- 1Department of Nutrition and Public Health, Universidad del Bío-Bío, Chillán, Chile
- 2Department of Public Health, Palacký University Olomouc, Olomouc, Czechia
- 3Servicio de Salud Ñuble, Chillán, Chile
- 4Austrian Agency for Health and Food Safety, Institute for Medical Microbiology and Hygiene, Vienna, Austria
- 5Intestinal Bacteriology Research Laboratory, Hospital Infantil de México Federico Gómez, Mexico City, Mexico
- 6Faculty of Medicine, Biological Sciences Graduate Program, Posgrado en Ciencias Biológicas, Universidad Nacional Autónoma de México, Mexico City, Mexico
- 7Microbiology and Probiotics Laboratory, Institute of Nutrition and Food Technology, Universidad de Chile, Santiago, Chile
- 8foodmicrobe.com, Nottinghamshire, United Kingdom
Cronobacter sakazakii is an enteropathogen that causes neonatal meningitis, septicemia, and necrotizing enterocolitis in preterm infants and newborns with a mortality rate of 15 to 80%. Powdered and dairy formulas (P-DF) have been implicated as major transmission vehicles and subsequently the presence of this pathogen in P-DF led to product recalls in Chile in 2017. The objective of this study was to use whole genome sequencing (WGS) and laboratory studies to characterize Cronobacter strains from the contaminated products. Seven strains were identified as C. sakazakii, and the remaining strain was Franconibacter helveticus. All C. sakazakii strains adhered to a neuroblastoma cell line, and 31 virulence genes were predicted by WGS. The antibiograms varied between strains. and included mcr-9.1 and blaCSA genes, conferring resistance to colistin and cephalothin, respectively. The C. sakazakii strains encoded I-E and I-F CRISPR-Cas systems, and carried IncFII(pECLA), Col440I, and Col(pHHAD28) plasmids. In summary, WGS enabled the identification of C. sakazakii strains and revealed multiple antibiotic resistance and virulence genes. These findings support the decision to recall the contaminated powdered and dairy formulas from the Chilean market in 2017.
Introduction
On June 2, 2017, the Chilean Ministry of Health issued a national and international food safety alert as a result of the presence of Cronobacter sakazakii in one batch of powdered infant formula (PIF) and one batch of dairy products (powder milk). This preventive measure was adopted due to the risk of disease associated with C. sakazakii in vulnerable populations (Parra-Flores et al., 2018b).
Cronobacter is a genus of pathogens formerly known as Enterobacter sakazakii and is now made up of seven species: C. sakazakii, C. malonaticus, C. universalis, C. turicensis, C. muytjensii, C. dublinensis, and C. condimenti (Iversen et al., 2008; Joseph et al., 2012; Stephan et al., 2014). The most susceptible population groups are newborns younger than 12 months and the elderly (Hariri et al., 2013; Patrick et al., 2014). The clinical profile is mainly meningitis, septicemia, or necrotizing enterocolitis, with a mortality ranging from 15 to 80% (Kleiman et al., 1981; Holý and Forsythe, 2014). The disease is associated with the consumption of contaminated rehydrated PIF. The pathogen has also been isolated from dairy products, infant cereals, milk substitutes, water, food preparation surfaces, and expressed breast milk (Baumgartner et al., 2009; Parra et al., 2015; Vojkovska et al., 2016; Morato-Rodríguez et al., 2018). The source of contamination is closely associated with powdered milk (PM) manufacturing plants and the ingredients used in its manufacture (Holý and Forsythe, 2014). Internationally, the incidence of Cronobacter spp. in PM ranges from 3 to 30% (Ling et al., 2021). In Chile, its incidence in PIF was 9.5% in 2015, 35% in 2017, and 4.7% in 2020 (Parra et al., 2015; Parra-Flores et al., 2018b, 2020).
The genus Cronobacter has diversified over the course of its evolution, with some species pathogenic to humans and other species whose impact on human health is still unknown (Forsythe, 2018). C. sakazakii and C. malonaticus were reported are the species with the highest clinical significance, having been involved in cases and outbreaks reported in the literature (Forsythe, 2018; Parra-Flores et al., 2021). However, information on the diversity, pathogenicity, and virulence of Cronobacter species obtained from various sources is still poorly understood.
Key aspects for the prognosis and development of the disease caused by C. sakazakii include the presence of virulence factors which may be plasmidborne (Shi et al., 2018; Aly et al., 2019), its adherence and invasiveness in cell lines (Cruz et al., 2011; Parra-Flores et al., 2018a; Holý et al., 2021), the presence of genes such as ompA, cpa, fliC, hly, sip, aut, plas, and inv (Cruz et al., 2011; Franco et al., 2011; Aldubyan et al., 2017; Holý et al., 2019), sialic acid utilization, as well as its capsule and endotoxin production (Ogrodzki and Forsythe, 2015). Another key aspect is resistance to beta-lactam antibiotics such as cephalothin, cefotaxime, ceftazidime, and ampicillin (Flores et al., 2011; Lee et al., 2012; Fei et al., 2017; Holý et al., 2021).
Whole-genome sequencing (WGS) studies generate a high degree of information content for pathogenic strains, including an accurate understanding of the taxonomic differences between them. WGS is used as a tool to identify and genotype pathogens (MLST, CRISPR-Cas, serogroup), as well as predict antibiotic-associated and virulence genes. Thus allowing more precise epidemiological links to be established (Leopold et al., 2014). WGS analysis and genome comparison, in addition to the use of in vivo and in vitro models, provide us with more accurate information about the pathogenic potential of C. sakazakii (Lehner et al., 2018).
In this study, we used WGS and laboratory studies to characterize the virulence, and antibiotic resistance genes of C. sakazakii strains isolated from powdered infant formula and powdered milk.
Materials and Methods
Strains Used in the Study
Eight suspected strains of Cronobacter isolated in 2017 from powdered infant formula and powdered milk were used. The strains were isolated after pre-enrichment in buffered peptone water (BPW) followed by Enterobacteriaceae enrichment broth (BD Difco, Sparks, MD, United States), isolation on Brilliance chromogenic agar CM 1035 (Oxoid Thermo-Fisher, United Kingdom) and purification on trypticase soy agar (BD Difco, Sparks, MD, United States) (Parra-Flores et al., 2018b). The isolates were presumptive identified as C. sakazakii or Cronobacter species unknown. using polymerase chain reaction (PCR) probes by gene amplification of 16S (Lehner et al., 2004), ompA (Mohan-Nair and Venkitanarayanan, 2006), rpoB (Stoop et al., 2009), cgcA (Carter et al., 2013), and fusA gene sequencing (Baldwin et al., 2009).
Reidentification of Cronobacter Isolates
For reidentification, the eight isolates were cultured on Columbia blood agar plates (bioMérieux, Marcy-l’Étoile, France) at 37°C for 24 h. Primary species identification from single colonies was carried out by matrix-assisted laser desorption ionization - time-of-flight mass spectrometry (MALDI-TOF-MS) (Bruker, Billerica, MA, United States) and MBT Compass IVD software 4.1.60 (Bruker), described by Lepuschitz et al. (2017).
Whole-Genome Sequencing
High quality genomic DNA from an overnight culture was obtained using the MagAttract high molecular weight (HMW) DNA kit (Qiagen, Hilden, Germany). The quantification of input DNA was performed with a Qubit 2.0 fluorometer (Thermo Fisher Scientific, Waltham, MA, United States) and the double-stranded DNA (dsDNA) BR assay kit (Thermo Fisher Scientific). WGS of C. sakazakii strains was performed as described (Lepuschitz et al., 2019). Default parameters were used for all software unless otherwise specified. Raw reads were quality controlled using FastQC v0.11.9. Trimmomatic v0.36 (Bolger et al., 2014) was used to remove adapter sequences and to trim the last 10 bp of each sequence and sequences with quality scores <20. Reads were assembled using SPAdes v3.11.1 (Bankevich et al., 2012). Contigs were filtered for a minimum coverage of 5× and a minimum length of 200 bp using SeqSphere + software v6.0.0 (Ridom GmbH, Würzburg, Germany).
A total of 3,678 targets were used to establish the core genome multilocus sequence typing (cgMLST) scheme using strain ATCC BAA-894 as reference. According to the determined cgMLST scheme, the genotypic relationships of isolates was visualized using a minimum spanning tree (MST) as per Lepuschitz et al. (2019). In addition, the sequences of the seven housekeeping genes (atpD, fusA, glnS, gltB, gyrB, infB, and ppsA) of the conventional multilocus sequence typing (MLST) scheme were extracted and compared with the Cronobacter MLST database, from which sequence types (STs) were assigned in silico (Forsythe et al., 2014). The strains in this study are ID 3196–3202 in the Cronobacter PubMLST database.
O-Serotyping
The presence of the serotype O region-specific gnd and galF genes was determined by WGS sequence analysis with the BIGSdb tool present in the PubMLST database1.
Cell Line Adhesion and Invasion Assay
The mouse neuroblastoma cell line N1E-115 (American Type Culture Collection, Manassas, VA, United States) was used for the assay. The N1E-115 cell line was cultured in Dulbecco’s modified Eagle medium (DMEM) supplemented with 4.5 g/L glucose (GIBCO, United States) and 7% fetal bovine serum (FBS) (GIBCO, United States). They were then differentiated in DMEM medium supplemented with 2% FBS and 1.25% dimethyl sulfoxide for 5 days. Cells were seeded in 24-well plates (Corning Life Sciences, United States) at 1 × 105 cells/ml and infected at 100:1 multiplicity of infection with each C. sakazakii isolate after culture in Luria broth. Infection was carried out for 4 h at 37°C in 5% CO2. After incubation, the cells were washed with 1 × phosphate-buffered saline (PBS), and C. sakazakii was removed by the addition of 1 ml of 0.1% Triton X-100 (Amresco, OH, United States). To quantify the colony-forming units (CFU) of bacteria attached to the N1E-115 cell, various dilutions were performed in Luria broth (Cruz et al., 2011).
