- 1Department of Biological Functions Engineering, Graduate School of Life Science and Systems Engineering, Kyushu Institute of Technology, Kitakyushu, Japan
- 2Institute of Environmental Science and Technology, The University of Kitakyushu, Kitakyushu, Japan
- 3Department of Microbiology and Parasitology, Faculty of Medicine, UNAM, Mexico City, Mexico
- 4Laboratory of Infectology, National Institute of Rehabilitation Luis Guillermo Ibarra Ibarra, Mexico City, Mexico
Bdellovibrio bacteriovorus is one of the predatory bacteria; therefore, it can act as a novel “living antibiotic,” unlike the current antibiotics. Here the predation of Escherichia coli by B. bacteriovorus was inhibited in the presence of Pseudomonas aeruginosa. This study investigated whether P. aeruginosa-induced predation inhibition is associated with bacterial quorum sensing (QS). Each las, rhl, or pqs QS mutant in P. aeruginosa was used to check the predatory activity of E. coli cells using B. bacteriovorus. As a result, the predatory activity of B. bacteriovorus increased in a mutant pqs QS system, whereas wild-type PA14 inhibited the predatory activity. Moreover, the addition of 4-hydroxy-2-heptylquinoline (HHQ) or the analog triggered the low predatory activity of B. bacteriovorus and killed B. bacteriovorus cells. Therefore, a defensive action of P. aeruginosa against B. bacteriovorus is activated by the pqs QS system, which produces some quinolone compounds such as HHQ.
Introduction
Bdellovibrio bacteriovorus is a small-sized Gram-negative bacterium belonging to the group Bdellovibrio and like organisms (BALOs). It is one of the obligate predatory bacteria which prey another Gram-negative bacterium (Stolp and Starr, 1963). Although B. bacteriovorus cannot prey upon Gram-positive bacterial species, it is known to be able to degrade the biofilm formed by Gram-negative bacteria and Gram-positive ones (Kadouri and O’Toole, 2005; Dashiff et al., 2011; Im et al., 2018). B. bacteriovorus also has a competitive inhibition against Staphylococcus aureus (Im et al., 2018). BALOs exist in a diverse environment such as waste sewage sludge (Koval et al., 2013), river (Hobley et al., 2012), sea (Pineiro et al., 2007; Chen et al., 2015; Tang et al., 2020), and various habitats on the ground (Jurkevitch et al., 2000). B. bacteriovorus usually grows through the invasion, digestion, and lysis of other Gram-negative bacteria as host cells; then, it again swims to find other new host cells, predate, and replicate further (Pérez et al., 2016). In the predation for Gram-negative bacteria, B. bacteriovorus takes 2–4 h to complete all the processes, including the attachment using type IVa pili (Evans et al., 2007; Mahmoud and Koval, 2010), the modification of peptidoglycan of host cells (Stolp and Starr, 1965; Kuru et al., 2017), the digestion, and the lysis, which some enzymes can handle as obtained from B. bacteriovorus (Thomashow and Rittenberg, 1978a,b; Lerner et al., 2012). Hence, B. bacteriovorus has the potential to be applied in many fields—for example, B. bacteriovorus may have a certain potential to kill multidrug-resistant bacteria (Iebba et al., 2014), remove harmful bacteria on food (Fratamico and Cooke, 1996), decrease waste sewage sludge (Semblante et al., 2017), and disperse biofilm on the pipes in wastewater treatment plants (Feng et al., 2016). To date, B. bacteriovorus is expected to be available for cystic fibrosis patients with chronic infections (Sockett and Lambert, 2004; Shatzkes et al., 2015) caused by Pseudomonas aeruginosa which is known as one of the critical strains of multidrug-resistant bacteria because B. bacteriovorus was discovered to prey on P. aeruginosa by some researchers (Stolp and Starr, 1963; Shanks et al., 2013; Iebba et al., 2014). However, other researchers also reported that P. aeruginosa is not preyed upon by B. bacteriovorus (Mukherjee et al., 2015). Thus, the predatory performance of B. bacteriovorus to P. aeruginosa strains is inconsistent; however, the reason is still unknown.
Most bacteria in nature do not live independently but communicate with each other through extracellular signal molecules to control their collective behavior. These signal molecules are mechanisms released into the environment and respond after they are taken up by the surrounding cells (Fuqua et al., 2001; Miller and Bassler, 2001; Withers et al., 2001). Quorum sensing (QS), one of the most essential systems for regulating bacterial functions, is a typical communication system used by many bacterial species to activate or repress a large number of genes, which form the QS regulon. Changes in their expression levels are achieved once the signal molecules reach a given threshold (Fuqua et al., 1994). It controls bacterial functions such as biofilm formation, resistance to acid stress, motility, competence, and morphological modifications (Huang et al., 2016; Johansen and Jespersen, 2017; de Almeida et al., 2018). Gram-negative bacteria use small diffusible molecules called autoinducers such as indole (Lee et al., 2015), acyl-homoserine lactones, autoinducer-2 (Galloway et al., 2011), and alkyl-quinolone (Pesci et al., 1999) among others—for example, P. aeruginosa also regulates the gene expression of some virulence factors by using four QS systems directly or indirectly: las regulation system, rhl regulation system, pqs regulation system, and iqs regulation system (Winson et al., 1995; Latifi et al., 1996; Pesci et al., 1997, 1999; McKnight et al., 2000; Gallagher et al., 2002; Kiratisin et al., 2002; Déziel et al., 2004; Xiao et al., 2006; Lee et al., 2013). P. aeruginosa uses 3-oxo-C12-homoserine lactone for the las QS system, C4-homoserine lactone for the rhl QS system, and integrated QS signal [IQS; 2-(2-hydroxyphenyl)-thiazole-4-carbaldehyde]. In particular, the pqs QS system is known as the most complicated one among the QS systems of P. aeruginosa since the system has three kinds of quinolone compounds: Pseudomonas quinolone signals [PQS; 2-heptyl-3-hydroxy-4(1H)-quinolone], 4-hydroxy-2-heptylquinolone (HHQ), and 2-heptyl-4-quinolinol-1-oxide (HQNO). Thus, all the QS signal molecules have the function of promoting or inhibiting other bacteria, fungi, protozoa, and nematodes (Fuqua et al., 1994; Burgess et al., 1999; Dubuis et al., 2007). Changes in the expression of autoinducer and QS-controlled compounds can also affect not only bacterial community dynamics but also host responses during infection. It has been reported that various QS regulatory molecules, such as acyl-homoserine lactones, quinolones, and phenazines, can interact with host cells and thereby affect various responses, including immune regulation (Liu et al., 2015). However, E. coli strains, including E. coli O157:H7 (Page et al., 2015), use indole as one of the signal molecules (Wang et al., 2001), and Dwidar et al. (2015) investigated that a high concentration (2 mM) of indole inhibits the predation of E. coli by B. bacteriovorus. Since 0.25–1.1 mM indole was detected in human feces (Karlin et al., 1985; Zuccato et al., 1993), indole may help E. coli to inactivate the predatory activity of B. bacteriovorus if they grow together. Thus, we hypothesized that the QS systems of Gram-negative bacteria might influence the B. bacteriovorus cells negatively.
Although the effect of indole on the predatory activity of B. bacteriovorus was investigated, there are no reports on how other QS molecules of Gram-negative bacteria influence the activity of B. bacteriovorus. In this study, the relationship between the QS systems of P. aeruginosa and the predatory activities of B. bacteriovorus was investigated.
Materials and Methods
Chemicals
The following compounds, 2-heptyl-3-hydroxy-4(1H)-quinolone (PQS; Pseudomonas quinolone signal), 4-hydroxy-2-heptylquinolone (HHQ), and 2-amino-6-chlorobenzoic acid (CABA), which is a pqs QS inhibitor, were purchased from Sigma-Aldrich Japan (Tokyo, Japan), and 2-heptyl-4-quinolinol-1-oxide (HQNO) was purchased from Cayman Chemical Company (Michigan, United States). As one of the quinolone analogs, nalidixic acid was also purchased from FUJIFILM Wako Pure Chemical Corporation, Ltd. (Osaka, Japan). PQS and HHQ were stocked in dimethyl sulfoxide (DMSO) to make an 80-mM standard solution. HQNO, nalidixic acid, and CABA were stocked in DMSO to be 8-mM, 16-mM, and 1.5-M stock solutions, respectively.
Bacterial Strains
B. bacteriovorus 109J (ATCC 43826), used as a predatory bacterium in this study, was purchased from the ATCC culture collection. The glycerol stock of B. bacteriovorus cells was made by mixing a cell culture of B. bacteriovorus and an equal volume of 2 × stock solution consisting of 10 g of yeast extract, 100 ml DMSO, 100 ml glycerol, 312 ml of 0.2 M NaH2PO4, and 488 ml of 0.2 M Na2HPO4 in 1 liter of the total stock solution, and the mixture was stored at −70°C. The Escherichia coli strains and P. aeruginosa strains used in this study are listed in Table 1.
Growth Condition and Predation Assay
These strains were initially streaked on Luria Bertani (LB) (Sambrook et al., 1989) agar plates and incubated overnight at 37°C. Then, a single colony of each strain was used for inoculation. For the cell preparation of prey cells, a single colony of E. coli and P. aeruginosa strains was inoculated into 100 ml of LB medium and aerobically incubated overnight at 37°C and 120 rpm. Mainly E. coli cells in the log phase (8–12 h) were used. The overnight culture was centrifuged for 10 min at 7,000 rpm and 4°C to wash the cell pellet using HEPES-based buffer, including 25 mM HEPES and 2 mM CaCl2 (the pH was adjusted to 7.6 using 5 M NaOH). Then, each washed pellet was suspended and adjusted at ∼1.5 or two of OD600 by the same HEPES buffer. The final cell suspensions were used for propagating B. bacteriovorus cells (when E. coli BW25113 was used) and for the predation assays in the experiments. For the predation assay using the double-layer method, as mentioned above, the top agar (3 ml) consisting of B. bacteriovorus culture (100 μl) and E. coli overnight culture (100 μl) was first poured to the bottom agar plate. After the agar has hardened, 2 μl of bacterial overnight culture and/or 2 μl of standard chemical solution was put on the center of each plate, and these plates were incubated at 30°C for 2–3 days. An inhibition zone (the area where E. coli cells were grown) was measured with a ruler at three points per plate. Three independent experiments were carried out.
Preparation of B. bacteriovorus Cells and Double-Layer Method
Initially, the purity of B. bacteriovorus stock was confirmed by checking the plaque formation in a double-layer method in which 3 ml nutrient broth (NB) top agar (kept warm at 50°C; 8 g/l NB, 0.286 g/l MgCl2, 0.222 g/l CaCl2, and 8 g/l agar in pure water) mixed with 100 μl B. bacteriovorus culture and 100 μl of E. coli growth culture (at 37°C and 120 rpm for 10 h) was poured to NB agar bottom plates consisting of 10 g/l agar concentration and incubated at 30°C for around a week. A piece of top agar with the plaque of B. bacteriovorus was sliced at 1 cm2 and transferred under sterile conditions to the E. coli cell suspension (60 ml). The mixture was incubated at 37°C and 120 rpm for 2–3 days until the cell turbidity, mainly derived from E. coli cells, drastically decreased. Then, a pure cell culture of B. bacteriovorus was obtained by removing E. coli cells from the culture with a 0.45-μm-pore-size membrane filter. The filtrate was used as the cell culture of B. bacteriovorus for all the experiments in this study.
