- 1Dipartimento di Scienze e Innovazione Tecnologica, Università del Piemonte Orientale, Alessandria, Italy
- 2Dipartimento di Scienze e Innovazione Tecnologica, Università del Piemonte Orientale, Vercelli, Italy
- 3SmartSeq s.r.l., spin-off of the Università del Piemonte Orientale, Alessandria, Italy
Arbuscular mycorrhizal fungi (AMF) are beneficial soil microorganisms that can establish symbiotic associations with Vitis vinifera roots, resulting in positive effects on grapevine performance, both in terms of water use efficiency, nutrient uptake, and replant success. Grapevine is an important perennial crop cultivated worldwide, especially in Mediterranean countries. In Italy, Piedmont is one of the regions with the longest winemaking tradition. In the present study, we characterized the AMF communities of the soil associated or not with the roots of V. vinifera cv. Pinot Noir cultivated in a vineyard subjected to conventional management using 454 Roche sequencing technology. Samplings were performed at two plant phenological stages (flowering and early fruit development). The AMF community was dominated by members of the family Glomeraceae, with a prevalence of the genus Glomus and the species Rhizophagus intraradices and Rhizophagus irregularis. On the contrary, the genus Archaeospora was the only one belonging to the family Archaeosporaceae. Since different AMF communities occur in the two considered soils, independently from the plant phenological stage, a probable role of V. vinifera in determining the AMF populations associated to its roots has been highlighted.
Introduction
Arbuscular mycorrhizal fungi (AMF) are beneficial symbiotic soil microorganisms that improve the plant nutritional state by increasing the interface area between roots and soil (Hodge et al., 2010). Moreover, the arbuscular mycorrhizal symbiosis provides other advantages for plants, such as better tolerance versus biotic or abiotic stresses (Hodge et al., 2010; Bona et al., 2011; Lingua et al., 2012; Degola et al., 2015) and improved fruit yield and quality (Baslam et al., 2013; Berta et al., 2014; Bona et al., 2015, 2017, 2018; Todeschini et al., 2018).
Grapevine (Vitis vinifera L.) is an important perennial crop cultivated in all continents where the climatic conditions are permissive. Italy is one of the five major grape producers in the world, with about 8,600,000 tons which represent 11% of the production in the world (IOV, 2019). It has been widely demonstrated that the inclusion of fresh grape and its derivates in the diet (Vislocky and Fernandez, 2010) and also the reasonable consumption of wine (Georgiev et al., 2014; Artero et al., 2015) can give beneficial effects for human health, decreasing the risk factors associated with cancer and age-related cognitive decline as well as cardiovascular and neurodegenerative diseases (Torres et al., 2018). Pinot Noir is a grapevine cultivar from which both white and red fine wines, with typical organoleptic characteristics, are produced all over the world. In particular, the surface area dedicated to this cultivation corresponds to 112,000 ha. Germany, Italy, and Switzerland in Europe and United States, New Zealand, and Australia in non-European countries are the main producers (IOV, 2017). Grapevines, during their life cycle, are subjected to various cultivation practices which can interfere with the native microbiota and also the fungal soil population. It is well documented that practices like tillage, as well as the use of fertilizers and/or pesticides, can reduce soil microbial biodiversity (Berruti et al., 2014; Trouvelot et al., 2015; Zaller et al., 2018; Nogales et al., 2019). The intensity and frequency of these practices vary according to the type of vineyard management, which can be classified into conventional, organic, and/or integrated (Likar et al., 2017; Zaller et al., 2018). The growth and development of grapevines are dependent on AMF (Linderman and Davis, 2001; Schreiner, 2005), and the occurrence of species specificity between V. vinifera and AMF has been observed (Holland et al., 2014). In addition, if compared to non-native ones, AMF native of a certain area are often reported to be more effective in plant growth promotion (Schreiner et al., 2006). In order to realize and manage a sustainable agricultural ecosystem, the study of AMF communities associated with grapevines, in the context of conventional management, becomes of great importance (Likar et al., 2013). Several works described the biodiversity of AMF in vineyards subjected to conventional management. The AMF community of two differently managed vineyards (tilled and covered) in Sardinia was characterized by Lumini et al. (2010). Conventional management of the vineyard leads to the development of different fungal and bacterial microbial communities according to specific local biogeographic factors (Likar et al., 2017). The differences between AMF communities in a vineyard and in nearby unmanaged areas were analyzed in order to highlight the impact of viticulture on AMF community diversity and composition (Holland et al., 2016). Finally, AMF biodiversity was studied in the roots of V. vinifera cv. Pinot Noir and Chardonnay in Burgundy (France) and Oregon (United States) (Bouffaud et al., 2016; Schreiner, 2020). In the past, the study of AMF communities was exclusively based on the morphological identification of isolated spores. More recently, this methodology has been complemented and/or replaced by molecular techniques, applied both to roots and soils as, for example, cloning followed by Sanger sequencing (Vasar et al., 2017). Then, since the early 2000s, the use of next-generation sequencing, including Roche 454 platform, allowed the analysis of a huge number of sequences (hundreds of thousands) per sample, enormously increasing the depth of investigation. Molecular approaches are based on nuclear ribosomal markers such as the small subunit (SSU) rRNA gene, the internal transcribed spacer region (ITS), and the large subunit (LSU) rRNA gene (Öpik and Davison, 2016). Based on the idea that Piedmont has winemaking tradition that we can define as historic, it becomes of extreme ecological and applicative importance to get information on the AMF communities associated with the vines. In this geographical zone, we therefore identified a vineyard cultivated with grapevine cv. Pinot Noir and subjected to conventional management. A detailed description of the native AMF communities of the soils associated or not with the grapevine roots at two plant phenological stages (flowering and early fruit development) was obtained.
Materials and Methods
Soil Sampling
The experimental vineyard is located in Mantovana (Predosa municipality, Alessandria, Southern Piedmont, Italy – altitude: 215 m a.s.l., latitude: 44.730294° N, and longitude: 8.6226556°E), and it is subjected to conventional management. Glyphosate treatment was performed in the vineyard in June. Trifloxistrobin and Fosetyl-Al + copper were employed as fungicides against Oidium spp. and Peronospora spp., respectively, and were distributed in June and July, coupled with one insecticide (thiamethoxan) and two sulfur treatments in July.
The soil, hereafter indicated as Bs, was sampled close to the vineyard, in a not cultivated area covered in part with grasses (Figure 1). The soil associated to the roots (Rs) of V. vinifera cv. Pinot Noir, grafted onto SO4 rootstock, was collected from grapevine roots entrapped in the soil cores taken near the plant. Samplings were carried out in May 2014 (Bs1S and Rs1S, flowering) and July 2014 (Bs2S and Rs2S, early fruit development). For each soil (Bs or Rs) and time point (1S or 2S), five samples were collected from the topsoil (5–30 cm). According to the Italian guide for soil analysis (GU 179/2002), for each plant, three soil cores were taken, pooled, and mixed to prepare one sample. The soil samples were stored at −20°C until DNA extraction.
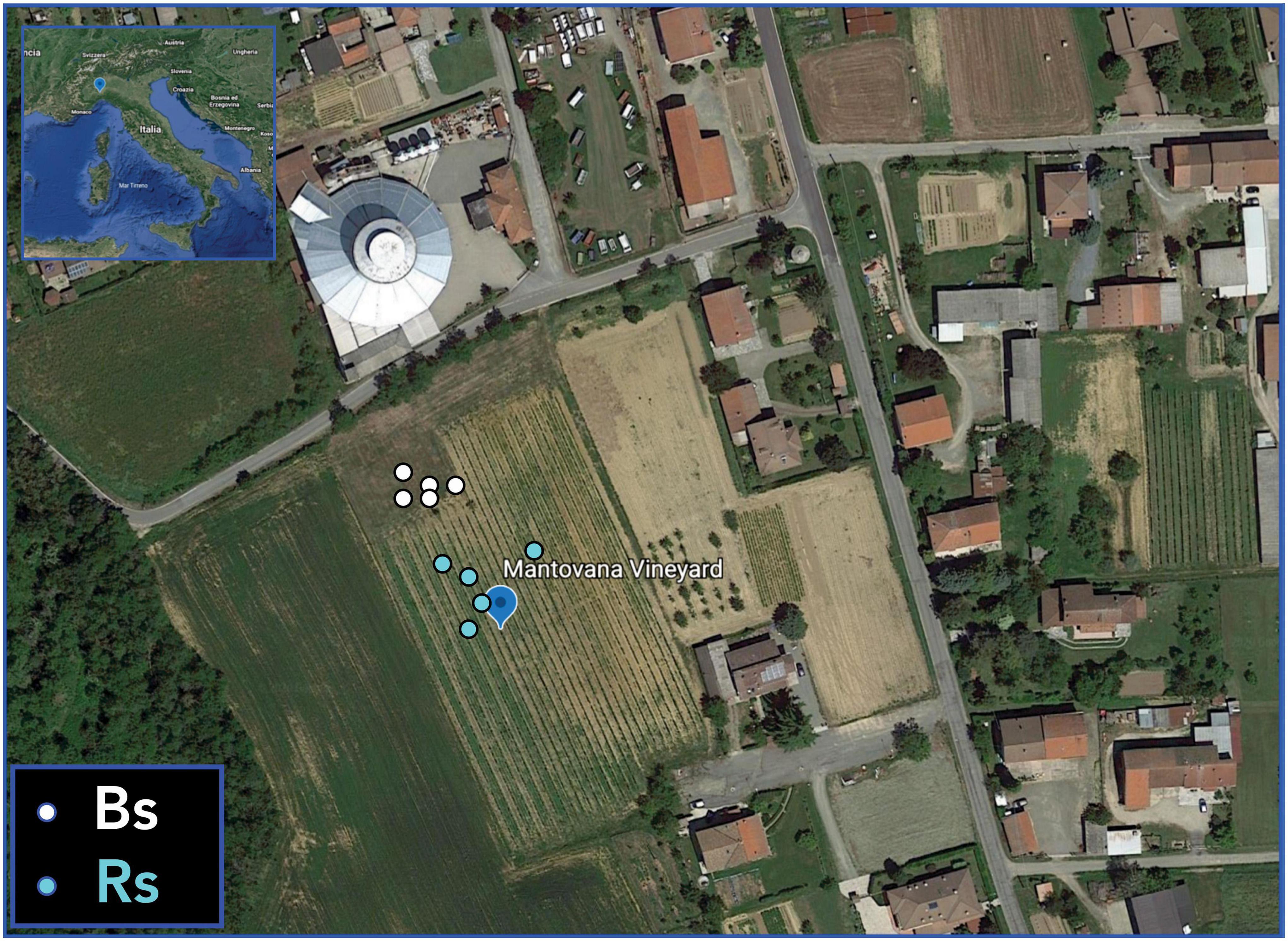
Figure 1. Vineyard aerial view showing the sampling points. The vineyard is located in Mantovana (Predosa municipality, Alessandria, Italy). The two soil sampling sites included one in an area just outside the borders of the vineyard in the absence of grapevines (Bs, white dots) and one inside the vineyard corresponding to the grapevine plants (Rs, cyan dots). Google Earth online version was used to produce this image (https://earth.google.com/web/).
