- 1Lactobio A/S, Copenhagen, Denmark
- 2Department of Dermatology, Bispebjerg Hospital, University of Copenhagen, Copenhagen, Denmark
- 3Department of Food Science, Faculty of Science, University of Copenhagen, Copenhagen, Denmark
Atopic dermatitis (AD) is a common inflammatory skin disease characterized by an epidermal barrier impairment, as well as a Th2/Th22-skewed immune response, both favoring skin colonization with Staphylococcus aureus. Colonization is strongly related to severity of the disease, and a reduction of S. aureus has been found to alleviate symptoms. Lactic acid bacteria (LAB) produce antimicrobial compounds such as organic acids and bacteriocins and are widely used as probiotics. The aim of this study was to isolate LAB and screen for antibacterial effect specifically toward S. aureus clonal complex type 1. A total of 680 LAB were isolated from fermented vegetables and swab samples from healthy volunteers (vaginal, stool and skin). Screening for antibacterial activity toward S. aureus, narrowed the field of isolates down to four LAB strains with high antibacterial activity. The activity varied according to the specific LAB strain and the origin of the strain. The results suggested different modes of action, including co-aggregation, expression of bacteriocins and production of specific organic acids. However, the ability to acidify the surroundings appeared as the main effect behind inhibition of S. aureus. Broth microdilution assays showed a significant reduction of S. aureus growth when using down to 10% cell free supernatant (CFS). Our results underline the use of specific living LAB or their CFS as potential future treatment strategies to reduce S. aureus colonization of AD skin.
Introduction
Atopic dermatitis (AD) is characterized by chronic cutaneous inflammation, epidermal barrier dysfunction and increased susceptibility to skin infections (Bieber, 2008; Brown, 2016). Colonization with S. aureus is found on the skin of ~70% of AD patients and is directly correlated with the severity of the disease (Totté et al., 2016; Geoghegan et al., 2018). Whether S. aureus is the primary trigger of inflammation or simply colonizes the skin due to inflammation is unknown, but reduction of S. aureus on the skin has been found to alleviate symptoms of the disease (Gong et al., 2006; Myles et al., 2018; Nakatsuji et al., 2021). A high proportion of S. aureus colonizing skin of AD patients are found to be resistant to the preferred topical antibiotic treatment with fusidic acid (Edslev et al., 2017) and alternatives to the traditional antibiotic therapies are therefore of interest.
Lactic acid bacteria (LAB) are a ubiquitous group of bacteria including lactobacilli, lactococci, enterococci, streptococci, leuconostoc, and pediococci. LAB are found in soil, water and plants but also on mucosal surfaces of humans and animals in the gastrointestinal and urogenital tracts, as well as on the human skin (Grice et al., 2009; Chu et al., 2017). LAB are generally considered beneficial due to their immunomodulating effect, their ability to alter the microbiota composition in some habitats and their positive effect on the gastro-intestinal health (Hill et al., 2014). LAB release bioactive molecules such as organic acids and antimicrobial peptides (AMP) that inhibit pathogen growth and interfere with the quorum sensing system of pathogens (Arief et al., 2015). Some LAB co-aggregate with pathogens facilitating removal of the pathogen from the skin via peristaltic elimination and thereby prevention of pathogen skin interaction (Lukic et al., 2017; Siedler et al., 2020). Another antimicrobial mechanism indicated by co-aggregation is competitive displacement of the pathogen with some LAB strains showing high affinity binding to epithelial cell receptors (Lukic et al., 2017; Spacova et al., 2020). Several studies demonstrate antimicrobial effects of LAB on S. aureus (Blanchet-Réthoré et al., 2017; Jayashree et al., 2018; Spacova et al., 2020; Delanghe et al., 2021; Musa et al., 2021) and recent findings indicate, that aggregation of lactobacilli to S. aureus is important for the ability of lactobacilli to prevent or reduce S. aureus adhesion to epithelial surfaces (Younes et al., 2016). Previous study has shown that topical application of LAB in a cream formulation can have beneficial effect on AD symptoms and S. aureus colonization on skin (Blanchet-Réthoré et al., 2017; Butler et al., 2020). Most studies target standard laboratory S. aureus without specifying the clonal type of S. aureus. However, findings indicate that a specific clonal lineage of S. aureus, clonal complex (CC) type 1, are more prevalent in AD patients, and are also associated with filaggrin gene (FLG) mutations (Irvine et al., 2011; Clausen et al., 2017). These findings suggest that the CC-1-type of S. aureus is important in colonization of the AD skin, and therefore a relevant target when studying the microbial interactions on the skin.
The aim of this study was to isolate novel LAB strains from several different habitats, establish a strain collection with substantial variation in lactobacilli species and screen for beneficial antibacterial properties toward AD prevalent S. aureus CC-type 1.
Materials and Methods
The study was divided into three screening parts, enabling the discovery of LAB with antibacterial activity toward S. aureus from a library collection of 680 LAB. A flow chart of the screening process is given in Figure 1.
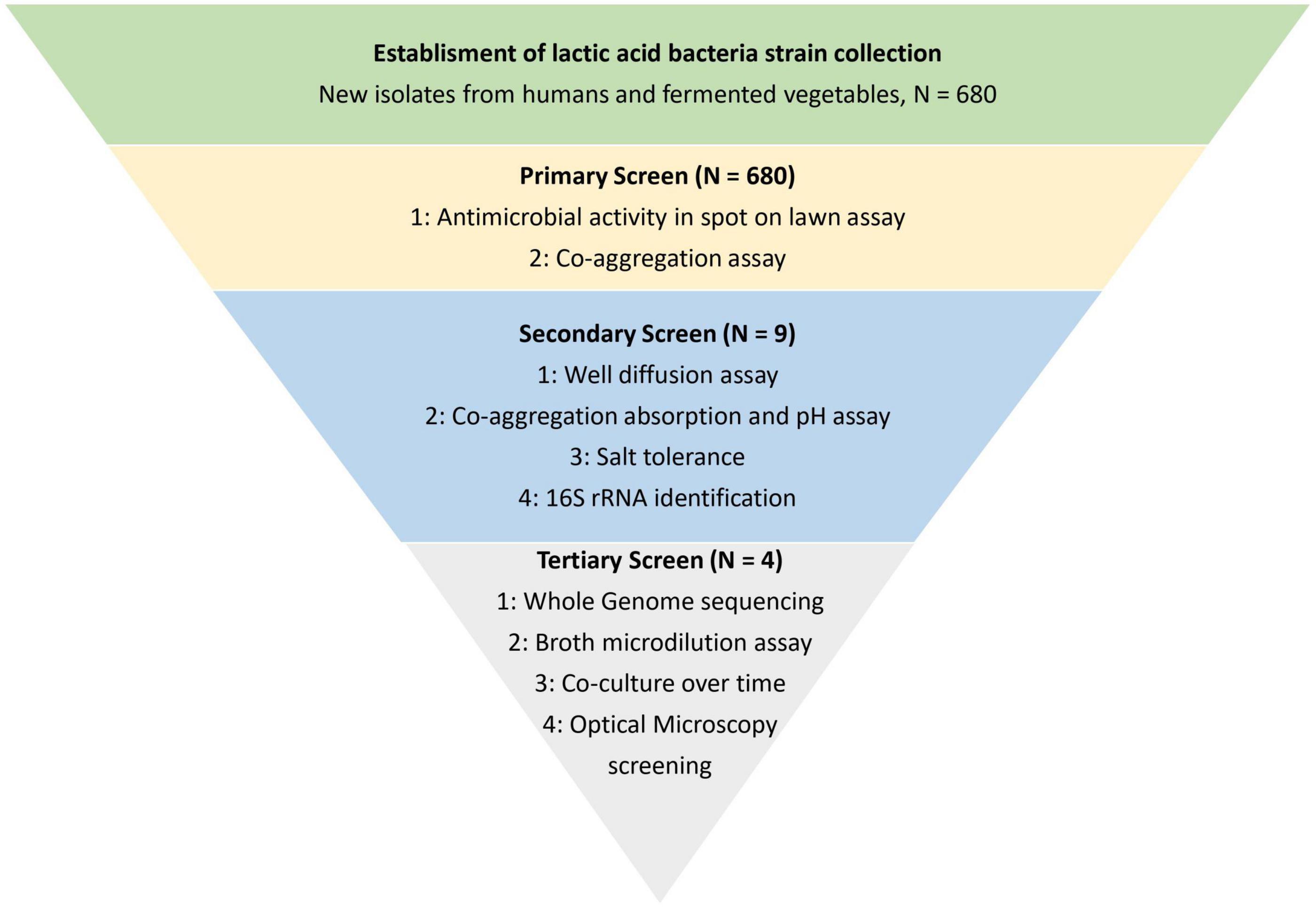
Figure 1. Schematic overview of the study. The study was divided into three screening parts for identification and characterization of lactic acid bacteria with antimicrobial activity toward Staphylococcus aureus CC-type 1. The screening was performed to narrow down the library of 680 lactic acid bacteria strains to four strains with the highest observed antibacterial activity.
