- 1Department of Biological Sciences, University of the Sciences, Philadelphia, PA, United States
- 2The Smith Center for Infectious Diseases and Urban Health, West Orange, NJ, United States
- 3Department of Pharmacology and Physiology, Drexel University College of Medicine, Philadelphia, PA, United States
- 4Center for Neuroimmunology and CNS Therapeutics, Institute of Molecular Medicine and Infectious Diseases, Drexel University College of Medicine, Philadelphia, PA, United States
A unique population of HIV-1 infected individuals can control infection without antiretroviral therapy. These individuals fall into a myriad of categories based on the degree of control (low or undetectable viral load), the durability of control over time and the underlying mechanism (i.e., possession of protective HLA alleles or the absence of critical cell surface receptors). In this study, we examine a cohort of HIV-1 infected individuals with a documented history of sustained low viral loads in the absence of therapy. Through in vitro analyses of cells from these individuals, we have determined that infected individuals with naturally low viral loads are capable of controlling spreading infection in vitro in a CD8+ T-cell dependent manner. This control is lost when viral load is suppressed by antiretroviral therapy and correlates with a clinical CD4:CD8 ratio of <1. Our results support the conclusion that HIV-1 controllers with low, but detectable viral loads may be controlling the virus due to an effective CD8+ T-cell response. Understanding the mechanisms of control in these subjects may provide valuable understanding that could be applied to induce a functional cure in standard progressors.
Introduction
Human immunodeficiency virus type-1 (HIV-1) infection causes disease by causing immunosuppression (Shearer, 1998; Elfaki, 2014). The degree of immunosuppression is closely and inversely linked to the concentration of CD4+ T-cells circulating in blood (Ingole et al., 2011; Merci et al., 2017). The average CD4+ T cell count is 1,000 cells/μL. HIV-1 infection causes a drop of 60 cells/uL per year on average (Schwartländer et al., 1993; Patrikar et al., 2014; Parsa et al., 2020). When the CD4+ T-cell count falls below 200 cells/uL, a person is at increased risk of opportunistic infections and malignancies (Egger et al., 2002; Institute of Medicine (US) Committee on Social Security HIV Disability Criteria, 2010; Opportunistic Infections Project Team of the Collaboration of Observational Hiv Epidemiological Research in Europe (Cohere) in EuroCoord, Young et al., 2012; Merci et al., 2017). Approximately 3–6 months after infection, plasma HIV-1 RNA concentration referred to as “viral load” (VL) reaches a steady state with a median of 40,000 copies/ml (c/ml). The level of viremia correlates to the rate of CD4+ T-cell loss (O’Brien W. A. et al., 1996; Mellors et al., 1997; de Wolf et al., 1997). CD4+ T-cells decrease ∼4% per year per log c/ml of HIV-1 RNA. The vast majority of people living with HIV (PLWH) have a VL > 1,000 c/ml of plasma. A very small percentage, < 1%, have a VL below the limit of detection, so-called undetectable.
Initially interested in studying the host factors of PLWH with naturally low viral loads (LVLs), we studied several individuals with VLs < 2,000 c/mL. As in published studies, isolated CD4+ T-cells from all LVLs supported HIV-1 in vitro growth. We next tested the ability of PHA-stimulated Peripheral Blood Mononuclear Cells (PBMCs) from LVLs to grow HIV-1. Surprisingly, the results segregated LVLs into two distinct groups, those with consistently undetectable VLs referred to as elite controllers (ECs) and those with VLs between 100–2,000 c/mL, which we refer to as LVLs. PHA-stimulated PBMCs from EC donors supported HIV-1 growth at a level similar to normal donor PBMCs. On the other hand, no growth could be detected in PBMCs from LVLs and we were able to associate this control with CD8+ T-cells.