The soil of the vineyard was clay-loam and acidic, as reported in Gamalero et al. (2020); moreover, the climatic conditions of the area, such as temperature, humidity, and rainfall, are detailed in Bona et al. (2019).
AMF Root Colonization
Mycorrhizal colonization was evaluated microscopically following the method of Trouvelot et al. (1986) as mycorrhizal frequency, degree of AMF root colonization, and arbuscule and vesicle abundance. Briefly, from grapevine roots, 30 randomly chosen 1-cm-long pieces were cut, cleared at 80°C for 90 min in 10% KOH, stained with 1% methyl blue in lactic acid, and mounted onto slides. The results were analyzed by ANOVA; differences were considered statistically significant for p-values less than 0.05.
DNA Extraction and Amplification
Power Soil R DNA Isolation Kit (MO BIO Laboratories, Inc., Carlsbad, CA, United States) was used, following the manufacturer’s instructions, to extract DNA from five samples, both of Bs and of the soil associated with the roots of V. vinifera (Rs), collected at flowering (1S) or at fruiting (2S) times. A hemi-nested PCR, employing as template the previously extracted DNA, was performed using LR1 and FLR2 (Cesaro et al., 2008) primers for the first amplification and LR1 and FLR4 (Cesaro et al., 2008) primers tagged with Multiplex Identifier sequences for 454 Pyrosequencing (Roche) for the second one. In particular, the FLR2 and FLR4 primers are specific for fungi and for Glomeromycota, respectively (Farmer et al., 2007). The reactions were performed at the conditions described in Massa et al. (2020).
Pyrosequencing employing 454 technology was performed on the products of the second PCR (size, 700 bp). DNA-carrying beads were loaded on a PicoTiterTM plate and surrounded by enzyme beads (sulfurylase and luciferase). The light signals were represented in flow grams and analyzed; a nucleotide sequence was determined for each read with the GS Amplicon Variant Analyzer software.
Bioinformatic Analysis
Data were analyzed using a custom bioinformatic pipeline as fully described in Massa et al. (2020). Raw sequence reads were demultiplexed. The reads with the following characteristics were discarded: (1) read length less than 200 nucleotides, (2) average Phred quality score less than 25 (Ewing et al., 1998), and (3) presence of at least one ambiguous base inside the read. Then, an alignment of each sequence was performed against our AMF LSU rDNA database, consisting of 3.803 univocal sequences downloaded from online sources: EBI and SILVA databases, and from the web site1 (Krüger et al., 2011). Our database was prepared as described in Massa et al. (2020). The alignment of each sequence was performed using BLASTN (Altschul et al., 1997). Two criteria were applied in order to identify the taxa at species level (named “known”): coverage ≥80% and similarity of sequences ≥97% according to Lindahl et al. (2013) and Hart et al. (2015). Following these criteria, chimeras were also removed. All the sequences that did not satisfy both afore-mentioned criteria were then aligned against themselves. After comparing one sequence to each other, all those with coverage ≥80% and similarity of sequences ≥97% were grouped together, and each group was named de novo (Massa et al., 2020).
Bioinformatic analysis was performed on a database containing the results normalized at 8,000 sequences. The rarefaction curves were plotted with the RAM package of R (R Core Team., 2018; Supplementary Figure 1).
Taxa Abundance and Biodiversity Analysis
In order to describe the distribution of “known” and de novo taxa in the different samples, the number of taxa with at least 10 sequences in one replicate was calculated, considering altogether the replicates for each sample (if the same taxon was present more than one time in the different replicates of the sample, it was counted as one). The freely available Venny version 2.1 software2 was used to construct the Venn diagrams of AMF taxa in Bs and Rs soils.
For abundance analysis, only taxa present with at least 10 sequences in one replicate were used (“known” reported in Supplementary Table 1 and de novo in Supplementary Table 2). Then, the de novo taxa were BLASTed against the NCBI database to give them a name (Supplementary Table 2) as fully described in Massa et al. (2020).
Using these data, the four AMF communities were compared also by analysis with MicrobiomeAnalyst, a freely available online software3, according to Berlanas et al. (2019) and to Sergaki et al. (2018), which allow community description by alpha diversity, heat trees, beta diversity, and linear discriminant analysis effect size (LDA-LEfSe).
In particular, alpha diversity analysis was performed using the phyloseq package (McMurdie and Holmes, 2013). The results were represented as box plots for each sample. The statistical significance was also estimated using either parametric or non-parametric tests.
Heat tree method was used to compare abundance at the species taxonomic level for space and time factors. Heat tree uses a hierarchical structure of taxonomic classifications to quantitatively (median abundance) and statistically (non-parametric Wilcoxon rank-sum test) describe taxon differences among communities. The resulting differential heat tree shows the relative abundance of each taxon in two different samples. Heat tree analysis was performed using R metacoder package (Foster et al., 2017).
Beta diversity was analyzed using the phyloseq package (McMurdie and Holmes, 2013). Principal coordinate analysis (PCoA) was applied using Bray–Curtis distance-based method. Permutational ANOVA (PERMANOVA) was employed for the evaluation of the statistical significance of the clustering pattern in ordination plots.
Moreover, LDA-LEfSe analysis using the non-parametric factorial Kruskal–Wallis sum-rank test was applied. Features were considered significant for adjusted p-value cutoff at 0.05 and LDA score at 1.0.
Finally, for each sample, considering the sequence abundance in the single replicates, the median value of the number of sequences was calculated, and only the taxa with a median higher than 0 (yellow lines in Supplementary Tables 1, 2) were considered to be assigned to the different taxonomic groups.
Data Availability
The genomic datasets are available in NCBI using BioProject ID PRJNA613620 containing the following BioSamples: SAMN14411203, SAMN14411449, SAMN14411451, and SAMN14411452 (project name: Vitis vinifera association with local AMF communities in an Italian vineyard at two different phenological stages).
Results
AMF Root Colonization
Arbuscular mycorrhizal fungi root colonization was checked in grapevine plants in both sampling times. The frequencies of colonization were 92.0 ± 2.5% at the first sampling and 94.2 ± 3.3% at the second sampling (p-value = 0.3658). The degrees of mycorrhizal colonization were 35.4 ± 5.5 and 44.5 ± 8.0% (p-value = 0.3379), the arbuscule abundances were 13.9 ± 3.6 and 16.8 ± 5.3% (p = 0.6547), and the vesicle abundances were 11.3 ± 3.0 and 17.6 ± 7.7% (p = 0.9491) in the first and the second sampling, respectively. No significant differences between the two sampling times were detected in all the considered parameters.
Taxa Abundance and Analysis of Biodiversity
Table 1 shows the real number of sequences for each replicate of Bs and Rs soils. On average, the number of obtained sequences was about 9,000. As the rarefaction curves reached a plateau (Supplementary Figure 1), the number of obtained sequences was adequate to properly describe the biodiversity of the AMF community in the samples. A total of 467 taxa (305 univocal taxa) were obtained from the two soils at the two sampling times, including 177 (87 univocal taxa) “known AMF” and 290 (218 univocal taxa) de novo taxa (Supplementary Tables 1, 2). Figure 2A shows the distribution of taxa in the different samples. In Bs soil, 108 and 118 taxa were obtained in the first and the second sampling time, respectively. In particular, 42 “known AMF” taxa occurred in Bs1S sample and 49 in Bs2S (factor time), while the number of de novo AMF taxa at the two sampling times was 66 and 69, respectively. In Rs soil, a total of 104 and 137 taxa was found in the first and the second sampling time, respectively. In particular, in this soil, 48 “known AMF” taxa were observed at the first sampling time while 38 taxa in the second one; on the contrary, the number of de novo AMF taxa was 56 and 99, respectively (factor time).
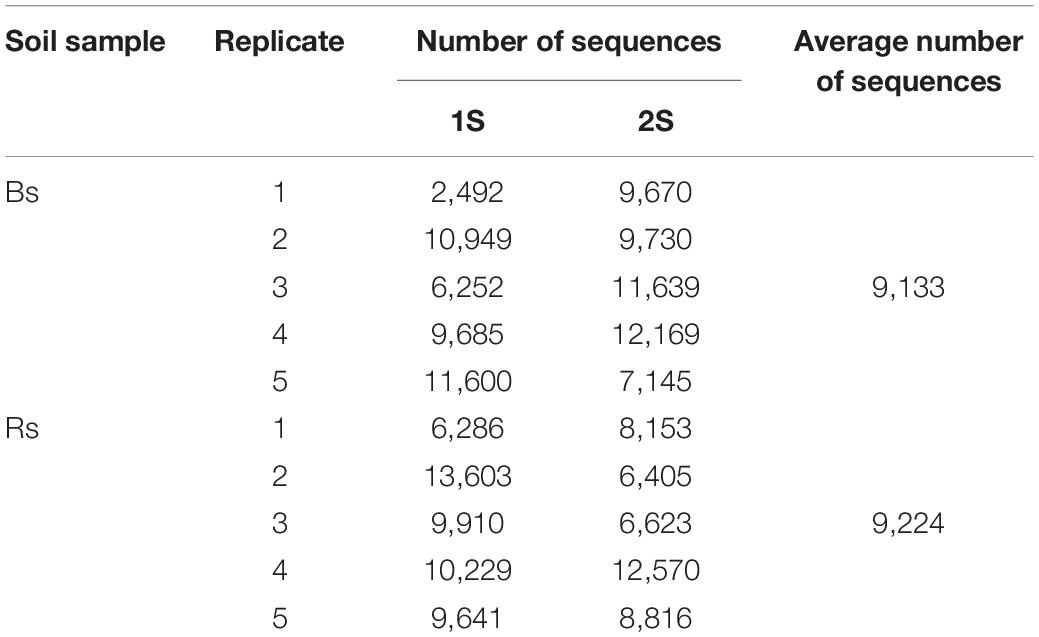
Table 1. Number of sequences obtained from the different replicates of the soils associated (Rs) or not (Bs) with the roots of Vitis vinifera cv. Pinot Noir at the two sampling times (1S = flowering; 2S = fruit development).
