- 1National Engineering Laboratory for Druggable Gene and Protein Screening, Northeast Normal University, Changchun, China
- 2State Key Laboratory of Microbial Technology, Shandong University, Qingdao, China
- 3NMPA Key Laboratory for Quality Control of Cell and Gene Therapy Medicine Products, Northeast Normal University, Changchun, China
- 4Guangdong Provincial Key Laboratory of Malignant Tumor Epigenetics and Gene Regulation, Department of Medical Research Center, Sun Yat-Sen Memorial Hospital, Guangzhou, China
- 5Faculty of Chemistry, Northeast Normal University, Changchun, China
Streptococcus mutans (S. mutans), the prime pathogen of dental caries, can secrete glucosyltransferases (GTFs) to synthesize extracellular polysaccharides (EPSs), which are the virulence determinants of cariogenic biofilms. Ursolic acid, a type of pentacyclic triterpene natural compound, has shown potential antibiofilm effects on S. mutans. To investigate the mechanisms of ursolic acid-mediated inhibition of S. mutans biofilm formation, we first demonstrated that ursolic acid could decrease the viability and structural integrity of biofilms, as evidenced by XTT, crystal violet, and live/dead staining assays. Then, we further revealed that ursolic acid could compete with the inherent substrate to occupy the catalytic center of GTFs to inhibit EPS formation, and this was confirmed by GTF activity assays, computer simulations, site-directed mutagenesis, and capillary electrophoresis (CE). In conclusion, ursolic acid can decrease bacterial viability and prevent S. mutans biofilm formation by binding and inhibiting the activity of GTFs.
Introduction
Most oral diseases, including periodontal diseases and especially dental caries, are prevalent and common public health problems worldwide (Jakubovics et al., 2021). Approximately 60–90% of school-aged children and almost all adults suffer from dental caries (Wong et al., 2017). Dental caries is a chronic bacterial infectious disease that occurs on the tooth surface (Taubman and Nash, 2006). Traditional methods used to prevent or cure dental caries include mechanical removal of plaque (e.g., toothbrushing), the usage of broad-spectrum antibiotics, and more comprehensive forms of clinical treatment (e.g., root canal therapy, surgery, and dental restorations; Chen et al., 2016). However, the effect of prevention can be limited by poor toothbrushing technique and drug resistance (Cummins, 2013). Dental treatment is expensive, averaging 5% of total health expenditures and 20% of out-of-pocket health expenditures in most high-income countries, and is beyond the capacity of healthcare systems in most low- and middle-income countries (Vernazza et al., 2021). Therefore, more effective preventive and therapeutic strategies for dental caries are needed.
Dental caries is a typical biofilm induction-related disease, and cariogenic biofilms are one of the main factors leading to bacterial infection since they can protect microorganisms by enhancing microbial resistance to the host’s immunologic defense and antibacterial agents (Takahashi and Nyvad, 2011; Pitts et al., 2017). Streptococcus mutans (S. mutans), the prime pathogen of dental caries, is an important contributor to the formation of cariogenic biofilms (Wang et al., 2020). S. mutans secretes three types of glucosyltransferases (GTFs), namely, GTF-I (also known as GtfB), GTF-SI (GtfC), and GTF-S (GtfD; Bowen and Koo, 2011). These GTFs can use dietary sucrose to synthesize extracellular polysaccharides (EPSs), and EPSs are the virulence determinants of cariogenic biofilms (Tamesada et al., 2004; Bowen and Koo, 2011; Jaña et al., 2018). GTF-I and GTF-SI catalyze mainly the synthesis of water-insoluble glucans, and GTF-S produces mainly water-soluble glucans (Monchois et al., 1999; Bowen et al., 2018). Water-insoluble glucans help bacteria adhering and aggregating on the tooth surface to form biofilms, while water-soluble glucan may supply a source of metabolizable carbohydrates for plaque bacteria and induce water-insoluble glucan formation (Jakubovics et al., 2021). Therefore, GTFs play key roles in causing and forming dental caries and are an effective target for the prevention and treatment of dental caries or other related diseases (e.g., infective endocarditis; Shun et al., 2005).
Ursolic acid, a type of pentacyclic triterpene compound, can be isolated in abundance from many foods (e.g., apples, olive, and basil) and medicinal plants (e.g., Malus pumila, Ocimum basiliacum, and Rosmarinus officinalis; Ikeda et al., 2008; Bacanlı et al., 2017; Hui et al., 2021). Ursolic acids have been reported to have many beneficial bioactivities, such as anticancer (Patlolla and Rao, 2012), anti-inflammatory (Pádua et al., 2014), antimicrobial (Kim et al., 2012), immunity regulation (Xu et al., 2019), and antiviral activities (Kong et al., 2013). In our previous research, we confirmed that the crude extract of Bergenia crassifolia leaves could inhibit S. mutans biofilm formation (Liu et al., 2017). In a screen of the active ingredients of Bergenia crassifolia leaves for antibiofilm activity, we found that ursolic acid had a significant effect. Here, we confirmed the antibiofilm activities of ursolic acid against S. mutans and further revealed the mechanism underlying the inhibitory effect of ursolic acid on GTF-mediated synthesis of EPSs. Our study provides a potential antimicrobial agent that can be used to prevent and cure oral and other GTF-related diseases.
Materials and Methods
Bacterial Strain, Growth Conditions, and Chemicals
S. mutans (ATCC 251175) was obtained from the Guangdong Microbiology Culture Center and was cultured in brain heart infusion (BHI) broth (Hopebio, Qingdao, China) supplemented with 1% sucrose at 37°C for 24h under aerobic conditions. After incubation, the bacterial concentration was 107cfu/ml, as determined by spectrophotometry (OD630=0.2). Ursolic acid was purchased from the National Institutes for Food and Drug Control with purity >98% and was dissolved in dimethyl sulfoxide.
Antimicrobial Activity Assay
The potential inhibitory activity of ursolic acid against S. mutans was determined by the microdilution method as described previously (Liu et al., 2017), with minor modifications. Briefly, ursolic acid was serially diluted twofold in BHI broth containing 1% sucrose, with the final concentration of ursolic acid ranging from 0.25 to 0.031mg/ml. Twenty microliters of sterile solution of resazurin sodium per well was added to the bacterial culture and incubated at 37°C for 24h. BHI broth alone was used as blank control, bacterial suspension alone was used as a noninhibition negative control, and chlorhexidine treatment at a final concentration of 0.6mg/ml was used as a positive control. As bacteria grow, resazurin is reduced to resorufin, resulting in the medium color changing from blue to pink (Palomino et al., 2002; Sarker et al., 2007). The lowest concentration of ursolic acid that could inhibit the medium color change from blue to pink was defined as the minimal inhibitory concentration (Palomino et al., 2002; Süntar et al., 2016). The bacterial cultures treated with ursolic acid at concentrations equal to or higher than the minimum inhibitory concentration (MIC) were transferred to BHI agar plates and incubated at 37°C for 24h. The lowest concentration that resulted in no visible bacterial colonies on the agar plates after incubation was defined as the minimal bactericidal concentration (MBC; Liu et al., 2017).
