- 1State Key Laboratory for Conservation and Utilization of Bio-resources in Yunnan, Yunnan Agricultural University, Kunming, China
- 2Qujing Branch of Yunnan Provincial Tobacco Company, Qujing, China
Tobacco leaf mildew caused by Rhizopus oryzae (Mucorales, Zygomycota) is an important and devastating post-harvest disease during flue-cured tobacco period, and also is known to cause diseases of fruits and vegetables. In this study, assessment of several candidate biological control agents were first tested in vitro to determine their antifungal activities and potential strains were further applied to tobacco leaves to prevent pathogen colonization during the tobacco-curing process. In vitro screening of 36 bacteria and the isolates of one fungus were performed for their antifungal activities against R. oryzae using dual culture method. Potential five isolates viz. Bacillus amyloliquefaciens B9601-Y2 (Y2), B. amyloliquefaciens YN201728 (YN28), Pseudomonas sp. (Pb), and B. amyloliquefaciens YN201732 (YN32) and T. harzianum B (Th-B) from total screened isolates have shown remarkable results for controlling the mycelial growth of R. oryzae. Finally, out of these five isolates, B. amyloliquefaciens B9601-Y2 potentially reduced the mycelial growth of fungal pathogen with great inhibitory effect. In order to get a better understanding of the biocontrol effect of B9601-Y2 in a flue-curing barn, various suspension density tests with two application methods involving spraying and soaking were examined. Two application methods of B. amyloliquefaciens B9601-Y2 had 98.60 and 98.15% control effects, respectively. In curing barn, the incidence in the treatment group was significantly reduced and tobacco leaves did not get mildew. Altogether, the study demonstrated that candidate bacterial strain B. amyloliquefaciens B9601-Y2 is a potential antagonist for the management of tobacco leaf mildew during flue-curing.
Introduction
Tobacco (Nicotiana tabacum L.) is one of the most important commercial crops widely cultivated in about 75 countries in many agricultural regions around the world. The tobacco industry achieved an annual output of 3 million tons in recent years (FAOSTAT, 2018). This crop is often used in cigarette blends or mixtures for pipe smoking and also a valuable medicinal plant as it has high alkaloid content. However, many post-harvest diseases such as frogeye leaf spot of tobacco (Dixon et al., 2018), blue mold (Blanco-Meneses et al., 2018), and tobacco soft rot (Wang R. et al., 2017) during production result in devastating losses. After harvesting from the field, leaves are stored for curing, redrying, aging, and fermentation, which negatively affect leaf carotenoids that slowly oxidize and importantly, cause degradation (Zhu et al., 2016; Popova et al., 2019).
During these processes, numerous diseases caused by phytopathogens such as Aspergillus, Penicillium, and Rhizopus reduce yields and monetary returns (Wang J. et al., 2017). Rhizopus oryzae causes leaf and petiole mildew and losses during flue-cured processing of tobacco leaves (Kortekamp et al., 2003; Wang et al., 2016; Pan et al., 2019). In Yunnan Province, China, the average annual loss due to diseases in curing barn is estimated to be 15–25% (Chen et al., 2020). Infection by R. oryzae usually begins at wound sites or dead cells and then gradually extends to leaves. Petiole surface covers with a dark brown mycelial mat of R. oryzae, resulting in decomposing and softening of internal tissues. The most characteristic symptoms of tobacco leaf mildew are a slimy, wet rot lesion that spreads from the rotting main petiole to the mid-veins and leaves, then appears as downy mold (Zheng et al., 2017). Disease outbreaks can occur rapidly and cause severe losses, especially under prolonged humid and warm conditions they may cause further losses through post-harvest infection in storage bins (Pan et al., 2019). Fungi that are air-borne and can produce a large number of spores, can survive for many years below 70°. The optimal growth temperature of R. oryzae ranges from 36.8 to 37.9° (Pan et al., 2019). This pathogen causes pre- and post-harvest crop losses worldwide, with a wide host range, such as banana (Kwon et al., 2012), peanut (Xu et al., 2015), sunflowers (Mathew et al., 2015; Zhou et al., 2018), sweet potato (Holmes and Stange, 2002; Wang J. et al., 2017), tomato (Khokhar et al., 2019) and yellow oleander (Cascabela thevetia) (Arif et al., 2017). The consequences of plant diseases range from major damage to minor nuisances. These problems have generated immense pressure and crisis on farmers to cut yield losses. Recently, several physical and chemical control measures have been used for the management of tobacco leaf mildew. Application of chemicals in the form of chlorothalonil, carbendazim, acid phosphorus, manganese, and zinc (Su et al., 2010); natural antimicrobials such as sodium d-isoascorbate, calcium salts, hydrogen peroxide, and azoxystrobin (Kortekamp, 2006) have been used to manage this disease. Different flue-cured tobacco varieties and the regulation of air flow in bulk curing barns (Popova et al., 2019) can also prevent and control mildew disease. However, synthetic chemicals can enhance crop productivity but also cause undesirable side effects, such as food contamination, environmental dispersal, and higher food production cost (Jiao et al., 2020). Owing to concerns about environmental contamination and harmful effects on non-target organisms, the demand for ecofriendly alternatives to chemical control has increased. Therefore, due to the widespread and refractory nature of the disease, no matter what flue-cured tobacco and storage technology is used, an alternative control of this disease is a serious challenge (Li et al., 2019; Zhang et al., 2020).
Biological control is an eco-friendly and cost-effective strategy, which can replace or integrate with chemical substances to achieve a high level of protection with sustainable production and also avoids the development of pathogen resistance (Cui et al., 2019; Jiao et al., 2020, 2021). This can be accomplished by protecting the host plant through the various biological activities of the bioagents by following appropriate application practices. Previous reports have shown that Pseudomonas aeruginosa as a biological control agent can effectively control tobacco bacterial wilt (TBW) and tobacco black shank (TBS) and even though it was found to be a good alternative to chemical fungicides (Ma et al., 2018) it has not been carried out in practice. The use of beneficial antagonists to control plant disease is a promising way to protect plants that can reduce the need for chemical substances.
In this study, we screened 37 isolates (36 bacteria and one fungus) for their antifungal activity against isolated R. oryzae by using dual culture and leaf disc assay. Antagonism—including biological control effect and microbial suspension is also studied here for disease management. We evaluated the ability of candidate biocontrol strains isolated from tobacco in the form of microbial suspension against leaf mildew disease caused by R. oryzae. In addition, we investigated candidate biocontrol strains against this pathogen under barn conditions on a large scale. Finally, the best strain B. amyloliquefaciens B9601-Y2 was used in different experiments to properly establish disease management strategies to maintain tobacco crop production with different application methods.
Materials and Methods
Tobacco Leaves and Microbial Strains
Rotten leaves of Nicotiana tabacum cv. Yunyan 105 were obtained from Geyi, Xuanwei, Yunnan Province, China in 2018 (Supplementary Figure 1). Tobacco leaves of susceptible cultivar Yunyan 105 with no signs of infection or apparent injury were also harvested from the same place (Xuanwei, Yunnan) during the commercial ripening period. Candidate microbes comprising 36 bacterial strains and 1 fungal strain (Supplementary Table 1), obtained in a previous study (Pan et al., 2019) and preserved in the Laboratory of Biocontrol and Plant Pathology (Yunnan Agricultural University, Kunming, China), were used in this study. Fungal pathogen R. oryzae was previously isolated from naturally infected tobacco leaves in Xuanwei (China). Luria Bertani broth (LB) and potato dextrose agar (PDA) culture media were used for the cultivation of bacteria and fungi, respectively.
Synergism Assay for Candidate Microbial Strains
Synergism was assessed for all the combined strains tested on LB agar plates. Burkholder’s “spot-on lawn” method (Burkholder et al., 1966) was used to screen the synergistic effect of bacterial strains. Bacterial strains were obtained from the initial stock and pre-grown on LB plates at 37° for 48 h. Then a fresh colony of each strain was dissolved in sterile saline (0.85% NaCl). Strains were confronted with each other onto LB plates to obtain a full interaction of all strains with each other. The plates were incubated for 2-3 days at 37° to check the synergistic effect of strains. Bacterial strains grown together were used for further study.
Inoculum Preparation of Candidate Microbes and Pathogen
Preserved candidate microbes were picked out by a sterile teasing needle and transferred to a fresh LB medium plate. The plate was incubated at 32°C for 72 h to allow bacterial growth. When a single bacterial colony appears, plates were kept at 4°C. A bacterial suspension was prepared by inoculating 100 mL of LB in Erlenmeyer flasks with a loopful of cells, and the flask was incubated on a rotary shaker at 180 rpm for 72 h at 37°C and diluted to different densities, and stored at 4°C.
For the pathogen, the method described by Sukhada et al. (2011) with minor modification was followed. Sterile filter paper discs with R. oryzae were cultured on a PDA medium plate containing 10 μg/mL chloramphenicol solution for 4 days. Plates were cultured at 32°C for 72 h for fungal sporulation. The 15 mL of sterile distilled water (SDW) was added to an inoculated plate to collect the spores/mycelia and the mixture was thoroughly ground. The spore suspension was carefully drawn, and adjusted to different concentrations through a hemocytometer (Sigma-Aldrich, China).
