- 1Postgraduate Program of Microbiology, Parasitology and Pathology, Department of Pathology, Federal University of Paraná, Curitiba, Brazil
- 2Department of Biomedicine, Centro Universitário Católica de Santa Catarina, Joinville, Brazil
- 3Postgraduate Program of Genetics, Federal University of Paraná, Curitiba, Brazil
- 4Department of General and Applied Biology, Biosciences Institute, State University of São Paulo, Rio Claro, Brazil
- 5Department of Pharmaceutical Sciences, College of Pharmacy, University of Kentucky, Lexington, KY, United States
- 6Center for Pharmaceutical Research and Innovation, College of Pharmacy, University of Kentucky, Lexington, KY, United States
Plant diseases caused by phytopathogens are responsible for significant crop losses worldwide. Resistance induction and biological control have been exploited in agriculture due to their enormous potential. In this study, we investigated the antimicrobial potential of endophytic fungi of leaves and petioles of medicinal plants Vochysia divergens and Stryphnodendron adstringens located in two regions of high diversity in Brazil, Pantanal, and Cerrado, respectively. We recovered 1,304 fungal isolates and based on the characteristics of the culture, were assigned to 159 phenotypes. One isolate was selected as representative of each phenotype and studied for antimicrobial activity against phytopathogens. Isolates with better biological activities were identified based on DNA sequences and phylogenetic analyzes. Among the 159 representative isolates, extracts from 12 endophytes that inhibited the mycelial growth (IG) of Colletotrichum abscissum (≥40%) were selected to expand the antimicrobial analysis. The minimum inhibitory concentrations (MIC) of the extracts were determined against citrus pathogens, C. abscissum, Phyllosticta citricarpa and Xanthomonas citri subsp. citri and the maize pathogen Fusarium graminearum. The highest activity against C. abscissum were from extracts of Pseudofusicoccum stromaticum CMRP4328 (IG: 83% and MIC: 40 μg/mL) and Diaporthe vochysiae CMRP4322 (IG: 75% and MIC: 1 μg/mL), both extracts also inhibited the development of post-bloom fruit drop symptoms in citrus flowers. The extracts were promising in inhibiting the mycelial growth of P. citricarpa and reducing the production of pycnidia in citrus leaves. Among the isolates that showed activity, the genus Diaporthe was the most common, including the new species D. cerradensis described in this study. In addition, high performance liquid chromatography, UV detection, and mass spectrometry and thin layer chromatography analyzes of extracts produced by endophytes that showed high activity, indicated D. vochysiae CMRP4322 and P. stromaticum CMRP4328 as promising strains that produce new bioactive natural products. We report here the capacity of endophytic fungi of medicinal plants to produce secondary metabolites with biological activities against phytopathogenic fungi and bacteria. The description of the new species D. cerradensis, reinforces the ability of medicinal plants found in Brazil to host a diverse group of fungi with biotechnological potential.
Introduction
Plant diseases caused by microorganisms lead to significant crop losses worldwide (Oerke, 2006; Cantrell et al., 2012; Tan et al., 2018; Sang and Kim, 2020). It is estimated that the fungal diseases cause approximately 20% of yield reductions in commercial crops and major foods worldwide (Wang et al., 2016; Fisher et al., 2018; Zhao et al., 2020). In Brazil, economically important crops such as citrus and maize are affected by different phytopathogens that can cause losses in production or render products unviable for export (Ferrigo et al., 2016; Guarnaccia et al., 2017; Ference et al., 2018).
The production of citrus is an important agricultural activity, and in Brazil it occupies a relevant position in the production of fruit, both for the fresh fruit market and to produce concentrate orange juice (Carvalho et al., 2019; González-González et al., 2020). However, this important crop is affected by different pathogens, such as Colletotrichum abscissum, Phyllosticta citricarpa, Xanthomonas citri subsp. citri (Silva-junior et al., 2014; Guarnaccia et al., 2017; Behlau, 2021). The post-bloom fruit drop (PDF) is caused by C. abscissum and affects citrus crops at the blossom stage, causing petal lesions, and abscission of young fruits (De Menezes et al., 2014; Silva-junior et al., 2014). The pathogen P. citricarpa causes the citrus black spot (CBS) disease, which affects fruits and leaves of citrus, and cause early fruit drop (Glienke et al., 2011; Guarnaccia et al., 2019; Hendricks et al., 2020). Moreover, citrus canker, caused by X. citri subsp. citri, is a major bacterial disease affecting citrus production in Brazil (Ference et al., 2018).
Maize production stands out in Brazilian agriculture. The country is the third largest global producer and the second largest maize exporter (Allen and Valdes, 2016). However, several species within the Fusarium graminearum species complex are the cause of major diseases in maize crops including F. graminearum, an important phytopathogen in maize crops, that frequently infects cobs and stalks of maize (Zea mays L.) causing the disease fusarium head blight (FHB). This pathogen results in visibly damaged kernels that can lower the grade, and, therefore, the value of the harvested crop (Ferrigo et al., 2016; Walder et al., 2017; Pfordt et al., 2020).
The control or prevention of pathogens is mainly based on the application of synthetic agrochemicals and the use of cultural practices. Although agrochemicals used represent 50% of citrus production costs, control of citrus pathogens is often unsatisfactory (Agostini et al., 2006; Qin et al., 2016; Schreuder et al., 2018; Guarnaccia et al., 2019; Hendricks et al., 2020), and the presence of agrochemicals residues may limit the export of these products (Food and Drug Administration, 2012). The continuous use of agrochemicals also led to an increase in the occurrence and selection of resistant fungal pathogens (Rodrigues et al., 2007; Deising et al., 2008; Hendricks et al., 2013). In addition, synthetic agrochemicals also led to concerns about environmental contamination and human health (Dayan et al., 2009; Kim et al., 2018). In this context, the discovery of new and safer compounds with activity against citrus and maize pathogens is desired.
Potential sources of new secondary metabolites are endophytic fungi that can produce chemically diverse compounds with a broad range of bioactivities (Aly et al., 2011; Tan et al., 2018). Endophytes are microorganisms that reside within the internal tissues of living plants, without visibly harming the host (Quiroga et al., 2001; Hardoim et al., 2015). These microorganisms, when associated with medicinal plants, possess high potential to produce new bioactive metabolites (Savi et al., 2018, 2019a; Singh et al., 2021). Microorganisms have been regarded as a promising source with the most potential for structurally novel antifungal metabolites because of the isolation of many natural fungicides from the microbial resource (Copping and Duke, 2007; Pham et al., 2019).
Among the endophytic fungi, the genus Diaporthe has been isolated from different host plants, and is reported as great source of compounds, especially with antibacterial and antifungal activities (Gomes et al., 2013; Santos et al., 2016; Noriler et al., 2019). Diaporthe species are commonly isolated from medicinal plants, found in a range of biomes and recent studies have demonstrated antifungal activity of Diaporthe endophytes isolated from medicinal plants against citrus phytopathogens such as C. abscissum and P. citricarpa (Tonial et al., 2017; Noriler et al., 2018, 2019; Savi et al., 2020). However, only a small fraction of the approximately one million known endophytes have been investigated (Savi et al., 2019a; Manganyi and Ateba, 2020). In addition, several rare medicinal plants produce important bioactive compounds to survive in unique environments and may host new and diverse fungal endophytes (Strobel, 2003), which have rarely been isolated and characterized (Tan et al., 2018).
The Cerrado (savanna) and Pantanal (wetland) are recognized as Brazilian biomes with high diversity of species (Myers et al., 2000; Strassburg et al., 2017; Guerreiro et al., 2019). Stryphnodendron adstringens (Martius) Coville (Forero, 1972) is a native plant in the Brazilian savanna, recognized by medicinal properties and was added in the “Plants for the Future Initiative Midwest region,” an action of the Brazilian Ministry of the Environment (Souza-Moreira et al., 2018; Pellenz et al., 2019; de Souza et al., 2020). This species has been harbored a richness endophytic fungi community with biotechnological potential (Carvalho et al., 2012; Noriler et al., 2018). Additionally, Vochysia divergens Pohl (Vochysiaceae) is one of the main medicinal tree species in the Pantanal region (de Carvalho Filho et al., 2021). Endophytic fungi reported by V. divergens have been showed antimicrobial, antifungal and cytotoxicity activities (Hokama et al., 2016; Savi et al., 2018; Noriler et al., 2018, 2019). Considering the need for more effective treatments of plant diseases caused by citrus and maize pathogens, the present study identified fungal endophytes of two medicinal plants, V. divergens and S. adstringens, and investigated their antimicrobial potential against the citrus pathogens C. abscissum, P. citricarpa, and X. citri subsp. citri, as well as against the maize pathogen F. graminearum.
Materials and Methods
Biological Material
Pathogens Cultures
The pathogens C. abscissum (CMRP704 – Ca142), P. citricarpa (CMRP06) and F. graminearum (LGMF1703) are deposited in the CMRP Taxonline Microbiological Collections of Paraná Network, at the Federal University of Paraná, Brazil1 and X. citri subsp. citri (X. citri; isolate 306 – IBSBF 1594) deposited in the IBSBF (culture collection of the Biological Institute Culture Collection of Phytopathogenic Bacteria).
Plants
The leaves and petioles of V. divergens and S. adstringens used for the isolation of endophytes were collected in January 2018 from two Brazilian regions: the Pantanal that is a wetland characterized by periods of flood and drought (Ivory et al., 2019), and the Cerrado that is a savannah characterized by the natural presence of fire (Strassburg et al., 2017). The leaves and petioles of V. divergens were collected in the Miranda River of the Pantanal (19°36′35″S 56°58′35″W) and on the Red River in Corumbá, Mato Grosso do Sul, Brazil. S. adstringens leaves and petioles were collected in the Cerrado along the BR262 (20°18′10.8″S 56°15′44.3″W). Samples were collected from 20 plants of S. adstringens and 20 plants of V. divergens. For both species, five leaves and five petioles were collected of each individual plant. The plant tissues were stored at 4°C and the isolation of endophytes was performed within 72 h.
Isolation of Endophytes
The leaves and petioles used for the isolation of endophytic fungi were processed using the protocol described by Petrini (1986), with modifications: the leaves and petioles were submerged in autoclaved water for 1 min, immersion in 70% ethanol (v/v) for 1 min, then for 3 min in sodium hypochlorite 3% (v/v), then for 1 min in 70% ethanol (v/v), and then, washed in sterilized distilled water for 1 min. After surface sterilization, the samples were cut into five pieces of 8 × 8 mm and aseptically transferred to Petri dishes containing potato dextrose agar (PDA) supplemented with nalidixic acid (50 μg/mL), at pH 5.8. The Petri dishes were incubated at 28°C for 30 days. The growth of endophytes was checked daily, and emerging mycelia were transferred to culture tubes containing PDA for further identification. To test the efficacy of surface disinfection method the last rinsed distilled water was collected and plated on the PDA plates and incubated at 28°C. The absence of fungal colonies growth from the last rinsed water plates indicates the efficacy of the surface sterilization procedure and confirming that the isolates were endophytes originated from within the host plant tissues.
After macroscopic characteristic analysis, the isolates were grouped into phenotypes according to colony color, growth rate, hyphal aspect, and presence/absence of spores. One isolate of each phenotype was randomly selected for molecular identification and bioprospecting. For each representative of the phenotypes, a pure culture was obtained from a single spore or hyphal tip according to Gilchrist-Saavedra et al. (2006).
DNA Extraction, PCR Amplification, Sequencing, and Phylogenetic Analyses
Genomic DNA was extracted from 3-day old cultures using the method described by Raeder and Broda (1985). PCR amplification of partial regions of ITS (internal transcribed spacer) using corresponding primer pairs V9G/ITS4 (White et al., 1990; De Hoog and Gerrits van den Ende, 1998). For the Diaporthe genus were also used partial sequences of beta-tubulin (tub2), translation elongation factor 1-α (tef1), calmodulin (cal), and histone H3 (his3) genes using corresponding primer pairs, e.g., Bt2a/Bt2b (Glass and Donaldson, 1995), EF1-728F/EF1-986R (Carbone and Kohn, 1999), CAL- 228F/CAL-737R (Carbone and Kohn, 1999), and CYLH3F/H3-1b (Glass and Donaldson, 1995; Crous et al., 2004), respectively. PCR reaction was performed using Top Taq Master Mix (QIAGEN), purified with the Exo1 and FastAP enzymes (Thermo Fisher Scientific, United States) and sequenced using the BigDye Terminator Cycle Sequencing Kit v 3.1 Kit. The products were purified with Sephadex G50 and submitted to an ABI3500® automated sequencer (Applied Biosystems, Foster City, CA, United States). Chromatograms were inspected using the MEGA 7 software (Kumar et al., 2015) and the consensus sequences were compared to those available in the NCBI/GenBank database (National Center for Biotechnology Information – http://www.ncbi.nlm.nih.gov/BLAST/) using the Blast tool, limiting to the sequences of the type strains.
The species found were also compared to the list of validated species of the MycoBank database2. The alignment was performed using the MAFFT version 7 (Katoh and Toh, 2008) and phylogeny based on Bayesian inference was performed in the MrBayes version 3.2.1 (Ronquist et al., 2011), using two parallel runs with one cold and three heated chains each, using the number of generations needed to reach split frequencies of ≤0.01 and a sampling frequency set to every 100 generations. The phylogenetic analyzes of Diaporthe genus were performed following the clades described by Gomes et al. (2013); Gao et al. (2017); Guarnaccia et al. (2018); Yang et al. (2018); Manawasinghe et al. (2019); Guo et al. (2020), and Hilário et al. (2021). In the present study we analyzed 173 Diaporthe isolates (Supplementary Table 1) (eight endophytic isolates from this study).
All the identified isolates were deposited in the CMRP Taxonline Microbiological Collections of Paraná Network (see text footnote 1) at the Federal University of Paraná (UFPR).
Taxonomy
The isolate CMRP4331 was cultivated in different culture media: PDA, oatmeal agar (OA), and 2% malt extract agar (MEA) at 25°C in darkness and colony diameters were measured 14 days after inoculation (Crous et al., 2009). Colony colors were rated according to Rayner (1970). To induce sporulation, the isolates were cultured on 2% tap water agar supplemented with sterile pine needles (PNA). Cultures were incubated at 25°C with a 12/12 h fluorescent light/dark cycle. 50 alpha conidia and 36 beta conidia were measured to calculate the mean size and standard deviation (SD). Moreover, the morphological characteristics were examined captured using an Olympus microscope equipped with an SC30 camera. Descriptions were deposited in the MycoBank database3.
Antifungal Activity
Production of Extracts
A total of 159 isolates representing each phenotype were used to prepare the extracts. The isolates were cultured for 7 days on PDA dishes, pH 5.8 at 28°C. Three mycelial disks (1.2 cm) of endophytes were inoculated into Erlenmeyer flasks (250 mL) containing 100 mL ME (Malt extract; Schulz et al., 1995) and cultured under agitation (180 rpm, 28°C) for 14 days. The mycelium was separated from the culture medium by filtration with Whatman n°4 filter paper. The fermentative liquid was mixed with 4% (w/v) XAD-16 resin and stirred overnight, followed by centrifugation. The resin was washed with distilled water (three times) and then extracted with MeOH (three times). The obtained methanol extract was dried using rotary evaporator at 40°C. The generated dry extracts of 159 isolates were diluted in methanol to a final concentration of 10 mg/mL (Savi et al., 2020).
