- 1Key Laboratory of Biology and Genetic Resources of Tropical Crops, Ministry of Agriculture, Institute of Tropical Bioscience and Biotechnology, Chinese Academy of Tropical Agricultural Sciences, Haikou, China
- 2College of Life Science, Hainan Normal University, Haikou, China
Banana is an important fruit crop. Fusarium wilt caused by Fusarium oxysporum f. sp. cubense tropical race 4 (Foc TR4) seriously threatens the global banana industry. It is difficult to control the disease spread using chemical measures. In addition, commercial resistant cultivars are also lacking. Biological control is considered as a promising strategy using antagonistic microbes. Actinomycetes, especially Streptomyces, are potential sources of producing novel bioactive secondary metabolites. Here, strain SCA2-4T with strong antifungal activity against Foc TR4 was isolated from the rhizospheric soil of Opuntia stricta in a dry hot valley. The morphological, physiological and chemotaxonomic characteristics of the strain were consistent with the genus Streptomyces. Based on the homology alignment and phylogenetic trees of 16S rRNA gene, the taxonomic status of strain SCA2-4T exhibited a paradoxical result and low bootstrap value using different algorithms in the MEGA software. It prompted us to further discriminate this strain from the closely related species by the multilocus sequence analysis (MLSA) using five house-keeping gene alleles (atpD, gyrB, recA, rpoB, and trpB). The MLSA trees calculated by three algorithms demonstrated that strain SCA2-4T formed a distinct clade with Streptomyces mobaraensis NBRC 13819T. The MLSA distance was above 0.007 of the species cut-off. Average nucleotide identity (ANI) values between strain SCA2-4T genome and two standard strain genomes were below 95-96% of the novel species threshold. Strain SCA2-4T was assigned to a novel species of the genus Streptomyces and named as Streptomyces huiliensis sp. nov. The sequenced complete genome of SCA2-4T encoded 51 putative biosynthetic gene clusters of secondary metabolites. Genome alignment revealed that ten gene clusters were involved in the biosynthesis of antimicrobial metabolites. It was supported that strain SCA2-4T showed strong antifungal activities against the pathogens of banana fungal diseases. Extracts abstracted from the culture filtrate of strain SCA2-4T seriously destroyed cell structure of Foc TR4 and inhibited mycelial growth and spore germination. These results implied that strain SCA2-4T could be a promising candidate for biological control of banana Fusarium wilt.
Introduction
Banana (Musa spp.) is one of the most popular fruits in the tropical and sub-tropical regions of the world. It is considered to be the fourth most important crop following rice, wheat and corn in the developing countries (Mohandas and Ravishankar, 2016). Banana Fusarium wilt seriously threatened the global banana industry. The disease was caused by the soil-borne fungi, containing four physiological races (abbreviated as Foc 1–Foc 4) based on their host specificity. Especially, tropical race 4 (Foc TR4) can infect almost all banana cultivars (Ploetz, 2015). They can survive in the soil as chlamydospores for more than 20 years (Ploetz, 2015). It is difficult to control the disease spread using chemical measures. In addition, commercial resistant cultivars are also lacking. Biological control is considered as a promising strategy using antagonistic microbes.
Actinobacteria are rich in bioactive secondary metabolites exhibiting antimicrobial, antitumor and/or antiviral activities (Watve et al., 2001; Guo et al., 2008). These microbes are widely distributed in diverse ecosystems such as soil, air and salt water. More than 50% of the known antibiotics come from Actinobacteria. The genus Streptomyces is the largest genus of Actinobacteria. Over 1000 species of Streptomyces are identified1. Notably, 75% of antibiotics are produced by Streptomyces species (Bérdy, 2005). Streptomyces have been used as biocontrol agents against soil-borne diseases such as Foc TR4. Streptomyces g10 isolated from a coastal mangrove exhibited a strong antagonistic activity against banana Fusarium wilt (Getha et al., 2005). Endophytic Streptomyces sp. S76 and Streptomyces sp. S96 showed antifungal activity against Foc TR4 (Cao et al., 2004, 2005). We previously reported Streptomyces sp. CB-75, SCA3-4, and JBS5-6 isolated from rhizosphere soil strongly inhibited mycelial growth of Foc TR4 and spore germination (Chen et al., 2018; Qi et al., 2019; Jing et al., 2020). However, Streptomyces species are still the most potential candidates to produce novel types of secondary metabolites (Bérdy, 2005). Based on their genome information, more than 90% of Streptomyces bioactive metabolites are waiting to be discovered (Watve et al., 2001; Guo et al., 2008). Therefore, the isolation and identification of Streptomyces remains a perennial attention.
In our present study, strain SCA2-4T was isolated from a rhizosphere soil sample of Opuntia stricta in a dry hot valley. The alignment of 16S rRNA gene and multilocus sequence analysis (MLSA) indicated that the strain had the closest taxonomic relationship with Streptomyces orinoci NBRC 13466T and Streptomyces mobaraensis NBRC 13819T. The MLSA distance and average nucleotide identity (ANI) demonstrated that the strain belonged to a novel Streptomyces species, named with Streptomyces huiliensis sp. nov. Extracts of strain SCA2-4T exhibited a strong antifungal activity against Foc TR4. Bioinformatics analysis showed that the strain genome contained a number of genes coding antifungal secondary metabolites, suggesting that it will have an application potential in biocontrol for phytopathogenic fungi.
Materials and Methods
Isolation of Actinobacteria
Soil sample was collected from rhizosphere of Opuntia stricta in a dry hot valley of the Huili County, Sichuan Province, China in 2014. One gram of soil sample was homogenized in 9 mL of sterile water and heated at 55°C for 20 min. The homogenate was diluted by a serial dilution method and spread on a starch casein agar (SCA) medium (Kuster and Williams, 1964) (10 g of soluble starch, 0.3 g of casein, 2.0 g of KNO3, 2.0 g of NaCl, 2.0 g of K2HPO4, 0.05 g of MgSO4⋅7H2O, 0.02 g of CaCO3, 0.01 g of FeSO4⋅H2O, and 18 g of agar in 1 L of sterile water, pH 7.0–7.4) supplemented with 50 mg/L of K2Cr2O7 and 50 mg/L of nystatin. The plates were incubated at 28°C for 7 days. Colonies with different morphological characteristics were selected and purified on the YE agar medium (4 g of yeast extract, 10 g of malt extract, 4 g of glucose and 20 g of agar in 1 L of distilled water, pH 7.3). The purified isolate was stocked in 20% (v/v) of glycerol at −80°C.
Antifungal Activity of Actinobacteria
Antagonistic activities of the purified actinobacteria against Foc TR4 (ATCC 76255) were screened on potato dextrose agar (PDA) plates using a conventional spot inoculation method (Qi et al., 2019). A hyphal block (5-mm diameter) of Foc TR4 was inoculated on the center of PDA plate. Four blocks (5-mm diameter) of the isolates were placed on four symmetrical points of PDA plate. Three replicates were set for each experiment. The plate inoculated only with pathogen was used as a control. Antifungal activity was detected until pathogenic mycelium covered the whole plate in control group at 28°C. The growth diameters of Foc TR4 were measured using a cross method (Qi et al., 2019). The inhibitory zone and the percentage of fungal growth inhibition were calculated using the following formula:
where C and T represented average growth diameters of tested pathogen in the control and treated plates, respectively (Aghighi et al., 2004).
Based on its strong antifungal activity against Foc TR4, strain SCA2-4T was selected as a target strain to determine antifungal activity against other fungi including F. oxysporum f. sp. cubense Race 1 (Foc 1; ACCC 31271), Curvularia fallax (ATCC 38579), and Curvularia lunata (ACCC 36365). These fungi were kindly provided by the Institute of Environment and Plant Protection, China Academy of Tropical Agricultural Sciences, Haikou, China.
Phylogenetic and Genomic Analyses of Strain SCA2-4T
Extraction of genomic DNA was performed as described by Ahmad et al. (2017). The whole genome of strain SCA2-4T was sequenced and assembled by the Shanghai Majorbio Bio-pharm Technology Co., Ltd., The complete 16S rRNA gene selected from its genome sequence was aligned with the EzTaxon server (Yoon et al., 2017a) and the NCBI database (Altschul et al., 1990). The phylogenetic trees were generated by the three algorithms including the Neighbor-Joining method, the Maximum-Likelihood method and the Maximum-Parsimony method in MEGA 7.0 (Kumar et al., 2016). The evolutionary distance was calculated by the Kimura’s two-parameter model (Kimura, 1980). The confidence level of phylogenetic tree was investigated using the bootstrap analysis on 1000 replicates (Felsenstein, 1985). In addition, five standard housekeeping genes including atpD (ATP synthase, beta subunit), gyrB (DNA gyrase B subunit), recA (recombinase A), rpoB (RNA polymerase, beta subunit), and trpB (tryptophan synthetase, beta subunit) were chosen from strain SCA2-4 T genome to perform a multilocus sequence analysis (MLSA) (Brady et al., 2008). The length of 9399 bp gene sequences including concatenated atpD, gyrB, recA, rpoB and trpB were compared with the five housekeeping genes of 16 related standard strains from GenBank (Supplementary Table 1). The MLSA phylogenetic trees were constructed using the Neighbor-Joining method (Saitou and Nei, 1987) the Maximum-Likelihood (Felsenstein, 1981) and the Maximum-Parsimony (Fitch, 1971). Average nucleotide identity (ANI) between genomes was calculated using the online OrthoANI (Yoon et al., 2017b). The G + C content of strain SCA2-4T was estimated in the light of sequenced complete genome. The genome sequences of standard strains were downloaded from the public genome database of EzBioCloud2.
Morphological Characteristics
The morphological profiles of strain SCA2-4T were observed using a scanning electron microscopy (ZEISS, Germany) after inoculation on YE agar medium at 28°C for 21 days. The sample was prepared as described by Kumar et al. (2014). The growth characteristics of strain SCA2-4T were detected on six standard ISP media, containing yeast extract-malt extract agar (ISP2 or YE), oatmeal agar (ISP3), inorganic salts-starch agar (ISP4), glycerol-asparagine agar (ISP5), peptone yeast-iron agar (ISP6), and tyrosine agar (ISP7) (Shirling and Gottlieb, 1966). The formation of melanoid pigments was observed on ISP6 and ISP7 at 28°C for 14 days. Colors of substrate and aerial mycelia as well as diffusible pigments were judged by comparing with the ISCC-NBS color charts (Kelly, 1958). The tolerance of NaCl (0–9%, at intervals of 1%, w/v) and temperature (16–46°C, at intervals of 1°C) for growth was examined on the YE plates. The effect of pH on growth was evaluated in the NB broth (1.0 g of yeast powder, 0.8 g of beef extract, 2.0 g of casein, and 10.0 g of glycerol) with pH 4–10 (at intervals of 1) at 28°C for 14 days. Physiological and biochemical characteristics were determined according to the methods of Williams et al. (1983).
Chemotaxonomic Characteristics
The biomass of strain SCA2-4T was collected by centrifugation at 8000 rpm for 20 min after growing in a shaken flask of the TSB broth (15.0 g of tryptone, 5.0 g of soytone, 30.0 g of sodium chloride, 1 L of sterile water, pH 7.1–7.5) at 25°C for 5 d. Respiration quinone was extracted from the lyophilized mycelia (0.5 g) by a reverse-phase partition high-performance liquid chromatography (HPLC) (Sasser, 1990). The cellular fatty acids were analyzed according to the standard Microbial Identification System (Sherlock Version 6.1, MIDI database) by a gas chromatography (56890N, Agilent Technologies) (Komagata and Suzuki, 1987). The polar lipids were extracted from dry mycelia and individually separated by a two-dimensional thin-layer chromatography (TLC) on silica gel plates (Art 5554, DC-Alufolien Kieselgel 60F 254, Merck, Germany) (Minnikin et al., 1979).
