- 1Key Laboratory of Tropical Marine Bio-Resources and Ecology, South China Sea Institute of Oceanology, Chinese Academy of Sciences, Guangzhou, China
- 2Southern Marine Science and Engineering Guangdong Laboratory (Guangzhou), Guangzhou, China
- 3Department of Marine Sciences, University of Connecticut, Groton, CT, United States
- 4Key Laboratory of the Ministry of Education for Coastal and Wetland Ecosystem, College of the Environment and Ecology, Xiamen University, Xiamen, China
- 5Guangdong Provincial Key Laboratory of Fishery Ecology and Environment, Key Laboratory of South China Sea Fishery Resources Exploitation and Utilization, Ministry of Agriculture and Rural Affairs, P. R. China, South China Sea Fisheries Research Institute, Chinese Academy of Fishery Sciences, Guangzhou, China
- 6State Key Laboratory of Marine Environmental Science, Marine Biodiversity and Global Change Research Center, Xiamen University, Xiamen, China
Ciliates are abundant microplankton that are widely distributed in the ocean. In this paper, the distribution patterns of ciliate diversity in the South China Sea (SCS) were analyzed by compiling community data from previous publications. Based on morphological identification, a total of 592 ciliate species have been recorded in the SCS. The ciliate communities in intertidal, neritic and oceanic water areas were compared in terms of taxonomy, motility and feeding habit composition, respectively. Significant community variation was revealed among the three areas, but the difference between the intertidal area and the other two areas was more significant than that between neritic and oceanic areas. The distributions of ciliates within each of the three areas were also analyzed. In the intertidal water, the community was not significantly different among sites but did differ among habitat types. In neritic and oceanic areas, the spatial variation of communities among different sites was clearly observed. Comparison of communities by taxonomic and ecological traits (motility and feeding habit) indicated that these traits similarly revealed the geographical pattern of ciliates on a large scale in the SCS, but to distinguish the community variation on a local scale, taxonomic traits has higher resolution than ecological traits. In addition, we assessed the relative influences of environmental and spatial factors on assembly of ciliate communities in the SCS and found that environmental selection is the major process structuring the taxonomic composition in intertidal water, while spatial processes played significant roles in influencing the taxonomic composition in neritic and oceanic water. Among ecological traits, environmental selection had the most important impact on distributions.
Introduction
Ciliates are common members of the microplankton, and usually dominate marine microzooplankton communities in terms of both species number and abundance (Azam and Malfatti, 2007; Lynn, 2008; De Vargas et al., 2015). By consuming phytoplankton while also serving as prey for metazoans, these protists act as an intermediate link of energy transfer in planktonic food webs (Pierce and Turner, 1992; Fenchel, 2008).
An increasing number of investigations on diversity and distribution of ciliates have been conducted worldwide (Dolan and Marrasé, 1995; Leakey et al., 1996; Pitta and Giannakourou, 2000; Ota and Taniguchi, 2003; Johansson et al., 2004; Gómez, 2007; Yang et al., 2020). However, one of the most hotly debated issues remains unresolved, i.e., whether or not ciliate distributions are spatially restricted. Some researchers find that global ciliate diversity is relatively low and local diversity covers a very high proportion of global diversity (Fenchel et al., 1997; Fenchel and Finlay, 2004), while others find an extremely high global diversity and that the proportion of the global species pool found locally is only moderate (Pierce and Turner, 1993; Foissner et al., 2008; Agatha, 2011). To address this issue, it is essential to explore the mechanisms that determine the assembly of ciliate communities (Dolan et al., 2007; Doherty et al., 2010). A large number of studies have confirmed that ciliate communities can be influenced by environmental variables including nutrients, salinity, pH, temperature, and biotic interactions (e.g., predators) (Urrutxurtu et al., 2003; Forster et al., 2012; Gimmler et al., 2016; Sun et al., 2017). Meanwhile, spatial factors (dispersal) have also been considered in the study of ciliate community assembly. Any dispersal limitation should lead to a decrease in community similarity with distance (Gong et al., 2015; Zhao et al., 2017; Pan et al., 2020). However, a growing number of studies have indicated that the influence of environmental and spatial variables on ciliates depends on study scale and ecosystem types (Langenheder and Ragnarsson, 2007; Martiny et al., 2011; Soininen et al., 2011; Hanson et al., 2012; Zhang et al., 2018). For example, the ciliate community structure in the mesopelagic zone is mainly controlled by depth and geographic distance (Grattepanche et al., 2016b; Sun et al., 2019), while environmental selection exhibits a greater influence on ciliates than spatial factors in intertidal sandy sediments at continental scale (Pan et al., 2020).
There is a growing interest in the diversity and distributions of ciliates, considered according to their ecological traits (e.g., Green et al., 2008; Soininen et al., 2016). Ecological traits can be more directly linked to species fitness or performance than taxonomical identity can (Laureto et al., 2015). Therefore, to better understand the relationships between communities and the environment, it is necessary to investigate the geographical patterns of ecological trait composition as well as potential mechanisms whereby they may affect community composition (McGill et al., 2006; Villéger et al., 2011).
As the largest semi-enclosed basin in the western Pacific Ocean and the largest marginal sea of China, the South China Sea (SCS) has an expansive area which covers both subtropical and tropical regions (Su, 2004). It hosts strong gradients in physico-chemical environments and is known as an important hotspot of marine biodiversity (Chen et al., 2001; Huang et al., 2004; Li et al., 2017; Sun et al., 2019). Many studies on the diversity and distribution of ciliates have been conducted in the SCS in the last two decades (Su et al., 2007; Liu et al., 2010, 2016a,b; Tan et al., 2010; Wang et al., 2013, 2014; Yu et al., 2014; Wu et al., 2016a,b,c, 2017, 2019; Hu et al., 2019; Huang et al., 2021). However, most of these studies were conducted on a small local scale, such as estuaries, bays, and reef islands; this has resulted in knowledge that is more or less patchy, and the overall ciliate distribution pattern in the SCS is still poorly known.
Here, we collect the available community data on ciliates from the published literature, and analyze distributions in the SCS. Our aims are to quantify the overall spatial patterns by considering the area as a whole, and to check for whether ciliates are regionally restricted or ubiquitously distributed by analyzing the community variations in terms of taxonomy and ecological trait compositions among intertidal, neritic and oceanic areas as well as among different sites within each area. In addition, we also assess the influence of environmental and spatial factors on the geographical distributions of ciliate communities.
Materials and Methods
Database
Data were compiled from different sources include one monograph (Hu et al., 2019) and all papers on marine ciliate biodiversity in SCS based on morphological identification from 1991 to 2018 (for the list of papers, see Liu et al., 2021). In total 592 species from 30 investigations were included in the data set (see Supplementary Table S1 in Supplement for a complete list). The community from each investigation was set as one sample in our analyses.
Community Composition Analyses
Considering spatial environmental variation, we firstly analyzed the large-scale patterns of ciliate community composition based on three geographical area groupings, i.e., the intertidal area (waters covering the area between the low and high tides), the neritic area (beyond the intertidal zone and over the continental shelf), and the oceanic area (from the shelf break to the deep sea) (Liu et al., 2021). Subsequently, in each area, the distribution variations of ciliates on a regional scale were analyzed based on 3–4 subarea groupings (Supplementary Figure S1). Further, for the samples from the intertidal area, five habits types (i.e., estuary, aquaculture pond, beach, mangrove and harbor) were identified and their specific communities were compared. In addition, ciliate abundance were compared among subareas for neritic and oceanic areas, and the abundance data in intertidal areas were not available from the publications and thus were not included in our analyses.
To compare the assemblage composition of each grouping, the samples belonging to same spatial area or subarea were pooled together and the species richness of all taxa were plotted at Class or Subclass levels. Moreover, we clarified the ecological trait composition of ciliates in terms of motility and feeding habits in the areas or subareas. For motility composition, the designation of species as being sessile, vagile or planktonic was made according to the literature (e.g., Foissner, 1992; Foissner et al., 1999; Coppellotti and Matarazzo, 2000; Xu et al., 2009). For feeding habit composition, the species were assigned to five types, which comprised detritivores, bacterivores, algivores, raptors, and non-selectives, according to the original sources in which the species were described, as well as the broader literature (Pratt and Cairns, 1985; Fernandez-Leborans, 2001; Fernandez-Leborans and Fernandez-Fernandez, 2002; Lynn, 2008). Indicator species analysis was performed to identify the species that characterized each areas using the package “Indicspecies” in R (Dufrene and Legendre, 1997). Only species with indicator values (IV) > 0.3 and p < 0.05 were considered good indicators.
Statistical Analyses
In order to facilitate taxonomic consistency between different data sources in community multivariate analyses, species-level data were aggregated to the genus level (presence/absence), which resulted in a data set comprising 207 ciliate genera. Then the genera presence/absence community data were Hellinger transformed prior to the analyses (Peres-Neto et al., 2006). We analyzed the patterns of ciliate communities with non-metric multidimensional scaling (NMDS). Analysis of similarity (ANOSIM) was used to statistically test for significant differences in communities of each area or subarea based on Bray-Curtis dissimilarity with 999 random permutations. The unweighted pair group method with arithmetic mean (UPGMA) hierarchical clustering algorithm was conducted to cluster pairs of communities for each subarea, and we tested robustness of these clusters with jackknife analysis, a non-parametric estimator based on 1,000 randomized subsamples. The relationships between the Bray-Curtis dissimilarity of ciliate communities and geographic distance were analyzed based on Spearman’s rank correlations for each of the three areas.
We explored the impacts of environmental and spatial variables on the communities defined by taxonomic, motility and feeding habit traits. We included 3 environmental variables: Habitat type, Salinity, and Chlorophyll a concentration (Chla). Seven habitat types (i.e., full-open water, semi-open water, estuary, aquaculture pond, beach, mangrove and harbor) were defined as categorical variables. Salinity and Chlorophyll a concentration were averaged for each sample from the original data sources. For some samples with Chlorophyll a data not available, the mean value of last decade was extracted from NASA GSFC.1 We followed the approach of the principal coordinates of neighbor matrices (PCNMs) analyses to calculate a set of spatial factors based on the latitude and longitude of each sample. Then, variation partitioning analysis (VPA) was used to evaluate the relative contribution of the environmental and spatial variables in shaping ciliate communities with adjusted R2 coefficients based on redundancy analysis (RDA) or canonical correspondence analysis (CCA). The relative contributions of both components were explained by pure environmental variables, pure spatial variables, and the combined effects of both environment and space. Before the RDA or CCA analysis, a forward selection was conducted to select significant explanatory variables (P < 0.05) for further analyses. Mantel tests were also performed to evaluate individual effects of environmental and spatial variables on each ciliate community. All analyses were performed using the “vegan” package in R (R Core Team, 2021). Considering the high similarity of community and environmental characters between neritic and oceanic areas, the data from these two areas were combined to analyze the responses of communities to environmental and spatial variables in open water.
Results
Distribution Variation of Ciliates Among Intertidal, Neritic, and Oceanic Water of the SCS
In total, 592 ciliates species, assigned to 14 classes/subclasses, 33 orders, 96 families, and 207 genera, have been detected in the SCS. The subclass Choreotrichia is the most abundant in terms of species number (202 species), which is much higher than the second most abundant subclass, Oligotrichia (84 species) (Supplementary Figure S2).