Bacterial Strains and Growth Conditions
A library of 680 LAB was established for screening in this study. LAB were isolated from different natural sources, including fermented vegetables and swab samples from vaginal, stool and skin of Danish healthy volunteers (Table 1), in compliance with the national laws on access to genetic resources. All LAB strains in the library were collected in compliance with the Bioconvention and the Nagoya protocol (The Convention on Biological Diversity, 2011). The LAB were isolated on de Man, Rogosa and Sharp (MRS) agar plates (69964, Millipore) incubated at 37°C under anaerobic conditions for 24 h. Colonies were randomly picked from the agar plate and purified prior to long term storage in MRS broth (69966, Millipore) with 20% glycerol (G5516, Sigma Aldrich) at −80°C.
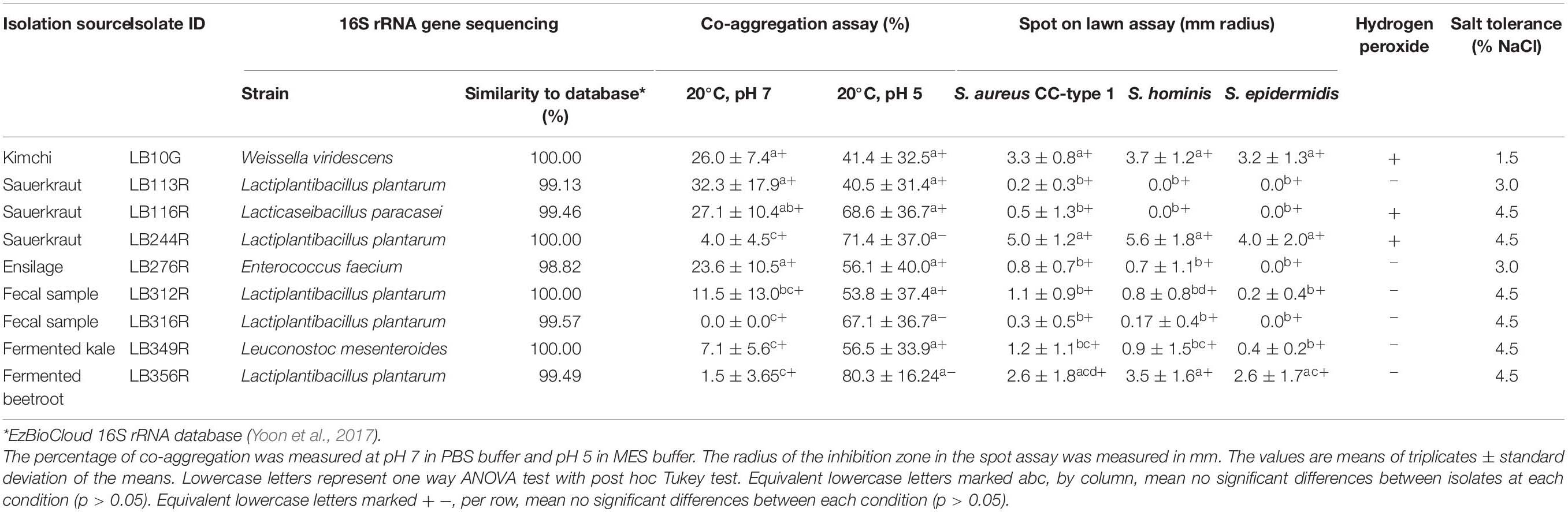
Table 1. Antimicrobial activity (as determined by spot on lawn assay and hydrogen peroxide production) against Staphyloccous aureus CC-type 1, co-aggregation with S. aureus CC-type 1, species level identification (by 16S rRNA gene sequencing) and salt tolerance of the 9 best performing LAB from the secondary screen.
Staphylococcus epidermidis HM-140 (BEI Resources), Staphylococcus hominis HM-119 (BEI Resources) and human origin isolate of Staphylococcus aureus clonal complex (CC) type 1 (University of Copenhagen) were used as target organisms in this study. The Staphylococcus strains were grown in Brain Heart Infusion (BHI) broth (53286, Millipore) at 37°C under aerobic conditions overnight.
Visual Co-aggregation (Primary Screen)
Visual co-aggregation between LAB isolates and S. aureus CC-type 1 was performed in the primary screen of all 680 LAB strains as a rapid screening according to Cisar et al. (1979) and Ekmekci et al. (2009). LAB isolates were grown in MRS broth and target organism S. aureus CC-type 1 in BHI broth over night at 37°C under aerobic conditions. The cultures were harvested by centrifugation at 10,000 × g for 5 min, washed twice using sterile phosphate-buffered saline (PBS) buffer (P5493, Sigma Aldrich) and hereafter resuspended in PBS buffer. LAB suspension (200 μL) was mixed with 200 μL of the target organism S. aureus CC-type 1 in 48-well plates and incubated overnight at room temperature under constant shaking at ~500 rpm. Control samples containing 200 μl of each bacterial suspension were maintained at the same time. Co-aggregation was scored according to the degree of co-aggregation ranging from 0 (no-aggregation) to four (large co-aggregates covering most of the well) (Cisar et al., 1979).
Spot on Lawn Assay (Primary Screen)
The spot on lawn assay was performed according to Zhang and Tamplin (2015) with some modifications. An overnight culture of S. aureus CC-type 1 was diluted to an OD of 1.0 (600 nm) and further diluted twofold. Two hundred microliter of the adjusted cell suspension was plated onto BHI agar plates (70138, Millipore) and the plates were left for drying for ~10 min. Overnight cultures of isolated LAB (20 μL) were spotted onto the dry S. aureus lawn. The inhibition halos around the spots were scored at four levels, 4, 3, 2 and 1, corresponding to radius (R) ≥ 4 mm, 2 mm ≤ R < 4 mm, 0.5 mm < R < 2 mm and 0 < R ≤ 0.5 mm, respectively (Zhang and Tamplin, 2015).
Absorbance Detection of Co-aggregation (Secondary Screen)
Co-aggregation between LAB isolates and S. aureus CC-type 1 was determined by measuring optical density (OD) at 600 nm according to Handley et al. (1987). The percentage of co-aggregation is calculated by following equation.
ODtarget, ODLAB, and ODmix represent the OD measure at 600 nm of individual pathogen, LAB and their mixture after incubation for 24 h. Lowercase letters represent significant differences between the LAB strains (p < 0.05) (Handley et al., 1987).
pH Effect on Co-aggregation (Secondary Screen)
According to Ekmekci et al. (2009) the pH of the solution can affect the co-aggregation ability. Hence, 2-(N-Morpholino) ethanesulfonic acid (MES) buffer (69892, Sigma-Aldrich) with a pH adjusted according to the pH of the skin (pH 5) was used instead of PBS for cell suspension and otherwise carried out as in section “Absorbance Detection of Co-aggregation (Secondary Screen).”
Well Diffusion Assay (Secondary Screen)
A well diffusion assay was performed with overnight cultures of LAB and the cell free supernatant (CFS) of LAB. The assay was performed according to Oldak et al. (2017) with some modifications. Overnight cultures of LAB were centrifuged (8,000 × g, 20 min, 4°C) and the supernatant filter sterilized (Filtropur S 0.2 μm membrane, Sarstedt) to remove excess cells. The overnight culture and CFS was filled in 7 mm wells in Mueller-Hinton agar plates inoculated with S. aureus CC-type 1, S. epidermidis or S. hominis and the radius of the inhibition zone measured (in mm) in triplicate of three independent experiments.
Hydrogen Peroxide Production (Secondary Screen)
Test for production of hydrogen peroxide was performed according to Marshall (1979). Overnight culture of isolated LAB was spotted (20 μL) onto MRS agar plates with 0.25 mg/ml 3,3′, 5,5′-tetramethylbenzidine (TMB) (Sigma-Aldrich) and 0.01 mg/ml of horseradish peroxidase (HRP) (Sigma-Aldrich). The plates were incubated at 37°C under anaerobic conditions for 24 h and hereafter exposed to oxygen in 4 h before visually determining hydrogen peroxide production due to blue pigmentation in the spots (Marshall, 1979).
Salt Tolerance of LAB (Secondary Screen)
To test the survival ability of LAB on skin, the salt tolerance of the LAB hit strains at NaCl concentrations of 1.5; 3; 4.5; and 6% in MRS broth were used. Overnight culture of LAB hit strains were inoculated into the salt broth concentrations and incubated over night at 37°C aerobically. Growth of LAB in the MRS + NaCl was determined visually (clear well when no growth).