Evaluation of Mycelial Growth Inhibition Against Pathogenic Fungi
The first screening of antifungal activity was evaluated against C. abscissum. 100 μL of the extract was added to a Petri dish with PDA medium, and a pathogen mycelial disk (8 mm) was subsequently placed in the center of the plate. The fungicide Carbendazim (Derosal®; 1.0 mg/mL) and methanol were used as positive and negative controls, respectively. The plates were incubated in BOD (Biochemical Oxygen Demand) at 24°C for 7 days. The inhibition of pathogen growth caused by each extract was evaluated by comparing the treatments with the controls (Savi et al., 2015, 2020; Hokama et al., 2016). The experiment was performed in triplicate and the data were submitted to Mann Whitney test in GraphPad Prims v. 6.01. The percentage of inhibition (Quiroga et al., 2001) was calculated with the following formula: Pi = (Cd – Td)/Cd, where: Pi = Percent inhibition; Cd = control growth diameter; Td = treatment growth diameter.
The extracts that showed the elite results in inhibiting the growth of the pathogen C. abscissum were selected for all other tests, including the evaluation of mycelial growth inhibition of P. citricarpa and F. graminearum that followed the same experimental design. The growth inhibition rates were analyzed 7 and 21 days after inoculation for F. graminearum and P. citricarpa, respectively. The experiments were performed in triplicate.
Determination of the Minimum Inhibitory Concentration for Pathogenic Fungi
The minimum inhibitory concentrations (MIC) analyzes were performed adapted from Tonial et al. (2017). MIC was determined in 96-well plates, with 100 μL malt extract medium in each well (C. abscissum and P. citricarpa). Potato dextrose medium was used for F. graminearum. 50 μL of the extract were pipetted into the adjacent well for serial dilutions, and 10 μL of conidial suspension of the pathogens C. abscissum, P. citricarpa, and F. graminearum (106 conidia/mL) were added in each well. The experiments were performed in triplicate and the plates were incubated at 28°C for 14 days. The MIC was determined as the lowest concentration of the extract capable of completely inhibiting pathogen growth (Hörner et al., 2008).
Determination of the Minimum Inhibitory Concentration for Pathogenic Bacteria
To perform tests against X. citri subsp. citri, this pathogen was cultivated in NYG/NYG-agar medium (nitrogen-yeast-glycerol: 5 gL–1 of peptone, 3 gL–1 of yeast extract, 2% glycerol; solid medium bacterial agar was added to 15 gL–1) at 29°C (Zamuner et al., 2020). The inhibitory concentration values (IC) of the extracts for X. citri were determined using the Resazurin Microtiter Assay Plate (REMA) adapted by Silva et al. (2013). Stock solutions were prepared by dissolving the extracts in NYG medium containing 1% DMSO (v/v) to the final concentration of 100 μg/mL. Exposure of the cells was done in 96-multiwell plates, in which each of the extracts was serially diluted per column of the plate (example: A1-H1, A2-H2, etc.) using NYG medium as the diluent to the resulting concentration range varying from 100 to 0.78 μg/mL. Cells of X. citri were cultivated in NYG from the starting OD600 nm of ∼0.1 until the OD600 nm of ∼0.40 (which contains 107 cells/mL). A fraction of this culture was added to each of the wells in order to give the final concentration of 105 cells/well in a total volume of 100 μL per well. The negative control was NYG medium without any extract, whereas the positive control was kanamycin at 20 μg/mL. The control of vehicle was 1% DMSO (v/v) mixed in the NYG medium. The REMA plates were incubated for 16 h at 29 ± 1°C. Development was performed by adding 15 μL of 0.1 mg/mL resazurin (Sigma-Aldrich, Germany) solution into each well, followed by 2-h incubation at 29 ± 1°C. Cell respiration was monitored by the fluorescence of resorufin (the reduced form of resazurin in the presence of NADH) using the BioTek plate reader Synergy H1N1 set to the excitation and emission wavelengths of 530 and 590 nm, respectively. The percentages of cell growth inhibition per extract concentration were plotted using the software Microcal Origin 8.0, and polynomial regression was used to estimate IC values (concentrations of extracts able to inhibit 100% of the cells in culture; Morão et al., 2018). To determine if an extract has bactericidal or bacteriostatic effect, aliquots from REMA were transferred to NYG-agar plates before resazurin was added by using a 96-plate replicator (8 × 12 Sigma-Aldrich, Germany). Plates were incubated at 29°C for 48 h to verify the ability of the treated cells to resume growth. Three independent experiments were performed in triplicates each.
Inhibition of Post-Bloom Fruit Drop Development in Citrus Flowers
To evaluate the ability of 12 extracts into inhibit the PFD development, citrus flowers were collected at the UFPR, Curitiba, Paraná, Brazil. Flowers without PFD symptoms were added in Petri dishes containing water agar medium (15 g of agar per 1 L of deionized H2O). In a petal of each flower were added 5 μL of endophyte extracts and 5 μL of conidial suspension of C. abscissum (106 conidia/mL). Methanol was used as negative control and fungicide Carbendazim (Derosal®; 1.0 mg/mL) was the positive control. The dishes were kept at 28°C for 48 h, and the qualitative analysis was performed. The presence of PFD symptoms in the flower was considered a negative activity against PFD, and the absence of symptoms was considered a positive activity of extract. The test was performed in triplicate (Savi et al., 2020).
Inhibition of Phyllosticta citricarpa Pycnidia Development in Citrus Leaves
To evaluate the potential of 12 extracts to inhibit the development of P. citricarpa pycnidia, autoclaved Citrus sinensis leaves were used (20 min; 120°C; 1 atm). Leaves disks (Ø 10 mm) with 10 μL of the extract were placed on Petri dishes with water agar medium. Four disks of 2 mm P. citricarpa mycelia were inoculated close to each leaf fragment. Methanol was used as negative control and fungicide Carbendazim (Derosal®; 1.0 mg/mL) was the positive control. The Petri dishes were maintained for 21 days at 28°C with 12 h photoperiod. The pycnidia of P. citricarpa formed above the leaves were counted under a stereoscopic microscope. This test was performed in triplicate (Santos et al., 2016).
General Experimental Procedures
High performance liquid chromatography, UV detection, and mass spectrometry (HPLC-UV/MS) analyses were accomplished with an Agilent Infinity Lab LC/MSD mass spectrometer (MS Model G6125B; Agilent Technologies, Santa Clara, CA, United States) equipped with an Agilent 1260 Infinity II Series Quaternary LC system and a Phenomenex NX-C18 column (250 × 4.6 mm, 5 μm) [Method: solvent A: H2O/0.1% formic acid, solvent B: CH3CN; flow rate: 0.5 mL/min; 0–30 min, 5–100% B (linear gradient); 30–35 min, 100% B; 35–36 min, 100–5% B; 36–40 min, 5% B]. Thin layer chromatography (TLC) was carried out using Polygram SIL G/UV254 (Macherey-Nagel and Co., Dueren, Germany).
Results
Phenotypes and Screening of the Antifungal Activity
A high number (1,304) of cultivable endophytic fungi were isolated from V. divergens and S. adstringens, and the isolates were grouped into 159 phenotypes based on morphological characteristics. The antifungal activity of the extracts produced from the fermentation of the 159 representative isolates (one of each phenotype) was determined (Supplementary Table 2). Twelve endophytic fungi produced metabolites that inhibited mycelial growth (IG) of the pathogen C. abscissum equal to or greater than 40%: CMRP4328 – IG: 83%, CMRP4323 – IG: 80%, CMRP4322 – IG: 75.3%, CMRP4325 – IG: 72.6%, CMRP4330 – IG: 71.3%, CMRP4332 – IG: 65.3%, CMRP4327 – IG: 51.3%, CMRP4324 – IG: 48.6%, CMRP4321 – IG: 46%, CMRP4326 – IG: 46%, CMRP4329 – IG: 40% and CMRP4331 – IG: 40% (Supplementary Table 2, Figure 1, and Supplementary Figures 1, 2).
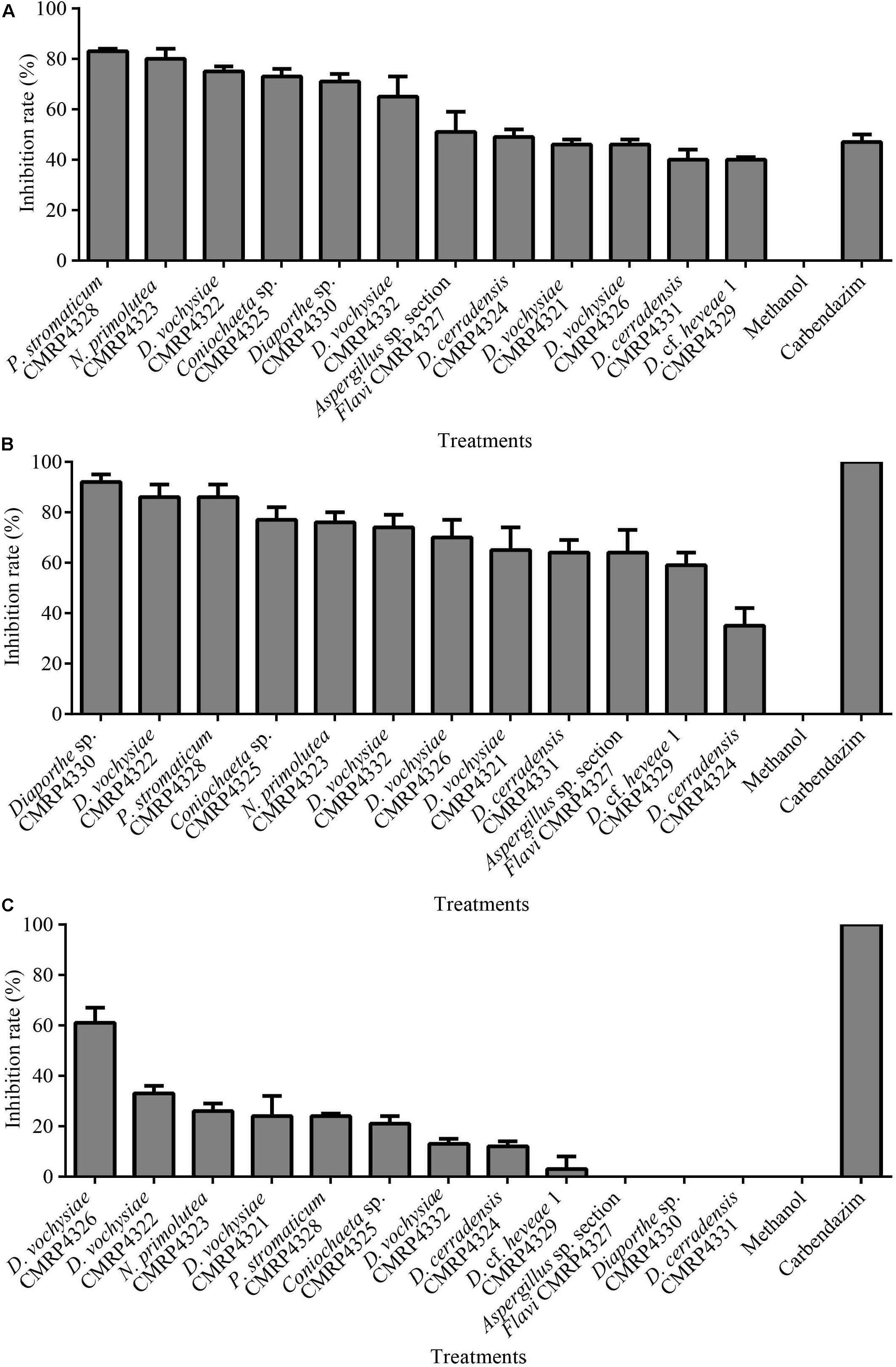
Figure 1. Mean values of mycelial growth inhibition (in%) of the pathogens Colletotrichum abscissum (A), Phyllosticta citricarpa (B), and Fusarium graminearum (C) in the presence of 100 μL of extracts obtained after culture of endophytes in malt extract medium. Note: Fungicide: 100 μL Carbendazim (Derosal; 0.1 mg/mL) was used as positive control.
Of the 12 isolates selected, seven were isolated from plants of S. adstringens (six from leaves and one from a petiole) located in the Cerrado biome, and five isolates were obtained from plants of V. divergens (two from leaves and three from petioles) of the Pantanal biome in Brazil (Supplementary Table 3).
Identification of Isolates
The 12 isolates were identified based on phylogenetic analysis using ITS (in addition to other loci) sequences as belonging to the Ascomycota phylum, with ten isolates belonging to the Sordariomycetes class, one to Eurotiomycetes and one to the Dothideomycetes class. Diaporthe was the genus with the highest number of isolates with antifungal potential, represented by eight isolates (Supplementary Table 3): the isolates CMRP4321, CMRP4322, CMRP4326, and CMRP4332 (D. sojae complex; Supplementary Figure 3), the CMRP4329 isolate (Supplementary Figure 4), the isolate CMRP4330 (D. arecae complex; Supplementary Figure 5) and CMRP4324 and CMRP4331 isolates (Figure 2). The other isolates were identified as Aspergillus section Flavi A. nomius-clade (CMRP4327; Supplementary Figure 6), Coniochaeta sp. (CMRP4325; Supplementary Figure 7), Nemania primolutea (CMRP4323; Supplementary Figure 8), and Pseudofusicoccum stromaticum (CMRP4328; Supplementary Figure 9 and Supplementary Table 4).
