- 1Microbiology Unit, Belgian Nuclear Research Centre (SCK CEN), Mol, Belgium
- 2Department of Microbial and Molecular Systems, Faculty of Bioscience Engineering, KU Leuven, Leuven, Belgium
Cupriavidus metallidurans strains display a decreased viability when incubated in rich medium at a temperature of 37°C compared to their normal growth temperature of 30°C, a phenomenon coined “temperature-induced mortality and mutagenesis” (TIMM). To scrutinize this aberrant phenotype further, the contributions of specific inducers and protective agents were determined. Different growth media, including lysogeny broth (LB) and Schatz, and components, including casamino acids, in particular amino acids (proline, cysteine, glycine, glutamine, leucine, histidine and phenylalanine) and ammonium, were found to induce TIMM at 37°C. Sorbitol was found to counteract TIMM. Furthermore, although TIMM is well conserved within the C. metallidurans species, multiple and strain-specific TIMM inducers exist. Twenty-nine percent of the TIMM survivors inherited resistance to TIMM. Whole-genome sequencing of two resistant derivatives revealed an important role of an uncharacterized oxidoreductase, indicating putative metabolic poisoning when grown in high-concentration nitrogen-containing media at 37°C.
Introduction
Type strain Cupriavidus metallidurans CH34, isolated from a non-ferrous metallurgical plant in Belgium (Mergeay et al., 1978), is mostly studied because of its resistance to numerous metals (Mergeay and Van Houdt, 2015). In addition, Mergeay et al. (1985) described an intriguing phenomenon of decreased viability (with typical survival frequencies ranging between 10–3 and 10–5) when C. metallidurans CH34 is grown in rich medium [i.e., lysogeny broth (LB)] at an increased temperature of 37°C compared to the normal growth temperature of 30°C. It was hypothesized that the phenomenon corresponded to a temperature-induced mutator phenotype as up to 80% of the surviving colonies exhibited one or more aberrant phenotypes, including auxotrophy for lysine, threonine, proline or serine; loss of autotrophy (growth on H2 and CO2); loss of nitrate utilization; loss of ammonium utilization as a nitrogen source; loss of tyrosine as a carbon source; and loss of cobalt/zinc resistance (Sadouk and Mergeay, 1993). Therefore, they coined this phenomenon as “temperature-induced mortality and mutagenesis” (TIMM). Next to rich LB medium, a similar phenotype was observed when methionine was specifically added to a mineral medium (Van der Lelie et al., 1992). Interestingly, most TIMM survivors, although isolated at a higher temperature, remained susceptible to an increase in temperature (Mergeay, 2000).
Growing C. metallidurans CH34 in LB medium at 37°C for 1 h had no effect on a variety of measured physiological parameters, including cell size, membrane permeability, membrane potential, intracellular esterase activities, intracellular pH and production of reactive oxygen species (ROS) (Baatout et al., 2005). However, during prolonged growth at 37°C, morphological changes such as filamentous cell formation, bead-like chains and cell clusters were induced (Arroua et al., 2014). This aberrant growth was hypothesized to be caused by impaired peptidoglycan synthesis or processing. Moreover, when cultures grown in LB medium were shifted from permissive to non-permissive temperature, a full growth arrest was induced after approximately two generations, which was probably caused by the depletion of an essential cell component and/or activity (Arroua et al., 2014). In addition, Arroua et al. (2014) suggested that the TIMM phenotype may be part of a more general stress response as the same morphological changes could be induced by sodium hypochlorite.
In this study, 22 different C. metallidurans strains were tested for their susceptibility to TIMM, and the TIMM phenotype was further scrutinized by testing different growth media for their ability to induce mortality at the restrictive temperature of 37°C. The potential mitigation of TIMM induction by adding a non-ionic osmolyte or ROS scavengers was scrutinized as well as the possible stable inheritance of TIMM resistance by C. metallidurans CH34.
Materials and Methods
Strains, Media and Culture Conditions
Bacterial strains and plasmids used in this study are listed in Tables 1, 2. C. metallidurans strains were routinely cultured at 30°C in Tris-buffered mineral medium (6.06 g/L Tris/HCl, 4.68 g/L NaCl, 1.49 g/L KCl, 1.07 g/L NH4Cl, 0.43 g/L Na2SO4, 0.2 g/L MgCl2⋅6H20, 0.03 g/L CaCl2⋅2H20, 0.04 g/L Na2HPO4⋅2H2O, 4.8 mg/L Fe(III)(NH4)citrate, 144 μg/L ZnSO4⋅7H2O, 99 μg/L MnCl2⋅4H2O, 62 μg/L H3BO3, 190 μg/L CoCl2⋅6H2O, 17 μg/L CuCl2⋅2H2O, 24 μg/L NiCl2⋅6H2O, and 36 μg/L Na2MoO4⋅2H2O) supplemented with 0.2% (w/v) sodium gluconate (MM284) as described previously (Mergeay et al., 1985). Escherichia coli strains were routinely cultured at 37°C in LB. Liquid cultures were grown in the dark on a rotary shaker at 150 rpm; for culturing on agar plates, 2% agar (Thermo Scientific, Oxoid) was added. C. metallidurans strains were also cultured on Schatz mineral salt medium, as described previously (Schatz and Bovell, 1952), supplemented with 0.2% (w/v) sodium gluconate. When appropriate, the following chemicals (Sigma-Aldrich or Thermo Scientific) were added to the growth medium at the indicated final concentrations: casamino acids (CA) (0.5% (w/v)), individual L-amino acids (0.5% (w/v)), sorbitol (0.44 M), NaCl (0.5–1% (w/v)), mannitol (50 mM), pyruvic acid (10 mM), kanamycin (50 and 1,500 μg/ml for E. coli and C. metallidurans, respectively), chloramphenicol (30 μg/ml), 5-bromo-4-chloro-3-indolyl β-galactopyranoside (X-Gal; 40 μg/ml), and isopropyl β-D-1-thiogalactopyranoside (IPTG; 0.1 mM).
