- 1Instituto de Ciências Biomédicas Abel Salazar, Universidade do Porto, Porto, Portugal
- 2Nephrology & Infectious Diseases R&D Group, INEB – Instituto de Engenharia Biomédica, i3S – Instituto de Investigação e Inovação em Saúde, Universidade do Porto, Porto, Portugal
- 3Nephrology Department, Hospital Universitari Doctor Josep Trueta, Girona, Spain
- 4Universidade Católica Portuguesa, CBQF - Centro de Biotecnologia e Química Fina – Laboratório Associado, Escola Superior de Biotecnologia, Porto, Portugal
- 5Instituto Português de Oncologia do Porto Francisco Gentil (IPO), Porto, Portugal
- 6Nephrology Department, Centro Hospitalar Universitário de São João, Porto, Portugal
- 7Faculdade de Medicina Dentária, Universidade do Porto, Porto, Portugal
- 8Laboratório para a Investigação Integrativa e Translacional em Saúde Populacional (ITR), Porto, Portugal
Chronic Kidney Disease (CKD) is a growing public-health concern worldwide. Patients exhibit compromised immunity and are more prone to infection than other populations. Therefore, oral colonization by clinically relevant members of the Enterobacteriaceae family, major agents of both nosocomial and dialysis-associated infections with frequent prevalence of antibiotic resistances, may constitute a serious risk. Thus, this study aimed to assess the occurrence of clinically relevant enterobacteria and their antibiotic resistance profiles in the oral cavity of CKD patients undergoing peritoneal dialysis (CKD-PD) and compare it to healthy controls. Saliva samples from all the participants were cultured on MacConkey Agar and evaluated regarding the levels of urea, ammonia, and pH. Bacterial isolates were identified and characterized for antibiotic resistance phenotype and genotype. The results showed that CKD-PD patients exhibited significantly higher salivary pH, urea, and ammonia levels than controls, that was accompanied by higher prevalence and diversity of oral enterobacteria. Out of all the species isolated, only the prevalence of Raoultella ornithinolytica varied significantly between groups, colonizing the oral cavity of approximately 30% of CKD-PD patients while absent from controls. Antibiotic resistance phenotyping revealed mostly putative intrinsic resistance phenotypes (to amoxicillin, ticarcillin, and cephalothin), and resistance to sulfamethoxazole (~43% of isolates) and streptomycin (~17%). However, all isolates were resistant to at least one of the antibiotics tested and multidrug resistance isolates were only found in CKD-PD group (31,6%). Mobile genetic elements and resistance genes were detected in isolates of the species Raoultella ornithinolytica, Klebsiella pneumoniae, Klebsiella oxytoca, Escherichia coli, and Enterobacter asburiae, mostly originated from CKD-PD patients. PD-related infection history revealed that Enterobacteriaceae were responsible for ~8% of peritonitis and ~ 16% of exit-site infections episodes in CKD-PD patients, although no association was found to oral enterobacteria colonization at the time of sampling. The results suggest that the CKD-induced alterations of the oral milieu might promote a dysbiosis of the commensal oral microbiome, namely the proliferation of clinically relevant Enterobacteriaceae potentially harboring acquired antibiotic resistance genes. This study highlights the importance of the oral cavity as a reservoir for pathobionts and antibiotic resistances in CKD patients undergoing peritoneal dialysis.
Introduction
Chronic kidney disease (CKD) is an increasing global health issue, defined as decreased kidney function shown by a glomerular filtration rate of less than 60 ml/min/1.73 m2, or markers of kidney damage, or both, for a duration of at least 3 months (Webster et al., 2017; Benjamin and Lappin, 2021). CKD is commonly progressive and when patients reach end-stage renal disease, they may require renal replacement therapies, such as hemodialysis, peritoneal dialysis (PD) or kidney transplantation (Murdeshwar and Anjum, 2021). CKD patients exhibit increased mortality and morbidity, especially associated with cardiovascular events (Webster et al., 2017; Benjamin and Lappin, 2021).
The decrease in kidney function leads to the accumulation of substances in the body that in normal conditions would be eliminated in the urine (Lisowska-Myjak, 2014). These substances, known as uremic toxins, build up inside the organism and cause alterations in various body sites, including the oral cavity, with higher levels of urea, ammonia and pH being commonly detected in the saliva of CKD patients (Gupta et al., 2015; Simões-Silva et al., 2017). These changes are thought to be major selective factors responsible for altering the oral microbiome, which has shown significant association with CKD status (Simões-Silva et al., 2017, 2018; Hu et al., 2018).
On the other hand, it has been hypothesized that the oral microbiome may contribute in some extent to the progression of CKD. Three mechanisms have been proposed that link oral infection, especially periodontitis, to secondary systemic effects (Li et al., 2000; Simões-Silva et al., 2018). These include the generalized spread of infection from the oral cavity as a result of transient bacteremia; generalized injury resulting from circulating toxins of oral microbial origin; and systemic inflammation as a result of immunological activation induced by oral microorganisms (Li et al., 2000; Simões-Silva et al., 2018).
Among other complications, the accumulation of uremic toxins in the body leads to a chronic inflammatory status which can cause the suppression of lymphocytes and cell-mediated immunity, making CKD patients more prone to infections than other populations (Gupta et al., 2015). In fact, infectious episodes persist as the main weakness of PD programs, with peritonitis and exit-site/tunnel infections remaining as the most common and relevant concerns for these patients (Mujais, 2006; Liakopoulos et al., 2017). While Gram-positive agents (mainly Staphylococcus spp. and Streptococcus spp.) are responsible for the majority of infections, Gram-negative bacteria (mainly Pseudomonas spp. and members of the family Enterobacteriaceae) are more likely to provoke more severe infections with poor outcomes (Li and Chow, 2011; Liakopoulos et al., 2017).
The Enterobacteriaceae family is of particular relevance in end-stage renal disease patients. Aside from being recognized agents of nosocomial infections, Enterobacteriaceae are responsible for 10 to 12% of all peritoneal dialysis-associated peritonitis, constituting a significant risk for patients on PD programs (Jain and Blake, 2006; Szeto et al., 2006). Regarding the oral microbiome, enterobacteria are frequent inhabitants of periodontal pockets (Gonçalves et al., 2007), potentially contributing to generalized inflammation and disease progression in these patients. Furthermore, Enterobacteriaceae are critical level pathogens regarding antibiotic resistance phenotypes. The mechanisms of antibiotic resistance in these bacteria may be intrinsic due to the presence of features encoded by chromosomal genes related with functions such as antibiotic inactivating enzymes and non-enzymatic paths (efflux pumps, permeability or target modifications) that are observed in all or most of the members of a given bacterial species (Ruppé et al., 2015). In contrast, acquired antibiotic resistance is due to gene mutation or, more frequently, to incorporation of genes received through mobile genetic elements, such as plasmids or phages that often harbor genes encoding ß-lactamases, aminoglycosides modifying enzymes, or non-enzymatic mechanisms (Cox and Wright, 2013).