XTT Reduction Assay
The effect of ursolic acid on the viability of biofilms was evaluated using a 2,3-bis (2-methyloxy-4-nitro-5-sulfophenyl)-2H-tetrazolium-5-carboxanilide (XTT) reduction assay as described previously (Duque et al., 2017), with some modification. Briefly, after 24h of incubation for cultured bacteria to form biofilms in 96-well microplates, supernatants were removed, and the wells were gently washed three times with PBS (pH 7.0) to remove nonadhered bacteria. Then, the cultures were incubated at 37°C for another 22h in 200μl of BHI broth including 100μl of ursolic acid at various concentrations. After removing the supernatants, 50μl of XTT (Sigma, St. Louis, MO, United States) reagent was added, and the microplate was kept in the dark for 2h at 37°C. Then, the absorbance of the colored product was detected at 490nm using an ELISA reader. Alternatively, after ursolic acid treatment, a crystal violet assay was used to evaluate the attachment of biofilm biomass as described previously (Cardoso Sá et al., 2012).
Live/Dead Bacterial Staining
Fluorescence staining was used to determine biofilm integrity as described previously (Liu et al., 2017). After biofilm formation, ursolic acid was added at a final concentration of 1/2 MIC followed by incubation at 37°C for another 18h. The culture was then removed from the supernatant and gently washed twice with sterile water. Staining was carried out by means of the Live/Dead BacLight Bacterial Viability Kit (L13152, Invitrogen, Carlsbad, CA, United States) for 30min at room temperature in the dark. Stained cells were observed under a fluorescence microscope (Nikon Eclipse 80i; Nikon Co., Japan). The kit utilizes a mixture of SYTO 9, a green-fluorescent nucleic acid stains and propidium iodide (PI), a red-fluorescent nucleic acid stains. SYTO 9 is a membrane-permeable fluorescent marker that stains all cells, while PI penetrates only bacteria with damaged membranes and stains dead cells red. Twenty fields per sample were randomly selected to analyze the intensity of red and green fluorescence.
Extracellular Polysaccharide Production Assay
The method for quantification of EPS production was conducted as described previously (Packiavathy et al., 2012). Briefly, the bacterial culture was incubated with or without ursolic acid at concentrations ranging from 0.008 to 0.125mg/ml at 37°C for 16h, the culture was centrifuged (4°C, 12000×g, 30min), and the supernatants and cells were collected. The water-soluble and water-insoluble glucans were prepared using the method described previously (Yano et al., 2012). Briefly, water-soluble glucans were obtained by ethanol precipitation of the supernatants. The cells were resuspended in 1M NaOH and centrifuged to collect supernatants, which were used to prepare water-insoluble glucans by ethanol precipitation. The phenol/H2SO4 method was used to quantify two types of EPS in the supernatant as described previously (Liu et al., 2017). The absorbance of the color was detected at 490nm using an ELISA reader.
Molecular Dynamics Simulations
The crystal structure of glucansucrase was downloaded from the Protein Data Bank (ID: 3AIC) as the receptor, and the preparation work was done using the software Gold 5.2 and AutoDockTool 1.5.6. The MD simulation of the system was performed for 20ns under the npt ensemble, and the data were saved every other 5ps. CPPTRAJ was used for data analysis. The MMPBSA.py module was used to compute the binding free energy between the protein and ligand.
To further confirm the binding site between GTF-SI and ursolic acid, the amino acids in the predicted binding site were replaced by other amino acids, as shown in Supplementary Table S1 Afterward, the mutant GTF-SI proteins were subjected to molecular docking under the same conditions as above.
GTF Activity Assay
The method for analyzing the enzymatic activity of the crude extract of GTFs was used as described previously by Koo et al (Koo et al., 2000). A 20-mL bacterial suspension of S. mutans was incubated in 200ml of BHI broth containing 1% sucrose at 37°C. After incubation, supernatants were collected, and ammonium sulfate was added at 60% saturation for 24h to prepare the protein. The crude enzymes were dissolved in PBS (pH 6.0). The reaction system mixture was the same as that described previously (Liu et al., 2017). The final concentration of ursolic acid ranging from 0.04 to 0.12mg/ml was used to measure the inhibition of the synthesis of EPSs. After incubation, the method to determine the amount of the two types of glucans was applied as described above.
Preparation of Recombinant GTF and Its Mutants
cDNA fragments of wild-type GTF-SI (GenBank: M22054.1) and two mutant GTF-SI variants (Supplementary Table S1) were synthesized and inserted into the NdeI and XhoI sites of pCold I to generate pCold-GTF-SI recombinant plasmids with a 6× histidine tag at the N-terminus of the proteins (Ito et al., 2011). The recombinant proteins were purified by using GE metal affinity resin and dialyzed. SDS-PAGE was used to analyze recombinant GTF-SI proteins (shown in Supplementary Figure S1).
Capillary Electrophoresis Assay
Capillary electrophoresis (CE) was used to quantify fructose production catalyzed by GTFs as described previously (Rizelio et al., 2012). Recombinant GTF-SI was crudely extracted from recombinant transformants by ultrasonic disruption. Then, 50μl of the crude enzyme solution together with or without ursolic acid at a final concentration of 0.03mg/ml or 0.02mg/ml was added to 1ml of sucrose (0.1M) and incubated at 37°C for 2h. After incubation, the culture was centrifuged (4°C, 12000×g, 10min), and the supernatants were collected and filtered with a 0.22-μm nylon membrane before being subjected to the CE assay. The CE assay was conducted in a CE system, and the conditions to detect fructose were as follows: The detection wavelength was set at 254nm; the temperature was maintained at 25°C; and fused silica capillaries had dimensions of 50cm in total length and 40cm in effective length, and the inner diameter and outer diameter were 50μm and 375μm, respectively. The sample injection was maintained at an elevation difference of 20cm from the nearest detector for 5s, and the separation voltage was 25kV. The capillary system was rinsed with 0.1M NaOH for 5min, distilled water for 3min, and PBS for 3min before each run.
CE can also be used to detect the binding of drugs and proteins, which was used in this study to analyze the interaction of ursolic acid with GTF-SI and its variants as described previously (Liang et al., 2020). GTF-SI and its variants were dissolved in PBS (20mm, pH=6.8) and filtered with a 0.22-μm nylon membrane. Ursolic acid was dissolved in the same solution as that used to dissolve the proteins, and the final concentration was 0.3mg/ml. Afterward, the GTF or its variants were incubated with ursolic acid for 2h. Finally, CE was conducted as described above with a few differences: The detection wavelength was 210nm, and the separation voltage was 20kV.