Bioinformatic Analysis of Strain SCA2-4T
The complete genome of strain SCA2-4T was sequenced using a paired-end sequencing method in the Illumina Hiseq × Ten platform (Illumina, San Diego, CA, United States) by the Shanghai Majorbio Bio-pharm Technology Co., Ltd., (Shanghai, China). Data were analyzed on the free online platform of the Majorbio Cloud Platform3. Clean sequencing data were optimized using the GapCloser v1.12 (Luo et al., 2012) and were subsequently assembled using the SOAPdenovo v2.04. The protein-coding genes were predicted by the Glimmer v3.02 (Delcher et al., 20074). Gene functions were annotated using six databases (NR, Swiss-Prot, Pfam, EggNOG, GO, and KEGG). The biosynthetic gene clusters of secondary metabolites were predicted using the online antiSMASH v 6.0.1 software (Kai et al., 20175).
Recovering Extracts From the Culture Filtrate of Strain SCA2-4T
In order to determine whether secondary metabolites of strain SCA2-4T can inhibit growth of Foc TR4 or not, it was first fermented in the broth medium (15 g of corn flour, 10 g of glucose, 0.5 g of K2HPO4, 0.5 g of NaCl, 0.5 g of MgSO4, 3 g of beef extract, 10 g of yeast extract, 10 g of soluble starch, 2 g of CaCO3, pH 7.2–7.4) at 150 rpm and 28°C for 7 days. The secondary metabolites in fermentation broth were extracted with ethyl acetate (v/v = 1:1). After mycelia were removed with the Whatman No.1 filter, secondary metabolites in organic solvent were collected using a separating funnel and evaporated using a rotary vacuum evaporator (EYELA, N-1300, Japan). The extracts were dissolved in 10% of dimethyl sulfoxide (DMSO) with 20 mg/mL of a final concentration.
Analysis of Minimum Inhibitory Concentration and Antifungal Activity of Extracts
Minimum inhibitory concentration (MIC) of extracts against Foc TR4 was analyzed using a 96-well plate (Nunc MicroWell, Roskilde, Denmark) (Wang et al., 2013). The stock solution was sterilized through a 0.22-μm sterile filter (Millipore, Bedford, MA, United States) and diluted to a concentration range of 100–0.781 μg/mL by a two-fold serial dilution method (Qi et al., 2019). Each well contained 80 μL of the Roswell Park Memorial Institute (RPMI) mycological media, one hundred microliters of fungal suspension at 1.0 × 10 5 CFU (colony-forming units)/mL and 20 μL of extract solution. Twenty microliters of 10% DMSO replacing extract solution was used as a negative control. The same volume of cycloheximide (50–0.391 μg/mL) or nystatin (50–0.391 μg/mL) replacing extract solution was used as a positive control. The 96-well plate was covered with a plastic membrane and cultured at 150 rpm for 24 h at 28°C. MIC value was determined as our description (Qi et al., 2019). Antifungal activity of extracts against Foc TR4 was assessed using an agar-well diffusion method (Tepe et al., 2005). 100 μL of extracts (16 × MIC) was added to each well. An equal amount of DMSO (10%, v/v) was used as a control. A 5 mm-diameter hyphal disk was placed on the plate center. The growth diameter of fungi was measured after cultured 28°C for 7 days. All experiments were repeated in triplicate. The growth inhibition percentage was calculated according to the above method.
Effect of Extracts on Cell Structure of Foc TR4
Effect of strain SCA2-4T extracts on cell structure of Foc TR4 was observed by a transmission electron microscope (TEM, HT7700, Hitachi, Japan) (Nogueira et al., 2010). Foc TR4 mycelia exposed to extracts were fixed with glutaraldehyde (2.5%, v/v) for 3 h and washed three times with PBS (0.1 mol/L, pH 7.0). Foc TR4 mycelia treated with 10% (v/v) of DMSO were used as a control. Mycelial samples were postfixed with 1% (w/v) of osmium tetroxide in PBS (0.1 mol/L, pH 7.3) for 3 h at room temperature and rinsed three times with same buffer. Thereafter, samples were dehydrated in a graded ethanol solution (70, 80, 90, 95, and 100%) for 20 min, respectively. Dehydrated specimens were then embedded in the Epon 812 resin at 37°C for 12 h, 45°C for 12 h, and 60°C for 24 h, respectively. The samples were cut into ultrathin sections (approximately 50 nm in thickness) using an ultramicrotome (EM UC6, Leica, Germany). After stained with saturated uranyl acetate and lead citrate, the sections were observed using TEM.
Effect of Extracts on Spore Germination of Foc TR4
Effect of strain SCA2-4T extracts on spore germination of Foc TR4 was examined by an optical microscope (Axio Scope A1, Carl ZEISS, Germany) (Chen et al., 2018). Foc TR4 spores were collected using a sterile L-shaped spreader and adjusted to a concentration of 1.0 × 105 CFU/mL with sterile distilled water. Ten microliters of extracts and an equal volume of spore suspension were mixed in a cavity glass slide at 28°C for 20 h. Three replications were set for each experiment. One hundred spores in per slide were observed for germination. The germination rate and the inhibition rate of spore germination were calculated according to the following formula: The germination rate = (A or B)/100 × 100% and the inhibition rate of spore germination = (A−B)/A × 100%. A and B were the number of germinated spores in the control and treatment groups, respectively.
Results and Discussion
Isolation and Antifungal Activity Assay of Actinobacteria
Forty-eight actinobacteria were isolated using the SCA medium from soil sample of the dry hot valley. Antagonistic activities against Foc TR4 were screened using a conventional spot inoculation method. Fourteen strains exhibited antifungal activities against Foc TR4. Strain SCA2-4T had a high antifungal activity and was selected for the following study. We further assessed its antifungal activities against other phytopathogenic fungi such as Foc 1, C. fallax and C. lunata (Figure 1). Compared with the growth diameter (85.00 mm ± 0.00) in the control group, the inhibitory zones of strain SCA2-4T against Foc TR4, Foc 1, C. fallax, and C. lunata were 53.17 mm ± 2.84, 37.83 mm ± 0.29, 58.83 mm ± 2.02, and 52.83 mm ± 1.26, respectively. The percentages of mycelial inhibition were 62.55, 44.51, 69.21, and 62.15%, respectively.
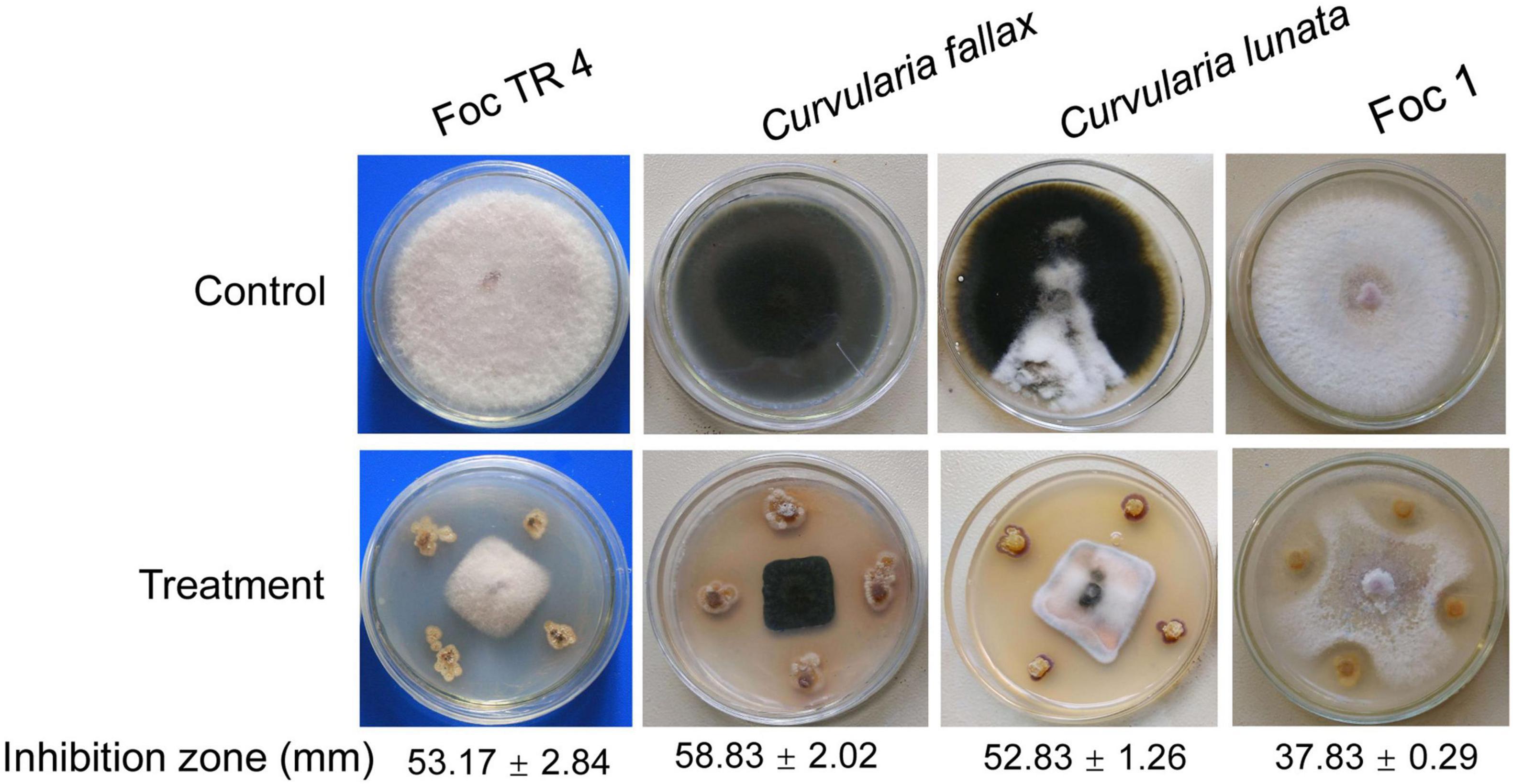
Figure 1. Antifungal activity of strain SCA2-4T against four banana fungal pathogens. Foc TR4: Fusarium oxysporum f. sp. cubense tropical race 4; Foc 1: Fusarium oxysporum f. sp. cubense 1.
Phylogenetic and Genomic Analyses of Strain SCA2-4T
Because 16S rRNA had a high resolving ability for relatedness between different organisms on the genus level (Stackebrandt and Goebel, 1994), these sequences were first used to identify the genus of strain SCA2-4T. A complete gene sequence of 16S rRNA (1523 bp, Genbank accession number MW547058) was selected from the sequenced genome of strain SCA2-4T. By aligned with the different databases, the strain belonged to a member of the genus Streptomyces. The 16S rRNA sequence of strain SCA2-4T shared 99.17% of similarity with Streptomyces mobaraensis NBRC 13819T. However, the phylogenetic trees constructed by the Neighbor-joining method showed that strain SCA2-4T fell into a subclade with S. orinoci NBRC 13466T (98.89% of similarity) with 54% of bootstrap value (Figure 2A). The taxonomic status of subclade was supported by the maximum-parsimony tree with 25% of low bootstrap value, but was not supported by the maximum-likelihood tree. The low bootstrap values generated by different algorithms indicated that strain SCA2-4T was not distinguished from closely related species using 16S rRNA genes. Likewise, the previous study also showed that 16S rRNA genes lacked sufficient resolution for closely related species due to its multiple copies and conservative feature in the single genome (Martens et al., 2008).