Antimicrobial resistant enterobacteria are quite prevalent in CKD patients and are the cause of serious drug-resistant infectious episodes (Wang et al., 2019). Probably because of frequent hospitalizations and frequent need for antibiotic therapy, patients with CKD tend to have more infections by drug resistant bacteria than people with normal kidney function (Fysaraki et al., 2013; Salloum et al., 2020). In 2014, outpatient hemodialysis facilities in the United States reported that, out of the isolates identified as agents of bloodstream infections and exit-site-related infections, 17.8% of Escherichia coli and 14.6% of Klebsiella spp. isolates were resistant to cephalosporins and 4.8% of Enterobacter spp. isolates were resistant to carbapenems (Nguyen et al., 2017). In another study conducted in a hemodialysis unit in a hospital in Algeria, 100% of K. pneumoniae isolates associated with catheter-related infections produced extended spectrum beta-lactamase and were resistant to at least gentamicin (Sahli et al., 2017). Regarding peritoneal dialysis, in PD patients who developed dialysis-associated peritonitis between 2002 and 2011, 54.3% of Enterobacteriaceae isolates were resistant to third-generation cephalosporins and 76.1% were resistant to fluoroquinolones (ciprofloxacin), and all of them produced extended spectrum beta-lactamase (Prasad et al., 2014). This data shows that a large percentage of enterobacteria responsible for dialysis-related infections in CKD patients also exhibit resistance to one or more antibiotic drugs.
Taking into consideration the above mentioned, this work aimed to assess the prevalence and antibiotic resistance profile of clinically relevant enterobacteria in the oral cavity of CKD patients undergoing PD.
Materials and Methods
Participant Recruitment
Chronic kidney disease patients undergoing peritoneal dialysis (CKD-PD, n = 44) followed at the outpatient clinic of the Nephrology Department of Centro Hospitalar Universitário de São João were invited to participate in this study. Exclusion criteria for this group included inability to give informed consent, pregnancy, recent history of infection (less than 3 months), recent history of antibiotic therapy (less than 3 months), and severe acute illness. Samples were collected according to patient attendance to the clinic over a period of 4 months. Relevant clinical and demographic information was gathered for each individual through a semi-structured interview and by reviewing the patient’s computerized clinical reports. The occurrence of PD-related infectious episodes associated with species from the Enterobacteriaceae family was retrieved from clinical records from the date of entrance in the PD program until the moment of oral sample collection.
The control group was recruited from the student body of the Faculdade de Medicina Dentária da Universidade do Porto (n = 37). Relevant clinical and demographic information was gathered through a semi-structured interview. Exclusion criteria for the control group included inability to give informed consent, pregnancy, recent history of infection (less than 3 months), recent history of antibiotic therapy (less than 3 months), and compromised oral health (occurrence of gum disease, such as gingivitis or periodontitis, and a caries index including decayed, missing or filled teeth superior to 5). Controls had no history of chronic or systemic diseases (such as diabetes, hypertension, obesity, allergies, autoimmune diseases, etc.), no history of genetic disorders, no history of renal disease, and had not been diagnosed for any other health condition.
The protocols for the study were approved by both the Ethics Committee for Health of Centro Hospitalar Universitário de São João (approval number 200/18) and the Ethics Committee for Health of Faculdade de Medicina Dentária da Universidade do Porto (approval number 12/2019) and followed the 1964 Helsinki declaration and its later amendments; all recruited participants gave their written informed consent.
Sample Collection
Unstimulated whole saliva was collected via the spitting method (Navazesh, 1993) into sterile containers, at least 1 h after eating or tooth brushing. Prior to the collection, the subjects did a water mouthwash to minimize typical dry-mouth sensation and to clean the oral cavity. Saliva collection began after swallowing the residual saliva present in the mouth and allowing newly produced saliva to accumulate. Part of the saliva samples (~1 ml) were stored at −20°C until biochemical analysis and the remaining volume was kept refrigerated for microbiological evaluation.
Saliva Biochemical Analysis
The biochemical analysis was carried out using a sequential injection analysis system with potentiometric detection for the determination of urea and ammonia in saliva samples as previously described (Thepchuay et al., 2020), and the pH was measured using pH strips (5.0–8.0, Duotest, Germany and 1–14, Merck, Germany).
Oral Microbiota Isolation and Identification
Up to 2 h after collection, refrigerated saliva samples were serially diluted with sterile 0.9% NaCl solution and were plated in triplicate in MacConkey Agar (VWR Chemicals BDH, Belgium). This culture medium was used in order to target non-fastidious non-strict anaerobic Gram-negative species. After aerobic incubation at 37°C for 48 h, the total number of colonies was counted and the results were expressed in colony-forming units per mL of saliva (CFU/ml).
From each saliva sample, all colonies with distinct appearances were reisolated in the same medium, which resulted in the selection of 2 to 3 isolates per individual saliva sample. Additionally, urease production was tested by incubating colonies on Urea Broth (Panreac, Spain) at 37°C for 24 h. Isolates were then stored in Brain Heart Infusion broth (BHI; Biolab Zrt., Hungary) supplemented with 10% glycerol at −80°C and were later identified by matrix-assisted laser desorption/ionization–time-of-flight mass spectrometry (MALDI-TOF MS, Bruker MALDI Biotyper).
The MALDI-TOF MS analysis was conducted directly from fresh (<24 h) bacterial colonies according to manufacturer’s instructions. When necessary, formic acid 70% was added to samples to improve identification. Only isolates which obtained an identification score value equal or higher than 2.0, corresponding to probable identification to species level, were considered properly identified.
Antibiotic Resistance Phenotype and Genotype
Antibiotic resistance phenotype was determined using the Kirby–Bauer disk-diffusion method to test the following antibiotics: amoxicillin (AML, 25 μg), gentamycin (GEN, 10 μg), ciprofloxacin (CIP, 5 μg), sulfamethoxazole/trimethoprim (SXT, 1.25/23.75 μg), tetracycline (TET, 30 μg), cephalothin (CP, 30 μg), meropenem (MEM, 10 μg), ceftazidime (CAZ, 30 μg), ticarcillin (TIC, 75 μg), colistin (CT, 50 μg), sulfamethoxazole (SUL, 25 μg) and streptomycin (STR, 10 μg; Oxoid™, Hampshire, UK). Assays were conducted as recommended for Enterobacteriaceae (CLSI, 2017). As a quality control, Escherichia coli strain ATCC 25922 was included in all assays.
PCR-based gene screening (primers and PCR conditions described in Supplementary Table S1) used DNA extracts obtained from fresh pure cultures: bacterial colonies were resuspended in 50 μl of nuclease-free water and heated for 10 min at 95°C; subsequently, the mixture was refrigerated on ice for 5 min and centrifuged at 14 000 rpm for 5 min for sedimentation of cell debris; the supernatant was used as template for PCR reactions (Wiedmann-al-Ahmad et al., 1994). PCR gel electrophoresis pictures are presented in Supplementary Figures S1–S9. The screened genetic determinants included resistance determinants to β-lactams (blaTEM, blaCTX-M, blaOXA and blaSHV), sulfonamides (sul1) and quinolones (qnrS), and mobile genetic elements related with genetic recombination and gene acquisition, plasmid replicon types (incF, incHI1 and incHI2), representative of incompatibility groups circulating among Enterobacteriaceae, and class 1 integron integrase (intI1).