Results
Effects of Ursolic Acid on Biofilms
Biofilms are the key factors in the induction of dental caries and periodontitis. We first measured the MIC and the MBC values of ursolic acid against S. mutans. The MIC value is the lowest concentration that could prevent the culture color from changing blue to pink. The MBC value is the lowest concentration that resulted in no visible bacterial colonies on the agar plates after incubation. As shown in Supplementary Figure S2 and shown in Table 1, both the MIC and MBC values of ursolic acid against S. mutans were 0.25mg/ml. Based on the concentration of antimicrobial activities, we evaluated the effect of ursolic acid on S. mutans biofilms by the XTT reduction method. As shown in Figure 1A, the bacterial viability within the biofilms decreased as the concentration of ursolic acid increased, while the difference was most significant at the concentration of 0.063mg/ml. Compared with the positive control, ursolic acid showed similar inhibitory effects on the bacterial viability of biofilms at 0.125mg/ml (Supplementary Figure S3). In addition, the crystal violet staining results showed that the amount of biofilm was decreased upon ursolic acid treatment, as shown in Figure 1B, which is consistent with the data of the XTT reduction assay.
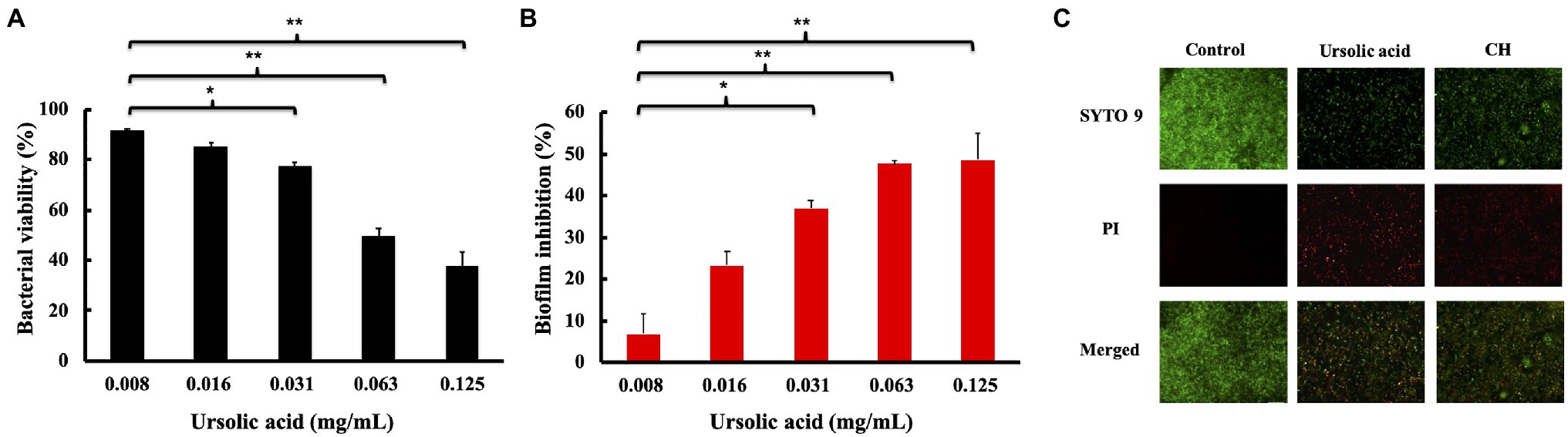
Figure 1. Effects of ursolic acid on biofilms. (A): Percentage of bacterial viability within biofilms detected by XTT assay; (B): Inhibitory percentage of ursolic acid on biofilm formation detected by crystal violet staining. Data are presented as the mean±standard deviation. *p<0.05 and **p<0.01. (C): Effects of ursolic acid on biofilm structure. SYTO 9: green fluorescence, which stains both the dead and live bacterial cells; PI: red fluorescence, which stains dead bacterial cells. Control: ursolic acid untreated biofilms; CH: chlorhexidine (the positive control).
To observe the direct effects of ursolic acid on biofilms, the Live/Dead BacLight Bacterial Viability kit was used to examine S. mutans bacteria within the biofilm, as shown in Figure 1. Compared with the control sample, the sample treated with ursolic acid had a large number of red-stained bacterial cells, indicating dead cells. Furthermore, the biofilms were thin. This result confirmed that ursolic acid could significantly destroy biofilms by affecting bacterial survival and adhesion.
Effects of Ursolic Acid on GTF Activity
EPSs are the key factors contributing to bacterial colonization, biofilm formation, maturation, and caries. Because EPSs are synthesized by GTFs, we explored the effect of ursolic acid on GTF activity in crude bacterial extracts in the presence of sucrose in vitro. As shown in Figure 2, ursolic acid inhibited the activity of GTFs, resulting in a decrease in the synthesis of EPS products. The inhibition of ursolic acid on water-soluble glucan production was stronger than that on water-insoluble glucan production at low concentrations of less than 0.06mg/ml, while this inhibition tendency was reversed at concentrations from 0.06mg/ml to 0.12mg/ml. Combined with the data above, these results suggest that ursolic acid could suppress the aggregation or adhesion of S. mutans to form cariogenic biofilms by preventing GTFs from synthesizing EPSs.
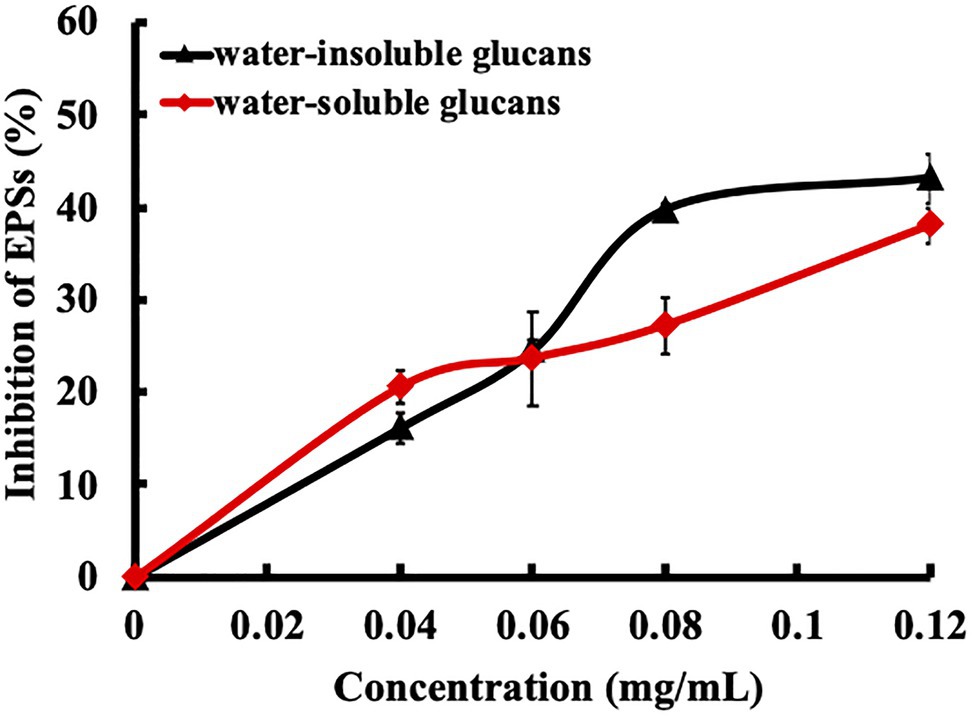
Figure 2. Effect of ursolic acid on glucosyltransferase (GTF) activities. GTFs were crudely extracted from bacterial culture medium and were then used to test the effect of ursolic acid on its activities by using an in vitro enzymatic reaction system. The final concentration of ursolic acid ranged from 0.04 to 0.12mg/ml. Extracellular polysaccharides (EPSs), including soluble and insoluble glucans, which are the catalytic products of GTFs, were measured as indicators of GTF activity. The data are presented as the mean ± standard deviation.