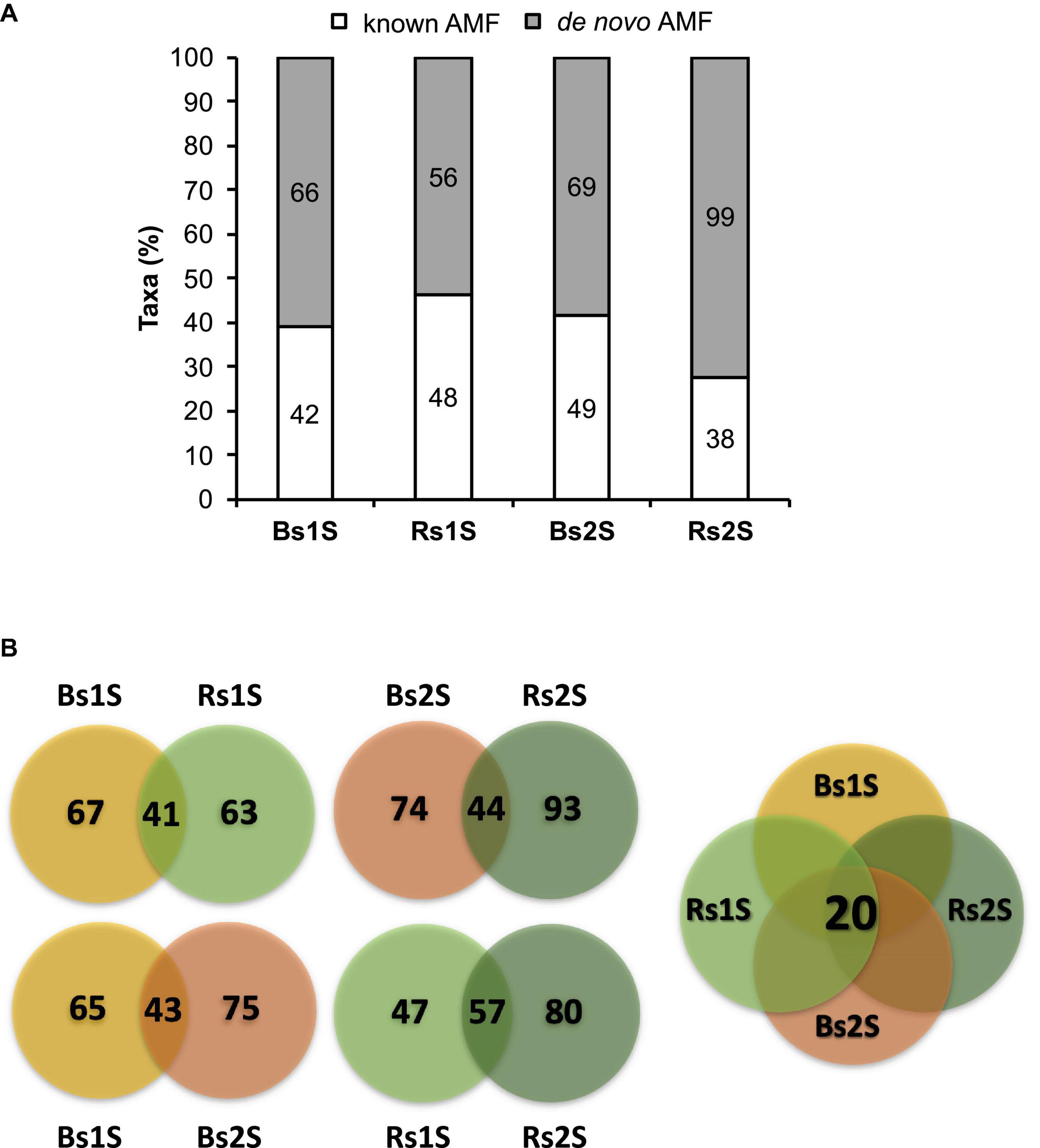
Figure 2. (A) Abundance of the taxa obtained from the soils associated (Rs) or not (Bs) with the roots of Vitis vinifera cv. Pinot Noir during the two sampling times (1S = flowering and 2S = fruit development). Bars represent the percentage of known (white) or de novo (gray) arbuscular mycorrhizal fungi taxa, while labels inside the bars indicate the actual number of taxa. (B) Venn diagrams showing the number of taxa that were exclusive or common to (a) Bs and Rs soils in the first (1S) or in the second (2S) sampling time (upper part on the left of the figure), (b) the first (1S) and the second (2S) sampling times in Bs or in Rs (lower part on the left of the figure), and (c) the four soil samples (Bs1S, Rs1S, Bs2S, and Rs2S—on the right of the figure). The Venn diagrams were calculated by the freely available Venny version 2.1 software (http://bioinfogp.cnb.csic.es/tools/venny).
Bs1S and Rs1S or Bs2S and Rs2S (factor space) shared 41 and 44 taxa, respectively (Figure 2B and Supplementary Table 3). The AMF communities in Bs soils at the two sampling times showed 43 taxa in common. On the other hand, the number of taxa shared between the two sampling times in Rs soil was 57. Finally, all samples had 20 taxa in common (Figure 2B and Supplementary Table 3).
To compare AMF alpha diversity, the number of observed species, Simpson and Shannon indices were calculated (Figure 3). For all these indices, differences were not significant, even if an increased number of observed species occurred in Rs2S compared to the other samples.
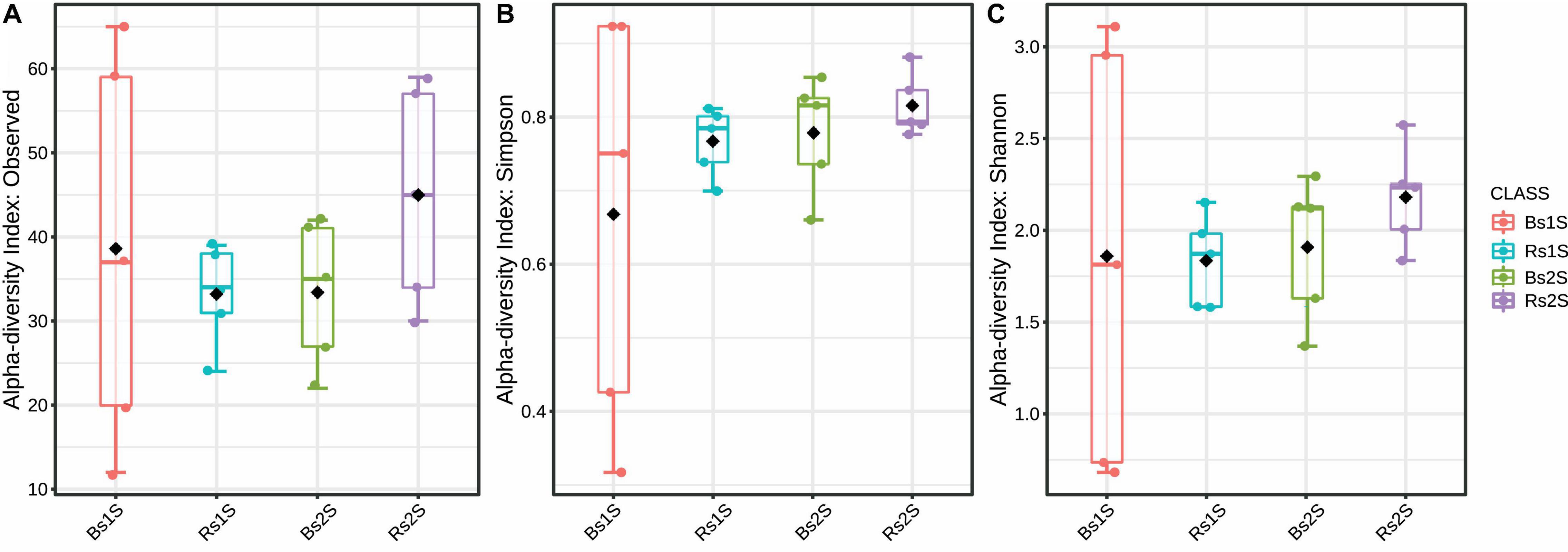
Figure 3. Alpha diversity indices. (A) Number of observed arbuscular mycorrhizal fungi species (p-value, 0.5393), (B) Simpson index (p-value, 0.4700), and (C) Shannon’s index (p-value, 0.8177) of biodiversity detected in the soils associated (Rs) or not (Bs) with the roots of Vitis vinifera cv. Pinot Noir at the two sampling times (1S = flowering and 2S = fruit development). Alpha diversity analysis was performed using the phyloseq package of MicrobiomeAnalyst, a freely available online software (https://www.microbiomeanalyst.ca).
The heat trees reported in Figures 4, 5 represented time and space effect on the AMF community. In particular, Figure 4A is relative to time effect in Bs and displays the increased (blue line) abundance of Rhizophagus irregularis in Bs2S compared to Bs1S (Supplementary Table 4). Figure 4B reports the time effect in the soil associated to V. vinifera roots and shows the increase (blue lines) of de novo_570 (uncultured Glomus), de novo_660 (R. irregularis), and de novo_10711 (uncultured Glomus) and the decrease (red line) of Septoglomus viscosum in the Rs2S compared to Rs1S samples (Supplementary Table 4). In Figure 5A, is represented the heat tree related to the space effect in the first sampling time: while Glomus sp., Rhizophagus irregularis, de novo_1903 (R. irregularis), and de novo_4639 (Glomus sp.) increased (blue lines) in Rs1S compared to Bs1S soil, de novo_358 (uncultured Archaeospora), de novo_581 (uncultured Archaeospora), de novo_627 (uncultured Archaeospora), and de novo_681 (uncultured Archaeospora) decreased (red lines) (Supplementary Table 4). Finally, Figure 5B shows the heat tree related to the space effect in the second sampling time. Although de novo_660 (R. irregularis) and de novo_10711 (uncultured Glomus) increased (blue lines) in Rs2S compared to Bs2S soil, de novo_358 (uncultured Archaeospora), de novo_479 (uncultured Glomerales), and de novo_581 (uncultured Archaeospora) decreased (red lines) (Supplementary Table 4).
