- 1Division of Bacteriology, Chiba Prefectural Institute of Public Health, Chiba, Japan
- 2Pathogen Genomics Center, National Institute of Infectious Diseases, Tokyo, Japan
- 3Division of Bacteriology, Ibaraki Prefectural Institute of Public Health, Mito, Japan
- 4Department of Microbiology, Tokyo Metropolitan Institute of Public Health, Tokyo, Japan
- 5Microbiological Testing and Research Division, Yokohama City Institute of Public Health, Yokohama, Japan
- 6Itako Public Health Center, Itako, Japan
- 7Laboratory of Cancer Genetics, Chiba Cancer Center Research Institute, Chiba, Japan
- 8Santé Publique France, The French National Public Health Agency, Saint-Maurice, France
- 9Center for Surveillance, Immunization, and Epidemiologic Research, National Institute of Infectious Diseases, Tokyo, Japan
- 10Faculty of Health Science, School of Medical Technology, Gunma Paz University, Takasaki, Japan
- 11French National Reference Center for E. coli, Shigella and Salmonella, Institute Pasteur, Paris, France
- 12Groupe de Recherche sur l’Adaptation Microbienne (GRAM 2.0, EA2656), Normandy University, UNICAEN, UNIROUEN, Caen, France
- 13Center for Emergency Preparedness and Response, National Institute of Infectious Diseases, Musashi-Murayama, Japan
In Japan’s Kanto region, the number of Salmonella enterica serovar Chester infections increased temporarily between 2014 and 2016. Concurrently with this temporal increase in the Kanto region, S. Chester isolates belonging to one clonal group were causing repetitive outbreaks in Europe. A recent study reported that the European outbreaks were associated with travelers who had been exposed to contaminated food in Morocco, possibly seafood. Because Japan imports a large amount of seafood from Morocco, we aimed to establish whether the temporal increase in S. Chester infections in the Kanto region was associated with imported Moroccan seafood. Short sequence reads from the whole-genome sequencing of 47 S. Chester isolates from people in the Kanto region (2014–2016), and the additional genome sequences from 58 isolates from the European outbreaks, were analyzed. The reads were compared with the complete genome sequence from a S. Chester reference strain, and 347 single nucleotide polymorphisms (SNPs) were identified. These SNPs were used in this study. Cluster and Bayesian cluster analyses showed that the Japanese and European isolates fell into two different clusters. Therefore, ΦPT and values were calculated to evaluate genetic differences between these clusters. The results revealed that the Japanese and European isolates were genetically distinct populations. Our root-to-tip analysis showed that the Japanese isolates originating from one clone had accumulated mutations, suggesting that an emergence of this organism occurred. A minimum spanning tree analysis demonstrated no correlation between genetic and geographical distances in the Japanese isolates, suggesting that the emergence of the serovar in the Kanto region did not involve person-to-person contact; rather, it occurred through food consumption. The dN/dS ratio indicated that the Japanese strain has evolved under positive selection pressure. Generally, a population of bacterial clones in a reservoir faces negative selection pressure. Therefore, the Japanese strain must have existed outside of any reservoir during its emergence. In conclusion, S. Chester isolates originating from one clone probably emerged in the Kanto region via the consumption of contaminated foods other than imported Moroccan seafood. The emerging strain may have not established a reservoir for survival in the food supply chain resulting in its disappearance after 2017.
Introduction
The number of Salmonella enterica serovar Chester infections increased temporarily during 2014–2016 in the Kanto region of Japan. We reported that an outbreak in 2016 from an unknown infection source was caused by S. enterica subsp. enterica serovar Chester in a part of the aforementioned Japanese region (Aoki et al., 2020). In this outbreak, seven S. Chester infection cases occurred in the adjacent prefectures of Chiba and Ibaraki in October and November of 2016. No epidemiological link among the cases except for an intrafamily case was found. Simultaneously, pulsed-field gel electrophoresis (PFGE) showed that all 10 of the isolates had almost identical band patterns (Aoki et al., 2020), which raises the possibility that these isolates originated from one clone. Non-typhoidal Salmonella (NTS) is a significant cause of foodborne illness worldwide (World Health Organization, 2018)1. In Japan, infections with S. Chester have been rare among all reported NTS infections (Katoh et al., 2015). Nevertheless, the number of S. Chester isolates in Tokyo, a part of the Kanto region, increased temporarily in 2014–2016 (Tokyo Metropolitan Infectious Disease Surveillance Center, 2020)2, while they did also in other areas of Kanto region, that is, Chiba Prefecture, Ibaraki Prefecture and Yokohama City. In the period, 44 S. Chester isolates were isolated in the Kanto region, which included nine isolates from the seven infection cases that we reported previously (Aoki et al., 2020), although S. Chester was not isolated before or after this period. Previously, the number of S. Oranienburg isolates, another rarely isolated serovar in Japan, increased in various areas of Japan in 1998–1999; subsequently, PFGE analysis demonstrated that the temporary increase was caused by a diffuse outbreak (Tsuji and Hamada, 1999). Therefore, the S. Chester isolates that emerged during 2014–2016 might belong to a single diffuse outbreak and include the outbreak isolates from 2016 (Aoki et al., 2020) in the Kanto region.
Looking outside of Japan, an interesting fact has emerged. In Europe, S. Chester isolates belonging to one clonal group have repetitively caused outbreaks in recent years (Fonteneau et al., 2017). In the summers of 2014 and 2015, a multinational outbreak caused by this bacterium occurred across several countries (e.g., France, Belgium, Netherlands, Spain, Denmark, and Sweden). From April to October in 2016, the number of S. Chester infections increased in France. Fonteneau et al. (2017) reported that most of the S. Chester cases had traveled to Morocco and eaten seafood there before the onset of symptoms. This implies that the S. Chester isolates belonging to the same clonal group had spread continuously in Morocco and that the spreading isolates have caused outbreaks every year in Europe, perhaps via the consumption of Moroccan seafood. As for Japan, a large amount of seafood (e.g., octopus, squid, and eel) has been imported from Morocco (Ministry of Agriculture, Forestry and Fisheries, 2019)3. Some cases of Salmonella spp. infections have previously been caused by seafood and fish consumption (Tsuji and Hamada, 1999; Heinitz et al., 2000). Therefore, we hypothesize that imported seafood from Morocco was one of the vehicle candidates for S. Chester infections in the Kanto region of Japan during 2014–2016; that is, the temporary increase in the infections in the Kanto region might be caused by the S. Chester isolates spreading in Morocco.
Several studies have recently shown the superiority of whole genome sequencing (WGS) data compared with PFGE data. PFGE has generally been used to determine whether bacterial isolates originate from the same outbreak. However, PFGE can only analyze nucleotide differences between isolates at a relatively small number of restriction enzyme sites (Kudva et al., 2004). Therefore, PFGE has sometimes failed to differentiate isolates originating from the same outbreak, that is, isolates derived from one clone, and isolates originating outside the outbreak. During 2014–2015, enterohemorrhagic Escherichia coli (EHEC) O121 isolates with similar PFGE patterns were isolated in various Japanese regions. Analysis of single nucleotide polymorphism (SNP) extracted from WGS data showed that only some of EHEC O121 isolates were derived from a single clone, while the WGS data showed that the remaining isolates were not from this clone (Lee et al., 2017). The superiority of WGS data analysis for molecular epidemiological analysis was also apparent in the cases of EHEC O157 (Yokoyama et al., 2018) and S. Agona (Yokoyama et al., 2019).