Viability Tests of Prey Cells in the Presence of B. bacteriovorus
E. coli and P. aeruginosa cell suspensions, as mentioned above, were concentrated to OD600 of 2.0. The E. coli cell suspensions (5 ml) were incubated with or without the inoculation of B. bacteriovorus cell culture (167 μl) (multiplicity of infection, MOI = 0.01–0.1). The MOI was calculated by dividing the viable count of B. bacteriovorus in a 167-μl cell culture by the viable count of E. coli in 5-ml cell suspension at ∼2 of the cell turbidity at OD600. B. bacteriovorus cell culture (167 μl) was also inoculated in a mixture of 2.5 ml E. coli and P. aeruginosa cell suspension. As a control, without B. bacteriovorus, the sample with only E. coli and P. aeruginosa cell suspension was also prepared. These mixtures were incubated at 30°C and 120 rpm for a week. Then, an appropriate serial dilution of tester samples was prepared by using 0.85% sterile NaCl solution. To evaluate the survival of the prey cells, serial dilution was spread on LB agar plates and incubated at 37°C under aerobic conditions overnight. Then, the colonies grown on each plate were counted. The distinction between E. coli colonies and P. aeruginosa colonies was determined visually based on the shape, size, and color of the colonies. Here the plates with pure cultures of E. coli and P. aeruginosa, respectively, were prepared as standards. Two or three independent experiments were carried out.
Pyocyanin Extraction
For pyocyanin extraction, a single colony of P. aeruginosa PA14 was inoculated in 5 ml of LB medium and incubated overnight at 37°C and 120 rpm. The overnight culture (1.83 ml) was inoculated in 0.25% casein-M9 medium (100 ml) to be 0.05 of the cell turbidity at OD600 (2.5 g casein, 6.0 g Na2HPO4, 3.0 g KH2PO4, 1.0 g NH4Cl, 0.5 g NaCl, 2 ml of 1 M MgSO4, and 0.1 ml of 1 M CaCl2 in 1 liter of water; the pH was adjusted to 7 using 5 M NaOH) and incubated for 24 h at 37°C and 180 rpm. Pyocyanin was extracted by using chloroform and 0.2 M HCl (Essar et al., 1990) from the overnight culture (100 ml) of P. aeruginosa PA14. The concentration of pyocyanin extracted was determined by measuring the absorbance at 520 nm using a V-530 spectrophotometer (JASCO Corporation, Tokyo, Japan). The molar absorptivity of acidic pyocyanin (ε = 2.46 mM–1 cm–1) (O’Malley et al., 2004) was used for the calculation of the concentration. The acidic pyocyanin solution was finally adjusted to pH ∼7 by adding 5 M NaOH and stocked at a concentration of 6.63 mM. The stock solution was filtered with a 0.20-μm membrane filter.
Viability Tests of Predatory Cells in the Presence of Quinolone Compounds
Each solution of quinolone compounds was mixed with B. bacteriovorus cell culture (0.5 ml) and HEPES buffer (4.5 ml) to be at a concentration of 80 μM. As a control including the only solvent, 2 μl DMSO was added to the B. bacteriovorus mixture in HEPES buffer without any chemical compound. The mixture was incubated at 30°C and 120 rpm. The mixture was collected in 0, 3, and 6 days to measure the number of viable predatory cells. In evaluating the viability of predatory cells, the double-layer method mentioned above was conducted. An appropriate serial dilution of the B. bacteriovorus cell culture was used to count the number (30–300) of plaques formed on the agar plates after incubation at 30°C for around a week. Two independent experiments were carried out. For the metabolic activity assay, 180 μl HEPES buffer (pH = 7.6) with 80 μM PQS, HHQ, HQNO, and nalidixic acid or 0.1% DMSO as the control condition, 10 μl of B. bacteriovorus culture (∼106 cells), and 10 μl of color reagent (WST solution: electron mediator reagent = 9:1) purchased from Kishida Chemical Co., Ltd. (Osaka, Japan) were mixed in a well of a 96-well plate. The plate was incubated at 30°C, and the microplate reader measured the aerobic conditions and the absorbance at 450 nm with time (every 12 h up to 48 h). Three independent experiments were carried out.
Quinolone Compound Extraction and Quantification
P. aeruginosa PA14 was cultured in LB medium with 0, 0.75, 1.5, and 3 mM CABA (solvent: DMSO, stock concentration: 1.5 M) by incubation at 37°C and 120 rpm for 10 h. The 5-ml culture was centrifuged at 13,000 rpm for 5 min and filtered using a 0.2-μm-pore-size filter. The supernatant (4 ml) was mixed with 4 ml ethyl acetate (Lépine et al., 2004; Lesic et al., 2007) to extract quinolone compounds. The organic solvent was further evaporated, and the dried fraction of quinolone compounds dissolved in 10 μl DMSO. The final DMSO solution was used to test the growth of B. bacteriovorus. Then, 2 μl of DMSO solution containing quinolone compounds or 100% DMSO as a control was put on the center of each plate with the cells of B. bacteriovorus and E. coli in the top agar as mentioned above. Then, these plates were incubated at 30°C for 3–4 days.
In accordance with Supplementary Tables 1, 2, LC-QTOF-MS was performed to quantify each quinolone compound in each fraction. Basically, the conditions and methods were followed as written by Kadokami and Ueno (2019). The ion transitions of each quinolone compound are shown in Supplementary Table 3. The calibrations of the concentrations prepared were 1, 5, 10, 50, 100, 500, and 1,000 ng/l of each chemical dissolved in methanol. Three independent experiments were carried out.
Isolation of Bdellovibrio-Like Bacteria From River Water
River water was collected from Onga River, Kitakyushu, Japan, and an aliquot of the river water (100 ml) was mixed with E. coli cells suspended with HEPES buffer at ∼2 of cell turbidity at OD600 (100 ml) to aggregate B. bacteriovorus from the river. The mixture was incubated at 30°C and 180 rpm for 2 days. The enriched culture was filtered by a 0.45-μm-pore-size filter, and the filtrate was used for the double-layer method as mentioned above to obtain a single plaque of Bdellovibrio-like bacteria. A piece of a top agar plate with the plaque was sliced and added to the E. coli cell suspension until the cell turbidity (OD600) decreased (for 3–4 days). A pure culture of a Bdellovibrio-like bacterium was obtained by repeating this step three times according to the method of Hobley et al. (2012). Three independent experiments were carried out.
Indole Production Assay
E. coli culture in LB medium was incubated at 30°C and 120 rpm, and the cell turbidity was measured at 600 nm after 24 h. The E. coli supernatant (0.5 ml) of the 24-h culture was reacted to 0.2 ml of Kovac’s reagent (mixed with 5 g of p-dimethylamino-benzaldehyde, 25 ml HCl, and 75 ml pentanol). The organic layer (0.1 ml), Kovac’s reagent, was mixed with 0.9 ml HCl-amyl alcohol mixture (30 ml HCl and 90 ml pentanol). The absorbance at 540 nm was measured using a V-530 spectrophotometer (JASCO Corporation, Tokyo, Japan) to determine the indole concentration (Kawamura-Sato et al., 1999). Three independent experiments were carried out.
Statistical Analysis
Compared with the control without any test reagent, each chemical sample was calculated using the means from at least duplicate data (n = 2 or 3). Graphics were created using Microsoft Office. According to the Shapiro–Wilk test, each data was normally distributed. Therefore, differences of some results were determined by one-way ANOVA, followed by Tukey’s test at a significance level of p < 0.05.
Results
Effect of P. aeruginosa on the Predation of E. coli by B. bacteriovorus
First, the effect of P. aeruginosa PA14 wild type on the predation of E. coli K-12 BW25113 by B. bacteriovorus was examined. Under regular conditions in the presence of E. coli and B. bacteriovorus, the number of E. coli cells decreased 1,000–10,000 times by the predation by B. bacteriovorus (Figure 1A). However, B. bacteriovorus could not prey E. coli cells in the presence of P. aeruginosa PA14 (Figure 1A). While the predatory activity of B. bacteriovorus was basically observed by checking a clear zone on the agar plate (Supplementary Figure 1A), the inhibition of predation by P. aeruginosa was seen on NB agar plates after incubation, as indicated in a result wherein E. coli cells grew in an area near to where the culture of P. aeruginosa PA14 was spotted on the agar plate (Supplementary Figure 1B). Without inoculating B. bacteriovorus cells, only the E. coli cells were grown on the agar plate (Supplementary Figure 1C): note that the reflection of the fluorescent lamp is more diffuse due to the turbidity of E. coli growth. Next, the involvement of P. aeruginosa QS in the predatory activity of B. bacteriovorus was investigated by using each QS mutant (lasI, rhlI, pqsA, or pqsH) since these genes are involved in the synthesis of each signal molecule. As shown in Figure 1B, whereas a large inhibitory zone was observed in the wild-type strain, lasI mutant, and rhlI mutant, the zone was reduced when pqsA or pqsH mutant was tested (Supplementary Figure 2, indicated in plate photos). The comparison clearly showed that the two mutants, pqsA and pqsH, do not have any inhibitory effect on the predation activity of E. coli by B. bacteriovorus (Figure 1B), whereas lasI and rhlI mutants showed almost the same predation inhibitory activity as the wild-type strain, PA14. Next, when the effect of a complementation assay with QS signal molecules, PQS and HHQ, on the predatory activity by B. bacteriovorus was investigated, the addition of PQS at the pqsH mutant or HHQ at the pqsA and pqsH mutants enhanced the inhibitory effect on the predation activity of B. bacteriovorus (Figure 2). In this experiment, the pqsA mutant strain was used as the strain that does not produce both PQS and HHQ, and the pqsH mutant strain was used as the strain that produces HHQ but not PQS. The recovery of predatory inhibitory activity in the pqsA and pqsH mutants was revealed by the size of the inhibition zone in the plate assays in the presence of PQS or HHQ (Supplementary Figure 3). Therefore, it was discovered that among the QS systems of P. aeruginosa, the pqs regulation system plays an essential role in inhibiting the predatory activity of B. bacteriovorus. HHQ rather than PQS is also more important to inhibit the predation activity of B. bacteriovorus because the inhibitory effect on the plate assays in the presence of HHQ was greater than that of PQS.
