- 1Department of Clinical Laboratory, The First People’s Hospital of Guiyang, Guiyang, China
- 2Laboratory of Bacterial Infectious Disease of Experimental Center, Guizhou Provincial Centre for Disease Control and Prevention, Guiyang, China
- 3School of Basic Medical Sciences, Guizhou Medical University, Guiyang, China
- 4Central Laboratory of the Second Affiliated Hospital, Guizhou University of Traditional Chinese Medicine, Guiyang, China
Candida tropicalis is an increasingly opportunistic pathogen that causes serious invasive candidiasis threatening a patient’s life. Traditional methods to detect C. tropicalis infection depends on time-consuming, culture-based gold-standard methods. So, we sought to establish a new method that could detect target pathogens quickly, accurately, and straightforwardly. Herein, a combination of multiple cross displacement amplification (MCDA) and lateral flow biosensors (LFB) was employed to detect C. tropicalis. In the MCDA system, 10 primers were designed to identify the specific genes of C. tropicalis and amplify the genes in an isothermal amplification device. Then, MCDA amplification reaction products could be identified visibly by color change, and all the amplification products would be tested by LFB with no special equipment. The results demonstrated that the optimal reaction condition of C. tropicalis-MCDA assay was 64°C within 30 min, and only 10 fg DNA was required in each reaction. No cross-reaction was found between C. tropicalis strains and non-C. tropicalis strains. For 300 sputum samples, the results showed that MCDA-LFB assay could rapidly and successfully detect all of the C. tropicalis-positive (28/300) samples detected by the gold-standard method. The entire procedure, including specimen processing (40 min), isothermal reaction (30 min) and result reporting (within 2 min), could be completed within 75 min. Briefly, the study results demonstrated that the detection ability of C. tropicalis-MCDA-LFB assay was better than culture methods with more simplicity, rapidity, sensitivity and specificity. Hence, MCDA-LFB strategy is an effective tool to rapidly detect C. tropicalis in clinical samples, especially in resource-poor areas.
Introduction
Invasive candidiasis is a well-known life-threatening disease and a major issue for a medical institution, leading to significant morbidity, mortality, and huge extra hospital costs (Fuchs et al., 2019; Jenks et al., 2020). Regarding epidemiological data, invasive candidiasis was ranked the fourth leading nosocomial infectious disease with an overall mortality rate of 40% (McCarty and Pappas, 2015). Although Candida albicans is the most common species cause of invasive candidiasis, C. tropicalis has become an ascendent non-albicans Candida species causing invasive candidiasis worldwide, and its prevalence varies across geographic regions (Jagdish et al., 2013; Arrua et al., 2015; Chakrabarti et al., 2015). Compared to other non-C. tropicalis Candida species, C. tropicalis is stated to be more clinically indistinguishable and differently resistant to antifungal drugs. Besides, some previous studies indicated that invasive candidiasis caused by C. tropicalis have higher mortality compared to those caused by other non-tropicalis Candida species (Wang et al., 2016a; Fan et al., 2017). C. tropicalis is a major opportunistic human pathogenic yeast that is prevalent in nature and can be found in the skin, vagina, mouth and digestive tract of healthy people (Patil et al., 2018; Wang Q. et al., 2020). When patients with low immunity, especially those with neutropenia, leukemia, tumors, and bone marrow transplants are susceptible to C. tropicalis, this causes various clinically relevant infections, including candidiasis and systemic disseminated infections resulting in significant morbidity and mortality (Wang et al., 2016b; Horváth et al., 2020).
While antifungal drugs can be employed to treat Candida infections, mortality rates continue to rise (Ala-Houhala et al., 2019; Arastehfar et al., 2020). Furthermore, the epidemiology change of invasive candidiasis is partly attributed to overusing antifungal drugs, and some studies suggested that C. tropicalis appears to be resistant to azole antifungals, especially fluconazole, compared to other Candida species (Chakrabarti et al., 2009; Xiao et al., 2015; Chen et al., 2019). With the emergence of antifungal resistance and epidemiology change among Candida species, rapid and accurate fungi detection is required to optimize antifungal therapy.
So far, C. tropicalis can be detected by the commonly used culture and several non-culture methods (Chai et al., 2010). However, 3–7 days are needed to isolate and identify, C. tropicalis isolates using conventional culture method, which is considered the gold-standard method. In order to improve the detection duration and sensitiveness of laboratory diagnosis. Several non-culture methods have been developed for C. tropicalis detection, including polymerase-chain-reaction (PCR) assays and real-time PCR (Ogata et al., 2015; Guo et al., 2016). However, these methods require expensive laboratory equipment and highly skilled professionals, which are not available in resource-limited settings (Avni et al., 2011). On the other hand, microscopic examination is inaccurate, and traditional culture-based methods require at least 72 h, leading to optimal treatment time loss. Compared with other methods, multiple cross displacement amplification (MCDA) is a novel nucleic acid amplification technique under isothermal conditions, which avoid many complicated steps and expensive instruments (Wang et al., 2015). Besides, it only takes 1 h to complete gene amplification with color change (Wang J. et al., 2020). The amplification products can be tested using gold nanoparticle-based lateral flow biosensors (LFB), a new method to detect microbial specific gene fragments (Wang et al., 2016c).
Herein, we aim to develop a rapid and straightforward MCDA-LFB assay using specific primers targeting the internal transcribed spacer II (ITS II) gene of C. tropicalis for detecting C. tropicalis strains. The optimal conditions, analytical sensitivity, specificity, and feasibility of this method were validated utilizing pure cultures and clinical samples.
Materials and Methods
Reagents and Instruments
The kit Qiagen QIAamp DNA mini kit (Qiagen, Beijing, China) was used to extract genomic DNA from fungi, bacterial and clinical. Isothermal amplification kits and colorimetric indicator (malachite green, MG) were provided by Beijing-Hai Tai Zheng Yuan Technology Co., Ltd. (Beijing, China). Dye streptavidin-coated polymer nanoparticles (Crimson red) were obtained from Bangs Laboratories, Inc. (Indiana, United States). Anti-FAM (rabbit anti-fluorescein antibody) and biotin-BSA (biotinylated bovine serum albumin) were obtained from Abcam. Co., Ltd. (Shanghai, China). Polymer nanoparticle-based lateral flow biosensor (LFB) materials, including sample pad, nitrocellulose membrane (NC), absorbent pad, conjugate pad and backing card were obtained from Jie-Yi Biotechnology. Co., Ltd. (Shanghai, China). Primers and labeled primers (Table 2 and Supplementary Table 1) used were synthesized by Beijing-Tsingke Biotechnology Co., Ltd. (Beijing, China).
Fungi, Bacteria Strains, Clinical Specimens, and Genomic DNA Preparation
A total of 37 fungi strains and 5 bacterial strains were utilized (Table 1), including 23 C. tropicalis and 14 non-C. tropicalis fungi strains. Except for C. tropicalis standard strain (ATCC13803) and C. albicans strain (ATCC10231), all the other strains were isolated from The First People’s Hospital of Guiyang, identified by culture and biochemical methods. All pure strains with 15% (w/v) glycerol broth were stored at −70°C. Candida strains were inoculated in the sabouraud dextrose agar (SDA) plate at 30°C, while bacteria strains were inoculated in the nutrient agar plate at 36°C. After 48 h of pure culture, all colonies were collected separately. To test the applicability of the C. tropicalis MCDA-LFB assay to clinical samples, 300 sputum samples were collected from June 1st to October 1st 2020 in the clinical microbiology laboratory, The First People’s Hospital of Guiyang. The protocol was approved by the Ethics Committee of The First People’s Hospital of Guiyang. Patients who provided sputum samples gave written informed consent, in accordance with the Declaration of Helsinki.