For the invasion assay, the preparation of the N1E-115 monolayers and the time for infection were as described for the adhesion assay. After 4 h of incubation, the infected monolayers were washed with 1 × PBS and incubated with 1 ml of DMEM plus 300 μg/ml lysozyme (Sigma-Aldrich, United States) and 100 μg/ml gentamicin (Sigma-Aldrich, United States) for 2 h at 37°C in 5% CO2. The cells were washed three times with 1 × PBS, separated with 1 ml of 0.1% Triton X-100, and plated on Luria-Bertani agar. Invasion frequencies were calculated as the number of bacteria that survived incubation with gentamicin and lysozyme divided by the total number of bacteria present in the absence of this antibiotic (bacterial adherence) (Cruz et al., 2011).
Both assays (adhesion, invasion) were repeated twice and performed in duplicate. The data are expressed as the means.
Detection of Virulence Factors in vitro
Seven genes were detected by PCR. The genes evaluated were plasminogen activator (cpa), presence of hemolysin (hly), siderophore-interacting protein (sip), invasin/intimin (inv), flagellin (fliC), autotransporter (aut), and outer membrane protein (ompA). The amplified products were stained and visualized on 1.5% agarose gel with 1.0 mg/ml ethidium bromide solution using an agarose gel imaging system (Mohan-Nair and Venkitanarayanan, 2006; Cruz et al., 2011; Holý et al., 2019).
Antibiotic Resistance Profile
The disk diffusion method was used in accordance with the recommendations of the Clinical and Laboratory Standards Institute (Clinical and Laboratory Standards Institute [CLSI], 2018). Commercial antibiotic disks consisting of ceftazidime (30 μg), cefotaxime (30 μg), amoxicillin-clavulanic acid (20/10 μg), ciprofloxacin (5 μg), cephalothin (30 μg), nalidixic acid (35 μg), gentamicin (10 μg), tetracycline (30 μg), chloramphenicol (30 μg), and ampicillin (10 μg) were used. Resistance/susceptibility profiles were characterized according to the manufacturer’s instructions. E. coli strain ATCC 25922 was used as quality control.
In silico Detection of Virulence and Antibiotic Resistance Genes
The existence of virulence genes was confirmed by using the task template feature in SeqSphere + for WGS data and the ResFinder tool from the Center for Genomic Epidemiology (CGE)2. Thresholds for the target scanning procedure were set as a required identity of ≥90% with the reference sequence and an aligned reference sequence ≥99%. For antimicrobial resistance genes, the Comprehensive Antibiotic Resistance Database was used with the default “perfect” and “strict” settings for sequence analysis (Jia et al., 2017), and Task Template AMRFinderPlus 3.2.3, available in Ridom SeqSphere + 7.0 software, was used with the EXACT method at the 100% setting and with BLAST alignment of protein sequences against the AMRFinderPlus database.
Plasmid Detection
The PlasmidFinder 2.1 and MobileElementFinder 1.0 tool were used to detect plasmids. We chose a minimum identity of 95 and 90%, respectively (see text footnote 2) (Carattoli et al., 2014; Johansson et al., 2021).
Profiling of CRISPR-Cas Loci
The search and characterization of CRISPR arrays and their association to Cas proteins was determined with CRISPRCasFinder and CRISPRDetect (Biswas et al., 2016; Couvin et al., 2018), available from the Institut de Biologie Intégrative de la Cellule in the Université Paris-Saclay server3 and University of Otago4. The types pf CRISPR systems were determinated with the CRISPRmap program (Lange et al., 2013).
Statistical Analysis
Statistical significance (p < 0.05) was determined using Student’s t-test for the adherence and invasion assays.
Results and Discussion
The eight strains initially identified in 2017 as Cronobacter were reidentified with MALDI-TOF MS as seven strains of Cronobacter sp. and one strain of Franconibacter helveticus (Table 1). At the time of original analysis, strain CH85 fusA gene sequence did not correspond with any known Cronobacter species. However, in the original paper (Parra-Flores et al., 2018b) it was designated as ‘Cronobacter spp.’ based on the results of the PCR probes. Due to the later recognition of the Franconibacter genus, the fusA sequence is now designated as ‘Franconibacter helveticus’ and this indicates that the original PCR probes lacked genus specificity. Subsequently, from WGS data and using average nucleotide identity (ANI), ribosomal MLST, and core genome MLST, six strains were confirmed as C. sakazakii ST1, one as C. sakazakii ST83, and the remaining strain as Franconibacter helveticus ST345. F. helveticus has previously been mis-identified as Cronobacter as they are closely related (Stephan et al., 2014; Jackson and Forsythe, 2016). In addition, the C. sakazakii strains identified as ST1 had the same O:1 serotype and the same gene loci for the O-antigen flanking genes gnd and galF (galF 2; gnd 1), in contrast to the ST83 isolate, whose loci were galF 21 and gnd 65.
The cgMLST scheme analysis revealed a cluster of six C. sakazakii ST1 strains with one to three allele differences and ST83 strain with 3,043 allele difference (Figure 1). C. sakazakii ST1 has been most frequently isolated from commercial PIF in various countries, from processing equipment for PIF manufacturing, and from patients with fatal meningitis, septicemia, or urinary tract infections (Joseph and Forsythe, 2012; Fei et al., 2015; Holý et al., 2021; Parra-Flores et al., 2021). Lepuschitz et al. (2017), in their multicenter study of infections caused by C. sakazakii in Europe, studied eight isolates identified as C. sakazakii ST1 that included two isolates from newborns’ feces with an epidemiological link to an outbreak in Austria in 2009. Furthermore, these eight isolates showed one allelic difference and were closely related to strain ATCC BAA-894, a strain isolated from a powdered infant formula in the United States in 2001, which was associated with an outbreak involving two newborns with necrotizing enterocolitis hospitalized in the same neonatal intensive care unit. C. sakazakii ST83 has also been isolated from severe clinical cases, in addition to being found in PIF and the environment, so it has been suggested that C. sakazakii strains ST4, ST1, ST8, ST12, and ST83 are the most likely to produce disease in infants and children (Himelright et al., 2002; Sonbol et al., 2013; Fei et al., 2017; Chase et al., 2017; Forsythe, 2018).
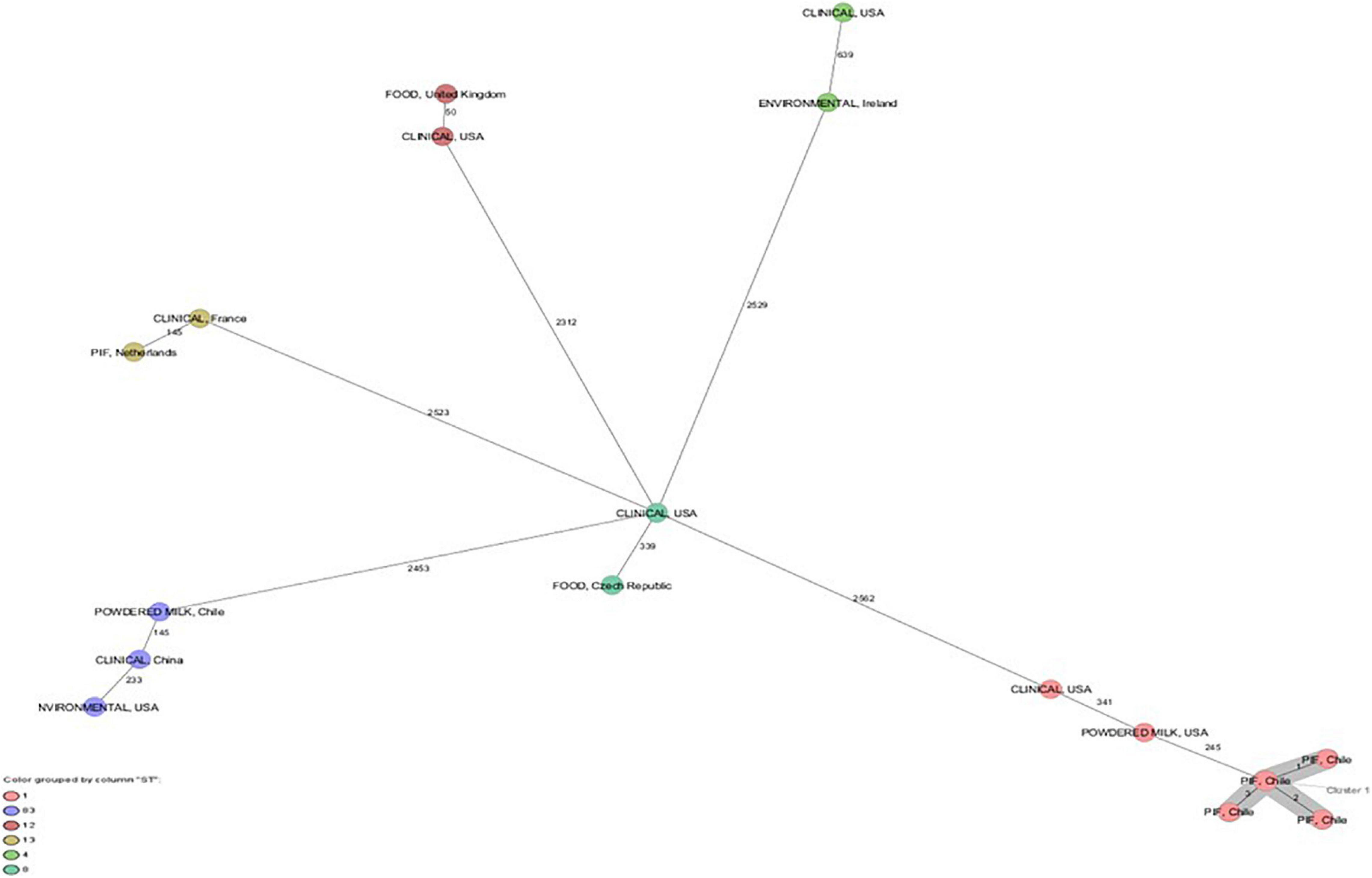
Figure 1. Minimum spanning tree (MST) of seven Cronobacter sakazakii strains from powdered infant formula and powdered milk isolated in Chile. In addition, C. sakazakii strains with ST1, ST4, ST8, ST12, ST13, and ST83 of clinical origin and food. Calculation of the MST was based on the defined cgMLST scheme comprising 3678 target genes from ATCC BAA-894. Isolates are represented as colored circles according to the classical MLST. Black numbers accord to the allelic difference between isolates. Isolates with closely related genotypes are marked as Cluster.