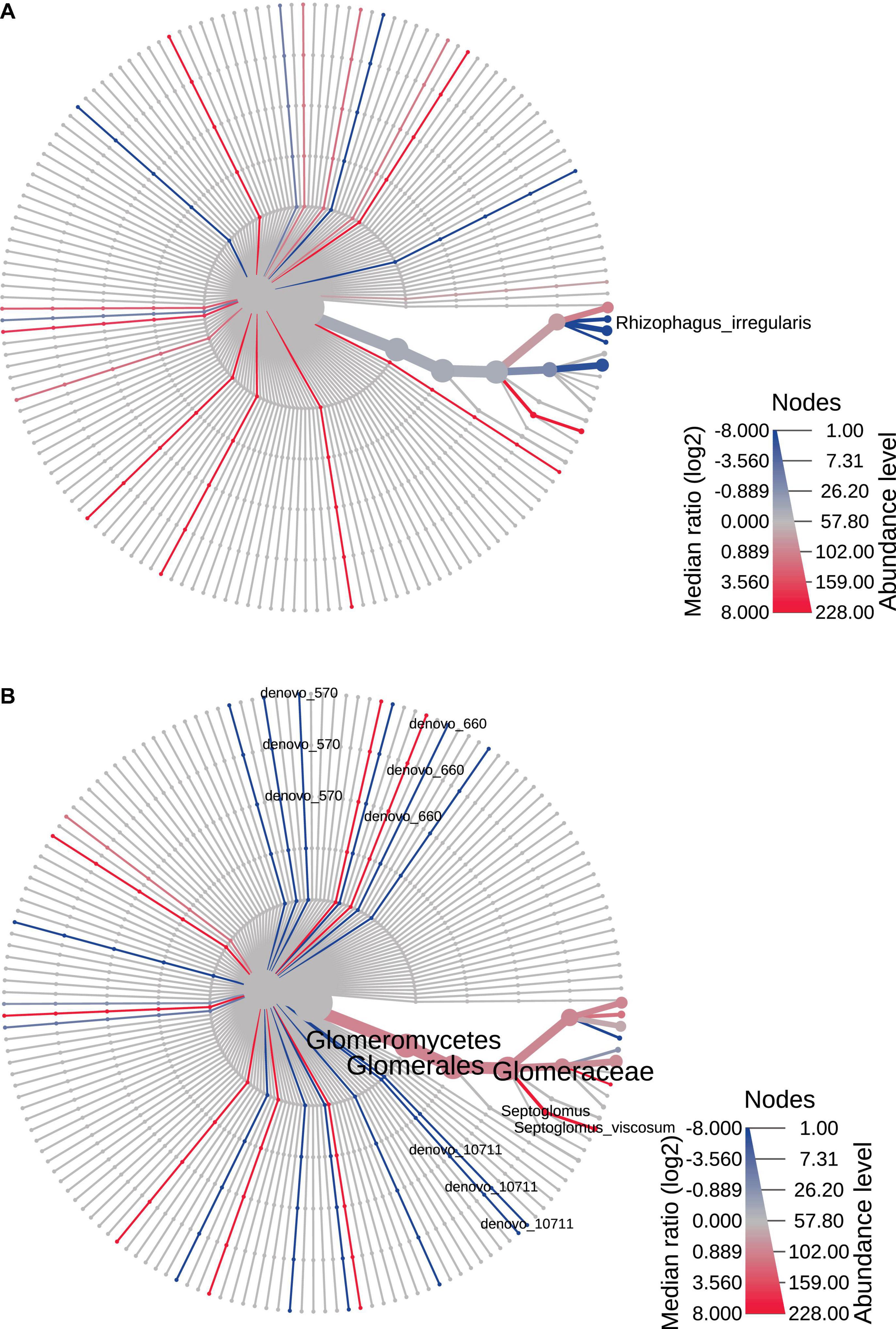
Figure 4. Heat tree based on the factor “time”, reporting the effect of sampling time on the hierarchical structure of taxonomic classifications (median abundance, non-parametric Wilcoxon rank–sum test). (A) Bs1S vs. Bs2S. (B) Rs1S vs. Rs2S. Heat tree analysis was performed using the R metacoder package of MicrobiomeAnalyst, a free available on-line software (https://www.microbiomeanalyst.ca).
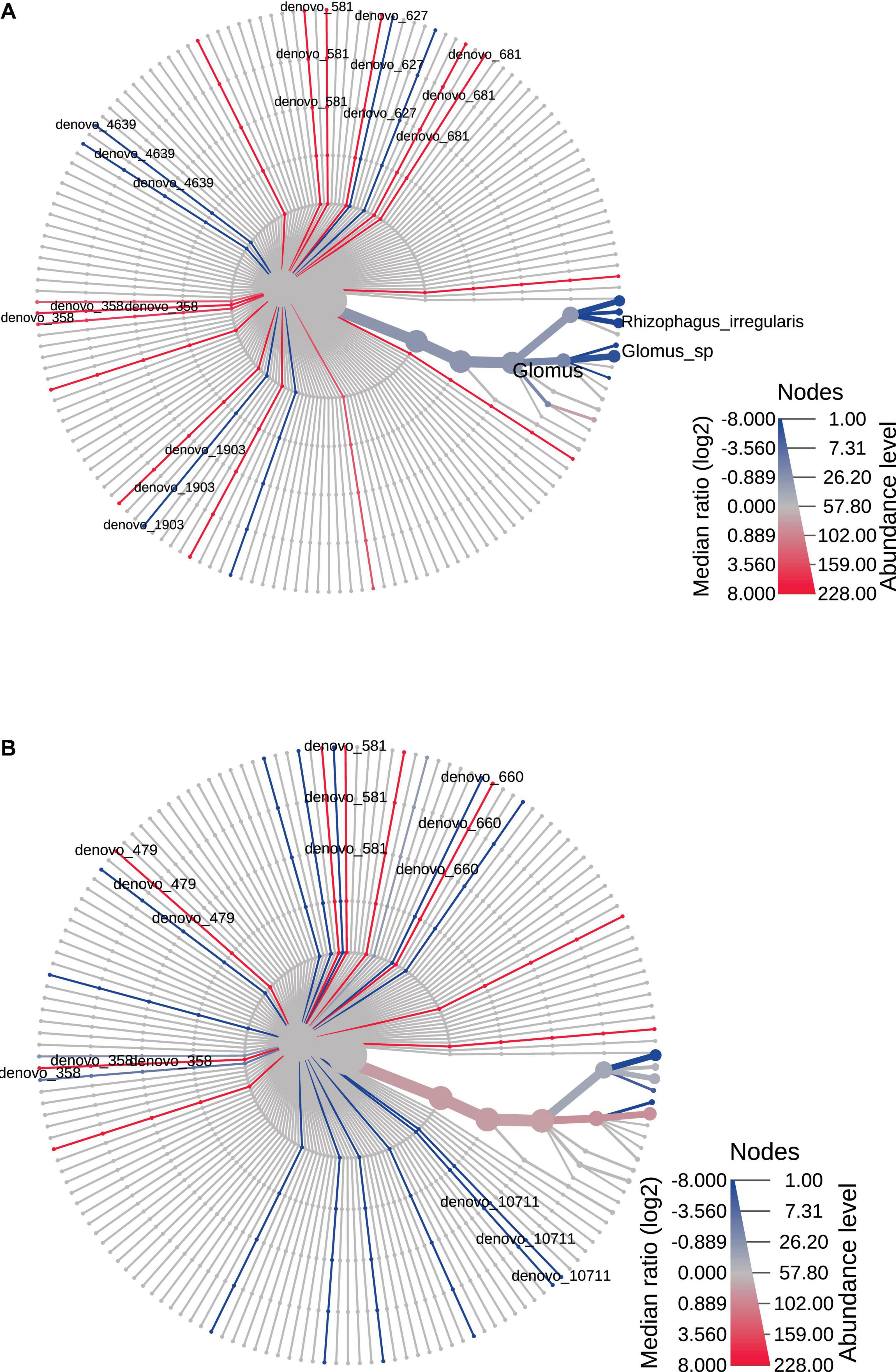
Figure 5. Heat tree based on the factor “space” reporting the effect of the presence of Vitis vinifera roots on the hierarchical structure of taxonomic classifications (median abundance, non-parametric Wilcoxon rank–sum test). (A) Bs1S vs. Rs1S. (B) Bs2S vs. Rs2S. Heat tree analysis was performed using the R metacoder package of MicrobiomeAnalyst, a freely available online software (https://www.microbiomeanalyst.ca).
Data analyzed by PCoA underlined a different community composition between the two soils (PERMANOVA p-value, <0.005), with Rs samples distributed more homogeneously than Bs ones at both sampling times (Figure 6A), but no difference occurred between the two sampling times (PERMANOVA p-value, 0.994) (Figure 6B).
