- 1NHC Key Laboratory of Food Safety Risk Assessment, China National Center for Food Safety Risk Assessment, Beijing, China
- 2UCD-Centre for Food Safety, School of Public Health, Physiotherapy and Sports Science, University College Dublin, Dublin, Ireland
Listeria innocua are Gram-positive rod-shaped bacteria, which are not generally infectious as opposed to Listeria monocytogenes. However, the comparatively high genomic similarity between both along with on occasion, their coexistence in similar ecological niches may present the opportunity for resistance or virulence gene transfer. In this study, three multi-drug resistant L. innocua originally cultured from food were put forward for long-read genome sequencing. Chromosome and plasmid genomes were assembled and annotated. Analysis demonstrated that the resistant phenotypes correlated well with genotypes. Three plasmids pLI42, pLI203, and pLI47-1 were identified which harbor resistance islands. Sequence alignments suggested that plasmids pLI42 and pLI203 were highly similar to a previously sequenced L. monocytogenes plasmid pLR1. Similarly, another three types of resistance gene islands were observed on chromosome, including tet(M) gene islands (transposon Tn916 orthologs), dfrG gene islands and optrA-erm(A) gene islands. All three L. innocua isolates possessed listeria pathogenicity island-4 (LIPI-4) which is linked to cases of mengitis. Further genome environment and phylogenic analysis of regions flanking LIPI-4 of L. innocua and L. monocytogenes showed that these may have common origins and with the potential to transmit from the former. Our findings raise the possible need to include both L. monocytogenes and L. innocua in food surveillance programs so as to further understand of the origins of antimicrobial resistance and virulence markers of public health importance in L. monocytogenes.
Introduction
Listeria species are Gram-positive and facultative anaerobic bacteria that exist in soil, water and the animal gut. Members of this genus are found to contaminate certain types of foods and the associated food processing environment thereby representing a risk for public health (Finlay, 2001). Nineteen species of Listeria have been reported (Orsi and Wiedmann, 2016). Among them, L. monocytogenes is considered as the only one that can cause listeriosis both in humans and animals (Vivant et al., 2013). Previously, Listeria innocua was generally considered as a non-virulent species with a closer evolutionary relationship than other members of the genus to L. monocytogenes (Buchrieser et al., 2003).
To better describe the genomic evolution and potential for horizontal gene transfer (HGT) between L. monocytogenes and L. innocua, the findings of comparative genomics analyses were reported in previous studies (Buchrieser et al., 2003; Hain et al., 2006). These data highlighted the close genetic relationship existing between L. monocytogenes and L. innocua. Phylogenetic studies using amplicons or Listeria house-keeping genes provided evidence that L. innocua and L. monocytogenes are indeed related genetically (Glaser et al., 2001b; Orsi and Wiedmann, 2016). The orthologous genes identified between both species are highly conserved. Further, data indicated that L. innocua may have evolved through gene elimination and acquisition from the same pathogenic ancestor of L. monocytogenes (Chen et al., 2009).
Although few in number, early studies described plasmid-mediated AMR and their transmission in L. innocua (Bertsch et al., 2014; Gomez et al., 2014; Escolar et al., 2017). Based on recent sequencing data all L. monocytogenes possessed listeria pathogenicity island-1 (LIPI-1) and inlAB (Reddy and Lawrence, 2014). Hypervirulent isolates of L. monocytogenes harbor LIPI-3 and LIPI-4 (Maury et al., 2016), in which LIPI-3 encodes listeriolysin S, a second hemolysin that enhances the survival of L. monocytogenes in polymorphonuclear neutrophils (PMN) while LIPI-4 encodes a cellobiose-family phosphotransferase system (PTS) (Cotter et al., 2008), that enhances invasion of the central nervous system (CNS) along with maternal-neonatal infection (MN). Most L. innocua isolates lack LIPI-1 and several important virulence genes including inlA and inlB, while other data reported on atypical L. innocua that harbored LIPI-1 or LIPI-3 (Volokhov et al., 2007; Clayton et al., 2014; Moura et al., 2019). Unlike other LIPIs, LIPI-4 orthologous has been reported to be found in many L. innocua isolates (Moura et al., 2019). Furthermore, few examples of HGT involving resistance and virulence genes between these two species have been reported (Bertsch et al., 2014).
In this study, we describe three MDR L. innocua LI42, LI47, and LI203, isolated from food samples in China. In order to extend our understanding of the genetic relationships and antibiotic resistance and virulence, all three were sequenced and compared with the closely related L. innocua and L. monocytogenes reference genomes, including two resistant L. monocytogenes in our previous study (Yan et al., 2019).
Materials and Methods
Bacterial Isolates
Three L. innocua isolates were isolated from food in China from year 2015 to 2016. The source information was listed in Supplementary Table 1. All isolates were confirmed by API listeria (Suarez et al., 2001).
Antibiotics Susceptibility Testing (AST)
All isolates were tested for antimicrobial susceptibility using broth microdilution against a panel of nine antimicrobial compounds commonly used in veterinary and human therapy and these data were interpreted according to the guidelines of the Clinical and Laboratory Standards Institute (CLSI) M45 (3rd edition) (Clsi Institute, 2015), where appropriate. Drugs tested included ampicillin (AMP), chloramphenicol (CHL), ciprofloxacin (CIP), erythromycin (ERY), gentamicin (GEN), meropenem (MEM), trimethoprim-sulfamethoxazole (SXT), tetracycline (TET), and vancomycin (VAN). All antibiotics were purchased from Sigma-Aldrich, Germany.
DNA Purification and Sequencing
Each isolate was grown in brain heart infusion (BHI) broth (Beijing Land Bridge) at 37°C and genomic DNA (gDNA) was purified using Omega EZNA® Bacterial DNA Kit (Omega Bio-tek, Norcross, GA, United States). The bacterial genomes were sequenced by Tianjin Biochip Corporation, using a PacBio RS II platform (Pacific Biosciences, Menlo Park, CA, United States). The sequencing depth is 1000X. De novo assembly was performed by SMRT Link (V6.0.0.47841).
Annotation of Genomes and AMR Genes
The chromosomes and plasmids of three L. innocua were annotated with the prokaryotic genome annotation tool Prokka (v1.12). Antibiotic resistance genes were extracted from these genome sequences using the ABRicate1 software package, where a combination of three reference databases CARD (Jia et al., 2017), ResFinder (Zankari et al., 2012), and NCBI AMRFinderPlus (Feldgarden et al., 2019) were used. Gene names were unified to the NCBI AMRFinderPlus references. All resistance genes were screened using the BLASTN algorithm with minimum nucleotide identity and alignment length coverage of 80%.
Assessment of Virulence Factors
The presence and integrity of virulence factors was assessed using L. monocytogenes EGD-e (NC_003210) as the reference genome for Internalin A (InlA), Internalin B (InlB), listeria pathogenicity island 1 (LIPI-1) (Glaser et al., 2001b; Toledo-Arana et al., 2009). L. monocytogenes F2365 (NC_002973) was used as reference genome for LIPI-3 with the protein sequences (LMOf2365_1113 to LMOf2365_1119) (Nelson et al., 2004). L. monocytogenes LM9005581 (CYWW00000000) was used as reference for LIPI-4 with the protein sequences (LM9005581_70009 to LM9005581_70014). Analysis was performed using the BLASTN algorithm with a minimum identity of 80%, coverage of 80%.
