- Department of Medical Microbiology and Infectious Diseases, Erasmus MC University Medical Center, Rotterdam, Netherlands
The role of plasmids in the complex pandemic of antimicrobial resistance is increasingly being recognized. In this respect, multiple mobile colistin resistance (mcr) gene-carrying plasmids have been described. However, the characteristics and epidemiology of these plasmids within local healthcare settings are largely unknown. We retrospectively characterized the genetic composition and epidemiology of plasmids from mcr-1-positive bacterial isolates identified from patients from a large academic hospital in the Netherlands. Clinical Gram-negative bacteria with an MIC > 2 μg/mL for colistin, obtained from patients hospitalized at the Erasmus MC University Medical Center Rotterdam during the years 2010–2018, were screened for presence of the mcr-1 gene. Extracted plasmids from mcr-1-positive isolates were sequenced using a combination of short- and long-read sequencing platforms, characterized by incompatibility type and genetic composition and compared to publicly available mcr-1-carrying plasmid sequences. In 21 isolates from 14 patients, mcr-1 was located on a plasmid. These plasmids were of diverse genetic background involving Inc types IncX4, IncI2(delta), IncHI2, as well as double Inc types IncHI2/IncN and IncHI2/IncQ. mcr-1-carrying plasmids were found in Escherichia coli, Klebsiella pneumoniae, and Kluyvera georgiana, and within the chromosome of an ST147 K. pneumoniae isolate. In depth analysis indicated intrapatient, interpatient, and interspecies transmission events of mcr-1-carrying plasmids. In addition, our results show that the mcr-1 gene resides in a rich environment full of other (mcr-1 negative) plasmids and of many different Inc types, enabling interplasmidal transfer events and facilitating widespread dissemination of the mcr-1 gene. Multiple mcr-1-carrying plasmid transmission events had likely occurred among isolates from hospitalized patients. Recognition and identification of plasmid transmission events within hospitals is necessary in order to design and implement effective infection control measures.
Introduction
The plasmid-mediated mobile colistin resistance gene (mcr-1) was first reported in 2015 and has been detected across all continents (Liu et al., 2016; Wang R. et al., 2018; Elbediwi et al., 2019). Since then, at least 10 different mcr genes have been identified, with mcr-1 being the most prevalent colistin resistance gene in humans and animals (Irrgang et al., 2016; Ye et al., 2016). The global spread of mcr-1 is a potential challenge to the effective use of antibiotics to treat multi-drug resistant microorganisms, particularly as colistin is considered as an “antibiotic of last resort” (Gregoire et al., 2017). Additionally, the location of mcr-1 within plasmids contributes to the gene’s mobility via horizontal transfer, thus allowing the spread of colistin resistance between different bacterial species. This phenomenon has been observed in livestock and more recently also in healthcare settings (Carattoli, 2013; Liu et al., 2016; Shen et al., 2018; Wang X. et al., 2018).
To date, mcr genes have been detected in plasmids of diverse incompatibility (Inc) types, with IncI2, IncHI2, and InX4 being the most abundant types identified (Matamoros et al., 2017). Plasmids carrying the mcr-1 gene have been mainly described in Escherichia coli and Klebsiella pneumoniae (Terveer et al., 2017; Wang et al., 2017), but have also been identified in other Enterobacterales (Tse and Yuen, 2016; Caselli et al., 2018). From a large study in Parisian hospitals, based on the genetic background of the strains, it was suggested human isolates carrying an mcr-1 plasmid may have an animal origin (Bourrel et al., 2019). In addition, nosocomial spread of the mcr-1 gene or mcr-1-containing plasmids has been rarely reported (Mendes et al., 2018; Mariani et al., 2020). This may be due to the current limited use of plasmid detection and characterization for hospital epidemiology and infection prevention and control purposes.
In this study, we analyzed the epidemiology and genetics of mcr-1-carrying plasmids isolated from patients admitted to a large tertiary care hospital in the Netherlands. We characterized plasmids responsible for the spread of mcr-1 in colistin-resistant bacteria isolated from patients and compared these to publicly available plasmid sequences. As a result, we were able to observe inter- and intrapatient transmission events as well as interspecies transmission events.
Materials and Methods
Study Design
This retrospective observational study was conducted at the Erasmus MC University Medical Center (Erasmus MC) in Rotterdam, the Netherlands. Highly resistant microorganisms (HRMO) and non-HRMO isolates (stored at −80°C) known not to be intrinsically resistant to colistin, from different collections, were included in the study (Supplementary File 1). Bacterial isolates collected from January 2010 until September 2018 were included in the study (Supplementary File 1). Enterobacterales, as well as Acinetobacter baumannii-calcoaceticus complex and Pseudomonas aeruginosa isolates were included (Supplementary File 1), all with an MIC > 2 μg/mL for colistin (as determined by the Vitek 2® system – bioMérieux, Marcy-l’Étoile, France). The applied cut-off value was validated by also screening isolates with an MIC ≤ 2 (Supplementary File 1). Approval to conduct the study was received from the medical ethics research committee of the Erasmus MC (MEC-2015-306).
Total DNA Extraction
A 1 μL loop of bacterial inoculum was suspended in 200 μL phosphate-buffered saline (PBS) solution. DNA extraction was performed using a MagNA Pure 96 (Roche Diagnostics, Basel, Switzerland) and the MagNA Pure DNA/Viral NA SV 2.0 kit with the Pathogen Universal 200 protocol. Total genomic DNA was subjected to amplified fragment length polymorphism (AFLP) fingerprinting (van Burgh et al., 2019) using two optimized conditions for E. coli with restriction enzymes HpyCH4IV and MseI using as selective residues C + GT and G + GA, respectively.
Real-Time PCR
The presence of the mcr-1 gene was determined using real-time PCR. Five μL of purified DNA, amplification primers 5′-GGTGGGTAAGCTTGCCAGTA-3′ (0.5 μM), 5′-GCGTCTTTGGCGTGATAAAT-3′ (0.5 μM) and probe 5′-FAM-AAAAGCCAGTGCGCCAAAAGATACC-BHQ-3′ (0.2 μM) (Eurogentec, Liège, Belgium) were combined in 20 μL amplification reactions in 1x LightCycler® 480 Probes Master (Roche Diagnostics). Thermocycling consisted of an initial denaturation for 5 min at 95°C followed by 50 cycles of 5 s at 95°C and 30 s at 60°C, performed on a LightCycler® 480 instrument (Roche Diagnostics). mcr-1-positive and negative control strains were included in each run.
Plasmid Extraction
A 10 μL loop of bacterial inoculum was suspended in 200 μL PBS solution. Plasmid DNA extraction was performed using the Bioline plasmid miniprep kit (Bioline, Ranst, Belgium). The protocol of the manufacturer was followed for low copy number plasmids including all optional steps, although the speed of all centrifugation steps was reduced to 4,000 × g (except the initial centrifugation step for the lysis of the bacterial cells, which was maintained at 16,000 × g). DNA concentration was measured using the Quant-iT PicoGreen dsDNA Assay Kit (Thermo Fisher Scientific, Waltham, MA, United States).
Total and Plasmid DNA Sequencing
Extracted plasmid DNA and/or total DNA were sequenced using Illumina and Nanopore sequencing platforms. Illumina sequencing library preparation was conducted with the NextEra DNA Flex Library Prep Kit (Illumina, San Diego, CA, United States). Sequencing was conducted using the Illumina iSeq 100 System (Illumina) generating 150 bp paired end reads. Library preparation for Nanopore sequencing was performed using the Rapid Barcoding Sequencing Kit SQK-RBK004 (Oxford Nanopore Technologies, Oxford, United Kingdom) according to the standard protocol described by the manufacturer.
