- 1Laboratory of Food Quality and Food Safety, Faculty of Biological and Agricultural Sciences, Mouloud Mammeri University, Tizi Ouzou, Algeria
- 2Food Biotechnology Department, Instituto de la Grasa, IG-CSIC, Seville, Spain
Olives from the Sigoise, Verdale, and Sevillana cultivars were elaborated as Spanish-style table olives by four Algerian factories, and the quality and food safety of the industrial table olives have been studied by the analysis of physicochemical and microbiological parameters. Differences were observed between the treatments carried out by the different factories throughout the manufacturing process, especially during the washing stage, but no significant differences were found between the analyzed samples for the concentration of sugars and polyphenols. The final pH values reached at the end of fermentation ranged between 5.04 and 4.27, and the titratable acidity was above 0.4% for all samples. Lactic and acetic acids were produced in mean concentrations of 0.68% and 0.21% as a result of lactic acid bacteria (LAB) and yeast metabolism, respectively. However, the presence of butyric, isobutyric, and propionic acids was also detected, and was related to the growth of undesirable spoilage microorganisms, responsible for secondary fermentations. The high-throughput sequencing of bacterial DNA suggested the dominance of LAB species belonging to genera Lactiplantibacillus, Leuconostoc, Pediococcus, Oenococcus, or Enterococcus. The Enterobacteriaceae family was detected during the first days of brining and in only one sample after 120 days of fermentation. Other spoilage microorganisms were found, such as Lentilactobacillus buchneri or the Pectinatus and Acetobacter genera, capable of consuming lactic acid and these played an essential role in the onset of spoilage. The Clostridium and Enterobacter genera, producers of butyric and propionic acids, were responsible for the malodorous fermentation present in the industrial samples that were analyzed. The study concluded that the safety of the table olives analyzed was compromised by the presence of undesirable microorganisms and microbial stability was not guaranteed. The elaboration process can be improved by reducing the washing steps and the time should be reduced to avoid the loss of fermentable matter, with the goal of reaching a pH < 4.0 after the fermentation and preventing the possibility of the growth of spoilage microorganisms and foodborne pathogens.
Introduction
Table olives are elaborated from the olive tree’s fruit (Olea europaea) and are considered to be the most popular and highly valued fermented vegetable in the Mediterranean countries, having been part of their diet for centuries. Algeria is one of the major table olive-producing countries, with an increased production in recent years estimated at 323,000 tons annually, representing more than 10.5% of the global production (International Olive Council [IOC], 2021).
Raw olives are inedible due to the bitter taste that the polyphenol oleuropein lends them. The main objective of table olives elaboration processes is to remove the bitterness by hydrolysis of the oleuropein, in order to make them palatable (Brenes and de Castro, 1998). There are many different elaboration methods depending on the region, cultivar, the stage of maturity of the olives, etc (Alves and Quintas, 2016; Grounta and Panagou, 2017; Ashaolu and Reale, 2020). Among the different types of commercial table olives, Spanish-style green olives are the most popular elaboration, characterized by an initial alkaline treatment with 2–3% sodium hydroxide (lye) for several hours, which hydrolyzes the oleuropein into non-bitter compounds (Brenes and de Castro, 1998). Then, the fruits are washed twice with tap water to remove the excess alkali, and finally, the olives are covered with brine (9–10% w/v of sodium chloride) to undergo fermentation by autochthonous microbiota for several months (Sánchez-Gómez et al., 2006; Heperkan, 2013).
The increasingly restrictive new environmental policies applied in many countries have forced table olive producers to eliminate the second wash in this type of elaboration, replacing it with a single one, although more intense (12–15 h), to reduce the volume of wastewaters (Sánchez-Gómez et al., 2006). However, Algerian regulations seem to be more flexible in this regard, and factories continue to carry out two washes (Mettouchi et al., 2016). Another trend currently carried out in many factories is to perform a controlled fermentation. Traditionally, fermentation is carried out by autochthonous lactic acid bacteria (LAB) and yeasts but the addition of starter cultures, a practice increasingly employed by processors, reduces the probability of spoilage (Panagou and Tassou, 2006; Rejano-Navarro et al., 2010; Benítez-Cabello et al., 2019; Anagnostopoulos et al., 2020). Also, extensive control of the physicochemical parameters during the table olives elaboration is necessary for an adequate management of the fermentation. The correct sodium chloride concentration, pH values around 4, and free acidity above 0.6% at the end of the process help extend the shelf life, avoid spoilage, and maintain a safe product (Sánchez et al., 2001; International Olive Council [IOC], 2004).
Spanish-style table olives occasionally present with microbial spoilage issues. The uncontrolled growth of certain microorganisms such as Enterobacteriaceae, Clostridium, Propionibacterium (Medina-Pradas and Arroyo-López, 2015; Grounta and Panagou, 2017), Cardiobacteriaceae, Ruminococcus (De Castro et al., 2018), and oxidative yeasts (Arroyo-López et al., 2012) can cause secondary fermentations with a consequent increase in the pH value of brines. Spoilage microorganisms associated with table olives can convert the lactic acid, generated by LAB during fermentation, into other organic acids and the formation of off-odors like putrid or rancid butter as a consequence of their metabolisms (Montaño et al., 1992; De Castro et al., 2018). The resulting higher pH values may lead to the proliferation of other foodborne pathogen microorganisms, that were previously inhibited. Improper acidification to pH above 4.6 enables the growth of the spore-forming Clostridium botulinum, responsible for the production of the botulinum toxin, generating a risk to the health of consumers (Medina-Pradas and Arroyo-López, 2015). However, table olives have a long history of food safety. The control of the pH, free acidity, and salt concentration in brine would prevent the growth of these non-desirable microorganisms, especially during the summer when temperatures rise (Sánchez-Gómez et al., 2006). Besides, different strategies such as the use of starter cultures to carry out the fermentation under control, the use of preservatives, and heat treatments as pasteurization, together with good manufacturing and hygiene practices, are useful tools as control measures to reduce alterations in table olives (Panagou and Tassou, 2006; Medina-Pradas et al., 2017).
The objective of this work was to study the quality and food safety of the industrial fermented green table olives elaborated as Spanish-style from different Algerian cultivars using the analysis of physicochemical and microbiological parameters.
Materials and Methods
Olive Fruits Processing and Sampling
Seventeen samples of brines and olive fruits from different table olive cultivars (Sevillana, Verdale, and Sigoise) elaborated as Spanish-style were collected in February 2020 from four Algerian factories, two of them located in Ain Defla, and the others in Boumerdes and Tizi Ouzou. Samples were collected at different stage of fermentation from industrial fermenters and transported within 24 h to the laboratory for the analysis. The elaboration process was different among the four producers, and the information related to each sample is reported in Table 1.
