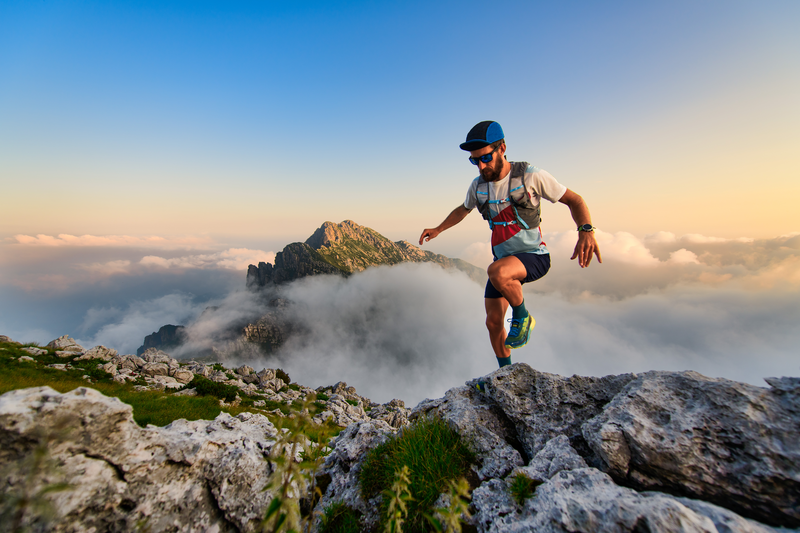
94% of researchers rate our articles as excellent or good
Learn more about the work of our research integrity team to safeguard the quality of each article we publish.
Find out more
ORIGINAL RESEARCH article
Front. Microbiol. , 20 May 2021
Sec. Food Microbiology
Volume 12 - 2021 | https://doi.org/10.3389/fmicb.2021.678903
This article is part of the Research Topic From Traditional to Modern: Progress of Molds and Yeasts in Fermented-Food Production View all 45 articles
Hong Qu Huangjiu (HQW) is distinguished by its inclusion of Monascus pigments, meaning that photosensitivity strongly affects the sensory quality of the wine. In this study, the effects of Flos sophorae immaturus (FSI) on the stability of Monascus pigments, the flavor profiles, and the sensory characteristics of HQW were investigated. After sterilization, the addition of FSI increased the preservation rate of Monascus pigments in HQW by up to 93.20%, which could be accounted for by the synergy of rutin and quercetin in FSI. The total content of the volatile flavor compounds in HQW increased significantly as the added amounts of FSI were increased, especially 3-methyl-1-butanol, 2-methyl-1-propanol, and short-chain fatty acid ethyl esters (SCFAEE). Sensory evaluation and partial least-squares regression revealed that the concentration of FSI significantly affected the aroma characteristics of HQW but had little effect on the mouthfeel. The addition of 0.9 mg/mL FSI yielded a satisfactory HQW with high scores in terms of mouthfeel and aroma. The strong correlation between fruit-aroma, full-body, and SCFAEE suggests that FSI might alter the aroma of HQW by enhancing the synthesis of SCFAEE. Summarily, treatment with FSI represents a new strategy for improving the stability of photosensitive pigments and thus adjusting the aroma of HQW or similar beverages.
Hong Qu Huangjiu (HQW), a typical form of Huangjiu (Chinese rice wine), has become increasingly popular in the rice wine market in recent years (Huang et al., 2019; Wang et al., 2020). Consumers favor HQW because of its bright red color, fine flavor, and health-promoting aspects (Park et al., 2016). The active ingredients of HQW have been proven to reduce blood pressure and blood lipid and cholesterol concentrations, and to influence metabolism and immune regulation in the human body (Lin et al., 2017; Mondal et al., 2019). Accordingly, HQW is referred to as a “ruby” Huangjiu wine.
Hong Qu Huangjiu is unique because its production involves Hong Qu (saccharification starter), which is inoculated with Monascus spp., introducing numerous pigments and enzymes (Feng et al., 2012; Patakova, 2013; Vendruscolo et al., 2016; Keekan et al., 2020). Monascus pigments contribute to the unique color and physiological effects of HQW. However, as a natural pigment, Monascus pigments undergo substantial photodegradation during storage, which negatively affects the appearance, sensory profile, and commercial value of HQW (Mapari et al., 2009; Wang et al., 2016; Sen et al., 2019). Although storage in brown bottles can reduce the degradation of Monascus pigments, these do not fully prevent the color fading of HQW during storage. To resolve this problem, manufacturers prefer the use of caramel coloring to mask the photodegradation of Monascus pigments. However, caramel coloring alters the original color of HQW and may have negative effects on food safety and human health (Vollmuth, 2018; Mateo-Fernández et al., 2019). Thus, the effective and inert stabilizers of Monascus pigments are needed to reduce the photodegradation of HQW during storage, and thus maintain and improve its sensory profile.
Numerous studies have reported that antioxidants effectively prevent the photodegradation of Monascus pigments (Dhale et al., 2018; Liu C. et al., 2020). Therefore, antioxidants may improve the final sensory profiles of HQW. However, the application of traditional food-industry antioxidants (e.g., Vitamin C, quercetin, and β-carotene) is limited by high cost or poor water-solubility (Qian et al., 2012; Horuz et al., 2017). Therefore, inexpensive natural herbs that contain abundant antioxidant compounds may be effective alternatives for reducing the photodegradation of Monascus pigments in HQW.
