- 1Shandong Provincial Research Center for Bioinformatic Engineering and Technique, School of Life Sciences and Medicine, Shandong University of Technology, Zibo, China
- 2Zibo Key Laboratory of Precise Gene Detection, Zibo, China
- 3State Key Laboratory of Infectious Disease Prevention and Control, National Institute for Viral Disease Control and Prevention, Chinese Center for Disease Control and Prevention, Beijing, China
The untranslated region (UTRs) of viral genome are important for viral replication and immune modulation. Japanese encephalitis virus (JEV) is the most significant cause of epidemic encephalitis worldwide. However, little is known regarding the characterization of the JEV UTRs. Here, systematic analyses of the UTRs of JEVs isolated from a variety of hosts worldwide spanning about 80 years were made. All the important cis-acting elements and structures were compared with other mosquito-borne Flaviviruses [West Nile virus (WNV), Yellow fever virus (YFV), Zika virus (ZIKV), Dengue virus (DENV)] and annotated in detail in the UTRs of different JEV genotypes. Our findings identified the JEV-specific structure and the sequence motif with unique JEV feature. (i) The 3’ dbsHP was identified as a small hairpin located in the DB region in the 3′ UTR of JEV, with the structure highly conserved among the JEV genotypes. (ii) The spacer sequence UARs of JEV consist of four discrete spacer sequences, whereas the UARs of other mosquito-borne Flaviviruses are continuous sequences. In addition, repetitive elements have been discovered in the UTRs of mosquito-borne Flaviviruses. The lengths, locations, and numbers of the repetitive elements of JEV also differed from other Flaviviruses (WNV, YFV, ZIKV, DENV). A 300 nt-length region located at the beginning of the 3′ UTR exhibited significant genotypic specificity. This study lays the basis for future research on the relationships between the JEV specific structures and elements in the UTRs, and their important biological function.
Introduction
Flaviviruses are enveloped viruses with 11 kb, positive-sense single-stranded RNA genome containing highly structured 5′ and 3′ untranslated region (UTRs) between which lies a single open reading frame (ORF) encoding three structural proteins (C-prM-E) and seven non-structural (NS) proteins (NS1, NS2A, NS2B, NS3, NS4A, NS4B, NS5) (Sumiyoshi et al., 1987; Liu and Qin, 2020). The NS proteins interact with certain cellular factors to form the viral replicase complex that directs the replication of the genomic RNA (Lindenbach et al., 2007; Garcia-Blanco et al., 2016; Wang et al., 2017; Sanford et al., 2019). The genome cyclizes so that a single minus strand can be synthesized with stem loop A (SLA) functioning to recruit the RNA dependent RNA polymerase (RdRp). The minus strand in the resulting double-strand serves as a template for multiple reinitiations of genome RNAs (Westaway et al., 2003; Lindenbach et al., 2007). Within the 5′ UTR, there are several conserved stem-loop (SL) structures. These include (i) SLA, which serves as a viral polymerase binding site (Filomatori et al., 2006); and (ii) Stem-loop B (SLB), which contains the upstream of AUG region (5′ UAR) (Alvarez et al., 2005) and 5′-UAR-flanking stem (UFS) (Liu et al., 2016) are involved in long-range RNA-RNA interactions and genome replication. The capsid-coding region hairpin (cHP) element lies within the coding region and aids in start codon recognition and viral replication (Clyde et al., 2008). The 5′ UAR along with 5′ downstream AUG region (DAR) (Friebe et al., 2011) and the 5′ cyclization sequence (5′ cCS) (Villordo and Gamarnik, 2009; Gebhard et al., 2011) cause genome circularization by hybridizing with their counterparts in the 3′ UTR (i.e., 3′ UAR, 3′ DAR, and 3′ cCS) (Hahn et al., 1987; Hodge et al., 2019). Besides the viral genome cyclization-related sequences, 3′ UTR has a panel of stem-loop structures that halt XRN1 exoribonuclease digestion. This results in the synthesis of sets of subgenomic flaviviral RNA (sfRNA) (Pijlman et al., 2008) which are associated with viral pathogenicity, host adaption, and immune evasion and immune evasion (Moon et al., 2012; Chapman et al., 2014; Filomatori et al., 2017).
The 5′ and 3′UTRs of the Flavivirus have been shown to be crucial for virus replication, immune regulation and pathogenicity (Brinton et al., 1986; Moon et al., 2012; Chang et al., 2013; Kieft et al., 2015). However, the studies of cis-acting elements in the UTRs have been mainly focused on Dengue virus (DENV), Yellow fever virus (YFV), West Nile virus (WNV), Tick-borne encephalitis virus (TBEV), and Zika virus (ZIKV) (Zeng et al., 2020). Although Japanese encephalitis virus (JEV) is arguably the most significant pathogen causing viral encephalitis within the Flaviviruses, few studies have been conducted to explore the functions and features of JEV UTRs. As a result, little is known about the primary and higher-order structures of JEV. Whether all of the functional important cis-acting elements that exist in the JEV UTRs share some features with the other Flaviviruses or whether JEV has some specific features has yet to be understood. It is largely unknown what the accurate locations and the sequence features of these important elements in JEV UTRs are.
Japanese encephalitis virus have evolved into five genetically different lineages in nature, diverged in the chronological order of GV, GIV, GIII, GII, and GI (Gao et al., 2015). JEV was initially isolated from viral encephalitis patients in 1935 in Japan and subsequently this strain was identified to be JEV GIII (Lewis et al., 1947). GIII has been the predominant genotype causing human and animal diseases in JE endemic areas until the late 20th century. In recent years, most JEV strains isolated from mosquito vectors, pigs, and humans in Asia belong to GI, thus replacing GIII as the dominant genotype in the Asian continent (Pan et al., 2011). GII primarily circulates in southeast Asia and northern Australia (Williams et al., 2000; Schuh et al., 2010), whereas GIV and GV are mainly confined to the tropical regions of Southeast Asia (Li et al., 2011; Kuwata et al., 2020; Woo et al., 2020). Both the inactivated vaccine (Nakayama) and the attenuated live vaccine (SA14-14-2) currently used for JE vaccination are derived from GIII (Ni et al., 1994; Halstead and Jacobsen, 2008; Erlanger et al., 2009). However, a growing number of reports suggest that these vaccines do not provide complete protection against GI and GV virus strains (Cao et al., 2016; Hegde and Gore, 2017). Collectively, these reports suggest that viral evolution, transmission ability, pathogenicity, and immunogenicity differ among JEV genotypes. Previous JEV studies were mainly focused on the protein-coding sequences, neglecting the study of the UTRs region. The differences in the UTRs among the JEV genotypes are poorly understood. In this study, a comprehensive study of 160 JEV strains including 67 isolates sequenced in our lab was conducted on the JEV UTRs. The results will assist our understanding of the role of UTRs in the transcription, replication and pathogenicity of JEV.
Materials and Methods
The Japanese Encephalitis Virus Untranslated Regions Data Analysis Pipeline
The following analyses were carried out in this work to investigate the primary sequence and secondary structural similarity and differences between representative Flaviviruses (WNV, YFV, ZIKV, DENV) and within the five genotypes of JEV UTRs. First, the JEV whole-genome sequence dataset was constructed, including 67 strains sequenced in our lab and 97 strains obtained from the NCBI database. Sequence inclusion criteria are described in the dataset construction section. The whole JEV genome dataset was then split into three parts to form the 5′UTR, ORF, and 3′UTR gene datasets. Meanwhile, the UTRs sequences of the representative Flaviviruses (WNV, YFV, ZIKV, DENV) were also downloaded as reference sequences for cis-acting element annotation and repeat sequences analysis. The general sequence feature analysis, primary sequence analysis, and higher-level structure analysis were performed using the JEV UTRs sequence datasets. The primary sequence analysis entailed sequence similarity, nucleotide composition, and repeat sequence analysis. The higher-level structural analysis comprised of the secondary structure and cis-acting elements annotation. Through these analyses, some genotypic sequence or structure features could be identified amongst the five JEV genotypes, if any. Such regions would then be selected for phylogenetic analysis. The software used in the analyses is listed in the flow chart of the JEV UTRs data analysis pipeline (Figure 1). The Supplementary Data 2 contains the detailed parameter settings utilized in each software as well as the analysis scripts. The datasets, sequence alignments, and the software used in the current study are available from the corresponding authors upon request.
The Japanese Encephalitis Virus Untranslated Regions Datasets Construction
In order to understand the sequence and structural features of the UTRs of JEV, the nucleotide sequences of the 5′ and 3′UTRs of JEVs were downloaded from GenBank as of January 2020. The complete UTRs sequences with clear background information including location, data, origin (vector or host) were selected, we excluded all missing at least one of the three metadata.
The Japanese Encephalitis Virus Untranslated Regions’ Sequence Analysis
The sequence analysis of the UTRs of the 160 JEV strains was conducted to explore the genetic features among the different JEV genotypes. First, CLUSTALW software (Thompson et al., 1997) was used to align the nucleotide sequences of the UTRs of JEV. The BioEdit and GeneDoc software were then used to perform sequence editing and nucleotide difference analysis in UTRs. The MegAlign incorporated in DNAStar software (Burland, 2000) was used to compute the sequence distance between different strains to generate the similarity matrix. The Mega-X program (Kumar et al., 2018) was used to analyze the base composition of the nucleotide sequences of the JEV UTRs. A scatter plot was then generated with ggplot2 package in RStudio1 to show the proportion of bases (A, U, G, C) of each strain in different genotypes. The statistical analyses were conducted using SPSS software.