Identification by 16S rRNA Gene Sequencing (Secondary Screen)
The isolates (9 in total) with most promising antimicrobial activity against the targeted Staphylococcus strains (see section “Bacterial Strains and Growth Conditions”) were identified by near full-length 16S rRNA gene sequencing (V1–V9 regions), sequencing services were provided by GENEWIZ Germany GmbH. Similarity-based search against EzBioCloud 16S rRNA gene sequence database was used for taxonomic identification (Yoon et al., 2017).
Analysis of Whole Genome Sequences (Tertiary Screen)
Four of the LAB hit strains were whole genome sequenced by Baseclear (Leiden, Netherlands) and annotated using Rapid Annotation Subsystem Technology (RAST) server1 to reveal virulence or antibiotic resistance encoding genes. ResFinder2 was used subsequently to analyze the four genomes for resistance genes (Bortolaia et al., 2020). The analysis is set to search for acquired antimicrobial resistance genes. The annotation program Bacteriocin Genome mining tool, BAGEL43 was used to reveal potential bacteriocin encoding genes (Van Heel et al., 2018).
Co-culture Assay—Inhibition Over Time (Tertiary Screening)
The antibacterial effect of LAB toward S. aureus CC-type 1 was determined over time according to Coman et al. (2014) and Tetili et al. (2017) with modifications. A suspension of 2 mL MRS broth mixed 1:4 with overnight LAB culture (~106 CFU/ml) was mixed with 2 mL BHI broth with 1% overnight culture of S. aureus CC-type 1 (~106 CFU/ml). The antibacterial effect of LAB was revealed by CFU counting of viable S. aureus on selective mannitol salt phenol red agar (MSA) (Millipore 63567) according to Jett et al. (1997). The CFU/ml of S. aureus CC-type 1 was determined after 0, 2, 4, 8, 12, and 24 h of incubation.
Turbidimetric Assay of S. aureus and LAB Cell Free Supernatant (Tertiary Screening)
A broth microdilution assay with various fractions of LAB CFS was used to determine the minimum percentage of CFS able to inhibit growth of S. aureus. The growth inhibition was measured by phase contrast microscopy and image analysis using an oCelloscope (BioSense Solution, Denmark). The inhibitory effect of LAB CFS on S. aureus CC-type 1 was measured over time as described previously with slight modifications (Fredborg et al., 2013). An overnight culture of S. aureus was diluted to a concentration of ~104 CFU/ml. An overnight culture of LAB (109 CFU/ml) was filtered through a 0.2 μm filter to remove all cells. The CFS was diluted into 75, 50, 25, and 10% of the original content using MRS broth. A 100 μL aliquot of diluted S. aureus cell suspension was mixed with 100 μL undiluted or diluted CFS in 96 well plates. The plate was sealed with oxygen permeable film (Sigma-Aldrich) and incubated in the oCelloScope instrument (BioSense Solution, Denmark) at 37°C for 18 h. The S. aureus growth was measured every 20 min as segmentation and extraction of surface area (SESA).
Statistical Analysis
Statistical comparisons were performed by one-way ANOVA test (p < 0.05 was considered as statistically significant). Tukey’s test was used to perform multiple comparisons between all means. All statistical studies were conducted in the statistical software R (version 4.0.0. R Core Team, 2020, Vienna, Austria).4
Results
Primary Screen
A total of 680 presumptive LAB isolates were cultivated on MRS agar from fermented vegetables (sauerkraut, Kimchi, and fermented vegetables) and from swab samples from healthy male and female Danish volunteers (vaginal, stool, and skin samples) and investigated for their antibacterial effect against S. aureus CC-type 1. As seen from Figure 2 isolates varied widely in this as determined by spot on lawn and co-aggregation assay. The outcome depended on source of origin and the specific strain. Especially LAB isolated from sauerkraut showed a high co-aggregation score while isolates from kimchi showed a high antimicrobial activity (Figure 2). Five strains with the highest observed co-aggregation abilities and four with the highest antibacterial activity on the spot assay were selected for further identification and characterization (Figure 1).
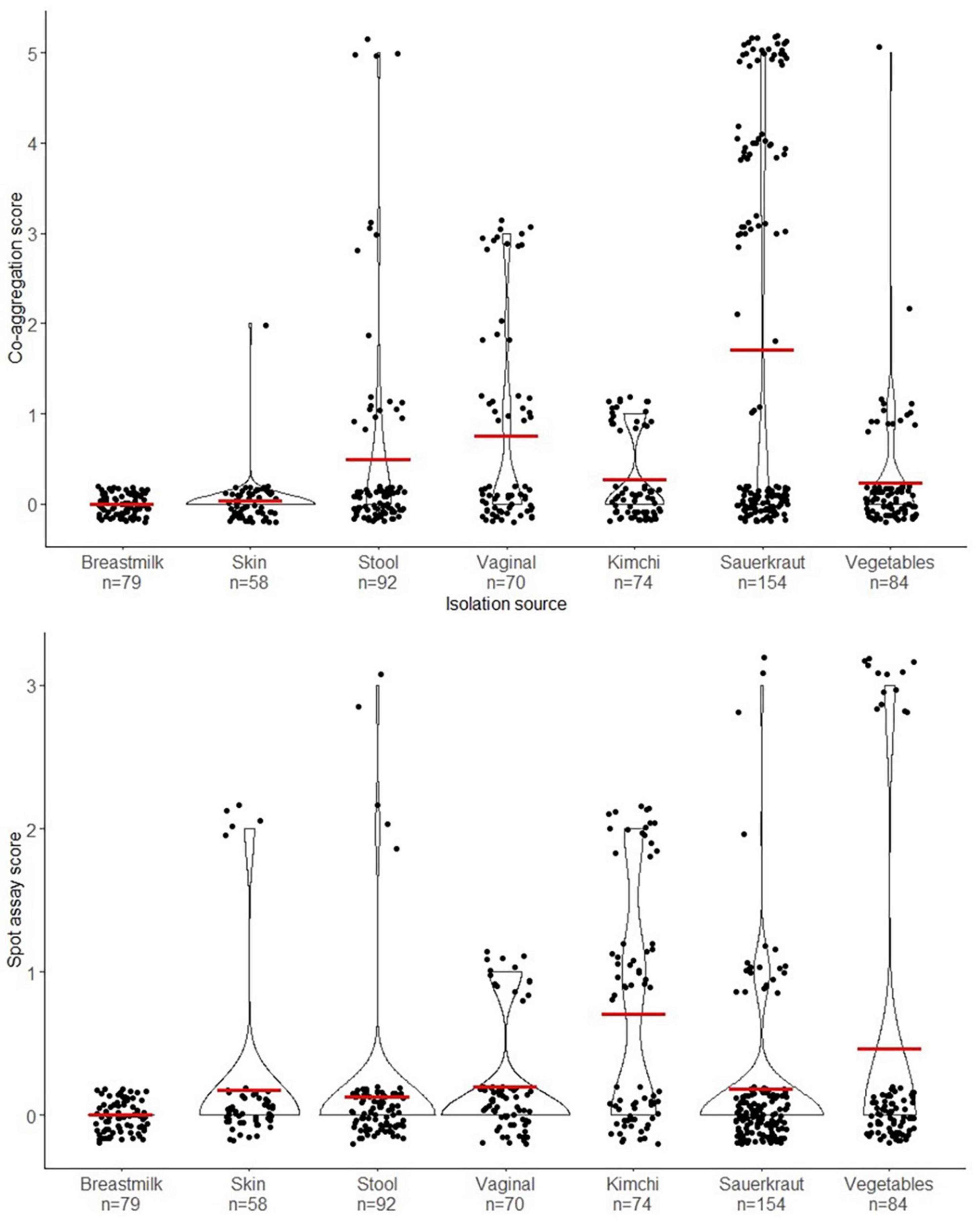
Figure 2. Violin plot illustrating the distribution of all the isolates from the primary screen and their isolation source. The upper graph shows the distribution according to co-aggregation score (0–5) and the lower graph shows the distribution of the isolates according to the spot on lawn assay score (0–5). The red crossbar shows the mean of the assay scores for each isolation source.
Secondary Screen
Identification by 16S rRNA Sequencing
Five of the nine isolates selected for further characterization were identified as Lactiplantibacillus plantarum, one as Lacticaseibacillus paracasei and the three remaining isolates were identified as Weissella viridescens, Enterococcus faecium, and Leuconostoc mesenteroides, respectively (Table 1).
Spectrophotometric Co-aggregation Assay
The LAB isolates that showed high co-aggregation score in the primary screen were studied thoroughly by measuring the percentage of co-aggregation (Table 1). W. viridescens LB10G, Lp. plantarum, LB113R and LB116R, and Enterococcus faecium LB276R showed the highest percentage of co-aggregation (p < 0.05) compared to the other hit strains at pH 7 (Table 1). When the pH was lowered to pH 5 to mimic the pH of the skin, the co-aggregation increased for all hit strains and considerably for Lp. plantarum LB244R, LB316R, and LB356R (p < 0.05).
