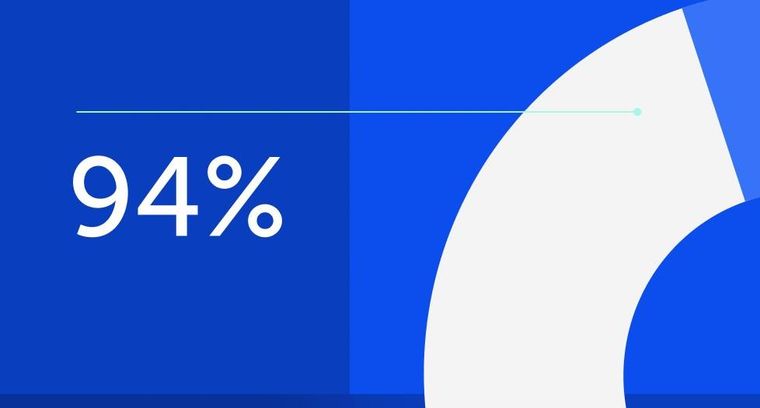
94% of researchers rate our articles as excellent or good
Learn more about the work of our research integrity team to safeguard the quality of each article we publish.
Find out more
ORIGINAL RESEARCH article
Front. Microbiol., 05 October 2021
Sec. Terrestrial Microbiology
Volume 12 - 2021 | https://doi.org/10.3389/fmicb.2021.715070
Autotrophic nitrification is regulated by canonical ammonia-oxidizing archaea (AOA) and bacteria (AOB) and nitrite-oxidizing bacteria (NOB). To date, most studies have focused on the role of canonical ammonia oxidizers in nitrification while neglecting the NOB. In order to understand the impacts of combined biochar and chemical fertilizer addition on nitrification and associated nitrifiers in plant rhizosphere soil, we collected rhizosphere soil from a maize field under four different treatments: no fertilization (CK), biochar (B), chemical nitrogen (N) + phosphorus (P) + potassium (K) fertilizers (NPK), and biochar + NPK fertilizers (B + NPK). The potential nitrification rate (PNR), community abundances, and structures of AOA, AOB, complete ammonia-oxidizing bacteria (Comammox Nitrospira clade A), and Nitrobacter- and Nitrospira-like NOB were measured. Biochar and/or NPK additions increased soil pH and nutrient contents in rhizosphere soil. B, NPK, and B + NPK treatments significantly stimulated PNR and abundances of AOB, Comammox, and Nitrobacter- and Nitrospira-like NOB, with the highest values observed in the B + NPK treatment. Pearson correlation and random forest analyses predicted more importance of AOB, Comammox Nitrospira clade A, and Nitrobacter- and Nitrospira-like NOB abundances over AOA on PNR. Biochar and/or NPK additions strongly altered whole nitrifying community structures. Redundancy analysis (RDA) showed that nitrifying community structures were significantly affected by pH and nutrient contents. This research shows that combined application of biochar and NPK fertilizer has a positive effect on improving soil nitrification by affecting communities of AOB and NOB in rhizosphere soil. These new revelations, especially as they related to understudied NOB, can be used to increase efficiency of agricultural land and resource management.
To meet the food needs of a large population, excess chemicals are often applied in agricultural production (Liu et al., 2015). However, chemical fertilizer use efficiency in Chinese agricultural ecosystem is typically low (Zhang et al., 2015). At the same time, the overuse and low use efficiency of chemical fertilizers have led to serious environmental problems such as soil acidification (Guo et al., 2010), increased greenhouse gas emissions (Hu et al., 2015), and surface water and groundwater pollution (Sebilo et al., 2013). This pathway is closely correlated with environmental problem and food production, which has attracted extensive attention in the past several decades (Koops et al., 2006).
Nitrification is a vital microbial-mediated N cycling process in which ammonia (NH3) is converted to nitrite (NO2–) and nitrate (NO3–). Traditional wisdom holds that autotrophic nitrification is mainly carried out by two different groups of nitrifiers: ammonia-oxidizing bacteria (AOB) and archaea (AOA) (Prosser and Nicol, 2012), and nitrite-oxidizing bacteria (NOB), which consist of six genera, namely, Nitrobacter, Nitrospira, Nitrococcus, Nitrotoga, Nitrolancetus, and Nitrospina (Attard et al., 2010; Daims et al., 2011). In 2015, what is exciting is that researchers found that complete ammonia-oxidizing bacteria (Comammox) of the genus Nitrospira sublineage II (NOB) were capable of oxidizing NH3 to NO3– in a single microorganism (Daims et al., 2015; van Kessel et al., 2015). A more recent study found that Comammox Nitrospira can be subdivided into two clades (A and B) based on the phylogeny of their ammonia monooxygenase (van Kessel et al., 2015). For decades, nitrification research has focused mainly on AOA and AOB, as they drive the first and often rate-limiting step of nitrification. In fact, nitrite oxidation can also become the limiting step for nitrification in disturbed soil ecosystems, and NOB has immense ecological significance as a principal source of NO3– that supports primary biological production on Earth (Galloway et al., 2008).
The impacts of various fertilization measures on canonical ammonia oxidizers have been widely studied in terrestrial ecosystems (He et al., 2007; Di et al., 2009; Ouyang et al., 2018). A large number of studies have shown that AOB are more dominant in high NH4+-N environments, while AOA prefer low NH4+-N environments (Shen et al., 2008; Jia and Conrad, 2009; Di et al., 2010; Verhamme et al., 2011; Ouyang et al., 2017). In contrast, we have limited information about the response of NOB to chemical fertilization in the agricultural ecosystem. For Comammox Nitrospira, clades A and B present different responses to NH4+-N concentrations (Wang et al., 2019a). Comammox Nitrospira clade A was found to contribute to nitrification in the agricultural soils with high NH4+-N input (Li et al., 2019), while Comammox Nitrospira clade B as well as AOA played a pivotal role in soil nitrification in low NH4+-N or oligotrophic soil conditions (Wang et al., 2019b). The Nitrobacter- and Nitrospira-like NOB are believed to be the two key NOB groups in soils, and their responses to N fertilization are totally different. Previous studies found that Nitrobacter-like NOB was more responsive than Nitrospira-like NOB to high urea additions (Ke et al., 2013; Han et al., 2018). In addition, the rhizosphere and associated root exudates could be the hotspots of nutrient cycling in agricultural soil (Liu et al., 2018; Yu et al., 2019), but there is limited information available regarding the impacts of N fertilizer on nitrification and related nitrifiers in rhizosphere soil.
Biochar is commonly used as a soil amendment to improve soil fertility (Novak et al., 2009), increases crop yields (Lehmann, 2007), reduces greenhouse gas (Cayuela et al., 2014), and enhances soil carbon sequestration (Lehmann and Joseph, 2015). Biochar improves soil quality due to its effects on soil physicochemical properties, including enhancement of water- and nutrient-holding capacities, soil pH, organic C content, and cation exchange capacity (Pandit et al., 2018). Besides, previous studies have indicated that biochar addition has a significant impact on soil nitrification process and canonical ammonia oxidizers, although across different soils the effect can be stimulating (Teutscherova et al., 2017; He et al., 2018; Shi et al., 2019), inhibiting (Wang et al., 2015; Li et al., 2020), or have no difference (He et al., 2016). For example, an increase in soil pH due to biochar input into soils usually elevates AOB abundance and nitrification rate in an acidic soil (He et al., 2018). Another study found that coconut husk biochar amendment enhanced nutrient retention by reducing nitrifier abundance and nitrification activity (Plaimart et al., 2020). He et al. (2016) observed that the application of biochar had no effect on nitrification activity and AOA and AOB communities in Cambosols with high pH. However, the impacts of the biochar on community abundance and structure of NOB are not yet clear, and a recent study found that the inhibitory effect of biochar amendment was linked with the abundance of Comammox Nitrospira in a highly acidic soil (Li et al., 2020). In addition, previous studies just concentrated on assessing the impact of biochar addition on canonical ammonia oxidizers in bulk soils, but little research attention has been given to investigate the effects of biochar amendment on root-associated nitrifying communities.
