- 1School of Food Science, Washington State University, Pullman, WA, United States
- 2Washington Tree Fruit Research Commission, Wenatchee, WA, United States
- 3Stemilt Growers LLC., Wenatchee, WA, United States
This study aimed to investigate the effects of low-dose continuous ozone gas in controlling Listeria innocua and quality attributes and disorders of Red Delicious apples during long-term commercial cold storage. Red Delicious apples were inoculated with a three-strain L. innocua cocktail at ∼6.2 log10 CFU/apple, treated with or without 1-methylcyclopropene, and then subjected to controlled atmosphere (CA) storage with or without continuous gaseous ozone in a commercial facility for 36 weeks. Uninoculated Red Delicious apples subjected to the above storage conditions were used for yeast/mold counts and quality attributes evaluation. The 36 weeks of refrigerated air (RA) or CA storage caused ∼2.2 log10 CFU/apple reduction of L. innocua. Ozone gas application caused an additional > 3 log10 CFU/apple reduction of L. innocua compared to RA and CA storage alone. During the 36-week CA storage, low-dose continuous gaseous ozone application significantly retarded the growth of yeast/mold, delayed apple firmness loss, and had no negative influence on ozone burn, lenticel decay, russet, CO2 damage, superficial scald, and soft scald of Red Delicious apples compared to CA-alone storage. In summary, the application of continuous low-dose gaseous ozone has the potential to control Listeria on Red Delicious apples without negatively influencing apple quality attributes.
Introduction
Listeria monocytogenes can cause deadly listeriosis in susceptible human populations and has a high mortality rate (CDC, 2017). L. monocytogenes is widespread in agricultural environments. The Food and Drug Administration enforces a zero tolerance for apples and other ready-to-eat foods (Shank et al., 1996; FDA, 2008). Apples are grown in an open environment and can therefore be subject to L. monocytogenes during production or subsequent postharvest packing (Angelo et al., 2017). L. monocytogenes has been implicated in two multistate caramel apple outbreaks (Angelo et al., 2017; Marus et al., 2019) and multiple recalls of several fresh apple varieties (FDA, 2017, 2019) and fresh-cut apples (FDA, 2016, 2020b). These outbreaks and recalls highlight the risk of L. monocytogenes contamination of apples and the importance of controlling L. monocytogenes on apples. After harvest, apples are usually subjected to cold storage for up to 12 months in controlled atmosphere (CA) refrigerated storage. Antimicrobial interventions during this long-term storage could provide potential interventional strategies in controlling Listeria on fresh apples (Sheng and Zhu, 2021).
Ozone is a strong oxidant and potent antimicrobial agent, which does not leave any residue on the treated produce (Guzel-Seydim et al., 2004). Ozone gas is approved by the Food and Drug Administration as a Generally Recognized as Safe agent (FDA, 2020a) and is approved for use in organic production and handling (USDA-NOP, 2021). Gaseous ozone at a fixed rate for a short duration has been investigated to control major food-borne pathogens, including Escherichia coli O157:H7 (Han et al., 2002), Salmonella (Das et al., 2006), and L. monocytogenes (Alwi and Ali, 2014; Concha-Meyer et al., 2014) on fresh produce. For example, ozone gas at 10 mg/L resulted in a ∼7 log10 CFU/tomato reduction of Salmonella Enteritidis inoculated on tomatoes within 1 h (Das et al., 2006). Gaseous ozone at 4 mg/L reduced L. monocytogenes by 3 log10 CFU/ml on fresh blueberries after 10-day storage at 4°C (Concha-Meyer et al., 2014). A 20-min gaseous ozone (23 mg/L) exposure caused 2.1–3.1 log10 CFU/apple reduction of L. monocytogenes on Empire apples (Murray et al., 2018). Ozone gas was also studied to control resident microbiota on different apple varieties during storage (Yaseen et al., 2015). A 60-day exposure of ozone gas at 0.5 mg/L under 1°C storage resulted in a 2.7–4.0 log reduction of Penicillium expansum on fresh apples, depending on the variety (Yaseen et al., 2015). Furthermore, ozone gas application during storage is beneficial to maintain fruit quality. For example, 1–3 mg/L gaseous ozone exposure was reported to maintain the firmness of apples (Antos et al., 2018) and reduced apple weight loss (Juhnevica-Radenkova et al., 2019). We previously showed that 30 weeks of continuous application of gaseous ozone at 87 μg/L in CA storage reduced Listeria by ∼5.0 log10 CFU/apple on Fuji apples without negatively impacting apple fruit quality (Sheng et al., 2018). However, this effect cannot directly transfer to other apple cultivars because each apple variety has its unique cuticular wax and resident microbiota compositions that impact Listeria persistence and response to antimicrobial interventions (Chai et al., 2020; Ku et al., 2020; Abdelfattah et al., in press).
Red Delicious apples, one of the most commercially sold apple cultivars in the United States (USAA, 2019), was recently implicated in a recall due to potential contamination of L. monocytogenes (FDA, 2019). Red Delicious apples are especially prone to developing watercore compared to other commercially grown apple varieties, possessing an increased risk of developing internal disorders such as internal browning during long-term CA storage (Mattheis, 2008), further leading to losses to the apple industry. The objective of this study was to investigate the effects of different commercial storage regimes in conjunction with different doses of continuous gaseous ozone in controlling Listeria innocua, which is phylogenetically related to L. monocytogenes (Buchrieser et al., 2003), on Red Delicious apples with or without 1-methylcyclopropene (1-MCP) pretreatment. The maintenance of quality attributes of Red Delicious apples under different storage conditions was further evaluated.
Materials and Methods
Listeria innocua Culture Preparation
Two L. innocua food isolates, NRRL 33314 and NRRL 33554, and one processing plant isolate L. innocua, NRRL 33197, were obtained from the USDA-ARS culture collection [National Center for Agricultural Utilization Research (NRRL), Peoria, IL, United States] and stored in trypticase soy broth (Becton, Dickinson and Company (BD), Sparks, MD, United States) supplemented with 0.6% yeast extract (TSBYE; Fisher Scientific, Fair Lawn, NJ, United States) (TSBYE) and 20% (v/v) glycerol at −80°C. Each frozen culture was subjected to two sequential transfers in TSBYE at 37°C for 24 h. The three-strain cocktail inoculum was prepared by combining an equal population of individual L. innocua strains. The individual L. innocua strain or a three-strain cocktail was enumerated by serially diluting and plating on TSAYE (TSBYE with 1.5% agar) plates.
Apple Inoculation
Fresh unwaxed Red Delicious apples, manually harvested at commercial maturity as indicated by the Cornell Starch index for a range of 2.8–3.5 (Blanpied and Silsby, 1992), were stored in a commercial storage room (Yakima, WA, United States) at ∼1°C and delivered to the laboratory before the study. Apples (∼200 g), free of cuts, bruises, or scars, were selected for this study. For L. innocua inoculation, apples were dip inoculated in the three-strain L. innocua inoculum diluted in sterile phosphate-buffered saline (1 × PBS, pH 7.4) to obtain the inoculation level of ∼6.2 log10 CFU/apple. The inoculated apples were held at room temperature (∼22°C) for 24 h before subjected to the respective storages. Forty inoculated apples were randomly sampled at 0 and 24 h, respectively, to confirm the initial bacterial level and the uniformity of inoculation.
Storage Treatment of Apples
Apples 24-h postinoculation were randomly packed into plastic crates; 40 apples per crate. The boxed inoculated apples were randomly separated into six groups, and half of them were treated with 1.0 mg/L gaseous 1-MCP air for 24 h before storage. Apples were then subjected to the refrigerated air (RA; 0.2 ± 0.1°C) and CA (0.2 ± 0.1°C, 3.1 ± 0.0% O2, 0.4 ± 0.0% CO2) with or without continuous gas at 60.2 ± 5.7 or 78.7 ± 13.2 μg/L in semicommercial RA/CA rooms (3,200 ft2) with the relative humidity of ∼90% in a commercial facility (Stemilt Growers LLC, Wenatchee, WA, United States). Ozone gas was generated by a commercial ozone generator (Guardian Ozone, Cocoa, FL, United States) and transferred automatically into storage room coupled with a proportional–integral–derivative algorithm to maintain ozone dose at the target level during storage. Ozone concentrations were initiated at the first week of storage and reached the target concentration at the beginning of the fourth week. Concurrently, separated sets of uninoculated apples were included in the above storage conditions for resident microflora enumeration and apple quality evaluation.
Inoculated apples were sampled after 3, 6, 12, 18, 24, 30, and 36 weeks of storage for L. innocua enumeration. Uninoculated apples were sampled after 6, 12, 24, and 36 weeks of storage for total plate counts (TPC) and yeast/mold counts. Apple quality attributes were assessed at harvest and at 6 and 9 months of storage. Forty apples per storage regime were sampled at each time point for microbial enumeration, quality attributes, and internal disorder evaluation, while 100 apples per storage regime were sampled at each time point for external disorder evaluation, where each apple was considered an experimental unit.