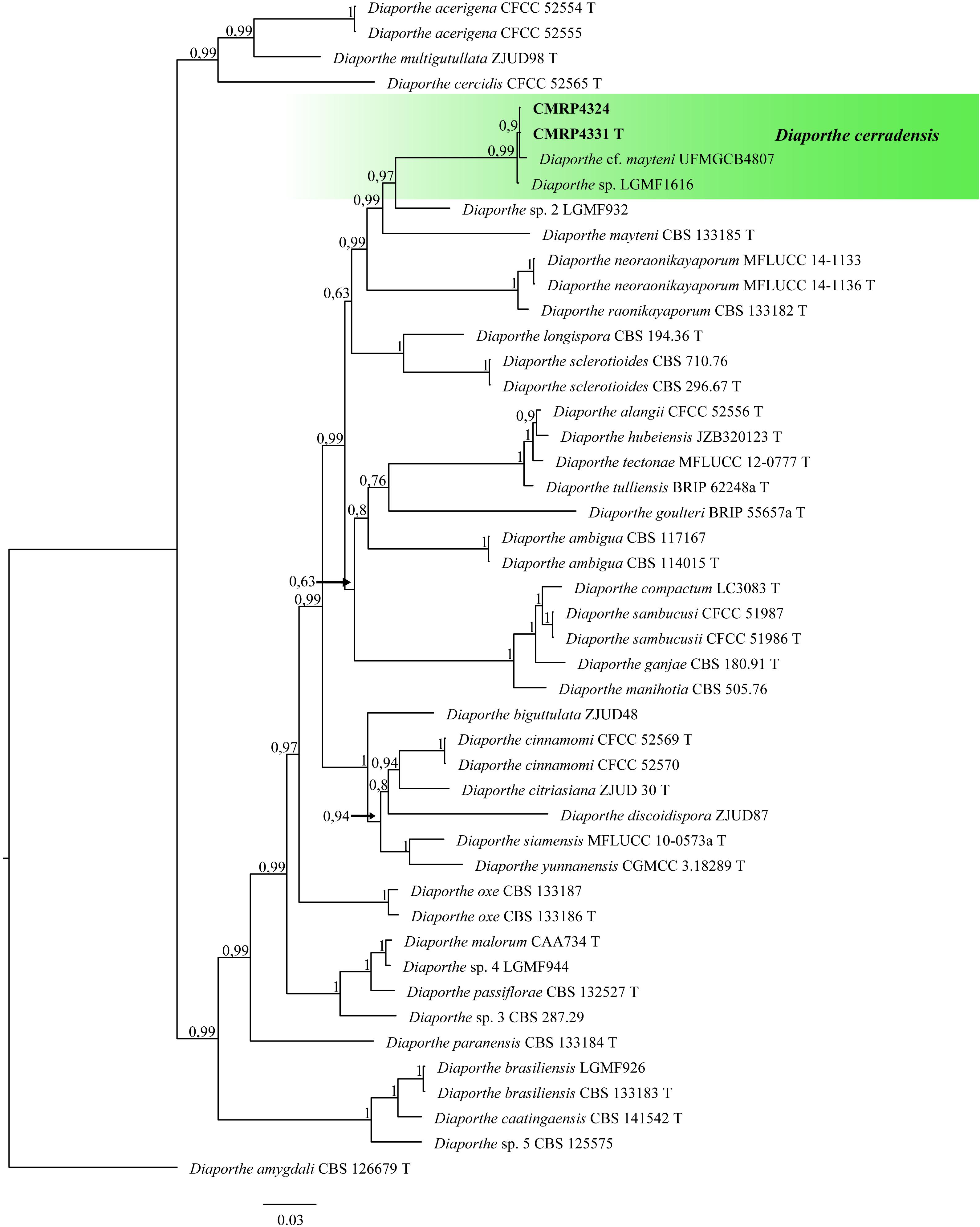
Figure 2. Bayesian Inference phylogenetic tree of Diaporthe species based on multiple alignment of ITS, tub2, tef1, his3, and cal partial sequences. The data matrix had 47 taxa and 2,133 characters. The species Diaporthe amygdali (CBS 126679 T) was used as outgroup. Strains marked with a “T” correspond to type sequences. The scale bar of 0.03 represents the number of changes. The sequence of the isolates here studied is presented with its isolation code (CMRP4331 and CMRP4324) highlighted in bold.
Phylogenetic Analyses
Diaporthe genus
Diaporthe vochysiae (CMRP4321, CMRP4322, CMRP4326, and CMRP4332)
In the D. sojae species complex, 60 representatives of the complex including the outgroup sequences of Diaporthe amygdali (CBS126679) were subjected to multi-locus phylogenetic analyses with concatenated ITS, tub2, tef1, his3, and cal sequences. A total of 2,439 characters including gaps (593 for ITS, 442 for tub2, 369 for tef1, 516 for his3, and 519 for cal) were used in the phylogenetic analysis, 647 characters were parsimony-informative, 921 were variable and 1453 characters were conserved.
In this clade, the isolates CMRP4321, CMRP4322, CMRP4326, and CMRP4332 were closely related to the type strain of D. vochysiae (Supplementary Figure 3), and therefore, we suggest them as belonging to this species.
Diaporthe cf. heveae 1 (CMRP4329)
The multi-locus phylogenetic analyses with 29 representative isolates including the outgroup sequences of D. perjuncta (CBS 114435 T). A total of 2,160 characters including gaps (525 for ITS, 396 for tub2, 326 for tef1, 451 for his3, and 459 for cal) with 620 characters being parsimony-informative, 792 were variable, and 1,315 characters were conserved. The isolate CMRP4329 was assigned as Diaporthe cf. heveae 1 (Supplementary Figure 4).
D. arecae species complex (CMRP4330)
The isolate CMRP4330 was aligned with 49 representative isolates from D. arecae species complex including D. caulivora (CBS 127268 T) as outgroup. Were analyzed and 2,112 characters including gaps (517 for ITS, 340 for tub2, 323 for tef1, 457 for his3, and 475 for cal) were included in the multi-locus dataset, 474 characters were parsimony-informative, 721 were variable, and 1,361 characters were conserved. The CMRP4330 isolate was positioned on a single branch and, therefore, we suggest that this isolate belongs to a new species of the genus Diaporthe, named Diaporthe sp. (Supplementary Figure 5), since all attempts to produce are productive structure have failed.
Diaporthe cerradensis (CMRP4331 and CMRP4324)
The phylogenetic tree to D. cerradensis was obtained with 47 representative isolates including the outgroup sequences of D. amygdali (CBS 126679 T), a total of 2,133 characters including gaps (505 for ITS, 399 for tub2, 356 for tef1, 469 for his3, and 404 for cal). 761 characters were parsimony-informative, 926 were variable, and 1,157 characters were conserved. Based on these multi-locus phylogenic analyses, the isolates CMRP4324 and CMRP4331 were described as a new species and named D. cerradensis (Figure 2). In addition to these, the results for the first 10 megablast sequences using GenBank4 are in Supplementary Tables 5–9. The highest similarity was for the isolate Diaporthe cf. mayteni for the partial tef1 (99.69%) and tub (99.59%). There are no his and cal sequences for Diaporthe cf. mayteni. Two endophytic strains were clustered in the same clade of D. cerradensis, the strain Diaporthe cf. mayteni UFMGCB4807 isolated from a leaf of Carapa guianensis (Ferreira et al., 2015) and Diaporthe sp. LGMF1616 isolated from a leaf of S. adstringens (Noriler et al., 2018), both also isolated in the Cerrado biome, Brazil. Below, we describe these new species.
Species Description
Diaporthe cerradensis CMRP4331
Diaporthe cerradensis: Iantas, Noriler & Glienke, sp. nov. MycoBank 839350;
Etymology: Named after the region where the strain was collected (Cerrado, Brazilian biome)
Pycnidial conidiomata globose, conical or irregular, solitary or aggregated, exposed on the PNA surface, dark brown to black, cream translucent conidial drops exuded from the ostioles, 170–350 μm diameter. Conidiophores hyaline, densely aggregated, unbranched, aseptate, subcylindrical to cylindrical, 4.5–6.5 × 2–4.3 μm. Conidiogenous cells hyaline and subcylindrical, tapering toward the apex 12.2–15.1 × 2–3.5 μm. Alpha conidia common, hyaline, fusiform, biguttulate, 6.4–8.3 × 2–3 μm, mean ± SD = 7.32 ± 0.38 × 2.31 ± 0.18 (n = 50). Beta conidia less common hyaline, aseptate, filiform, curved 18.8–29.6 × 1–1.6 μm, mean ± SD = 24.8 ± 2.7 × 1.4 ± 0.1 μm (n = 36). Gamma conidia not observed.
Culture characteristics: Colonies on flat PDA, aerial mycelium forming concentric rings with cotton texture, white and light yellow on the surface, colonies reaching 72 mm in diameter after 2 weeks at 25°C; reverse yellow amber. In flat OA, with a white aerial mycelium, olive gray on the surface, colonies reaching 65 mm in diameter; reverse white, gray and greenish olivaceous. In the flat MEA, aerial white mycelium and amber patches on the surface, colonies reaching 52 mm diameter; reverse brown (Figure 3).
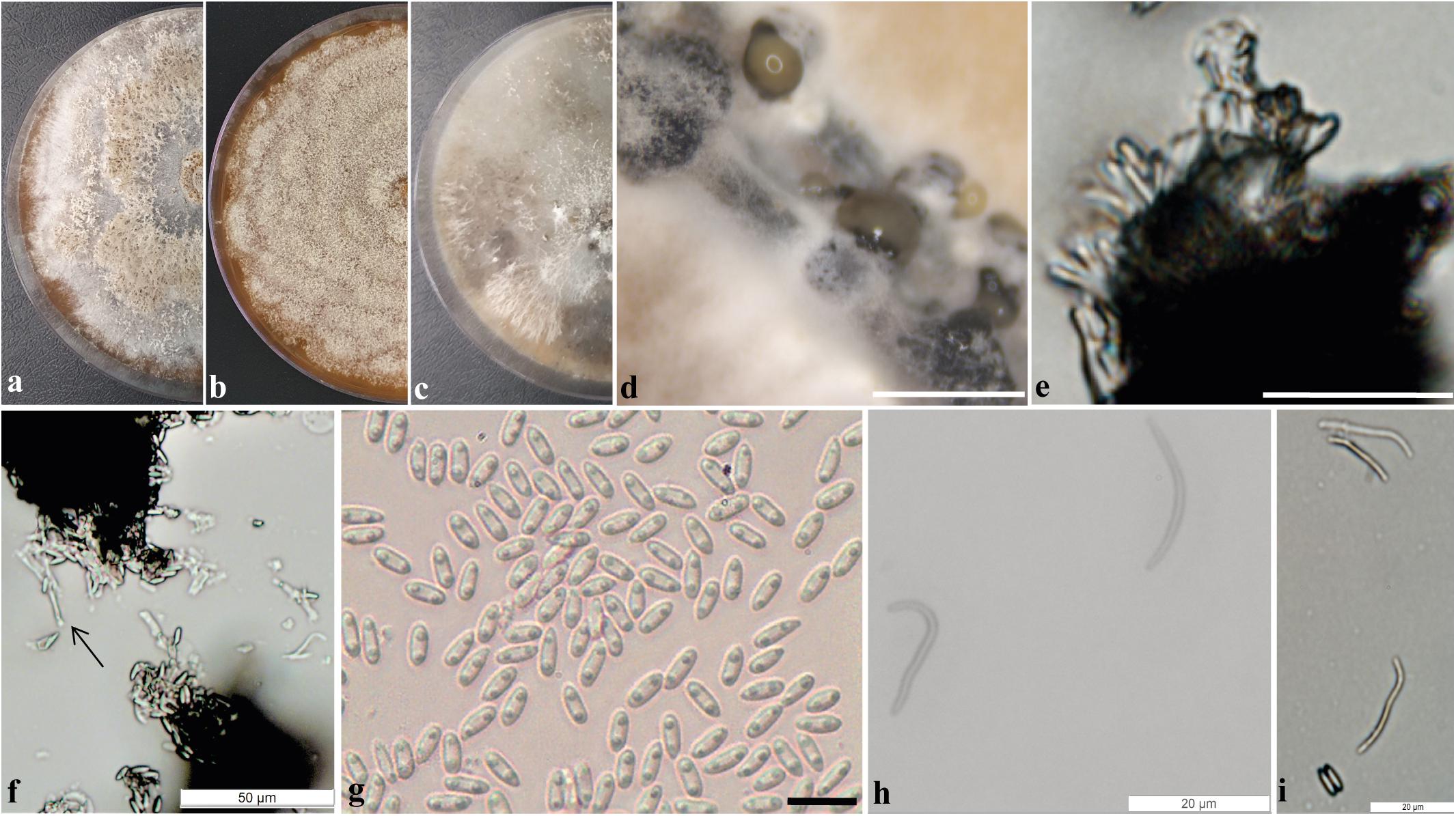
Figure 3. Diaporthe cerradensis (CMRP4331). (a–c) Colonies on PDA, MEA, and OA, respectively; (d) conidiomata sporulating on PNA; (e) conidiophores; (f) conidiogenous cells; (g) alpha conidia; (h) beta conidia; and (i) alpha and beta conidia. — Scale bars: d = 200 μm; e = 50 μm; and g = 10 μm.
Specimen examined: Brazil, Miranda, Cerrado, Mato Grosso do Sul, 20°18′10.8″S, 56°15′44.3″W, endophytic species isolated from a leaf of S. adstringens (popular name Barbatimão), January 05, 2018 by C. Glienke and D. C. Savi. Holotype: UPCB97125 (Herbarium of the Department of Botany code, University Federal of Paraná), ex-type culture CMRP4331 CMRP – Taxonline Microbiological Collections of Paraná Network (see text footnote 1) at the UFPR.
Notes: Diaporthe cerradensis forms a well-supported and independent clade distinct from the previously described species of Diaporthe. D. cerradensis is most closely related to the species D. mayteni, D. raonikayaporum, and D. neoraonikayaporum. However, D. cerradensis differs from these species in the ITS sequences (36 different unique fixed alleles by D. mayteni, 30 by D. raonikayaporum, and 29 by D. neoraonikayaporum), tef1 (27 different unique fixed alleles by D. mayteni, 45 by D. raonikayaporum, and 41 by D. neoraonikayaporum), tub2 (19 different unique fixed alleles by D. mayteni, 26 by D. raonikayaporum, 23 by D. neoraonikayaporum), his3 (30 different unique fixed alleles by D. mayteni and 40 by D. raonikayaporum), and cal (21 different unique fixed alleles by D. mayteni, 28 by D. raonikayaporum, 26 by D. neoraonikayaporum) loci. Furthermore, D. cerradensis differs from D. mayteni in having larger conidiogenous cells (12.2–15.1 × 2–3.5 vs 10–13 × 2–3 μm) and alpha conidia (6.4–8.3 × 2–3 vs 5–7 × 2–3 μm; Gomes et al., 2013). Conidiogenous cells of D. cerradensis are also larger than those of D. raonikayaporum (12.2–15.1 × 2–3.5 vs 5–10 × 2–3 μm; Gomes et al., 2013). In addition, alpha conidia of D. cerradensis are larger than those of D. neoraonikayaporum (6.4–8.3 × 2–3 vs 4–6 × 1.4–1.9 μm; Doilom et al., 2017).
Aspergillus sp. section Flavi A. nomius-clade (CMRP4327)
The isolate CMRP4327 was aligned with 54 sequences of 37 accepted species of the genus Aspergillus section Flavi, and Aspergillus muricatus (NRRL35674 T) was used as the outgroup. The alignment of the partial sequences of ITS consisted of 610 characters, 426 of these were conserved, 83 were parsimony informative, and 161 were uninformative. In the phylogenetic tree, the isolate CMRP4327 is closely related to the species A. nomius and A. pseudonomius (Supplementary Figure 6) and therefore, was denominated as belonging to the A. nomius clade.
Coniochaeta sp. (CMRP4325)
The phylogenetic analysis comprises the isolate CMRP4325, 35 species accepted in the Coniochaeta genus, and Cheatosphaeria garethjonessi MFLU161019 that was used as outgroup. The alignment of partial sequence of the ITS consisted of 612 characters, of which 331 were conserved, 171 were parsimony informative, and 261 uninformative. In the phylogenetic analysis, the isolate CMRP4325 is in the same branch as the Coniochaeta boothii species but seems to be a distinct form (Supplementary Figure 7). Multigene analysis will be necessary for the identification of this isolate.
Nemania primolutea (CMRP4323)
The alignment consisted of the isolate CMRP4323, 13 accepted species of the Nemania genus, and Biscogniauxia nummularia (MUCL51395) as the outgroup taxa. The ITS analysis comprises of 679 characters, which 322 were conserved, 175 were parsimony informative, and 290 were uninformative. Based on this phylogenetic analysis, the isolate CMRP4323 was identified as N. primolutea (Supplementary Figure 8).