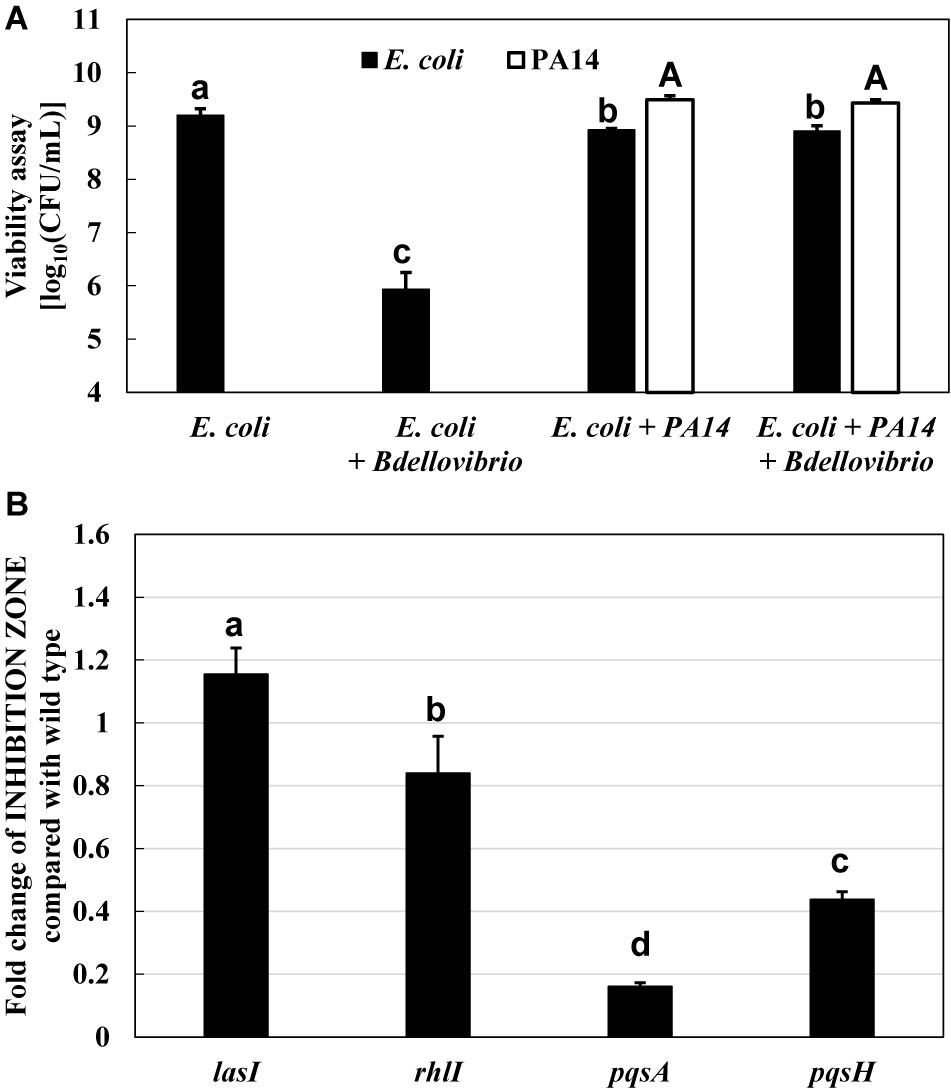
Figure 1. Inhibition of the predatory activity of Bdellovibrio bacteriovorus in the presence of Pseudomonas aeruginosa. (A) Dynamics of viable cells of Escherichia coli or/and P. aeruginosa PA14 with or without B. bacteriovorus 109J. Each cell suspension (initial cell turbidity was adjusted to be ∼2 at OD600) in HEPES buffer was incubated for 72 h at 30°C and 120 rpm under aerobic condition (n = 2). (B) Effect of P. aeruginosa quorum-sensing mutants (las, rhl, and pqs) on the predation plate assays using a mixture of B. bacteriovorus 109J and E. coli BW25113. In the plate assays using E. coli BW25113 as a prey cell, the area of the inhibition zone was compared in the presence of each P. aeruginosa strain (P. aeruginosa wild type, lasI mutant, rhlI mutant, pqsA mutant, or pqsH mutant), n = 3. The value among each sample with different letters is significantly different (p < 0.05).
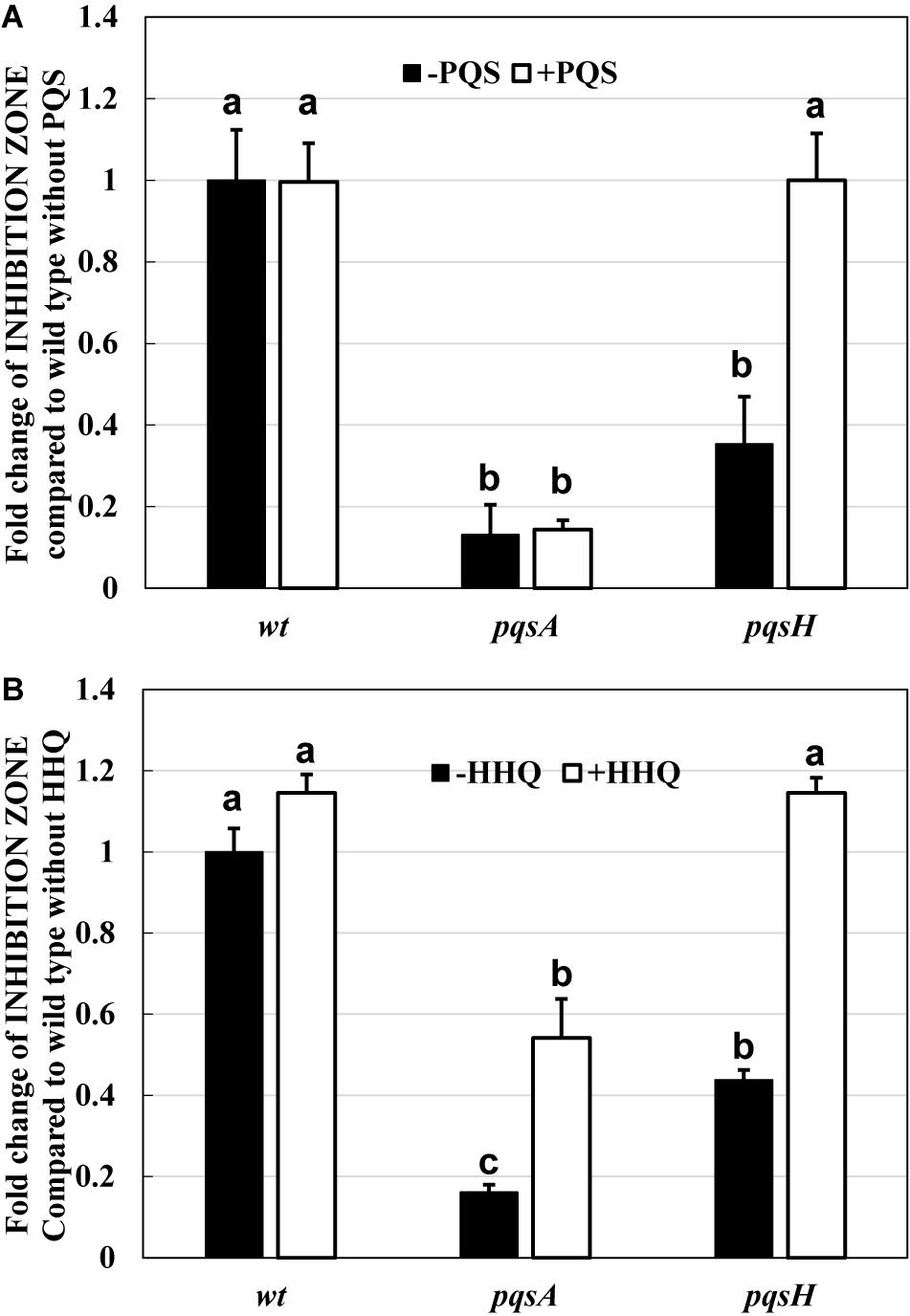
Figure 2. Restoration of the inhibitory effect by two quinolone compounds, PQS (A) and HHQ (B), on the predation plate assays using a mixture of Bdellovibrio bacteriovorus 109J and Escherichia coli BW25113 in the presence of Pseudomonas aeruginosa wild type, pqsA mutant, or pqsH mutant. Each PQS or HHQ standard solution (solvent: DMSO, concentration: 160 nmol) or DMSO solution (control) was placed on the assay plate along with the cell culture of P. aeruginosa wild type, pqsA mutant, or pqsH mutant. All the plates were incubated at 30°C for 4 days. PQS, Pseudomonas quinolone signal, 2-heptyl-3-hydroxy-4(1H)-quinolone; HHQ, 2-heptyl-4-quinolone. n = 3. The value among each sample with different letters is significantly different (p < 0.05).
Quinolone Signals Are Essential to the Predation Inhibitory Effect of B. bacteriovorus
The next question is whether the predation inhibitory activity to B. bacteriovorus is due to each signal molecule (PQS, HHQ, or HQNO) itself or the activation of the pqs regulation system by the exogenous addition of PQS, HHQ, or HQNO. First, the predatory activity of B. bacteriovorus was evaluated at a final amount of 8, 16, 32, 64, 128, and 160 nmol potential inhibitor by plate assays under the condition that only each pqs QS signal molecule (PQS, HHQ, or HQNO) was present without using P. aeruginosa culture. As a result, HHQ was the most effective compound in inhibiting the predatory activity of B. bacteriovorus (Figure 3 and Supplementary Figure 4). The inhibitory effect was even higher when tested at a final amount of 8, 16, 32, 64, 128, and 160 nmol. Moreover, 100% DMSO addition as the solvent control has no inhibition (Supplementary Figure 4). Additionally, the inhibitory effect of the PQS, HHQ, or HQNO was also evaluated at 4, 8, and 16 μM in assays using a liquid culture, by which the predation activity of B. bacteriovorus can be evaluated by reducing the turbidity mainly derived from E. coli cells. As shown in Figure 4, the predatory activity of B. bacteriovorus was inhibited according to the concentration of PQS, HHQ, or HQNO tested. The concentrations tested should be reasonable because a similar concentration of PQS, HHQ, and HQNO was detected from the culture of P. aeruginosa (Lesic et al., 2007). Particularly, HHQ and HQNO inhibited the activity of B. bacteriovorus compared to the control with DMSO. At higher concentrations (20, 40, 80, and 160 μM) of each quinolone compound, the inhibitory effect was also more clearly observed (Supplementary Figure 5), as shown in the result wherein complete inhibition of predation activity was found at high concentrations of HQNO (over 20 μM) and HHQ (over 40 μM). Moreover, since pyocyanin is known as a compound produced by the pqs QS system (Gallagher et al., 2002), the effect of pyocyanin on the predation activity was examined. Consequently, pyocyanin had no effect on the predation activity of B. bacteriovorus (Supplementary Figure 6). The results altogether indicate that PQS, HHQ, and HQNO produced by P. aeruginosa are essential compounds that inhibit the predation activity of B. bacteriovorus directly.