Listeria innocua Enumeration
Each apple was transferred to a stomacher bag (Fisher Scientific) containing 10 ml of sterile 1 × PBS and hand-rubbed for 80 s. Rub solutions were 10-fold serially diluted with sterile 1 × PBS, plated on TSAYE plates, and then overlaid with modified Oxford agar (MOX) (BD) to discern Listeria from background bacteria (Shen et al., 2019), and incubated at 37°C for 48 h. L. innocua produced typical black colonies surrounded by black halos on MOX agar plates. For samples below the detection limit (10 CFU/apple), 1.0 ml of rub solution was enriched in buffered Listeria enrichment buffer (BLEB) (BD) at 30°C for 48 h; the presence/absence of Listeria was reported. The enrichment culture was streaked onto both MOX and CHROMagarTM Listeria (CHROMagar, Paris, France) plates, respectively, to qualitatively detect the presence of Listeria (Sheng et al., 2017). A sample size of 10 apples per replication with four independent replicates per storage regime was sampled at each time point.
Resident Microbiota Enumeration
Uninoculated apples were processed the same way as inoculated apples. Rub solutions at appropriate dilutions were plated on duplicate TSAYE plates and potato dextrose agar (PDA; BD) plates, respectively, for TPC and yeast/mold counts. TSAYE plates were incubated at 37°C for 24 h, while PDA plates were incubated at room temperature (22°C) for 5 days. A sample size of 10 apples per replication with four independent replicates per storage regime was sampled at each time point.
Fruit Quality Analysis
Fruit quality attributes including firmness, total soluble solids (TSS), and titratable acidity (TA) were evaluated on uninoculated apples at harvest and at 6 and 9 months after cold storage per the published method (Sheng et al., 2018). Briefly, fruit firmness was measured with a fruit texture analyzer (FTA GS-15-643, Güss Manufacturing, Ltd., Strand, South Africa) using a 1-cm-diameter probe on a peeled area (∼3 cm2) on both the sun-exposed and shaded sides of each apple. TSS content was measured using an Atago PR-32 digital Brix refractometer (Atago Co., Ltd., Tokyo, Japan). TA of fruit juice was represented by the percentage of malic acid content (grams of malic acid per 100 g fresh weight of apples) and measured using a potentiometric titrator (Titrando 888 and 875 Robotic USB Sample Processor XL, Metrohm, Riverview, FL, United States). A sample size of 10 apples per replication with four independent replicates per storage regime was used for apple quality analysis.
Fruit Disorder Analysis
Fruits were inspected for external disorders at harvest and after 6 and 9 months of cold storage. External disorders including ozone burn (surface pitting on apple exposed side), superficial scald (brown patches and discoloration on the skin), lenticel decay (dark brown pits around lenticels), decay (visible mycelium or spore masses), russet (periderm formation on skin), and CO2 damage (snowflake-like patches on the skin) were visually inspected following standard criteria as previously described (Sheng et al., 2018). Soft scald, characterized by smooth or irregularly shaped brown lesions on apple surfaces (DeEll, 2013), was also evaluated. Apples were sliced three times for evaluation of internal disorders including watercore and internal browning. Watercore was characterized by the accumulation of sorbitol-rich fluid in the intercellular spaces in flesh adjacent to the vasculature (Marlow and Loescher, 1984). Internal browning was characterized by the brown discoloration of the flesh that usually originated near the core area (Argenta et al., 2001). The results were reported as apples with respective disorder/total apples (%) within individual treatment per storage period. A sample size of 10 apples per replicate with four independent replicates per storage regimen was used for internal disorder assessment. For external disorder evaluations, a sample size of 100 apples was used per each storage regime. Apples were evaluated after 1 and 7 days at room temperature (22°C).
Statistical Analysis
Data were analyzed with IBM SPSS 19.0 (Chicago, IL, United States). Mean difference was discerned by one-way analysis of variance (ANOVA) followed by Tukey multiple comparison test. Values of p < 0.05 were considered statistically significant.
Results
Survival of Listeria innocua on Red Delicious Apples Under Commercial Cold Storage Conditions With Different Doses of Gaseous Ozone
During the first 3 weeks of cold storage, the populations of L. innocua on apples were reduced by 0.7–0.9 log10 CFU/apple under all storage conditions, before the ozone concentration had reached the target concentration (Figure 1). There was 2.1–2.2 log10 CFU/apple reduction of L. innocua on Red Delicious apples under RA storage or CA storage with or without 1-MCP treatment during 36 weeks of storage (Figure 1). Low-dose continuous gaseous ozone application, regardless of dose and 1-MCP treatment, dramatically increased L. innocua reduction on Red Delicious apples compared to that under RA or CA storage, which resulted in an additional 3.3–3.5 log10 CFU/apple reduction at the end of 24 weeks of storage followed by no further reduction during subsequent storage (Figure 1 and Table 1).
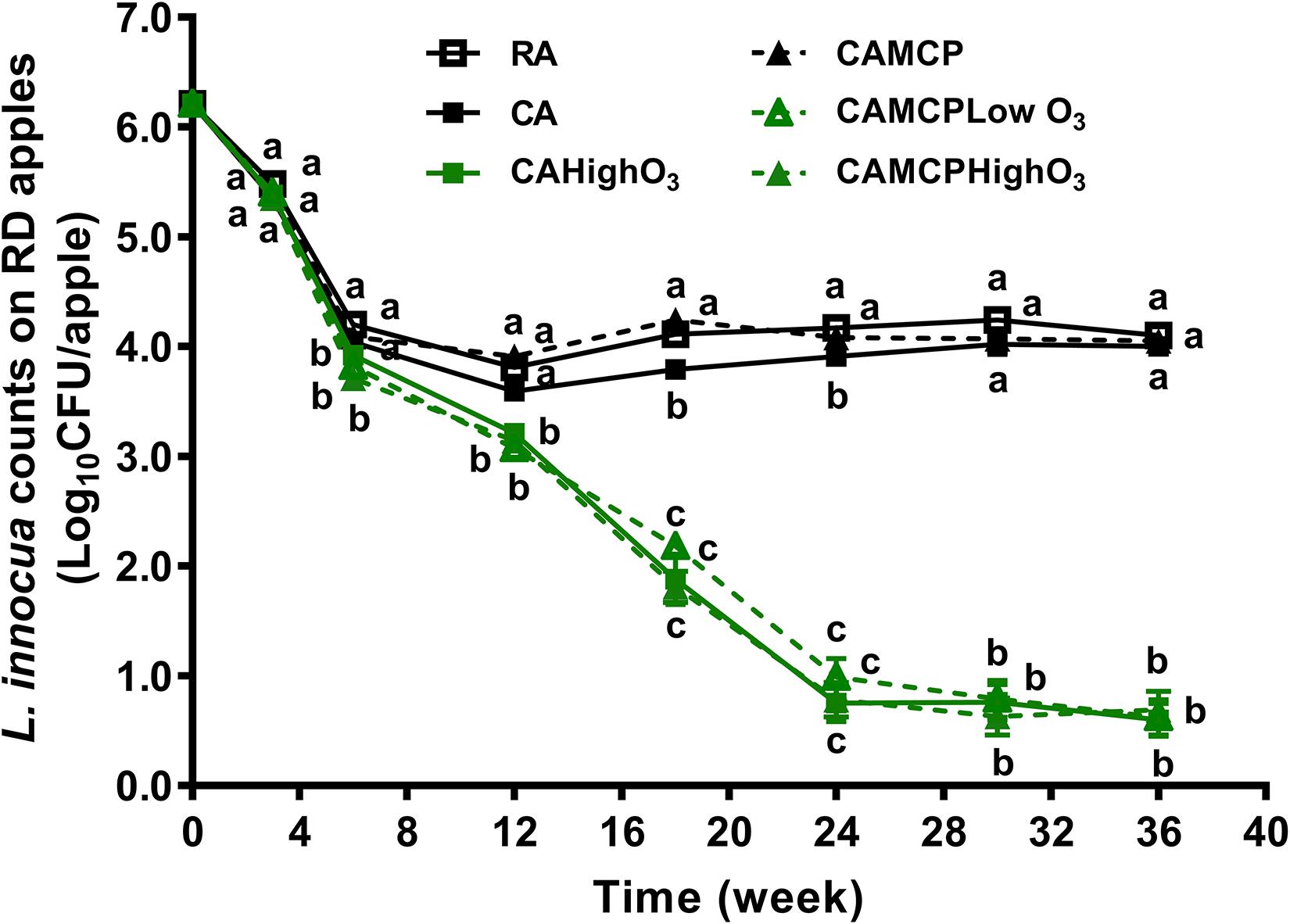
Figure 1. Survival of L. innocua on Red Delicious apples during 36 weeks of commercial cold storage. a– cMean at each sampling point without a common letter differ significantly (p < 0.05). Mean ± SEM, n = 32–40. MCP, apples were treated with 1-MCP prior to cold storage; CAHighO3, CA storage with continuous gaseous O3 application at 78.7 ± 13.2 μg/L; CAMCPHighO3, CA storage with continuous gaseous O3 application at 78.7 ± 13.2 μg/L, where apples were treated with 1-MCP prior to cold storage; CAMCPLowO3, CA storage with continuous gaseous O3 application at 60.2 ± 5.7 μg/L, where apples were treated with 1-MCP prior to cold storage.