Genomic Comparison
Sequence comparison was executed between chromosomes or plasmids on average nucleotide identity (ANI) based on BLASTN alignment, using pyani (v0.2.7). ANIb values of each pair of samples was calculated and classified into two groups via species. A one-tailed student t-test was performed. A circular genome comparison graph was performed with BRIG (v0.95). Sequence comparisons were done using BLASTN and visualized using EasyFig (v2.2.3).
Core Genome Alignment and Phylogenetic Tree
Core genomes of all assemblies were calculated using Roary (v3.11.0). The core genomes were aligned with MAFFT (v7.313). Maximum Likelihood phylogenetic tree of the aligned genomes was performed using FastTree (v2.1.10). The phylogenetic tree was illustrated by adjusting the mid-point as root.
Sequence Data Accession Numbers
Accession numbers for complete genome sequences are SAMN18079989 (LI42), SAMN18080006 (LI47), and SAMN18080009 (LI203).
Conjugation Experiments
Conjugation experiments were performed using L. innocua LI42, LI47, and LI203 as the donors, L. monocytogenes ATCC 19115, L. monocytogenes ST9 isolate and E. coli J53 (NaN3 resistant) as recipients. For the selection of the transconjugants between L. innocua and L. monocytogenes, blood agar plate was supplemented with 4 mg/L tetracycline, and the colonies were separated by hemolysis test. For the selection of the transconjugants between L. innocua and E. coli J53, MacConkey Agar (MAC) plate was supplemented with 4 mg/L tetracycline and 100 mg/L NaN3. The Colonies grew on these selective plates were further confirmed by PCR amplification of hly and tet(S) genes for listeria spp., and tet(S) for E. coli J53.
Results
Pheno- and Genotypic Characterization of Three L. innocua Isolates
Listeria innocua LI42, LI47, and LI203 were found to express the same MDR phenotype resistant to chloramphenicol, erythromycin, tetracycline and trimethoprim-sulfamethoxazole as shown in Figure 1, albeit somewhat different minimum inhibitory concentration (MIC) values. AMR genotypes corresponded well with the phenotypes described by AST analysis, where the three MDR isolates harbored resistance genes, respectively, for aminoglycoside, macrolide-lincosamide-streptogramin B (MLSb), phenicol, tetracycline, and sulfamethoxazole resistance. Complete LIPI-4 orthologs were found in all three L. innocua isolates.
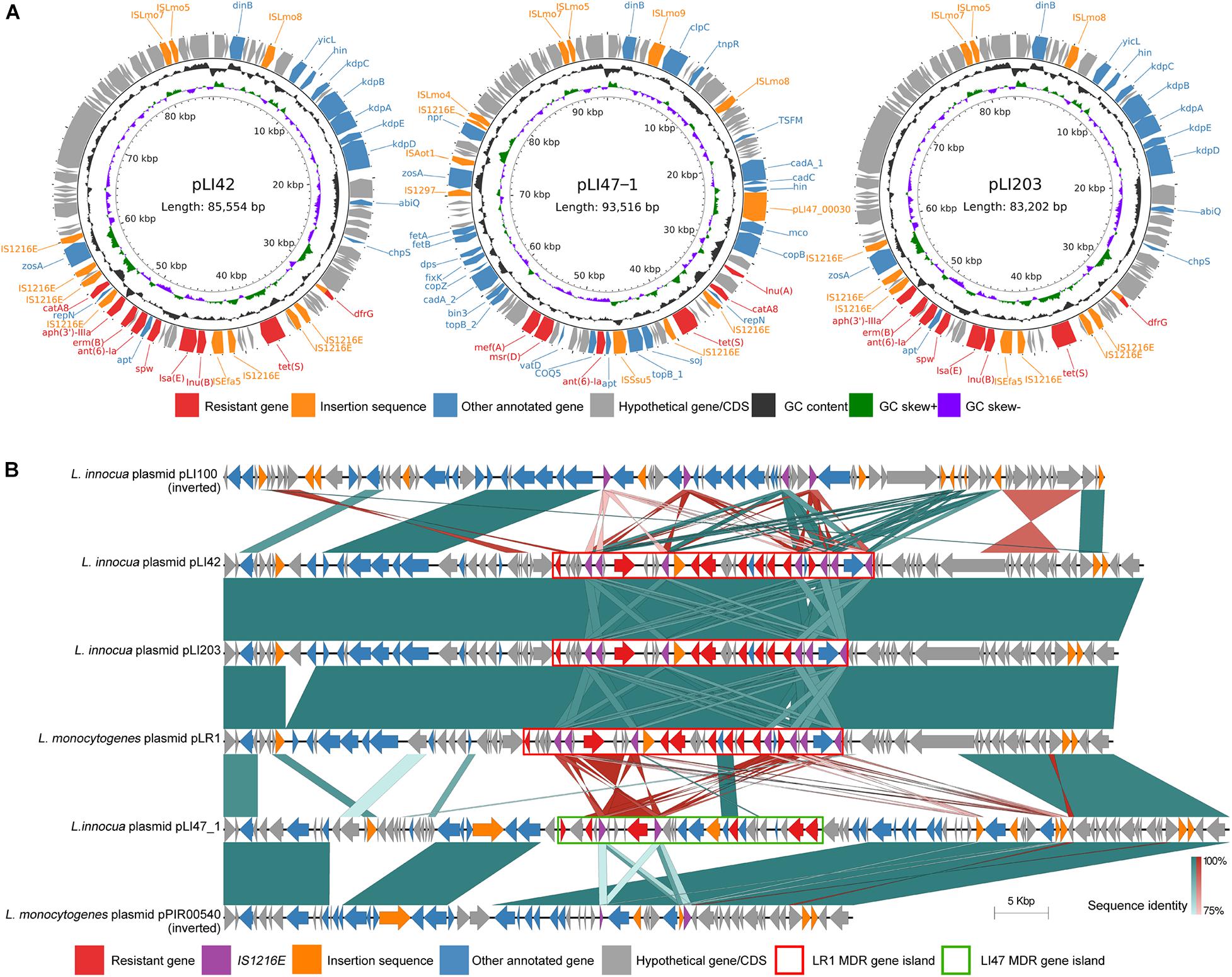
Figure 1. Circular schematic map and linear sequence comparison of three plasmids carrying antibiotic resistance genes. (A) The sequence structure of three sequenced L. innocua plasmid pLI42, pLI47-1, and PLI203. CDSs were shown as arrows. Antibiotic resistance genes were highlighted as red. Insertion sequences were highlighted by orange. Other annotated genes were denoted by blue. The inner two rings represented GC Skew[(G – C)/(G + C), G and C represents guanine content cytosine content] and GC content. (B) Comparison of three L. innocua plasmid pLI47-1, pLI203, and PLI42 sequences with a closely related reference L. innocua plasmid pLI100 and two L. monocytogenes reference plasmid pLR1 and pPIR00540. CDSs were shown as arrows and colored based on gene functions. Copies of insertion sequence IS1216E was specially highlighted as purple. Green or red shades between each two sequences represented direct or inverted nucleotide identity between covered regions with at least 75% identity. Reference plasmids pLI100 and pPIR00540 sequences were reversed to be aligned with other sequences. L. monocytogenes LR1 MDR gene island and the orthologs were marked in red frames. L. innocua LI47 MDR gene island was marked in green frame.