The Mode of Interaction Between Ursolic Acid and GTF-SI
The catalytic center of GTF-SI has been shown to include two subsites for EPS synthesis: Subsite+1 contains the key amino acids for sucrose to bind, and subsite-1 contains the key amino acids for glucosyl moiety polymerization to form EPSs (Ito et al., 2011). In particular, Trp517 provides a platform for the acceptor glycosyl moiety of sucrose, and Tyr430 participates in hydrophobic interactions with carbon atoms of the glycosyl moiety in subsite+1; however, Asp909 and Tyr916 are related to recognition of the glucosyl moiety of the primary sucrose and formation of the glycosyl-enzyme intermediate in subsite-1 (Ito et al., 2011). As shown in Figure 3A, the simulation data showed that ursolic acid formed hydrogen bonds with Tyr430 and Asp909 of GTF-SI and had hydrophobic interactions with Leu433, Leu434, Phe907, Trp517, and Tyr916, which are the key sites for catalyzing sucrose to synthesize EPSs. Furthermore, the MD simulation results showed that the binding free energy of GTF-SI to ursolic acid was similar to that of sucrose (Supplementary Table S2). Then, we made two mutant GTF-SI variants in which the predicted amino acids that are essential for ursolic acid binding were replaced with other amino acids (Supplementary Table S2) to further verify the binding indicated by the simulation. The simulation showed that the number of amino acids in either GTF-SI variant that formed hydrogen bonds and hydrophobic interactions with ursolic acid decreased compared with that in wild-type GTF-SI (Figures 3B,C), and the interaction and binding free energy of the GTF-SI variants with ursolic acid were also decreased (Supplementary Table S2, S3).
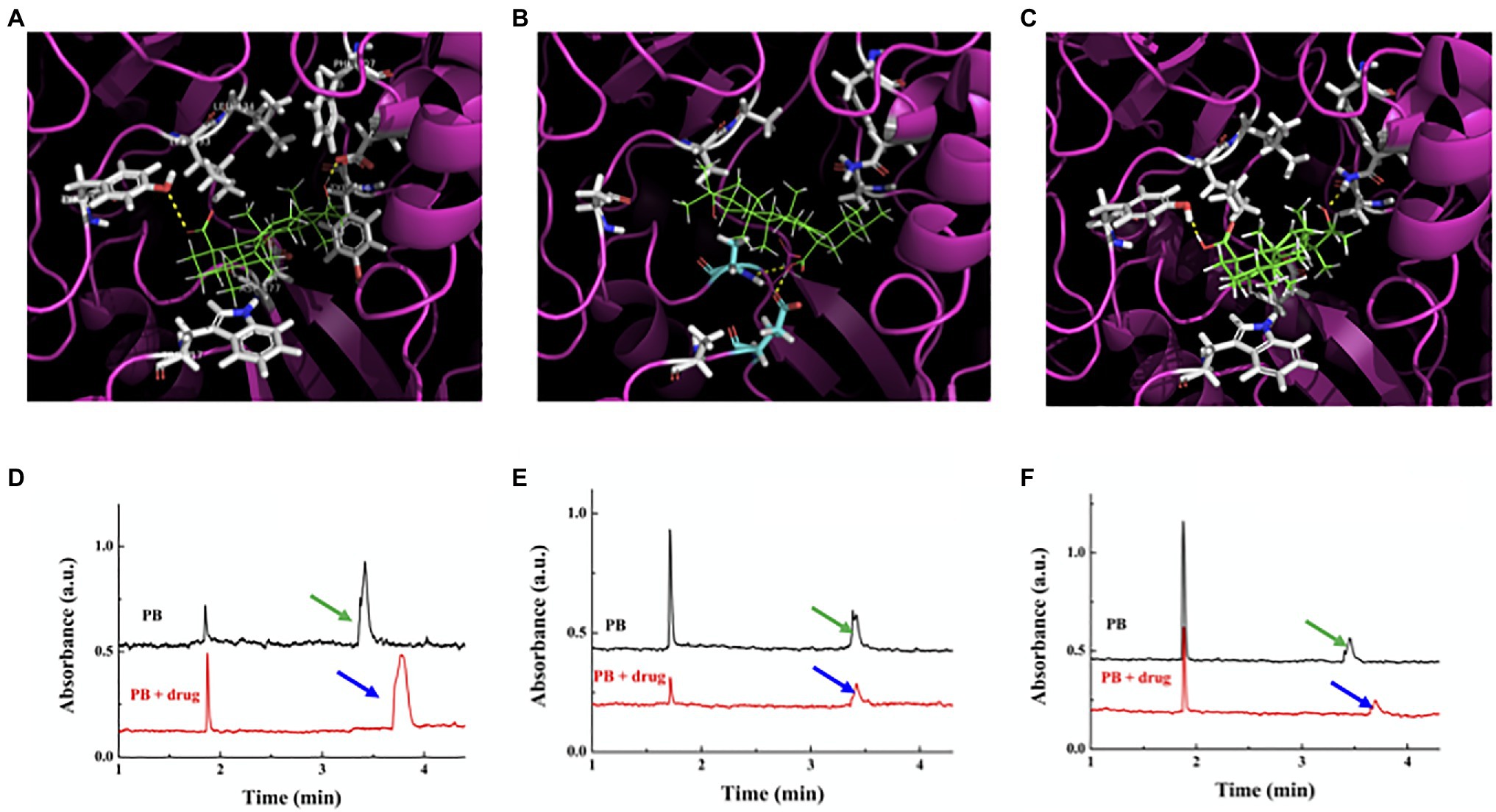
Figure 3. Interaction between ursolic acid and GTF-SI. (A-C) Binding model of ursolic acid with GTF-SI and its variants simulated by Gold software. (A) Wild-type GTF-SI; (B) GTF-SI variant A; (C) GTF-SI variant B. Ursolic acid, as the ligand, is shown in green. The protein is shown as a cartoon with pink color. The yellow dotted lines represent hydrogen bonds. (D-F): Interaction between ursolic acid and GTF-SI measured by CE assay. The black line represents the migration time of GTF-SI and its variants, whereas the red line is the migration time of GTF-SI or its variants together with ursolic acid. (D) Wild-type GTF-SI; (E) GTF-SI variant A; (F) GTF-SI variant B. Arrows point to the peak of the protein (black)/protein and ursolic acid complex (red).