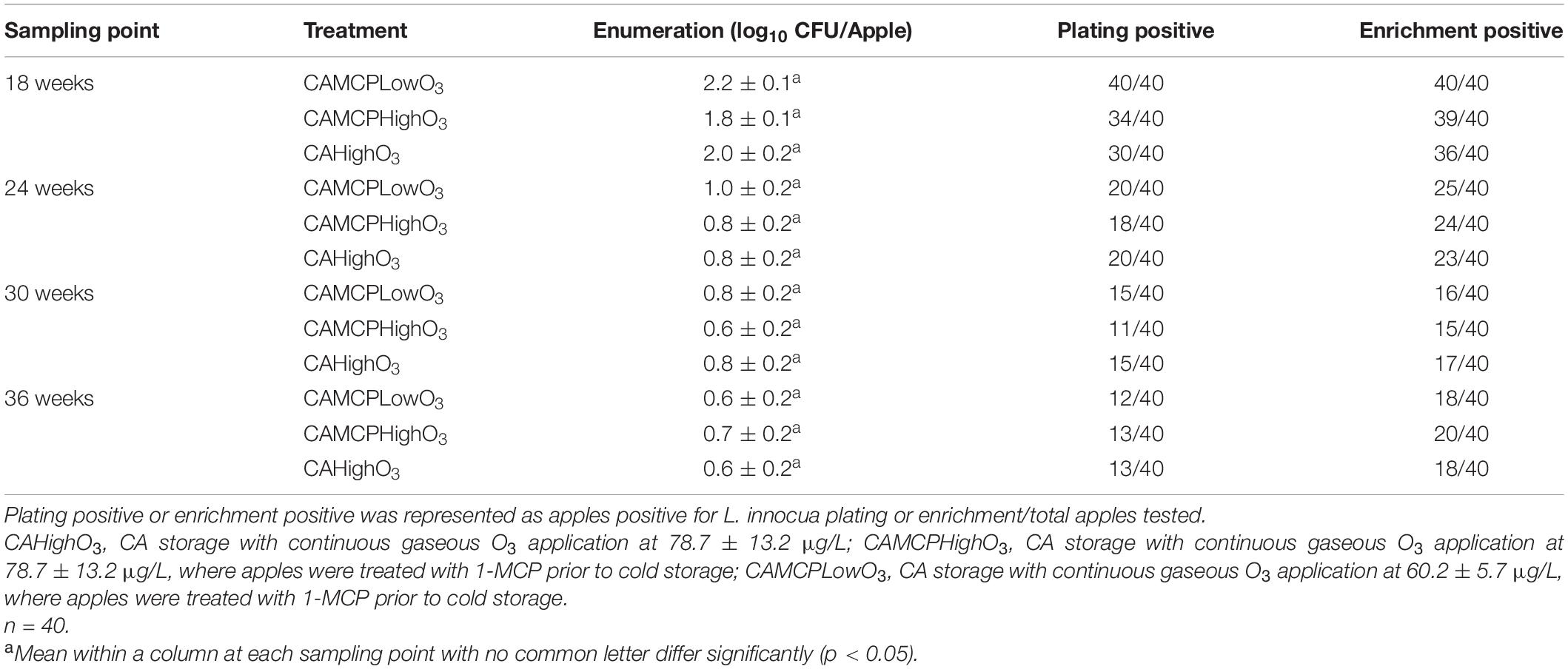
Table 1. Listeria innocua-positive Red Delicious apples under CA with gaseous ozone and/or 1-MCP storage after 18 weeks.
Resident Microflora on Red Delicious Apples Under Commercial Cold Storage Conditions With Different Doses of Gaseous Ozone
The resident bacteria on uninoculated Red Delicious apples were 3.8 log10 CFU/apple before storage (Figure 2). The population of resident bacteria on apples increased by 0.9–1.0 log10 CFU/apple under RA or CA storage pretreated with or without 1-MCP (Figure 2). The level of resident bacteria was relatively stable during subsequent RA and CA storage (Figure 2). Gaseous ozone application decreased resident bacteria by 1.2–1.3 log10 CFU/apple after 36 weeks of storage regardless of ozone dose and 1-MCP pretreatment (Figure 2).
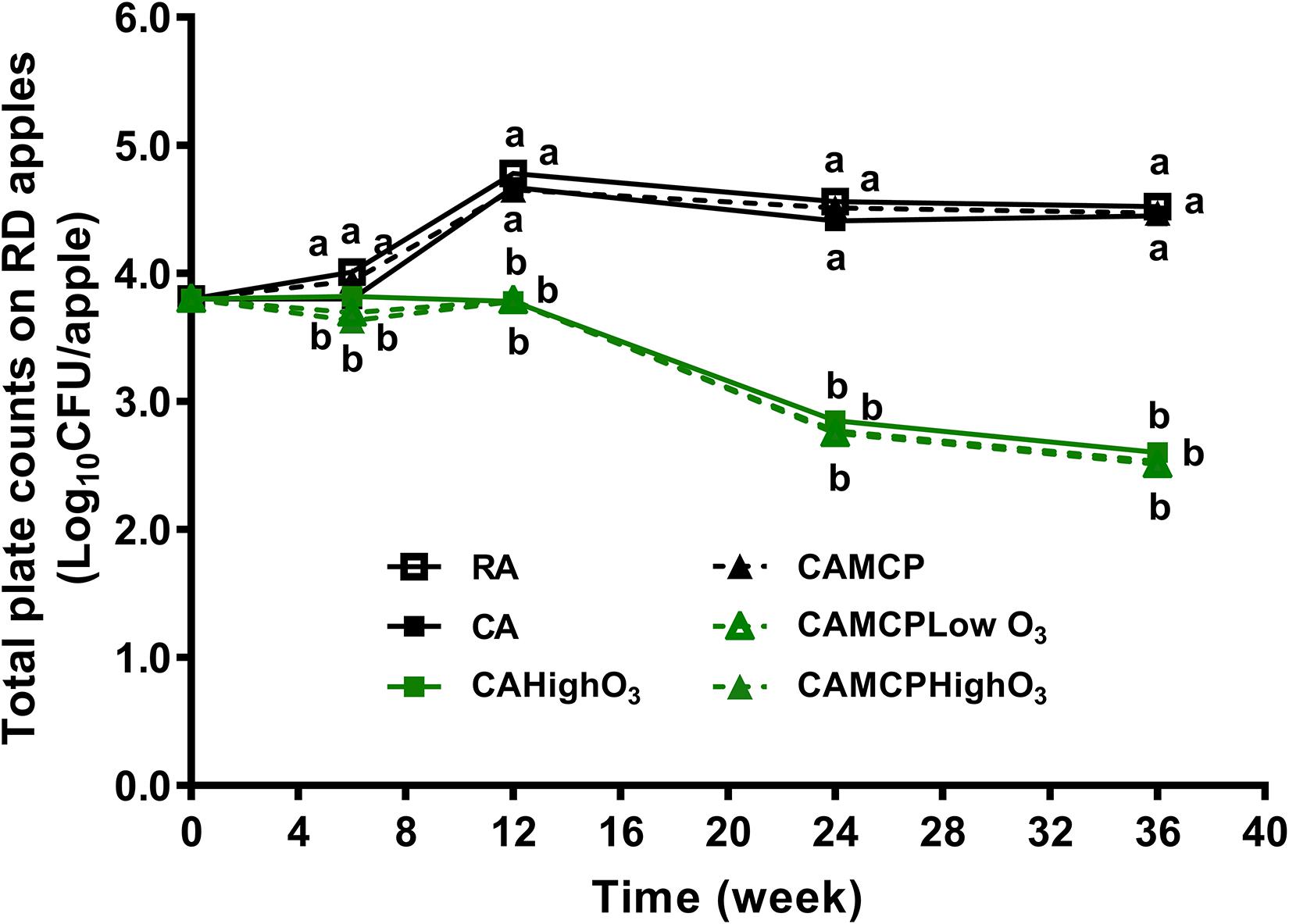
Figure 2. Resident bacteria on Red Delicious apples during 36 weeks of commercial cold storage. a,bMean at each sampling point without common letter differ significantly (p < 0.05). Mean ± SEM, n = 40. MCP, apples were treated with 1-MCP prior to cold storage; CAHighO3, CA storage with continuous gaseous O3 application at 78.7 ± 13.2 μg/L; CAMCPHighO3, CA storage with continuous gaseous O3 application at 78.7 ± 13.2 μg/L, where apples were treated with 1-MCP prior to cold storage; CAMCPLowO3, CA storage with continuous gaseous O3 application at 60.2 ± 5.7 μg/L, where apples were treated with 1-MCP prior to cold storage.