Sequencing Data Assembly and Genome Annotation
Fastq files from Illumina and Nanopore sequencing were combined in a hybrid assembly using Unicycler v0.4 (Wick et al., 2017) with default parameters and visualized using Bandage (Wick et al., 2015). The assembled plasmid sequences were further validated by read mapping and visual checking for assembly errors. Plasmid sequences were annotated using Prokka v1.13 (Seemann, 2014). Syntenic regions were visualized using Geneious v2019.1 (Biomatters, Auckland, New Zealand). Whole plasmid alignment was performed using the progressiveMauve algorithm from Mauve 3.6 (Darling et al., 2004). Plasmidfinder (Carattoli et al., 2014) was used to identify plasmid Inc type(s). In order to distinguish between large plasmids and a possible chromosomal location of the mcr-1 gene, selected bacterial isolates were additionally sequenced via Nanopore sequencing on total genomic DNA. The presence of antibiotic resistance genes/mechanisms were identified using a stand-alone version of RGI (Jia et al., 2017) v5.1.0 based on the CARD database v3.0.5 (including Perfect and Strict hits) and ResFinder (Zankari et al., 2012) 3.2 (database 2019-10-01). Mobilization module characterization was based on MOBscan (Garcillán-Barcia et al., 2020) and the insertion elements were identified based on ISfinder (Siguier et al., 2006).
The NCBI genomic repository was searched for mcr-1 containing sequences1 (2019-05-07) ignoring sequences shorter than 1.5 kbp or larger than 1 Mbp as well as ignoring sequences that were annotated as (cloning) vectors and sequences lacking an Inc type. Search results were further filtered by the presence of mcr-1 by a BLAST search (49) against the mcr-1 sequence (MH143576.1) using default parameters. kSNP v3.01 (Gardner et al., 2015) was used for k-mer based SNP analysis of plasmid sequences of similar Inc types. K-mer chooser was used to define the optimal k-mer value for each Inc type. ITOL (Letunic and Bork, 2016) was used for visualizing the maximum likelihood trees based on core and accessory SNPs. Integron identification was performed using Integron Finder v2.0 (Cury et al., 2016). When applicable, the multi locus sequence type (MLST) was identified based on each species’ corresponding MLST scheme (Jolley et al., 2018).
Results
Patient and Strain Characteristics
Three hundred and twenty-nine bacterial isolates were screened from a total of 270 patients. Ninety-six isolates (29.2%) were described as extended-spectrum beta-lactamase (ESBL)-positive, and eight isolates (2.4%) were stored because of the presence of a carbapenemase gene (Supplementary File 1). Real-time PCR demonstrated the presence of the mcr-1 gene in 22 isolates, derived from 14 individual patients The oldest mcr-1 positive isolates in this collection dated back to two patients admitted in 2010. Fourteen out of the 22 mcr-1 positive isolates were ESBL-positive and all isolates were imipenem and meropenem susceptible. The mcr-1 positive samples included isolates of E. coli (n = 19), K. pneumoniae (n = 2) and Kluyvera georgiana (n = 1), and were cultured from rectum (n = 13), urine (n = 6), throat (n = 1), vagina (n = 1), and blood (n = 1).
Eight out of 14 patients were male (57.1%), and the mean age was 51 (range 12–73). Five adult patients and two pediatric patients were treated for a hematologic disease at the moment of their positive culture. None of the 14 patients died within 28 days after the first positive culture. Out of the 14 patients, seven patients were previously admitted to the Erasmus MC, and two patients were hospitalized abroad within 1 year before the positive culture. No additional mcr-1 positive isolates were identified when screening isolates with an MIC ≤ 2 (Supplementary File 1).
Plasmid Architecture
Hybrid assembly of short and long sequencing reads generated multiple plasmid sequences per isolate (Tables 1, 2 and Supplementary Table 1).
All mcr-1 genes encoded the mcr-1.1 variant. The mcr-1 gene was surrounded by different genes in different Inc type plasmids, but for each Inc type there were remarkable similarities. MOBscan analysis indicated the presence of relaxases encoding genes of MOBH (Plasmid 2, 10, 19, and 20) and MOBP (Plasmid 1, 3, 4, 5, 6, 7, 8, 11, 12, 13, 14, 15, 16, 18, and 21). For two plasmids (9 and 17) no result was obtained. Analysis of mcr-1 plasmid architectures revealed regions with similarities among the plasmids (Figure 1), with a conserved group of 23 genes – dedicated to pili formation and conjugal transfer – in IncI2(delta) mcr-1 plasmids (Figure 1).
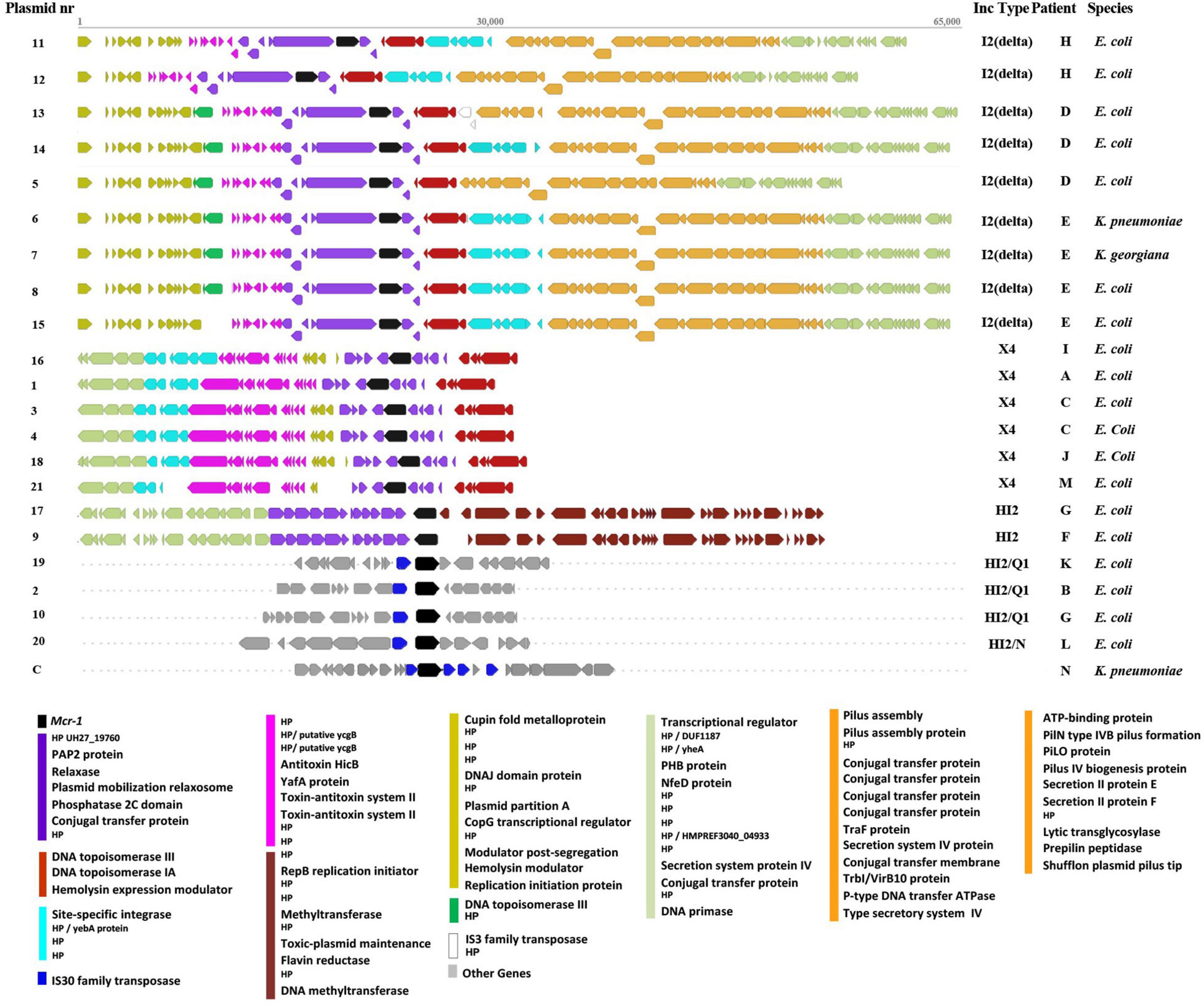
Figure 1. Graphical representation of genomic regions in plasmids containing the mcr-1 gene and annotated using Prokka. Conserved regions as defined by Mauve alignment are represented by different colors. Each arrow represents a gene and dots indicate that the presented region is part of a much larger plasmid/chromosome. HP, hypothetical protein encoding gene.
