- 1Institute of Biotechnology and Chemical Engineering, I-Shou University, Kaohsiung, Taiwan
- 2Department of Physical Examination Center, Kaohsiung Veterans General Hospital, Kaohsiung, Taiwan
- 3Department of Nursing, Meiho University, Pingtung, Taiwan
- 4Department of Pediatrics, Kaohsiung Veterans General Hospital, Kaohsiung, Taiwan
- 5Department of Medicine, National Yang-Ming University, Taipei, Taiwan
- 6Department of Nursing, Fooyin University, Kaohsiung, Taiwan
- 7Department of Internal Medicine, National Cheng Kung University Hospital, Tainan, Taiwan
- 8Department of Medicine, College of Medicine, National Cheng Kung University, Tainan, Taiwan
Colistin is the last resort antimicrobial for treating multidrug-resistant gram-negative bacterial infections. The plasmid-mediated colistin resistance gene, mcr-1, crucially influences colistin’s resistance transmission. Human fecal carriages of mcr-1-positive Escherichia coli (E. coli) were detected in many regions worldwide; however, only a few studies have focused on children. Therefore, we identified the prevalence and risk factors of mcr-1-positive E. coli in fecal carriages among community children in Southern Taiwan. In this study, 510 stool samples were collected from April 2016 to August 2019 from the pediatric department at a medical center in Southern Taiwan. These samples were collected within 3 days after admission and were all screened for the presence of the mcr-1 gene. Diet habits, travel history, pet contact, and medical history were also obtained from participants to analyze the risk factors of their fecal carriages to mcr-1-positive E. coli. Antimicrobial susceptibility testing was determined using the VITEK 2 system and the broth microdilution test. Twelve mcr-1-positive E. coli. were isolated from 2.4% of the fecal samples. Through multivariate analysis, frequent chicken consumption (at least 3 times per week) had a significantly positive association with the presence of mcr-1-positive E. coli in fecal carriages (adjust odds ratio 6.60, 95% confidence interval1.58– 27.62, p = 0.033). Additionally, multidrug resistance was more common in mcr-1-positive E. coli. (75.0% vs. 39.5%, p = 0.031) than in non-mcr-1-positive Escherichia coli. Furthermore, the percentage of extraintestinal pathogenic E. coli in mcr-1-positive isolates was 83.3%. Some multi-locus sequence types in our mcr-1-positive E. coli were also similar to those isolated from food animals in the literature. The prevalence of fecal carriages of mcr-1-positive E. coli was low among community children in Southern Taiwan. Our data shows that chicken consumption with a higher frequency increases the risk of mcr-1-positive E. coli. in fecal carriages.
Introduction
Antibiotic resistance is a global public health challenge of our time. Colistin (polymyxin E), first discovered in 1947, is a polycationic peptide antibiotic (Storm et al., 1977). It mainly targets the bacterial cell membrane and increases its permeability, which results in the leakage of cell contents, thereby ultimately causing cell death (Luo et al., 2020). Furthermore, it has broad-spectrum antimicrobial activities, and it is used to treat multidrug-resistant gram-negative infections (Poirel et al., 2017). Before 2015, studies showed that colistin resistance was regulated by mutation in chromosomal genes (Olaitan et al., 2014). However, in 2016, Liu et al. (2016) discovered the plasmid-mediated colistin resistance gene—mcr-1. Since then, the mcr-1 gene has been identified as part of several bacterial species in humans, animals, and the environment, thus, posing a great threat to treatment (Elbediwi et al., 2019).
The human gut is a reservoir of antimicrobial resistance genes. In the gut, antimicrobial resistance genes can spread through horizontal gene transfer (Rolain, 2013; McInnes et al., 2020), which plays an important role in transmitting drug-resistant bacteria. Previous studies have also reported that the prevalence of mcr-1-positive Enterobacteriaceae in fecal carriages was 0.4–15.0% in Asia (Chan et al., 2018; Shen et al., 2018; La et al., 2019; Wu et al., 2019), 0–0.35% in Europe (Terveer et al., 2017; Zurfluh et al., 2017b), and 38.3% in South America (Giani et al., 2018), showing great variability in different geographic areas and populations. In the aspects of risk factors, several literatures from China reported antibiotic use before admission, the consumption of meat and aquaculture products were linked to mcr-1-positive Enterobacteriaceae in fecal carriages (Wang et al., 2017b; Shen et al., 2018). Furthermore, traveling to Southeast Asia has also been associated with mcr-1-positive Escherichia coli (E. coli) in fecal carriages (Nakayama et al., 2018). Despite these results, most studies on the risk factors for mcr-1 Enterobacteriaceae in fecal carriages were from the adult population, and studies among children were scarce. Furthermore, E. coli is a common inhabitant of the human intestinal tract and has the highest mcr prevalence among all bacterial species (Elbediwi et al., 2019). Therefore, we conducted a prospective study to identify the prevalence and risk factors of mcr-1-positive E. coli in fecal carriages of community children in Southern Taiwan.
Materials and Methods
Study Population and Data Collection
This prospective study was conducted from April 2016 to August 2019 at the pediatric department of Kaohsiung Veterans General Hospital in Southern Taiwan. Children aged below 18 years who were admitted at the pediatric department were enrolled. We contacted them as soon as they were available after admission. If they agreed to participate in the study after an interview, we described how their stool samples would be collected, after which they were given a standardized questionnaire that included demographic data, hospitalization in the last 3 months, antibiotic use in the last 3 months, history of traveling abroad, dietary habits, intake of unboiled drinking water, and history of pet contact. All participants and their legal guardians were then provided with an informed written consent. Exclusion criteria were non-willing participants, those unwilling to complete the informed written consent, those without fecal samples within 3 days after admission, and those with incomplete data in their questionnaire. The Ethics Committee of the Kaohsiung Veterans General Hospital (VGHKS 16-CT2–04, VGHKS 18-CT3–11) approved this study.
Isolation of E. coli and Detection of the mcr-1 Gene
Fecal samples were collected using cotton swabs, and then the swabs were transported in a COPAN Transystem® (Copan Diagnostics, Inc., Brescia, Italy). Subsequently, the swabs were plated on a CHROMagar™ ECC plate (CHROMagar, Paris, France) and incubated without CO2 at 35–37°C for 24 h. E. coli colonies presented blue coloration, and up to two E. coli colonies were randomly selected for further analysis. Furthermore, all selected E. coli strains were screened for mcr-1 genes. We used polymerase chain reaction with the primers; CLR5-F (5′-CGGTCAGTCCGTTTGTTC-3′) and CLR5-R (5′-CTTGGTCGGTCTGTA GGG-3′) for mcr-1 gene screening (Liu et al., 2016). Additionally, all selected E. coli strains were spread on a CHROMagar™ ESBL plate (CHROMagar, Paris, France) to recognize the extended-spectrum β-lactamase (ESBL) E. coli isolates. We also checked for ESBL genes and carbapenemase genes, including; blaCTX–M, blaSHV, blaTEM, blaOXA–1, blaIMP, and blaVIM using polymerase chain reaction (PCR) with previously described primers and methods (Chia et al., 2005; Sidjabat et al., 2009; Wu et al., 2019). Moreover, we used polymerase chain reaction to identify whether mcr-1-positive E. coli isolates belonged to extraintestinal pathogenic E. coli (ExPEC). Therefore, ExPEC was defined as E. coli isolates harboring at least two of the following five genes: S and F1C fimbriae (focG + sfaS), kpsM II, papA, afa, and iutA (Johnson et al., 2003). The E. coli multilocus sequence typing (MLST) scheme was also determined among mcr-1-positive E. coli isolates using seven housekeeping gene sequences (adk, fumC, gyrB, icd, mdh, purA, and recA) (Chia et al., 2005). Then, we used the goeBURST algorithm1 to evaluate the genetic relatedness of mcr-1-positive E. coli (Francisco et al., 2009).