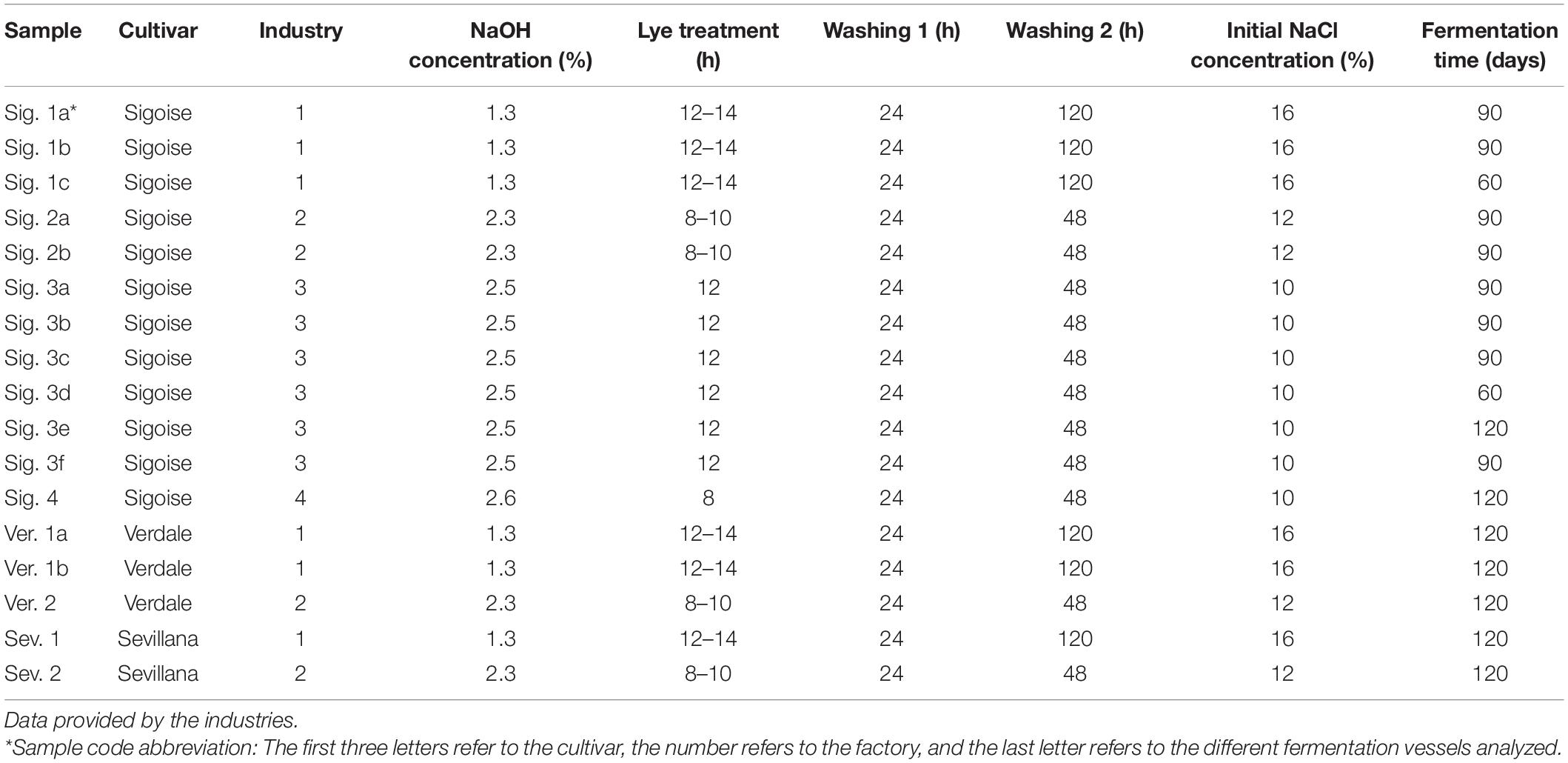
Table 1. Characteristic processing of the Spanish-style green olives brines collected from industrial tanks.
In parallel, brine samples of the Sigoise fermentation (Sig. 4) were collected periodically to monitor the fermentation process at 1, 3, 8, 20, 38, 47, and 120 days of fermentation. Also, samples of fruits were collected at the beginning and end of the fermentation process.
Physicochemical Analysis
Titratable acidity (expressed as % of lactic acid) and pH of brines were measured using a Metrohm 670 Titroprocessor (Herisau, Switzerland). The concentration of NaCl was analyzed by titration with a silver nitrate solution (0.086 N) and expressed as percent (%).
Analysis of Sugars and Organic and Volatile Acids
Sugars (sucrose, glucose, fructose, and mannitol), organic acids (lactic and acetic acids), and ethanol were extracted and analyzed from olive fruits as described elsewhere (Medina et al., 2007). The concentration of sugars in olive brines was determined by mixing 0.5 ml of brine with 1 g of the acidic resin Amberlite IR-120 and 1 g of the basic resin Amberlite IRA-93, and 1.5 ml of sorbitol (0.05%). After 30 min of occasional agitation, the mixture was filtered through a 0.22-μm pore size nylon filter and injected in the HPLC. The organic acids (lactic and acetic acids) and ethanol were analyzed by filtering 1 ml of brine directly through a 0.22-μm pore size nylon filter before injection. The chromatographic system for the analysis of the sugars and the organic acids was the same as described by Medina et al. (2007). Volatile acid compounds in olive brines (butyric, isobutyric, and propionic acids) were analyzed by HS-SPME-GC-MS following the procedure described elsewhere (Garcia-Villalba et al., 2012). All samples were analyzed in duplicate.
Analysis of Phenolic Compounds
The phenolic compounds in brines were analyzed as described by Medina et al. (2007). An aliquot of 0.25 ml of brines was diluted in 0.5 ml of distilled water and 0.25 ml of syringic acid (2 mM). The mixtures were filtered through a 0.22-μm pore size nylon filter, and aliquots of 20 μl were injected in the HPLC. All samples were analyzed in duplicate. The results were expressed as the sum of the phenols hydroxytyrosol, hydroxytyrosol 4-glucoside, tyrosol, and salidroside.
Microbiological Analysis
The viable and culturable microbial population were determined by plating the fermentation brines and their decimal dilutions (in 9 g/L NaCl) with a Spiral Plater (Don Whitley Scientific Ltd., Shipley, United Kingdom). Enterobacteriaceae, mesophilic aerobic bacteria, yeasts, and LAB were counted on VRBG agar (Sigma-Aldrich, St. Louis, MO, United States), Plate Count Agar (Sigma-Aldrich), Yeast Malt Agar with oxytetracycline (Oxoid Ltd., Basingstoke, United Kingdom), and De Man, Rogosa and Sharpe agar (Biokar Diagnostics, Beauvais, France) supplemented with 0.02% sodium azide (Sigma-Aldrich), respectively. Enterobacteriaceae were incubated at 37°C for 24 h, mesophilic aerobic bacteria, yeasts, and LAB were set at 32°C for 48 h, and the numbers of colony-forming units were counted with a Scan 500 colony counter (Interscience, Saint-Nom-la-Bretèche, France).
Molecular Identification of the Bacterial Population
A selection of four different colonies from VRBG agar plate (sample Ver. 2) were isolated according to their morphology as the main types of bacteria found. The total DNA of the isolates was extracted directly from pelleted cells by the rapid chloroform method described by Ruiz-Barba et al. (2005). The isolates were identified to species level by 16S rRNA gene sequence analysis of a PCR fragment (ca. 1400 bp) amplified using the primer pair 8f (5′-AGAGTTTGATYMTGGCTCAG-3′) and 1492r (5′-GGTTACCTTGTTACGACTT-3′) as previously described in Pérez-Díaz et al. (2019).
Bacterial DNA Extraction and High-Throughput Sequencing
Culture-independent methods were applied to assess the whole microbiota in selected industrial samples. A volume of 5 ml of brine was spun at 9,000 g for 5 min. Then, the pellet was washed twice with saline solution (0.9% NaCl). DNA isolation was performed using the PowerFood® Microbial DNA Isolation Kit (MoBio, Carlsbad, CA, United States) according to the manufacturer’s instructions. Purified DNA samples (∼10 ng/μl) were sent to the Sequencing and Bioinformatic Service of FISABIO (Valencia, Spain) for amplification, library preparation of the 16S rRNA gene of bacteria, and massive sequencing of 16S rDNA amplicons using a MiSeq Illumina platform. Metagenomic libraries, sequencing, and bioinformatic analysis were carried out as described elsewhere (Medina et al., 2018).
Statistical Analysis
Data were expressed as mean values ± standard deviation. Statistical software version 7.0 was used for data processing (Statistical for Windows, Tulsa, OK, United States). Comparison among mean values was performed by one-way analysis of variance followed by Duncan’s multiple range test, and the differences were considered significant when p < 0.05.