Flos sophorae immaturus (FSI) is the dried flower bud of Sophora japonica L. This herb has a high flavonoid content and is considered a healthy food because of its high antioxidant activity (Abdelhady et al., 2015; Li and Fan, 2020). FSI has been widely used for 2,000 years in China as an important traditional Chinese medicine, due to its antitumor, anti-inflammatory, and antibacterial activities (Liu T. et al., 2019; Huang et al., 2020). Many studies have reported that the antioxidant compounds of FSI are responsible for its efficacy (Yao et al., 2011; Zhang et al., 2011; Hu et al., 2016; Wang et al., 2019). Therefore, FSI may prevent the photodegradation of Monascus pigments in HQW during storage.
In our previous study (submitted for publication), we observed that FSI preserved Monascus pigments to a greater extent than honeysuckle (a natural herb with high antioxidant activity) and some traditional antioxidants (e.g., Vc, EDTA, β-cyclodextrin, and quercetin). Thus, this study aimed to analyze the ability of FSI to preserve Monascus pigments in HQW during different brewing stages. Specifically, we evaluated the effects of FSI on the oenological properties, flavor profiles, and sensory characteristics in the resulting HQW. This study provides a basis for improving the pigment stability and sensory quality of HQW and broadens the applications for FSI as a novel stabilizer in beverages containing photosensitive pigments.
Hong Qu (Saccharification starter) made using Monascus spp. was purchased from a commercial production facility in Gutian, Fujian province, China. FSI was picked from Linyi, Shandong province, China. Chlorogenic acid, rutin, and quercetin were purchased from Shanghai Yuanye Bio-Technology Co., Ltd. (Shanghai, China). All reagents and standards were purchased at Sigma Aldrich (Shanghai, China). n-Alkane (C7-C40; Supelco, Bellefonte, PA, United States) standards were used to determine the retention index.
Ten grams of Hong Qu was added in 1 L of 15% ethanol, ultrasonicated for 20 min at room temperature, and filtrated to obtain the Monascus pigments-solution. To obtain an extract, fresh FSI was dehydrated and dried in an oven at 40°C to a constant level of moisture. The dry FSI was then thoroughly milled using a grinder to produce powder, which was passed through a 60-mesh sieve. Two grams of the sieved powder were mixed with 40 mL of 40% ethanol at 70°C, ultrasonicated for 20 min, and filtered immediately. The filtrate was pooled and concentrated using a rotary evaporator at 55°C. Finally, a dry powder was obtained by freeze drying and stored in a desiccator in the dark at 4°C.
Ultraviolet (UV) was used to accelerate the degradation of Monascus pigments-solution. Chlorogenic acid, rutin, quercetin, and FSI were, respectively, mixed with Monascus pigments-solution to prepare solutions at a concentration of 0.5 mg/mL. A volume of 50 mL mixed solution was placed in a UV-transparent quartz dish with a radius of 8 cm for UV irradiation, while the pure Monascus pigments-solution was set as the control. The maximum absorbance (Amax) of Monascus pigments-solution and HQW were determined using a SpectraMax M5e spectrofluorometer (Molecular Devices Ltd., San Jose, CA, United States). The Monascus pigments-preservation rate was calculated using the following formula:
where Ax and A0 are the Amax values of pigments treated and untreated with FSI, respectively.
The possibly antioxidant constituent of FSI was analyzed using a Waters high-performance liquid chromatograph (HPLC) system (e2695-Empower system, MA, United States). An equivalent of 5.0 mg of FSI was solubilized in 25 mL methanol, filtered through a 0.22 μm filter membrane, and separated on a Sepax HP-C18 column (4.6 mm × 250 mm, 5 μm) at 25°C. The mobile phase was composed of 100% acetonitrile/0.01 mol/L ammonium acetate (0 min, 10:90 and 30 min, 30:70). The flow rate was 1.0 mL/min, the injection volume was 10 μL, and the UV detection wavelength was set at 350 nm.
Hong Qu Huangjiu was brewed according to the method described by Yang et al. (2017). FSI was correspondingly added during Hong Qu soaking, on day 5 of fermentation (representative of the fermentation stage), and cooled after sterilization. The FSI concentration in the wine was adjusted to 0, 0.1, 0.3, 0.5, 0.7, and 0.9 mg/mL. As the photodegradation of Monascus pigments in HQW generally occurs during storage, the young wine was stored in the dark at 4°C for 160 days, and changes were monitored. The aged wine was regularly sampled, and samples were stored at −80°C for further analysis.
Reducing sugar was detected using the method described by Miller (1959). Total acidity was determined according to Code of China Standard No. GB/T 5517-2010 (GB/T 5517-2010), and ethanol was determined using the HPLC method reported by Di Egidio et al. (2010), with the following conditions: a Carbomix H-NP (300 mm × 7.8 mm) column at a temperature of 55°C, a mobile phase of 2.5 mM sulfuric acid at a flow rate of 0.6 mL/min and using a differential detector. The amino acid nitrogen content in a 5-mL aliquot of HQW digested at 420 °C for 150 min was determined using an automatic Kjeldahl apparatus (Kjeltec 8400, FOSS Co., Sweden).
Volatile flavor components were extracted from HQW by solid-phase microextraction and analyzed by gas chromatography–mass spectrometry (GC-MS), according to the method reported by Yang et al. (2019). A 5-mL aliquot of each sample [diluted to 6% (v/v) ethanol] was placed in a 20-mL headspace glass vials with 2 g of sodium chloride and 10 μL internal standard of 4-methyl-2-pentanol (250 μg/mL). The volatile compounds were extracted for 30 min at 50°C using a 50 μm DVB/CAR/PDMS fiber. Then, the extracted fiber head was desorbed at the GC injection port at 250°C for 7 min for GC-MS analysis. The chromatographic conditions of GC-MS system (Thermo Fisher Inc., United States) equipped with a DB-WAX column (60 m × 0.25 mm × 0.25 μm, Agilent Technologies, United States) were as follows: programmed at an initial temperature of 40°C for 3 min, increased to 210°C at 6°C/min, and then, at 8°C/min to 230°C that is maintained for 15 min. Helium was delivered at a flow rate of 1 mL/min as the carrier gas. The conditions of the mass detector and identification of volatile compounds were operated as the method described by Yang et al. (2019). The quantity of volatile compounds was determined by the internal standard method.