Therefore, the aim of this study was to clarify whether the temporal increase in S. Chester infections in the Kanto region of Japan was associated with imported seafood consumption from Morocco. First, we performed molecular epidemiological and population genetic analyses using WGS data to elucidate the relationship between S. Chester isolates from the Kanto region and those from Europe. Second, we investigated whether a correlation exists between the genetic and geographical distances of the Japanese isolates using a molecular epidemiological analysis to confirm that the Kanto region’s temporal increase occurred via contaminated food. Third, our WGS data was used to assess whether selective pressure featured in the outbreaks involving S. Chester. We then discuss why the S. Chester isolates emerging in the Kanto region during 2014–2016 were not isolated after 2017.
Materials and Methods
Collection of S. Chester Isolates Isolated in the Kanto Region of Japan
Salmonella Chester isolates isolated in the Kanto region (47 isolates) during 2014–2016 were used in this study (Supplementary Table 1 and Supplementary Figure 1). A traditional contact survey was carried out by local health centers in each municipality. When a local public health institute in Japan receives a report that a food poisoning or infection case has occurred, the institute microbiologically examines the pathogens to identify which one(s) is/are causing the case. For this study, we made inquiries to all local public health institutes in the Kanto region as to whether the number of S. Chester isolates derived from human and food sources had increased recently. On the inquiry, it became clear that the number of S. Chester isolates from humans increased in Tokyo Metropolitan, Chiba Prefecture, Ibaraki Prefecture and Yokohama City during 2014–2016. However, S. Chester isolates were not isolated by these institutes before or after this period. In this study, nine S. Chester isolates from people in Tokyo, 18 from Chiba, 11 from Ibaraki, and nine from Yokohama were collected (Supplementary Table 1).
SNP Detection From WGS Data
The short sequence reads from 58 European S. Chester isolates (ST1954) that were collected from humans, chickens and food in the multinational European outbreaks were obtained in a previous study (Fonteneau et al., 2017). These reads are deposited in the National Center for Biotechnology Information Short Read Archive (BioProject PRJNA248792). The short sequence reads from the European isolates were kindly provided by the Institute Pasteur (France) to the National Institute of Infectious Diseases (NIID), Japan.
As for the Japanese isolates, the short sequence reads from the 47 S. Chester isolates from the Kanto region of Japan were obtained by the NIID. DNA was extracted from the Japanese isolates and purified using the Isoplant II DNA extraction kit (Nippon Gene Inc., Tokyo, Japan). The genomic DNA libraries were prepared using the Nextera XT DNA sample prep kit (Illumina). The pooled libraries were subjected to multiplexed paired-end sequencing (300 mer × 2) using MiSeq (Illumina, San Diego, CA, United States). The adapter sequences were trimmed from the short reads, and low quality bases with Phred scores less than 15 were eliminated using the Skewer program (Jiang et al., 2014) to obtain sequences of at least 50-mers in length. In the NIID, the short reads from the Japanese and European isolates were mapped using the BWA-mem program (Li and Durbin, 2010) against the S. Chester ATCC 11997 strain’s complete genome sequence (4,660,922 bp, GenBank accession number CP019178.1) as a reference (Supplementary Table 2). After excluding prophage, repeat, indel, biallelic, and recombination regions from the complete genome sequence, 91.122% (4,293,757 bp) of the genome was used for variant detection. Variant calling was performed using VarScan ver. 2.3.4 (Koboldt et al., 2009), resulting in the identification of 347 SNPs (Supplementary Table 3). Out of the 347 SNPs, 120 and 271 SNPs were detected in the Japanese and European isolates, respectively. Forty-four SNPs were common to both the Japanese and European isolates. The 347 SNPs were used for further analyses in this study.
Molecular Epidemiology
To perform multilocus sequence typing (MLST) on the Japanese isolates, the assembled sequence contigs from the A5-MiSeq pipeline (Coil et al., 2015) were analyzed using the web tools available from the Center for Genomic Epidemiology website4 (Larsen et al., 2012). The MLST classified the Salmonella isolates into sequence types (STs) based on the analysis of seven housekeeping genes (i.e., aroC, dnaN, hemD, hisD, purE, sucA, and thrA) (Achtman et al., 2012). The chromosomal positions of these housekeeping genes in the S. Chester ATCC 11997 strain (Accession number CP019178.1) were 1,427,763–1,428,848 for aroC, 1,406–2,506 for dnaN, 4,565,282–4,566,022 for hemD, 1,788,720–1,790,024 for hisD, 1,788,720–1,790,024 for purE, and 3,104,010–3,106,100 for sucA, and 3,872,960–3,873,009 for thrA. The European isolates were classified as belonging to ST1954 based on the MLST analysis in our previous study (Fonteneau et al., 2017).
Cluster analysis was conducted using MEGA 7 software (Kumar et al., 2016). The 347 SNPs from the Japanese and European isolates were imported into the software, and a dendrogram was reconstructed by the maximum-likelihood method with the Tamura-Nei model and with 1,000 bootstraps performed for the statistics. The other conditions for reconstructing the dendrogram were set by the default settings.
Bayesian cluster analysis using the 347 SNPs from the Japanese and European isolates was conducted using STRUCTURE Version 2.3.4 software (Pritchard et al., 2000). The setting options were as follows: length of burn-in period was 50,000, the Markov Chain Monte Carlo number was 100,000, the ancestry model was the “admixture model,” and the frequency model was “allele frequency correlated.” To determine the number of clusters (K) to show how the isolates could be grouped, delta K was calculated using STRUCTURE Harvester Ver. 0.6.93 software (Earl and von Holdt, 2012).
Population Genetic Analysis
The difference between the Japanese and European isolates was evaluated using ΦPT, which is an analogue of Fst (Peakall and Smouse, 2012). Genetic Analysis in Excel (GenAlEx), version 6.51b2 software, an add-on package from Microsoft Excel, was used to calculate a ΦPT value with 999 permutations. ΦPT was calculated as the proportion of the variance among populations from:
Where VAP was the variance among the populations and VWP was the variance within a population. If a ΦPT value differed significantly from zero, the Japanese and European isolates would be different populations.
Linkage disequilibrium in the Japanese and European isolates was evaluated using the standardized index of association () (Haubold and Hudson, 2000). LIAN Ver. 3.7 software was used to calculate for each of the Japanese and European isolates from the ratio of the variance of the observed mismatches in the test set (VD) to the variance expected for a state of linkage equilibrium (Ve), scaled by the number of loci used in the analysis (l), from:
The significance between VD and Ve was determined by the Monte Carlo simulations with 103 resamplings.
Comparison of Genetic Traits Between the Japanese and European Isolates
A root-to-tip analysis was performed using Path-O-Gen software to evaluate whether changes occurred in the diversity of the Japanese and European isolates as time proceeds (Rambaut et al., 2016). In the root-to-tip analysis, the X-axis shows the isolation date for each isolate, and the Y-axis shows the genetic distance to each isolate from the root on the dendrogram. If the isolates originating from the same clone are assumed to have a uniform mutation rate, they would show a proportionate relationship between the X-and Y-axes.
The frequencies of the SNPs causing non-synonymous (PN) and synonymous (PS) substitutions in the amino acids were compared to evaluate the selection pressure on each of the Japanese and European isolates as follows (Bierne and Eyre-Walker, 2004). Of the 347 SNP loci detected in the WGS data, 300 and 47 of them were on coding and non-coding regions, respectively. Out of the 300 SNPs, there were two types of nucleotide bases in 66 of the SNPs in the sequences from the Japanese isolates and 210 from the European isolates. LN and Ls represent the number of SNP loci causing non-synonymous and synonymous substitutions, respectively. We designated the minor allele to be the lesser allele belonging to the two alleles in a SNP locus. If two alleles in a SNP locus are equal, either allele was designated as the minor allele. SLN x and SLS x represents the number of Japanese and European isolates where the minor alleles at the xth SNP locus cause non-synonymous and synonymous substitutions, respectively. N is the number of Japanese and European isolates. PN and PS can be calculated from:
When the dN/ dS ratios were >1 and <1, the genes possessed by each group were considered to have been subjected to positive or negative selection, respectively (Stukenbrock and Bataillon, 2012). Where dN/dS = 1 this indicates that the group has evolved under neutral evolution.