The Repeat Sequences Analysis of Japanese Encephalitis Virus and Representative Mosquito-Borne Flaviviruses
The UTR sequence alignments were generated using the CLUSTALW software. The GenDoc program was subsequently employed to extract the consensus sequences from the alignments. To determine whether there are repetitive sequences located in the UTRs of representative arboviral Flaviviruses (WNV, YFV, ZIKV, DENV) were also downloaded from GenBank for analysis. The NovoPro online tool that is based on the k-mer algorithm (Lerat, 2010) was used to search repeat sequences in the viral UTRs sequences. The core number setting of the minimum repeat sequence followed three principles: (1) The repetitive sequence unit should be obtained; (2) The longest repetitive sequence should be obtained; (3) There should be no overlap between two or more repetitive sequence units. Based on the three principles, 5 and 8 were the best minimum repeat sequence core numbers for the 5′ and 3′ UTRs, respectively. The IBS 1.0.3 software (Liu et al., 2015) was used for results visualization.
Higher-Order Structure Analysis of the Untranslated Regions of Japanese Encephalitis Virus
Prediction of the JEV UTR secondary structure was done using the Mfold software v 3.6 (Zuker, 2003). The parameters used included a folding temperature of 37°C, an ionic condition of 1M NaCl with no divalent ions, and a 5% suboptimality. The upper bound of the number of computed folding and the maximum upper bound of the total number of single-stranded bases allowed in a bulge or interior loop were set at 25. The other parameters were set at default, and the initial ΔG was selected as the smallest structure to obtain the Vienna format file. The representative Flaviviruses’ (WNV and YFV) genome sequences with confirmed UTR secondary structures were used as references to validate the parameter settings. Taken the representative Flaviviruses as reference, a 50 nt-length sequence located after the start codon within the ORF that forms secondary structures, which are essential for genome cyclization, was also included in the present analysis. The VARNA program (Darty et al., 2009) was finally used for visualization of the UTR secondary structure.
The Phylogenetic Analysis Based on the Japanese Encephalitis Virus Untranslated Regions
According to results of sequence alignment of 3′UTRs, it is clear that the 3′ variable region (VR) of JEV 3′UTR, which comprises 300 nt, exhibits clear genotype-specific features. Thus, this region was selected to conduct the phylogenetic analysis. Phylogenetic analyses based on the 5′ and 3′UTRs, as well as on the 3′VR sequences, were all performed using both Maximun Likehood (ML) and Neighbor-Joining (NJ) methods within MEGAX software. The best-fit substitution model of each dataset was estimated using ModelFinder (Kalyaanamoorthy et al., 2017) incorporated in the PhyloSuite software (Zhang et al., 2020). In order to verify the consistency of the phylogenetic trees generated using different gene regions, the phylogenetic tree were also generated using ORF, preM and E gene sequences. The topology of the phylogenetic trees based on the different gene datasets were compared using the Robinson–Foulds (RF) metric (Robinson and Foulds, 1981; Smith, 2020) to validate the accuracy of JEV genotyping based on the VR sequences. The ML and NJ trees were constructed with 1000 bootstrap replicates.
Results
The Japanese Encephalitis Virus Untranslated Regions Datasets Construction
After removing the sequence with insufficient background information and incomplete nucleotides, the sequences of 93 JEV strains were selected, together with the sequences of 67 strains from our lab, to form a final whole-genome sequence dataset containing 160 JEV strains. Then the JEV whole-genome sequence dataset was split into three parts to form the 5′ UTR, ORF, and 3′ UTR gene datasets. The 160 JEV strains are representing the samples isolated from kinds of mosquitoes (n = 82)with Culex tritaeniorhynchus as the major species (n = 46), midges (n = 2), bats (n = 6), pigs (n = 29), horses (n = 2), and humans (n = 38). The isolation dates ranged from 1935 to 2018. The isolation sites including China (n = 121), Japan (n = 17), India (n = 7), South Korea (n = 4), South Korea (n = 2), Vietnam (n = 1), Laos (n = 1), Australia (n = 1), Angola (n = 1), Indonesia (n = 1), Malaysia (n = 1), Singapore (n = 1), and Philippines (n = 1). The geographical distribution extends from latitude 15°S to latitude 45°N. The 160 strains of JEV belongs to five genotypes: GI (n = 78), GII (n = 1), GIII (n = 77), GIV (n = 1), and GV (n = 3) (detailed information in Supplementary Table 1).
The Sequence Analyses of the Japanese Encephalitis Virus Untranslated Regions
Both of the 5′ and 3′ UTRs sequences were compared and analyzed using CLUSTALW and GenDoc software. It is found that the length of the 5′ UTR region of JEV is 95 nt (only GI is 96 nt). The 5′ UTRs are more conserved compared with the 3′ UTRs. There are nucleotide differences that exist in both the 5′ and 3′ UTRs among the different genotypes (Tables 1–3 and Supplementary Table 1). The 3′UTR ranges in length from 557 to 596 nt, and the nucleotide sequence of the 3′UTRs is more variable than that of the 5′UTRs. There is a 300 nt-length region, starting from the stop codon, that is the most variable and exhibits a clear genotype specificity (Tables 1, 3). In JEV and other arboviral Flaviviruses, the 5′UTR initiates with an adenine (A) that is immediately connected with the 5′cap structure (m7GpppAmpN) and terminates with the CU. However, we identified that four out of 160 JEV strains were not terminated with CU.
The Nucleotide Similarity and Composition of the Japanese Encephalitis Virus Untranslated Regions
The nucleotide similarity analysis for the 5′ and 3′UTRs of 160 JEV strains isolated from different vectors, hosts and locations during 1938–2018 revealed that the 5′UTRs exhibit a nucleotide similarity ranges from 84.5 to 100%, with an average similarity of μ = 97.62. The nucleotide similarity of GI and GIII are 90.7–100% and 95.9–100%, respectively. The 3′UTRs exhibit nucleotide similarity ranges from 79.3% to 100%, with a μ = 94.47%. The nucleotide similarity of GI and GIII are 95.5–100% and 88.6–100%, respectively. The detailed similarity of the 5′ and 3′UTRs for each JEV genotype are presented (Tables 1, 4). The JEV 5′UTRs are rich in AT (A + T content reaches 62%), and the 3′UTRs are rich in GC dinucleotides (A + T content is 47%) (Figure 2 and Table 1).
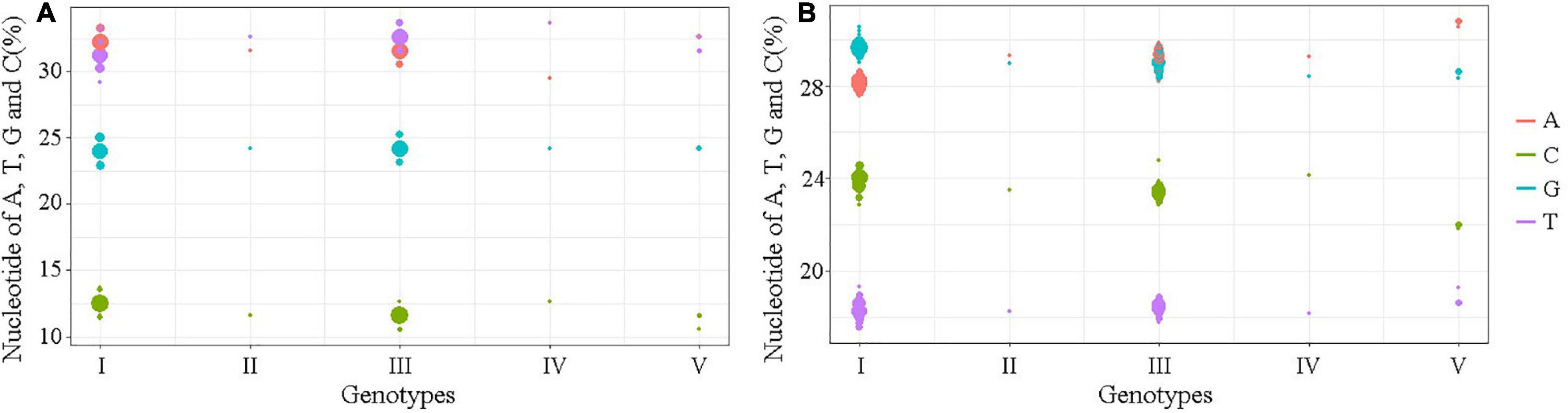
Figure 2. The A, T, G, and C contents of the UTRs of different JEV genotypes. (A) The nucleotide contents of the 5′UTRs. (B) The nucleotide contents of the 3′UTRs. The abscissa indicates JEV genotypes and the ordinate represents the percentage of nucleotide contents. The red bar identifies adenine (A), green identifies cytosine (C), blue identifies guanine (G) and violet identifies thymine (T).