Screening Candidate Microbes for Their Antifungal Activities
Dual-Culture Antagonism Assay
Biocontrol strains preserved in the laboratory were assessed for their ability to inhibit R. oryzae on PDA medium as described previously (Sukhada et al., 2011), with some modifications. Rhizopus oryzae spore suspension was adjusted to 104 spores/mL with SDW and spores concentration was measured by a hemocytometer (Sigma-Aldrich, China). An aliquots of 100 μL spore suspension was spread on a fresh PDA plate and incubated at 32°C. After 5 h, a single candidate bacterial colony was stirred with a sterilized teasing needle and then immersed in four sites, approximately 2 cm away from the edge of the PDA plate. The plates were incubated in a growth chamber at 32 °C. Each bacterial isolate was tested three times. Antifungal potentials of Trichoderma harzianum and T. viride were evaluated as performed previously with slight modifications (Meena et al., 2017). Five days old culture of T. harzianum TH-B was used in PDA plates using the mycelial plugs (5 mm) in the center. In the same plates, tested pathogen R. oryzae was placed at the opposite side of the plates. The control plates consist of pathogen only without the application of biocontrol strains. After 2 days, the number of inhibition zones (IZ) between the fungus and the bacteria was recorded (An IZ > 0.2 cm indicated that the antagonist bacteria have an antifungal effect).
Tobacco Leaf Disc Interaction Test
In detached leaf disc assay, the healthy leaves of susceptible cultivar Yunyan 105 were chosen. The leaves were cut into small discs with a diameter of 1.5 cm and rinsed four times with SDW (1 min each disc), then left to dry at room temperature. Bacteria suspensions were diluted to 108 CFU/mL by using the plate count method as performed previously (Munir et al., 2020). Fungal pathogen suspension was prepared as described above and the concentration was adjusted to 104 spores/mL in SDW by using a hemocytometer with the addition of 0.1 g/L Tween 80. Both sides of leaf discs were sprayed by prepared spore suspension using a manual sprayer. The bacterial suspension was sprayed on both sides of the dried leaf disc and transferred to a petri dish, covered with a parafilm, and incubated in a growth chamber at 32°C for 5 h to make interaction. Bacteria were recovered from the leaf disc by washing the leaf disc with 1,000 μL of SDW. An aliquot of 200 μL of dilutions was spread on a PDA plate and incubated at 32°C for 3 days. The spore germination on the plate indicated the interaction between bacterial isolates and pathogens. The assay consisted of 10 leaf discs with three replicates.
Screening of Selected Candidate Strains for Their Biocontrol Capacity Against Tobacco Leaf Mildew in Field Curing Barns
Effect of Different Inoculum Concentrations
Five (5) biocontrol isolates viz. B. amyloliquefaciens B9601-Y2 (Y2), B. amyloliquefaciens YN201728(YN28), B. amyloliquefaciens YN201732(YN32), Pseudomonas sp. (Pb), and T. harzianum B (Th-B) with potential antifungal effects in dual culture and disc interaction from the above experiments were further applied in the curing barn located at Shimo Village, Geyi Township, and Xuanwei City, Yunnan Province. Biocontrol bacteria and fungi were prepared according to the above methods. Among them, original concentrations of the bacterial strains viz. B. amyloliquefaciens B9601-Y2 (Y2), B. amyloliquefaciens YN201728(YN28), B. amyloliquefaciens YN201732(YN32), and Pseudomonas sp. (Pb) strains was 106 CFU/mL, while T. harzianum B (Th-B) spore suspension concentration was 108 spores/mL. All biocontrol agents were applied at the original concentration and concentration of 10 fold dilution.
After collecting tobacco leaves in the field, the petioles of 30∼40 cm were soaked in different concentrations and different types of biocontrol suspensions for 10 min. Fresh tobacco leaves were taken out and stretched to air-dry naturally, then flue-cured tobacco shall follow the normal flue-cured tobacco process. The control group was kept untreated. After 7 days, disease incidence was observed and recorded. According to the above method, the disease index and control effect was calculated. In this experiment, 10 bars of tobacco leaves (100 pieces/bar) were treated with each biocontrol suspension and the experiment was repeated 3 times.
Effect of Different Microbial Mixtures and Application Methods
Further, Y2, Pb, and Th-B were selected as mixed application strains. Y2, Pb bacterial suspension, and Trichoderma Th-B spore suspension were prepared according to the above method and two of them were mixed with 1:1 to prepare Y2+Pb, Y2+Th-B, Th-B+Pb suspension. The positive control is treated by soaking in single strain suspension, while negative control was treated with SDW without soaking. Healthy tobacco petioles were first washed with SDW, sterilized on the surface by soaking in 70% ethanol for 30 s, and rinsed with sterilized water 4 times (30 s each), then dried at room temperature. Pathogen spore suspension (104 spores/mL) was sprayed onto the petiole surface. After air-drying, petioles were immersed for 10 min in single and mixed applications of different strains. The dried petioles were placed in sterilized petri dishes, sealed with plastic wrap to maintain high humidity, incubated at 32°C, and monitored routinely after every 24 h.
After the seventh day of observation, the disease grade scale (DG) was categorized from 0 to 9 based on lesion area: (0) no symptoms; (1) lesion area smaller than 5% of the total petioles area; (3) 5–25% of petioles area infected; (5) 25–50% of petioles area infected; (7) 50–75% of petioles area infected; (9) larger than 75% infected area.
The disease severity was assessed by disease rate (DR) and disease index (DI) (Alahmad et al., 2018), and relative control effect (RCE) is used to assess resistance:
Where DGmax is the maximum value of disease grades, ni is the number of petioles with each disease grade, m is the total number of rot petioles, n is the total number of petioles observed, DIc is the value of the control disease index. The 10 bars of tobacco leaves (100 pieces/bar) were treated with each biocontrol suspension and the experiment was repeated 3 times.
Evaluation of the Biocontrol Effect of Different Inoculum Concentrations of Bacillus amyloliquefaciens Strain B9601-Y2 Under Laboratory Conditions
The antifungal activity of highly effective strain B. amyloliquefaciens B9601-Y2 (Y2) at a different concentration was tested against R. oryzae on tobacco petiole. The bacterial suspension was prepared as described above. The density of biocontrol bacteria culture was adjusted to 105, 106, and 107 CFU/mL with SDW. Fungal spore inoculum was prepared by mixing 104 spores/mL spore suspension. Healthy tobacco petioles were first washed as described above. Spore suspension (104 spores/mL) was sprayed onto the petiole surface for 10 min, as mentioned above. After 3 days of observation, DI, DR, and REC were calculated. The concentration of suspension of each biological agent consisted of 10 petioles with 3 replicates for the confirmation of results.
Evaluation of the Biocontrol Effect of Bacillus amyloliquefaciens Strain B9601-Y2 in Field Curing Barns
Effect of Different Inoculum Concentrations
In 2018, pre-selected B. amyloliquefaciens Y2 was screened for its ability to reduce tobacco leaf mildew at Shimo Village, Geyi Township, and Xuanwei City, Yunnan Province. The cultivation of this crop followed the standard agronomic practices. Fresh tobacco leaves were soaked 40-50 cm, the petiole was covered with the fermentation broth of Y2 at a concentration of 105, 106, and 107 CFU/mL for 10 min before roasting. The fourth without treatment was kept as a control to assess the disease. Treatments in each curing barn consisted of 3 replicates in a completely randomized block design. Each experiment was carried out three times. Disease evaluation followed the same procedure as above.
Effect of Different Application Methods
Effective strains were selected after in vitro and in vivo bioassay, to evaluate their effectiveness under field conditions against tobacco leaf mildew during curing at Seka Village, Reshui Township, Yunnan Province. Standard agronomic practices were followed in crop cultivation. Fresh tobacco leaves were soaked (300 leaves for each concentration) in a suspension of Y2 at 105 CFU/mL or sprayed (1,000 leaves for each treatment) on the tobacco petiole top until the drop dripped. An untreated control was used as a negative test. In a completely randomized block design, treatments in each curing barn involved three replicates. Disease assessment was performed as described above after 7 days. Each experiment was carried out three times. Disease evaluation followed the same procedure as above.
Statistical Analysis
The experiment was carried out in a completely randomized design. All data were subjected to analysis of variance (ANOVA) at a 0.05 significance level, using the SPSS version 22.0 (SPSS Inc., Chicago, IL). Duncan’s multiple range test was applied to separate means. All data are expressed as the mean ± SDs from independent experiments.
Results
Lab Screening of Candidate Bacteria Against Fungal Pathogen
Antifungal Activity in vitro
Thirty-seven (37) microbial strains from our previous study (Pan et al., 2019) were inoculated on ϕ9cm PDA plates spread with 100 μL spore suspension of R. oryzae. All selected bacterial strains were also tested against each other to check synergism (Supplementary Figure 2). The results showed that most of the strains can grow easily with each other. Four (4) bacterial strains Y2, Pb, YN28, YN32, and a fungal strain T. harzianum Th-B showed antagonistic potential against R. oryzae. Initially, these five candidate isolates against R. oryzae were used to test in vitro on tobacco leaf discs. However, the antifungal effect of biocontrol bacteria was not obvious in the plate confrontation experiment (Figure 1). Bacillus amyloliquefaciens Y2 showed a very good antifungal effect against R. oryzae through the growth of mycelia on the PDA plates (Supplementary Table 1) and revealed a better antagonistic effect compared to other strains by involving a maximum reduction in leaf disc assay.