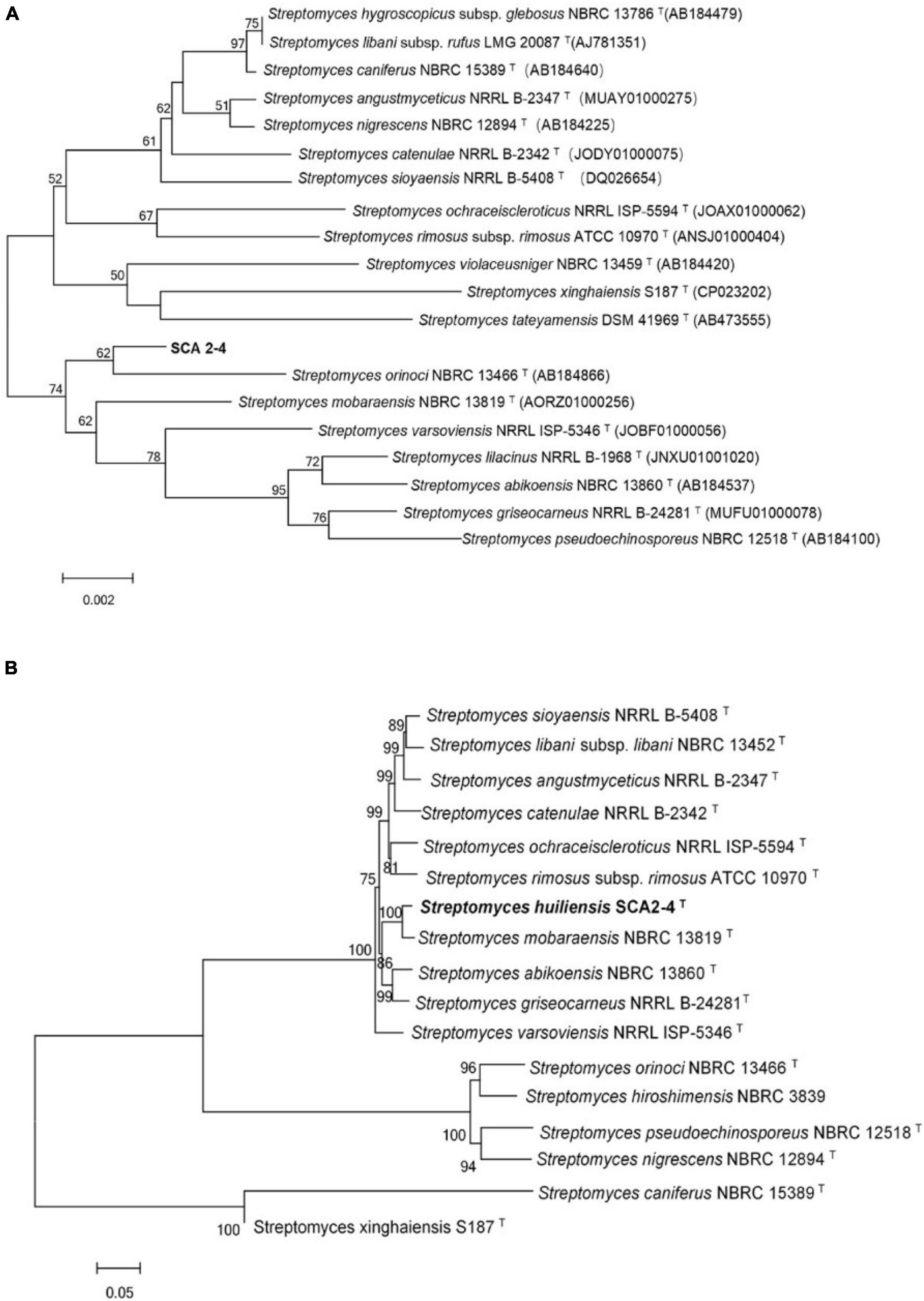
Figure 2. Phylogenetic trees of strain SCA2-4T based on 16S rRNA and house-keeping genes. Bootstrap percentages (based on 1000 replications) were shown at branching points. Bar, 0.002 or 0.05 substitutions per nucleotide position. (A) Construction of phylogenetic tree based on the complete 16S rRNA sequences using the Neighbor-Joining method. Accession numbers of the selected genes were listed in brackets. (B) Construction of phylogenetic tree based on concatenated five house-keeping genes (atpD, gyrB, recA, rpoB, and trpB) using the Neighbor-Joining method. Strain names and accession numbers were provided in Supplementary Table 1.
In order to further determine the phylogenetic status of strain SCA2-4T, the multilocus sequence analysis (MLSA) was performed using the sequences of atpD, gyrB, recA, rpoB, and trpB genes. The MLSA was considered as an alternative method for refining the Streptomyces systematics due to its efficiency of inter- and intra-species resolution and reproducibility (Rong and Huang, 2010). The Neighbor-joining tree based on MLSA demonstrated that strain SCA2-4T formed a distinct clade with S. mobaraensis NBRC 13819T with 100% of bootstrap value (Figure 2B). The taxonomic status of subclade was well supported by trees constructed by other two algorithms. These five protein-coding genes were also selected to identify Streptomyces (Rong et al., 2010; Huang et al., 2019). Rong and Huang (2012) found high correlation between the five-gene MLSA and the DNA-DNA hybridization in Streptomyces. The five-gene nucleotide sequence distance above 0.007 could be considered as an independent species. Our results showed the distance of MLSA between strain SCA2-4T and other selected strains ranged from 0.024 to1.020 (Supplementary Table 2), suggesting that strain SCA2-4T might be a novel species.
Although the conventional DNA-DNA hybridization was often used to clarify the species of strains, the technique was time-consuming, labor-intensive and low reproducible (Stackebrandt and Goebel, 1994; Goris et al., 2007). Average nucleotide identity (ANI) was a robust and sensitive means to compare genetic relatedness among strains by the alignment of genome sequences (Konstantinidis and Tiedje, 2005; Goris et al., 2007). However, the limited availability of strain whole-genome sequences restricted the use of ANI in taxonomic purposes. Based on the above results, we selected two standard strains (S. orinoci NBRC 13466T, = NRRL B-3379T, GenBank Accession No. NZ_PHNC00000000.1 and S. mobaraensis NBRC 13819T, = DSM 40847, GenBank Accession No. NZ_VOKX00000000.1) to further identify the species of strain SCA2-4T. We compared with strain SCA2-4T genome (DDBJ/ENA/GenBank Accession No. JAIQLH000000000) and two standard strain genomes. ANI values were 80.44 and 90.76%, respectively, which were below the threshold value of 95–96% for distinguishing a novel species (Richter and Rossello-Mora, 2009; Chun et al., 2018). Thus, strain SCA2-4T was identified as a novel species of the genus Streptomyces.
Phenotypic Characteristics
Strain SCA2-4T was an aerobic and Gram-positive bacterium. SEM analysis revealed that the strain produced well-developed and branched substrate and aerial mycelium that could not differentiate into spore chains (Figure 3A). It could grow well on ISP2, ISP3 and ISP6 media, followed by ISP4, ISP5 and ISP7 media. Aerial hypha demonstrated gray white, reddish brown, and earthy yellow colors on ISP2 and ISP4 media, respectively. None of aerial mycelia was produced on other two media. The hyphal substrate colors on ISP2-7 media were dark brown, light yellow, light yellow, gold, iron gray, and dark brown, respectively. Strain SCA2-4T produced brown or light brown soluble pigment on different media except for ISP3-5 (Figure 3B). These morphological and cultural characteristics were consistent with those of the genus Streptomyces. By contrast, two standard strains selected grew well on all ISP media. Their cultural characteristics were compared in Table 1.
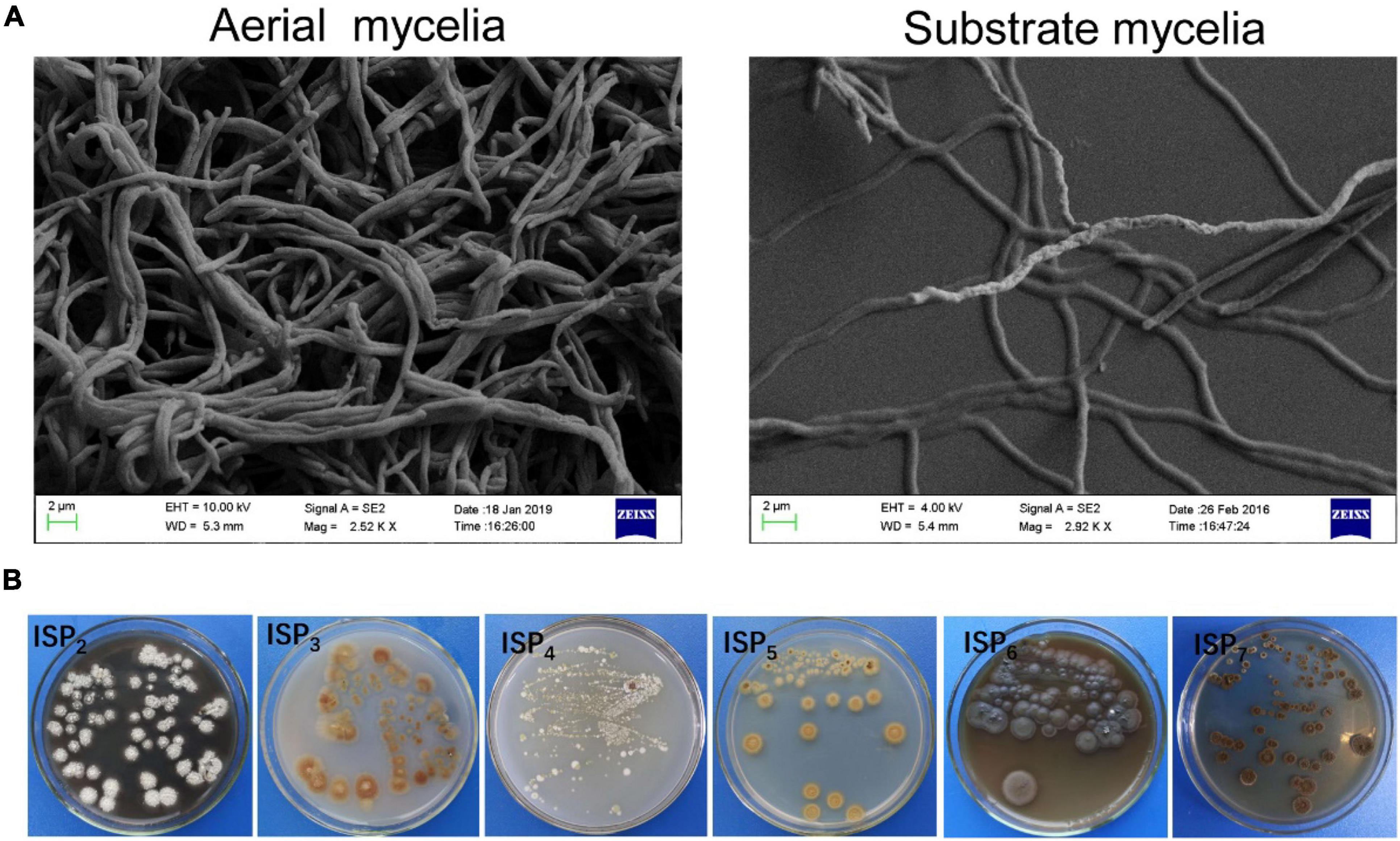
Figure 3. Mycelial and morphological characteristics of SCA2-4T. (A) Aerial and substrate mycelia were detected on the YE agar medium at 28°C for 21 days using the scanning electron microscope. (B) Cultural characteristics of strain 2-4T on six ISP media.