Antimicrobial Susceptibility Testing
The VITEK 2 system was used for the antimicrobial susceptibility testing of all selected E. coli strains. The following 17 antibiotics were included: ampicillin-sulbactam, piperacillin-tazobactam, piperacillin, cefazolin, cefoxitin, cefixime, ceftriaxone, ceftazidime, cefepime, ertapenem, imipenem, amikacin, gentamycin, ciprofloxacin, minocycline, tigecycline, and sulfamethoxazole-trimethoprim. Additionally, we used the broth dilution method to determine the antimicrobial susceptibility of colistin, sulfamethoxazole, trimethoprim, ciprofloxacin, tetracycline, meropenem, azithromycin, nalidixic acid, cefotaxime, chloramphenicol, tigecycline, ceftazidime, ampicillin, and gentamicin among all mcr-1-positive E. coli isolates. Minimum inhibitory concentration (MIC) was interpreted based on the 2020 CLSI guidelines (Clinical and Laboratory Standards Institute, 2020).
Statistical Analysis
We used SPSS version 20.0 for Windows (SPSS Inc., Armonk, NY, United States) to perform our statistical analyses. For univariate analysis, Chi-square or Fisher’s exact tests were used to analyze categorical variables. An independent t-test was also used to analyze continuous variables. Furthermore, variables with a p-value of <0.1 in the univariate analysis were assessed using binary logistic regression analysis in the multivariate analysis. A p-value of<0.05 was considered statistically significant.
Results
We collected 510 non-duplicated fecal specimens from patients in our pediatric ward. Among these specimens, 268 yielded 514 E. coli isolates. The characteristics of these 510 participants are demonstrated in Table 1. The participants’ ages ranged from 1 day to 17 years (mean age was 1.5 years), and males accounted for 58.6% (299/510). Hospitalization and antibiotic therapy in the last 3 months were recorded in 9.6% (49/510) and 7.8% (40/510) of participants, respectively. In addition, 7.5% (38/510) of the participants had traveled abroad in the last 12 months. Among them, 10 had been to China, 23 to Japan, 2 to Korea, 5 to Southeast Asian nations, and one to the United States. The prevalence of fecal carriages of mcr-1-positive E. coli was 2.4% (12/510). Looking further, we divided the study period into three segments. The prevalence was 1.6% (2/124) from April 2016 to March 2017, 2.5% (8/324) from April 2017 to March 2018, and 3.2% (2/62) from April 2018 to August 2019.
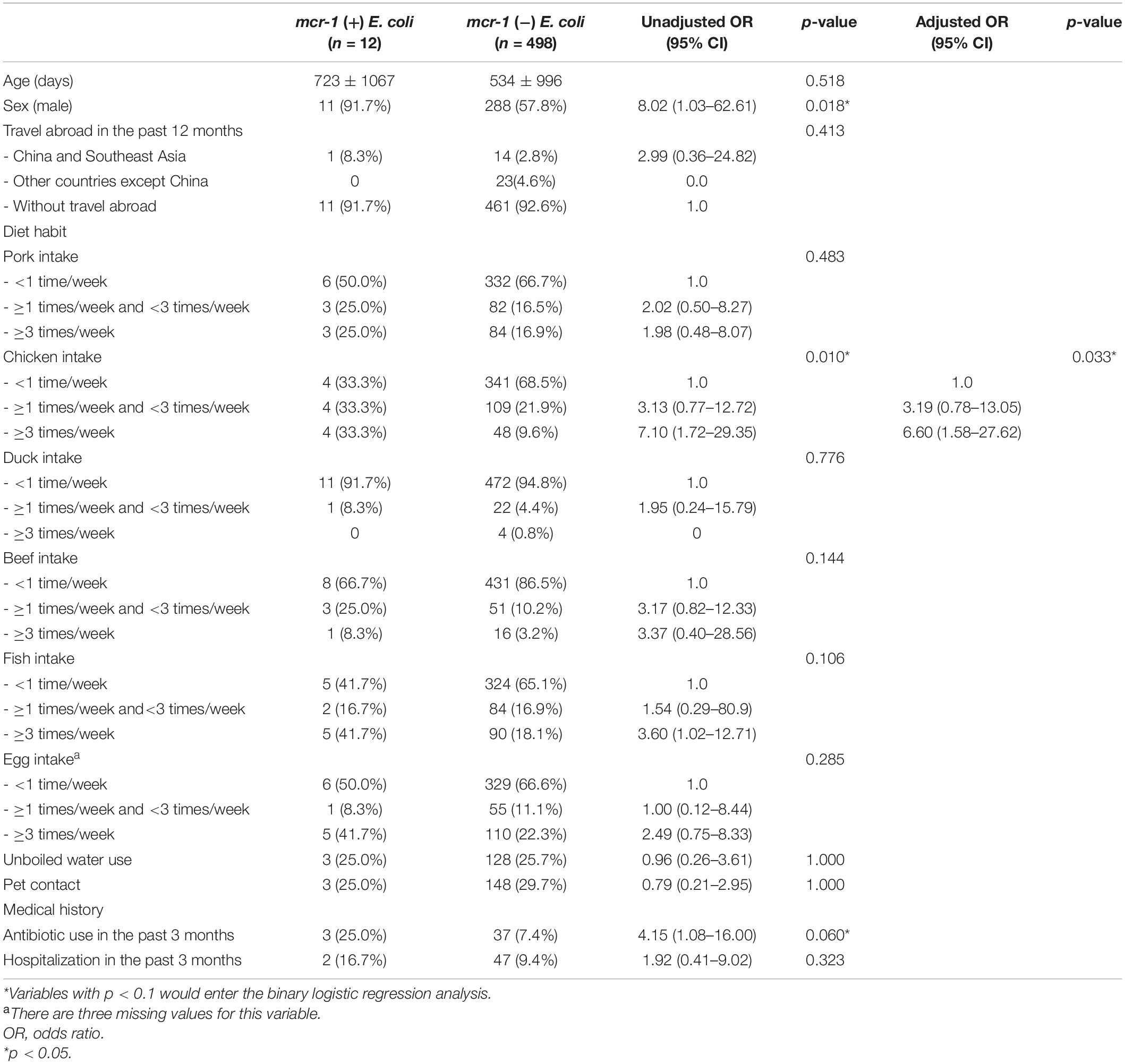
Table 1. Characteristics of 510 participants with and without mcr-1 Escherichia coli in their fecal carriages.
Subsequently, we compared the variables between individuals with and without fecal carriages of mcr-1-positive E. coli through univariate analysis. Individuals with fecal carriages of mcr-1-positive E. coli were predominantly male [Odds Ratio (OR) 8.02, 95% confidence interval (CI) 1.03–62.61] and people frequently ate chicken (OR 3.13, 95% CI 0.77–12.72), with a consumption of between 1 and 3 times per week. Another set of included individuals (OR 7.10, 95% CI 1.72–29.35) had a chicken consumption frequency of at least 3 times per week. Furthermore, antibiotic use in the past 3 months was borderline associated with mcr-1-positive E. coli carriage (OR 4.15, 95% CI 1.08–16.00) (Table 1). Through binary logistic regression analysis, chicken consumption with a higher frequency (least 3 times per week) was the only factor that had a significant association with fecal carriages of mcr-1-positive E. coli (adjust OR 6.60, 95% CI 1.58–27.62, p = 0.033) (Table 1).
The distribution of reasons for admission between participants with and without mcr-1-positive E. coli in fecal carriages are presented in Table 2, showing that individuals that were hospitalized due to infectious diseases had a higher proportion of mcr-1-positive E. coli in their fecal carriages than those hospitalized due to non-infectious diseases (3.6 and 0.9%, respectively, p = 0.041), and individuals hospitalized due to urinary tract infection (UTI) had the highest proportion (7.4%) of mcr-1-positive E. coli in their fecal carriages.