Although two IncHI2 plasmids were detected of approximately 55 kbp and 206 kbp, respectively, three other mcr-1 positive IncHI2 plasmids were of a double Inc type. More specifically, in two cases a plasmid with Inc type IncHI2 had merged with another plasmid carrying either IncQ or IncN, resulting in a large plasmid (>200 kbp) of double Inc type (Table 1). These double Inc type mcr-1-carrying plasmids clustered independently from each other and from the plasmids with single IncHI2 type in SNP analysis. While IncI2 is observed as one of the most commonly identified mcr-1-carrying plasmids in public repositories, in our samples we only detected IncI2(delta), as the most prevalent Inc type in our study and no IncI2 was found (Table 2). Three novel additional multi-Inc types plasmids were identified in this study, adding to the known (multi-)Inc type mcr-1-carrying plasmids.
In mcr-1-carrying plasmids, additional antibiotic resistance genes were identified. The blaCTX–M gene encoding CTX-M-64 was detected in five IncI2(delta) plasmids and encoding CTX-M-14 was detected in two IncHI2 mcr-1 plasmids (Supplementary Table 2). Plasmid 20 carried seven antibiotic resistance genes including a blaTEM–1 and aadA while plasmid 2 carried a large number of 21 antibiotic resistance genes, including multiple aminoglycoside antibiotic resistance genes. In addition, plasmids 20 and 2 carried heavy metal resistance genes, with plasmid 20 possessing genes for copper resistance and two operons; one of cation efflux system proteins, and one of four copper resistance proteins. Plasmid 2 carried an operon related to mercury resistance involving mercuric transport proteins and a mercury reductase protein).
In addition to the mcr-1-carrying plasmids, up to eight (average four) other plasmids were identified per strain. The size range of these additional plasmids was 1.3–163 kbp (Supplementary Table 1). Twenty different Inc types were identified in the non-mcr-1 plasmids including the Inc types that have already been described in mcr-1 positive plasmids. Another double Inc type plasmid was observed (IncFIA/IncFIB) in an E. coli isolate.
Plasmid Transmissions in Patients
A comparison of plasmids in this study with publicly available plasmid sequences was made to investigate whether there was transmission or multiple independent acquisitions of similar/identical plasmids between and within patients. A total of 314 plasmid sequences from GenBank were available for comparison (Table 1). Plasmids of selected Inc types were compared in a SNP analysis by kSNP (Figure 2). In general, public plasmid sequences were very different from plasmids from our study as the latter ones formed completely separate branches in the dendrograms.
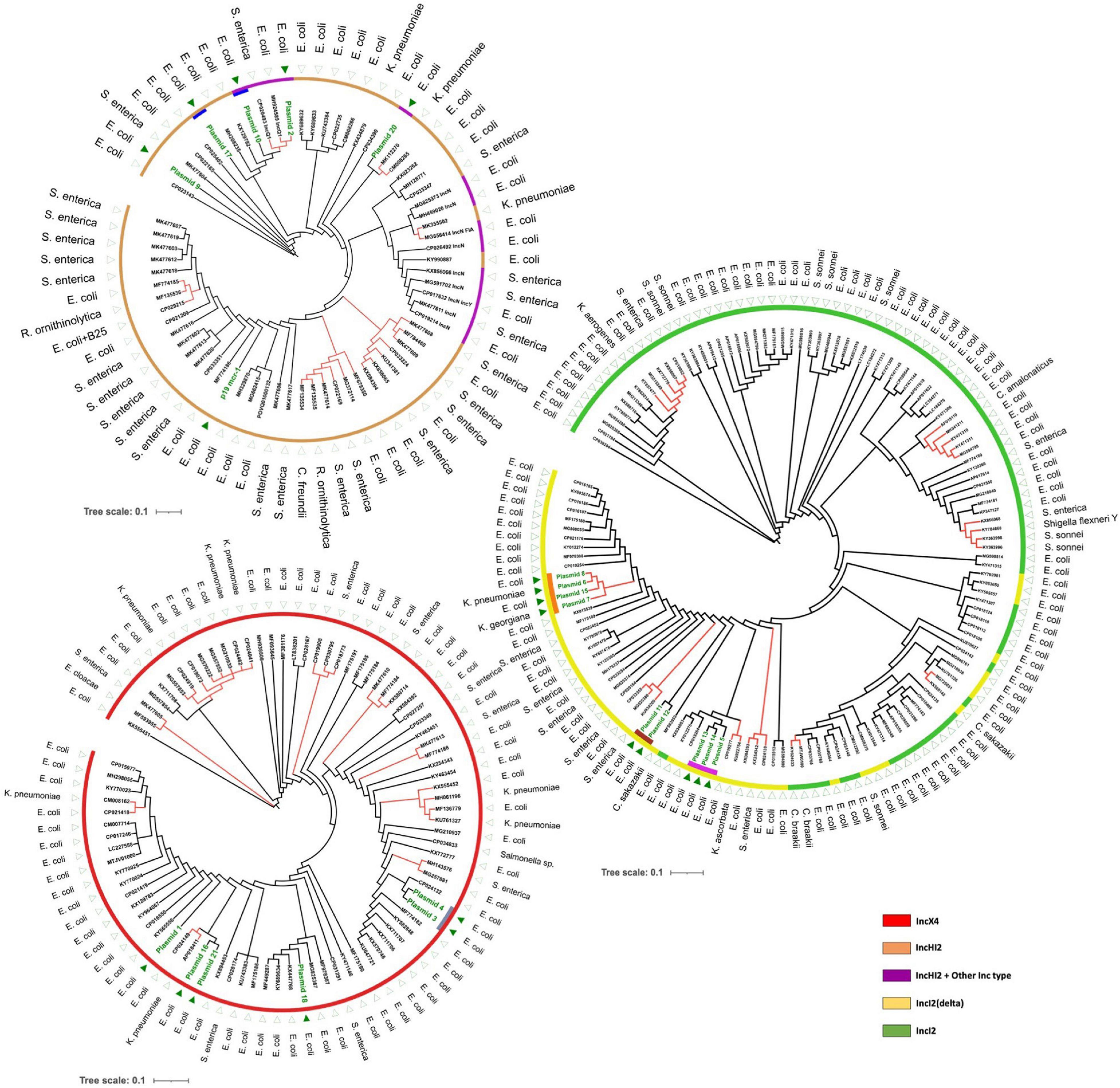
Figure 2. Maximum likelihood trees based on SNP analysis (core and accessory SNPs) of mcr-1-carrying plasmids using k-mers defined by kSNP. Separate figures are provided for plasmids of IncX4, IncI2/IncI2(delta), and IncHI2 (including IncHI2/IncQ1 and IncHI2/IncN). Dark green text and triangles are plasmids from this study isolates; colored bars indicate plasmids from the same patient, the color of the outer circles correspond to Inc types. Red branches indicate plasmid clusters involving different bacterial species.