Several authors have studied the invasiveness and adhesion processes in vitro in different cell lines, such as CaCo-2, HEp-2, HBMECs, IEC-6, and N1E-115, as an initial stage of pathogenesis by C. sakazakii (Mange et al., 2006; Cruz et al., 2011, Holý et al., 2019). Adherence, as a first step, alters the epithelium, making it possible for the pathogen to cross the mucosa and then migrate to the bloodstream (Hunter and Bean, 2013). In our study, 100% of the strains adhered the N1E-115 cell line with ranges from 2.2 to 16.3 × 106 CFU/mL. Strain CH45 (ST1) was the most adherent and strain CH50 (ST83) the least. In the invasion assay only four strains (50%) invaded with frequencies from 0.0002 to 0.00009% (Figure 2). These values are similar to those reported by Holý et al. (2019), where 100% and 66.7% of the C. sakazakii clinical strains evaluated adhered to and invaded the N1E-115 cell line, respectively. Recently, Holý et al. (2021), when evaluating clinical strains of C. sakazakii with the N1E-115 cell line, found a value of 2.2 × 106 CFU/mL for adherence, which is much lower than those found in our study. However, the rates of invasion of C. sakazakii in our study are lower than those reported by various authors, such as Mange et al. (2006); Townsend et al. (2007), Parra-Flores et al. (2018a), and Holý et al. (2019). Recently, Veronica et al. (2020) found that N1E-115 neuroblastoma cells are highly permissive to infection by a wild-type C. sakazakii strain, suggesting that flagellum and outer membrane proteins are necessary to promote invasion of N1E-115 cells, but not adherence.
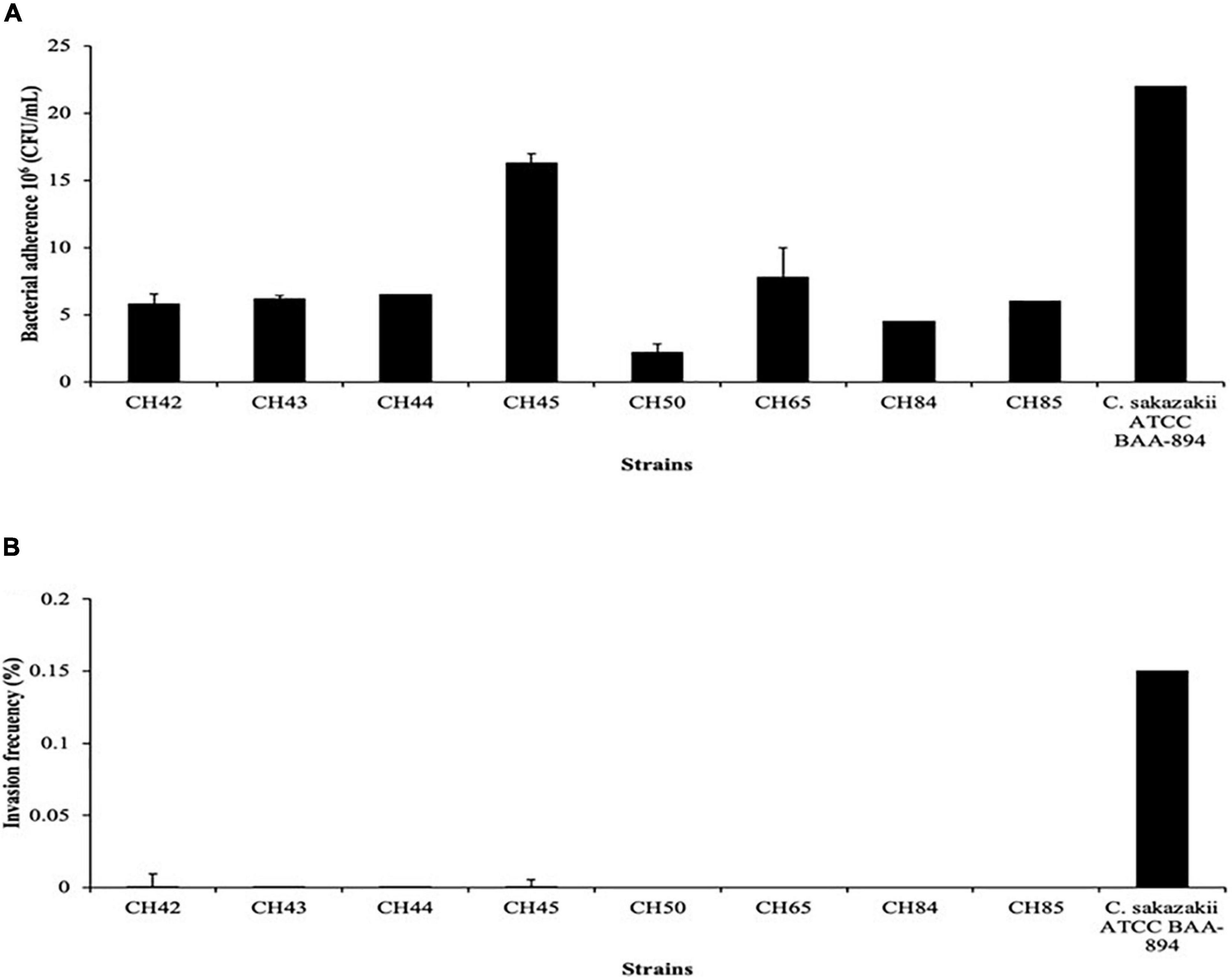
Figure 2. Bacterial adherence (A) and invasion frequency (B) of Cronobacter sakazakii strains on neuroblastoma (NT) cell line.
In regard to the presence of putative virulence factors by PCR, all C. sakazakii strains were positive for six genes (hlyA, ompA, aut, fliC, sip, and cpa), and all were negative for the inv gene (Table 2). Holý et al. (2019) found that only 76% of strains expressed the inv gene among strains with good levels of adherence but low invasion rates in HT-29 and N1E-115 cell lines. The inv gene encodes a protein mediating bacterial adhesion and basolateral and apical invasion in epithelial cells. The main function of this protein is as an invasin and was previously described by Chandrapala et al. (2014).
Thirty-one virulence genes were detected in silico, which were grouped into flagellar proteins, outer membrane proteins (ompA), chemotaxis (motB), hemolysins (hlyIII), invasion (lpxA), plasminogen activator (cpa), colonization (mviM), transcriptional regulator (sdiA), macrophage survival, sialic acid utilization (nanA, nanK), and toxins-antitoxins (TA) (fic) (Table 3). These results are consistent with PCR findings, with the exception of the inv gene, which was not detected by PCR. However, invasion genes such as lpx were present in the genome, and therefore further studies are needed to evaluate the specificity of the inv gene.
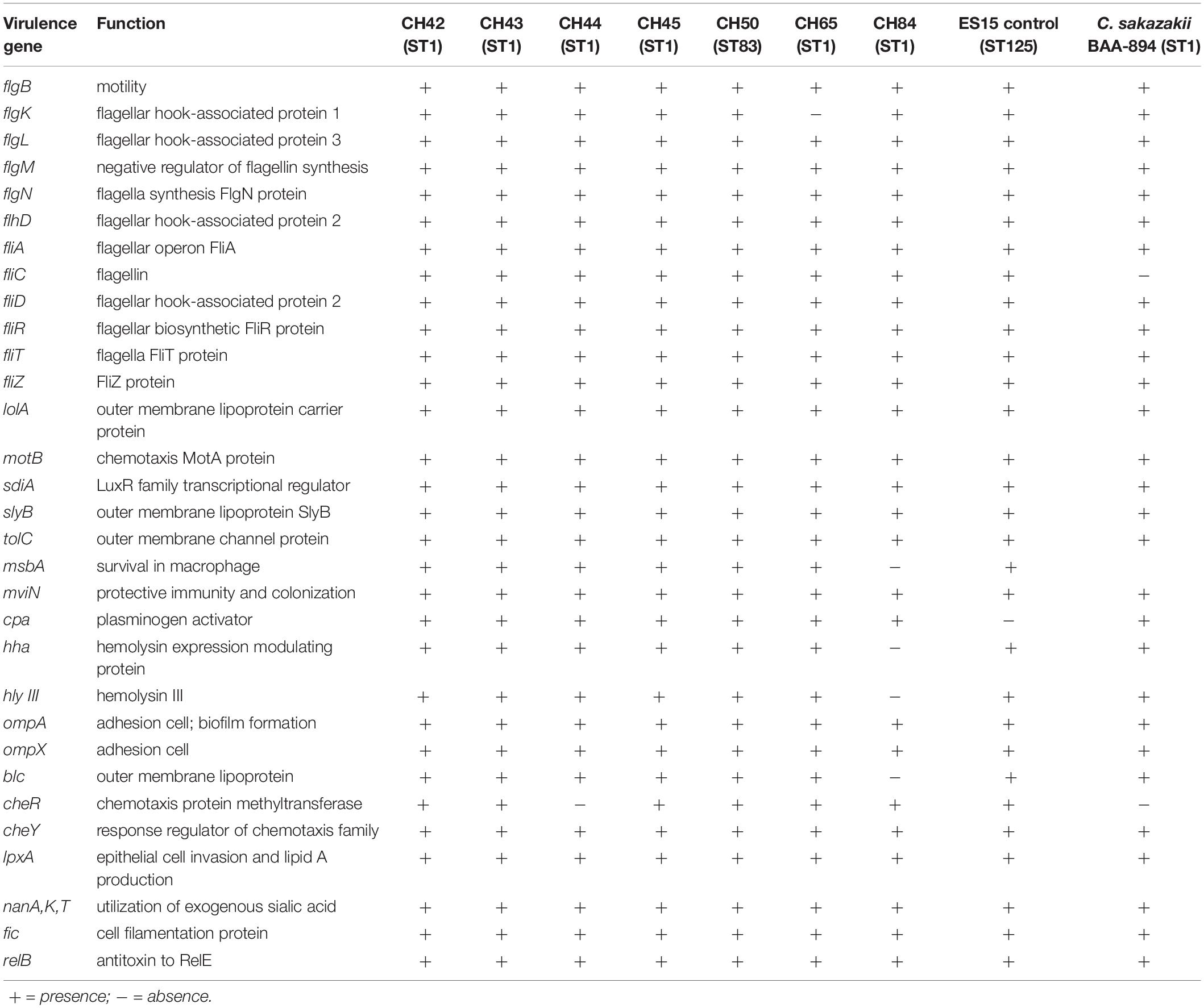
Table 3. Putative virulence and other genes distribution among seven strains of Cronobacter sakazakii by whole-genome sequencing (WGS).