CD8+ T-cells are known to play a critical role in the control of viral infection and their temporal appearance has been associated with a reduction in plasma viremia following acute HIV infection (Koup et al., 1994). To underscore the importance of CD8+ T-cells in the control of viral replication, previous studies have demonstrated that a robust rebound of plasma viremia results from antibody-mediated depletion of CD8+ T-cells from antiretroviral therapy (ART) treated simian immunodeficiency virus infected rhesus macaques (Cartwright et al., 2016; McBrien et al., 2020a,b). In addition, simian human immunodeficiency virus infected rhesus macaques who gained viral control when treated with combinatorial broadly neutralizing antibodies (bNAbs) became susceptible to infection when depleted of CD8+ T-cells, thus providing further evidence which supports the indispensable role of CD8+ T-cells in the control of viral replication (Nishimura et al., 2017). It was also observed that there was a minimal viral load which persisted in rhesus macaques who gained viral control when treated with bNAbs but their viral loads were so low they required detection via ultrasensitive assays (Nishimura et al., 2017). It was postulated that although negligible, low viral loads in these animals permitted a sustained CD8+ T-cell response sufficient to control infection which is not achieved when viral load levels are further suppressed by treatment with ART such that when ART is interrupted there is an inability of CD8+ T-cells to control viral replication (Nishimura et al., 2017). Therefore, in the presence of a low viral load, we speculate that CD8+ T-cells retain the ability to control viral replication.
In this study, we report on the clinical and cellular factors associated with LVL inhibition of HIV in vitro (IHI). IHI is only seen in donors who have a detectable, but low viral load and a “flipped ratio” or CD4:CD8 < 1. IHI is lost when viral load is suppressed by ART and is mediated by CD8+ T-cells in an HLA-restricted fashion. Our results support the conclusion that HIV-1 infected individuals with a low viral load set point controls the virus through a CD8+ T-cell response. This response fades quickly after viremia is suppressed, establishing that this mechanism is different than the mechanism(s) involved in the control of viremia in ECs. Understanding how these subjects are different from standard progressors with detectable CTL response may lead to the development of immunomodulatory therapies that can induce a functional cure.
Results
LVL Donor PBMCs Are Resistant to HIV Infection
For this study, we recruited ART naïve HIV-1 seropositive donors with viral loads below 2,000 copies/mL which we define as low viral load (LVL) donors, donors with a history of high viral loads (HVLs), elite controllers (ECs), or normal donors (Table 1). We performed a tissue culture infectious dose 50 (TCID50) assay to determine the relative susceptibility of PBMCs from each donor to infection using a common viral stock (Figure 1A). Normal donors and ECs showed the most susceptibility to infection in vitro. HVLs were less susceptible to infection, with no difference between HVLs suppressed on therapy and those that are therapy naïve. LVLs had the lowest average susceptibility to in vitro infection with HIV-1. However, LVLs were not statistically significant from HVLs off therapy. Blood CD4+ T-cell counts (Figure 1B) of LVLs remain high confirming that these donors control viral loads and maintain healthy CD4+ T-cell counts, unlike HVLs. We further analyzed the ability of PBMCs from normal donors, HVL donors on therapy and LVL donors to control spreading infection. PBMCs were activated with PHA and IL-2 for 48 h, washed, infected with NL4-3, and virus accumulation in the supernatant was measured every 2 days by p24 ELISA. Interestingly, PBMCs from LVLs suppressed de novo HIV-1 infection (Figure 2A). When the same assay was performed on uninfected normal donor and HVL donors PBMCs, we observed robust viral production by day four and six post-infection. As prior studies suggested, CD4+ T-cells from controllers (both LTNPs and ECs) could support viral replication (Cao et al., 1995; Sáez-Cirión et al., 2007; Julg et al., 2010; O’Connell et al., 2011), these data suggest that other cell types present in PBMCs may be mediating suppression in our system.