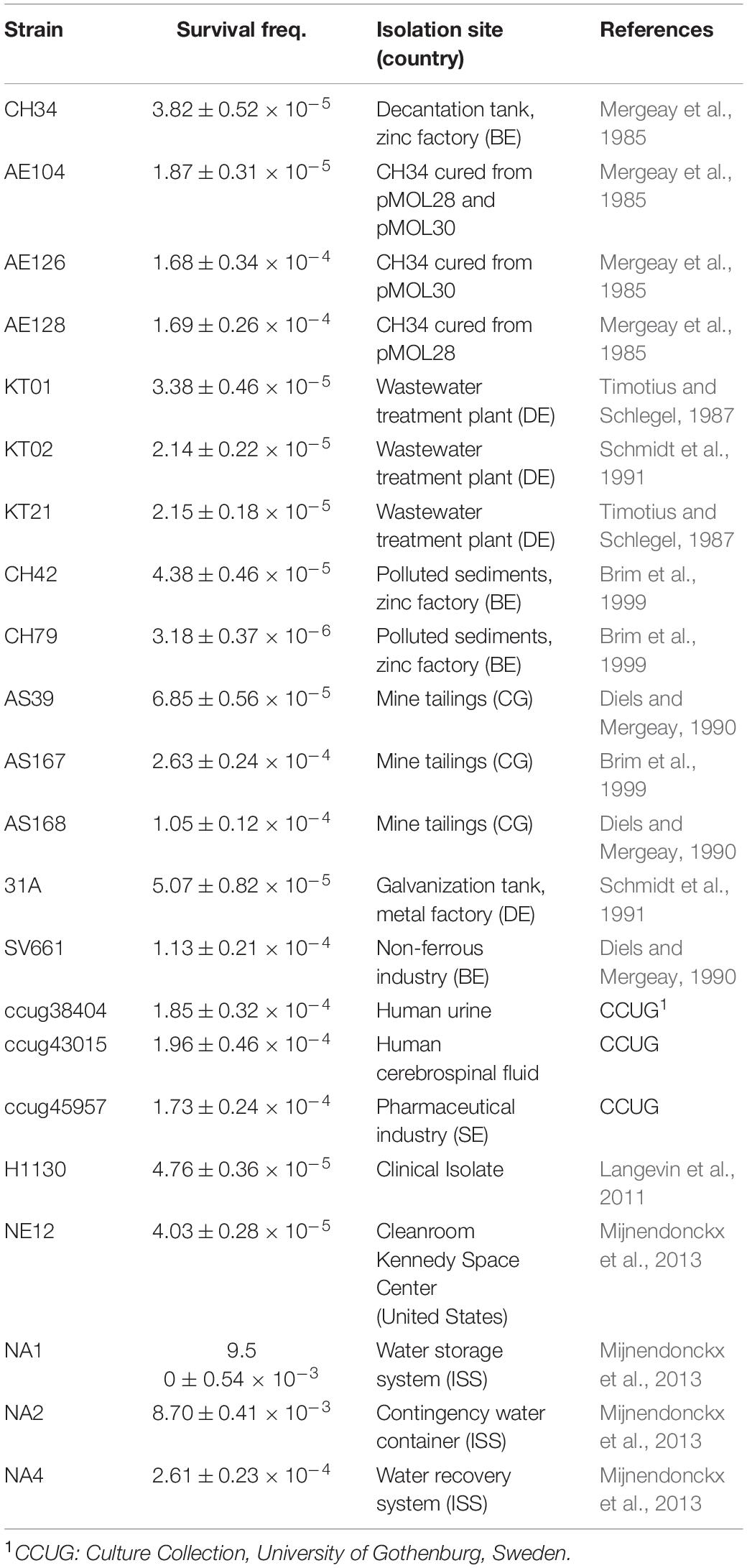
Table 2. Survival frequency and isolation site/place of the different C. metallidurans strains used in this study as determined by the ratio of viable count on LB agar at 37 to 30°C.
TIMM Assay and Isolation of TIMM-Resistant Mutants
In this study, unless cited otherwise, LB agar plates were used to study the TIMM phenotype. The TIMM assay comprised cultivating a 10-fold serial dilution of stationary-phase cells on agar plates at 30°C (permissive temperature) and 37°C (restrictive temperature). After an incubation time of 2 days, the survival frequency was calculated as viable cell count at 37°C divided by viable cell count at 30°C. A survival frequency of 10–2 or less was scored as a positive TIMM phenotype. Additional TIMM assays were performed to determine the frequency of inherited TIMM resistance. Ten independent C. metallidurans CH34 lines were cultivated in MM284 at 30°C up to the stationary phase and 109 cells were pelleted. One-hundred-microliter aliquots of a 10-fold serial dilution in saline were spread on MM284 agar plates containing 0.5% (w/v) L-phenylalanine and incubated for 2 days at 37°C. Next, 10 survivors of each independent line, thus totaling 100 survivors for each strain, were purified at 30°C. Finally, these survivors were retested for TIMM resistance by being spread on different agar plates, including LB, Schatz medium and MM284 containing 0.5% (w/v) L-phenylalanine or CA.
Fitness Analysis
The fitness of stable TIMM-resistant CH34 mutant strains was compared with that of the parental CH34 by culturing equally mixed cell suspensions in triplicate. To enable selection, a reference C. metallidurans CH34 strain, i.e., CH34-KmR, containing a kanamycin resistance cassette in gene Rmet_0221, coding for a hypothetical protein, was used in the competition assays. The different strains, i.e., CH34-TIMMR1, CH34-TIMMR2, CH34 and CH34-KmR (Table 1), were first individually cultivated up to the stationary phase (MM284, 30°C) and subsequently used to inoculate tubes with 4 ml MM284 (biological triplicate) with mixed cultures of CH34-KmR and the TIMM-resistant mutant or parent, respectively, at a final concentration of 105 cells/ml. The tubes were incubated with shaking at 30°C, and 20 μl aliquots were withdrawn at different time points. Cell enumeration by total viable count was performed by spreading 100 μl of a serial 10-fold dilution in sterile PBS on MM284 agar and counting colonies after 2 days at 30°C. Plates with 30–300 colonies were selected as master plates for replica plating onto MM284 + 1,500 μg/ml kanamycin agar plates, a condition where only CH34-KmR will survive, and the CFU/ml was determined again after 2 days of incubation at 30°C. The fitness of the different strains compared to the reference strain was determined as the ratio of CFU/ml on MM284 to MM284 + 1,500 μg/ml kanamycin.
Construction Insertional Inactivation Mutant
The Rmet_1009 gene including the 1-kb region upstream and downstream was amplified by PCR (Phusion High-Fidelity DNA polymerase, Thermo Scientific) with the primer pair Rmet_1009_5′-FW/_3′-RV providing Mph1103I/EcoRI recognition sites. This PCR product was cloned as a Mph1103I/EcoRI fragment into PstI/EcoRI-digested pK18mob. The resulting pK18mob-1009 plasmid from an E. coli DG1 transformant selected on LB Km50 was further confirmed by sequencing prior to amplifying of the flanking sequences of Rmet_1009 by inverse PCR (Phusion High-Fidelity DNA polymerase) with the primer pair Rmet_1009_5′-RV/_3′-RW, providing SpeI/BspTI restriction sites. At the same time, the tet gene from pACYC184 was amplified by PCR (Phusion High-Fidelity DNA polymerase) with the primer pair Tet_Fw-Rv, providing SpeI/BspTI restriction sites. Afterward, this PCR product was cloned as a SpeI/BspTI fragment into the former inverse PCR product. The resulting pK18mob-1009::tet plasmid from an E. coli DG1 transformant selected on LB Tc20 Km50 was further confirmed by sequencing prior to conjugation (biparental with E. coli MFDpir as a donor) to C. metallidurans CH34, CH34-TIMMR1 and CH34-TIMMR2. The resulting transformants selected on MM284 Tc20 were replica-plated on MM284 Tc20 and MM284 Km1500. Transformants resistant to Tc20 but sensitive to Km1500 were further confirmed by sequencing and were designated CH34 Δ1009, CH34-TIMMR1 Δ1009, and CH34-TIMMR2 Δ1009. All primers used in this study are listed in Supplementary Table 1.