Then, CE technology was used to further determine the interaction between GTFs and ursolic acid in vitro. As shown in Figure 3D, compared with that of the control, the migration time of the protein was prolonged by the addition of ursolic acid, which indicated that ursolic acid could bind with GTF-SI to change the charge-mass ratio, leading to a migration time-shift. The CE results further showed that the retention time of the variant A or variant B proteins was shorter than that of wild-type GTF-SI after the addition of ursolic acid, suggesting that the interaction between GTF-SI and ursolic acid became weaker or even lost as several key amino acids at the binding site were replaced (Figures 3E,F). These results indicate that ursolic acid might inhibit the enzymatic activity of GTFs through direct binding and that Phe907, Asp909 and Tyr916 in GTF-SI might be the key amino acids responsible for ursolic acid binding.
Effects of Ursolic Acid on EPSs and Fructose Production
The process of GTF synthesis of EPS includes two steps: First, the GTF enzyme binds with sucrose, and then, EPS synthesis is catalyzed. After sucrose binds with subsite+1 of the GTF, the glucosyl group of sucrose dissociates from subsite+1 and then binds subsite-1 of the GTF to form an intermediate that catalyzes EPS production, while the fructosyl group of sucrose dissociates from subsite+1 of the GTF to produce the by-product, namely, fructose (Ito et al., 2011). Therefore, the production of fructose and EPSs would decrease when the binding site and catalytic site of the GTF are occupied by a molecule other than sucrose. Since the amino acids in GTF-SI that are essential for ursolic acid binding are also indispensable for sucrose recognition and since the MD simulation results showed that the binding free energy of GTF-SI to ursolic acid was similar to that of sucrose (Supplementary Table S2), we hypothesized that ursolic acid may suppress GTF activity by competitively blocking sucrose binding. To test this hypothesis, we evaluated the effects of ursolic acid on the production of EPS and fructose. To confirm the effect of ursolic acid on EPS synthesis by S. mutans, water-soluble glucans and water-insoluble glucans were obtained from bacterial culture treated with or without ursolic acid for 16h, and the phenol/H2SO4 method was used to detect the content of both types of EPSs. As shown in Figure 4A, ursolic acid at concentrations of 0.008mg/ml to 0.125mg/ml inhibited the production of both water-soluble and water-insoluble glucans. Additionally, the amount of fructose produced by crude recombinant GTF-SI was further measured in the presence of sucrose and ursolic acid for 2h. As shown in Figure 4B, the amount of fructose production decreased with the addition of ursolic acid to the enzymatic reaction system, and the effect of a high concentration was more obvious than that of a low concentration. Based on this in combination with the data above, we proposed that ursolic acid could compete with sucrose to occupy the catalytic center of the GTF, which then inhibits the enzymes that synthesize EPSs and eventually prevents bacterial adhesion to form biofilms.
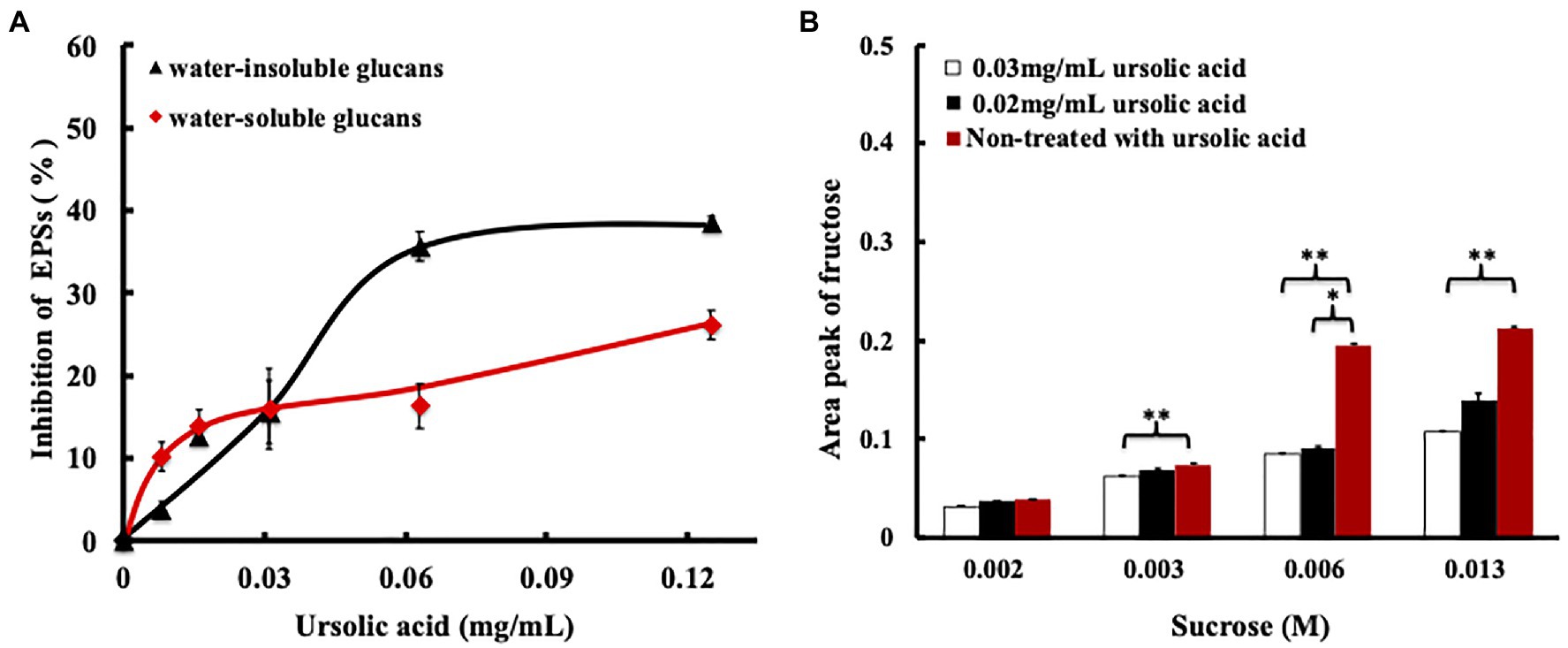
Figure 4. Effect of ursolic acid on GTF catalyzed products. (A) Inhibitory percentage of ursolic acid on EPS production determined by phenol/H2SO4 analysis. After incubation with ursolic acid for 16h, the water-soluble glucans and water-insoluble glucans were prepared from bacterial culture using the method described above. Phenol/H2SO4 analysis was used to quantify the two types of EPSs. Data are presented as the mean ± standard deviation; (B) Effect of ursolic acid on fructose production by GTFs determined via CE assay. Data are presented as the mean ± standard deviation. *p<0.05 and **p<0.01.
Discussion
Dental plaque is an oral bacterial biofilm that plays a key role in oral diseases (Adler et al., 2013). Biofilm formation enhances bacterial tolerance to drugs or the environment; thus, inhibition of biofilm formation and damage to the biofilm are the effective ways to prevent or cure oral diseases (Chenicheri et al., 2017). S. mutans is the principal bacterium that forms biofilms by adhesion to teeth and is considered the most important pathogen for dental caries. In the present study, we investigated the effects of ursolic acid on the growth and cariogenicity of biofilms formed by S. mutans. The results indicated that ursolic acid could effectively prevent biofilm formation of S. mutans through two pathways: (i) Ursolic acid decreased the viability of planktonic and sessile bacteria; and (ii) ursolic acid inhibited GTF-mediated synthesis of EPSs by competing with sucrose to occupy the catalytic center of the enzyme, which decreased bacterial adhesion and biofilm formation. Therefore, ursolic acid has great potential to be developed as an oral protective or therapeutic drug for oral disease.