The sensory evaluation team comprised 10 judges (five men and five women) who had been trained to evaluate the sensory attributes of HQW samples using a series of reference substances, according to the ISO Standard No. 8586-1 (ISO, 1993; Supplementary Table 1). After the judges reached a consensus on the sensory characteristics of all substances, they were given a sensory assessment card that listed all attributes with their definitions and 10-point scales (from 0 to 9). The scoring criteria were as follows: 0 meant very weak, 1–2 means weak, 3–4 meant average, 6 meant medium, 7–8 means high, and 9 meant extremely high. The evaluation was conducted in a room with a uniform light source and without noise or other distracting stimuli. The HQW samples were presented to the judges in a random order, in marked tulip-shaped glasses.
Each sample was analyzed in triplicate, and the results were expressed as mean values ± standard deviations (SD). Duncan’s test was used to analyze the significant differences between data using SPSS 19.0 software (SPSS Inc., Chicago, IL, United States). Partial least squares regression (PLSR) was used to explore the relationship among volatile flavor compounds, sensory attributes, and HQW samples containing different concentrations of FSI using UNSCRAMBLER ver. 9.7 (CAMO ASA, Oslo, Norway). TBtools (Chen et al., 2020) were used to visualize the differentiation of flavor compounds in different wines.
Photosensitive Monascus pigments may be degraded during the Hong Qu soaking stage of the brewing processes. Therefore, we investigated the preservation rates of Monascus pigments in HQW samples treated with different concentrations of FSI during different brewing stages (soaking, fermentation, and sterilization) (Figure 1). We found that the pigment-preservation rate increased with FSI concentration. Specifically, when 0.1–0.9 mg/mL FSI was added during Hong Qu soaking, the Monascus pigment-preservation rates increased by 20.68, 34.01, 39.33, 44.75, and 40.82% relative to the control (Figure 1A). When FSI was added during HQW fermentation, the pigment-preservation rate increased by 25.74, 36.25, 43.16, 58.46, and 53.02% relative to the control (Figure 1B). When FSI was added after sterilization, the rate increased by 34.89, 52.88, 66.87, 86.13, and 93.20% relative to the control (Figure 1C). After sterilization, the addition of 0.9 mg/mL FSI resulted in the greatest preservation rate of Monascus pigments. A similar pattern was observed for the appearance of HQW (Figure 1D). After natural aging for 160 d, the color of the control HQW had faded distinctly, whereas that of FSI-treated HQW remained satisfactory.
Figure 1. The preservation rate of Monascus pigments in HQW treated with different concentrations of FSI during Hong Qu soaking (A), wine fermentation (B), and after sterilization (C) during 160-days storage. Appearance comparison between HQW containing 0.9 mg/mL FSI and the control HQW (D).
The effective antioxidant compositions in FSI were analyzed using HPLC, with rutin, chlorogenic acid, and quercetin were used as references (Figure 2A). The result showed that FSI contained abundant rutin and quercetin and their contents were 68.64 and 23.75 mg/g, respectively (Figure 2B). Furthermore, the effects of rutin, quercetin, and FSI on the preservation rate of Monascus pigments under UV were compared (Figure 2C). After irradiation by UV for 10 h, the preservation rate of Monascus pigments in the control, solutions containing FSI, rutin, and quercetin correspondingly decreased from 100 to 33.2, 80.58, 68.05, and 70.53%. FSI exhibited better ability in the preservation of Monascus pigments than rutin or quercetin.
Figure 2. Effective constituent of FSI in the preservation of Monascus pigments. HPLC results of reference substances (A) and FSI (B). Effect of quercetin, rutin, and FSI on the preservation rate of Monascus pigments (C). Component 1: chlorogenic acid; Component 2: rutin; Component 3: quercetin.
We next evaluated the oenological properties of HQW treated with FSI during different brewing stages (Figure 3). When FSI was added during Hong Qu soaking or fermentation, the reducing sugar content in the resultant HQW increased significantly. In contrast, adding FSI after sterilization had little effect on this parameter (Figure 3A). Otherwise, the addition of FSI during different brewing stages had little effect on the ethanol, total acidity, and amino-acid nitrogen contents in the final wine samples (Figures 3B–D). Because the addition of FSI after sterilization had little effect on the oenological properties and yielding the best protection against Monascus pigments loss, only wine samples to which FSI had been added after sterilization were further analyzed.
Figure 3. The oenological properties of HQW treated with various concentrations of FSI during different brewing stages, comprising reducing sugar content (A), total acidity (B), amino acid nitrogen content (C), and ethanol content (D). **Indicates significant differences (p < 0.01, Duncan’s tests) between different HQW. NS indicates no significant difference between the wine samples.
The volatile flavor compounds of aged HQW treated with FSI after sterilization were analyzed (Table 1). A total of 47 compounds were detected, comprising 10 alcohols, 22 esters, six aldehydes, three phenols, two ketones, and four acids. The FSI had little effect on the detected number of flavor compounds, whereas the relative contents of flavor compounds were different in HQW samples containing different concentrations of FSI (Figure 4). In particular, the total content of volatile flavor compounds in HQW increased significantly as the added amounts of FSI were increased. HQW containing 0.9 mg/mL FSI exhibited the highest flavor-compound content (98.72 mg/L), followed by HQW containing 0.7 mg/mL FSI (95.61 mg/L), which were 10.64 and 7.15% higher than the control HQW, respectively (Table 1).