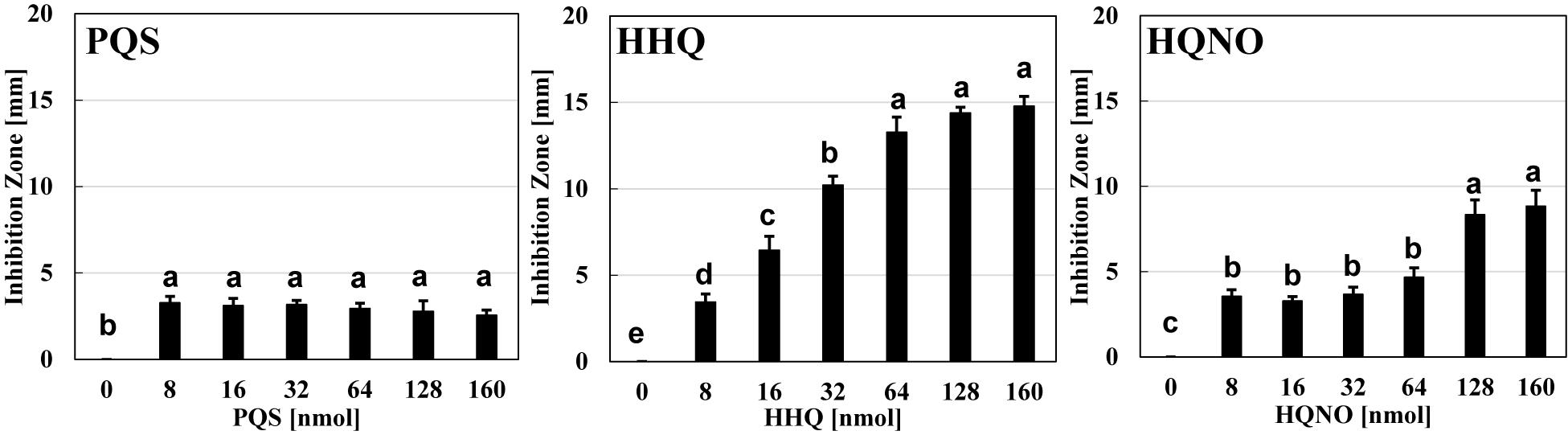
Figure 3. Effect of each quinolone compound itself on the predation plate assays using a mixture of Bdellovibrio bacteriovorus 109J and Escherichia coli BW25113. The inhibition zone for the predation plate assay using a mixture of B. bacteriovorus 109J and E. coli BW25113 was compared in the presence of PQS, HHQ, or HQNO compound (0, 8, 16, 32, 64, 128, or 160 nmol) placed on the plate after incubation at 30°C for 4 days. The 0-nmol sample is the control with DMSO alone. PQS, Pseudomonas quinolone signal, 2-heptyl-3-hydroxy-4(1H)-quinolone; HHQ, 2-heptyl-4-quinolone; HQNO, 2-heptyl-4-quinolinol 1-oxide. n = 3. The value among each sample with different letters is significantly different (p < 0.05).
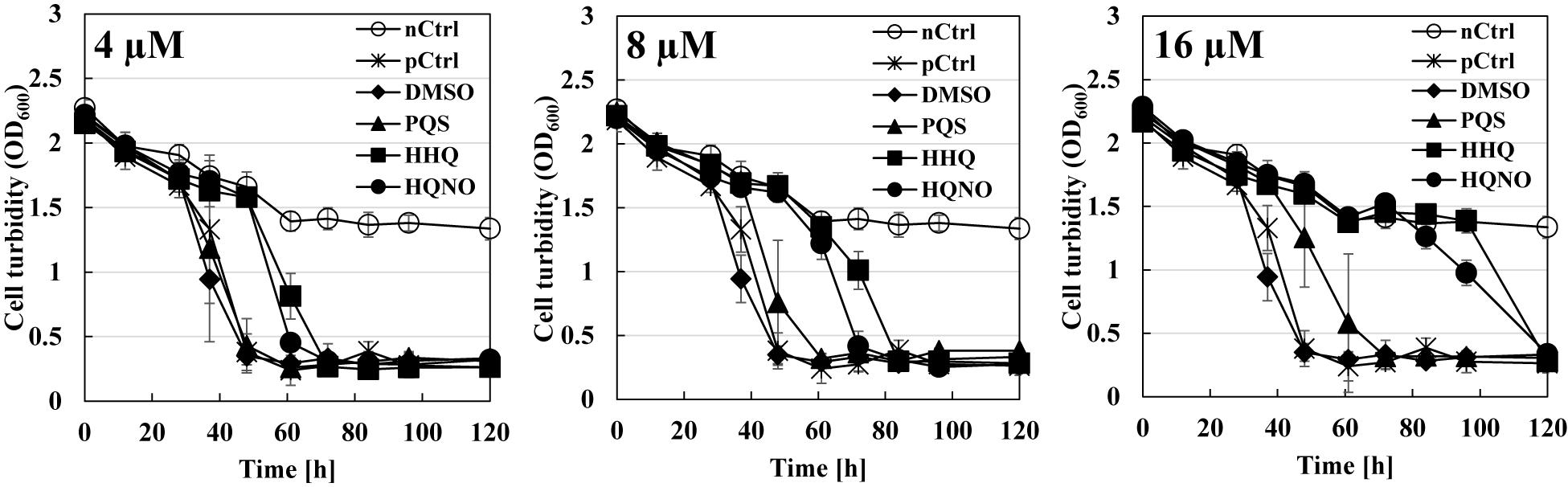
Figure 4. Effect of each quinolone compound itself on the predation liquid assays using a mixture of Bdellovibrio bacteriovorus 109J and Escherichia coli BW25113. Each cell turbidity of E. coli BW25113 (OD600 nm ∼ 2) was monitored with time after inoculating the cells of B. bacteriovorus under 4 μM (left), 8 μM (middle), or 16 μM (right) of each PQS/HHQ/HQNO compound or DMSO which was used as a solvent for the compounds (as the control). “nCtrl” means a sample consisting of only the E. coli BW25113 cells in HEPES buffer without any compound, whereas “pCtrl” means a mixture of E. coli cells and B. bacteriovorus cells in HEPES buffer without any compound. PQS, Pseudomonas quinolone signal, 2-heptyl-3-hydroxy-4(1H)-quinolone; HHQ, 2-heptyl-4-quinolone; HQNO, 2-heptyl-4-quinolinol 1-oxide. n = 3.
Effect of Quinolone Compounds on B. bacteriovorus Cells
The next point is how quinolone compounds act directly on B. bacteriovorus cells in the absence of prey E. coli cells. The viability of B. bacteriovorus cells in HEPES buffer was monitored for 6 days with or without the addition of 80 μM of each quinolone compound, including nalidixic acid, one of the quinolone compounds, as a positive control. As a result, the number of B. bacteriovorus cells drastically reduced in the presence of HHQ or HQNO (Figure 5A). These compounds may affect the electron mediators of B. bacteriovorus since HQNO kills cells by flow inhibition of electrons, such as cytochrome bc1 complex (Hazan et al., 2016). Corroborating evidence was obtained from the results using the WST-8 colorimetric assay kit to evaluate the metabolic activity of B. bacteriovorus under HEPES buffer with or without 80 μM of each quinolone signal. As shown in Figure 5B, the metabolic activity of B. bacteriovorus was also reduced in the presence of HHQ or HQNO. Hence, our results indicate that quinolone signal compounds produced by P. aeruginosa act directly to inactivate B. bacteriovorus cells.
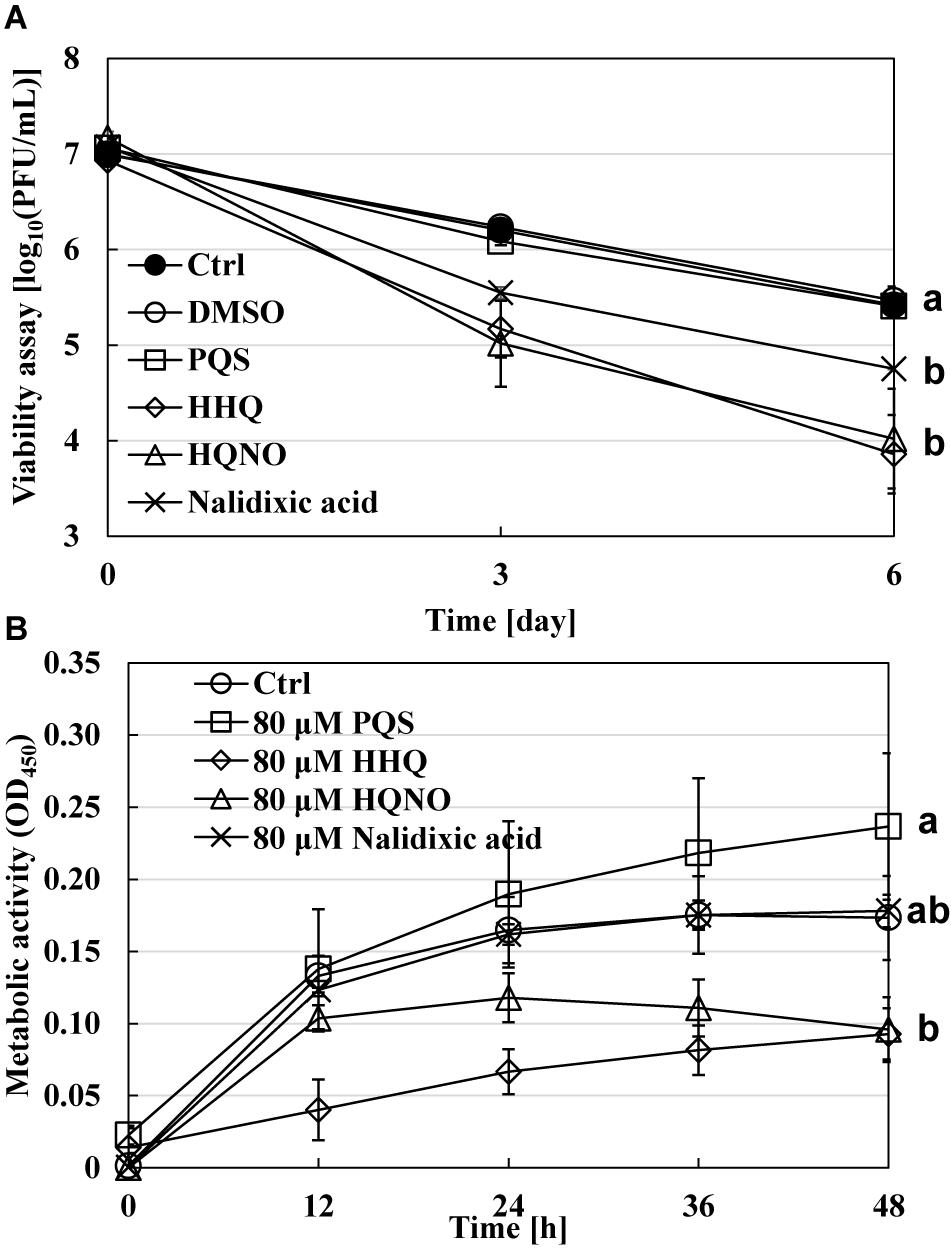
Figure 5. Effect of each quinolone compound itself on the viability of Bdellovibrio bacteriovorus cells. (A) The dynamics of viable cells of B. bacteriovorus in HEPES buffer with or without 80 μM of PQS/HHQ/HQNO/nalidixic acid. The incubation was conducted for 6 days at 30°C and 120 rpm under aerobic condition. n = 2. (B) The metabolic activity of B. bacteriovarus cells with or without 80 μM of PQS, HHQ, HQNO, or nalidixic acid for 48 h at 30°C left to stand in LB medium. “Ctrl” means a mixture of B. bacteriovorus cells and DMSO as a control solvent. PQS, Pseudomonas quinolone signal, 2-heptyl-3-hydroxy-4(1H)-quinolone; HHQ, 2-heptyl-4-quinolone; HQNO, 2-heptyl-4-quinolinol 1-oxide. n = 3. The value among each sample with different letters is significantly different (p < 0.05).