A minimum spanning tree (MST) analysis was performed using PopART software Version 1.7 (Leigh and Bryant, 2015) to evaluate how the Japanese isolates emerged geographically as the time advanced. All the SNPs in the sequences from the Japanese isolates were imported into the software, and an MST was reconstructed using the default epsilon value (0).
The presence of SNPs in four genes (i.e., mutS, mutL, mutH, and uvrD) within the mismatch repair system (Sheng et al., 2020) was investigated. The chromosomal positions of these genes in the S. Chester strain ATCC 11997 (Accession number CP019178.1) were 956,162–958,729 for mutS, 4,121,661–4,123,517 for mutL, 3,712,806–3,713,201 for mutT, and 4,551,149–4,553,311 for uvrD. When at least one SNP was located in these genes, we examined whether the SNP caused either non-synonymous or synonymous substitutions.
DNA Sequence Data Deposition
The short sequence reads from the 47 Japanese isolates have been deposited in the Sequence Read Archive in the DNA Data Bank of Japan (Supplementary Table 4).
Results
Discrimination of the Japanese and European Isolates
The MLST analysis showed that all the Japanese isolates belong to ST1954, which is the same sequence type as that of the European isolates (Fonteneau et al., 2017). In detail, the nucleotide sequences in each of the seven housekeeping genes (i.e., aroC, dnaN, hemD, hisD, purE, sucA, and thrA) were exactly the same among the Japanese and European isolates. This MLST result is consistent with no SNPs being detected in these seven genes among the Japanese and European isolates, as determined by mapping the short sequence reads from these isolates against the reference genome sequence (Supplementary Table 3).
The cluster analysis showed that the Japanese and European isolates fell into two clusters (i.e., clusters A and B), one of which only contains the Japanese isolates (cluster A), while the other contains only the European isolates (cluster B) (Figure 1). We found that cluster B includes the S. Chester isolates from travelers to Morocco, one chicken isolate from Morocco, and two isolates derived from food in Belgium. In this dendrogram, there were no S. Chester isolates that were not included in cluster A or B. The genetic distances from the root to clusters A and B were 0.052 and 0.043, respectively. Therefore, the cut-off value for the genetic distance we used to define a cluster was determined to be 0.04 in this study. The maximum pair-wise genetic distance between the S. Chester isolates in cluster A (i.e., isolate 29149) and cluster B (i.e., 201110105) was 0.327 (Supplementary Table 5). The pair-wise comparison of the genetic distances between all 105 S. Chester isolates is shown in Supplementary Table 5.
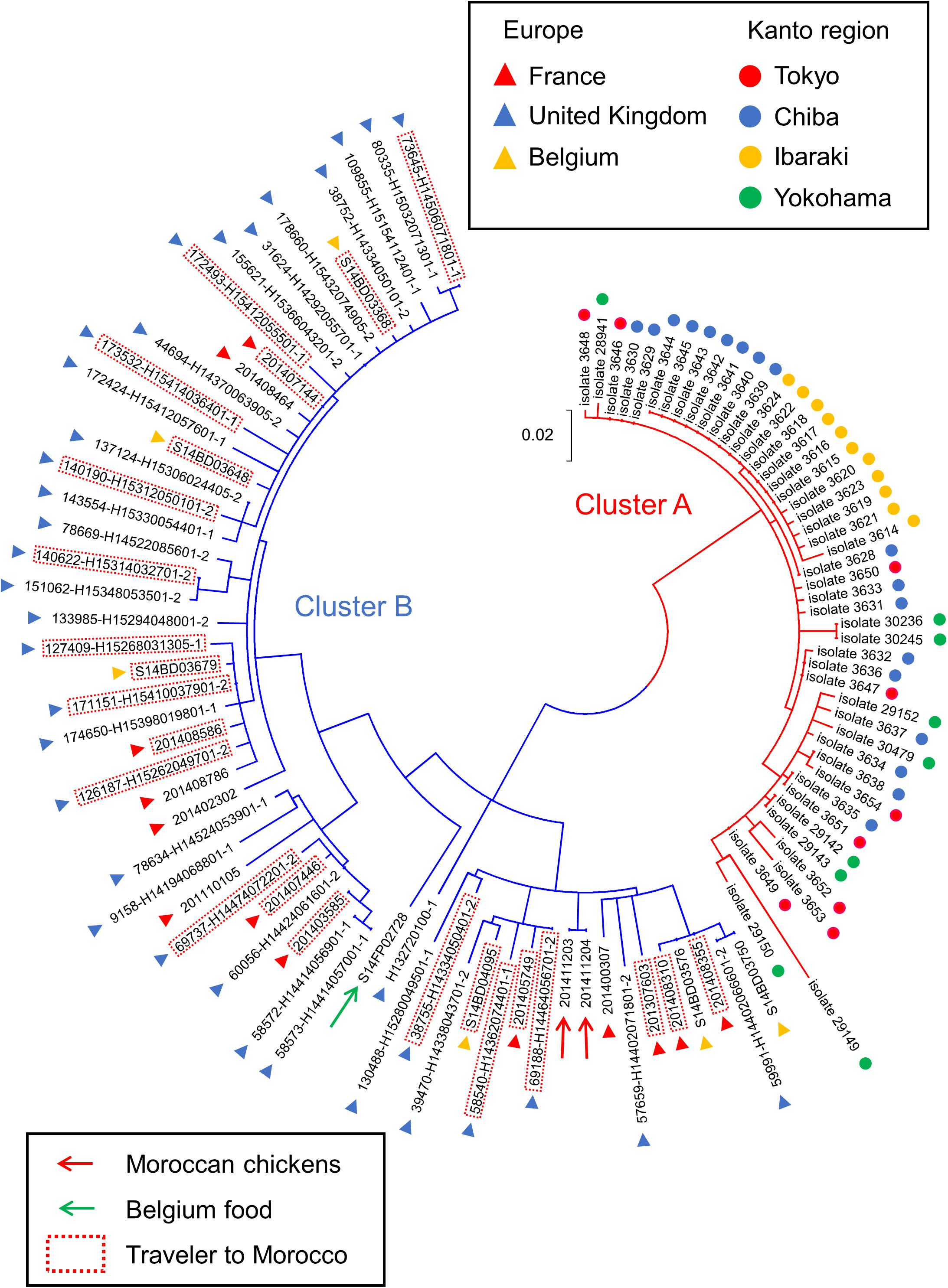
Figure 1. Dendrogram reconstructed from SNPs from Salmonella Chester isolated in the Kanto region of Japan and Europe. Branches for the isolates included in clusters A and B are indicated in red and blue, respectively. Countries and areas where the isolates were collected are indicated by colored circles and triangles. Isolates derived from travelers to Morocco are surrounded by red dotted lines. Red and green arrows show the isolates obtained from chickens or food, respectively. The scale bar indicates the genetic distance.
The division of the two clusters on the dendrogram was also confirmed by Bayesian cluster analysis. This analysis showed that the highest Delta K value was obtained when a population was partitioned in two sub-populations (Figure 2A). Among the 10 runs under the assumption of K = 2, the highest log likelihood value was obtained in the third run with ln P(D) = −3479.8. In the third run, when the isolates were ordered according to their posterior probability values (Q) (Supplementary Table 1), they formed two groups that were completely concordant with the two clusters on the dendrogram (Figure 2). The Q values of all the Japanese isolates in group A and the values of all the Europe isolates in group B were >0.99.