Antibacterial Activity
The antibacterial effect of the nine most promising LAB was tested against three different staphylococci species, namely S. aureus and coagulase negative S. epidermidis and S. hominis (Table 1). W. viridescens LB10G, Lp. plantarum LB244R and LB356R showed the strongest inhibition toward all three species of staphylococci (Table 1). On the contrary, Lp. plantarum LB113R and LB116R which had the highest co-aggregation ratio exerted limited antibacterial activity toward the target organisms. No significant difference in antibacterial activity of LAB against the three Staphylococci species were found.
Hydrogen Peroxide Production
Of the nine hit strains, W. viridescens LB10G, Lc. paracasei LB116R, and Lp. plantarum LB244R showed hydrogen peroxide production (Table 1).
Stress Tolerance Toward NaCl
To simulate survival under the relatively high NaCl concentrations bacteria might encounter on the skin, the nine hit strains were tested for their salt tolerance. Lp. plantarum LB244R, LB312R, LB316R, and LB356R showed the highest tolerance (4.5% added NaCl) while W. viridescens LB10G showed the lowest tolerance (1.5% added NaCl) (Table 1).
Tertiary Screen
From the secondary screen, four of the LAB strains, namely W. viridescens LB10G, Lp. plantarum LB113R, and Lp. plantarum LB244R and LB356R, were chosen for further characterization. The strains were selected due to their high co-aggregation abilities or due to their high antibacterial activity in the well diffusion assay.
Basic Genome Sequence Analysis of LAB Strains
Several genes involved in bacteriocin production were identified in the Lp. plantarum LB244R and LB356R genome sequences (Supplementary Table 1). These genes are located in the well characterized bacteriocin plantaricin (pln) loci consisting of operons with plantaricin encoding genes and genes involved in the three-component regulatory networks (Diep et al., 2009). The loci found in Lp. plantarum LB244R contains the operons plnGHSTUVW, plnABCD, and plnEFI, indicating a quorum sensing regulation of the plantaricin EF complex and the cognate immunity protein by the three-component regulatory network, consisting of the inducing factor plantaricin A, the histidine kinase plnB and the two regulators plnC and plnD (Diep et al., 1995, 2009; Nes et al., 1996; Goel et al., 2020). The plnEF complex was found in Lp. plantarum LB356R as well, along with plnJK and plnN. No known bacteriocin encoding genes were found in W. viridescens LB10G and Lp. plantarum LB113R.
Additionally, genes involved in the production of antibacterial metabolites were found in the genome of all four strains. Hydroxyisocaproate dehydrogenase responsible for the conversion of 2-hydroxyisocaproic acid (HICA) was found in Lp. plantarum LB244R and LB356R. HICA has previously been identified as antibacterial toward S. aureus (Park et al., 2017).
The RAST analysis of the four LAB genomes did not reveal any virulence or toxin encoding genes (Figure 3). Genes encoding tetracycline and beta-lactam resistance were found in the RAST analysis in conserved domains of LB244R and LB356R, however, these genes were not classified as being functional and additional analysis with the server ResFinder did not reveal any resistance genes in the four strains (Bortolaia et al., 2020). Minimum inhibitory concentration (MIC) assays with tetracycline and ampicillin did not reveal resistance, according to the cut-off values determined by the European Food Safety Authority (EFSA, 2012) (data not shown). Beta-lactamase resistance gene was found in LB113R, however, MIC assays showed susceptibility toward ampicillin. No resistance genes were found in W. viridescens LB10G.
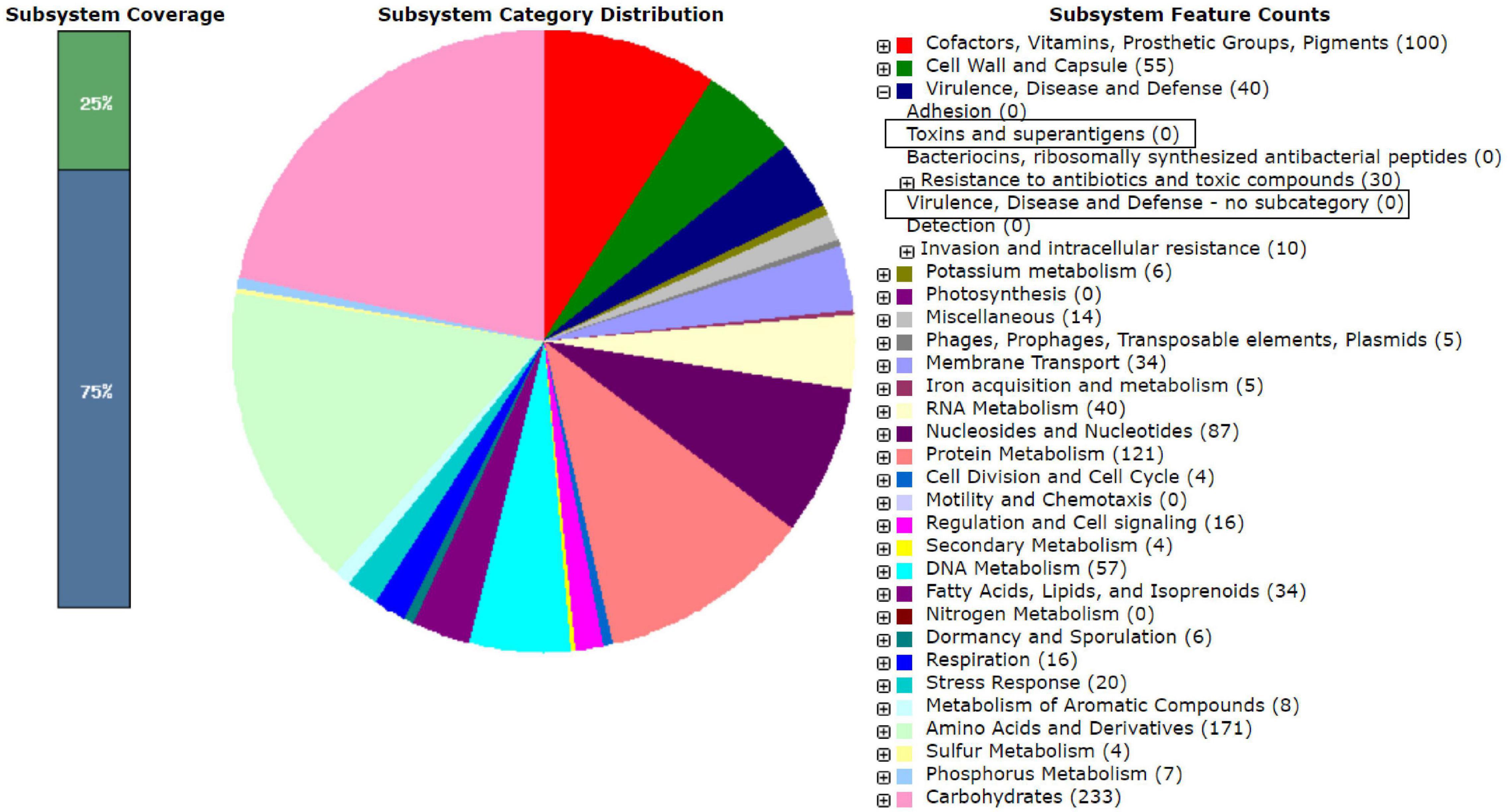
Figure 3. RAST analysis on the genome sequence of the Lactiplantibacillus plantarum subsp. plantarum LB244R. The marked boxes show that no genes encoding toxins or virulence were found in the genome.
The Four Hit Strains Inhibit S. aureus CC-Type 1 in Co-culture
The inhibitory effect of the four LAB strains on S. aureus CC-type 1 was determined during 24 h in a co-culture assay (Figure 4). After 4 h of incubation, the viable cell count of S. aureus CC-type 1 was significantly reduced in the co-cultures for all four strains (2.5–3.0 log CFU/ml, p < 0.001). After 8 hs of incubation no viable cells were detected in the co-culture with LB356R and after 12 h of incubation, no viable S. aureus cells were detected for all four co-cultures (detection limit 100 CFU/g).