Additionally, it was reported that indole represses the predatory activity of B. bacteriovorus (Dwidar et al., 2015); it was tested whether E. coli can produce indole from several quinolone compounds derived from P. aeruginosa. Supplementary Figure 7 shows the indole production in E. coli in the presence of PQS, HHQ, or HQNO. As a result, there was no difference in the amount of indole produced with or without each quinolone compound. Therefore, it is fairly unlikely that the inhibition of the predatory activity of B. bacteriovorus may be caused by indole produced from quinolone compounds.
Relationship Between pqs Regulation System and Predation Activity of B. bacteriovorus
The relationship between the pqs regulation system and the predation activity of B. bacteriovorus was further examined using CABA, a pqs QS inhibitor (Lesic et al., 2007). Figure 6A shows the inhibitory effect of B. bacteriovorus at several concentrations (0.75, 1.5, and 3 mM CABA), at which the growth of P. aeruginosa was not inhibited (Supplementary Figure 8). The results clearly show that the predatory activity of B. bacteriovorus was not inhibited according to the increasing concentration of CABA. Additionally, the actual concentration of HHQ in each culture was verified by LC-QTOF-MS analyses (Figure 6C). As a result, lower concentrations of HHQ were detected from the cultures at higher concentrations of CABA. A similar tendency was observed for the PQS concentration but not for the HQNO one (Figures 6B,D). Because the concentration of HHQ is highly correlated with that of CABA compared to PQS and HQNO, the HHQ produced is a key factor to inhibit the predation activity of B. bacteriovorus.
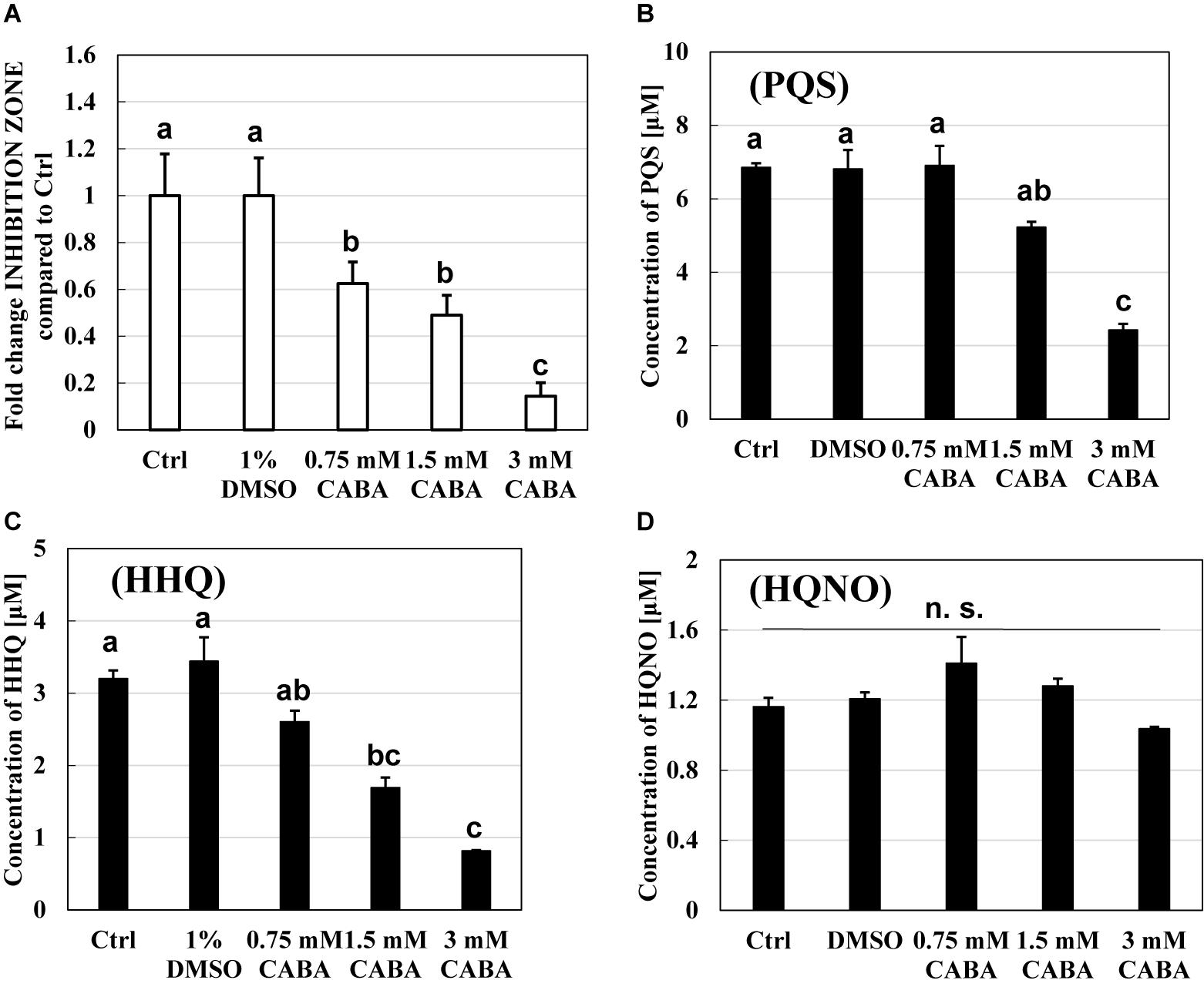
Figure 6. Effect of a pqs quorum-sensing inhibitor, 2-amino-6-chlorobenzoic acid (CABA), on the predation inhibition by Pseudomonas aeruginosa and the production of PQS, HHQ, or HQNO. (A) The reduction of predation inhibitory effect in the presence of CABA. The predation plate assays using a mixture of Bdellovibrio bacteriovorus 109J and Escherichia coli BW25113 were conducted at 30°C for 3 days in the presence of quinolone compounds extracted from the culture of P. aeruginosa wild type containing 0, 0.75, 1.5, or 3 mM of CABA. (B) PQS, (C) HHQ, and (D) HQNO concentration in the extracts of bacterial culture of P. aeruginosa PA14 after incubation at 37°C and 120 rpm for 10 h under LB medium with 0, 0.75, 1.5, or 3 mM of CABA. The concentration of HHQ extracted was determined by LC-QTOF-MS. Ctrl and 1% DMSO mean that each sample from the culture uses only LB medium or uses LB with 1% DMSO as solvent control. PQS, Pseudomonas quinolone signal, 2-heptyl-3-hydroxy-4(1H)-quinolone; HHQ, 2-heptyl-4-quinolone; HQNO, 2-heptyl-4-quinolinol 1-oxide. n = 3. The value among each sample with different letters is significantly different (p < 0.05). n = 3. n. s., not significant.
Effect of Quinolone Compounds on Other B. bacteriovorus Isolates
Another question is whether the inhibitory effect of B. bacteriovorus by the quinolone compounds occurs only in B. bacteriovorus strain 109J that has been used in this study. To answer the question, two strains of Bdellovibrio-like bacteria were isolated from a river sample and similarly evaluated for their effect on quinolone compounds. First, 16S ribosomal RNA gene homology analysis was conducted on these two strains, and it was discovered that both strains had 99% homology (TAE1: 99.36%, TAE2: 99.79%) with the B. bacteriovorus strain 109J complete sequence. Second, the two B. bacteriovorus strains were also inhibited in the presence of quinolone compounds (Figure 7). No inhibition zone is observed in the control condition with DMSO alone, as shown in Supplementary Figure 4. In both strains, HHQ showed the highest predation inhibitory effect. These results support the above-mentioned point that HHQ should be a key compound to inhibit the predation activity of B. bacteriovorus.
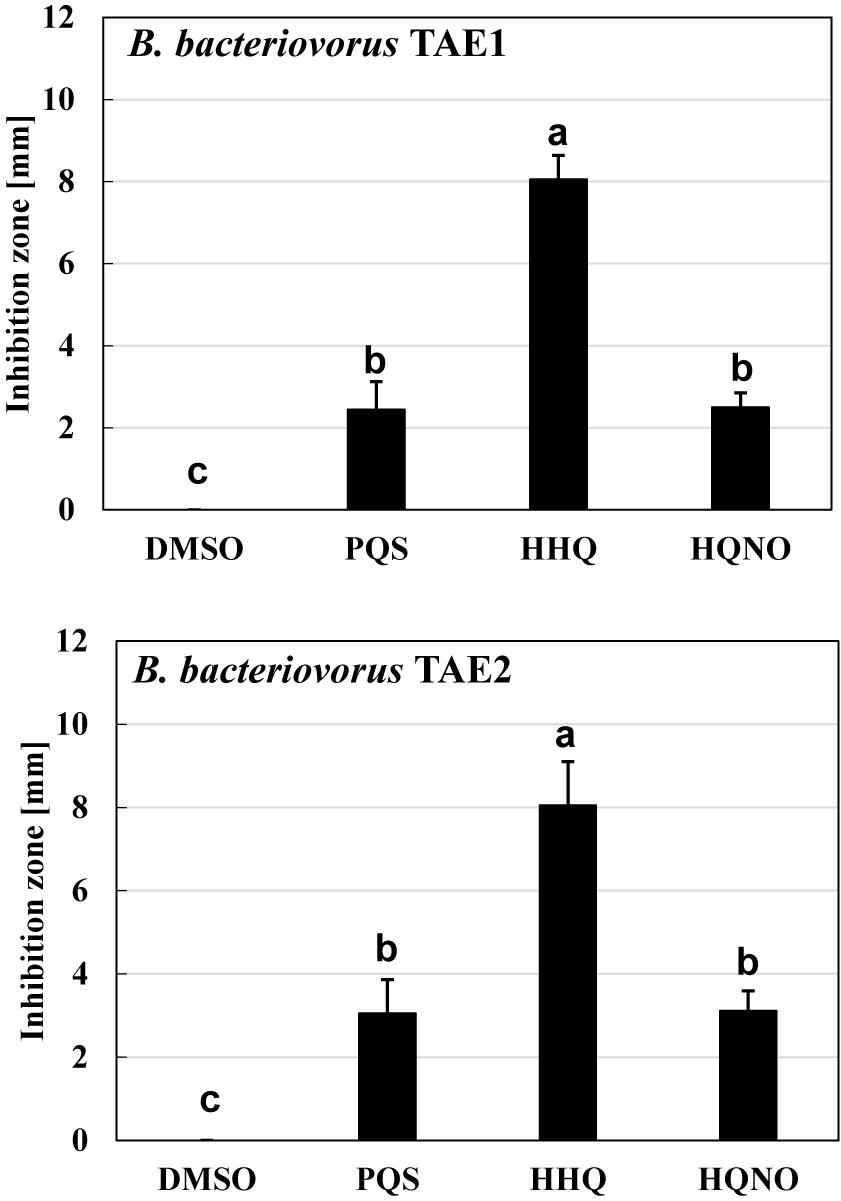
Figure 7. The effect of quinolone compounds on two environmental isolates of Bdellovibrio bacteriovorus. The predation plate assay was conducted using two isolates (TAE1 and TAE2) of B. bacteriovorus. The inhibition zone for the predation plate assay uses a mixture of each B. bacteriovorus isolate, and Escherichia coli BW25113 was compared in the presence of PQS, HHQ, or HQNO compound (16 nmol) placed on the plate after incubation at 30°C for 4 days. The DMSO sample is the solvent control with DMSO alone. PQS, Pseudomonas quinolone signal, 2-heptyl-3-hydroxy-4(1H)-quinolone; HHQ, 2-heptyl-4-quinolone; HQNO, 2-heptyl-4-quinolinol 1-oxide. n = 3. The value among each sample with different letters is significantly different (p < 0.05).