The comparison of species richness among the three areas revealed that the intertidal area possesses a remarkably higher number of ciliate species than neritic and oceanic areas. A Venn diagram shows that the number of species found only in one area is dramatically higher than that of shared species (only 9 species were found in all three areas), and the number of species shared by neritic and oceanic communities is greater than those shared between intertidal and other two areas (Figure 1B). The taxonomic compositions of ciliates in neritic and oceanic areas are generally similar to each other at class level due to a high proportion of subclass Choreotrichia, but significantly differ from that of intertidal areas in which the proportions of many classes are similar (Figure 1A). In terms of the motility and feeding habits, intertidal communities also displayed clear differences from other two areas. For motility-based composition, the proportion of planktonic species is much lower than that of vagile species in the intertidal area, while on the other hand in neritic and oceanic areas, the vagiles represented the least abundant motility group (Figure 1C). For feeding habit composition, the abundances of most feeding types are generally equal in intertidal water except raptors, while the proportions of algivores and non-selectives are notably lower than others in neritic and oceanic areas (Figure 1D). In addition, we observed a variation in indicator species in the three areas (Figure 1E), i.e., no indicator species was found in the intertidal area, whereas many Tintinnopsis species were identified as the indicators for the neritic area, and rare tintinnids with hyaline loricas constitute the indicators of the oceanic area.
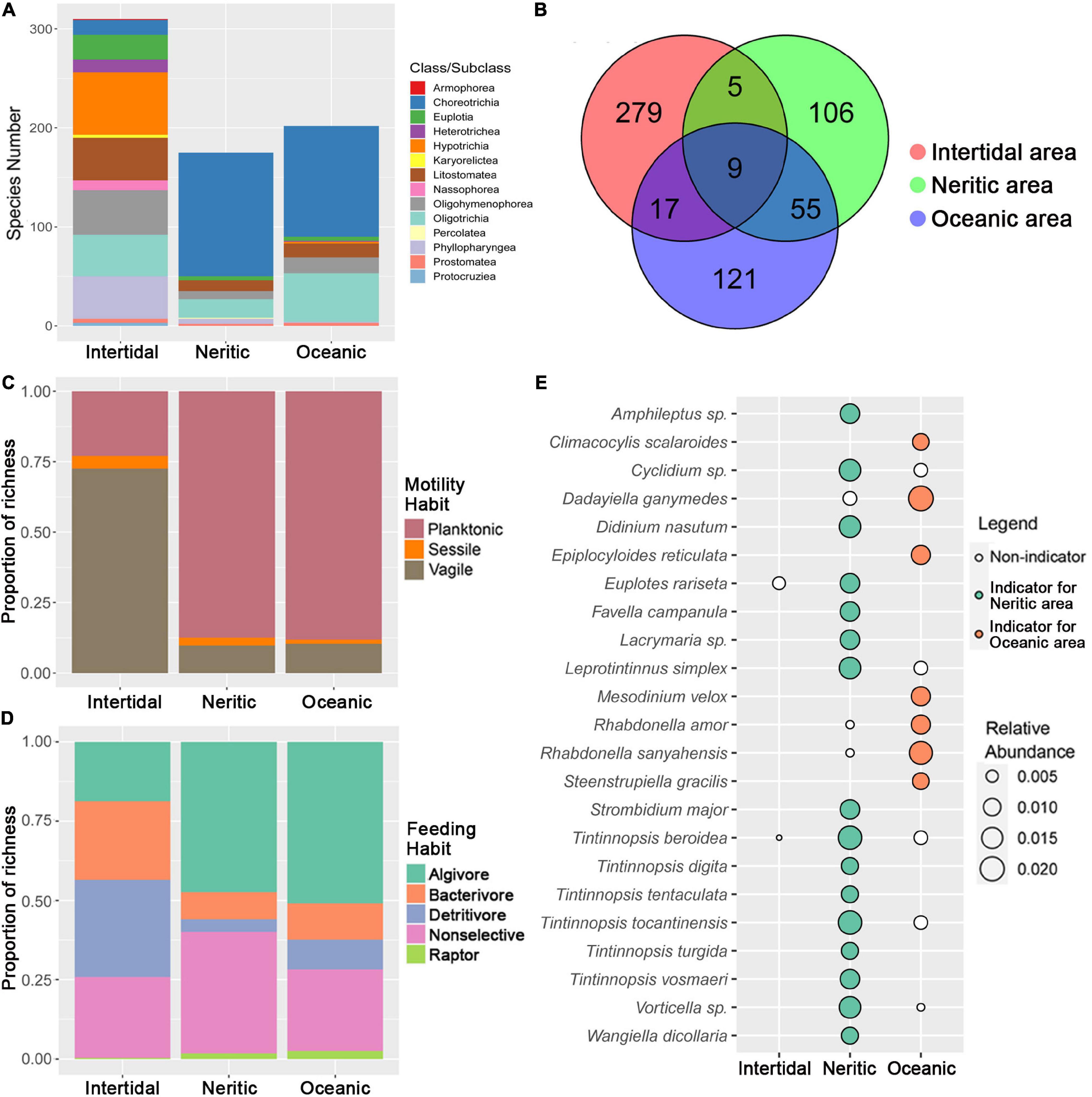
Figure 1. Comparison of ciliate diversity and composition among the three areas in SCS. (A) Taxonomy composition patterns of ciliates at class/subclass level in the three areas. (B) Venn diagram showing the number of species that are unique and shared among the three areas. (C) Proportion of motility habit grouping of ciliates in the three areas. (D) Proportion of feeding habit grouping of ciliates in the three areas. (E) Indicator species in the three areas (no indicator species was identified in the Intertidal area); the size of the bubble indicates the relative abundance of each species in each area.
The similarity analyses revealed that communities exhibited significant differences in the three areas in terms of taxonomy, motility and feeding habits (ANOSIM, P < 0.01, Table 1). However, the pairwise tests of the three areas showed that the communities from neritic and oceanic areas cannot be separated by motility and feeding habits, but were significantly different for taxonomic composition. In addition, for all three kinds of community composition, the NMDS indicated that the communities in neritic and oceanic water cluster together, and separated from intertidal communities, of which most samples were widely scattered in the plot (Figure 2A). The dissimilarity of ciliate communities among the samples in intertidal water were significantly higher than neritic and oceanic water in terms of taxonomy, motility and feeding habits compositions, but the differences between neritic and oceanic areas were not significant for motility and feeding habits compositions (Figure 2B).
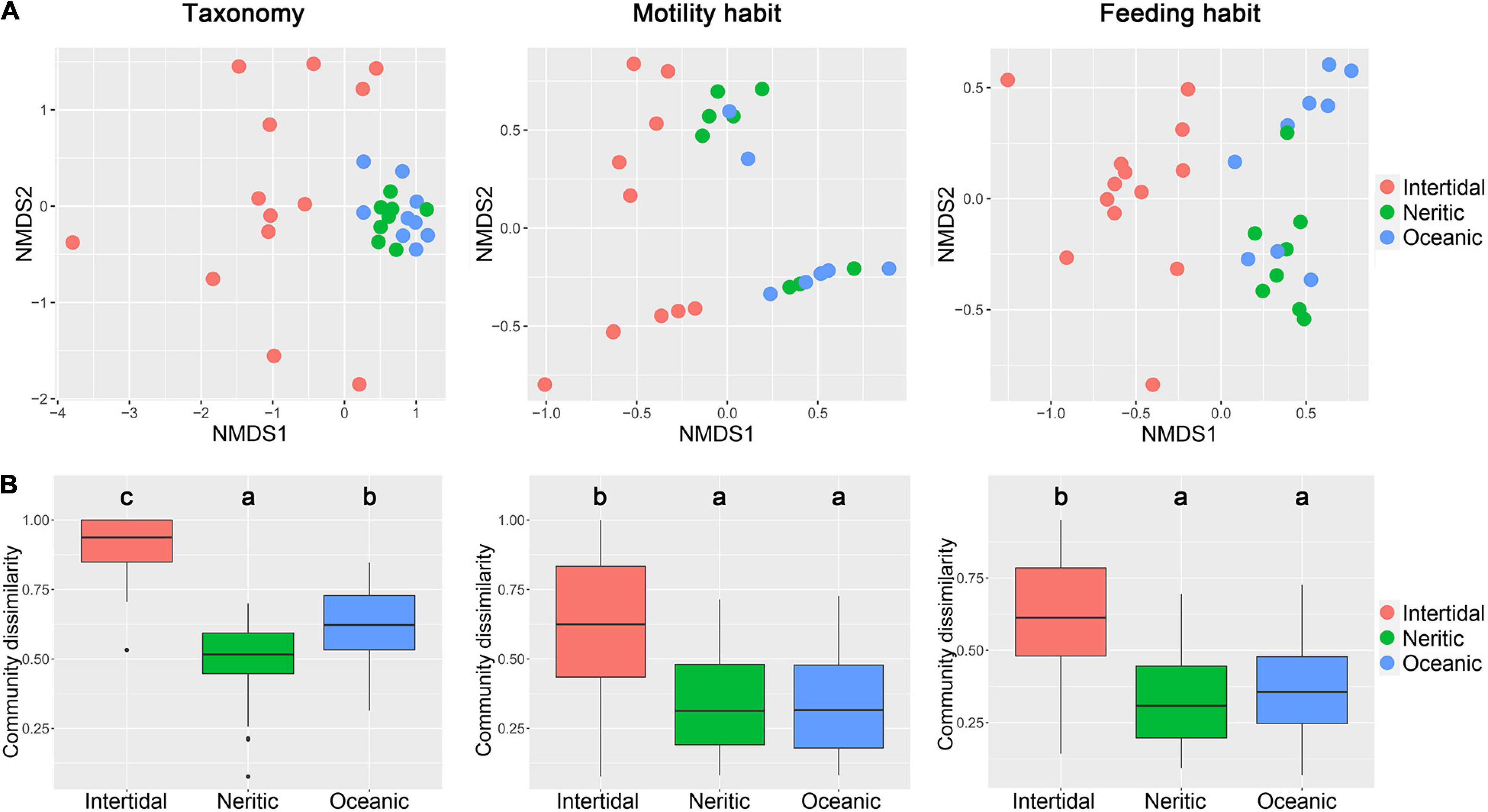
Figure 2. Comparison of ciliate communities in terms of taxonomy, motility, and feeding habits compositions among the three areas in the SCS. (A) Non-metric multidimensional scaling (NMDS) ordination of samples in the three areas based on Bray–Curtis dissimilarity. (B) Dissimilarity of ciliate communities among the samples in each area.
Distribution Patterns of Ciliate Diversity in Intertidal Water of the SCS
For ciliates in intertidal water, the cluster analyses showed that the taxonomy-based communities that clustered together did not come from the same geographic subarea but from similar habitats (Supplementary Figure S3). This is in accord with the ANOSIM analyses (Table 1), in which the communities in the three intertidal subareas could not be distinguished (r = −0.159, P = 0.907), but the communities grouped by habitat types were clearly different (P = 0.005). Therefore, subsequent community analyses were all conducted based on habitat grouping. However, for motility- and feeding habits-based communities, the ANOSIM analyses did not reveal community variations in either subareas or habitats (P > 0.1, Table 1).