Although our dataset was relatively small, we observed multiple occasions of plasmid transfer within and between bacterial species. First, the most striking observation involved plasmids 6, 7, and 8 that were identical mcr-1 carrying plasmids of IncI2(delta), and which were isolated from three different species including an E. coli, a K. pneumoniae, and a K. georgiana isolate cultured from the same patient over a 3-day period in 2015 – a clear indication of interspecies plasmid transmission (Table 1 and Figure 3). K. georgiana and K. pneumoniae, while a colistin MIC of >8, were isolated from a rectum sample, and E. coli, while a colistin MIC of 8, was isolated from a throat sample. All three isolates produced ESBL. Additionally, the same patient carried another genotypically different E. coli also containing an IncI2(delta) mcr-1-carrying plasmid (plasmid nr 15). This plasmid was closely related to the other plasmids isolated from this patient but lacked the DNA topoisomerase III gene that was found in the other plasmids (Figure 1). All four plasmids from this patient grouped together in a single branch well separated from other plasmids indicating the plasmids to be identical by descent (plasmids 6, 7, and 8 share 100% sequence identity while with plasmid 15 is 99.8% identical).
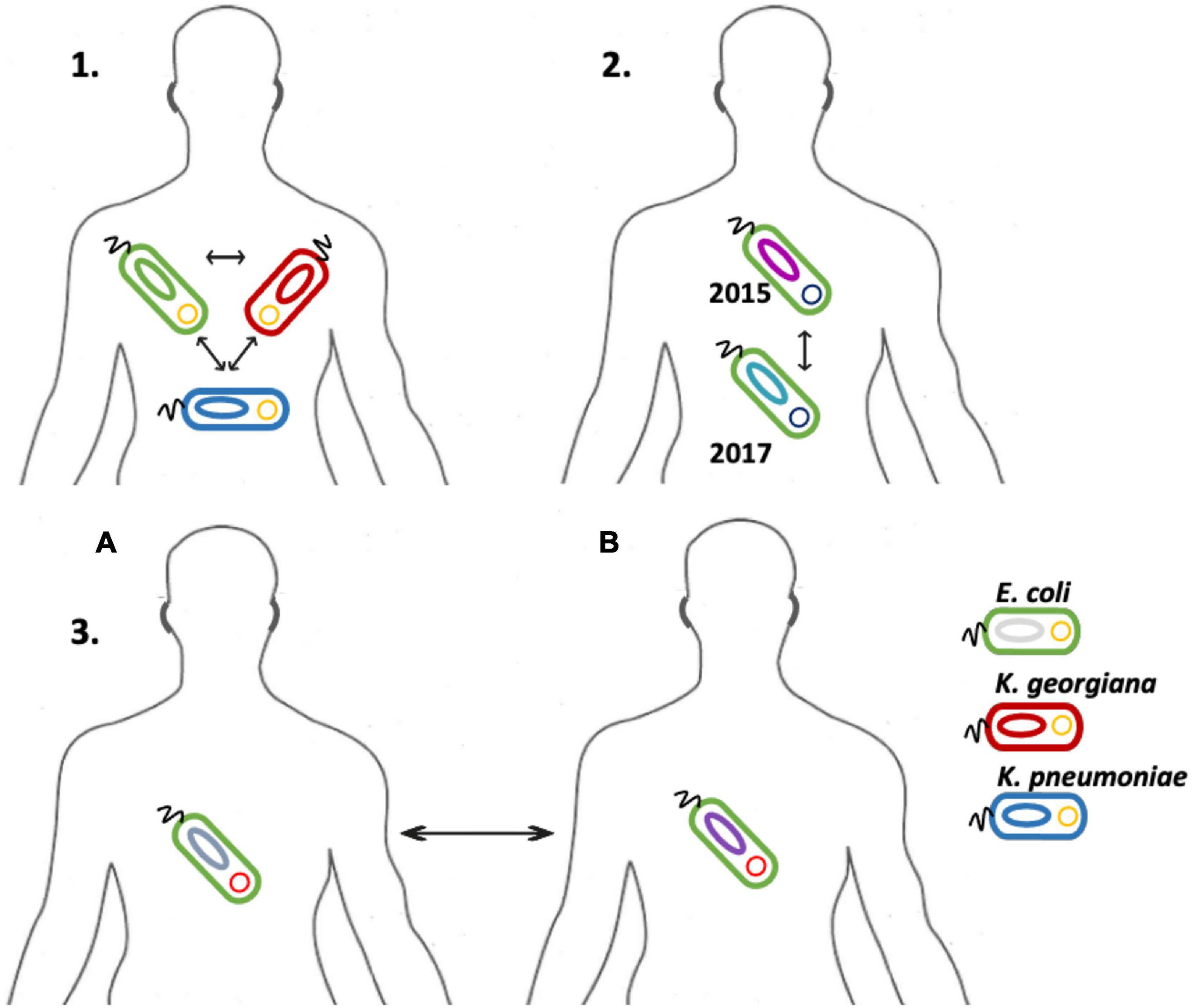
Figure 3. Illustration of the transmission events detected in this study’s dataset. The possible plasmid transfer among species is depicted in three separate cases. Case 1, an IncI2(delta) mcr-1-carrying-plasmid has been transferred among three different species of E. coli, K. pneumoniae and K. georgiana in a patient. Case 2, an IncI2(delta) mcr-1-carrying-plasmid was transferred among two different E. coli strains, one isolate detected at 2015 and one at 2017 in the same patient. Case 3, an IncX4 mcr-1-carrying-plasmid was transferred among two different patients in two different E. coli strains.
Second, we identified three matching IncI2(delta) plasmids (plasmids 5, 13, and 14) hosted by three E. coli isolates from the same patient (Figure 3). Plasmid 5 was from an isolate of 2015, plasmid 13 was from a genotypically indistinguishable isolate from 2016 and plasmid 14 from a genotypically unrelated isolate from 2016. All three plasmids clustered closely together in SNP analysis, although the two most recent ones carried a genomic region containing a site-specific integrase gene and hypothetical proteins that were absent in the 2015 isolate (Figure 1). In addition, plasmid 14 was lacking the IS3 transposase gene that was found in plasmid 13.
Interestingly, we detected double Inc type mcr-1 plasmids in E. coli isolates cultured from patients hospitalized in the years 2010, 2015, and 2017. An IncHI2/IncQ1 plasmid (plasmid 10) was identified in one of two identical E. coli isolates colonizing the same patient in two different time periods (2015 and 2016). The second E. coli isolate from this patient contained a different IncHI2 plasmid (plasmid 17), with a gene content similar to an IncHI2 plasmid (plasmid 9) originating from a different E. coli isolate cultured from a different patient in 2015 (Table 1). In contrast, all three IncHI2 plasmids clustered separate from each other and from other plasmids. Another IncHI2/IncQ1 plasmid (plasmid 2) was detected from an E. coli isolate cultured from a patient hospitalized in 2010.
In one isolate, the mcr-1 gene was not carried on a plasmid but was found in the chromosome. In the K. pneumoniae isolate of ST147, the mcr-1 gene was surrounded by a hypothetical protein encoding gene and three ISApl1 insertion elements (IS30 family transposases) (Li et al., 2017; Poirel et al., 2017) in a 6.7 kbp genomic region (Figure 1). A Blast search indicated that only the sequence surrounding this 6.7 kbp region matched the chromosome of several other K. pneumoniae isolates (Supplementary Figure 1).