Table 1. Identification and relative contents of volatile flavor compounds in aged HQW (160 d) containing with different concentrations of FSI.
Figure 4. Differentiation of volatile flavor compounds in HQW containing different concentrations of FSI. The 0.1 FSI, 0.3 FSI, 0.5 FSI, 0.7 FSI, and 0.9 FSI represented HQW containing 0.1, 0.3. 0.5, 0.7, and 0.9 mg/mL FSI, respectively. The color and size of the circle represent the relative content of the flavor compound (the data has been standardized). The redder (the bigger) the circle, the higher the content of the flavor compound, and the bluer (the smaller) the circle, the lower content of the flavor compound.
As shown in Figure 4, esters were the most abundant aroma compounds, followed by alcohols and aldehydes. However, the total content of alcohols was the greatest, and thus responsible for the differentiation of total flavor compounds in different HQW. The addition of FSI significantly increased the total alcohol content in HQW. The highest alcohol content (62.36 mg/L) was observed in HQW containing 0.9 mg/L FSI, and this represented a 15.48% relative to the control. Among the detected alcohols, 3-methyl-1-butanol, 2-methyl-1-propanol, and 2-phenylethanol accounted for more than 95% of the total alcohols. Furthermore, the 3-methyl-1-butanol content gradually increased with the amount of FSI and was highest in HQW containing 0.9 mg/L FSI. The content of 2-phenylethanol in HQW containing FSI was significantly higher than that in the control, although the concentration of FSI had little effect on this parameter. Most of the detected esters in HQW were fatty-acid ethyl-esters (FAEE) (Figure 4 and Table 1)., comprising short-chain (SC, C2-C5), medium-chain (MC, C6-C12), and long-chain (LC, C13-C18) FAEE according to the chain length (Gao et al., 2018). As the FSI concentration was increased, the relative contents of SCFAEE and long-chain fatty acids ethyl esters (LCFAEE) in HQW also gradually increased. Compared to the control wine, the addition of 0.9 mg/L FSI increased the SCFAEE content by 20.95% in the resultant HQW, but only increased the LCFAEE content by 3.03% (Table 1). Benzaldehyde, phenylacetaldehyde, and furfural were identified as the key aldehydes in HQW (Yang et al., 2017; Liu Z. et al., 2020). The content of benzaldehyde determined the total content of aldehydes in each HQW (Table 1). However, no significant difference was observed between the total aldehyde contents in HQW with and without FSI, indicating that the addition of FSI had little effect on the aldehyde content of HQW during aging.
The aged HQW samples treated with different FSI concentrations after sterilization were subjected to a sensory evaluation. The mouthfeel characteristic of astringency, full-body, and aftertaste-continuation varied little in HQW containing different concentrations of FSI (Figure 5). In contrast, a significant difference in the aroma of alcohol, cereal, and fruity was observed. Different wines were perceived to have similar levels of sweet taste, however, the wines received significantly different scores for the taste of sour, despite a lack of significant differences in the total acidity. In summary, although the overall mouthfeel characteristics were similar between HQW samples with and without FSI, the concentration of FSI significantly affected the aroma characteristics of the wine. HQW treated with 0.9 mg/mL FSI received the highest scores for the total mouthfeel and aroma.
Figure 5. Scores of sensorial attributes for HQW treated with different concentrations of FSI after sterilization and aged for 160 days.
A multivariate analysis of the variance-PLSR model was performed to study the relationship between flavor compounds and sensory attributes in samples of HQW with and without FSI. A total of 53 identified flavor compounds were designed as the X-matrix, while nine sensory attributes and six wine samples were designed as Y-matrix (Figure 6). The two-factor model explained 63% of the variance in X-matrix and 76% of that in Y-matrix, indicating that this model satisfactorily explained the flavor compounds and sensory attributes. Except for nonanal, benzaldehyde, ethyl nonanoate, ethyl dodecanoate, ethyl stearate, ethyl (9E)-9-octadecenoate, acetic acid, hexanoic acid, octanoic acid, 2-octanone, 2-furanmethanol, and 2-methoxy-phenol, all of the sensory attributes and flavor compounds were located between the small and big ellipses, where the flavor compounds could be considered as correlated with sensory attributes (Xiao et al., 2014).
Figure 6. Correlataion loadings plot between the fifty-three volatile flavor compounds and nine sensory attributes in HQW containing different concentrations of FSI (The codes were defined in Table 1).
Monascus pigments, as the distinguished component in HQW, its photosensitivity characteristic significantly affects the sensory quality of the resulting wine. During the manufacturing processes of HQW, Monascus pigments may be degraded from the stage of Hong Qu soaking, wine fermentation, and sterilization. Though the pigment-preservation rate of HQW increased with FSI concentration, adding FSI after sterilization resulted in the greatest reduction of Monascus pigment loss in HQW, especially when added at a concentration of 0.9 mg/mL.