Among the five habitats, the species number detected in mangroves is much higher than in the other habitats, while that in aquaculture ponds is lowest (Figure 3A). For the taxonomy (Figure 3A), motility (Figure 3B), and feeding habits (Figure 3C) based communities, obvious differences can be found among the habitats. Taking feeding habit composition as an example, the algivores and detritivores represent the dominant feeding types in both estuaries and harbors, and the non-selective omnivores dominated the ciliate communities in aquaculture ponds and beach habitats, while the proportion of bacterivores in mangroves is much higher than that in other habits.
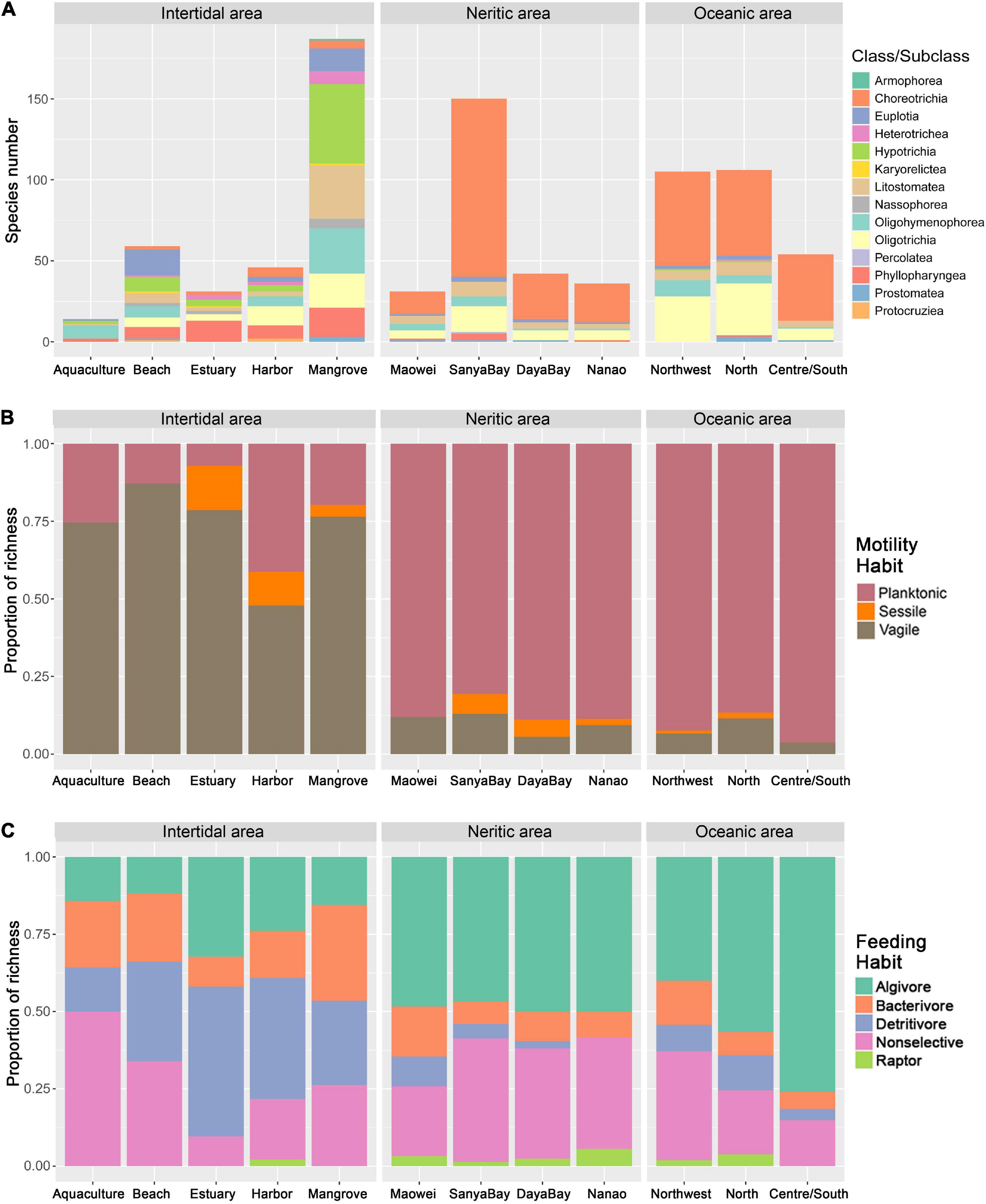
Figure 3. Community variations of ciliate diversity among the subareas in SCS. (A) Comparison of ciliate species richness and composition at the class/subclass level among the subgroups. (B) Relative proportion of motility habit groupings among the subgroups. (C) Relative proportion of feeding habit groupings among the subgroups.
The Spearman correlograms comparing community dissimilarity and geographical distances among samples did not indicate significant correlations with distance for all taxonomy, motility and feeding habit communities (Figure 4), which means that the community dissimilarity between any two samples did not vary with increasing distance.
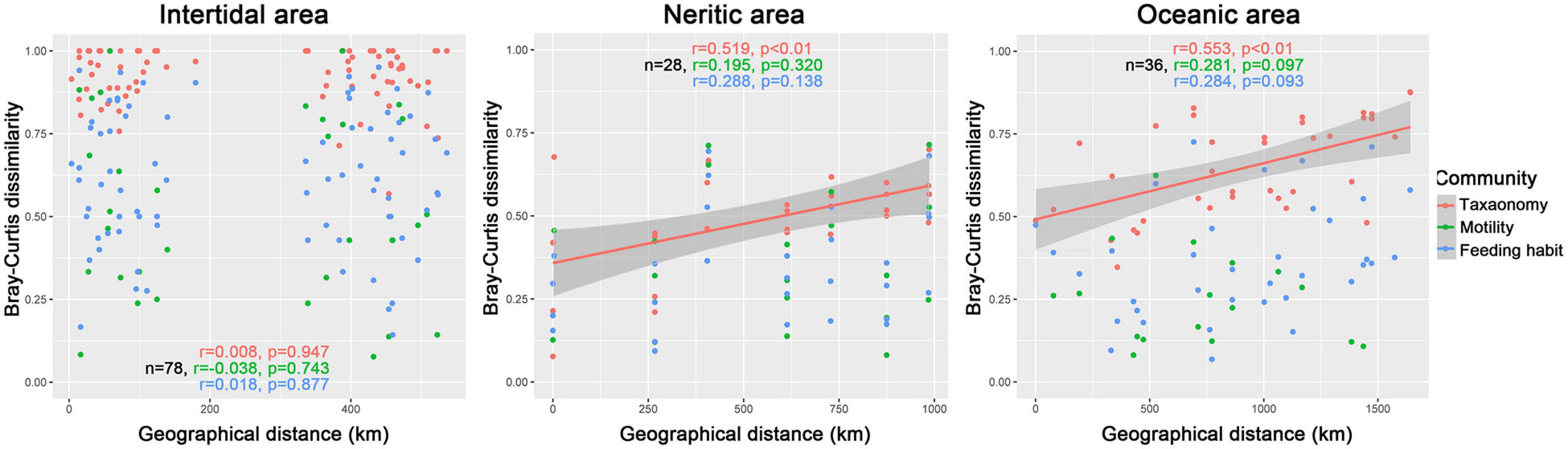
Figure 4. Spearman’s rank correlations between the pairwise Bray–Curtis dissimilarity of communities and the geographical distance of samples in intertidal, neritic, and oceanic water. The n is the number of comparisons for samples, the r is the correlation coefficient for taxonomy (red text and symbols), motility (green text and symbols), and feeding (blue text and symbols) habits compositions.
Distribution Patterns of Ciliate Diversity in Neritic Water of the SCS
In neritic areas, the taxonomy-based ciliate communities exhibited striking differences among the four subareas in the ANOSIM analyses (r = 0.458, P = 0.014, Table 1). In the cluster analyses, the communities from same subarea generally clustered together except for Sanya Bay (Supplementary Figure S3). However, for communities defined by motility and feeding habits, variations in subareas were not distinguished by the ANOSIM analyses (Table 1).
Communities defined by feeding habits showed that the relative proportions of most feeding types in the Nanao, Sanya Bay, and Daya Bay are similar, but clearly different from those in Maowei where the proportions of bacterivores and detritivores are higher than in the other three subareas and the proportion of non-selective omnivores is lower (Figure 3C). The motility habit compositions generally are similar among the four subareas (Figure 3B). In addition, the species richness of ciliates in Sanya Bay is remarkably higher than other subareas and that in Maowei is lowest (Figure 3A). In terms of average abundances of ciliates, the highest value occurred in Daya Bay, followed by Nanao and Maowei, and the lowest value was found in Sanya Bay (Figure 5).
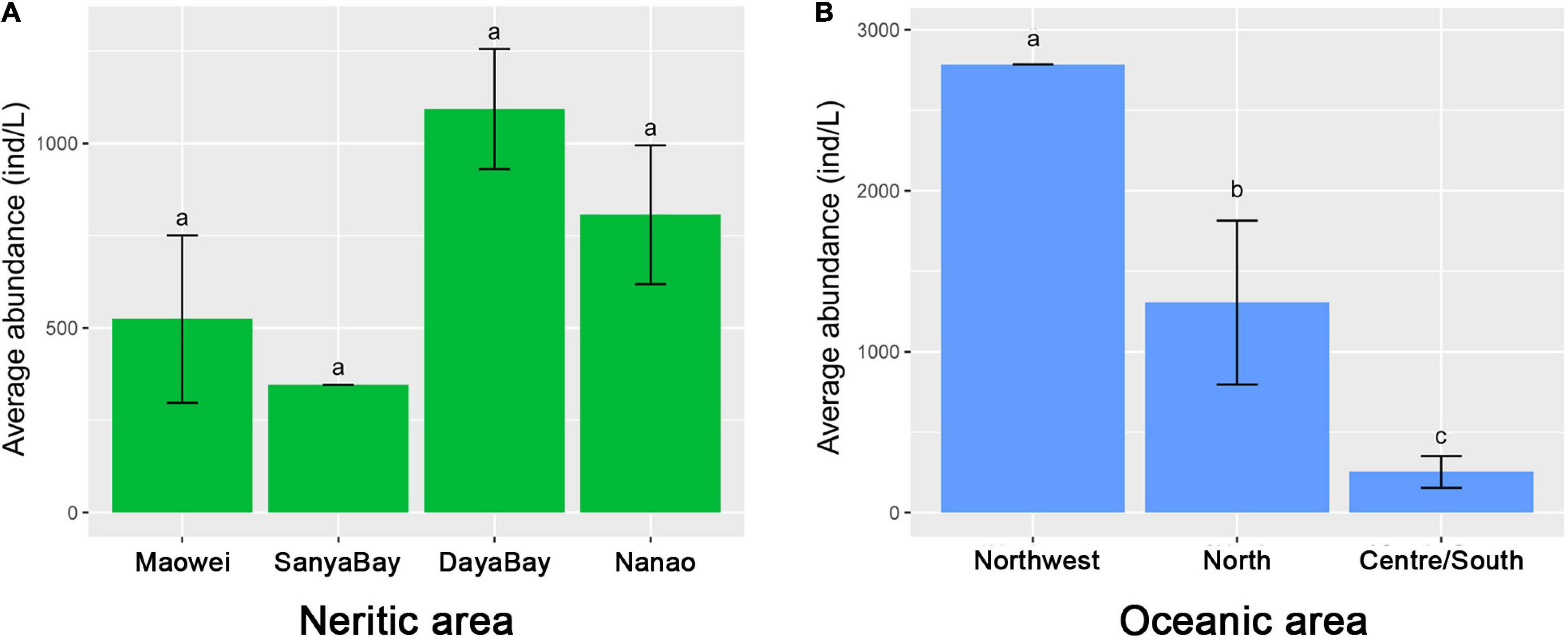
Figure 5. Comparison of ciliate abundances among the subareas in neashore and oceanic areas of SCS. (A) Average abundance of ciliates in the four subareas in neritic water. (B) Average abundance of ciliates in the four subareas in oceanic water.