The yeast/mold counts of uninoculated Red Delicious apples were 4.7 log10 CFU/apple at the beginning of storage, which increased by 1.1–1.3 log10 CFU/apple after 36 weeks of RA or CA storage (Figure 3). The gaseous ozone application, regardless of dose and 1-MCP treatment, resulted in a 0.7 log10 CFU/apple reduction of yeast/mold counts after 36 weeks of storage (Figure 3).
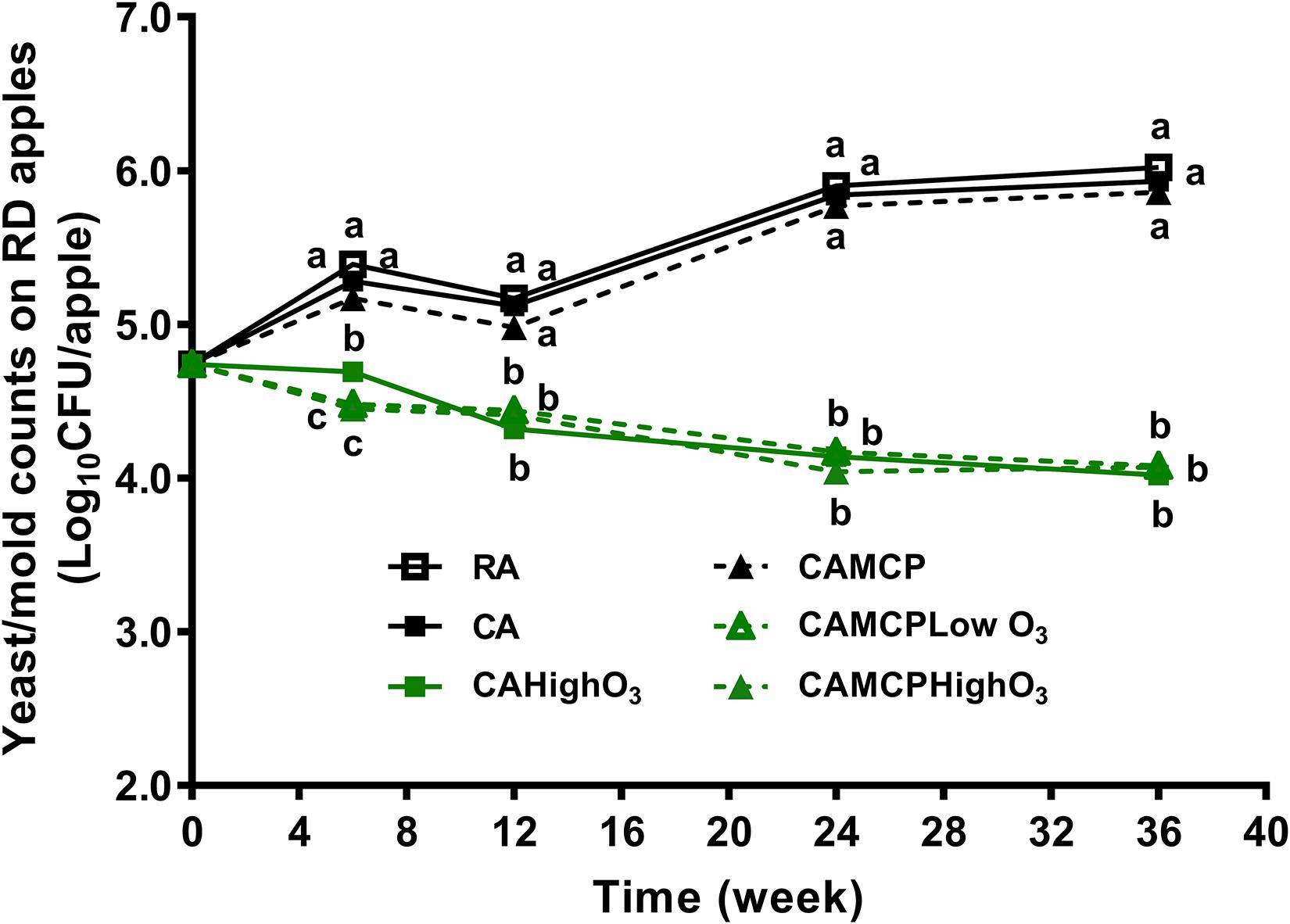
Figure 3. Yeast/mold counts on Red Delicious apples during 36 weeks of commercial cold storage. a– cMean at each sampling point without common letter differ significantly (p < 0.05). Mean ± SEM, n = 40. MCP, apples were treated with 1-MCP prior to cold storage; CAHighO3, CA storage with continuous gaseous O3 application at 78.7 ± 13.2 μg/L; CAMCPHighO3, CA storage with continuous gaseous O3 application at 78.7 ± 13.2 μg/L, where apples were treated with 1-MCP prior to cold storage; CAMCPLowO3, CA storage with continuous gaseous O3 application at 60.2 ± 5.7 μg/L, where apples were treated with 1-MCP prior to cold storage.
Quality Attributes and Disorders of Red Delicious Apples Under Commercial Cold Storage Conditions With Different Doses of Gaseous Ozone
The TSS of Red Delicious apples did not differ among storage treatments at 6 and 9 months (Table 2). Red Delicious apples under RA storage had a significantly lower firmness and TA compared with CA with 1-MCP treatment or with gaseous ozone at 6- and 9-month storages (Table 2). Ozone gas application or 1-MCP treatment in CA storage significantly retarded fruit firmness loss compared to CA storage alone at 6 and 9 months of storage, where the firmness was 4.4 and 4.2 kg for CA storage but was 5.5–6.5 and 5.2–6.8 kg for CA storage with MCP treatment and ozone gas application (Table 2).
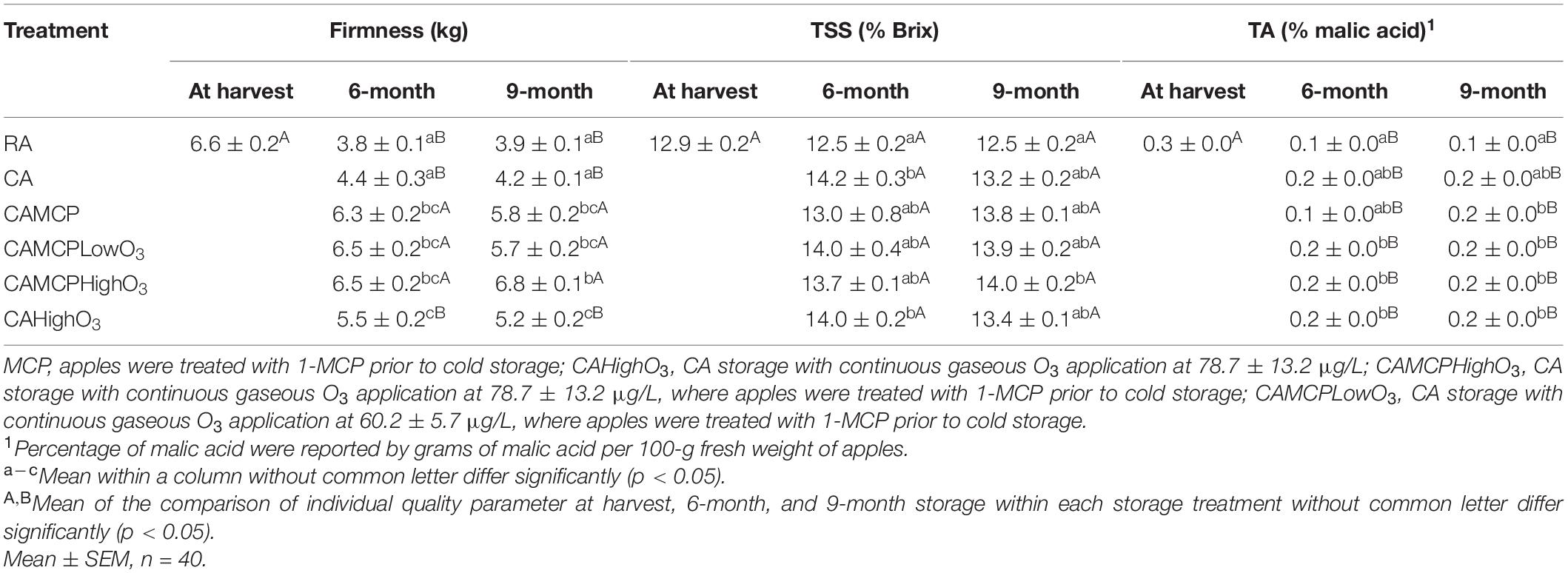
Table 2. Fruit quality attributes of Red Delicious apples after cold storage under different conditions.