Typically, the ionization reaction occurs in a water solution of Monascus pigments under light, generating superoxide anions, hydroxyl radicals, and radicals originated by the side chain fracture (Feng et al., 2012). These radicals undergo an addition reaction with the double bonds in the pigment structure, which caused the disappearance of conjugated double bonds in Monascus pigments, and led to the loss of color (Mapari et al., 2009; Patakova, 2013). The antioxidant substances formed chemical bonds between its hydroxyl and the ketone group on the molecular structure of Monascus pigments, thereby stabilizing the structure of pigments (Kennedy et al., 1999). Reportedly, FSI is rich in antioxidant substances, such as rutin, quercetin, and chlorogenic acid, which might be related to its ability to preserve pigment under light (Hu et al., 2016). According to the HPLC analysis, the major antioxidant constituents of FSI were rutin and quercetin, and the content of rutin was significantly higher than quercetin (Figure 2). After UV irradiation, FSI exhibited better ability in the preservation of Monascus pigments than rutin or quercetin, suggesting that the combination of antioxidants enhanced the protective effect on Monascus pigments. However, the combination of multiple antioxidants greatly increases the cost. As a natural extraction product, FSI can replace the conventional antioxidants to protect natural pigments from light irradiation.
While adding FSI after HQW sterilization, it had little effect on the oenological properties, indicating that there was no need to change the production parameters due to the FSI treatment (Figure 3). However, FSI treatment contributed greatly to the flavor profiles of HQW. During the fermentation of HQW, the antioxidant constituents in FSI can prevent the growth and reproduction of microorganisms that contributed greatly to the stability of Monascus pigments (Tang et al., 2019). This may responsible for the reason that adding FSI after sterilization exhibited the best inhibitory effect on the Monascus pigment photodegradation. In addition, adding FSI during the Hong Qu soaking process or fermentation process will inhibit the metabolism of yeast, which generates a range of volatile flavor compounds and results in the specific flavor characteristic of HQW (Chen and Xu, 2012; Cai et al., 2018). This phenomenon affects its utilization as it reduces the sugar by microorganisms (including yeast), contributing to the formation of flavor compounds in HQW. After sterilization, the fresh HQW had an insufficient aroma and a rough taste, and a variety of chemical reactions such as oxidation, esterification, and hydrolysis reactions gradually occur (Jones et al., 2008; Tao et al., 2014). The added FSI may promote the chemical reactions among some flavor compounds, thereby affecting the flavor profiles of HQW. Generally, the flavor of Huangjiu is attributed to the combination of alcohols, esters, and aldehydes that make up the structural components of HQW aroma (Liu Z. et al., 2020). With FSI treatment, a significant difference in the total content of alcohols was observed in HQW samples containing different FSI concentrations, and FSI strongly enhanced the formation of 3-methyl-1-butanol and 2-methyl-1-propanol. In contrast, the total content of esters in HQW with FSI was generally lower than that in the control wine, except for HQW containing 0.7 and 0.9 mg/L FSI (Figure 4 and Table 1). At nearly all concentrations of FSI, the content of MCFAEE was significantly lower in treated HQW samples relative to the control; however, treatment with 0.9 mg/L FSI led to a higher content of MCFAEE relative to the control. These data suggested that the addition of FSI significantly promoted the accumulation of SCFAEE during HQW aging. As for aldehydes, the addition of FSI had little effect on the aldehyde content of HQW during aging. Thus, the FSI treatment improves the flavor profiles of HQW by affecting the production of alcohols and esters, especially 3-methyl-1-butanol, 2-methyl-1-propanol, and SCFAEE. Combining with the sensory evaluation (Figure 5), the treatment of 0.9 mg/mL FSI considerably highlighted the alcohol-aroma, cereal-aroma, and fruit-aroma in the aged HQW, consistent with the content of high alcohols and esters shown in Figure 4. The similar score of sweet taste in different HQW samples corresponded well with oenological properties, as the sweet attribute is partly related to the content of reducing sugar (Figure 3). The sour taste, which is generally related to the presence of organic acids (Dysvik et al., 2020), scored different levels suggesting that the FSI might have significantly affected the flavor-active organic acids in HQW.
As shown in Figure 6, the HQW samples treated with different FSI concentrations were located in different quadrants, suggesting that the concentration of FSI had a significant effect on the sensory characteristic of the resultant HQW. HQW treated with 0.7 mg/mL FSI exhibited a similar sensory profile as the control wine, and this profile was closely related to the presence of MCFAEE, 1-octen-3-ol, 3-methylthiopropanol, isoamyl acetate, diethyl succinate, ethyl tetradecanoate, and continuation. However, treatment with 0.7 mg/mL FSI was strongly correlated with a sour taste. Treatment with 0.1 mg/mL FSI was correlated to astringency mouthfeel, indicating that different FSI concentrations increased certain sensory attributes. Treatment with 0.9 mg/mL FSI correlated strongly with a full body and fruit aroma, and was favored by consumers. The sensory characteristic of the resultant HQW depended greatly on the concentration of FSI. The addition of 0.9 mg/mL FSI yielded a satisfactory result, consistent with the sensory evaluation (Figure 5). The key attributes of the fruit and alcohol aroma were closely related to the presence of SCFAEE and to that of 1-butanol and 2-phenylethanol, respectively. The high odor activity values of ethyl acetate, ethyl butanoate, ethyl hexanoate, and 2-phenylethanol might be responsible for the fruit aroma (Yang et al., 2018). Cereal-aroma was strongly correlated with the presence of LCFAEE, 2-nonanone, ethyl-2-hydroxypropanoate and 2-methyl-1-propanol, and different types of flavor compounds (esters, alcohols, aldehydes, and ketones) suggested a complex origin for this aroma attribute (Mo et al., 2010; Liu S. et al., 2019). Both a full body and fruit aroma were strongly correlated with the presence of SCFAEE. Combining with the result of flavor compounds that the SCAFEE content was significantly accumulated by adding FSI (Figure 4), it suggested that FSI may alter the aroma of HQW by enhancing the synthesis of SCFAEE. Thus, the addition of FSI to HQW after sterilization may be a novel alternative strategy for obtaining HQW with a high-quality flavor profile.