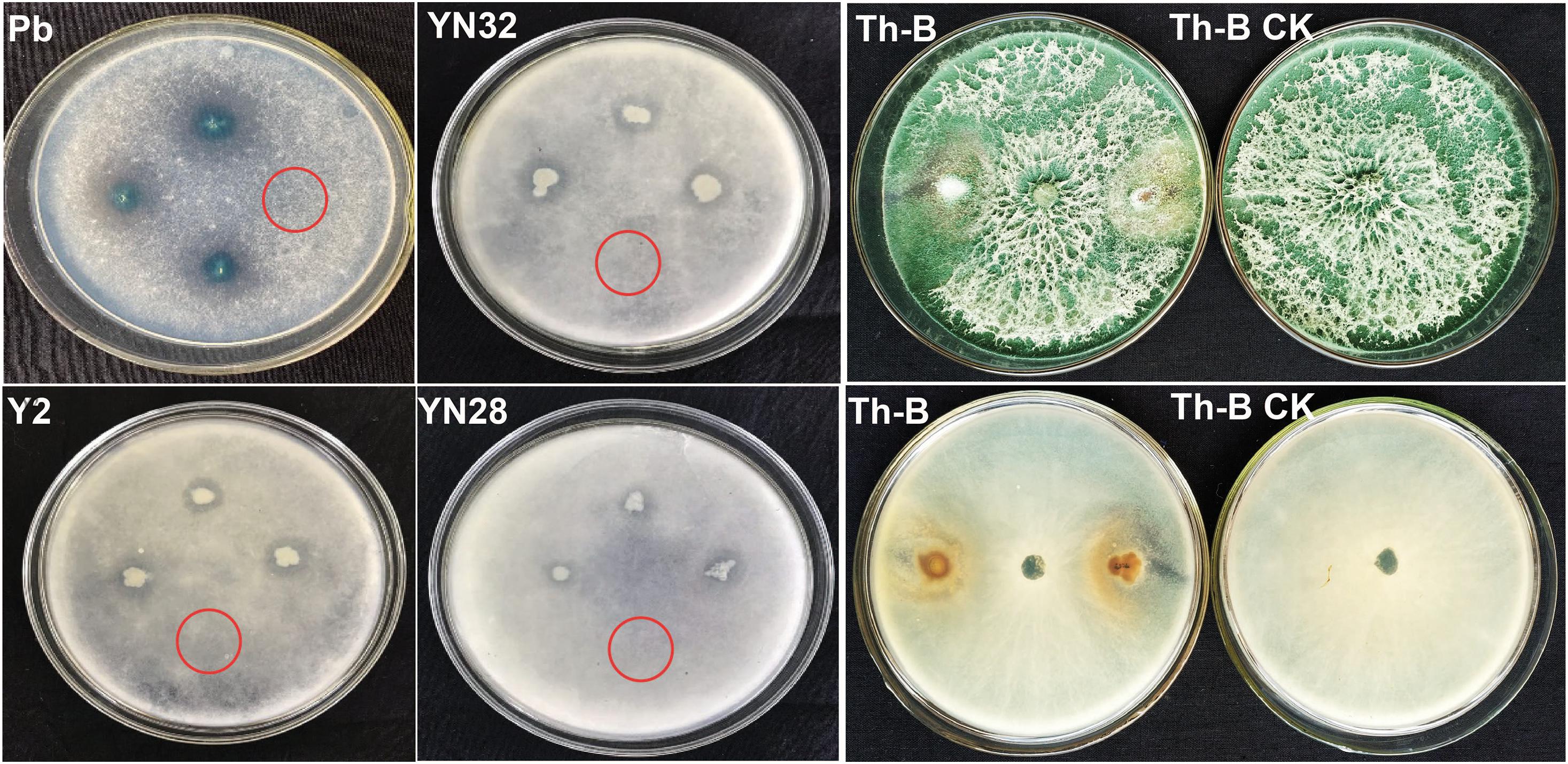
Figure 1. Lab screening of antagonist against fungal pathogen. Biocontrol agents with different inhibitory effects against Rhizopus oryzae. Pseudomonas sp. (Pb), B. amyloliquefaciens YN201732 (YN32), B. amyloliquefaciens B9601-Y2 (Y2), and B. amyloliquefaciens YN201728 (YN28) were tested in vitro. Trichoderma (Th-B) displaying antifungal activity against fungal pathogen, which occupied all the space in the plate. Inverted section of Th-B showing that the candidate strain antagonizes the pathogen. The red circle indicates that the control treatments showed no inhibition.
Tobacco Leaf Disc Interaction Test
According to the screening experiment, four strains of bacteria Y2, Pb, YN32, YN28 and one strain of Trichoderma Th-B have a certain inhibitory effect on the growth of fungal pathogen (Figure 2). Therefore, these five candidate strains were selected for the leaf disc experiment. After a period of moisturizing culture, the candidate strains on the leaf disc surface were eluted, and the plate was cultivated. Compared with the control group, R. oryzae in the eluate of leaves treated with Y2, Pb, and YN28 no longer grow. It shows that Y2, Pb, and YN28 have obvious inhibitory effects on the growth of the R. oryzae pathogen, and Y2 has the best inhibitory effect. Bacillus amyloliquefaciens YN32 and T. harzianum Th-B can also inhibit the growth of the fungal pathogen in the eluate. Therefore, these five candidate strains were selected for further field control experiments.
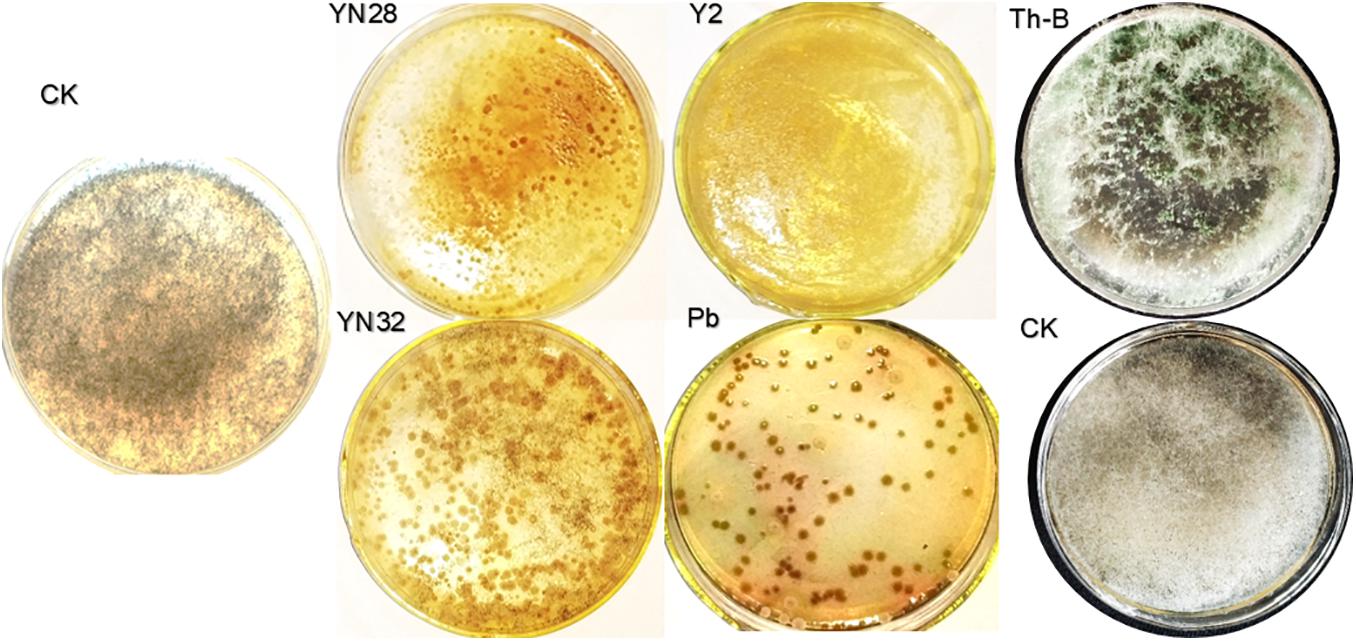
Figure 2. Tobacco leaf disc interaction test. Both sides of leaf discs were sprayed by prepared spore suspension using a manual sprayer. Spore suspension of the pathogen and candidate biocontrol strains brought in interaction in the growth chamber for 5 h. An aliquot of 200 μL of dilutions was spread on a PDA plate and incubated at 32°C for 3 days.
In vivo Evaluation of the Antifungal Activity Against Rhizopus oryzae
Confirmation of the Preventive Effect Under Curing Barn Conditions
Based on the above experimental results, the five strains were selected to verify the control effect in the curing barn. Mature tobacco leaves with petiole were collected from the field and soaked in different concentrations of various suspensions of microbial strains. Tested strains significantly affected disease incidence and R. oryzae in the same way as reported in the lab. Bacillus amyloliquefaciens Y2 and Trichoderma strain Th-B had a good control effect on tobacco leaf mildew. The strains YN28 and YN32 displayed a good control effect but these strains reduced the quality of cured tobacco leaves. After curing, the leaves soaked in YN28 and YN32 were generally green, which greatly reduced the quality of tobacco leaves. Therefore, YN28 and YN32 bacterial strains were no longer used in the following experiments (Table 1).