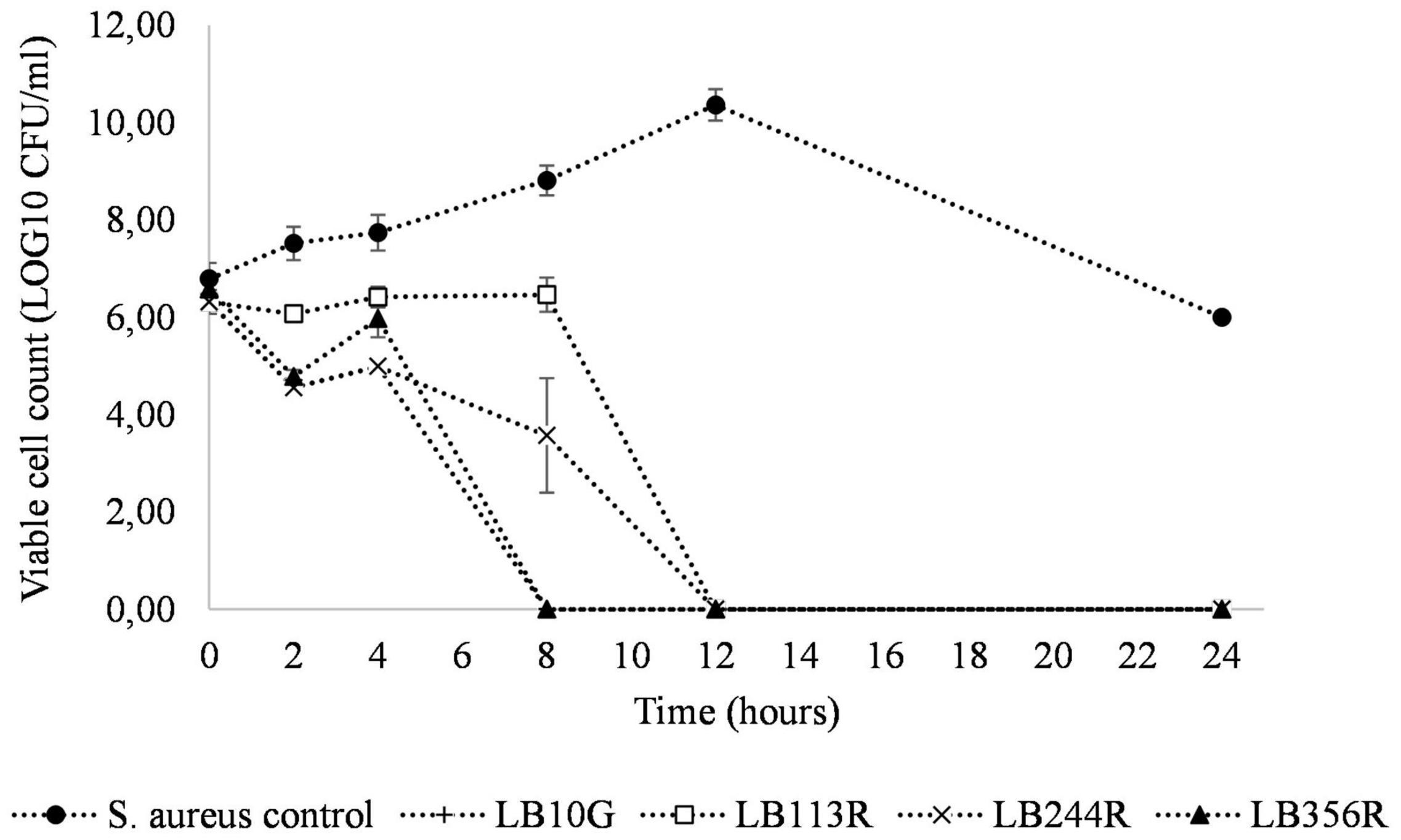
Figure 4. Co-culture of Staphylococcus aureus CC-type 1 and the four hit strains Lactiplantibacillus plantarum subsp. plantarum LB113R, LB244R and LB356R and Weissella viridescens LB10G over time. The growth of S. aureus is detected on S. aureus selective MSA agar plates with a detection limit of 100 CFU/g. The values are the mean ± standard deviations of triplicates repeated in two individual experiments.
Cell Free Supernatant of LAB Inhibits S. aureus CC-Type 1
CFS from all four LAB strains selected in the tertiary screen inhibited growth of S. aureus CC-type 1 (Figure 5A). Lp. plantarum LB113R as well as Lp. plantarum LB244R and LB356R showed complete inhibition after 2 h. W. viridescens LB10G did not inhibit S. aureus CC-type 1 to the same extent, but growth of S. aureus CC-type 1 was still significantly reduced from 2.79 SESA to 1.19 SESA (p < 0.001) after 8 h of incubation. Broth microdilution of Lp. plantarum LB244R and LB356R CFS showed reduction in growth using as low as 10% CFS (Figure 5B). The lag phase of the CC-type 1 was prolonged when adding 10 and 25% of Lp. plantarum LB244R and LB356R CFS to the culture, however, after 12 h of incubation, the growth was equal to the control sample. Complete inhibition of growth was detected using 100 and 50% of Lp. plantarum LB244R and LB356R CFS.
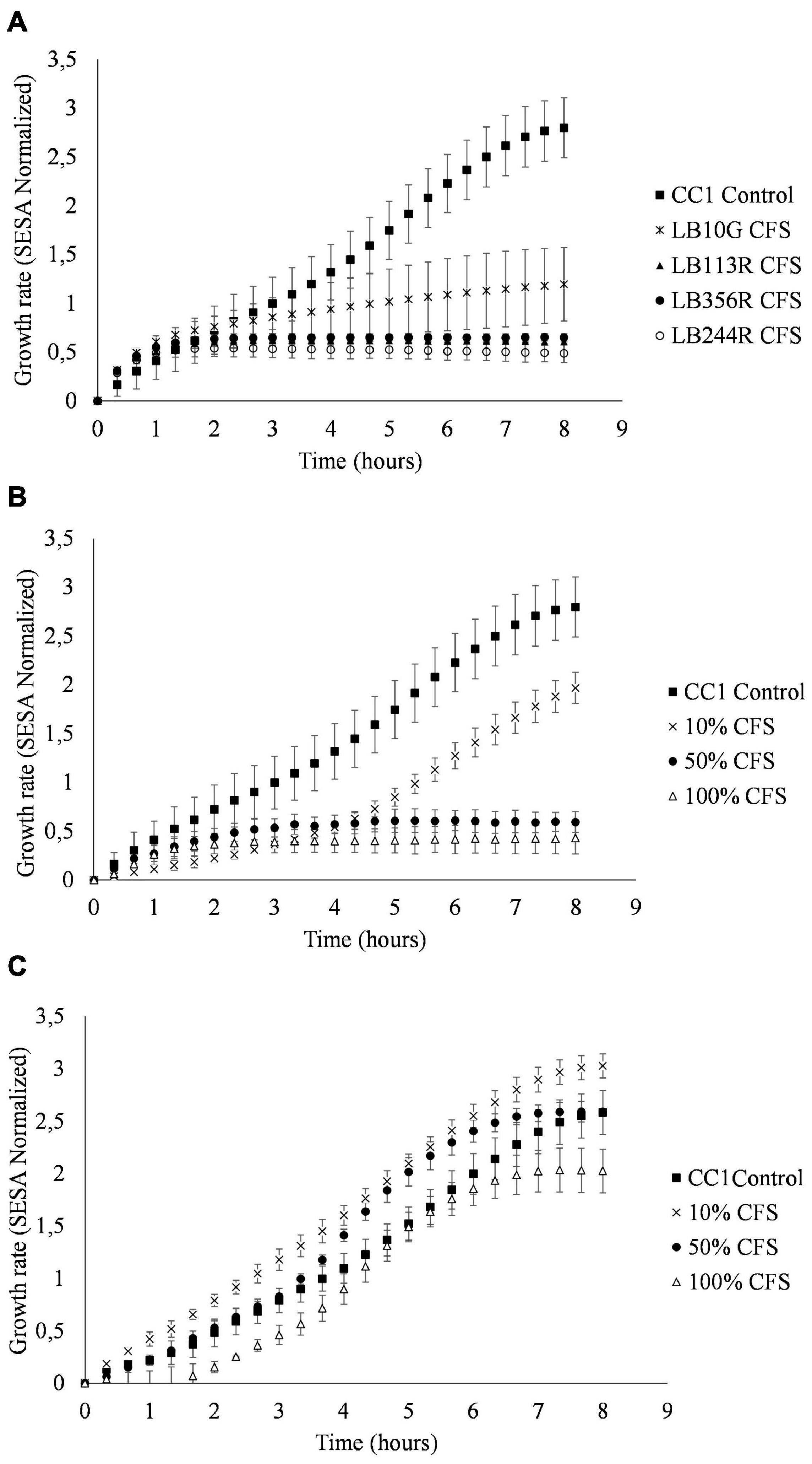
Figure 5. Culture assays measuring the growth of S. aureus CC-type 1 when exposed to cell-free supernatants from Lactiplantibacillus plantarum subsp. plantarum LB224R. Growth over time assessed by the oCelloscope using the normalized segmentation and extraction of surface area (SESA) algorithm. (A) S. aureus CC-type 1 growth inhibition by CFS from the LAB strains selected in the tertiary screen (B) Broth microdilution determination of S. aureus CC-type 1 growth inhibition by percentages of added LB244R CFS. (C) Determination of pH effect on antibacterial activity of CFS. S. aureus CC-type 1 is cultured with pH neutralized LB244R CFS (pH 6). The values are the mean ± standard deviations of triplicates repeated in two individual experiments.