Genome Wide Characterization of Three Listeria innocua Genomes
As shown in Table 1, long-read sequencing of L. innocua LI42, LI47, LI203 facilitated the construction of the complete genome sequence in each case including their chromosomes and plasmids. Listeria innocua LI42 and LI203 genomes each contain one chromosome and one plasmid (pLI42 or pLI203), while L. innocua LI47 contained a chromosome and two plasmids (pLI47-1 and pLI47-2).
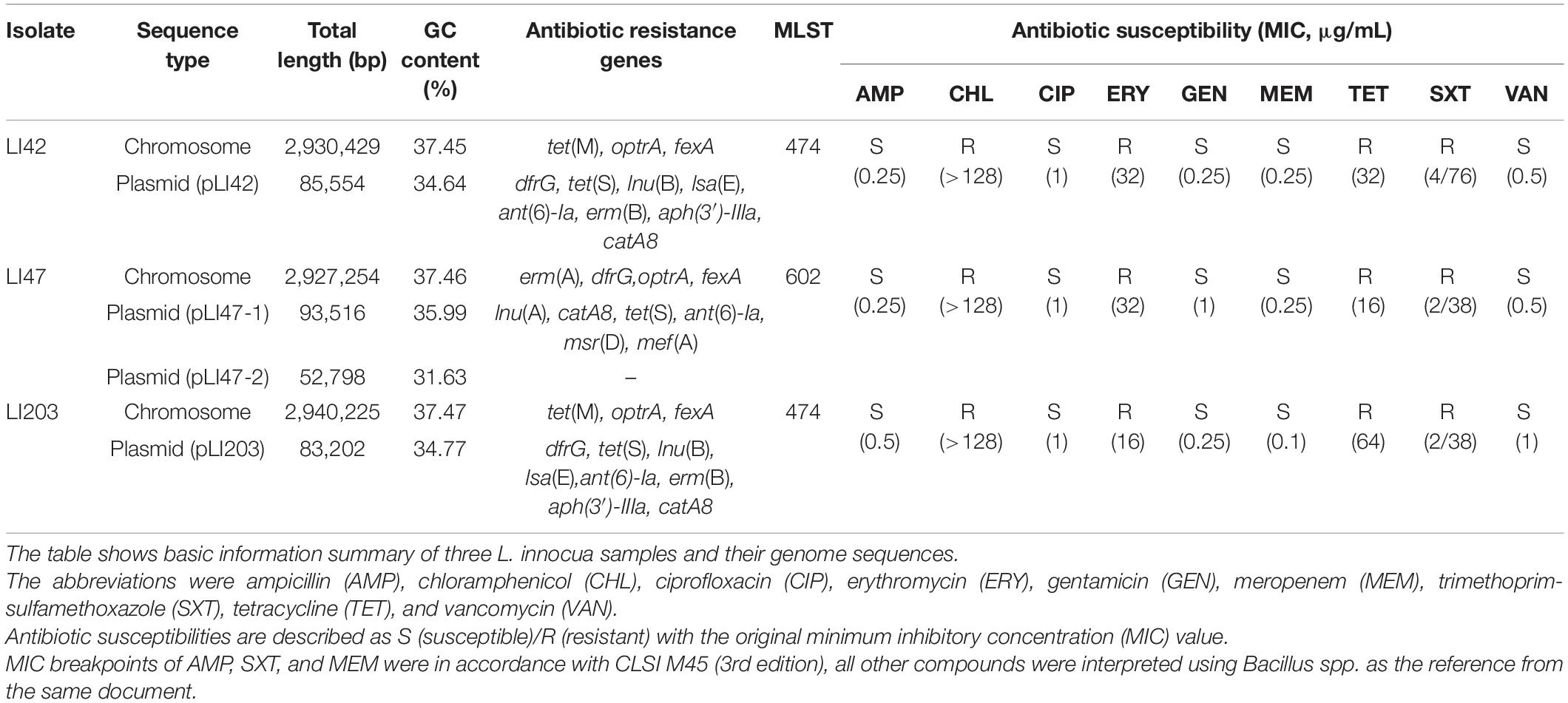
Table 1. Summary for complete genome sequencing and antimicrobial resistance pheno- and genotypes of three L. innocua isolates.
Comparative Genomic Analysis of Three Listeria innocua Plasmids
Annotation of resistance genes showed that plasmid pLI42, pLI47-1, and pLI203 carried multiple antibiotic resistance genes including ant(6)-Ia, aph(3′)-IIIa, catA8, dfrG, erm(B), lnu(A), lnu(B), lsa(E), msr(D), mef(A), spw and tet(S), associating resistances of amikacin, aminoglycoside, chloramphenicol, kanamycin, lincosamide, macrolide, tetracycline, trimethoprim, streptogramin, and streptomycin. Multiple copies of insertion sequences were also noted (Figure 1A).
Our previous study reporting on L. monocytogenes from foods in China described a multidrug resistant (MDR) gene island dfrG-tet(S)-lnu(B)-lsa(E)-spw-ant(6)_Ia-erm(B)-aphA-catA8 from L. monocytogenes LR1 (SAMN10434273) (Yan et al., 2019). The recent long-read re-sequencing of this strain confirmed that this gene island is located on a plasmid, denoted as plasmid pLR1. Average nucleotide identities between each pair of plasmids pLI42, pLI203 and L. monocytogenes MDR plasmid pLR1 sequences showed >95.0% coverages and >99.9% identities between each two sequences, demonstrating that plasmids pLI42 and pLI203 are close orthologs of plasmid pLR1.
Linear sequence comparison was performed involving plasmids pLI42, pLI47-1, and pLI203 along with three plasmids pLI100 (L. innocua) from L. innocua Clip11262 (Glaser et al., 2001a), pLR1 (L. monocytogenes), and pPIR00540 (L. monocytogenes) as the closest common hits of online BLAST result of the three plasmids against NCBI nt/nr database (Portmann et al., 2018), which clearly displayed the ortholog regions (Figure 1B). Although plasmid pLI203 lacked the chloramphenicol resistance gene catA8, plasmids pLI42, pLI203, and pLR1 shared a similar overall genetic backbone covering the previously described LR1 MDR gene island. Listeria innocua LI47 MDR gene island mapped to plasmid pLI47-1 demonstrated a unique resistance gene arrangement lnuA-catA8-tet(S)-ant(6)Ia-msr(D)-mef(A). The conserved flanking region of L. innocua LI47 MDR gene island on plasmid pLI47-1 was identical to plasmid pPIR00540.