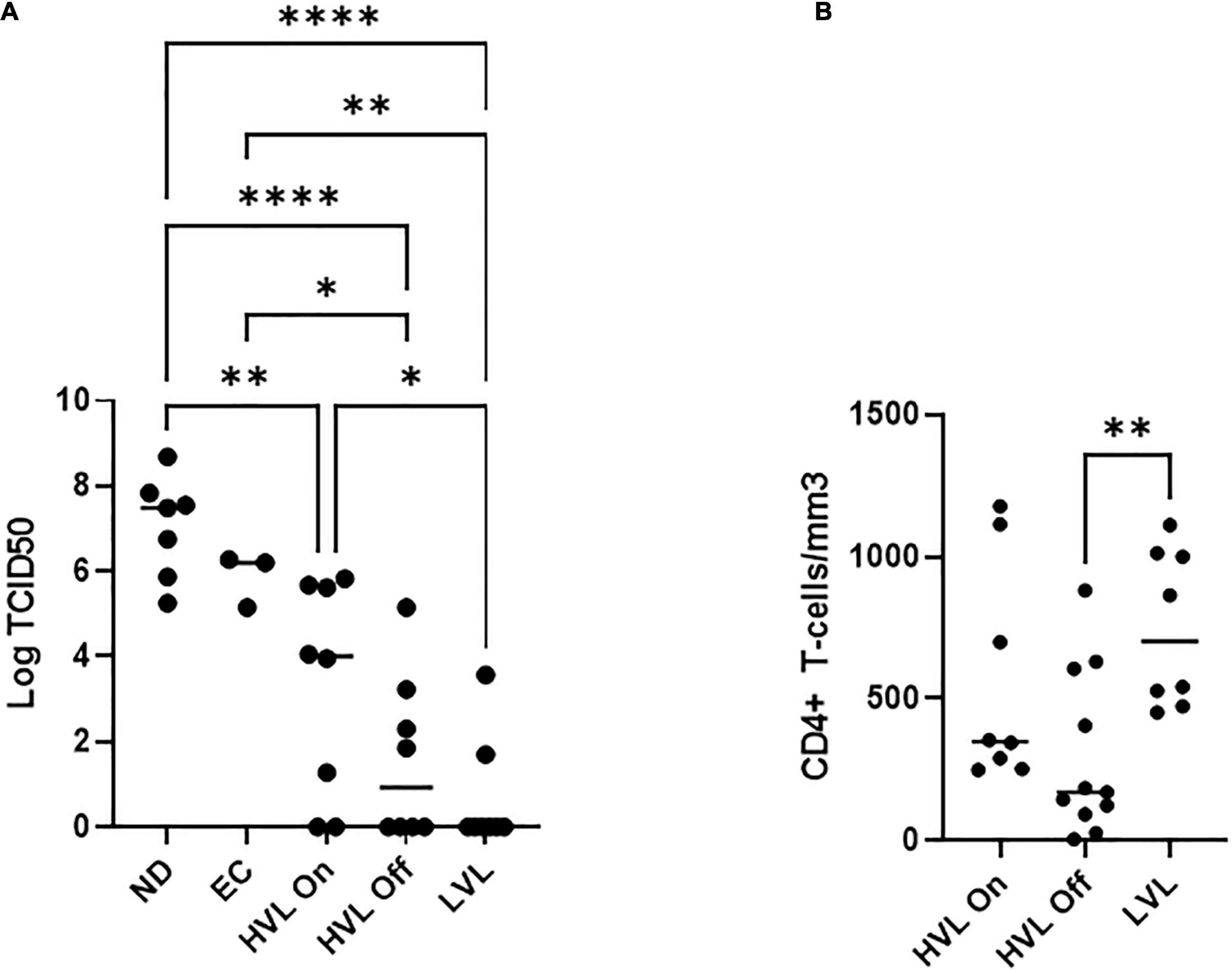
Figure 1. PBMCs from different classes of HIV-1 infected individuals show different susceptibility to de novo infection. (A) Limiting dilutions of NL4-3 were used to infect arrays of PBMCs from normal donors (ND), elite controllers (EC), high viral load on or off therapy (HVL On, HVL Off), or low viral load (LVL) donors in 96 well plates. p24 ELISA was used to determine productive infection in a given well and TCID50 was calculated. One-way ANOVA with Tukey’s multiple comparison was performed. P-value: ∗ < 0.05, ∗∗ < 0.01, **** < 0.0001. (B) CD4+ T-cell counts in the blood of HVL (on/off therapy) and LVL. Mann-Whitney test was used to compare groups ∗∗P < 0.01.