Effect of P. aeruginosa Mutant Strains That Do Not Produce Quinolone Compounds on the Predation by B. bacteriovorus
Considering the previous results, it might be thought that B. bacteriovorus can prey on P. aeruginosa mutant strains that do not produce quinolone compounds. However, P. aeruginosa (pqsA or pqsH mutant) with a small inhibition zone in Figure 1B was not preyed upon by B. bacteriovorus when P. aeruginosa cell suspension was added to B. bacteriovorus (Supplementary Figure 9). For P. aeruginosa to be preyed upon by B. bacteriovorus, it was considered that not only quinolone compounds but also other predation inhibitors had to be avoided.
Next, it was investigated whether it would be possible for B. bacteriovorus to prey upon E. coli in the presence of P. aeruginosa mutant strains, which do not produce quinolone compounds. As a result, B. bacteriovorus was unable to prey upon E. coli even in the presence of P. aeruginosa mutant strains (pqsA or pqsH) with a small inhibition zone, as shown in Figure 1B (Supplementary Figure 10).
These results suggest that quinolone compounds are not the only factor inhibiting the predatory activity of B. bacteriovorus but that there are other factors.
Discussion
The reason for the presence or absence of predatory activity against P. aeruginosa by B. bacteriovorus has still been a big mystery to date. P. aeruginosa, a Gram-negative bacterium like E. coli, has been reported to be preyed upon by B. bacteriovorus (Stolp and Starr, 1963; Shanks et al., 2013; Iebba et al., 2014; Pérez et al., 2016), while other researchers have reported such to be not preyed upon (Mukherjee et al., 2015). Since the different behavior regarding predatory activity was considered to be because of the barrier caused by P. aeruginosa rather than the property of B. bacteriovorus itself, we first investigated the relationship between each QS system derived from P. aeruginosa and the predatory activity of B. bacteirovorus in this study to narrow down the virulence factors produced by P. aeruginosa (Winson et al., 1995; Latifi et al., 1996; Pesci et al., 1997, 1999; McKnight et al., 2000; Gallagher et al., 2002; Kiratisin et al., 2002; Déziel et al., 2004; Xiao et al., 2006; Lee et al., 2013) against B. bacteriovorus. These results clearly show that quinolone compounds derived from P. aeruginosa negatively affect the predation of E. coli by B. bacteriovorus (Figures 1, 2, 3). For the complementary experiments shown in Figure 2, the pqsA mutant strain, which lacked the first step in the PQS synthesis pathway (Gallagher et al., 2002; Coleman et al., 2008), was not complemented by exogenous PQS since it could not produce HHQ, but the pqsH mutant strain, which lacked the conversion gene of HHQ to PQS at the last step in the PQS synthesis pathway (Gallagher et al., 2002; Déziel et al., 2004; Dubern and Diggle, 2008; Schertzer et al., 2009), was complemented by exogenous PQS since it could upregulate PQS synthesis (Xiao et al., 2006) and produce HHQ. However, it is considered to have been complemented in the pqsH mutant strain with exogenous HHQ since pqsA is upregulated by PqsR receiving HHQ (Rampioni et al., 2016). However, for the pqsA mutant strain, the size of the inhibition zone may be due to the exogenous HHQ itself, as shown in Figure 3, rather than complementation. Additionally, among the quinolone compounds, HHQ and HQNO directly inactivated the activity of B. bacteriovorus (Figures 4, 5). In general, similar phenomena likely occur in nature since P. aeruginosa produces 4–40 μM PQS, 0.5–4 μM HHQ, and ∼75 μM HQNO in a pure culture system (Lépine et al., 2004; Lesic et al., 2007; Ji et al., 2016). As the inhibitory mechanism, it inhibits the activity of electron mediators such as cytochrome bc1 complex (Hazan et al., 2016). However, HHQ exhibits bacteriostatic activity in a species-specific manner (Reen et al., 2011), but the detailed mechanism is still unknown. Figure 4 and Supplementary Figure 6 suggest that HHQ (over 40 μM) and HQNO (over 20 μM) are inhibited, but HHQ is as effective as or more effective than HQNO at lower concentrations (4–16 μM). Similarly, in the plate experiment (Figure 3), only HHQ showed a more significant inhibition zone than PQS at a low number of compounds (16–32 nmol), while not only HHQ but also HQNO showed a larger inhibition zone than PQS at high amounts of compounds (over 64 nmol). Considering the solubility of each compound in liquid conditions and its diffusion on the plate, the inhibitory mechanism of each quinolone compound against B. bacteriovorus needs to be clarified in the future. The effects of HHQ and HQNO are almost the same; however, the concentration of HHQ produced is higher than that of HQNO in the actual culture of P. aeruginosa (Figure 6A). Additionally, the predatory activity of B. bacteriovorus was restored in the presence of a pqs regulation inhibitor (Lesic et al., 2007), CABA (Figures 6B,D). Thus, the inhibition of predatory activity by HHQ was clarified as a barrier mechanism of P. aeruginosa against B. bacteriovorus. Since HHQ, which had the highest inhibition in the plate assay, was the focus of this study, instrumental analysis was conducted using the P. aeruginosa culture after about 10 h. Therefore, it looks like only the HHQ concentration correlated with the size of the inhibition zone. This may also correlate with the degree of inhibition of HQNO when P. aeruginosa is incubated for a longer time (data not shown). Since these pqs QS signals produced by P. aeruginosa are known to act as not only the regulation of gene expression but also an antibiotic for some bacterial species (Reen et al., 2011; Szamosvári et al., 2019), the predation activity assay of B. bacteriovorus strains tested may be inactivated as shown in Figure 7.
Although the pqs regulation system of P. aeruginosa is one of the defense mechanisms against B. bacteriovorus, this predator was unable to prey on pqs mutants that could not produce quinolone compounds such as HHQ (Supplementary Figure 9). The results show that P. aeruginosa may have another mechanism that evades the attack of B. bacteriovorus. In the predation evaluation by plate assays, the inhibition zone of pqs mutants was small, suggesting a different inhibitory mechanism other than extracellular secretory substances. Further research is needed to elucidate the mechanism of the presence or absence of predatory activity of B. bacteriovorus against P. aeruginosa.
So far, it has been reported that indole, which is one of the QS molecules, and cyanide inhibits the activity of B. bacteriovorus (Dwidar et al., 2015; Mun et al., 2017); however, there is no knowledge about its relationship with other QS compounds. Other than that, Varon and Shilo (1981) reported that the predation of B. bacteriovorus was inhibited by sodium dodecyl sulfate (SDS). Surfactants, not limited to artificially added SDS, are produced by various microorganisms such as bacteria and yeast. In Supplementary Figures 9, 10, in the presence of the quinolone compound non-producing P. aeruginosa strain, B. bacteriovorus could not prey upon not only P. aeruginosa but also E. coli. Thus, the strategy should not be to avoid only one of the extracellular inhibitors to allow B. bacteriovorus to prey upon P. aeruginosa. As a summary of this research, our results showed that quinolone signals related to the QS of P. aeruginosa, especially HHQ and HQNO, inhibit the predation activity of B. bacteriovorus. Among the quinolone compounds, HHQ was the most effective quinolone compound against B. bacteriovorus. It was discovered that quinolone compounds such as HHQ act as bactericidal on B. bacteriovorus, unlike indole that suppresses viability without killing it. As shown in the results of this research, the accumulation of knowledge to understand the relationship between predator bacteria and other bacteria is indispensable for the application and usage of B. bacteriovorus in the future.
Data Availability Statement
The raw data supporting the conclusions of this article will be made available by the authors, without undue reservation.
Author Contributions
YH, YN, TMo, and KK performed the experiments. TMa, KK, and RG-C supervised the project. YH initially wrote the manuscript. TMa revised the manuscript. All authors designed the study, discussed the results, contributed to the article, and approved the submitted version.
Funding
This study was supported by a research fund of Kitakyushu City.
Conflict of Interest
The authors declare that the research was conducted in the absence of any commercial or financial relationships that could be construed as a potential conflict of interest.
Publisher’s Note
All claims expressed in this article are solely those of the authors and do not necessarily represent those of their affiliated organizations, or those of the publisher, the editors and the reviewers. Any product that may be evaluated in this article, or claim that may be made by its manufacturer, is not guaranteed or endorsed by the publisher.
Acknowledgments
We wish to thank Prof. Thomas K. Wood (Pennsylvania State University, United States) for providing all mutant gene strains of P. aeruginosa and E. coli. We would also like to thank Enago (www.enago.jp) for the English language review.
Supplementary Material
The Supplementary Material for this article can be found online at: https://www.frontiersin.org/articles/10.3389/fmicb.2021.722579/full#supplementary-material
References
Baba, T., Ara, T., Hasegawa, M., Takai, Y., Okumura, Y., Baba, M., et al. (2006). Construction of Escherichia coli K-12 in-frame, single-gene knockout mutants: the Keio collection. Mol. Syst. Biol. 2. doi: 10.1038/msb4100050
Burgess, J. G., Jordan, E. M., Bregu, M., Mearns-Spragg, A., and Boyd, K. G. (1999). Microbial antagonism: a neglected avenue of natural products research. J. Biotechnol. 70, 27–32.