Pseudofusicoccum stromaticum (CMRP4328)
The phylogenetic analysis was performed with the partial sequences of ITS and tef1 of the isolate CMRP4328 and 15 sequences of 9 accepted species of the Pseudofusicoccum genus and using Endomelanconiopsis microspora (CBS353.97) as the outgroup taxa. The alignment of the partial sequence of ITS consisted of 575 characters, with 491 conserved sites, 16 were parsimony informative, and 74 uninformative. The partial tef1 (Translation elongation factor 1 alpha) alignment consisted of 308 characters, with 211 conserved sites, 16 were parsimony informative, and 95 uninformative. In the phylogenetic tree the isolate CMRP4328 grouped with P. stromaticum and therefore, it was identified as belonging to this species (Supplementary Figure 9).
Biological Activity
Extracts From Endophytic Fungi Inhibit the Mycelial Growth and Germination of Conidia of Plant Pathogens
In the screening for antifungal activity among the 159 extracts evaluated, 12 extracts showed highest mycelium growth inhibition against C. abscissum (Figure 1A) and were selected to be evaluated for their activity against the pathogens P. citricarpa and F. graminearum. Seven extracts inhibited more than 70% of the mycelial growth of the pathogen P. citricarpa (Figure 1B): Diaporthe sp. CMRP4330 (IG: 92%, p = 0.01), P. stromaticum CMRP4328 (IG: 86%, p = 0.01), D. vochysiae CMRP4322 (IG: 86%, p = 0.01), Coniochaeta sp. CMRP4325 (IG: 77%, p = 0.05), N. primolutea CMRP4323 (IG: 76%, p = 0.05), D. vochysiae CMRP4332 (IG: 74%, p = 0.05), and D. vochysiae CMRP4326 (IG: 70%, p = 0.05). Against the maize pathogen F. graminearum the extract from D. vochysiae CMRP4326 inhibited 61% of mycelial growth (p = 0.01; Figure 1C). D. cerradensis CMRP4324 and CMRP4331 inhibited the mycelial growth of citrus pathogens C. abscissum (IG = 49 and 40%, respectively) and P. citricarpa (IG = 35 and 64%, respectively).
For the 12 selected extracts, we also evaluated the minimum concentrations that inhibit the germination of the conidia of the pathogens. The values are showed in Table 1, and the underlined values represent extracts that also showed evidenced activity in the inhibition of mycelium growth (Figure 1 and Supplementary Figure 2). The extracts produced by the isolates D. vochysiae CMRP4322 completely inhibited the conidial germination of C. abscissum with the MIC value 1 μg/mL, and other isolates such as N. primolutea CMRP4323 (MIC: 40 μg/mL), D. cerradensis CMRP4331 (MIC: 40 μg/mL), D. vochysiae CMRP4326 (MIC: 40 μg/mL), and P. stromaticum CMRP4328 (MIC: 40 μg/mL) showed the lowest MIC values against C. abscissum.
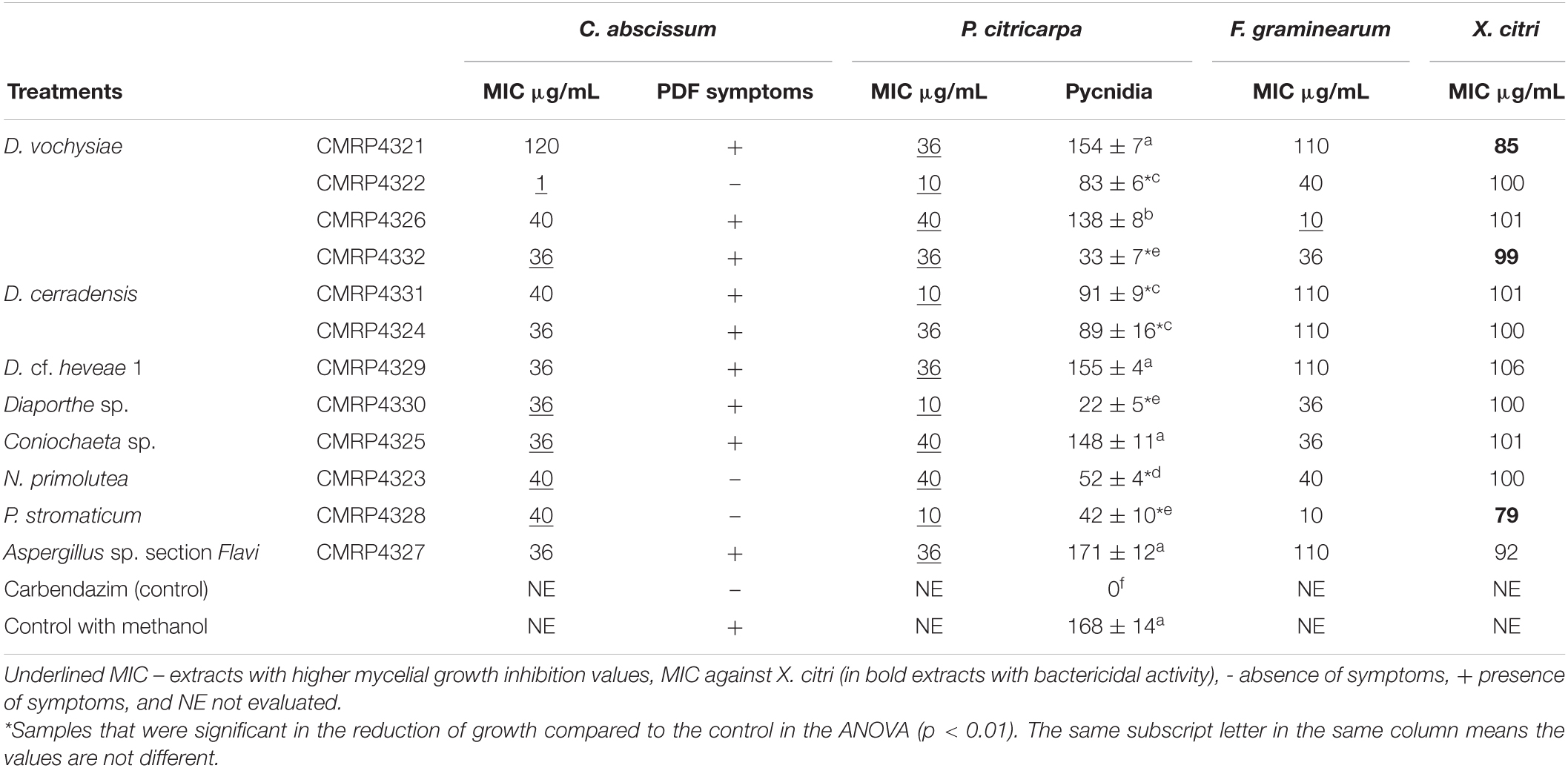
Table 1. Minimum inhibitory concentration (μg/mL) of extracts produced by the endophytes against the pathogens Colletotrichum abscissum, Phyllosticta citricarpa, Fusarium graminearum, and Xanthomonas citri subsp. citri (in bold extracts with bactericidal activity), post-bloom fruit drop (PFD) symptoms in the presence of extracts and number of pycnidia produced in leaves by P. citricarpa in the presence of extracts.
The lowest MIC values (10 μg/mL) that inhibit the germination of P. citricarpa conidia were produced by four isolates, including the new species D. cerradensis CMRP4331, and D. vochysiae CMRP4322, Diaporthe sp. CMRP4330, and P. stromaticum CMRP4328 (Table 1). The extracts of the isolates D. vochysiae CMRP4326 and P. stromaticum CMRP4328 inhibited the conidia germination of F. graminearum with MIC values of 10 μg/mL.
Extracts of Endophytic Fungi Have Activity Against Citrus Pathogenic Bacteria
Four extracts showed inhibitory activity against X. citri subsp. citri in which the IC values were below 100 μg/mL: P. stromaticum CMRP4328 (MIC: 79 μg/mL), D. vochysiae CMRP4321 (MIC: 85 μg/mL) Aspergillus sp. section Flavi (MIC: 92 μg/mL), and D. vochysiae CMRP4332 (MIC: 99 μg/mL). Extracts from D. vochysiae CMRP4321, P. stromaticum CMRP4328, and D. vochysiae CMRP4332 displayed bactericidal action (Table 1).
Inhibition of Post-Bloom Fruit Drop Development in Citrus Flowers
The ability of the 12 extracts, which showed great antifungal activity against C. abscissum, to inhibit the PFD symptoms in citrus flowers, was evaluated. This qualitative analysis assesses the presence or absence of symptoms of PFD (typical peach-orange necrotic lesions). After 48 h of inoculation, the extracts produced by D. vochysiae CMRP4322, N. primolutea CMRP4223, and P. stromaticum CMRP4328 completely inhibit symptoms in flowers (Supplementary Table 1 and Figure 4). The lesions observed in the flowers treated with the other extracts are similar to the typical PFD lesion shown in Figure 4a.
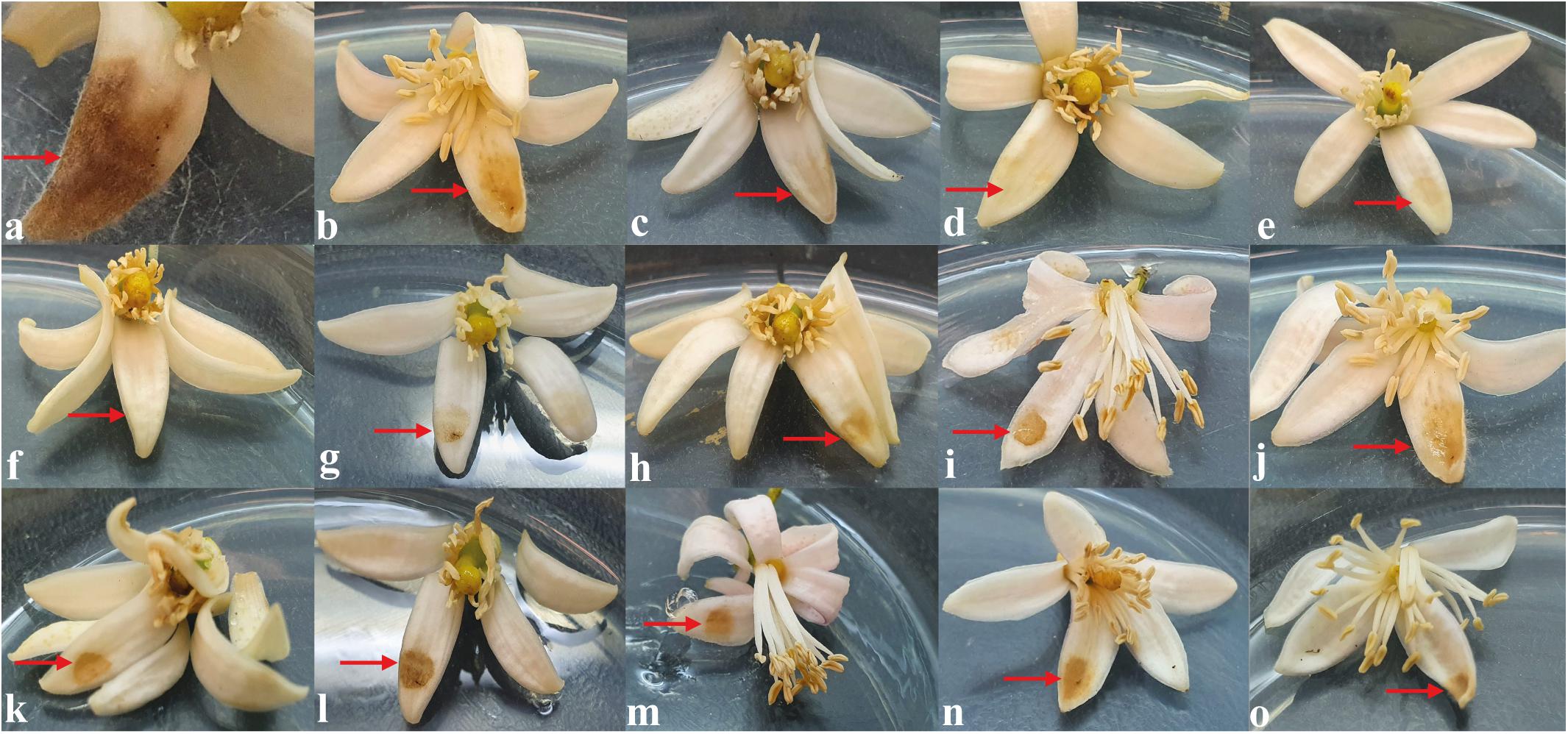
Figure 4. In vitro assay to evaluate the activity of the extracts to inhibit the development of post-bloom fruit drop (PFD) in citrus flowers, 48 h after inoculation with 5 μL of conidial suspension of Colletotrichum abscissum (106 conidia/mL; red arrows) added with: (a) 5 μL of saline solution (showing the characteristic symptom of PDF), (b) 5 μL of methanol (control), (c) 10 μL with carbendazim (Derosal – control), and 5 μL of each endophytic extracts: (d) Diaporthe vochysiae CMRP4322, (e) Nemania primolutea CMRP4323, (f) Pseudofusicoccum stromaticum CMRP4328, (g) D. vochysiae CMRP4321, (h) D. vochysiae CMRP4326, (i) D. vochysiae CMRP4332, (j) D. cerradensis CMRP4331, (k) D. cerradensis CMRP4324, (l) D. cf. heveae 1 CMRP4329, (m) Diaporthe sp. CMRP4330, (n) Coniochaeta sp. CMRP4325, and (o) Aspergillus sp. section Flavi CMRP4327.
Inhibition of P. citricarpa Pycnidia Development in Citrus Leaves
We also evaluated the ability of extracts to inhibit the formation of P. citricarpa pycnidia in citrus leaves, and five extracts were able to inhibit more than 50% of pycnidia production (Supplementary Table 1 and Figure 5), Diaporthe sp. CMRP4330 (86%; Figure 5d), D. vochysiae CMRP4332 (81%; Figure 5e), P. stromaticum CMRP4328 (75%; Figure 5f), and N. primolutea CMRP4323 (69%; Figure 5g). We highlight the extracts from strains Diaporthe sp. CMRP4330 and P. stromaticum CMRP4328 that were able to reduce pycnidia formation and the mycelial growth.