In the present study, a field trial was conducted to study the influence of biochar and/or NPK additions on soil potential nitrification rate (PNR), community abundances, and structures of nitrifiers in rhizosphere soils of maize using a shaken-slurry procedure, real-time PCR, and terminal restriction fragment length polymorphism (T-RFLP). The objective of this research was to: (1) identify the responses of PNR and nitrifying community abundances and structures to biochar and/or NPK additions in rhizosphere soil, as well as their relationships with soil chemical properties; and (2) to evaluate the role of canonical ammonia oxidizers and nitrite oxidizers on PNR in rhizosphere soil. We hypothesized that: (1) combined application of biochar and/or chemical fertilizer would stimulate soil nitrification rate in the tested soil; and (2) soil properties including pH and nutrient contents in rhizosphere soil disturbed by biochar and/or chemical fertilizer addition would directly influence the community abundances and structures of nitrifiers.
The field site was located in Wengyuan County, Guangdong Province, China (24°35′N, 114°13′E), where the conventional farming system is maize (Zea mays L.) monoculture. This area has a subtropical monsoon climate, with an average annual temperature of 20.6°C and average annual precipitation of 1,778.8 mm. The soil is classified as Ferralic Cambisol, which is widespread in South China. A local high-yielding variety of maize (Yedan 13) was used in this experiment.
The field experiment was conducted on July 2017. Briefly, the experiment consisted of four treatments with three replicates. The four treatments in the plot were as follows: (1) without fertilizer (CK); (2) soil with biochar at 20 t ha–1 without fertilizer (B); (3) chemical nitrogen, phosphorus, and potassium fertilizers (NPK); and (4) biochar (20 t ha–1) plus NPK (B + NPK). Each field plot was 30 m2 (6 m × 5 m), and a 1-m buffer was set between adjacent plots. The biochar used in this study derived from sugarcane straw, which was pyrolyzed to biochar at 550°C. Its basic properties are according to Li et al. (2020). Chemical fertilizers were applied in the form of urea, a fusion of calcium/magnesium phosphate, and potassium chloride, respectively. The N fertilizer was applied at the rate of 250 kg ha–1 year–1 according to local conventional fertilization management. Approximately 40% of N was applied as basal fertilizer, and the remaining 60% of N was applied as topdressing fertilizer. All P and K fertilizers and biochar were applied as basal fertilizers at the rate of 90 kg ha–1 year–1, 90 kg ha–1 year–1, and 20 t ha–1, respectively. Biochar and inorganic fertilizers were uniformly applied to the topsoil and immediately plowed by tillage before sowing.
Soil samples were collected at the maize reproductive stage on October 2019, when the rhizosphere effects tend to be the most activated (Cheng et al., 2003). The rhizosphere sampled at the 0–20-cm depth from 10 maize plants were taken from each plot. The 10 samples from a given plot were then mixed to form a composite sample. Rhizosphere soil samples were taken by carefully picking up the maize root, gently shaking it, and then brushing the soil attached to the root into a collection bag (Wang et al., 2017). The samples were mixed thoroughly and sieved through a 2-mm sieve. One part of each soil sample was stored at −80°C for DNA extraction and molecular biology analyses, and the remaining sample was stored at 4°C for PNR and chemical property analyses.
All soil parameters were analyzed according to the methods of Lu (2000). Fresh soil samples were analyzed for soil nitrate-N (NO3–-N) and ammonium-N (NH4+-N). The fresh soils were air-dried for measuring soil pH, organic matter (OM), total nitrogen (TN), total phosphorus (TP), and available phosphorus (AP). Soil pH was measured with a water:soil ratio of 2.5 using a pH meter. OM was determined using the K2Cr2O7 oxidation method. TN was assessed using the Kjeldahl method. TP was digested by H2SO4/HClO4 and measured by the molybdenum blue method. AP in soil was extracted with ammonium fluoride-hydrochloric acid and assayed by molybdenum–antimony anti-colorimetric method. NH4+-N and NO3–-N were extracted with 2 M KCl solution and then determined on a San++ Continuous Flow Analyzer.
Soil PNR was measured using a shaken soil-slurry method with supplemental NH4+ (Kong et al., 2019). Briefly, 15 g of fresh soil was mixed with 100 ml buffer solution (1.0 mM NH4+, 1.0 mM PO3–, pH = 7.2) in a 250-ml bottle and then shaken at 150 rpm for 48 h at 25°C. Ten milliliters of suspension was taken at 0-, 24-, and 48-h intervals, respectively. The resulting supernatant was centrifuged at 10,000 rpm for 5 min, and the supernatant was analyzed for NO3–-N using the San++ Continuous Flow Analyzer.
Soil DNA was extracted from 0.25 g fresh soil using MoBio PowerSoil DNA isolation Kit (MoBio Laboratory, Carlsbad, CA, United States). The quality and integrity of total DNA were assessed by 1% agarose gel electrophoresis. Quantification of functional marker genes (AOA, AOB, and Comammox Nitrospira clade A amoA, Nitrobacter-like nxrA, and Nitrospira-like nxrB) was carried out by quantitative PCR using a CFX96 Optical Real-Time Detection System (Bio-Rad, United States). The primer pairs and amplification procedures for quantification of functional genes are listed in Supplementary Table 1. The SYBR Premix Ex TaqTM Kit (TaKaRa Biotechnology Co., Dalian, China) was used to conduct quantitative PCR reactions. The PCR reaction (25 μl total) contained 12.5 μl 2 × SYBR Premix Ex TaqTM (Takara, Dalian), 0.5 μl of each primer, 2 μl DNA template (1–10 ng), and 9.5 μl dd H2O. Standard curves were obtained using plasmid DNA containing right inserts of the functional genes. Specific amplification was checked through a melting curve and a 1.5% agarose gel. However, we failed to amplify the band of Comammox Nitrospira clade B according to the gel electrophoresis detection system, and thus Comammox Nitrospira clade B was excluded from further analyses. The amplification efficiencies resulted in values of 88–95% for all functional genes, and the r2 values were > 0.99.
The AOA, AOB, Comammox Nitrospira, and Nitrobacter- and Nitrospira-like NOB community structures were analyzed by T-RFLP using the fluorescently labeled (6-FAM) forward primer (Ke et al., 2013; Hu et al., 2015). The PCR primer pairs and amplification conditions for these functional genes are shown in Supplementary Table 1. Each 50-μl reaction mixture consisted of 25 μl 2 × Premix (TaKaRa), 1, 10 μM forward and reverse primers, and 2 μl DNA template (10 ng). The labeled PCR products were separated by 1.5% agarose gel electrophoresis. After purification, the PCR products were then digested with restriction enzymes listed in Supplementary Table 2. The size of the terminal restriction fragments (T-RFs) and relative abundance were analyzed by an ABI 3730XL DNA analyzer (Applied Biosystems, Carlsbad, United States). GeneMarker 2.2 software was used to analyze T-RFLP profiles, and only peak heights > 100 fluorescence units were selected for the next step analysis. The relative abundance of each T-RF was calculated as the peak height percentage of a specific T-RF in the whole T-RFLP profile.