Biocontrol Effect With Different Application Methods
The above three screened strains viz. B. amyloliquefaciens B9601-Y2 (Y2), Pseudomonas sp. (Pb), and T. harzianum B (Th-B) were tested for mixed control. The results showed that both mixed and single application had higher than 95% control effects and the differences in control effects between different treatments were not significant (Table 2). Initially, the experiment was carried out using individual strains through a spray method and also by a combination of strains in soak methods. We found that spraying with individual strains can effectively control the disease, so further experiments were not carried out with combination during the spraying method. A low dose of candidate strain was used in curing the barn.
Effect of Various Concentrations of Bacterial Suspensions
We found that candidate strain B. amyloliquefaciens B9601-Y2 displayed the best antifungal activities against the fungal pathogen in different assays, therefore, it was chosen for further experiments. Briefly, spore suspension of R. oryzae and candidate strain B. amyloliquefaciens B9601-Y2 suspension were sprayed onto sterilized fresh tobacco discs, respectively. At the same time, the pathogen was sprayed on tobacco discs, which served as a control group. Leaves became softened and gradually spread in later stages and white hair-like mycelium appeared out of the wound. Tobacco discs were rotten and the mycelium stuck to the surface of the disc (petiole) due to high humidity. Diseased tissue emitted mildew with an odor smell and DI is 53.33%. The lesions formed in a treatment group approximately 1 day later than the control group. The infection rate was lower and the disease index was significantly reduced with higher than 95% control efficacy (Figure 3), indicating that B. amyloliquefaciens B9601-Y2 has potential in the prevention and control of tobacco leaf mildew. The disease indexes at the concentrations of 105, 106, and 107 CFU/mL of Y2 strain were 2.59, 1.48, and 0.00, respectively. The control effects are 95.14, 97.22, and 100.00%, respectively, indicating that the effect of increasing concentration on the control effect is not obvious (Table 3).
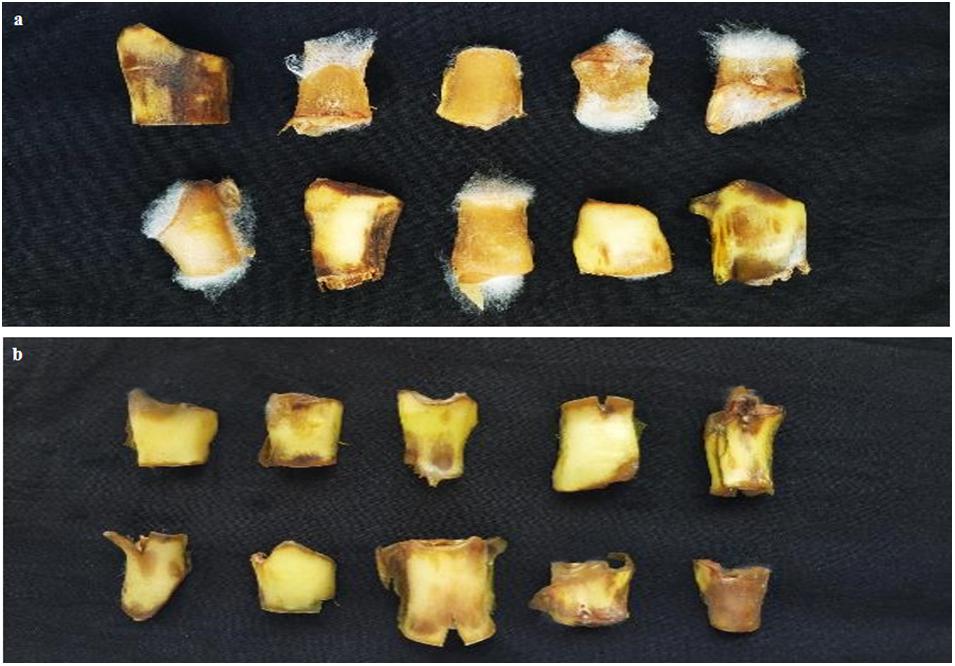
Figure 3. Effect of B. amyloliquefaciens Y2 was used as a biocontrol agent against R. oryzae in vivo. (a) The control showed white hair like mycelium of the fungal pathogen; (b) treatment with B. amyloliquefaciensY2 indicated the inhibition of pathogen). The experiment was repeated three times.
Large-Scale Evaluation of Y2 in Curing Barn
Effect of Different Concentrations on the Control Effect in Curing Barn
To clarify the effect of concentration, the experiment was conducted in a curing barn naturally infested with R. oryzae in 2018 at Geyi Township, Xuanwei City, Yunnan. In the control group, initially, water-stained spots at the petiole of tobacco appeared, then become brown and rotten. Diseased parts were shrunk and softened and then extended from the base along the leaf vein to the tip of the leaf with a large number of white hyphae on the surface. The mycelium later became gray/black and eventually moldy and decayed tobacco leaves started expanding. However, the incidence in the treatment group was significantly reduced and the tobacco leaves did not get rot (Figure 4). Fewer leaves were affected by mold and the disease level was low. The control effect of all treatments reached as high as 98%, but there was no significant difference between groups (Table 4).
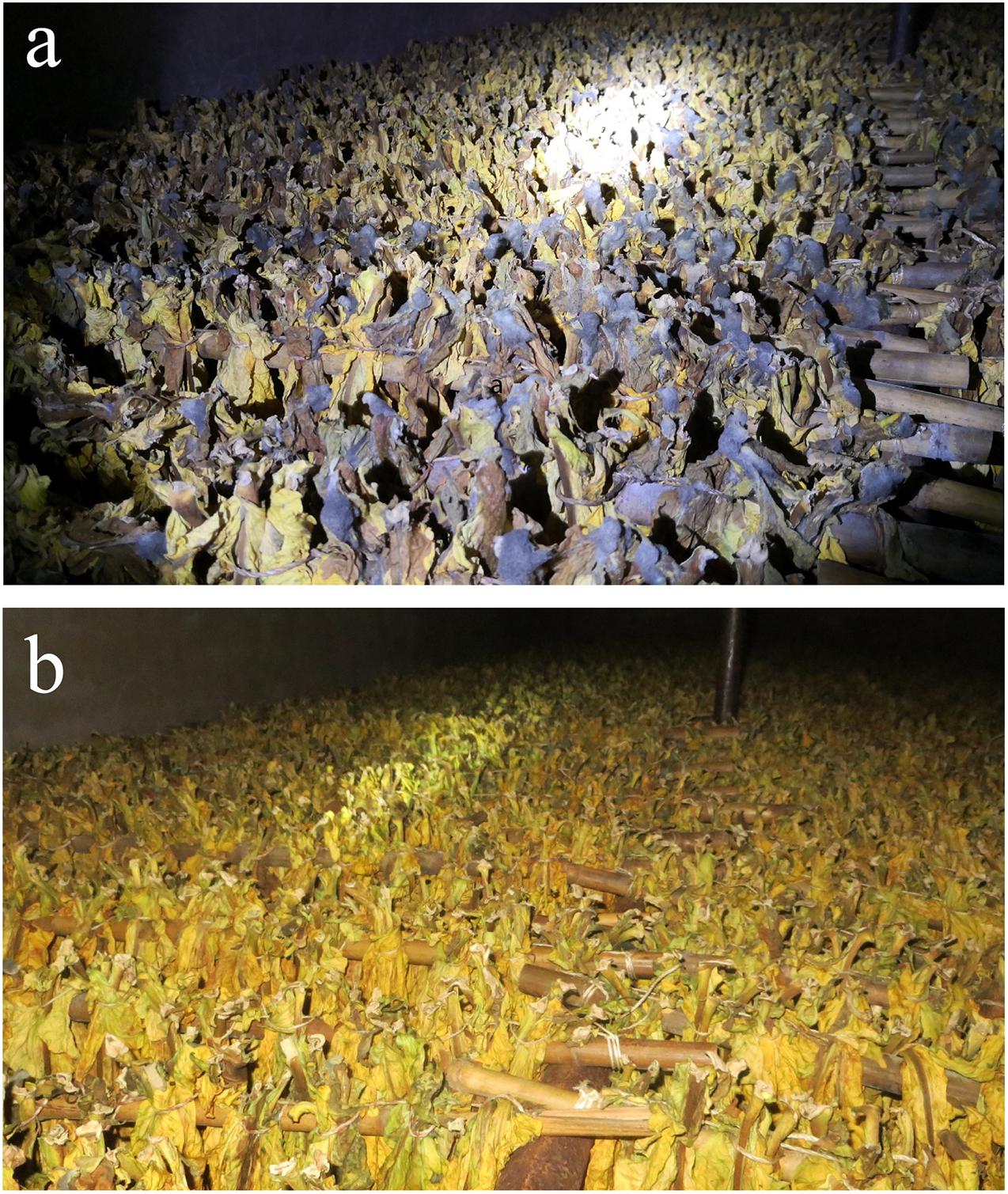
Figure 4. Inhibition of pathogen in curing barn. The experiment was conducted in field conditions naturally infested with fungal pathogen R. oryzae. (a) Infested tobacco leaves with R. oryzae. (b) Effect of B. amyloliquefaciensY2 was used as a biocontrol agent against R. oryzae under field condition.