Data Analysis
All data analysis was carried out using IBM SPSS Statistics for Windows, Version 23.0 (IBM Corp, NY, United States). When appropriate, the chi-square independence test was used to analyze hypotheses regarding the categorical variables, using continuity correction for 2×2 Tables. T-test for independent samples was used for the continuous variables, using Levene’s test for equal variances. In order to evaluate potential confounders like age and sex, binary logistic regression (Enter method) was applied for the presence of Raoultella ornithinolytica as dependent variable and the type of participant as another covariate with bootstrapping technique with 95% of confidence. A significance level of 0.05 was considered.
Results
Clinical Characterization of CKD-PD Patients
Clinical information relating to the CKD-PD patients is included in Table 1. The most prevalent etiology of CKD was diabetic nephropathy and, at the time of sampling, the average time on PD therapy was 32 months, ranging from 2 months up to 12.4 years. Regarding salivary biochemical parameters, pH, urea, and ammonia levels were all significantly higher in CKD-PD patients compared to healthy controls (Table 2).
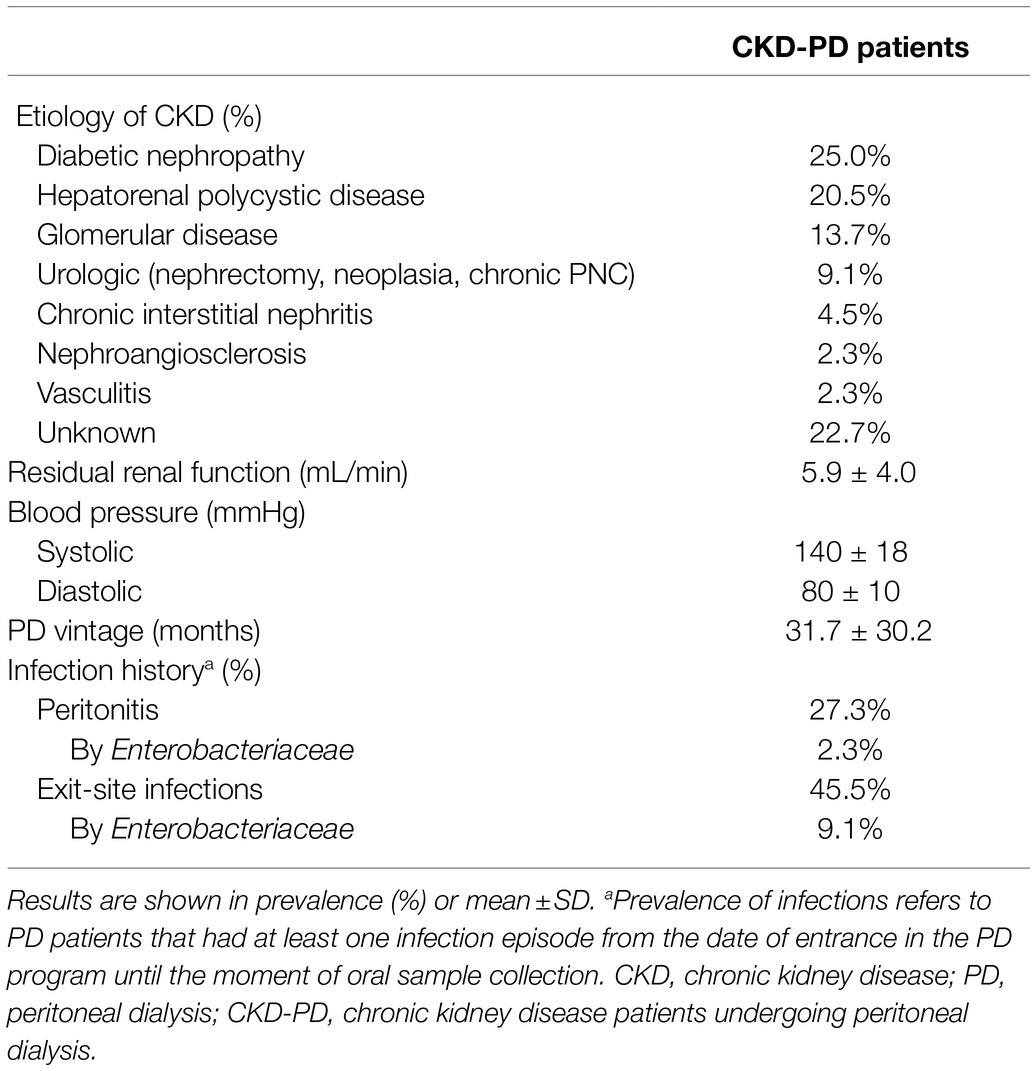
Table 1. CKD-PD patients’ clinical information, including etiology of CKD, residual renal function, blood pressure, time on PD, and PD-related infection history.
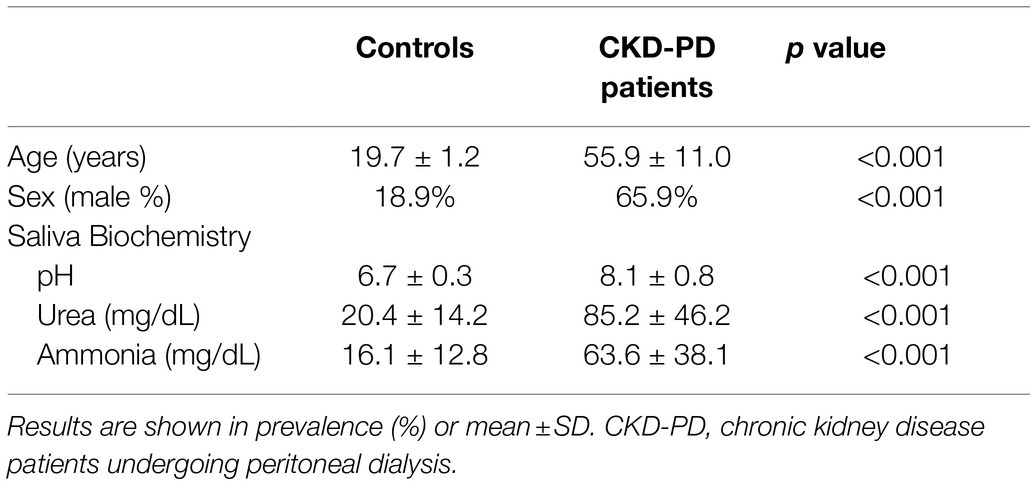
Table 2. Demographic information and biochemical salivary parameters of healthy controls and CKD-PD patients.
Oral Enterobacteriaceae Prevalence
CKD-PD patients exhibited significantly higher microbial counts and Enterobacteriaceae prevalence (Table 3). Enterobacteriaceae diversity was also higher in CKD-PD patients, with only three species being isolated from healthy controls while seven species (two in common with the controls and an additional five) were isolated from the study group, from a total of eight isolated species (Table 3). Six of the total eight species tested positive for urease production (excluding Escherichia coli and Klebsiella aerogenes; Table 3). Out of all Enterobacteriaceae species identified, only Raoultella ornithinolytica exhibited significantly higher prevalence in CKD-PD patients, being absent from the oral cavity of healthy controls.