Insertion sequence (IS) elements IS3, IS6, IS21, IS1595, IS1380, ISLre2, and Tn3 were also annotated, along with the resistance gene dfrG, which is also known as transposes ISSsu9 (Holden et al., 2009). Multiple copies of IS1216E were noted on these plasmids. Each of plasmids pLI42, pLI203, and pLR1 had six direct repeats of IS1216E. Plasmids pLI47-1 and pPIR00540 contain two direct repeats, while three direct repeats and a single inverted repeat are found in plasmid pLI100.
Comparative Genomic Analysis of Resistance Gene Islands Located on Chromosomes
Listeria innocua LI42, LI47, and LI203 chromosomes were discovered harboring three different types of resistance gene island including tet(M) gene island (∼10,925 bp, found on the LI42 and LI203 chromosomes, associated with tetracycline resistance), dfrG gene island (∼3,310 bp, mapped on the LI47 chromosome, and associated with trimethoprim resistance) and optrA-erm(A) gene island (∼18,861 bp, integrally found on the LI47 chromosome, partially found on LI42 and LI203 chromosome, associated with macrolide, florfenicol and oxazolidinone resistance).
Nucleotide comparison confirmed that the tet(M) and dfrG gene-containing islands are highly identical (>99%) with their orthologs in L. monocytogenes LR8 (SAMN10434278), a feature reported in our earlier study (Yan et al., 2019). Specifically, tet(M) is an identical ortholog of transposon Tn916, which is found among several different bacteria (Kathariou et al., 1987; Bertsch et al., 2013), while the dfrG gene is known to exist as an independent antibiotic resistance gene and also associated with an insertion sequence element IS1595. The insert locations for the tet(M) gene-containing island in L. innocua LI42 and LI203 chromosomes differ from that of L. monocytogenes LR8 (as shown in Figure 2A). Meanwhile, the dfrG gene-containing island also mapped to plasmids pLI42 and pLR1, suggesting horizontal movement between both plasmids and chromosomes. The latter contains a pair of short direct repeats (∼63 bp) flanking both ends, and module inserts reversibly at the corresponding location on the chromosomes of L. innocua LR8 and LI42 (Figure 2B).
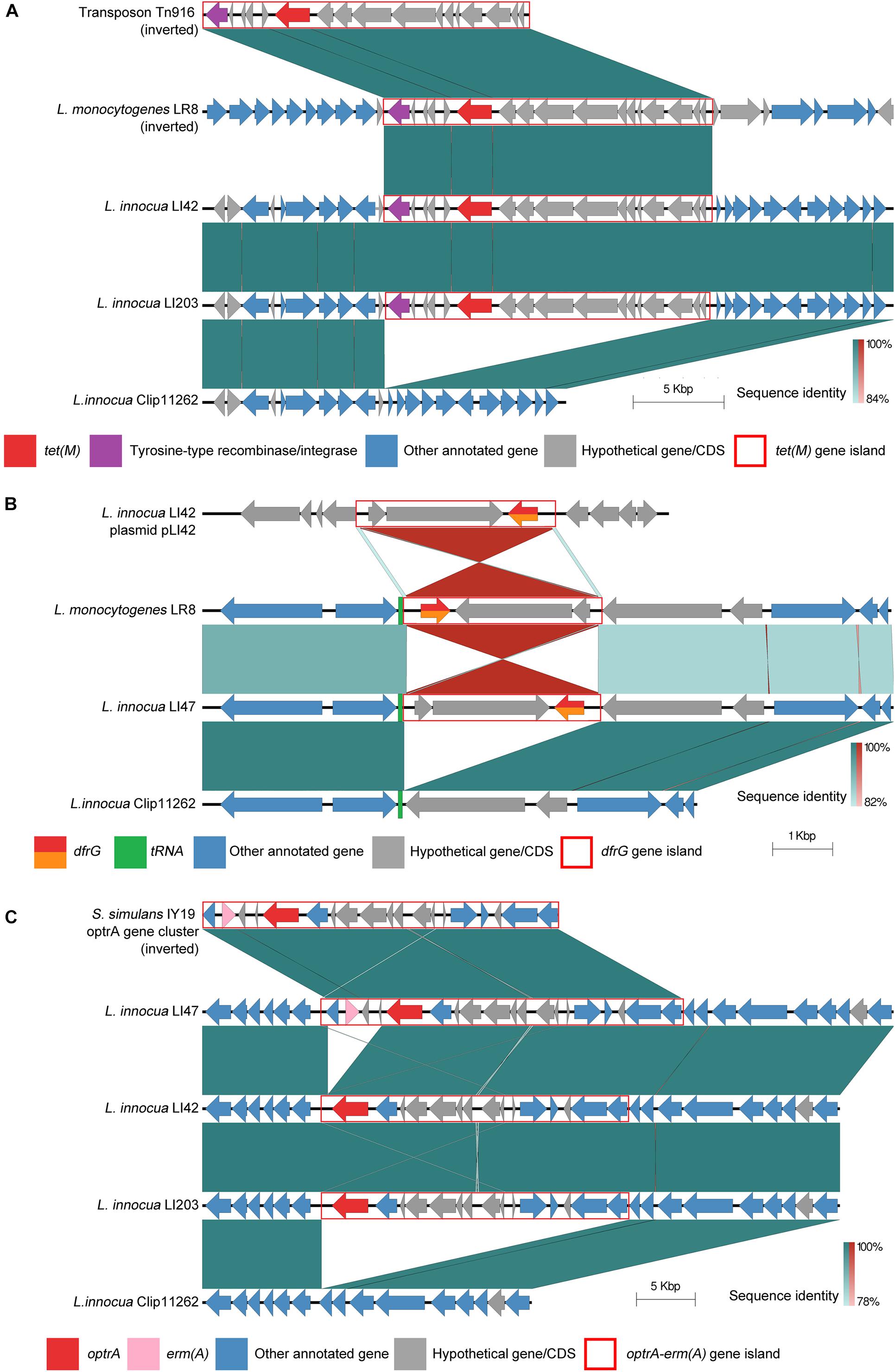
Figure 2. Linear sequence comparison of resistance genes and its flanking region on chromosome. The tet(M) gene islands (A), dfrG gene islands (B), and optrA-erm(A) gene islands (C) and their flanking regions of LI42, LI47 or LI203 chromosomes was compared with their most closely related orthologs from nr/nt database and the susceptible L. innocua reference genome Clip11262. (A) Comparison of the tet(M) gene islands on LI42 and LI203 chromosomes with L. monocytogenes LR8 and transposon TN916. (B) Comparison of dfrG gene island in LI47 chromosome with plasmid pLI42 and L. monocytogenes LR8 chromosome. (C) Comparison of optrA-erm(A) gene islands in LI47, LI42, and LI203 chromosomes with S. simulans IY19 optrA gene cluster. Resistance genes and genes related to insertion or recombination were highlighted. Resistance gene islands were marked in red frames. Green or red shades between each two sequences represented direct or inverted nucleotide identity between covered regions.