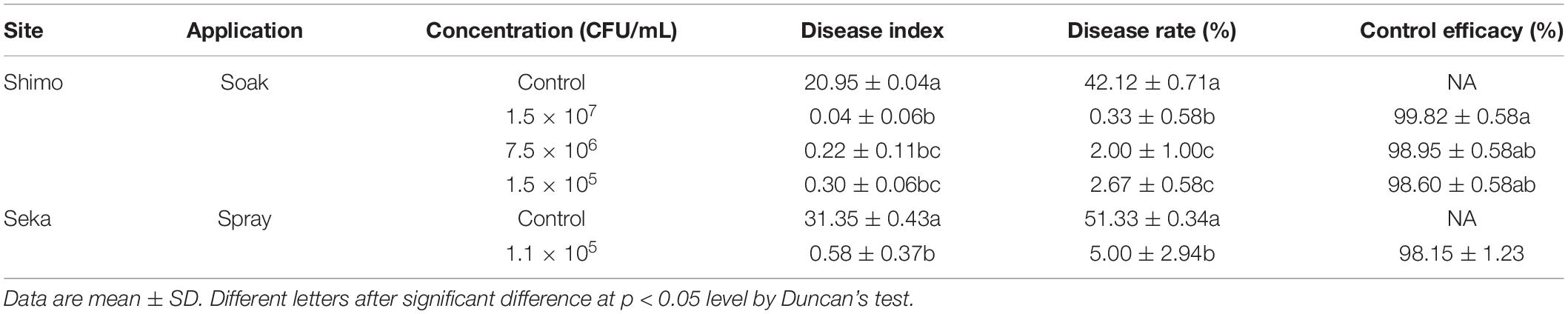
Table 4. Effect of Bacillus amyloliquefaciens Y2 on tobacco rot in curing barns (Shimo, Yunnan and Seka, Yunnan) experiments.
Effects of Different Application Methods on the Control Effect in the Field Curing Barn
Bacillus amyloliquefaciens Y2 had a satisfactory effect for controlling tobacco leaf mildew. In order to understand the effect of application methods on results, the experiment was conducted in a curing barn naturally infested with R. oryzae in 2018 at Reshui Township, Xuanwei City, Yunnan. Two application methods, spraying, and soaking, had 98.60 and 98.15% control effect, respectively, showing that there was no significant difference between the two methods (Table 4).
Discussion
Tobacco leaf mildew is commonly found throughout China where the pathogen Rhizopus oryzae can infect petioles and leaves during the flue-curing process with typical symptoms emerging at a later stage. Several physical and chemical control measures have been used to inhibit the growth of R. oryzae on tobacco leaves (Amadioha, 2001; Amadioha and Markson, 2007; Chaurasia et al., 2017; Uyar and Uyar, 2019). However, fumigation with chemicals can cause undesirable side effects, such as product contamination, environmental diffusion, and increase the production cost of goods. Despite the urgent need for effective and environmentally friendly alternatives, research is limited due to the lack of plant screening systems. To date, no significant measures have been taken to control this disease. A total of 37 candidate microbes were identified and all of these were tested to check for synergism against each other. The antagonists with inhibitory effects on each other were considered as outliers. We established that B. amyloliquefaciens B9601-Y2 is an effective biological control agent when used to soak or spray tobacco petioles in the curing barn, thereby significantly reducing the disease by 90% or above. Our previous study proved that this strain can tolerate high temperatures, i.e, temperature-tolerant during curing barn makes it one of the suitable candidates to control tobacco leaf mildew caused by R. oryzae (Pan et al., 2019).
Rhizopus has been a serious threat to a wide variety of foods stuff, fruits, and crops in agricultural and urban environments in many tropical and subtropical regions. The use of beneficial antagonistic microorganisms is a promising method of protecting plants from air-borne pathogens, which can reduce the need for chemical fungicides (Cartwright and SpurrJr., 1998; Sukhada et al., 2011; Widmer, 2014). Several microorganisms have been studied to inhibit the growth of Rhizopus. Pichia membranefaciens had a significant biocontrol effect against Rhizopus stolonifer on nectarine fruits, with an incidence rate of 60% reduction (Qing and Shiping, 2000). Zahavi et al. (2000) found two isolates, Candida guilliermondii, and Acremonium cephalosporium can control decay in grapes caused by R. stolonifera (Zahavi et al., 2000). Six antagonistic agents viz. Trichoderma asperellum, T. atroviride, T. fasciculatum, T. harzianum, T. viride, and T. virens have been found to manage the R. oryzae pathogen of tomato rot (Alka and Prajapati, 2017). Moreover, disease severity in TBW and black shank significantly reduced by Pseudomonas aeruginosa (Ma et al., 2018). In addition, three P. fluorescens isolates were used to control the postharvest disease of apple (Wallace et al., 2017) and another strain P. chlororaphis to control Ceratocystis fimbriata in postharvest sweet potatoes (Zhang et al., 2019).
Similarly, in this study, T. harizanium Th-B and Pseudomonas (Pb) also showed growth inhibition of Rhizopus on culture media and tobacco leaf discs, but their antifungal interactions or potential control effect were not examined in large-scale on plants. Therefore, further large-scale research on the biocontrol effect of Th-B and Pseudomonas Pb will be needed.
This study started with in vitro analyses of the antifungal ability of 37 isolates against R. oryzae. Five remarkable isolates showed strong antagonistic activity and were further found to be effective in inhibiting the growth of R. oryzae in curing barn. In plant assays, we showed that before the establishment of R. oryzae infection, better biological control efficiency can be obtained by spraying B9601-Y2, which provides prospects for practical applications during flue-curing. In addition, T. harzianum Th-B and Pseudomonas Pb also showed significant inhibitory effects against R. oryzae in dual-culture and tobacco leaf disc assays, indicating their potential as biocontrol agents. Moreover, isolates YN32 and YN28, isolated from tobacco seeds, can also reduce disease incidence, but also delay the yellowing process which is considered to be a decline in quality. The study also tested whether a single strain or multiple strains of biocontrol agents can better control tobacco leaf mildew in the curing barn. The results showed that there was no significant difference between the two methods. Using mixtures of strains compared to using single strains may increase the chances of host colonization. The polymicrobial approach plays a significant role in excluding devastating pathogens (De Vrieze et al., 2018). The role of using each strain in combination is important to explore the properties of individual strain (Lindemann et al., 2016). Using a single strain or combination of strains depends mainly on the performance and target pathogen. A consortium of Trichoderma and Pseudomonas displayed significant effects against blast disease, but the effectiveness was reduced against blight disease (Jambhulkar et al., 2018). Pseudomonas simiae and P. aeruginosa strains have emerged as potential candidates in agriculture for disease control (Ma et al., 2018; Pieterse et al., 2020). Plant diseases can be managed efficiently through P. aeruginosa, which displayed excellent root colonizing ability and produced a wide range of metabolites during abiotic and biotic stress (Illakkiam et al., 2013). The P. aeruginosa NXHG29 was also reported for its excellent biocontrol ability against wilt disease caused by Fusarium oxysporum f.sp. cubense on banana (Li et al., 2012). In addition, a range of soil-borne pathogens has been successfully controlled through P. aeruginosa (Singh et al., 2010; Illakkiam et al., 2013). Apart from beneficial properties, this strain must be handled with much care as this strain is also an opportunistic pathogen and may lead to bloodstream, skin and soft tissue infections, and pneumonia (Driscoll et al., 2007). Cyanide production by Pseudomonas sp. causes an inhibitory effect on plant growth (Saharan and Nehra, 2011; Parikh and Jha, 2012), for example in lettuce and barnyard grass (Kremer and Souissi, 2001), P. fluorescens suppressed the emergence of green foxtail (Setaria viridis L.) (Daigle et al., 2002).
A 4 year field study on Botrytis cinerea in grapevines using a consortium of strains showed that a combination does not work to control disease, but B. subtilis or Aureobasidium pullulans alone is effective (Pertot et al., 2017). Another important factor to be considered is the strain used to control a particular disease, i.e., isolation source, available in a culture collection or a commercial strain. Different strains always have a differential mode of action against pathogens (Hunziker et al., 2015).
In this study, the above three isolates were evaluated as potential biocontrol agents against R. oryzae. However, antagonists for commercial use must fulfill different requirements. In addition to dealing with specific target plant pathogens, they must have appropriate market size, ecological characteristics, production costs, safety, environmental risks, and the possibility of intellectual property protection (Köhl et al., 2011). On the one hand, only B. amyloliquefaciens B9601-Y2 has been studied for safety and ecology, while strain B9601-Y2 has been patented and commercially used. Hence, B. amyloliquefaciens B9601-Y2 was finally selected for large-scale evaluation in curing barn trials. The genus Bacillus is known to inhibit a variety of bacterial, fungal, and fungal-like pathogens (Belbahri et al., 2017; Liu et al., 2018). Many studies have reported that members of the Bacillus act as elicitors for the induction of systemic resistance as well as plant growth promoters (Ramírez and Kloepper, 2010; Dinesh et al., 2015; Shao et al., 2015; Islam et al., 2016; Borriss, 2020). The ability to compete with the pathogen is related to the production of secondary metabolites (Boottanun et al., 2017; Chen et al., 2019) that possess antimicrobial activities (Alenezi et al., 2015, 2016, 2017; Mefteh et al., 2017), competition for essential nutrients (Wu et al., 2012), or stimulation of the host plant immune system (Chowdhury et al., 2015).