Red Delicious apples under RA storage had a higher incidence of superficial scald (at 6- and 9-month of storage) and rot (at 9-month of storage) compared to CA storage with or without 1-MCP treatment (Table 3). Compared to CA storage, gaseous ozone application in CA storage did not influence the incidence of superficial scald, ozone burn, lenticel decay, russet, CO2 damage, and soft scald of Red Delicious apples up to 9 months of storage (Table 3).
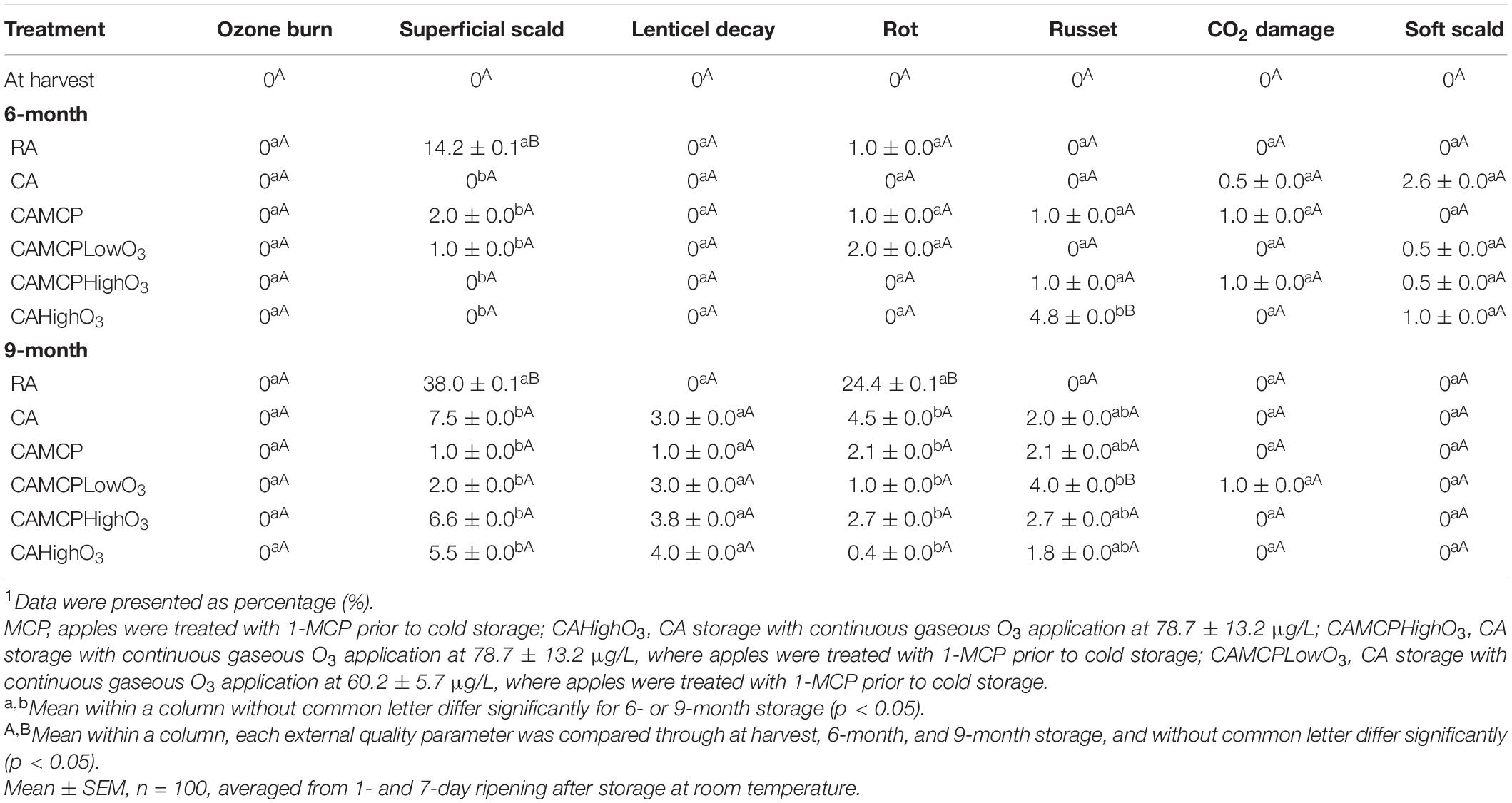
Table 3. External disorders analysis of Red Delicious apples after cold storage under different conditions1.
The incidence of watercore was decreased in Red Delicious apples under all storage treatments after 6- and 9-month of storage (Table 4). The internal browning incidence was significantly increased in Red Delicious apples under RA and CA storage, with or without 1-MCP at 6 and 9 months, compared to that at harvest (Table 4). The application of gaseous ozone at 60.2–78.7 μg/L during CA storage mitigated the internal browning incidence of Red Delicious apples (Table 4).
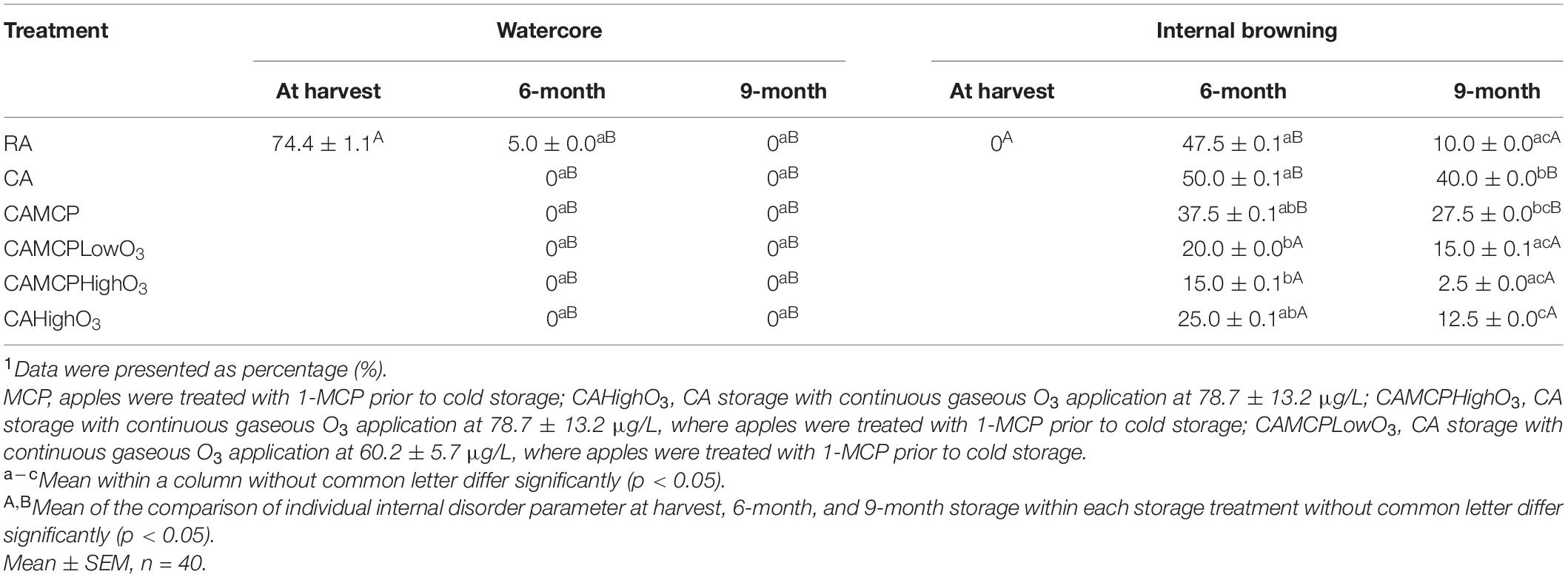
Table 4. Internal disorder analysis of Red Delicious apples after cold storage under different conditions1.