The Repeat Sequences in the Japanese Encephalitis Virus Untranslated Regions
The repeat sequences in the JEV UTRs were analyzed using the NovoPro online tool and IBS 1.0.3 software. We found that both the JEV 5′ and 3′UTRs consist of different numbers of repeat sequence elements. The numbers, locations and nucleotide sequence composition of the repeat units of the 5′ and 3′UTRs are different among the five JEV genotypes. The detailed information is shown in Figure 3 and Table 1.
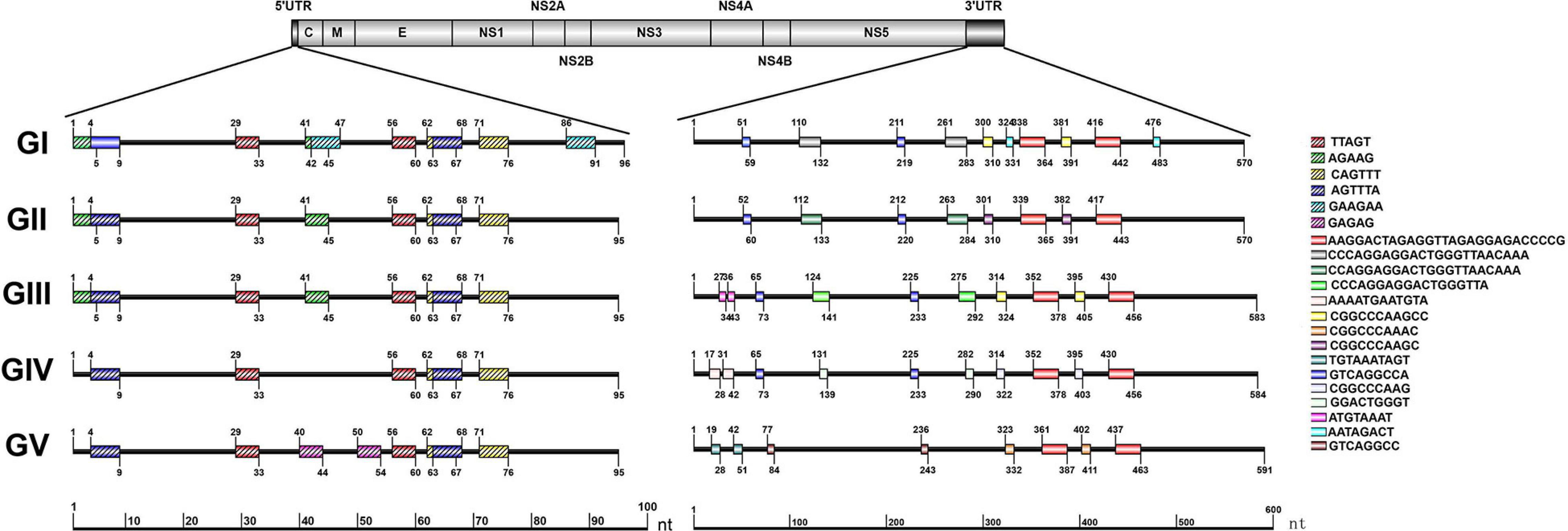
Figure 3. Schematic localization of the repeat sequences in UTRs of different genotypes of JEV. Different color boxes indicate different repeat sequence units. The start and end positions of the repeat sequence unit is labeled upward and downward lines, respectively.
The Repeat Sequences in Representative Mosquito-Borne Flaviviruses
The repeat sequences identification in representative mosquito-borne Flaviviruses including WNV, YFV, ZIKV, and DENV were conducted using the NovoPro online tool and IBS 1.0.3 software. The results showed that the repeat sequence elements exist in the 5′ and 3′UTRs of all the analyzed arboviral Flaviviruses. There are clearly differences in the length, number, position, and nucleotide sequence composition among the different arboviral Flaviviruses. The detailed information is shown in Figure 4.
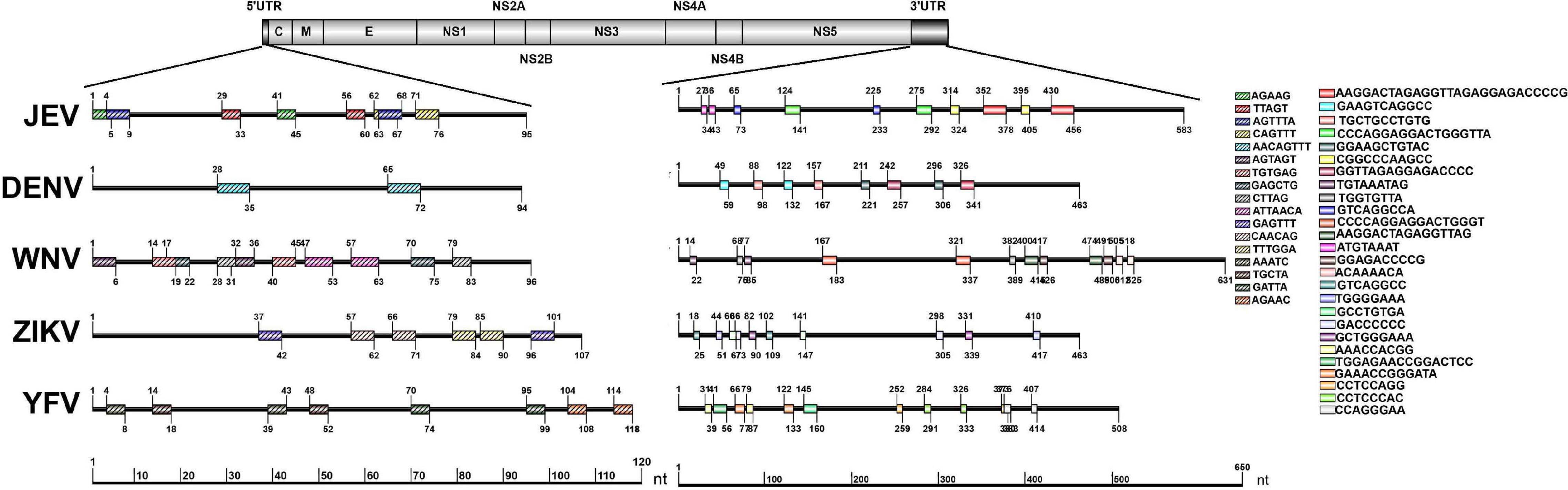
Figure 4. Schematic localization of the repeat sequences in UTRs of medically important Flaviviruses. Different color boxes indicate different repeat sequence units. The start and end positions of the repeat sequence unit is labeled upward and downward lines, respectively.
The Secondary Structures and Important Functional Sequences in the Japanese Encephalitis Virus 5′ Termini
As shown in Figure 5 and Table 1, the 5′UTRs consist of two stem-loop structures termed SLA and SLB. Within the SLB lies the UAR and the UFS. There are three elements sited after the ATG start codon within the capsid-coding region, termed the DAR, the cHP and the 5′cCS. Except for GIV, the secondary structures of the JEV 5′UTR are conserved among the five JEV genotypes. The 5′UTRs start with an adenine (A) connecting to the 5′ cap structure (m7GpppAmpA), directly linked to the SLA. SLA has a conserved structure with a length of about 70 nucleotides. GIV has a unique secondary structure in that instead of a direct connection between the 5′ cap and SLA, a U-rich sequence is inserted. Furthermore, the morphology of SLA of GIV differs from those of other genotypes.