Genome Sequencing and Analysis
Whole-genome sequencing of two CA TIMM-resistant CH34 derivatives, i.e. strains CH34-TIMMR1 and CH34-TIMMR2 (Table 1), was performed to identify mutations responsible for the stable inheritance of TIMM resistance. The strains were cultivated by inoculating 4 ml LB at 30°C, and total DNA was extracted using the QIAamp DNA Mini Kit (Qiagen, Netherlands). The quantity and quality of extracted DNA were measured using a NanoDropTM 1000 spectrophotometer (Thermo Scientific, United States). Ten micrograms of DNA were sent for Illumina sequencing (BaseClear, Netherlands). Point mutations (SNPs and small indels) and large insertions and deletions (>200 bp) were identified as described by Mijnendonckx et al. (2019). Sequencing data are available within the Sequencing Read Archive (SRA) of NCBI under the bioProject PRJNA641768.
Results
TIMM Is Well Conserved Among C. metallidurans Strains
As detailed in the introduction, C. metallidurans CH34 exhibits a decreased viability when cultivated on nutrient-rich agar plates at 37°C, and up to 80% of the surviving colonies exhibited one or more aberrant phenotypes, including loss of autotrophy; auxotrophy for lysine, threonine, proline, or serine; or loss of ammonium utilization as a nitrogen source (Mergeay et al., 1985; Van der Lelie et al., 1992; Sadouk and Mergeay, 1993; Mijnendonckx et al., 2013). Besides strain CH34, other strains isolated from metal-contaminated industrial environments showed this TIMM phenotype as well (Brim et al., 1999). Here, we confirmed this phenotype for a subset of these strains (KT01, KT02, KT21, CH42, CH79, AS39, AS167, AS168, 31A and SV661), which were recently included in a comparative genomic hybridization study to type strain CH34 (Van Houdt et al., 2012), and demonstrated it for more recently isolated strains from anthropogenic environments not typified by metal contamination (ccug45957, NE12, NA1, NA2 and NA4) and from human infections (ccug38404, ccug43015 and H1130) (Table 2). All these C. metallidurans strains displayed increased mortality on LB agar at 37°C with a frequency of survivors ranging from 10–4 to 10–6 (Table 2). In addition, the CH34 derivatives cured from one or both megaplasmids (AE104, AE126 and AE128) displayed increased mortality (Table 2).
TIMM-Mediated Cell Mortality After Prolonged Exposure at 37°C
Survival of C. metallidurans CH34 under TIMM-inducing conditions was further assessed in the function of incubation time. At different time points post-plating, LB agar plates were shifted from restrictive to permissive temperatures, and the viability was determined after 2 days of incubation at 30°C following the shift from 37 to 30°C (Figure 1). It was demonstrated that cell mortality was initiated during prolonged exposure to 37°C. Approximately, a 4-log reduction in viability was observed after 24 h of exposure. Viability further decreased 1 log (48 h at 37°C) and remained more or less stable at 72 and 96 h. Thus, a reduced viable cell count during TIMM conditions resulted from cell death and not merely by a decreased or anomalous growth rate.

Figure 1. Tenfold serial dilution of a stationary phase culture of CH34 spotted on LB agar plates and incubated at 37°C (upper row) for 0, 24, 48, 72 and 96 h before shifting to an 48 h incubation period at 30°C (lower row).
TIMM-Inducing and Protective Media
Previously, TIMM induction was observed when cells were incubated at 37°C on LB agar plates or on MM284 agar plates supplemented with L-methionine (Van der Lelie et al., 1992). Here, we scrutinized different growth media and possible protective agents for their ability to either induce or inhibit TIMM at 37°C (Table 3). In contrast with previous observations, cells incubated on MM284 containing 0.5% (w/v) L-methionine did not display TIMM. Modifications of LB medium (a mixture of 1% (w/v) sodium chloride, 0.5% (w/v) yeast extract and 1% (w/v) peptone) were made in order to identify and evaluate specific TIMM inducers. Media composed of only sodium chloride with either peptone or yeast extract were tested, and both induced TIMM (Table 3). Both peptone and yeast extract have a high peptide and amino acid content with yeast extract also comprising different vitamins and sugars. Subsequently, the role of amino acids was further analyzed using MM284 containing 0.5% (w/v) CA. CA, the hydrolysis product of casein, comprise small peptides and amino acids with the exception of tryptophan. C. metallidurans CH34 grown on MM284 containing 0.5% (w/v) CA showed a survival frequency at 37°C comparable to LB, confirming that amino acids play an important role in TIMM induction. In addition, other C. metallidurans strains, including NA1, NA2, NA4 and ccug43015, were tested, and CA promoted TIMM for all tested strains (data not shown).
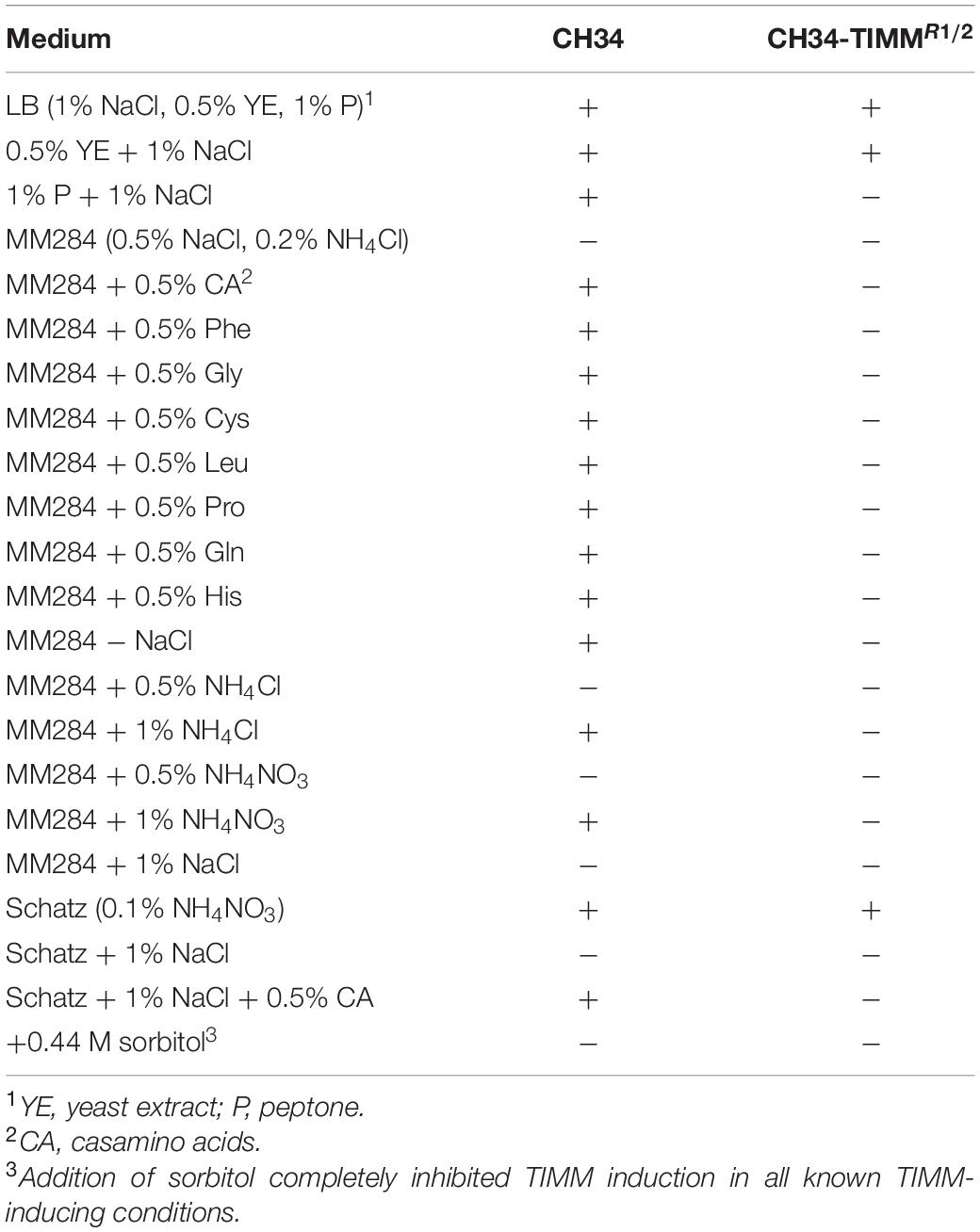
Table 3. Different media and strains used in this study to evaluate TIMM induction (+ for induction and − for no induction) as determined by the ratio of viable count at 37 to 30°C.