For taxonomy-based communities, there were significant and positive relationships between geographical distance and dissimilarity of ciliate communities (Figure 4), while for both motility and feeding habit communities, no significant relationships were exhibited between geographical distance and dissimilarity (Figure 4).
Distribution Patterns of Ciliate Diversity in Oceanic Water of the SCS
In oceanic water, the ANOSIM analyses revealed the differences in ciliate communities among the three subareas. This is clear in terms of taxonomic composition (R = 0.588, P = 0.008, Table 1), but not significant in terms of motility and feeding habits. In the cluster analyses, the communities from the Northwest and Centre/South subareas generally form their own clades, but those from North SCS nested within other subareas (Supplementary Figure S3).
The feeding habit composition of ciliates displayed clear differences among the three subareas, i.e., the proportion of algivores gradually increased while that of non-selective omnivores decreased from Northwest to Centre/South (Figure 3C). The motility habit compositions are generally similar among the subareas (Figure 3B). For taxonomic composition, the species richness in Centre/South is distinctly lower than that in other subareas (Figure 3A). The average abundance in Centre/South is lowest among the subareas, and that in Northwest is dramatically higher than the others (Figure 5).
For communities in terms of taxonomic composition, significant positive relationships were revealed between the geographical distance and dissimilarity of ciliate communities, whereas the relationships were not significant in terms of motility and feeding habit composition (Figure 4).
Impacts of Environmental and Spatial Variables on Ciliate Communities in the SCS
The CCA/RDA ordination showed that Habitat type was the environmental factors that significantly affected all communities and subcommunities, and Chla was related to the taxonomic and feeding habit communities of the entire SCS, while Salinity was not related to either community (Supplementary Figure S4). Regarding spatial variables, no variable was found to be significantly related to intertidal community composition, while 1–3 variables were revealed to have relationships with the other communities (Supplementary Figure S4).
The VPA demonstrated that the community variation in terms of taxonomic composition for the entire SCS was related significantly to both environment and space (P < 0.05), and the environmental variables had much higher explanatory power for community variation than spatial variables (20.8% vs. 0%) (Table 2). For open water areas, VPA revealed that both environmental and spatial variables had significant (P < 0.05) influence on the communities, although the fraction of the variance explained by spatial variables is slightly higher than environmental variables (7.4% vs. 5.9%). For intertidal communities, no significant spatial variable was selected as an explanatory factor, and environmental variables alone had an explanatory power of 4.5% for community variations. Mantel tests showed consistent results with the above analyses, that the correlations between variations in ciliate communities and environmental or spatial variables in both the entire SCS and just open water were significant (P < 0.05), whereas the intertidal communities were primarily governed only by environment (Table 3).
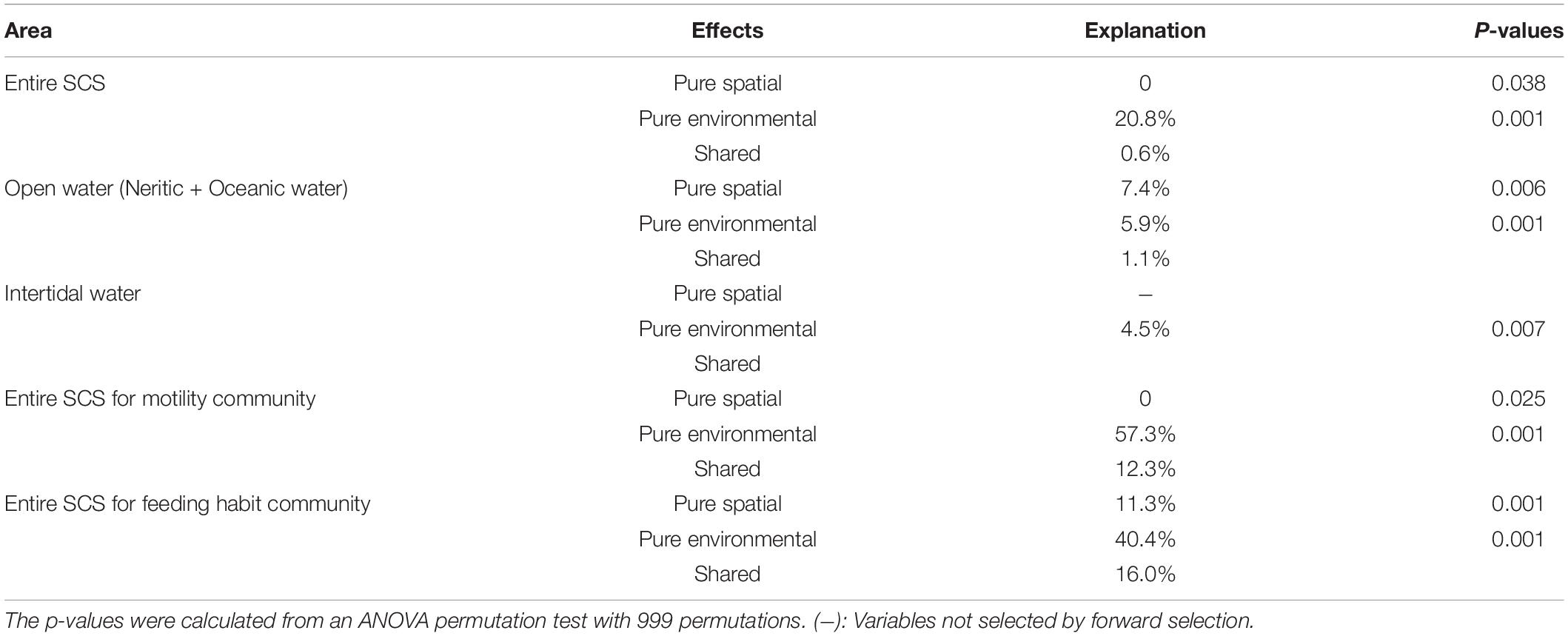
Table 2. Effects of spatial and environmental variables on the ciliate communities in different areas of SCS analyzed by redundancy analysis (RDA).
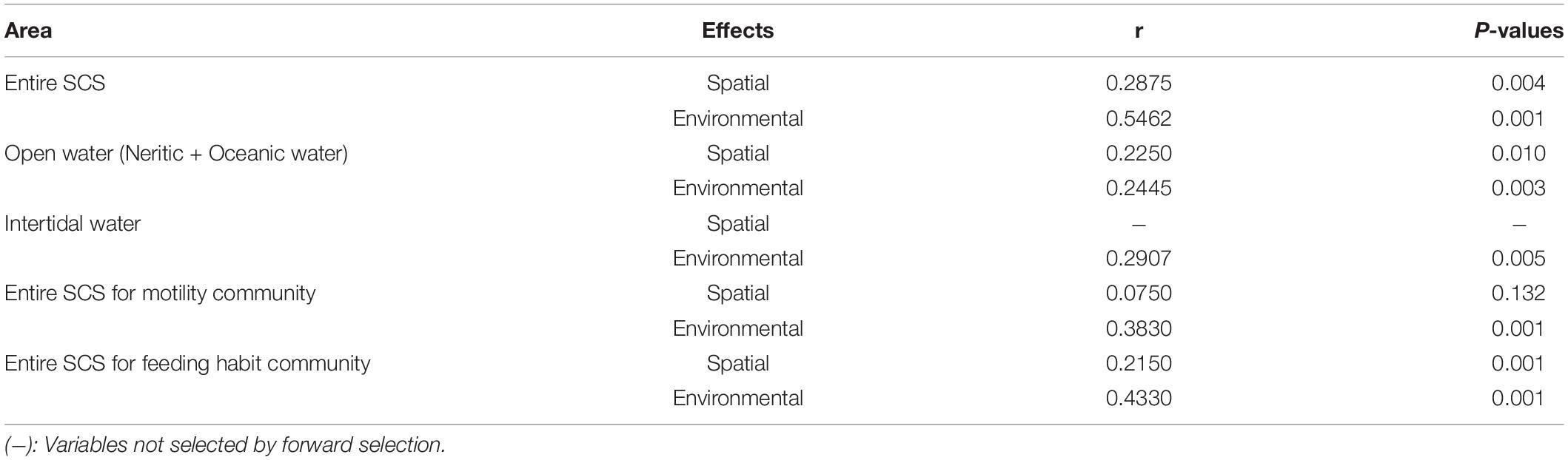
Table 3. Mantel tests for the correlation between community and spatial, environmental variables using Pearson’s coefficient.
For ciliate communities defined by motility composition, the VPA revealed that the community variations in the entire SCS are mostly determined by the environmental variables which by themselves explained up to 57.3% of the variance. The spatial variables by themselves did not explain a significant amount of the variance, although they contributed a 12.3% proportion of explanation together with environmental variables (Table 2). In Mantel tests, community variations were not significantly related to spatial variables but they were to environmental variables (Table 3). For feeding habit composition communities, the environmental variables by themselves exhibited notably higher contributions to the community variation in the entire SCS than the spatial variables did (40.4% vs. 11.3%) in the VPA, and both environmental and spatial variables were found in Mantel tests to be significantly related to the community variations.
Discussion
Comparison of Ciliate Diversity Among Intertidal, Neritic, and Oceanic Areas
Our data aggregation found 592 ciliates species recorded in the SCS based on microscropy studies, which is less than some other seas of the world. For instance, 789 planktonic ciliates have been found in the Baltic Sea (Mironova et al., 2009) and 620 in the Caspian (Alekperov, 2007). Apparently, the diversity of ciliates in the SCS is still not well studied, especially in the wide oceanic areas such as the central and southern SCS where the area accounts for 2/3 of SCS but only four investigations have been conducted (Liu et al., 2021). For the taxonomic composition of ciliates, species of the subclasses Choreotrichia and Oligotrichia represent the dominant group in the SCS (Supplementary Figure S2). This group is also found to dominate in other seas like the Yellow Sea in China, the northwest Atlantic, the south Atlantic, the Baltic Sea and, globally, in the Tara Oceans data (Mironova et al., 2009; Santoferrara and Alder, 2009; Song et al., 2009; Agatha, 2011; Gimmler et al., 2016). The high species richness in this group is not surprising, given that it is a common planktonic taxon, widely distributed in all habitats especially open waters and easily collected in surveys (Dolan et al., 2013).