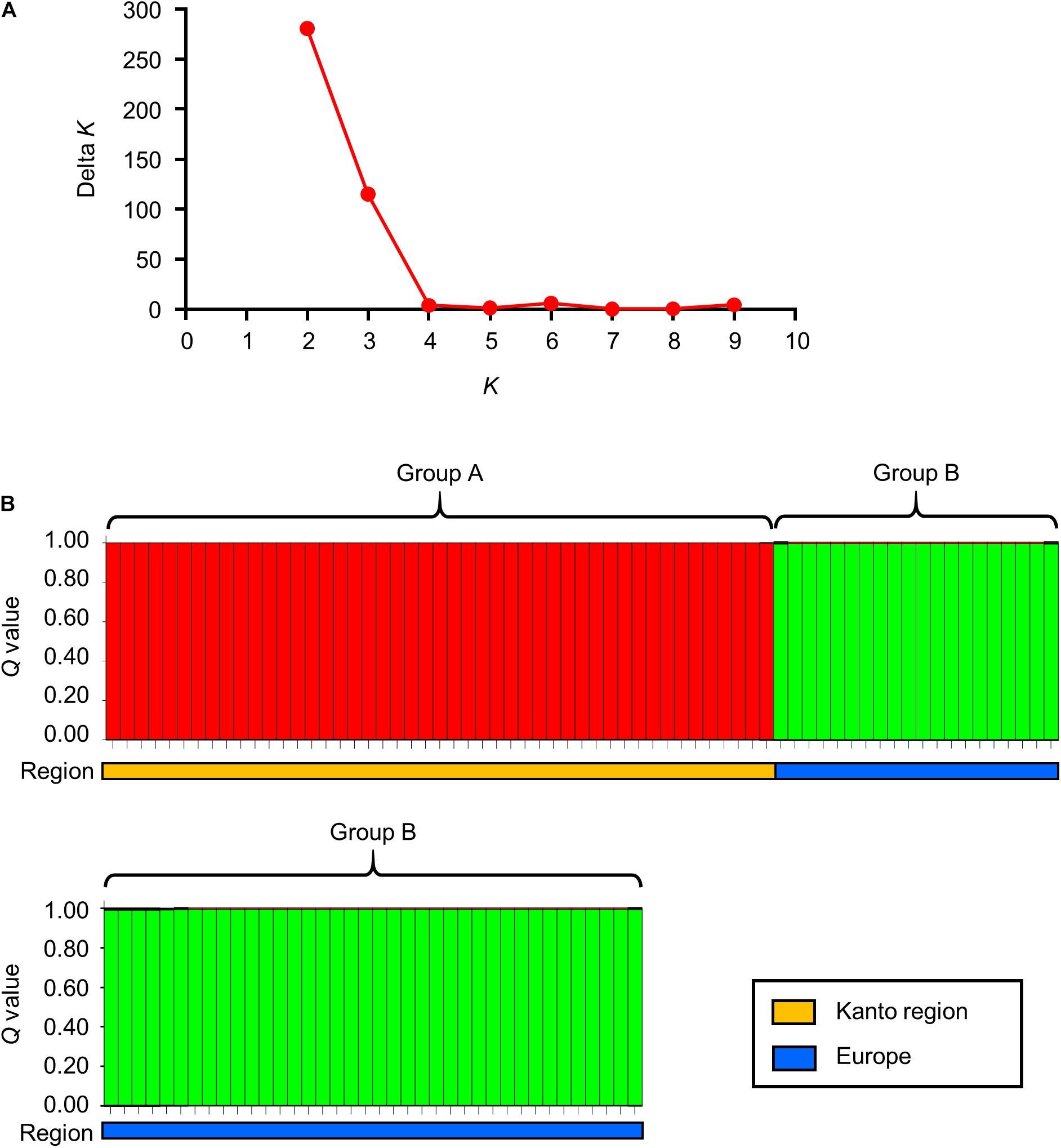
Figure 2. Bayesian cluster analysis of S. Chester isolated in the Kanto region of Japan and Europe. (A) This graph represents Delta K (Y-axis) against the assumed sub-populations (X-axis). (B) This panel shows the genetic structure of the 105 S. Chester isolates from the Kanto region and from Europe under the assumption of K = 2. (B) This panel contains 105 red and light green squares, and the height of each square indicates the posterior probability value (Q) of the isolate. These red and light green squares represent groups A and B, respectively. The orange and blue bars below the 105 squares indicate the region where each isolate originated (i.e., the Kanto region or Europe). The details of this analysis are described in Supplementary Table 1.
The division of the Japanese and European isolates into these two groups was supported by the ΦPT value. The value was 0.700 (P = 0.001), indicating that the two groups belonged to different populations. Furthermore, the values showed that the linkage disequilibrium in each group was significantly greater than zero (Table 1), indicating that the Japanese and European isolates could have diverged from different most recent common ancestors.
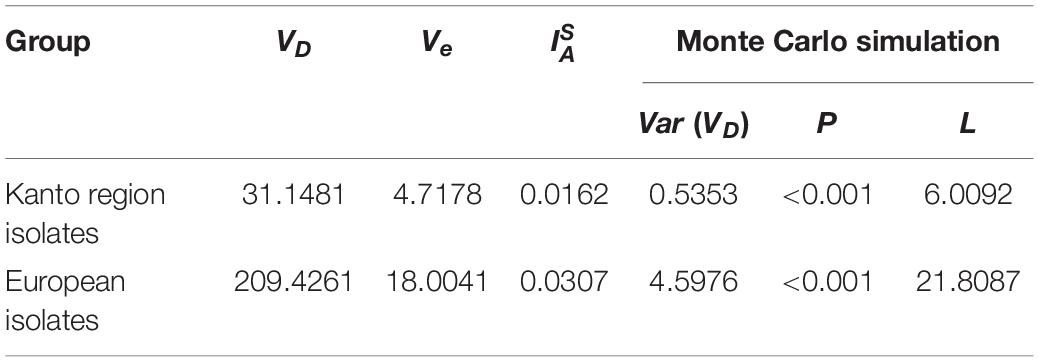
Table 1. Linkage disequilibrium for single nucleotide polymorphism (SNPs) from Salmonella Chester isolated in the Kanto region of Japan or Europe.
Different Genetic Traits Between the Japanese and European Isolates
Our root-to-tip analysis revealed a different trend in the diversity change between the Japanese and European isolates. A positive correlation was observed between diversity in the Japanese isolates and their isolation dates, with the exception of isolate 29149 (Figure 3A), indicating that genetic diversity in the Japanese S. Chester isolates (excepting isolate 29149) increased as time advanced after the isolates originated from a single clone. In contrast, no such tendency was found when the European isolates were analyzed (Figure 3B).