The OmpA and OmpX proteins of C. sakazakii are involved in basolateral adhesion in CaCo2 and INT-407 cell lines, in addition to a possible involvement in the crossing of the blood–brain barrier by Cronobacter spp. (Mange et al., 2006; Kim et al., 2010). The Cpa protein is related to serum resistance and systemic spread of C. sakazakii. The cpa locus could be considered specific to C. sakazakii and C. universalis (Franco et al., 2011). However, highly virulent clinical ST8 strains of C. sakazakii that bear plasmid pESA3 have been found to lack the cpa gene, suggesting the likely presence of other virulence genes as responsible for the disease (Jang et al., 2020). Hemolysins (Hly) are outer membrane proteins or exoproteins found in various pathogens belonging to the Enterobacteriaceae family, such as Escherichia coli, Klebsiella, Enterobacter, and Gram-positive pathogens such as Bacillus cereus, with hemolytic capacity (Mare et al., 2020; Mazzantini et al., 2020). This hly gene was found when analyzing the C. sakazakii BAA-894 strain that was isolated from the neonatal intensive care unit outbreak in 2001 (Himelright et al., 2002).
Twelve genes associated with flagellar proteins, synthesis, operons, and flagellin (fliC) were found (Table 3). Their main functions are bacterial motility, adherence capacity, biofilm formation, and stimulation of proinflammatory responses through receptor TLR5 signaling (Proudy et al., 2008). Aldubyan et al. (2017), described the prevailing role of flagella in the adherence and invasion of pathogenic fliC-containing bacteria. However, some authors have proposed that flagellar motility in C. sakazakii is not necessary for biofilm formation (Ye et al., 2015), showing that self-aggregation is a biological function of the flagellum that favors decreased motility (Hoeflinger and Miller, 2017). Dingle et al. (2011) described, in Clostridium difficile mutant strains, the important role of the major flagellar subunits FliC and FliD in their increased adherence to Caco-2 cells and their greater virulence than wild-type. The presence of the nanA and nanK genes, encoding the ability to use of exogenous sialic acid as a carbon source, is another important virulence factor. This particular feature is considered an evolutionary adaptation of C. sakazakii, as this compound is found naturally in breast milk and is added supplementally to PIF due to its association with brain development as it is a major component of gangliosides (Forsythe, 2018). Sohanpal et al. (2007) demonstrated how sialic acid can modify bacterial surfaces by regulating the expression of enzymes such as sialidase and adhesins or inhibiting transcription factors of the fimB gene, part of the fim operon, which are virulence factors that mediate epithelial cell adhesion and invasion (Severi et al., 2007). Moreover, the use of sialic acid by bacterial cells has been linked to several virulence factors. The bacterial glycolipid capsule is an example of host molecular adaptation, as it helps the pathogen circumvent host immune responses. Neonatal meningitic Escherichia coli K1 uses sialic acid to modify its cell surface, and Cronobacter spp. produce capsular material when cultured in milk (Caubilla-Barron and Forsythe, 2007). However, there is no evidence of genes encoding for a sialic acid capsule in C. sakazakii strains.
Few reports have identified possible the presence of toxins associated with C. sakazakii. In our study we found the typical fic TA gene and the relB gene, which encodes the relE antitoxin. Toxin–antitoxin (TA) systems are small genetic elements found in plasmids, phage genomes and chromosomes of different bacterial species. Furthermore, these TA genes have a prominent role in the physiology of bacterial stress, such as in the stabilization of horizontally acquired mobile elements. They are also involved in a persistence phenotype in some species, such as E. coli and Salmonella (Deter et al., 2017; Walling and Butler, 2019). Finkelstein et al. (2019) found, in preliminary studies of C. sakazakii isolates, that two typical TA genes, fic and hipA, followed species-specific evolutionary lines. When expanding their focus to evaluate the presence of five TA in C. sakazakii, they found that some strains contained either a toxin or an antitoxin component but not both. Only 55 of the 63 strains tested possessed three of these genes (fic, relB, and parDE), pointing to possible nucleotide polymorphisms at these loci or to the absence of the genes. Additionally, the only strains that contained all 22 TA homologs were the C. sakazakii ST1.
Five strains of C. sakazakii were resistant in vitro to cephalothin, four to ampicillin, and two to ceftazidime, amoxicillin/clavulanic acid and nalidixic acid. In addition, one strain was resistant to four of the 10 antibiotics tested (40%) and two strains to two antibiotics (20%) (Table 4). Resistance of C. sakazakii to cephalothin, ceftazidime, and ampicillin has been evidenced in several previous studies, and a quasi-intrinsic resistance to cephalothin by Cronobacter spp. has been proposed (Kim et al., 2008; Molloy et al., 2009; Flores et al., 2011; Chon et al., 2012). Among clinical strains of C. sakazakii, Holý et al. (2019) did not find antibiotic resistant strains. In contrast, a more recent study in five C. sakazakii strains isolated from powdered milk distributed in Latin America found 100% to be resistant to cefotaxime and ampicillin, 60% to cefepime, 40% to amikacin, and 20% to cephalothin. One strain of C. sakazakii was resistant to six of the 12 antibiotics tested (54.5%), while another strain was resistant to five (50%) (Parra-Flores et al., 2020). These outcomes should be studied further due to the emergence of multidrug-resistant C. sakazakii strains, such as the one causing neonatal meningitis in China that was resistant to eight antibiotics (Zeng et al., 2018), which represents a clear health risk for infants.
Regarding the in silico presence of antibiotic resistance genes, all strains of C. sakazakii had the same efflux genes (adeF, H-NS, msbA, marA, kpnF, kpnE, emrR, emrB, rsmA, and cRP), one antibiotic inactivation gene (ampH), and four antibiotic target alteration genes (pBP3, glpT, eF-Tu, and marR), which confer antibiotic resistance to beta-lactams, fluoroquinolones, aminoglycosides, and phosphonates (Table 5). The marA gene, whose transcriptional function regulates multidrug efflux and modulates membrane permeability, was found in all isolates. Aly et al. (2019) found msbA, emrR, H-NS, emrB, marA, CRP, and PBP3 to be associated with resistance to several antibiotics. Lepuschitz et al. (2019) found that out of 21 C. sakazakii isolates, 12 carried the efflux genes emrB, msbA, and patA; the antibiotic-efflux-modulating regulatory system genes CRP, marA, emrR, marR, and H-NS; the antibiotic target protection gene msrB; and the fosfomycin resistance determinant glpT. This aspect becomes particularly relevant in the context of increasing antibiotic resistance (Falagas et al., 2019) considering that fosfomycin is considered a useful antibiotic for patients with multidrug-resistant bacterial infections and since in our study the glpT gene was found in 100% of the isolates.