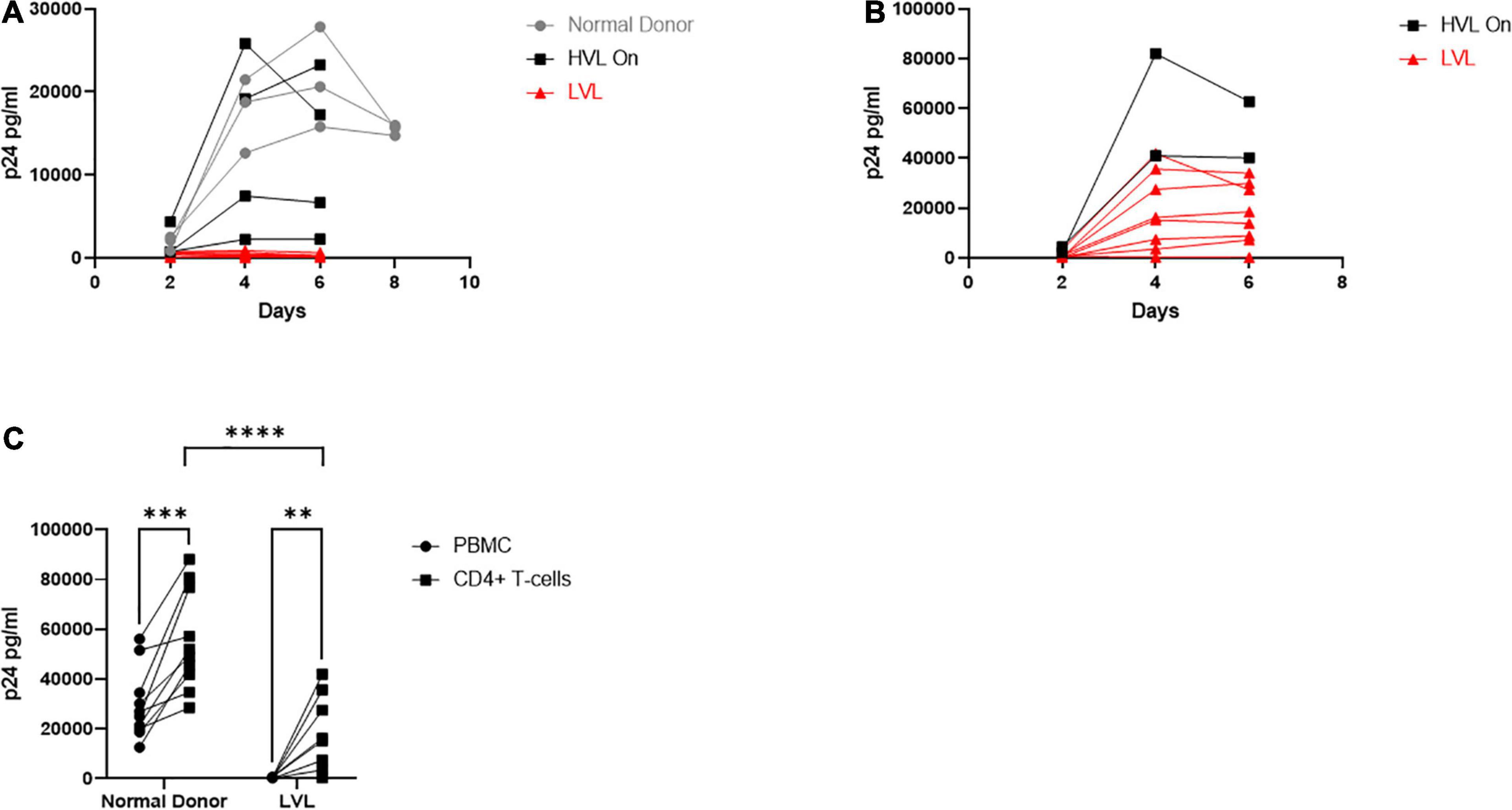
Figure 2. Total PBMCs from low viral load (LVL) donors do not support HIV spreading infection in vitro. (A) Total PBMCs collected from normal donors (gray, n = 3), high viral load donors on ART (HVL, black, n = 4) and ART naïve low viral load donors (LVL, red, n = 8) were induced with PHA and IL-2 then infected with NL4-3 48 h later. Supernatant was collected every 48 h post infection and virus levels were determined by p24 ELISA. (B) CD4+ T-cells were isolated from PBMCs from HVLs (black, n = 2) and LVLs (red, n = 8) by magnetic bead based isolation. Resulting CD4+ T-cells were induced with PHA and IL-2 then infected with NL4-3 48 h later. Supernatant was collected every 48 h post infection and virus levels were determined by p24 ELISA. (C) Measurement of peak p24 production in total PBMCs or isolated CD4+ T-cells from the indicated groups. Two-way ANOVA with Sidak’s multiple comparison test was performed. P-value: ∗∗ < 0.01, ∗∗∗ < 0.001, **** < 0.0001.