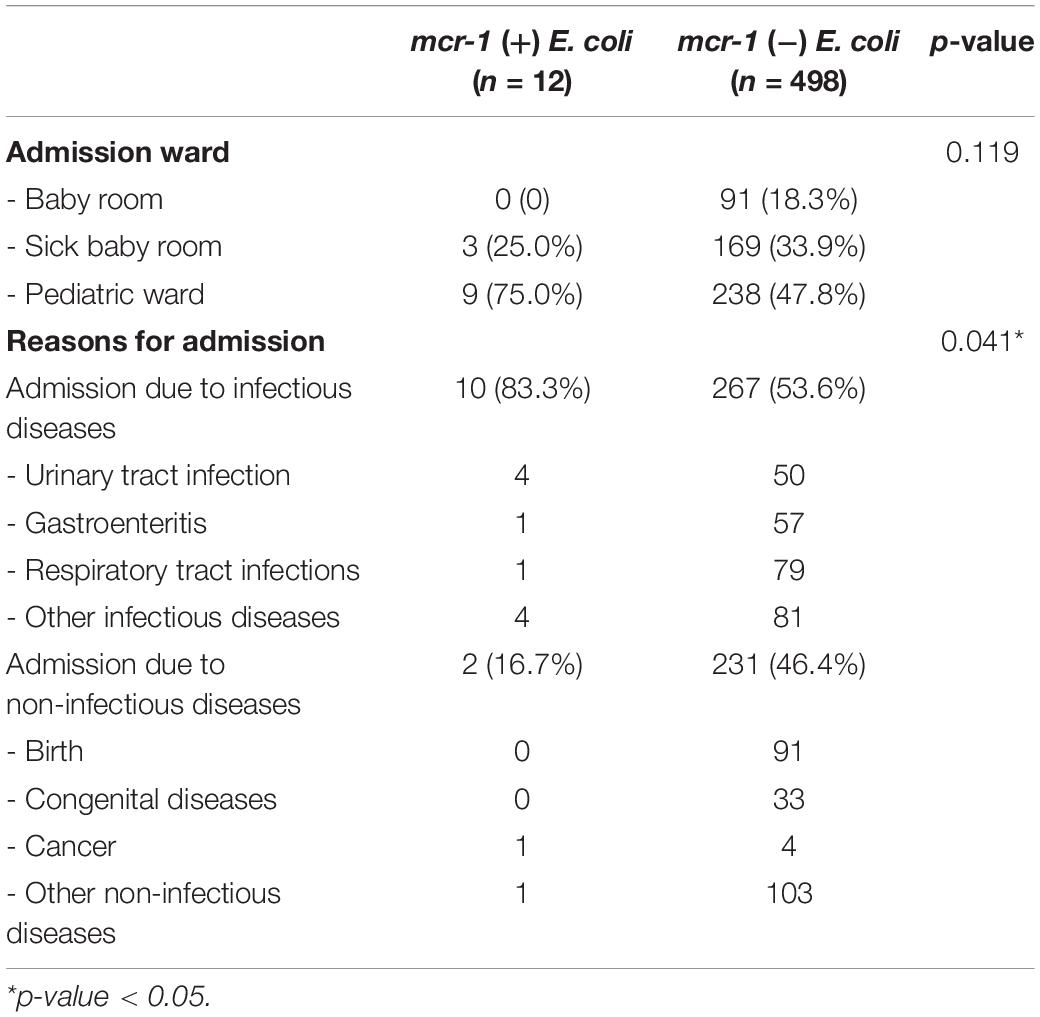
Table 2. The distribution of reasons for admitting participants with and without mcr-1-positive E. coli in their fecal carriages.
In Table 3, we compare the antibiotic susceptibility results between mcr-1-positive and non-mcr-1-positive E. coli isolates determined using Vitek. Results showed that mcr-1-positive E. coli isolates were more prone to be resistant to cefoxitin (50.0% vs. 9.0%, p = 0.001), minocycline (41.7% vs. 15.2%, p = 0.031), and sulfamethoxazole-trimethoprim (75.0% vs. 40.5%, p = 0.018) than non-mcr-1-positive E. coli isolates. Multidrug resistance was defined as the non-susceptibility to at least 1 antibiotic in 3 or more antimicrobial categories (Magiorakos et al., 2012). Multidrug resistance (75.0% vs. 39.5%, p = 0.031) was also more common in mcr-1-positive E. coli isolates. Non-mcr-1 -positive E. coli isolates were all susceptible to colistin. All E. coli isolates were susceptible to ertapenem, imipenem, amikacin, and tigecycline. Moreover, the MIC and molecular characteristics of mcr-1-positve E. coli isolates are shown in Table 4. Most mcr-1-positive E. coli isolates exhibited colistin MICs of 4–8 μg/mL. Two isolates possessed the ESBL-producing phenotype. One had blaCTX–M and blaTEM, and the other had blaTEM. Among mcr-1-positive E. coli isolates, 10 (83.3%) belonged to ExPEC.
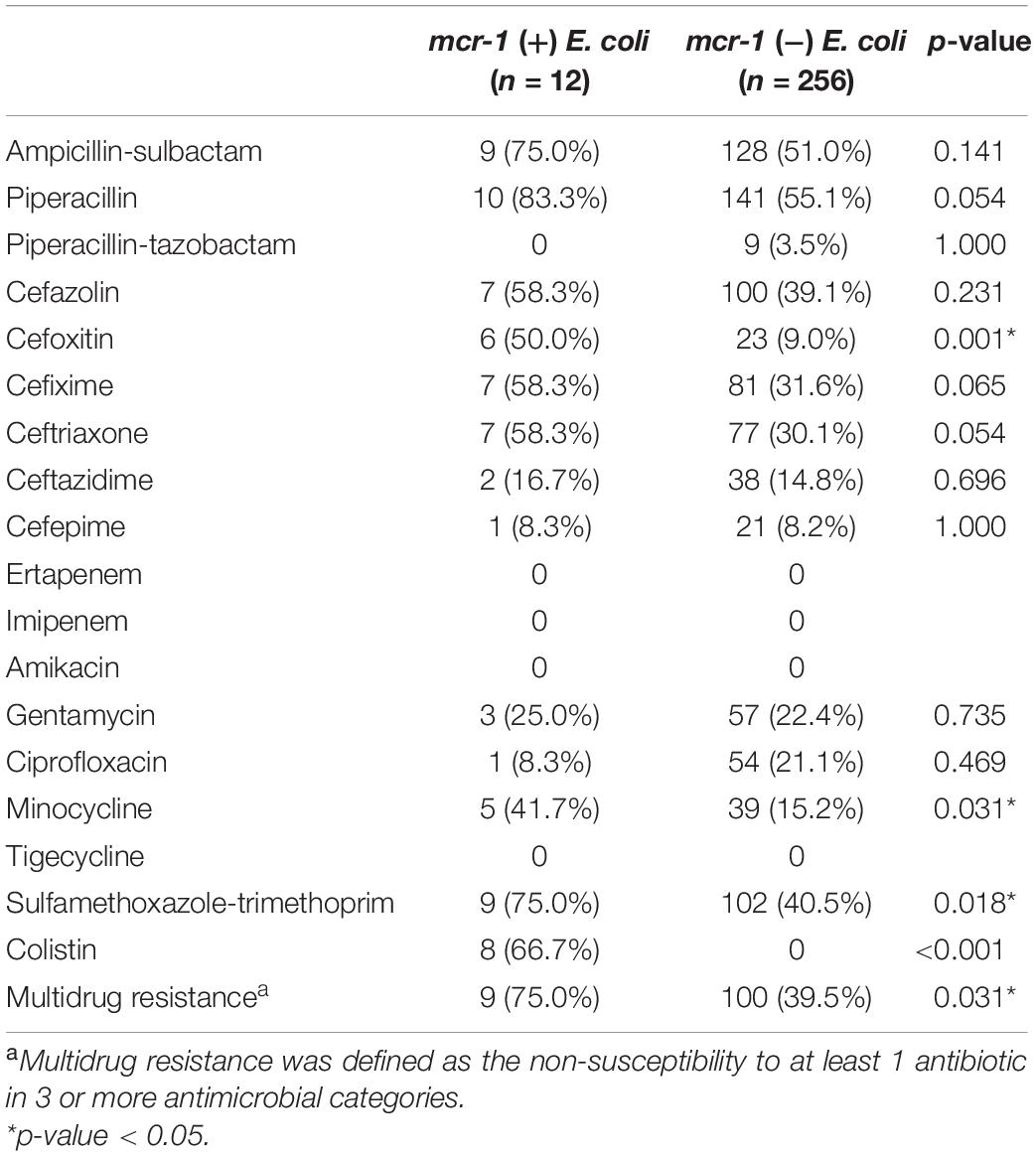
Table 3. Comparing antibiotic resistance profiles between mcr-1-positve E. coli and non-mcr-1-positve E. coli. using Vitek.