Discussion
Red Delicious apples at 36 weeks of RA and CA commercial cold storages exhibited a 2.1–2.2 log10 CFU/apple reduction of L. innocua. Similarly, a 2.5–3.0 log10 CFU/apple reduction of L. innocua was detected on Fuji apples at 30 weeks of RA and CA cold storages (Sheng et al., 2018). Consistently, a 10-day CA storage at 4°C reduced L. monocytogenes by 1.2 log10 CFU/ml on fresh highbush blueberries (Concha-Meyer et al., 2014). This study found a similar reduction of L. innocua on the surface of Red Delicious apples during 36 weeks of RA and CA storages. However, more reduction of L. innocua was observed in Fuji apples under RA storage than that under CA storage (Sheng et al., 2018). L. monocytogenes showed better survival on endive under RA storage at 4°C compared to that under CA storage (5% CO2, 5%O2, 90% N2), where an additional 1.0 log reduction of L. monocytogenes occurred on endive under CA storage after 14 days (Niemira et al., 2005). The exact reasons for the different behavior of L. innocua in different apple varieties/studies are unknown. Different Listeria strains are known to have different behavior under 56-day CA (5% O2, 10% CO2, and 85% N2) storage at 4°C (Razavilar and Genigeorgis, 1992). Given that the same L. innocua cocktail was used in this study as previous reports using Fuji apples, the resident microbial community associated with each apple variety, the source of apples, and the apple surface wax composition might be partially responsible for the divergence, warranting future research.
The gaseous ozone application at 60.2–78.7 μg/L during 24 weeks of CA storage resulted in > 5 log10 CFU/apple reductions of L. innocua on Red Delicious apples, which is consistent with the finding on Fuji apples (Sheng et al., 2018). The 1-MCP application, a common practice for apple fruit subjected to long-term CA storage (Mattheis, 2008), did not interfere the antilisterial efficacy of gaseous ozone throughout the entire storage. Considering practical L. monocytogenes contamination loads were less than 3.5 log10 CFU/fruit (Chen et al., 2016), the gaseous ozone application provides a viable in-storage intervention strategy in controlling L. monocytogenes on fresh apples. It is worth noting that the maximal reduction of L. innocua was achieved at 24 weeks of CA storage with gaseous ozone; ozone application during subsequent storage did not cause additional reduction of L. innocua. A similar phenomenon was also observed in Fuji apples, where lethality of ozone gas against L. innocua reached a plateau at 24 weeks of storage (Sheng et al., 2018). A 6-h exposure to 1 mg/L ozone reduced L. monocytogenes on fresh-cut bell peppers by 2 log10 CFU/g, and prolonged exposure of 24 h did not result in any further reduction (Alwi and Ali, 2014). Gaseous ozone is highly mobile and reactive compared to aqueous ozone intervention on produce surfaces (Singh et al., 2002), and it induces cell leakage by increasing membrane fluidity, reducing membrane integrity, and disrupting cell osmotic balance (Zhang et al., 2011; Ersoy et al., 2019). The L. innocua on apple surfaces might be protected from ozone by dead cells or microcrack/niches on the apple surfaces (Macauley et al., 2006; Selma et al., 2008).
The initial populations of resident bacteria and yeast/mold counts on Red Delicious apples were 3.8 and 4.7 log10 CFU/apple, which was similar with previous observations on Fuji apples (Sheng et al., 2018) and Red Delicious apples (Kreske et al., 2006), indicating apple surfaces are covered with a high population of background microflora. TPC and yeast/mold counts increased by ∼1 log10 CFU/apple on Red Delicious apples under RA and CA storage after 36 weeks. Similarly, TPC and yeast/mold counts increased on sweet cherries under CA storage at 1°C for 30 days (Serradilla et al., 2013). The yeast/mold counts of Fuji apples increased by ∼1 log10 CFU/apple during 30 weeks of RA storage (Sheng et al., 2018). In contrast, TPC of Fuji apples under RA and CA storage and the yeast/mold population of Fuji apples under CA remained relatively stable during 30 weeks (Sheng et al., 2018). This difference might be due to different initial background resident microbiota compositions as well as the different surface properties of Fuji and Red Delicious apples.
Ozone gas application at 60.2–78.7 μg/L in CA storage suppressed the resident microflora on Red Delicious apples. This was consistent with the finding on Fuji apples, where supplementation of 87 μg/L gaseous ozone in CA storage retarded the growth of resident bacteria and yeasts and molds on apples at 30 weeks of storage (Sheng et al., 2018). Concordantly, continuous provision of 0.5 mg/L ozone gas during 15 days of storage at 12°C reduced resident microbiota, including total mesophilic bacteria and yeast/mold counts, by ∼0.2 log10 CFU/g on tomatoes (Tuffi et al., 2012). A 45-min ozone gas treatment at 2.8 mg/L reduced TPC and yeast/mold counts by 1.5 and 0.7 log10 CFU/mushroom on mushrooms (Akata et al., 2015).
Fruit quality is an important driver of consumer preference. Improper cold storage leads to a significant loss of fruit quality (Davis and Blair, 1936). CA storage is known to maintain the fruit quality longer (Tasdelen and Bayindirli, 1998) and retard apple decay during long-term storage (Xuan and Streif, 2005). Consistently, Red Delicious apples under RA storage had a higher incidence of superficial scald and decay compared to CA storage, pretreated with or without 1-MCP treatment, during 36 weeks of storage.
The supplementation of low-dose continuous gaseous ozone in CA storage significantly retarded the firmness loss in Red Delicious apples compared to that in RA or CA storage alone. Similarly, the application of gaseous ozone in CA storage after 24 weeks significantly decreased the firmness loss in Fuji apples compared to that in RA storage (Sheng et al., 2018). However, the changes of apple firmness were similar for Fuji apples under CA storage alone or supplemented with ozone gas (Sheng et al., 2018). Accordingly, the application of gaseous ozone at 0.8 mg/L during 6 months of cold storage retarded the loss of flesh firmness in Ledzenu and Auksis apples (Juhnevica-Radenkova et al., 2019).
The 1-MCP pretreatment was beneficial to maintain the firmness in Red Delicious apples during 9-month CA storage. Consistently, Empire apples pretreated with 1-MCP were firmer than apples without 1-MCP treatment after 10 months of 0.5°C CA storage (Jung and Watkins, 2011); Red Delicious apples treated with 1-MCP were firmer than those without 1-MCP treatment after 6 months of 0°C storage (Mir et al., 2001). 1-MCP is a known ethylene action inhibitor, which binds to ethylene receptor and prevents ethylene from binding and eliciting its action, thus retarding fruit ripening and firmness loss (Deell et al., 2008).
Watercore is a physiological disorder where fluid accumulates in the intercellular air spaces in flesh adjacent to the vasculature (Marlow and Loescher, 1984). Red Delicious and Fuji apples are two cultivars that are prone to developing watercore (Mattheis, 2008). In this study, the incidence of watercore in Red Delicious apples at harvest was high. This might be due to the fact that Red Delicious apples typically, when achieving the optimum background color for commercial harvest requirements, are more likely to have watercore at harvest (Watkins et al., 1992). The incidence of watercore was significantly reduced during cold storage regardless of storage treatment/period. In agreement, the incidence of watercore decreased from 2.6 to 0.1 as expressed by watercore rating in Fuji apples after 160 days of storage at 0°C (Kasai and Arakawa, 2010). In this study, the internal browning developed in Red Delicious apples under all storage conditions after 6- and 9-month storages but was mitigated by supplementing with gaseous ozone in CA storage compared with CA storage alone. In Fuji apples, no internal browning was found after 6 months of RA or CA storage (Sheng et al., 2018). Consistent with our finding on Fuji apples (Sheng et al., 2018), continuous low-dose gaseous ozone application did not cause ozone burn and had no impact on superficial scald, lenticel decay, russet, CO2 damage, and soft scald on Red Delicious apples over 9 months of CA storage.
Conclusion
The counts of L. innocua on Red Delicious apples decreased by ∼2 log CFU/apple over 9 months of commercial RA or CA storage. The reduction of L. innocua was magnified 1,000-fold by continuous low-dose ozone gas treatment at 60.2–78.7 μg/L; > 5.0 log10 CFU/apple reduction was achieved after 24 weeks of storage. In addition, low-dose gaseous ozone application in CA storage retarded the growth of resident microbiota, delayed apple firmness loss, improved apple quality, and had no negative impacts on external disorders of Red Delicious apples compared to CA storage without ozone gas. Thus, low-dose continuous gaseous ozone application is a promising strategy for the apple industry to control Listeria and microbial decay on Red Delicious apples. Further studies on different ozone dosage and apple varieties are warranted to ensure the safety and quality of fresh apples.
Data Availability Statement
The raw data supporting the conclusions of this article will be made available by the authors, without undue reservation.
Author Contributions
XS: investigation, data analysis, and writing–original draft. YS, ZH, LS, and MM: investigation. YH and TG: sample preparation. IH and RB: writing–review and editing. M-JZ: conceptualization, supervision, data analysis, writing–review and editing, and funding acquisition. All authors contributed to the article and approved the submitted version.
