, Z., Behrens, A. M., Ginat, N., Tzeng, S. Y., Lu, X., Sivan, S., et al. (2018). Biofilm-inspired encapsulation of probiotics for the treatment of complex infections. Adv. Mater. 30:e1803925. doi: 10.1002/adma.201803925
Liu, L., Guo, S., Chen, X., Yang, S., Deng, X., Tu, M., et al. (2021). Metabolic profiles of Lactobacillus paraplantarum in biofilm and planktonic states and investigation of its intestinal modulation and immunoregulation in dogs. Food Funct. 12, 5317–5332. doi: 10.1039/d1fo00905b
Liu, L., Wu, R., Zhang, J., and Li, P. (2018). Overexpression of luxSPromotes stress resistance and biofilm Formation of lactobacillus paraplantarumL-ZS9 by regulating the expression of multiple genes. Front. Microbiol. 9:2628. doi: 10.3389/fmicb.2018.02628
Namarta, K., Jatinder, S., and Manpreet, K. (2020). Microbiota in vaginal health and pathogenesis of recurrent vulvovaginal infections: a critical review. Ann. Clin. Microbiol. Antimicrob. 19:5. doi: 10.1186/s12941-020-0347-4
Nardini, P., Ñahui Palomino, R. A., Parolin, C., Laghi, L., Foschi, C., Cevenini, R., et al. (2016). Lactobacillus crispatus inhibits the infectivity of Chlamydia trachomatis elementary bodies, in vitro study. Sci. Rep. 6:29024. doi: 10.1038/srep29024
O’Hanlon, D. E., Moench, T. R., and Cone, R. A. (2013). Vaginal pH and microbicidal lactic acid when lactobacilli dominate the microbiota. PLoS One 8:e80074. doi: 10.1371/journal.pone.0080074
Oliver, J. C., Laghi, L., Parolin, C., Foschi, C., Marangoni, A., Liberatore, A., et al. (2020). Metabolic profiling of Candida clinical isolates of different species and infection sources. Sci. Rep. 10:16716. doi: 10.1038/s41598-020-73889-1
Parolin, C., Abruzzo, A., Giordani, B., Oliver, J. C., Marangoni, A., Luppi, B., et al. (2021). Anti-candida activity of hyaluronic acid combined with lactobacillus crispatus lyophilised supernatant: a new antifungal strategy. Antibiotics 10:628. doi: 10.3390/antibiotics10060628
Parolin, C., Marangoni, A., Laghi, L., Foschi, C., Palomino, R. A. Ñ, Calonghi, N., et al. (2015). Isolation of vaginal lactobacilli and characterization of anti-candida activity. PLoS One 10:e0131220. doi: 10.1371/journal.pone.0131220
Ravel, J., Gajer, P., Abdo, Z., Schneider, G. M., Koenig, S. S. K., McCulle, S. L., et al. (2011). Vaginal microbiome of reproductive-age women. Proc. Natl. Acad. Sci. U. S. A. 108, 4680–4687. doi: 10.1073/pnas.1002611107
Rieu, A., Aoudia, N., Jego, G., Chluba, J., Yousfi, N., Briandet, R., et al. (2014). The biofilm mode of life boosts the anti-inflammatory properties of Lactobacillus. Cell. Microbiol. 16, 1836–1853. doi: 10.1111/cmi.12331
Rodrigues, C. F., Silva, S., and Henriques, M. (2014). Candida glabrata: a review of its features and resistance. Eur. J. Clin. Microbiol. Infect. Dis. 33, 673–688. doi: 10.1007/s10096-013-2009-3
Rönnqvist, D., Forsgren-Brusk, B., and EGrahn-Håkansson, E. (2006). Lactobacilli in the female genital tract in relation to other genital microbes and vaginal pH. Acta Obstet. Gynecol. Scand. 85, 726–735. doi: 10.1080/00016340600578357
Salas-Jara, M., Ilabaca, A., Vega, M., and García, A. (2016). Biofilm Forming Lactobacillus: new Challenges for the Development of Probiotics. Microorganisms 4:35. doi: 10.3390/microorganisms4030035