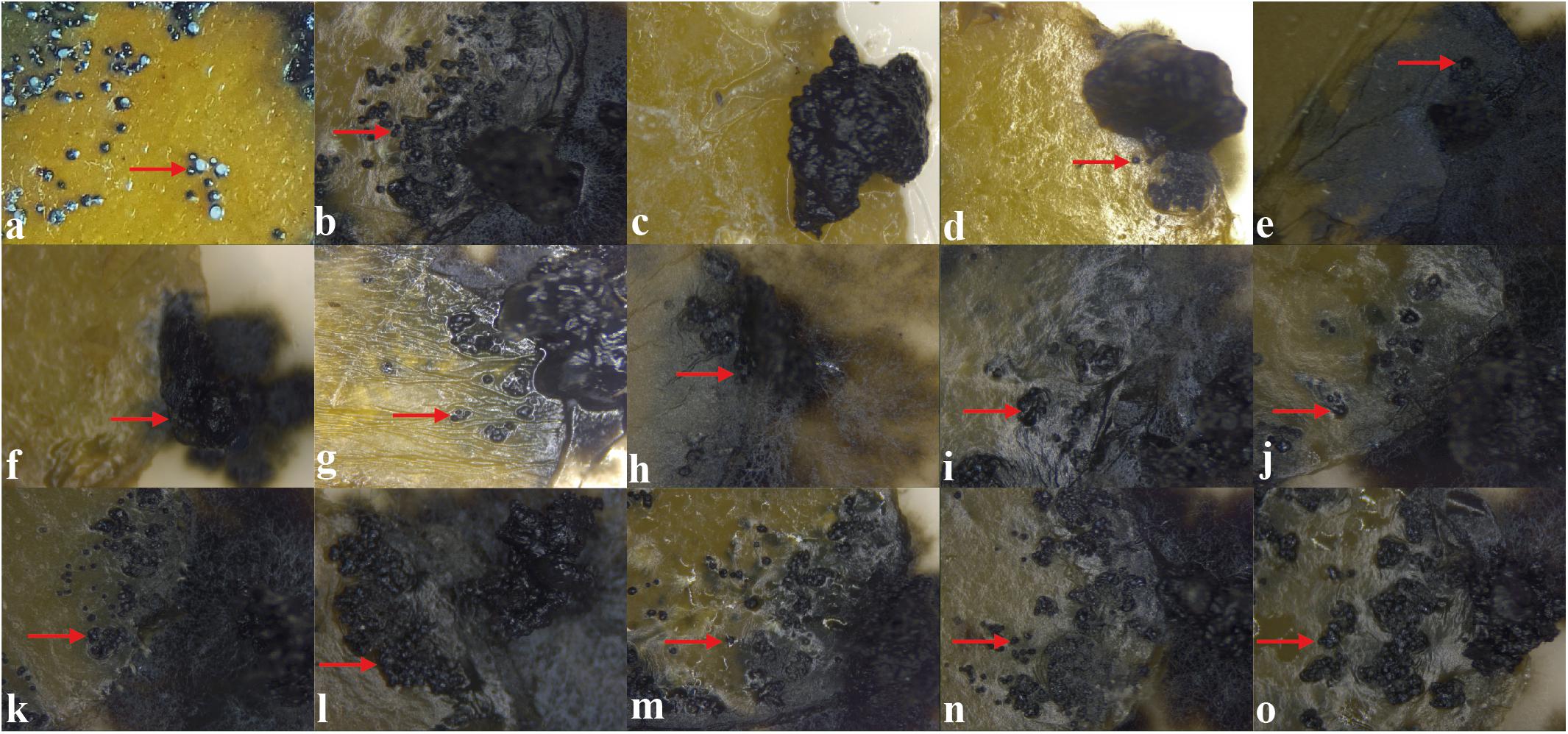
Figure 5. In vitro assay to evaluate the activity of the extracts to inhibit the development of pycnidia in citrus leaves, 21 days after the inoculation of mycelial disks of Phyllosticta citricarpa added with: (a) 10 μL of saline solution (showing characteristic pycnidia), (b) 10 μL of methanol (control), (c) 10 μL of carbendazim (Derosal; control), and 10 μL of each endophytic extracts: (d) Diaporthe sp. CMRP4330, (e) D. vochysiae CMRP4332, (f) Pseudofusicoccum stromaticum CMRP4328, (g) Nemania primolutea CMRP4323, (h) D. vochysiae CMRP4322, (i) D. cerradensis CMRP4324, (j) D. cerradensis CMRP4331, (k) D. vochysiae CMRP4326, (l) Coniochaeta sp. CMRP4325, (m) D. vochysiae CMRP4321, (n) D. cf. heveae 1 CMRP4329, and (o) Aspergillus sp. section Flavi CMRP4327. The red arrows represent the pycnidia formation.
HPLC-UV/MS and TLC Analyses of Selected Fungal Extracts
The bioactive extracts produced by the top interesting 12 endophytic fungi (D. vochysiae CMRP4321, CMRP4322, CMRP4326, and CMRP4332, D. cerradensis CMRP4324 and CMRP431, D. cf. heveae 1 CMRP4329, Diaporthe sp. CMRP4330, N. primolutea CMRP4323, Coniochaeta sp. CMRP4325, Aspergillus sp. section Flavi CMRP4327, and P. stromaticum CMRP4328) have been subjected for chemical screening (TLC and HPLC-UV/MS analyses) followed by metabolite data analysis. The extracts generated from the small-scale fermentations of these fungal strains have been dissolved in MeOH and subjected to HPLC-UV/MS and TLC analyses. The major class of compounds are aflatoxins and xanthones (Figure 6). The HPLC-UV/MS analyses of these extracts displayed several interesting UV/vis and MS peaks (Supplementary Figures 10–42). For example, HPLC-UV/MS analyses of the extracts produced by four strains (D. vochysiae CMRP4321, CMRP4322, and CMRP4332, and P. stromaticum CMRP4328) indicate the presence of several interesting compounds with similar UV/vis chromophores (Supplementary Figures 10–16, 30, 31, 38–41). In the same manner, the LCMS analysis of the fungus Coniochaeta sp. CMRP4325 extract showed two major peaks with molecular weights of 324 and 306 Daltons, respectively, with Δm/z = 18 Daltons difference, consistent with loss of water. AntiBase (Laatsch, 2017) search afforded no matched hits, which likely indicates two new fungal metabolites (Supplementary Figures 22, 23). The HPLC-UV/MS analysis of another fungus extract (Aspergillus sp. section Flavi CMRP4327) was very rich in terms of its metabolites (Supplementary Figures 26–29) and shows several peaks with similar UV/vis that matched with the aflatoxin class of compounds (Figure 6). The LC mass spectra of these peaks revealed three peaks with different retention times, but the same molecular weight (MW 338) as well as other major peaks with MW of 354, 312, and 422 Daltons. In addition, the TLC of the Aspergillus sp. section Flavi CMRP4327 extract (Supplementary Figure 42) displayed several blue-fluorescent UV bands at 254/365 nm. The extract of D. cf. heveae 1 CMRP4329 showed three major HPLC peaks with similar UV/vis chromophores, with a major peak of MS = 324 Daltons. No mass match for these compounds were found in an AntiBase search, indicating new compounds in this crude extract. The fungus strain Diaporthe sp. CMRP4330 displayed two major peaks (HPLC Rt = 20.96 and 21.19 min) with a similar UV/vis spectrum and the same molecular weight (MW 338; Supplementary Figure 34). An AntiBase search showed no hits matching the molecular weights and UV/vis of these two HPLC peaks, which indicates novelty of these two compounds. The generated extracts by the remaining fungal strains (N. primolutea CMRP4323, D. cerradensis CMRP4324 and CMRP4331, and D. vochysiae CMRP4326) did not show major peaks in the HPLC-MS analyses, however, they might contain some none-UV/vis bioactive compounds, or the extracts produced from these strains did not contain compound with good solubility in MeOH.
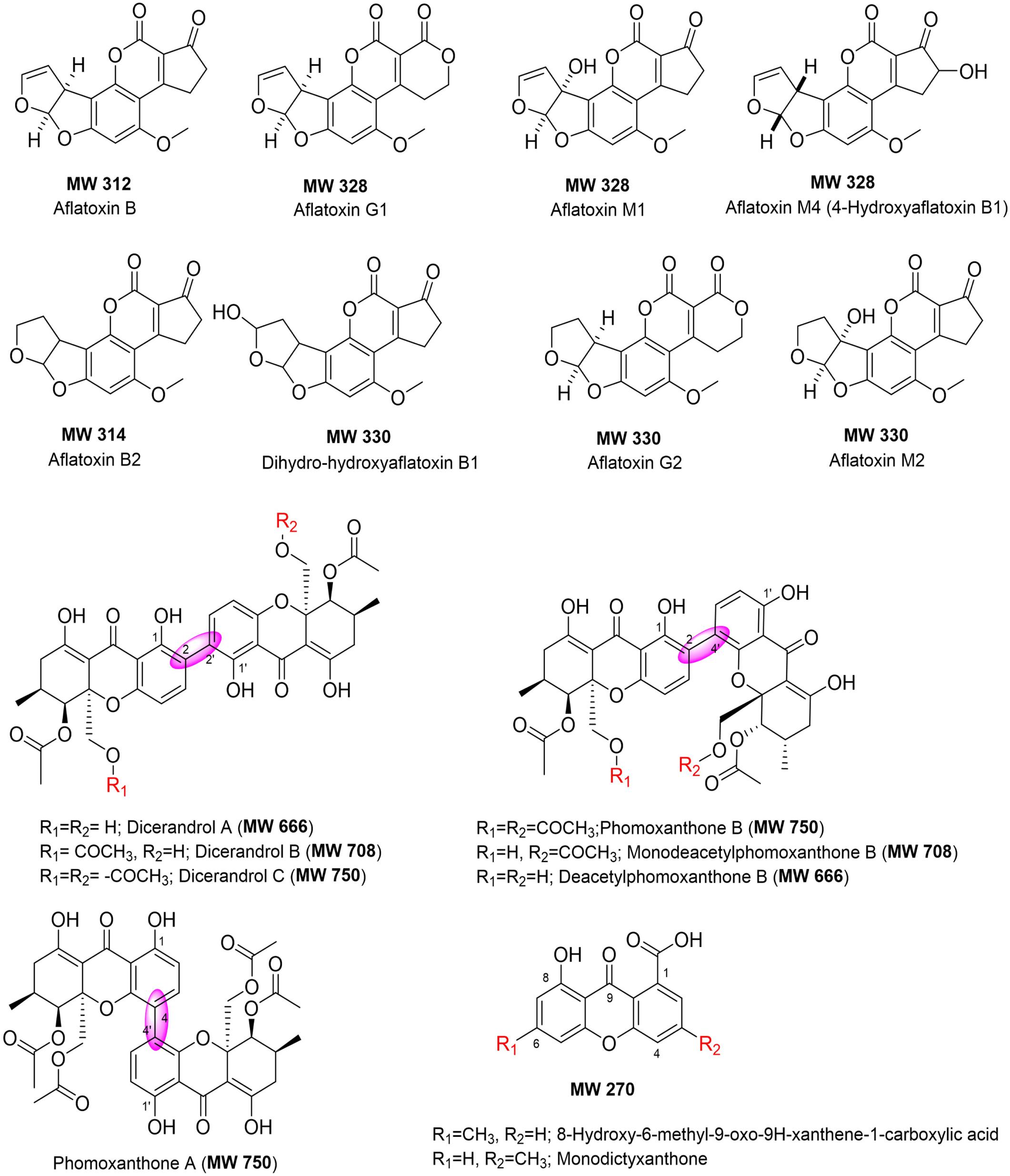
Figure 6. Chemical structures of compounds identified in the extracts of selected endophytic fungi (using HPLC-UV/vis, MS and TLC analyses, and AntiBase search).
Discussion
Plant diseases caused by microorganisms remain one of the major obstacles in agriculture, and more effective treatments for diseases caused by pathogens of citrus and maize are necessary. An ecologically attractive alternative to control plant diseases is the use of secondary metabolites produced by endophytes (Khare et al., 2018; Savi et al., 2019a). In the past decade we isolated a large number of endophytic fungi from leaves and petioles of the medicinal plants V. divergens and S. adstringens, respectively, located in two highly diverse biomes in Brazil: Pantanal and Cerrado (Carvalho et al., 2012; Hokama et al., 2016; Noriler et al., 2018). Here, we report on 12 endophytes producing secondary metabolites that were able to inhibit the mycelial growth of important pathogens of citrus (C. abscissum, P. citricarpa, and X. citri). We also report on extracts that inhibited the maize pathogenic fungus F. graminearum.
The isolates with biological activity (Supplementary Table 3) belong to the Ascomycota phylum, one of the most prevalent eukaryotic groups in the world (Park et al., 2017). In addition, Sordariomycetes was the most prevalent class among the endophytes selected. Diaporthe was the dominant genus among the endophytes that showed potential for production of compounds with antifungal activity. The genus Diaporthe belongs to the family Diaporthaceae and is composed of hundreds of species (Chepkirui and Stadler, 2017). We performed multilocus DNA sequence analyses using five loci (ITS, tef1, tub2, his3, and cal) commonly used in previous phylogenetic studies of Diaporthe species (Gomes et al., 2013; Guarnaccia et al., 2018; Yang et al., 2018; Guo et al., 2020). The final phylogenetic trees distinguished four species of the Diaporthe genus that showed potential against plant pathogens, including the new species D. cerradensis described in this study. This species was collected in the Cerrado, the Brazilian savanna and the extracts produced by two representatives of this species (CMRP4324 and CMRP4331) showed interesting results, especially against the pathogenic citrus fungi C. abscissum and P. citricarpa.
The genus Diaporthe is a rich source of bioactive secondary metabolites, including volatile and non-volatile compounds, which have been shown to be effective against plant pathogens (Singh et al., 2011; Noriler et al., 2019; Savi et al., 2020; Xu et al., 2021). This genus has also been recognized as a producer of several new compounds of great interest in biotechnology (Nicoletti and Fiorentino, 2015; Santos et al., 2016; Tahir et al., 2017). Santos et al. (2016) reported that endophytic isolates of D. terenbinthifolii were able to colonize citrus plants and produce secondary metabolites with activity against P. citricarpa, suggesting that this species could be used as a potential biological controller of the CBS disease. The multilocus phylogenetic analyses revealed four isolates belonging to the D. vochysiae species. This species was described by Noriler et al. (2019), in which compounds produced by the isolate LGMF1583 were characterized and tested, showing compounds with activity against human pathogenic bacteria.
Fungi endophytes isolates from two Brazilian biomes, Cerrado and Pantanal showed potential to produce secondary metabolites with activity against phytopathogens. In the present study, we isolated the endophyte D. vochysiae from both biomes and four strains showed potential to inhibit the growth of both target phytopathogens of this study. This species was initially isolated from the Pantanal biome as an endophyte of V. divergens (Noriler et al., 2019) and the extract produced from this isolate showed activities against human pathogens. The four strains belonging to the new species D. cerradensis were isolated from plants of the Cerrado biome, two obtained in this study, one (LGMF1616) obtained by Noriler et al. (2018) also from S. adstringens leaves and one isolate (UFMGCB4807, previously called Diaporthe cf. mayteni) was obtained as an endophyte from plant leaves of C. guianensis by Ferreira et al. (2015).
Both plant tissues (leaf and petiole) harbored endophytes producers of secondary metabolites with biological activity. One example is the species D. cf. heveae 1 isolated in the present study as an endophyte of petiole of S. adstringens, and isolated from leaf of S. adstringens by Noriler et al. (2018). The secondary metabolites from both strains showed activity against plant pathogens (Noriler et al., 2018; Savi et al., 2020). Our data reinforces the importance of both Brazilian biomes as a source of endophytes with biotechnological potential. Further studies can provide more information about fungi endophytic community structures across tissues and biomes.