The use of CA as a sole carbon or nitrogen source or as an additional carbon/nitrogen nutrient to the available sodium gluconate and ammonium in MM284 presented no differential effect on TIMM induction. Next, all 20 amino acids were individually added to MM284 at a final concentration of 0.5% (w/v), and seven different amino acids induced TIMM (Tables 3, 4). Interestingly, not all C. metallidurans strains displayed the same sensitivity toward particular amino acids (Table 4), indicating strain-specific inducers and the possible involvement of multiple pathways. The individual amino acids that induced the TIMM phenotype for at least one of the C. metallidurans strains included proline, cysteine, glycine, glutamine, leucine and histidine with only phenylalanine being an inducer for all tested strains (Table 4).
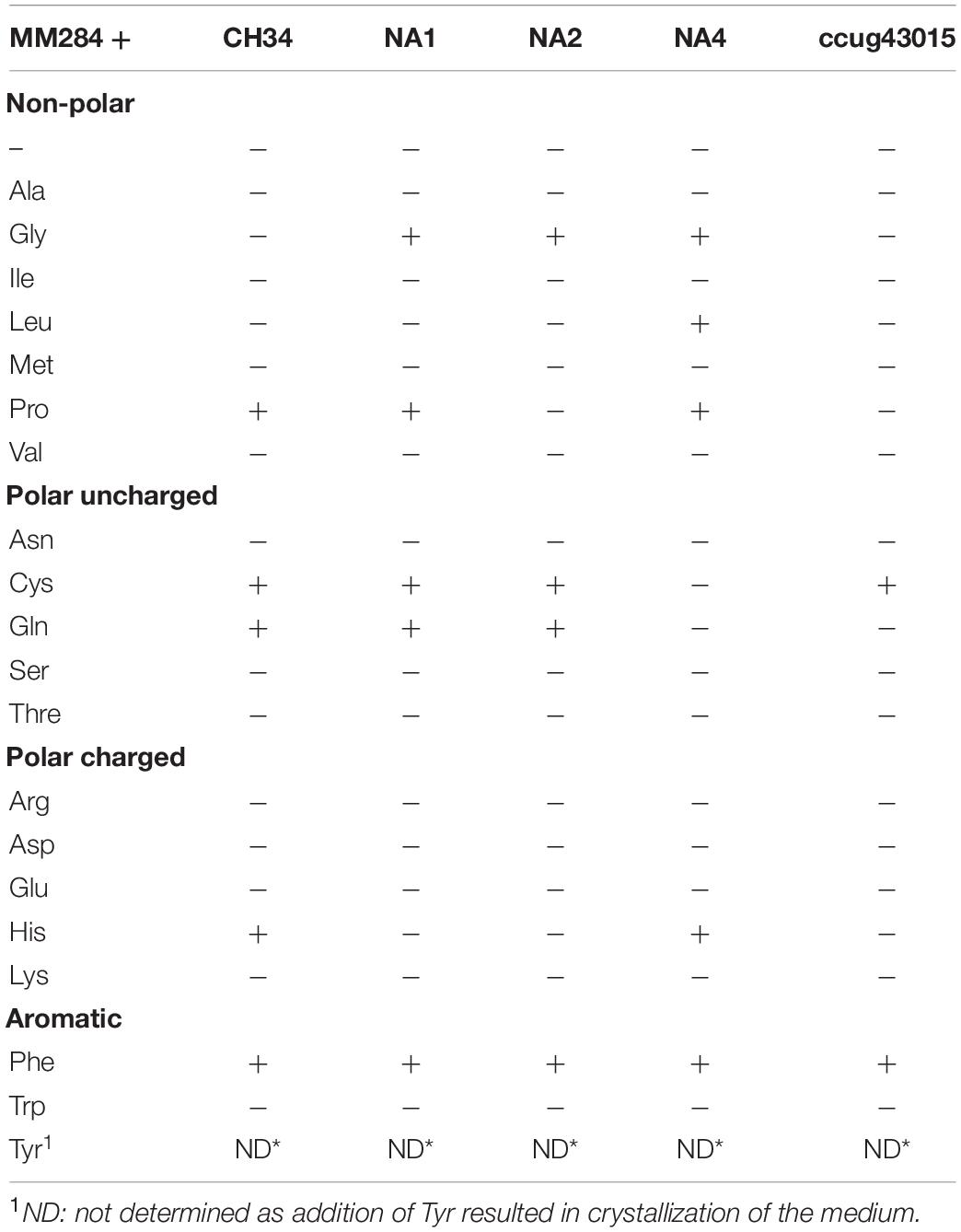
Table 4. Effect of supplementing a specific amino acid to MM284 at a final concentration of 0.5% (w/v) on TIMM induction for different C. metallidurans strains (+ for induction and − for no induction) as determined by the ratio of viable count at 37 to 30°C.
Next, the effect of surplus inorganic nitrogen, i.e., NH4Cl and NH4NO3, on TIMM induction was tested. It was observed that a gradual increase in inorganic nitrogen induced TIMM, indicating that excess nitrogen resulted in aberrant survival at 37°C (Table 3). In addition, growth on a mineral medium with low salt concentrations, i.e. Schatz medium (Table 3), and on carbon dioxide and hydrogen gas (data not shown) also induced TIMM. None of these additions affected survival at 30°C.
Since the possible aberrant catabolism of nutrients at 37°C might lead to the production of ROS, the addition of ROS scavengers was evaluated in the search of TIMM protective agents. Although addition of mannitol or pyruvic acid to MM284 containing 0.5% (w/v) L-phenylalanine exerted a positive effect at 37°C, a complete mitigation of the TIMM phenotype was not reached, indicating that ROS production probably does not, at least solely, cause the observed mortality (data not shown). In a next step, the effect of a non-ionic compatible osmolyte was assessed. Such compounds can exert favorable effects on macromolecule–solvent interactions, e.g. via stabilizing the cell membrane and increasing the protein thermal stability (Yancey, 2001). Addition of sorbitol, which cannot be used as a carbon source (Van Houdt et al., 2018), completely inhibited TIMM induction in all known TIMM-inducing conditions (Table 3). Finally, increasing the osmolarity of the mineral salt medium Schatz to levels approximating MM284 mitigated TIMM induction. The impact of the osmotic potential was further demonstrated by the fact that MM284 deprived of NaCl promoted TIMM. Is important to note that addition of CA to Schatz containing 1% NaCl again promoted TIMM, indicating that osmolarity and a nutrient-rich medium, although both counteracted by the addition of sorbitol, probably promoted TIMM via a different pathway.