To adjust for pH effect of the CFS on the viability of S. aureus CC-type 1, the pH of the CFS was neutralized to around pH 6. When the pH of the CFS was adjusted, the inhibitory effect of the CFS was lost for all four LAB strains when using 10 and 50% CFS. A prolonged lag phase and slightly reduced growth from 2.58 to 2.02 SESA (p = 0.365) was detected when using 100% pH neutralized CFS (Figure 5C).
Discussion
The present study selectively identified novel isolated LAB strains potentially suitable for reduction of S. aureus colonization of AD skin. The antibacterial effect was tested against S. aureus CC-type 1, which is the most prevalent CC-type in AD (Clausen et al., 2017).
The isolates showing the highest antibacterial effect toward S. aureus were primarily LAB isolated from fermented vegetables, with LAB isolated from human swab samples being less inhibitory on average (Figure 2). This indicates that the microenvironment of the bacteria, may lead to a differentiation of the competitive mechanisms between species according to their natural microenvironment (Oldak et al., 2017). LAB isolated from sauerkraut (salt concentration of 1.5–3 %) revealed a rather high salt tolerance of 4.5%, suggesting that the LAB isolates would be capable of prolonged survival on the skin environment with high salt concentrations.
The antibacterial effect of LAB was tested toward S. hominis and S. epidermidis, two skin commensals, to study whether the effect specifically targets S. aureus or a variety of skin bacteria. The results showed antibacterial effect toward all three staphylococci, with no significant difference (p < 0.05) in inhibition among them (Table 1). The antibacterial effect of LAB toward S. aureus was very specific to the individual LAB isolate. The findings showed that Lp. plantarum LB244R and LB356R had the highest antibacterial activity (Table 1) while the isolates LB113R and LB116R showed good co-aggregation abilities (Table 1), but very little antibacterial effect in the spot tests (Table 1). Overnight culture of all four strains had an acidic pH of ~3.8 but only Lp. plantarum LB244R and LB356R showed antibacterial effect in the spot test (Table 1). These differences in activity suggest that the inhibitory effect is not only due to lowered environmental pH. Analysis of the genome sequences of LB244R and LB356R revealed genes encoding the bacteriocin plantaricin EF and JK complex. Further analysis would be to test whether these are expressed by the LAB cultures and if the expression is upregulated upon exposure to S. aureus. Selegård et al. (2019) have showed that plantaricins EF and JK significantly lyse S. epidermidis and following studies by Musa et al. (2021) showed how the two-peptide Plantaricin NC8 αβ eliminated S. aureus and counteracting its inflammatory and cytotoxic effect (Selegård et al., 2019; Musa et al., 2021). No bacteriocin encoding genes were found in the genome of LB113R, supporting the spot assay results revealing no antibacterial effect of LB113R. No effect was observed for the CFS or heat-treated LAB cells on the spot assays (data not shown), suggesting that the growth conditions or the direct interaction between the LAB and S. aureus on the spot agar plate affect the production of antibacterial compounds. The liquid co-culture of LAB CFS and S. aureus CC-type 1 showed a high antibacterial effect of the LAB CFS in contrast to the spot assay on solid agar. The antibacterial effect of the LAB CFS was lost when pH was neutralized indicating most of the effect being due to acidic pH and organic acids produced by LAB.
The results of the optical screening with LAB CFS indicate the importance of acidic pH to maintain antibacterial effect of the bioactive compounds found in the CFS. Neutralized pH can affect the produced bacteriocins and the effect of these. Studies by Barbosa et al. (2016) showed that plantaricins lost their activity at a pH higher than pH 6, indicating that changing the pH alters the effect of the bacteriocins in the CFS (Barbosa et al., 2016). Additionally, organic acids can, in their undissociated form, penetrate the cytoplasmic membrane, causing intracellular acidification and a following collapse of the transmembrane proton motive force (Arena et al., 2016).
Production of organic acids by LAB may have a positive effect on the AD skin (Lee et al., 2016). The pH of AD skin infected with S. aureus is found to be higher than the pH of normal skin, which increases the vulnerability of the skin barrier and might promote S. aureus colonization (Ali and Yosipovitch, 2013; Panther and Jacob, 2015; Clausen et al., 2019). According to Miajlovic et al. (2010), lowered pH led to the reduction of protein expression of surface proteins promoting colonization of host tissue by S. aureus. Hence, the acid produced by LAB might also affect the pH of the skin and hereby decrease the colonization of S. aureus.
Specific clonal lineages of S. aureus are detected in AD patients, with S. aureus CC-type 1 as the most prevalent among AD patients with filaggrin mutations (Clausen et al., 2019; Iwamoto et al., 2019). Recent studies have shown that in comparison to standard laboratory S. aureus strains, clinical S. aureus strains from AD skin promote an AD-specific immune environment by altering the T cell response resulting in a Th2/Th22 skewed immune response (Iwamoto et al., 2017, 2019) suggested that the S. aureus derived cell wall proteins and secreted virulence factors represent a future therapeutic target (Iwamoto et al., 2019). Future studies could be to investigate the effect of LAB on the expression of these virulence factors and whether the LAB through microbial interactions could shift the gene expression of S. aureus toward a commensal state.
While RAST-based analysis of the whole-genome sequenced isolates showed that isolates LB244R and LB335R carried genes encoding resistance against tetracycline and beta-lactams in conserved regions of their genome and isolate LB113R carried genes encoding resistance against beta-lactams. However, more detailed inspection of the genomes using ResFinder could not confirm this. Further, when tested against tetracycline and ampicillin, these isolates were all susceptible at concentrations well below the cut-off values recommended by the European Food Safety Authority (EFSA, 2012). Horizontal transfer of genes encoding antibiotic resistance from these strains does therefore not seem to be risk. The ability to decarboxylate amino acids is a common trait among LAB and might result in the formation of biogenic amines (Barbieri et al., 2019). The isolates obtained in the present study was not tested for their ability to produce biogenic amines. However, as the isolates are intended for use as skin targeting probiotics, the ability to form biogenic amines does not seem like a major risk either.
The strength of this study is the large library of LAB with broad diversity of origin and how this affects their antimicrobial activity. Subsequently, the screening was targeted specifically toward S. aureus CC-type 1, which is dominant on the skin of AD patients. However, the results presented remain preliminary as the isolates have not yet been tested in vivo. Furthermore, future studies are required to illuminate in more detail how the LAB will affect and interact with the AD skin cells upon colonization of S. aureus CC-type 1.
Conclusion
In summary, four LAB candidates were found to inhibit growth of S. aureus CC-type 1. The results indicate that the antibacterial activity is caused primarily by acidic pH and might be due to a combination of different modes of action including co-aggregation, bacteriocin production and other antibacterial metabolites. These findings suggest that specific LAB strains hold potential as live biotherapeutic preventing S. aureus colonization of AD skin.
Data Availability Statement
The data presented in the study are deposited in the GenBank repository, accession numbers JAIFOK000000000 (LB10G), JAIFOL000000000 (LB113R), MZ855496 (LB116R), JAIFOR000000000 (LB244R), MZ855497 (LB276R), MZ855498 (LB312R), MZ855499 (LB316R), MZ855500 (LB349R), and JAIFOM000000000 (LB356R).
Author Contributions
SK, CV, TA, M-LC, and IC formulated and designed the study. IC performed the experimental work, described in this manuscript, processed, analyzed the data, and prepared the manuscript. DN carefully edited the first draft of the manuscript. All authors interpreted the experimental results along with the preparation of the manuscript draft, and gave their approval to the final version of the manuscript. The manuscript was written through contributions of all authors.
Funding
This study was supported by the Lactobio A/S and the Innovation Fund Denmark through funding of an industrial Ph.D-project (Ref.no. 8053-00218B).
Conflict of Interest
SK and CV have financial relationships as CEO and COO of Lactobio A/S. IC is an employee of Lactobio.
The remaining authors declare that the research was conducted in the absence of any commercial or financial relationships that could be construed as a potential conflict of interest.
The authors declare that this study received funding from Lactobio A/S. The funder had the following involvement in the study: study design, data collection and analysis, decision to publish and preparation of the manuscript.