Clone libraries of AOA, AOB, Comammox amoA genes, and Nitrobacter-like nxrA and Nitrospira-like nxrB genes were constructed using the primers and reaction conditions in Supplementary Table 2. PCR products from each treatment were purified and ligated into the pMD19-T vector and transformed into competent E. coli top 10 cells. Positive clones for each functional gene were randomly selected for sequencing using an ABI 3730 sequencer (Applied Biosystems). Sequences in each clone library and the most similar sequences obtained from GenBank were used to construct the phylogenetic analysis. The phylogenetic analysis was conducted to construct a neighbor-joining tree using the Kimura two-parameter distance with 1,000 replicates to produce bootstrap values within MEGA 6.0. In order to identify the T-RFs of nitrifiers (AOA, AOB, Comammox, Nitrobacter, Nitrospira), clone libraries for each functional gene were constructed using the same primers as T-RFLP without the 6-FAM label. Virtual digests with restriction enzyme were carried out on the sequences retrieved from the clone libraries to allow the assignment of phylogenetic identify to individual T-RF. The obtained DNA sequences were deposited at the GenBank database under the following accession numbers: MZ614254–MZ614291 for AOA amoA gene, MZ614368–MZ614403 for AOB amoA gene, MZ614292–MZ614317 for Comammox Nitrospira amoA gene, MZ614318–MZ614339 for Nitrobacter-like nxrA gene, and MZ614340–MZ614367 for Nitrospira-like nxrB gene.
The effects of different treatments on soil chemical properties, PNR, the abundances of AOB, AOA, Comammox Nitrospira clade A, and Nitrobacter- and Nitrospira-like NOB were analyzed by one-way analysis of variance (ANOVA) based on the Duncan test with SPSS 20.0. Pearson correlation analysis was conducted to assess the relationships between soil chemical properties, PNR, and abundances of AOB, AOA, Comammox Nitrospira clade A, and Nitrobacter- and Nitrospira-like NOB using “corrplot” package in R software. Principal coordinate analysis (PCoA) was used to analyze the differences in nitrifying communities among the various treatments. Permutational multivariate analysis of variance (PERMANOVA) was used to test the differences in community structure among the different treatments. The detrended correspondence analysis (DCA) was performed by Canoco 5.0 to determine the overall functional changes of the nitrifying communities and found that the lengths of gradient for AOA, AOB, Comammox, nxrA, and nxrB were 0.3, 0.86, 0.28, 0.67, and 0.2, respectively. Therefore, redundancy analysis (RDA) was conducted to investigate the relationships between soil chemical properties and nitrifier community structure. Monte Carlo permutation test with 999 unrestricted permutations was used to determine the extent of the environmental parameter(s) that were able to explain the variation in the nitrifying communities. A random forest model was conducted using the “randomforest” and “A3” packages in R to select the main predictors of PNR. The mean square error (MSE) was calculated for each tree and averaged across 5,000 trees.
The effects of biochar and/or NPK addition on soil properties were analyzed (Table 1). Soil pH values ranged between 5.99 and 6.49 across the four different treatments. Compared with CK (pH = 5.99), biochar and/or NPK additions significantly increased soil pH (p < 0.05), with the highest value in the B treatment, which remained around 6.49 in rhizosphere soil. Similarly, compared with CK, biochar and/or NPK amendment obviously increased the contents of soil OM, AP, TP, TN, and NO3– in rhizosphere soil, with the highest values in the B and B + NPK treatments. The highest concentration of NH4+ was found in the soil treated with NPK alone, followed by the B + NPK treatment, which was much higher than the CK treatment.
The soil PNR had a different response to biochar and/or NPK amendment (Figure 1A). The PNR was 0.13 mg NO3–-N kg–1 day–1 in the CK treatment, while B, NPK, and B + NPK treatments significantly increased PNR by 4.08, 2.00, and 16.92 times, respectively.
Figure 1. Potential nitrification activities (PNR) (A); ammonia-oxidizing archaea (AOA) (B), bacteria (AOB) (C), and Comammox Nitrospira clade A (D) amoA gene abundances; Nitrobacter-like nxrA (E) and Nitrospira-like nxrB (F) gene abundances in rhizosphere soil. Error bars represent standard errors (n = 3). Different letters indicate significant differences across different treatments (p < 0.05). Treatments: CK, no fertilizer; B, biochar; NPK, nitrogen, phosphate, and potassium fertilizers; B + NPK, biochar + NPK.
The abundances of AOA, AOB, Comammox Nitrospira clade A amoA, Nitrobacter-like NOB nxrA, and Nitrospira-like NOB nxrB genes were measured using real-time PCR assay. AOA abundance ranged from 4.84 × 107 to 7.34 × 107 copies g–1 soil in rhizosphere soil (Figure 1B). Compared to the CK treatment, NPK and B + NPK treatments showed significantly elevated AOA abundance (p < 0.05), while the B treatment alone had no effect on it (p > 0.05). The abundances of AOB ranged from 3.75 × 106 to 5.10 × 107 copies g–1 soil and the abundance of Comammox Nitrospira clade A ranged from 7.12 × 107 to 3.09 × 108 copies g–1 soil (Figures 1C,D). Biochar and/or NPK additions significantly increased the abundances of AOB and Comammox Nitrospira clade A (p < 0.05), with the highest values (5.10 × 107 and 3.09 × 108 copies g–1 soil, respectively) in the B + NPK treatment (p < 0.01).
Nitrobacter- and Nitrospira-like NOB abundances ranged from 7.17 × 105 to 2.32 × 107 copies g–1 soil and 1.15 × 108 to 2.72 × 108 copies g–1 soil, respectively (Figures 1E,F). The highest Nitrobacter- and Nitrospira-like NOB abundances were detected in the B + NPK treatment, while the lowest values were observed in the CK treatment. The abundances of Nitrobacter- and Nitrospira-like NOB in the NPK and B treatments were higher than those in the CK treatment.
The PCoA of AOA, AOB, Comammox, Nitrobacter-like NOB, and Nitrospira-like NOB community structure revealed that the first two axes explained 96.55, 99.22, 95.28, 95.11, and 96.75% of the total variance, respectively (Figures 2B,D,F,H,J). In addition, the community structure of these nitrifiers under CK treatment was significantly separated from those in the other fertilization treatments (B, NPK, B + NPK), which was further supported by PERMANOVA analysis (Figures 2B,D,F,H,J). These results indicated that biochar and/or chemical fertilization changed the nitrifier community structure.
Figure 2. Treatment effects on nitrifying communities in rhizosphere soil characterized by terminal restriction fragment length polymorphism (T-RFLP). The T-RFLP profiles and principal coordinate analysis (PCoA) graphs of ammonia-oxidizing archaea (AOA) amoA (A,B), ammonia-oxidizing bacteria (AOB) amoA (C,D), Comammox Nitrospira amoA (E,F), Nitrobacter-like nxrA (G,H), and Nitrospira-like nxrB (I,J) genes across treatments. Permutational multivariate analysis of variance (PERMANOVA) was used to test the differences in community structure among the different treatments. Treatments: CK, no fertilizer; B, biochar; NPK, nitrogen, phosphate, and potassium fertilizers; B + NPK, biochar + NPK. OM, soil organic matter; AP, available P; TP, total P; TN, total N; NO3–, nitrate-N; NH4+, ammonium-N.