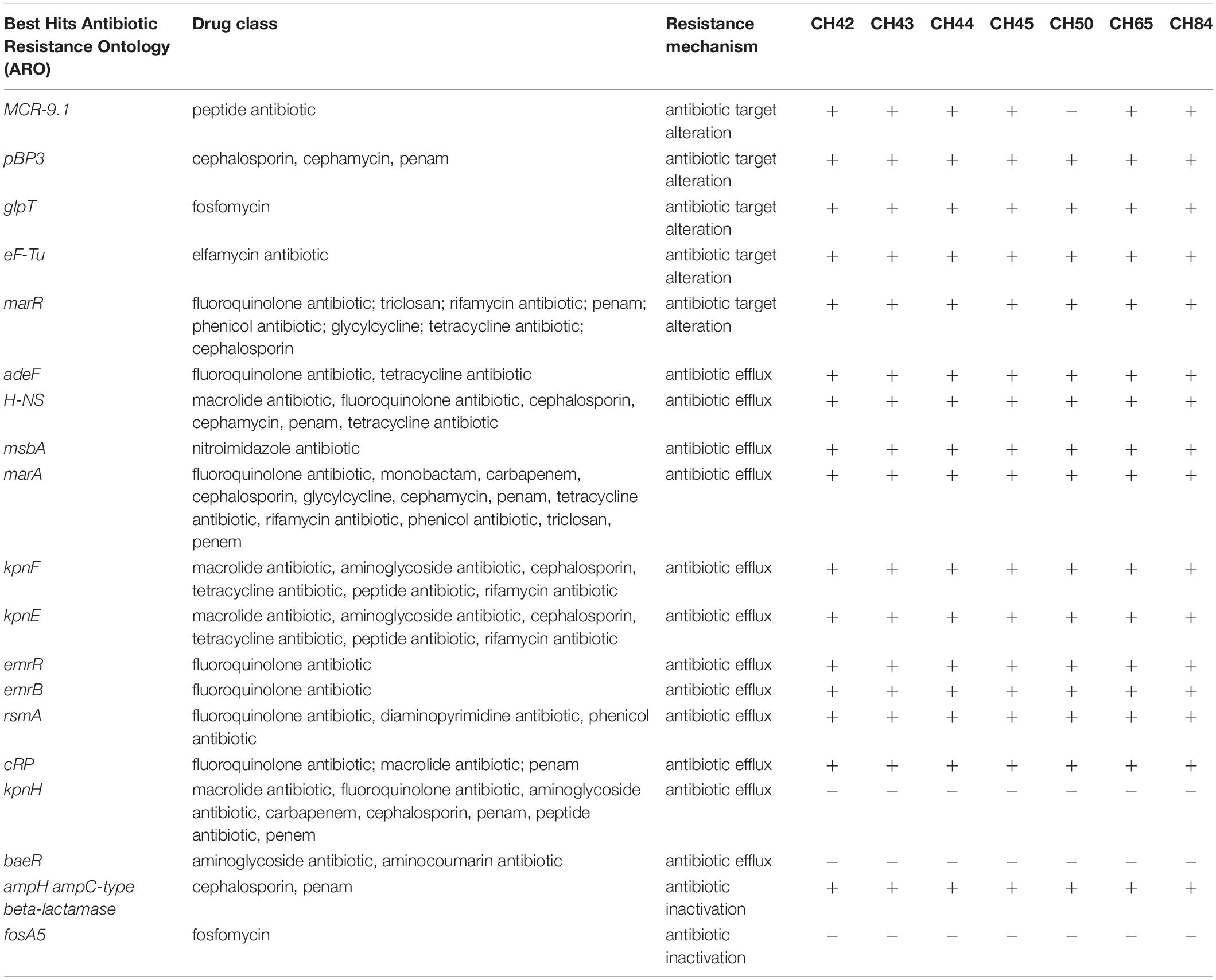
Table 5. Antibiotic-resistance genes identified by Comprehensive Antibiotic Resistance Database (CARD) of C. sakazakii strains.
Antibiotic overuse in food environments and the presence of several antibiotic resistance operons (marA) can favor the development of resistance to different antibiotics in Cronobacter spp. (Kim et al., 2008; Chon et al., 2012; Holý et al., 2021). In addition, we found the mcr-9.1 and blaCSA genes, conferring resistance to colistin and cephalothin, respectively. The mcr-9.1 gene is considered a plasmid-borne colistin resistance gene that can generate colistin resistance in various enteropathogens. These genes can silently circulate undetected unless induced by colistin (Carroll et al., 2019; Kieffer et al., 2019). The presence of mobile colistin-resistant (mcr) genes causes worldwide concern because colistin is considered the last resort for treating infections caused by multidrug-resistant Enterobacteriaceae (Borowiak et al., 2020). Müller et al. (2014) first described the β-lactamase class C resistance gene family blaCSA. Members of this family of β-lactamases are not inducible and are considered cephalosporinases. Jang et al. (2020) found class C bla resistance gene variants identified as CSA-2 or CSA-1. On the other hand, Holý et al. (2021) found blaCSA genes providing cephalothin resistance in all C. sakazakii strains isolated from powdered milk produced in the Czechia between 2010–2014.
All isolates studied here carried plasmids Col440I and Col (pHHAD28) and one isolate IncFII (pECLA), which are associated with antibiotic resistance genes (Ramsamy et al., 2020; Khezri et al., 2021). The presence of terC, from the plasmid-encoded tellurium resistance (ter) operon, is highly associated with infected patients compared to asymptomatic colonized patients. Furthermore, it is associated with pathogenesis of Klebsiella pneumoniae as a horizontally transferable factor that promotes robust intestinal colonization in the presence of the autochthonous microbiota (Vornhagen et al., 2020).
All C. sakazakii strains showed CRISPR arrays, and three strains had both I-E and I-F type arrays. CRISPR-Cas systems are related to the acquisition of horizontally acquired genetic material and have been recognized as an immunity system. These systems acquire information by means of viruses and plasmids. They consist of a guide RNA (gRNA) and a non-specific endonuclease associated with Cas-encoding genes. In the present study, when analyzing the genomes of the seven strains of C. sakazakii, we found that 100% (7/7) presented repeated sequences and spacers, forming arrays associated with CRISPR systems type I-F and I-E (Table 6). Regarding the CRISPR arrays, it was found that in system type I-E, there were five different consensus repeat sequences associated with it, of which the sequences GTGTTCCCCGCGCGAGCGGGGATAAACCG and CTGTTCCCCGCGCGAGCGGGGATAAACCG were the most frequent and were found in six of the seven strains in the study. In the case of the type I-F system, two different associated repeated sequences were found, of which the sequence GTTCACTGCCGTACAGGCAGCTTAGAAA was the most frequent and the sequence TTTCTAAGCTGCCTGTAC GGCAGTGAAC was characteristic of strains CH43 and CH84. Even though the sequences of each system may be the same, the numbers and lengths of repeated sequences and spacers differentiate them. With respect to the above, it was found that strains CH42, CH43, CH44, CH45, and CH84 presented the largest arrays, with up to a maximum of 30 repeat sequences and 29 spacers in the case of system I-E and 13 repeat sequences and 12 spacers in system I-E.
The relevance of studying the diversity of the CRISPR genes is that these systems can be used as a typing method of different microorganisms. Ogrodzki and Forsythe (2016), in analyzing 29 C. sakazakii ST1 genomes, found the same operon structure type I-E and three spacer arrays with conserved patterns. In addition, CRISPR spacer matrix profiles allow better intraspecies discrimination than MLST, so they have been proposed as a future tool for epidemiological studies of outbreaks by Cronobacter species (Makarova and Koonin, 2015). Although it was initially proposed that C. sakazakii had only one type of CRISPR system, Zeng et al. (2017) and Ogrodzki and Forsythe (2017) found that 94.5% of C. sakazakii strains had more than one CRISPR array, which were present in conserved areas of their genomes. The cas1 and cas2 genes are indispensable for the integration and processing of information acquired by the bacterium: In their absence, the system loses the ability to acquire information.
In our study, the use of WGS led to the reidentification of C. sakazakii and the determination of multiple virulence and antibiotic resistance genes in PIF and dairy products intended for consumption by infants. This situation should be analyzed in greater depth due to the growing international commercialization of powdered milk as a base product to manufacture other dairy products and byproducts. In Chile, legislation has just been passed regarding the obligation of producers of PIF and dairy products to disclose the origin of the powdered milk that is reused to prepare fluid milks and dairy by products. This requirement seeks to maintain an active record of information for authorities and supply visible information on the product’s retail label so consumers can make informed choices.
Considering that powdered milks are consumed by those who are susceptible to Cronobacter infection, the presence of virulence factors and antibiotic resistance in strains of C. sakazakii isolated from these products should be more closely monitored due to the direct relationship they have with the severity of the disease associated with this pathogen. Therefore, health authorities need to carry out more activities with preventive control measures for these foods along with campaigns to encourage the use of powdered milk rehydration water at 70°C, as indicated by the World Health Organization, which reports that this temperature has a proven effect of significantly decreasing the risk of C. sakazakii disease in already reconstituted milks (Parra-Flores et al., 2018b).
Conclusion
Cronobacter sakazakii strains isolated from powdered infant formula and powdered milk showed diverse virulence factors as well resistance to beta-lactam antibiotics in silico and in vitro. These findings reinforce the governmental decision to recall all involved powdered and dairy formulas in Chile in 2017. Continued surveillance of these products is necessary due to the risk associated with product contamination by C. sakazakii and consumption by the immunologically vulnerable infant population.
Data Availability Statement
The datasets presented in this study can be found in online repositories. The names of the repository/repositories and accession number(s) can be found below: https://pubmlst.org/organisms/cronobacter-spp/, 3195–3201.
Author Contributions
JP-F, OH, SL, AC-C, MT, and GF conceived the experiments and prepared the manuscript. JP-F, AC-C, SL, and MT conducted the laboratory work. JP-F, FR, EM-S, AR-F, JX-C, WR, and SF drafted the manuscript. All authors reviewed and approved the final manuscript.
Funding
This research was funded the Research Directorate of the Universidad del Bío-Bío, Projects 191520 4/R and GI 195420/EF.
Conflict of Interest
The authors declare that the research was conducted in the absence of any commercial or financial relationships that could be construed as a potential conflict of interest.
Acknowledgments
We wish to thank the Chilean Ministry of Health and the Research Directorate of the Universidad del Bío-Bío.