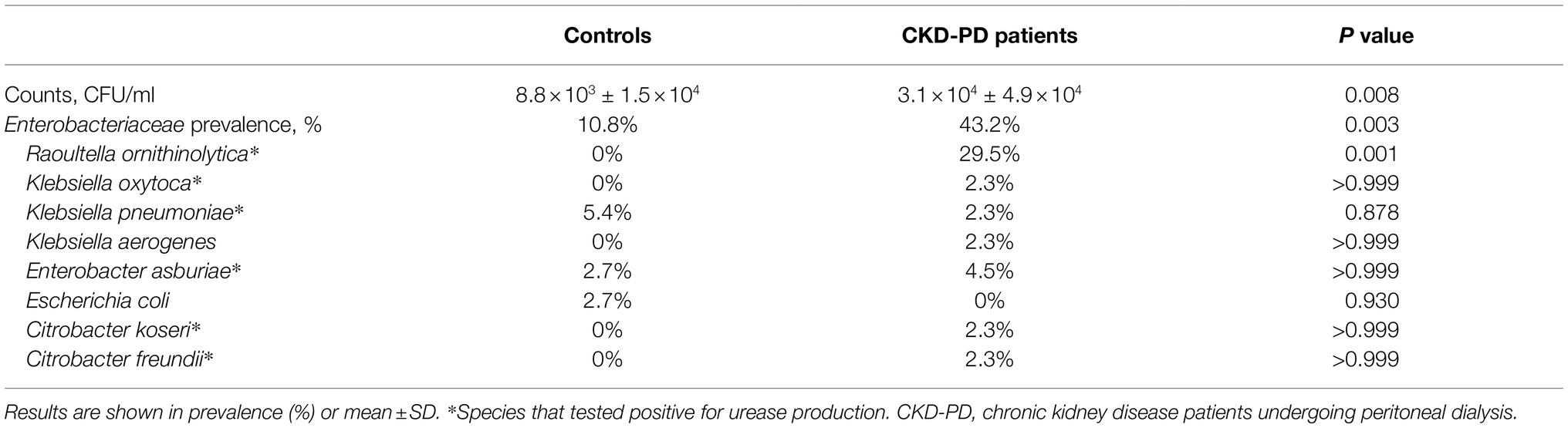
Table 3. Bacterial counts in MacConkey Agar and prevalence of participants colonized by urease producing (*) and non-producing Enterobacteriaceae species.
Because controls and CKD patients vary significantly in terms of age and gender, binary logistic regression was applied for the presence of Raoultella ornithinolytica as dependent variable containing three independent variables: sex, age and type of participants. The full model with bootstrapping technique containing all predictors was statically significant (χ2 (3, N = 81) = 18.041, p < 0.001). According to the bootstrapping technique, only the type of participants (controls or CKD) made a unique statistically significant contribution (p < 0.001) in the model (CKD patients’ coefficient, B = 20.299; CI95% = 17.675; 22.954). On the other hand, statistical significance was not attained for sex nor age, respectively (p = 0.760) and (p = 0.956).
Enterobacteriaceae-Related Infection History
During the complete period of PD therapy, around one quarter of the studied population had at least one peritonitis and around half of the patients had at least one ESI (Table 1). When one looks to the total number of infectious episodes (Table 4), Enterobacteriaceae were responsible for 7.7% of all peritonitis and for 16.2% of all ESI episodes, and the identified infectious agents include the genera Klebsiella, Enterobacter and Escherichia. No association was found between previous Enterobacteriaceae infectious agents and the oral enterobacteria colonization of the CKD-PD patients at the time of sampling.
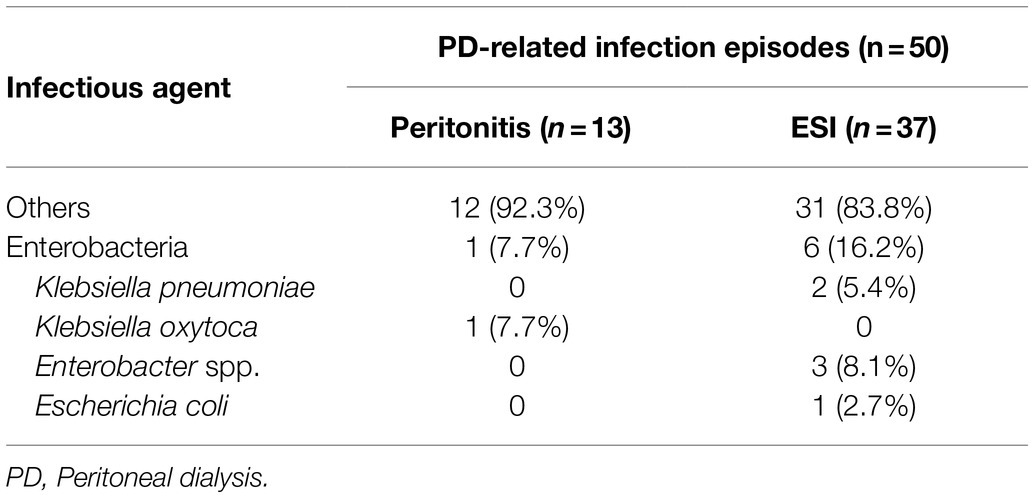
Table 4. Total number of PD-related infection episodes, including peritonitis and exit-site infections (ESI), and the infectious agent identified.
Antibiotic Resistance Profiling
Enterobacteriaceae isolates (n = 23) obtained from different individuals exhibited mainly intrinsic antibiotic resistance phenotypes (CLSI, 2017; EUCAST, 2020; Supplementary Table S2). Specifically, resistance to amoxicillin (in all isolates of Klebsiella spp., one isolate of Citrobacter freundii, 2 out of 3 isolates of Enterobacter spp., and 12 out of 14 isolates from R. ornithinolytica), resistance to ticarcillin (2 out of 3 isolates of Klebsiella spp. and in 10 out of 14 isolates from R. ornithinolytica), and resistance to cephalothin (in all isolates of Enterobacter spp.) were observed. In addition to intrinsic antibiotic resistance, sulfamethoxazole (in Enterobacter asburiae, Escherichia coli, Klebsiella pneumoniae, and Raoultella ornithinolytica isolates), and streptomycin (in Enterobacter asburiae and Raoultella ornithinolytica isolates) resistances were also observed. Only 6 isolates (2 isolates of Enterobacter asburiae and 4 of Raoultella ornithinolytica) exhibited multidrug resistance, all of them originated from CKD-PD patients. These results are depicted in Supplementary Table S2. Overall, out of the 23 Enterobacteriaceae isolates, about 83% were resistant to amoxicillin, 52% showed resistance to ticarcillin, 43% to sulfamethoxazole, 35% to ciprofloxacin and 17% to streptomycin. 100% of the isolates were resistant to at least one of the antibiotics tested. The percentage of sensitivity to the studied antibiotics is presented in Supplementary Figure S10.
Among the genes screened, neither the antibiotic resistance genes blaTEM, blaOXA and qnrS, nor the plasmid replicon types incHI1 and incHI2 were detected. The other genetic determinants were observed in one Escherichia coli isolate from a healthy control (sul1); in 2 of the 3 Enterobacter asburiae isolates from CKD-PD patients and controls (sul1, n = 1 and sul1 and intI1, n = 1); in the Klebsiella oxytoca isolate from a CKD-PD patient (blaCTX-M, normally associated with the plasmid replicon type incF); in 2 isolates of Klebsiella pneumoniae from controls (blaSHV, n = 2, probably intrinsic in this species, and sul1, n = 1); and in 4 of the 14 Raoultella ornithinolytica isolates from CKD-PD patients (sul1 and intI1, n = 3 and intI1, n = 1). These results are depicted in Supplementary Table S2. Overall, the blaSHV gene alone or with sul1 and blaCTX-M with replicon type incF were detected in 4.3% of isolates. The sul1 gene alone was found in 8.7% of isolates and sul1 with intI1 were found in 17.4% of isolates. None of the tested genes were detected in approximately 57% of isolates. The prevalence of these genetic elements is presented in Supplementary Figure S11.