All plasmid sequences were also screened for insertion sequences using ISfinder software (Supplementary Table 4). In all IncX4 plasmids a single insertion sequence was found (IS26). In four plasmids of the IncI2(delta) type no known insertion sequences were found and in four IncI2(delta) plasmids only an ISEnca1 was found. In the remaining plasmid of the IncI2(delta) type two IS elements were found. In contrast, in the larger plasmids of IncHI2 type up to 20 insertion sequences were identified with several insertion sequences occurring multiple times. This involved the previously mentioned ISApl1 and many others. Only few insertion sequences flanked the mcr-1 gene (arbitrarily set at ≤3 kbp distance), this only involved IncHI2 plasmids (or IncHI2 double Inc type plasmids) and only involved ISApl1.
Discussion
A systematic screening of clinical isolates over an 8-year period to determine the prevalence of mcr-1 resulted in a low mcr-1 prevalence, while isolates at risk of carrying mcr-1 were selected for inclusion in this study. Despite the low prevalence, interspecies and intrapatient plasmid-based mcr-1 transfer events were detected, including one event involving chromosomal integration of the gene. The mcr-1-carrying plasmids identified were mainly of Inc types IncX4, IncI2(delta), and IncHI2, although mcr-1 plasmids with double Inc types were also observed.
We identified several transmission events of mcr-1 plasmids, including a unique case of interspecies intrapatient plasmid transmission involving E. coli, K. pneumoniae and K. georgiana. K. georgiana has not been described before as carrying such plasmid. In general, Kluyvera spp. are considered mainly environmental bacteria and only infrequently cause infections in humans. However, they can be reservoirs of multiple antibiotic resistance genes and are considered progenitors of cefotaximases and the fosA3 gene (Rodriguez et al., 2018). In fact, in the genus of Kluyvera, an mcr-1-carrying plasmid was so far only reported in Kluyvera ascorbata (KU922754) (Zhao and Zong, 2016). This plasmid was different from the plasmid present in the K. georgiana and was more similar to three E. coli plasmids (13, 14, and 5) (Figure 2).
It is well established that mcr-1 plasmids may efficiently spread between different species using laboratory conditions (Denervaud Tendon et al., 2017). By plotting the host species onto the dendrograms depicted in Figure 2 it can be recognized that interspecies transfer of mcr-1 plasmids had occurred on multiple occasions in vivo as many examples are visible where (near-)identical plasmids were found in different species. These potential transfers of the same plasmid among species were not restricted to a particular Inc type but were observed in, IncX4-, IncI2-, IncI2(delta)-, and IncHI2-type plasmids and included E. coli, K. pneumoniae, Salmonella enterica, Citrobacter freundii, Cronobacter sakazakii, and Raoultella ornithinolytica.
Previous plasmid studies applied pairwise sequence comparison or average nucleotide identity (ANI) analysis (Dona et al., 2017; Dominguez et al., 2019) which is mainly applied to large sequences such as chromosomal comparisons. In this study, we compare plasmid sequences by combining a k-mer based analysis with a functional analysis. On the one hand k-mer based analysis can be applied to any sequence size and can indicate phylogenetic variations, and on the other hand functional analysis provides information regarding the genotype and phenotype (Salgado-Camargo et al., 2020). The functional comparison can result in multiple similarities among plasmids of same Inc type but k-mer based SNP analysis can indicate remarkable differences. Utilizing either one of these methods alone may restrict the amount of information obtained and actually prevent a full characterization of plasmids.
Relatively little is known about the stability of plasmids upon transfer between different strains or even between different species. Our analysis shows a remarkable heterogeneity between plasmids of identical Inc type indicating that attributing a possible transfer of plasmids by only addressing Inc types may only be presumptive (Mo et al., 2017; Han et al., 2018). A full characterization of plasmids better allows addressing these issues and also enables a more detailed study of the lateral and longitudinal stability of plasmids. The presence of highly similar plasmids found over a 3-year period in two different strains from two different patients suggests these plasmids to be stable in this time frame despite its transfer between isolates. Whether or not this is valid for all plasmids remains to be investigated. The exact transmission route was not identified in the bacterial isolates, but may have involved other patients, the innate hospital environment, or even routes in the community (including a common source), as well as animals.
Interestingly, in this study, we report one of the oldest confirmed cases of a clinical patient, hospitalized in 2010, with an E. coli isolate harboring an mcr-1-carrying IncX4 plasmid. The oldest mcr-1-carrying plasmid from a human isolate with a defined Inc type was reported in 2008 in an IncI2 plasmid carried by Shigella sonnei (Pham Thanh et al., 2016; Nang et al., 2019). Additionally, a plasmid of combined IncHI2/IncQ1 was reported in our study, in an E. coli isolate from 2010, indicating that these two Inc types merged into one plasmid more than a decade ago. These double Inc type plasmids are indicative of the complexity of mcr-1 transmission and have been recognized before in public plasmid repositories (Kudirkiene et al., 2018). The impact of double Inc types in plasmids is currently unknown. However, it would be plausible that the range of host species able to carry such plasmids may be increased. Additionally, the results of two plasmids merging could affect plasmid fitness and/or the acquisition of multiple AMR genes.
The plasmids identified in our dataset derived from strains isolated from hospitalized patients were of the IncI2(delta) type (45% of plasmids in this study) which deviates from the public data set where IncI2(delta) represents only 14% of plasmids. In contrast, the most prevalent Inc types in the public dataset involved IncI2 (28%). This difference is most likely related to the hosts carrying the isolates where IncI2 might be more prevalent in farm- and dietary animal isolates and IncI2(delta) might be more prevalent in human clinical isolates. In contrast IncX4 appears to be equally present in this study and the public collection. The IncI2(delta) mcr-1-carrying plasmids contained a genomic region with numerous genes dedicated to pili formation and conjugation (Hu et al., 2019). Such a conserved region in IncI2(delta) plasmids may be connected to a higher transmission capacity considering our observations of IncI2(delta) plasmid transmissions.
Several non-mcr-1 antibiotic resistance genes were detected within the plasmids found in our study, including genes generating resistance to fosfomycin, cephalosporin, aminoglycosides, carbapenems, and tetracycline. Interestingly, one mcr-1-carrying plasmid (plasmid nr 2) was detected containing 21 AMR genes, associated with resistance to nine different classes of antibiotics. The presence and potential spread of this 263 kbp plasmid within our academic medical center raises major concerns.
In addition to mcr-1 carrying plasmids, all bacterial isolates from our study also contained plasmids of various other Inc types, which provides a rich genetic environment for inter-plasmidal transmission of the mcr-1 gene. Furthermore, almost all Inc types from publicly available mcr-1 plasmid sequences, were detected in non-mcr-1-carrying plasmids obtained in only a very small number of characterized isolates from our study, indicating that inter-plasmidal recombination events may already have taken place on a large scale. Such events may also have formed the basis for the multi-Inc type plasmids that we observed. The multi-Inc types plasmids described in this study contained a different MOB type relaxase encoding genes from the single Inc type plasmids. The identified ISApl1 transposase gene may play an important role in this process, as it may contribute to DNA recombination events by cutting and inserting DNA regions into target bacterial chromosomes or plasmids (Wang R. et al., 2018). Previously, it was established that the insertion sequence ISApl1 was responsible for the mobilization of the mcr-1 gene (Li et al., 2017). Our results confirm presence of this mechanism in IncHI2 plasmids. However, in other Inc type plasmids this insertion sequence was not detected and only few (if any) other insertion sequence were detected. Despite the lack of an ISApl1 in IncI2 and IncX4 plasmids, the mcr-1 gene was flanked by the pap2 gene as the likely remains of Tn6330 (ISApl1-mcr-1-pap2-ISApl1) which is identified as a primary vehicle for mcr-1 mobilization (Poirel et al., 2017; Snesrud et al., 2018). In the large IncHI2 plasmids only one copy of ISApl1 was maintained. The loss of the ISApl1 copy/copies after mobilization is a common event and may have contributed to the stabilization of the mcr-1 cassette which appears to have remained intact among the plasmids of the same Inc type.