Dental caries is a secondary bacterial disease that affects the health and quality of life of half of the world’s population (Hwang et al., 2014). Attention is needed to develop effective prevention and treatment methods. The results of this study indicated that ursolic acid inhibits planktonic S. mutans. Previously, it was reported that ursolic acid could have an inhibitory effect on other cariogenic pathogens, such as Streptococcus sobrius (do Nascimento et al., 2014). Therefore, ursolic acid may have significant antimicrobial activity against pathogenic bacteria involved in dental caries. Traditionally, fluoride, a common component of oral care products, is used to support oral hygiene and health. However, some studies have found that excess fluoride is unsafe for children and adults, as it may induce color nonuniformity of teeth and loss of potency (Wong et al., 2010). Chlorhexidine, a broad-spectrum antibiotic, is widely used to prevent or cure oral diseases (Charugundla et al., 2015). It was reported that S. mutans has some drug resistance against chlorhexidine (López-Jornet et al., 2012). Therefore, it is necessary to develop new effective components to decrease the drug resistance of oral pathogens. Interestingly, ursolic acid showed inhibitory activity on biofilms at 1/2 MIC, similar to chlorhexidine (Supplementary Figure S3). Moreover, ursolic acid has low toxicity and is versatile in terms of its biological activity, evidenced by its antiviral, liver protective, and whitening effects (Zou et al., 2019; Liu et al., 2021). Therefore, ursolic acid has great potential to be used as a lead compound in the development of an effective inhibitor for dental caries and as a protective agent for oral health.
Biofilm formation is a dynamic process that includes adhesion, aggregation, and maturation (Arciola et al., 2012). GTFs can synthesize EPSs by using sucrose as a substrate, which provides an adherent ability for bacterial colonization to promote biofilm formation and development (Hellmuth et al., 2008; Chen et al., 2013). Therefore, GTFs are key pathogenic factors in the induction of dental caries. It has been reported that subsite-1 and subsite+1 are the main partial catalytic centers of GTF-SI for sucrose binding and EPS formation (Ito et al., 2011). The primary bound sucrose is attacked by a proton to induce hydrolysis, and then, the glucosyl group binds to subsite-1 of GTF as an intermediate, while fructose is released from subsite+1 of the enzyme (Ito et al., 2011). Amino acid residues such as Arg475, Asp477, Glu515, Asp588, and Tyr916 construct subsite-1 of GTF for catalyzing glucan formation, while the amino acid residues located at subsite+1, such as Tyr430, Leu433, and Trp517, constitute the critical domains for recognition of the moiety (Ito et al., 2011). The molecular docking analysis showed that ursolic acid is sandwiched by Tyr430 and Asp909 to occupy the catalytic center and that the 3-hydroxyl group of ursolic acid points toward the active center. The experimental results confirmed that ursolic acid could bind with GTFs and that the binding ability disappeared as the action site was replaced by other amino acids. Quantitative analysis of EPSs and fructose showed that ursolic acid could decrease the levels of EPSs and fructose. These results confirm that ursolic acid competes with sucrose to occupy the catalytic center of GTFs, which may lead to the failure of GTFs to use sucrose as a substrate to synthesize EPSs (as shown in Figures 2, 4). In addition, the recognition of the glucosyl moiety of primary sucrose by GTFs and the intermediate formation at subsite-1 are well conserved in other Streptococcus species related to dental caries and infective endocarditis, such as Streptococcus gordonii (Hellmuth et al., 2008). Therefore, ursolic acid could be developed as an antimicrobial agent for dental caries or Streptococcus-related diseases.
Conclusion
We characterized the mechanism of S. mutans biofilm formation inhibition by ursolic acid. More importantly, ursolic acid can bind to GTFs instead of sucrose to interfere with microbial adhesion and aggregation through interactions with seven amino acids (Tyr430, Asp909, Leu433, Leu434, Phe907, Trp517, and Tyr916) of the enzyme, which is necessary for biofilm formation and even destroys mature biofilms, to prevent and cure dental caries and other oral diseases. Thus, ursolic acid has the potential to be developed as a drug or oral cleaning product to protect against and cure oral disease and other GTF-related diseases in the clinic. Finally, Leu433, Leu434, and Phe907 may serve as new target sites to screen inhibitors of GTFs to develop new antimicrobials.
Data Availability Statement
The data sets presented in this study can be found in online repositories. The names of the repository/repositories and accession number(s) can be found in the article/Supplementary Material.
Author Contributions
LY, BM, FC, and CZ performed the experiments. LY and SL designed the study, carried out the analysis and interpretation, and drafted and revised the manuscript. HY, YL, and ZL designed the study and analyzed the data. YC, SZ, YX, YJ, WS, LL, and WG assisted with the data analysis. All authors contributed to the article and approved the submitted version.
Funding
This study was supported by grants from the following foundations: the National Natural Science Foundation of China (no. 81700709), the Fundamental Research Funds for the Central Universities (nos. 135130006, 130029804, 111498001, and 131004006), the Research Foundation of Jilin Provincial Science and Technology Development (nos. 20200602023ZP, 20200901002SF, and 20200404124YY), the Foundation of Jilin Province Development and Reform Commission (no. 2020C015), the funds of the Education Department of Jilin Province (no. JJKH20201177KJ), and the Foundation of Human Resources and Social Security Department of Jilin Province (no. 2020009).
Conflict of Interest
The authors declare that the research was conducted in the absence of any commercial or financial relationships that could be construed as a potential conflict of interest.
Publisher’s Note
All claims expressed in this article are solely those of the authors and do not necessarily represent those of their affiliated organizations, or those of the publisher, the editors and the reviewers. Any product that may be evaluated in this article, or claim that may be made by its manufacturer, is not guaranteed or endorsed by the publisher.
Acknowledgments
This article is dedicated to my advisor Yuxin Li, who passed away last year, and commemorates his guidance in my life.