Funding
This study was funded by the Washington Tree Fruit Research Commission and partially by USDA-NIFA 2020-68012-31822.
Conflict of Interest
RB was employed by Stemilt Growers LLC.
The remaining authors declare that the research was conducted in the absence of any commercial or financial relationships that could be construed as a potential conflict of interest.
Publisher’s Note
All claims expressed in this article are solely those of the authors and do not necessarily represent those of their affiliated organizations, or those of the publisher, the editors and the reviewers. Any product that may be evaluated in this article, or claim that may be made by its manufacturer, is not guaranteed or endorsed by the publisher.
Acknowledgments
We would like to thank Guardian Ozone for their generous support on gaseous ozone application and Stemilt Growers LLC for providing R&D cold storage facilities. We would also like to thank Enrique Garcia-Perez for assisting in sample storage and the research interns at Washington Tree Fruit Research Commission for helping with the transportation of apples.
References
Abdelfattah, A., Freilich, S., Bartuv, R., Zhimo, V. Y., Kumar, A., Biasi, A., et al. (in press). Global analysis of the apple fruit microbiome: are all apples the same? Environ. Microbiol. 1462–2920. doi: 10.1111/1462-2920.15469
Akata, I., Torlak, E., and Erci, F. (2015). Efficacy of gaseous ozone for reducing microflora and foodborne pathogens on button mushroom. Postharvest Biol. Technol. 109, 40–44. doi: 10.1016/j.postharvbio.2015.06.008
Alwi, N. A., and Ali, A. (2014). Reduction of Escherichia coli O157. Listeria monocytogenes and Salmonella enterica sv. Typhimurium populations on fresh-cut bell pepper using gaseous ozone. Food Control 46, 304–311. doi: 10.1016/j.foodcont.2014.05.037
Angelo, K. M., Conrad, A. R., Saupe, A., Dragoo, H., West, N., Sorenson, A., et al. (2017). Multistate outbreak of Listeria monocytogenes infections linked to whole apples used in commercially produced, prepackaged caramel apples: United States, 2014-2015. Epidemiol. Infect. 145, 848–856. doi: 10.1017/s0950268816003083
Antos, P., Piechowicz, B., Gorzelany, J., Matlok, N., Migut, D., Jozefczyk, R., et al. (2018). Effect of ozone on fruit quality and fungicide residue degradation in apples during cold storage. Ozone Sci. Eng. 40, 482–486. doi: 10.1080/01919512.2018.1471389
Argenta, L., Fan, C., and Mattheis, J. (2001). Development Of Internal Browning In Fuji Apples During Storage. https://citeseerx.ist.psu.edu/viewdoc/download?doi=10.1.1.502.434&rep=rep1&type=pdf (accessed July 27, 2021).
Blanpied, G. D., and Silsby, K. J. (1992). Predicting Harvest Date Windows For Apples. https://ecommons.cornell.edu/bitstream/handle/1813/3299/Predicting%20Harvest%20Date%20Window%20for%20Apples.pdf?sequence=2&isAllowed=y (accessed January 6, 2021).
Buchrieser, C., Rusniok, C., Kunst, F., Cossart, P., Glaser, P., and Consortium, L. (2003). Comparison of the genome sequences of Listeria monocytogenes and Listeria innocua: clues for evolution and pathogenicity. FEMS Immunol. Med. Microbiol. 35, 207–213. doi: 10.1016/s0928-8244(02)00448-0
CDC (2017). Listeria (Listeriosis). https://www.cdc.gov/listeria/index.html (accessed July 27, 2021).
Chai, Y. F., Li, A., Wai, S. C., Song, C. C., Zhao, Y. Y., Duan, Y. Q., et al. (2020). Cuticular wax composition changes of 10 apple cultivars during postharvest storage. Food Chem. 324:126903. doi: 10.1016/j.foodchem.2020.126903
Chen, Y., Burall, L. S., Luo, Y., Timme, R., Melka, D., Muruvanda, T., et al. (2016). Listeria monocytogenes in stone fruits linked to a multistate outbreak: enumeration of cells and whole-genome sequencing. Appl. Environ. Microbiol. 82, 7030–7040. doi: 10.1128/aem.01486-16
Concha-Meyer, A., Eifert, J., Williams, R., Marcy, J., and Welbaum, G. (2014). Survival of Listeria monocytogenes on fresh blueberries (Vaccinium corymbosum) stored under controlled atmosphere and ozone. J. Food Prot. 77, 832–836. doi: 10.4315/0362-028x.jfp-13-441
Das, E., Gurakan, G. C., and Bayindirli, A. (2006). Effect of controlled atmosphere storage, modified atmosphere packaging and gaseous ozone treatment on the survival of Salmonella Enteritidis on cherry tomatoes. Food Microbiol. 23, 430–438. doi: 10.1016/j.fm.2005.08.002
DeEll, J. R. (2013). Maturity And Storage Of “Honeycrisp” Apples. http://www. omafra.gov.on.ca/english/crops/hort/news/orchnews/2013/on-0913a10.htm#: ∼:text=Soft%20scald%20is%20a%20low,the%20disorder%20continues%20to% 20develop (accessed January 6, 2021).
Deell, J. R., Ayres, J. T., and Murr, D. P. (2008). 1-methylcyclopropene concentration and timing of postharvest application alters the ripening of ‘McIntosh’ apples during storage. Horttechnology 18, 624–630. doi: 10.21273/horttech.18.4.624
Ersoy, Z. G., Barisci, S., and Dinc, O. (2019). Mechanisms of the Escherichia coli and Enterococcus faecalis inactivation by ozone. LWT Food Sci. Technol. 100, 306–313. doi: 10.1016/j.lwt.2018.10.095
FDA (2020a). 21CFR184.1563 Ozone. https://www.accessdata.fda.gov/scripts/cdrh/cfdocs/cfcfr/CFRSearch.cfm?fr=184.1563 (accessed July 27, 2021).
FDA (2020b). Country Fresh Expands Voluntary Recall. https://www.fda.gov/safety/recalls-market-withdrawals-safety-alerts/country-fresh-expands-volun tary-recall (accessed July 27, 2021).
FDA (2008). Compliance Policy Guide (CPG) Sec 555.320 Listeria monocytogenes. https://www.fda.gov/regulatory-information/search-fda-guidance-documents/cpg-sec-555320-listeria-monocytogenes (accessed July 27, 2021).
FDA (2016). Fresh From Texas Recalls Apple Product Because Of Possible Health Risk. https://www.fda.gov/Safety/Recalls/ucm494345.html (accessed January 6, 2021).
FDA (2017). Jack brown Produce, Inc. Recalls Gala, Fuji, Honeycrisp and Golden Delicious Apples Due To Possible Health Risk. https://www.fda.gov/Safety/Recalls/ucm589722.html (accessed January 6, 2021).
FDA (2019). North Bay Produce Voluntarily Recalls Fresh Apples Because Of Possible Health Risk. https://www.fda.gov/safety/recalls-market-withdrawals- safety-alerts/north-bay-produce-voluntarily-recalls-fresh-apples-because-possi ble-health-risk#:∼:text=The%20recalled%20apples%20were%20shipped,North %20Carolina%2C%20Texas%20and%20Wisconsin (accessed January 6, 2021).