Characterization of Stable Inherited TIMM Resistance
Cupriavidus metallidurans CH34 was subjected to a directed evolution experiment to determine the frequency of inherited TIMM resistance in initial TIMM survivors randomly selected after 2 days of incubation at 37°C. Ten independent lines were plated on MM284 with 0.5% (w/v) L-phenylalanine and incubated at 37°C for 48 h. The latter medium was chosen because of its basic and defined composition and because L-phenylalanine induced TIMM in all C. metallidurans strains. Next, 10 TIMM survivors of each independent line, totaling 100 TIMM survivors, were purified at 30°C and subsequently retested for their TIMM resistance on L-phenylalanine, CA, LB and Schatz medium. The frequency of inherited resistance for C. metallidurans CH34 was 29% with lower cross-resistance to other TIMM-inducing media (Figure 2A).
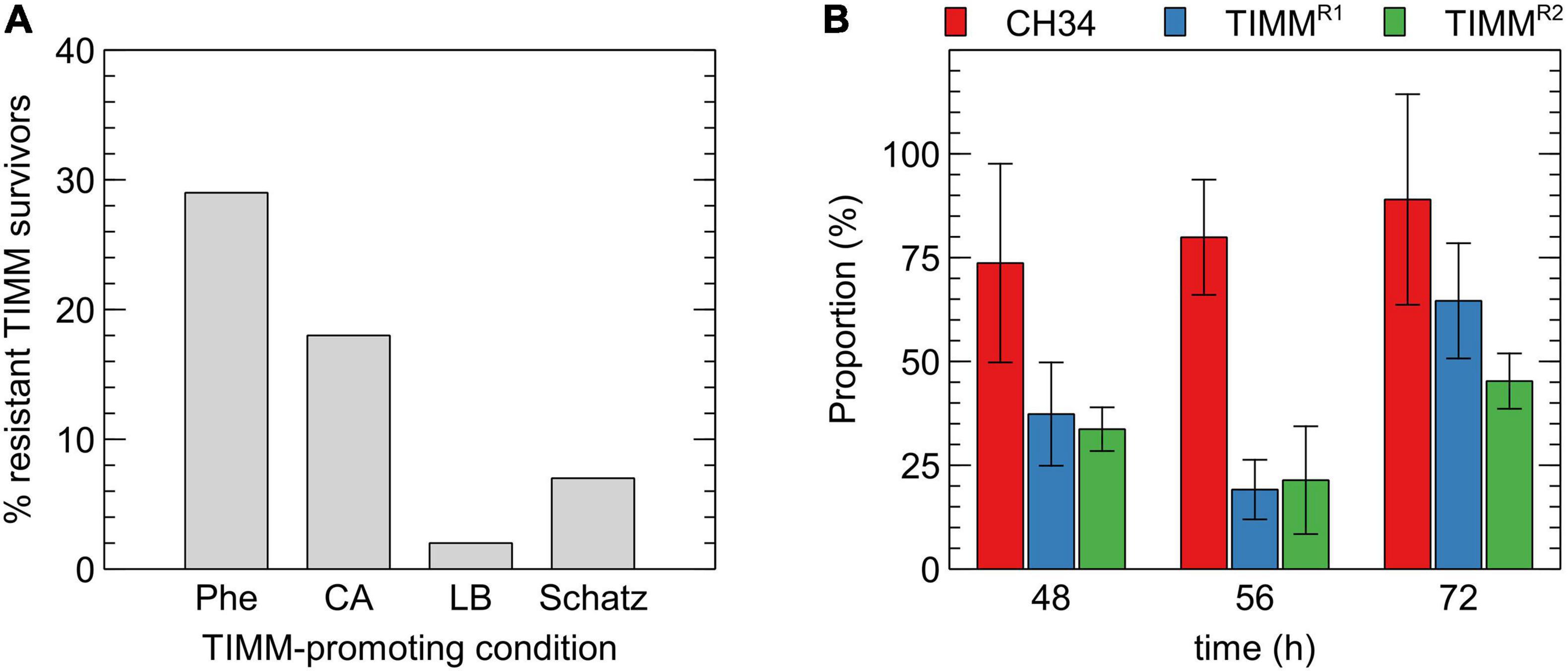
Figure 2. Characterization of TIMM survivors. (A) Inherited resistance of 100 TIMM survivors, originally isolated on MM284 containing 0.5% (w/v) L-phenylalanine, to different TIMM-promoting conditions as determined by the ratio of viable count at 37 to 30°C. Phe: L-phenylalanine, CA: casamino acids. (B) Relative fitness of the parental strain CH34 (red), CH34-TIMMR1 (blue) and CH34-TIMMR2 (green) in MM284 at 30°C, as determined by the ratio of viable count of the strains to CH34-KmR in function of time.
Analysis of TIMM-Resistant CH34 Derivatives
Two stable TIMM-resistant CH34 derivatives, i.e. strains CH34-TIMMR1 and CH34-TIMMR2, from two independent lines were further analyzed (Table 1). The fact that TIMM survivors displayed a lower cross-resistance to other TIMM-inducing media indicated the possible involvement of numerous or additional pathways in the different growth media. Therefore, strains CH34-TIMMR1 and CH34-TIMMR2 were selected since they displayed inherited resistance to the applied stress (e.g., L-phenylalanine) and amino acids (CA) but not to LB and Schatz medium.
Reduced Fitness of TIMM-Resistant Derivatives in Non-restrictive Conditions
It was previously reported that a large fraction of the TIMM survivors, ranging from 2 to 42%, showed additional mutations resulting in other aberrant phenotypes (Mergeay et al., 1985; Sadouk and Mergeay, 1993; Mergeay, 2000; Mijnendonckx et al., 2013). A fitness analysis was performed to find out if throughout the selective TIMM condition, additional detrimental mutations occurred that hitchhiked with the mutations relieving the selective pressure. The fitness of strains CH34-TIMMR1 and CH34-TIMMR2 was compared to that of the CH34 parent in a co-cultured growth competition assay under controlled laboratory conditions (Figure 2B). Although the experiment displayed a high variation, it demonstrated that the parental strain exerted a fitness advantage over the mutants when grown in MM284 at 30°C, indicating that the TIMM-resistant derivatives probably harbored accompanying detrimental mutations leading to a decreased fitness at the tested non-selective conditions.