The ciliate communities in intertidal, neritic and oceanic water are clearly different as identified by ANOSIM, suggesting that the variation of communities occurs across a large spatial scale in the SCS. Our further analyses revealed that the community in intertidal water is more distinctive compared with the other two areas. First, the species richness of ciliates in intertidal water was remarkably higher than in the other two areas (Figure 1A). This was in accordance with some previous studies that found alpha diversity of planktonic ciliates decreased with increasing distance from shore (Doherty et al., 2010; Tamura et al., 2011). The high ciliate diversity in intertidal water may be explained by the diverse habitats and heterogeneous environmental characters there, which thus supplies a wide range of niches for ciliates (Liu et al., 2021). Second, the composition patterns of ciliates in intertidal water differs strikingly from other areas in terms of taxonomy, motility and feeding habits (Figures 1A,C,D). Moreover, the number of species shared by intertidal and neritic or oceanic communities is very low (Figure 1B), indicating a high dissimilarity of taxonomic composition at the species level between intertidal and the other two areas. Similar results have also been found for distributions of tintinnids in the northwest Atlantic where distinct assemblage patterns were revealed between intertidal and oceanic areas, and the OTUs found in nearshore areas were nearly or completely undetectable in oceanic areas (Santoferrara et al., 2016, 2018). Third, our NMDS also showed the communities from neritic and oceanic water cluster together, and were clearly separated from intertidal communities. The notable differences in ciliate communities between intertidal and open water areas is likely due to the influence of environmental factors. It is well known that environmental properties are distinctly different between the intertidal and open water, which can create specific environmental niches, and lead to distinct ciliate community composition (Liu et al., 2021). This is confirmed by our motility and feeding habits composition patterns in the three areas. In intertidal water, the seagrasses and reefs supply extensive attachment surfaces for periphytonic (sessile and vagile) ciliates which thus dominate the community. In both neritic and oceanic areas, the open water is lacking in surfaces for attachment and hence more suitable for a planktonic lifestyle, so the proportion of planktonic species is higher than that of other groups. Meanwhile, the diverse habitats in intertidal areas supply diverse food resources for ciliates, and thus more feeding habit types can exist there compared with the neritic and oceanic areas.
It was notable that in NMDS, the samples from intertidal water are separately distributed, while those from neritic and oceanic water cluster together. Further, the community dissimilarity among samples is significantly higher in the intertidal than neritic and oceanic water in terms of taxonomic, motility, and feeding habit communities (Figure 2). Similar results were also found in previous studies on the New England Shelf, where the ciliate communities in nearshore stations were distinct from nearby ones, whereas communities are more similar among samples within midshelf and oceanic stations (Grattepanche et al., 2016a). All these observations indicate that the ciliate communities from distinct sampling sites are more variable in intertidal water compared to open water. Since intertidal waters are generally impacted by various processes such as human activities and river discharge, the environmental characters were significantly heterogeneous there and affected the distributions of ciliates, which thus leads to the patchy distribution of ciliates (Liu et al., 2021). In contrast, the waters from neritic and oceanic areas are more impacted by ocean currents, and are more homogeneous with similar hydrologic characters, and this results in relatively high similarity of communities.
Our results showed the proportion of the subclass Choreotrichia in total species number is significantly higher in both neritic and oceanic than intertidal water (Figure 1A). Since choreotrichs are mostly composed of tintinnid ciliates, this finding is consistent with some conclusions that species richness of tintinnids increased from coastal to oceanic area (Dolan et al., 2013; Li et al., 2016). Although the composition patterns of the neritic and oceanic communities are highly similar at the class level, the indicator species for these two areas revealed their composition difference at the species level (Figure 1E). Their abundances generally match with their biogeographic preferences, especially for tintinnids. For example, the indicators of neritic areas mostly consist of species of the genera Tintinnopsis, Favella, and Leprotintinnus. According to the tintinnid biogeographic categories established by Dolan et al. (2013), these three genera are classified to neritic assemblages because they are restricted to nearshore waters, and this is consistent with our results. In addition, species of the genera Rhabdonella, Epiplocyloides, and Climacocylis were identified as indicators of oceanic water in our study. According to the same tintinnid biogeographic categories (Dolan et al., 2013), these three genera belong to the warm water group. As the oceanic area of SCS located in the tropical zone with higher water temperature, our result confirms their preference for warm water. Besides, from neritic to oceanic areas the indicator tintinnids have shown a switch from species with agglutinated loricae (with mineral particles like species of Tintinnopsis and Leprotintinnus) to those with hyaline loricae (like species of Rhabdonella, Epiplocyloides, and Climacocylis). This can be explained by the finding that the agglutinated species require sufficient small mineral particles to facilitate lorica formation and are thus limited to neritic areas where the concentrations of mineral particles are high, whereas for the hyaline tintinnids the mineral particles are not needed to their lorica structuring and thus they prefer oceanic waters (Gold and Morales, 1976; Rassoulzadegan, 1980; Middlebrook et al., 1987; Lynn et al., 1991; Dolan et al., 2013).
Distribution Variations of Ciliates Among the Subareas in Intertidal, Neritic, and Oceanic Areas
In intertidal water, spatial variation of ciliate communities was not found among sampling regions in both cluster and ANOSIM analyses, but the communities grouped by habitat types were clearly separated (Supplementary Figure S3 and Table 1). Among the habitat type groupings, the composition patterns also exhibited notable variations in our study especially for feeding habit trait (Figure 3). It is widely accepted that the variation of feeding habit composition can be attributed to the responses of ciliate communities to environmental and spatial changes in marine water (Xu et al., 2018, Xu and Soininen, 2019). In our study, for example, the proportion of bacterivores in mangroves is higher than other habitats, which was apparently correlated with the higher bacteria production due to the sufficient accumulation of organic detritus in mangroves compared with other habitats. In the estuarine zone, the high inflow of nutrients from freshwater facilitates the growth of phytoplankton, resulting in the high proportion of algivores. Meanwhile, the strong mixture and interaction of river discharge and ocean intrusion in the estuarine zone gives rise to a high concentration of dissolved organic matter, which can explain the high proportion of detritivores (Figure 3C). In addition to community composition, we also found differences in species richness in the five habitat types (Figure 3A). For example, the fewest species were detected in aquaculture ponds, which agrees with most previous studies (Stoeck et al., 2018; Forster et al., 2019), and indicates the high impact of the nutrient enrichment due to the accumulation of waste food and fecal matter, as well as the ecotoxic effects of discharged medicines (Wilson et al., 2009; Burridge et al., 2010; Pawlowski et al., 2014). The mangrove wetlands possess the highest species richness among the five habitats (Figure 3A). This result is well supported by the high number of new ciliate species reported in mangrove wetlands in the past decade (Liu et al., 2017, 2019; Bai et al., 2019; Hu et al., 2019; Song et al., 2019). The reason for the high species richness in mangroves is twofold. First, the mangrove has complex environmental composition which can supply a wide range of ecological niches for ciliates. This can be confirmed by our result for the feeding habit composition (Figure 3C), in which the proportions of all feeding types were mostly even in mangroves, suggesting it is suitable for ciliates with various feeding styles. Second, the water in mangroves is rich in nutrients which leads to sufficient food.
In neritic water, the spatial variations of ciliate communities at a local scale can be clearly identified in cluster and ANOSIM analyses in terms of taxonomy. Regarding the feeding habit community composition, we found that Maowei clearly differs from other subareas by having a higher proportion of bacterivores and detritivores (Figure 3C). Maowei is located in a relatively closed bay where the water interaction with the open ocean is weak but the inflow of freshwater from rivers is strong. These processes contribute to the high concentrations of dissolved organic matter and bacteria, and thus lead to a high growth of bacterivores and detritivores (Liu et al., 2016a). The spatial variations of ciliate communities among the four neritic subareas can also be found in the species richness and abundance. It is notable that the species richness is strikingly high in Sanya Bay (Figure 3A), which can probably be attributed to the suitable habitat for ciliates supplied by extensive distribution of coral reef there (Su et al., 2008; Tan et al., 2010). The communities in Daya Bay and Nanao are higher in abundance than in other subareas (Figure 5). Aquaculture is practiced extensively in Daya Bay and Nanao, and the feed generally increases the concentration of nutrients and phytoplankton as the food source for ciliates, which thus leads to high abundance (Wu et al., 2016b, 2017, 2019).
In oceanic areas, the variation of ciliate communities among the three subareas is seen in the results of cluster and ANOSIM analyses in terms of taxonomy. In addition, the community variations also can be observed in the feeding habit composition, i.e., the proportion of algivores increased but that of other feeding types decreased from north to south (Figure 3C). Since the nutrient level is lower in oceanic water than the shelf/slope, the microplankton ecology in the southern SCS tends to be simpler and the proportion of tintinnids in ciliate assemblages becomes higher compared with those in the northern SCS (Figure 3A). Given that most tintinnids feed on phytoplankton, the high proportion of algivores in southern or central SCS is easily explained. In addition to community composition, abundance and species richness are significantly different at the local scale (Figures 3A, 5). Those values in Central/South SCS are smaller than North and Northwest SCS, suggesting the decrease in ciliate diversity from shelf/slope to the oceanic realm. The same trend was revealed in the southwestern Atlantic where the density of ciliates decreased ca. Twofold from the Argentine shelf to oceanic waters (Santoferrara and Alder, 2009, 2012). In shelf and slope areas, the environmental characters were significantly affected by coastal currents and upwelling, which carried nutrients, and thus sustained a high biomass of phytoplankton or bacteria as food for ciliates (Liu et al., 2010; Wang et al., 2014). The oceanic area is characterized by the oligotrophic and high salinity water with low concentration of Chl-a, thus explaining the low ciliate abundance and diversity.
Different Mechanisms Shaping Ciliate Communites in Intertidal and Open Waters
We found no variation among sampling regions in intertidal areas (Figure 4), but it was clearly present among habitat groupings (Supplementary Figure S3 and Table 1), which suggests that the influence of habitat types or site-specific environmental conditions on communities is stronger than that of spatial variation. This finding was supported by CCA and VPA results, in which only environmental variables showed significant contributions to the community variation in intertidal water, but no spatial variable corresponds to this variation (Supplementary Figure S4 and Table 2). In neritic and oceanic areas, by contrast, the ciliate communities from different geographic subareas displayed clear variation, and significant and positive relationships between the geographical distance and community dissimilarity were revealed (Figure 4, Supplementary Figure S3, and Table 1). Moreover, VPA showed that a larger explained fraction of the community variation was attributed to the spatial than environmental variables in the open water (Table 2).