Guzel-Seydim, Z. B., Greene, A. K., and Seydim, A. C. (2004). Use of ozone in the food industry. LWT Food Sci. Technol. 37, 453–460. doi: 10.1016/j.lwt.2003.10.014
Han, Y., Floros, J. D., Linton, R. H., Nielsen, S. S., and Nelson, P. E. (2002). Response surface modeling for the inactivation of Escherichia coli O157 : H7 on green peppers (Capsicum annuum) by ozone gas treatment. J. Food Sci. 67, 1188–1193. doi: 10.1111/j.1365-2621.2002.tb09475.x
Juhnevica-Radenkova, K., Radenkovs, V., Kundzins, K., and Seglina, D. (2019). Effect of ozone treatment on the microstructure, chemical composition and sensory quality of apple fruits. Food Sci. Technol. Int. 25, 252–267. doi: 10.1177/1082013218815285
Jung, S. K., and Watkins, C. B. (2011). Involvement of ethylene in browning development of controlled atmosphere-stored ‘Empire’ apple fruit. Postharvest Biol. Technol. 59, 219–226. doi: 10.1016/j.postharvbio.2010.08.019
Kasai, S., and Arakawa, O. (2010). Antioxidant levels in watercore tissue in ‘Fuji’ apples during storage. Postharvest Biol. Technol. 55, 103–107. doi: 10.1016/j.postharvbio.2009.08.008
Kreske, A. C., Ryu, J. H., and Beuchat, L. R. (2006). Evaluation of chlorine, chlorine dioxide, and a peroxyacetic acid-based sanitizer for effectiveness in killing Bacillus cereus and Bacillus thuringiensis spores in suspensions, on the surface of stainless steel, and on apples. J. Food Prot. 69, 1892–1903. doi: 10.4315/0362-028x-69.8.1892
Ku, K. M., Chiu, Y. C., Shen, C. L., and Jenks, M. (2020). Leaf cuticular waxes of lettuce are associated with reduced attachment of the foodborne pathogen Salmonella spp. at harvest and after postharvest storage. LWT Food Sci. Technol. 117:108657. doi: 10.1016/j.lwt.2019.108657
Macauley, J. J., Qiang, Z. M., Adams, C. D., Surampalli, R., and Mormile, M. R. (2006). Disinfection of swine wastewater using chlorine, ultraviolet light and ozone. Water Res. 40, 2017–2026. doi: 10.1016/j.watres.2006.03.021
Marlow, G. C., and Loescher, W. H. (1984). Watercore. Hortic. Rev. 6, 189–251. doi: 10.1002/9781118060797.ch6
Marus, J. R., Bidol, S. M. A. S., Oni, O., Parker-Strobe, N., Otto, M., Pereira, E., et al. (2019). Notes From The Field: Outbreak Of Listeriosis Likely Associated With Prepackaged Caramel Apples-United States, 2017. https://www.cdc.gov/mmwr/volumes/68/wr/mm6803a5.html (accessed January 6, 2021).
Mattheis, J. P. (2008). How 1-methylcyclopropene has altered the washington state apple industry. Hortscience 43, 99–101. doi: 10.21273/hortsci.43.1.99
Mir, N. A., Curell, E., Khan, N., Whitaker, M., and Beaudry, R. M. (2001). Harvest maturity, storage temperature, and 1-MCP application frequency alter firmness retention and chlorophyll fluorescence of ‘Redchief Delicious’ apples. J. Am. Soc. Hortic. Sci. 126, 618–624.
Murray, K., Moyer, P., Wu, F., Goyette, J. B., and Warriner, K. (2018). Inactivation of Listeria monocytogenes on and within apples destined for caramel apple production by using sequential forced air ozone gas followed by a continuous advanced oxidative process treatment. J. Food Prot. 81, 357–364. doi: 10.4315/0362-028x.jfp-17-306
Niemira, B. A., Fan, X. T., and Sokorai, K. J. B. (2005). Irradiation and modified atmosphere packaging of endive influences survival and regrowth of Listeria monocytogenes and product sensory qualities. Radiat. Phys. Chem. 72, 41–48. doi: 10.1016/j.radphyschem.2004.01.005
Razavilar, V., and Genigeorgis, C. (1992). Interactive effect of temperature, atmosphere and storage time on the probability of colony formation on blood agar by four Listeria species. J. Food Prot. 55, 88–92. doi: 10.4315/0362-028x-55.2.88
Selma, M. V., Ibanez, A. M., Cantwell, M., and Suslow, T. (2008). Reduction by gaseous ozone of Salmonella and microbial flora associated with fresh-cut cantaloupe. Food Microbiol. 25, 558–565. doi: 10.1016/j.fm.2008.02.006
Serradilla, M. J., Villalobos, M. D., Hernandez, A., Martin, A., Lozano, M., and Cordoba, M. D. (2013). Study of microbiological quality of controlled atmosphere packaged ‘Ambrunes’ sweet cherries and subsequent shelf-life. Int. J. Food Microbiol. 166, 85–92. doi: 10.1016/j.ijfoodmicro.2013.06.006
Shank, F. R., Elliot, E. L., Wachsmuth, I. K., and Losikoff, M. E. (1996). US position on Listeria monocytogenes in foods. Food Control 7, 229–234. doi: 10.1016/s0956-7135(96)00041-2
Shen, X., Sheng, L., Gao, H., Hanrahan, I., Suslow, T., and Zhu, M.-J. (2019). Enhanced efficacy of peroxyacetic acid against Listeria monocytogenes on fresh apples at elevated temperature. Front. Microbiol. 10:1196. doi: 10.3389/fmicb.2019.01196
Sheng, L. N., and Zhu, M. J. (2021). Practical in-storage interventions to control foodborne pathogens on fresh produce. Compr. Rev. Food Sci. Food Saf. 20, 3135–4210.
Sheng, L., Edwards, K., Tsai, H. C., Hanrahan, I., and Zhu, M. J. (2017). Fate of Listeria monocytogenes on fresh apples under different storage temperatures. Front. Microbiol. 8:1396. doi: 10.3389/fmicb.2017.01396
Sheng, L., Hanrahan, I., Sun, X., Taylor, M. H., Mendoza, M., and Zhu, M.-J. (2018). Survival of Listeria innocua on Fuji apples under commercial cold storage with or without low dose continuous ozone gaseous. Food Microbiol. 76, 21–28. doi: 10.1016/j.fm.2018.04.006
Singh, N., Singh, R. K., Bhunia, A. K., and Stroshine, R. L. (2002). Efficacy of chlorine dioxide, ozone, and thyme essential oil or a sequential washing in killing Escherichia coli O157 : H7 on lettuce and baby carrots. LWT Food Sci. Technol. 35, 720–729. doi: 10.1006/fstl.2002.0933
Tasdelen, O., and Bayindirli, L. (1998). Controlled atmosphere storage and edible coating effects on storage life and quality of tomatoes. J. Food Process. Preserv. 22, 303–320. doi: 10.1111/j.1745-4549.1998.tb00352.x
Tuffi, R., Lovino, R., Canese, S., Cafiero, L., and Vitali, F. (2012). Effects of exposure to gaseous ozone and negative air ions on control of epiphytic flora and the development of Botrytis cinerea and Penicillium expansum during cold storage of strawberries and tomatoes. Ital. J. Food Sci. 24:102.
USAA (2019). Apple Industry At-A-Glance: Top 10 Varieties Produced In U.S. http://usapple.org/the-industry/apple-industry-at-a-glance/ (accessed January 6, 2021).
USDA-NOP (2021). §205.605; Nonagricultural (Nonorganic) Substances Allowed As Ingredients In Or On Processed Products Labeled As “Organic” Or “Made With Organic (Specified Ingredients Or Food Group(s)).”. https://www.ecfr.gov/cgi-bin/text-idx?SID=a6a0935ddf00e166695f4c2138bd58d8&mc=true&node=pt7.3.205&rgn=div5#se7.3.205_1270 (accessed January 6, 2021).
Watkins, C. B., Brookfield, P. L., and Harker, F. R. (1992). “Development of maturity indices for the ‘Fuji’apple cultivar in relation to watercore incidence,” in International Symposium on Pre-and Postharvest Physiology of Pome-fruit, ed. R. Marcelle (St.-Truiden: ISHS), 267–276. doi: 10.17660/actahortic.1993.326.29
Xuan, H., and Streif, J. (2005). Effect of 1-MCP on the respiration and ethylene production as well as on the formation of aroma volatiles in ‘Jonagold’ apple during storage. Acta Hortic. 682, 1203–1210. doi: 10.17660/actahortic.2005.682.160
Yaseen, T., Ricelli, A., Turan, B., Albanese, P., and D’onghia, A. M. (2015). Ozone for post-harvest treatment of apple fruits. Phytopathol. Mediterr. 54, 94–103.
Keywords: Red Delicious apple, Listeria innocua, 1-methylcyclopropene, gaseous ozone, quality
Citation: Shen X, Su Y, Hua Z, Sheng L, Mendoza M, He Y, Green T, Hanrahan I, Blakey R and Zhu M-J (2021) Effectiveness of Low-Dose Continuous Gaseous Ozone in Controlling Listeria innocua on Red Delicious Apples During 9-Month Commercial Cold Storage. Front. Microbiol. 12:712757. doi: 10.3389/fmicb.2021.712757
Received: 21 May 2021; Accepted: 18 August 2021;
Published: 29 September 2021.
Edited by:
Mostafa Ghanem, University of Maryland, College Park, United StatesReviewed by:
Loris Pinto, Italian National Research Council, ItalyAlejandro Castillo, Texas A&M University, United States
Copyright © 2021 Shen, Su, Hua, Sheng, Mendoza, He, Green, Hanrahan, Blakey and Zhu. This is an open-access article distributed under the terms of the Creative Commons Attribution License (CC BY). The use, distribution or reproduction in other forums is permitted, provided the original author(s) and the copyright owner(s) are credited and that the original publication in this journal is cited, in accordance with accepted academic practice. No use, distribution or reproduction is permitted which does not comply with these terms.
*Correspondence: Mei-Jun Zhu, meijun.zhu@wsu.edu