DNA was extracted from each strain using a QIAamp DNA extraction kit (Qiagen, Beijing, China) following manufacturer’s instructions, and concentration and purity were measured with a Nanodrop 2000 (Beijing, China) at A260/280. DNA from C. tropicalis (ATCC13803) was employed as a positive control to confirm performance and choose optimal temperature and sensitivity, while DNA from C. albicans (ATCC10231) was deployed as a negative control. For clinical samples, DNA was extracted from 200 μL of the sputum sample using the QIAamp DNA extraction kit (Qiagen,Beijing,China) according to the manufacturer’s instructions. DNA was eluted by adding 60 μL of sterile, nuclease-free water. The extracted DNA were stored at −20°C until use.
MCDA Assay Primers Design and Synthesis
Primer Explorer V4 and Primer 5.0 were utilized to design MCDA primers targeting C. tropicalis specific gene ITS II (Accession No. AF268095.1). Based on design principle of MCDA primer, 10 primers used for C. tropicalis detection were designed and then blasted on NCBI to confirm their specificity (Wang et al., 2015). The details of MCDA primers’ locations and sequences were indicated in Figure 1 and Table 2. All of the primers used were synthesized by Beijing-Tsingke Biotechnology Co., Ltd. (Beijing, China) with HPLC purification grade.
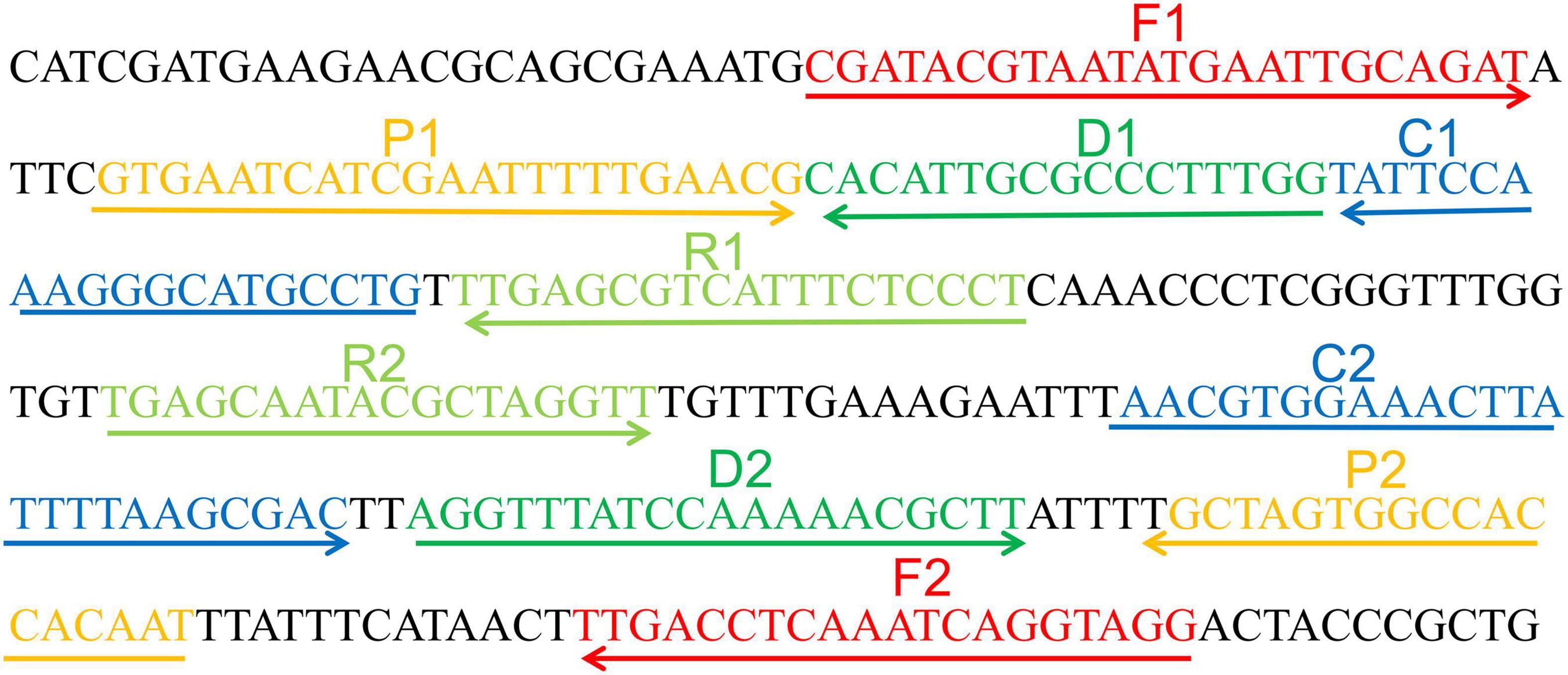
Figure 1. Appropriate primer design for C. tropicalis MCDA-LFB assay and primer sequence positions in the selected fragment including ITS II.
Preparation of Nanoparticle-Based Lateral Flow Biosensor
Lateral flow biosensor (LFB) used in this study was prepared according to previously reported (Wang et al., 2015). In short, the biosensor was composed of four sections, including conjugate pad, sample pad, NC membrane, and absorbent pad. Dye streptavidin-coated polymer nanoparticles were laminated in the conjugate pad. Anti-FAM (0.25 mg/ml) and biotin-BSA (2.5 mg/ml) were conjugated onto the NC membrane for test line (TL) and control line (CL), respectively. The prepared LFB were preserved with a desiccant gel at room temperature.
The C. tropicalis-MCDA Reaction
Based on the standard MCDA reaction (Wang et al., 2015), the total volume of MCDA reaction system was 25 μL, containing 0.4 μM each of displacement primers (F1 and F2), 0.8 μM each of amplification primers (C1∗, C2, R1, R2, D1∗, and D2), 1.6 μM each of cross primers (CP1 and CP2), 12.5 μL of 2 × reaction mix (Hai Tai Zheng Yuan, Beijing, China), 1.25 μL (10U) Bst DNA polymerase (Hai Tai Zheng Yuan, Beijing, China) and 1 μL DNA template from isolated strain or 5 μL DNA template from clinical samples were used. Also, 10 ng genomic DNA of C. albicans ATCC10231 and Klebsiella pneumoniae was used as negative control, and 1 μL double distilled water was served as blank control.
Both colorimetric indicator (malachite green, MG) and lateral flow biosensor (LFB) methods were employed to determine and verify the C. tropicalis-MCDA products. When using MG, noticeable change was observed in the positive reaction solution from colorless to light green, while the negative and blank controls remained colorless. With LFB assay, two lines included test line (TL) and control line (CL), which appeared in positive reactions, but only CL was observed in negative controls and blank control.
Optimal Temperature of C. tropicalis-MCDA Assay
In the MCDA reaction system, temperature was essential for influencing amplification efficiency. the amplification temperature of C. tropicalis-MCDA assay was optimized from 61 to 68°C with 1°C interval. Amplification mixtures with 1 μL of template of C. albicans were used as negative controls (NCs), and 1 μL of distilled water (DW) was used as a blank control. The MCDA amplicons were monitored using loopamp real-time turbidimeter LA-320C (Eiken Chemical Co., Ltd., Japan). Turbidity >0.1 was considered as positive result.