The presence of mcr-1 on the chromosome of one of the colistin-resistant K. pneumoniae (ST147) bacterial isolates adds evidence to reports that the mcr-1 gene may be found on the chromosome of several bacterial species (Falgenhauer et al., 2016; Yu et al., 2016; Singh et al., 2018). In our isolate, we identified an mcr-1 gene region surrounded by multiple ISApl1 insertion elements (IS30 family). The region was identified as ISApl1-(mcr-1)-ISApl1-ISApl1-orf-ISApl1 and was previously identified on a large IncHI2 plasmid (Wang W. et al., 2018). The international high-risk K. pneumoniae ST147 clone has been associated with the carriage of blaNDM and blaOXA–48, but, to our knowledge, no cases of mcr-1 carriage have been reported (Bahramian et al., 2019; Volozhantsev et al., 2019). The isolate from our study did not carry a major carbapenemase gene, but multiple other resistance mechanisms were found (Supplementary Table 3).
In 2016, it was stated that within the Dutch healthcare system screening for the presence of mcr-1 in colistin-resistant bacteria from hospitalized patients is not indicated (Kluytmans-van den Bergh et al., 2016). However in our study, we showed evidence suggesting multiple transmission events of mcr-1-carrying plasmids, despite the gene only being identified from a relatively small set of mcr-1 positive strains from a single medical center. Insufficient monitoring of mcr-1 transfer within plasmids, bacterial species and patients may lead to misleading prevalence estimates within healthcare settings. Furthermore, mcr-1 is a highly mobile gene, present in multiple types of plasmids with different attributes and found in many species (Elbediwi et al., 2019), which together with our findings indicates that an increased detection and monitoring of this gene is actually required, especially for high-risk clinical settings, e.g., hematology ward.
In order to facilitate epidemiological surveillance of mcr-1 and other AMR plasmids, new tools need to be developed to allow diagnostic laboratories to detect mcr-1 and AMR plasmid transmission events in hospitals and other relevant settings. A better understanding of the epidemiology of plasmid transmission events on local, national and global level is necessary for the development and implementation of measures to control the spread of the mcr-1 gene.
Data Availability Statement
The datasets presented in this study can be found in online repositories. The names of the repository/repositories and accession number(s) can be found below: https://www.ebi.ac.uk/ena, PRJEB37819.
Author Contributions
NS performed the genomics analysis and composed the manuscript. AV performed strain selection and epidemiological study and composed the manuscript. MV assisted in patient and strain selection and supervised the clinical aspect. WZ contributed in laboratory experiments. AH and JH performed nanopore sequencing and contributed in genomics analysis. JS and CK supervised the complete study, contributed in defining the epidemiology and genomics points for the study, and contributed to the supervision of the study. All authors contributed to the article and approved the submitted version.
Acknowledgments
The mcr-1 positive isolate that was used as a positive control was kindly provided by the COMBAT consortium (Arcilla et al., 2016).
Conflict of Interest
The authors declare that the research was conducted in the absence of any commercial or financial relationships that could be construed as a potential conflict of interest.
Publisher’s Note
All claims expressed in this article are solely those of the authors and do not necessarily represent those of their affiliated organizations, or those of the publisher, the editors and the reviewers. Any product that may be evaluated in this article, or claim that may be made by its manufacturer, is not guaranteed or endorsed by the publisher.
Supplementary Material
The Supplementary Material for this article can be found online at: https://www.frontiersin.org/articles/10.3389/fmicb.2021.727435/full#supplementary-material
Supplementary Figure 1 | Organization of the chromosomal region containing mcr-1 in ST147 K. pneumoniae.
Supplementary Table 1 | Overview of non-mcr-1 plasmids including size and Inc types.
Supplementary Table 2 | Table showing mcr-1 plasmids that contained additional antibiotic resistance genes and the corresponding antibiotic classes.
Supplementary Table 3 | Additional antibiotic resistance genes present in ST147 K. pneumoniae carrying mcr-1 in its chromosome.
Supplementary Table 4 | Overview of insertion sequences detected in the plasmids of this study. Numbers indicate the count of that IS element in the plasmid sequence. Of these, boldface numbers in red indicate the number flanking the mcr-1 gene (distance ≤3 kbp).
Supplementary File 1 | Inclusion of isolates and patients January 2010 until September 2018 information. The graphic representation indicates the sample collection process followed in this study.
Footnotes
References
Arcilla, M. S., van Hattem, J. M., Matamoros, S., Melles, D. C., Penders, J., de Jong, M. D., et al. (2016). Dissemination of the mcr-1 colistin resistance gene. Lancet Infect. Dis. 16, 147–149. doi: 10.1016/s1473-3099(15)00540-x
Bahramian, A., Shariati, A., Azimi, T., Sharahi, J. Y., Bostanghadiri, N., Gachkar, L., et al. (2019). First report of New Delhi metallo-beta-lactamase-6 (NDM-6) among Klebsiella pneumoniae ST147 strains isolated from dialysis patients in Iran. Infect. Genet. Evol. 69, 142–145. doi: 10.1016/j.meegid.2019.01.030
Bourrel, A. S., Poirel, L., Royer, G., Darty, M., Vuillemin, X., Kieffer, N., et al. (2019). Colistin resistance in Parisian inpatient faecal Escherichia coli as the result of two distinct evolutionary pathways. J. Antimicrob. Chemother. 74, 1521–1530. doi: 10.1093/jac/dkz090
Carattoli, A. (2013). Plasmids and the spread of resistance. Int. J. Med. Microbiol. 303, 298–304. doi: 10.1016/j.ijmm.2013.02.001
Carattoli, A., Zankari, E., Garcia-Fernandez, A., Voldby Larsen, M., Lund, O., Villa, L., et al. (2014). In silico detection and typing of plasmids using PlasmidFinder and plasmid multilocus sequence typing. Antimicrob. Agents Chemother. 58, 3895–3903. doi: 10.1128/aac.02412-14
Caselli, E., D’Accolti, M., Soffritti, I., Piffanelli, M., and Mazzacane, S. (2018). Spread of mcr-1-driven colistin resistance on hospital surfaces, Italy. Emerg. Infect. Dis. 24, 1752–1753. doi: 10.3201/eid2409.171386
Cury, J., Jove, T., Touchon, M., Neron, B., and Rocha, E. P. (2016). Identification and analysis of integrons and cassette arrays in bacterial genomes. Nucleic Acids Res. 44, 4539–4550. doi: 10.1093/nar/gkw319
Darling, A. C., Mau, B., Blattner, F. R., and Perna, N. T. (2004). Mauve: multiple alignment of conserved genomic sequence with rearrangements. Genome Res. 14, 1394–1403. doi: 10.1101/gr.2289704
Denervaud Tendon, V., Poirel, L., and Nordmann, P. (2017). Transferability of the mcr-1 colistin resistance gene. Microb. Drug Resist. 23, 813–814. doi: 10.1089/mdr.2016.0191
Dominguez, J. E., Faccone, D., Tijet, N., Gomez, S., Corso, A., Fernandez-Miyakawa, M. E., et al. (2019). Characterization of Escherichia coli carrying mcr-1-plasmids recovered from food animals from argentina. Front. Cell. Infect. Microbiol. 9:41. doi: 10.3389/fcimb.2019.00041
Dona, V., Bernasconi, O. J., Pires, J., Collaud, A., Overesch, G., Ramette, A., et al. (2017). Heterogeneous genetic location of mcr-1 in colistin-resistant Escherichia coli isolates from humans and retail chicken meat in Switzerland: emergence of mcr-1-carrying IncK2 plasmids. Antimicrob. Agents Chemother. 61, e01245–17.