Biochar and/or NPK addition significantly affected the relative abundances of the major canonical ammonia oxidizers and nitrite oxidizers as indicated by T-RFLP analysis (Figure 2). Eight major T-RFs were obtained from the T-RFLP profile of the AOA amoA gene using the enzyme HpyCH4V (Figure 2A). The relative abundance of T-RF 76 bp was decreased by single biochar addition, while it was increased by single NPK amendment. However, the application of combined biochar with NPK (B + NPK) had little impact on its relative abundance compared with the CK treatment in rhizosphere soil. Biochar and/or NPK addition significantly reduced the relative abundances of T-RFs by 157, 205, and 249 bp. The relative abundance of T-RF 166 bp was enhanced in the B treatment but decreased in the NPK and B + NPK treatments. The relative abundance of 217 bp was decreased in the B treatment compared with CK treatment, while NPK and B + NPK treatments did not influence it. The relative abundance of T-RF 257 bp was increased in the B and B + NPK treatments, while NPK treatment showed no effect on it, compared with CK treatment. AOA amoA sequences obtained from the clone library were assigned to Nitrosotalea and Nitrososphaera clusters (Supplementary Figure 1). The T-RF 76 bp was affiliated with both Nitrosotalea and Nitrososphaera clusters. The T-RFs of 166, 205, and 249 bp were assigned to Nitrosotalea, while the T-RFs of 217 and 257 bp belonged to Nitrososphaera.
For AOB, there were also six main T-RFs reflecting the relative abundance using the enzyme MspI (Figure 2C). The relative abundance of T-RF 42 bp was not detected in the CK and NPK treatments, but it was significantly increased in the B and B + NPK treatments. The B and B + NPK treatments significantly elevated the relative abundance of T-RF 56 bp, whereas this T-RF decreased in the NPK treatment. The relative abundance of T-RFs 94 bp was significantly decreased in the B, NPK, and B + NPK treatments. The relative abundance of T-RF 156 bp was significantly decreased in the B and B + NPK treatments but significantly increased in the NPK treatment. The relative abundance of T-RF 234 bp was significantly decreased in the B and B + NPK treatments. The relative abundance of T-RF 257 bp was significantly increased in the NPK and B + NPK treatments. AOB amoA sequences obtained from the clone library were assigned to Nitrosospira and Nitrosomonas (Supplementary Figure 2). The T-RF 56 bp was affiliated with both Nitrosospira and Nitrosomonas. The T-RFs of 156, 234, and 257 bp were assigned to Nitrosospira, while the T-RFs of 217 and 257 bp belonged to Nitrososphaera. Six main T-RFs were obtained from the T-RFLP profile of Comammox Nitrospira amoA across all the soil samples (Figure 2E). The relative abundance of T-RF 73 bp was significantly decreased in the B and B + NPK treatments compared with the CK treatment, while the relative abundances of T-RFs 85, 134, and 141 bp were significantly increased. The relative abundance of T-RF 85 bp was significantly decreased in the NPK treatment while the relative abundance of T-RF 121 bp significantly increased in the B treatment, compared with the CK treatment. However, biochar and/or NPK addition did not affect the relative abundance of T-RF 78 bp. The phylogenetic analysis showed that all Comammox amoA sequences were assigned to Comammox clade A (Supplementary Figure 3). Eight and six major T-RFs were obtained from the T-RFLP profile of Nitrobacter-like nxrA and Nitrospira-like nxrB genes across soil samples, respectively (Figures 2G,I). For Nitrobacter-like NOB, the relative abundance of T-RF 266 bp was the dominant Nitrobacter-like nxrA genotype and significantly increased in the B and B + NPK treatments (Figure 2G). Besides, the relative abundances of T-RFs 55, 81, 121, and 193 bp were significantly decreased in the B and B + NPK treatments, while NPK treatment had no impact on them. The relative abundance of T-RF 106 bp was significantly increased in the NPK and B + NPK treatments, compared with the CK treatment. The relative abundance of T-RF 198 bp was only significantly increased in the B + NPK treatment. The phylogenetic analysis showed that the T-RFs of 55, 106, and 198 bp were only affiliated with Nitrobacter alkalicus, while T-RF 226 bp was assigned to both Nitrobacter alkalicus and Nitrobacter winogradskyi (Supplementary Figure 4). For Nitrospira-like NOB, the relative abundances of T-RF 98, 123, 182, and 192 bp were major fragments of the Nitrospira-like NOB nxrB gene (Figure 2I). The relative abundance of T-RF 98 bp in rhizosphere soil was significantly increased in B, NPK, and B + NPK treatments, while the relative abundances of T-RFs 123 and 182 bp were significantly decreased. The relative abundance of T-RF 192 bp was significantly increased in the B + NPK treatment, while single biochar or NPK addition had little impact on it. The phylogenetic analysis showed that T-RF 98 bp was assigned to Nitrospira lineage I and II. T-RF 123 bp was affiliated with Namibia soil cluster, Nitrospira lineage V and VI, while T-RF 182 bp belonged to Nitrospira lineage II and V.
As shown in Figure 3, Pearson correlation analyses indicated that AOA abundance was positively correlated with NH4+ (p < 0.001), while AOB and Comammox Nitrospira clade A abundances were positively correlated with pH, OM, TN, and NO3– (p < 0.05 or p < 0.001). The abundance of Nitrobacter-like NOB was positively correlated with pH, OM, and NO3– (p < 0.05, p < 0.01, or p < 0.001) while the abundance of Nitrospira-like NOB was positively correlated with pH, OM, AP, TP, TN, and NO3– (p < 0.01 or p < 0.001).
Figure 3. Pearson correlation analyses of soil chemical properties and potential nitrification rate (PNR), and nitrifying community abundances in rhizosphere soil. Blue denotes the significance level p < 0.05, the smaller shapes and darker color denote the larger correlation coefficients; red denotes the significance level p > 0.05, the larger shapes and darker color denote the smaller correlation coefficients. The correlation coefficients are shown in the lower left panel, * indicates p < 0.05, ** indicates p < 0.01, and *** indicates p < 0.001. OM, soil organic matter; AP, available P; TP, total P; TN, total N; NO3–, nitrate-N; NH4+, ammonium-N; AOA, ammonia-oxidizing archaea; AOB, ammonia-oxidizing bacteria; Comammox Nitrospira, complete ammonia oxidizers; Nitrobacter, Nitrobacter-like NOB; Nitrospira, Nitrospira-like NOB.
The correlations between soil properties and nitrifiers community structure were assessed by RDA (Figure 4). Overall, the first two axes explained 92.47, 98.63, 94.10, 93.54, and 93.60% of the total variability in the community structures of AOA, AOB, Comammox Nitrospira, Nitrobacter-, and Nitrospira-like NOB, respectively. Among the multiple soil variables, pH and most nutrient parameters were the primary factors influencing nitrifier community structure (Figures 4A–D).
Figure 4. Redundancy analysis (RDA) evaluates the relationships between soil chemical properties and nitrifying communities in rhizosphere soil. (A) Ammonia-oxidizing archaea (AOA), (B) ammonia-oxidizing bacteria (AOB), (C) Comammox Nitrospira, (D) Nitrobacter-like NOB, and (E) Nitrospira-like NOB. Treatments: CK, no fertilizer; B, biochar; NPK, nitrogen, phosphate, and potassium fertilizers; B + NPK, biochar + NPK. OM, soil organic matter; AP, available P; TP, total P, TN: total N; NO3–, nitrate-N; NH4+, ammonium-N.