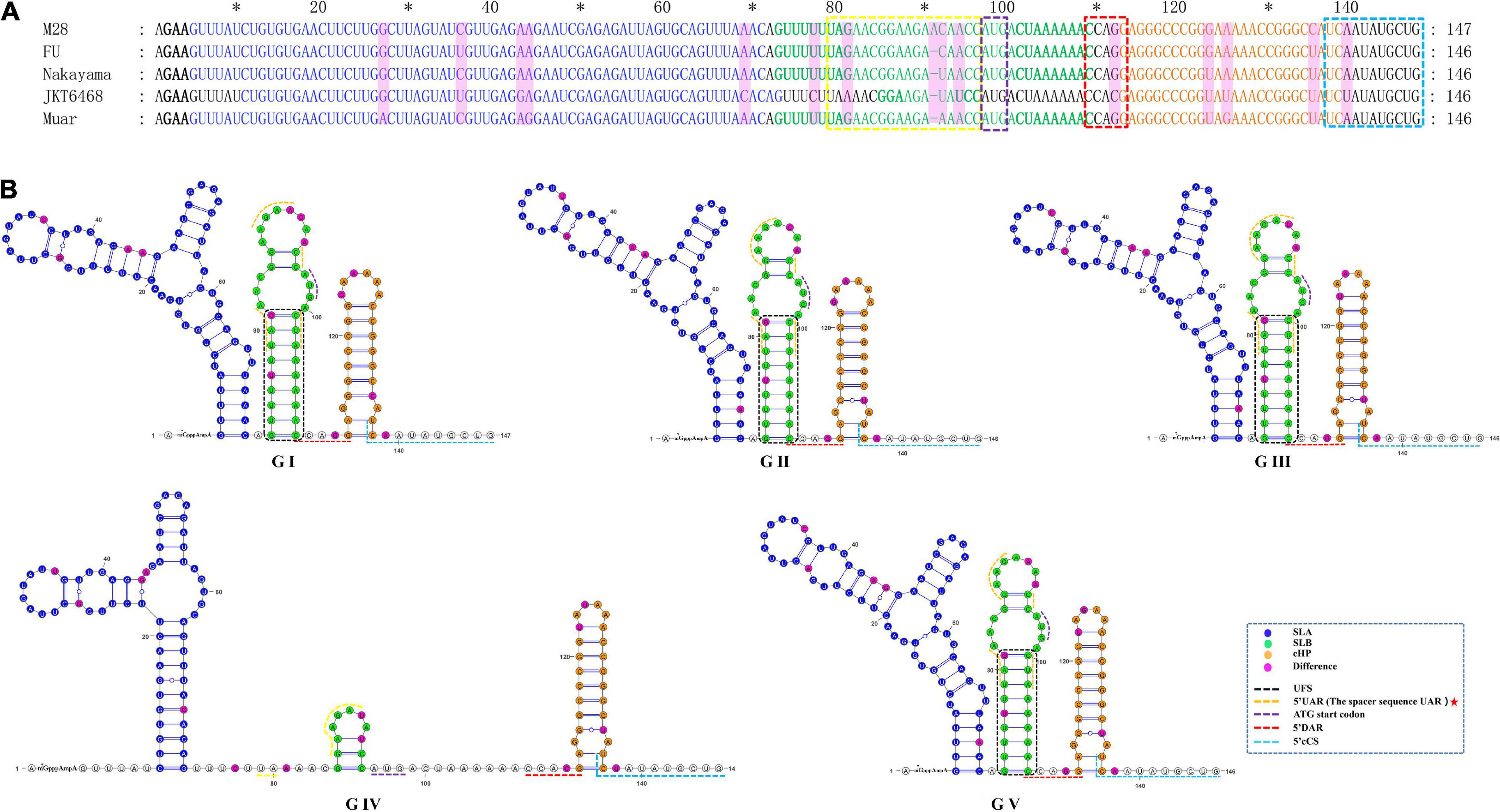
Figure 5. The primary and higher-order structures of the 5′ UTRs of JEV. (A) The primary sequences of the JEV 5′ UTRs. (B) The higher order structures of the JEV 5′ UTRs. Differently colored nucleotide bases signify different cis-acting elements or significant units: bold black, 5′ Cap (m7GpppAmpA); blue, the stem-loop A (SLA); green, the stem-loop B (SLB); yellow box, the upstream AUG region (5′ UAR); blue box, the ATG start codon; bold green, the 5′ UAR-flanking stem (UFS); orange, the capsid-coding region hairpin element (cHP); red box, the 5′ downstream AUG region (5′ DAR); aquamarine, 5′ cyclization sequence (5′cCS). The mutation sites are colored magenta. The mentioned units are colored similarly in the higher order structures. Black dashed box, yellow dashed lines and red dashed lines, respectively, denote the UFS, 5′ URA, and 5′ DAR, which were overlapped with other structures and sequences. The red pentagram represents the sequence motif with unique JEV feature.
SLB sits closest to the SLA, and contains UFS and 5′UAR. The SLB starting sequence (5′-GUUUUU-3′) and the sequence within the coding region (4 nucleotides behind the start codon, 5′-AAAAAC-3′) are reversely complementary, which form a stem of 6 base pairs in length, termed the 5′UAR-flanking stem (UFS) (Figure 5). An 11 nt-length bulge structure is connected to the JEV UFS structure (stem), which includes both the 6 nt-length 5′UTR sequence and the 5 nt-length sequence from the start codon of the capsid protein-coding sequence (5′-ATGAC-3′). Among all the medically important arboviral Flaviviruses (DENV 1-4, YFV, ZIKA, WNV, TBEV), only DENV 4 and ZIKV have this bulge structure, but it lacks an ATG start codon. This structural feature is unique in the JEV 5′ termini. The UAR sequence is located within the top loop of SLB, and the size and nucleotide composition of the top loop structures are different among the five JEV genotypes.
There is a clear difference that exists in the loop structure in JEV GI, due to the adenine insertion at position 92, as well as a mutation (93 T > C), resulting in the top loop structure change of SLB. The UARs of JEV are not continuous sequences but consists of four interspaced sequences (Denoted by yellow dashed lines in Figure 5). When compared to other mosquito-borne Flaviviruses (WNV, YFV, ZIKV, DENV), which have continuous UARs, the feature of JEV sequence is unique. We defined the JEV’s UARs as spacer sequence UAR. The 5’ DAR is located downstream of SLB, within the linker of SLB and cHP (Denoted by red dashed lines in Figure 5). It is conserved among JEV genotypes. The cHP contains a CG-rich stem and an A-rich top loop. The cHP’s loop structure of GI is larger than GIII. The 5′ cCS is an 11nt long conserved sequence motif within JEV genotypes and is located downstream the cHP.(5′ cCS:5′-TCA ATATGCTG-3′). Except for JEV GIV, the cCS sequences of the other four JEV genotypes differ only in the position of the starting codon. The JEV GIV’s cCS sequence is 5′-TCTATATGCTG-3′, located 39 nt downstream of the ATG start site. There is a mutation (A > T) at the third position within the cCS sequence (Figure 5).
The Secondary Structures and Important Functional Sequences in the Japanese Encephalitis Virus 3’UTR
We found the 3′UTR of JEV consists of three domains, termed domain I, II, III (Figure 6). Domain I comprised approximately 300 nt, which is immediately downstream of the stop codon, and is highly variable and termed the variable region (VR). The VR contains a very high variable region (VVR) and three stem-loop stem structures. The VVR ranges from 48 to 74 nt in length and is enriched in AU dinucleotides. The secondary structures of the VVR of the different JEV genotypes are significantly different (Figure 6). There are two SL structures that are resistant to host 5′-3′exoribonuclease (XRN1) activity, termed xrRNA1 and xrRNA2, which are conserved in the secondary structure of the five JEV genotypes. In xrRNA1, a three-nucleotide spacer sequence (5′-C-G-G-3′) located in the long-arm top loop pairs with a reverse complementary spacer sequence (5′-C-C-G-3′) that lies in the stem structure to form the pseudoknot (PK) PK1 (Figure 6, labeled with black dashed line). In xrRNA2, a three-nucleotide sequence (5′-GCU-3′) located in the long-arm top loop pairs with a 3-nucleotide sequence (5′-AGC-3′) that lies in the linear sequences between the SL structures to form PK2. The xrRNA1 and xrRNA2 structures of JEV are separated by a long SL structure, which is 73 nt in length. The structure is moderately conserved in the different JEV genotypes, and we named it the 3′UTR variable region stem loop (3′vrSL). There is a 25 nt-length conserved sequence, termed the 3′UTR variable region conserved sequences 1(3′vrCSl), which lies at the top loop of the 3′vrSL. A short SL structure with a length of approximately 11 nt is located after the xrRNA2 structure. The stem structures of the short SL structure are highly conserved in the five JEV genotypes, whereas the loop sequences are divergent. For genotypes I-III, the loop sequences are conserved (5′-GUUGA-3′), and for GIV and GV, the sequences are 5′-CAUGA-3′ and 5′-GAAAA-3′, respectively (Figure 6). Domain II consists of two dumbbell structures (DB1, DB2) and a 15 nt-length small hairpin structure. Theses structures are highly conserved among the five JEV genotypes. After searching the 3′UTRs of other mosquito-borne Flavivirus (WNV, YFV, ZIKV, and DENV), it is found that the 15 nt-length small hairpin structure could only be identified in the DB region in 3′UTRs of JEV. Thus, we named the it the 3′UTR DB region small hairpin (3′dbsHP). The long-arm top loop of DB1 (5′-GAUGCAA-3′) pairs with a reverse complementary sequence (5′-UUGCAUC-3′), which lies in the 3′dbsHP, to form PK3. The long-arm top loop of DB2 (5′-GCUGU-3′) pairs with a reverse complementary sequence (5′-ACAGC-3′) in the 3′dbsHP to form PK4 (Figure 6). The two PKs serve to further stabilize the DB structures. Besides the PKs located in the 3′dbsHP, partial sequences of 3′cCS sequence (5′-CAG CAU AUU GA-3′) overlaps the 3′dbsHP (Figure 6, bright pink dashed box). In the internal loop and the short arm structure of both DB1 and DB2, there are 27 nt-length repeated conserved sequences (-AAGGACUAGAGGUUUAGAGGA GACCCG-), designated the 3′UTR dumbbell structure repeated conserved sequences (3′dbRCS).