Results and Discussion
The industrial table olive samples were elaborated with a Spanish-style procedure, but the differences between the factories are noticeable, as shown in Table 1. The concentration of the NaOH solution and the duration used for the alkaline treatment ranged from 1.3 to 2.6% and 8–14 h, respectively. It is recommended that the alkaline solution must penetrate 2/3 parts the distance of the pulp to the pit for adequate bitterness removal (Conte et al., 2020). Thus, in those treatments with lower lye concentrations, the duration was longer, and vice versa. Differences were reported in the subsequent washing steps, being 24 h for the first washing, and 120 and 48 h for the second washing for factory 1 and 2–4, respectively. The main objective of the washing stage is the removal of the excess of sodium hydroxide from the flesh. Thus, excessive washing causes loss of soluble sugars and polyphenols to be lost from the fruit to wastewaters (Papadaki and Mantzouridou, 2016). Nowadays, the current trend requires the reduction of washing steps to only one with a duration of 6–12 h to minimize the volume of wastewaters generated. Therefore, it seems that the Algerian table olive factories should optimize the duration of both the alkaline and subsequent washing stages.
Glucose was the primary sugar in olive brines, as shown in Figure 1A. The highest concentration was reached at day 20 after brining and it decreased drastically at day 40 when the glucose was entirely consumed until the end of fermentation. The mannitol reached maximum values at around day 40, and its consumption was lower than glucose and decreased at the end of fermentation (Figure 1A). Sucrose was not detected and fructose showed low concentrations at the time of brining (Figure 1A). The sugars of the final fruits showed a reduction of 96.11, 83.88, and 27.83% for the glucose, fructose, and mannitol, respectively, compared to the raw fruit (data not shown). Glucose showed low concentrations or was not detected in the industrial brines listed in Table 2, which implied that it had been consumed during the fermentation (except for the sample Sig. 1a).
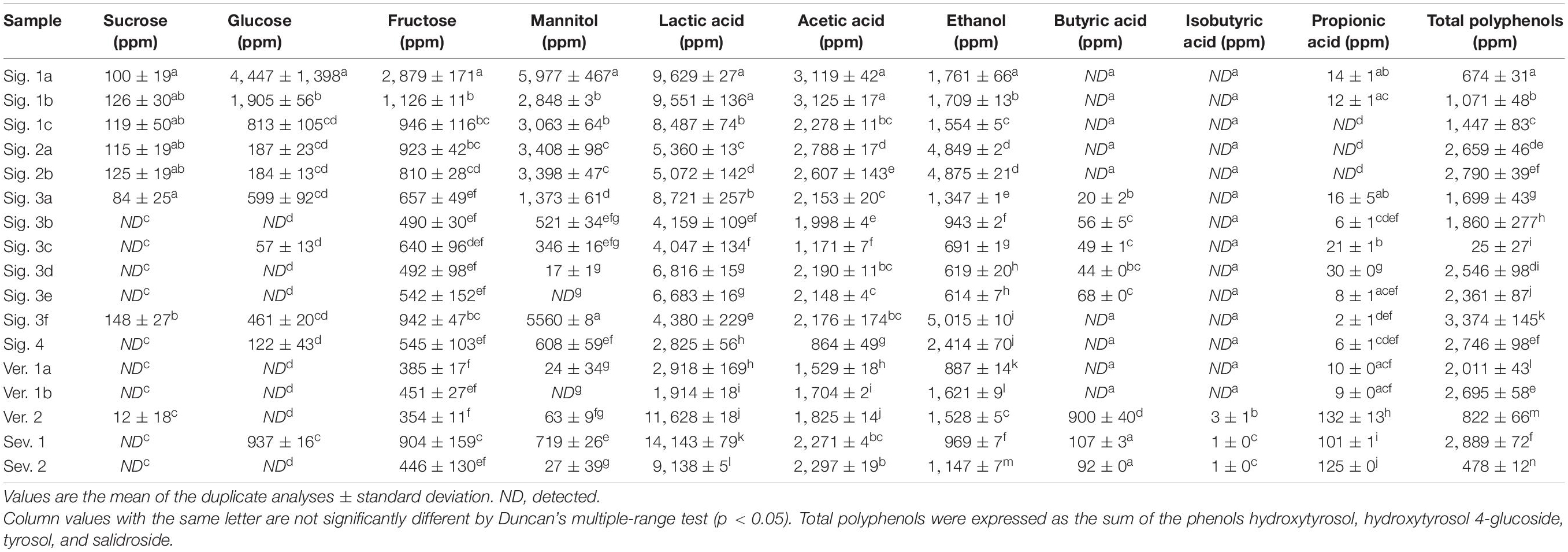
Table 2. Sugars, organic acids, and total polyphenol concentration in industrial Spanish-style green olive brines after fermentation.
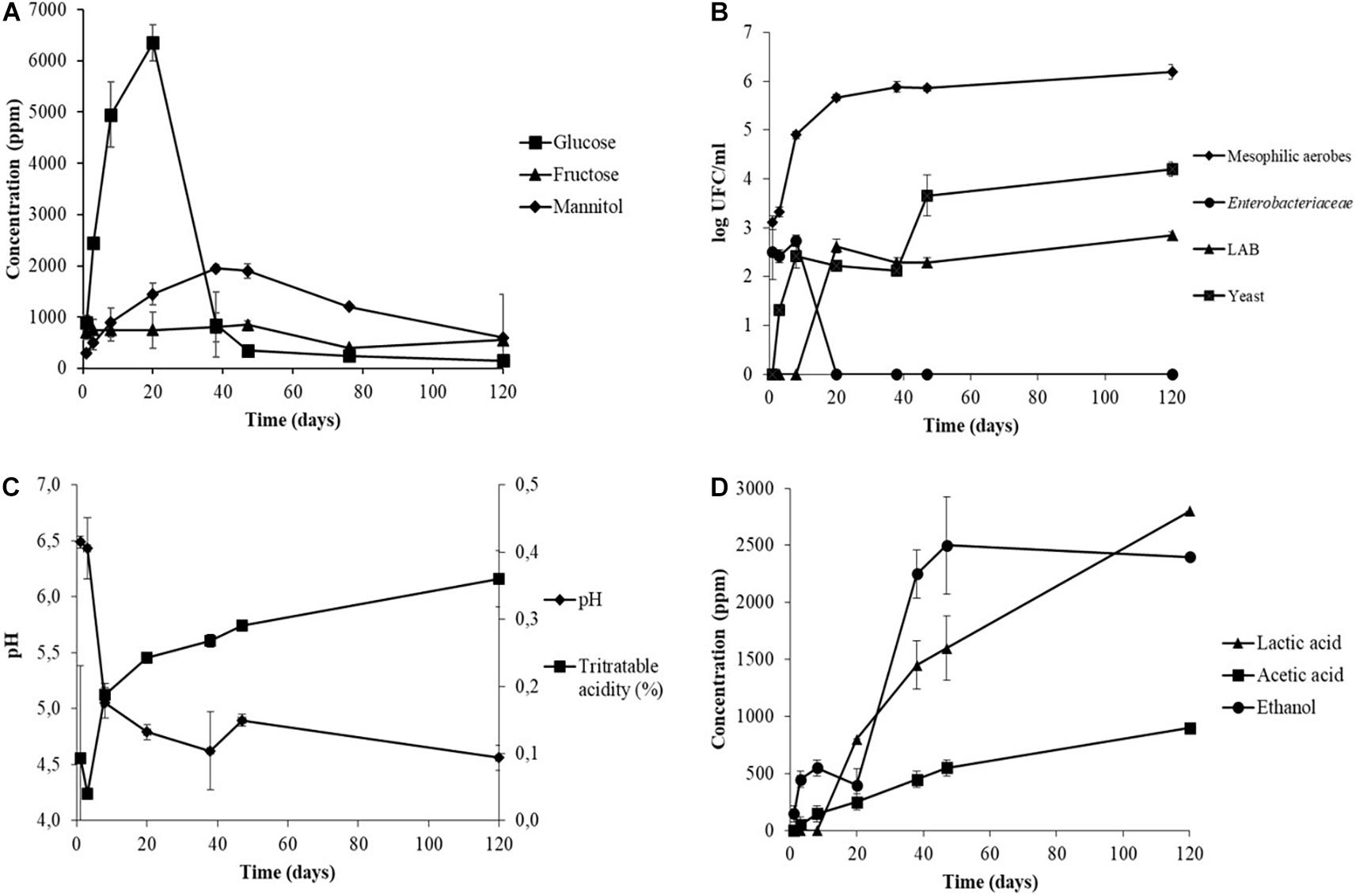
Figure 1. Monitoring of pH and titratable acidity (A), organic acids (B), sugars (C), and viable microbial population (D) during the industrial processing of Sigoise cultivar elaborated as Spanish-style green olives (sample Sig. 4). Standard deviation of duplicates is depicted on the error bars.