Limit of Detection (LoD) and Optimal Isothermal Amplification Time of C. tropicalis-MCDA Assay
To evaluate LoD of MCDA assay for C. tropicalis, a serial dilution of C. tropicalis strain (ATCC13803) genomic DNA from 10 ng to 100 ag (10 ng, 10 pg, 1 pg, 100 fg, 10 fg, 1 fg, and 100 ag per microliter) were used to determine the LoD. As a template, 1 μL genomic DNA was added into the amplification reaction system. Meanwhile, the serially diluted templates were applied for optimizing the isothermal amplification time during the reaction stage, four different times (10–40 min, with 10 min interval) were compared under the same reaction conditions, and all of the amplified products at each time point including 10, 20, 30, and 40 min were detected by MG and LFB, and each reaction was repeated three times.
Specificity of C. tropicalis-MCDA Assay
To assess the analytical specificity of C. tropicalis-MCDA assay, the genomic DNA (at least 10 fg per microliters) was extracted from 23 C. tropicalis strains and 18 non-C. tropicalis strains, respectively, which were amplified under the best conditions. All MCDA products were detected by lateral flow biosensor (LFB), and each test was repeated at least three times.
Application of C. tropicalis -MCDA Detection in Clinical Samples
To evaluate applying C. tropicalis-MCDA detection in clinical samples, 300 sputum samples were collected from The First People’s Hospital of Guiyang and were detected using the gold-standard method (the culture method) and C. tropicalis-MCDA. Traditional culture methods included colony morphology, Gram staining and biochemical identification. The traditional detection method was performed first, MCDA-LFB was performed after a certain amount of sputum samples were collected, culture and molecular testing were done by two different people, and it was not known which one contained the target fungus before the experiment. The C. tropicalis-MCDA detection was performed as described above. The results of C. tropicalis-MCDA-LFB were compared with that of culture assay.
Results
Selection of the Set of Primers
To confirm effectiveness of the four sets of primers (Supplementary Table 1) for C. tropicalis, MCDA reactions were performed at a constant temperature of 62°C for 40 min using C. tropicalis (ATCC13803) genomic DNA templates. The most effective set of primers was selected by inspection of the curves from loopamp real-time turbidimeter LA-320C for use as future experimental primers (Supplementary Figure 1). The first set primers is considered to be the most effective for C. tropicalis-MCDA amplification, because a threshold value of 0.1 of absorbance that indicated positive amplification from the C. tropicalis-MCDA reaction was reached most quickly. Then, to confirm the effectiveness of the MCDA primers we selected (Figure 1 and Table 1), the amplification products were monitored by two different methods, including colorimetric indicator (malachite green, MG) and lateral flow biosensor (LFB). The results showed that the positive results were observed when the nucleic acid from C. tropicalis, but not with C. albicans, Klebsiella pneumoniae, and the blank control (Figures 2A,B). Hence, the C. tropicalis-MCDA primers for ITS II gene detection in the current study were valid for the establishment of C. tropicalis MCDA-LFB.
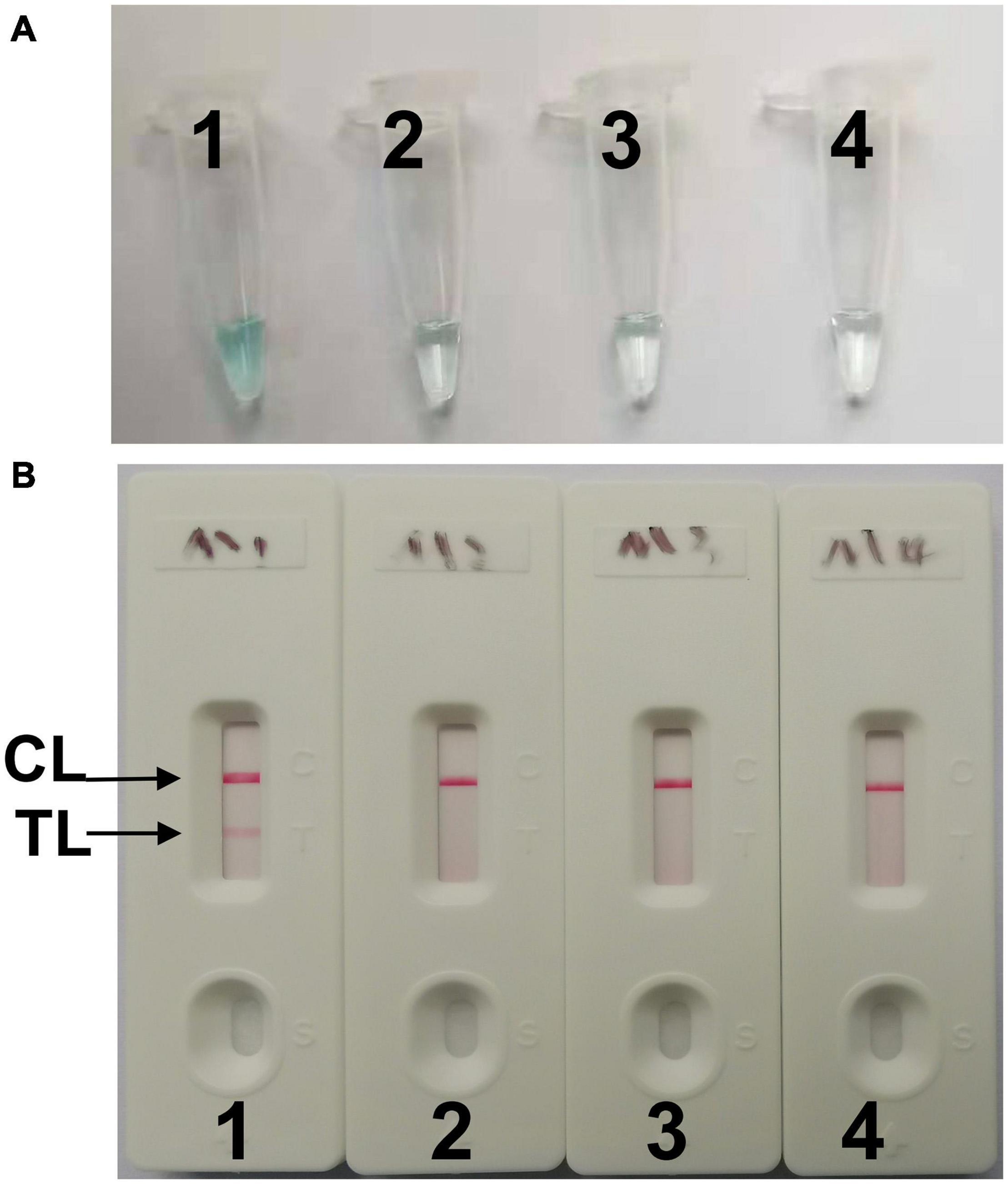
Figure 2. Confirming of C. tropicalis-MCDA products. (A) The C. tropicalis-MCDA amplification products were analyzed with MG reagents through visually observation of the color change. (B) Lateral flow biosensor (LFB) was applied for visual detection of C. tropicaliss-MCDA products, two lines contained test line (TL) and the control line (CL) appeared in positive samples, but only the CL observed in negative samples. Tube 1/Biosensor 1: positive amplification of C. tropicalis (ATCC13803); Tube 2/Biosensor 2: negative amplification of C. albicans (ATCC10231); 3: negative amplification of Klebsiella pneumoniae; Tube 4/Biosensor 4: blank control (DW).