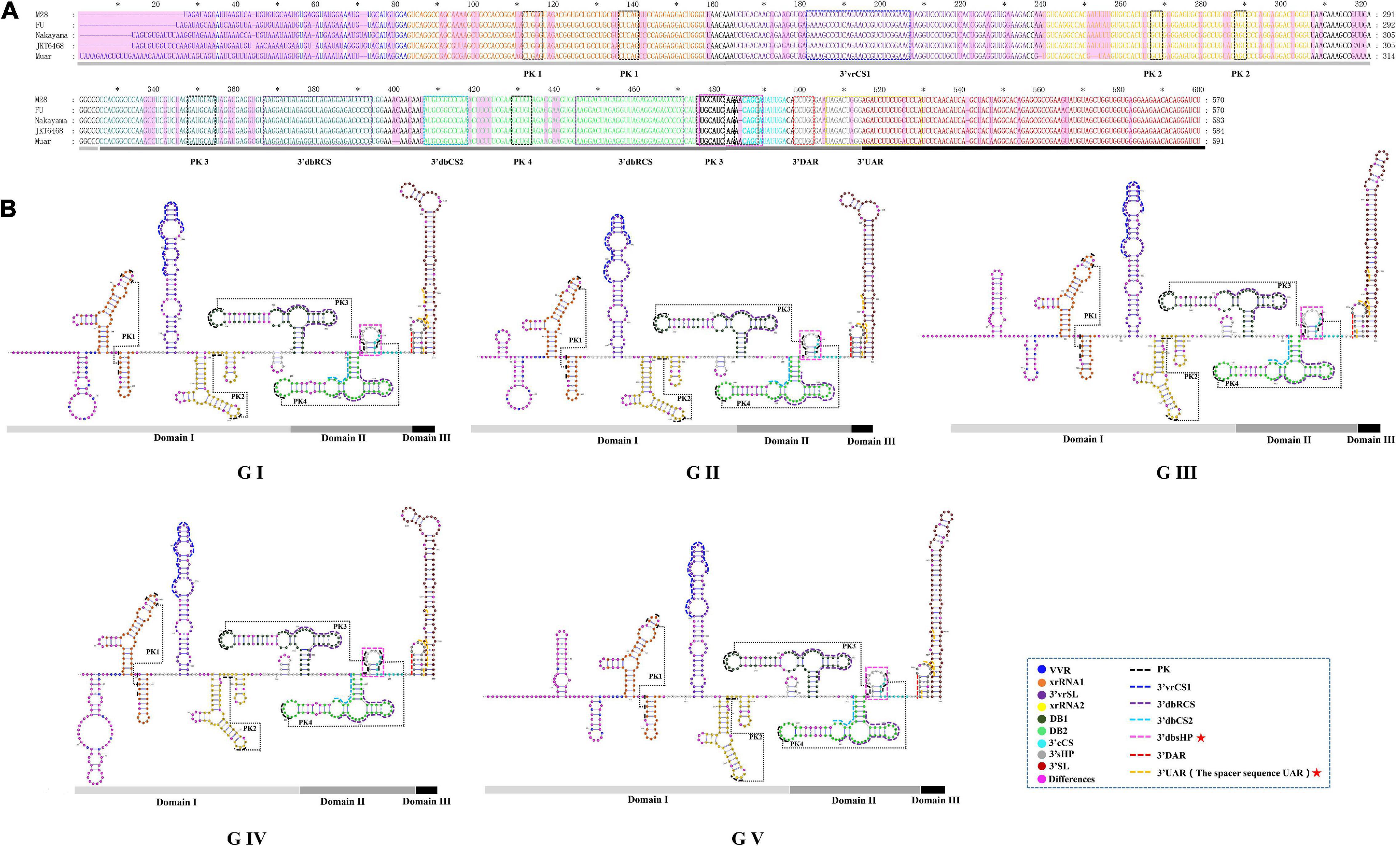
Figure 6. The primary and higher order structures of the 3′UTRs of JEV. (A) The primary sequences of the JEV 3′UTRs. (B) The higher order structures of the JEV 3′UTRs. Differently colored nucleotide bases signify different structures, cis-acting elements or significant sequence motifs: blue, the very variable region (VVR); orange, the exoribonuclease resistant RNA (xrRNA1); purple, the 3′UTR variable region stem loop (3′vrSL); light yellow, the exoribonuclease resistant RNA (xrRNA2); dark green, dumbbell structure 1 (DB1); light green, dumbbell structure 2 (DB2); bold (black and aquamarine), the 3′UTR DB region small hairpin (3′dbsHP); aquamarine, 3′cyclization sequence (3′cCS); gray, the 3′UTR short hairpin (3′sHP); brick red, the 3′UTR stem loop (3′SL). The cis-acting elements or sequence motifs which were overlapped with other structures were labeled with different colored dashed line box: black, pseudoknot PK; blue, the 3′UTR variable region conserved sequences 1 (3′vrCS1); purple, the 3′UTR dumbbell structure repeated conserved sequences (3′dbRCS); light blue, the 3′UTR dumbbell structure conserved sequences 2 (3′dbCS2); bright pink, the 3′UTR DB region small hairpin (3′dbsHP); red, the 3′downstream AUG region (3′DAR); yellow, the 3′ upstream AUG region (3′UAR). The magenta blocks represent the differences within five JEV genotypes. The Domain I, II, and III are indicated with light gray, dark gray and black bars, respectively. The mentioned units are labeled similarly in the higher order structures. The red pentagram represents the JEV specific structure or sequence motifs.
Domain III is considered to be highly conserved across the Flaviviruses and is also known as a highly conserved region (HCR). However, we found that there are structural differences that exist in the JEV genotypes. Domain III is approximately 100 nt in length, consisting of a hairpin named the 3′UTR short hairpin (3′sHP) and a long terminal SL structure termed the 3′UTR stem loop (3′SL). The 3′SL structures are almost identical among GI to GIII. However, the shapes of the 3′SL structures of GIV and GV differ from those of GI to GIII (Figure 6). The sequence (5′-CCUGG-3 ′) that lies in the stem of 3′sHP is 3′DAR. In the 3′SL structure, the 3′UAR, which consist of four discrete spacer sequences, that complement the 5′ UAR (Figure 6).
Sequence Feature Information-Guided Japanese Encephalitis Virus Genome Typing
Prior to generate the NJ phylogenetic tree, the average evolutionary divergence over all sequence pairs was computed. The average divergence values for 5′UTR, 3′UTR, and 3′VR are 0.09 ± 0.01, 0.07 ± 0.01, and 0.04 ± 0.01, respectively. The results proves the datasets are suitable for generating distance based phylogenetic trees. The ModelFinder program was used to select the best-fit nucleotide substitution model based on the Bayesian information criterion (BIC) before generating the ML tree. The best-fit models for the 3′VR, PrM, E and the ORF gene datasets were GTR + G4, GTR + G4, GTR + I + G4, GTR + F + I + G4, respectively. The phylogenetic analyses showed that the JEV isolates can be clustered into five genotypes based on the JEV 3′UTR′s VR gene sequences using both ML and NJ methods. The normalized RF values of the 3′VR tree vs. the ORF, E, and preE trees were 0.29, 0.31, and 0.34, respectively. Further analysis revealed that the main branches of the different phylogenetic trees were identical (Figure 7).
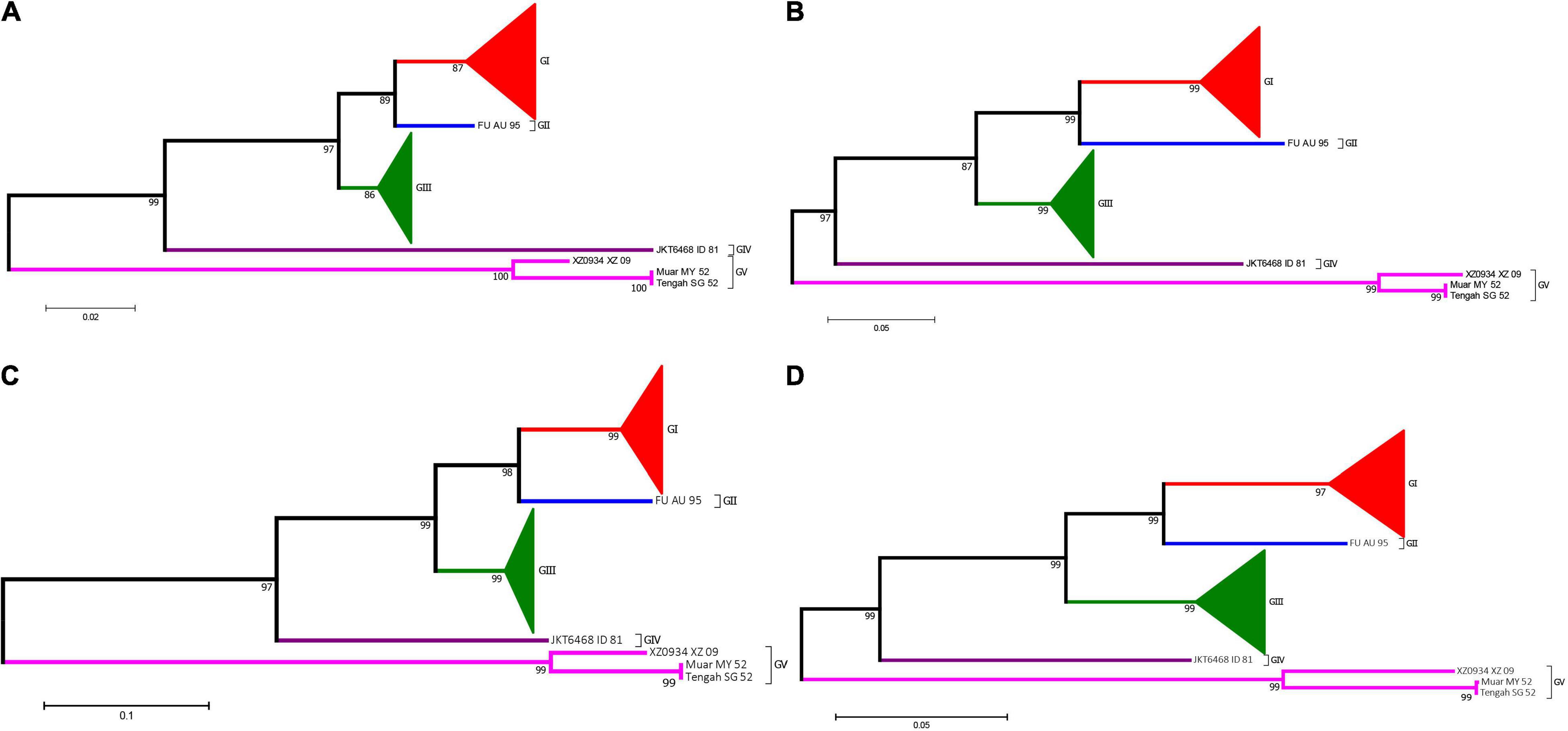
Figure 7. Phylogenetic analysis of JEV. (A) The phylogenetic tree based on the sequences (1–300 nt) of domain I (VR) within the 3′UTRs of JEV. (B) The phylogenetic tree based on the PrM gene of JEVs. (C) The phylogenetic tree based on the E gene of JEVs. (D) The phylogenetic tree based on the ORF gene of JEVs. Red, blue, green, purple and violet were used to mark genotype I, II, III, IV, and V of JEV, respectively. The triangles were used to condense strains of the same genotypes.