Extracts produced by the isolates D. vochysiae CMRP4322, P. stromaticum CMRP4328, and N. primolutea CMRP4323 also inhibited the mycelial growth of C. abscissum more than the fungicide carbendazim, demonstrating the potential of the metabolites from these endophytic isolates to control citrus pathogens. These same extracts inhibited the development of PFD symptoms in the flowers (Figures 1A, 4). These results are promising since the treatment with the fungicide carbendazim can reduce, but not eliminate the phytopathogen development in field (Savi et al., 2020). In addition to the inhibition of mycelial growth, the isolate D. vochysiae CMRP4322 also inhibited the germination of C. abscissum conidia at lower than 1 μg/mL concentrations. These results are quite interesting due to the PFD cycle caused by the production of conidia in already infected flowers, which are then splashed on surrounding leaves, twigs and floral buds that remain after the blossom period (Agostini et al., 1992; Silva-junior et al., 2014; Pinho et al., 2015; Silva A. O. et al., 2016; Savi et al., 2019b). In Brazil, PDF disease leads to large losses in citrus production, estimated 80%, when rainy periods occur during the bloom phase (Goes et al., 2008; Silva A. O. et al., 2016).
Isolates D. vochysiae CMRP4322, Diaporthe sp. CMRP4330, and P. stromaticum CMRP4328 produced extracts that significantly inhibited the mycelial growth of P. citricarpa. We also showed that these extracts considerably reduced the formation of pycnidia on leaf surfaces (Figures 1B, 5). P. citricarpa affects fruit, leaves and twigs of several citrus hosts causing diverse symptoms (Kotzé, 2000; Guarnaccia et al., 2019). To control the CBS several fungicide applications may be necessary, leading to an increase in the costs of citrus production. However, if this disease is not fully controlled, it depreciates the commercial value of the fruits (Silva G. J. et al., 2016; Schreuder et al., 2018; Hendricks et al., 2020). In addition, citrus fruits with CBS symptoms are subject to quarantine regulations in the European Union restricting market access for countries with the presence of CBS disease and reduce the availability of citrus fruits to consumers in the off-season in Europe (Agostini et al., 2006; EU, 2015; Moyo et al., 2020).
Four isolates, D. vochysiae CMRP4322, Diaporthe sp. CMRP4330, D. cerradensis CMRP4331, and P. stromaticum CMRP4328 showed low values of MIC (10 μg/mL) capable of inhibiting the germination of P. citricarpa conidia. According to Sposito et al. (2011) and Tran et al. (2018), conidia are important sources of inoculum of P. citricarpa and play an important role in increasing the prevalence and spread of CBS disease. Therefore, these four extracts may be explored as an alternative option for CBS control. Endophytic microorganisms or their secondary metabolites can be used as alternatives to reduce the application of fungicides in the control of CBS and PFD (Santos et al., 2016; Tonial et al., 2017; Savi et al., 2020).
Three extracts analyzed (D. vochysiae CMRP4321 and CMRP4332, as well as P. stromaticum CMRP4328) were bactericidal against X. citri subsp. citri. This pathogenic bacterium causes citrus canker, a serious disease that affects citrus production worldwide (Fu et al., 2020). X. citri can colonize all above ground tissues of citrus plants, including young leaves, thorns, shoots, and fruits (Ferreira et al., 2016; Ference et al., 2018). As one of the most important citrus pathogens, X. citri causes serious economic losses due to reduced crop yield and quality, the costs of eradication measures, containment and obstacles to fruit exports. Moreover, the control of this bacterium results in an important environmental alert related to increased use of copper-based pesticides and the development of resistance to antimicrobials (Miller et al., 2009; Robène et al., 2020; Behlau, 2021).
The isolate D. vochysiae CMRP4326 inhibited the mycelial growth of a maize pathogen F. graminearum. Moreover, two extracts of isolates D. vochysiae CMRP4326 and P. stromaticum CMRP4328 completely inhibited the germination of conidia of this pathogen at low concentration (10 μg/mL). F. graminearum is an important pathogen affecting the yield and production of cereals, including maize and wheat worldwide (Schöneberg et al., 2016). In addition to causing direct yield loss through tissue rotting, F. graminearum can also cause crop contamination with mycotoxins, rendering the grain unsafe for human and livestock consumption and imposing higher costs on producers (Arunachalam and Doohan, 2013; Maresca, 2013; Pfordt et al., 2020). Different strategies are used to reduce the impact of FBH, including crop rotation, tillage practices, and fungicide application and planting of less susceptible cultivars (Jones et al., 2018). These strategies reached only low to moderate success (Wegulo et al., 2011; Mousa et al., 2015). Therefore, our results point to a promising alternative since secondary metabolites reported here prevent the germination of asexual spores, which is essential to break the cycle of the diseases.
HPLC-UV/MS analyses of the extracts produced by four strains (D. vochysiae CMRP4321, CMRP4322, and CMRP4332, and P. stromaticum CMRP4328) indicate the presence of several interesting compounds with similar UV/vis chromophores. AntiBase (Laatsch, 2017) search using MS and UV/vis, resulted in several xanthone-analogs (Figure 6) that matches with MS and UV/vis, including dicerandrol A (MW 666), dicerandrol B (MW 708), dicerandrol C (MW 750); phomoxanthone B (MW 750), or its mono- or di-deacetylated analogs (MW 708 and 666, respectively), all of them have been reported previously as fungal metabolites (Isaka et al., 2001; Wagenaar and Clardy, 2001; Zhang et al., 2008; Cao et al., 2012; Choi et al., 2013; Ding et al., 2013; Ronsberg et al., 2013; Kumla et al., 2017). The HPLC-UV/MS analysis of another fungus extract (Aspergillus sp. section Flavi CMRP4327) was very rich in metabolites (Supplementary Figures 26–29). AntiBase (Laatsch, 2017) and literature search using the aforementioned data indicates that this strain is a potential producer of aflatoxins (Asao et al., 1965; Benkerroum, 2020; Zhang and Banerjee, 2020). However, no aflatoxin analogs have been reported previously with MW 338, which designates this strain a potential producer of new aflatoxin-analogs (Laatsch, 2017).
Based on the aforementioned LCMS and TLC results, this work highlights that some of the selected strains including D. vochysiae CMRP4322, D. cf. heveae 1 CMRP4329, and Diaporthe sp. CMRP4330, Coniochaeta sp. CMRP4325, Aspergillus sp. section Flavi CMRP4327, and P. stromaticum CMRP4328, can now be considered promising strains for potential discovery of new bioactive natural products, and have been selected for future studies (including scale-up fermentations, isolation, and structure elucidation of the new bioactive compounds produced by these strains). Results will be published later somewhere else.
The compounds produced by endophytic microorganisms have been showing promise as a more environmentally friendly alternative (Wang et al., 2019). We reported here the capacity of 12 endophytic fungi isolated from tissues of the medicinal plants V. divergens and S. adstringens to produce secondary metabolites with biological activities against phytopathogenic fungi and bacteria. These metabolites directly affect the mycelium growth, germination of conidia, or symptoms development of important fungi pathogens of citrus (C. abscissum and P. citricarpa), and act also inhibiting the mycelial growth of the maize pathogen F. graminearum. In addition, some of these metabolites revealed bactericidal activity against an important pathogenic bacterium of citrus, X. citri subsp. citri. The herein described D. cerradensis is a new species of the Diaporthe genus. Its discovery again shows the capacity of medicinal plants found in Brazil to host a diverse group of fungi with biotechnological potential. Further studies are needed to identify the bioactive compounds and also for the field evaluation of the endophytes or their metabolites in the control of plant pathogens.
Data Availability Statement
The datasets presented in this study can be found in online repositories. The names of the repository/repositories and accession number(s) can be found below: [Diaporthe cerradensis MB#839350 – (https://www.mycobank.org/)].
Author Contributions
CG, DS, JI, and KS: conceived and designed the experiments. JI, RS, SN, BA, GD, and HF: performed the experiments. JI, DS, KS, and CG: analyzed the data. JI, DS, CG, KS, GD, and HF: writing – original draft. JI, DS, CH, JR, KS, and JT: writing – review and editing. CG, JR, KS, and JT: supervision. All authors read and approved the final manuscript.
Funding
This work was supported by the INCT Citrus CNPq 465440/2014-2 Brazil and CNPq grant 309971/2016-0 and 424738/2016-3 to CG, CAPES-Brazil – grant to JI, and FAPESP 2015/50162-2 – grant to HF. This work was also supported by National Institutes of Health grants R01 CA243529, R01 GM115261, the Center of Biomedical Research Excellence (COBRE) in Pharmaceutical Research and Innovation (CPRI, NIH P20 GM130456), the University Professorship in Pharmacy (to JR), the University of Kentucky College of Pharmacy and the National Center for Advancing Translational Sciences (UL1TR000117 and UL1TR001998).
Conflict of Interest
The authors declare that the research was conducted in the absence of any commercial or financial relationships that could be construed as a potential conflict of interest.
Publisher’s Note
All claims expressed in this article are solely those of the authors and do not necessarily represent those of their affiliated organizations, or those of the publisher, the editors and the reviewers. Any product that may be evaluated in this article, or claim that may be made by its manufacturer, is not guaranteed or endorsed by the publisher.
Supplementary Material
The Supplementary Material for this article can be found online at: https://www.frontiersin.org/articles/10.3389/fmicb.2021.714750/full#supplementary-material
Footnotes
- ^ www.cmrp-taxonline.com
- ^ http://www.mycobank.org/
- ^ www.MycoBank.org
- ^ https://blast.ncbi.nlm.nih.gov
References
Agostini, J. P., Peres, N. A., Mackenzie, S. J., Adaskaveg, J. E., and Timmer, L. W. (2006). Effect of fungicides and storage conditions on postharvest development of citrus sblack spot and survival of Guignardia citricarpa in fruit tissues. Plant Dis. 90, 1419–1424. doi: 10.1094/PD-90-1419
Agostini, J. P., Timmer, L. W., and Mitchell, D. J. (1992). Morphological and pathological characteristics of strains of Colletotrichum gloeosporioides form Citrus. APS 82, 1377–1382.
Allen, E., and Valdes, C. (2016). Brazil’s corn industry and the effect on the seasonal pattern of U.S. corn exports. U.S. Department of Agriculture, Economic Research Service.
Aly, A. H., Debbab, A., and Proksch, P. (2011). Fungal endophytes: unique plant inhabitants with great promises. J. Appl. Microbiol. Biotechnol. 90, 1829–1845. doi: 10.1007/s00253-011-3270-y
Arunachalam, C., and Doohan, F. M. (2013). Trichothecene toxicity in eukaryotes: Cellular and molecular mechanisms in plants and animals. Toxicol. Lett. 217, 149–158. doi: 10.1016/j.toxlet.2012.12.003
Asao, T., Büchi, G., Abdel-Kader, M. M., Chang, S. B., Wick, E. L., and Wogan, G. N. (1965). The structures of aflatoxins B and G1. J. Am. Chem. Soc. 87, 882–886. doi: 10.1021/ja01082a031
Behlau, F. (2021). An overview of citrus canker in Brazil. Trop. Plant Pathol. 46, 1–12. doi: 10.1007/s40858-020-00377-2
Benkerroum, N. (2020). Aflatoxins: producing-molds, structure, health issues and incidence in Southeast Asian and Sub-Saharan African Countries. Int. J. Environ. Res. Public Health 17:1215. doi: 10.3390/ijerph17041215
Cantrell, C. L., Dayan, F. E., and Duke, S. O. (2012). Natural products as sources for new pesticides. J. Nat. Prod. 75, 1231–1242. doi: 10.1021/np300024u
Cao, S., McMillin, D. W., Tamayo, G., Delmore, J., Mitsiades, C. S., and Clardy, J. (2012). Inhibition of tumor cells interacting with stromal cells by xanthones isolated from a Costa Rican Penicillium sp. J. Nat. Prod. 75, 793–797. doi: 10.1021/np2009863
Carbone, I., and Kohn, L. M. (1999). A method for designing primer sets for speciation studies in filamentous ascomycetes. Mycol. 91, 553–556. doi: 10.1080/00275514.1999.12061051
Carvalho, C. R., Gonçalves, V. N., Pereira, C. B., Johann, S., Galliza, I. V., and Rosa, C. A. (2012). The diversity, antimicrobial and anticancer activity of endophytic fungi associated with the medicinal plant Stryphnodendron adstringens (Mart.) Coville (Fabaceae) from the Brazilian savannah. Symbiosis 57, 95–107. doi: 10.1007/s13199-012-0182-2
Carvalho, S. A. D., Girardi, E. A., Mourão Filho, F. D. A. A., Ferrarezi, R. S., and Coletta Filho, H. D. (2019). Advances in citrus propagation in Brazil. Rev. Bras. Frutic. 41:e422. doi: 10.1590/0100-29452019422
Chepkirui, C., and Stadler, M. (2017). The genus Diaporthe: a rich source of diverse and bioactive metabolites. Mycol. Prog. 16, 477–494. doi: 10.1007/s11557-017-1288-y
Choi, J. N., Kim, J., Ponnusamy, K., Lim, C., Kim, J. G., Muthaiya, M. J., et al. (2013). Identification of a new phomoxanthone antibiotic from Phomopsis longicolla and its antimicrobial correlation with other metabolites during fermentation. J. Antibiot. 66, 231–233. doi: 10.1038/ja.2012.105
Copping, L. G., and Duke, S. O. (2007). Natural products that have been used commercially as crop protection agents. Pest Manag. Sci. 63, 524–554. doi: 10.1002/ps.1378
Crous, P. W., Groenewald, J. Z., and Risède, J. (2004). Calonectria species and their cylindrocladium anamorphs species with sphaeropedunculate vesicles. Stud. Mycol. 50, 415–430.
Crous, P. W., Verkley, G. J. M., and Groenewald, J. Z. (2009). Fungal Biodiversity. CBS Laboratory Manual Series 1. Utrecht, Netherlands: Westerdijk Fungal Biodiversity Institute.
Dayan, F. E., Cantrell, C. L., and Duke, S. O. (2009). Natural products in crop protection. Bioorg. Med. Chem. 17, 4022–4034. doi: 10.1016/j.bmc.2009.01.046
de Carvalho Filho, C. G., Kellner Filho, L. C., Picão, B. W., Ragozoni, A. A., Ribeiro, A. B., Nicolella, H. D., et al. (2021). Chemical composition and in vitro cytotoxicity of Corynespora olivacea (V18) associated with Vochysia divergens. BASR 2, 1244–1254. doi: 10.34115/basrv5n2-046
De Hoog, G. S., and Gerrits van den Ende, A. H. (1998). Molecular diagnostics of clinical strains of filamentous Basidiomycetes. Mycoses 41, 183–189. doi: 10.1111/j.1439-0507.1998.tb00321.x
De Menezes, H. D., Rodrigues, G. B., Teixeira, S. P., Massola, N. S. Jr., Bachmann, L., Wainwright, M., et al. (2014). In vitro photodynamic inactivation of plant-pathogenic fungi Colletotrichum acutatum and Colletotrichum gloeosporioides with novel phenothiazinium photosensitizers. Appl. Environ. Microbiol. 80, 1623–1632. doi: 10.1128/AEM.02788-13
de Souza, U. J. B., Vitorino, L. C., and Bessa, L. A. (2020). Trends in the scientific literature on Stryphnodendron adstringens (Leguminosae): an important Brazilian medicinal tree. Multi. Sci. J. 1, 8–15. doi: 10.33837/msj.v3i1.1108
Deising, H. B., Reimann, S., and Pascholati, S. F. (2008). Mechanisms and significance of fungicide resistance. Braz. J. Microbiol. 39, 286–295. doi: 10.1590/s1517-83822008000200017
Ding, B., Yuan, J., Huang, X., Wen, W., Zhu, X., Liu, Y., et al. (2013). New dimeric members of the phomoxanthone family: phomolactonexanthones A, B and deacetylphomoxanthone C isolated from the fungus Phomopsis sp. Mar. Drugs 11, 4961–4972. doi: 10.3390/md11124961
Doilom, M., Dissanayake, A. J., Wanasinghe, D. N., Boonmee, S., Liu, J. K., Bhat, D. J., et al. (2017). Microfungi on tectona grandis (teak) in Northern Thailand. Fungal Divers 1, 107–182. doi: 10.1007/s13225-016-0368-7
EU. (2015) Guidelines for Phyllosticta citricarpain the Union Territory. Available Online at: https://gd.eppo.int/taxon/GUIGCI/distribution/BD.