Stivala, A., Carota, G., Fuochi, V., and Furneri, P. M. (2021). Lactobacillus rhamnosus AD3 as a promising alternative for probiotic products. Biomolecules 11:94. doi: 10.3390/biom11010094
Sturme, M. H. J., Nakayama, J., Molenaar, D., Murakami, Y., Kunugi, R., Fujii, T., et al. (2005). An agr-like two-component regulatory system in Lactobacillus plantarum is involved in production of a novel cyclic peptide and regulation of adherence. J. Bacteriol. 187, 5224–5235. doi: 10.1128/JB.187.15.5224-5235.2005
Tortelli, B., Lewis, W., Allsworth, J., Member-Meneh, N., Foster, L., Reno, H., et al. (2020). Associations between the vaginal microbiome and Candida colonization in women of reproductive age. Physiol. Behav. 176, 139–148. doi: 10.1016/j.ajog.2019.10.008.Associations
van den Nieuwboer, M., van Hemert, S., Claassen, E., and de Vos, W. M. (2016). Lactobacillus plantarum WCFS1 and its host interaction: a dozen years after the genome. Microb. Biotechnol. 9, 452–465. doi: 10.1111/1751-7915.12368
Van der Maaten, L., and Hinton, G. (2008). Multiobjective evolutionary algorithms to identify highly autocorrelated areas: the case of spatial distribution in financially compromised farms. Ann. Oper. Res. 219, 187–202. doi: 10.1007/s10479-011-0841-3
van der Veer, C., Hertzberger, R. Y., Bruisten, S. M., Tytgat, H. L. P., Swanenburg, J., Angelino-Bart, A., et al. (2018). Comparative genomics of human Lactobacillus crispatus isolates reveals genes for glycosylation and glycogen degradation: implications for in vivo dominance of the vaginal microbiota. bioRxiv [Preprint]. doi: 10.1101/441972
Ventolini, G. (2015). Vaginal lactobacillus: biofilm formation in vivo – clinical implications. Int. J. Womens. Health 7, 243–247. doi: 10.2147/IJWH.S77956
Wang, S., Wang, Q., Yang, E., Yan, L., Li, T., and Zhuang, H. (2017). Antimicrobial compounds produced by vaginal Lactobacillus crispatus are able to strongly inhibit Candida albicans growth, hyphal formation and regulate virulence-related gene expressions. Front. Microbiol. 8:564. doi: 10.3389/fmicb.2017.00564
Keywords: Lactobacillus, Candida, biofilm, metabolome, vaginal microbiota, health benefits
Citation: Parolin C, Croatti V, Laghi L, Giordani B, Tondi MR, De Gregorio PR, Foschi C and Vitali B (2021) Lactobacillus Biofilms Influence Anti-Candida Activity. Front. Microbiol. 12:750368. doi: 10.3389/fmicb.2021.750368
Received: 30 July 2021; Accepted: 04 October 2021;
Published: 29 October 2021.
Edited by:
M. Pilar Francino, Fundación para el Fomento de la Investigación Sanitaria y Biomédica de la Comunitat Valenciana (FISABIO), SpainReviewed by:
Patrick Van Dijck, VIB-KU Leuven Center for Microbiology, Flanders Institute for Biotechnology, BelgiumSónia Silva, University of Minho, Portugal
Copyright © 2021 Parolin, Croatti, Laghi, Giordani, Tondi, De Gregorio, Foschi and Vitali. This is an open-access article distributed under the terms of the Creative Commons Attribution License (CC BY). The use, distribution or reproduction in other forums is permitted, provided the original author(s) and the copyright owner(s) are credited and that the original publication in this journal is cited, in accordance with accepted academic practice. No use, distribution or reproduction is permitted which does not comply with these terms.
*Correspondence: Carola Parolin, carola.parolin@unibo.it