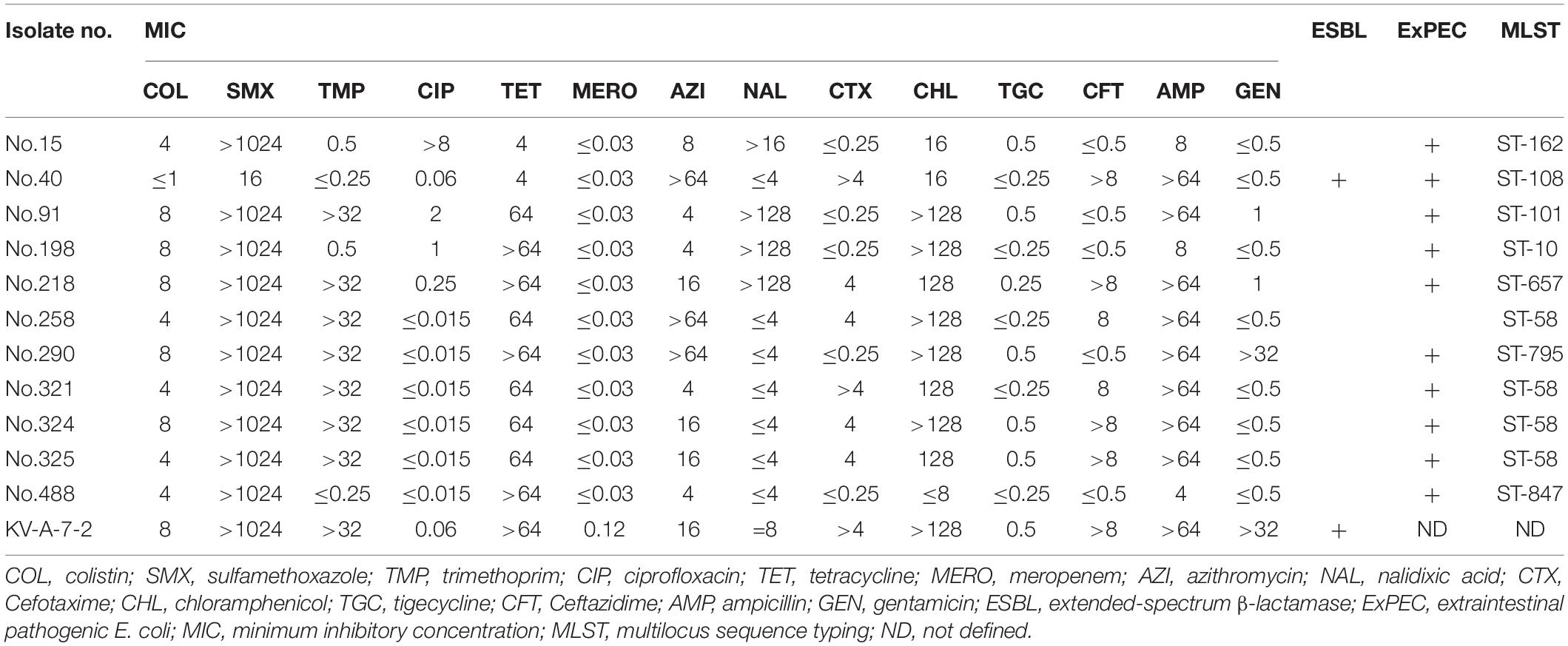
Table 4. Antibiotic susceptibility based on minimum inhibitory concentration and the molecular characteristics of 12 mcr-1-positive E. coli isolates.
Sequence type (ST) 58 accounted for the highest proportion (n = 4, 33.3%). The genetic relatedness of mcr-1-positive E. coli in the study and those isolated from food animals reported in the literatures of Taiwan was evaluated using goeBURST’s algorithm (Figure 1; based on data from the MLST database)2 (Kuo et al., 2016; Liu J. Y. et al., 2020). Results showed that ST10, ST101, and ST162 were detected in both our participants’ fecal samples and food animals, including chicken, pork, and beef, reported in the literatures of Taiwan (Kuo et al., 2016; Liu J. Y. et al., 2020).
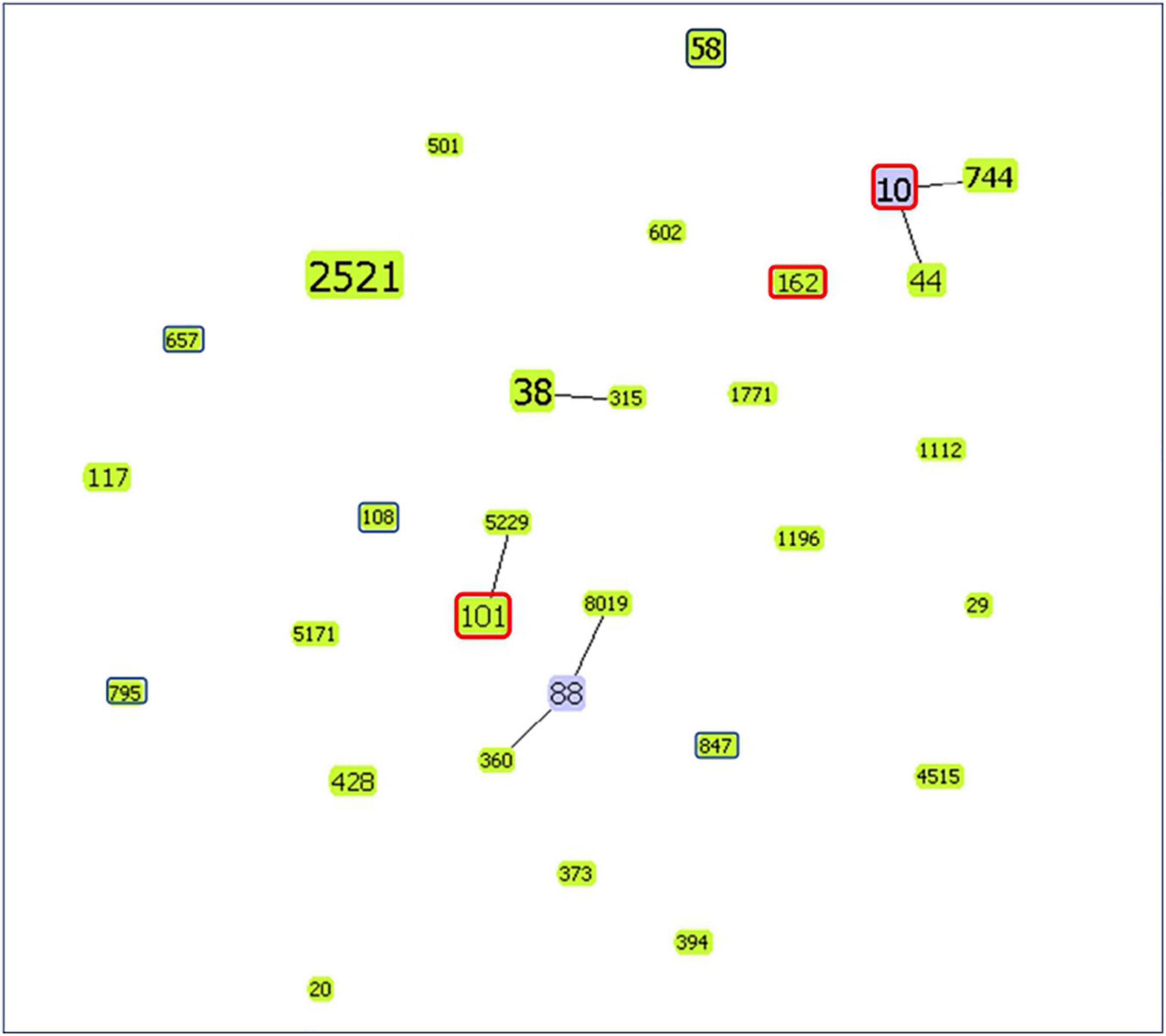
Figure 1. goeBURST diagram showing the genetic relatedness of mcr-1-positive E. coli isolates from the study and food animals reported in the literatures of Taiwan (Kuo et al., 2016; Liu J. Y. et al., 2020). The number within the node is the ST. Node size is based on the number of isolates with specific STs. ST of isolates in the study (from fecal samples of children) is marked with a dark blue frame. However, isolates with the same ST from the study and food animals of Taiwan reported in the literatures are marked with a red frame. Others without frame markings are ST of isolates from food animals in the literature of Taiwan. (Node color: light green: group founder; light blue: common node).