A few studies have compared the relative influences of selective and neutral processes for assembly of microbial communities in marine ecosystems (Chen et al., 2017; Mo et al., 2018; Zhang et al., 2018). Selective processes explain the community variation by emphasizing the influence of environmental factors, while neutral processes highlight the roles of spatial factors (Hanson et al., 2012; Zhou and Ning, 2017; Zhang et al., 2018). In our study, the distinct distribution patterns of ciliates between intertidal and open (neritic and oceanic) water could be attributed to these two types of mechanisms, respectively. Our VPA results indicate that environmental selection is the major process structuring the ciliate assemblages in intertidal water, while spatial processes (dispersal) played significant roles in influencing the biogeography of ciliates in neritic and oceanic water. These findings concur with Grattepanche et al. (2016a), who found that distance to the shore has a more powerful role in structuring ciliate assemblages in oceanic water than specific features of the environment do, while the variation of some environments such as biotic factors and water circulation drove the distribution of ciliate communities in nearshore stations. Furthermore, the different mechanisms in open and intertidal waters of SCS might be ascribed to their distinct motility habit composition. In our results, the ciliate community in intertidal water was dominated by the periphytonic (sessile and vagile) group (Figure 1C). Since the movements of periphyton were limited to substrates, local environmental factors might be more important than spatial factors in shaping their biogeography. In contrast, in neritic and oceanic areas the planktonic ciliates, which were characterized by high and random dispersal rate, dominated the community, and thus the dispersal limitation contributed the most in affecting their distribution.
Comparison of Distributions of Ciliate Communities in Terms of Taxonomy, Motility, and Feeding Habits
Since ecological traits consider organisms as dynamic entities that interact with their environment, trait-based approaches make science more predictive and able to forecast ecosystem alterations occurring under rapid environmental changes (Laureto et al., 2015; Xu and Soininen, 2019). Our study provided an opportunity to evaluate potential significance of taxonomy and ecological traits in clarifying the community variation of ciliates. In our NMDS results, similar distribution patterns of the communities in SCS were found in terms of the taxonomic, motility and feeding habit compositions (Figure 2). The ANOSIM analyses showed that the community variations of ciliates among the intertidal, neritic, and oceanic areas can be clearly distinguished for all taxonomic, motility, and feeding habit compositions. Similarly, both species and trait compositions showed spatial variations of benthic ciliates along the coast of China (Xu and Soininen, 2019). These suggest that taxonomic and ecological traits can give the same result in exploring the geographical patterns of ciliates on a large scale. However, the pairwise comparison of the communities of the three areas showed that the variations between neritic and oceanic areas were not revealed by motility and feeding habits but only by taxonomy (Figure 2B and Table 1). This indicates that the environmental differences of neritic and oceanic areas have no influence to filter the ecological traits of ciliates, but produce a strong effect on their species composition assembly, which can be confirmed by the distinct indicator species in the two areas.
At a local scale, the ciliate taxonomic compositions exhibited significant differences among the habitats or subareas for intertidal, neritic and oceanic areas, while the community variations of motility and feeding habits among the subgroupings were not distinguished (Table 1). Moreover, in neritic and oceanic water, the significant and positive relationship between geographical distance and dissimilarity was exhibited for taxonomy-based communities, while no significant correlations were observed for the motility and feeding habit communities (Figure 3). Our results suggest that taxonomic traits have higher resolution than ecological traits to distinguish the community variation at the local scale. At small spatial scales, ecology trait compositions were influenced by mass effects due to the high dispersal ability of ciliates, resulting in spatially homogenized communities (Xu and Soininen, 2019).
Regarding the influence of environment and space on the community variation of ciliates in the entire SCS, VPA and Mantel tests showed similar results for both taxonomic and ecological traits—that environmental variables exhibited a higher contribution to the community variation than spatial variables did (Tables 2, 3). Moreover, the explanatory power of environmental variables alone for motility and feeding habit community variations was notably higher than for taxonomy-based community variations. Considering that our chosen traits (motility and feeding habits) are closely related to environmental conditions, it is not difficult to understand that environmental selection (habitat) must play an important role in structuring the ecological trait assemblages of ciliates. Similar findings were reported in a study on trait composition of diatom communities, with a stronger correlation with environmental variables compared to spatial factors (Soininen et al., 2016). Therefore, ecological traits are a good choice for clarifying the selective mechanisms of community variation. In addition, our RDA showed that feeding habit communities were also significantly influenced by Chla. Considering that Chla is the important indicator of food sources for ciliates, this correlation between feeding communities and Chla indicates the response of ciliates to food availability, as found in some other studies (Sun et al., 2019; Liu et al., 2021; Yang et al., 2020).
Future Prospects
The data on ciliate diversity from previous studies in our analyses were all based on morphological identification. Due to the differences among the studies in identifiers’ subjective knowledge of ciliate taxonomy and discrepancies in sample treatment (e.g., the samples were fixed with Lugol’s in some studies and formalin in others), there may be some inconsistencies of taxonomy in our data. To reduce this, species-level data were aggregated to genus level (presence/absence data) in our analyses. To eliminate this issue, future studies should be carried out uniformly as far as possible. For example, samples should be treated following standard protocols, and ciliate species identification should be done by the same investigator with professional taxonomy training or by applying molecular methods (Agatha, 2011; Santoferrara and McManus, 2017).
In addition, since environmental data in some previous studies is unavailable, the relationship between ciliate communities and environmental factors (temperature, salinity, etc.) was not analyzed in the present work. Therefore, further investigation should pay more attention to the influence of environmental factors on ciliates to clarify the deterministic processes relating to ciliate distributions in the SCS.
Data Availability Statement
The datasets presented in this study can be found in online repositories. The names of the repository/repositories and accession number(s) can be found in the article/Supplementary Material.
Author Contributions
WL and YT conceived the research. WL wrote the manuscript. GM, XL, HH, and WZ critically reviewed the findings and improved the manuscript. All authors contributed to the article and approved the submitted version.
Funding
This work was supported by the Natural Science Foundation of China (Nos. 32070517 and 31761133001), the Key Special Project for Introduced Talents Team of Southern Marine Science and Engineering Guangdong Laboratory (Guangzhou) (GML2019ZD0405), and the Science and Technology Basic Resources Investigation Program of China (No. 2017FY201404).
Conflict of Interest
The authors declare that the research was conducted in the absence of any commercial or financial relationships that could be construed as a potential conflict of interest.
Publisher’s Note
All claims expressed in this article are solely those of the authors and do not necessarily represent those of their affiliated organizations, or those of the publisher, the editors and the reviewers. Any product that may be evaluated in this article, or claim that may be made by its manufacturer, is not guaranteed or endorsed by the publisher.
Acknowledgments
We thank to Dr. Sabine Agatha (University of Salzburg) for her kind suggestions to improve the manuscript.
Supplementary Material
The Supplementary Material for this article can be found online at: https://www.frontiersin.org/articles/10.3389/fmicb.2021.689688/full#supplementary-material
Footnotes
References
Agatha, S. (2011). Global diversity of aloricate Oligotrichea (Protista Ciliophora Spirotricha) in marine and brackish sea water. PLoS One 6:e22466. doi: 10.1371/journal.pone.0022466
Alekperov, I. (2007). Fauna of free-living ciliates in Azerbaijan: modern studies and perspectives. Protistology 5:11.
Azam, F., and Malfatti, F. (2007). Microbial structuring of marine ecosystems. Nat. Rev. Micro. 5, 782–791. doi: 10.1038/nrmicro1747
Bai, Y., Wang, R., Liu, W., Warren, A., Zhao, Y., and Hu, X. (2019). Redescriptions of three tintinnine ciliates (Ciliophora: Tintinnina) from coastal waters in China based on lorica features cell morphology and rDNA sequence data. Eur. J. Protist. 72:12659.
Burridge, L., Weis, J. S., Cabello, F., Pizarro, J., and Bostick, K. (2010). Chemical use in salmon aquaculture: a review of current practices and possible environmental effects. Aquaculture 306, 7–23. doi: 10.1016/j.aquaculture.2010.05.020
Chen, C. T. A., Wang, S.-L., Wang, B.-J., and Pai, S.-C. (2001). Nutrient budgets for the South China Sea basin. Mar. Chem. 75, 281–300. doi: 10.1016/s0304-4203(01)00041-x
Chen, W., Pan, Y., Yu, L., Yang, J., and Zhang, W. (2017). Patterns and processes in marine microeukaryotic community biogeography from Xiamen coastal waters and intertidal sediments, southeast China. Front. Microbiol. 8:1912. doi: 10.3389/fmicb.2017.01912
Coppellotti, O., and Matarazzo, P. (2000). Ciliate colonization of artificial substrates in the Lagoon of Venice. J. Mar. Biol. Assoc. U.K. 80, 419–427. doi: 10.1017/s0025315400002113
De Vargas, C., Audic, S., Henry, N., Decelle, J., Mahé, F., Logares, R., et al. (2015). Ocean plankton eukaryotic plankton diversity in the sunlit ocean. Science 348:1261605.
Doherty, M., Tamura, M., Costas, B., Ritchie, M., Mcmanus, G. B., and Katz, L. A. (2010). Ciliate diversity and distribution across an environmental and depth gradient in Long Island Sound. U.S.A. Environ. Microbiol. 12, 886–898. doi: 10.1111/j.1462-2920.2009.02133.x
Dolan, J. R., and Marrasé, C. (1995). Planktonic ciliate distribution relative to a deep chlorophyll maximum: Catalan Sea, N.W. Mediterranean, June 1993. Deep Sea Res.1 Oceanogr. Res. Pap. 42, 1965–1987. doi: 10.1016/0967-0637(95)00092-5
Dolan, J. R., Montagnes, D. J. S., Agatha, S., Coats, D. W., and Stoecker, D. K. (2013). The Biology and Ecology of Tintinnid Ciliates: Models for Marine Plankton. Oxford: John Wiley & Sons, Ltd.
Dolan, J., Ritchie, M., and Ras, J. (2007). The “neutral” community structure of planktonic herbivores, tintinnid ciliates of the microzooplankton, across the SE tropical pacific ocean. Biogeosci. Discuss. 4, 561–593.
Dufrene, M., and Legendre, P. (1997). Species assemblages and indicator species: the need for a flexible asymmetrical approach. Ecol. Monogr. 67, 345–366. doi: 10.2307/2963459
Fenchel, T. (2008). The microbial loop-25 years later. J. Exp. Mar. Biol. Ecol. 366, 99–103. doi: 10.1016/j.jembe.2008.07.013
Fenchel, T., and Finlay, B. J. (2004). The ubiquity of small species: patterns of local and global diversity. Bioscience 54, 777–784. doi: 10.1641/0006-3568(2004)054[0777:tuossp]2.0.co;2
Fenchel, T., Esteban, G. F., and Finlay, B. J. (1997). Local versus global diversity of microorganisms: cryptic diversity of ciliated protozoa. Oikos 80, 220–225. doi: 10.2307/3546589
Fernandez-Leborans, G. (2001). Relative importance of protozoan functional groups in three marine sublittoral areas. J. Mar. Biol. Ass. U.K. 81, 735–750. doi: 10.1017/s0025315401004544
Fernandez-Leborans, G., and Fernandez-Fernandez, D. (2002). Protist functional groups in a sublittoral estuarine epibenthic area. Estuaries 25, 382–392. doi: 10.1007/bf02695981
Foissner, W. (1992). “Evaluation water quality using protozoa and saprobity index,” in Protocols in Protozoology. Society of Protozoologists, eds J. J. Soldo and A. T. Lee (Lawrence, KS: Society of Protozoologists). B–11.1–B–11.20.