Supplementary Material
The Supplementary Material for this article can be found online at: https://www.frontiersin.org/articles/10.3389/fmicb.2021.694922/full#supplementary-material
Footnotes
- ^ http://pubmlst.org/Cronobacter
- ^ http://www.genomicepidemiology.org
- ^ https://crisprcas.i2bc.paris-saclay.fr
- ^ http://crispr.otago.ac.nz/CRISPRDetect/predict_crispr_array.html
References
Aldubyan, M., Almami, I., Benslimane, F., Alsonosi, A., and Forsythe, S. (2017). Comparative outer membrane protein analysis of high and low-invasive strains of Cronobacter malonaticus. Front. Microbiol. 8:2268. doi: 10.3389/fmicb.2017.02268
Aly, M. A., Domig, K. J., Kneifel, W., and Reimhult, E. (2019). Whole genome sequencing-based comparison of food isolates of Cronobacter sakazakii. Front. Microbiol. 10:1464. doi: 10.3389/fmicb.2019.01464
Baldwin, A., Loughlin, M., Caubilla-Barron, J., Kucerova, E., Manning, G., Dowson, C., et al. (2009). Multilocus sequence typing of Cronobacter sakazakii and Cronobacter malonaticus reveals stable clonal structures with clinical significance which do not correlate with biotypes. BMC Microbiol. 9:23. doi: 10.1186/1471-2180-9-223
Bankevich, A., Nurk, S., Antipov, D., Gurevich, A., Dvorkin, M., Kulikov, A., et al. (2012). SPAdes: a new genome assembly algorithm and its applications to single-cell sequencing. J. Comput. Biol. 19, 455–477.
Baumgartner, A., Grand, M., Liniger, M., and Iversen, C. (2009). Detection and frequency of Cronobacter spp. (Enterobacter sakazakii) in different categories of ready-to-eat foods other than infant formula. Int. J. Food Microbiol. 136, 159–154. doi: 10.1016/j.ijfoodmicro.2009.04.009
Biswas, A., Staals, R., Morales, S., Fineran, P., and Brown, C. (2016). CRISPRDetect: a flexible algorithm to define CRISPR arrays. BMC Genomics 17:356. doi: 10.1186/s12864-016-2627-0
Bolger, A., Lohse, M., and Usadel, B. (2014). Trimmomatic: a flexible trimmer for Illumina sequence data. Bioinformatics 30, 2114–2120. doi: 10.1093/bioinformatics/btu170
Borowiak, M., Baumann, B., Fischer, J., Thomas, K., Deneke, C., Hammerl, J. A., et al. (2020). Development of a novel mcr-6 to mcr-9 multiplex PCR and assessment of mcr-1 to mcr-9 occurrence in colistin-resistant Salmonella enterica isolates from environment, feed, animals and food (2011–2018) in Germany. Front. Microbiol. 11:80. doi: 10.3389/fmicb.2020.00080
Carattoli, A., Zankari, E., García-Fernández, A., Voldby Larsen, M., Lund, O., Villa, L., et al. (2014). In silico detection and typing of plasmids using plasmid finder and plasmid multilocus sequence typing. Antimicrob. Agents Chemother. 58, 3895–3903. doi: 10.1128/AAC.02412-14
Carroll, L., Gaballa, A., Guldimann, C., Sullivan, G., Henderson, L., and Wiedmann, M. (2019). Identification of novel mobilized colistin resistance gene mcr-9 in a multidrug-resistant, colistin-susceptible Salmonella enterica serotype Typhimurium isolate. mBio 10:e00853-19. doi: 10.1128/mBio.00853-19
Carter, L., Lindsey, L. A., Grim, C. J., Sathyamoorthy, V., Jarvis, K. G., Gopinath, G., et al. (2013). Multiplex PCR assay targeting a diguanylate cyclase-encoding gene, cgcA, to differentiate species within the genus Cronobacter. Appl. Environ. Microbiol. 79, 734–737. doi: 10.1128/AEM.02898-12
Caubilla-Barron, J., and Forsythe, S. (2007). Dry stress and survival time of Enterobacter sakazakii and other Enterobacteriaceae in dehydrated powdered infant formula. J. Food Prot. 70, 2111–2117. doi: 10.4315/0362-028X-70.9.2111
Chandrapala, D., Kim, K., Choi, Y., Senevirathne, A., Kang, D., Ryu, S., et al. (2014). Putative inv is essential for basolateral invasion of caco-2 cells and acts synergistically with ompA to affect in vitro and in vivo virulence of Cronobacter sakazakii ATCC 29544. Infect. Immun. 82, 1755–1765. doi: 10.1128/IAI.01397-13
Chase, H. R., Gopinath, G. R., Eshwar, A. K., Stoller, A., Fricker-Feer, C., Gangiredla, J., et al. (2017). Comparative genomic characterization of the highly persistent and potentially virulent Cronobacter sakazakii ST83, CC65 strain H322 and other ST83 strains. Front. Microbiol. 8:1136. doi: 10.3389/fmicb.2017.01136
Chon, J., Song, K., Kim, S., Hyeon, J., and Seo, K. (2012). Isolation and characterization of Cronobacter from desic-cated foods in Korea. J. Food Sci. 77, 354–358. doi: 10.1111/j.1750-3841.2012.02750.x
Clinical and Laboratory Standards Institute [CLSI] (2018). Performance Standards for Antimicrobial Disk Susceptibility Tests; Approved Standard, 13th Edn, Vol. 38. Wayne, PA: CLSI.
Couvin, D., Bernheim, A., Toffano-Nioche, C., Touchon, M., Michalik, J., Néron, B., et al. (2018). CRISPRCasFinder, an update of CRISRFinder, includes a portable version, enhanced performance and integrates search for Cas proteins. Nucleic Acids Res. 46, 246–251. doi: 10.1093/nar/gky425
Cruz, A., Xicohtencatl, J., Gonzalez, B., Bobadilla, M., Eslava, C., and Rosas, I. (2011). Virulence traits in Cronobacter species isolated from different sources. Can. J. Microbiol. 57, 735–744. doi: 10.1139/w11-063
Deter, H. S., Jensen, R. V., Mather, W. H., and Butzin, N. C. (2017). Mechanisms for differential protein production in toxin–antitoxin systems. Toxins 9:211. doi: 10.3390/toxins9070211
Dingle, T. C., Mulvey, G. L., and Armstrong, G. D. (2011). Mutagenic analysis of the Clostridium difficile Flagellar proteins, FliC and FliD, and their contribution to virulence in Hamsters. Infect. Immun. 79, 4061–4067. doi: 10.1128/iai.05305-11
Falagas, M., Athanasaki, F., Voulgaris, G., Triarides, N., and Vardakas, K. (2019). Resistance to fosfomycin: mechanisms, frequency and clinical consequences. Int. J. Antimicrob. Agents 53, 22–28. doi: 10.1016/j.ijantimicag.2018.09.013
Fei, P., Jiang, Y., Feng, J., Forsythe, S. J., Li, R., Zhou, Y., et al. (2017). Antibiotic and desiccation resistance of Cronobacter sakazakii and C. malonaticus isolates from powdered infant formula and processing environments. Front. Microbiol. 8:316. doi: 10.3389/fmicb.2017.00316
Fei, P., Man, C., Lou, B., Forsythe, S., Chai, Y., Li, R., et al. (2015). Genotyping and source tracking of the Cronobacter sakazakii and C. malonaticus isolated from powdered infant formula and an infant formula production factory in China. Appl. Environ. Microbiol. 81, 5430–5439. doi: 10.1128/AEM.01390-15
Finkelstein, S., Negrete, F., Jang, H., Gangiredla, J., Mammel, M., Patel, I. R., et al. (2019). Prevalence, distribution, and phylogeny of type two toxin-antitoxin genes possessed by Cronobacter species where C. sakazakii homologs follow sequence type lineages. Microorganisms 7:554. doi: 10.3390/microorganisms7110554
Flores, J. P., Arvizu, S., Silva, J., and Fernández, E. (2011). Two cases of hemorrhagic diarrhea caused by Cronobacter sakazakii in hospitalized nursing infants associated with the consumption of powdered infant formula. J. Food Prot. 74, 2177–2181. doi: 10.4315/0362-028X.JFP-11-257
Forsythe, S. J. (2018). Updates on the Cronobacter Genus. Annu. Rev. Food Sci. Technol. 25, 23–44. doi: 10.1146/annurev-food-030117-012246
Forsythe, S. J., Dickins, B., and Jolley, K. A. (2014). Cronobacter, the emergent bacterial pathogen Enterobacter sakazakii comes of age; MLST and whole genome sequence analysis. BMC Genomics 15:1121. doi: 10.1186/1471-2164-15-1121
Franco, A. A., Kothary, M., Gopinath, G., Jarvis, K., Grim, C. J., Hu, L., et al. (2011). Cpa, the outer membrane protease of Cronobacter sakazakii, activates plasminogen and mediates resistance to serum bactericidal activity. Infect. Immun. 79, 1578–1587. doi: 10.1128/IAI.01165-10
Hariri, S., Joseph, S., and Forsythe, S. J. (2013). Cronobacter sakazakii ST4 strains and neonatal meningitis, United States. Emerg. Infect. Dis. 19, 175–177. doi: 10.3201/eid1901.120649
Himelright, I., Harris, E., Lorch, V., and Anderson, M. (2002). Enterobacter sakazakii infections associated with the use of powdered infant formula – Tennessee, 2001. J. Am. Med. Assoc. 287, 2204–2205. doi: 10.1001/jama.287.17.2204
Hoeflinger, J. L., and Miller, M. J. (2017). Cronobacter sakazakii ATCC 29544 autoaggregation requires FliC flagellation, not motility. Front. Microbiol. 8:301. doi: 10.3389/fmicb.2017.00301
Holý, O., Cruz-Cordova, A., Xicohtencatl-Cortés, J., Hochel, I., Parra-Flores, J., Petrzelova, J., et al. (2019). Occurrence of virulence factors in Cronobacter sakazakii and Cronobacter malonaticus originated from clinical samples. Microb. Pathog. 127, 250–256. doi: 10.1016/j.micpath.2018.12.011
Holý, O., and Forsythe, S. J. (2014). Cronobacter species as emerging causes of healthcare-associated infection. J. Hosp. Infect. 86, 169–177. doi: 10.1016/j.jhin.2013.09.011
Holý, O., Parra-Flores, J., Lepuschitz, S., Alarcón-Lavín, M. P., Cruz-Córdova, A., Xicohtencatl-Cortes, J., et al. (2021). Molecular characterization of Cronobacter sakazakii strains isolated from powdered milk. Foods 10:20. doi: 10.3390/foods10010020
Hunter, C., and Bean, J. (2013). Cronobacter: an emerging opportunistic pathogen associated with neonatal meningitis, sepsis and necrotizing enterocolitis. J. Perinatol. 33, 581–585. doi: 10.1038/jp.2013.26