Discussion
Overall, our results show that the accumulation of uremic toxins in patients with advanced CKD and the consequent changes in the oral milieu (such as the increase in salivary urea, ammonia and pH) may indeed act as selecting factors on the oral microbiome, leading to an oral dysbiosis with proliferation of pathobionts in the oral cavity. In fact, 6 of the 8 species identified in Table 3 tested positive for urease production. Thus, it was observed that more urease-producing species were found in the oral cavity of CKD patients in comparison to the oral cavity of healthy controls, which is expected to be due to the previously reported adaptation of the microbiome to the altered conditions of the oral milieu, evidenced in Table 2 (Hu et al., 2018; Rysz et al., 2021).
The increased prevalence of Enterobacteriaceae spp. is significantly relevant in CKD patients. While enterobacteria are only occasionally detected in the oral cavity of healthy individuals, colonization is much more prevalent in immunocompromised patients, people who come in contact with hospital environments frequently, and people suffering from hyposalivation, oral lesions, and systemic disease, a scenario that fits CKD patients (Peleg and Hooper, 2010; Posse et al., 2017). Perhaps, all these reasons also contributed to the higher prevalence of Enterobacteriaceae in the oral microbiota of our CKD patients as well as to the fact that Enterobacteriaceae were responsible for approximately 8% of the peritoneal dialysis-associated peritonitis and 16% of exit-site infections in our group of patients, highlighting the clinical relevance of our results.
The increased prevalence of Raoultella ornithinolytica in CKD patients undergoing PD is a particularly significant finding. Formerly classified as Klebsiella ornithinolytica, R. ornithinolytica, a histamine-producing aquatic-commensal enterobacteria, is a virulent pathogen of community-acquired and emerging nosocomial infections, particularly associated with invasive procedures (Seng et al., 2016; Hajjar et al., 2018, 2020). R. ornithinolytica is able to occasionally survive in human saliva and a case report has pointed it as a cause of primary peritonitis in humans (Sibanda, 2014; Seng et al., 2016), thus aggravating the risk of its presence in the oral cavity of patients undergoing peritoneal dialysis. Regarding the oral microbiome, the high rate of colonization by Raoultella ornithinolytica is a very interesting finding in itself, since this species is still not described in the Human Oral Microbiome Database.1
Although no association was found between previous Enterobacteriaceae infectious agents in the group of CKD patients and oral colonization by enterobacteria at the time of sampling, the difficulty in the identification of R. ornithinolytica and common misidentification as belonging to the Klebsiella genus should be considered, as a misidentification of the infectious agent is a possibility (Ponce-Alonso et al., 2016). Additionally, the hypothesis of an oral-peritoneal association regarding infection cannot be discarded without further studies, especially when all the Enterobacteriaceae-related infectious episodes reported in these patients occurred at least 1 year prior to the collection of the samples used in this study.
It is important to note that all the species identified in this study are clinically relevant and constitute potential cause of infection, with many being associated with PD-related infections (Barraclough et al., 2009; Li and Chow, 2011). Although contamination concerns for patients undergoing peritoneal dialysis typically tend to focus on contamination from external origins, the oral microbiome may be a major source of contamination both through external and internal routes (Li and Chow, 2011; Smith and Nehring, 2021). Bacteria inhabiting the oral cavity can translocate into the bloodstream as a result from routine daily activities, such as tooth brushing or invasive dental procedures, becoming opportunistic infectious agents in distant body sites such as the peritoneum (Smith and Nehring, 2021). In fact, the risk associated with the presence of pathogenic bacteria in the oral-nasal cavities of CKD patients is already recognized, which is why these patients are frequently screened for nasal carriage of Staphylococcus aureus as a way to prevent infection and the efficacy of prophylactic intranasal antibiotics for the treatment of confirmed nasal carriage of S. aureus has been tested in several prospective studies (Grothe et al., 2014; Li et al., 2016). Furthermore, the presence of these bacteria in the oral cavity can stimulate the immune system and contribute to the inflammatory state in CKD, which then leads to cardiovascular events, the main cause of death in these patients (Li et al., 2000; Webster et al., 2017; Höfer et al., 2021).
The oral cavity has also recently been highlighted as a potential reservoir of antibiotic resistance genes (Roberts and Mullany, 2010; Jiang et al., 2018; de Sousa Moreira Almeida et al., 2020). Resistance against amoxicillin was observed in 83% of isolates, although in these cases it is possibly intrinsic (Cox and Wright, 2013). However, putative acquired genetic elements related with antibiotic resistance were observed in 10 out of 23 oral isolates, 3 from healthy individuals (sul1 in Escherichia coli and blaSHV in Klebsiella pneumoniae) and 7 from CKD patients (sul1, intI1, blaCTX-M and incF in Enterobacter asburiae, Raoultella ornithinolytica and Klebsiella oxytoca). Curiously, the 5 isolates harboring the gene intI1 and the one exhibiting the high mobile replicon type incF and blaCTX-M genes all originated from CKD patients. These observations raise the interest to investigate the vulnerability of some population groups to be colonized by bacteria harboring acquired antibiotic resistance genes and mobile genetic elements (de Sousa Moreira Almeida et al., 2020). Overall, regarding the resistance phenotype, 100% of the Enterobacteriaceae isolates were resistant to at least one of the antibiotics tested and only 6 isolates, all originating from CKD-PD patients, exhibited multidrug resistance. Genetic elements related to resistance were also detected in approximately 43% of isolates. Antibiotic-resistant enterobacteria are known agents of dialysis-associated infections in CKD patients, frequently originating serious life-threatening complications (Wang et al., 2019). Therefore, it is important to keep screening isolates from CKD patients for antimicrobial resistances, in order to plan efficient therapeutic interventions in case of infection.
Our study presents several strengths and some limitations. The fact that the control and CKD groups differ in age and gender may constitute a limitation of our study, although a binary logistic regression was applied using the prevalence of Raoultella ornithinolytica (the only member of the Enterobacteriaceae family with a significantly different prevalence between groups) as the dependent variable and sex, age, and type of participants as independent variables, confirming that only the type of participants (controls or CKD-PD) made a unique statistically significant contribution. Moreover, though ideally the groups should display similar demographic characteristics, several studies have reported that the oral microbiome does not suffer significant alterations throughout adulthood, with no significant differences being reported by gender or age groups (adults and young adults) at species level. Alterations in the healthy oral microbiota are only significant before adulthood or after the age of 65–70 years-old (Crielaard et al., 2011; Belstrøm et al., 2014; Lira-Junior et al., 2018; Verma et al., 2021). As so, the healthy young adults constituting the control group in this study are nevertheless representative of healthy reference values. Regarding methodology, although MALDI-TOF MS is a universally used method that has been routinely used in clinical microbiology (He et al., 2010; Tsuchida et al., 2020) and is considered extremely adequate for the identification of enterobacteria (Richter et al., 2013), it presents some limitations, as is the example of the inability to differentiate Escherichia coli from Shigella species (Devanga Ragupathi et al., 2017).