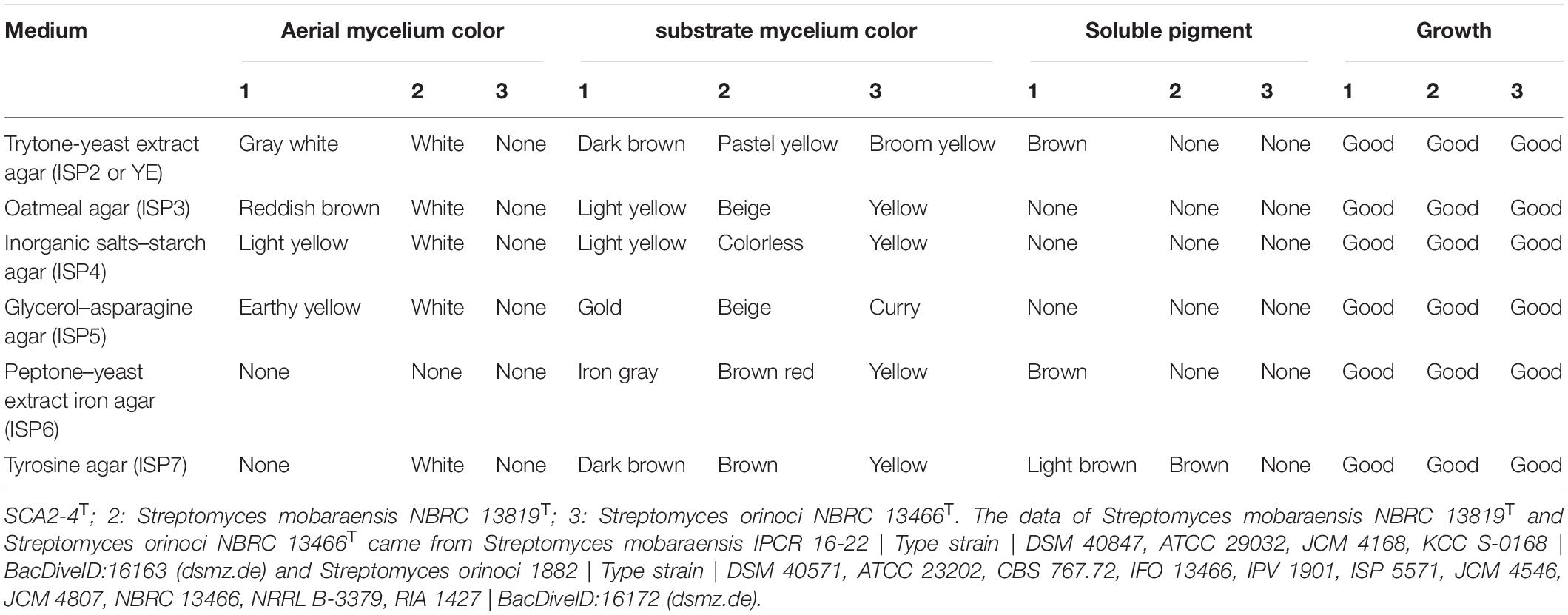
Table 1. Comparison of growth characteristics between strain SCA2-4T and closely related strains on six standard ISP media.
In addition, strain SCA2-4T could grow at pH from 5.0 to 8.0 (optimum pH 7.0), temperature from 17–45°C (optimum 28°C) and NaCl from 0 to 6% (w/v). It could degrade Tween 80, tyrosine, gelatin and nitrate, but not resolve urea, Tween 20, starch and cellulose. Strain SCA2-4T produced melanoid pigment, but not H2S. It could utilize carbon sources such as L-Arabinose, D-fructose, D-glucose, inositol, D-raffinose, L-rhamnose, and sucrose, but not Cellulose, D-mannitol and D-xylose (Table 2). In addition, strain SCA2-4T used L-phenylalanine, ammonium sulfate, L-hydroxyproline, L (+)-cysteine, histidine, glycine, valine, and ammonium oxalate as sole nitrogen sources, but not ammonium acetate, ammonium nitrate, ammonium molybdate tetrahydrate, L-arginine and glutamate. The strain was sensitive to rifampicin, but resistant to ampicillin, chloramphenicol, streptomycin, penicillin-G, gentamicin, nystatin, tetracycline, neomycin sulfate and kanamycin sulfate (Supplementary Table 3).
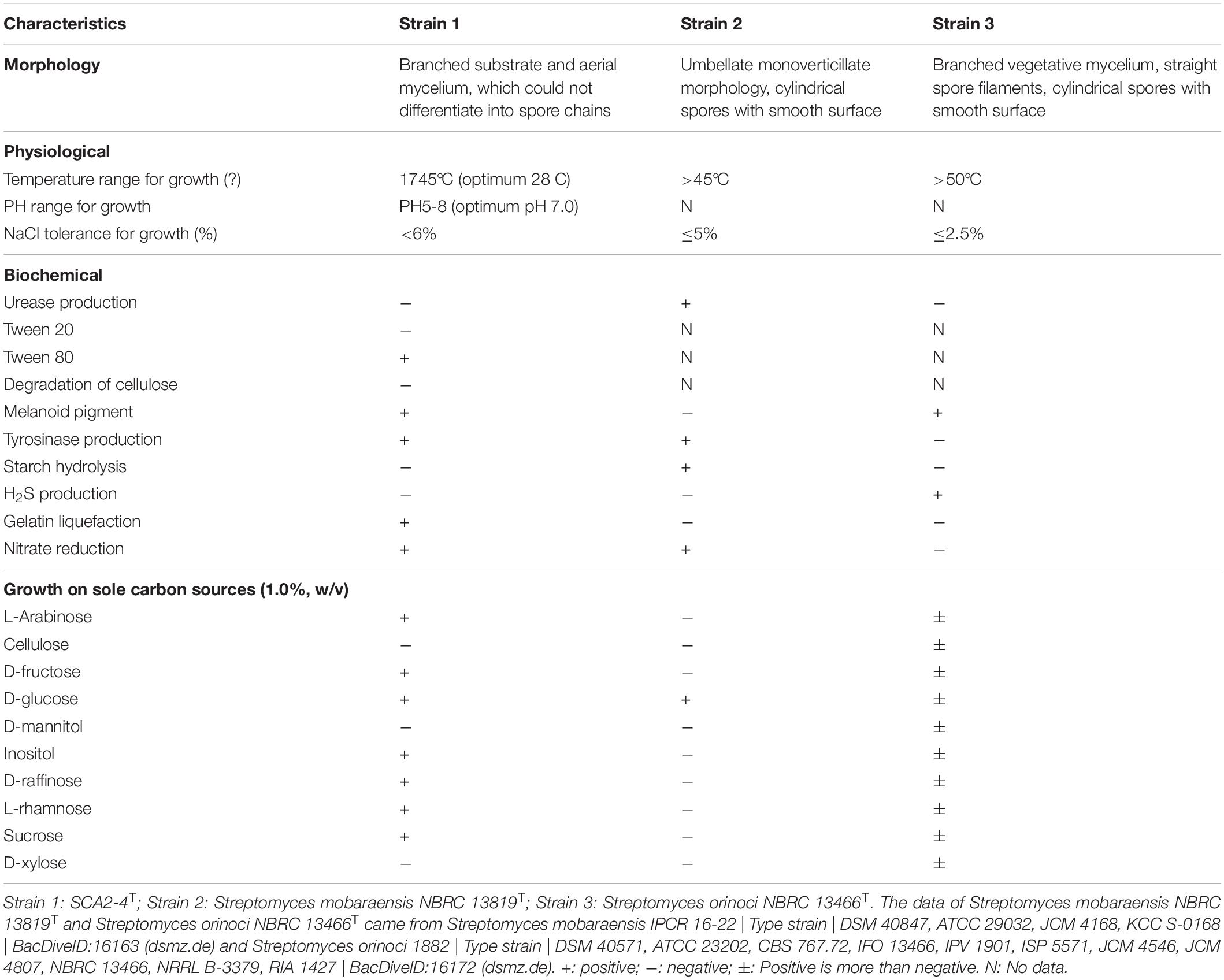
Table 2. Morphological, physiological and biochemical characteristics of strain SCA2-4T and two standard strains.
Chemotaxonomic Characteristics
By analysis, the predominant menaquinones of strain SCA2-4T were MK-9(H4) (40.0%) and MK-9(H6) (44.0%). MK-9(H2) (7.0%), and MK-9(H4) (9.0%) were minor components. The composition of fatty acids included Antesio-C15:0 (35.01%), Iso-C15:0 (28.01%) and C16:1ω7c (11.03%) (Table 3). The major polar lipids consisted of diphosphatidylglycerol, unidentified phospholipid, and phosphatidylethanolamine. We also identified phosphatidylglycerol, two unidentified lipids, phosphatidylinositol and phosphatidylinositol mannoside (Figure 4). The chemotaxonomic characteristics of strain SCA2-4T were consistent with those of the genus Streptomyces.