Elbediwi, M., Li, Y., Paudyal, N., Pan, H., Li, X., Xie, S., et al. (2019). Global burden of colistin-resistant bacteria: mobilized colistin resistance genes study (1980-2018). Microorganisms 7:461. doi: 10.3390/microorganisms7100461
Falgenhauer, L., Waezsada, S. E., Gwozdzinski, K., Ghosh, H., Doijad, S., Bunk, B., et al. (2016). Chromosomal locations of mcr-1 and bla CTX-M-15 in Fluoroquinolone-Resistant Escherichia coli ST410. Emerg. Infect. Dis. 22, 1689–1691.
Garcillán-Barcia, M. P., Redondo-Salvo, S., Vielva, L., and de la Cruz, F. (2020). MOBscan: automated Annotation of MOB Relaxases. Methods Mol. Biol. 2075, 295–308. doi: 10.1007/978-1-4939-9877-7_21
Gardner, S. N., Slezak, T., and Hall, B. G. (2015). kSNP3.0: SNP detection and phylogenetic analysis of genomes without genome alignment or reference genome. Bioinformatics 31, 2877–2878. doi: 10.1093/bioinformatics/btv271
Gregoire, N., Aranzana-Climent, V., Magreault, S., Marchand, S., and Couet, W. (2017). Clinical Pharmacokinetics and Pharmacodynamics of Colistin. Clin Pharmacokinet. 56, 1441–1460. doi: 10.1007/s40262-017-0561-1
Han, J., Pendleton, S. J., Deck, J., Singh, R., Gilbert, J., Johnson, T. J., et al. (2018). Impact of co-carriage of IncA/C plasmids with additional plasmids on the transfer of antimicrobial resistance in Salmonella enterica isolates. Int. J. Food Microbiol. 271, 77–84. doi: 10.1016/j.ijfoodmicro.2018.01.018
Hu, B., Khara, P., and Christie, P. J. (2019). Structural bases for F plasmid conjugation and F pilus biogenesis in Escherichia coli. Proc. Natl. Acad. Sci. U. S. A. 116, 14222–14227. doi: 10.1073/pnas.1904428116
Irrgang, A., Roschanski, N., Tenhagen, B. A., Grobbel, M., Skladnikiewicz-Ziemer, T., Thomas, K., et al. Prevalence of mcr-1 in E. coli from livestock and food in Germany, 2010–2015. PLoS One. 11:e0159863. doi: 10.1371/journal.pone.0159863
Jia, B., Raphenya, A. R., Alcock, B., Waglechner, N., Guo, P., Tsang, K. K., et al. (2017). CARD 2017: expansion and model-centric curation of the comprehensive antibiotic resistance database. Nucleic Acids Res. 45, D566–D573.
Jolley, K. A., Bray, J. E., and Maiden, M. C. J. (2018). Open-access bacterial population genomics: BIGSdb software, the PubMLST.org website and their applications. Wellcome Open Res. 3:124. doi: 10.12688/wellcomeopenres.14826.1
Kluytmans-van den Bergh, M. F., Huizinga, P., Bonten, M. J., Bos, M., De Bruyne, K., Friedrich, A. W., et al. (2016). Presence of mcr-1-positive Enterobacteriaceae in retail chicken meat but not in humans in the Netherlands since 2009. Euro Surveill. 21:30149.
Kudirkiene, E., Andoh, L. A., Ahmed, S., Herrero-Fresno, A., Dalsgaard, A., Obiri-Danso, K., et al. (2018). The use of a combined bioinformatics approach to locate antibiotic resistance genes on plasmids from whole genome sequences of Salmonella enterica Serovars from humans in Ghana. Front Microbiol. 9:1010. doi: 10.3389/fmicb.2018.01010
Letunic, I., and Bork, P. (2016). Interactive tree of life (iTOL) v3: an online tool for the display and annotation of phylogenetic and other trees. Nucleic Acids Res. 44, W242–W245.
Li, R., Xie, M., Zhang, J., Yang, Z., Liu, L., Liu, X., et al. (2017). Genetic characterization of mcr-1-bearing plasmids to depict molecular mechanisms underlying dissemination of the colistin resistance determinant. J. Antimicrob. Chemother. 72, 393–401. doi: 10.1093/jac/dkw411
Liu, Y. Y., Wang, Y., Walsh, T. R., Yi, L. X., Zhang, R., Spencer, J., et al. (2016). Emergence of plasmid-mediated colistin resistance mechanism mcr-1 in animals and human beings in China: a microbiological and molecular biological study. Lancet Infect. Dis. 16, 161–168. doi: 10.1016/s1473-3099(15)00424-7
Mariani, B., Corbella, M., Merla, C., Tallarita, M., Piralla, A., Girello, A., et al. (2020). Bloodstream infections caused by Escherichia coli carrying mcr-1 gene in hospitalized patients in northern Italy from 2012 to 2018. Infection 48, 223–230. doi: 10.1007/s15010-019-01377-4
Matamoros, S., van Hattem, J. M., Arcilla, M. S., Willemse, N., Melles, D. C., Penders, J., et al. (2017). Global phylogenetic analysis of Escherichia coli and plasmids carrying the mcr-1 gene indicates bacterial diversity but plasmid restriction. Sci. Rep. 7:15364. doi: 10.1038/s41598-017-15539-7
Mendes, A. C., Novais, A., Campos, J., Rodrigues, C., Santos, C., Antunes, P., et al. (2018). mcr-1 in carbapenemase-producing Klebsiella pneumoniae with hospitalized patients, Portugal, 2016-2017. Emerg. Infect. Dis. 24, 762–766. doi: 10.3201/eid2404.171787
Mo, S. S., Sunde, M., Ilag, H. K., Langsrud, S., and Heir, E. (2017). Transfer potential of plasmids conferring extended-spectrum-cephalosporin resistance in Escherichia coli from poultry. Appl. Environ. Microbiol. 83, e00654–17. doi: 10.1128/AEM.00654-17
Nang, S. C., Li, J., and Velkov, T. (2019). The rise and spread of mcr plasmid-mediated polymyxin resistance. Crit. Rev. Microbiol. 45, 131–161. doi: 10.1080/1040841X.2018.1492902
Pham Thanh, D., Thanh Tuyen, H., Nguyen Thi, Nguyen, T., Chung The, H., Wick, R. R., et al. (2016). Inducible colistin resistance via a disrupted plasmid-borne mcr-1 gene in a 2008 Vietnamese Shigella sonnei isolate. J. Antimicrob. Chemother. 71, 2314–2317. doi: 10.1093/jac/dkw173
Poirel, L., Kieffer, N., and Nordmann, P. (2017). In vitro study of ISApl1-mediated mobilization of the colistin resistance gene mcr-1. Antimicrob. Agents Chemother. 61, e00127–17. doi: 10.1128/AAC.00127-17
Rodriguez, M. M., Ghiglione, B., Power, P., Naas, T., and Gutkind, G. (2018). Proposing Kluyvera georgiana as the origin of the plasmid-mediated resistance gene fosA4. Antimicrob. Agents Chemother. 62, e00710–18. doi: 10.1128/AAC.00710-18
Salgado-Camargo, A. D., Castro-Jaimes, S., Gutierrez-Rios, R. M., Lozano, L. F., Altamirano-Pacheco, L., Silva-Sanchez, J., et al. (2020). Structure and evolution of Acinetobacter baumannii plasmids. Front. Microbiol. 11:1283. doi: 10.3389/fmicb.2020.01283