Discussion
This study provides comprehensive and detailed information of the sequence features and the exact sequence composition, position and distribution of the Flavivirus-conserved cis-acting elements in the JEV UTRs. The 5′ and 3′ terminal structures of JEV share the general characteristics of Flaviviruses, the reported structures and cis-acting elements of other Flaviviruses are all existed in the UTRs of JEV. The structure and cis-acting elements’ sequence comparison with other mosquito-borne Flaviviruses (WNV, YFV, ZIKV, DENV) demonstrated the existence of a JEV-specific structure and a sequence motif with a unique feature of JEV UTRs. (i) The 3′ dbsHP, which is a small hair-pin structure located in the DB region within the 3′ UTR. The 3′ dbHP is highly conserved among five JEV genotypes and contains three functional important sequences (PK3, PK4, and 3′ cCS) that involved in DB structure maintaining and viral genome cyclization. (ii) The spacer sequence UARs. The UARs of JEV consist of four discrete spacer sequences whereas the UARs of other mosquito-borne Flaviviruses (WNV, YFV, ZIKV, DENV) are continuous sequences. During viral RNA syntheses, the 5′UAR hybridizes with 3′UAR to form a complementary pairing structure that aids viral genome cyclization. The molecular dynamics and the function of the segmented UARs in JEV genome cyclization remain unclear. Given the importance of JEV, it is worth exploring the molecular functions of the unique structure and cis-acting element existent in the UTRs.
Our findings demonstrated that repeated sequences are found not only in the UTR of JEV but also in those of the other medically important mosquito-borne Flaviviruses, including WNV, YFV, ZIKV, and DENV. However, these repeated sequences differ in nucleotide composition, length and position among the different viruses. These results indicate that the UTRs of Flaviviruses contain repeated sequences, but these sequences are not consistently conserved across species. Although repeated sequences are present in the UTRs of GI–V JEV, their lengths, distances between sequences, distributions in the UTR, distances between the repeated sequences and the initiation (termination) codons differ among the genotypes. Despite differences in host origin, regional distribution and isolation time, the length and position of the repeated sequences are highly conserved within the same JEV genotype. Therefore, these repeated sequences are important molecular markers for distinguishing JEV genotypes. The interaction between the conserved regions of the viral sequence and binding proteins is a long-term evolutionary interaction between the virus and the host cell. Even minor mutations in the nucleotide sequence can affect the binding affinity of the interacting proteins (Jin, 2001). In vitro experiments have demonstrated that removal of all repeat sequences from Alphaviruses maintains their viabilities but reduces their titers (Jin, 2001). However, the decrease in virus titer significantly varied among the different host cells (Zhai et al., 2008). This suggests that the repeated sequences can directly affect the replication efficiency of the virus and play varying roles in different host cells, demonstrating a significant host specificity. The latest research reveals that the repeated conserved sequences in the 3’UTR are involved in subgenomic flaviviral RNA production and viral virulence, indicating the importance of the repeated sequences for Flaviviruses (Zhang et al., 2020). JEV has the longest repeated sequences compared with other Flaviviruses, indicating that the use of long repeated sequences for completing the virus lifecycle is unique to JEV. These repeated sequences might play an important role in the successful viral replication and transmission. Nonetheless, further studies will be required to confirm the influence of these repeated sequences on viral biological functions.
In the present study, we find that the 3′UTRs are more divergent than the 5′UTRs in both the primary sequence and the higher-order structures of JEV. The JEV 3′UTR consists of three domains. Domain I is considered to be the most variable region within the 3′UTRs among all the arthropod-borne Flaviviruses, and thus this region has been termed the variable region (VR) (Proutski et al., 1997; Ng et al., 2017). In our study, it was also demonstrated that domain I is the most variable region within JEV and that this 300 nt-length region exhibits obvious genotype-specific features.
Currently, JEV can be classified into five genotypes (GI to GV), and the distribution, host range and pathogenesis among the five genotypes are quite different (Wang and Liang, 2015; Gao et al., 2019). In particular, JEV has already spread from its traditional endemic region in Asia to Europe (Platonov et al., 2012; Ravanini et al., 2012), and JEV-infected patients have even been identified in Africa (Simon-Loriere et al., 2017). Therefore, identification of the JEV genotype has an important significance for the prevention and treatment of JE. The current methods for JEV genotyping are all based on the protein-coding region sequences, such as the E or the PrM gene (Chen et al., 1990, 1992; Solomon et al., 2003; Wang et al., 2007). Our study demonstrated that the JEV 3′UTR VR region exhibits a strong genotype-specific and that this specificity does not change with the isolation time, distribution or the host (vector). Therefore, this 300 nt-long nucleotide sequence is ideal for JEV genotyping. The phylogenetic analysis results, based on the complete ORF genome sequence (10,300 nt), the E gene (1500 nt), the PrM gene (500 nt) and the 3′UTR conserved sequence (300 nt) using different phylogenetic algorithms, generated consistent results (Figure 6), which further suggests that the VR region sequence within the 3′UTR is an ideal sequence marker for JEV genotyping. Whether the VR region sequences of other Flaviviruses also exhibit genotype-specific features and could have been used for genome typing needs further evaluation.
Our study compared the sequence and predicted structural differences in the 5′ and 3′ UTRs of 160 JEV strains isolated over an 80-year period. GI and GIII are the most widespread genotypes of JEV and cause human viral encephalitis and animal diseases (Zheng et al., 2012). All the previously reported GI and GIII strains were included and analyzed to systematically compare them and identify any nucleotide changes attributed to time, host, vector, and geographical distribution. We revealed nucleotide differences in the 5′ and 3′ UTR associated with genotype but not isolation time, host, vector, or geographical distribution. The imbalance in sequence information across the JEV genotypes in the JEV sequence dataset is because only a few JEV strains belonging to GII, GIV, and GV (Williams et al., 2000; Li et al., 2011; Woo et al., 2020) have been isolated in nature. Enhanced surveillance of JEV in nature to obtain sufficient genomic sequence information of different JEV genotypes to improve the understanding of its comprehensive genetic characteristics is highly recommended.
Data Availability Statement
The original contributions presented in the study are included in the article/Supplementary Material, further inquiries can be directed to the corresponding author/s.
Author Contributions
HL and GL carried out the study design and contributed to the revision of the manuscript. HL, JZ, and YN performed the experiment and data analysis. GL and HL wrote the manuscript. All authors read and approved the final manuscript.
Funding
This work was supported by grants from the National Natural Science Foundation of China (81301479,81290342), a Development Grant of the State Key Laboratory of Infectious Disease Prevention and Control (2014SKLID103 and 2018SKLID309), the Key R&D projects in Zibo city (2020kj100011), and the Natural Science Foundation of Shandong Province, China (ZR2013HQ008). The funders had no role in study design, data collection and analysis, decision to publish, or preparation of the manuscript.
Conflict of Interest
The authors declare that the research was conducted in the absence of any commercial or financial relationships that could be construed as a potential conflict of interest.
Publisher’s Note
All claims expressed in this article are solely those of the authors and do not necessarily represent those of their affiliated organizations, or those of the publisher, the editors and the reviewers. Any product that may be evaluated in this article, or claim that may be made by its manufacturer, is not guaranteed or endorsed by the publisher.