Publisher’s Note
All claims expressed in this article are solely those of the authors and do not necessarily represent those of their affiliated organizations, or those of the publisher, the editors and the reviewers. Any product that may be evaluated in this article, or claim that may be made by its manufacturer, is not guaranteed or endorsed by the publisher.
Acknowledgments
Clinical isolates of S. aureus were provided by Statens Serum Institut and University of Copenhagen. Technical assistance of Katja Billekop Olsen was highly appreciated.
Supplementary Material
The Supplementary Material for this article can be found online at: https://www.frontiersin.org/articles/10.3389/fmicb.2021.733847/full#supplementary-material
Footnotes
- ^ http://rast.nmpdr.org/
- ^ https://cge.cbs.dtu.dk/services/ResFinder/
- ^ http://bagel4.molgenrug.nl/index.php
- ^ https://www.R-project.org/
References
Ali, S. M., and Yosipovitch, G. (2013). Skin pH: from basic science to basic skin care. Acta Derm. Venereol. 93, 261–267. doi: 10.2340/00015555-1531
Arena, M. P., Silvain, A., Normanno, G., Grieco, F., Drider, D., Spano, G., et al. (2016). Use of Lactobacillus plantarum strains as a bio-control strategy against food-borne pathogenic microorganisms. Front. Microbiol. 7:464. doi: 10.3389/fmicb.2016.00464
Arief, I. I., Budiman, C., Jenie, B. S. L., Andreas, E., and Yuneni, A. (2015). Plantaricin IIA-1A5 from Lactobacillus plantarum IIA-1A5 displays bactericidal activity against Staphylococcus aureus. Benef. Microbes 6, 603–613. doi: 10.3920/BM2014.0064
Barbieri, F., Montanari, C., Gardini, F., and Tabanelli, G. (2019). Biogenic amine production by lactic acid bacteria: a review. Foods 8:17. doi: 10.3390/FOODS8010017
Barbosa, M., Todorov, S. D., Ivanova, I. V., Belguesmia, Y., Choiset, Y., Rabesona, H., et al. (2016). Characterization of a two-peptide plantaricin produced by Lactobacillus plantarum MBSa4 isolated from Brazilian salami. Food Control 60, 103–112. doi: 10.1016/j.foodcont.2015.07.029
Blanchet-Réthoré, S., Bourdès, V., Mercenier, A., Haddar, C. H., Verhoeven, P. O., and Andres, P. (2017). Effect of a lotion containing the heat-treated probiotic strain Lactobacillus johnsonii NCC 533 on Staphylococcus aureus colonization in atopic dermatitis. Clin. Cosmet. Investig. Dermatol. 10, 249–257. doi: 10.2147/CCID.S135529
Bortolaia, V., Kaas, R. S., Ruppe, E., Roberts, M. C., Schwarz, S., Cattoir, V., et al. (2020). ResFinder 4.0 for predictions of phenotypes from genotypes. J. Antimicrob. Chemother. 75, 3491–3500. doi: 10.1093/jac/dkaa345
Butler, É, Lundqvist, C., and Axelsson, J. (2020). Lactobacillus reuteri DSM 17938 as a novel topical cosmetic ingredient: a proof of concept clinical study in adults with atopic dermatitis. Microoganisms 8:1026. doi: 10.3390/microorganisms8071026
Chu, D. M., Ma, J., Prince, A. L., Antony, K. M., Seferovic, M. D., and Aagaard, K. M. (2017). Maturation of the infant microbiome community structure and function across multiple body sites and in relation to mode of delivery. Nat. Med. 23, 314–326. doi: 10.1038/nm.4272
Cisar, J. O., Kolenbrander, P. E., and McIntire, F. C. (1979). Specificity of coaggregation reactions between human oral streptococci and strains of Actinomyces viscosus or Actinomyces naeslundii. Infect. Immun. 24, 742–752.
Clausen, M. D., Edslev, S. M., Nørreslet, L. B., Sørensen, J. A., Andersen, P. S., and Agner, T. (2019). Temporal variation of Staphylococcus aureus clonal complexes in atopic dermatitis: a follow-up study. Br. J. Dermatol. 180, 181–186. doi: 10.1111/bjd.17033
Clausen, M. L., Edslev, S. M., Andersen, P. S., Clemmensen, K., Krogfelt, K. A., and Agner, T. (2017). Staphylococcus aureus colonization in atopic eczema and its association with filaggrin gene mutations. Br. J. Dermatol. 177, 1394–1400. doi: 10.1111/bjd.15470
Coman, M. M., Verdenelli, M. C., Cecchini, C., Silvi, S., Orpianesi, C., Boyko, N., et al. (2014). In vitro evaluation of antimicrobial activity of Lactobacillus rhamnosus IMC 501®, Lactobacillus paracasei IMC 502® and SYNBIO® against pathogens. J. Appl. Microbiol. 117, 518–527. doi: 10.1111/jam.12544
Delanghe, L., Spacova, I., Malderen, J., Van, Oerlemans, E., and Claes, I. (2021). The role of lactobacilli in inhibiting skin pathogens. Biochem. Soc. Trans. 49, 617–627. doi: 10.1042/BST20200329
Diep, D. B., Håvarstein, L. S., and Nes, I. F. (1995). A bacteriocin-like peptide induces bacteriocin synthesis in Lactobacillus plantarum C11. Mol. Microbiol. 18, 631–639.
Diep, D. B., Straume, D., Kjos, M., Torres, C., and Nes, I. F. (2009). An overview of the mosaic bacteriocin pln loci from Lactobacillus plantarum. Peptides 30, 1562–1574. doi: 10.1016/j.peptides.2009.05.014
Edslev, S. M., Clausen, M.-L., Agner, T., Stegger, M., and Andersen, P. S. (2017). Genomic analysis reveals different mechanisms of fusidic acid resistance in Staphylococcus aureus from Danish atopic dermatitis patients. J. Antimicrob. Chemother. 73, 856–861. doi: 10.1093/jac/dkx481
Ekmekci, H., Aslim, B., and Ozturk, S. (2009). Characterization of vaginal Lactobacilli coaggregation ability with Escherichia coli. Microbiol. Immunol. 53, 59–65. doi: 10.1111/j.1348-0421.2009.00115.x
EFSA (2012). Guidance on the assessment of bacterial susceptibility to antimicrobials of human and veterinary importance. EFSA J. 10:2740. doi: 10.2903/j.efsa.2012.2740
Fredborg, M., Andersen, K. R., Jørgensen, E., Droce, A., Olesen, T., Jensen, B. B., et al. (2013). Real-time optical antimicrobial susceptibility testing. J. Clin. Microbiol. 51, 2047–2053. doi: 10.1128/JCM.00440-13
Geoghegan, J. A., Irvine, A. D., and Foster, T. J. (2018). Staphylococcus aureus and atopic dermatitis: a complex and evolving relationship. Trends Microbiol. 26, 484–497. doi: 10.1016/j.tim.2017.11.008
Goel, A., Halami, P. M., and Tamang, J. P. (2020). Genome analysis of Lactobacillus plantarum isolated from some indian fermented foods for bacteriocin production and probiotic marker genes. Front. Microbiol. 11:40. doi: 10.3389/fmicb.2020.00040
Gong, J. Q., Lin, L., Lin, T., Hao, F., Zeng, F. Q., Bi, Z. G., et al. (2006). Skin colonization by Staphylococcus aureus in patients with eczema and atopic dermatitis and relevant combined topical therapy: a double-blind multicentre randomized controlled trial. Br. J. Dermatol. 155, 680–687. doi: 10.1111/j.1365-2133.2006.07410.x
Grice, E. A., Kong, H. H., Conlan, S., Deming, C. B., Davis, J., Young, A. C., et al. (2009). Topographical and temporal diversity of the human skin microbiome. Science 324, 1190–1193.