Chen, H., Brinkac, L. M., Mishra, P., Li, N., Lymperopoulou, D. S., Dickerson, T. L., et al. (2015). Draft genome sequences for the obligate bacterial predators Bacteriovorax spp. of four phylogenetic clusters. Stand. Genomic Sci. 10:11. doi: 10.1186/1944-3277-10-11
Coleman, J. P., Hudson, L. L., McKnight, S. L., Farrow, J. M., Calfee, M. W., Lindsey, C. A., et al. (2008). Pseudomonas aeruginosa PqsA is an anthranilate-coenzyme A ligase. J. Bacteriol. 190, 1247–1255. doi: 10.1128/JB.01140-07
Dashiff, A., Junka, R. A., Libera, M., and Kadouri, D. E. (2011). Predation of human pathogens by the predatory bacteria Micavibrio aeruginosavorus and Bdellovibrio bacteriovorus. J. Appl. Microbiol. 110, 431–444. doi: 10.1111/j.1365-2672.2010.04900.x
Datsenko, K. A., and Wanner, B. L. (2000). One-step inactivation of chromosomal genes in Escherichia coli K-12 using PCR products. Proc. Natl. Acad. Sci. U.S.A. 97, 6640–6645. doi: 10.1073/pnas.120163297
de Almeida, F. A., Carneiro, D. G., de Oliveira Mendes, T. A., Barros, E., Pinto, U. M., de Oliveira, L. L., et al. (2018). N-dodecanoyl-homoserine lactone influences the levels of thiol and proteins related to oxidation-reduction process in Salmonella. PLoS One 13:e0204673. doi: 10.1371/journal.pone.0204673
Déziel, E., Lépine, F., Milot, S., He, J., Mindrinos, M. N., Tompkins, R. G., et al. (2004). Analysis of Pseudomonas aeruginosa 4-hydroxy-2-alkylquinolines (HAQs) reveals a role for 4-hydroxy-2-heptylquinoline in cell-to-cell communication. Proc. Natl. Acad. Sci. U.S.A. 101, 1339–1344. doi: 10.1073/pnas.0307694100
Dubern, J. F., and Diggle, S. P. (2008). Quorum sensing by 2-alkyl-4-quinolones in Pseudomonas aeruginosa and other bacterial species. Mol. Biosyst. 4, 882–888. doi: 10.1039/b803796p
Dubuis, C., Keel, C., and Haas, D. (2007). Dialogues of root-colonizing biocontrol peudomonads. Eur. J. Plant Pathol. 119, 311–328. doi: 10.1007/s10658-007-9157-1
Dwidar, M., Nam, D., and Mitchell, R. J. (2015). Indole negatively impacts predation by Bdellovibrio bacteriovorus and its release from the bdelloplast. Environ. Microbiol. 17, 1009–1022. doi: 10.1111/1462-2920.12463
Essar, D. W., Eberly, L., Hadero, A., and Crawford, I. P. (1990). Identification and characterization of genes for a second anthranilate synthase in Pseudomonas aeruginosa: interchangeability of the two anthranilate synthase and evolutionary implications. J. Bacteriol. 172, 884–900. doi: 10.1128/jb.172.2.884-900.1990
Evans, K. J., Lambert, C., and Sockett, R. E. (2007). Predation by Bdellovibrio bacteriovorus HD100 requires type IV pili. J. Bacteriol. 189, 4850–4859. doi: 10.1128/JB.01942-06
Feng, S., Tan, C. H., Cohen, Y., and Rice, S. A. (2016). Isolation of Bdellovibrio bacteriovorus from a tropical wastewater treatment plant and predation of mixed species biofilms assembled by the native community members. Environ. Microbiol. 18, 3923–3931. doi: 10.1111/1462-2920.13384
Fratamico, P. M., and Cooke, P. H. (1996). Isolation of Bdellovibrios that prey on Escherichia coli O157:H7 and Salmonella species and application for removal of prey from stainless steel surfaces. J. Food Saf. 16, 161–173. doi: 10.1111/j.1745-4565.1996.tb00157.x
Fuqua, C., Parsek, M. R., and Greenberg, E. P. (2001). Regulation of gene expression by cell-to-cell communication: acyl-homoserine lactone quorum sensing. Annu. Rev. Genet. 35, 439–468. doi: 10.1146/annurev.genet.35.102401.090913
Fuqua, W. C., Winans, S. C., and Greenberg, E. P. (1994). Quorum sensing in bacteria: the LuxR-LuxI family of cell density- responsive transcriptional regulators. J. Bacteriol. 176, 269–275. doi: 10.1128/jb.176.2.269-275.1994
Gallagher, L. A., McKnight, S. L., Kuznetsova, M. S., Pesci, E. C., and Manoil, C. (2002). Functions required for extracellular quinolone signaling by Pseudomonas aeruginosa. J. Bacteriol. 184, 6472–6480. doi: 10.1128/JB.184.23.6472-6480.2002
Galloway, W. R. J. D., Hodgkinson, J. T., Bowden, S. D., Welch, M., and Spring, D. R. (2011). Quorum sensing in Gram-negative bacteria: small-molecule modulation of AHL and AI-2 quorum sensing pathways. Chem. Rev. 111, 28–67. doi: 10.1021/cr100109t
Hazan, R., Que, Y. A., Maura, D., Strobel, B., Majcherczyk, P. A., Hopper, L. R., et al. (2016). Auto poisoning of the respiratory chain by a quorum-sensing-regulated molecule favors biofilm formation and antibiotic tolerance. Curr. Biol. 26, 195–206. doi: 10.1016/j.cub.2015.11.056
Hobley, L., Lerner, T. R., Williams, L. E., Lambert, C., Till, R., Milner, D. S., et al. (2012). Genome analysis of a simultaneously predatory and prey-independent, novel Bdellovibrio bacteriovorus from the River Tiber, supports in silico predictions of both ancient and recent lateral gene transfer from diverse bacteria. BMC Genomics 13:670. doi: 10.1186/1471-2164-13-670
Huang, J., Shi, Y., Zeng, G., Gu, Y., Chen, G., Shi, L., et al. (2016). Acyl-homoserine lactone-based quorum sensing and quorum quenching hold promise to determine the performance of biological wastewater treatments: an overview. Chemosphere 157, 137–151. doi: 10.1016/j.chemosphere.2016.05.032
Iebba, V., Totino, V., Santangelo, F., Gagliardi, A., Ciotoli, L., Virga, A., et al. (2014). Bdellovibrio bacteriovorus directly attacks Pseudomonas aeruginosa and Staphylococcus aureus cystic fibrosis isolates. Front. Microbiol. 5:280. doi: 10.3389/fmicb.2014.00280
Im, H., Dwidar, M., and Mitchell, R. J. (2018). Bdellovibrio bacteriovorus HD100, a predator of Gram-negative bacteria, benefits energetically from Staphylococcus aureus biofilms without predation. ISME J. 12, 2090–2095. doi: 10.1038/s41396-018-0154-5
Ji, C., Sharma, I., Pratihar, D., Hudson, L. L., Maura, D., Guney, T., et al. (2016). Designed small-molecule inhibitors of the anthranilyl-CoA synthetase PqsA block quinolone biosynthesis in Pseudomonas aeruginosa. ACS Chem. Biol. 11, 3061–3067. doi: 10.1021/acschembio.6b00575
Johansen, P., and Jespersen, L. (2017). Impact of quorum sensing on the quality of fermented foods. Curr. Opin. Food Sci. 13, 16–25. doi: 10.1016/j.cofs.2017.01.001
Jurkevitch, E., Minz, D., Ramati, B., and Barel, G. (2000). Prey range characterization, ribotyping, and diversity of soil and rhizosphere Bdellovibrio spp. isolated on phytopathogenic bacteria. Appl. Environ. Microbiol. 66, 2365–2371. doi: 10.1128/AEM.66.6.2365-2371.2000
Kadokami, K., and Ueno, D. (2019). Comprehensive Target Analysis for 484 Organic Micropollutants in Environmental Waters by the Combination of Tandem Solid-Phase Extraction and Quadrupole Time-of-Flight Mass Spectrometry with Sequential Window Acquisition of All Theoretical Fragment-Ion Spectra Acquisition. Anal. Chem. 91, 7749–7755. doi: 10.1021/acs.analchem.9b01141
Kadouri, D., and O’Toole, G. A. (2005). Susceptibility of biofilms to Bdellovibrio bacteriovorus attack. Appl. Environ. Microbiol. 71, 4044–4051. doi: 10.1128/AEM.71.7.4044-4051.2005
Karlin, D. A., Mastromarino, A. J., Jones, R. D., Stroehlein, J. R., and Lorentz, O. (1985). Fecal skatole and indole and breath methane and hydrogen in patients with large bowel polyps or cancer. J. Cancer Res. Clin. Oncol. 109, 135–141. doi: 10.1007/BF00391888
Kawamura-Sato, K., Shibayama, K., Horii, T., Iimuma, Y., Arakawa, Y., and Ohta, M. (1999). Role of multiple efflux pumps in Escherichia coli in indole expulsion. FEMS Microbiol. Lett. 179, 345–352. doi: 10.1111/j.1574-6968.1999.tb08748.x
Kiratisin, P., Tucker, K. D., and Passador, L. (2002). LasR, a transcriptional activator of Pseudomonas aeruginosa virulence genes, functions as a multimer. J. Bacteriol. 184, 4912–4919. doi: 10.1128/JB.184.17.4912-4919.2002
Koval, S. F., Hynes, S. H., Flannagan, R. S., Pasternak, Z., Davidov, Y., and Jurkevitch, E. (2013). Bdellovibrio exovorus sp. nov., a novel predator of Caulobacter crescentus. Int. J. Syst. Evol. Microbiol. 63, 146–151. doi: 10.1099/ijs.0.039701-0
Kuru, E., Lambert, C., Rittichier, J., Till, R., Ducret, A., Derouaux, A., et al. (2017). Fluorescent D-amino-acids reveal bi-cellular cell wall modifications important for Bdellovibrio bacteriovorus predation. Nat. Microbiol. 2, 1648–1657. doi: 10.1038/s41564-017-0029-y
Latifi, A., Foglino, M., Tanaka, K., Williams, P., and Lazdunski, A. (1996). A hierarchical quorum-sensing cascade in Pseudomonas aeruginosa links the transcriptional activators LasR and RhIR (VsmR) to expression of the stationary-phase sigma factor RpoS. Mol. Microbiol. 21, 1137–1146. doi: 10.1046/j.1365-2958.1996.00063.x
Lee, J., Wu, J., Deng, Y., Wang, J., Wang, C., Wang, J., et al. (2013). A cell-cell communication signal integrates quorum sensing and stress response. Nat. Chem. Biol. 9, 339–343. doi: 10.1038/nchembio.1225
Lee, J. H., Wood, T. K., and Lee, J. (2015). Roles of indole as an interspecies and interkingdom signaling molecule. Trends Microbiol. 23, 707–718. doi: 10.1016/j.tim.2015.08.001
Lépine, F., Milot, S., Déziel, E., He, J., and Rahme, L. G. (2004). Electrospray/mass spectrometric identification and analysis of 4-hydroxy-2-alkylquinolines (HAQs) produced by Pseudomonas aeruginosa. J. Am. Soc. Mass Spectrom. 15, 862–869. doi: 10.1016/j.jasms.2004.02.012
Lerner, T. R., Lovering, A. L., Bui, N. K., Uchida, K., Aizawa, S. I., Vollmer, W., et al. (2012). Specialized peptidoglycan hydrolases sculpt the intra-bacterial niche of predatory Bdellovibrio and increase population fitness. PLoS Pathog. 8:e1002524. doi: 10.1371/journal.ppat.1002524
Lesic, B., Lépine, F., Déziel, E., Zhang, J., Zhang, Q., Padfield, K., et al. (2007). Inhibitors of pathogen intercellular signals as selective anti-infective compounds. PLoS Pathog. 3:e0030126. doi: 10.1371/journal.ppat.0030126
Liberati, N. T., Urbach, J. M., Miyata, S., Lee, D. G., Drenkard, E., Wu, G., et al. (2006). An ordered, nonredundant library of Pseudomonas aeruginosa strain PA14 transposon insertion mutants. Proc. Natl. Acad. Sci. U.S.A. 103, 2833–2838. doi: 10.1073/pnas.0511100103
Liu, Y. C., Chan, K. G., and Chang, C. Y. (2015). Modulation of host biology by Pseudomonas aeruginosa quorum sensing signal molecules: messengers or traitors. Front. Microbiol. 6:1226. doi: 10.3389/fmicb.2015.01226
Mahmoud, K. K., and Koval, S. F. (2010). Characterization of type IV pili in the life cycle of the predator bacterium Bdellovibrio. Microbiology 156, 1040–1051. doi: 10.1099/mic.0.036137-0
McKnight, S. L., Iglewski, B. H., and Pesci, E. C. (2000). The Pseudomonas quinolone signal regulates rhl quorum sensing in Pseudomonas aeruginosa. J. Bacteriol. 182, 2702–2708. doi: 10.1128/JB.182.10.2702-2708.2000
Miller, M. B., and Bassler, B. L. (2001). Quorum sensing in bacteria. Annu. Rev. Microbiol. 55, 165–199. doi: 10.1146/annurev.micro.55.1.165
Mukherjee, S., Brothers, K. M., Shanks, R. M. Q., and Kadouri, D. E. (2015). Visualizing Bdellovibrio bacteriovorus by using the tdTomato fluorescent protein. Appl. Environ. Microbiol. 82, 1653–1661. doi: 10.1128/AEM.03611-15
Mun, W., Kwon, H., Im, H., Choi, S. Y., Monnappa, A. K., and Mitchell, R. J. (2017). Cyanide production by chromobacterium piscinae shields it from Bdellovibrio bacteriovorus HD100 predation. MBio. doi: 10.1128/mBio.01370-17
O’Malley, Y. Q., Reszka, K. J., Spitz, D. R., Denning, G. M., and Britigan, B. E. (2004). Pseudomonas aeruginosa pyocyanin directly oxidizes glutathione and decreases its levels in airway epithelial cells. Am. J. Physiol. Lung Cell. Mol. Physiol. 287, L94–L103.