Supplementary Material
The Supplementary Material for this article can be found online at: https://www.frontiersin.org/articles/10.3389/fmicb.2021.730045/full#supplementary-material
Footnote
References
Alvarez, D. E., Lodeiro, M. F., Luduena, S. J., Pietrasanta, L. I., and Gamarnik, A. V. (2005). Long-range RNA-RNA interactions circularize the dengue virus genome. J. Virol. 79, 6631–6643. doi: 10.1128/JVI.79.11.6631-6643.2005
Brinton, M. A., Fernandez, A. V., and Dispoto, J. H. (1986). The 3′-nucleotides of flavivirus genomic RNA form a conserved secondary structure. Virology 153, 113–121. doi: 10.1016/0042-6822(86)90012-7
Burland, T. G. (2000). DNASTAR’s Lasergene sequence analysis software. Methods Mol. Biol. 132, 71–91. doi: 10.1385/1-59259-192-2:71
Cao, L., Fu, S., Gao, X., Li, M., Cui, S., Li, X., et al. (2016). Low protective efficacy of the current japanese encephalitis vaccine against the emerging Genotype 5 Japanese encephalitis virus. PLoS Negl. Trop. Dis. 10:e0004686. doi: 10.1371/journal.pntd.0004686
Chang, R. Y., Hsu, T. W., Chen, Y. L., Liu, S. F., Tsai, Y. J., Lin, Y. T., et al. (2013). Japanese encephalitis virus non-coding RNA inhibits activation of interferon by blocking nuclear translocation of interferon regulatory factor 3. Vet. Microbiol. 166, 11–21. doi: 10.1016/j.vetmic.2013.04.026
Chapman, E. G., Moon, S. L., Wilusz, J., and Kieft, J. S. (2014). RNA structures that resist degradation by Xrn1 produce a pathogenic Dengue virus RNA. Elife 3:e01892. doi: 10.7554/eLife.01892.021
Chen, W. R., Rico-Hesse, R., and Tesh, R. B. (1992). A new genotype of Japanese encephalitis virus from Indonesia. Am. J. Trop. Med. Hyg. 47, 61–69. doi: 10.4269/ajtmh.1992.47.61
Chen, W. R., Tesh, R. B., and Rico-Hesse, R. (1990). Genetic variation of Japanese encephalitis virus in nature. J. Gen. Virol. 71, 2915–2922. doi: 10.1099/0022-1317-71-12-2915
Clyde, K., Barrera, J., and Harris, E. (2008). The capsid-coding region hairpin element (cHP) is a critical determinant of dengue virus and West Nile virus RNA synthesis. Virology 379, 314–323. doi: 10.1016/j.virol.2008.06.034
Darty, K., Denise, A., and Ponty, Y. (2009). VARNA: interactive drawing and editing of the RNA secondary structure. Bioinformatics 25, 1974–1975. doi: 10.1093/bioinformatics/btp250
Erlanger, T. E., Weiss, S., Keiser, J., Utzinger, J., and Wiedenmayer, K. (2009). Past, present, and future of Japanese encephalitis. Emerg. Infect. Dis. 15, 1–7. doi: 10.3201/eid1501.080311
Filomatori, C. V., Carballeda, J. M., Villordo, S. M., Aguirre, S., Pallares, H. M., Maestre, A. M., et al. (2017). Dengue virus genomic variation associated with mosquito adaptation defines the pattern of viral non-coding RNAs and fitness in human cells. PLoS Pathog. 13:e1006265. doi: 10.1371/journal.ppat.1006265
Filomatori, C. V., Lodeiro, M. F., Alvarez, D. E., Samsa, M. M., Pietrasanta, L., and Gamarnik, A. V. (2006). A 5’ RNA element promotes dengue virus RNA synthesis on a circular genome. Genes Dev. 20, 2238–2249. doi: 10.1101/gad.1444206
Friebe, P., Shi, P. Y., and Harris, E. (2011). The 5’ and 3’ downstream AUG region elements are required for mosquito-borne flavivirus RNA replication. J. Virol. 85, 1900–1905. doi: 10.1128/JVI.02037-10
Gao, X., Liu, H., Li, M., Fu, S., and Liang, G. (2015). Insights into the evolutionary history of Japanese encephalitis virus (JEV) based on whole-genome sequences comprising the five genotypes. Virol. J. 12:43. doi: 10.1186/s12985-015-0270-z
Gao, X., Liu, H., Li, X., Fu, S., Cao, L., Shao, N., et al. (2019). Changing geographic distribution of japanese Encephalitis virus Genotypes, 1935-2017. Vector Borne Zoonotic Dis. 19, 35–44. doi: 10.1089/vbz.2018.2291
Garcia-Blanco, M. A., Vasudevan, S. G., Bradrick, S. S., and Nicchitta, C. (2016). Flavivirus RNA transactions from viral entry to genome replication. Antivir. Res. 134, 244–249. doi: 10.1016/j.antiviral.2016.09.010
Gebhard, L. G., Filomatori, C. V., and Gamarnik, A. V. (2011). Functional RNA elements in the dengue virus genome. Viruses 3, 1739–1756. doi: 10.3390/v3091739
Hahn, C. S., Hahn, Y. S., Rice, C. M., Lee, E., Dalgarno, L., Strauss, E. G., et al. (1987). Conserved elements in the 3’ untranslated region of flavivirus RNAs and potential cyclization sequences. J. Mol. Biol. 198, 33–41. doi: 10.1016/0022-2836(87)90455-4
Halstead, S. B., and Jacobsen, J. (2008). “Japanese Encephalitis vaccines,” in Vaccines, 5th Edn, eds S. A. Plotkin, W. A. Orenstein, and P. A. Offit (Amsterdam: Elsevier).
Hegde, N. R., and Gore, M. M. (2017). Japanese encephalitis vaccines: immunogenicity, protective efficacy, effectiveness, and impact on the burden of disease. Hum. Vaccin. Immunother. 13, 1–18. doi: 10.1080/21645515.2017.1285472
Hodge, K., Kamkaew, M., Pisitkun, T., and Chimnaronk, S. (2019). Flavors of Flaviviral RNA structure: towards an integrated view of RNA function from translation through Encapsidation. Bioessays 41:e1900003. doi: 10.1002/bies.201900003
Kalyaanamoorthy, S., Minh, B. Q., Wong, T. K., von Haeseler, A., and Jermiin, L. S. (2017). ModelFinder: fast model selection for accurate phylogenetic estimates. Nat. Methods 14, 587–589. doi: 10.1038/nmeth.4285
Kieft, J. S., Rabe, J. L., and Chapman, E. G. (2015). New hypotheses derived from the structure of a flaviviral Xrn1-resistant RNA: conservation, folding, and host adaptation. RNA Biol. 12, 1169–1177. doi: 10.1080/15476286.2015.1094599
Kumar, S., Stecher, G., Li, M., Knyaz, C., and Tamura, K. (2018). MEGA X: molecular evolutionary genetics analysis across computing platforms. Mol. Biol. Evol. 35, 1547–1549. doi: 10.1093/molbev/msy096
Kuwata, R., Torii, S., Shimoda, H., Supriyono, S., Phichitraslip, T., Prasertsincharoen, N., et al. (2020). Distribution of Japanese Encephalitis virus, Japan and Southeast Asia, 2016-2018. Emerg. Infect. Dis. 26, 125–128. doi: 10.3201/eid2601.190235
Lerat, E. (2010). Identifying repeats and transposable elements in sequenced genomes: how to find your way through the dense forest of programs. Heredity 104, 520–533. doi: 10.1038/hdy.2009.165
Lewis, L., Taylor, H. G., Sorem, M. B., Norcross, J. W., and Kindsvatter, V. H. (1947). Japanese B encephalitis: clinical observations in an outbreak on okinawa shima. Arch. Neurol. Psychiatry 57, 430–463. doi: 10.1001/archneurpsyc.1947.02300270048004
Li, M. H., Fu, S. H., Chen, W. X., Wang, H. Y., Guo, Y. H., Liu, Q. Y., et al. (2011). Genotype v Japanese encephalitis virus is emerging. PLoS Negl. Trop. Dis. 5:e1231. doi: 10.1371/journal.pntd.0001231
Lindenbach, B. D., Thiel, H. J., and Rice, C. M. (2007). “Flaviviridae: the viruses and their replication,” in Fields Virology, 5th Edn, eds D. M. Knipe and P. M. Howley (Philadelphia, PA: Lippincott Williams & Wilkins).