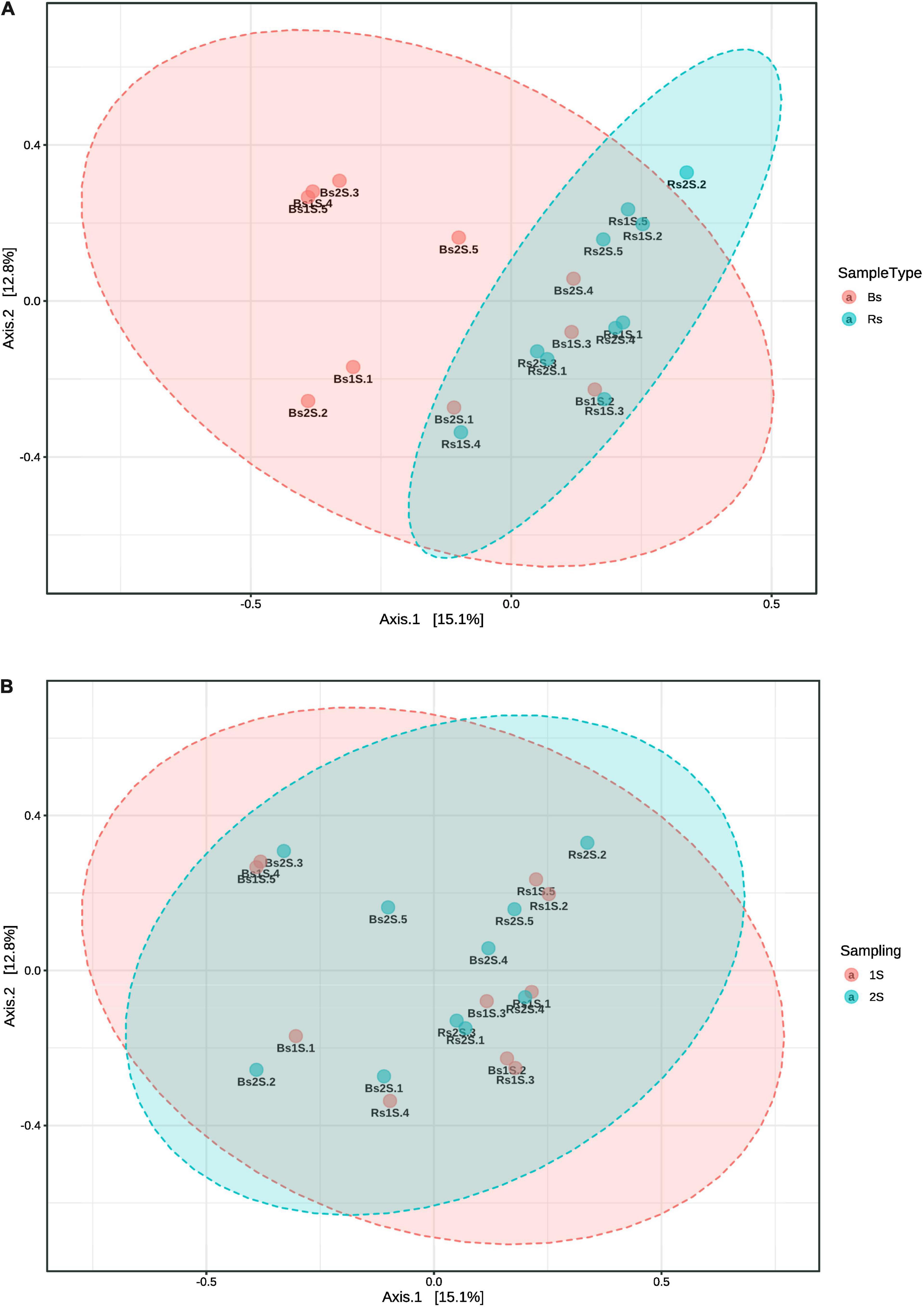
Figure 6. Comparison by principal coordinate analysis of the ecological distance (based on Bray–Curtis distance method) of the different compartments. (A) Soil effect (Bs = soil not associated to grapevine roots, Rs = soil associated with the roots of Vitis vinifera cv. Pinot Noir); PERMANOVA F-value: 1.787, R-square: 0.090312, and p-value < 0.005. (B) Sampling time effect (1S = flowering and 2S = fruit development); PERMANOVA F-value: 0.48119, R-square: 0.026037, and p-value < 0.994. Beta diversity analysis was performed using the phyloseq package of MicrobiomeAnalyst, a freely available online software (https://www.microbiomeanalyst.ca).
The LEfSe results, presented in Figure 7 and Supplementary Table 5, showed the 13 taxa that better explained the differences in the AMF community analyzed. In particular, Glomus sp. showed a LDA score of 6.1 (p-value, 0.033341), the highest values in Rs1S followed by Bs2S. Other important taxa present in the soil associated to V. vinifera roots (Rs) were de novo_10711 that was uncultured Glomus (LDA score, 3.8), de novo_570 that was uncultured Glomus (LDA score, 3.79), de novo_10732 that was Rhizophagus intraradices (LDA score, 3.77), de novo_660 that was R. irregularis (LDA score, 3.58), and de novo_11975 that was uncultured Rhizophagus (LDA score, 3.51). On the contrary, the seven de novo taxa – de novo_358 that was uncultured Archaeospora (LDA score, 5.62), R. irregularis (LDA score, 5.47), de novo_561 that was uncultured Archaeospora (LDA score, 5.16), de novo_581 that was uncultured Archaeospora (LDA score, 4.62), de novo_627 that was uncultured Archaeospora (LDA score, 4.52), de novo_681 that was uncultured Archaeospora (LDA score, 3.63), and de novo_919 that was uncultured Archaeospora (LDA score, 3.58) – mostly explained the differences in Bs soil.
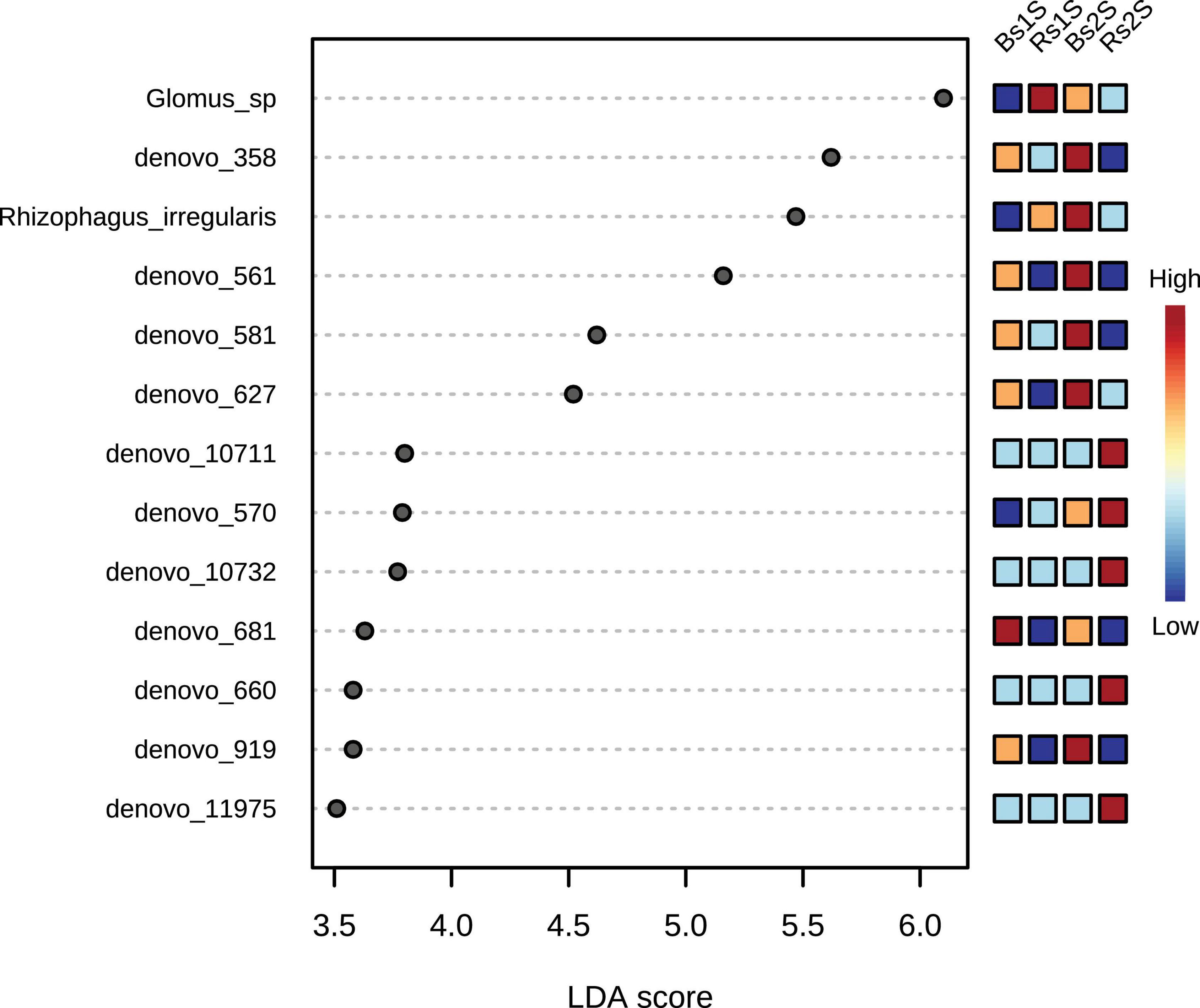
Figure 7. Linear discriminant analysis–effect size (LDA–LEfSe) results using non-parametric factorial Kruskal–Wallis sum–rank test. Adjusted p-value cutoff = 0.05 and LDA score = 1.0; Glomus sp. (LDA score 6.1); de novo_358 – uncultured Archaeospora (LDA score 5.62), Rhizophagus irregularis (LDA score 5.47), de novo_561 – uncultured Archaeospora (LDA score 5.16), de novo_581 – uncultured Archaeospora (LDA score 4.62), de novo_627 – uncultured Archaeospora (LDA score 4.52), de novo_10711 – uncultured Glomus (LDA score 3.8), de novo_570 – uncultured Glomus (LDA score 3.79), de novo_10732 – Rhizophagus intraradices (LDA score 3.77), de novo_681 – uncultured Archaeospora (LDA score 3.63), de novo_660 – Rhizophagus irregularis (LDA score 3.58), de novo_919 – uncultured Archaeospora (LDA score 3.58), and de novo_11975 – uncultured Rhizophagus (LDA score 3.51). LEfSe analysis was performed with MicrobiomeAnalyst, a freely available online software (https://www.microbiomeanalyst.ca).
The de novo AMF taxa were named after being BLASTed against NCBI database (Supplementary Table 2). Then, for each sample, all the “known AMF” (yellow lines in Supplementary Table 1) and the de novo-BLASTed (yellow lines in Supplementary Table 2) taxa belonging to the same taxonomic group were added (Figure 8A). Two taxa corresponded to higher AMF classification levels (subphylum and order; Figure 8A – cyan area on the left). Many taxa were included in the family Glomeraceae (Figure 8A – central green area); the genus Glomus was the most abundant group (22 taxa in Rs2S). All the other remaining taxa of the family Glomeraceae belonged to the genus Rhizophagus, with the exception of S. viscosum. All the taxa belonging to the family Archaeosporaceae were included in only one genus (Archaeospora; Figure 8A – orange area on the right).