Discussion
The prevalence of mcr-1-positive E. coli in fecal carriages of patients included in the study was 2.4% (12/510), which was lower than what had been reported in children (4–38.3%) from either community or hospital settings in other countries, such as China, Bolivia and India (Bi et al., 2017; Hu et al., 2017; Giani et al., 2018; Purohit et al., 2019). However, the results were similar to other recent studies in Taiwan, including data from clinical isolates and commensal E. coli (Lee et al., 2019; Wu et al., 2019). These findings demonstrated that the prevalence of the mcr-1 gene was still low in Taiwan. However, an upward trend of the prevalence over time was found. Furthermore, in this study at the individual level, a significant predictor of fecal carriages in mcr-1-positive E. coli was chicken consumption. A study from China of approximately 90% adults also demonstrated that meat consumption, especially pork and mutton, was associated with mcr-1 prevalence in a provincial region’s level (Shen et al., 2018). Another epidemiological study from southern China using vegetarian and non-vegetarian classifications did not find diet as a risk factor for mcr-1 in fecal carriages (Wang et al., 2017b). Additionally, previous studies revealed a correlation of drug-resistance bacteria between contaminated food and the human gut. Sørensen et al. (2001) observed the same resistant strains in stool after ingestion of chickens containing those resistant bacteria. Donabedian et al. (2003) also observed related pulsed-field gel electrophoresis patterns of gentamicin-resistant isolates from human stools and meat. A recent review article indicated that foodborne transmission was a pathway of mcr-1-positive E. coli transmission (Elbediwi et al., 2019). Literature also revealed that chicken had a higher percentage of the mcr-1 gene than other meats (Kuo et al., 2016). Therefore, chicken consumption is a possible pathway to transmit mcr-1-positive E. coli to humans. Nevertheless, more studies should investigate the detailed mechanisms.
Traveling abroad was proposed as a risk factor for drug-resistant gene transmission (Hu et al., 2020). Limited studies on the relation between traveling abroad and fecal carriages of mcr-1-positive E. coli have been reported. von Wintersdorff et al. (2016) and Nakayama et al. (2018) demonstrated that traveling to Southeast Asia or southern Africa increases the risk to mcr-1-positive E. coli in fecal carriages. However, traveling abroad was not associated with mcr-1-positive E. coli in fecal carriages in this study. The period between the time of travel and fecal collection may affect the results. In those two studies, fecal samples were collected within no more than 3 weeks after travel events. In the study presented here, travel history was traced back to 12 months before the fecal sample collection. Previous studies revealed that the proportion of drug-resistant bacterial colonization declined as time after international travels increased. For example, only about 10% of fecal colonization of ESBL E. coli cases remained positive after 12 months follow-up (von Wintersdorff et al., 2016; Arcilla et al., 2017; OstholmBalkhed et al., 2018). Additionally, people in Taiwan have more opportunities of contacting people from China and Southeast Asia due to their geographic location, which may weaken the influence of traveling to these countries. Therefore, more studies should investigate the relationship between international travels and mcr-1-positive E. coli in fecal carriages.
A higher proportion of participants who were hospitalized due to UTI were positive for mcr-1-positive E. coli in the study. The association between UTI pathogens and the gut microbiome had been reported before (Moreno et al., 2008; Paalanne et al., 2018). Paalanne et al. (2018) revealed that the gut microbiome was associated with the risk of febrile UTI in children. However, we did not collect urine data from the participants in the study. The relevance of UTI pathogens and fecal carriages of mcr-1-positive E. coli thus needs further study. The four participants with UTI with mcr-1-positive E. coli isolates were under 5 years. Furthermore, two of them had a history of UTI and antibiotic exposure in the last 12 months. Except for the undefined one, the other three isolates belonged to ExPEC. Interestingly, the positive rate of mcr-1-positive E. coli presence in the fecal carriage of participants with acute gastroenteritis was low (1.7%, 1/58).
Additionally, variations were observed in genotypes among the 12 mcr-1-positive E. coli cases. ST58 was the most common type in the study. E. coli ST58 has been isolated from different samples, including humans, the environment, food animals, and wildlife (Fuentes-Castillo et al., 2021). Furthermore, mcr-1-positive E. coli ST58 was found in chicken, turkey, cattle, and the environment (Brennan et al., 2016; Donà et al., 2017; Zurfluh et al., 2017a; Sacramento et al., 2018; Zajac et al., 2019). Similarly, mcr-1-positive E. coli ST162 was also isolated from ducks, chickens, turkeys, and dogs (Lim et al., 2016; Gelbicova et al., 2019; Zhang et al., 2019; Liu Y. Y. et al., 2020). E. coli ST101 and ST10 were the widespread clones that harbored mcr-1 genes (Elbediwi et al., 2019). mcr-1-positive E. coli ST162, ST101, and ST10 have been reported in food animals and meats in Taiwan as well (Kuo et al., 2016; Liu J. Y. et al., 2020). Our preliminary data also showed that plasmid analysis from mcr-1-positive E. coli in children was similar to the plasmid sequence reported in the animals (unpublished data). Most E. coli ST108 have been isolated from chicken (Yang et al., 2014; Agabou et al., 2016; Pietsch et al., 2018). E. coli ST657 has been reported from rectal swabs of humans (Yang et al., 2014; Wang et al., 2017a). E. coli ST795 was found in retail vegetables and pigs (Hammerum et al., 2012; Luo et al., 2017; Xu et al., 2017). Additionally, E. coli ST-847 has been isolated from retail vegetables, pigs, cattle, and birds (Guenther et al., 2012; Leimbach et al., 2015; Freitag et al., 2018; Wang et al., 2020). These genotypes isolates, including ST-108, ST-657, ST-795, and ST-847, have been reported to carry different drug-resistant genes. This study is the first to reveal that these STs of E. coli carried the mcr-1 gene.
Some limitations exist in this study. First, the diet information relied on self-reports provided by the participants’ parents or caregivers. Recall bias is inevitable. Second, the number of participants with mcr-1-positive E. coli in fecal carriages was relatively small, which would not detect certain risk factors. Third, mcr-1 gene is detected mainly from Enterobacteriaceae. Although E. coli has the highest mcr prevalence among all bacterial species (Elbediwi et al., 2019), there are still some common bacterial species with mcr gene, such as Klebsiella pneumoniae, that have not been analyzed in the study. Furthermore, the study was limited to mcr-1 gene, which is the most widely disseminated mcr gene worldwide (Ling et al., 2020). However, other mcr variants may exist. Last, the diversity of STs of mcr-1-positive E. coli not only means clonal expansion but also the possibility of certain plasmid transmission in the community. In our preliminary result, some of the plasmid in the mcr-1 positive E. coli had the same sequence as the plasmid identified in food animals, as reported by studies published in Taiwan (unpublished data).