Soil PNR was significantly correlated with soil pH, OM, TN, and NO3– (p < 0.05, p < 0.01, or p < 0.001) (Figure 3). In addition, soil PNR was significantly and positively correlated with the abundances of AOB, Comammox Nitrospira clade A, and Nitrobacter-like, and Nitrospira-like NOB (Figure 3). Random forest analysis was conducted to reveal the main factors in predicting the PNR in the study. Nitrospira-like NOB, AOB, Comammox Nitrospira clade A, and Nitrobacter-like NOB abundances were the major predictors for PNR (Figure 5). In addition, PNR was also predicted by OM and NO3–, which was consistent with Pearson’s correlation analysis (Figures 3, 5).
Figure 5. Random forest model predicts the relative importance of soil chemical properties and abundances of nitrifying communities, as measured by percentage of increase in mean square error (MSE) of the potential nitrification rate (PNR). OM, soil organic matter; AP, available P; TP, total P; TN, total N; NO3–, nitrate-N; NH4+, ammonium-N; AOA, ammonia-oxidizing archaea; AOB, ammonia-oxidizing bacteria; Comammox Nitrospira, complete ammonia oxidizers; Nitrobacter, Nitrobacter-like NOB; Nitrospira, Nitrospira-like NOB.
In this study, we characterized the effect of biochar and/or NPK fertilizer additions on PNR to better understand how these measures influenced the soil nitrification process. PNR was very weak in the CK treatment, while NPK addition alone significantly increased it, probably due to the absence of substrate NH4+ (He et al., 2016). Addition of biochar alone also significantly stimulated soil PNR compared to the CK treatment, indicating that biochar addition had a positive effect on PNR, which was in line with a recent study that manure-based biochar increased the gross nitrification rate in rhizosphere soil using the 15N isotope labeling technique (Pokharel et al., 2021). Moreover, PNR in the B + NPK treatment was observed higher than in the NPK treatment, demonstrating that application of combined biochar with NPK had a synergistic effect on soil nitrification. Biochar may significantly improve soil structure and aeration due to its high porosity and low bulk density (Joseph et al., 2010), thereby providing a suitable environment for a high nitrification rate. In addition, biochar elevates soil organic matter, leading to a high C/N ratio that could stimulate the soil nitrification rate (Bi et al., 2017). Furthermore, biochar is usually alkaline and its application could increase soil pH, another factor that could promote soil nitrification (Cayuela et al., 2014). In our study, Pearson correlation analysis showed a positive relationship between PNR and pH and nutrient contents including OM and TN. Overall, these results supported our hypothesis that biochar and/or NPK addition had positive effects on PNR as they elevate soil pH and nutrient availability.
Canonical ammonia oxidizers (AOA and AOB) carry out the first step of nitrification, in which ammonia is converted to nitrite by encoding the subunit of ammonia monooxygenase, the limiting factor in soil nitrification (Shi et al., 2019). In agreement with previous studies (Leininger et al., 2006; He et al., 2018; Yu et al., 2019), AOA dominated in abundance compared to AOB in the tested soil. Single biochar amendment had little impact on AOA abundance, which was contrary to a previous study (Xiao et al., 2019). The higher AOA abundance in NPK and B + NPK treatments compares with CK, indicating that substrate NH4+ concentration may be the limiting factor for AOA growth in the selected soil. The positive correlation between AOA and NH4+ concentration supported our argument. Additionally, the abundance of AOB significantly enhanced in the biochar and/or NPK treatments, accompanied by higher nitrification rates in these soils. Meanwhile, a strong positive relationship was found between AOB abundance and soil PNR (r = 0.98, p < 0.001), which indicated that AOB might play a more important role than AOA in soil nitrification activity in biochar and/or NPK treatments. Particularly, the highest abundance of AOB and PNR was observed in biochar + NPK treatment, suggesting that the application of combined biochar and NPK could increase nitrification rates by increasing AOB abundance in rhizosphere soil. Consistent with our findings, He et al. (2018) showed that the application of biochar increased AOB abundance and PNR in oxisols with low pH compared with CK. Another study also reported that AOB abundance was strongly correlated with net nitrification rate and that AOB abundance followed a similar trend as NO3– in biochar amendment treatments under a microcosm experiment, implying that AOB could contribute more to nitrification in degraded acid soil when biochar and N fertilizer is added together (Teutscherova et al., 2017). A large number of studies have indicated that the application of biochar would provide a better living environment by increasing soil pH (liming effect) (Dai et al., 2016), heighten nutrient retention and soil fertility (Hagemann et al., 2017), and improve soil aeration (Cayuela et al., 2014), and these factors are often linked with the growth of AOA and AOB (Xiao et al., 2019).
The T-RFLP analysis showed that single biochar amendment significantly altered the community structures of canonical ammonia oxidizers in the rhizosphere soil. This result differs from a previous study that showed single biochar addition did not significantly change AOA and AOB community structures in an intensive vegetable soil (Bi et al., 2017). In this case, we suspect that the effect of biochar addition on community structures of canonical ammonia oxidizers strongly depends on soil type, with fundamental soil properties affecting the effect of biochar. In addition, this study also found that the community structures of AOA and AOB were significantly altered by NPK amendment, which was in line with a previous study that the application of inorganic fertilizer containing N showed significant impact on AOA and AOB community structures in acidic red soil (He et al., 2007). Furthermore, the community structures of AOA and AOB were significantly shifted by the combined application of biochar and NPK, which partly aligns with previous studies showing that combined application of biochar with N fertilizer influenced AOB but not AOA community structure (Bi et al., 2017; Lin et al., 2017). Based on the RDA analysis, the reason that significant changes in community structures of canonical ammonia oxidizers were observed in our study could be elevated pH and nutrient contents after biochar and/or NPK amendment.
In our study, we found that Comammox Nitrospira clade A outnumbered canonical ammonia oxidizers (AOA and AOB). Similar results were also reported in a subtropical paddy soil (Liu et al., 2019), pasture soil (Li et al., 2019), and forest soil (Hu and He, 2017; Osburn and Barrett, 2020). A higher abundance of Comammox Nitrospira has been observed in environments with low NH3 concentration (Hu and He, 2017), and Nitrospira inopinata, the only pure strain of Comammox bacteria, is assumed to have an oligotrophic lifestyle in a previous study (Kits et al., 2017). However, in contrast to traditional understanding, we found that the application of NPK or combined biochar and NPK (i.e., eutrophic conditions) significantly elevated Comammox Nitrospira clade A abundance, which was significantly and positively correlated with soil nitrification rate. These results provide new evidence that Comammox Nitrospira clade A may play an active role in nitrification of tested rhizosphere soil-amended biochar and/or NPK fertilizer. Single biochar addition significantly increased Nitrobacter-like NOB rather than Nitrospira-like NOB abundance, indicating that biochar showed a positive effect on it. Previous studies reported that Nitrobacter-like NOB had a lower affinity than Nitrospira-like NOB for N substrate and could be stimulated by high N levels (Wagner et al., 2002; Attard et al., 2010; Ke et al., 2013; Han et al., 2018). For example, Ma et al. (2016) found that Nitrobacter-like NOB abundance in a pasture soil elevated linearly with increasing N fertilizer amendment gradient while Nitrospira-like NOB was not obviously affected. Wertz et al. (2011) also observed that N fertilization enhanced Nitrobacter-like NOB abundance but not Nitrospira-like NOB in forest soils. However, in our study, the abundances of Nitrobacter- and Nitrospira-like NOB were both significantly increased in NPK treatment, which was also consistent with a recent study (Kong et al., 2019). Thus, the response of Nitrospira-like NOB to N fertilization is likely dependent on soil type or other complex ecosystem features and needs to be more thoroughly investigated in future. Furthermore, the higher abundances of Nitrobacter- and Nitrospira-like NOB were observed in B + NPK treatment compared with NPK alone, suggesting that biochar and NPK interact to exert a greater effect on NOB abundance. In our study, Pearson correlation analysis showed that changes in the abundances of Comammox Nitrospira clade A, Nitrobacter- and Nitrospira-like NOB were significantly and positively correlated with soil pH and multiple nutrient contents, suggesting that soil pH and nutrient availability may explain in part their promoting effect on the growth of nitrite oxidizers.