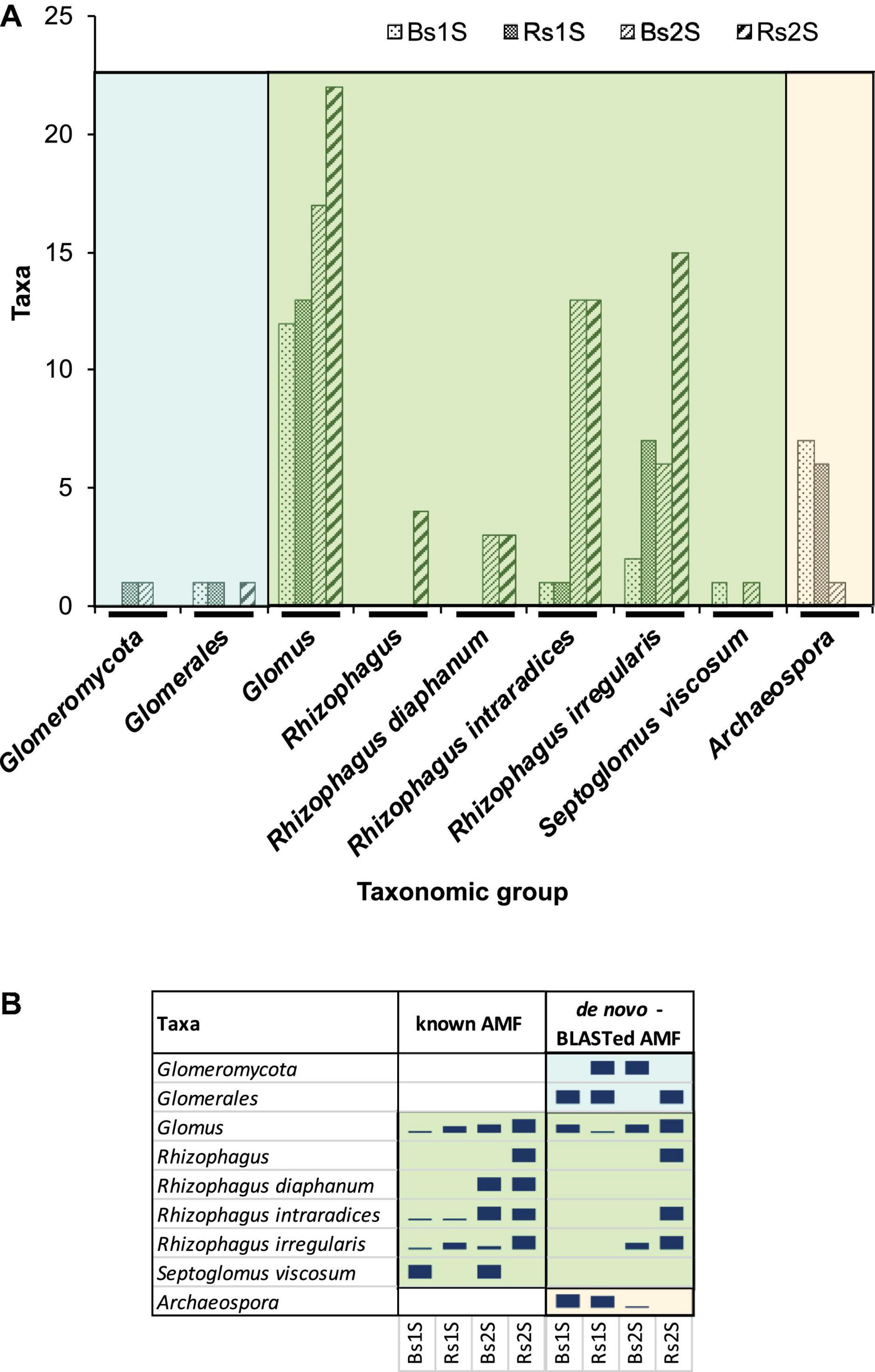
Figure 8. (A) Number of taxa belonging to the different taxonomic groups – obtained by adding the “de novo-BLASTed AMF” taxa with the “known AMF” ones on the basis of the group to which they belonged – in the different samples (Bs = soil not associated to grapevine roots, Rs = soil associated with the roots of Vitis vinifera cv. Pinot Noir) at the two sampling times (1S = flowering and 2S = fruit development). (B) Relative abundance of taxa obtained considering separately “known AMF” and “de novo-BLASTed AMF”, belonging to each taxonomic group, in Bs and Rs soils at the two sampling times (1S = flowering and 2S = fruit development).
Most of the taxonomic groups were detected in both soils, with the exception of unidentified species of the genus Rhizophagus that were observed only in Rs2S and S. viscosum that was detected only in Bs soils at both sampling times (Figure 8A). Moreover, the species Rhizophagus diaphanum was present in both soils, but only at the second sampling time (Figure 8A).
The de novo AMF taxon identification by NCBI permitted to highlight the presence of the genus Archaeospora that did not appear among the “known AMF” taxa (Figure 8B). In fact, the “known AMF” taxa belonged only to the family Glomeraceae (Figure 8B).
All the identified de novo AMF taxa corresponded to uncultured AMF, with the exception of four taxa: one belonging to Glomeromycota, five to the genus Glomus, three to the species R. irregularis, and one to the species R. intraradices (Supplementary Table 2).
Discussion
Arbuscular mycorrhizal fungi are widespread symbionts able to colonize the roots of a lot of terrestrial plant species, including V. vinifera (Trouvelot et al., 2015). The characterization of AMF communities associated to grapevines is of interest by both economic and historical viewpoints. In fact, Piedmont is one the most important Italian regions for vineyard cultivation and wine production (ISTAT, 2018), and since 2014, the UNESCO World Heritage list has included the hills of the Piedmont area covering the Langhe, Roero, and Monferrato4. Therefore, we focused our attention on the AMF community associated to the Pinot Noir grapevine cultivar, which is largely cultivated in Piedmont.
At the beginning, the mycorrhizal colonization of grapevine roots was evaluated to assess the actual interaction between the plant and the AM fungi present in the soil. The plant roots were colonized by AMF at levels that were similar to those reported in the literature (Schreiner, 2005, 2020; Likar et al., 2013; Bouffaud et al., 2016; Massa et al., 2020), and no significant differences between the two sampling times were observed. This is in contrast with the findings of Schreiner (2005) who reported that the mycorrhizal colonization of V. vinifera cv. Pinot Noir roots increased before bud break in the spring, reaching values of about 50–60% of root length in early summer, that remained constant until leaf senescence in late fall.
Fragments resulting from pyrosequencing analysis were 700 bp in length, so they have a larger size if compared to those obtained in previously published works (Lumini et al., 2010; Holland et al., 2014). A total of 467 taxa have been found. This result is consistent with that reported by Holland et al. (2016), in which 816 taxa were obtained using the SSU rDNA marker for studying the AMF biodiversity in different vineyards in Canada. Similarly, Massa et al. (2020) found 528 taxa of AMF in an integrated pest-managed (IPM) vineyard in Piedmont (Italy). Considering the distribution of taxa among the different samples, the highest number of taxa was observed in Rs2S. The two soils showed different taxa: in the second sampling, only 44 taxa were shared by both soils, while 74 were present only in Bs2S and 93 only in Rs2S. Moreover, only 20 taxa were common to the two soils at the two sampling times. Compared to the AMF community characterization performed in the IPM vineyard considered in Massa et al. (2020), in the present work, focused on a conventionally managed vineyard, a higher number of taxa shared between the two soils and a lower number of taxa exclusive of each soil were observed at the two sampling times. On the contrary, considering the sampling time, both Bs and Rs soils shared numbers of taxa similar to those reported in Massa et al. (2020). However, a lower number of exclusive taxa for each sampling time was observed. The differences between the AMF community described in these two studies could be due to the different vineyard management (IPM vs. conventional), but the impact of chemical–physical soil parameters cannot be ruled out.
The biodiversity indices (number of observed species – Shannon and Simpson’s indices) did not show significant differences according to soil type (Bs vs. Rs) and sampling time (1S vs. 2S). Our results confirmed what was previously reported by Schreiner and Mihara (2009), demonstrating that AMF vineyard communities did not change with season succession, but differed according to the vineyard age and type of soil. Consistently, the impact of seasonality on the biodiversity of AMF has been described in crops other than grapevines (Dumbrell et al., 2011; Bouamri et al., 2014; Vargas-Gastélum et al., 2015).
Going into deeper detail and considering time and space as separate factors, some significant differences have been highlighted. In particular, an increase of R. irregularis in Bs and an increase of R. irregularis and uncultured Glomus, combined with a reduction of S. viscosum in Rs, were observed in the second sampling time compared to the first one. Moreover, in both sampling times, an increase of Glomus and R. irregularis and a decrease in uncultured Archaeospora were recorded in Rs compared to Bs.
Finally, the phylogenetic distance obtained by PCoA supported the difference between Bs and Rs soils, confirming what was previously discussed. These data are in accordance with what was reported in other scientific works, which demonstrated the effect of the plant in selecting the associated AM fungal population (Cesaro et al., 2008; Holland et al., 2014).
Consistently with Massa et al. (2020), no fungal sequences belonging to Claroideoglomeraceae, Acaulosporaceae, Gigasporaceae, and Diversisporaceae were detected even if the primers used were specific for all AMF. Many fungal taxa corresponded to Glomeraceae, which is one of the most represented family in agricultural lands (Cesaro et al., 2008; Berruti et al., 2014) and also in vineyards (Balestrini et al., 2010; Lumini et al., 2010; Holland et al., 2014; Trouvelot et al., 2015; Bouffaud et al., 2016; Massa et al., 2020). This abundance could be explained by the high growth rate and the fast recovery of the hyphal network following the disturbance caused by agricultural practices (Berruti et al., 2014; Trouvelot et al., 2015) that are typical of the fungi belonging to this family. Although the genus Glomus was the most abundant, all the other remaining taxa of the family Glomeraceae, with the exception of S. viscosum, belonged to the genus Rhizophagus.
In agreement with Schreiner and Mihara (2009); Balestrini et al. (2010), Oehl and Koch (2018); Massa et al. (2020), and Schreiner (2020), we found AMF members of the family Archaeosporaceae represented only by one genus, Archaeospora.
The AMF community of a conventionally managed vineyard (with a prevalence of Glomeraceae and Archaeosporaceae) described in this work partly overlapped with those characterized in other vineyards subjected to a different management (Schreiner and Mihara, 2009; Balestrini et al., 2010; Holland et al., 2016; Massa et al., 2020). However, a lot of uncontrolled variables such as soil tillage, cover crops, manure application, and quality and amount of herbicides, fertilizers, and pesticides can influence the AMF diversity or community composition (Turrini et al., 2017).