Optimal Temperature of C. tropicalis-MCDA Assay
To identify the optimal temperature of C. tropicalis-MCDA assay, the C. tropicalis standard strain (ATCC13803) DNA was employed as a template, and MCDA amplifications were carried out from 61 to 68°C with 10 pg per tube of C. tropicalis. The turbidity of all reaction products was monitored using loopamp real-time turbidimeter LA-320C. As shown in Figure 3, 64°C was considered as an optimum reaction temperature for C. tropicalis-MCDA amplification, because a threshold value of 0.1 of absorbance that indicated positive amplification from the C. tropicalis-MCDA reaction was reached most quickly at 64°C. Herein, the optimal temperature of 64°C was employed for the subsequent C. tropicalis-MCDA examinations conducted in this report.
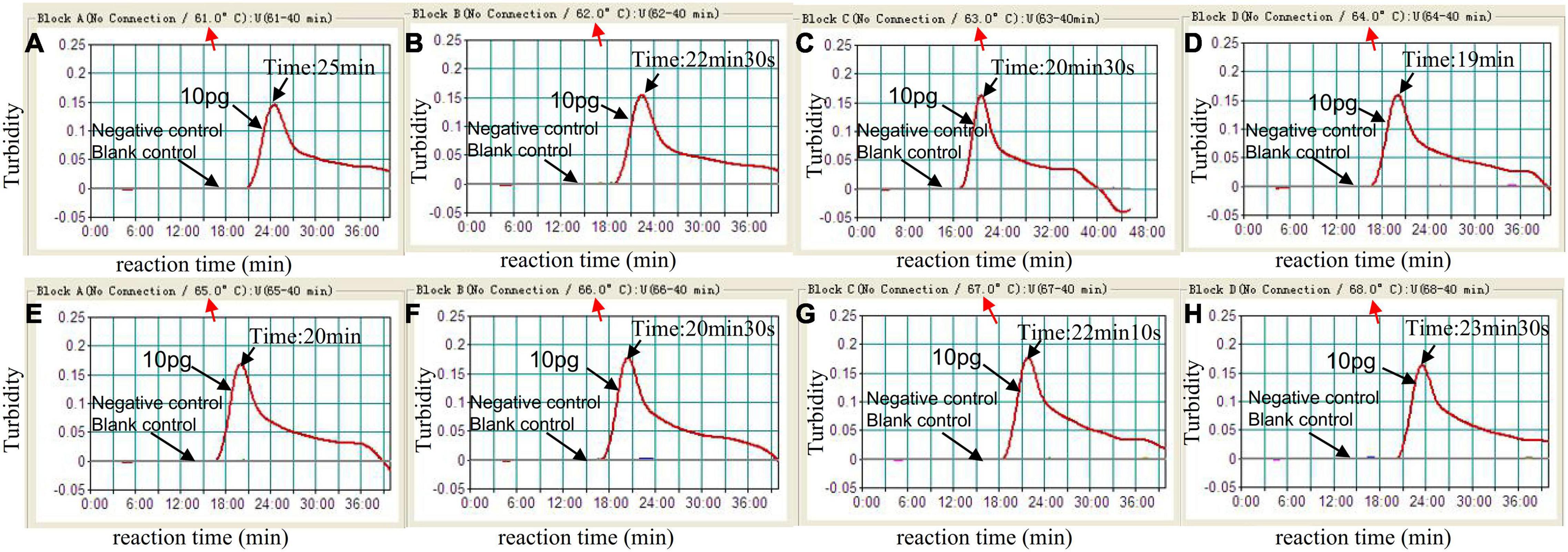
Figure 3. Optimal amplification temperature for C. tropicalis-MCDA assay. By using a real-time measurement to monitor the turbidity of C. tropicalis-MCDA reactions. The corresponding curves were displayed in the panels. The negative control was C. albicans, and the blank control was sterile double-distilled water. Abscissa represents reaction time (min), ordinate represents turbidity. The threshold value was 0.1, and the turbidity >0.1 was considered as positive amplification. Eight kinetic curves (A–H) were generated from 61 to 68°C (1°C intervals), with 10 pg C. tropicalis DNA per reaction. The optimal C. tropicalis-MCDA reaction temperature was 64°C.
Limit of Detection and Optimized Time of MCDA for C. tropicalis Detection
To determine the LoD of C. tropicalis-MCDA assay, a serial dilution of the C. tropicalis genomic DNA (10 ng, 10 pg, 1 pg, 100 fg, 10 fg, 1 fg, and 100 ag per microliter) was used in MCDA assays. As observed in Figure 4, when the dilution exceeded 10 fg, MCDA tubes presented colorless and only control band in LFB. While other dilutions MCDA tubes were presented bright blue, and two red bands were observed in LFB; one was control line, and the other was test line.
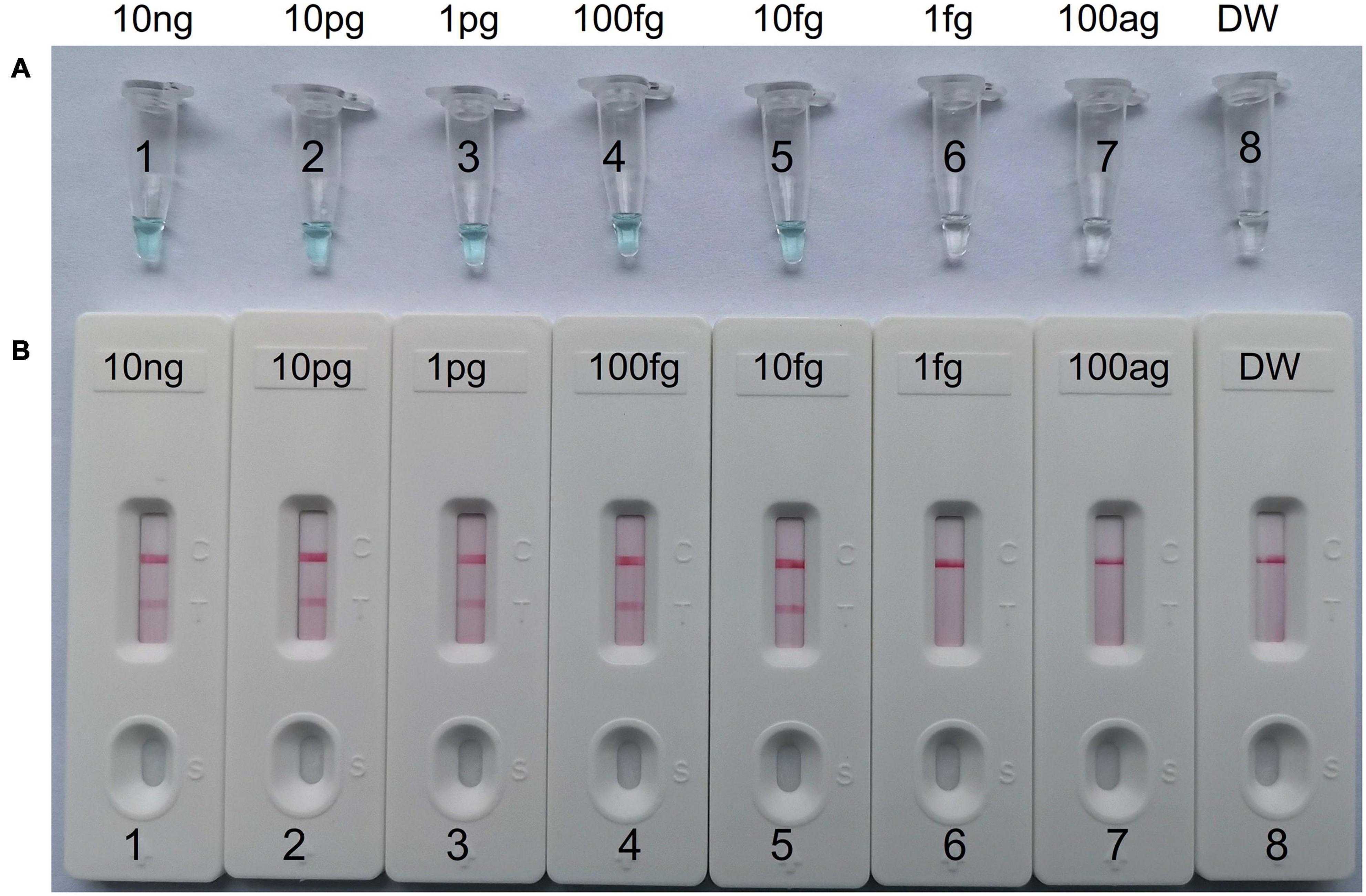
Figure 4. The detection limit of C. tropicalis-MCDA-LFB assays. Two measurement techniques, including (A) a colorimetric indicator (MG) and (B) lateral flow biosensor. A series of dilutions (10 ng, 10 pg, 1 pg, 100 fg, 10 fg, 1 fg, and 100 ag) of C. tropicalis ATCC13803 DNA and a blank control (DW) were operated according to standard MCDA reactions.