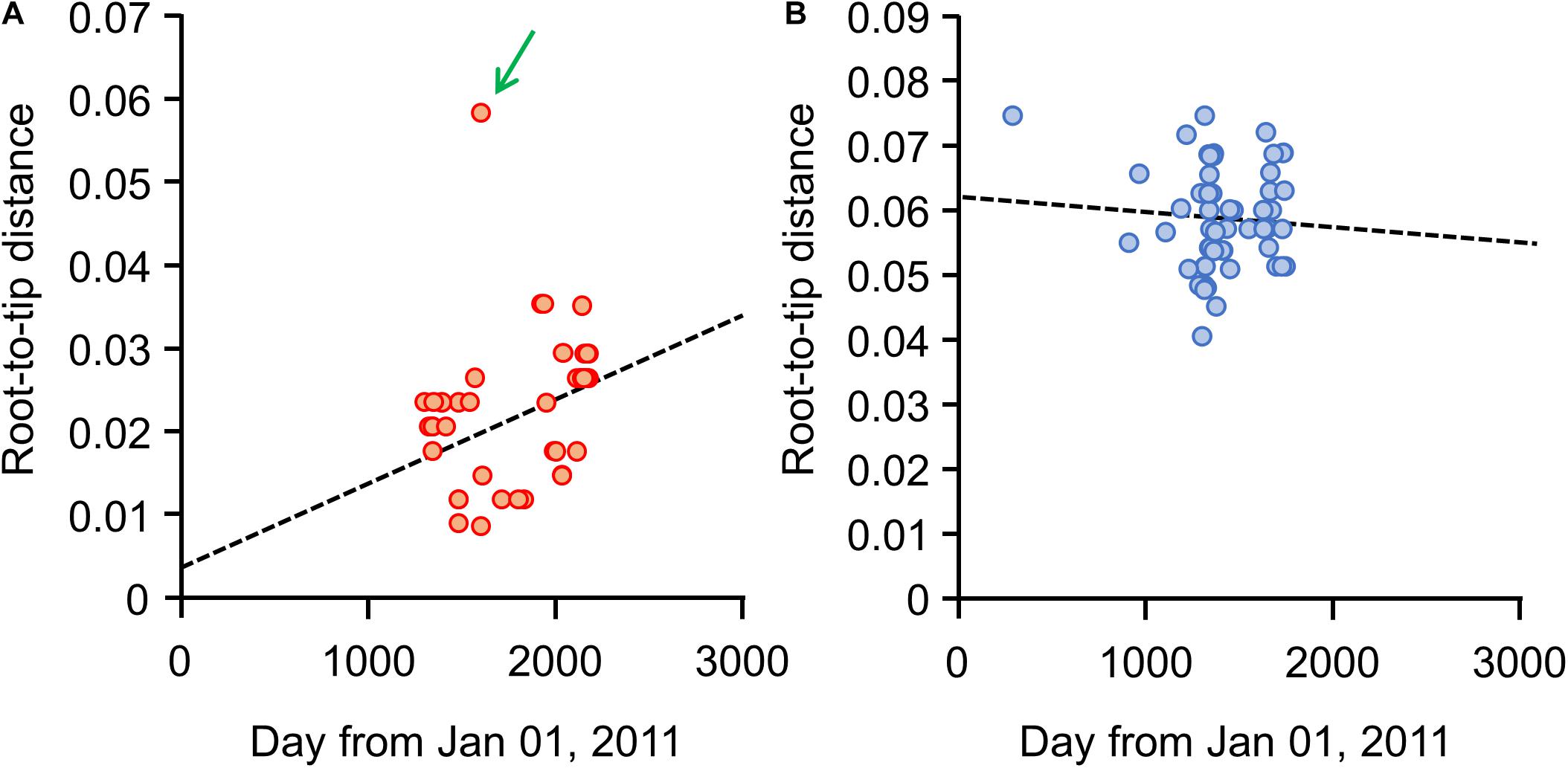
Figure 3. Root-to-tip analysis for each S. Chester isolated in the Kanto region of Japan or Europe. The X-axis represents the isolation date of each S. Chester isolate as the days lapsed from January 01, 2011. The Y-axis represents the genetic distance to each isolate from the root in the dendrogram for isolates from (A) the Kanto region or (B) from Europe. Dotted lines represent the regression lines drawn from (A) the Kanto region’s isolates, excluding isolate 29149, and (B) the European isolates. A green arrow indicates isolate 29149.
The MST analysis also showed that chronological variation accumulated in the Japanese isolates. Because isolate 29149 was set apart from the more clustered positions of the other isolates in the root-to-tip analysis (Figure 3A), it was excluded so as to evaluate the maximum pair-wise distances in the MST (Figures 4A,B). The maximum pair-wise distances between two isolates in 2014, 2015, and 2016 were 3, 12, and 16, respectively (Figure 4A). In contrast, no correlation was observed between genetic distance and geographic distance in the Japanese isolates. The maximum pair-wise distance for the isolates from Tokyo and Chiba was 11, while that of Tokyo and Yokohama was 16 (Figure 4B), although the geographic distances between Tokyo-Chiba and Tokyo-Yokohama (distances between the local authorities’ offices) were 39 and 27 km, respectively.
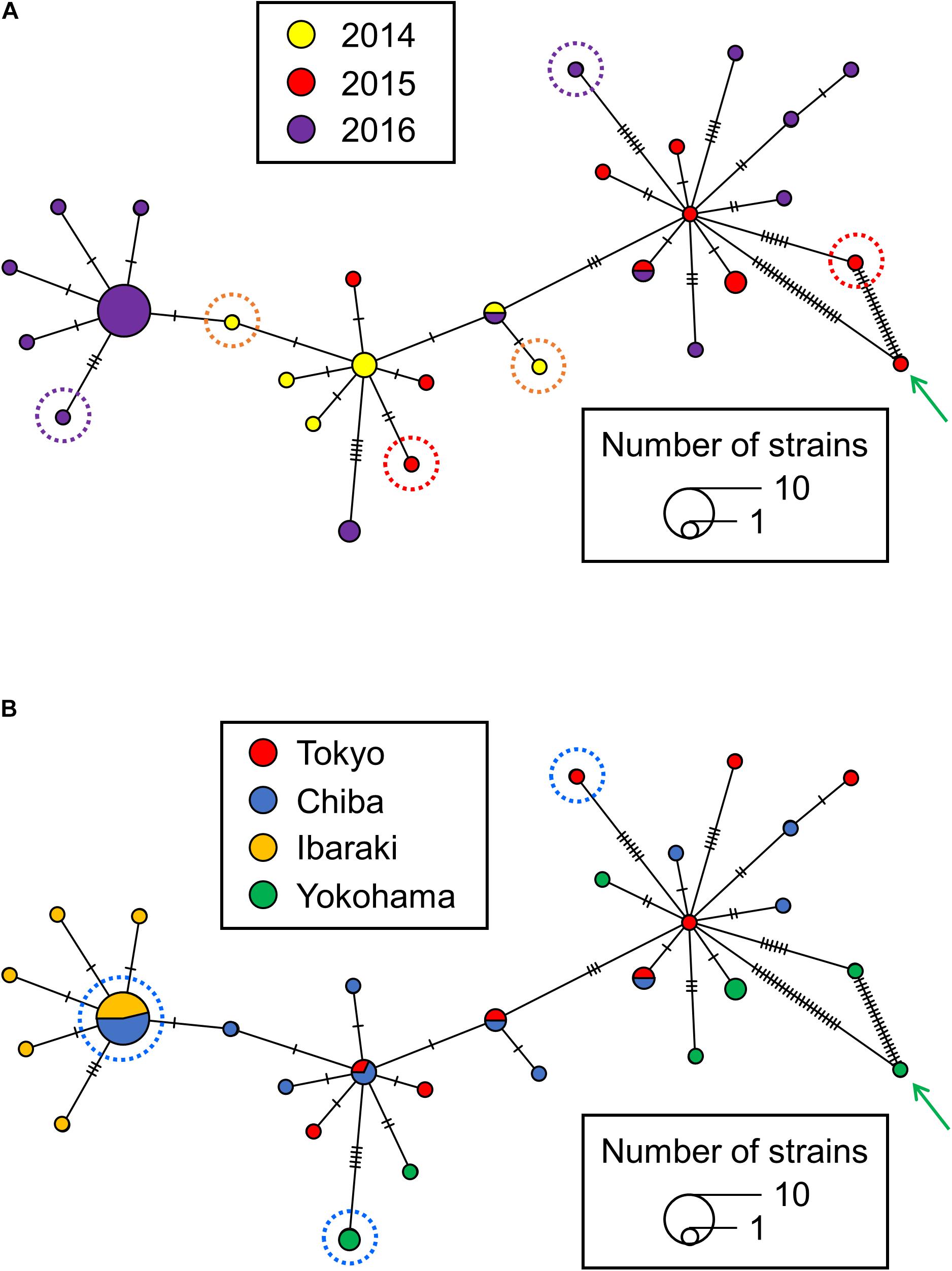
Figure 4. A minimum spanning tree (MST) reconstructed from the single nucleotide polymorphism (SNPs) from S. Chester isolated in the Kanto region of Japan. The colors of the nodes in panels (A,B) indicate the years and areas in which the S. Chester stains were isolated, respectively. (A) The isolates surrounded by orange, red and purple dotted lines show the maximum pair-wise distances between two isolates in 2014, 2015, and 2016, respectively. (B) The isolates surrounded by blue dotted lines show the maximum pair-wise distances of the isolates from Tokyo and Chiba and those of the Tokyo and Yokohama isolates. A green arrow indicates isolate 29149. A crossing bar on a connecting line indicates one SNP.