In conclusion, the prevalence of mcr-1-positive E. coli in fecal carriages is low among community children in Southern Taiwan who were included in this study. Nevertheless, we can found a gradual upward trend of the prevalence from 2016 to 2019. This phenomenon shows the need for continuous follow-up. A higher frequency of chicken consumption was also associated with fecal carriages of mcr-1-positive E. coli. However, the genetic relationship between our colonized isolates and isolates from chicken in Taiwan warrants further evaluation.
Data Availability Statement
The raw data supporting the conclusions of this article will be made available by the authors, without undue reservation.
Ethics Statement
The studies involving human participants were reviewed and approved by VGHKS 16-CT2–04, VGHKS 18-CT3–11. Written informed consent to participate in this study was provided by the participants’ legal guardian/next of kin.
Author Contributions
P-CW, M-FC, and J-LW: conceptualization. P-CW, M-FC, W-YH, and C-HH: methodology. W-LC, M-FC, and C-HH: validation. P-CW, W-LC, and J-LW: formal analysis. P-CW and J-LW: investigation and writing—original draft preparation. W-YH and C-HH: data curation. J-LW and C-HH: writing—review and editing. C-HH: supervision. M-FC and J-LW: funding acquisition. All authors have read and agreed to the published version of the manuscript.
Funding
This work was supported by research grants from the Ministry of Science and Technology, Taiwan (MOST 110-2314-B-006-093-MY2), Veterans Affairs Council, Taiwan (VAC110-005-01), Kaohsiung Veterans General Hospital, Kaohsiung, Taiwan (KSVGH110-G01-1 and KSVGH110-G01-2), and Ministry of Science and Technology, Taiwan (MOST 108-2314-B-075B-002-MY3).
Conflict of Interest
The authors declare that the research was conducted in the absence of any commercial or financial relationships that could be construed as a potential conflict of interest.
Publisher’s Note
All claims expressed in this article are solely those of the authors and do not necessarily represent those of their affiliated organizations, or those of the publisher, the editors and the reviewers. Any product that may be evaluated in this article, or claim that may be made by its manufacturer, is not guaranteed or endorsed by the publisher.
Acknowledgments
We thank the members of C-HH’s laboratory at the I-Shou University for their excellent work in completing the E. coli isolation and PCR.
Abbreviations
CI, confidence interval; E. coli, Escherichia coli; ESBL, extended-spectrum β-lactamase; ExPEC, extraintestinal pathogenic E. coli; MIC, minimum inhibitory concentration; MLST, multilocus sequence typing; OR, odds ratio; UTI, urinary tract infection.
Footnotes
References
Agabou, A., Lezzar, N., Ouchenane, Z., Khemissi, S., Satta, D., Sotto, A., et al. (2016). Clonal relationship between human and avian ciprofloxacin-resistant Escherichia coli isolates in North-Eastern Algeria. Eur. J. Clin. Microbiol. Infect. Dis. 35, 227–234. doi: 10.1007/s10096-015-2534-3
Arcilla, M. S., van Hattem, J. M., Haverkate, M. R., Bootsma, M. C. J., van Genderen, P. J. J., Goorhuis, A., et al. (2017). Import and spread of extended-spectrum β-lactamase-producing Enterobacteriaceae by international travellers (COMBAT study): a prospective, multicentre cohort study. Lancet Infect. Dis. 17, 78–85. doi: 10.1016/s1473-3099(16)30319-x
Bi, Z., Berglund, B., Sun, Q., Nilsson, M., Chen, B., Tarnberg, M., et al. (2017). Prevalence of the mcr-1 colistin resistance gene in extended-spectrum beta-lactamase-producing Escherichia coli from human faecal samples collected in 2012 in rural villages in Shandong Province, China. Int. J. Antimicrob. Agents. 49, 493–497. doi: 10.1016/j.ijantimicag.2016.12.018
Brennan, E., Martins, M., McCusker, M. P., Wang, J., Alves, B. M., Hurley, D., et al. (2016). Multidrug-Resistant Escherichia coli in Bovine Animals, Europe. Emerg. Infect. Dis. 22, 1650–1652. doi: 10.3201/eid2209.160140
Chan, W. S., Au, C. H., Ho, D. N., Chan, T. L., Ma, E. S., and Tang, B. S. (2018). Prospective study on human fecal carriage of Enterobacteriaceae possessing mcr-1 and mcr-2 genes in a regional hospital in Hong Kong. BMC Infect. Dis. 18:81. doi: 10.1186/s12879-018-2987-y
Chia, J. H., Chu, C., Su, L. H., Chiu, C. H., Kuo, A. J., Sun, C. F., et al. (2005). Development of a multiplex PCR and SHV melting-curve mutation detection system for detection of some SHV and CTX-M beta-lactamases of Escherichia coli, Klebsiella pneumoniae, and Enterobacter cloacae in Taiwan. J. Clin. Microbiol. 43, 4486–4491. doi: 10.1128/JCM.43.9.4486-4491.2005
Clinical and Laboratory Standards Institute (2020). Performance Standards for Antimicrobial Susceptibility Testing, 30 Edn. Wayne, PA: Clinical and Laboratory Standards Institute.
Donà, V., Bernasconi, O. J., Pires, J., Collaud, A., Overesch, G., Ramette, A., et al. (2017). Heterogeneous Genetic Location of mcr-1 in Colistin-Resistant Escherichia coli Isolates from Humans and Retail Chicken Meat in Switzerland Emergence of mcr-1-Carrying IncK2 Plasmids. Antimicrob. Agents Chemother. 61, e1245–e1217.
Donabedian, S. M., Thal, L. A., Hershberger, E., Perri, M. B., Chow, J. W., Bartlett, P., et al. (2003). Molecular characterization of gentamicin-resistant Enterococci in the United States: evidence of spread from animals to humans through food. J. Clin. Microbiol. 41, 1109–1113. doi: 10.1128/jcm.41.3.1109-1113.2003
Elbediwi, M., Li, Y., Paudyal, N., Pan, H., Li, X., Xie, S., et al. (2019). Global Burden of Colistin-Resistant Bacteria: Mobilized Colistin Resistance Genes Study (1980-2018). Microorganisms. 7:461. doi: 10.3390/microorganisms7100461
Francisco, A. P., Bugalho, M., Ramirez, M., and Carriço, J. A. (2009). Global optimal eBURST analysis of multilocus typing data using a graphic matroid approach. BMC Bioinformatics 10:152. doi: 10.1186/1471-2105-10-152
Freitag, C., Michael, G., Li, J., Kadlec, K., Wang, Y., Hassel, M., et al. (2018). Occurrence and characterisation of ESBL-encoding plasmids among Escherichia coli isolates from fresh vegetables. Vet. Microbiol. 219, 63–69. doi: 10.1016/j.vetmic.2018.03.028
Fuentes-Castillo, D., Navas-Suarez, P. E., Gondim, M. F., Esposito, F., Sacristan, C., Fontana, H., et al. (2021). Genomic characterization of multidrug-resistant ESBL-producing Escherichia coli ST58 causing fatal colibacillosis in critically endangered Brazilian merganser (Mergus octosetaceus). Transbound. Emerg. Dis. 68, 258–266. doi: 10.1111/tbed.13686
Gelbicova, T., Barakova, A., Florianova, M., Jamborova, I., Zelendova, M., Pospisilova, L., et al. (2019). Dissemination and Comparison of Genetic Determinants of mcr-Mediated Colistin Resistance in Enterobacteriaceae via Retailed Raw Meat Products. Front. Microbiol. 10:2824. doi: 10.3389/fmicb.2019.02824