To obtain the optimal amplification time for the C. tropicalis MCDA assay, four amplification times (10, 20, 30, and 40 min) were tested at 64°C, respectively. The amplification products were tested with LFB. The results confirmed that the LoD level of C. tropicalis ATCC10231 genomic DNA (10 fg per reaction) was tested when the amplification last 30 and 40 min (Figure 5). Therefore, 30 min was considered an appropriate amplification time for C. tropicalis MCDA assay.
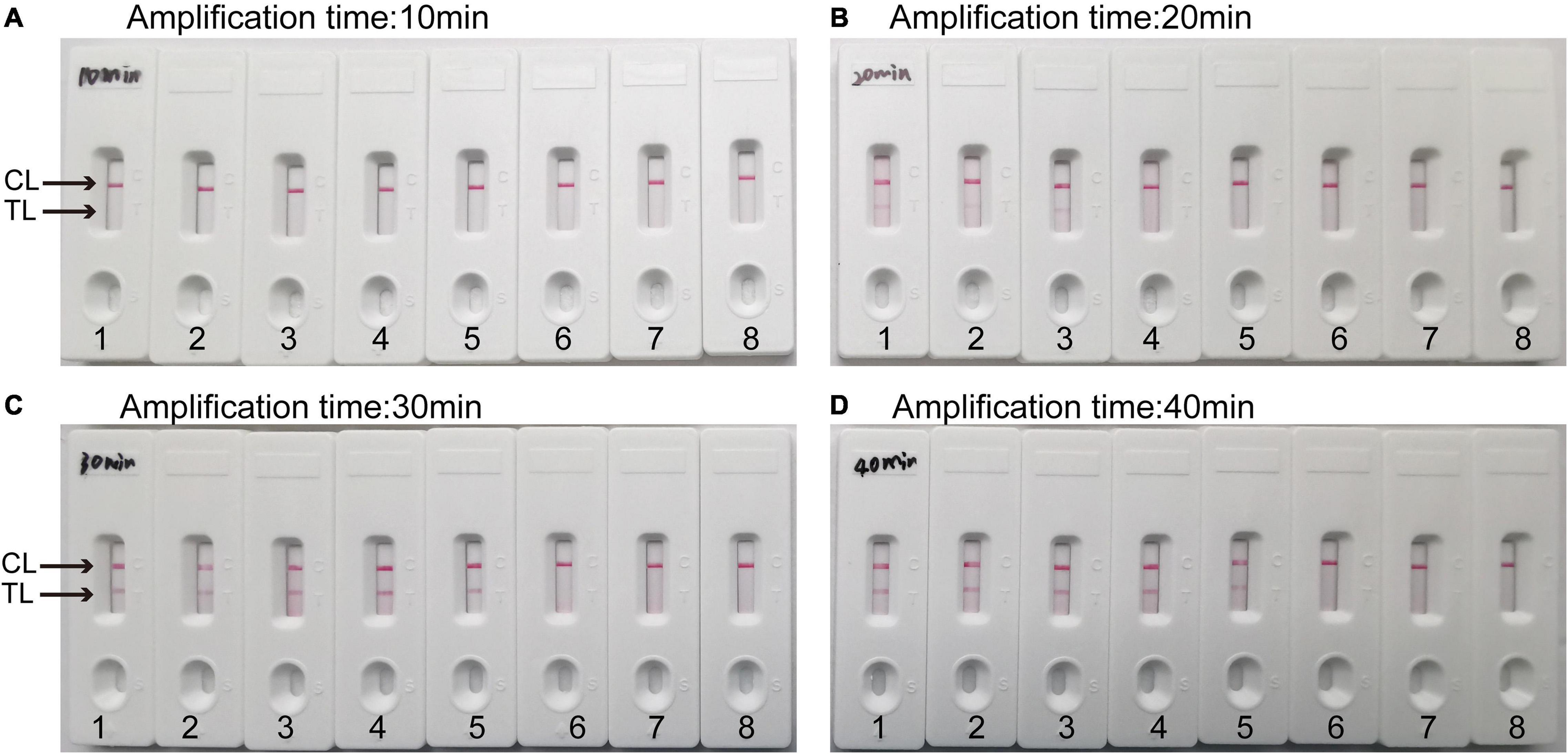
Figure 5. Optimal detection time required for C. tropicalis MCDA-LFB assay. Four different reaction times ((A) 10 min; (B) 20 min; (C) 30 min; (D) 40 min) were evaluated at 64°C. Biosensors 1–7 represent C. tropicalis ATCC13803 DNA levels of 10 ng, 10 pg, 1 pg, 100 fg, 10 fg, 1 fg, and 100 ag per reaction, respectively; 8 represents a blank control (DW). The best amplification time was observed when MCDA lasted for 30 min (C).
Specificity of MCDA-LFB for C. tropicalis Detection
The results showed that two red lines appeared at the location of TL and CL on the strips for the C. tropicalis strain, but only one line appeared at the location of CL for all the non-C. tropicalis strains and blank control (Figure 6), LFB devices were labeled C. tropicalis (Ctr) as positives after the test results were obtained. So, the results suggested that MCDA-LFB was highly specific for C. tropicalis detection.