Liu, W., Xie, Y., Ma, J., Luo, X., Nie, P., Zuo, Z., et al. (2015). IBS: an illustrator for the presentation and visualization of biological sequences. Bioinformatics 31, 3359–3361. doi: 10.1093/bioinformatics/btv362
Liu, Z. Y., and Qin, C. F. (2020). Structure and function of cis-acting RNA elements of flavivirus. Rev. Med. Virol. 30:e2092. doi: 10.1002/rmv.2092
Liu, Z. Y., Li, X. F., Jiang, T., Deng, Y. Q., Ye, Q., Zhao, H., et al. (2016). Viral RNA switch mediates the dynamic control of flavivirus replicase recruitment by genome cyclization. Elife 5:e17636. doi: 10.7554/eLife.17636.044
Moon, S. L., Anderson, J. R., Kumagai, Y., Wilusz, C. J., Akira, S., Khromykh, A. A., et al. (2012). A noncoding RNA produced by arthropod-borne flaviviruses inhibits the cellular exoribonuclease XRN1 and alters host mRNA stability. RNA 18, 2029–2040. doi: 10.1261/rna.034330.112
Ng, W. C., Soto-Acosta, R., Bradrick, S. S., Garcia-Blanco, M. A., and Ooi, E. E. (2017). The 5’ and 3’ untranslated regions of the flaviviral genome. Viruses 9:137. doi: 10.3390/v9060137
Ni, H., Burns, N. J., Chang, G. J., Zhang, M. J., Wills, M. R., Trent, D. W., et al. (1994). Comparison of nucleotide and deduced amino acid sequence of the 5’ non-coding region and structural protein genes of the wild-type Japanese encephalitis virus strain SA14 and its attenuated vaccine derivatives. J. Gen. Virol. 75(Pt 6), 1505–1510. doi: 10.1099/0022-1317-75-6-1505
Pan, X. L., Liu, H., Wang, H. Y., Fu, S. H., Liu, H. Z., Zhang, H. L., et al. (2011). Emergence of genotype I of Japanese encephalitis virus as the dominant genotype in Asia. J. Virol. 85, 9847–9853. doi: 10.1128/JVI.00825-11
Pijlman, G. P., Funk, A., Kondratieva, N., Leung, J., Torres, S., and van der, et al. (2008). A highly structured, nuclease-resistant, noncoding RNA produced by flaviviruses is required for pathogenicity. Cell Host Microbe 4, 579–591. doi: 10.1016/j.chom.2008.10.007
Platonov, A., Rossi, G., Karan, L., Mironov, K., Busani, L., and Rezza, G. (2012). Does the Japanese encephalitis virus (JEV) represent a threat for human health in Europe? detection of JEV RNA sequences in birds collected in Italy. Euro. Surveill. 17:20241. doi: 10.2807/ese.17.32.20241-en
Proutski, V., Gould, E. A., and Holmes, E. C. (1997). Secondary structure of the 3’ untranslated region of flaviviruses: similarities and differences. Nucleic Acids Res. 25, 1194–1202. doi: 10.1093/nar/25.6.1194
Ravanini, P., Huhtamo, E., Ilaria, V., Crobu, M. G., Nicosia, A. M., Servino, L., et al. (2012). Japanese encephalitis virus RNA detected in Culex pipiens mosquitoes in Italy. Euro. Surveill. 17:20221. doi: 10.2807/ese.17.28.20221-en
Robinson, D. F., and Foulds, L. R. (1981). Comparison of phylogenetic trees. Math. Biosci. 53, 131–147. doi: 10.1016/0025-5564(81)90043-2
Sanford, T. J., Mears, H. V., Fajardo, T., Locker, N., and Sweeney, T. R. (2019). Circularization of flavivirus genomic RNA inhibits de novo translation initiation. Nucleic Acids Res. 47, 9789–9802. doi: 10.1093/nar/gkz686
Schuh, A. J., Li, L., Tesh, R. B., Innis, B. L., and Barrett, A. D. (2010). Genetic characterization of early isolates of Japanese encephalitis virus: genotype II has been circulating since at least 1951. J. Gen. Virol. 91(Pt 1), 95–102. doi: 10.1099/vir.0.013631-0
Simon-Loriere, E., Faye, O., Prot, M., Casademont, I., Fall, G., Fernandez-Garcia, M. D., et al. (2017). Autochthonous Japanese Encephalitis with yellow fever coinfection in Africa. N. Engl. J. Med. 376, 1483–1485. doi: 10.1056/NEJMc1701600
Smith, M. R. (2020). Information theoretic generalized robinson-foulds metrics for comparing phylogenetic trees. Bioinformatics 36, 5007–5013. doi: 10.1093/bioinformatics/btaa614
Solomon, T., Ni, H., Beasley, D. W., Ekkelenkamp, M., Cardosa, M. J., and Barrett, A. D. (2003). Origin and evolution of Japanese encephalitis virus in southeast Asia. J. Virol. 77, 3091–3098. doi: 10.1128/JVI.77.5.3091-3098.2003
Sumiyoshi, H., Mori, C., Fuke, I., Morita, K., Kuhara, S., Kondou, J., et al. (1987). Complete nucleotide sequence of the Japanese encephalitis virus genome RNA. Virology 161, 497–510. doi: 10.1016/0042-6822(87)90144-9
Thompson, J. D., Gibson, T. J., Plewniak, F., Jeanmougin, F., and Higgins, D. G. (1997). The CLUSTAL_X windows interface: flexible strategies for multiple sequence alignment aided by quality analysis tools. Nucleic Acids Res. 25, 4876–4882. doi: 10.1093/nar/25.24.4876
Villordo, S. M., and Gamarnik, A. V. (2009). Genome cyclization as strategy for flavivirus RNA replication. Virus Res. 139, 230–239. doi: 10.1016/j.virusres.2008.07.016
Wang, H. Y., Takasaki, T., Fu, S. H., Sun, X. H., Zhang, H. L., Wang, Z. X., et al. (2007). Molecular epidemiological analysis of Japanese encephalitis virus in China. J. Gen. Virol. 88, 885–894. doi: 10.1099/vir.0.82185-0
Wang, H., and Liang, G. (2015). Epidemiology of Japanese encephalitis: past, present, and future prospects. Ther. Clin. Risk Manag. 11, 435–448. doi: 10.2147/TCRM.S51168
Wang, H., Han, M., Qi, J., Hilgenfeld, R., Luo, T., Shi, Y., et al. (2017). Crystal structure of the C-terminal fragment of NS1 protein from yellow fever virus. Sci. China Life Sci. 60, 1403–1406. doi: 10.1007/s11427-017-9238-8
Westaway, E. G., Mackenzie, J. M., and Khromykh, A. A. (2003). Kunjin RNA replication and applications of Kunjin replicons. Adv. Virus Res. 59, 99–140. doi: 10.1016/S0065-3527(03)59004-2
Williams, D. T., Wang, L. F., Daniels, P. W., and Mackenzie, J. S. (2000). Molecular characterization of the first Australian isolate of Japanese encephalitis virus, the FU strain. J. Gen. Virol. 81(Pt 10), 2471–2480. doi: 10.1099/0022-1317-81-10-2471
Woo, J. H., Jeong, Y. E., Jo, J. E., Shim, S. M., Ryou, J., Kim, K. C., et al. (2020). Genetic characterization of japanese encephalitis virus Genotype 5 isolated from patient, South Korea, 2015. Emerg. Infect. Dis. 26, 1002–1006. doi: 10.3201/eid2605.190977
Zeng, M., Duan, Y., Zhang, W., Wang, M., Jia, R., Zhu, D., et al. (2020). Universal RNA secondary structure insight into Mosquito-Borne Flavivirus (MBFV) cis-Acting RNA biology. Front. Microbiol. 11:473. doi: 10.3389/fmicb.2020.00473
Zhai, Y. G., Wang, H. Y., Sun, X. H., Fu, S. H., Wang, H. Q., Attoui, H., et al. (2008). Complete sequence characterization of isolates of Getah virus (genus Alphavirus, family Togaviridae) from China. J. Gen. Virol. 89, 1446–1456. doi: 10.1099/vir.0.83607-0
Zhang, Q. Y., Li, X. F., Niu, X., Li, N., Wang, H. J., Deng, C. L., et al. (2020). Short Direct Repeats in the 3’ untranslated region are involved in Subgenomic Flaviviral RNA Production. J. Virol. 94:e1175-19. doi: 10.1128/JVI.01175-19
Zheng, Y., Li, M., Wang, H., and Liang, G. (2012). Japanese encephalitis and Japanese encephalitis virus in mainland China. Rev. Med. Virol. 22, 301–322. doi: 10.1002/rmv.1710
Keywords: Japanese encephalitis virus, Flavivirus, secondary structure, 5′untranslated region, 3′untranslated region
Citation: Liu H, Zhang J, Niu Y and Liang G (2021) The 5′ and 3′ Untranslated Regions of the Japanese Encephalitis Virus (JEV): Molecular Genetics and Higher Order Structures. Front. Microbiol. 12:730045. doi: 10.3389/fmicb.2021.730045
Received: 24 June 2021; Accepted: 22 September 2021;
Published: 28 October 2021.
Edited by:
Han Xia, Key Laboratory of Special Pathogens and Biosafety, Wuhan Institute of Virology, Chinese Academy of Sciences (CAS), ChinaReviewed by:
Margo A. Brinton, Georgia State University, United StatesAdriano de Bernardi Schneider, University of California, San Diego, United States
Copyright © 2021 Liu, Zhang, Niu and Liang. This is an open-access article distributed under the terms of the Creative Commons Attribution License (CC BY). The use, distribution or reproduction in other forums is permitted, provided the original author(s) and the copyright owner(s) are credited and that the original publication in this journal is cited, in accordance with accepted academic practice. No use, distribution or reproduction is permitted which does not comply with these terms.
*Correspondence: Hong Liu, liuhongseminar@hotmail.com; Guodong Liang, gdliang@hotmail.com
†These authors have contributed equally to this work