Our study showed that biochar and/or NPK amendment significantly changed the community structures of nitrite oxidizers (Comammox Nitrospira, and Nitrobacter- and Nitrospira-like NOB) in rhizosphere soil. To better understand the complex interaction of factors, it is necessary to explore how soil chemical properties influenced nitrite oxidizers community structures under biochar and/or NPK addition. The RDA analysis suggested that the variability of nitrite oxidizers was remarkably affected by soil pH and multiple nutrients (Figure 4), in agreement with recent studies (Han et al., 2018; Wang et al., 2019a; Yu et al., 2019). Wang et al. (2019a) found that pH, OM, and available N are key factors affecting Comammox community structure. Han et al. (2018) reported that Nitrobacter-like community structure was strongly influenced by pH and soil organic carbon content and the Nitrospira-like NOB community structure was significantly explained by pH and TN. Therefore, biochar and/or NPK amendment resulted in a shift of soil pH and nutrient availability, which are the key factors affecting community structures of nitrite oxidizers in the rhizosphere soil.
Our present study revealed that biochar and/or NPK addition significantly increased the soil nitrification rate in rhizosphere soil of maize. Single biochar addition significantly increased AOB, Comammox Nitrospira clade A, Nitrobacter-like NOB and Nitrospira-like NOB abundances, while it showed no impact on AOA abundance. Biochar and/or NPK addition also changed nitrifying community structures, and pH and nutrient availability were key factors influencing them. We found positive correlations between AOB, Comammox Nitrospira clade A, and Nitrobacter- and Nitrospira-like NOB abundances and potential nitrification rates, indicating that these nitrifiers may play important roles in soil nitrification.
The original contributions presented in the study are included in the article/Supplementary Material, further inquiries can be directed to the corresponding author/s.
QW contributed to the design of the research and revised the manuscript. PS and ZZ conducted the experiment and participated in drafting the manuscript. PF, WC, and YR contributed to analyze the results. All authors have read and approved the final manuscript.
This work was supported by the National Natural Science Foundation of China (41807054) and Academician Innovation Team Project (No. RZ2000008513KY).
The authors declare that the research was conducted in the absence of any commercial or financial relationships that could be construed as a potential conflict of interest.
All claims expressed in this article are solely those of the authors and do not necessarily represent those of their affiliated organizations, or those of the publisher, the editors and the reviewers. Any product that may be evaluated in this article, or claim that may be made by its manufacturer, is not guaranteed or endorsed by the publisher.
We would like to thank Stephen Gaughran at Princeton University for his assistance with english language and grammatical editing.
The Supplementary Material for this article can be found online at: https://www.frontiersin.org/articles/10.3389/fmicb.2021.715070/full#supplementary-material
Attard, E., Poly, F., Commeaux, C., Laurent, F., Terada, A., Smets, B. F., et al. (2010). Shifts between Nitrospira- and Nitrobacter-like nitrite oxidizers underlie the response of soil potential nitrite oxidation to changes in tillage practices. Environ. Microbiol. 12, 315–326. doi: 10.1111/j.1462-2920.2009.02070.x
Bi, Q. F., Chen, Q. H., Yang, X. R., Li, H., Zheng, B. X., Zhou, W. W., et al. (2017). Effects of combined application of nitrogen fertilizer and biochar on the nitrification and ammonia oxidizers in an intensive vegetable soil. Amb. Express 7:198. doi: 10.1186/s13568-017-0498-7
Cayuela, M. L., van Zwieten, L., Singh, B. P., Jeffery, S., Roig, A., and Sánchez-Monedero, M. A. (2014). Biochar’s role in mitigating soil nitrous oxide emissions: a review and meta-analysis. Agri. Ecosyst. Environ. 191, 5–16. doi: 10.1016/j.agee.2013.10.009
Cheng, W., Johnson, D., and Fu, S. (2003). Rhizosphere effects on decomposition: controls of plant species, phenology, and fertilization. Soil Sci. Soc. America J. 67, 1418–1427. doi: 10.2136/sssaj2003.1418
Dai, Z. M., Zhang, X. J., Tang, C., Muhammad, N., Wu, J. J., Brookes, P. C., et al. (2016). Potential role of biochars in decreasing soil acidification-a critical review. Sci. Total Environ. 78, 1601–1611. doi: 10.1016/j.scitotenv.2016.12.169
Daims, H., Lebedeva, E. V., Pjevac, P., Han, P., Herbold, C., Albertsen, M., et al. (2015). Complete nitrifification by Nitrospira bacteria. Nature 528, 504–509. doi: 10.1038/nature16461
Daims, H., Lücker, S., Paslier, D. L., and Wagner, M. (2011). “Diversity, environmental genomics, and ecophysiology of nitrite-oxidizing bacteria,” in Nitrification, eds B. B. Ward, D. J. Arp, and M. G. Klotz (Washington, DC: ASM Press), 295–322.