The dN/dS ratios of the sequences from the Japanese and European isolates were 1.89 and 0.94, respectively. These values indicate that the Japanese isolates have been subjected to positive selection pressure, and that the European isolates have been subjected to negative selection pressure. While positive selection pressure causes individuals in a population to accumulate mutations, negative selection pressure excludes individuals with mutations that differ from the population (Tanner and Kingsley, 2018). No SNPs were located in the mismatch repair system genes (mutS, mutL, mutH, and uvrD) (Supplementary Table 3).
Discussion
Our results refute the hypothesis that the temporary increase in S. Chester infections in the Kanto region of Japan was related to the S. Chester isolates spreading continuously in Morocco. The cluster and Bayesian cluster analyses showed that the Japanese and European isolates fell into two different clusters; thus, it is possible that the Japanese and European isolates are genetically distinct populations. Therefore, population genetic analysis was performed using ΦPT and values to confirm the genetic difference between the Japanese and European isolates in the two clusters. These values revealed that the Japanese and European isolates were genetically different from each other and were derived from each of the most recent ancestors. Fonteneau et al. (2017) suggested that S. Chester isolates belonging to the same clonal group had spread continuously in Morocco and that the spreading isolates (i.e., the European isolates) have caused outbreaks in Europe via Moroccan seafood. Therefore, it is likely that the temporary increase of S. Chester infections in Japan was caused by an infectious source other than the seafood imported from Morocco.
Our study has revealed that the S. Chester isolates originating from one clone emerged in the Kanto region via contaminated food items, which differed from the infection source of the European outbreaks. In general, when bacterial strains emerge via person-to-person contact, genetic distances in the strains are positively correlated with the geographical distances among the places where the strains are isolated (Brown et al., 2019). Conversely, bacterial strains emerging via the food supply chain lack this correlation. In our study, the MST for the Japanese isolates shows no correlation between the genetic and geographical distances in the isolates, suggesting that S. Chester emerged in the Kanto region via the food supply chain but not by person-to-person contact. Generally, the emergence of a foodborne pathogen requires two factors. The first factor is the introduction of a pathogen into a new host (Morse, 1995; Whittam et al., 1998). Newly emerging foodborne pathogens in the environment or in hosts outside of the human food supply chain would be introduced into a new host in the human food supply chain. However, there is no evidence that the Japanese isolates were introduced into a new host via the food supply chain because S. Chester was not reported in livestock and food samples by the national surveillance systems during its new emergence in the Kanto region between 2014 and 2016 (Ministry of Agriculture, Forestry and Fisheries, 2018; National Veterinary and Assay Laboratory, 2020)56. The second factor relating to new emergence is the acquisition of genetic variations (Whittam et al., 1998). The root-to-tip analysis showed that the Japanese isolates originating from one clone accumulated mutations over time, suggesting that they can be defined as one strain and that this strain met the second factor’s requirement. However, it seems likely that the newly emerging S. Chester isolates were unable to establish themselves and adapt to their new environment because none were isolated after 2017 in Japan at the point of writing this paper.
The results of our tests to detect selective pressure (the dN/dS ratio) acting on the isolates may explain why the Japanese S. Chester strain was not isolated after 2017. In general, the population of bacterial clones in a reservoir of persistent infection is subject to negative selection pressure, and the pressure excludes some clones with mutations that differ from the population (Tanner and Kingsley, 2018). Conversely, when a population of bacterial clones is subject to positive selection pressure outside the reservoirs, the pressure causes the clones to accumulate mutations (i.e., the diversity of the population increases). The dN/dS ratio for the Japanese strain was >1, indicating that the genes possessed by these isolates have been subjected to positive selection (Stukenbrock and Bataillon, 2012). Therefore, the emerging Japanese strain has been under positive selection pressure, suggesting that it existed outside of any reservoir during its emergence. As to why S. Chester was not isolated in the Kanto region after 2017, the organism would have been unable to find suitable reservoirs in which to survive in the human food supply chain. In contrast, the dN/dS ratio for the European isolates was <1, indicating that the genes in these isolates have been subjected to negative selection (Stukenbrock and Bataillon, 2012). Our root-to-tip analysis showed that genetic diversity in the European isolates has decreased over time (Figure 3B). These results suggest that the European isolates have already adapted to the reservoirs existing in the European human food supply chain. Fonteneau et al. (2017) also raised the possibility of persistent contamination sources in Morocco. Thus, the different fate of the Japanese strain and the European isolates may have been determined by their colonizing opportunities toward a suitable host.
We chose not to exclude isolate 29149 from Yokohama City from our analysis of the Japanese isolates when we evaluated the selective pressure acting on the isolates from Kanto. However, the root-to-tip analysis revealed that isolate 29149 was set apart from the other isolates in its position in this analysis, although the other positions were positively correlated. Similarly, the MST analysis showed that isolate 29149 was distant from the other isolates. These results indicate that isolate 29149 accumulated mutations faster than the other Japanese isolates did. Nevertheless, mutations in the mismatch repair genes encoding MutS, MutL, MutH, and UvrD proteins did not contribute to this phenomenon, despite these genes being the most critical factors leading to hypermutability in bacteria (Sheng et al., 2020), because no SNPs were identified in these genes in the WGS data for isolate 29149. One possible explanation for this may be that the additional genetic changes were induced by antibiotic use and bacteriological examination procedures. In antibiotic treatment of clinical cases, bacteria respond to antibiotic stress resulting in mutations occurring in the bacterial genome (Levert et al., 2010). Subculturing bacteria during their examination and detection can also cause genome variation (Iguchi et al., 2006; Yokoyama et al., 2017). However, we have no information with which to elucidate the influence of such factors. In this study, the dN/dS ratio of the Japanese isolates other than isolate 29149 was 1.92. Therefore, even if isolate 29149 had been excluded from this study, this would not have changed our conclusion that the genes examined in the Japanese strain have undergone positive selection.
A limitation of the present study is that the WGS data from the Japanese S. Chester isolates could not be compared with the data from the isolates collected in Japan before 2013. Our data revealed that the Japanese isolates emerging during 2014–2016 originated from one clone. It is possible that an S. Chester clone became established in its host in the Japanese environment and the clone emerging from this time may have arisen from the pre-existing clone. This possibility, however, seems unlikely. In the first place, infections with S. Chester have been rare among all the reported NTS infections in Japan (Katoh et al., 2015). If the emerging S. Chester clone had been present in the Japanese environment, similar emergences of the S. Chester isolates investigated in this study would have occurred. There was only one large S. Chester outbreak in 1998. However, the isolates from 1998 were classified as belonging to ST343 using MLST. In the present study, the Japanese strains all belonged to ST1954. Therefore, the genetic distance between our Japanese isolates and the isolates from 1998 was too large to evaluate their genetic relationships. Even if the S. Chester isolates isolated before 2013 were to be collected from all the local public health institutes throughout Japan and the WGS data were compared between our Japanese isolates and the pre-2013 collected isolates, it is unlikely that the comparison would provide new insights into the findings of this study.