Iversen, C., Mullane, N., Mc Cardell, B., Tall, B. D., Lehner, A., Fanning, S., et al. (2008). Cronobacter gen. nov., a new genus to accommodate the biogroups of Enterobacter sakazakii, and proposal of Cronobacter sakazakii gen. nov. comb. nov., C. malonaticus sp. nov., C. turicensis sp. nov., C. muytjensii sp. nov., C. dublinensis sp. nov., Cronobacter genomospecies 1, and of three subspecies, C. dublinensis sp. nov. subsp. dublinensis subsp. nov., C. dublinensis sp. nov. subsp. lausannensis subsp. nov., and C. dublinensis sp. nov. subsp. lactaridi subsp. nov. Int. J. Syst. Evol. Microbiol. 58, 1442–1447. doi: 10.1099/ijs.0.65577-0
Jackson, E., and Forsythe, S. J. (2016). Comparative study of Cronobacter identification according to phenotyping methods. BMC Microbiol. 16:146. doi: 10.1186/s12866-016-0768-6
Jang, H., Chase, H. R., Gangiredla, J., Grim, C. J., Patel, I. R., Kothary, M. H., et al. (2020). Analysis of the molecular diversity among Cronobacter species isolated from filth flies using targeted PCR, pan genomic DNA microarray, and whole genome sequencing analyses. Front. Microbiol. 11:561204. doi: 10.3389/fmicb.2020.561204
Jia, B., Raphenya, A. R., Alcock, B., Waglechner, N., Guo, P., Tsang, K., et al. (2017). CARD 2017: expansion and model-centric curation of the comprehensive antibiotic resistance database. Nucleic Acids Res. 45, D566–D573. doi: 10.1093/nar/gkw1004
Johansson, M., Bortolaia, V., Tansirichaiya, S., Aarestrup, F., Roberts, A., and Petersen, T. (2021). Detection of mobile genetic elements associated with antibiotic resistance in Salmonella enterica using a newly developed web tool: MobileElementFinder. J. Antimicrob. Chemother. 76, 101–109. doi: 10.1093/jac/dkaa390
Joseph, S., Cetinkaya, E., Drahovska, H., Levican, A., Figueras, M., and Forsythe, S. (2012). Cronobacter condimenti sp. Nov., isolated from spiced meat, and Cronobacter universalis sp. nov., a species designation for Cronobacter sp. Geneomoespecies 1, recovered from a leg infection, water and food ingredients. Int. J. Syst. Evol. Microbiol. 62, 1277–1283. doi: 10.1099/ijs.0.032292-0
Joseph, S., and Forsythe, S. (2012). Insights into the emergent bacterial pathogen Cronobacter spp., generated by multilocus sequence typing and analysis. Front. Microbiol. 3:397. doi: 10.3389/fmicb.2012.00397
Khezri, A., Avershina, E., and Ahmad, R. (2021). Plasmid identification and plasmid-mediated antimicrobial gene detection in norwegian isolates. Microorganisms 9:52. doi: 10.3390/microorganisms9010052
Kieffer, N., Royer, G., Decousser, J. W., Bourrel, A. S., Palmieri, M., and Ortiz, et al. (2019). mcr-9, an inducible gene encoding an acquired phosphoethano-lamine transferase in Escherichia coli, and its origin. Antimicrob. Agents Chemother. 63:e00965-19. doi: 10.1128/AAC.00965-19
Kim, K., Jang, S., Kim, S., Park, J., Heu, S., and Ryu, S. (2008). Prevalence and genetic diversity of Enterobacter sakazakii in ingredients of infant foods. Int. J. Food Microbiol. 122, 196–203. doi: 10.1016/j.ijfoodmicro.2007.11.072
Kim, K., Kim, K., Choi, J., Lim-Jeong, A., Lee, J., Hwang, S., et al. (2010). Outer membrane proteins a (OmpA) and x (OmpX) are essential for basolateral invasion of Cronobacter sakazakii. Appl. Environ. Microbiol. 76, 5188–5198. doi: 10.1128/AEM.02498-09
Kleiman, M., Allen, S., Neal, P., and Reynolds, J. (1981). Meningoencephalitis and compartmentalization of the cerebral ventricles caused by Enterobacter sakazakii. J. Clin. Microbiol. 14, 352–354.
Lange, S., Alkhnbashi, O., Rose, D., Will, S., and Backofen, R. (2013). CRISPRmap: an automated classification of repeat conservation in prokaryotic adaptive immune systems. Nucleic Acids Res. 41, 8034–8044. doi: 10.1093/nar/gkt606
Lee, Y.-D., Park, J., and Chang, H. (2012). Detection, antibiotic susceptibility and biofilm formation of Cronobacter spp. from various foods in Korea. Food Control. 24, 225–230. doi: 10.1016/j.foodcont.2011.09.023
Lehner, A., Tall, B. D., Fanning, S., and Srikumar, S. (2018). Cronobacter spp.—opportunistic foodborne pathogens: an update on evolution, osmotic adaptation and pathogenesis. Curr. Clin. Micro Rpt. 5, 97–105. doi: 10.1007/s40588-018-0089-7
Lehner, A., Tasara, T., and Stephan, R. (2004). 16S rRNA gene based analysis of Enterobacter sakazakii strains from different sources and development of a PCR assay for identification. BMC Microbiol. 4:43–49. doi: 10.1186/1471-2180-4-43
Leopold, S., Goering, R., Witten, A., Harmsen, D., and Mellmann, A. (2014). Bacterial whole-genome sequencing revisited: portable, scalable, and standardized analysis for typing and detection of virulence and antibiotic resistance genes. J. Clin. Microbiol. 52, 2365–2370. doi: 10.1128/JCM.00262-14
Lepuschitz, S., Ruppitsch, W., Pekard-Amenitsch, S., Forsythe, S. J., Cormican, M., Mach, R. L., et al. (2019). Multicenter study of Cronobacter sakazakii infections in humans, Europe, 2017. Emerg. Infect. Dis. 25, 515–522. doi: 10.3201/eid2503.181652
Lepuschitz, S., Sorschag, S., Springer, B., Allerberger, F., and Ruppitsch, W. (2017). Draft genome sequence of carbapenemase-producing Serratia marcescens isolated from a patient with chronic obstructive pulmonary disease. Genome Announc. 5:e01288-17. doi: 10.1128/genomeA.01288-1
Ling, N., Jiang, Y., Zeng, H., Ding, Y., and Forsythe, S. (2021). Advances in our understanding and distribution of the Cronobacter genus in China. J. Food Sci. 86, 276–283. doi: 10.1111/1750-3841.15577
Makarova, K. S., and Koonin, E.V. (2015). “Annotation and classification of CRISPR-Cas systems,” in CRISPR. Methods in Molecular Biology, Vol. 1311, eds M. Lundgren, E. Charpentier and P. Fineran (New York, NY: Humana Press). doi: 10.1007/978-1-4939-2687-9_4
Mange, J. P., Stephan, R., Borel, L., Wild, P., Kim, K. S., Pospischil, A., et al. (2006). Adhesive properties of Enterobacter sakazakii to human epithelial and brain microvascular endothelial cells. BMC Microbiol. 6:58–68. doi: 10.1186/1471-2180-6-58
Mare, A., Man, A., Toma, F., Ciurea, C. N., Coşeriu, R. L., Vintilă, C., et al. (2020). Hemolysin-producing strains among diarrheagenic Escherichia coli isolated from children under 2 years old with diarrheal disease. Pathogens 9:1022. doi: 10.3390/pathogens9121022
Mazzantini, D., Fonnesu, R., Celandroni, F., Calvigioni, M., Vecchione, A., Mrusek, D., et al. (2020). GTP-dependent FlhF homodimer supports secretion of a hemolysin in Bacillus cereus. Front. Microbiol. 11:879. doi: 10.3389/fmicb.2020.00879
Mohan-Nair, M. K., and Venkitanarayanan, K. S. (2006). Cloning and sequencing of the ompA gene of Enterobacter sakazakii and development of an ompA-targeted PCR for rapid detection of Enterobacter sakazakii in infant formula. Appl. Environ. Microbiol. 72:2539. doi: 10.1128/AEM.72.4.2539-2546.2006
Molloy, C., Cagney, C., O’Brien, S., Iversen, C., Fanning, S., and Duffy, G. (2009). Surveillance and characterization by pulsed-field gel electrophoresis of Cronobacter spp in farming and domestic environments, food production animals and retails foods. Int. J. Food Microbiol. 136, 198–238. doi: 10.1016/j.ijfoodmicro.2009.07.007
Morato-Rodríguez, M., Velandia-Rodríguez, D., Castañeda, S., Crosby, M., and Vera, H. (2018). Cronobacter spp. in common breast milk substitutes, Bogotá, Colombia. Emerg. Infect. Dis. 24, 1907–1909. doi: 10.3201/eid2410.172021
Müller, A., Hächler, H., Stephan, R., and Lehner, A. (2014). Presence of AmpC beta-lactamases, CSA-1, CSA-2, CMA-1, and CMA-2 Conferring an unusual resistance phenotype in Cronobacter sakazakii and Cronobacter malonaticus. Microb. Drug Resist. 20, 275–280. doi: 10.1089/mdr.2013.0188
Ogrodzki, P., and Forsythe, S. (2015). Capsular profiling of the Cronobacter genus and the association of specific Cronobacter sakazakii and C. malonaticus capsule types with neonatal meningitis and necrotizing enterocolitis. BMC Genomics 16:758. doi: 10.1186/s12864-015-1960-z
Ogrodzki, P., and Forsythe, S. (2016). CRISPR–cas loci profiling of Cronobacter sakazakii pathovars. Fut. Microbiol. 11, 1507–1519. doi: 10.2217/fmb-2016-0070
Ogrodzki, P., and Forsythe, S. (2017). DNA-sequence based typing of the Cronobacter genus using MLST, CRISPR-cas array and capsular profiling. Front. Microbiol. 8:1875. doi: 10.3389/fmicb.2017.01875
Parra, J., Oliveras, L., Rodriguez, A., Riffo, F., Jackson, E., and Forsythe, S. (2015). Riesgo por Cronobacter sakazakii en leches en polvo para la nutrición de lactantes. Rev. Chil. Nut. 42, 83–89. doi: 10.4067/S0717-75182015000100011
Parra-Flores, J., Aguirre, J., Juneja, V., Jackson, E., Cruz-Córdova, A., Silva-Sanchez, J., et al. (2018a). Virulence and antibiotic resistance profiles of Cronobacter sakazakii and Enterobacter spp. involved in the diarrheic hemorrhagic outbreak in Mexico. Front. Microbiol. 9:2206. doi: 10.3389/fmicb.2018.02206
Parra-Flores, J., Cerda-Leal, F., Contreras, A., Valenzuela-Riffo, N., Rodriguez, A., and Aguirre, J. (2018b). Cronobacter sakazakii and microbiological parameters in dairy formulas associated with a food alert in Chile. Front. Microbiol. 9:1708. doi: 10.3389/fmicb.2018.01708
Parra-Flores, J., Cruz-Córdova, A., Acuña, S., Riffo-Sepúlveda, F., Maury-Sintjago, E., Rodriguez-Fernández, A., et al. (2021). “Cronobacter spp. in milk,” in Reference Module in Food Science, (Amsterdam: Elsevier), 1–9.