Di, H. J., Cameron, K. C., He, J. Z., Shen, J. P., Winefield, C. S., O’Callaghan, M., et al. (2010). Ammonia-oxidizing bacteria and archaea grow under contrasting soil nitrogen conditions. FEMS Microbiol. Ecol. 72, 386–394. doi: 10.1111/j.1574-6941.2010.00861.x
Di, H. J., Cameron, K. C., Shen, J. P., Winefield, C. S., O’Callaghan, M., Bowatte, S., et al. (2009). Nitrification driven by bacteria and not archaea in nitrogen-rich grassland soils. Nat. Geosci. 2, 621–624. doi: 10.1038/ngeo613
Galloway, J. N., Townsend, A. R., Erisman, J. W., Bekunda, M., Cai, Z. C., Freney, J. R., et al. (2008). Transformation of the nitrogen cycle: recent trends, questions, and potential solutions. Science 320, 889–892. doi: 10.1126/science.1136674
Guo, J. H., Liu, X. J., Zhang, Y., Shen, J. L., Han, W. X., Zhang, W. F., et al. (2010). Significant acidification in major Chinese croplands. Science 327, 1008–1010. doi: 10.1126/science.1182570
Hagemann, N., Joseph, S., Schmidt, H. P., Kammann, C. I., Harter, J., Borch, T., et al. (2017). Organic coating on biochar explains its nutrient retention and stimulation of soil fertility. Nat. Commun. 8:1089. doi: 10.1038/s41467-017-01123-0
Han, S., Zeng, L. Y., Luo, X. S., Xiong, X., Wen, S. L., Wang, B. R., et al. (2018). Shifts in Nitrobacter- and Nitrospira-like nitrite-oxidizing bacterial communities under long-term fertilization practices. Soil Biol. Biochem. 124, 118–125. doi: 10.1016/j.soilbio.2018.05.033
He, J. Z., Shen, J. P., Zhang, L. M., Zhu, Y. G., Zheng, Y. M., Xu, M. G., et al. (2007). Quantitative analyses of the abundance and composition of ammonia-oxidizing bacteria and ammonia-oxidizing archaea of a Chinese upland red soil under long-term fertilization practices. Environ. Microbiol. 9, 2364–2374. doi: 10.1111/j.1462-2920.2007.01481.x
He, L. L., Bi, Y. C., Zhao, J., Pittelkow, C. M., Zhao, X., Wang, S. Q., et al. (2018). Population and community structure shifts of ammonia oxidizers after four-year successive biochar application to agricultural acidic and alkaline soils. Sci. Total Environ. 620, 1105–1115. doi: 10.1016/j.scitotenv.2017.11.029
He, L. L., Liu, Y., Zhao, J., Bi, Y. C., Zhao, X., Wang, S. Q., et al. (2016). Comparison of straw-biochar-mediated changes in nitrification and ammonia oxidizers in agricultural oxisols and cambosols. Biol. Fertil. Soils 52, 137–149. doi: 10.1007/s00374-015-1059-3
Hu, H. W., Chen, D. L., and He, J. Z. (2015). Microbial regulation of terrestrial nitrous oxide formation: understanding the biological pathways for prediction of emission rates. FEMS Microbiol. Rev. 39, 729–749. doi: 10.1093/femsre/fuv021
Hu, H. W., and He, J. Z. (2017). Comammox—a newly discovered nitrification process in the terrestrial nitrogen cycle. J. Soils Sediments 17, 2709–2717. doi: 10.1007/s11368-017-1851-9
Jia, Z. J., and Conrad, R. (2009). Bacteria rather than archaea dominate microbial ammonia oxidation in an agricultural soil. Environ. Microbiol. 11, 1658–1671. doi: 10.1111/j.1462-2920.2009.01891.x
Joseph, S. D., Camps-Arbestain, M., Lin, Y., Munroe, P., Chia, C. H., Hook, J., et al. (2010). An investigation into the reactions of biochar in soil. Soil Res. 487, 501–515. doi: 10.1071/SR10009
Ke, X., Angel, R., Lu, Y., and Conrad, R. (2013). Niche differentiation of ammonia oxidizers and nitrite oxidizers in rice paddy soil. Environ. Microbiol. 15, 2275–2292. doi: 10.1111/1462-2920.12098
Kits, K. D., Sedlacek, C. J., Lebedeva, E. V., Han, P., Bulaev, A., Pjevac, P., et al. (2017). Kinetic analysis of a complete nitrifier reveals an oligotrophic lifestyle. Nature 54, 269–272. doi: 10.1038/nature23679
Kong, Y. L., Ling, N., Xue, C., Chen, H., Ruan, Y., Guo, J. J., et al. (2019). Long-term fertilization regimes change soil nitrification potential by impacting active autotrophic ammonia oxidizers and nitrite oxidizers as assessed by DNA stable isotope probing. Environ. Microbiol. 21, 1224–1240. doi: 10.1038/nature23679
Koops, H. P., Purkhold, U., Pommerening-Röser, A., Timmermann, G., and Wagner, M. (2006). “The lithoautotrophic ammonia-oxidizing bacteria,” in The Prokaryotes: Proteobacteria: Alpha and Beta Subclasses, eds M. Dworkin, S. Falkow, E. Rosenberg, K.-H. Schleifer, and E. Stackebrandt (New York, NY: Springer New York), 778–811.
Lehmann, J., and Joseph, S. (2015). Biochar for Environmental Management: Science, Technology and Implementation, 2nd Edn. London: Earthscan.
Leininger, S., Urich, T., Schloter, M., Schwark, L., Qi, J., Nicol, G., et al. (2006). Archaea predominate among ammonia-oxidizing prokaryotes in soils. Nature 442, 806–809. doi: 10.1038/nature04983
Li, C. Y., Hu, H. W., Chen, Q. L., Chen, D., and He, J. Z. (2019). Comammox Nitrospira play an active role in nitrification of agricultural soils amended with nitrogen fertilizers. Soil Biol. Biochem. 138:107609. doi: 10.1016/j.soilbio.2019.107609
Li, S., Chen, D. W., Wang, C., Chen, D., and Wang, Q. (2020). Reduced nitrification by biochar and/or nitrification inhibitor is closely linked with the abundance of Comammox Nitrospira in a highly acidic sugarcane soil. Biol. Fertil. Soils 56, 1219–1228. doi: 10.1007/s00374-020-01499-0
Lin, Y. X., Ding, W. X., Liu, D. Y., He, T. H., Yoo, G. Y., Yuan, J. J., et al. (2017). Wheat straw-derived biochar amendment stimulated N2O emissions from rice paddy soils by regulating the amoA genes of ammonia-oxidizing bacteria. Soil Biol. Biochem. 113, 89–98. doi: 10.1016/j.soilbio.2017.06.001
Liu, T., Wang, Z., Wang, S., Zhao, Y., Wright, A. L., and Jiang, X. (2019). Responses of ammonia-oxidizers and comammox to different long-term fertilization regimes in a subtropical paddy soil. Europ. Soil Biol. 93:103087. doi: 10.1016/j.ejsobi.2019.103087
Liu, X. R., Li, J., Yu, L., Pan, H., Liu, H. Y., Liu, Y. M., et al. (2018). Simultaneous measurement of bacterial abundance and composition in response to biochar in soybean field soil using 16S rRNA gene sequencing. Land Degrad. Dev. 29, 2172–2182. doi: 10.1002/ldr.2838
Liu, Y. B., Pan, X. B., and Li, J. S. (2015). A 1961-2010 record of fertilizer use, pesticide application and cereal yields: a review. Agro. Sustainable Dev. 35, 83–93. doi: 10.1007/s13593-014-0259-9
Ma, W. B., Jiang, S. J., Assemien, F. E. L., Qin, M. S., Ma, B. B., Xie, Z., et al. (2016). Response of microbial functional groups involved in soil N cycle to N, P and NP fertilization in Tibetan alpine meadows. Soil Biol. Biochem. 101, 195–206. doi: 10.1016/j.soilbio.2016.07.023
Novak, J. M., Bussche, R. W. J., Laird, D. L., Ahmedna, M., Watts, D. W., and Niandou, M. A. S. (2009). Impact of biochar amendment on fertility of a southeastern coastal plain soil. Soil Sci. 174, 105–112. doi: 10.1097/SS.0b013e3181981d9a
Osburn, E. D., and Barrett, J. E. (2020). Abundance and functional importance of complete ammonia-oxidizing bacteria (comammox) versus canonical nitrifiers in temperate forest soils. Soil Biol. Biochem. 145:107801. doi: 10.1016/j.soilbio.2020.107801