Ference, C. M., Gochez, A. M., Behlau, F., Wang, N., Graham, J. H., and Jones, J. B. (2018). Recent advances in the understanding of Xanthomonas citri ssp. citri pathogenesis and citrus canker disease management. Mol. Plant Pathol. 19, 1302–1318. doi: 10.1111/mpp.12638
Ferreira, M. C., Vieira, M. D. L. A., Zani, C. L., de Almeida Alves, T. M., Junior, P. A. S., Murta, S. M., et al. (2015). Molecular phylogeny, diversity, symbiosis and discover of bioactive compounds of endophytic fungi associated with the medicinal Amazonian plant Carapa guianensis Aublet (Meliaceae). Biochem. Syst. Ecol. 59, 36–44. doi: 10.1016/j.bse.2014.12.017
Ferreira, R. M., Moreira, L. M., Ferro, J. A., Soares, M. R. R., Laia, M. L., Varani, A. M., et al. (2016). Unravelling potential virulence factor candidates in Xanthomonas citri. subsp. citri by secretome analysis. Peer J. 4:e1734. doi: 10.7717/peerj.1734
Ferrigo, D., Raiola, A., and Causin, R. (2016). Fusarium toxins in cereals: occurrence, legislation, factors promoting the appearance and their management. Molecules 21:627. doi: 10.3390/molecules21050627
Fisher, M. C., Hawkins, N. J., Sanglard, D., and Gurr, S. J. (2018). Worldwide emergence of resistance to antifungal drugs challenges human health and food security. Science 360, 739–742. doi: 10.1126/science.aap7999
Food and Drug Administration (2012). Carbendazim in Orange Juice Products. Available Online at: http://www.fda.gov/food/foodborneillnesscontaminants/pesticides/ucm288004.htm
Forero, E. (1972). Studies in Stryphnodendron (Leguminosae Mimosoideae) including two new taxa. Brittonia 24, 143–147. doi: 10.2307/2805864
Fu, H., Zhao, M., Xu, J., Tan, L., Han, J., Li, D., et al. (2020). Citron C-05 inhibits both the penetration and colonization of Xanthomonas citri subsp. citri to achieve resistance to citrus canker disease. Hortic. Res. 7, 1–12. doi: 10.1038/s41438-020-0278-4
Gao, Y., Liu, F., Duan, W., Crous, P. W., and Cai, L. (2017). Diaporthe is paraphyletic. IMA Fungus 1, 153–187. doi: 10.5598/imafungus.2017.08.01.11
Gilchrist-Saavedra, L., Fuentes-Dávila, G., Martinez-Cano, C., López-Atilano, R. M., and Duveiller, E. (2006). Practical Guide to the Identification of Selected Diseases of Wheat and Barley, 2nd Edn. Mexico: CIMMYT.
Glass, N. L., and Donaldson, G. C. (1995). Development of primer sets designed for use with the PCR to amplify conserved genes from filamentous ascomycetes. ASM 61, 1323–1330.
Glienke, C., Pereira, O. L., Stringari, D., Fabris, J., Kava-Cordeiro, V., and Galli-Terasawa, L. (2011). Endophytic and pathogenic Phyllosticta species, with reference to those associated with citrus black spot. Persoonia 26, 47–56. doi: 10.3767/003158511X569169
Goes, A., Garrido, R. B. O., Reis, R. F., Baldassari, R. B., and Soares, M. A. (2008). Evaluation of fungicide applications to sweet orange at different flowering stages for control of postbloomfruit drop caused by Colletotrichum acutatum. Crop Prot. 27, 71–76. doi: 10.1016/j.cropro.2007.04.007
Gomes, R. R., Glienke, C., Videira, S. I. R., Lombard, L., Groenewald, J. Z., and Crous, P. W. (2013). Diaporthe: a genus of endophytic, saprobic and plant pathogenic fungi. Persoonia 31, 1–41. doi: 10.3767/003158513X666844
González-González, M. G., Gómez-Sanchis, J., Blasco, J., Soria-Olivas, E., and Chueca, P. (2020). Citrus Yield: a dashboard for mapping yield and fruit quality of citrus in precision agriculture. Agronomy 10:128. doi: 10.3390/agronomy10010128
Guarnaccia, V., Gehrmann, T., Silva-Junior, G. J., Fourie, P. H., Haridas, S., and Vu, D. (2019). Phyllosticta citricarpa and sister species of global importance to Citrus. Mol. Plant Pathol. 20, 1619–1635. doi: 10.1111/mpp.12861
Guarnaccia, V., Groenewald, J. Z., Li, H., Glienke, C., Carstens, E., and Hattingh, V. (2017). First report of Phyllosticta citricarpa and description of two new species, P. paracapitalensis and P. paracitricarpa, from citrus in Europe. Stud. Mycol. 87, 161–185. doi: 10.3767/003158515X688433
Guarnaccia, V., Groenewald, J. Z., Woodhall, J., Armengol, J., Cinelli, T., and Eichmeier, A. (2018). Diaporthe diversity and pathogenicity revealed from a broad survey of grapevine diseases in Europe. Persoonia Mol. Phylogeny Evol. Fungi. 40, 135–153. doi: 10.3767/persoonia.2018.40.06
Guerreiro, R. L., Bergier, I., McGlue, M. M., Warren, L. V., de Abreu, U. G. P., Abrahão, J., et al. (2019). The soda lakes of Nhecolândia: a conservation opportunity for the Pantanal wetlands. PECON 1, 9–18. doi: 10.1016/j.pecon.2018.11.002
Guo, Y. S., Crous, P. W., Bai, Q., Fu, M., Yang, M. M., and Wang, X. H. (2020). High diversity of Diaporthe species associated with pear shoot canker in China. Persoonia 45, 132–162. doi: 10.3767/persoonia.2020.45.05
Hardoim, P. R., Van Overbeek, L. S., Berg, G., Pirttilä, A. M., Compant, S., and Campisano, A. (2015). The hidden world within plants: ecological and evolutionary considerations for defining function of microbial endophytes. Microbiol. Mol. Biol. Rev. 79, 293–320. doi: 10.1128/MMBR.00050-14
Hendricks, K. E., Christman, M. C., and Roberts, P. D. (2020). The effect of weather and location of fruit within the tree on the incidence and severity of citrus black spot on Fruit. Sci. Rep. 10, 1–12. doi: 10.1038/s41598-020-58188-z
Hendricks, K. E. M., Donahoo, R. S., Roberts, P. D., and Christman, M. C. (2013). Effect of copper on growth characteristics and disease control of the recently introduced Guignardia citricarpa on citrus in Florida. Am. J. Plant Sci. 4, 282–290. doi: 10.4236/ajps.2013.42037
Hilário, S., Santos, L., and Alves, A. (2021). Diaporthe amygdali, a species complex or a complex species? Fungal Biol. 7, 505–518. doi: 10.1016/j.funbio.2021.01.006
Hokama, Y., Savi, D. C., Assad, B., Aluizio, R., Gomes-Figueiredo, J., and Adamoski, D. (2016). “Endophytic fungi isolated from Vochysia divergens in the Pantanal, Mato Grosso do Sul: diversity, phylogeny and biocontrol of Phyllosticta citricarpa,” in Endophytic Fungi: Diversity, Characterization and Biocontrol, 4th Edn, ed. E. Hughes (Hauppauge, NY: Nova), 1–25.
Hörner, M., Giglio, V. F., Santos, A. J. R. W. A., Westphalen, A. B., Iglesias, B. A., Martins, P. R., et al. (2008). Triazenes and antibacterial activity. Rev. Bras. Cienc. Farm. 44, 441–449. doi: 10.1590/S1516-93322008000300014
Isaka, M., Jaturapat, A., Rukseree, K., Danwisetkanjana, K., Tanticharoen, M., and Thebtaranonth, Y. (2001). Phomoxanthones A and B, novel xanthone dimers from the endophytic fungus Phomopsis species. J. Nat. Prod. 64, 1015–1018. doi: 10.1021/np010006h
Ivory, S. J., McGlue, M. M., Spera, S., Silva, A., and Bergier, I. (2019). Vegetation, rainfall, and pulsing hydrology in the Pantanal, the world’s largest tropical wetland. Environ. Res. Lett. 14:124017. doi: 10.1088/1748-9326/ab4ffe
Jones, S., Farooqi, A., Foulkes, J., Sparkes, D., Linforth, R., and Ray, R. V. (2018). Canopy and ear traits associated with avoidance of Fusarium head blight in wheat. Front. Plant Sci. 9:1021. doi: 10.3389/fpls.2018.01021
Katoh, K., and Toh, H. (2008). Recent developments in the MAFFT multiple sequence alignment program. Brief. Bioinform. 9, 286–298. doi: 10.1093/bib/bbn013
Khare, E., Mishra, J., and Arora, N. K. (2018). Multifaceted interactions between endophytes and plant: developments and prospects. Front. Microbiol. 9:2732. doi: 10.3389/fmicb.2018.02732
Kim, B., Han, J. W., Ngo, M. T., Le Dang, Q., Kim, J. C., Kim, H., et al. (2018). Identification of novel compounds, oleanane-and ursane-type triterpene glycosides, from Trevesiapalmata: Their biocontrol activity against phytopathogenic fungi. Sci. Rep. 8:1452. doi: 10.1038/s41598-018-32956-4
Kotzé, J. M. (2000). “Black spot,” in Compendium of Citrus Diseases, eds L. W. Timmer, S. M. Garnsey, and J. H. Graham (St. Paul, MN: American Phytopathological Society Press Inc), 23–25.
Kumar, S., Stecher, G., and Tamura, K. (2015). MEGA7: molecular evolutionary genetics analysis version 7 for bigger datasets. Mol. Biol. Evol. 33, 1870–1874. doi: 10.1093/molbev/msw054
Kumla, D., Shine Aung, T., Buttachon, S., Dethoup, T., Gales, L., Pereira, J. A., et al. (2017). A new dihydrochromone dimer and other secondary metabolites from cultures of the marine sponge-associated Fungi Neosartorya fennelliae KUFA 0811 and Neosartorya tsunodae KUFC 9213. Mar. Drugs 15:375. doi: 10.3390/md15120375
Manawasinghe, I. S., Dissanayake, A. J., Li, X., Liu, M., Wanasinghe, D. N., Xu, J., et al. (2019). High genetic diversity and species complexity of Diaporthe associated with grapevine dieback in China. Front. Microbiol. 10:1936. doi: 10.3389/fmicb.2019.01936
Manganyi, M. C., and Ateba, C. N. (2020). Untapped potentials of endophytic fungi: a review of novel bioactive compounds with biological applications. Microorganisms 8:1934. doi: 10.3390/microorganisms8121934
Maresca, M. (2013). From the gut to the brain: Journey and pathophysiological effects of the food associated trichothecene mycotoxin deoxynivalenol. Toxins 5, 784–820.
Miller, S. A., Beed, F. D., and Harmon, C. L. (2009). Plant disease diagnostic capabilities and networks. Annu. Rev. Phytopathol. 47, 15–38.
Morão, L. G., Polaquini, C. R., Kopacz, M., Torrezan, G. S., Ayusso, G. M., Dilarri, G., et al. (2018). A simplified curcumin targets the membrane of Bacillus subtilis. Microbiol. Open 8:e683. doi: 10.1002/mbo3.683
Mousa, W. K., Shearer, C. R., Limay-Rios, V., Zhou, T., and Raizada, M. N. (2015). Bacterial endophytes from wild maize suppress Fusarium graminearum in modern maize and inhibit mycotoxin accumulation. Front. Plant Sci. 6:805. doi: 10.3389/fpls.2015.00805
Moyo, P., Fourie, P. H., Masikane, S. L., de Oliveira Fialho, R., Mamba, L. C., du Plooy, W., et al. (2020). The effects of postharvest treatments and sunlight exposure on the reproductive capability and viability of Phyllosticta citricarpa in citrus black spot fruit lesions. Plants 9:1813. doi: 10.3390/plants9121813
Myers, N., Mittermeier, R. A., Mittermeier, C. G., Da Fonseca, G. A., and Kent, J. (2000). Biodiversity hotspots for conservation priorities. Nature 6772, 853–858. doi: 10.1038/35002501
Nicoletti, R., and Fiorentino, A. (2015). Plant bioactive metabolites and drugs produced by endophytic fungi of Spermatophyta. Agriculture 5, 918–970. doi: 10.3390/agriculture5040918
Noriler, S. A., Savi, D. C., Aluizio, R., Palácio-Cortes, A. M., Possiede, Y. M., and Glienke, C. (2018). Bioprospecting and structure of fungal endophyte communities found in the Brazilian Biomes, Pantanal, and Cerrado. Front. microbiol. 9:1526. doi: 10.3389/fmicb.2018.01526
Noriler, S. A., Savi, D. C., Ponomareva, L. V., Rodrigues, R., Rohr, J., Thorson, J. S., et al. (2019). Vochysiamides A and B: Two new bioactive carboxamides produced by the new species Diaporthe vochysiae. Fitoterapia 138:104273. doi: 10.1016/j.fitote.2019.104273
Park, Y. H., Kim, Y., Mishra, R. C., and Bae, H. (2017). Fungal endophytes inhabiting mountain-cultivated ginseng (Panax ginseng Meyer): Diversity and biocontrol activity against ginseng pathogens. Sci. Rep. 7, 1–10. doi: 10.1038/s41598-017-16181-z
Pellenz, N. L., Barbisan, F., Azzolin, V. F., Marques, L. P. S., Mastella, M. H., Teixeira, C. F., et al. (2019). Healing activity of Stryphnodendron adstringens (Mart.), a Brazilian tannin-rich species: A review of the literature and a case series. Wound Med. 1:100163. doi: 10.1016/j.wndm.2019.100163
Petrini, O. (1986). Taxonomy of Endophytic Fungi of Aerial Plant Tissues. Microbiology of the Phyllosphere. Cambridge: Cambridge University Press, 175–187.