In this study, we demonstrated that the photodegradation of Monascus pigments in HQW could be reduced significantly by adding FSI to wine after sterilization. Specifically, FSI considerably enhanced the accumulation of alcohols and esters as the added amounts were increased, especially 3-methyl-1-butanol, 2-methyl-1-propanol, and SCFAEE, in HQW. Furthermore, FSI enhanced the alcohol, cereal, and fruit aromas in HQW. HQW treated with 0.9 mg/mL FSI received the highest scores for the mouthfeel and aroma. A PLSR analysis of the HQW samples revealed that a pleasing aroma was strongly related to the presence of SCFAEE, the accumulation of which was significantly enhanced by FSI. Therefore, we inferred that FSI might alter the aroma of HQW by enhancing the synthesis of SCFAEE. Taken together, our results suggest the considerable potential of FSI treatment as a strategy for reducing the photodegradation of Monascus pigments, thus improving the color stability, flavor profile, and sensory characteristics of the resulting HQW. Our work provides a new strategy for improving the pigment stability and sensory quality of HQW and other beverages containing photosensitive pigments. However, further studies are needed to explore the bioactive constituents of FSI in detail and the mechanisms by which FSI enhances the formation of SCFAEE.
The raw data supporting the conclusions of this article will be made available by the authors, without undue reservation.
We obtained informed consent from participants in the sensory evaluation in accordance with the Code of Ethics of the World Medical Association (Declaration of Helsinki).
YY: experiment, investigation, software, data curation, and writing—original draft. YX: conceptualization, methodology, project administration, and writing—review and editing. XS: methodology, project administration, writing—review and editing. ZM: experiment, resources, and methodology. HQ: experiment, resources, methodology, and visualization. LT: conceptualization, software, and investigation. LA: supervision, writing—original draft, and writing—review and editing. All authors contributed to the article and approved the submitted version.
This work was financially supported by the National Natural Science Foundation of China (No. 32001674), the Shanghai Agriculture Applied Technology Development Program, China (2019-02-08-00-07-F01152); the Shanghai Technical Standard Program, China (18DZ2200200); and the Shanghai Engineering Research Center of food microbiology program (19DZ2281100).
The authors declare that the research was conducted in the absence of any commercial or financial relationships that could be construed as a potential conflict of interest.
The Supplementary Material for this article can be found online at: https://www.frontiersin.org/articles/10.3389/fmicb.2021.678903/full#supplementary-material
PLSR, partial least squares regression; SCFAEE, short-chain fatty acid ethyl esters; MCFAEE, medium-chain fatty acids ethyl esters; LCFAEE, long-chain fatty acids ethyl esters.
Abdelhady, M., Kamal, A., Othman, S., Mubarak, M., and Hadda, T. (2015). Total polyphenolic content, antioxidant, cytotoxic, antidiabetic activities, and polyphenolic compounds of Sophora japonica grown in Egypt. Med. Chem. Res. 24, 482–495. doi: 10.1007/s00044-014-1101-2
Cai, H., Zhang, T., Zhang, Q., Luo, J., Cai, C., and Mao, J. (2018). Microbial diversity and chemical analysis of the starters used in traditional Chinese sweet rice wine. Food Microbiol. 73, 319–326. doi: 10.1016/j.fm.2018.02.002
Chen, C., Chen, H., Zhang, Y., Thomas, H. R., Frank, M. H., He, Y., et al. (2020). TBtools-an integrative toolkit developed for interactive analyses of big biological data. Mol. Plant 13, 1194–1202. doi: 10.1016/j.molp.2020.06.009
Chen, S., and Xu, Y. (2012). The influence of yeast strains on the volatile flavour compounds of Chinese rice wine. J. Instit. Brewing 116, 190–196. doi: 10.1002/j.2050-0416.2010.tb00417.x
Dhale, M., Javagal, M., and Puttananjaiah, M. (2018). Protective and antioxidative effect of rubropunctatin against oxidative protein damage induced by metal catalyzed reaction. Int. J. Biol. Macromol. 116, 409–416. doi: 10.1016/j.ijbiomac.2018.04.170
Di Egidio, V., Sinelli, N., Giovanelli, G., Moles, A., and Casiraghi, E. (2010). NIR and MIR spectroscopy as rapid methods to monitor red wine fermentation. Eur. Food Res. Technol. 230, 947–955. doi: 10.1007/s00217-010-1227-5
Dysvik, A., La Rosa, S., Liland, K., Myhrer, K., Østlie, H., De Rouck, G., et al. (2020). Co-fermentation involving Saccharomyces cerevisiae and Lactobacillus Species tolerant to brewing-related stress factors for controlled and rapid production of sour beer. Front. Microbiol. 11:279. doi: 10.3389/fmicb.2020.00279
Feng, Y., Shao, Y., and Chen, F. (2012). Monascus pigments. Appl. Microbiol. Biotechnol. 96, 1421–1440. doi: 10.1007/s00253-012-4504-3
Gao, Q., Cao, X., Huang, Y., Yang, J., Chen, J., Wei, L., et al. (2018). Overproduction of fatty acid ethyl esters by the oleaginous yeast Yarrowia lipolytica through metabolic engineering and process optimization. ACS Synthetic Biol. 7, 1371–1380. doi: 10.1021/acssynbio.7b00453
Horuz, E., Bozkurt, H., Karataş, H., and Maskan, M. (2017). Effects of hybrid (microwave-convectional) and convectional drying on drying kinetics, total phenolics, antioxidant capacity, vitamin C, color and rehydration capacity of sour cherries. Food Chem. 230, 295–305. doi: 10.1016/j.foodchem.2017.03.046
Hu, Z., Li, S., Li, C., and Li, Z. (2016). Chitosan-assisted isolation and antioxidant evaluation of flavonoids from Sophora japonica L. BMC Plant Biology 16:10–24. doi: 10.1186/s12870-016-0904-3
Huang, R., Zhang, Y., Zhang, Y., Zhang, L., Pei, L., Shu, G., et al. (2020). Evaluation of the synergetic effect of Yupingfeng san and Flos Sophorae Immaturus based on free radical scavenging capacity. Biomed. Pharmacother. 128, 110265. doi: 10.1016/j.biopha.2020.110265
Huang, Z., Guo, W., Zhou, W., Li, L., Xu, J., Hong, J., et al. (2019). Microbial communities and volatile metabolites in different traditional fermentation starters used for Hong Qu glutinous rice wine. Food Res. Int. 121, 593–603. doi: 10.1016/j.foodres.2018.12.024
ISO (1993). Sensory Analysis—General Guidance for Selection, Training and Monitoring of Assessors. Switzerland: International Organization for Standardization Geneva.