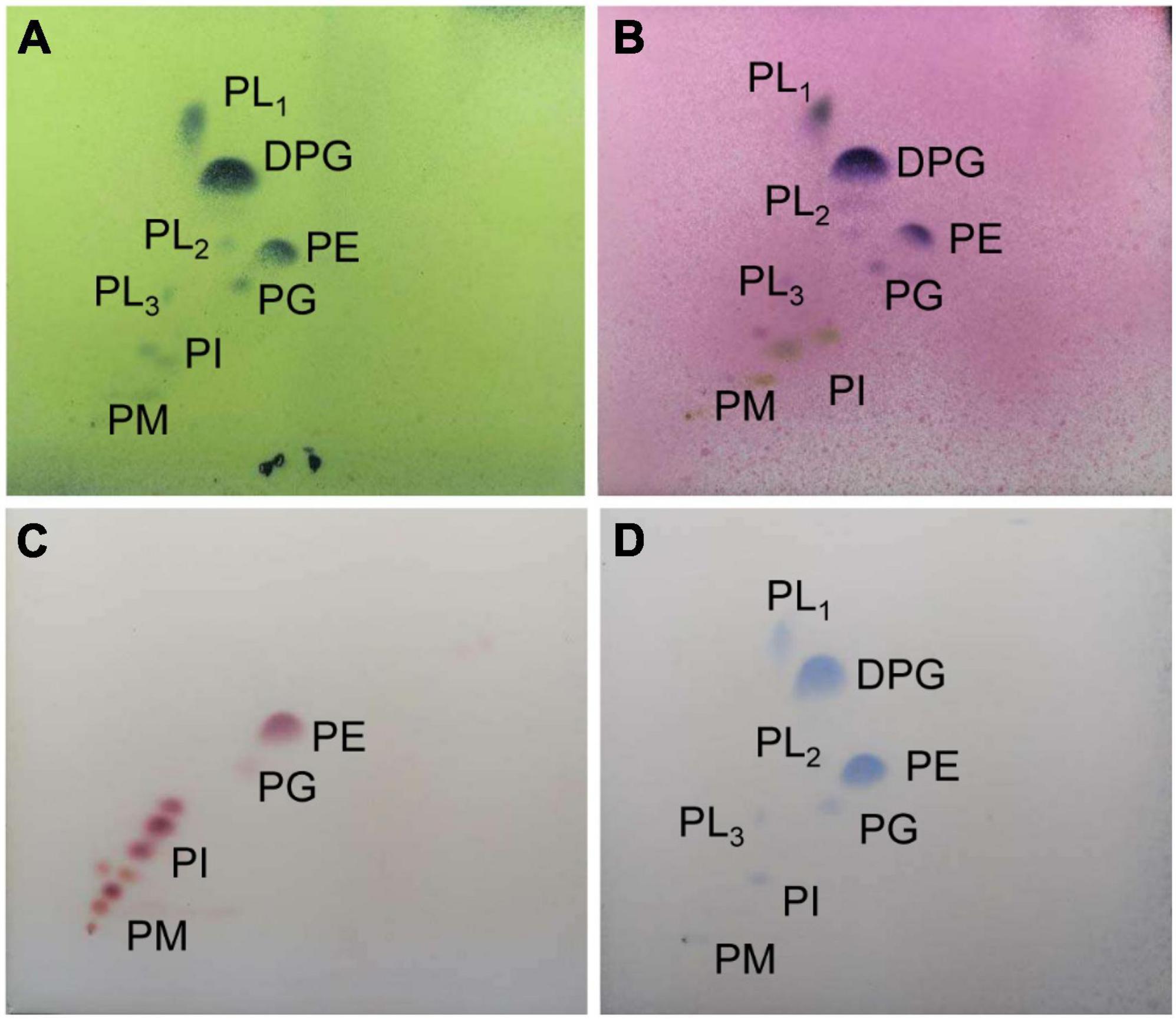
Figure 4. The phospholipid profile of strain SCA2-4 T sprayed with phosphomolybdic acid (A), a-naphthol (B), ninhydrin (C), and phosphate (D). The horizontal and vertical directions were developed using the chloroform-methanol-water (65:25:4) (v/v) and the chloroform-methanol-acetic acid-water (80:12:15:4) (v/v), respectively. DPG, diphosphatidylglycerol; PE, phosphatidylethanolamine; PL1-3, unidentified phospholipids; PG, phosphatidylglycerol; PI, Phosphatidylinositol; PIM, phosphatidylinositolmannoside.
Bioinformatic Analysis
Strain SCA2-4T genome was sequenced and contained 7681513 bp. The genome with 73.19% of GC content included one rRNA gene, sixty-nine tRNA genes and 7137 coding sequences (CDS) (Figure 5A and Table 4). By annotation, 73.41, 34.22, and 60.95% of CDS were assigned to COG, KEGG, and GO, respectively (Table 4). In the COG categories, 34.26, 17.58, and 14.28% of genes participated in metabolism, information storage and processing as well as cellular processes and signaling, respectively. Significantly, 33.88% of COG genes were unknown function (Figure 5B and Supplementary Table 4). These coding sequences were responsible for different function profiles in the KEGG pathways (Figure 5C). By analysis of antiSMASH software, fifty-one gene clusters related to the secondary metabolite biosynthesis were identified in genome sequences of strain SCA2-4T (Supplementary Table 5). They included nine PKS I gene clusters, eight terpene gene clusters, five NRPS gene clusters, four PKS III gene clusters, three other gene clusters, two lasso peptide gene clusters, two PKS II gene clusters, two siderophore gene clusters, two otherks gene clusters, two lantipeptide gene clusters, one PKS III-siderophore gene cluster, one fused gene cluster, one arylpolyene gene cluster, one lantipeptide-lasso peptide gene cluster, one lantipeptide-NRPS gene cluster, one ladderane gene cluster, one indole gene cluster, one PKS III-terpene gene cluster, one terpene-NRPS gene cluster, one bacteriocin gene cluster, one PKS I-NRPS gene cluster and one thiopeptide gene cluster (Supplementary Table 6). The gene and chemical structures of sixteen secondary metabolites with more than 70% of sequence similarity were listed in Supplementary Figure 1.
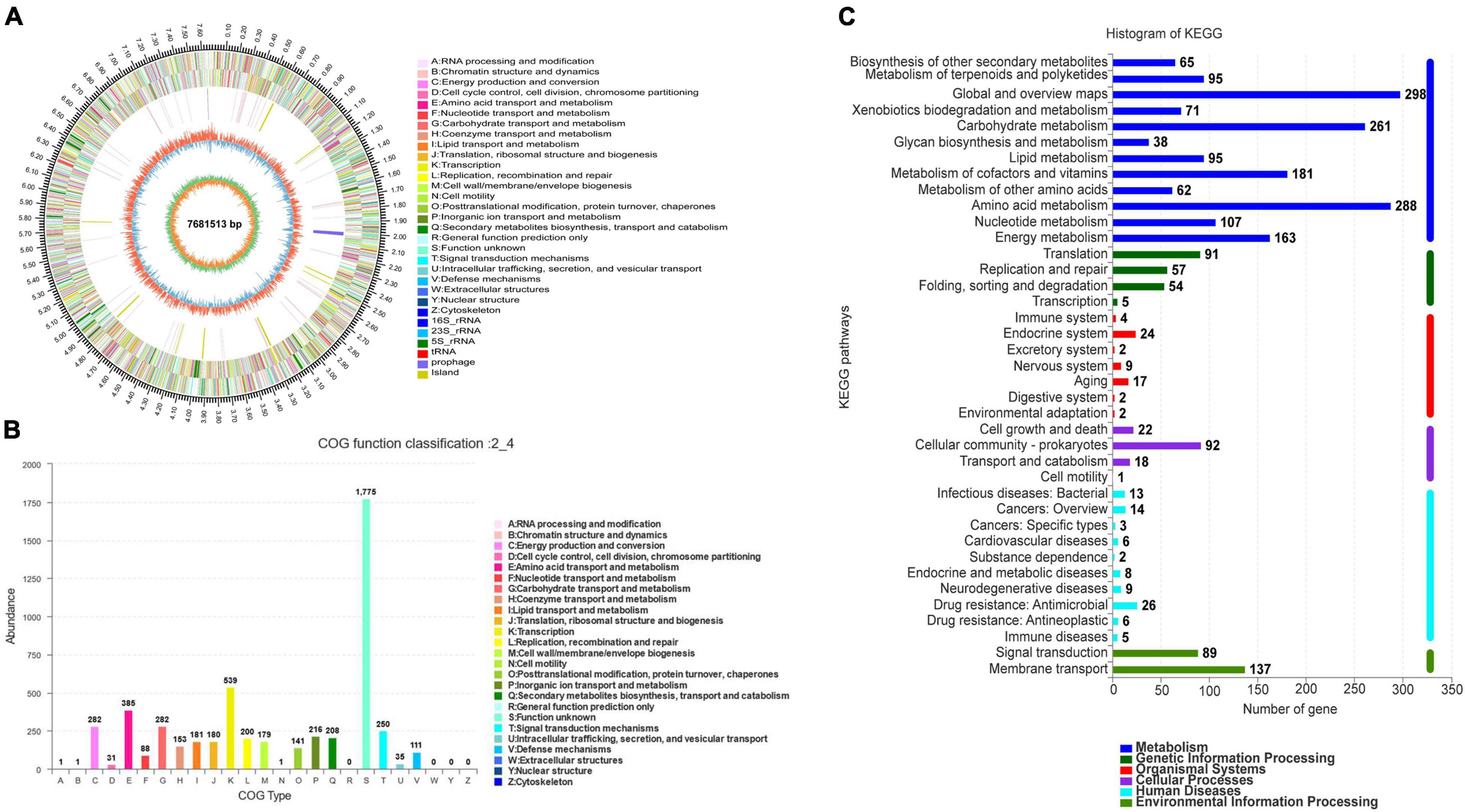
Figure 5. Genome information and function annotation of strain SCA2-4T. (A) Circular map of strain SCA2-4T genome. From outside to center, ring 1 was the mark of genome size. Rings 2 and 3 represented CDS on forward/reverse strand. Different colors indicated the functional category of CDS. Ring 4 was tRNA and rRNA. Ring 5 showed the G + C content. The outward red part indicated that the GC content of this region was higher than the average GC content of the whole genome. The inward blue part indicated that the GC content of this region was lower than the average GC content of the whole genome, followed by G + C skew in ring 6. (B) The COG annotation of strain SCA2-4T genome. (C) The KEGG pathway annotation of strain SCA2-4T genome.
Genome analysis further revealed that ten gene clusters were involved in the biosynthesis of antimicrobial metabolites, including naringenin (Salehi et al., 2019), piericidin A1 (Engl et al., 2018), simocyclinone (Schimana et al., 2000), granaticin (Snipes et al., 1979), medermycin (Takano et al., 1976), cyclothiazomycin b1 (Mizuhara et al., 2011), cyclothiazomycin C (Cox et al., 2014), caboxamycin (Hohmann et al., 2009), rhizomide A-C (Wang et al., 2018), and alkylresorcinol (Dey et al., 2013). Among them, gene clusters encoding naringenin, simocyclinone, cyclothiazomycin C and alkylresorcinol showed 100% of similarity with the known genes. Thirteen gene clusters might participate in the biosynthesis of anticancer agents including naringenin, aranciamycin, AT2433 (Singh et al., 2015), rebeccamycin (Bush et al., 1987), staurosporine (Nakano and Omura, 2009), K-252a (Nakano and Omura, 2009), cladoniamide (Loosley et al., 2013), oviedomycin (Lombó et al., 2004), landomycin (Henkel et al., 1990), simocyclinone, granaticin, medermycin, and caboxamycin.
Notably, one gene cluster was responsible for in the biosynthesis of siderophore bacillibactin. Siderophores had a competition ability for ferric iron (Fe3+) by the receptors of the siderophore-producing strains (Sheng et al., 2020). They played an important role in enhancing plant resistance and reducing pathogen infection (Gu et al., 2020). Sheng et al. (2020) reported that Brevibacillus brevis GZDF3 had strong antifungal activity against Candida albicans by producing siderophores. The previous study showed that Streptomyces S96 lacked antagonistic activity against Foc TR4 by adding an excess ferric iron (Cao et al., 2005). The role of siderophore bacillibactin in antifungal activity of strain SCA2-4T against plant pathogenic fungi including Foc TR4 needs further study. In addition, several unmatched gene clusters were also found in the genome of strain SCA2-4T, which might participate in the biosynthesis of some key secondary metabolites.
Description of Streptomyces huiliensis sp. nov.
Streptomyces huiliensis (hui.li.en’sis. N.L. masc. adj. huiliensis of Huili, a county in China, referring to the place where the type strain was first isolated). The strain was Gram-positive, aerobic and non-motile. It formed branched substrate and aerial mycelia and could grow good on all of ISP agars. Aerial mycelia with gray white, reddish brown, light yellow and earthy yellow were formed on ISP2-4, but not aerial mycelia were detected on ISP6 and ISP7. Substrate mycelia were dark brown on ISP2, light yellow on ISP3-4, gold on ISP5, iron gray and dark brown on ISP6 and ISP7. Brown soluble pigments were produced on ISP2, but not on ISP3-4. Melanin was produced on ISP6 or ISP. The strain could grow at 17–45°C (optimum 28°C), pH 5–8 (optimum pH 7) and 0–6% (w/v) NaCl. It could degrade Tween 80, tyrosine, gelatin and nitrate, but not urea, Tween 20, starch and cellulose. The strain did not produce H2S. It utilized L-Arabinose, D-fructose, D-glucose, inositol, D-raffinose, L-rhamnose and sucrose as sole carbon sources, but not Cellulose, D-mannitol and D-xylose. It could also use L-phenylalanine, ammonium sulfate, L-hydroxyproline, L (+)-cysteine, histidine, glycine, valine and ammonium oxalate as sole nitrogen sources, but not ammonium acetate, ammonium nitrate, ammonium molybdate tetrahydrate, L-arginine and glutamate. strain SCA2-4T was resistant to ampicillin, chloramphenicol, streptomycin, Penicillin-G, gentamicin, nystatin, tetracycline, neomycin sulfate and kanamycin sulfate, but sensitive to rifampicin.