The L. innocua LI42 chromosome harbors the complete optrA-erm(A) gene island. It is identical to Staphylococcus simulans IY19 optrA gene cluster (MF805730), which was reported in an earlier study (Sun et al., 2018). In L. innocua LI47 and LI203 a partial optrA-erm(A) gene island only was noted, and which is devoid of erm(A) gene. The insertion locations of this gene island were consistent among L. innocua LI42, LI47 and LI203. Flanking regions of all three resistance gene islands were identical in comparison with the susceptible L. innocua reference genome Clip11262.
Genome Environment Analysis of LIPI-4
Genomic comparisons were performed on LIPI-4 and its flanking region in L. innocua and L. monocytogenes in order to discover the degree to which it may be conserved between these species. The LIPI-4 ortholog was located at the corresponding position on the chromosomes of L. innocua LI42, LI47,and LI203 and the reference L. monocytogenes N2306. The sequence context of LIPI-4 was found to be consistent. The reference L. monocytogenes EGD-e was devoid of LIPI-4, while possessing an identical LIPI-4 flanking region (as shown in Figure 3).
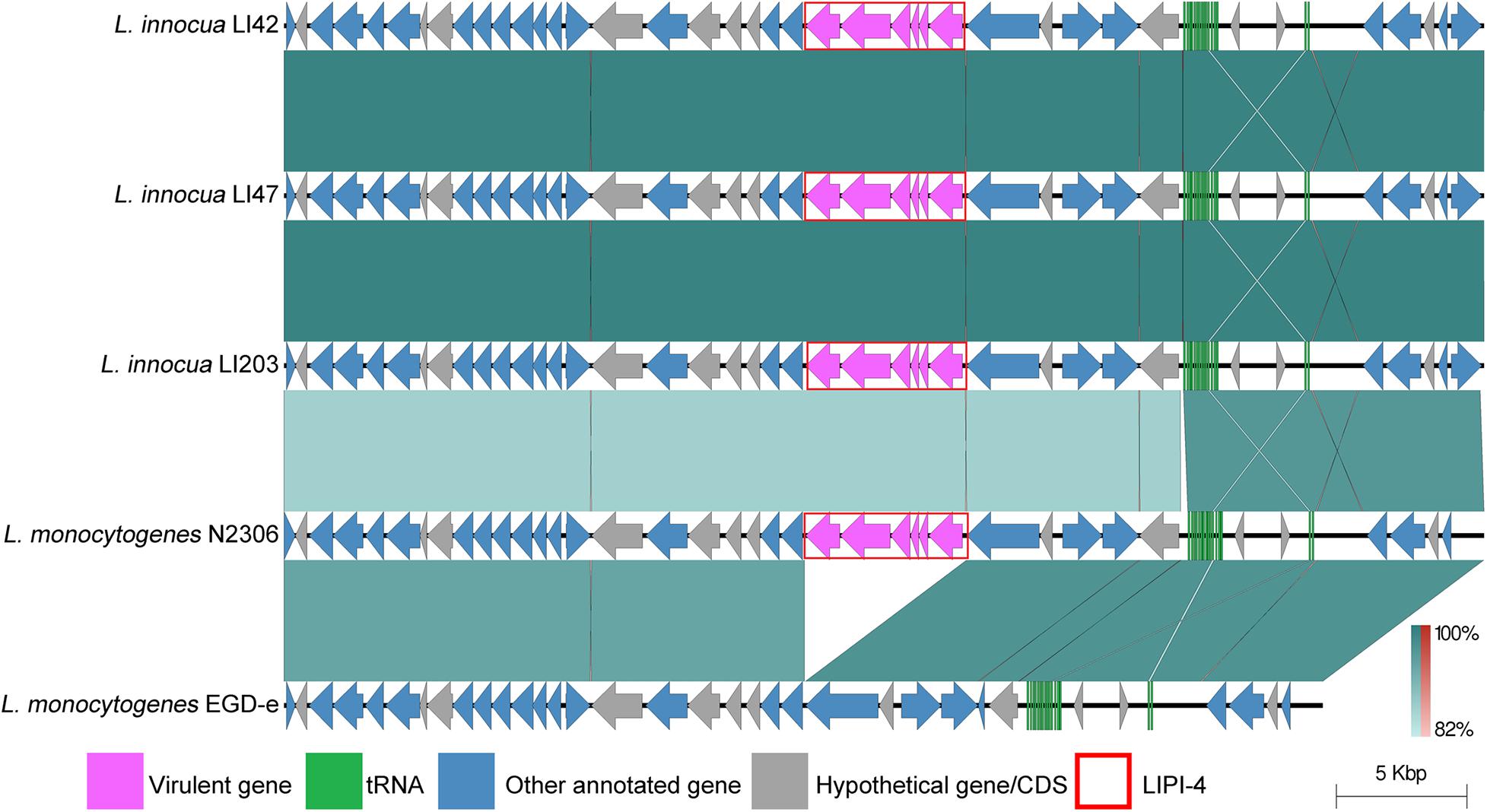
Figure 3. Linear sequence comparison of LIPI-4 and its flanking regions. Sequences of LIPI-4 and their flanking regions on LI42, LI47, and LI203 genomes were compared with their ortholog in L. monocytogenes reference genome N2306 and EGD-e. Virulence genes were highlighted in pink arrows and LIPI-4 regions were marked in red frame. Green or red shades between each two sequences represented direct or inverted nucleotide identity between covered regions.
A 10-kbp upstream and downstream region flanking LIPI-4 were assessed for a comparative analysis of their genomic features. These regions were found to be identical among the L. innocua strains, and in L. monocytogenes with/without LIPI-4. The LIPI-4 locus of both species shared an identical lacE-celA-lacF-chbG-manR-pagL gene arrangement. Average sequence identity of each gene within/between species is shown in Supplementary Table 2. L. monocytogenes lacking LIPI-4 demonstrated highly similar orthologous regions to the LIPI-4 adjacent regions, especially for the lineage-I strains. The sequence identity between the non-LIPI-4 orthologs and the LIPI-4 containing L. monocytogenes lineage-I was approximately 100%.
Discussion
Genomic Features of Antibiotic Resistance
In this study, three L. innocua each expressing an MDR phenotype were studied. When sequenced, their corresponding antibiotic resistant-encoding genes were found to map either to the bacterial chromosome or to plasmids contained therein. In the case of these plasmids that were identified, an ortholog of L. monocytogenes MDR-expressing plasmid pLR1 were detected in L. innocua LI42 and LI203. The same plasmid in a L. monocytogenes isolate was also recovered in China, and denoted as plasmid pNH1 (Yan et al., 2020). A novel MDR expressing plasmid pLI47-1 is also described in this study. Sequence comparisons highlighted the mosaic nature of MDR gene islands in both of these plasmids wherein their resistance genes appeared to arise from multiple origins including Staphylococcus aureus, Enterococcus faecium, and Lactococcus lactis, with IS1216E likely to play a decisive role in the recombination steps contributing to their formation. Similar observations have been reported earlier by others (Kang et al., 2019; Moroi et al., 2019; Iimura et al., 2020).