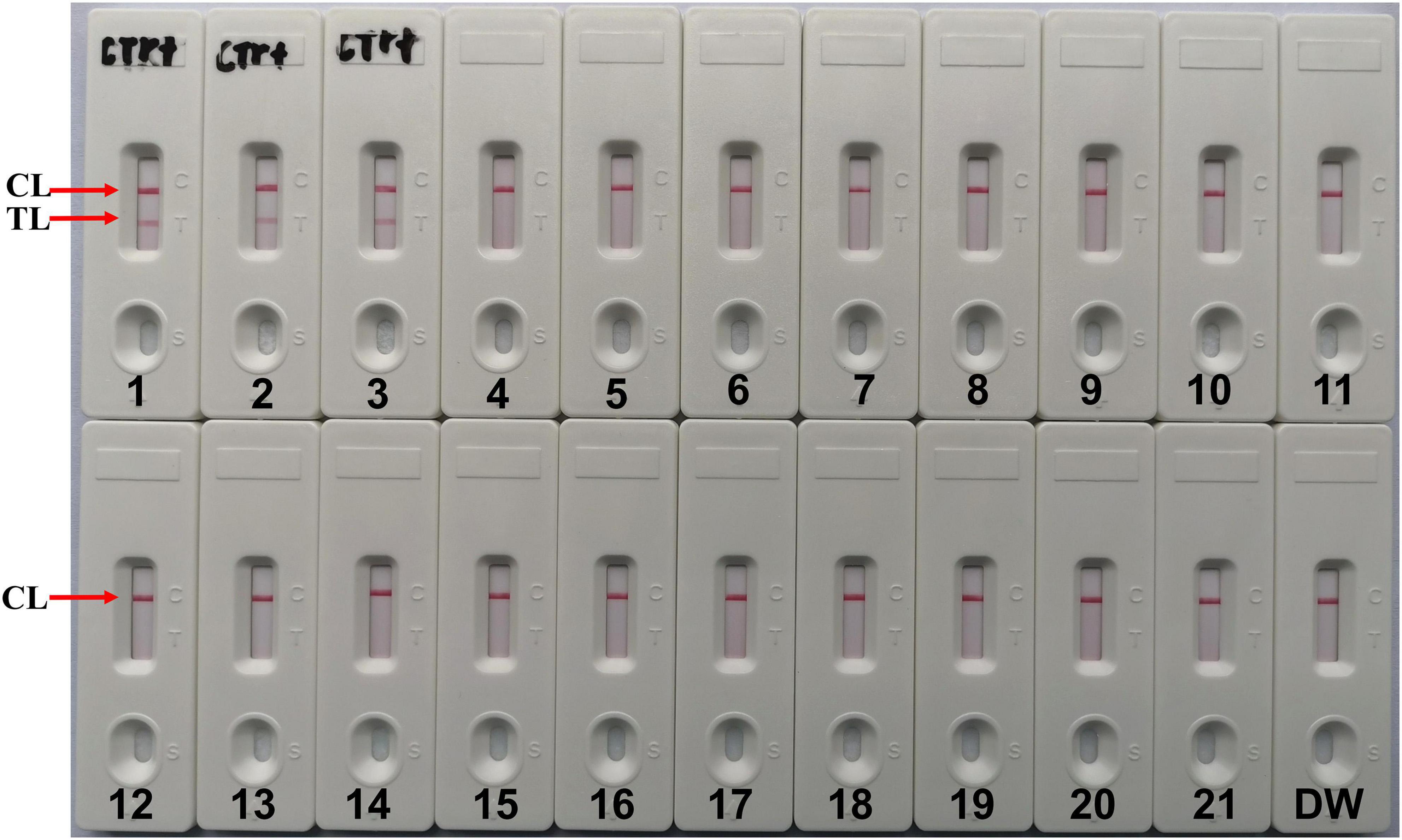
Figure 6. Specificity of LFB assays detecting C. tropicalis-MCDA products. The C. tropicalis-MCDA-LFB assay was evaluated with different genomic DNA as templates. Both the control line and the test line were visible in LFB for all C. tropicalis, and only the control line was appeared in non- C. tropicalis. LFB devices were labeled C. tropicalis (Ctr) as positives after the test results were obtained. 1, Positive control (C. tropicalis ATCC13803), 2–3, isolated C. tropicalis strains; 4–6, Candida albicans; 7, Candida dubliniensis; 8, Candida stellatoidea; 9, Candida guilliermondi; 10, Candida krusei; 11–12, Candida parapsilosis; 13, Candida glabrata; 14, Cryptococcus neoformans; 15, Penicillium mameffei; 16, Aspergillus flavus; 17, Staphylococcus aureus; 18, Enterococcus faecium; 19, Klebsiella pneumoniae; 20, Pseudomonas aeruginosa; 21, Acinetobacter baumannii; 22, a blank control (DW).
Application of MCDA-LFB Assay in Clinical Samples for C. tropicalis Detection
To further confirm the application of MCDA-LFB as a valuable tool for C. tropicalis detection in clinic, we analyzed 300 sputum samples from clinic using culture methods and C. tropicalis-MCDA-LFB assay. As C. tropicalis positive bloodstream samples are not frequently obtained in our clinic, but positive sputum samples could be readily obtained (Xie et al., 2019), sputum samples were chosen to investigate the clinical utility of the test. The results are shown in Table 3. Of the 300 clinical sputum samples, 28 sputum samples were detected successfully by MCDA-LFB assay, which had been confirmed positive by culture assays. The C. tropicalis-MCDA-LFB assay results were the same as traditional culture results. Therefore, such results suggested that MCDA-LFB was a feasible alternative to traditional culture and could potentially provide results in nucleic acid analysis.
Discussion
C. tropicalis is a diploid dimorphic opportunistic fungus belonging to a member of genus Candida. C. tropicalis has recently become one of the most common non-Candida albicans Candida species (NAC), even more prevalent than C. albicans in some countries. It is reported that non-Candida albicans Candida species are clinically indistinguishable and displays different resistance to antifungal drugs and degrees of virulence (Fan et al., 2017). Furthermore, mortality rates associated with non-Candida albicans Candida species infection are unacceptably high (40–70%), especially patients with immunosuppressed and neutropenic or bone marrow transplant (Xiao et al., 2020). So rapid diagnosis and accurate medication are key to improving the survival rate. However, traditional methods (culture-based techniques and colony morphology) and PCR-based technology for detecting C. tropicalis are time-consuming. Consequently, developing a new method to identify and diagnose C. tropicalis straightforwardly, rapidly, sensitively, and specifically is required.
This study combined multiple cross displacement amplification (MCDA) with lateral flow biosensors (LFB) to detect C. tropicalis. The MCDA is an isothermal amplification system that requires only a simple water bath or heater without special equipment and professional training (Wang et al., 2017). The LFB labeled with FAM and biotin could detect amplification products (Wang et al., 2018). We could read the test result from the lines on LFB for approximately 2 min. Compared to molecular diagnostic assays, such as PCR-based methods, 10 primers were designed to identify the specific genes of C. tropicalis in MCDA system, and the study results showed that the optimal reaction condition of C. tropicalis-MCDA assay was 64°C within 30 min, and only 10 fg DNA was required in each reaction. This indicates that MCDA is a rapid, sensitive and straightforward method for detecting trace amounts of C. tropicalis. The specificity of C. tropicalis-MCDA-LFB assay was confirmed using the template DNA isolated from 23 C. tropicalis strains and 18 non-C. tropicalis strains, and all C. tropicalis-positive products from MCDA were successfully identified using LFB, not for non-C. tropicalis strains, which indicated that C. tropicalis-MCDA-LFB assay could be regarded as an applied tool for detecting C. tropicalis with high specificity. The entire detection procedure of C. tropicalis-MCDA-LFB assay, including template preparation (about 30 min), isothermal reaction (30 min), and LFB result reading (approximately 2 min), could be performed within 70 min. Though MCDA amplification products could be detected by gel electrophoresis analysis and turbidity analysis, we chose LFB in this study. In the detection procedure, using LFB could avoid many tedious steps such as gel electrophoresis analysis, and LFB result reading does not require professional training. Furthermore, we can obtain experiment result only by waiting for approximately 2 min, implying that LFB is easier and shortens the detection time than other methods.