Bacillus amyloliquefaciens B9601-Y2, isolated from the rhizosphere of wheat in North China, has been found to inhibit a broad spectrum of pathogenic fungi; promote growth and rooting of crops and vegetables; improve the drought tolerance of wheat, corn, and broad bean; reduce the number of nematodes in tomato and tobacco roots and colonize many crops (Hao et al., 2012; He et al., 2013; Scholz et al., 2014; Cui et al., 2019). The antifungal lipopeptides bacillomycin D, fengycin, siderophore bacillibactin, and dipeptide bacilysin are produced by Y2 (He et al., 2013) and could be possible disease suppressive compounds against R. oryzae. The most active antifungal lipopeptides reported in this strain were bacillomycin D and fengycin. These compounds are responsible for suppressing spore germination and mycelium of pathogenic fungi such as Fusarium oxysporum, Monilinia fructicola, and Rhizoctonia solani (Koumoutsi et al., 2004; Liu et al., 2011). It has also been used as biocontrol of numerous plant diseases caused by Bipolaris maydis, Fusarium verticillioides (Cui et al., 2015), Cochlioboluscarbonum, Curvularia lunata, and Exserohilum turcicum (Xie et al., 2017), Botrytis cinerea, Colletotrichum spp., Rhizoctonia solani, and Sclerotinia sclerotiorum (Wu et al., 2012). In the present study, B. amyloliquefaciens B9601-Y2 was demonstrated as a successful biological control agent against tobacco leaf mildew disease caused by R. oryzae by soaking and spraying during the tobacco flue-curing process. Both of methods, spraying and soaking, had 98.60 and 98.15% control effect, respectively, showing that there was no significant difference between the two methods. Soaking is the conventional delivery system, using biocontrol agents to manage plant diseases. Both of these methods might play a role in antibiosis, competition, induction of resistance, and parasitism in targeting surface pathogens only. The timing of bacterial spray is an important factor that can facilitate the development of successful applications against tobacco pathogens.
Conclusion
In summary, R. oryzae is an important fungal pathogen causing post-harvest tobacco disease during the flue-cured tobacco period and causes severe economic losses to the most economically valuable non-food crops in the world. Bacillus amyloliquefaciens B9601-Y2 effectively reduced the pathogen in vitro and under curing barn conditions on a large scale, implying it could successfully reduce the use of pesticides in tobacco crops. We conclude that both methods of spraying and soaking tobacco leaves in a biocontrol agent do not show much difference and a significant control effect was found when it was tested against pathogen R. oryzae. In addition, more research is needed to understand the most important mechanisms of B. amyloliquefaciens B9601-Y2 that can help to control this pathogen.
Data Availability Statement
The original contributions presented in the study are included in the article/Supplementary Material, further inquiries can be directed to the corresponding author/s.
Author Contributions
YH, YW, and YC conceived and designed the study and experiments. ZF, SM, YL, PBH, YX, and PH performed the experiments. ZF, YL, and PH analyzed the data. ZF, SM, and YH wrote the manuscript. All authors contributed to the final draft of the manuscript.
Funding
This research was financially supported by the Yunnan Tobacco Company ([2018]121).
Conflict of Interest
The authors declare that the research was conducted in the absence of any commercial or financial relationships that could be construed as a potential conflict of interest.
Supplementary Material
The Supplementary Material for this article can be found online at: https://www.frontiersin.org/articles/10.3389/fmicb.2021.683365/full#supplementary-material
Supplementary Figure 1 | Tobacco petioles showing symptoms in field conditions. (A,B) Petioles with Rhizopus oryzae infection are indicated by an arrow pointing to the middle.
Supplementary Figure 2 | Synergism assays for different bacterial strains. Red circle indicating two bacterial strains used together.
Supplementary Table 1 | Candidate microbial strains used in the study.
References
Alahmad, S., Simpfendorfer, S., Bentley, A. R., and Hickey, L. T. (2018). Crown rot of wheat in Australia: Fusarium pseudograminearum taxonomy, population biology and disease management. Australas. Plant Pathol. 47, 285–299. doi: 10.1007/s13313-018-0554-z
Alenezi, F. N., Rekik, I., Bełka, M., Ibrahim, A. F., Luptakova, L., Jaspars, M., et al. (2016). Strain-level diversity of secondary metabolism in the biocontrol species Aneurinibacillus migulanus. Microbiol. Res. 182, 116–124. doi: 10.1016/j.micres.2015.10.007
Alenezi, F. N., Rekik, I., Chenari Bouket, A., Luptakova, L., Weitz, H. J., Rateb, M. E., et al. (2017). Increased biological activity of Aneurinibacillus migulanus strains correlates with the production of new gramicidin secondary metabolites. Front. Microbiol. 8:517.
Alenezi, F. N., Weitz, H. J., Belbahri, L., Nidhal, J., Luptakova, L., Jaspars, M., et al. (2015). Draft genome sequence of Aneurinibacillus migulanus NCTC 7096. Genome Announc. 3, e00234–15.
Alka, P. R., and Prajapati, B. K. (2017). Effect of Trichoderma Spp. and its culture filtrate antagonists on growth and management of Rhizopus rot of tomato fruit in vitro and in vivo. J. Pharm. Phytochem. 6, 394–398.
Amadioha, A. C. (2001). Fungitoxic effects of some leaf extracts against Rhizopus oryzae causing tuber rot of potato. Arch. Phytopathol. Plant Prot. 33, 499–507. doi: 10.1080/03235400109383372
Amadioha, A. C., and Markson, A. A. (2007). Control of storage rot of cassava tuber caused by Rhizopus oryzae using some plant extracts. Arch. Phytopathol. Plant Prot. 40, 381–388. doi: 10.1080/03235400500222248
Arif, S., Liaquat, F., Khizar, M., Shah, I. H., Inam, W., Chaudhary, H. J., et al. (2017). First report of Rhizopus oryzae causing fruit rot of yellow oleander in Pakistan. Can. J. Plant Pathol. 39, 361–364. doi: 10.1080/07060661.2017.1363819
Belbahri, L., Chenari Bouket, A., Rekik, I., Alenezi, F. N., Vallat, A., Luptakova, L., et al. (2017). Comparative genomics of Bacillus amyloliquefaciens strains reveals a core genome with traits for habitat adaptation and a secondary metabolites rich accessory genome. Front. Microbiol. 8:1438.
Blanco-Meneses, M., Carbone, I., and Ristaino, J. B. (2018). Population structure and migration of the tobacco blue mold pathogen, Peronospora tabacina, into North America and Europe. Mol. Ecol. 27, 737–751. doi: 10.1111/mec.14453
Boottanun, P., Potisap, C., Hurdle, J. G., and Sermswan, R. W. (2017). Secondary metabolites from Bacillus amyloliquefaciens isolated from soil can kill Burkholderia pseudomallei. AMB Express 7, 1–11.
Borriss, R. (2020). “Phytostimulation and biocontrol by the plant-associated Bacillus amyloliquefaciens FZB42: an update,” in Phyto-Microbiome in Stress Regulation, eds M. Islam, M. Rahman, P. Pandey, C. Jha, and A. Aeron (Singapore: Springer), 1–20. doi: 10.1007/978-981-15-2576-6_1
Burkholder, P. R., Pfister, R. M., and Leitz, F. H. (1966). Production of a pyrrole antibiotic by a marine bacterium. Appl. Microbiol. 14, 649–653. doi: 10.1128/am.14.4.649-653.1966
Cartwright, D. K., and Spurr, H. W. Jr. (1998). Biological control of Phytophthora parasitica var. Nicotianae on tobacco seedlings with non-pathogenic binucleate Rhizoctonia fungi. Soil Biol. Biochem. 30, 1879–1884. doi: 10.1016/s0038-0717(98)00019-4
Chaurasia, S., Chaurasia, S., Chaurasia, A. K., Chaurasia, S., and Chaurasia, R. K. (2017). In vitro inhibitory effect of fungicides on mycelial growth of Rhizopus oryzae (Went & Prins Geerl.). Int. J. Adv. Res. Biol. Sci. 4, 176–183.
Chen, E., Zhang, M., Su, J., Sun, Z., Zhu, K., Zhou, Z., et al. (2019). Prevention and control of mildew of tobacco leaves by regulating air movement in bulk curing barns. J. Hun. Univ. Arts Sci.(Sci. Technol.) 31, 56–59.
Chen, Q. L., Cai, L., Wang, H. C., Cai, L. T., Goodwin, P., Ma, J., et al. (2020). Fungal composition and diversity of the tobacco leaf Phyllosphere during curing of leaves. Front. Microbiol. 11:2136.
Chowdhury, S. P., Hartmann, A., Gao, X., and Borriss, R. (2015). Biocontrol mechanism by root-associated Bacillus amyloliquefaciens FZB42–a review. Front. Microbiol. 6:780.
Cui, W., He, P., Munir, S., He, P., Li, X., Li, Y., et al. (2019). Efficacy of plant growth promoting bacteria Bacillus amyloliquefaciens B9601-Y2 for biocontrol of southern corn leaf blight. Biol. Control 139:104080. doi: 10.1016/j.biocontrol.2019.104080
Cui, W., He, P., Shang, J., Li, Y., Wu, Y., and He, Y. (2015). Effects of Bacillus amyloliquefaciens B9601-Y2 on diseases control and growth-promotion of maize. J. Maize Sci. 23, 153–158.
Daigle, D. J., Connick, W. J., and Boyetchko, S. M. (2002). Formulating a weed-suppressive bacterium in “Pesta” 1. Weed Technol. 16, 407–413. doi: 10.1614/0890-037x(2002)016[0407:fawsbi]2.0.co;2
De Vrieze, M., Germanier, F., Vuille, N., and Weisskopf, L. (2018). Combining different potato-associated Pseudomonas strains for improved biocontrol of Phytophthora infestans. Front. Microbiol. 9:2573.