Foissner, W., Berger, H., and Schaumburg, J. (1999). Identification and Ecology Of Limnetic Plankton Ciliates. Deggendorf: Informationsberichte Des Bayerischen Landesamtes Für Wasserwirtschaft. Deggendorf: Bayerisches Landesamt für Wasserwirtschaft.
Foissner, W., Chao, A., and Katz, L. A. (2008). Diversity and geographic distribution of ciliates (Protista: Ciliophora). Biodivers. Conserv. 17, 345–363. doi: 10.1007/s10531-007-9254-7
Forster, D., Behnke, A., and Stoeck, T. (2012). Meta-analyses of environmental sequence data identify anoxia and salinity as parameters shaping ciliate communities. Syst. Biodivers. 10, 277–288. doi: 10.1080/14772000.2012.706239
Forster, D., Filker, S., Kochems, R., Breiner, H., Cordier, T., Pawlowski, J., et al. (2019). A Comparison of different ciliate metabarcode genes as bioindicators for environmental impact assessments of salmon aquaculture. J. Eukary. Microbiol. 66, 294–308. doi: 10.1111/jeu.12670
Gimmler, A., Korn, R., Vargas, C. D., Audic, S., and Stoeck, T. (2016). The tara oceans voyage reveals global diversity and distribution patterns of marine planktonic ciliates. Sci. Rep. 6:33555.
Gold, K., and Morales, E. A. (1976). Studies on the sizes, shapes, and the development of the lorica of agglutinated Tintinnida. Biol. Bull. 150, 377–392. doi: 10.2307/1540679
Gómez, F. (2007). Trends on the distribution of ciliates in the open pacific ocean. Acta. Oecol. 32, 188–202. doi: 10.1016/j.actao.2007.04.002
Gong, J., Shi, F., Ma, B., Dong, J., Pachiadaki, M., Zhang, X., et al. (2015). Depth shapes α-and β-diversities of microbial eukaryotes in surficial sediments of coastal ecosystems. Environ. Microbiol. 17, 3722–3737. doi: 10.1111/1462-2920.12763
Grattepanche, J.-D., McManus, G. B., and Katz, L. A. (2016a). Patchiness of ciliate communities sampled at varying spatial scales along the New England Shelf. PLoS One 11:e0167659. doi: 10.1371/journal.pone.0167659
Grattepanche, J.-D., Santoferrara, L. F., McManus, G. B., and Katz, L. A. (2016b). Unexpected biodiversity of ciliates in marine samples from below the photic zone. Mol. Ecol. 25, 3987–4000. doi: 10.1111/mec.13745
Green, J. L., Bohannan, B. J., and Whitaker, R. J. (2008). Microbial biogeography:from taxonomy to traits. Science 320, 1039–1043. doi: 10.1126/science.1153475
Hanson, C. A., Fuhrman, J. A., Horner-Devine, M. C., and Martiny, J. B. H. (2012). Beyond biogeographic patterns: processes shaping the microbial landscape. Nat. Rev. Microbiol. 10:845.
Hu, X. Z., Lin, X. F., and Song, W. B. (2019). Ciliate Atlas: Species Found in the South China Sea. Beijing: Science Press.
Huang, H., Yang, J., Huang, S., Gu, B., Wang, Y., Wang, L., et al. (2021). Spatial distribution of planktonic ciliates in the western Pacific Ocean: along the transect from Shenzhen (China) to Pohnpei (Micronesia). Mar. Life Sci. Technol. 3, 103–115. doi: 10.1007/s42995-020-00075-7
Huang, L. M., Jian, W. J., Song, X. Y., Huang, X. P., Liu, S., Qian, P. Y., et al. (2004). Species diversity and distribution for phytoplankton of the Pearl River estuary during rainy and dry seasons. Mar. Pollut. Bull. 49, 588–596. doi: 10.1016/j.marpolbul.2004.03.015
Johansson, M., Gorokhova, E., and Larsson, U. (2004). Annual variability in ciliate community structure potential prey and predators in the open northern Baltic Sea proper. J. Plankton. Res. 26, 67–80. doi: 10.1093/plankt/fbg115
Langenheder, S., and Ragnarsson, H. (2007). The role of environmental and spatial factors for the composition of aquatic bacterial communities. Ecology 88, 2154–2161. doi: 10.1890/06-2098.1
Laureto, L., Cianciaruso, M., and Samia, D. (2015). Functional diversity:an overview of its history and applicability. J. Nat. Conserv. 13, 112–116. doi: 10.1016/j.ncon.2015.11.001
Leakey, R. J. G., Burkill, P. H., and Sleigh, M. A. (1996). Planktonic ciliates in the northwestern Indian Ocean: their abundance and biomass in waters of contrasting productivity. J. Plankton. Res. 18, 1063–1071. doi: 10.1093/plankt/18.6.1063
Li, H., Zhao, Y., Chen, X., Zhang, W., and Xiao, T. (2016). Interaction between neritic and warm water tintinnids in surface waters of east china sea. Deep Sea. Res. 2 Top. Stud. Oceanogr. 124, 84–92. doi: 10.1016/j.dsr2.2015.06.008
Li, J., Jiang, X., Li, G., Jing, Z., Zhou, L., Ke, Z., et al. (2017). Distribution of picoplankton in the northeastern south china sea with special reference to the effects of the kuroshio intrusion and the associated mesoscale eddies. Sci. Total Environ. 589, 1–10. doi: 10.1016/j.scitotenv.2017.02.208
Liu, H. X., Chen, M. R., Shen, P. P., Huang, H. H., Dai, M., and Qi, Z. H. (2016a). A first description of ciliate assemblages in a subtropical eutrophic bay South China Sea: species assemblage and environmental correlates. Aquat. Living Resour. 29:304. doi: 10.1051/alr/2016027
Liu, H. X., Shen, P. P., Li, C. H., Chen, Z. Z., Qi, Z. H., and Huang, H. H. (2016b). Composition and distribution of planktonic ciliates in the southern South China Sea during late summer: comparison between surface and 75 m deep layer. J. Ocean. Univ. China. 15, 171–176. doi: 10.1007/s11802-016-2712-7
Liu, H. X., Tan, Y. H., Huang, L. M., Song, X. Y., and Huang, J. R. (2010). Composition and distribution of ciliates in northern South China Sea during summer. Acta Ecol. Sin. 30, 2340–2346.
Liu, W. W., Jiang, J. M., Xu, Y., Pan, X. M., Qu, Z. S., Luo, X. T., et al. (2017). Diversity of free-living marine ciliates (Alveolata Ciliophora): faunal studies in coastal waters of China during the years 2011-2016. Eur. J. Protist. 61, 424–438. doi: 10.1016/j.ejop.2017.04.007
Liu, W. W., Shin, M. K., Yi, Z. Z., and Tan, Y. H. (2021). Progress in studies on the diversity and distribution of planktonic ciliates (Protista. Ciliophora) in the South China Sea. Mar. Life Sci. Tech. 3, 28–43. doi: 10.1007/s42995-020-00070-y
Liu, W. W., Zhang, K. X., Chen, C. Z., Li, J. Q., Tan, Y. H., Warren, A., et al. (2019). Overview of the biodiversity and geographic distribution of aloricate oligotrich ciliates (Protozoa Ciliophora Spirotrichea) in coastal waters of southern China. Syst. Biodiver. 17, 787–800. doi: 10.1080/14772000.2019.1691081
Lynn, D. H. (2008). The Ciliated Protozoa. Characterization, Classification, and Guide to the Literature. Dordrecht: Springer Verlag.
Lynn, D. H., Roff, J. C., and Hopcroft, R. R. (1991). Annual abundance and biomass of aloricate ciliates in tropical neritic waters off kingston, Jamaica. Mar. Biol. 110, 437–448. doi: 10.1007/bf01344362
Martiny, J. B. H., Eisen, J. A., Penn, K., Allison, S. D., and Horner-Devine, M. C. (2011). Drivers of bacterial b-diversity depend on spatial scale. Proc. Natl. Acad. Sci. U.S.A. 108, 7850–7854. doi: 10.1073/pnas.1016308108
McGill, B. J., Enquist, B. J., Weiher, E., and Westoby, M. (2006). Rebuilding community ecology from functional traits. Trends Ecol. Evol. 21, 178–185. doi: 10.1016/j.tree.2006.02.002
Middlebrook, K. H., Emerson, C. W., Roff, J. C., and Lynn, D. H. (1987). Distribution and abundance of tintinnids in the Quoddy region of the Bay of Fundy. Can. J. Zool. 65, 594–601. doi: 10.1139/z87-092
Mironova, E. I., Telesh, I. V., and Skarlato, S. O. (2009). Planktonic ciliates of the baltic sea (a review). Inland Water Biol. 2, 13–24. doi: 10.1134/s1995082909010039
Mo, Y., Zhang, W., Yang, J., Lin, Y., Yu, Z., and Lin, S. (2018). Biogeographic patterns of abundant and rare bacterioplankton in three subtropical bays resulting from selective and neutral processes. ISME J. 12, 2198–2210. doi: 10.1038/s41396-018-0153-6
Ota, T., and Taniguchi, A. (2003). Standing crop of planktonic ciliates in the East China Sea and their potential grazing impact and contribution to nutrient regeneration. Deep Sea Res. Pt. II 50, 423–442. doi: 10.1016/s0967-0645(02)00461-7
Pan, Y., Yang, J., McManus, G. B., Lin, S., and Zhang, W. (2020). Insights into protist diversity and biogeography in intertidal sediments sampled across a range of spatial scales. Limnol. Oceanogr. 65, 1103–1115. doi: 10.1002/lno.11375
Pawlowski, J., Esling, P., Lejzerowicz, F., Cedhagen, T., and Wilding, T. A. (2014). Environmental monitoring through protist next generation sequencing metabarcoding: assessing the impact of fish farming on benthic Foraminifera communities. Mol. Ecol. Resour. 14, 1129–1140. doi: 10.1111/1755-0998.12261
Peres-Neto, P. R., Legendre, P., Dray, S., and Borcard, D. (2006). Variation partitioning of species data matrices: estimation and comparison of fractions. Ecology 87, 2614–2625. doi: 10.1890/0012-9658(2006)87[2614:vposdm]2.0.co;2
Pierce, R., and Turner, J. (1992). Ecology of planktonic ciliates in marine food webs. Rev. Aquat. Sci. 6, 139–181.
Pierce, R., and Turner, J. (1993). Global biogeography of marine Tintinnids. Mar. Ecol. Prog. Ser. 94, 11–26. doi: 10.3354/meps094011
Pitta, P., and Giannakourou, A. (2000). Planktonic ciliates in the oligotrophic Eastern Mediterranean: vertical, spatial distribution and mixotrophy. Mar. Ecol. Prog. Ser. 194, 269–282. doi: 10.3354/meps194269
Pratt, J. R., and Cairns, J. (1985). Functional groups in the protozoa: roles in differing ecosystems. J. Protozool. 32, 415–423. doi: 10.1111/j.1550-7408.1985.tb04037.x
R Core Team (2021). R: A Language and Environment For Statistical Computing. R Foundation. Vienna, Austria. Available online at: https://www.R-project.org (accessed July 20, 2021).