The evolution of the microbial population observed for sample Sig. 4 is shown in Figure 1B. Mesophilic aerobes were the main group detected and reached a population above 5 log CFU/ml in the first days of brining. Instead, the presence of LAB and yeasts were detected after 10–20 days of fermentation and reached values of 2.85 and 4.20 log CFU/ml at the end of fermentation, respectively. Enterobacteriaceae were present during the first days of brining, reaching a population of 4.96 CFU/ml at day 8, and were not detected at day 20 when the pH was below 5 (Figures 1B,C). The population of LAB was very low in comparison to those found by other researchers in the spontaneous table olive fermentation of different cultivars processed as Spanish-style such as Conservolea (Chranioti et al., 2018), Manzanilla and Gordal (De Castro et al., 2018, 2019). Usually, LAB are detected after 48–72 h of brining (Lanza, 2013). Among the factors that could have limited the adaptation of LAB are the brine environment and nutrient availability (Grounta and Panagou, 2017). The low concentration of sugars at the beginning of fermentation and their consumption by other microorganisms like mesophilic aerobes, yeasts, or Enterobacteria delayed the growth of LAB. Conversely, the industrial brines showed a high LAB population in a range of 4.32–7.52 log CFU/ml at the end of fermentation (Table 3), following those observed by previous authors (except for samples Sig. 3f, Sig. 4, and Sev. 1).
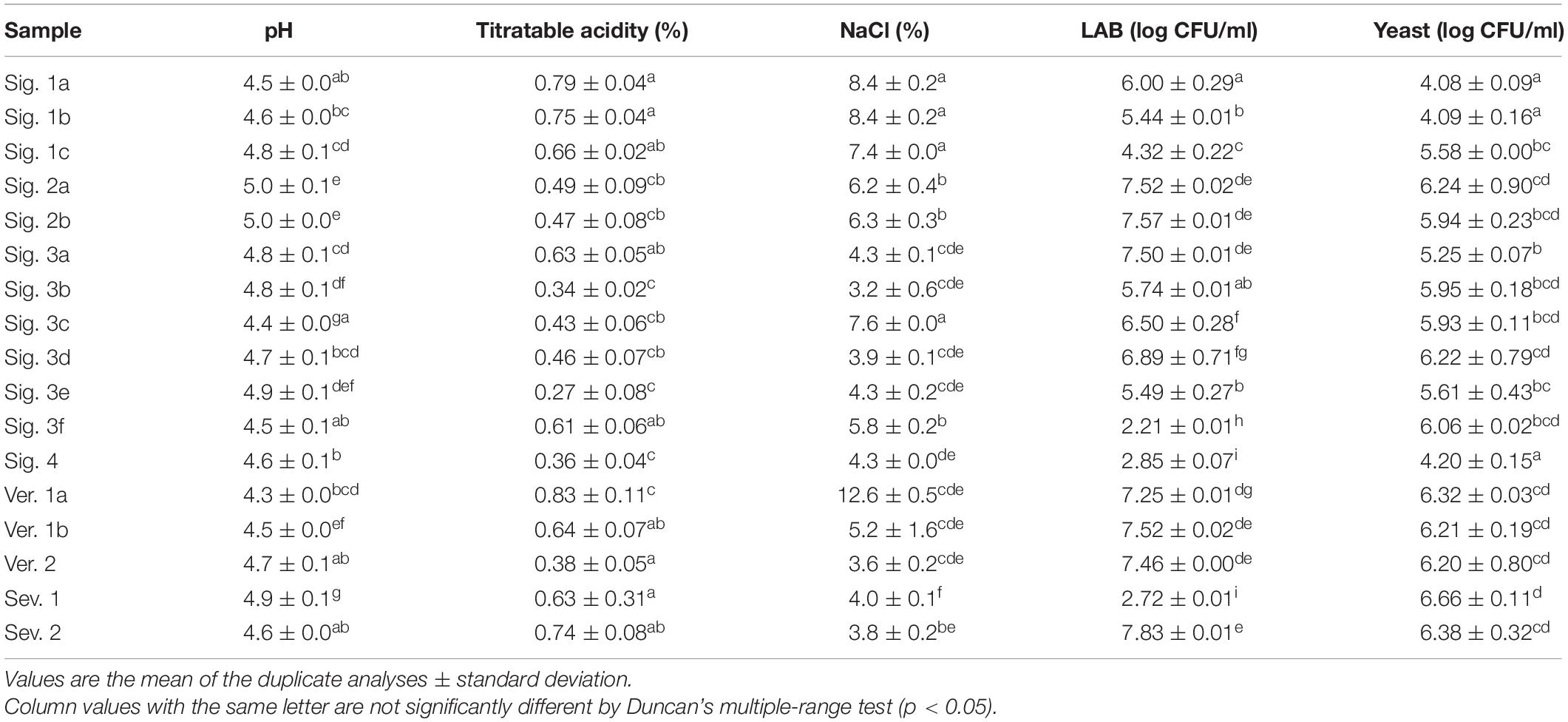
Table 3. Chemical characteristics and viable microbial population of industrial Spanish-style green olive brines after fermentation.
As expected, sugars were the primary source of carbon for the fermentative microorganisms to produce organic acids during the fermentation process, and consequently, the titratable acidity increased, and the pH decreased (Figure 1C). Most of the industrial samples reached a titratable acidity between 0.43 and 0.85%, but four of them maintained lower values (Table 3). Higher acidity values are usually achieved at the final stage of fermentation, as those found by Lucena-Padrós and Ruiz-Barba (2019) for industrial table olive brines of Manzanilla. Conversely, Chranioti et al. (2018) also found low acidity during the fermentation of Conservolea and it was attributed to the low content of sugars in the brine, as observed in our study. De Castro et al. (2019) noticed acidity values below 0.40% for uninoculated Manzanilla and Gordal table olive brines at 120 days of fermentation, and were corrected with the supplementation of glucose to reach values around 0.60%, extending the fermentation time.
Lactic acid was the main organic acid detected in the industrial table olive brines and was correlated with the evolution of the LAB during the fermentation process due to their fermentative metabolism (Figure 1D). It should be noted that the low concentration of lactic acid generated in the industrial brine samples may be due to the lack of fermentable sugars in the brines (Table 2) caused by excessive washing of the fruits during the elaboration steps (Table 1). Furthermore, the presence of certain polyphenols with antimicrobial activity can inhibit the growth of LAB (Medina et al., 2007). The brine samples showed an average total phenols concentration of 1,821 ppm (Table 2) although the antimicrobial phenolic compounds were not detected. The phenolic content of olives is drastically affected by the alkaline treatment and the successive washing stages during the elaboration as Spanish-style table olives (Medina et al., 2008; Mettouchi et al., 2016; Ait Chabane et al., 2019). In addition, the LAB population showed values below 2.85 log CFU/ml in some specific samples (Table 3), and the high concentration of NaCl of the initial brines could also inhibit the growth of them in favor of yeasts (Arroyo-López et al., 2012). It should be noted that despite the low number of LAB, other groups of microorganisms such as certain Enterobacteriaceae, present in the first step of fermentation, are capable of producing lactic acid although with a lower yield. In studies carried out with Manzanilla, Gordal, and Hojiblanca varieties, researchers observed that the LAB population was more abundant than yeasts, considered to be a good sign of an adequate fermentation (Sánchez et al., 2018; De Castro et al., 2019). The formation of acetic acid and the ethanol in our samples implies a high activity of yeast metabolism. The dominance of yeasts during the fermentation process creates a product with a milder taste and less self-preservation due to a nutrient competition for nutrients with LAB and the subsequent low acid production (Arroyo-López et al., 2012).