Dinesh, R., Anandaraj, M., Kumar, A., Bini, Y. K., Subila, K. P., and Aravind, R. (2015). Isolation, characterization, and evaluation of multi-trait plant growth promoting rhizobacteria for their growth promoting and disease suppressing effects on ginger. Microbiol. Res. 173, 34–43. doi: 10.1016/j.micres.2015.01.014
Dixon, E., Kennedy, B., Pearce, R., and Pfeufer, E. (2018). Occurrence of frogeye leaf spot, caused by Cercospora nicotianae, on greenhouse tobacco transplants in Kentucky. Plant Dis. 102:1036. doi: 10.1094/pdis-10-17-1548-pdn
Driscoll, J. A., Brody, S. L., and Kollef, M. H. (2007). The epidemiology, pathogenesis and treatment of Pseudomonas aeruginosa infections. Drugs 67, 351–368. doi: 10.2165/00003495-200767030-00003
FAOSTAT (2018). Food and Agriculture Organization of the United Nations Statistical Database. Rome: FAOSTAT.
Hao, K., He, P., Blom, J., Rueckert, C., Mao, Z., Wu, Y., et al. (2012). The genome of plant growth-promoting Bacillus amyloliquefaciens subsp. plantarum strain YAU B9601-Y2 contains a gene cluster for mersacidin synthesis. J. Bacteriol. 194, 3264–3265. doi: 10.1128/jb.00545-12
He, P., Hao, K., Blom, J., Rückert, C., Vater, J., Mao, Z., et al. (2013). Genome sequence of the plant growth promoting strain Bacillus amyloliquefaciens subsp. plantarum B9601-Y2 and expression of mersacidin and other secondary metabolites. J. Bacteriol. 164, 281–291. doi: 10.1016/j.jbiotec.2012.12.014
Holmes, G. J., and Stange, R. R. (2002). Influence of wound type and storage duration on susceptibility of sweet potatoes to Rhizopus soft rot. Plant Dis. 86, 345–348. doi: 10.1094/pdis.2002.86.4.345
Hunziker, L., Bönisch, D., Groenhagen, U., Bailly, A., Schulz, S., and Weisskopf, L. (2015). Pseudomonas strains naturally associated with potato plants produce volatiles with high potential for inhibition of Phytophthora infestans. Appl. Environ. Microbiol. 81, 821–830. doi: 10.1128/aem.02999-14
Illakkiam, D., Ponraj, P., Shankar, M., Muthusubramanian, S., Rajendhran, J., and Gunasekaran, P. (2013). Identification and structure elucidation of a novel antifungal compound produced by Pseudomonas aeruginosa PGPR2 against Macrophomina phaseolina. Appl. Biochem. Biotechnol. 171, 2176–2185. doi: 10.1007/s12010-013-0469-7
Islam, S., Akanda, A. M., Prova, A., Islam, M. T., and Hossain, M. M. (2016). Isolation and identification of plant growth promoting rhizobacteria from cucumber rhizosphere and their effect on plant growth promotion and disease suppression. Front. Microbiol. 6:1360.
Jambhulkar, P. P., Sharma, P., Manokaran, R., Lakshman, D. K., Rokadia, P., and Jambhulkar, N. (2018). Assessing synergism of combined applications of Trichoderma harzianum and Pseudomonas fluorescens to control blast and bacterial leaf blight of rice. Eur. J. Plant Pathol. 152, 747–757. doi: 10.1007/s10658-018-1519-3
Jiao, R., Munir, S., He, P., Li, X., Wu, Y., Wang, J., et al. (2021). Bacillus amyloliquefaciens YN201732 produces lipopeptides with promising biocontrol activity against fungal pathogen Erysiphe cichoracearum. Front. Cell. Infect. Microbiol. 11:387.
Jiao, R., Munir, S., He, P., Yang, H., Wu, Y., Wang, J., et al. (2020). Biocontrol potential of the endophytic Bacillus amyloliquefaciens YN201732 against tobacco powdery mildew and its growth promotion. Biol. Control 143:104160. doi: 10.1016/j.biocontrol.2019.104160
Khokhar, I., Wang, J., Jia, Y., and Yan, Y. (2019). First report of Rhizopus soft rot on tomato (Lycopersicon esculentum) caused by Rhizopus oryzae in China. Plant Dis. 103, 1041–1041. doi: 10.1094/pdis-10-18-1848-pdn
Köhl, J., Postma, J., Nicot, P., Ruocco, M., and Blum, B. (2011). Stepwise screening of microorganisms for commercial use in biological control of plant-pathogenic fungi and bacteria. Biol. Control 57, 1–12. doi: 10.1016/j.biocontrol.2010.12.004
Kortekamp, A. (2006). Expression analysis of defence-related genes in grapevine leaves after inoculation with a host and a non-host pathogen. Plant Physiol. Biochem. 44, 58–67. doi: 10.1016/j.plaphy.2006.01.008
Kortekamp, A., Schmidtke, M., and Serr, A. (2003). Infection and decay of tobacco caused by Rhizopus oryzae Die Infektion und Fäulnis von Tabak verursacht durch Rhizopus oryzae. Zeitschrift für Pflanzenkrankheiten und Pflanzenschutz. J. Plant Dis. Protec. 110, 535–543.
Koumoutsi, A., Chen, X. H., Henne, A., Liesegang, H., Hitzeroth, G., Franke, P., et al. (2004). Structural and functional characterization of gene clusters directing nonribosomal synthesis of bioactive cyclic lipopeptides in Bacillus amyloliquefaciens strain FZB42. J. Bacteriol. 186, 1084–1096. doi: 10.1128/jb.186.4.1084-1096.2004
Kremer, R. J., and Souissi, T. (2001). Cyanide production by rhizobacteria and potential for suppression of weed seedling growth. Curr. Microbiol. 43, 182–186. doi: 10.1007/s002840010284
Kwon, J. H., Ryu, J. S., Chi, T. T. P., Shen, S. S., and Choi, O. (2012). Soft rot of Rhizopus oryzae as a postharvest pathogen of banana fruit in Korea. Mycobiology 40, 214–216. doi: 10.5941/myco.2012.40.3.214
Li, J., Pu, T., Ji, S., Zhang, L., Zhang, L., Duan, S., et al. (2019). Effects of biochar on three pesticide residues in flue-cured tobacco. J. Agric. Sci. Technol. (Beijing) 21, 99–106.
Li, P., Ma, L., Feng, Y. L., Mo, M. H., Yang, F. X., Dai, H. F., et al. (2012). Diversity and chemotaxis of soil bacteria with antifungal activity against Fusarium wilt of banana. J. Indust. Microbiol. Biotechnol. 39, 1495–1505. doi: 10.1007/s10295-012-1163-4
Lindemann, S. R., Bernstein, H. C., Song, H.-S., Fredrickson, J. K., Fields, M. W., Shou, W., et al. (2016). Engineering microbial consortia for controllable outputs. ISME J. 10, 2077–2084. doi: 10.1038/ismej.2016.26
Liu, C., Yang, Z., He, P., Munir, S., Wu, Y., Ho, H., et al. (2018). Deciphering the bacterial and fungal communities in clubroot-affected cabbage rhizosphere treated with Bacillus subtilis XF-1. Agric. Ecosyst. Environ. 256, 12–22. doi: 10.1016/j.agee.2018.01.001
Liu, J., Zhou, T., He, D., Li, X.-Z., Wu, H., Liu, W., et al. (2011). Functions of lipopeptides bacillomycin D and fengycin in antagonism of Bacillus amyloliquefaciens C06 towards Monilinia fructicola. J. Mol. Microbiol. Biotechnol. 20, 43–52. doi: 10.1159/000323501
Ma, L., Zhang, H. Y., Zhou, X. K., Yang, C. G., Zheng, S. C., Duo, J. L., et al. (2018). Biological control tobacco bacterial wilt and black shank and root colonization by bio-organic fertilizer containing bacterium Pseudomonas aeruginosa NXHG29. Appl. Soil Ecol. 129, 136–144. doi: 10.1016/j.apsoil.2018.05.011
Mathew, F. M., Prasifka, J. R., Gaimari, S. D., Shi, L., Markell, S. G., and Gulya, T. J. (2015). Rhizopus oryzae associated with Melanagromyza splendida and stem disease of sunflowers (Helianthus annuus) in California. Plant Health Prog. 16, 39–42. doi: 10.1094/php-rs-14-0042
Meena, M., Swapnil, P., Zehra, A., Dubey, M. K., and Upadhyay, R. (2017). Antagonistic assessment of Trichoderma spp. by producing volatile and non-volatile compounds against different fungal pathogens. Arch. Phytopathol. Plant Protec. 50, 629–648. doi: 10.1080/03235408.2017.1357360
Mefteh, F. B., Daoud, A., Chenari Bouket, A., Alenezi, F. N., Luptakova, L., Rateb, M. E., et al. (2017). Fungal root microbiome from healthy and brittle leaf diseased date palm trees (Phoenix dactylifera L.) reveals a hidden untapped arsenal of antibacterial and broad spectrum antifungal secondary metabolites. Front. Microbiol. 8:307.
Munir, S., Li, Y., He, P., Huang, M., He, P., He, P., et al. (2020). Core endophyte communities of different citrus varieties from citrus growing regions in China. Sci. Rep. 10:3648.