Ouyang, Y., Evans, S. E., Friesen, M. L., and Tiemann, L. K. (2018). Effect of nitrogen fertilization on the abundance of nitrogen cycling genes in agricultural soils: a meta-analysis of field studies. Soil Biol. Biochem. 127, 71–78. doi: 10.1016/j.soilbio.2018.08.024
Ouyang, Y., Norton, J. M., and Stark, J. M. (2017). Ammonium availability and temperature control contributions of ammonia oxidizing bacteria and archaea to nitrification in an agricultural soil. Soil Biol. Biochem. 113, 161–172. doi: 10.1016/j.soilbio.2017.06.010
Pandit, N. R., Mulder, J., Hale, S. E., Zimmerman, A. R., Pandit, B. H., and Cornelissen, G. (2018). Multi-year double cropping biochar field trials in Nepal: finding the optimal biochar dose through agronomic trials and cost-benefit analysis. Sci. Total Environ. 63, 1333–1341. doi: 10.1016/j.scitotenv.2018.05.107
Plaimart, J., Acharya, K., Mrozik, W., Davenport, R. J., Vinitnantharat, S., and Werner, D. (2020). Coconut husk biochar amendment enhances nutrient retention by suppressing nitrification in agricultural soil following anaerobic digestate application. Environ. Pollut. 268:115684. doi: 10.1016/j.envpol.2020.115684
Pokharel, P., Qi, L., and Chang, S. X. (2021). Manure-based biochar decreases heterotrophic respiration and increases gross nitrification rates in rhizosphere soil. Soil Biol. Biochem. 154:108147. doi: 10.1016/j.soilbio.2021.108147
Prosser, J. I., and Nicol, G. W. (2012). Archaeal and bacterial ammonia-oxidisers in soil: the quest for niche specialisation and differentiation. Trends Microbiol. 20, 523–531. doi: 10.1016/j.tim.2012.08.001
Sebilo, M., Mayer, B., Nicolardot, B., Pinay, G., and Mariotti, A. (2013). Long-term fate of nitrate fertilizer in agricultural soils. Proc. Natl. Acad. Sci. U. S. A. 110, 18185–18189. doi: 10.1073/pnas.1305372110
Shen, J. P., Zhang, L. M., Zhu, Y. G., Zhang, J. B., and He, J. Z. (2008). Abundance and composition of ammonia-oxidizing bacteria and ammonia-oxidizing archaea communities of an alkaline sandy loam. Environ. Microbiol. 10, 1601–1611. doi: 10.1111/j.1462-2920.2008.01578.x
Shi, R. Y., Ni, N., Nkoh, J. N., Li, J. Y., Xu, R. K., and Qian, W. (2019). Beneficial dual role of biochars in inhibiting soil acidification resulting from nitrification. Chemosphere 234, 43–51. doi: 10.1016/j.chemosphere.2019.06.030
Teutscherova, N., Vazquez, E., Masaguer, A., Navas, M., Scow, K. M., Schmidt, R., et al. (2017). Comparison of lime- and biochar-mediated pH changes in nitrification and ammonia oxidizers in degraded acid soil. Biol. Fertil. Soils 53, 811–821. doi: 10.1007/s00374-017-1222-0
van Kessel, M. A., Speth, D. R., Albertsen, M., Nielsen, P. H., den Camp, H. J. O., Kartal, B., et al. (2015). Complete nitrification by a single microorganism. Nature 528, 555–559. doi: 10.1038/nature16459
Verhamme, D. T., Prosser, J. I., and Nicol, G. W. (2011). Ammonia concentration determines differential growth of ammonia-oxidising archaea and bacteria in soil microcosms. ISME J. 5, 1067–1071. doi: 10.1038/ismej.2010.191
Wagner, M., Loy, A., Nogueira, R., Purkhold, U., Lee, N., and Daims, H. (2002). Microbial community composition and function in wastewater treatment plants. Antonie van Leeuwen. Inter. Gen. Molecu. Microbiol. 81, 665–680. doi: 10.1023/A:1020586312170
Wang, C., Zheng, M. M., Song, W. F., Wen, S. L., Wang, B. R., Zhu, C. Q., et al. (2017). Impact of 25 years of inorganic fertilization on diazotrophic abundance and community structure in an acidic soil in southern China. Soil Biol. Biochem. 113, 240–249. doi: 10.1016/j.soilbio.2017.06.019
Wang, J. C., Wang, J. L., Rhodes, G., He, J. Z., and Ge, Y. (2019a). Adaptive responses of comammox Nitrospira and canonical ammonia oxidizers to long-term fertilizations: implications for the relative contributions of different ammonia oxidizers to soil nitrogen cycling. Sci. Total Environ. 668, 224–233. doi: 10.1016/j.scitotenv.2019.02.427
Wang, Z., Cao, Y., Zhu-Barker, X., Nicol, G. W., Wright, A. L., Jia, Z., et al. (2019b). Comammox Nitrospira clade B contributes to nitrification in soil. Soil Biol. Biochem. 135, 392–395. doi: 10.1016/j.soilbio.2019.06.004
Wang, Z. Y., Zong, H. Y., Zheng, H., Liu, G. C., Chen, L., and Xing, B. S. (2015). Reduced nitrification and abundance of ammonia-oxidizing bacteria in acidic soil amended with biochar. Chemosphere 138, 576–583. doi: 10.1016/j.chemosphere.2015.06.084
Wertz, S., Leigh, A. K., and Grayston, S. J. (2011). Effects of long-term fertilization of forest soils on potential nitrification and on the abundance and community structure of ammonia oxidizers and nitrite oxidizers. FEMS Microbiol. Ecol. 79, 142–154. doi: 10.1111/j.1574-6941.2011.01204.x
Xiao, Z. G., Rasmann, S., Yue, L., Lian, F., Zou, H., and Wang, Z. Y. (2019). The effect of biochar amendment on N-cycling genes in soils: a meta-analysis. Sci. Total Environ. 696:133984. doi: 10.1016/j.scitotenv.2019.133984
Yu, L., Homyak, P. M., Kang, X. X., Brookes, P. C., Ye, Y. K., Lin, Y. N., et al. (2019). Changes in abundance and composition of nitrifying communities in barley (Hordeum vulgare L.) rhizosphere and bulk soils over the growth period following combined biochar and urea amendment. Biol. Fertil. Soils 56, 169–183. doi: 10.1007/s00374-019-01410-6
Keywords: nitrification, AOA, AOB, Comammox Nitrospira, NOB, rhizosphere soil
Citation: Sun P, Zhao Z, Fan P, Chen W, Ruan Y and Wang Q (2021) Ammonia- and Nitrite-Oxidizing Bacteria are Dominant in Nitrification of Maize Rhizosphere Soil Following Combined Application of Biochar and Chemical Fertilizer. Front. Microbiol. 12:715070. doi: 10.3389/fmicb.2021.715070
Received: 26 May 2021; Accepted: 09 September 2021;
Published: 05 October 2021.
Edited by:
Rich Boden, University of Plymouth, United KingdomReviewed by:
Xuesong Luo, Huazhong Agricultural University, ChinaCopyright © 2021 Sun, Zhao, Fan, Chen, Ruan and Wang. This is an open-access article distributed under the terms of the Creative Commons Attribution License (CC BY). The use, distribution or reproduction in other forums is permitted, provided the original author(s) and the copyright owner(s) are credited and that the original publication in this journal is cited, in accordance with accepted academic practice. No use, distribution or reproduction is permitted which does not comply with these terms.
*Correspondence: Qing Wang, d2FuZ3FpbmczMTYwMDBAMTYzLmNvbQ==
Disclaimer: All claims expressed in this article are solely those of the authors and do not necessarily represent those of their affiliated organizations, or those of the publisher, the editors and the reviewers. Any product that may be evaluated in this article or claim that may be made by its manufacturer is not guaranteed or endorsed by the publisher.
Research integrity at Frontiers
Learn more about the work of our research integrity team to safeguard the quality of each article we publish.