Rassoulzadegan, F. (1980). Granulometric analysis of the particles used by a tintinnid Stenosemella ventricosa during lorica building. Protistology 16, 507–510.
Santoferrara, L. F., and Alder, V. A. (2012). Abundance and diversity of tintinnids (planktonic ciliates) under contrasting levels of productivity in the argentine shelf and drake passage. J. Sea Res. 71, 25–30. doi: 10.1016/j.seares.2012.04.002
Santoferrara, L. F., and McManus, G. B. (2017). Integrating dimensions of biodiversity in choreotrichs and oligotrichs of marine plankton. Eur. J. Protistol. 61, 323–330. doi: 10.1016/j.ejop.2017.04.004
Santoferrara, L. F., Grattepanche, J.-D., Katz, L. A., and McManus, G. B. (2016). Patterns and processes in microbial biogeography: do molecules and morphologies give the same answers? ISME J. 10, 1779–1790. doi: 10.1038/ismej.2015.224
Santoferrara, L. F., Rubin, E., and McManus, G. B. (2018). Global and local DNA (meta) barcoding reveal new biogeography patterns in tintinnid ciliates. J. Plank. Res. 3, 209–221. doi: 10.1093/plankt/fby011
Santoferrara, L., and Alder, V. (2009). Abundance trends and ecology of planktonic ciliates of the south-western atlantic (35-63°S): a comparison between neritic and oceanic environments. J. Plank. Res. 31, 837–851. doi: 10.1093/plankt/fbp033
Soininen, J., Jamoneau, A., Rosebery, J., and Passy, S. I. (2016). Global patterns and drivers of species and trait composition in diatoms. Glob. Ecol. Biogeogr. 25, 940–950. doi: 10.1111/geb.12452
Soininen, J., Korhonen, J. J., Karhu, J., and Vetterli, A. (2011). Disentangling the spatial patterns in community composition of prokaryotic and eukaryotic lake plankton. Limnol. Oceanog. 56, 508–520. doi: 10.4319/lo.2011.56.2.0508
Song, W. B., Warren, A., and Hu, X. Z. (2009). Free-living Ciliates in the Bohai and Yellow Seas China. Beijing: Science Press.
Song, W., Xu, D. P., Zhang, Q. Q., Liu, W. W., Warren, A., and Song, W. B. (2019). Taxonomy and phylogeny of two poorly studied genera of marine oligotrich ciliates including descriptions of two news pecies: Cyrtostrombidium paraboreale sp n and Apostrombidium orientale sp n (Ciliophora: Spirotrichea). Eur. J. Protist. 70, 1–16. doi: 10.1016/j.ejop.2019.05.001
Stoeck, T., Kochems, R., Forster, D., Lejzerowicz, F., and Pawlowski, J. (2018). Metabarcoding of benthic ciliate communities shows high potential for environmental monitoring in salmon aquaculture. Ecol. Ind. 85, 153–164. doi: 10.1016/j.ecolind.2017.10.041
Su, J. L. (2004). Overview of the South China Sea circulation and its influence on the coastal physical oceanography near the Pearl River. Cont. Shelf Res. 24, 1745–1760. doi: 10.1016/j.csr.2004.06.005
Su, Q., Huang, L. M., Tan, Y. H., Wang, H. K., Dong, J. D., Chen, Q. H., et al. (2008). Microzooplankton community composition and grazing pressure in coral reefs of Sanya Bay. Mar. Sci. Bull. 2, 28–36.
Su, Q., Huang, L. M., Tan, Y. H., Xu, R. L., Li, T., Xu, Z. Z., et al. (2007). Preliminary study of microzooplankton grazing and community composition in the north of South China Sea in autumn. Mar. Sci. Bull. 2, 43–53.
Sun, P., Huang, L. Y., Xu, D. P., Warren, A., Huang, B. Q., Wang, Y., et al. (2019). Integrated space-time dataset reveals high diversity and distinct community structure of ciliates in mesopelagic waters of the northern South China Sea. Front. Microbiol. 10:2178. doi: 10.3389/fmicb.2019.02178
Sun, P., Huang, L., Xu, D., Huang, B., Chen, N., and Warren, A. (2017). Marked seasonality and high spatial variation in estuarine ciliates are driven by exchanges between the ‘abundant’and ‘intermediate’biospheres. Sci. Rep. 7:9494.
Tamura, M., Katz, L. A., and McManus, G. B. (2011). Distribution and diversity of oligotrich and choreotrich ciliates across an environmental gradient in a large temperate estuary. Aquat. Microb. Ecol. 64, 51–67. doi: 10.3354/ame01509
Tan, Y. H., Huang, L. M., Huang, X. P., Su, Q., Shi, X., and Huang, J. R. (2010). The relationships between ciliate composition abundance and environmental factors in Sanya Bay coral reef waters. Acta Ecol. Sin. 30, 6835–6844.
Urrutxurtu, E., Orive, E., and Sota, A. D. L. (2003). Seasonal dynamics of ciliated protozoa and their potential food in an eutrophic estuary (bay of Biscay). Estuar. Coast. Shelf S. 57, 1169–1182. doi: 10.1016/s0272-7714(03)00057-x
Villéger, S., Novack-Gottshall, P. M., and Mouillot, D. (2011). The multidimensionality of the niche reveals functional diversity changes in benthic marine biotas across geological time. Ecol. Lett. 14, 561–568. doi: 10.1111/j.1461-0248.2011.01618.x
Wang, Y. B., Zhang, W. J., Lin, Y. S., Cao, W. Q., Zheng, L. M., and Yang, J. (2014). Phosphorus nitrogen and chlorophyll-a are significant factors controlling ciliate communities in summer in the northern Beibu Gulf South China Sea. PLoS One 9:e101121. doi: 10.1371/journal.pone.0101121
Wang, Y. B., Zhang, W. J., Lin, Y. S., Zheng, L. M., Cao, W. Q., and Yang, J. (2013). Spatial pattern of the planktonic ciliate community and its relationship with the environment in spring in the northern Beibu Gulf South China Sea. Oceanol. Hydrobiol. St. 42, 470–479. doi: 10.2478/s13545-013-0103-x
Wilson, A., Magill, S., and Black, K. D. (eds) (2009). “Review of environmental impact assessment and monitoring in salmon aquaculture,” in FAO. Environmental Impact Assessment and Monitoring in Aquaculture. FAO Fisheries and Aquaculture Technical Paper, eds FAO Fisheries and Aquaculture Department (Rome: FAO).
Wu, F. X., Dai, M., Huang, H. H., and Qi, Z. H. (2019). Plankton ciliate community responses to different aquatic environments in Nan’ao Island a representative mariculture base in the South China Sea. Mar. Freshw. Res. 70, 426–436. doi: 10.1071/mf18153
Wu, F. X., Huang, H. H., Huang, J. R., and Liu, H. X. (2016a). Spatial pattern of the planktonic ciliate community in spring in Baisha Bay South China Sea and its relationship with its environment. J. Trop. Ocean 3, 79–86.
Wu, F. X., Huang, H. H., Tan, Y. H., and Qi, Z. H. (2016b). Planktonic ciliate community and its relationship with environmental factors in South China Sea during autumn. Acta Sci. Nat. Univ. Sunyatseni 5, 89–95.
Wu, F. X., Huang, J. R., Dai, M., Liu, H. X., and Huang, H. H. (2016c). Using ciliates to monitor different aquatic environments in Daya Bay South China Sea. Can. J. Zool. 94, 265–273. doi: 10.1139/cjz-2015-0202
Wu, F. X., Huang, J. R., Qi, Z. H., and Huang, H. H. (2017). Spatial and seasonal variations in the planktonic ciliate community and its relationship with environmental factors in Daya Bay the South China Sea. Oceanol. Hydrobiol. St. 44, 212–222. doi: 10.1515/ohs-2017-0021
Xu, H., Min, G. S., Choi, J. K., Jung, J. H., and Park, M. H. (2009). An approach to analyses of periphytic ciliate colonization for monitoring water quality using a modified artificial substrate in korean coastal waters. Mar. Pollut. Bull. 58, 1278–1285. doi: 10.1016/j.marpolbul.2009.05.003
Xu, Y., and Soininen, J. (2019). Spatial patterns of functional diversity and composition in marine benthic ciliates along the coast of china. Mar. Ecol. Prog. 627, 49–60. doi: 10.3354/meps13086
Xu, Y., Stoeck, T., Forster, D., Ma, Z., Zhang, L., and Fan, X. (2018). Environmental status assessment using biological traits analyses and functional diversity indices of benthic ciliate communities. Mar. Pollut. Bull. 131, 646–654. doi: 10.1016/j.marpolbul.2018.04.064
Yang, J., Huang, S., Fan, W., Warren, A., Jiao, N., and Xu, D. (2020). Spatial distribution patterns of planktonic ciliate communities in the East China Sea: potential indicators of water masses. Mar. Pollut. Bull. 156:111253. doi: 10.1016/j.marpolbul.2020.111253
Yu, Y., Zhang, W. C., Cai, Y. M., Feng, M. P., Li, H. B., and Xiao, T. (2014). Distribution patterns of planktonic ciliates in the northern South China Sea in winter and summer 2009. Oceanol. Limnol. Sin. 45, 839–847.
Zhang, W. J., Pan, Y. B., Yang, J., Chen, H. H., Holohan, B., Vaudrey, J., et al. (2018). The diversity and biogeography of abundant and rare intertidal marine microeukaryotes explained by environment and dispersal limitation. Environ. Microbiol. 20, 462–476. doi: 10.1111/1462-2920.13916
Zhao, F., Filker, S., Xu, K., Huang, P., and Zheng, S. (2017). Patterns and drivers of vertical distribution of the ciliate community from the surface to the abyssopelagic zone in the western pacific ocean. Front. Microbiol. 8:2559. doi: 10.3389/fmicb.2017.02559
Keywords: biogeography, community assembly, intertidal, motility, neritic, oceanic, feeding habit
Citation: Liu W, McManus GB, Lin X, Huang H, Zhang W and Tan Y (2021) Distribution Patterns of Ciliate Diversity in the South China Sea. Front. Microbiol. 12:689688. doi: 10.3389/fmicb.2021.689688
Received: 01 April 2021; Accepted: 03 August 2021;
Published: 03 September 2021.
Edited by:
Gordon T. Taylor, Stony Brook University, United StatesReviewed by:
Bing Yang, Sichuan Academy of Giant Panda, Chengdu, ChinaDiogo Antonio Tschoeke, Federal University of Rio de Janeiro, Brazil
Copyright © 2021 Liu, McManus, Lin, Huang, Zhang and Tan. This is an open-access article distributed under the terms of the Creative Commons Attribution License (CC BY). The use, distribution or reproduction in other forums is permitted, provided the original author(s) and the copyright owner(s) are credited and that the original publication in this journal is cited, in accordance with accepted academic practice. No use, distribution or reproduction is permitted which does not comply with these terms.
*Correspondence: Yehui Tan, tanyh@scsio.ac.cn