Giani, T., Sennati, S., Antonelli, A., Di Pilato, V., di Maggio, T., Mantella, A., et al. (2018). High prevalence of carriage of mcr-1-positive enteric bacteria among healthy children from rural communities in the Chaco region, Bolivia, September to October 2016. Euro Surveill. 23:1800115. doi: 10.2807/1560-7917.ES.2018.23.45.1800115
Guenther, S., Aschenbrenner, K., Stamm, I., Bethe, A., Semmler, T., Stubbe, A., et al. (2012). Comparable high rates of extended-spectrum-beta-lactamase-producing Escherichia coli in birds of prey from Germany and Mongolia. PLoS One 7:e53039. doi: 10.1371/journal.pone.0053039
Hammerum, A. M., Jakobsen, L., Olsen, S. S., and Agerso, Y. (2012). Characterization of CTX-M-14- and CTX-M-15-producing Escherichia coli of porcine origin. J. Antimicrob. Chemother. 67, 2047–2049. doi: 10.1093/jac/dks148
Hu, Y., Matsui, Y., and Lee, W. R. (2020). Risk factors for fecal carriage of drug-resistant Escherichia coli: a systematic review and meta-analysis. Antimicrob. Resist. Infect. Control. 9:31. doi: 10.1186/s13756-020-0691-3
Hu, Y. Y., Wang, Y. L., Sun, Q. L., Huang, Z. X., Wang, H. Y., Zhang, R., et al. (2017). Colistin resistance gene mcr-1 in gut flora of children. Int. J. Antimicrob. Agents. 50, 593–597. doi: 10.1016/j.ijantimicag.2017.06.011
Johnson, J. R., Gajewski, A., Lesse, A. J., and Russo, T. A. (2003). Extraintestinal pathogenic Escherichia coli as a cause of invasive nonurinary infections. J. Clin. Microbiol. 41, 5798–5802. doi: 10.1128/JCM.41.12.5798-5802.2003
Kuo, S. C., Huang, W. C., Wang, H. Y., Shiau, Y. R., Cheng, M. F., and Lauderdale, T. L. (2016). Colistin resistance gene mcr-1 in Escherichia coli isolates from humans and retail meats. Taiwan. J. Antimicrob. Chemother. 71, 2327–2329. doi: 10.1093/jac/dkw122
La, M. V., Lee, B., Hong, B. Z. M., Yah, J. Y., Koo, S. H., Jiang, B., et al. (2019). Prevalence and antibiotic susceptibility of colistin-resistance gene (mcr-1) positive Enterobacteriaceae in stool specimens of patients attending a tertiary care hospital in Singapore. Int. J. Infect. Dis. 85, 124–126. doi: 10.1016/j.ijid.2019.05.029
Lee, Y. L., Lu, M. C., Shao, P. L., Lu, P. L., Chen, Y. H., Cheng, S. H., et al. (2019). Nationwide surveillance of antimicrobial resistance among clinically important Gram-negative bacteria, with an emphasis on carbapenems and colistin: Results from the Surveillance of Multicenter Antimicrobial Resistance in Taiwan (SMART) in 2018. Int. J. Antimicrob. Agents. 54, 318–328. doi: 10.1016/j.ijantimicag.2019.06.009
Leimbach, A., Poehlein, A., Witten, A., Scheutz, F., Schukken, Y., Daniel, R., et al. (2015). Complete Genome Sequences of Escherichia coli Strains 1303 and ECC-1470 Isolated from Bovine Mastitis. Genome Announc. 3, e182–e115. doi: 10.1128/genomeA.00182-15
Lim, S. K., Kang, H. Y., Lee, K., Moon, D. C., Lee, H. S., and Jung, S. C. (2016). First Detection of the mcr-1 Gene in Escherichia coli Isolated from Livestock between 2013 and 2015 in South Korea. Antimicrob. Agents Chemother. 60, 6991–6993. doi: 10.1128/AAC.01472-16
Ling, Z., Yin, W., Shen, Z., Wang, Y., Shen, J., and Walsh, T. (2020). Epidemiology of mobile colistin resistance genes mcr-1 to mcr-9. J Antimicrob. Chemother. 75, 3087–3095. doi: 10.1093/jac/dkaa205
Liu, J. Y., Liao, T. L., Huang, W. C., Liu, Y. M., Wu, K. M., Lauderdale, T. L., et al. (2020). Increased mcr-1 in pathogenic Escherichia coli from diseased swine, Taiwan. J. Microbiol. Immunol. Infect. 53, 751–756. doi: 10.1016/j.jmii.2018.10.011
Liu, Y. Y., Sun, Y. W., Sun, H. R., Luo, X. W., He, D. D., Wu, H., et al. (2020). Characterization of the IncHI2 plasmid pTW4 harboring tet(M) from an isolate of Escherichia coli ST162. J. Antibiot. 73, 876–880. doi: 10.1038/s41429-020-0337-y
Liu, Y. Y., Wang, Y., Walsh, T. R., Yi, L. X., Zhang, R., Spencer, J., et al. (2016). Emergence of plasmid-mediated colistin resistance mechanism MCR-1 in animals and human beings in China: a microbiological and molecular biological study. Lancet Infect. Dis. 16, 161–168. doi: 10.1016/S1473-3099(15)00424-7
Luo, J., Yao, X., Lv, L., Doi, Y., Huang, X., Huang, S., et al. (2017). Emergence of mcr-1 in Raoultella ornithinolytica and Escherichia coli Isolates from Retail Vegetables in China. Antimicrob. Agents Chemother. 61, e1139–e1117. doi: 10.1128/AAC.01139-17
Luo, Q., Wang, Y., and Xiao, Y. (2020). Prevalence and transmission of mobilized colistin resistance (mcr) gene in bacteria common to animals and humans. Biosafety Health 2, 71–78. doi: 10.1016/j.bsheal.2020.05.001
Magiorakos, A. P., Srinivasan, A., Carey, R. B., Carmeli, Y., Falagas, M. E., Giske, C. G., et al. (2012). Multidrug-resistant, extensively drug-resistant and pandrug-resistant bacteria: an international expert proposal for interim standard definitions for acquired resistance. Clin. Microbiol. Infect. 18, 268–281. doi: 10.1111/j.1469-0691.2011.03570.x
McInnes, R. S., McCallum, G. E., Lamberte, L. E., and van Schaik, W. (2020). Horizontal transfer of antibiotic resistance genes in the human gut microbiome. Curr. Opin. Microbiol. 53, 35–43. doi: 10.1016/j.mib.2020.02.002
Moreno, E., Andreu, A., Pigrau, C., Kuskowski, M. A., Johnson, J. R., and Prats, G. (2008). Relationship between Escherichia coli strains causing acute cystitis in women and the fecal E. coli population of the host. J. Clin. Microbiol. 46, 2529–2534. doi: 10.1128/JCM.00813-08
Nakayama, T., Kumeda, Y., Kawahara, R., Yamaguchi, T., and Yamamoto, Y. (2018). Carriage of colistin-resistant, extended-spectrum beta-lactamase-producing Escherichia coli harboring the mcr-1 resistance gene after short-term international travel to Vietnam. Infect Drug Resist. 11, 391–395. doi: 10.2147/IDR.S153178
Olaitan, A. O., Morand, S., and Rolain, J. M. (2014). Mechanisms of polymyxin resistance: acquired and intrinsic resistance in bacteria. Front. Microbiol. 5:643. doi: 10.3389/fmicb.2014.00643
OstholmBalkhed, A., Tarnberg, M., Nilsson, M., Nilsson, L. E., Hanberger, H., Hallgren, A., et al. (2018). Duration of travel-associated faecal colonisation with ESBL-producing Enterobacteriaceae - A one year follow-up study. PLoS One 13:e0205504. doi: 10.1371/journal.pone.0205504
Paalanne, N., Husso, A., Salo, J., Pievilainen, O., Tejesvi, M. V., Koivusaari, P., et al. (2018). Intestinal microbiome as a risk factor for urinary tract infections in children. Eur. J. Clin. Microbiol. Infect. Dis. 37, 1881–1891. doi: 10.1007/s10096-018-3322-7