Pan, Z., Cai, Y., He, P., Yu, Y., Xie, J., Wu, Y., et al. (2019). Isolation, identification and characterization of the pathogen of tobacco leaf mold during flue-curing. Chin. Tobacco Sci. 40, 42–47.
Parikh, K., and Jha, A. (2012). Biocontrol features in an indigenous bacterial strain isolated from agricultural soil of Gujarat. India J. Soil Sci. Plant Nutr. 12, 245–252. doi: 10.4067/s0718-95162012000200004
Pertot, I., Giovannini, O., Benanchi, M., Caffi, T., Rossi, V., and Mugnai, L. (2017). Combining biocontrol agents with different mechanisms of action in a strategy to control Botrytis cinerea on grapevine. Crop Protec. 97, 85–93. doi: 10.1016/j.cropro.2017.01.010
Pieterse, C. M., Berendsen, R. L., De Jonge, R., Stringlis, I. A., Van Dijken, A. J., Van Pelt, J. A., et al. (2020). Pseudomonas simiae WCS417: star track of a model beneficial rhizobacterium. Plant Soil 461, 245–263. doi: 10.1007/s11104-020-04786-9
Popova, V., Ivanova, T., Prokopov, T., Nikolova, M., Stoyanova, A., and Zheljazkov, V. D. (2019). Carotenoid-related volatile compounds of tobacco (Nicotiana tabacum L.) essential oils. Molecules 24:3446. doi: 10.3390/molecules24193446
Qing, F., and Shiping, T. (2000). Postharvest biological control of Rhizopus rot of nectarine fruits by Pichia membranefaciens. Plant Dis. 84, 1212–1216. doi: 10.1094/pdis.2000.84.11.1212
Ramírez, C. A., and Kloepper, J. W. (2010). Plant growth promotion by Bacillus amyloliquefaciens FZB45 depends on inoculum rate and P-related soil properties. Biol. Fertil. Soils 46, 835–844. doi: 10.1007/s00374-010-0488-2
Saharan, B., and Nehra, V. (2011). Plant growth promoting rhizobacteria: a critical review. Life Sci. Med. Res. 21, 1–30.
Scholz, R., Vater, J., Budiharjo, A., Wang, Z., He, Y., Dietel, K., et al. (2014). Amylocyclicin, a novel circular bacteriocin produced by Bacillus amyloliquefaciens FZB42. J. Bacteriol. 196, 1842–1852. doi: 10.1128/jb.01474-14
Shao, J., Xu, Z., Zhang, N., Shen, Q., and Zhang, R. (2015). Contribution of indole-3-acetic acid in the plant growth promotion by the rhizospheric strain Bacillus amyloliquefaciens SQR9. Biol. Fertil. Soils 51, 321–330. doi: 10.1007/s00374-014-0978-8
Singh, N., Kumar, S., Bajpai, V. K., Dubey, R., Maheshwari, D., and Kang, S. C. (2010). Biological control of Macrophomina phaseolina by chemotactic fluorescent Pseudomonas aeruginosa PN1 and its plant growth promotory activity in chir-pine. Crop Protec. 29, 1142–1147. doi: 10.1016/j.cropro.2010.04.008
Su, J., Yang, C., Mi, J., Zhang, G., and Lu, Z. (2010). Effects of chemicals for controlling mildew in petioles of tobacco cultivar Honghuadajinyuan during flue-curing. Acta Tabacaria Sin. 16, 64–66.
Sukhada, M., Manjula, R., and Rawal, R. D. (2011). Evaluation of Arbuscular mycorrhiza and other biocontrol agents against Phytophthora parasitica var. nicotianae infecting papaya (Carica papaya cv. Surya) and enumeration of pathogen population using immunotechniques. Biol. Control 58, 22–29. doi: 10.1016/j.biocontrol.2011.03.013
Uyar, G. E. O., and Uyar, B. (2019). Effects of ethanol and ultraviolet-C treatments on inactivation of Rhizopus oryzae spores which cause postharvest rot. Food Sci. Technol. 39, 691–695. doi: 10.1590/fst.04618
Wallace, R. L., Hirkala, D. L., and Nelson, L. M. (2017). Postharvest biological control of blue mold of apple by Pseudomonas fluorescens during commercial storage and potential modes of action. Postharvest Biol. Technol. 133, 1–11. doi: 10.1016/j.postharvbio.2017.07.003
Wang, H. C., Huang, Y. F., Tang, X. G., Cai, L. T., Chen, Q. Y., Wang, M. S., et al. (2016). Leaf and stem rot of tobacco (Nicotiana tabacum) caused by Rhizopus oryzae in closed curing barns in Guizhou Province of China. Plant Dis. 100, 536–536. doi: 10.1094/pdis-08-15-0935-pdn
Wang, J., Wang, Y., Zhao, T., Dai, P., and Li, X. (2017). Characterization of the pathogen causing a new bacterial vein rot disease in tobacco in China. Crop Protec. 92, 93–98. doi: 10.1016/j.cropro.2016.10.025
Wang, R., Gao, B., Chen, S., Ma, J., Li, X., and Xue, C. (2017). First report of Rhizopus oryzae causing soft rot on storage roots of sweet potato in China. Plant Dis. 101:1039. doi: 10.1094/pdis-09-16-1272-pdn
Widmer, T. L. (2014). Screening Trichoderma species for biological control activity against Phytophthora ramorum in soil. Biol. Control 79, 43–48. doi: 10.1016/j.biocontrol.2014.08.003
Wu, X., Wu, Y., Zhao, Z., Rainer, B., Mao, Z., Chen, X., et al. (2012). Effects of seed mixing with 4 PGPR strains on growth and yield of broad bean under drought conditions. Arid Zone Res. 29, 203–207.
Xie, L., He, P., Wu, Y., Xiao, C., Yi, J., and He, Y. (2017). Control effects of Bacillus amyloliquefaciens B9601-Y2 on corn leaf spots. J. Maize Sci. 25, 130–135.
Xu, M. L., Yang, J. G., Wang, F. L., Wu, J. X., and Chi, Y. C. (2015). First report of Rhizopus arrhizus (syn. R. oryzae) causing root rot of peanut in China. Plant Dis. 99:1448. doi: 10.1094/pdis-02-15-0235-pdn
Zahavi, T., Cohen, L., Weiss, B., Schena, L., Daus, A., Kaplunov, T., et al. (2000). Biological control of Botrytis, Aspergillus and Rhizopus rots on table and wine grapes in Israel. Postharvest Biol. Technol. 20, 115–124. doi: 10.1016/s0925-5214(00)00118-6
Zhang, Q., Geng, Z., Li, D., and Ding, Z. (2020). Characterization and discrimination of microbial community and co-occurrence patterns in fresh and strong flavor style flue-cured tobacco leaves. Microbiol. Open 9:965.
Zhang, Y., Li, T., Liu, Y., Li, X., Zhang, C., Feng, Z., et al. (2019). Volatile organic compounds produced by Pseudomonas chlororaphis subsp. aureofaciens SPS-41 as biological fumigants to control Ceratocystis fimbriata in postharvest sweet potatoes. J. Agric. Food Chem. 67, 3702–3710. doi: 10.1021/acs.jafc.9b00289
Zheng, S., Wu, C., Bao, Y., and Wang, Y. (2017). Influential parameters analysis on heating performance of heat pump in baking process. Procedia Eng. 205, 810–817. doi: 10.1016/j.proeng.2017.10.015
Zhou, H., Wang, D., Zhao, J., Dong, B., Zhang, X., Wen, C., et al. (2018). First report of Rhizopus head rot of sunflower caused by Rhizopus arrhizus (syn. R. oryzae) in Xinjiang and Gansu provinces of China. Plant Dis. 102, 1173–1173. doi: 10.1094/pdis-10-17-1528-pdn
Keywords: Bacillus, fungi, Nicotiana tabacum, Rhizopus oryzae, tobacco
Citation: Pan Z, Munir S, Li Y, He P, He P, Wu Y, Xie Y, Fu Z, Cai Y and He Y (2021) Deciphering the Bacillus amyloliquefaciens B9601-Y2 as a Potential Antagonist of Tobacco Leaf Mildew Pathogen During Flue-Curing. Front. Microbiol. 12:683365. doi: 10.3389/fmicb.2021.683365
Received: 20 March 2021; Accepted: 17 May 2021;
Published: 14 July 2021.
Edited by:
Samantha Chandranath Karunarathna, Key Laboratory for Plant Diversity and Biogeography of East Asia, Kunming Institute of Botany, Chinese Academy of Sciences, ChinaReviewed by:
Kasun Thambugala, University of Sri Jayewardenepura, Sri LankaChayanard Phukhamsakda, Jilin Agriculture University, China
Copyright © 2021 Pan, Munir, Li, He, He, Wu, Xie, Fu, Cai and He. This is an open-access article distributed under the terms of the Creative Commons Attribution License (CC BY). The use, distribution or reproduction in other forums is permitted, provided the original author(s) and the copyright owner(s) are credited and that the original publication in this journal is cited, in accordance with accepted academic practice. No use, distribution or reproduction is permitted which does not comply with these terms.
*Correspondence: Yongzhan Cai, eW9uZ3poYW5jYWkyMDEzQDE2My5jb20=; Yueqiu He, eW5maDIwMDdAMTYzLmNvbQ==
†These authors have contributed equally to this work