Stable TIMM-Resistant CH34 Derivatives Harbor Numerous Mutations
To further characterize the inherited TIMM resistance, whole-genome sequencing was performed. CH34-TIMMR1 and CH34-TIMMR2 harbored 14 and 11 mutations, respectively, including three common mutations (Table 5). Mutations were caused by insertions, deletions, and point and frameshift mutations (Table 5). Five mutations targeted genes encoded by either pMOL30 or pMOL28, but these are not likely to be responsible for the observed TIMM resistance as the plasmid-free derivative of CH34, i.e. strain AE104, could also evolve TIMM resistance. One large common deletion, approximately 60 kb, contained the hox/cbb locus and explained the observed loss of autotrophy of both strains. Loss of autotrophy is one of the most observed aberrant phenotypes identified under TIMM survivors but is not common to all TIMM survivors (Sadouk and Mergeay, 1993; Mergeay, 2000; Mijnendonckx et al., 2013). Only one other mutation was shared by both TIMM survivors, a single-nucleotide substitution in Rmet_1009, encoding an oxidoreductase subunit. An insertional knockout mutant was generated in CH34, CH34-TIMMR1 and CH34-TIMMR2. Inactivation of Rmet_1009 had no effect on TIMM resistance in CH34-TIMMR1 and CH34-TIMMR2 but resulted in acquisition of TIMM resistance in CH34 (Figure 3). The latter indicated an important role of Rmet_1009 in CA-induced TIMM.
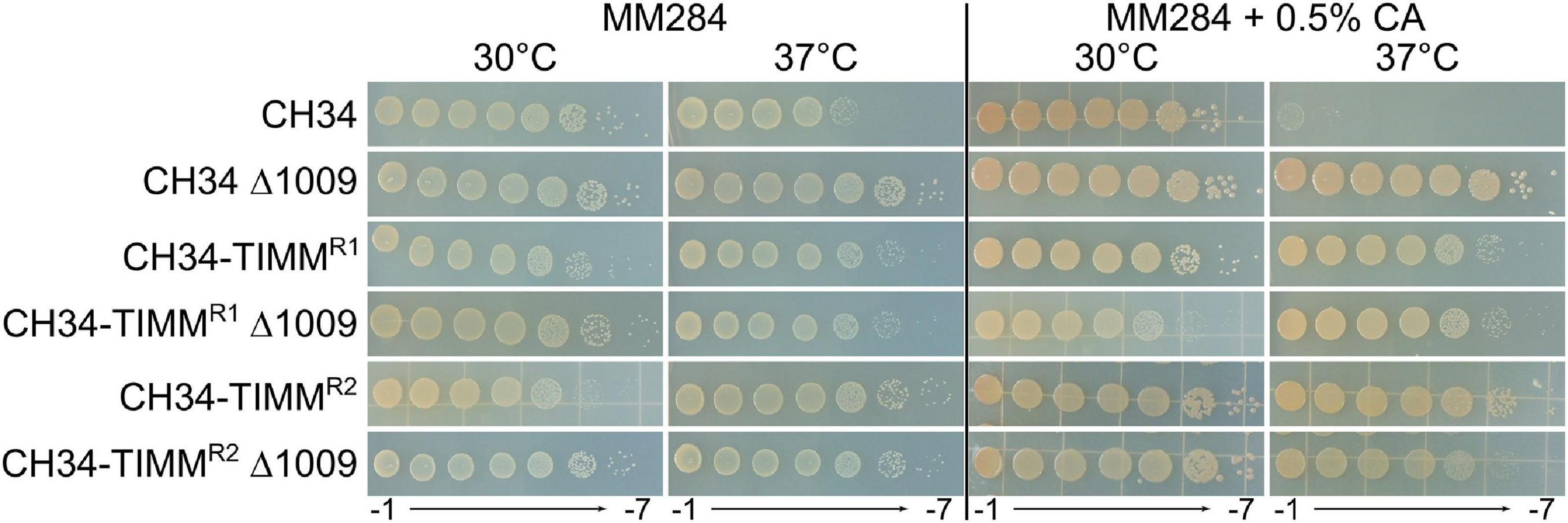
Figure 3. Tenfold serial dilution of stationary-phase cultures of CH34, CH34-TIMMR1 and CH34-TIMMR2, and their Rmet_1009 insertional knockout mutants spotted on MM284 agar plates with and without 0.5% (w/v) CA and incubated for 2 days at 30 and 37°C.
Discussion
Elevating the temperature above the normal optimum can damage a broad spectrum of cellular components leading to metabolic malfunction and ultimately cell death. Here, we further investigated the previously described observations that C. metallidurans CH34 exhibits a decreased survival frequency when cultivated at 37°C on rich medium (Mergeay et al., 1985; Van der Lelie et al., 1992; Sadouk and Mergeay, 1993; Mijnendonckx et al., 2013). This study revealed that all C. metallidurans strains tested displayed increased mortality on LB agar plates at 37°C, including more recently isolated strains such as those from human infection. The latter indicated that the TIMM phenomenon does not impede its survival and proliferation within the human body. Putatively, the environmental conditions, i.e. amino acids and ammonium concentrations, encountered do not induce TIMM (Hladky and Barrand, 2014; Simha and Ganesapillai, 2017). Furthermore, our observations reinforce the conservation of this phenotype in C. metallidurans strains, indicating the involvement of conserved cellular housekeeping processes and its use as a phylogenetic discriminatory trait. Cultivation of C. metallidurans CH34 on LB agar plates at 37°C indicated that the lower viable count at 37°C compared to 30°C resulted from induced mortality and not by reduced or stalled cell division.
The ability to induce TIMM was also tested for different inducers, and three different conditions were found that promoted mortality at the non-permissive temperature. It was shown that yeast extract and peptone, both containing a high peptide and amino acid content with yeast extract also comprising various vitamins and sugars, could separately promote TIMM. Moreover, it was demonstrated that both components promoted TIMM in at least two different ways as TIMM survivors resistant to peptone but not yeast extract were isolated. Alternatively, CA, another product containing small peptides and amino acids with the exception of tryptophan, promoted increased mortality at 37°C either as a sole carbon or nitrogen source or as an additional nutrient to MM284. In addition, seven different amino acids, namely, proline, cysteine, glycine, glutamine, leucine, histidine and phenylalanine, could induce TIMM. However, only phenylalanine promoted TIMM for all strains tested. Since different strain-specific inducers were found, the possible involvement of multiple and strain-specific pathways is proposed. In addition, increasing the level of inorganic nitrogen also induced mortality at 37°C. Second, Schatz medium, a mineral medium with low salt concentrations, also induced TIMM at 37°C. Schatz medium has a higher water activity than MM284 and thus renders the cell in a more hyperosmotic state where water will transport from the medium to the cell, putting pressure on the cell membrane. Third, C. metallidurans CH34 grown autotrophically also exhibited increased mortality at 37°C.
Scrutinizing protective agents able to inhibit TIMM showed that a complete mitigation of the TIMM phenotype was reached by the addition of a non-ionic osmolyte but not by ROS scavengers, indicating that ROS is not the primary or main cause of the observed mortality at 37°C. Addition of sorbitol to all known TIMM-promoting conditions protected the cells. A second protective factor for Schatz medium was increasing the osmolarity of the growth medium by the addition of salt approximating the level in MM284. However, the protective function of salt, but not of sorbitol, in Schatz medium was counteracted by the addition of CA. Thus, sorbitol exerts a general protective function to alleviate mortality of the cells at 37°C. Sorbitol has been shown to promote cell growth of Zymomonas mobilis in environments with high sugar concentration and had also a protective function under heat and ethanol stress, although the molecular mechanisms involved in tolerance to the stress conditions are still unclear (Loos et al., 1994; Sootsuwan et al., 2013). In yeast suffering cell wall defects, an osmotic stabilizer, i.e. sorbitol, was required for growth and proliferation, especially at elevated temperature, and it was postulated that cells might employ a non-lethal morphology checkpoint to monitor appropriate turgor pressure before undergoing further growth (Martin et al., 1993; Harrison et al., 2001; Hohmann, 2002). Increasing osmolarity of the growth medium or addition of sorbitol likely promotes cell membrane stability, which might be crucial for cell survival and further growth at 37°C.