MK-9(H4) (40.0%) and MK-9(H6) (44.0%) were found as the major menaquinones of the strain. The major fatty acids were composed of Antesio-C15:0 (35.01%), Iso-C15:0 (28.01%) and C16:1ω7c (11.03%). The major polar lipids consisted of diphosphatidylglycerol, one unidentified phospholipid, and phosphatidylethanolamine.
Strain SCA2-4T (= GDMCC 4.215T) was isolated from the soil sample collected from rhizosphere of O. stricta in a dry hot valley of the Huili County, Sichuan Province, China. The 16S rRNA gene sequence was submitted into GenBank with accession number MW547058. This Whole Genome Shotgun project of the strain has been deposited at DDBJ/ENA/GenBank under the accession JAIQLH000000000. The version described in this paper is version JAIQLH010000000. The genome was 7681513 bp with 73.19% of GC content.
Antifungal Activity and MIC of Extracts Against Foc TR4
In order to determine whether the secondary metabolites of strain SCA2-4T had antifungal activity or not, extracts were isolated with ethyl acetate. Minimum inhibitory concentration (MIC) of extracts ranged from 100 to 0.781 μg/mL against Foc TR4. Two standard antibiotics cycloheximide and nystatin used as positive controls. The results displayed that MIC of extracts was 6.25 μg/mL and MIC values of two standard antibiotics cycloheximide and nystatin were 1.563 and 6.25 μg/mL, respectively. The results indicated that secondary metabolites of strain 2-4T had a strong inhibitory activity on Foc TR4 (Figure 6). Inhibition zone and mycelial inhibition rate reached 31.83 mm ± 2.36 and 42.47% (Supplementary Table 7), respectively.
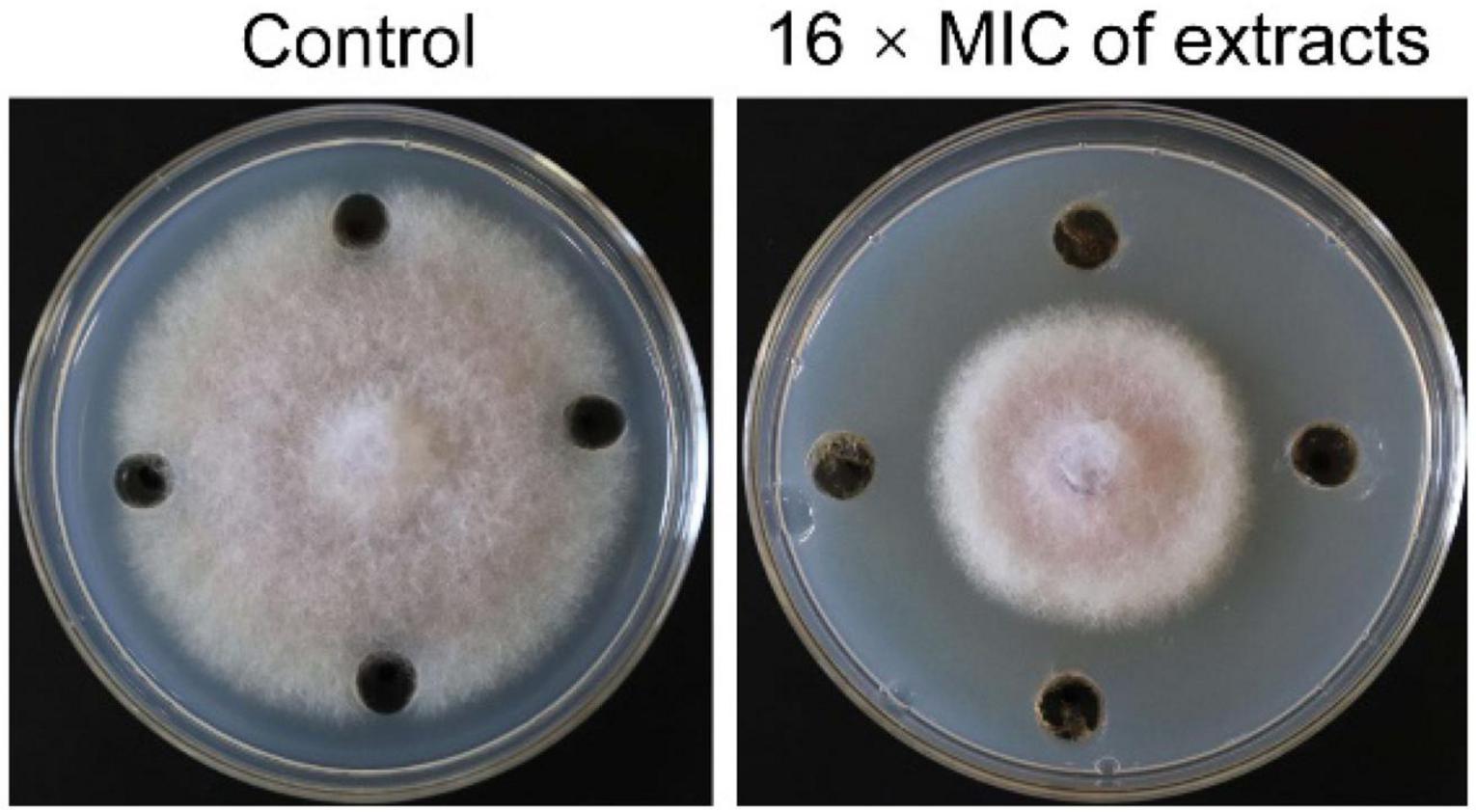
Figure 6. Inhibition assay of strain SCA2-4T extracts on the mycelial growth of Foc TR4. 10% of DMSO treatment was used as control.
Effect of Extracts on Spore Germination and Cell Structure of Foc TR4
To further analyze antifungal mechanism of strain SCA2-4T extracts, inhibition activity of extracts on spore germination of Foc TR4 was investigated (Figure 7A). The results showed that spore germination of Foc TR4 was obviously inhibited by extracts of strain SCA2-4T. Compared with 94.33 ± 2.08% of spore germination rate in the control, the inhibition rate was 89.08% in the treatment group. In addition, cell ultrastructure of Foc TR4 was detected using TEM after treatment. By contrast, cells of Foc TR4 mycelia treated with 10% of DMSO kept an intact and discernible structure of cell membrane with the uniform cytochylema. All organelles such as mitochondria, nuclei and vacuoles had normal morphological characteristics (Figure 7B). However, fungal cell wall and cytoplasmic membrane treated with 16 × MIC of extracts became irregular and thickened. Many organelles such as nuclei and vacuoles were broken. Mitochondrial structures were disrupted, suggesting that extracts caused serious damage to cell structure of strain SCA2-4T extracts.
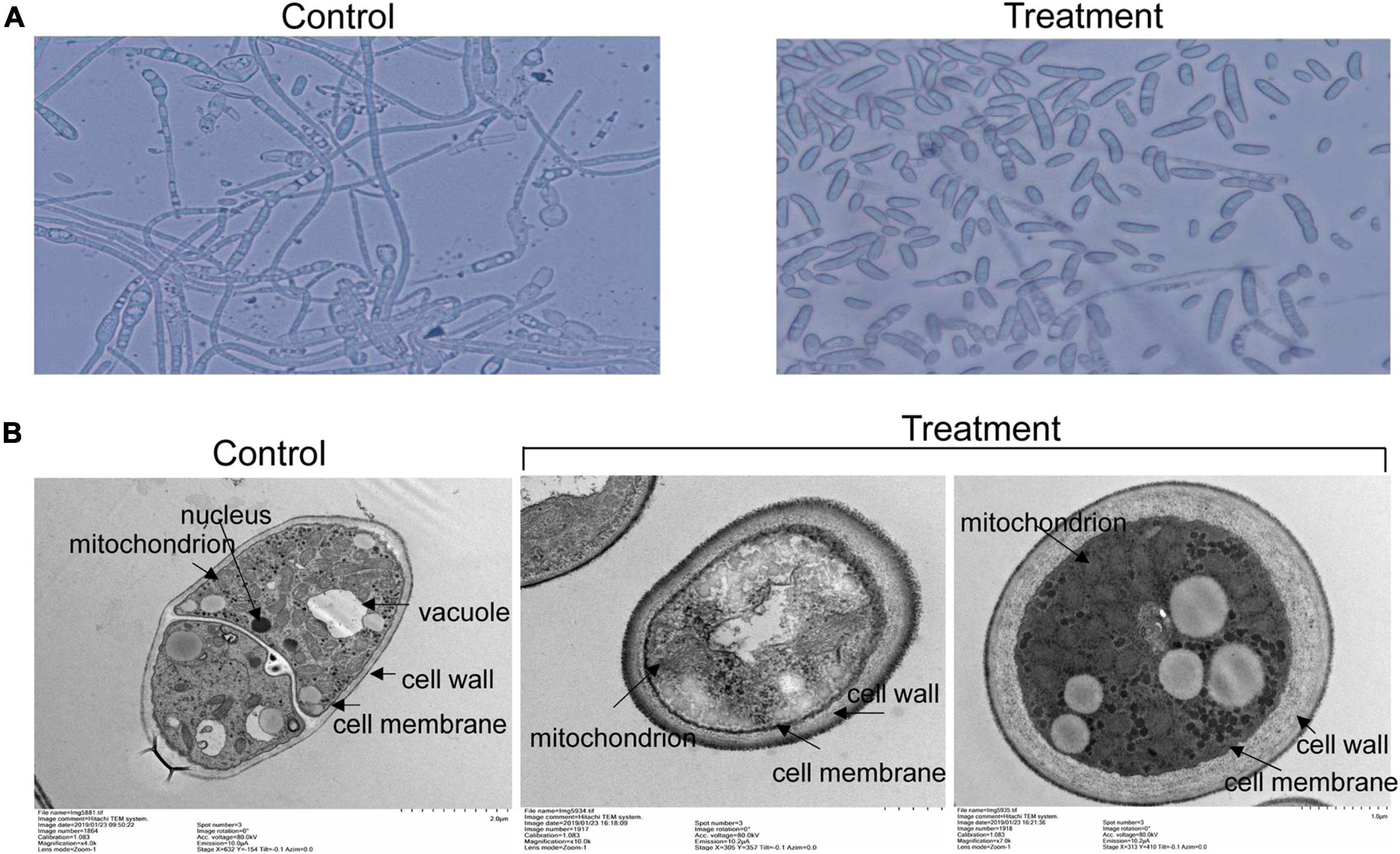
Figure 7. Effects of strain SCA2-4T extracts on spore germination and cell structure of Foc TR4. 10% of DMSO treatment was used as control. (A) Measurement of spore germination after treated with 16 × MIC of strain SCA2-4T extracts. (B) Cell structure of Foc TR4 treated with 16 × MIC of strain SCA2-4T extracts was detected using the transmission electron microscopy.