The >99% sequence identities between plasmid pLI42 and pLI203 in L. innocua and pLR1 in L. monocytogenes showed that these plasmids were highly homologous. Meanwhile, the isolates harboring these plasmids were isolated from different years, for L. monocytogenes isolate LR1 harboring pLR1 was discovered in 2012, while the L. innocua isolates harboring pLI42 and pLI203 were discovered in 2015 and 2016. These evidences may indicate the potential mobilizing nature of these plasmids between Listeria species. However, our conjugation experiment showed that pLI42, pLI47-1 and pLI203 in L. innocua were non-conjugative to L. monocytogenes or E. coli (data not shown). A previous study also reported that the homologous plasmid pNH1 was non-conjugative between L. monocytogenes strains (Yan et al., 2020). Thus, the specific mobilizing mechanism of the plasmids still needs to be revealed.
On chromosomes, three different types of antibiotic resistance gene-containing islands were discovered in L. innocua in this study. The tet(M) gene-containing islands were found to be orthologs of transposon Tn916. The differences in the sequence context identified in L. monocytogenes LR8 and the two L. innocua isolates suggested its ability to transfer and recombine at different positions on bacterial chromosome. The short direct repeat sequences flanking dfrG gene-containing islands may facilitate insertion into the genome, a feature noted on both chromosomes as well as plasmids. The flanking regions of dfrG islands in L. innocua LR8 and LI47 are identical, while the insertional orientation of the module is opposite, reflecting the flexibility of this step. There were no orthologs of optrA-erm(A) gene-containing islands found in any L. monocytogenes genomes analyzed to date. However, its identification in S. simulans (Sun et al., 2018), a non-closely related species of L. innocua, as well as their significant identity, suggested a potentially recent horizontal gene transfer, which possibly hints at its horizontal transmitting nature. Additionally, the GC content of optrA-erm(A) gene island is 35.1%. Comparing with L. monocytogenes (∼38.1%) and L. innocua (∼37.5%), it is closer to S. simulans (∼35.9%, S. simulans strain NCTC11046, NZ_LS483313). Thus S. simulans is more likely to be the original host of this gene island.
Discovery of L. monocytogenes MDR plasmids and resistance gene-containing islands on chromosome in L. innocua confirmed the fact that the latter can act as a gene sink, collecting AMR determinants from a range of sources. Antimicrobial resistant L. innocua have the potential to constitute a serious threat to public health through possible transferring of resistance genes to susceptible L. monocytogenes.
Genomic and Evolutionary Features and of Virulence
L. innocua containing LIPIs are usually considered as “atypical”’ (Volokhov et al., 2007; Clayton et al., 2014; Moura et al., 2019). Nonetheless, LIPI-4 is recently reported widespread among this species (Moura et al., 2019). In this study, LIPI-4 was identified in all three L. innocua studied. The existence of the LIPI-4 orthologs in L. innocua suggested a possibility of gain or loss of virulence genes during evolution.
A LIPI-4 phylogenetic tree created using 10 L. innocua and 11 L. monocytogenes clearly clustered by species (Supplementary Figure 1). The tree was split precisely into two branches in accordance with their species. The L. innocua branch had longer internal evolutionary distances compared to the other, implying a longer evolutionary history. The core genome phylogenetic tree of L. innocua and L. monocytogenes indicated an explicit clustering by species. The L. monocytogenes branch was clustered by lineages, while L. monocytogenes LIPI-4 were interspersed in the L. monocytogenes lineage -I cluster (Supplementary Figure 2). All L. monocytogenes containing LIPI-4 were found in lineage -I, and in this case the branch containing L. monocytogenes lineages -II and -III collapsed. There was no explicit common ancestor for the L. monocytogenes containing LIPI-4.
To better discover the evolutionary pathway of LIPI-4, Ka/Ks values were calculated for both L. innocua and L. monocytogenes, except wherein the gene had no mutation among all pairs (Supplementary Table 3; Wang et al., 2009, 2010). When the Ka/Ks ratio was less than 1 for all L. innocua and monocytogenes LIPI-4 genes, this implied that LIPI-4 was under purifying selection for both species. All genes of LIPI-4 orthologous were in identical order and shared high similarity in gene sequence individually. All L. monocytogenes harboring LIPI-4 belonged to lineage -I. Both genomes and LIPI-4 containing regions of L. monocytogenes were less diverse comparing with those of L. innocua. Additionally, no LIPI-4-absent L. innocua strain had been reported. Combining the above observations, it may imply that LIPI-4 of both species may originate from same ancestor. The obviously slower differentiation rate of L. monocytogenes LIPI-4 than that of L. innocua, suggested L. innocua may acquire the LIPI-4 earlier than L. monocytogenes.
Since phylogeny of LIPI-4 and genome showed no branch crossing, together with the result of the sequence comparison where average identity of LIPI-4 was 4% lower than the overall genome average identity between the two species, it is less likely that horizontal gene transfer of LIPI-4 arose in from contemporary L. innocua. Moreover, as no other species besides L. innocua and L. monocytogenes have been found to harbor a LIPI-4 ortholog or found through online BLASTN toward NCBI nt/nr database. It could be assumed that LIPI-4 may have transferred from a progenitor of L. innocua to a later L. monocytogenes lineage -I. However, the origins and transferring path of LIPI-4 remains unknown.
Conclusion
L. innocua, shared resistance and virulence genes with its infamous close relative L. monocytogenes, is not totally innocuous. As reports have highlighted, L. innocua and L. monocytogenes are commonly detected together in the same ecological niches (Franco et al., 1995; Wagner et al., 2007; Kim et al., 2017; Zhao et al., 2021). This implies the possibility of virulence/resistance gene transferring between these two species on the other side. Therefore, it would be better for public health that L. innocua need to be taken into consideration to refine the risk assessment of L. monocytogenes during future food surveillance and monitoring.
Data Availability Statement
Accession numbers for complete genome sequences are SAMN18079989 (LI42), SAMN18080006 (LI47), and SAMN18080009 (LI203).
Author Contributions
All authors listed have made a substantial, direct and intellectual contribution to the work, and approved it for publication.
Funding
This study was supported by grant (2017YFC1601402) from the Ministry of Science and Technology of China and the project of talent and training and development of China National Centre for Food Safety Risk Assessment (523 Project).
Conflict of Interest
The authors declare that the research was conducted in the absence of any commercial or financial relationships that could be construed as a potential conflict of interest.
Publisher’s Note
All claims expressed in this article are solely those of the authors and do not necessarily represent those of their affiliated organizations, or those of the publisher, the editors and the reviewers. Any product that may be evaluated in this article, or claim that may be made by its manufacturer, is not guaranteed or endorsed by the publisher.