In conclusion, in this work, a difference in AMF communities was observed between the two considered soils (Bs and Rs) independently from the plant phenological stage, suggesting a possible role of V. vinifera in modulating the AMF populations associated to its roots.
Overall, looking at the improvement in the global sustainability of viticulture practices, this study broadens the knowledge already gained by other works (Novello et al., 2017; Bona et al., 2019; Gamalero et al., 2020; Massa et al., 2020) regarding the microbiota associated with Pinot Noir grapevines cultivated in a geographic region historically dedicated to viticulture.
Data Availability Statement
The datasets presented in this study can be found in online repositories. The names of the repository/repositories and accession number(s) can be found below: https://www.ncbi.nlm.nih.gov/, PRJNA613620.
Author Contributions
PC cooperated in biological experiments, performed sample preparation for pyrosequencing, prepared the figures, analyzed data, and wrote the manuscript. NM organized the sampling, cooperated to data elaboration, prepared the figures, and wrote the manuscript. EB wrote the manuscript. GN participated to the sampling and performed DNA extraction. VT, EG, and GB cooperated in manuscript writing. LB performed pyrosequencing and bioinformatic analyses. FM cooperated in bioinformatic analyses. GL coordinated biological experiments, bioinformatic analyses, and manuscript writing. All authors revised the manuscript.
Funding
This research is original and has received financial support from the Università del Piemonte Orientale and Regione Piemonte PSR F.E.A.S.R. 2007/2013.
Conflict of Interest
LB and FM were employed by the company SmartSeq s.r.l.
The remaining authors declare that the research was conducted in the absence of any commercial or financial relationships that could be construed as a potential conflict of interest.
Acknowledgments
The authors would like to thank Agrion Foundation for Research, Innovation and Technological Development of Piedmont Agriculture for support during the field experiment.
Supplementary Material
The Supplementary Material for this article can be found online at: https://www.frontiersin.org/articles/10.3389/fmicb.2021.676610/full#supplementary-material
Supplementary Figure 1 | Rarefaction curves.
Supplementary Table 1 | List of IDs for “known” taxa identified by large subunit rDNA database.
Supplementary Table 2 | Identification of de novo taxa by BLAST analysis against the NCBI database.
Supplementary Table 3 | List of the IDs of “known” taxa (identified by large subunit rDNA database) and de novo taxa that were common between different soils (Bs or Rs) and in different sampling times (1S or 2S).
Supplementary Table 4 | Heat tree difference table reporting the results (p-values) of the non-parametric Wilcoxon rank–sum test used to depict the taxonomic differences between AMF communities.
Supplementary Table 5 | Linear discriminant analysis effect size results using non-parametric factorial Kruskal–Wallis sum–rank test.
Footnotes
- ^ http://www.amf-phylogeny.com/amphylo_species.html
- ^ www://bioinfogp.cnb.csic.es/tools/venny
- ^ https://www.microbiomeanalyst.ca
- ^ http://whc.unesco.org/en/list/1390
References
Altschul, S. F., Madden, T. L., Schaffer, A. A., Zhang, J., Zhang, Z., Miller, W., et al. (1997). Gapped BLAST and PSI-BLAST: a new generation of protein database search programs. Nucleic Acid Res. 25, 3389–3402. doi: 10.1093/nar/25.17.3389
Artero, A., Artero, A., Tarín, J. J., and Cano, A. (2015). The impact of moderate wine consumption on health. Maturitas 80, 3–13. doi: 10.1016/j.maturitas.2014.09.007
Balestrini, R., Magurno, F., Walker, C., Lumini, E., and Bianciotto, V. (2010). Cohorts of arbuscular mycorrhizal fungi (AMF) in Vitis vinifera, a typical Mediterranean fruit crop. Environ. Microbiol. Rep. 2, 594–604. doi: 10.1111/j.1758-2229.2010.00160.x
Baslam, M., Esteban, R., García-Plazaola, J. I., and Goicoechea, N. (2013). Effectiveness of arbuscular mycorrhizal fungi (AMF) for inducing the accumulation of major carotenoids, chlorophylls and tocopherol in green and red leaf lettuces. Appl. Microbiol. Biotechnol. 97, 3119–3128. doi: 10.1007/s00253-012-4526-x
Berlanas, C., Berbegal, M., Elena, G., Laidani, M., Cibriain, J. F., Sagües, A., et al. (2019). The fungal and bacterial rhizosphere microbiome associated with grapevine rootstock genotypes in mature and young vineyards. Front. Microbiol. 10:1142. doi: 10.3389/fmicb.2019.01142
Berruti, A., Borriello, R., Orgiazzi, A., Barbera, A. C., Lumini, E., and Bianciotto, V. (2014). “Arbuscular mycorrhizal fungi and their value for ecosystem management,” in Biodiversity - The Dynamic Balance of the Planet, ed. O. Grillo (UK: IntechOpen), 159–191.
Berta, G., Copetta, A., Gamalero, E., Bona, E., Cesaro, P., Scarafoni, A., et al. (2014). Maize development and grain quality are differentially affected by mycorrhizal fungi and a growth-promoting pseudomonad in the field. Mycorrhiza 24, 161–170. doi: 10.1007/s00572-013-0523-x
Bona, E., Cantamessa, S., Massa, N., Manassero, P., Marsano, F., Copetta, A., et al. (2017). Arbuscular mycorrhizal fungi and plant growth-promoting pseudomonads improve yield, quality and nutritional value of tomato: a field study. Mycorrhiza 27, 1–11. doi: 10.1007/s00572-016-0727-y
Bona, E., Lingua, G., Manassero, P., Cantamessa, S., Marsano, F., Todeschini, V., et al. (2015). AM fungi and PGP pseudomonads increase flowering, fruit production, and vitamin content in strawberry grown at low nitrogen and phosphorus levels. Mycorrhiza 25, 181–193. doi: 10.1007/s00572-014-0599-y
Bona, E., Marsano, F., Massa, N., Cattaneo, C., Cesaro, P., Argese, E., et al. (2011). Proteomic analysis as a tool for investigating arsenic stress in Pteris vittata roots colonized or not by arbuscular mycorrhizal symbiosis. J. Proteomics 74, 1338–1350. doi: 10.1016/j.jprot.2011.03.027
Bona, E., Massa, N., Novello, G., Boatti, L., Cesaro, P., Todeschini, V., et al. (2019). Metaproteomic characterization of the Vitis vinifera rhizosphere. FEMS Microbiol. Ecol. 95, 1–16. doi: 10.1093/femsec/fiy204
Bona, E., Todeschini, V., Cantamessa, S., Cesaro, P., Copetta, A., Lingua, G., et al. (2018). Combined bacterial and mycorrhizal inocula improve tomato quality at reduced fertilization. Sci. Hortic. 234, 160–165. doi: 10.1016/j.scienta.2018.02.026
Bouamri, R., Dalpé, Y., and Serrhini, M. N. (2014). Effect of seasonal variation on arbuscular mycorrhizal fungi associated with date palm. Emir. J. Food Agric. 26, 977–986. doi: 10.9755/ejfa.v26i11.18985
Bouffaud, M. L., Bernaud, E., Colombet, A., van Tuinen, D., Wipf, D., and Redecker, D. (2016). Regional-scale analysis of arbuscular mycorrhizal fungi: the case of Burgundy vineyards. J. Int. des Sci. la Vigne du Vin 50, 1–8. doi: 10.20870/oeno-one.2016.50.1.49
Cesaro, P., van Tuinen, D., Copetta, A., Chatagnier, O., Berta, G., Gianinazzi, S., et al. (2008). Preferential colonization of Solanum tuberosum L. roots by the fungus Glomus intraradices in arable soil of a potato farming area. Appl. Environ. Microbiol. 74, 5776–5783. doi: 10.1128/AEM.00719-08
Degola, F., Fattorini, L., Bona, E., Sprimuto, C. T., Argese, E., Berta, G., et al. (2015). The symbiosis between Nicotiana tabacum and the endomycorrhizal fungus Funneliformis mosseae increases the plant glutathione level and decreases leaf cadmium and root arsenic contents. Plant Physiol. Biochem. 92, 11–18. doi: 10.1016/j.plaphy.2015.04.001
Dumbrell, A. J., Ashton, P. D., Aziz, N., Feng, G., Nelson, M., Dytham, C., et al. (2011). Distinct seasonal assemblages of arbuscular mycorrhizal fungi revealed by massively parallel pyrosequencing. New Phytol. 190, 794–804. doi: 10.1111/j.1469-8137.2010.03636.x
Ewing, B., Hillier, L., Wendl, M., and Green, P. (1998). Base-calling of automated sequencer traces using Phred. I. Accuracy assessment. Genome Res. 8, 175–185. doi: 10.1101/gr.8.3.175
Farmer, M. J., Li, X., Feng, G., Zhao, B., Chatagnier, O., Gianinazzi, S., et al. (2007). Molecular monitoring of field-inoculated AMF to evaluate persistence in sweet potato crops in China. Appl. Soil Ecol. 35, 599–609. doi: 10.1016/j.apsoil.2006.09.012
Foster, Z. S. L., Sharpton, T. J., and Gru, N. J. (2017). Metacoder: an R package for visualization and manipulation of community taxonomic diversity data. PLoS Comput. Biol. 13:e1005404. doi: 10.1371/journal.pcbi.1005404
Gamalero, E., Bona, E., Novello, G., Boatti, L., Mignone, F., Massa, N., et al. (2020). Discovering the bacteriome of Vitis vinifera cv. Pinot Noir in a conventionally managed vineyard. Sci. Rep. 10:6453. doi: 10.1038/s41598-020-63154-w
Georgiev, V., Ananga, A., and Tsolova, V. (2014). Recent advances and uses of grape flavonoids as nutraceuticals. Nutrients 6, 391–415. doi: 10.3390/nu6010391
Hart, M. M., Aleklett, K., Chagnon, P. L., Egan, C., Ghignone, S., Helgason, T., et al. (2015). Navigating the labyrinth: a guide to sequence-based, community ecology of arbuscular mycorrhizal fungi. New Phytol. 207, 235–247. doi: 10.1111/nph.13340
Hodge, A., Helgason, T., and Fitter, A. H. (2010). Nutritional ecology of arbuscular mycorrhizal fungi. Fungal Ecol. 3, 267–273. doi: 10.1016/j.funeco.2010.02.002
Holland, T. C., Bowen, P., Bogdanoff, C., and Hart, M. M. (2014). How distinct are arbuscular mycorrhizal fungal communities associating with grapevines? Biol. Fertil. Soils 50, 667–674. doi: 10.1007/s00374-013-0887-2
Holland, T. C., Bowen, P. A., Bogdanoff, C. P., Lowery, T. D., Shaposhnikova, O., Smith, S., et al. (2016). Evaluating the diversity of soil microbial communities in vineyards relative to adjacent native ecosystems. Appl. Soil Ecol. 100, 91–103. doi: 10.1016/j.apsoil.2015.12.001
IOV. (2017). Distribution of the World’s Grapevine Varieties. ISBN: 979-10-91799-89-8. Paris: International organization of vine and wine.