Additional TIMM assays with C. metallidurans CH34 revealed a frequency of inherited stable TIMM resistance of 29%, with lower cross-resistance to other TIMM-inducing media, confirming the existence of multiple TIMM-promoting pathways. Two stable inherited C. metallidurans CH34 TIMM survivors showed multiple mutational changes, including insertions, deletions, point and frameshift mutations, and the redistribution of seven mobile genetic elements (MGEs), including IS1088, ISRme15, IS1090, IS1086, IS1087B and Tn6048. Transposition frequency of IS elements can be promoted by stress challenges, and increased transposition of various IS elements under elevated temperature conditions was observed in Burkholderia multivorans ATCC 17616 (Ohtsubo et al., 2005). Furthermore, both TIMM survivors tested displayed loss of autotrophy, which was confirmed by a large 60-kb deletion comprising the cbb/hox locus, and this excision was most probably caused by IS1071-mediated deletion (Mijnendonckx et al., 2013). The many mutational changes observed in these two stable C. metallidurans CH34 TIMM survivors resemble the activation of a transient temperature-induced hypermutator phenotype somewhere during the selective condition.
One of the mutations shared by both TIMM survivors was a single-nucleotide substitution in Rmet_1009, resulting in a K62R substitution in the encoded oxidoreductase. As deletion of Rmet_1009 in the parental strain resulted in TIMM resistance, it is likely that this K62R substitution presents a loss-of-function mutation. The Rmet_1009 gene encodes for a RutE-like oxidoreductase, although not located in a rutABCDEFG operon as in E. coli. The rut operon, which is under the control of the nitrogen regulatory protein C (NtrC) (Zimmer et al., 2000), is involved in the degradation of exogenous pyrimidines as the sole nitrogen source (Loh et al., 2006; Kim et al., 2010). Interestingly, this degradation only occurs at room temperature and not at 37°C, probably due to the inadequate ability to remove toxic malonic semialdehyde at higher temperatures (Loh et al., 2006; Kim et al., 2010). Currently, we do not know if Rmet_1009, similar to RutE, reduces malonic semialdehyde to 3-hydroxypropionic acid and if C. metallidurans CH34 carries other genes that encode for proteins that enable the removal of malonic semialdehyde, similar to Cupriavidus necator H16 (Arenas-López et al., 2019). Nevertheless, our results point toward metabolic poisoning of C. metallidurans grown in high-concentration nitrogen-containing media at 37°C.
Data Availability Statement
The datasets presented in this study can be found in online repositories. The names of the repository/repositories and accession number(s) can be found below: https://www.ncbi.nlm.nih.gov/, PRJNA641768.
Author Contributions
RV and AA: conceptualization, writing–review and editing, and supervision. RV, JV, and AA: methodology. RV, JV, WH, PM, and AP: validation, formal analysis, and investigation. RV: data curation and visualization. RV and JV: writing original draft preparation. NL: project administration. All authors have read and agreed to the published version of the manuscript.
Conflict of Interest
The authors declare that the research was conducted in the absence of any commercial or financial relationships that could be construed as a potential conflict of interest.
Supplementary Material
The Supplementary Material for this article can be found online at: https://www.frontiersin.org/articles/10.3389/fmicb.2021.698330/full#supplementary-material
Supplementary Table 1 | Primers used in this study.
References
Arenas-López, C., Locker, J., Orol, D., Walter, F., Busche, T., Kalinowski, J., et al. (2019). The genetic basis of 3-hydroxypropanoate metabolism in Cupriavidus necator H16. Biotechnol. Biofuels 12:150.
Arroua, B., Bellanger, X., Guilloteau, H., Mathieu, L., and Merlin, C. (2014). Atypical stress response to temperature and NaOCl exposure leading to septation defect during cell division in Cupriavidus metallidurans CH34. FEMS Microbiol. Lett. 353, 32–39.
Baatout, S., De Boever, P., and Mergeay, M. (2005). Temperature-induced changes in bacterial physiology as determined by flow cytometry. Ann. Microbiol. 55, 73–80.
Brim, H., Heyndrickx, M., de Vos, P., Wilmotte, A., Springael, D., Schlegel, H. G., et al. (1999). Amplified rDNA restriction analysis and further genotypic characterisation of metal-resistant soil bacteria and related facultative hydrogenotrophs. Syst. Appl. Microbiol. 22, 258–268. doi: 10.1016/s0723-2020(99)80073-3
Diels, L., and Mergeay, M. (1990). DNA probe-mediated detection of resistant bacteria from soils highly polluted by heavy metals. Appl. Environ. Microbiol. 56, 1485–1491. doi: 10.1128/aem.56.5.1485-1491.1990
Ferrieres, L., Hemery, G., Nham, T., Guerout, A. M., Mazel, D., Beloin, C., et al. (2010). Silent mischief: bacteriophage Mu insertions contaminate products of Escherichia coli random mutagenesis performed using suicidal transposon delivery plasmids mobilized by broad-host-range RP4 conjugative machinery. J. Bacteriol. 192, 6418–6427. doi: 10.1128/jb.00621-10
Harrison, J. C., Bardes, E. S., Ohya, Y., and Lew, D. J. (2001). A role for the Pkc1p/Mpk1p kinase cascade in the morphogenesis checkpoint. Nat. Cell Biol. 3, 417–420. doi: 10.1038/35070104
Hladky, S. B., and Barrand, M. A. (2014). Mechanisms of fluid movement into, through and out of the brain: evaluation of the evidence. Fluids Barriers CNS 11:26. doi: 10.1186/2045-8118-11-26
Hohmann, S. (2002). Osmotic stress signaling and osmoadaptation in yeasts. Microbiol. Mol. Biol. Rev. 66, 300–372. doi: 10.1128/mmbr.66.2.300-372.2002
Kim, K. S., Pelton, J. G., Inwood, W. B., Andersen, U., Kustu, S., and Wemmer, D. E. (2010). The Rut pathway for pyrimidine degradation: novel chemistry and toxicity problems. J. Bacteriol. 192, 4089–4102. doi: 10.1128/jb.00201-10
Langevin, S., Vincelette, J., Bekal, S., and Gaudreau, C. (2011). First case of invasive human infection caused by Cupriavidus metallidurans. J. Clin. Microbiol. 49, 744–745. doi: 10.1128/jcm.01947-10
Loh, K. D., Gyaneshwar, P., Markenscoff Papadimitriou, E., Fong, R., Kim, K. S., Parales, R., et al. (2006). A previously undescribed pathway for pyrimidine catabolism. Proc. Natl. Acad. Sci. U.S.A. 103, 5114–5119. doi: 10.1073/pnas.0600521103
Loos, H., Kramer, R., Sahm, H., and Sprenger, G. A. (1994). Sorbitol promotes growth of Zymomonas mobilis in environments with high concentrations of sugar: evidence for a physiological function of glucose-fructose oxidoreductase in osmoprotection. J. Bacteriol. 176, 7688–7693. doi: 10.1128/jb.176.24.7688-7693.1994
Martin, H., Arroyo, J., Sanchez, M., Molina, M., and Nombela, C. (1993). Activity of the yeast MAP kinase homologue Slt2 is critically required for cell integrity at 37 degrees C. Mol. Gen. Genet. 241, 177–184. doi: 10.1007/bf00280215
Mergeay, M. (2000). “Bacteria adapted to industrial biotopes: metal-resistant Ralstonia,” in Bacterial Stress Responses, eds G. Storz and R. Hengge-Aronis (Washington, DC: American Society for Microbiology), 403–414.