When one looks to the study strengths, firstly, it highlights how the uremic state in CKD can cause a dysbiosis of the oral microbiome and lead to a proliferation of enterobacteria, which are clinically relevant pathogens for patients on PD programs. Additionally, the identification of Raoultella ornithinolytica as a colonizer of the oral cavity is of extreme importance. The isolation of R. ornithinolytica from saliva samples has been reported before in the literature (Heggendorn et al., 2013; Derafshi et al., 2017), but never in such a high prevalence as in this study and never as an oral colonizer of CKD patients. It is important to keep in mind that this is an emerging species both in terms of infectious episodes in humans and in terms of resistance to antibiotic agents (Seng et al., 2016; Hajjar et al., 2020), two factors that make R. ornithinolytica a clinically relevant cause of concern, not only to CKD patients, but to the general population. The profiling of Enterobacteriaceae isolates in terms of antibiotic resistance, both regarding resistance phenotypes and the presence of genetic elements, is another strong point of this paper. As mentioned before, antibiotic-resistant enterobacteria are responsible for a significant percentage of infections in CKD patients (Prasad et al., 2014; Nguyen et al., 2017; Sahli et al., 2017; Wang et al., 2019), which makes their screening crucial for the clinical follow-up of said patients, given it can provide information for efficient antibiotic prescription in case of infection. Of note that infections are a major problem in peritoneal dialysis programs, leading to patients’ deaths or exclusion from peritoneal dialysis programs (Li et al., 2016). Therefore, the search of predisposing factors that lead to peritonitis and catheter-related infections in CKD-PD patients is of outmost importance. Finally, our study also contributes to the profiling of antibiotic resistance genes in the oral microbiome. In recent years, the oral cavity has been highlighted as a reservoir for resistance genes (de Sousa Moreira Almeida et al., 2020) and the profiling of these genes provides extremely relevant information for dentistry and the treatment of oral infections.
Conclusion
Our results suggest that the accumulation of uremic toxins in CKD patients induces alterations in the oral milieu, which, in turn, exert selective pressure on the oral microbiome, leading to its dysbiosis with significantly increased prevalence of urease-producing enterobacteria, specifically of Raoultella ornithinolytica, an opportunistic enterobacteria. Antibiotic resistance phenotyping revealed mostly intrinsic resistances, although resistances to sulfamethoxazole and streptomycin were also detected. Multidrug resistance was exclusively found in CKD-PD patients isolates. These results, together with the detection of acquired antibiotic resistance genes, alert to the possibility of the oral microbiota as a potential reservoir of resistance genes.
Enterobacteriaceae are known opportunistic pathogens of CKD patients, and this CKD-induced oral dysbiosis can potentially lead to an increase of resistant infectious and inflammatory episodes, contributing, in turn, to the progression of the disease and to its mortality rate. For all these reasons, studying the oral microbiome and its associated resistome and comprehend its role in infection episodes and in the progression of systemic diseases is of extreme relevance to ensure the health and well-being of CKD patients undergoing peritoneal dialysis.
Data Availability Statement
The raw data supporting the conclusions of this article will be made available by the authors, without undue reservation.
Ethics Statement
The studies involving human participants were reviewed and approved by Ethics Committee for Health of Centro Hospitalar Universitário de São João: (approval number 200/18) and Ethics Committee for Health of Faculdade de Medicina Dentária da Universidade do Porto: (approval number 12/2019). The patients/participants provided their written informed consent to participate in this study.
Author Contributions
RM, AR, and BS-M contributed to conception and design of the study. CC, AM-R, CC, NS, LP, CF and AG contributed to data acquisition. CC, AM-R, CC, CF, RM, AR, CM and BS-M contributed to data analysis and interpretation. CC, CF, AA, RM, AR, CM and BS-M performed the statistical analysis. CC wrote the first draft of the manuscript. All authors contributed to manuscript revision, read, and approved the submitted version.
Funding
This work is a result of the project POCI-01-0145-FEDER-029777, co-financed by Competitiveness and Internationalisation Operational Programme (POCI), under the PORTUGAL 2020 Partnership Agreement, through the European Regional Development Fund (ERDF) and through national funds by the FCT – Fundação para a Ciência e a Tecnologia. CC fellowship was supported by FCT/MCTES scholarship with the reference 2020.08540.BD. This work and CF were financially supported by FEDER through project “Assessing the risks associated with environmental antibiotic resistant bacteria: propagation and transmission to humans” (PTDC/CTA-AMB/28196/2017) – Programa Operacional Competitividade e Internacionalização, and by National Funds from FCT – Fundação para a Ciência e a Tecnologia and was hosted by CBQF through FCT project UIDB/50016/2020.
Conflict of Interest
The authors declare that the research was conducted in the absence of any commercial or financial relationships that could be construed as a potential conflict of interest.
Publisher’s Note
All claims expressed in this article are solely those of the authors and do not necessarily represent those of their affiliated organizations, or those of the publisher, the editors and the reviewers. Any product that may be evaluated in this article, or claim that may be made by its manufacturer, is not guaranteed or endorsed by the publisher.
Acknowledgments
We would like to thank the kind participants in our study.
Supplementary Material
The Supplementary Material for this article can be found online at: https://www.frontiersin.org/articles/10.3389/fmicb.2021.736685/full#supplementary-material
Footnotes
References
Barraclough, K., Hawley, C. M., McDonald, S. P., Brown, F. G., Rosman, J. B., Wiggins, K. J., et al. (2009). Corynebacterium peritonitis in Australian peritoneal dialysis patients: predictors, treatment and outcomes in 82 cases. Nephrol. Dial. Transplant. 24, 3834–3839. doi: 10.1093/ndt/gfp322
Belstrøm, D., Holmstrup, P., Nielsen, C. H., Kirkby, N., Twetman, S., Heitmann, B. L., et al. (2014). Bacterial profiles of saliva in relation to diet, lifestyle factors, and socioeconomic status. J. Oral Microbiol. 6:23609. doi: 10.3402/jom.v6.23609
Benjamin, O., and Lappin, S. L. (2021). “End-stage renal disease,” in StatPearls [Internet] (Treasure Island (FL): StatPearls Publishing).
CLSI (2017). Performance Standards for Antimicrobial Susceptibility Testing. 27th Edn. Wayne, PA: Clinical and Laboratory Standards Institute.