IOV. (2019). 2019 Statistical Report on World Vitiviniculture. Paris: International organization of vine and wine.
Krüger, M., Krüger, C., Walker, C., Stockinger, H., and Schüßler, A. (2011). Phylogenetic reference data for systematics and phylotaxonomy of arbuscular mycorrhizal fungi from phylum to species level. New Phytol. 193, 970–984. doi: 10.1111/j.1469-8137.2011.03962.x
Likar, M., Hančević, K., Radić, T., and Regvar, M. (2013). Distribution and diversity of arbuscular mycorrhizal fungi in grapevines from production vineyards along the eastern Adriatic coast. Mycorrhiza 23, 209–219. doi: 10.1007/s00572-012-0463-x
Likar, M., Stres, B., Rusjan, D., Potiseka, M., and Regvara, M. (2017). Ecological and conventional viticulture gives rise to distinct fungal and bacterial microbial communities in vineyard soils. Appl. Soil Ecol. 113, 86–95.
Lindahl, B. D., Nilsson, R. H., Tedersoo, L., Abarenkov, K., Carlsen, T., Pennanen, T., et al. (2013). Methods Fungal community analysis by high-throughput sequencing of amplified markers - a user’s guide. New Phytol. 199, 288–299. doi: 10.1111/nph.12243
Linderman, R. G., and Davis, E. A. (2001). Comparative response of selected grapevine rootstocks and cultivars to inoculation with different mycorrhizal fungi. Am. J. Enol. Vitic. 52, 8–11.
Lingua, G., Bona, E., Todeschini, V., Cattaneo, C., Marsano, F., Berta, G., et al. (2012). Effects of heavy metals and arbuscular mycorrhiza on the leaf proteome of a selected poplar clone: a time course analysis. PLoS One 7:e38662. doi: 10.1371/journal.pone.0038662
Lumini, E., Orgiazzi, A., Borriello, R., Bonfante, P., and Bianciotto, V. (2010). Disclosing arbuscular mycorrhizal fungal biodiversity in soil through a land-use gradient using a pyrosequencing approach. Environ. Microbiol. 12, 2165–2179. doi: 10.1111/j.1462-2920.2009.02099.x
Massa, N., Bona, E., Novello, G., Todeschini, V., Boatti, L., Mignone, F., et al. (2020). AMF communities associated to Vitis vinifera in an Italian vineyard subjected to integrated pest management at two different phenological stages. Sci. Rep. 10:9197. doi: 10.1038/s41598-020-66067-w
McMurdie, P. J., and Holmes, S. (2013). Phyloseq: an R package for reproducible interactive analysis and graphics of microbiome census data. PLoS One 8:e61217. doi: 10.1371/journal.pone.0061217
Nogales, A., Santos, E. S., Abreu, M. M., Arán, D., Victorino, G., Pereira, E. S., et al. (2019). Mycorrhizal inoculation differentially affects grapevine’s performance in copper contaminated and non-contaminated soils. Front. Plant Sci. 9:1906. doi: 10.3389/fpls.2018.01906
Novello, G., Gamalero, E., Bona, E., Boatti, L., Mignone, F., Massa, N., et al. (2017). The rhizosphere bacterial microbiota of Vitis vinifera cv. Pinot Noir in an integrated pest management vineyard. Front. Microbiol. 8:1528. doi: 10.3389/fmicb.2017.01528
Oehl, F., and Koch, B. (2018). Diversity of arbuscular mycorrhizal fungi in no-till and conventionally tilled vineyards. J. Appl. Bot. Food Qual. 91, 56–60. doi: 10.5073/JABFQ.2018.091.008
Öpik, M., and Davison, J. (2016). Uniting species- and community-oriented approaches to understand arbuscular mycorrhizal fungal diversity. Fungal Ecol. 24, 106–113. doi: 10.1016/j.funeco.2016.07.005
R Core Team. (2018). R: A Language and Environment for Statistical Computing. Vienna: R Foundation for Statistical Computing.
Schreiner, R. P. (2005). Spatial and temporal variation of roots, arbuscular mycorrhizal fungi, and plant and soil nutrients in a mature Pinot Noir (Vitis vinifera L.) vineyard in Oregon, USA. Plant Soil 276, 219–234. doi: 10.1007/s11104-005-4895-0
Schreiner, R. P. (2020). Depth structures the community of arbuscular mycorrhizal fungi amplified from grapevine (Vitis vinifera L.) roots. Mycorrhiza 30, 149–160. doi: 10.1007/s00572-020-00930-6
Schreiner, R. P., and Mihara, K. L. (2009). The diversity of arbuscular mycorrhizal fungi amplified from grapevine roots (Vitis vinifera L.) in Oregon vineyards is seasonally stable and influenced by soil and vine age. Mycologia 101, 599–611. doi: 10.3852/08-169
Schreiner, R. P., Scagel, C. F., and Baham, J. (2006). Nutrient uptake and distribution in a mature “pinot noir” vineyard. HortScience 41, 336–345. doi: 10.21273/hortsci.41.2.336
Sergaki, C., Lagunas, B., Lidbury, I., Gifford, M. L., and Schäfer, P. (2018). Challenges and approaches in microbiome research: from fundamental to applied. Front. Plant Sci. 9:1205. doi: 10.3389/fpls.2018.01205
Todeschini, V., Aitlahmi, N., Mazzucco, E., Marsano, F., Gosetti, F., Robotti, E., et al. (2018). Impact of beneficial microorganisms on strawberry growth, fruit production, nutritional quality, and volatilome. Front. Plant Sci. 9:1611. doi: 10.3389/fpls.2018.01611
Torres, N., Antolín, M. C., and Goicoechea, N. (2018). Arbuscular mycorrhizal symbiosis as a promising resource for improving berry quality in grapevines under changing environments. Front. Plant Sci. 9:897. doi: 10.3389/fpls.2018.00897
Trouvelot, A., Kough, J. L., and Gianinazzi-Pearson, V. (1986). “Mesure du taux de mycorrhization VA d’un système radiculaire. Recherche de méthodes d’estimation ayant une signification functionelle,” in Mycorrhizae: Physiology and Genetics, eds V. Gianinazzi-Pearson and S. Gianinazzi (Paris: INRA), 217–221.
Trouvelot, S., Bonneau, L., Redecker, D., van Tuinen, D., Adrian, M., and Wipf, D. (2015). Arbuscular mycorrhiza symbiosis in viticulture: a review. Agron. Sustain. Dev. 35, 1449–1467. doi: 10.1007/s13593-015-0329-7
Turrini, A., Agnolucci, M., Palla, M., Tomé, E., Tagliavini, M., Scandellari, F., et al. (2017). Species diversity and community composition of native arbuscular mycorrhizal fungi in apple roots are affected by site and orchard management. Appl. Soil Ecol. 116, 42–54. doi: 10.1016/j.apsoil.2017.03.016
Vargas-Gastélum, L., Romero-Olivares, A. L., Escalante, A. E., Rocha-Olivares, A., Brizuela, C., and Riquelme, M. (2015). Impact of seasonal changes on fungal diversity of a semi-arid ecosystem revealed by 454 pyrosequencing. FEMS Microbiol. Ecol. 91, 1–13. doi: 10.1093/femsec/fiv044
Vasar, M., Andreson, R., Davison, J., Jairus, T., Moora, M., Remm, M., et al. (2017). Increased sequencing depth does not increase captured diversity of arbuscular mycorrhizal fungi. Mycorrhiza 27, 761–773. doi: 10.1007/s00572-017-0791-y
Vislocky, L. M., and Fernandez, M. L. (2010). Biomedical effects of grape products. Nutr. Rev. 68, 656–670. doi: 10.1111/j.1753-4887.2010.00335.x
Zaller, J. G., Cantelmo, C., Dos Santos, G., Muther, S., Gruber, E., Pallua, P., et al. (2018). Herbicides in vineyards reduce grapevine root mycorrhization and alter soil microorganisms and the nutrient composition in grapevine roots, leaves, xylem sap and grape juice. Environ. Sci. Pollut. Res. 25, 23215–23226. doi: 10.1007/s11356-018-2422-3
Keywords: Vitis vinifera, arbuscular mycorrhizal fungi, biodiversity, conventional management, soil, grapevine roots
Citation: Cesaro P, Massa N, Bona E, Novello G, Todeschini V, Boatti L, Mignone F, Gamalero E, Berta G and Lingua G (2021) Native AMF Communities in an Italian Vineyard at Two Different Phenological Stages of Vitis vinifera. Front. Microbiol. 12:676610. doi: 10.3389/fmicb.2021.676610
Received: 05 March 2021; Accepted: 08 June 2021;
Published: 19 July 2021.
Edited by:
Sabine Dagmar Zimmermann, Délégation Languedoc Roussillon (CNRS), FranceReviewed by:
Cristiana Sbrana, Institute of Agricultural Biology and Biotechnology, Italian National Research Council, ItalyLuciano Avio, University of Pisa, Italy
Copyright © 2021 Cesaro, Massa, Bona, Novello, Todeschini, Boatti, Mignone, Gamalero, Berta and Lingua. This is an open-access article distributed under the terms of the Creative Commons Attribution License (CC BY). The use, distribution or reproduction in other forums is permitted, provided the original author(s) and the copyright owner(s) are credited and that the original publication in this journal is cited, in accordance with accepted academic practice. No use, distribution or reproduction is permitted which does not comply with these terms.
*Correspondence: Nadia Massa, nadia.massa@uniupo.it