Supplementary Material
The Supplementary Material for this article can be found online at: https://www.frontiersin.org/articles/10.3389/fmicb.2021.694361/full#supplementary-material
Supplementary Figure 1 | LIPI-4 phylogenetic tree of 4 L. innocua isolates with reference L. innocua and L. monocytogenes. Phylogenetic tree was built with FastTree and modified by FigTree using LIPI-4 sequences of 4 L. innocua and from NCBI nt/nr database. Root was set as midpoint.
Supplementary Figure 2 | Genome phylogenetic tree of 4 L. innocua isolates with reference L. innocua and L. monocytogenes. Phylogenetic tree was built with FastTree (v2.1.10) and modified by FigTree using whole genome sequences of 4 L. innocua and completely assembled reference genomes of L. monocytogenes and L. innocua from NCBI assembly database. Root was set as midpoint. L. innocua strains were marked in blue. L. monocytogenes strains with LIPI-4 were marked in red, while the strains without LIPI-4 were marked in yellow.
Footnotes
References
Bertsch, D., Muelli, M., Weller, M., Uruty, A., Lacroix, C., and Meile, L. (2014). Antimicrobial susceptibility and antibiotic resistance gene transfer analysis of foodborne, clinical, and environmental Listeria spp. isolates including Listeria monocytogenes. Microbiologyopen 3, 118–127. doi: 10.1002/mbo3.155
Bertsch, D., Uruty, A., Anderegg, J., Lacroix, C., Perreten, V., and Meile, L. (2013). Tn6198, a novel transposon containing the trimethoprim resistance gene dfrG embedded into a Tn916 element in Listeria monocytogenes. J. Antimicrob. Chemother. 68, 986–991. doi: 10.1093/jac/dks531
Buchrieser, C., Rusniok, C., Kunst, F., Cossart, P., and Glaser, P. (2003). Comparison of the genome sequences of Listeria monocytogenes and Listeria innocua: clues for evolution and pathogenicity. FEMS Immunol. Med. Microbiol. 35, 207–213. doi: 10.1016/s0928-8244(02)00448-0
Chen, J., Jiang, L., Chen, X., Luo, X., Chen, Y., Yu, Y., et al. (2009). Listeria monocytogenes serovar 4a is a possible evolutionary intermediate between L. monocytogenes serovars 1/2a and 4b and L. innocua. J. Microbiol. Biotechnol. 19, 238–249.
Clayton, E. M., Daly, K. M., Guinane, C. M., Hill, C., Cotter, P. D., and Ross, P. R. (2014). Atypical Listeria innocua strains possess an intact LIPI-3. BMC Microbiol. 14:58. doi: 10.1186/1471-2180-14-58
Clsi Institute (2015). Methods for Antimicrobial Dilution and Disk Susceptibility Testing of Infrequently Isolated or Fastidious Bacteria: 3rd Edition M45. Wayne, PA: CLSI.
Cotter, P. D., Draper, L. A., Lawton, E. M., Daly, K. M., Groeger, D. S., Casey, P. G., et al. (2008). Listeriolysin S, a novel peptide haemolysin associated with a subset of lineage I Listeria monocytogenes. PLoS Pathog. 4:e1000144. doi: 10.1371/journal.ppat.1000144
Escolar, C., Gómez, D., Del Carmen Rota García, M., Conchello, P., and Herrera, A. (2017). Antimicrobial Resistance Profiles of Listeria monocytogenes and Listeria innocua Isolated from Ready-to-Eat Products of Animal Origin in Spain. Foodborne Pathog. Dis. 14, 357–363. doi: 10.1089/fpd.2016.2248
Feldgarden, M., Brover, V., Haft, D. H., Prasad, A. B., Slotta, D. J., Tolstoy, I., et al. (2019). Validating the AMRFinder Tool and Resistance Gene Database by Using Antimicrobial Resistance Genotype-Phenotype Correlations in a Collection of Isolates. Antimicrob. Agents Chemother. 63, 483–419. doi: 10.1128/aac.00483-19
Finlay, B. B. (2001). Microbiology. Cracking Listeria’s password. Science 292, 1665–1667. doi: 10.1126/science.1062045
Franco, C. M., Quinto, E. J., Fente, C., Rodriguez-Otero, J. L., Dominguez, L., and Cepeda, A. (1995). Determination of the Principal Sources of Listeria spp. Contamination in Poultry Meat and a Poultry Processing Plant. J. Food Prot. 58, 1320–1325. doi: 10.4315/0362-028x-58.12.1320
Glaser, P., Frangeul, L., Buchrieser, C., Amend, A., Baquero, F., Berche, P., et al. (2001a). From the pathogenic to the innocuous: comparison of the Listeria monocytogenes and the Listeria innocua genomes. Bethesda: GenBank.
Glaser, P., Frangeul, L., Buchrieser, C., Rusniok, C., Amend, A., Baquero, F., et al. (2001b). Comparative genomics of Listeria species. Science 294, 849–852. doi: 10.1126/science.1063447
Gomez, D., Azon, E., Marco, N., Carraminana, J. J., Rota, C., Arino, A., et al. (2014). Antimicrobial resistance of Listeria monocytogenes and Listeria innocua from meat products and meat-processing environment. Food Microbiol. 42, 61–65. doi: 10.1016/j.fm.2014.02.017
Hain, T., Steinweg, C., and Chakraborty, T. (2006). Comparative and functional genomics of Listeria spp. J. Biotechnol. 126, 37–51. doi: 10.1016/j.jbiotec.2006.03.047
Holden, M. T., Hauser, H., Sanders, M., Ngo, T. H., Cherevach, I., Cronin, A., et al. (2009). Rapid evolution of virulence and drug resistance in the emerging zoonotic pathogen Streptococcus suis. PLoS One 4:e6072. doi: 10.1371/journal.pone.0006072
Iimura, M., Hayashi, W., Arai, E., Natori, T., Horiuchi, K., Matsumoto, G., et al. (2020). Identification of a multiresistant mosaic plasmid carrying a new segment of IS1216E-flanked optrA with integrated Tn551-ermB element in linezolid-resistant Enterococcus faecalis human isolate. J. Glob. Antimicrob. Resist. 22, 697–699. doi: 10.1016/j.jgar.2020.06.030
Jia, B., Raphenya, A. R., Alcock, B., Waglechner, N., Guo, P., Tsang, K. K., et al. (2017). CARD 2017: expansion and model-centric curation of the comprehensive antibiotic resistance database. Nucleic Acids Res. 45, D566–D573. doi: 10.1093/nar/gkw1004
Kang, Z. Z., Lei, C. W., Kong, L. H., Wang, Y. L., Ye, X. L., Ma, B. H., et al. (2019). Detection of transferable oxazolidinone resistance determinants in Enterococcus faecalis and Enterococcus faecium of swine origin in Sichuan Province, China. J. Glob. Antimicrob. Resist. 19, 333–337. doi: 10.1016/j.jgar.2019.05.021
Kathariou, S., Metz, P., Hof, H., and Goebel, W. (1987). Tn916-induced mutations in the hemolysin determinant affecting virulence of Listeria monocytogenes. J. Bacteriol. 169, 1291–1297. doi: 10.1128/jb.169.3.1291-1297.1987