The alteration of the aroma and the presence of these bad odors (rancid butter, putrid, etc.) were observed for all the samples at the time of sampling, although they showed different intensities that could not be quantified by a sensorial panel. Among the volatile organic acids, the presence of butyric, isobutyric, and propionic acids in many of the industrial brines (Table 2) must be related to the growth of undesirable spoilage microorganisms (De Castro et al., 2018) whose appearance in table olives is related to an inadequate pH and salt concentration.
Although initial NaCl concentrations were above 10% (data provided by factories), almost half of the samples showed a concentration of NaCl less than 5% (Table 3), which involves a lack of control and correction during the elaboration process. De Castro et al. (2018) noticed that table olive samples with low NaCl concentration developed an off-odor typically deriving from butyric and putrid fermentation. Also, Tataridou and Kotzekidou (2015) considered that NaCl concentration less than 4% induces the formation of a mold layer on the top of the fermentation tank, that also causes alterations in the final table olives. In general, a combination of a salt concentration higher than 5% and a pH below 4.0 are limiting factors of undesirable microbial growth and guarantee correct preservation conditions (Rejano-Navarro et al., 2010; Arroyo-López et al., 2012; Tataridou and Kotzekidou, 2015). As observed in Table 3, the pH values of the samples ranged from 5.0 to 4.3, higher than those reached by other authors for Spanish-style table olives (Tataridou and Kotzekidou, 2015; Lucena-Padrós and Ruiz-Barba, 2019). A slight increase in pH during fermentation could enhance the spoilage produced by Enterobacteriaceae and other microbial groups (Lanza, 2013), and maintaining pH values below 4.6 could also prevent the germination of spores of C. botulinum (Valero et al., 2020). Considering the multiple factors that will influence undesirable microbiota, both spoilage and pathogenic, it was deemed relevant to carry out a genomic analysis to know the relative abundance of the different microorganisms present in selected industrial brines samples.
The high throughput sequencing of 16S rRNA gene generated a total of 1,088,785 high-quality sequences with a mean of 136,098 sequences per sample (Supplementary Table 1). Diversity estimators (Shannon, Simpson, and Chao1) were used to analyze the microbial community (Supplementary Table 1). Supplementary Table 2 shows the 63 main bacterial OTUs assigned at family, genus, or species shared among all samples studied.
The Lactiplantibacillus genus was the predominant bacterial OTU in all the samples analyzed, showing a relative abundance of 87.5–46.2% (Figure 2). This genus has been also identified as the most abundant in natural table olives (Anagnostopoulos et al., 2020; López-García et al., 2021), and in commercial table olives (Benítez-Cabello et al., 2020). The dominance of species such as Lactiplantibacillus pentosus and Lactiplantibacillus plantarum has been well described in table olive fermentation as the main lactic acid producer of microorganisms, but it is tough to identify at a species level using 16S sequencing (Benítez-Cabello et al., 2019; Bautista-Gallego et al., 2020). Furthermore, other species such as Levilactobacillus brevis, Loigolactobacillus coryniformis, and Paucilactobacillus oligofermentans were detected in the brines with maximum relative abundance values of 8.2, 22.1, and 20.4% for specific samples, respectively. Moreover, other LAB belonging to Leuconostoc, Pediococcus, Oenococcus, or Enterococcus genera, previously described by other authors in table olive fermentations (De Castro et al., 2002; Medina et al., 2018; Anagnostopoulos et al., 2020; Benítez-Cabello et al., 2020; López-García et al., 2021), were also detected with a broad representation in the samples.
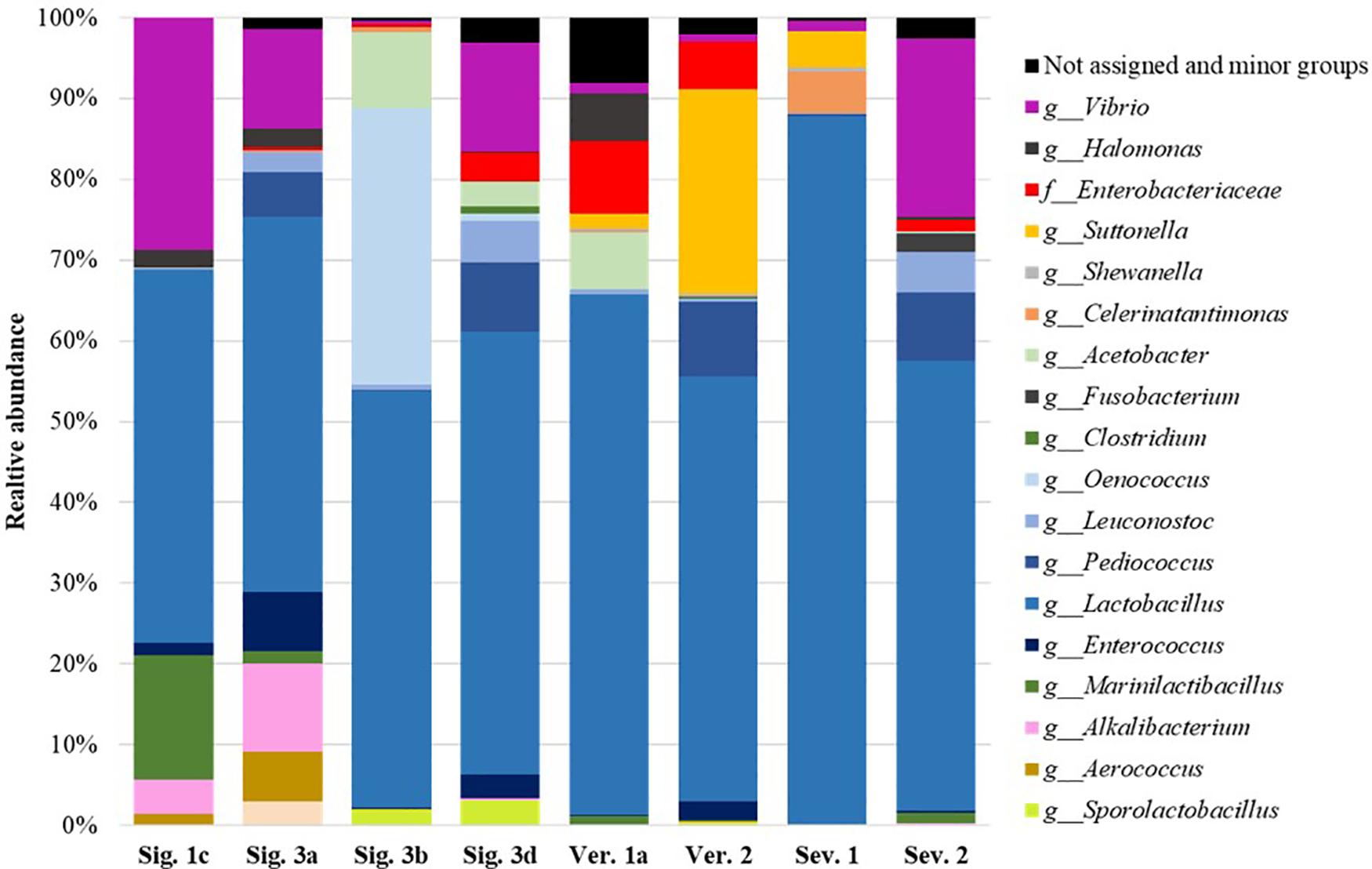
Figure 2. Relative abundance (%) of the bacterial genera or family obtained by sequencing analysis of selected industrial samples after fermentation.