Supplementary Material
The Supplementary Material for this article can be found online at:
https://www.frontiersin.org/articles/10.3389/fmicb.2021.743305/full#supplementary-material
References
Adler, C. J., Dobney, K., Weyrich, L. S., Kaidonis, J., Walker, A. W., Haak, W., et al. (2013). Sequencing ancient calcified dental plaque shows changes in oral microbiota with dietary shifts of the Neolithic and industrial revolutions. Nat. Genet. 45, 450–455. doi: 10.1038/ng.2536
Arciola, C. R., Campoccia, D., Speziale, P., Montanaro, L., and Costerton, J. W. (2012). Biofilm formation in staphylococcus implant infections. a review of molecular mechanisms and implications for biofilm-resistant materials. Biomaterials 33, 5967–5982. doi: 10.1016/j.biomaterials.2012.05.031
Bacanlı, M., Başaran, A. A., and Başaran, N. (2017). The antioxidant, cytotoxic, and antigenotoxic effects of galangin, puerarin, and ursolic acid in mammalian cells. Drug Chem. Toxicol. 40, 256–262. doi: 10.1080/01480545.2016.1209680
Bowen, W. H., Burne, R. A., Wu, H., and Koo, H. (2018). Oral biofilms: pathogens, matrix, and Polymicrobial interactions in microenvironments. Trends. Microbiol. 26, 229–242. doi: 10.1016/j.tim.2017.09.008
Bowen, W. H., and Koo, H. (2011). Biology of Streptococcus mutans-derived glucosyltransferases: role in extracellular matrix formation of cariogenic biofilms. Caries Res. 5, 69–86. doi: 10.1159/000324598
Cardoso Sá, N., Cavalcante, T. T., Araújo, A. X., dos Santos, H. S., Albuquerque, M. R., Bandeira, P. N., et al. (2012). Antimicrobial and antibiofilm action of Casbane Diterpene from croton nepetaefolius against oral bacteria. Arch. Oral Biol. 57, 550–555. doi: 10.1016/j.archoralbio.2011.10.016
Charugundla, B. R., Anjum, S., and Mocherla, M. (2015). Comparative effect of fluoride, essential oil and chlorhexidine mouth rinses on dental plaque and gingivitis in patients with and without dental caries: a randomized controlled trial. Int. J. Dent. Hyg. 13, 104–109. doi: 10.1111/idh.12094
Chen, L., Ren, Z., Zhou, X., Zeng, J., Zou, J., and Li, Y. (2016). Inhibition of Streptococcus mutans biofilm formation, extracellular polysaccharide production, and virulence by an oxazole derivative. App. Microbial. cell. physiol. 100, 857–867. doi: 10.1007/s00253-015-7092-1
Chen, Y. P., Zhang, P., Guo, J. S., Fang, F., Gao, X., and Li, C. (2013). Functional groups characteristics of EPS in biofilm growing on different carriers. Chemosphere 92, 633–638. doi: 10.1016/j.chemosphere.2013.01.059
Chenicheri, S., R, U., Ramachandran, R., Thomas, V., and Wood, A. (2017). Insight into Oral biofilm: primary, secondary and residual caries and Phyto-challenged solutions. Open Dent. J. 11, 312–333. doi: 10.2174/1874210601711010312
Cummins, D. (2013). Dental caries: a disease which remains a public health concern in the 21st century–the exploration of a breakthrough technology for caries prevention. J. Clin. Pediatr. Dentisitry. 24, A1–A14.
do Nascimento, P. G., Lemos, T. L., Bizerra, A. M., Arriaga, Â. M., Ferreira, D. A., Santiago,, et al. (2014). Antibacterial and antioxidant activities of ursolic acid and derivatives. Molecules 19, 1317–1327. doi: 10.3390/molecules19011317
Duque, C., Aida, K. L., Pereira, J. A., Teixeira, G. S., Caldo-Teixeira, A. S., Perrone, L. R., et al. (2017). In vitro and in vivo evaluations of glass-ionomer cement containing chlorhexidine for atraumatic restorative treatment. J. Appl. Oral Sci. 25, 541–550. doi: 10.1590/1678-7757-2016-0195
Hellmuth, H., Wittrock, S., Kralj, S., Dijkhuizen, L., Hofer, B., and Seibel, J. (2008). Engineering the glucan-sucrase GTFR enzyme reaction and glycosidic bond specificity: toward tailor-made polymer and oligosaccharide products. Biochemistry 47, 6678–6684. doi: 10.1021/bi800563r
Hui, Y., Wen, S., Lihong, W., Chuang, W., and Chaoyun, W. (2021). Molecular structures of nonvolatile components in the Haihong fruit wine and their free radical scavenging effect. Food Chem. 15:129298. doi: 10.1016/j.foodchem.2021.129298
Hwang, G., Klein, M. I., and Koo, H. (2014). Analysis of the mechanical stability and surface detachment of mature Streptococcus mutans biofilms by applying a rage of external shear forces. Biofouling 30, 1079–1091. doi: 10.1080/08927014.2014.969249
Ikeda, Y., Murakami, A., and Ohigashi, H. (2008). Ursolic acid: an anti-and pro-inflammatory triterpenoid. Mol. Nutr. Food Res. 52, 26–42. doi: 10.1002/mnfr.200700389
Ito, K., Ito, S., Shimamura, T., Weyand, S., Kawarasaki, Y., Misaka, T., et al. (2011). Crystal structure of Glucansucrase from the dental caries pathogen Streptococcus mutans. J. Mol. Biol. 408, 177–186. doi: 10.1016/j.jmb.2011.02.028
Jakubovics, N. S., Goodman, S. D., Mashburn-Warren, L., Stafford, G. P., and Cieplik, F. (2021). The dental plaque biofilm matrix. Periodontol. 86, 32–56. doi: 10.1111/prd.12361
Jaña, G. A., Mendoza, F., Osorio, M. I., Alderete, J. B., Fernandes, P. A., Ramos, M. J., et al. (2018). A QM/MM approach on the structural and stereoelectronic factors governing glycosylation by GTF-SI from Streptococcus mutans. Org. Biomol. Chem. 16, 2438–2447. doi: 10.1039/C8OB00284C
Kim, S. G., Min, J. K., Jin, D., Park, S. N., and Kook, J. K. (2012). Antimicrobial effect of ursolic acid and oleanolic acid against methicillin-resistant Staphylococcus aureus. J. Microbiol. 48, 212–215. doi: 10.7845/kjm.2012.029
Kong, L., Li, S., Liao, Q., Zhang, Y., Sun, R., Zhu, X., et al. (2013). Oleanolic acid and ursolic acid: novel hepatitis C virus antivirals that inhibit NS5B activity. Antivir. Res. 98, 44–53. doi: 10.1016/j.antiviral.2013.02.003
Koo, H., Gomes, B. P., Rosalen, P. L., Ambrosano, G. M., Park, Y. K., and Cury, J. A. (2000). In vitro antimicrobial activity of propolis and Arnica Montana against oral pathogens. Arch. Oral Biol. 45, 141–148. doi: 10.1016/S0003-9969(99)00117-X
Liang, C., Li, Y., Bai, M., Huang, Y., Yang, H., Liu, L., et al. (2020). Hypericin attenuates nonalcoholic fatty liver disease and abnormal lipid metabolism via the PKA-mediated AMPK signaling pathway in vitro and in vivo. Pharmacol. Res. 153:104657. doi: 10.1016/j.phrs.2020.104657
Liu, H. R., Ahmad, N., Lv, B., and Li, C. (2021). Advances in production and structural derivatization of the promising molecule ursolic acid. Biotechnol. J. 6:e2000657. doi: 10.1002/biot.202000657