Conclusion
An actinobacterial strain SCA2-4T was isolated from the rhizospheric soil of O. stricta in a dry hot valley and selected for its excellent antifungal activity against Foc TR4. Strain SCA2-4T was identified as a novel species of the genus Streptomyces in the light of genotype and phenotype data. The strain was proposed as Streptomyces huiliensis sp. nov. The whole genome analysis showed that strain SCA2-4T contained 51 putative biosynthetic gene clusters. Especially, ten gene clusters were involved in the biosynthesis of antimicrobial metabolites. The strain also exhibited high antifungal activity against pathogens of other banana fungal diseases such as Foc 1, C. fallax, and C. lunata. Extracts of strain SCA2-4T seriously destroyed fungal cell structure of Foc TR4, inhibited the mycelial growth and spore germination. MIC was 6.25 μg/mL against Foc TR4. These results implied that this strain could be a promising candidate for biological control of banana Fusarium wilt.
Data Availability Statement
The datasets presented in this study can be found in online repositories. The names of the repository/repositories and accession number(s) can be found in the article/Supplementary Material.
Author Contributions
DQ, LiZ, DZ, LZ, JX, and WW developed the ideas and designed the experimental plans. WW and JX supervised the research and provided the fund support. DQ, LiZ, DZ, MZ, and YW performed experiments. DQ, WW, and JX provided the materials. DQ, LZ, and WW analyzed the data and prepared the manuscript. All authors contributed to the article and approved the submitted version.
Funding
This work was supported by the National Key Research and Development Program of China (2020YFD1000104), the National Natural Science Foundation of China (31770476 and 32072504), the Natural Science Foundation of Hainan (2019RC293 and 320CXTD441), and the China Agriculture Research System (CARS-31).
Conflict of Interest
The authors declare that the research was conducted in the absence of any commercial or financial relationships that could be construed as a potential conflict of interest.
Publisher’s Note
All claims expressed in this article are solely those of the authors and do not necessarily represent those of their affiliated organizations, or those of the publisher, the editors and the reviewers. Any product that may be evaluated in this article, or claim that may be made by its manufacturer, is not guaranteed or endorsed by the publisher.
Acknowledgments
We thank Xiaolong Huang and Zhufeng Gao for providing your help to this work.
Supplementary Material
The Supplementary Material for this article can be found online at: https://www.frontiersin.org/articles/10.3389/fmicb.2021.722661/full#supplementary-material
Supplementary Figure 1 | Genome-wide analysis of strain SCA2-4T for gene clusters of the biosynthesis of secondary metabolites by the online antiSMASH v4.2.0 software.
Supplementary Table 1 | Allele sequence accession numbers of the Streptomyces used for the present study.
Supplementary Table 2 | MLSA distance values for selected strains in this study.
Supplementary Table 3 | Nitrogen-source utilization and antibiotic sensitivity of strain SCA2-4T.
Supplementary Table 4 | Functional cluster of orthologous genes (COG) classification of predicted genes in strain SCA2-4T.
Supplementary Table 5 | Biosynthetic gene clusters of secondary metabolite of strain SCA2-4T on the online antiSMASH v 6.0.1.
Supplementary Table 6 | Cluster number and gene number shown in different cluster types of strain SCA2-4T.
Supplementary Table 7 | Inhibitory activity and MIC of extracts of strain SCA2-4T against Foc TR4.
Footnotes
- ^ http://www.bacterio.net/
- ^ https://www.ezbiocloud.net/search?tn=Nocardioides
- ^ http://www.majorbio.com/
- ^ http://ccb.jhu.edu/software/glimmer/index.shtml
- ^ http://antismash.secondarymetabolites.org/#!/start
References
Aghighi, S., Shahidi Bonjar, G. H., Saadoun, I., Rawashdeh, R., and Batayneh, S. (2004). First report of antifungal spectra of activity of Iranian actinomycetes strains against Alternaria solani, Alternaria alternata, Fusarium solani, Phytophthora megasperma, Verticillium dahliae and Saccharomyces cerevisiae. Asian J. Plant Sci. 3, 463–471. doi: 10.3923/ajps.2004.463.471
Ahmad, M. S., Elgendy, A. O., Ahmed, R. R., Hassan, H. M., Elkabbany, H. M., and Merdash, A. G. (2017). Exploring the antimicrobial and antitumor potentials of Streptomyces sp. AGM12-1 isolated from Egyptian soil. Front. Microbiol. 8:438. doi: 10.3389/fmicb.2017.00438
Altschul, S. F., Gish, W., Miller, W., Myers, E. W., and Lipman, D. J. (1990). Basic local alignment search tool. J. Mol. Biol. 215, 403–410. doi: 10.1016/S0022-2836(05)80360-2
Brady, C., Cleenwerck, I., Venter, S., Vancanneyt, M., Swings, J., and Coutinho, T. (2008). Phylogeny and identification of Pantoea species associated with plants, humans and the natural environment based on multilocus sequence analysis (MLSA). Syst. Appl. Microbiol. 31, 447–460. doi: 10.1016/j.syapm.2008.09.004
Bush, J. A., Long, B. H., Catino, J. J., and Bradner, W. T. (1987). Production and biological activity of rebeccamycin, a novel antitumor agent. J. Antibiot. 40, 668–678. doi: 10.7164/antibiotics.40.668
Cao, L., Qiu, Z., Dai, X., Tan, H., Lin, Y., and Zhou, S. (2004). Isolation of endophytic actinomycetes from roots and leaves of banana (Musa acuminata) plants and their activities against Fusarium oxysporum f. sp. cubense. World J. Microb. Biotechnol. 20, 501–504. doi: 10.1023/B:WIBI.0000040406.30495.48
Cao, L., Qiu, Z., You, J., Tan, H., and Zhou, S. (2005). Isolation and characterization of endophytic Streptomycete antagonists of Fusarium wilt pathogen from surface-sterilized banana roots. FEMS Microbiol. Lett. 247, 147–152. doi: 10.1016/j.femsle.2005.05.006
Chen, Y. F., Zhou, D. B., Qi, D. F., Gao, Z. F., Xie, J. H., and Luo, Y. P. (2018). Growth promotion and disease suppression ability of a Streptomyces sp. CB-75 from banana rhizosphere soil. Front. Microbiol. 8:2704. doi: 10.3389/fmicb.2017.02704
Chun, J., Oren, A., Ventosa, A., Christensen, H., Arahal, D. R., Da, C. M., et al. (2018). Proposed minimal standards for the use of genome data for the taxonomy of prokaryotes. Int. J. Syst. Evol. Micr. 68, 461–466. doi: 10.1099/ijsem.0.002516
Cox, C. L., Tietz, J. I., Sokolowski, K., Melby, J. O., Doroghazi, J. R., and Mitchell, D. A. (2014). Nucleophilic 1,4-additions for natural product discovery. ACS Chem. Biol. 9, 2014–2022. doi: 10.1021/cb500324n
Delcher, A. L., Bratke, K. A., Powers, E. C., and Salzberg, S. L. (2007). Identifying bacterial genes and endosymbiont DNA with Glimmer. Bioinformatics 23, 673–679. doi: 10.1093/bioinformatics/btm009
Dey, E. S., Ahmadi-Afzadi, M., Nybom, H., and Tahir, I. (2013). Alkylresorcinols isolated from rye bran by supercritical fluid of carbon dioxide and suspended in a food-grade emulsion show activity against Penicillium expansum on apples. Arch. Phytopathol. Plant Prot. 46, 105–119. doi: 10.1080/03235408.2012.73471
Engl, T., Kroiss, J., Kai, M., Nechitaylo, T. Y., Svato, A., and Kaltenpoth, M. (2018). Evolutionary stability of antibiotic protection in a defensive symbiosis. Proc. Natl. Acad. Sci. U.S.A. 115, E2020–E2029. doi: 10.1073/pnas.1719797115
Felsenstein, J. (1981). Evolutionary trees from DNA sequences: a maximum likelihood approach. J. Mol. Evol. 17, 368–376. doi: 10.1007/BF01734359
Felsenstein, J. (1985). Confidence limits on phylogenies: an approach using the bootstrap. Evolution 39, 783–791. doi: 10.1111/j.1558-5646.1985.tb00420.x
Fitch, W. M. (1971). Toward defining the course of evolution: minimum change for a specific tree topology. Syst. Biol. 20, 406–416. doi: 10.1093/sysbio/20.4.406
Getha, K., Vikineswary, S., Wong, W. H., Seki, T., Ward, A., and Goodfellow, M. (2005). Evaluation of Streptomyces sp. strain g10 for suppression of fusarium wilt and rhizosphere colonization in pot-grown banana plantlets. J. Ind. Microbiol. Biotechnol. 32, 24–32. doi: 10.1007/s10295-004-0199-5
Goris, J., Konstantinidis, K. T., Klappenbach, J. A., Coenye, T., and Tiedje, J. M. (2007). DNA-DNA hybridization values and their relationship to whole-genome sequence similarities. Int. J. Syst. Evol. Microbiol. 57, 81–91. doi: 10.1099/ijs.0.64483-0
Gu, S., Yang, T., Shao, Z., Wang, T., and Pommier, T. (2020). Siderophore-mediated interactions determine the disease suppressiveness of microbial consortia. mSystems 5, e811–e819. doi: 10.1128/mSystems.00811-19
Guo, Y. P., Zheng, W., Rong, X. Y., and Huang, Y. (2008). A multilocus phylogeny of the Streptomyces griseus 16s rRNA gene clade: use of multilocus sequence analysis for Streptomycete systematics. Int. J. Syst. Evol. Microbiol. 58, 149–159. doi: 10.1099/ijs.0.65224-0
Henkel, T., Rohr, J., Beale, J. M., and Schwenen, L. (1990). Landomycins, new angucycline antibiotics from Streptomyces sp. I. structural studies on landomycins A-D. J. Antibiot. 43, 492–503. doi: 10.7164/antibiotics.43.492
Hohmann, C., Schneider, K., Bruntner, C., Irran, E., Nicholson, G., Bull, A. T., et al. (2009). Caboxamycin, a new antibiotic of the benzoxazole family produced by the deep-sea strain Streptomyces sp. NTK 937. J. Antibiot. 62, 99–104. doi: 10.1038/ja.2008.24
Huang, X. L., Kong, F. D., Zhou, S. Q., Huang, D. Y., Zheng, J. P., and Zhu, W. M. (2019). Streptomyces tirandamycinicus sp. nov. a novel marine sponge-derived actinobacterium with antibacterial potential against Streptococcus agalactiae. Front. Microbiol. 10:482. doi: 10.3389/fmicb.2019.00482
Jing, T., Zhou, D. B., Zhang, M. Y., Yun, T. Y., Qi, D. F., Wei, Y. Z., et al. (2020). Newly isolated Streptomyces sp. JBS5-6 as a potential biocontrol agent to control banana fusarium wilt: genome sequencing and secondary metabolite cluster profiles. Front. Microbiol. 11:602591. doi: 10.3389/fmicb.2020.602591
Kai, B., Thomas, W., Chevrette, M. G., Lu, X., Schwalen, C. J., Kautsar, S. A., et al. (2017). Antismash 4.0-improvements in chemistry prediction and gene cluster boundary identification. Nucleic Acids Res. 45, W36–W41. doi: 10.1093/nar/gkx319
Kelly, K. L. (1958). Centroid notations for the revised ISCC-NBC color name blocks. J. Res. Nat. Bur. Stand. 61:427.