LVL CD4+ T-Cells Are Susceptible to the HIV Infection
The resistance of PBMCs from LVLs to infection could be due to many factors, including resistance to infection of CD4+ and/or CD8+ T-cell anti-HIV activity. To decipher between a CD4+ and CD8+ T-cell mediated phenotype, we performed an HIV-1 spreading infection assay on an isolated CD4+ T-cell population (Figure 2B). CD4+ T-cells were isolated from uninduced PBMCs from HVLs and LVLs using Miltenyi CD4+ T-cell negative isolation kit, purity of > 90% confirmed by flow cytometry and cells were activated and infected as above. All CD4+ T-cells isolated from LVLs were susceptible to HIV-1 infection except one (AEM9650) (Figure 2B). HLA typing revealed none of the HLA-B mutations commonly associated with viral resistance (data not shown). Comparing total virus output from infected PBMCs and CD4+ T-cells at days of peak replication, revealed that although CD4+ T-cells from LVLs supported HIV-1 infection, significantly less virus was produced compared to normal donors (Figure 2C).
Control of Viral Replication in vitro by LVLs Is Mediated by CD8+ T-Cells
We next investigated the role of CD8+ T-cells in the ability of LVLs to control viral replication in vitro (Figure 3). We depleted CD8+ T-cells from PBMCs from LVLs using a magnetic bead-based method. Depletion was verified by flow cytometry and we confirmed > 90% depletion of CD8+ T-cells. Cells were activated and PBMCs were infected as above. Depletion of CD8+ T-cells from PBMCs of two LVL donors resulted in susceptibility to HIV-1 infection (Figures 3A,B). Isolated CD8+ T-cells were also titrated back in to the PBMCs and this addback restored the suppressive phenotype at a ratio of 1:1 and 1:2 PBMCs:CD8s in one donor (Figure 3A) and at 1:2 PBMCs:CD8s in another donor (Figure 3B). We next tested whether CD8+ T-cells alone were sufficient for suppression of viral replication in CD4+ T-cells from an LVL. Populations of CD4+ and CD8+ T-cells were isolated from PBMCs of an LVL donor and a normal donor, activated and infected as above. As expected, isolated CD4+ T-cells were susceptible to viral replication (Figures 3C,D). Titration of CD8+ T-cells back with CD4+ T-cells at all ratios tested mediated suppression of viral replication from the LVL (Figure 3C) and not from the normal donor (Figure 3D). These results suggest that CD8+ T-cells are both necessary and sufficient to mediate the observed suppression of HIV-1 replication in vitro.
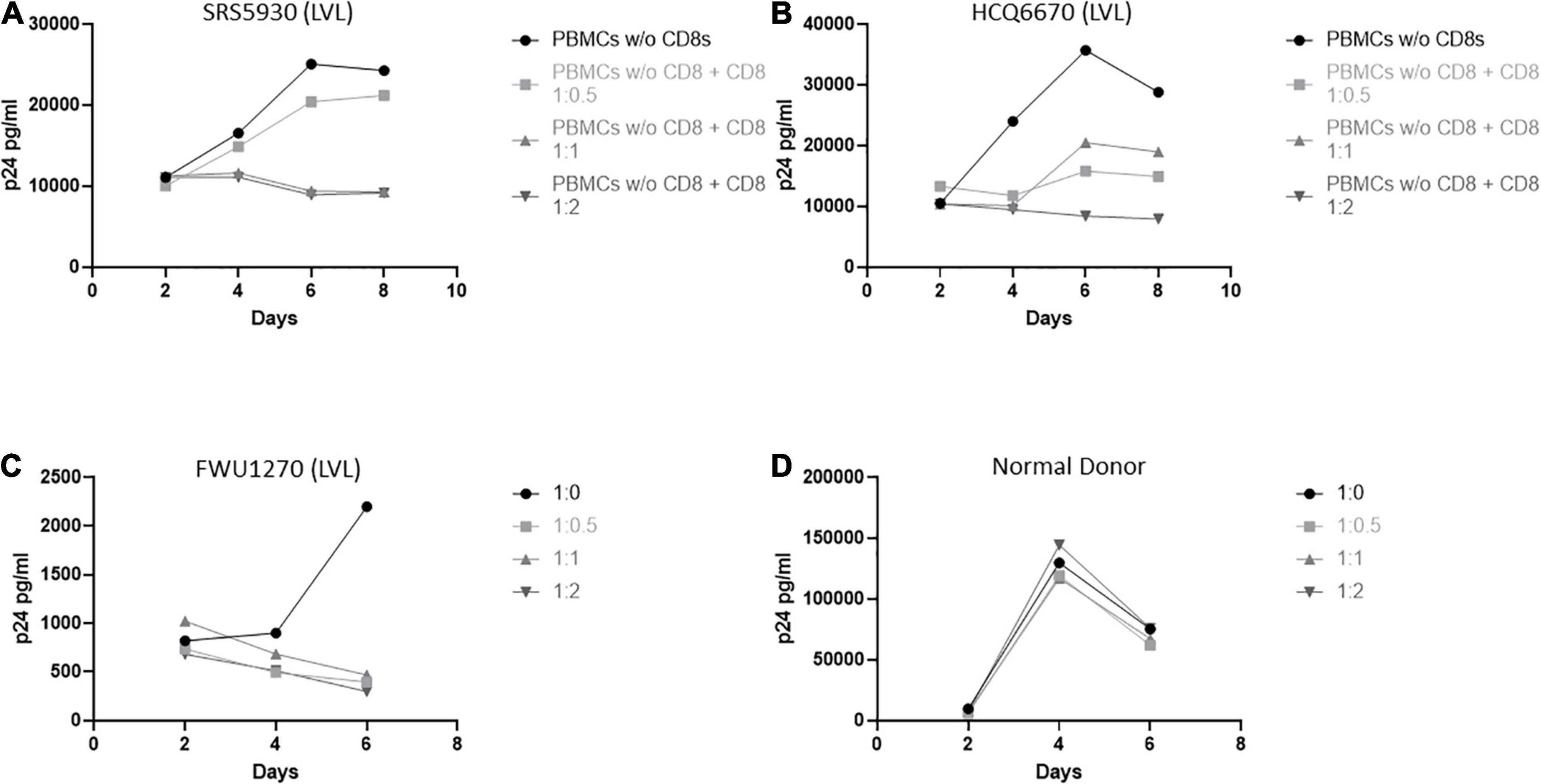