Seemann, T. (2014). Prokka: rapid prokaryotic genome annotation. Bioinformatics 30, 2068–2069. doi: 10.1093/bioinformatics/btu153
Shen, Y., Wu, Z., Wang, Y., Zhang, R., Zhou, H. W., Wang, S., et al. (2018). Heterogeneous and Flexible Transmission of mcr-1 in Hospital-Associated Escherichia coli. mBio 9, e00943–18. doi: 10.1128/mBio.00943-18
Siguier, P., Perochon, J., Lestrade, L., Mahillon, J., and Chandler, M. (2006). ISfinder: the reference centre for bacterial insertion sequences. Nucleic Acids Res. 34, D32–D36. doi: 10.1093/nar/gkj014
Singh, S., Pathak, A., Kumar, A., Rahman, M., Singh, A., Gonzalez-Zorn, B., et al. (2018). Emergence of chromosome-borne colistin resistance gene mcr-1 in clinical isolates of Klebsiella pneumoniae from India. Antimicrob. Agents Chemother. 62, e01885–17. doi: 10.1128/AAC.01885-17
Snesrud, E., McGann, P., and Chandler, M. (2018). The birth and demise of the ISApl1-mcr-1-ISApl1 composite transposon: the vehicle for transferable colistin resistance. mBio 9, e02381–17. doi: 10.1128/mBio.02381-17
Terveer, E. M., Nijhuis, R. H. T., Crobach, M. J. T., Knetsch, C. W., Veldkamp, K. E., Gooskens, J., et al. (2017). Prevalence of colistin resistance gene (mcr-1) containing Enterobacteriaceae in feces of patients attending a tertiary care hospital and detection of a mcr-1 containing, colistin susceptible E. coli. PLoS One 12:e0178598. doi: 10.1371/journal.pone.0178598
Tse, H., and Yuen, K. Y. (2016). Dissemination of the mcr-1 colistin resistance gene. Lancet Infect. Dis. 16, 145–146. doi: 10.1016/S1473-3099(15)00532-0
van Burgh, S., Maghdid, D. M., Ganjo, A. R., Mansoor, I. Y., Kok, D. J., Fatah, M. H., et al. (2019). PME and Other ESBL-positive multiresistant Pseudomonas aeruginosa isolated from hospitalized patients in the region of Kurdistan, Iraq. Microb. Drug Resist. 25, 32–38. doi: 10.1089/mdr.2018.0036
Volozhantsev, N. V., Shpirt, A. M., Kislichkina, A. A., Shashkov, A. S., Verevkin, V. V., Fursova, N. K., et al. (2019). Structure and gene cluster of the capsular polysaccharide of multidrug resistant carbapenemase OXA-48-producing Klebsiella pneumoniae strain KPB536 of the genetic line ST147. Res. Microbiol. 171, 74–79. doi: 10.1016/j.resmic.2019.10.001
Wang, R., van Dorp, L., Shaw, L. P., Bradley, P., Wang, Q., Wang, X., et al. (2018). The global distribution and spread of the mobilized colistin resistance gene mcr-1. Nat. Commun. 9:1179. doi: 10.1038/s41467-018-03205-z
Wang, W., Baloch, Z., Zou, M., Dong, Y., Peng, Z., Hu, Y., et al. (2018). Complete genomic analysis of a Salmonella enterica Serovar Typhimurium isolate cultured from ready-to-eat pork in China carrying one large plasmid containing mcr-1. Front. Microbiol. 9:616. doi: 10.3389/fmicb.2018.00616
Wang, X., Liu, Y., Qi, X., Wang, R., Jin, L., Zhao, M., et al. (2017). Molecular epidemiology of colistin-resistant Enterobacteriaceae in inpatient and avian isolates from China: high prevalence of mcr-negative Klebsiella pneumoniae. Int. J. Antimicrob. Agents 50, 536–541. doi: 10.1016/j.ijantimicag.2017.05.009
Wang, X., Wang, Y., Wang, Y., Zhang, S., Shen, Z., and Wang, S. (2018). Emergence of the colistin resistance gene mcr-1 and its variant in several uncommon species of Enterobacteriaceae from commercial poultry farm surrounding environments. Vet. Microbiol. 219, 161–164. doi: 10.1016/j.vetmic.2018.04.002
Wick, R. R., Judd, L. M., Gorrie, C. L., and Holt, K. E. (2017). Unicycler: resolving bacterial genome assemblies from short and long sequencing reads. PLoS Comput. Biol. 13:e1005595. doi: 10.1371/journal.pcbi.1005595
Wick, R. R., Schultz, M. B., Zobel, J., and Holt, K. E. (2015). Bandage: interactive visualization of de novo genome assemblies. Bioinformatics 31, 3350–3352. doi: 10.1093/bioinformatics/btv383
Ye, H., Li, Y., Li, Z., Gao, R., Zhang, H., Wen, R., et al. (2016). Diversified mcr-1-harbouring plasmid reservoirs confer resistance to colistin in human gut microbiota. mBio. 7:e00177. doi: 10.1128/mBio.00177-16
Yu, H., Qu, F., Shan, B., Huang, B., Jia, W., Chen, C., et al. (2016). Detection of the mcr-1 colistin resistance gene in carbapenem-resistant Enterobacteriaceae from different hospitals in China. Antimicrob. Agents Chemother. 60, 5033–5035. doi: 10.1128/AAC.00440-16
Zankari, E., Hasman, H., Cosentino, S., Vestergaard, M., Rasmussen, S., Lund, O., et al. (2012). Identification of acquired antimicrobial resistance genes. J. Antimicrob. Chemother. 67, 2640–2644. doi: 10.1093/jac/dks261
Keywords: genomics, bacterial drug resistance, colistin, plasmids, transmission
Citation: Strepis N, Voor in ’t holt AF, Vos MC, Zandijk WHA, Heikema AP, Hays JP, Severin JA and Klaassen CHW (2021) Genetic Analysis of mcr-1-Carrying Plasmids From Gram-Negative Bacteria in a Dutch Tertiary Care Hospital: Evidence for Intrapatient and Interspecies Transmission Events. Front. Microbiol. 12:727435. doi: 10.3389/fmicb.2021.727435
Received: 18 June 2021; Accepted: 16 August 2021;
Published: 06 September 2021.
Edited by:
Axel Cloeckaert, Institut National de Recherche pour l’Agriculture, L’Alimentation et L’Environnement (INRAE), FranceReviewed by:
Thomas Jové, INSERM U1092 Anti-Infectieux Supports Moléculaires des Résistances et Innovations Thérapeutiques, FranceNicolas Kieffer, University of Gothenburg, Sweden
Copyright © 2021 Strepis, Voor in ’t holt, Vos, Zandijk, Heikema, Hays, Severin and Klaassen. This is an open-access article distributed under the terms of the Creative Commons Attribution License (CC BY). The use, distribution or reproduction in other forums is permitted, provided the original author(s) and the copyright owner(s) are credited and that the original publication in this journal is cited, in accordance with accepted academic practice. No use, distribution or reproduction is permitted which does not comply with these terms.
*Correspondence: Corné H. W. Klaassen, Yy5oLncua2xhYXNzZW5AZXJhc211c21jLm5s
†These authors have contributed equally to this work and share first authorship
‡These authors have contributed equally to this work and share last authorship