Pfordt, A., Ramos Romero, L., Schiwek, S., Karlovsky, P., and von Tiedemann, A. (2020). Impact of environmental conditions and agronomic practices on the prevalence of Fusarium species associated with ear-and stalk rot in maize. Pathogens 9:236. doi: 10.3390/pathogens9030236
Pham, J. V., Yilma, M. A., Feliz, A., Majid, M. T., Maffetone, N., Walker, J. R., et al. (2019). A review of the microbial production of bioactive natural products and biologics. Front. Microbiol. 10:1404. doi: 10.3389/fmicb.2019.01404
Pinho, D. B., Lopes, U. P., Pereira, O. L., Silveira, A. L., and de Goes, A. (2015). Colletotrichum abscissum Pinho., O.L. Pereira, sp. nov. fungal planet description sheets. Persoonia 34, 236–237. doi: 10.3767/003158515X688433
Qin, C. F., He, M. H., Chen, F. P., Zhu, W., Yang, L. N., and Wu, E. J. (2016). Comparative analyses of fungicide sensitivity and SSR marker variations indicate a low risk of developing azoxystrobin resistance in Phytophthora infestans. Sci. Rep. 6:20483. doi: 10.1038/srep20483
Quiroga, E. N., Sampietro, A. R., and Vattuone, M. (2001). Screening antifungal activity of selected medicinal plants. J. Ethnopharmacol. 74, 89–96. doi: 10.1016/S0378-8741(00)00350-0
Raeder, J., and Broda, P. (1985). Rapid preparation of DNA from filamentous fungi. Let. Appl. Microbiol. 1, 17–20. doi: 10.1111/j.1472-765X.1985.tb01479.x
Robène, I., Maillot-Lebon, V., Chabirand, A., Moreau, A., Becker, N., Moumène, A., et al. (2020). Development and comparative validation of genomic-driven PCR-based assays to detect Xanthomonas citripv. citri in citrus plants. BMC Microbiol. 20:296. doi: 10.1186/s12866-020-01972-8
Rodrigues, M. B. C., Andreote, F. D., Sposito, M. B., Aguillar-Vildoso, C. I., Araujo, W. L., and Pizzirani-Kleiner, A. A. (2007). Resistência a benzimidazois por Guignardia citricarpa. Pesq. Agropec. Bras. 42, 323–327. doi: 10.1590/S0100-204X2007000300004
Ronquist, F., Teslenko, M., Van Der Mark, P., Ayres, D. L., and Darling, A. (2011). MrBayes 3.2: efficient Bayesian phylogenetic inference and model choice across a large model space. Syst. Biol. 61, 539–542. doi: 10.1093/sysbio/sys029
Ronsberg, D., Debbab, A., Mandi, A., Vasylyeva, V., Bohler, P., Stork, B., et al. (2013). Pro-apoptotic and immunostimulatory tetrahydroxanthone dimers from the endophytic fungus Phomopsis longicolla. J. Org. Chem. 78, 12409–12425. doi: 10.1021/jo402066b
Sang, H., and Kim, J. I. (2020). Advanced strategies to control plant pathogenic fungi by host-induced gene silencing (HIGS) and spray-induced gene silencing (SIGS). Plant Biotechnol. Rep. 14, 1–8. doi: 10.1007/s11816-019-00588-3
Santos, P. J. C., Savi, D. C., Gomes, R. R., Goulin, E. H., da costa Senkiv, C., and Tanaka, F. A. (2016). Diaporthe endophytica and D. terebinthifolii from medicinal plants for biological control of Phyllosticta citricarpa. Microbiol. Res. 186, 153–160. doi: 10.1016/j.micres.2016.04.002
Savi, D. C., Aluizio, R., and Glienke, C. (2019a). Brazilian plants: an unexplored source of endophytes as producers of active metabolites. Planta Med. 1, 1–12. doi: 10.1055/a-0847-1532
Savi, D. C., Haminiuk, C. W. I., Sora, G. T. S., Adamoski, D. M., Kensiki, J., and Winnischofer, S. M. B. (2015). Antitumor, antioxidant and antibacterial activities of secondary metabolites extracted by endophytic actinomycetes isolated from Vochysia divergens. Int. J. Pharm. Chem. Biol. Sci. 5, 347–356.
Savi, D. C., Noriler, S., Ponomareva, L. V., Thorson, J., Rohr, J., Glienke, C., et al. (2020). Dihydroisocoumarins produced by Diaporthe cf. heveae LGMF1631 inhibiting citrus pathogens. Folia Microbiol. 65, 381–392. doi: 10.1007/s12223-019-00746-8
Savi, D. C., Rossi, B. J., Rossi, G. R., Ferreira-Maba, L. S., Bini, I. H., da Silva Trindade, E., et al. (2019b). Microscopic analysis of colonization of Colletotrichum abscissum in citrus tissues. Microbiol. Res. 226, 27–33. doi: 10.1016/j.micres.2019.05.005
Savi, D. C., Shaaban, K. A., Gos, F. M. W. R., Ponomareva, L. V., Thorson, J. S., Glienke, C., et al. (2018). Phaeophleospora vochysiae Savi&Glienke sp. nov. Isolated from Vochysia divergens found in the pantanal, Brazil, produces bioactive secondary metabolites. Sci. Rep. 8:3122. doi: 10.1038/s41598-018-21400-2
Schöneberg, T., Martin, C., Wettstein, F. E., Bucheli, T. D., Mascher, F., and Bertossa, M. (2016). Fusarium and mycotoxin spectra in Swiss barley are affected by various cropping techniques. Food Addit. Contam. Part A Chem. Anal. Control. 33, 1608–1619. doi: 10.1080/19440049.2016.1219071
Schreuder, W., du Plooy, W., Erasmus, A., Savage, C., Basson, E., Lennox, C., et al. (2018). Postharvest fungicide treatments and cold storage control citrus black spot infections. Crop. Prot. 112, 332–342. doi: 10.1016/j.cropro.2018.06.020
Schulz, K. F., Chalmers, I., Hayes, R. J., and Altman, D. G. (1995). Empirical evidence of biasdimensions of methodological quality associated with estimates of treatment effects in controlled trials. JAMA 273, 408–412. doi: 10.1001/jama.273.5.408
Silva, A. O., Savi, D. C., Gomes, F. B., Gos, F. M. W. R., Silva, G. J., and Glienke, C. (2016). Identification of Colletotrichum species associated with postbloom fruit drop in Brazil through GAPDH sequencing analysis and multiplex PCR. Eur. J. Plant Pathol. 147, 731–748. doi: 10.1007/s10658-016-1038-z
Silva, C. I, Regasini, L. O., Petronio, M. S., Silva, D. H., Bolzani, V. S., Belasque, J. Jr., et al. (2013). Antibacterial activity of alkylgallates against Xanthomonas citrisubsp. citri. J. Bacteriol. Res. 195, 85–94. doi: 10.1128/JB.01442-12
Silva, G. J. Jr., Scapin, M. S., Silva, F. P., Silva, A. R. P., Behlau, F., and Ramos, H. H. (2016). Spray volume and fungicide rates for citrus black spot control based on tree canopy volume. Crop Prot. 85, 38–45. doi: 10.1016/j.cropro.2016.03.014
Silva-junior, G. J., Spósito, M. B., Marin, D. R., and Amorim, L. (2014). Efficacy and timing of application of fungicides for control of citrus postbloom fruit drop. Crop. Prot. 59, 51–56. doi: 10.1016/j.cropro.2014.01.012
Singh, A., Singh, D. K., Kharwar, R. N., White, J. F., and Gond, S. K. (2021). Fungal endophytes as efficient sources of plant-derived bioactive compounds and their prospective applications in natural product drug discovery: insights, avenues, and challenges. Microorganisms 9:197. doi: 10.3390/microorganisms9010197
Singh, S. K., Strobel, G. A., Knighton, B., Geary, B., Sears, J., and Ezra, D. (2011). An endophytic Phomopsis sp. possessing bioactivity and fuel potential with its volatile organic compounds. Microb. Ecol. 61, 729–739. doi: 10.1007/s00248-011-9818-7
Souza-Moreira, T. M., Queiroz-Fernandes, G. M., and Pietro, R. C. (2018). Stryphnodendron species known as “barbatimão”: a comprehensive report. Molecules 4:910. doi: 10.3390/molecules23040910
Sposito, M. B., Amorim, L., Bassanezi, R. B., Yamamoto, P. T., Felippe, M. R., and Czermainski, A. B. C. (2011). Relative importance of inoculum sources of guignardiacitricarpa on the citrus black spot epidemic in Brazil. Crop Prot. 30, 1546–1552. doi: 10.1016/j.cropro.2011.08.007
Strassburg, B. B. N., Brooks, T., Feltran-Barbieri, R., Iribarrem, A., Crouzeilles, R., Loyola, R., et al. (2017). Moment of truth for the Cerrado hotspot. Nat. Ecol. Evol. 1, 1–3. doi: 10.1038/s41559-017-0099
Strobel, G. A. (2003). Endophytes as sources of bioactive products. Microbes Infect. 5, 535–544. doi: 10.1016/S1286-4579(03)00073-X
Tahir, H. A., Gu, Q., Wu, H., Niu, Y., Huo, R., and Gao, X. (2017). Bacillus volatiles adversely affect the physiology and ultra-structure of Ralstonia solanacearum and induce systemic resistance in tobacco against bacterial wilt. Sci. Rep. 7:40481. doi: 10.1038/srep40481
Tan, X., Zhou, Y., Zhou, X., Xia, X., Wei, Y., and He, L. (2018). Diversity and bioactive potential of culturable fungal endophytes of Dysosma versipellis; a rare medicinal plant endemic to China. Sci. Rep. 8:5929. doi: 10.1038/s41598-018-24313-2
Tonial, F., Maia, B. H. L. N. S., Savi, D. C., Vicente, V. A., and Gomes, R. R. (2017). Biological activity of Diaporthe terebinthifolii extracts against Phyllosticta citricarpa. FEMS Microbiol. Lett. 364:fnx026. doi: 10.1093/femsle/fnx026
Tran, N. T., Miles, A. K., Smith, M. W., Dietzgen, R. G., and Drenth, A. (2018). Pathogenicity of phyllostictacitricarpa ascospores on Citrus spp. Plant Dis. 102, 1386–1393. doi: 10.1094/PDIS-08-17-1331-RE
Wagenaar, M. M., and Clardy, J. (2001). Dicerandrols, new antibiotic and cytotoxic dimers produced by the fungus Phomopsis longicolla isolated from an endangered mint. J. Nat. Prod. 64, 1006–1009. doi: 10.1021/np010020u
Walder, F., Schlaeppi, K., Wittwer, R., Held, A. Y., Vogelgsang, S., and Van der Heijden, M. G. (2017). Community profiling of Fusarium in combination with other plant-associated fungi in different crop species using SMRT sequencing. Front. Plant Sci. 8:2019. doi: 10.3389/fpls.2017.02019
Wang, H., Tian, R., Tian, Q., Yan, X., Huang, L., and Ji, Z. (2019). Investigation on the antifungal ingredients of Saccharothrix yanglingensis Hhs. 015, an antagonistic endophytic actinomycete isolated from cucumber plant. Molecules 24:3686. doi: 10.3390/molecules24203686
Wang, J. F., He, W. J., Huang, X. L., Tian, X. P., Liao, S. R., Yang, B., et al. (2016). Antifungal new oxepine-containing alkaloids and xanthones from the deep-sea-derived fungus Aspergillus versicolor SCSIO 05879. J. Agr. Food. Chem. 64, 2910–2916. doi: 10.1021/acs.jafc.6b00527
Wegulo, S. N., Bockus, W. W., Nopsa, J. H., De Wolf, E. D., Eskridge, K. M., and Peiris, K. H. (2011). Effects of integrating cultivar resistance and fungicide application on fusarium head blight and deoxynivalenol in winter wheat. Plant Dis. 95, 554–560. doi: 10.1094/PDIS-07-10-0495
White, T. J., Bruns, T., Lee, J., and Taylor, J. (1990). “Amplification and direct sequencing of fungal ribosomal RNA genes for phylogenetics,” in PCR Protocols: A Guide to Methods and Applications, eds M. A. Innis, D. H. Gelfand, J. J. Sninsky, and T. J. White (San Diego, CA: Academic Press), 315–322.
Xu, T. C., Lu, Y. H., Wang, J. F., Song, Z. Q., Hou, Y. G., Liu, S. S., et al. (2021). Bioactive secondary metabolites of the genus diaportheand anamorph phomopsis from terrestrial and marine habitats and endophytes: 2010–2019. Microorganisms 9:217. doi: 10.3390/microorganisms9020217
Yang, Q., Fan, X. L., Guarnaccia, V., and Tian, C. M. (2018). High diversity of Diaporthe species associated with dieback diseases in China, with twelve new species described. MycoKeys. 39:97. doi: 10.3897/mycokeys.39.26914
Zamuner, C. F. C., Dilarri, G., Cavalca, L. B., Behlau, F., Silva, T. G., Bacci, M. Jr., et al. (2020). A cinnamaldehyde-based formulation as an alternative to sodium hypochlorite for post-harvest decontamination of citrus fruit. Trop. Plant Pathol. 45, 701–709. doi: 10.1007/s40858-020-00338-9
Zhang, K., and Banerjee, K. (2020). A review: sample preparation and chromatographic technologies for detection of aflatoxins in foods. Toxins (Basel) 12:539. doi: 10.3390/toxins12090539
Zhang, W., Krohn, K., Zia, U., Florke, U., Pescitelli, G., Di Bari, L., et al. (2008). New mono- and dimeric members of the secalonic acid family: blennolides A-G isolated from the fungus Blennoria sp. Chemistry. 14, 4913–4923. doi: 10.1002/chem.200800035
Keywords: phytopathogens, endophytes, secondary metabolites, natural product, endophytic fungi
Citation: Iantas J, Savi DC, Schibelbein RdS, Noriler SA, Assad BM, Dilarri G, Ferreira H, Rohr J, Thorson JS, Shaaban KA and Glienke C (2021) Endophytes of Brazilian Medicinal Plants With Activity Against Phytopathogens. Front. Microbiol. 12:714750. doi: 10.3389/fmicb.2021.714750
Received: 25 May 2021; Accepted: 03 August 2021;
Published: 01 September 2021.
Edited by:
Jia Liu, Chongqing University of Arts and Sciences, ChinaReviewed by:
Sunil Kumar Deshmukh, The Energy and Resources Institute (TERI), IndiaMadhuree Kumari, Indian Institute of Science (IISc), India
Copyright © 2021 Iantas, Savi, Schibelbein, Noriler, Assad, Dilarri, Ferreira, Rohr, Thorson, Shaaban and Glienke. This is an open-access article distributed under the terms of the Creative Commons Attribution License (CC BY). The use, distribution or reproduction in other forums is permitted, provided the original author(s) and the copyright owner(s) are credited and that the original publication in this journal is cited, in accordance with accepted academic practice. No use, distribution or reproduction is permitted which does not comply with these terms.
*Correspondence: Khaled A. Shaaban, a2hhbGVkX3NoYWFiYW5AdWt5LmVkdQ==; Chirlei Glienke, Y2guZ2xpZW5rZUBnbWFpbC5jb20=
†ORCID: Chirlei Glienke, orcid.org/0000-0003-3394-0659; Jucélia Iantas, orcid.org/0000-0002-1203-7543; Jürgen Rohr, orcid.org/0000-0001-6447-5951; Jon S. Thorson, orcid.org/0000-0002-7148-0721; Khaled A. Shaaban, orcid.org/0000-0001-7638-4942