Conclusion
We conclude that the S. Chester isolates originating from one clone emerged in Japan’s Kanto region via contaminated foods other than imported Moroccan seafood. However, the emerging isolates could not establish any reservoirs for survival in the human food supply chain in Japan, and S. Chester was not isolated in the Kanto region after 2017.
Data Availability Statement
The datasets presented in this study can be found in online repositories. The names of the repository/repositories and accession number(s) can be found in the article/Supplementary Material.
Author Contributions
NA, EY, KM, and SH conceptualized and designed this study. NA, EY, YA, NK, YM, and SF isolated S. Chester from human stools, and identified the isolates as S. Chester. TS, YM, NJ-D, SL, KM, and MK performed WGS on the S. Chester isolates. TS, NJ-D, SL, and MK processed and analyzed the WGS data. NA, TS, EY, MK, and SH performed molecular epidemiological and population genetic analyses using the SNPs extracted from the WGS data. KI, KN, MS and HK assisted with project development and data analysis. NA, EY, KM, and SH wrote the draft manuscript. All authors have commented on and agreed upon the final manuscript.
Funding
This work was supported by grants from the Japanese Society for the Promotion of Science (KAKENHI) (Grant Numbers: JP19K10590 and JP20K18920), Health and Labour Sciences Research Grant (Research on Food Safety) from the Ministry of Health, Labour and Welfare, Japan (Grant Number: JPMH20KA1004), and the Research Program on Emerging and Re-emerging Infectious Diseases from the Japan Agency for Medical Research and Development (AMED) (Grant Numbers: JP20fk0108120 and JP19fk0108103).
Conflict of Interest
The authors declare that the research was conducted in the absence of any commercial or financial relationships that could be construed as a potential conflict of interest.
Publisher’s Note
All claims expressed in this article are solely those of the authors and do not necessarily represent those of their affiliated organizations, or those of the publisher, the editors and the reviewers. Any product that may be evaluated in this article, or claim that may be made by its manufacturer, is not guaranteed or endorsed by the publisher.
Acknowledgments
We thank the staff at the Kaiso Health and Welfare Center, Itako Public Health Center, and all the public health centers that participated in the epidemic prevention of S. Chester infections in the Kanto Region. We are grateful to Yoshihiro Aoki, Yudai Watanabe, and Katsuhiko Kitazawa for providing S. Chester isolates from patients in Asahi General Hospital. We thank T. Doi, T. Yamada, and J. Kobayashi of the National Institute of Infectious Diseases for their technical support.
Supplementary Material
The Supplementary Material for this article can be found online at: https://www.frontiersin.org/articles/10.3389/fmicb.2021.705679/full#supplementary-material
Footnotes
- ^ https://www.who.int/news-room/fact-sheets/detail/salmonella-(non-typhoidal)
- ^ http://idsc.tokyo-eiken.go.jp/epid/
- ^ https://www.maff.go.jp/j/tokei/kouhyou/kokusai/houkoku_gaikyou.html
- ^ http://www.genomicepidemiology.org/
- ^ https://www.maff.go.jp/j/syouan/nouan/kome/k_yasai/
- ^ https://www.maff.go.jp/nval/yakuzai/yakuzai_p3.html
References
Achtman, M., Wain, J., Weill, F. X., Nair, S., Zhou, Z., Sangal, V., et al. (2012). Multilocus sequence typing as a replacement for serotyping in Salmonella enterica. PLoS Pathog. 8:e1002776. doi: 10.1371/journal.ppat.1002776
Aoki, Y., Watanabe, Y., Kitazawa, K., Ando, N., Hirai, S., and Yokoyama, E. (2020). Emergence of Salmonella enterica subsp. enterica serovar Chester in a rural area of Japan. J. Vet. Med. Sci. 82, 580–584. doi: 10.1292/jvms.20-0033
Bierne, N., and Eyre-Walker, A. (2004). The genomic rate of adaptive amino acid substitution in Drosophila. Mol. Biol. Evol. 21, 1350–1360. doi: 10.1093/molbev/msh134
Brown, T. S., Challagundla, L., Baugh, E. H., Omar, S. V., Mustaev, A., Auld, S. C., et al. (2019). Pre-detection history of extensively drug-resistant tuberculosis in KwaZulu-Natal, South Africa. Proc. Natl. Acad. Sci. U. S. A. 116, 23284–23291. doi: 10.1073/pnas.1906636116
Coil, D., Jospin, G., and Darling, A. E. (2015). A5-miseq: an updated pipeline to assemble microbial genomes from Illumina MiSeq data. Bioinformatics 31, 587–589. doi: 10.1093/bioinformatics/btu661
Earl, D. A., and von Holdt, B. M. (2012). STRUCTURE HARVESTER: a website and program for visualizing STRUCTURE output and implementing the Evanno method. Conserv. Genet. Resour. 4, 359–361. doi: 10.1007/s12686-011-9548-7
Fonteneau, L., Jourdan Da Silva, N., Fabre, L., Ashton, P., Torpdahl, M., Müller, L., et al. (2017). Multinational outbreak of travel-related Salmonella Chester infections in Europe, summers 2014 and 2015. Euro Surveill. 22:30463. doi: 10.2807/1560-7917.ES.2017.22.7.30463
Haubold, B., and Hudson, R. R. (2000). LIAN 3.0: detecting linkage disequilibrium in multilocus data. Linkage analysis. Bioinformatics 16, 847–848. doi: 10.1093/bioinformatics/16.9.847
Heinitz, M. L., Ruble, R. D., Wagner, D. E., and Tatini, S. R. (2000). Incidence of Salmonella in fish and seafood. J. Food Prot. 63, 579–592. doi: 10.4315/0362-028x-63.5.579
Iguchi, A., Iyoda, S., Terajima, J., Watanabe, H., and Osawa, R. (2006). Spontaneous recombination between homologous prophage regions causes large-scale inversions within the Escherichia coli O157:H7 chromosome. Gene 372, 199–207. doi: 10.1016/j.gene.2006.01.005
Jiang, H., Lei, R., Ding, S. W., and Zhu, S. (2014). Skewera fast and accurate adapter trimmer for next-generation sequencing paired-end reads. BMC Bioinformatics 15:182. doi: 10.1186/1471-2105-15-182
Katoh, R., Matsushita, S., Shimojima, Y., Ishitsuka, R., Sadamasu, K., and Kai, A. (2015). Serovars and drug-resistance of Salmonella strains isolated from domestic chicken meat in Tokyo (1992-2012). Kansenshogaku Zasshi 89, 46–52. doi: 10.11150/kansenshogakuzasshi.89.46
Koboldt, D. C., Chen, K., Wylie, T., Larson, D. E., McLellan, M. D., Mardis, E. R., et al. (2009). VarScan: variant detection in massively parallel sequencing of individual and pooled samples. Bioinformatics 25, 2283–2285. doi: 10.1093/bioinformatics/btp373
Kudva, I. T., Griffin, R. W., Murray, M., John, M., Perna, N. T., Barrett, T. J., et al. (2004). Insertions, deletions, and single-nucleotide polymorphisms at rare restriction enzyme sites enhance discriminatory power of polymorphic amplified typing sequences, a novel strain typing system for Escherichia coli O157:H7. J. Clin. Microbiol. 42, 2388–2397. doi: 10.1128/JCM.42.6.2388-2397.2004