Page, J. A., Lubbers, B., Maher, J., Ritsch, L., and Gragg, S. E. (2015). Investigation into the efficacy of Bdellovibrio bacteriovorus as a novel preharvest intervention to control Escherichia coli O157:H7 and Salmonella in cattle using an in vitro model. J. Food Prot. 78, 1745–1749. doi: 10.4315/0362-028X.JFP-15-016
Pérez, J., Moraleda-Muñoz, A., Marcos-Torres, F. J., and Muñoz-Dorado, J. (2016). Bacterial predation: 75 years and counting! Environ. Microbiol. 18, 766–779. doi: 10.1111/1462-2920.13171
Pesci, E. C., Milbank, J. B. J., Pearson, J. P., Mcknight, S., Kende, A. S., Greenberg, E. P., et al. (1999). Quinolone signaling in the cell-to-cell communication system of Pseudomonas aeruginosa. Proc. Natl. Acad. Sci. U.S.A. 96, 11229–11234. doi: 10.1073/pnas.96.20.11229
Pesci, E. C., Pearson, J. P., Seed, P. C., and Iglewski, B. H. (1997). Regulation of las and rhl quorum sensing in Pseudomonas aeruginosa. J. Bacteriol. 179, 3127–3132. doi: 10.1128/jb.179.10.3127-3132.1997
Pineiro, S. A., Stine, O. C., Chauhan, A., Steyert, S. R., Smith, R., and Williams, H. N. (2007). Global survey of diversity among environmental saltwater Bacteriovoracaceae. Environ. Microbiol. 9, 2441–2450. doi: 10.1111/j.1462-2920.2007.01362.x
Rampioni, G., Falcone, M., Heeb, S., Frangipani, E., Fletcher, M. P., Dubern, J. F., et al. (2016). Unravelling the genome-wide contributions of Specific 2-Alkyl-4-Quinolones and PqsE to quorum sensing in Pseudomonas aeruginosa. PLoS Pathog. 12:e1006029. doi: 10.1371/journal.ppat.1006029
Reen, F. J., Mooij, M. J., Holcombe, L. J., Mcsweeney, C. M., Mcglacken, G. P., Morrissey, J. P., et al. (2011). The Pseudomonas quinolone signal (PQS), and its precursor HHQ, modulate interspecies and interkingdom behaviour. FEMS Microbiol. Ecol. 77, 413–428. doi: 10.1111/j.1574-6941.2011.01121.x
Sambrook, J., Fritsch, E. F., and Maniatis, T. (1989). Molecular Cloning: A Laboratory Manual. Cold Spring Harbor, NY: Cold Spring Harbor Laboratory Press.
Schertzer, J. W., Boulette, M. L., and Whiteley, M. (2009). More than a signal: non-signaling properties of quorum sensing molecules. Trends Microbiol. 17, 189–195. doi: 10.1016/j.tim.2009.02.001
Semblante, G. U., Phan, H. V., Hai, F. I., Xu, Z. Q., Price, W. E., and Nghiem, L. D. (2017). The role of microbial diversity and composition in minimizing sludge production in the oxic-settling-anoxic process. Sci. Total Environ. 60, 558–567. doi: 10.1016/j.scitotenv.2017.06.253
Shanks, R. M. Q., Davra, V. R., Romanowski, E. G., Brothers, K. M., Stella, N. A., Godboley, D., et al. (2013). An eye to a kill: using predatory bacteria to control Gram-negative pathogens associated with ocular infections. PLoS One 8:e66723. doi: 10.1371/journal.pone.0066723
Shatzkes, K., Chae, R., Tang, C., Ramirez, G. C., Mukherjee, S., Tsenova, L., et al. (2015). Examining the safety of respiratory and intravenous inoculation of Bdellovibrio bacteriovorus and Micavibrio aeruginosavorus in a mouse model. Sci. Rep. 5:12899. doi: 10.1038/srep12899
Sockett, R. E., and Lambert, C. (2004). Bdellovibrio as therapeutic agents: A predatory renaissance? Nat. Rev. Microbiol. 2, 669–675. doi: 10.1038/nrmicro959
Stolp, H., and Starr, M. P. (1963). Bdellovibrio bacteriovorus gen. et sp. n., a predatory, ectoparasitic, and bacteriolytic microorganism. Antonie Van Leeuwenhoek 29, 217–248. doi: 10.1007/BF02046064
Szamosvári, D., Schuhmacher, T., Hauck, C. R., and Böttcher, T. (2019). A thiochromenone antibiotic derived from the Pseudomonas quinolone signal selectively targets the Gram-negative pathogen Moraxella catarrhalis. Chem. Sci. 10, 6624–6628. doi: 10.1039/c9sc01090d
Tang, B. L., Yang, J., Chen, X. L., Wang, P., Zhao, H. L., Su, H. N., et al. (2020). A predator–prey interaction between a marine Pseudoalteromonas sp. and Gram-positive bacteria. Nat. Commun. 11:285. doi: 10.1038/s41467-019-14133-x
Thomashow, M. F., and Rittenberg, S. C. (1978a). Intraperiplasmic growth of Bdellovibrio bacteriovorus 109J: attachment of long-chain fatty acids to Escherichia coli peptidoglycan. J. Bacteriol. 135, 1015–1023. doi: 10.1128/jb.135.3.1015-1023.1978
Thomashow, M. F., and Rittenberg, S. C. (1978b). Intraperiplasmic growth of Bdellovibrio bacteriovorus 109J: solubilization of Escherichia coli peptidoglycan. J. Bacteriol. 135, 998–1007. doi: 10.1128/jb.135.3.998-1007.1978
Varon, M., and Shilo, M. (1981). Inhibition of the predatory activity of Bdellovibrio by various environmental pollutants. Microbial. Ecology. doi: 10.1007/BF0203249
Wang, D., Ding, X., and Rather, P. N. (2001). Indole can act as an extracellular signal in Escherichia coli. J. Bacteriol. 183, 4210–4216. doi: 10.1128/JB.183.14.4210-4216.2001
Winson, M. K., Camarat, M., Latifi, A., Foglino, M., Chhabrat, S. R., Daykin, M., et al. (1995). Multiple N-acyl-L-homoserine lactone signal molecules regulate production of virulence determinants and secondary metabolites in Pseudomonas aeruginosa. Proc. Natl. Acad. Sci. U.S.A. 92, 9427–9431.
Withers, H., Swift, S., and Williams, P. (2001). Quorum sensing as an integral component of gene regulatory networks in gram-negative bacteria. Curr. Opin. Microbiol. 4, 186–193. doi: 10.1016/S1369-5274(00)00187-9
Xiao, G., Déziel, E., He, J., Lépine, F., Lesic, B., Castonguay, M. H., et al. (2006). MvfR, a key Pseudomonas aeruginosa pathogenicity LTTR-class regulatory protein, has dual ligands. Mol. Microbiol. 62, 1689–1699. doi: 10.1111/j.1365-2958.2006.05462.x
Keywords: inhibition factors, Escherichia coli, quorum sensing, Pseudomonas quinolone signal, Pseudomonas aeruginosa, Bdellovibrio bacteriovorus, predatory bacteria
Citation: Hoshiko Y, Nishiyama Y, Moriya T, Kadokami K, López-Jácome LE, Hirano R, García-Contreras R and Maeda T (2021) Quinolone Signals Related to Pseudomonas Quinolone Signal-Quorum Sensing Inhibits the Predatory Activity of Bdellovibrio bacteriovorus. Front. Microbiol. 12:722579. doi: 10.3389/fmicb.2021.722579
Received: 09 June 2021; Accepted: 12 August 2021;
Published: 10 September 2021.
Edited by:
Malka Halpern, University of Haifa, IsraelReviewed by:
Manuel Alcalde-Rico, Pontificia Universidad Católica de Valparaíso, ChileJose L. Martinez, Consejo Superior de Investigaciones Científicas (CSIC), Spain
Carey Lambert, University of Nottingham, United Kingdom
Copyright © 2021 Hoshiko, Nishiyama, Moriya, Kadokami, López-Jácome, Hirano, García-Contreras and Maeda. This is an open-access article distributed under the terms of the Creative Commons Attribution License (CC BY). The use, distribution or reproduction in other forums is permitted, provided the original author(s) and the copyright owner(s) are credited and that the original publication in this journal is cited, in accordance with accepted academic practice. No use, distribution or reproduction is permitted which does not comply with these terms.
*Correspondence: Toshinari Maeda, toshi.maeda@life.kyutech.ac.jp