Jones, P. R., Gawel, R., Francis, I. L., Francis, I. L., and Waters, E. J. (2008). The influence of interactions between major white wine components on the aroma, flavour and texture of model white wine. Food Q. Pref. 19, 596–607. doi: 10.1016/j.foodqual.2008.03.005
Keekan, K., Hallur, S., Modi, P., and Shastry, R. (2020). Antioxidant activity and role of culture condition in the optimization of red pigment production by Talaromyces purpureogenus KKP through response surface methodology. Curr. Microbiol. 77, 1780–1789. doi: 10.1007/s00284-020-01995-4
Kennedy, J., Auclair, K., Kendrew, S., Park, C., Vederas, J., and Hutchinson, C. (1999). Modulation of polyketide synthase activity by accessory proteins during lovastatin biosynthesis. Science 284, 1368–1372. doi: 10.1126/science.284.5418.1368
Li, Y., and Fan, L. (2020). Comparative studies on the stabilization of flos sophorae immaturus beverages by various hydrocolloids. LWT 123:109117. doi: 10.1016/j.lwt.2020.109117
Lin, C., Lin, T., and Pan, T. (2017). Alleviation of metabolic syndrome by monascin and ankaflavin: the perspective of Monascus functional foods. Food Funct. 8, 2102–2109. doi: 10.1039/C7FO00406K
Liu, C., Yan, Y., Zhang, X., Mao, Y., Ren, X., Hu, C., et al. (2020). Regulating the pro-and antioxidant capabilities of bimetallic nanozymes for the detection of Fe 2+ and protection of Monascus pigments. Nanoscale. 12, 3068–3075. doi: 10.1039/C9NR10135G
Liu, S., Yang, L., Zhou, Y., He, S., Li, J., Sun, H., et al. (2019). Effect of mixed moulds starters on volatile flavor compounds in rice wine. LWT 112:108215. doi: 10.1016/j.lwt.2019.05.113
Liu, T., Wang, S., Ma, H., Jin, H., Li, J., Yang, X., et al. (2019). Microwave-assisted extraction combined with in-capillary [Fe (ferrozine) 3] 2+-CE-DAD to screen active components with the ability to chelate ferrous ions from Flos Sophorae Immaturus (Flos Sophorae). Molecules 24:3052. doi: 10.3390/molecules24173052
Liu, Z., Wang, Z., Sun, J., and Ni, L. (2020). The dynamics of volatile compounds and their correlation with the microbial succession during the traditional solid-state fermentation of gutian hong qu glutinous rice wine. Food Microbiol. 86:103347. doi: 10.1016/j.fm.2019.103347
Mapari, S. A., Meyer, A. S., and Thrane, U. (2009). Photostability of natural orange- red and yellow fungal pigments in liquid food model systems. J. Agric. Food Chem. 57, 6253–6261. doi: 10.1021/jf900113q
Mateo-Fernández, M., Alves-Martínez, P., Río-Celestino, D., Font, R., Merinas-Amo, T., and Alonso-Moraga, Á (2019). Food safety and nutraceutical potential of caramel colour class IV using in vivo and in vitro assays. Foods 8:392. doi: 10.3390/foods8090392
Miller, G. (1959). Use of dinitrosalicylic acid reagent for determination of reducing sugar. Anal. Chem. 31, 426–428. doi: 10.1021/ac60147a030
Mo, X., Xu, Y., and Fan, W. (2010). Characterization of aroma compounds in Chinese rice wine Qu by solvent-assisted flavor evaporation and headspace solid-phase microextraction. J. Agric. Food Chem. 58, 2462–2469. doi: 10.1021/jf903631w
Mondal, S., Pandit, S. G., Puttananjaiah, M. H., Harohally, N. V., and Dhale, M. A. (2019). Structural and functional characterization of new pigment molecule monashin from monascus purpureus CFR410-11. Proc. Biochem. 82, 173–178. doi: 10.1016/j.procbio.2019.04.004
Park, K., Liu, Z., Park, C., and Ni, L. (2016). Microbiota associated with the starter cultures and brewing process of traditional Hong Qu glutinous rice wine. Food Sci. Biotechnol. 25, 649–658. doi: 10.1007/s10068-016-0115-6
Patakova, P. (2013). Monascus secondary metabolites: production and biological activity. J. Indust. Microbiol. Biotechnol. 40, 169–181. doi: 10.1007/s10295-012-1216-8
Qian, C., Decker, E., Xiao, H., and McClements, D. (2012). Nanoemulsion delivery systems: influence of carrier oil on β-carotene bioaccessibility. Food Chem. 135, 1440–1447. doi: 10.1016/j.foodchem.2012.06.047
Sen, T., Barrow, C., and Deshmukh, S. (2019). Microbial pigments in the food industrychallenges and the way forward. Front. Nut. 6:7. doi: 10.3389/fnut.2019.00007
Tang, R., Luo, J., Wang, W., Liu, D., Wang, G., and Guo, X. (2019). Rutin’s natural source flos sophorae as potential antioxidant and improver of fungal community in Chinese sausages. LWT 101, 435–443. doi: 10.1016/j.lwt.2018.11.064