Mergeay, M., Houba, C., and Gerits, J. (1978). Extrachromosomal inheritance controlling resistance to cadmium, cobalt, copper and zinc ions: evidence from curing a Pseudomonas. Arch. Physiol. Biochem. 86, 440–442.
Mergeay, M., Nies, D., Schlegel, H. G., Gerits, J., Charles, P., and Van Gijsegem, F. (1985). Alcaligenes eutrophus CH34 is a facultative chemolithotroph with plasmid-bound resistance to heavy metals. J. Bacteriol. 162, 328–334. doi: 10.1128/jb.162.1.328-334.1985
Mergeay, M., and Van Houdt, R. (eds) (2015). Metal Response in Cupriavidus metallidurans, Volume I: From Habitats to Genes and Proteins. Switzerland: Springer International Publishing.
Mijnendonckx, K., Ali, M. M., Provoost, A., Janssen, P., Mergeay, M., Leys, N., et al. (2019). Spontaneous mutation in the AgrRS two-component regulatory system of Cupriavidus metallidurans results in enhanced silver resistance. Metallomics 11, 1912–1924. doi: 10.1039/c9mt00123a
Mijnendonckx, K., Provoost, A., Ott, C. M., Venkateswaran, K., Mahillon, J., Leys, N., et al. (2013). Characterization of the survival ability of Cupriavidus metallidurans and Ralstonia pickettii from space-related environments. Microb. Ecol. 65, 347–360. doi: 10.1007/s00248-012-0139-2
Ohtsubo, Y., Genka, H., Komatsu, H., Nagata, Y., and Tsuda, M. (2005). High-temperature-induced transposition of insertion elements in Burkholderia multivorans ATCC 17616. Appl. Environ. Microbiol. 71, 1822–1828. doi: 10.1128/aem.71.4.1822-1828.2005
Sadouk, A., and Mergeay, M. (1993). Chromosome mapping in Alcaligenes eutrophus CH34. Mol. Gen. Genet. 240, 181–187. doi: 10.1007/bf00277055
Schafer, A., Tauch, A., Jager, W., Kalinowski, J., Thierbach, G., and Puhler, A. (1994). Small mobilizable multi-purpose cloning vectors derived from the Escherichia coli plasmids pK18 and pK19: selection of defined deletions in the chromosome of Corynebacterium glutamicum. Gene 145, 69–73. doi: 10.1016/0378-1119(94)90324-7
Schatz, A., and Bovell, C. Jr. (1952). Growth and hydrogenase activity of a new bacterium, Hydrogenomonas facilis. J. Bacteriol. 63, 87–98. doi: 10.1128/jb.63.1.87-98.1952
Schmidt, T., Stoppel, R. D., and Schlegel, H. G. (1991). High-level nickel resistance in Alcaligenes xylosoxydans 31A and Alcaligenes eutrophus KTO2. Appl. Environ. Microbiol. 57, 3301–3309. doi: 10.1128/aem.57.11.3301-3309.1991
Simha, P., and Ganesapillai, M. (2017). Ecological Sanitation and nutrient recovery from human urine: how far have we come? A review. Sustain. Environ. Res. 27, 107–116. doi: 10.1016/j.serj.2016.12.001
Sootsuwan, K., Thanonkeo, P., Keeratirakha, N., Thanonkeo, S., Jaisil, P., and Yamada, M. (2013). Sorbitol required for cell growth and ethanol production by Zymomonas mobilis under heat, ethanol, and osmotic stresses. Biotechnol. Biofuels 6:180. doi: 10.1186/1754-6834-6-180
Timotius, K., and Schlegel, H. G. (1987). Aus Abwässern isolierte nickel-resistente Bakterien. Nachr. Akad. Wiss. Gött. II. Math. Physik. Kl. 3, 15–23.
Van der Lelie, D., Sadouk, A., Ferhat, A., Taghavi, S., Toussaint, A., and Mergeay, M. (1992). “Stress and survival in Alcaligenes eutrophus CH34: effects of temperature and genetic rearrangements,” in Gene Transfers and Environment, ed. M. Gauthier (Berlin: Springer), 27–32. doi: 10.1007/978-3-642-77450-8_5
Van Houdt, R., Monsieurs, P., Mijnendonckx, K., Provoost, A., Janssen, A., Mergeay, M., et al. (2012). Variation in genomic islands contribute to genome plasticity in Cupriavidus metallidurans. BMC Genomics 13:111. doi: 10.1186/1471-2164-13-111
Van Houdt, R., Provoost, A., Van Assche, A., Leys, N., Lievens, B., Mijnendonckx, K., et al. (2018). Cupriavidus metallidurans strains with different mobilomes and from distinct environments have comparable phenomes. Genes (Basel) 9:E507.
Yancey, P. H. (2001). Water stress, osmolytes and proteins. Am. Zool. 41, 699–709. doi: 10.1093/icb/41.4.699
Zimmer, D. P., Soupene, E., Lee, H. L., Wendisch, V. F., Khodursky, A. B., Peter, B. J., et al. (2000). Nitrogen regulatory protein C-controlled genes of Escherichia coli: scavenging as a defense against nitrogen limitation. Proc. Natl. Acad. Sci. U.S.A. 97, 14674–14679. doi: 10.1073/pnas.97.26.14674
Keywords: Cupriavidus, growth temperature, amino acids, nitrogen, osmotic pressure
Citation: Van Houdt R, Vandecraen J, Heylen W, Leys N, Monsieurs P, Provoost A and Aertsen A (2021) Phenotypic and Genetic Characterization of Temperature-Induced Mutagenesis and Mortality in Cupriavidus metallidurans. Front. Microbiol. 12:698330. doi: 10.3389/fmicb.2021.698330
Received: 21 April 2021; Accepted: 11 June 2021;
Published: 09 July 2021.
Edited by:
Ulrike Kappler, The University of Queensland, AustraliaReviewed by:
Triwibowo Yuwono, Gadjah Mada University, IndonesiaAbdul Rehman, University of the Punjab, Pakistan
Copyright © 2021 Van Houdt, Vandecraen, Heylen, Leys, Monsieurs, Provoost and Aertsen. This is an open-access article distributed under the terms of the Creative Commons Attribution License (CC BY). The use, distribution or reproduction in other forums is permitted, provided the original author(s) and the copyright owner(s) are credited and that the original publication in this journal is cited, in accordance with accepted academic practice. No use, distribution or reproduction is permitted which does not comply with these terms.
*Correspondence: Rob Van Houdt, rvhoudto@sckcen.be