The Enterobacteriaceae family is frequently found in table olives after brining when pH is still alkaline or neutral and decreases during the first days of fermentation (Lanza, 2013; Anagnostopoulos et al., 2020; Benítez-Cabello et al., 2020). In fact, Enterobacteriaceae were not detected by plating after 20 days in Sig. 4 brine (Figure 1), and in any samples at the end of fermentation, except for the brine Ver. 2 at 120 days, which showed a 3.38 × 104 CFU/ml. However, Enterobacteriaceae were found with high abundance in the samples analyzed by sequencing (Figure 2 and Supplementary Table 2). Benítez-Cabello et al. (2020) also found Enterobacteriaceae present in the most of commercial table olives but with median values below 0.15% of relative abundance. Most of the time, their presence does not cause side effects, but it is well known that certain species or strains can cause illnesses. Selected colonies from VRBD plates isolated from Ver. 2 brine were identified by 16S sequencing as Providencia rettgeri, Providencia vermicola, and Proteus vulgaris, previously identified in Arbequina (Hurtado et al., 2008) and Nocellara Etnea table olive fermentations (Randazzo et al., 2017). These species can cause gastroenteritis and urinary tract infections (Chart, 2012; Wang and Pan, 2014). Apart from the pathogenic species included in this family, these bacteria cause the formation of gas-pocket in the olive fruits and produce metabolites that generate off-odors and off flavors in the final products (Lanza, 2013).
The high pH of some industrial brines above 4.6 (Table 3) denotes that an appropriate lactic fermentation did not occur. Also, linked to the low NaCl concentration, undesirable microorganisms responsible for secondary fermentations could be acting. The presence of Pectinatus brassicae (in five out of seven samples analyzed, brines Sig. 3b, Sig. 3d, Ver. 1a, Ver. 2, and Sev. 2) and Lentilactobacillus buchneri (in brines Ver. 2 and Sev. 2) (Supplementary Table 2) suggests an initial stage of secondary fermentation as previously described for pickle fermentations (Breidt et al., 2013; Johanningsmeier and McFeeters, 2013; Medina et al., 2016). These microorganisms can metabolize lactic acid, produced by fermentation, into propionic acid, as detected in these brines (Table 2). Likewise, the presence of Acetobacter genus in five out of seven brines (brines Sig. 3b, Sig. 3d, Ver. 1a, Ver. 2, and Sev. 2) plays an essential role in the onset of spoilage by converting the lactic acid into acetic acid (Johanningsmeier and McFeeters, 2013; Medina et al., 2016), as well as certain oxidative yeasts (Franco and Pérez-Díaz, 2012; Medina-Pradas and Arroyo-López, 2015). Only the brine sample Sig. 1c did not show butyric, isobutyric, and propionic acids, which is related to the absence of the Pectinatus and Acetobacter genera (Table 2 and Supplementary Table 2).
Lactic acid consumption in table olives compromises the preservative power of fermented olives and increases the pH values, which can allow for the growth of other undesirable microorganisms detected in the samples as the Clostridium and Enterobacter genera, also found in some analyzed samples, which can metabolize the lactic acid and generate butyric and propionic acids, respectively (Franco and Pérez-Díaz, 2012; Breidt et al., 2013). Both generate aromas of manure, decomposing organic matter or rancid butter, symptoms of putrid and butyric fermentations (Gililland and Vaughn, 1943) or promote the so-called “zapatería” in spoilt table olives (Kawatomari and Vaughn, 1956; Plastourgos and Vaughn, 1957; Bautista-Gallego et al., 2020). This malodorous fermentation produces olives that are completely unacceptable to the consumer.
From a food safety point of view, most of the brines analyzed in this study showed a pH above 4.6, which could favor the germination of C. botulinum spores (Medina-Pradas and Arroyo-López, 2015). Although this species has not been identified in our samples by massive sequencing, these chemical characteristics do not guarantee the safety of the product. Table olives after the fermentation should maintain a pH < 4.0 and the NaCl in brine >5% to prevent any possibility of growth of this foodborne pathogen (Lanza, 2013).
The Vibrio genus was also present in all the samples reaching relative abundance even greater than 20% in specific brines (Figure 2 and Supplementary Table 2). It is common to find this genus in table olive fermentation brines and its origin is the salt or marine sources used for the brines (Lucena-Padrós et al., 2015; Medina et al., 2018; Lucena-Padrós and Ruiz-Barba, 2019; Benítez-Cabello et al., 2020). However, within this genus, Vibrio metschnikovii has been identified as a pathogenic bacterium associated with human diseases causing gastroenteritis or septicemia (Matté et al., 2007).
In addition to the aforementioned microorganisms, it is also worth highlighting the prominent presence of specific genera in the industrial brines studied, such as the Celerinatantimonas genus, previously identified at the end of fermentation in directly brined table olives (Medina et al., 2018; López-García et al., 2021), in commercial table olives (Benítez-Cabello et al., 2020) and as the dominant bacteria in black olive natural fermentation (Penland et al., 2020). The role played by this microorganism during the fermentation of table olives is still unknown, but it was linked to the pH decrease and acetic, citric, and lactic acid production (Penland et al., 2020). Also, the presence of the Marinilactibacillus genus, previously identified in green table olive fermentations (Lucena-Padrós and Ruiz-Barba, 2016; López-García et al., 2021), and in commercialized table olive biofilms (Benítez-Cabello et al., 2020), or the Suttonella genus, previously found in table olive spoilages, was outstanding (De Castro et al., 2018; López-García et al., 2021).
Conclusion
The elaboration of table olives is a complex biochemical process in which the indigenous microbiota present in the fruits consume the nutrients to carry out a lactic fermentation to attain proper preservation. In the table olive samples analyzed from the four Algerian factories, adequate pH and acidity values were not reached to guarantee quality and safe table olives. The high pH values and low acidity are consequences of the low initial concentrations of sugars or because its consumption was carried out by other microorganisms different from lactic acid bacteria. High-throughput sequencing revealed the presence of undesirable microorganisms in the cover brines, some of them considered to be foodborne pathogens, so microbial stability is not guaranteed in these fermented table olives.
The Algerian elaboration process can be improved by reducing the washing steps to a single washing (6–12 h), which would avoid the loss of fermentable matter and other biocompounds, and also help to reduce the amount of wastewater originated in the process, thus minimizing the environmental impact. Likewise, the addition of external fermentable matter and/or acid at the beginning of brining is a widespread practice among processors, and would help toward good growth of LAB and inhibit Enterobacteria and other undesirable microbiota, avoiding the secondary fermentations that make the product unacceptable to the consumer. Also, the use of starter cultures favors the growth of LAB, supplanting the undesirable indigenous microbiota and guaranteeing a proper fermentation for the maintenance of the correct preservation conditions. From the point of view of food safety, most of the brines analyzed in this study showed a pH above 4.6, which could favor the germination of C. botulinum spores (reference microorganism).
Table olives should maintain a NaCl concentration above 5% throughout the elaboration process, and keep values of pH below 4.0 after the fermentation, the time in which it is recommended that NaCl is raised above 8% to prevent any possibility of growth of spoilage microorganisms and foodborne pathogens, and guarantee high-quality standards.
Data Availability Statement
The original contributions presented in the study are included in the article/Supplementary Material, further inquiries can be directed to the corresponding author/s.
Author Contributions
CS, EM, MB, and AO: conception and design of study. CS, EM, CR, and AM: acquisition data. CS, EM, CR, AM, and MB: analysis and/or interpretation of data. CS and EM: drafting the manuscript. CR, MB, AO, and AM: revising the manuscript. All authors contributed to the article and approved the submitted version.
Funding
This research was supported by the Algerian Ministry of Higher Education and Scientific Research (PNE program 2019-2020), and the grant RYC2018-024752-I funded by MCIN/AEI/10.13039/501100011033 and FSE “El FSE invierte en tu futuro”.
Conflict of Interest
The authors declare that the research was conducted in the absence of any commercial or financial relationships that could be construed as a potential conflict of interest.
Publisher’s Note
All claims expressed in this article are solely those of the authors and do not necessarily represent those of their affiliated organizations, or those of the publisher, the editors and the reviewers. Any product that may be evaluated in this article, or claim that may be made by its manufacturer, is not guaranteed or endorsed by the publisher.