Kim, Y. S., Sohn, K. M., Kang, S. J., Jung, S. I., Kim, S. W., Chang, H. H., et al. (2017). Epidemiology and treatment of the commonest form of listeriosis: meningitis and bacteraemia. Infection 25, 210–216. doi: 10.1007/s15010-016-0939-2
Maury, M. M., Tsai, Y. H., Charlier, C., Touchon, M., Chenal-Francisque, V., Leclercq, A., et al. (2016). Uncovering Listeria monocytogenes hypervirulence by harnessing its biodiversity. Nat. Genet. 48, 308–313. doi: 10.1038/ng.3501
Moroi, H., Kimura, K., Ido, A., Banno, H., Jin, W., Wachino, J. I., et al. (2019). Erythromycin-Susceptible but Clindamycin-Resistant Phenotype of Clinical ermB-PCR-Positive Group B Streptococci Isolates with IS1216E-Inserted ermB. Jpn. J. Infect. Dis. 72, 420–422. doi: 10.7883/yoken.JJID.2019.015
Moura, A., Disson, O., Lavina, M., Thouvenot, P., Huang, L., Leclercq, A., et al. (2019). Atypical Hemolytic Listeria innocua Isolates Are Virulent, albeit Less than Listeria monocytogenes. Infect. Immun. 87, 758–718. doi: 10.1128/iai.00758-18
Nelson, K. E., Fouts, D. E., Mongodin, E. F., Ravel, J., DeBoy, R. T., Kolonay, J. F., et al. (2004). Whole genome comparisons of serotype 4b and 1/2a strains of the food-borne pathogen Listeria monocytogenes reveal new insights into the core genome components of this species. Nucleic Acids Res. 32, 2386–2395. doi: 10.1093/nar/gkh562
Orsi, R. H., and Wiedmann, M. (2016). Characteristics and distribution of Listeria spp., including Listeria species newly described since 2009. Appl. Microbiol. Biotechnol. 100, 5273–5287. doi: 10.1007/s00253-016-7552-2
Portmann, A. C., Fournier, C., Gimonet, J., Ngom-Bru, C., Barretto, C., and Baert, L. (2018). A Validation Approach of an End-to-End Whole Genome Sequencing Workflow for Source Tracking of Listeria monocytogenes and Salmonella enterica. Front. Microbiol. 9:446. doi: 10.3389/fmicb.2018.00446
Reddy, S., and Lawrence, M. L. (2014). Virulence characterization of Listeria monocytogenes. Methods Mol. Biol. 1157, 157–165. doi: 10.1007/978-1-4939-0703-8_13
Suarez, M., Gonzalez-Zorn, B., Vega, Y., Chico-Calero, I., and Vazquez-Boland, J. A. (2001). A role for ActA in epithelial cell invasion by Listeria monocytogenes. Cell Microbiol. 3, 853–864. doi: 10.1046/j.1462-5822.2001.00160.x
Sun, C., Zhang, P., Ji, X., Fan, R., Chen, B., Wang, Y., et al. (2018). Presence and molecular characteristics of oxazolidinone resistance in staphylococci from household animals in rural China. J. Antimicrob. Chemother. 73, 1194–1200. doi: 10.1093/jac/dky009
Toledo-Arana, A., Dussurget, O., Nikitas, G., Sesto, N., Guet-Revillet, H., Balestrino, D., et al. (2009). The Listeria transcriptional landscape from saprophytism to virulence. Nature 459, 950–956. doi: 10.1038/nature08080
Vivant, A. L., Garmyn, D., and Piveteau, P. (2013). Listeria monocytogenes, a down-to-earth pathogen. Front. Cell Infect. Microbiol. 3:87. doi: 10.3389/fcimb.2013.00087
Volokhov, D. V., Duperrier, S., Neverov, A. A., George, J., Buchrieser, C., and Hitchins, A. D. (2007). The presence of the internalin gene in natural atypically hemolytic Listeria innocua strains suggests descent from L. monocytogenes. Appl. Environ. Microbiol. 73, 1928–1939. doi: 10.1128/aem.01796-06
Wagner, M., Auer, B., Trittremmel, C., Hein, I., and Schoder, D. (2007). Survey on the Listeria contamination of ready-to-eat food products and household environments in Vienna, Austria. Zoonoses Public Health 54, 16–22. doi: 10.1111/j.1863-2378.2007.00982.x
Wang, D. P., Wan, H. L., Zhang, S., and Yu, J. (2009). Gamma-MYN: a new algorithm for estimating Ka and Ks with consideration of variable substitution rates. Biol. Direct 4:20. doi: 10.1186/1745-6150-4-20
Wang, D., Zhang, Y., Zhang, Z., Zhu, J., and Yu, J. (2010). KaKs_Calculator 2.0: a toolkit incorporating gamma-series methods and sliding window strategies. Genomics Proteom. Bioinformat. 8, 77–80. doi: 10.1016/s1672-0229(10)60008-3
Yan, H., Yu, R., Li, D., Shi, L., Schwarz, S., Yao, H., et al. (2020). A novel multiresistance gene cluster located on a plasmid-borne transposon in Listeria monocytogenes. J. Antimicrob. Chemother. 75, 868–872. doi: 10.1093/jac/dkz545
Yan, S., Li, M., Luque-Sastre, L., Wang, W., Hu, Y., Peng, Z., et al. (2019). Susceptibility (re)-testing of a large collection of Listeria monocytogenes from foods in China from 2012 to 2015 and WGS characterization of resistant isolates. J. Antimicrob. Chemother. 74, 1786–1794. doi: 10.1093/jac/dkz126
Zankari, E., Hasman, H., Cosentino, S., Vestergaard, M., Rasmussen, S., Lund, O., et al. (2012). Identification of acquired antimicrobial resistance genes. J. Antimicrob. Chemother. 67, 2640–2644. doi: 10.1093/jac/dks261
Keywords: Listeria innocua, multi-drug resistance, whole genome sequencing, listeria pathogenicity island 4, genome environment analysis
Citation: Li M, Yan S, Fanning S, Li F and Xu J (2021) Whole Genome Analysis of Three Multi-Drug Resistant Listeria innocua and Genomic Insights Into Their Relatedness With Resistant Listeria monocytogenes. Front. Microbiol. 12:694361. doi: 10.3389/fmicb.2021.694361
Received: 13 April 2021; Accepted: 21 June 2021;
Published: 23 July 2021.
Edited by:
Aloysius Wong, Kean University-Wenzhou, ChinaCopyright © 2021 Li, Yan, Fanning, Li and Xu. This is an open-access article distributed under the terms of the Creative Commons Attribution License (CC BY). The use, distribution or reproduction in other forums is permitted, provided the original author(s) and the copyright owner(s) are credited and that the original publication in this journal is cited, in accordance with accepted academic practice. No use, distribution or reproduction is permitted which does not comply with these terms.
*Correspondence: Shaofei Yan, eWFuc2hhb2ZlaUBjZnNhLm5ldC5jbg==; Jin Xu, eHVqaW5AY2ZzYS5uZXQuY24=
†These authors have contributed equally to this work