Handley, P. S., Harty, D. W. S., Wyatt, J. E., Brown, C. R., Doran, J. P., and Gibbs, A. C. C. (1987). A comparison of the adhesion, coaggregation and cell-surface hydrophobicity properties of fibrillar and fimbriate strains of Streptococcus salivarius. J. Gen. Microbiol. 133, 3207–3217. doi: 10.1099/00221287-133-11-3207
Hill, C., Guarner, F., Reid, G., Gibson, G. R., Merenstein, D. J., Pot, B., et al. (2014). Expert consensus document: the international scientific association for probiotics and prebiotics consensus statement on the scope and appropriate use of the term probiotic. Nat. Rev. Gastroenterol. Hepatol. 11, 506–514. doi: 10.1038/nrgastro.2014.66
Irvine, A. D., McLean, W. H. I., and Leung, D. Y. M. (2011). Filaggrin mutations associated with skin and allergic diseases. N. Engl. J. Med. 365, 1315–1327. doi: 10.1056/NEJMra1011040
Iwamoto, K., Moriwaki, M., Miyake, R., and Hide, M. (2019). Staphylococcus aureus in atopic dermatitis: strain-specific cell wall proteins and skin immunity. Allergol. Int. 68, 309–315. doi: 10.1016/j.alit.2019.02.006
Iwamoto, K., Moriwaki, M., Niitsu, Y., Saino, M., Takahagi, S., Hisatsune, J., et al. (2017). Staphylococcus aureus from atopic dermatitis skin alters cytokine production triggered by monocyte-derived Langerhans cell. J. Dermatol. Sci. 88, 271–279. doi: 10.1016/j.jdermsci.2017.08.001
Jayashree, S., Karthikeyan, R., Nithyalakshmi, S., Ranjani, J., Gunasekaran, P., and Rajendhran, J. (2018). Anti-adhesion property of the potential probiotic strain Lactobacillus fermentum 8711 against methicillin-resistant Staphylococcus aureus (MRSA). Front. Microbiol. 9:411. doi: 10.3389/fmicb.2018.00411
Jett, B. D., Hatter, K. L., Huycke, M. M., and Gilmore, M. S. (1997). Simplified agar plate method for quantifying viable bacteria. Biotechniques 23, 648–650. doi: 10.2144/97234bm22
Lee, N. R., Lee, H. J., Yoon, N. Y., Kim, D., Jung, M., and Choi, E. H. (2016). Application of topical acids improves atopic dermatitis in murine model by enhancement of skin barrier functions regardless of the origin of acids. Ann. Dermatol. 28, 690–696. doi: 10.5021/ad.2016.28.6.690
Lukic, J., Chen, V., Strahinic, I., Begovic, J., Lev-Tov, H., Davis, S. C., et al. (2017). Probiotics or pro-healers: the role of beneficial bacteria in tissue repair. Wound Repair Regen. 25, 912–922. doi: 10.1111/wrr.12607
Marshall, V. M. (1979). A note on screening hydrogen peroxide-producing lactic acid bacteria using a non-toxic chromogen. J. Appl. Bacteriol. 47, 327–328. doi: 10.1111/j.1365-2672.1979.tb01762.x
Miajlovic, H., Fallon, P. G., Irvine, A. D., and Foster, T. J. (2010). Effect of filaggrin breakdown products on growth of and protein expression by Staphylococcus aureus. J. Allergy Clin. Immunol. 126, 1184–1190.e3. doi: 10.1016/j.jaci.2010.09.015
Musa, A., Wiman, E., Selegård, R., Aili, D., Bengtsson, T., and Khalaf, H. (2021). Plantaricin NC8 αβ prevents Staphylococcus aureus-mediated cytotoxicity and inflammatory responses of human keratinocytes. Sci. Rep. 11:12514.
Myles, I. A., Earland, N. J., Anderson, E. D., Moore, I. N., Kieh, M. D., Williams, K. W., et al. (2018). First-in-human topical microbiome transplantation with Roseomonas mucosa for atopic dermatitis. JCI Insight 3:e120608. doi: 10.1172/jci.insight.120608
Nakatsuji, T., Hata, T. R., Tong, Y., Cheng, J. Y., Shafiq, F., Butcher, A. M., et al. (2021). Development of a human skin commensal microbe for bacteriotherapy of atopic dermatitis and use in a phase 1 randomized clinical trial. Nat. Med. 27, 700–709. doi: 10.1038/s41591-021-01256-2
Nes, I. F., Diep, D. B., Håvarstein, L. S., Brurberg, M. B., Eijsink, V., and Holo, H. (1996). Biosynthesis of bacteriocins in lactic acid bacteria. Antonie Van Leeuwenhoek 70, 113–128. doi: 10.1007/BF00395929
Oldak, A., Zielinska, D., Rzepkowska, A., and Kolozyn-Krajewska, D. (2017). Comparison of antibacterial activity of Lactobacillus plantarum strains isolated from two different kinds of regional cheeses from poland: oscypek and korycinski cheese. Biomed. Res. Int. 2017:6820369. doi: 10.1155/2017/6820369
Panther, D., and Jacob, S. (2015). The importance of acidification in atopic eczema: an underexplored avenue for treatment. J. Clin. Med. 4, 970–978. doi: 10.3390/jcm4050970
Park, B., Hwang, H., Chang, J. Y., Hong, S. W., Lee, S. H., Young, M., et al. (2017). Identification of 2-hydroxyisocaproic acid production in lactic acid bacteria and evaluation of microbial dynamics during kimchi ripening. Sci. Rep. 7:10904. doi: 10.1038/s41598-017-10948-0
R Core Team (2020). R: A Language and Environment for Statistical Computing. Vienna: R Foundation for Statistical Computing. Available online at: https://www.R-project.org/
Selegård, R., Musa, A., Nyström, P., Aili, D., Bengtsson, T., and Khalaf, H. (2019). Plantaricins markedly enhance the effects of traditional antibiotics against Staphylococcus epidermidis. Future Microbiol. 14, 195–205. doi: 10.2217/fmb-2018-0285
Siedler, S., Rau, M. H., Bidstrup, S., Vento, J. M., Aunsbjerg, S. D., Bosma, E. F., et al. (2020). ‘Competitive exclusion is a major bioprotective mechanism of Lactobacilli against fungal spoilage in fermented milk products’. Appl. Environ. Microbiol. 86, e2312–e2319. doi: 10.1128/AEM.02312-19
Spacova, I., Neill, C. O., and Lebeer, S. (2020). Lacticaseibacillus rhamnosus GG inhibits infection of human keratinocytes by Staphylococcus aureus through mechanisms involving cell surface molecules and pH reduction. Benef. Microbes 11, 703–715. doi: 10.3920/BM2020.0075
Tetili, F., Bendali, F., Perrier, J., and Sadoun, D. (2017). Anti-staphylococcal enterotoxinogenesis of Lactococcus lactis in algerian raw milk cheese. Food Technol. Biotechnol. 55, 511–518. doi: 10.17113/ftb.55.04.17.5105
The Convention on Biological Diversity (2011). The Nagoya Protocol on Access and Benefit-sharing. Available online at: https://www.cbd.int/abs/ (accessed March 25, 2021).
Totté, J. E. E., van der Feltz, W. T., Hennekam, M., van Belkum, A., van Zuuren, E. J., and Pasmans, S. G. M. A. (2016). Prevalence and odds of Staphylococcus aureus carriage in atopic dermatitis: a systematic review and meta-analysis. Br. J. Dermatol. 175, 687–695. doi: 10.1111/bjd.14566
Van Heel, A. J., De Jong, A., Song, C., Viel, J. H., Kok, J., and Kuipers, O. P. (2018). BAGEL4: a user-friendly web server to thoroughly mine RiPPs and bacteriocins. Nucleic Acids Res. 46, W278–W281. doi: 10.1093/nar/gky383
Yoon, S. H., Ha, S. M., Kwon, S., Lim, J., Kim, Y., Seo, H., et al. (2017). Introducing EzBioCloud: a taxonomically united database of 16S rRNA and whole genome assemblies. Int. J. Syst. Evol. Microbiol. 67, 1613–1617. doi: 10.1099/ijsem.0.001755
Younes, J. A., Reid, G., van der Mei, H. C., and Busscher, H. J. (2016). Lactobacilli require physical contact to reduce staphylococcal TSST-1 secretion and vaginal epithelial inflammatory response. Pathog. Dis. 74:ftw029. doi: 10.1093/femspd/ftw029
Keywords: lactobacilli, antimicrobial compounds, inhibitory activity, skin, atopic dermatitis, Staphylococcus aureus
Citation: Christensen IB, Vedel C, Clausen M-L, Kjærulff S, Agner T and Nielsen DS (2021) Targeted Screening of Lactic Acid Bacteria With Antibacterial Activity Toward Staphylococcus aureus Clonal Complex Type 1 Associated With Atopic Dermatitis. Front. Microbiol. 12:733847. doi: 10.3389/fmicb.2021.733847
Received: 30 June 2021; Accepted: 23 August 2021;
Published: 17 September 2021.
Edited by:
Santi M. Mandal, Indian Institute of Technology Kharagpur, IndiaReviewed by:
Noraznawati Ismail, Universiti Malaysia Terengganu, MalaysiaDorota Zielińska, Warsaw University of Life Sciences, Poland
Takeshi Zendo, Kyushu University, Japan
Copyright © 2021 Christensen, Vedel, Clausen, Kjærulff, Agner and Nielsen. This is an open-access article distributed under the terms of the Creative Commons Attribution License (CC BY). The use, distribution or reproduction in other forums is permitted, provided the original author(s) and the copyright owner(s) are credited and that the original publication in this journal is cited, in accordance with accepted academic practice. No use, distribution or reproduction is permitted which does not comply with these terms.
*Correspondence: Ida B. Christensen, ic@lactobio.com