More importantly, we successfully used C. tropicalis-MCDA-LFB assay to detect sputum samples from clinic. Compared with culture-based method, MCDA-LFB method is more rapid and straightforward, with 100% positive rate and 100% negative rate. Compared with real-time PCR assay for candida species, the C. tropicalis-MCDA-LFB technique is more time-saving, the real-time PCR requires 2∼3 h during the whole process (Guo et al., 2016). However, the detection time of entire MCDA-LFB assay procedure was less than 1.5 h, including sample processing. It showed that our developed method to detect C. tropicalis is faster than culture-based and real-time PCR method. Since C. tropicalis-MCDA-LFB assay can report results within only 1.5 h, clinicians can provide targeted therapies to patients more quickly, thus reducing exposure to broad-spectrum antibiotics.
However, our method has limitations. Firstly, the MCDA-LFB detection is a qualitative determination for C. tropicalis, and could not quantify target pathogen amount in the sample. so we cannot distinguish the infection from colonization. It is challenging to assess the dosage and efficacy of antibiotics. A more precise study will be designed for quantification of the amount of C. tropicalis in clinical samples with MCDA-LFB assay. Secondly, we only use the method to detect clinical sputum samples, and other specimens that can be tested in this way are unknown. In recent years, the proportion of bloodstream infection and urinary tract infection caused by C. tropicalis has increased, especially in intensive care unit patients, especially in those with malignancies, receiving broad-spectrum antibiotics or undergoing prolonged catheterization (Gharanfoli et al., 2019; Wang J. et al., 2020). So, we will focus on testing other clinical samples such as blood and urine in MCDA-LFB method. Moreover, MCDA is an isothermal amplification system requiring several multiple pairs of primers that easily carry contamination leading to false-positive results. Efforts have been made to solve this problem, and if we hold the optimum reaction temperature and reaction time, we can reduce false-positive results. So far, the MCDA-LFB assay has been successfully used to detect pathogens such as Staphylococcus aureus, Acinetobacter baumannii, Shigella spp., and Candida albicans (Wang et al., 2016d, 2018; Cheng et al., 2019; Zhao et al., 2019).
Conclusion
In this work, we successfully developed an MCDA-LFB assay to detect C. tropicalis simply, rapidly, and precisely. Compared with culture-based methods and molecular diagnostic assays, MCDA-LFB assay avoids sophisticated processes and does not require expensive equipment and skilled technical personnel. The limit of detection (LoD) of novel assay for C. tropicalis detection from isolate was as little as 10 fg, suggesting that MCDA-LFB assay was very sensitive. More importantly, the novel assay is more time-saving that can reduce detection time, and help clinicians provide targeted therapies for patients more quickly. In short, MCDA-LFB assay with accurate and timely detection, and not require expensive equipment and skilled technical personnel, it is amenable to generalize, especially in resource-poor areas.
Data Availability Statement
The original contributions presented in the study are included in the article/Supplementary Material, further inquiries can be directed to the corresponding author/s.
Ethics Statement
Written informed consent was obtained from the minor(s)’ legal guardian/next of kin for the publication of any potentially identifiable images or data included in this article.
Author Contributions
YW, XT, and SL conceived and designed the experiments. YW, XZ, JC, XT, and XC performed the experiments. YW, JC, and HY analyzed the data. YW, XZ, XC, and SL wrote the manuscript. All authors contributed to the article and approved the submitted version.
Funding
This study was supported by Zhu Ke He Tong [2020]-10-6 and [2019] Zhu Wei Jian Ke Ji He Tong Zi Di 001 from the Science and Technology Department of Guiyang city of Guizhou Province, and was supported by Qian Ke He [2018]1094, Qian Ke He Zhi Cheng [2021] Yi Ban 440, Qian Ke He Platform talent [2018]-5606, and Qian Ke He Platform [2018]-5767 from the Science and Technology Department of Guizhou Province.
Conflict of Interest
The authors declare that the research was conducted in the absence of any commercial or financial relationships that could be construed as a potential conflict of interest.
Supplementary Material
The Supplementary Material for this article can be found online at: https://www.frontiersin.org/articles/10.3389/fmicb.2021.681488/full#supplementary-material
References
Ala-Houhala, M., Valkonen, M., Kolho, E., Friberg, N., and Anttila, V. J. (2019). Clinical and microbiological factors associated with mortality in candidemia in adult patients 2007-2016. Infect. Dis. 51, 824–830. doi: 10.1080/23744235.2019.1662941
Arastehfar, A., Lass-Flörl, C., Garcia-Rubio, R., Daneshnia, F., Ilkit, M., Boekhout, T., et al. (2020). The quiet and underappreciated rise of drug-resistant invasive fungal pathogens. J. Fungi 6:138. doi: 10.3390/jof6030138
Arrua, J. M. M., Rodrigues, L. A. S., Pereira, F. O., and Lima, E. O. (2015). Prevalence of Candida tropicalis and Candida krusei in onychomycosis in joão pessoa, paraiba, brazil from 1999 to 2010. An. Acad. Bras. Cienc. 87, 1819–1822.
Avni, T., Leibovici, L., and Paul, M. (2011). Pcr diagnosis of invasive candidiasis: systematic review and meta-analysis. J. Clin. Microbiol. 49, 665–670. doi: 10.1128/jcm.01602-10
Chai, L. Y., Denning, D. W., and Warn, P. (2010). Candida tropicalis in human disease. Crit. Rev. Microbiol. 36, 282–298. doi: 10.3109/1040841X.2010.489506
Chakrabarti, A., Chatterjee, S. S., Rao, K. L., Zameer, M. M., Shivaprakash, M. R., Singhi, S., et al. (2009). Recent experience with fungaemia: change in species distribution and azole resistance. Scand. J. Infect. Dis. 41, 275–284. doi: 10.1080/00365540902777105
Chakrabarti, A., Sood, P., Rudramurthy, S. M., Chen, S., Kaur, H., Capoor, M., et al. (2015). Incidence, characteristics and outcome of ICU-acquired candidemia in India. Intens. Care. Med. 41, 285–295. doi: 10.1007/s00134-014-3603-2
Chen, P. Y., Chuang, Y. C., Wu, U. I., Sun, H. Y., Wang, J. T., Sheng, W. H., et al. (2019). Clonality of fluconazole-nonsusceptible Candida tropicalis in bloodstream infections, Taiwan, 2011-2017. Emerg. Infect. Dis. 25, 1660–1667. doi: 10.3201/eid2509.190520
Cheng, X., Yang, J., Wang, M., Wu, P., Du, Q., He, J., et al. (2019). Visual and rapid detection of Acinetobacter baumannii by a multiple cross displacement amplification combined with nanoparticles-based biosensor assay. AMB Express 9:30. doi: 10.1186/s13568-019-0754-0
Fan, X., Xiao, M., Liao, K., Kudinha, T., Wang, H., Zhang, L., et al. (2017). Notable increasing trend in Azole non-susceptible Candida tropicalis causing invasive candidiasis in China (August 2009 to July 2014): molecular epidemiology and clinical azole consumption. Front. Microbiol. 8:464. doi: 10.3389/fmicb.2017.00464