Cox, G., and Wright, G. D. (2013). Intrinsic antibiotic resistance: mechanisms, origins, challenges and solutions. Int. J. Med. Microbiol. 303, 287–292. doi: 10.1016/j.ijmm.2013.02.009
Crielaard, W., Zaura, E., Schuller, A. A., Huse, S. M., Montijn, R. C., and Keijser, B. J. F. (2011). Exploring the oral microbiota of children at various developmental stages of their dentition in the relation to their oral health. BMC Med. Genet. 4:22. doi: 10.1186/1755-8794-4-22
de Sousa Moreira Almeida, V., Azevedo, J., Leal, H. F., de Queiroz, A. T. L., da Silva Filho, H. P., and Reis, J. N. (2020). Bacterial diversity and prevalence of antibiotic resistance genes in the oral microbiome. PLoS One 15:e0239664. doi: 10.1371/journal.pone.0239664
Derafshi, R., Bazargani, A., Ghapanchi, J., Izadi, Y., and Khorshidi, H. (2017). Isolation and identification of nonoral pathogenic bacteria in the oral cavity of patients with removable dentures. J. Int. Soc. Prev. Commun. Dent. 7, 197–201. doi: 10.4103/jispcd.JISPCD_90_17
Devanga Ragupathi, N. K., Muthuirulandi Sethuvel, D. P., Inbanathan, F. Y., and Veeraraghavan, B. (2017). Accurate differentiation of Escherichia coli and Shigella serogroups: challenges and strategies. New Microbes New Infect. 21, 58–62. doi: 10.1016/j.nmni.2017.09.003
EUCAST (2020). Intrinsic Resistance and Unusual Phenotypes, version 3.2. Available at: https://www.eucast.org/fileadmin/src/media/PDFs/EUCAST_files/Expert_Rules/2020/Intrinsic_Resistance_and_Unusual_Phenotypes_Tables_v3.2_20200225.pdf
Fysaraki, M., Samonis, G., Valachis, A., Daphnis, E., Karageorgopoulos, D. E., Falagas, M. E., et al. (2013). Incidence, clinical, microbiological features and outcome of bloodstream infections in patients undergoing hemodialysis. Int. J. Med. Sci. 10, 1632–1638. doi: 10.7150/ijms.6710
Gonçalves, M. O., Coutinho-Filho, W. P., Pimenta, F. P., Pereira, G. A., Pereira, J. A. A., Mattos-Guaraldi, A. L., et al. (2007). Periodontal disease as reservoir for multi-resistant and hydrolytic enterobacterial species. Lett. Appl. Microbiol. 44, 488–494. doi: 10.1111/j.1472-765X.2007.02111.x
Grothe, C., Taminato, M., Belasco, A., Sesso, R., and Barbosa, D. (2014). Screening and treatment for Staphylococcus aureus in patients undergoing hemodialysis: A systematic review and meta-analysis. BMC Nephrol. 15:202. doi: 10.1186/1471-2369-15-202
Gupta, M., Gupta, M., and Abhishek, (2015). Oral conditions in renal disorders and treatment considerations – A review for pediatric dentist. Saudi Dent. J. 27, 113–119. doi: 10.1016/j.sdentj.2014.11.014
Hajjar, R., Ambaraghassi, G., Sebajang, H., Schwenter, F., and Su, S. H. (2020). Raoultella ornithinolytica: emergence and resistance. Infect. Drug. Resist. 13, 1091–1104. doi: 10.2147/IDR.S191387
Hajjar, R., Schwenter, F., Su, S.-H., Gasse, M.-C., and Sebajang, H. (2018). Community-acquired infection to Raoultella ornithinolytica presenting as appendicitis and shock in a healthy individual. J. Surg. Case Rep. 2018:rjy097. doi: 10.1093/jscr/rjy097
He, Y., Li, H., Lu, X., Stratton, C. W., and Tang, Y.-W. (2010). Mass spectrometry biotyper system identifies enteric bacterial pathogens directly from colonies grown on selective stool culture media. J. Clin. Microbiol. 48, 3888–3892. doi: 10.1128/JCM.01290-10
Heggendorn, F. L., Gonçalves, L. S., Dias, E. P., Silva Junior, A., Galvão, M. M., and Lutterbach, M. T. (2013). Detection of sulphate-reducing bacteria in human saliva. Acta Odontol. Scand. 71, 1458–1463. doi: 10.3109/00016357.2013.770163
Höfer, K., Turnowsky, A., Ehren, R., Taylan, C., Plum, G., Witte, H., et al. (2021). The impact of a needs-oriented dental prophylaxis program on bacteremia after toothbrushing and systemic inflammation in children, adolescents, and young adults with chronic kidney disease. Pediatr. Nephrol. doi: 10.1007/s00467-021-05153-1
Hu, J., Iragavarapu, S., Nadkarni, G. N., Huang, R., Erazo, M., Bao, X., et al. (2018). Location-specific oral microbiome possesses features associated with CKD. Kidney Int. Rep 3, 193–204. doi: 10.1016/j.ekir.2017.08.018
Jain, A. K., and Blake, P. G. (2006). Non-pseudomonas gram-negative peritonitis. Kidney Int. 69, 1107–1109. doi: 10.1038/sj.ki.5000257
Jiang, S., Zeng, J., Zhou, X., and Li, Y. (2018). Drug resistance and gene transfer mechanisms in respiratory/oral bacteria. J. Dent. Res. 97, 1092–1099. doi: 10.1177/0022034518782659
Li, P. K.-T., and Chow, K. M. (2011). Infectious complications in dialysis – epidemiology and outcomes. Nat. Rev. Nephrol. 8, 77–88. doi: 10.1038/nrneph.2011.194
Li, X., Kolltveit, K. M., Tronstad, L., and Olsen, I. (2000). Systemic diseases caused by oral infection. Clin. Microbiol. Rev. 13, 547–558. doi: 10.1128/CMR.13.4.547
Li, P. K.-T., Szeto, C. C., Piraino, B., de Arteaga, J., Fan, S., Figueiredo, A. E., et al. (2016). ISPD peritonitis recommendations: 2016 update on prevention and treatment. Perit. Dial. Int. 36, 481–508. doi: 10.3747/pdi.2016.00078
Liakopoulos, V., Nikitidou, O., Kalathas, T., Roumeliotis, S., Salmas, M., and Eleftheriadis, T. (2017). Peritoneal dialysis-related infections recommendations: 2016 update. What is new? Int. Urol. Nephrol. 49, 2177–2184. doi: 10.1007/s11255-017-1632-9
Lira-Junior, R., Åkerman, S., Klinge, B., Boström, E. A., and Gustafsson, A. (2018). Salivary microbial profiles in relation to age, periodontal, and systemic diseases. PLoS One 13:e0189374. doi: 10.1371/journal.pone.0189374
Lisowska-Myjak, B. (2014). Uremic toxins and their effects on multiple organ systems. Nephron Clin. Pract. 128, 303–311. doi: 10.1159/000369817
Mujais, S. (2006). Microbiology and outcomes of peritonitis in North America. Kidney Int. 70, 55–62. doi: 10.1038/sj.ki.5001916
Murdeshwar, H. N., and Anjum, F. (2021). “Hemodialysis,” in StatPearls [Internet] (Treasure Island (FL): StatPearls Publishing).
Navazesh, M. (1993). Methods for collecting saliva. Ann. N. Y. Acad. Sci. 694, 72–77. doi: 10.1111/j.1749-6632.1993.tb18343.x
Nguyen, D. B., Shugart, A., Lines, C., Shah, A. B., Edwards, J., Pollock, D., et al. (2017). National healthcare safety network (NHSN) dialysis event surveillance report for 2014. Clin. J. Am. Soc. Nephrol. 12, 1139–1146. doi: 10.2215/CJN.11411116
Peleg, A. Y., and Hooper, D. C. (2010). Hospital-acquired infections due to gram-negative bacteria. N. Engl. J. Med. 362, 1804–1813. doi: 10.1056/NEJMra0904124
Ponce-Alonso, M., Rodríguez-Rojas, L., del Campo, R., Cantón, R., and Morosini, M. I. (2016). Comparison of different methods for identification of species of the genus Raoultella: report of 11 cases of Raoultella causing bacteraemia and literature review. Clin. Microbiol. Infect. 22, 252–257. doi: 10.1016/j.cmi.2015.10.035
Posse, J. L., Dios, P. D., and Scully, C. (2017). “Systemic bacteria transmissible by kissing,” in Saliva Protection and Transmissible Diseases (London, U.K: Elsevier Inc.), 29–51.