Parra-Flores, J., Maury-Sintjago, E., Rodriguez-Fernández, A., Acuña, S., Cerda, F., Aguirre, J., et al. (2020). Microbiological quality of powdered infant formula in Latin America. J. Food Prot. 83, 534–541. doi: 10.4315/0362-028X.JFP-19-399
Patrick, M., Mahon, B., Greene, S., Rounds, J., Conquist, A., Wymore, K., et al. (2014). Incidence of Cronobacter spp. infections, United States, 2003–2009. Emerg. Infect. Dis. 20, 1520–1523. doi: 10.3201/eid2009.140545
Proudy, I., Bouglé, D., Coton, E., Coton, M., Leclercq, R., and Vergnaud, M. (2008). Genotypic characterization of Enterobacter sakazakii isolates by PFGE, BOX-PCR and sequencing of the fliC gene. J. Appl. Microbiol. 104, 26–34. doi: 10.1111/j.1365-2672.2007.03526.x
Ramsamy, Y., Mlisana, K. P., Allam, M., Amoako, D. G., Abia, A. L. K., Ismail, A., et al. (2020). Genomic analysis of carbapenemase-producing extensively drug-resistant Klebsiella pneumoniae isolates reveals the horizontal spread of p18-43_01 plasmid encoding blaNDM-1 in South Africa. Microorganisms 8:137. doi: 10.3390/microorganisms8010137
Severi, E., Hood, D. W., and Thomas, G. H. (2007). Sialic acid utilization by bacterial pathogens. Microbiology 153, 2817–2822. doi: 10.1099/mic.0.2007/009480-0
Shi, L., Liang, Q., Zhan, Z., Feng, J., Zhao, Y., and Chen, et al. (2018). Co-occurrence of 3 different resistance plasmids in a multi-drug resistant Cronobacter sakazakii isolate causing neonatal infections. Virulence 9, 110–120. doi: 10.1080/21505594.2017.1356537
Sohanpal, B. K., Friar, S., Roobol, J., Plumbridge, J. A., and Blomfield, I. C. (2007). Multiple co-regulatory elements and IHF are necessary for the control of fimB expression in response to sialic acid and N-acetylglucosamine in Escherichia coli K−12. Mol. Microbiol. 63, 1223–1236. doi: 10.1111/j.1365-2958.2006.05583.x
Sonbol, H., Joseph, S., McAuley, C., Craven, H., and Forsythe, S. (2013). Multilocus sequence typing of Cronobacter spp. from powdered infant formula and milk powder production factories. Int. Dairy J. 30, 1–7. doi: 10.1016/j.idairyj.2012.11.004
Stephan, R., Grim, C., Gopinath, G., Mammel, M., Sathyamoorthy, V., Trach, L., et al. (2014). Re-examination of the taxonomic status of Enterobacter helveticus, Enterobacter pulveris and Enterobacter turicensis as members of the genus Cronobacter and their reclassification in the genera Franconibacter gen. nov. and Siccibacter gen. nov. as Franconibacter helveticus comb. nov., Franconibacter pulveris comb. nov. and Siccibacter turicensis comb. nov., respectively. Int. J. Syst. Evol. Microbiol. 10, 3402–3410. doi: 10.1099/ijs.0.059832-0
Stoop, B., Lenher, A., Iversen, C., and Fanning, S. (2009). Development and evaluation of rpoB based PCR systems to differentiate the six proposed species within the genus Cronobacter. Int. J. Food Microbiol. 136, 165–168. doi: 10.1016/j.ijfoodmicro.2009.04.023
Townsend, S., Hurrell, E., Gonzalez-Gomez, I., Lowe, J., Frye, J., Forsythe, S., et al. (2007). Enterobacter sakazakii invades brain capillary endothelial cells, persists in human macrophages influencing cytokine secretion and induces severe brain pathology in the neonatal rat. Microbiology 153, 3538–3547. doi: 10.1099/mic.0.2007/009316-0
Veronica, E.-K., Sara, A. O., Everardo, C. Q., Héctor, Q., Oscar, M. C., Elizabeth, F. R., et al. (2020). Proteomics profiles of Cronobacter sakazakii and a fliF mutant: adherence and invasion in mouse neuroblastoma cells. Microb. Pathog. 149:104595. doi: 10.1016/j.micpath.2020.104595
Vojkovska, H., Karpiskova, R., Orieskova, M., and Drahovska, H. (2016). Characterization of Cronobacter spp. isolated from food of plant origin and environmental samples collected from farms and from supermarkets in the Czech Republic. Int. J. Food Microbiol. 217, 130–136. doi: 10.1016/j.ijfoodmicro.2015.10.017
Vornhagen, J., Bassis, C., Ramakrishnan, S., Hein, R., Mason, S., Bergman, Y., et al. (2020). A plasmid locus associated with Klebsiella clinical infections encodes a microbiome-dependent gut fitness factor. bioRxiv 2:963322. doi: 10.1101/2020.02.26.963322
Walling, L. R., and Butler, J. S. (2019). Toxins targeting transfer RNAs: translation inhibition by bacterial toxin–antitoxin systems. Wiley Interdiscip. Rev. RNA 10:e1506. doi: 10.1002/wrna.1506
Ye, Y. W., Ling, N., Jiao, R., Wu, Q. G., Han, Y. J., and Gao, J. N. (2015). Effects of culture conditions on the biofilm formation of Cronobacter sakazakii strains and distribution of genes involved in biofilm formation. LWT-Food Sci. Technol. 62, 1–6. doi: 10.1016/j.lwt.2015.01.035
Zeng, H., Lei, T., He, W., Zhang, J., Liang, B., Li, C., et al. (2018). Novel multidrug resistant Cronobacter sakazakii causing meningitis in neonate, China, 2015. Emerg. Infect. Dis. 24, 2121–2124. doi: 10.3201/eid2411.180718
Keywords: Cronobacter sakazakii, powdered formula, virulence, antibiotic resistance genes, CRISPR-cas
Citation: Parra-Flores J, Holý O, Riffo F, Lepuschitz S, Maury-Sintjago E, Rodríguez-Fernández A, Cruz-Córdova A, Xicohtencatl-Cortes J, Mancilla-Rojano J, Troncoso M, Figueroa G, Ruppitsch W and Forsythe S (2021) Profiling the Virulence and Antibiotic Resistance Genes of Cronobacter sakazakii Strains Isolated From Powdered and Dairy Formulas by Whole-Genome Sequencing. Front. Microbiol. 12:694922. doi: 10.3389/fmicb.2021.694922
Received: 14 April 2021; Accepted: 03 June 2021;
Published: 30 June 2021.
Edited by:
Fabian Horn, Federal Office of Consumer Protection and Food Safety (BVL), GermanyReviewed by:
Hyein Jang, United States Food and Drug Administration, United StatesPeng Fei, Henan University of Science and Technology, China
Copyright © 2021 Parra-Flores, Holý, Riffo, Lepuschitz, Maury-Sintjago, Rodríguez-Fernández, Cruz-Córdova, Xicohtencatl-Cortes, Mancilla-Rojano, Troncoso, Figueroa, Ruppitsch and Forsythe. This is an open-access article distributed under the terms of the Creative Commons Attribution License (CC BY). The use, distribution or reproduction in other forums is permitted, provided the original author(s) and the copyright owner(s) are credited and that the original publication in this journal is cited, in accordance with accepted academic practice. No use, distribution or reproduction is permitted which does not comply with these terms.
*Correspondence: Julio Parra-Flores, juparra@ubiobio.cl
†These authors have contributed equally to this work