Tao, Y., García, J. F., and Sun, D. W. (2014). Advances in wine aging technologies for enhancing wine quality and accelerating wine aging process. Crit. Rev. Food Sci. Nut. 54, 817–835. doi: 10.1080/10408398.2011.609949
Vendruscolo, F., Bühler, R., de Carvalho, J., de Oliveira, D., Moritz, D., Schmidell, W., et al. (2016). Monascus: a reality on the production and application of microbial pigments. Appl. Biochem. Biotechnol. 178, 211–223. doi: 10.1007/s12010-015-1880-z
Vollmuth, T. (2018). Caramel color safety–an update. Food Chem. Toxicol. 111, 578–596. doi: 10.1016/j.fct.2017.12.004
Wang, J., Li, L., Tan, J., Song, X., Chen, D., Xu, J., et al. (2019). Variations in the components and antioxidant and tyrosinase inhibitory activities of Styphnolobium japonicum (L.) schott extract during flower maturity stages. Chem. Biodiversity 16:e1800504. doi: 10.1002/cbdv.201800504
Wang, J., Yuan, C., Gao, X., Kang, Y., Huang, M., Wu, J., et al. (2020). Characterization of key aroma compounds in Huangjiu from northern China by sensory-directed flavor analysis. Food Res. Int. 134:109238. doi: 10.1016/j.foodres.2020.109238
Wang, L., Dai, Y., Chen, W., Shao, Y., and Chen, F. (2016). Effects of light intensity and color on the biomass, extracellular red pigment, and citrinin production of Monascus ruber. J. Agric. Food Chem. 64, 9506–9514. doi: 10.1021/acs.jafc.6b04056
Xiao, Z., Yu, D., Niu, Y., Chen, F., Song, S., Zhu, J., et al. (2014). Characterization of aroma compounds of Chinese famous liquors by gas chromatography–mass spectrometry and flash GC electronic-nose. J. Chromatography B 945, 92–100. doi: 10.1016/j.jchromb.2013.11.032
Yang, Y., Xia, Y., Lin, X., Wang, G., Zhang, H., Xiong, Z., et al. (2018). Improvement of flavor profiles in Chinese rice wine by creating fermenting yeast with superior ethanol tolerance and fermentation activity. Food Res. Int. 108, 83–92. doi: 10.1016/j.foodres.2018.03.036
Yang, Y., Xia, Y., Wang, G., Tao, L., Yu, J., and Ai, L. (2019). Effects of boiling, ultra-high temperature and high hydrostatic pressure on free amino acids, flavor characteristics and sensory profiles in Chinese rice wine. Food Chem. 275, 407–416. doi: 10.1016/j.foodchem.2018.09.128
Yang, Y., Xia, Y., Wang, G., Zhang, H., Xiong, Z., Yu, J., et al. (2017). Comparison of oenological property, volatile profile, and sensory characteristic of Chinese rice wine fermented by different starters during brewing. Int. J. Food Propert. 20, S3195–S3211. doi: 10.1080/10942912.2017.1325900
Yao, W., Wang, S., Chen, Y., and Wang, H. (2011). Composition and antibacterial activity of essential oils of Flos sophorae immaturus. Int. J. Food Propert. 14, 903–913. doi: 10.1080/10942910903474419
Keywords: Hong Qu Huangjiu, Flos sophorae immaturus, Monascus pigments, flavor profiles, sensory characteristic
Citation: Yang Y, Xia Y, Song X, Mu Z, Qiu H, Tao L and Ai L (2021) The Potential of Flos sophorae immaturus as a Pigment-Stabilizer to Improve the Monascus Pigments Preservation, Flavor Profiles, and Sensory Characteristic of Hong Qu Huangjiu. Front. Microbiol. 12:678903. doi: 10.3389/fmicb.2021.678903
Received: 10 March 2021; Accepted: 12 April 2021;
Published: 20 May 2021.
Edited by:
Xucong Lv, Fuzhou University, ChinaReviewed by:
Huaixiang Tian, Shanghai Institute of Technology, ChinaCopyright © 2021 Yang, Xia, Song, Mu, Qiu, Tao and Ai. This is an open-access article distributed under the terms of the Creative Commons Attribution License (CC BY). The use, distribution or reproduction in other forums is permitted, provided the original author(s) and the copyright owner(s) are credited and that the original publication in this journal is cited, in accordance with accepted academic practice. No use, distribution or reproduction is permitted which does not comply with these terms.
*Correspondence: Lianzhong Ai, YWlsaWFuemhvbmcxQDEyNi5jb20=; YWlsaWFuemhvbmdAaG90bWFpbC5jb20=
Disclaimer: All claims expressed in this article are solely those of the authors and do not necessarily represent those of their affiliated organizations, or those of the publisher, the editors and the reviewers. Any product that may be evaluated in this article or claim that may be made by its manufacturer is not guaranteed or endorsed by the publisher.
Research integrity at Frontiers
Learn more about the work of our research integrity team to safeguard the quality of each article we publish.