Supplementary Material
The Supplementary Material for this article can be found online at: https://www.frontiersin.org/articles/10.3389/fmicb.2021.729436/full#supplementary-material
References
Ait Chabane, F., Rovellini, P., Boucheffa, S., Medina, E., and Tamendjari, A. (2019). Quality of lipid fraction during Spanish-style table olives processing of Sigoise and Azzeradj cultivars. Food Control. 111:e107059. doi: 10.1016/j.foodcont.2019.107059
Alves, M., and Quintas, C. (2016). “Traditional green table olives from the south of portugal in traditional foods,” in Integrating Food Science And Engineering Knowledge Into The Food Chain, eds K. Kristbergsson and J. Oliveira (Boston, MA: Springer), 367–375. doi: 10.1007/978-1-4899-7648-2_30
Anagnostopoulos, D. A., Kamilari, E., and Tsaltas, D. (2020). Evolution of bacterial communities, physicochemical changes and sensorial attributes of natural whole and cracked picual table olives during spontaneous and inoculated fermentation. Front. Microbiol. 11:1128. doi: 10.3389/fmicb.2020.01128
Arroyo-López, F. N., Romero-Gil, V., Bautista-Gallego, J., Rodríguez-Gómez, F., Jiménez-Díaz, R., and García-García, P. (2012). Yeasts in table olive processing: desirable or spoilage microorganisms. Int. J. Food Microbiol. 160, 42–49. doi: 10.1016/j.ijfoodmicro.2012.08.003
Ashaolu, T. J., and Reale, A. (2020). A Holistic review on Euro-Asian lactic acid bacteria fermented cereal and vegetables. Microorganisms 8:1176. doi: 10.3390/microorganisms8081176
Bautista-Gallego, J., Medina, E., Sánchez, B., Benítez-Cabello, A., and Arroyo- López, F. N. (2020). Role of lactic acid bacteria in fermented vegetables. Grasas Aceites 71:e358. doi: 10.3989/gya.0344191
Benítez-Cabello, A., Rodríguez-Gómez, F., Morales, M. L., Garrido-Fernández, A., Jiménez-Díaz, R., and Arroyo-López, F. N. (2019). Lactic acid bacteria and yeast inocula modulate the volatile profile of Spanish-style green table olive fermentations. Foods 8:e280. doi: 10.3390/foods8080280
Benítez-Cabello, A., Romero-Gil, V., Medina-Pradas, E., Garrido-Fernández, A., and Arroyo-López, F. N. (2020). Exploring bacteria diversity in commercialized table olive biofilms by metataxonomic and compositional data analysis. Sci. Rep. 10:11381. doi: 10.1038/s41598-020-68305-7
Breidt, F., Medina, E., Wafa, D., Pérez-Díaz, I., Franco, W., Huang, H., et al. (2013). Characterization of cucumber fermentation spoilage bacteria by enrichment culture and 16S rDNA cloning. J. Food Sci. 78:M470. doi: 10.1111/1750-3841.12057
Brenes, M., and de Castro, A. (1998). Transformation of oleuropein and its hydrolysis products during Spanish-style green olive processing. J. Sci. Food Agric. 77, 353–358. doi: 10.1002/(SICI)1097-0010(199807)77:3<353::AID-JSFA50>3.0.CO;2-G
Chart, H. (2012). “Klebsiella, Enterobacter, proteus and other Enterobacteria: pneumonia; urinary tract infection; opportunist infection,” in Medical Microbiology, 18th Edn, eds D. Greenwood, M. Barer, R. Slack, and W. Irving (London: Elsevier), 290–297.
Chranioti, C., Kotzekidoub, P., and Gerasopoulosa, D. (2018). Effect of starter cultures on fermentation of naturally and alkali-treated cv. Conservolea green olives. LWT Food Sci. Technol. 89, 403–408. doi: 10.1016/j.lwt.2017.11.007
Conte, P., Fadda, C., Del Caro, A., Urgeghe, P. P., and Piga, A. (2020). Table olives: an overview on effects of processing on nutritional and sensory quality. Foods 9:514.
De Castro, A., Montaño, A., Casado, F. J., Sánchez, A. H., and Rejano, L. (2002). Utilization of Enterococcus casseliflavus and Lactobacillus pentosus as starter culture for Spanish-style green olive fermentation. Food Microbiol. 19, 637–644.
De Castro, A., Sánchez, A. H., Cortés-Delgado, A., López-López, A., and Montaño, A. (2019). Effect of Spanish-style processing steps and inoculation with Lactobacillus pentosus starter culture on the volatile composition of cv. Manzanilla green olives. Food Chem. 271, 543–549. doi: 10.1016/j.foodchem.2018.07.166
De Castro, A., Sánchez, A. H., López-López, A., Cortés-Delgado, A., Medina, E., and Montaño, A. (2018). Microbiota and metabolite profiling of spoiled Spanish-style green table olives. Metabolites 8:e73. doi: 10.3390/metabo8040073
Franco, W., and Pérez-Díaz, I. M. (2012). Role of selected oxidative yeasts and bacteria in cucumber secondary fermentation associated with spoilage of the fermented fruit. Food Microbiol. 32, 338–344. doi: 10.1016/j.fm.2012.07.013
Garcia-Villalba, R., Giménez-Bastida, J. A., Garcia-Conesa, M. T., Tomás-Barberán, F. A., Espín, J. C., and Larrosa, M. (2012). Alternative method for gas chromatography-mass spectrometry analysis of short-chain fatty acids in faecal samples. J. Sep. Sci. 35, 1906–1913. doi: 10.1002/jssc.201101121
Gililland, J. R., and Vaughn, R. H. (1943). Characteristics of butyric acid bacteria from olives. J. Bacteriol. 46, 315–322.
Grounta, A., and Panagou, P. (2017). “Olives fermentation,” in Lactic Acid Fermentation Of Fruits And Vegetables, ed. S. Paramithiotis (Boca Ratón, FL: CRC Press), 157–180.
Heperkan, D. (2013). Microbiota of table olive fermentations and criteria of selection for their use as starters. Front. Microbiol. 4:e143. doi: 10.3389/fmicb.2013.00143
Hurtado, A., Reguant, C., Esteve-Zarzoso, B., Bordons, A., and Rozès, N. (2008). Microbial population dynamics during the processing of Arbequina table olives. Food Res. Int. 41, 738–744. doi: 10.1016/j.foodres.2008.05.007
International Olive Council [IOC] (2004). Trade Standard Applying To Table Olives. Document COI/OT/NC No. 1. Madrid: IOC.
International Olive Council [IOC] (2021). Available online at: https://www.internationaloliveoil.org/wp-content/uploads/2020/12/OT-W901-23-11-2020-P.pdf. [Accessed June 10, 2021].
Johanningsmeier, S. D., and McFeeters, R. F. (2013). Metabolism of lactic acid in fermented cucumbers by Lactobacillus buchneri and related species, potential spoilage organisms in reduced salt fermentations. Food Microbiol. 35, 129–135. doi: 10.1016/j.fm.2013.03.004
Kawatomari, T., and Vaughn, R. H. (1956). Species of clostridium associated with zapatera spoilage of olives. J. Food. Sci. 21, 481–490.