Fuchs, B. B., Eatemadpour, S., Martel-Foley, J. M., Stott, S., Toner, M., Mylonakis, E., et al. (2019). Rapid isolation and concentration of pathogenic fungi using inertial focusing on a chip-based platform. Front. Cell. Infect. Microbiol. 9:27. doi: 10.3389/fcimb.2019.00027
Gharanfoli, A., Mahmoudi, E., Torabizadeh, R., Torabizadeh, R., Katiraii, F., and Faraji, S. (2019). Isolation, characterization and molecular identification of Candida species from urinary tract infections. Curr. Med. Mycol. 5, 33–36. doi: 10.18502/cmm.5.2.1159
Guo, Y., Yang, J. X., and Liang, G. W. (2016). A real-time PCR assay based on 5.8S rRNA gene (5.8S rDNA) for rapid detection of Candida from whole blood samples. Mycopathologia 181, 405–413. doi: 10.1007/s11046-015-9977-z
Horváth, E., Sipiczki, M., Csoma, H., and Miklós, I. (2020). Assaying the effect of yeasts on growth of fungi associated with disease. BMC Microbiol. 20:320. doi: 10.1186/s12866-020-01942-0
Jagdish, C., Nidhi, S., Kaur, S. S., and Satinder, G. (2013). Epidemiology of Candida blood stream infections: experience of a tertiary care centre in north india. J. Infect. Dev. Ctries 7, 670–675. doi: 10.3855/jidc.2623
Jenks, J. D., Cornely, O. A., Chen, S. C., Thompson, G. R. III, and Hoenigl, M. (2020). Breakthrough invasive fungal infections: who is at risk? Mycoses 63, 1021–1032. doi: 10.1111/myc.13148
McCarty, T. P., and Pappas, P. G. (2015). Invasive candidiasis. Infect. Dis. Clin. North. Am. 30, 103–124. doi: 10.1016/j.idc.2015.10.013
Ogata, K., Matsuda, K., Tsuji, H., and Nomoto, K. (2015). Sensitive and rapid RT-qPCR quantification of pathogenic Candida species in human blood. J. Microbiol. Methods 117, 128–135. doi: 10.1016/j.mimet.2015.07.021
Patil, A. H., Datta, K. K., Behera, S. K., Kasaragod, S., Pinto, S. M., Koyangana, S. G., et al. (2018). Dissecting Candida pathobiology: post-translational modifications on the Candida tropicalis Proteome. OMICS 22, 544–552. doi: 10.1089/omi.2018.0093
Wang, H., Xu, Y. C., and Hsueh, P. R. (2016a). Epidemiology of candidemia and antifungal susceptibility in invasive Candida species in the asia-pacific region. Future Microbiol. 11, 1461–1477. doi: 10.2217/fmb-2016-0099
Wang, Y., Shi, C., Liu, J. Y., Li, W. J., Zhao, Y., and Xiang, M. J. (2016b). Multilocus sequence typing of Candida tropicalis shows clonal cluster enrichment in azole-resistant isolates from patients in Shanghai. China. Infect. Genet. Evol. 44, 418–424. doi: 10.1016/j.meegid.2016.07.026
Wang, Y., Wang, Y., Xu, J., and Ye, C. (2016c). Development of multiple cross displacement amplification label-based gold nanoparticles lateral flow biosensor for detection of Shigella spp. Front. Microbiol. 7:1834. doi: 10.3389/fmicb.2016.01834
Wang, Y., Wang, Y., Zhang, L., Xu, J. G., and Ye, C. Y. (2016d). Visual and multiplex detection of nucleic acid sequence by multiple cross displacement amplification coupled with gold nanoparticle-based lateral flow biosensor. Sens. Actuators B Chem. 241, 1283–1293. doi: 10.1016/j.snb.2016.10.001
Wang, J., Chen, H., Lin, X., Ji, C., and Chen, B. (2020). Multiple cross displacement amplification-a more applicable technique in detecting Pseudomonas aeruginosa of ventilator-associated pneumonia (vap). Crit. Care 24:306. doi: 10.1186/s13054-020-03003-4
Wang, Q., Li, C., Tang, D., and Tang, K. (2020). Molecular epidemiology of Candida tropicalis isolated from urogenital tract infections. Microb. Open 9:e1121. doi: 10.1002/mbo3.1121
Wang, Y., Wang, Y., Ma, A. J., Li, D. X., Luo, L. J., Liu, D. X., et al. (2015). Rapid and sensitive isothermal detection of nucleic-acid sequence by multiple cross displacement amplification. Sci. Rep. 5:11902.
Wang, Y., Wang, Y., Wang, H., Xu, J., and Ye, C. (2017). A label-free technique for accurate detection of nucleic acid-based self-avoiding molecular recognition systems supplemented multiple cross-displacement amplification and nanoparticles based biosensor. Artif. Cells Nanomed. Biotechnol. 46, 1671–1684. doi: 10.1080/21691401.2017.1389748
Wang, Y., Yan, W., Fu, S., Hu, S., Wang, Y., Xu, J., et al. (2018). Multiple cross displacement amplification coupled with nanoparticles-based lateral flow biosensor for detection of Staphylococcus aureus and identification of methicillin-resistant S.aureus. Front. Microbiol. 9:907. doi: 10.3389/fmicb.2018.00907
Xiao, M., Chen, S. C., Kong, F., Xu, X. L., Yan, L., Kong, H. S., et al. (2020). Distribution and antifungal susceptibility of Candida species causing candidemia in China: an update from the CHIF-NET study. J. Infect. Dis. 221, 139–147. doi: 10.1093/infdis/jiz573
Xiao, M., Fan, X., Chen, S. C., Wang, H., Sun, Z. Y., Liao, K., et al. (2015). Antifungal susceptibilities of Candida glabrata species complex, Candida krusei, Candida parapsilosis species complex and Candida tropicalis causing invasive candidiasis in China: 3 year national surveillance. J. Antimicrob. Chemother. 70, 802–810. doi: 10.1093/jac/dku460
Xie, C. Y., Xiong, Y., Sun, J., Yang, Z. L., and Hu, Y. (2019). Clinical isolates distribution and drug resistance in nosocomial infections of Candida tropicalis. Chin. J. Mycol. 14, 107–109.
Zhao, F., Niu, L., Yan, L., Nong, J., Wang, C., Wang, J., et al. (2019). Establishment and application of multiple cross displacement amplification coupled with lateral flow biosensor (MCDA-LFB) for visual and rapid detection of Candida albicans in clinical samples. Front. Cell. Infect. Microbiol. 9:102. doi: 10.3389/fcimb.2019.00102
Keywords: Candida tropicalis, multiple cross displacement amplification, lateral flow biosensor, limit of detection, clinical samples
Citation: Wang Y, Zhao X, Cheng J, Tang X, Chen X, Yu H and Li S (2021) Development and Application of a Multiple Cross Displacement Amplification Combined With Nanoparticle-Based Lateral Flow Biosensor Assay to Detect Candida tropicalis. Front. Microbiol. 12:681488. doi: 10.3389/fmicb.2021.681488
Received: 16 March 2021; Accepted: 18 May 2021;
Published: 10 June 2021.
Edited by:
Hector Mora Montes, University of Guanajuato, MexicoReviewed by:
Noemí de-los-Santos-Álvarez, University of Oviedo, SpainDonna MacCallum, University of Aberdeen, United Kingdom
Copyright © 2021 Wang, Zhao, Cheng, Tang, Chen, Yu and Li. This is an open-access article distributed under the terms of the Creative Commons Attribution License (CC BY). The use, distribution or reproduction in other forums is permitted, provided the original author(s) and the copyright owner(s) are credited and that the original publication in this journal is cited, in accordance with accepted academic practice. No use, distribution or reproduction is permitted which does not comply with these terms.
*Correspondence: Yu Wang, wangzhongyuwy@163.com; Shijun Li, zjumedjun@163.com
†These authors have contributed equally to this work