Pietsch, M., Irrgang, A., Roschanski, N., Brenner Michael, G., Hamprecht, A., Rieber, H., et al. (2018). Whole genome analyses of CMY-2-producing Escherichia coli isolates from humans, animals and food in Germany. BMC Genomics 19:601. doi: 10.1186/s12864-018-4976-3
Poirel, L., Jayol, A., and Nordmann, P. (2017). Polymyxins: Antibacterial Activity, Susceptibility Testing, and Resistance Mechanisms Encoded by Plasmids or Chromosomes. Clin. Microbiol. Rev. 30, 557–596. doi: 10.1128/CMR.00064-16
Purohit, M. R., Lindahl, L. F., Diwan, V., Marrone, G., and Lundborg, C. S. (2019). High levels of drug resistance in commensal E. coli in a cohort of children from rural central India. Sci. Rep. 9:6682. doi: 10.1038/s41598-019-43227-1
Rolain, J. M. (2013). Food and human gut as reservoirs of transferable antibiotic resistance encoding genes. Front. Microbiol. 4:173. doi: 10.3389/fmicb.2013.00173
Sacramento, A. G., Fernandes, M. R., Sellera, F. P., Munoz, M. E., Vivas, R., Dolabella, S. S., et al. (2018). Genomic analysis of MCR-1 and CTX-M-8 co-producing Escherichia coli ST58 isolated from a polluted mangrove ecosystem in Brazil. J. Glob. Antimicrob. Resist. 15, 288–289. doi: 10.1016/j.jgar.2018.10.024
Shen, Y., Zhou, H., Xu, J., Wang, Y., Zhang, Q., Walsh, T. R., et al. (2018). Anthropogenic and environmental factors associated with high incidence of mcr-1 carriage in humans across China. Nat. Microbiol. 3, 1054–1062. doi: 10.1038/s41564-018-0205-8
Sidjabat, H. E., Paterson, D. L., Adams-Haduch, J. M., Ewan, L., Pasculle, A. W., Muto, C. A., et al. (2009). Molecular epidemiology of CTX-M-producing Escherichia coli isolates at a tertiary medical center in western Pennsylvania. Antimicrob. Agents Chemother. 53, 4733–4739. doi: 10.1128/AAC.00533-09
Sørensen, T. L., Blom, M., Monnet, D. L., Frimodt-Møller, N., Poulsen, R. L., and Espersen, F. (2001). Transient intestinal carriage after ingestion of antibiotic-resistant Enterococcus faecium from chicken and pork. N. Engl. J. Med. 345, 1161–1166. doi: 10.1056/NEJMoa010692
Storm, D. R., Rosenthal, K. S., and Swanson, P. E. (1977). Polymyxin and related peptide antibiotics. Annu. Rev. Biochem. 46, 723–763. doi: 10.1146/annurev.bi.46.070177.003451
Terveer, E. M., Nijhuis, R. H. T., Crobach, M. J. T., Knetsch, C. W., Veldkamp, K. E., Gooskens, J., et al. (2017). Prevalence of colistin resistance gene (mcr-1) containing Enterobacteriaceae in feces of patients attending a tertiary care hospital and detection of a mcr-1 containing, colistin susceptible E. coli. PLoS One 12:e0178598. doi: 10.1371/journal.pone.0178598
von Wintersdorff, C. J., Wolffs, P. F., van Niekerk, J. M., Beuken, E., van Alphen, L. B., Stobberingh, E. E., et al. (2016). Detection of the plasmid-mediated colistin-resistance gene mcr-1 in faecal metagenomes of Dutch travellers. J. Antimicrob. Chemother. 71, 3416–3419. doi: 10.1093/jac/dkw328
Wang, M., Zeng, Z., Jiang, F., Zheng, Y., Shen, H., Macedo, N., et al. (2020). Role of enterotoxigenic Escherichia coli prophage in spreading antibiotic resistance in a porcine-derived environment. Environ. Microbiol. 22, 4974–4984. doi: 10.1111/1462-2920.15084
Wang, Y., Tian, G. B., Zhang, R., Shen, Y., Tyrrell, J. M., Huang, X., et al. (2017b). Prevalence, risk factors, outcomes, and molecular epidemiology of mcr-1-positive Enterobacteriaceae in patients and healthy adults from China: an epidemiological and clinical study. Lancet Infect. Dis. 17, 390–399. doi: 10.1016/S1473-3099(16)30527-8
Wang, Y., Lo, W. U., Lai, R. W., Tse, C. W., Lee, R. A., Luk, W. K., et al. (2017a). IncN ST7 epidemic plasmid carrying blaIMP-4 in Enterobacteriaceae isolates with epidemiological links to multiple geographical areas in China. J. Antimicrob. Chemother. 72, 99–103. doi: 10.1093/jac/dkw353
Wu, P. C., Wang, J. L., Hsueh, P. R., Lin, P. H., Cheng, M. F., Huang, I. F., et al. (2019). Prevalence and risk factors for colonization by extended-spectrum beta-lactamase-producing or ST 131 Escherichia coli among asymptomatic adults in community settings in Southern Taiwan. Infect. Drug Resist. 12, 1063–1071. doi: 10.2147/IDR.S201086
Xu, Y., Bai, X., Jin, Y., Hu, B., Wang, H., Sun, H., et al. (2017). High Prevalence of Virulence Genes in Specific Genotypes of Atypical Enteropathogenic Escherichia coli. Front. Cell Infect. Microbiol. 7:109. doi: 10.3389/fcimb.2017.00109
Yang, X., Liu, W., Liu, Y., Wang, J., Lv, L., Chen, X., et al. (2014). F33: A-: B-, IncHI2/ST3, and IncI1/ST71 plasmids drive the dissemination of fosA3 and bla CTX-M-55/-14/-65 in Escherichia coli from chickens in China. Front. Microbiol. 5:688. doi: 10.3389/fmicb.2014.00688
Zajac, M., Sztromwasser, P., Bortolaia, V., Leekitcharoenphon, P., Cavaco, L. M., Zietek-Barszcz, A., et al. (2019). Occurrence and Characterization of mcr-1-Positive Escherichia coli Isolated From Food-Producing Animals in Poland, 2011-2016. Front. Microbiol. 10:1753. doi: 10.3389/fmicb.2019.01753
Zhang, P., Wang, J., Wang, X., Bai, X., Ma, J., Dang, R., et al. (2019). Characterization of Five Escherichia coli Isolates Co-expressing ESBL and MCR-1 Resistance Mechanisms From Different Origins in China. Front. Microbiol. 10:1994. doi: 10.3389/fmicb.2019.01994
Zurfluh, K., Stephan, R., Widmer, A., Poirel, L., Nordmann, P., Nüesch, H. J., et al. (2017b). Screening for fecal carriage of MCR-producing Enterobacteriaceae in healthy humans and primary care patients. Antimicrob. Resist. Infect. Control. 6:28. doi: 10.1186/s13756-017-0186-z
Keywords: mcr-1, risk factor, prevalence, community children, fecal carriage, Taiwan
Citation: Wu P-C, Cheng M-F, Chen W-L, Hung W-Y, Wang J-L and Hung C-H (2021) Risk Factors and Prevalence of mcr-1-Positive Escherichia coli in Fecal Carriages Among Community Children in Southern Taiwan. Front. Microbiol. 12:748525. doi: 10.3389/fmicb.2021.748525
Received: 28 July 2021; Accepted: 25 October 2021;
Published: 19 November 2021.
Edited by:
Krassimira Hristova, Marquette University, United StatesReviewed by:
Michael Brouwer, Wageningen University and Research, NetherlandsGerald Larrouy-Maumus, Imperial College London, United Kingdom
Copyright © 2021 Wu, Cheng, Chen, Hung, Wang and Hung. This is an open-access article distributed under the terms of the Creative Commons Attribution License (CC BY). The use, distribution or reproduction in other forums is permitted, provided the original author(s) and the copyright owner(s) are credited and that the original publication in this journal is cited, in accordance with accepted academic practice. No use, distribution or reproduction is permitted which does not comply with these terms.
*Correspondence: Jiun-Ling Wang, aml1bmxpbmd3YW5nQGdtYWlsLmNvbQ==; Chih-Hsin Hung, Y2hodW5nQGlzdS5lZHUudHc=