Prasad, K. N., Singh, K., Rizwan, A., Mishra, P., Tiwari, D., Prasad, N., et al. (2014). Microbiology and outcomes of peritonitis in northern India. Perit. Dial. Int. 34, 188–194. doi: 10.3747/pdi.2012.00233
Richter, S. S., Sercia, L., Branda, J. A., Burnham, C. A., Bythrow, M., Ferraro, M. J., et al. (2013). Identification of Enterobacteriaceae by matrix-assisted laser desorption/ionization time-of-flight mass spectrometry using the VITEK MS system. Eur. J. Clin. Microbiol. Infect. Dis. 32, 1571–1578. doi: 10.1007/s10096-013-1912-y
Roberts, A. P., and Mullany, P. (2010). Oral biofilms: A reservoir of transferable, bacterial, antimicrobial resistance. Expert Rev. Anti-Infect. Ther. 8, 1441–1450. doi: 10.1586/eri.10.106
Ruppé, É., Woerther, P.-L., and Barbier, F. (2015). Mechanisms of antimicrobial resistance in gram-negative bacilli. Ann. Intensive Care 5, 61. doi: 10.1186/s13613-015-0061-0
Rysz, J., Franczyk, B., Ławiński, J., Olszewski, R., Ciałkowska-Rysz, A., and Gluba-Brzózka, A. (2021). The impact of CKD on uremic toxins and gut microbiota. Toxins 13, 252. doi: 10.3390/toxins13040252
Sahli, F., Feidjel, R., and Laalaoui, R. (2017). Hemodialysis catheter-related infection: rates, risk factors and pathogens. J. Infect. Public Health 10, 403–408. doi: 10.1016/j.jiph.2016.06.008
Salloum, S., Tawk, M., and Tayyara, L. (2020). Bacterial resistance to antibiotics and associated factors in two hospital centers in Lebanon from January 2017 to June 2017. Infect. Prev. Pract. 2:100043. doi: 10.1016/j.infpip.2020.100043
Seng, P., Boushab, B. M., Romain, F., Gouriet, F., Bruder, N., Martin, C., et al. (2016). Emerging role of Raoultella ornithinolytica in human infections: a series of cases and review of the literature. Int. J. Infect. Dis. 45, 65–71. doi: 10.1016/j.ijid.2016.02.014
Sibanda, M. (2014). Primary peritonitis caused by Raoultella ornithinolytica in a 53-year-old man. JMM Case Rep. 1. doi: 10.1099/jmmcr.0.002634
Simões-Silva, L., Ferreira, S., Santos-Araujo, C., Tabaio, M., Pestana, M., Soares-Silva, I., et al. (2018). Oral colonization of staphylococcus species in a peritoneal dialysis population: A possible reservoir for PD-related infections? Can. J. Infect. Dis. Med. Microbiol. 2018:5789094. doi: 10.1155/2018/5789094
Simões-Silva, L., Silva, S., Santos-Araujo, C., Sousa, J., Pestana, M., Araujo, R., et al. (2017). Oral yeast colonization and fungal infections in peritoneal dialysis patients: A pilot study. Can. J. Infect. Dis. Med. Microbiol. 2017:4846363. doi: 10.1155/2017/4846363
Smith, D. A., and Nehring, S. M. (2021). “Bacteremia,” in StatPearls [Internet] (Treasure Island (FL): StatPearls Publishing).
Szeto, C. C., Chow, V. C., Chow, K. M., Lai, R. W., Chung, K. Y., Leung, C. B., et al. (2006). Enterobacteriaceae peritonitis complicating peritoneal dialysis: a review of 210 consecutive cases. Kidney Int. 69, 1245–1252. doi: 10.1038/sj.ki.5000037
Thepchuay, Y., Costa, C. F. F. A., Mesquita, R. B. R., Sampaio-Maia, B., Nacapricha, D., and Rangel, A. O. S. S. (2020). Flow-based method for the determination of biomarkers urea and ammoniacal nitrogen in saliva. Bioanalysis 12, 455–465. doi: 10.4155/bio-2020-0036
Tsuchida, S., Umemura, H., and Nakayama, T. (2020). Current status of matrix-assisted laser desorption/ionization–time-of-flight mass spectrometry (MALDI-TOF MS) in clinical diagnostic microbiology. Molecules 25:4775. doi: 10.3390/molecules25204775
Verma, D., Srivastava, A., Garg, P. K., Akhter, Y., Dubey, A. K., Mishra, S., et al. (2021). Taxonomic profiling and functional characterization of the healthy human oral bacterial microbiome from the north Indian urban sub-population. Arch. Microbiol. 203, 927–939. doi: 10.1007/s00203-020-02084-7
Wang, T. Z., Kodiyanplakkal, R. P. L., and Calfee, D. P. (2019). Antimicrobial resistance in nephrology. Nat. Rev. Nephrol. 15, 463–481. doi: 10.1038/s41581-019-0150-7
Webster, A. C., Nagler, E. V., Morton, R. L., and Masson, P. (2017). Chronic kidney disease. Lancet 389, 1238–1252. doi: 10.1016/S0140-6736(16)32064-5
Keywords: chronic kidney disease, oral microbiome, oral dysbiosis, peritoneal dialysis, Enterobacteriaceae, Raoultella ornithinolytica, antibiotic resistance
Citation: Costa CFFA, Merino-Ribas A, Ferreira C, Campos C, Silva N, Pereira L, Garcia A, Azevedo &, Mesquita RBR, Rangel AOSS, Manaia CM and Sampaio-Maia B (2021) Characterization of Oral Enterobacteriaceae Prevalence and Resistance Profile in Chronic Kidney Disease Patients Undergoing Peritoneal Dialysis. Front. Microbiol. 12:736685. doi: 10.3389/fmicb.2021.736685
Edited by:
Lucio Souza Gonçalves, Estácio de Sá University, BrazilReviewed by:
Anthony Ayodeji Adegoke, University of Uyo, NigeriaAshish Kumar Singh, Jagatpur Post Graduate College, India
Majid Validi, Shahrekord University of Medical Sciences, Iran
Janine Ribeiro, Universidade Ceuma, Brazil
Emma Hernandez-Sanabria, Flanders Institute for Biotechnology, Belgium
Copyright © 2021 Costa, Merino-Ribas, Ferreira, Campos, Silva, Pereira, Garcia, Azevedo, Mesquita, Rangel, Manaia and Sampaio-Maia. This is an open-access article distributed under the terms of the Creative Commons Attribution License (CC BY). The use, distribution or reproduction in other forums is permitted, provided the original author(s) and the copyright owner(s) are credited and that the original publication in this journal is cited, in accordance with accepted academic practice. No use, distribution or reproduction is permitted which does not comply with these terms.
*Correspondence: Benedita Sampaio-Maia, Ym1haWFAZm1kLnVwLnB0