Kumar, S., Stecher, G., and Tamura, K. (2016). MEGA7: molecular evolutionary genetics analysis version 7.0 for bigger datasets. Mol. Biol. Evol. 33, 1870–1874. doi: 10.1093/molbev/msw054
Larsen, M. V., Cosentino, S., Rasmussen, S., Friis, C., Hasman, H., Marvig, R. L., et al. (2012). Multilocus sequence typing of total-genome-sequenced bacteria. J. Clin. Microbiol. 50, 1355–1361. doi: 10.1128/JCM.06094-11
Lee, K. I., Morita-Ishihara, T., Iyoda, S., Ogura, Y., Hayashi, T., Sekizuka, T., et al. (2017). A geographically widespread outbreak investigation and development of a rapid screening method using whole genome sequences of enterohemorrhagic Escherichia coli O121. Front. Microbiol. 8:701. doi: 10.3389/fmicb.2017.00701
Leigh, J. W., and Bryant, D. (2015). PopART: full-feature software for haplotype network construction. Methods Ecol. Evol. 6, 1110–1116. doi: 10.1111/2041-210X.12410
Levert, M., Zamfir, O., Clermont, O., Bouvet, O., Lespinats, S., Hipeaux, M. C., et al. (2010). Molecular and evolutionary bases of within-patient genotypic and phenotypic diversity in Escherichia coli extraintestinal infections. PLoS Pathog. 6:e1001125. doi: 10.1371/journal.ppat.1001125
Li, H., and Durbin, R. (2010). Fast and accurate long-read alignment with burrows-wheeler transform. Bioinformatics 26, 589–595. doi: 10.1093/bioinformatics/btp698
Ministry of Agriculture, Forestry and Fisheries (2018). Study on Prevalence of Enterohemorrhagic Escherichia coli and Salmonella spp. in fresh vegetables [in Japanese]. Available online at: https://www.maff.go.jp/j/syouan/nouan/kome/k_yasai/ (accessed August 31, 2020)
Ministry of Agriculture, Forestry and Fisheries (2019). Overview of Foreign Trade of Agricultural, Forestry, and Fishery Products [in Japanese]. Available online at: https://www.maff.go.jp/j/tokei/kouhyou/kokusai/houkoku_gaikyou.html (accessed August 15, 2020)
Morse, S. S. (1995). Factors in the emergence of infectious diseases. Emerg. Infect. Dis. 1, 7–15. doi: 10.3201/eid0101.950102
National Veterinary and Assay Laboratory. (2020). Report on the Japanese Veterinary Antimicrobial Resistance Monitoring System [in Japanese]. Available online at: https://www.maff.go.jp/nval/yakuzai/yakuzai_p3.html (accessed August 31, 2020)
Peakall, R., and Smouse, P. E. (2012). GenAlEx 6.5: genetic analysis in Excel. Population genetic software for teaching and research–an update. Bioinformatics 28, 2537–2539. doi: 10.1093/bioinformatics/bts460
Pritchard, J. K., Stephens, M., and Donnelly, P. (2000). Inference of population structure using multilocus genotype data. Genetics 2155, 945–959.
Rambaut, A., Lam, T. T., Max Carvalho, L., and Pybus, O. G. (2016). Exploring the temporal structure of heterochronous sequences using TempEst (formerly Path-O-Gen). Virus Evol. 2:vew007. doi: 10.1093/ve/vew007
Sheng, H., Huang, J., Han, Z., Liu, M., Lü, Z., Zhang, Q., et al. (2020). Genes and proteomes associated with increased mutation frequency and multidrug resistance of naturally occurring mismatch repair-deficient Salmonella hypermutators. Front. Microbiol. 11:770. doi: 10.3389/fmicb.2020.00770
Stukenbrock, E. H., and Bataillon, T. (2012). A population genomics perspective on the emergence and adaptation of new plant pathogens in agro-ecosystems. PLoS Pathog. 8:e1002893. doi: 10.1371/journal.ppat.1002893
Tanner, J. R., and Kingsley, R. A. (2018). Evolution of Salmonella within hosts. Trends Microbiol. 26, 986–998. doi: 10.1016/j.tim.2018.06.001
Tokyo Metropolitan Infectious Disease Surveillance Center. (2020). Information on Microbial Test in Tokyo (Monthly reports) [in Japanese]. Available online at: http://idsc.tokyo-eiken.go.jp/epid/ [Accessed August 15, 2020]
Tsuji, H., and Hamada, K. (1999). Outbreak of salmonellosis caused by ingestion of cuttlefish chips contaminated by both Salmonella Chester and Salmonella Oranienburg. Jpn. J. Infect. Dis. 52, 138–139.
Whittam, T. S., McGrawSean, E. A., and Reid, S. D. (1998). 5 Pathogenic Escherichia coli O157:H7: a model for emerging infectious diseases. Biomed. Res. Rep. 1, 163–183. doi: 10.1016/S1874-5326(07)80029-9
World Health Organization. (2018). Salmonella (Non-Typhoidal). Available online at: https://www.who.int/news-room/fact-sheets/detail/salmonella-(non-typhoidal) (accessed Jun 26, 2020)
Yokoyama, E., Hirai, S., Ishige, T., and Murakami, S. (2017). Single nucleotide polymorphisms in the whole genome sequence data of Shiga toxin producing Escherichia coli O157:H7/H- strains by cultivation. Curr. Microbiol. 75, 425–430. doi: 10.1007/s00284-017-1208-z
Yokoyama, E., Hirai, S., Ishige, T., and Murakami, S. (2018). Application of whole genome sequence data in analyzing the molecular epidemiology of Shiga toxin-producing Escherichia coli O157:H7/H. Int. J. Food Microbiol. 264, 39–45. doi: 10.1016/j.ijfoodmicro.2017.10.019
Yokoyama, E., Torii, Y., Shigemura, H., Ishige, T., Yanagimoto, K., Uematsu, K., et al. (2019). Isolation of Salmonella enterica serovar Agona strains and their similarities to strains derived from a clone caused a serovar shift in broilers. J. Infect. Chemother. 25, 71–74. doi: 10.1016/j.jiac.2018.07.003
Keywords: Salmonella Chester, emerging, molecular epidemiological analysis, population genetic analysis, whole genome sequence data, single-nucleotide polymorphisms, selection pressure
Citation: Ando N, Sekizuka T, Yokoyama E, Aihara Y, Konishi N, Matsumoto Y, Ishida K, Nagasawa K, Jourdan-Da Silva N, Suzuki M, Kimura H, Le Hello S, Murakami K, Kuroda M, Hirai S and Fukaya S (2021) Whole Genome Analysis Detects the Emergence of a Single Salmonella enterica Serovar Chester Clone in Japan’s Kanto Region. Front. Microbiol. 12:705679. doi: 10.3389/fmicb.2021.705679
Received: 06 May 2021; Accepted: 05 July 2021;
Published: 27 July 2021.
Edited by:
George Grant, University of Aberdeen, United KingdomReviewed by:
Andrea Isabel Moreno Switt, Pontificia Universidad Católica de Chile, ChileValentine Usongo, Health Canada, Canada
Copyright © 2021 Ando, Sekizuka, Yokoyama, Aihara, Konishi, Matsumoto, Ishida, Nagasawa, Jourdan-Da Silva, Suzuki, Kimura, Le Hello, Murakami, Kuroda, Hirai and Fukaya. This is an open-access article distributed under the terms of the Creative Commons Attribution License (CC BY). The use, distribution or reproduction in other forums is permitted, provided the original author(s) and the copyright owner(s) are credited and that the original publication in this journal is cited, in accordance with accepted academic practice. No use, distribution or reproduction is permitted which does not comply with these terms.
*Correspondence: Shinichiro Hirai, hirai@nih.go.jp
†Present address: Kumiko Ishida, Ryugasaki Public Health Center, Ibaraki, Japan