Liu, Y., Xu, Y., Song, Q., Wang, F., Sun, L., Liu, L., et al. (2017). Anti-biofilm activities from Bergenia crassifolia leaves against Streptococcus mutans. Front. Microbiol. 8:1738. doi: 10.3389/fmicb.2017.01738
López-Jornet, P., Plana-Ramon, E., Leston, J. S., and Pons-Fuster, A. (2012). Short-term side effects of 0.2% alcohol-free chlorhexidine mouthrinse in geriatric patients: a randomized, double-blind, placebo-controlled study. Gerodontology 29, 292–298. doi: 10.1111/j.1741-2358.2012.00671.x
Monchois, V., Willemot, R. M., and Monsan, P. (1999). Glucansucrases: mechanism of action and structure–function relationships. FEMS. Microbiol. Rev. 23, 131–151. doi: 10.1016/S0168-6445(98)00041-2
Packiavathy, I. A. S. V., Agilandeswari, P., Musthafa, K. S., Pandian, S. K., and Ravi, A. V. (2012). Antibiofilm and quorum sensing inhibitory potential of Cuminum cyminum and its secondary metabolite methyl eugenol against gram negative bacterial pathogens. Food Res. Int. 45, 141–148. doi: 10.1016/j.foodres.2011.10.022
Pádua, T. A., de Abreu, B. S., Costa, T. E., Nakamura, M. J., Valente, L. M., Henriques, M. d., et al. (2014). Anti-inflammatory effects of methyl ursolate obtained from a chemically derived crude extract of apple peels: potential use in rheumatoid arthritis. Arch. Pharm. Res. 37, 1487–1495. doi: 10.1007/s12272-014-0345-1
Palomino, J. C., Martin, A., Camacho, M., Guerra, H., Swings, J., and Portaels, F. (2002). Resazurin microtiter assay plate: simple and inexpensive method for detection of drug resistance in mycobacterium tuberculosis. Antimicrob. Agents Chemother. 46, 2720–2722. doi: 10.1128/AAC.46.8.2720-2722.2002
Patlolla, J. M., and Rao, C. V. (2012). Triterpenoids for cancer prevention and treatment: current status and future prospects. Curr. Pharm. Biotechnol. 13, 147–155. doi: 10.2174/138920112798868719
Pitts, N. B., Zero, D. T., Marsh, P. D., Ekstrand, K., Weintraub, J. A., Ramos-Gomez, F., et al. (2017). Dental caries. Nature Review Disease Primers 3:17030. doi: 10.1038/nrdp.2017.30
Rizelio, V. M., Tenfen, L., da Silveira, R., Gonzaga, L. V., Costa, A. C., and Fett, R. (2012). Development of a fast capillary electrophoresis method for determination of carbohydrates in honey samples. Talanta 93, 62–66. doi: 10.1016/j.talanta.2012.01.034
Sarker, S. D., Nahar, L., and Kumarasamy, Y. (2007). Microtitre plate-based antibacterial assay incorporating resazurin as an indicator of cell growth, and its application in the in vitro antibacterial screening of phytochemicals. Methods 42, 321–324. doi: 10.1016/j.ymeth.2007.01.006
Shun, C. T., Lu, S. Y., Yeh, C. Y., Chiang, C. P., Chia, J. S., and Chen, J. Y. (2005). Glucosyltransferases of viridans streptococci are modulins of interleukin-6 induction in infective endocarditis. Infect. Immunnity. 73, 3261–3270. doi: 10.1128/IAI.73.6.3261-3270.2005
Süntar, I., Oyardı, O., Akkol, E. K., and Ozçelik, B. (2016). Antimicrobial effect of the extracts from Hypericum perforatum against oral bacteria and biofilm formation. Pharm. Biol. 54, 1065–1070. doi: 10.3109/13880209.2015.1102948
Takahashi, N., and Nyvad, B. (2011). The role of bacteria in the caries process: ecological perspectives. J. Dent. Res. 90, 294–303. doi: 10.1177/0022034510379602
Tamesada, M., Kawabata, S., Fujiwara, T., and Hamada, S. (2004). Synergistic effects of streptococcal glucosyl-transferases on adhesive biofilm formation. J. Dent. Res. 83, 874–879. doi: 10.1177/154405910408301110
Taubman, M. A., and Nash, D. A. (2006). The scientific and public-health imperative for a vaccine against dental caries. Nat. Rev. Immunol. 6, 555–563. doi: 10.1038/nri1857
Vernazza, C. R., Birch, S., and Pitts, N. B. (2021). Reorienting Oral health services to prevention: economic perspectives. J. Dent. Res. 100, 576–582. doi: 10.1177/0022034520986794
Wang, C., van der Mei, H. C., Busscher, H. J., and Ren, Y. (2020). Streptococcus mutans adhesion force sensing in multi-species oral biofilms. npj. Biofilms. Microbiomes. 6, 1–9. doi: 10.1038/s41522-020-0135-0
Wong, M. C., Glenny, A. M., Tsang, B. W., Lo, E. C., Worthington, H. V., and Marinho, V. C. (2010). Topical fluoride as a cause of dental fluorosis in children. Cochrane Database Syst. Rev. 1:CD007693. doi: 10.1038/s41522-020-0135-0
Wong, A., Subar, P. E., and Young, D. A. (2017). Dental caries: an update on dental trends and therapy. Adv. Pediatics. 64, 307–330. doi: 10.1016/j.yapd.2017.03.011
Xu, H., Zhang, M., Li, X. L., Li, H., Yue, L. T., Zhang, X. X., et al. (2019). Low and high doses of ursolic acid ameliorate experimental autoimmune myasthenia gravis through different pathways. J. Neuroimmunol. 330, 181–183. doi: 10.1016/j.jneuroim.2019.03.016
Yano, A., Kikuchi, S., Takahashi, T., Kohama, K., and Yoshida, Y. (2012). Inhibitory effects of the phenolic fraction from the pomace of Vitis coignetiae on biofilm formation by Streptococcus mutans. Arch. Oral Biol. 57, 711–719. doi: 10.1016/j.archoralbio.2012.01.001
Keywords: ursolic acid, biofilms, extracellular polysaccharides, glucosyltransferases, mechanism
Citation: Liu Y, Huang Y, Fan C, Chi Z, Bai M, Sun L, Yang L, Yu C, Song Z, Yang X, Yi J, Wang S, Liu L, Wang G and Zheng L (2021) Ursolic Acid Targets Glucosyltransferase and Inhibits Its Activity to Prevent Streptococcus mutans Biofilm Formation. Front. Microbiol. 12:743305. doi: 10.3389/fmicb.2021.743305
Edited by:
Prescilla Emy Nagao, Rio de Janeiro State University, BrazilReviewed by:
Geelsu Hwang, University of Pennsylvania, United StatesLatifa Bousarghin, University of Rennes 1, France
Copyright © 2021 Liu, Huang, Fan, Chi, Bai, Sun, Yang, Yu, Song, Yang, Yi, Wang, Liu, Wang and Zheng. This is an open-access article distributed under the terms of the Creative Commons Attribution License (CC BY). The use, distribution or reproduction in other forums is permitted, provided the original author(s) and the copyright owner(s) are credited and that the original publication in this journal is cited, in accordance with accepted academic practice. No use, distribution or reproduction is permitted which does not comply with these terms.
*Correspondence: Luguo Sun, sunluguo01@163.com; Lihua Zheng, zhenglh015@nenu.edu.cn
†These authors have contributed equally to this work