Lanza, B. (2013). Abnormal fermentations in table-olive processing: microbial origin and sensory evaluation. Front. Microbiol. 4:e91. doi: 10.3389/fmicb.2013.00091
López-García, E., Benítez-Cabello, A., Ramiro-García, J., Romero-Gil, V., Rodríguez-Gómez, F., and Arroyo-López, F. N. (2021). New insights into microbial diversity of the traditional packed table olives aloreña de málaga through metataxonomic analysis. Microorganisms 9:561. doi: 10.3390/microorganisms9030561
Lucena-Padrós, H., and Ruiz-Barba, J. L. (2016). Diversity and enumeration of halophilic and alkaliphilic bacteria in Spanish-style green table-olive fermentations. Food Microbiol. 53, 53–62. doi: 10.1016/j.fm.2015.09.006
Lucena-Padrós, H., and Ruiz-Barba, J. L. (2019). Microbial biogeography of Spanish-style green olive fermentations in the province of Seville, Spain. Food Microbiol. 82, 259–268. doi: 10.1016/j.fm.2019.02.004
Lucena-Padrós, H., González, J. M., Caballero-Guerrero, B., Ruiz-Barba, J. L., and Maldonado-Barragán, A. (2015). Vibrio olivae sp. nov., isolated from Spanish-style green-olive fermentations. Int. J. Sys. Evol. Microbiol. 65, 1895–1901. doi: 10.1099/ijs.0.000196
Matté, M. H., Baldassi, L., Barbosa, M. L., Malucelli, M. I. C., Nitrini, S. M. O. O., and Matté, G. R. (2007). Virulence factors of Vibrio metschnikovii strains isolated from fish in Brazil. Food Control. 18, 747–751. doi: 10.1016/j.foodcont.2006.03.012
Medina, E., Brenes, M., García-García, P., Romero, C., and de Castro, A. (2018). Microbial ecology along the processing of Spanish olives darkened by oxidation. Food Control. 86, 35–41. doi: 10.1016/j.foodcont.2017.10.035
Medina, E., Brenes, M., Romero, C., García, A., and de Castro, A. (2007). Main antimicrobial compounds in table olives. J. Agric. Food Chem. 55, e9817–e9823.
Medina, E., Pérez-Díaz, I. M., Breidt, F., Hayes, J. S., Franco, W., Butz, N., et al. (2016). Bacterial ecology of fermented cucumber rising pH spoilage as determined by non-culture based methods. J. Food Sci 81, M121–M129. doi: 10.1111/1750-3841.13158
Medina, E., Romero, C., de Castro, A., Brenes, M., and García, A. (2008). Inhibitors of lactic acid fermentation in Spanish-style green olive brines of the Manzanilla variety. Food Chem. 110, 932–937. doi: 10.1016/j.foodchem.2008.02.084
Medina-Pradas, E., and Arroyo-López, F. N. (2015). Presence of toxic microbial metabolites in table olives. Front. Microbiol. 6:e873. doi: 10.3389/fmicb.2015.00873
Medina-Pradas, E., Pérez-Díaz, I. M., Garrido-Fernández, A., and Arroyo-López, F. N. (2017). “Review of vegetable fermentations with particular emphasis on processing modifications, microbial ecology, and spoilage,” in The Microbiological Quality Of Food, eds A. Bevilacqua, M. R. Corbo, and M. Sinigaglia (New York, NY: Elsevier), 211–230.
Mettouchi, S., Sacchi, R., Ould-Moussa, Z. E. D., Paduano, A., Savarese, M., and Tamendjari, A. (2016). Effect of Spanish style processing on the phenolic compounds and antioxidant activity of Algerian green table olives. Grasas Aceites 67:e114. doi: 10.3989/gya.0378151
Montaño, A., de Castro, A., Rejano, L., and Sánchez, A. H. (1992). Analysis of zapatera olives by gas and high-performance liquid chromatography. J. Chromatogr. 594, 259–267. doi: 10.1016/0021-9673(92)80338-u
Panagou, E., and Tassou, C. C. (2006). Changes in volatile compounds and related biochemical profile during controlled fermentation of cv. Conservolea green olives. Food Microbiol. 23, 738–746. doi: 10.1016/j.fm.2006.02.005
Papadaki, E., and Mantzouridou, F. (2016). Current status and future challenges of table olive processing wastewaters valorization. Biochem. Eng. J. 112, 103–113.
Penland, M., Deutsch, S. M., Falentin, H., Pawtowski, A., Poirier, E., Visenti, G., et al. (2020). Deciphering microbial community dynamics and biochemical changes during nyons black olive natural fermentations. Front. Microbiol. 11:586614. doi: 10.3389/fmicb.2020.586614
Pérez-Díaz, I. M., Hayes, J. S., Medina, E., Webber, A. M., Butz, N., Dickey, A. N., et al. (2019). Assessment of the non-lactic acid bacteria microbiota in fresh cucumbers and commercially fermented cucumber pickles brined with 6% NaCl. Food Microbiol. 77, 10–20. doi: 10.1016/j.fm.2018.08.003
Plastourgos, S., and Vaughn, R. H. (1957). Species of Propionibacterium associated with zapatera spoilage of olives. Appl. Microbiol. 5, 267–271. doi: 10.1128/am.5.4.267-271.1957
Randazzo, C., Todaro, A., Pino, A., Pitino, I., Corona, O., and Caggia, C. (2017). Microbiota and metabolome during controlled and spontaneous fermentation of Nocellara Etnea table olives. Food Microbiol. 65, 136–148. doi: 10.1016/j.fm.2017.01.022
Rejano-Navarro, L., Montaño, A., Casado, F. J., Sánchez, A. H., and de Castro, A. (2010). “Table olives: varieties and variations,” in Olives and Olive Oil in Health and Disease Prevention, eds V. R. Preedy and R. R. Watson (Cambridge, MA: Academic Press), 5–15.
Ruiz-Barba, J. L., Maldonado, A., and Jimenez-Díaz, R. (2005). Small-scale total DNA extraction from bacteria and yeast for PCR applications. Anal. Biochem. 347, 333–335. doi: 10.1016/j.ab.2005.09.028
Sánchez, A. H., López-López, A., Cortés-Delgado, A., Beato, V. M., Medina, E., de Castro, A., et al. (2018). Effect of post-fermentation and packing stages on the volatile composition of Spanish-style green table olives. Food Chem. 239, 343–353. doi: 10.1016/j.foodchem.2017.06.125
Sánchez, A. H., Rejano, L., Montaño, A., and de Castro, A. (2001). Utilization at high pH of starter cultures of lactobacilli for Spanish-style green olive fermentation. Int. J. Food Microbiol. 67, 115–122. doi: 10.1016/s0168-1605(01)00434-2
Sánchez-Gómez, A. H., García-García, P., and Rejano-Navarro, L. (2006). Trends in table olive production. Grasas Aceites 57, 86–94.
Tataridou, M., and Kotzekidou, M. (2015). Fermentation of table olives by oleuropeinolytic starter culture in reduced salt brines and inactivation of Escherichia coli O157:H7 and Listeria monocytogenes. Int. J. Food Microbiol. 208, 122–130. doi: 10.1016/j.ijfoodmicro.2015.06.001
Valero, A., Olague, E., Medina-Pradas, E., Garrido-Fernández, A., Romero-Gil, V., Cantalejo, M. J., et al. (2020). Influence of acid adaptation on the probability of germination of Clostridium sporogenes spores against pH, NaCl and time. Foods 9:127. doi: 10.3390/foods9020127
Keywords: lactic acid fermentation, spoilage, Sevillana, Verdale, Sigoise
Citation: Sab C, Romero C, Brenes M, Montaño A, Ouelhadj A and Medina E (2021) Industrial Processing of Algerian Table Olive Cultivars Elaborated as Spanish Style. Front. Microbiol. 12:729436. doi: 10.3389/fmicb.2021.729436
Received: 23 June 2021; Accepted: 07 October 2021;
Published: 04 November 2021.
Edited by:
Cinzia Lucia Randazzo, University of Catania, ItalyReviewed by:
Anna Reale, Institute of Food Sciences, National Research Council, Consiglio Nazionale delle Ricerche (CNR), ItalyDimitrios A. Anagnostopoulos, University of Thessaly, Greece
Copyright © 2021 Sab, Romero, Brenes, Montaño, Ouelhadj and Medina. This is an open-access article distributed under the terms of the Creative Commons Attribution License (CC BY). The use, distribution or reproduction in other forums is permitted, provided the original author(s) and the copyright owner(s) are credited and that the original publication in this journal is cited, in accordance with accepted academic practice. No use, distribution or reproduction is permitted which does not comply with these terms.
*Correspondence: Eduardo Medina, emedina@ig.csic.es