Kimura, M. (1980). A simple method for estimating evolutionary rates of base substitutions through comparative studies of nucleotide sequences. J. Mol. Evol. 16, 111–120. doi: 10.1007/BF01731581
Komagata, K., and Suzuki, K.-I. (1987). Lipid and cell-wall analysis in bacterial systematics. Methods Microbiol. 19, 161–207.
Konstantinidis, K. T., and Tiedje, J. M. (2005). Genomic insights that advance the species definition for prokaryotes. Proc. Natl. Acad. Sci. U.S.A. 102, 2567–2572. doi: 10.1073/pnas.0409727102
Kumar, S., Stecher, G., and Tamura, K. (2016). MEGA7: molecular evolutionary genetics analysis version 7.0 for bigger datasets. Mol. Biol. Evol. 33, 1870–1874. doi: 10.1093/molbev/msw054
Kumar, V., Naik, B., Gusain, O., and Bisht, G. S. (2014). An actinomycete isolate from solitary wasp mud nest having strong antibacterial activity and kills the Candida cells due to the shrinkage and the cytosolic loss. Front. Microbiol. 5:446. doi: 10.3389/fmicb.2014.00446
Kuster, E., and Williams, S. T. (1964). Selection of media for isolation of Streptomycetes. Nature 202, 928–929. doi: 10.1038/202928a0
Lombó, F., Braña, A. F., Salas, J. A., and Méndez, C. (2004). Genetic organization of the biosynthetic gene cluster for the antitumor angucycline oviedomycin in Streptomyces antibioticus ATCC 11891. Chembiochem 5, 1181–1187. doi: 10.1002/cbic.200400073
Loosley, B. C., Andersen, R. J., and Dake, G. R. (2013). Total synthesis of cladoniamide G. Org. Lett. 15, 1152–1154. doi: 10.1021/ol400055v
Luo, R., Liu, B., Xie, Y., Li, Z., and Liu, Y. (2012). SOAPdenovo2: an empirically improved memory-efficient short-read de novo assembler. GigaScience 1:18. doi: 10.1186/2047-217X-1-18
Martens, M., Dawyndt, P., Coopman, R., Gillis, M., Vos, P. D., and Willems, A. (2008). Advantages of multilocus sequence analysis for taxonomic studies: a case study using 10 housekeeping genes in the genus ensifer (including former sinorhizobium). Int. J. Syst. Evol. Micrrbiol. 58, 200–214. doi: 10.1099/ijs.0.65392-0
Minnikin, D., Collins, M., and Goodfellow, M. (1979). Fatty acid and polar lipid composition in the classification of Cellulomonas, Oerskovia and related taxa. J. Appl. Bacteriol. 47, 87–95. doi: 10.1111/j.1365-2672.1979.tb01172.x
Mizuhara, N., Kuroda, M., Ogita, A., Tanaka, T., Usuki, Y., and Fujita, K. I. (2011). Antifungal thiopeptide cyclothiazomycin B1 exhibits growth inhibition accompanying morphological changes via binding to fungal cell wall chitin. Bioorgan. Med. Chem. 19, 5300–5310. doi: 10.1016/j.bmc.2011.08.010
Mohandas, S., and Ravishankar, K. V. (2016). Banana: Genomics and Transgenic Approaches for Genetic Improvement. Singapore: Springer, 211–226.
Nakano, H., and Omura, S. (2009). Chemical biology of natural indolocarbazole products: 30 years since the discovery of staurosporine. J. Antibiot. 62, 17–26. doi: 10.1038/ja.2008.4
Nogueira, J. H. C., GoncAlez, E., Galleti, S. R., Facanali, R., Marques, M., and Felício, J. D. (2010). Ageratum conyzoides essential oil as aflatoxin suppressor of aspergillus flavus. Int. J. Food Microbiol. 137, 55–60. doi: 10.1016/j.ijfoodmicro.2009.10.017
Ploetz, R. C. (2015). Fusarium wilt of banana. Phytopathology 105, 1512–1521. doi: 10.1094/phyto-04-15-0101-rvw
Qi, D. F., Zou, L. P., Zou, D. B., Chen, Y. F., Gao, Z. F., Feng, R. J., et al. (2019). Taxonomy and broad-spectrum antifungal activity of Streptomyces sp. SCA3-4 isolated from rhizosphere soil of Opuntia stricta. Front. Microbiol. 10:1390. doi: 10.3389/fmicb.2019.01390
Richter, M., and Rossello-Mora, R. (2009). Shifting the genomic gold standard for the prokaryotic species definition. Proc. Natl. Acad. Sci. U.S.A. 106, 19126–19131. doi: 10.1073/pnas.0906412106
Rong, X. Y., and Huang, Y. (2010). Taxonomic evaluation of the Streptomyces griseus clade using multilocus sequence analysis and DNA-DNA hybridization, with proposalto combine 29 species and three subspecies as 11 genomic species. Int. J. Syst. Evol. Microbiol. 60, 696–703. doi: 10.1099/ijs.0.012419-0
Rong, X. Y., and Huang, Y. (2012). Taxonomic evaluation of the Streptomyces hygroscopicus clade using multilocus sequence analysis and DNA-DNA hybridization, validating the MSLA scheme for systematics of the whole genus. Syst. Appl. Microbiol. 35, 7–18. doi: 10.1016/j.syapm.2011.10.004
Rong, X. Y., Liu, N., Ruan, J. S., and Huang, Y. (2010). Multilocus sequence analysis of Streptomyces griseus isolates delineating intraspecific diversity in terms of both taxonomy and biosynthetic potential. Antonie Van Leeuwenhoek 98:237. doi: 10.1007/s10482-010-9447-z
Saitou, N., and Nei, M. (1987). The neighbor-joining method: a new method for reconstructing phylogenetic trees. Mol. Biol. Evol. 4, 406–425. doi: 10.1093/oxfordjournals.molbev.a040454
Salehi, B., Fokou, P., Sharifi-Rad, M., Zucca, P., Pezzani, R., Martins, N., et al. (2019). The therapeutic potential of naringenin: a review of clinical trials. Pharmaceuticals 12:11. doi: 10.3390/ph12010011
Sasser, M. (1990). Identification of Bacteria by Gas Chromatography of Cellular Fatty Acids. Technical Note 101. Newark, DE: MIDI Inc.
Schimana, J., Fiedler, H. P., Groth, I., Suessmuth, R., Beil, W., Walker, M., et al. (2000). Simocyclinones, novel cytostatic angucyclinone antibiotics produced by Streptomyces antibioticus Tü 6040. I. taxonomy, fermentation, isolation and biological activities. J. Antibiot. 53, 779–787. doi: 10.7164/antibiotics.53.779
Sheng, M. M., Jia, H. K., Zhang, G. Y., Zeng, L. N., Zhang, T. T., Long, Y. H., et al. (2020). Siderophore production by rhizosphere biological control bacteria Brevibacillus brevis GZDF3 of Pinellia ternata and its antifungal effects on Candida albicans. J. Microbiol. Biotechnol. 30, 689–699. doi: 10.4014/jmb.1910.10066
Shirling, E. B., and Gottlieb, D. (1966). Methods for characterization of Streptomyces species. Int. J. Syst. Evol. Micr. 16, 313–340. doi: 10.1099/00207713-16-3-313
Singh, S., Kim, Y., Wang, F., Bigelow, L., Endres, M., Kharel, M. K., et al. (2015). Structural characterization of Atms13, a putative sugar aminotransferase involved in indolocarbazole AT2433 aminopentose biosynthesis. Proteins 83, 1547–1554. doi: 10.1002/prot.24844
Snipes, C. E., Chang, C. J., and Floss, H. G. (1979). Biosynthesis of the antibiotic granaticin. J. Am. Chem. Soc. 101, 701–706. doi: 10.1021/ja00497a036
Stackebrandt, E., and Goebel, B. M. (1994). Taxonomic note: a place for DNA-DNA reassociation and 16S rRNA sequence analysis in the present species definition in bacteriology. Int. J. Syst. Bacteriol. 44, 846–849. doi: 10.1099/00207713-44-4-846
Takano, S., Hasuda, K., Ito, A., Koide, Y., Ishii, F., Haneda, I., et al. (1976). A new antibiotic, medermycin. J. Antibiot. 29, 765–768. doi: 10.7164/antibiotics.29.765
Tepe, B., Daferera, D., Sokmen, A., Sokmen, M., and Polissiou, M. (2005). Antimicrobial and antioxidant activities of the essential oil and various extracts of Salvia tomentosa Miller (Lamiaceae). Food Chem. 90, 333–340. doi: 10.1016/j.foodchem.2003.09.013
Wang, X., Zhou, H. B., Chen, H. N., Jing, X. S., Zheng, W. T., Li, R. J., et al. (2018). Discovery of recombinases enables genome mining of cryptic biosynthetic gene clusters in Burkholderiales species. Proc. Natl. Acad. Sci. U.S.A. 115, E4255–E4263. doi: 10.1073/pnas.1720941115
Wang, X. N., Radwan, M. M., Taráwneh, A. H., Gao, J. T., Wedge, D. E., Rosa, L. H., et al. (2013). Antifungal activity against plant pathogens of metabolites from the endophytic fungus Cladosporium cladosporioides. J. Agric. Food Chem. 61, 4551–4555. doi: 10.1021/jf400212y
Watve, M. G., Tickoo, R., Jog, M. M., and Bhole, B. D. (2001). How many antibiotics are produced by the genus Streptomyces? Arch Microbiol. 176, 386–390. doi: 10.1007/s002030100345
Williams, S. T., Goodfellow, M., Alderson, G., Wellington, E. M. H., Sneath, P. H. A., and Sackin, M. J. (1983). Numerical classification of Streptomyces and related genera. J. Gen. Microbiol. 129, 1743–1813. doi: 10.1099/00221287-129-6-1743
Yoon, S. H., Ha, S. M., Kwon, S., Lim, J., Kim, Y., Seo, H., et al. (2017a). Introducing ezbiocloud: a taxonomically united database of 16S rRNA and whole genome assemblies. Int. J. Syst. Evol. Microbiol. 67, 1613–1617. doi: 10.1099/ijsem.0.001755
Keywords: Streptomyces, novel species, identification, banana Fusarium wilt, antifungal activities, genome sequencing
Citation: Qi D, Zou L, Zhou D, Zhang M, Wei Y, Zhang L, Xie J and Wang W (2021) Identification and Antifungal Mechanism of a Novel Actinobacterium Streptomyces huiliensis sp. nov. Against Fusarium oxysporum f. sp. cubense Tropical Race 4 of Banana. Front. Microbiol. 12:722661. doi: 10.3389/fmicb.2021.722661
Received: 10 June 2021; Accepted: 14 October 2021;
Published: 04 November 2021.
Edited by:
Eustachio Tarasco, University of Bari Aldo Moro, ItalyReviewed by:
Sukhendu Mandal, University of Calcutta, IndiaNatsuko Ichikawa, National Institute for Testing and Evaluation (NITE), Israel
Copyright © 2021 Qi, Zou, Zhou, Zhang, Wei, Zhang, Xie and Wang. This is an open-access article distributed under the terms of the Creative Commons Attribution License (CC BY). The use, distribution or reproduction in other forums is permitted, provided the original author(s) and the copyright owner(s) are credited and that the original publication in this journal is cited, in accordance with accepted academic practice. No use, distribution or reproduction is permitted which does not comply with these terms.
*Correspondence: Lu Zhang, luzhangtest@163.com, zhanglutest@163.com; Jianghui Xie, 2453880045@qq.com, xiejianghui@itbb.org.cn; Wei Wang, wangweisys@ahau.edu.cn