Figure 3. CD8+ T-cells are necessary and sufficient for control of viral replication by LVLs in vitro. (A,B) CD8+ T-cells were depleted from PBMCs of two LVLs. Depleted PBMCs and CD8+ T-cells were then stimulated for 48 h with PHA and IL-2 before being combined at the indicated ratio of PBMC:CD8 and infected with NL4-3. Supernatant was collected every 48 h post infection and virus levels were determined by p24 ELISA. CD4+ and CD8+ T-cells were isolated from PBMCs from (C) an LVL or (D) a normal donor. Isolated T-cells were induced with PHA and IL-2, combined as above (CD4:CD8) then infected with NL4-3 48 h later. Supernatant was collected every 48 h post infection and virus levels were determined by p24 ELISA.
HLA Compatibility Is Required for Induced CD8+ T-Cell Anti-HIV Function in Heterologous CD4+ T-Cells
It was previously reported by Killian et al., that an HLA class I match is required for HIV-1 inhibition by uninduced heterologous CD8+ T-cells (Mackewicz et al., 1998; Killian et al., 2018). In our donor cohort, we were able to identify several donors with matching HLA types (Table 2). To test the requirement of HLA compatibility for the ability of CD8+ T-cells from LVL donors to suppress HIV replication, we co-cultured activated PBMCs from an LVL donor with HLA-matched and non-HLA-matched isolated, activated heterologous CD4+ T-cells and infected as above (Figure 4). Of the LVLs in our study we chose to work with NIM1164 as this donor has matched HLA types with multiple other donors. We performed experiments to examine the ability of PBMCs from NIM1164 to suppress viral replication in isolated CD4+ T-cells from YCX1164, a primary relative containing several HLA matches (Figure 4A), BTS1096 a normal donor with matches in HLA-B, HLA-C, and DRB1 (Figure 4B), HCQ6670 a LVL with matches in HLA-A, HLA-B, and HLA-C (Figure 4C), BRW1143 an HVL with matches in HLA-B, HLA-C, and DRB1 (Figure 4D) and HFK1114 a normal donor with no matching HLA alleles (Figure 4F). The analysis of HIV-1 spreading infection in CD4+ T-cells and LVL PBMCs co-cultures at all CD4:PBMC ratios demonstrated marked inhibition of HIV-1 replication in cultures with matching HLAs compared to CD4+ T-cells alone (Figures 4A–E). Only minimal HIV-1 suppression was observed when LVL PBMCs were mixed with allogeneic CD4+ T-cells without matching HLA (Figure 4E). This suggests that HLA-class I compatibility is required for allogeneic suppression of HIV-1 replication by CD8+ T-cells from LVLs.
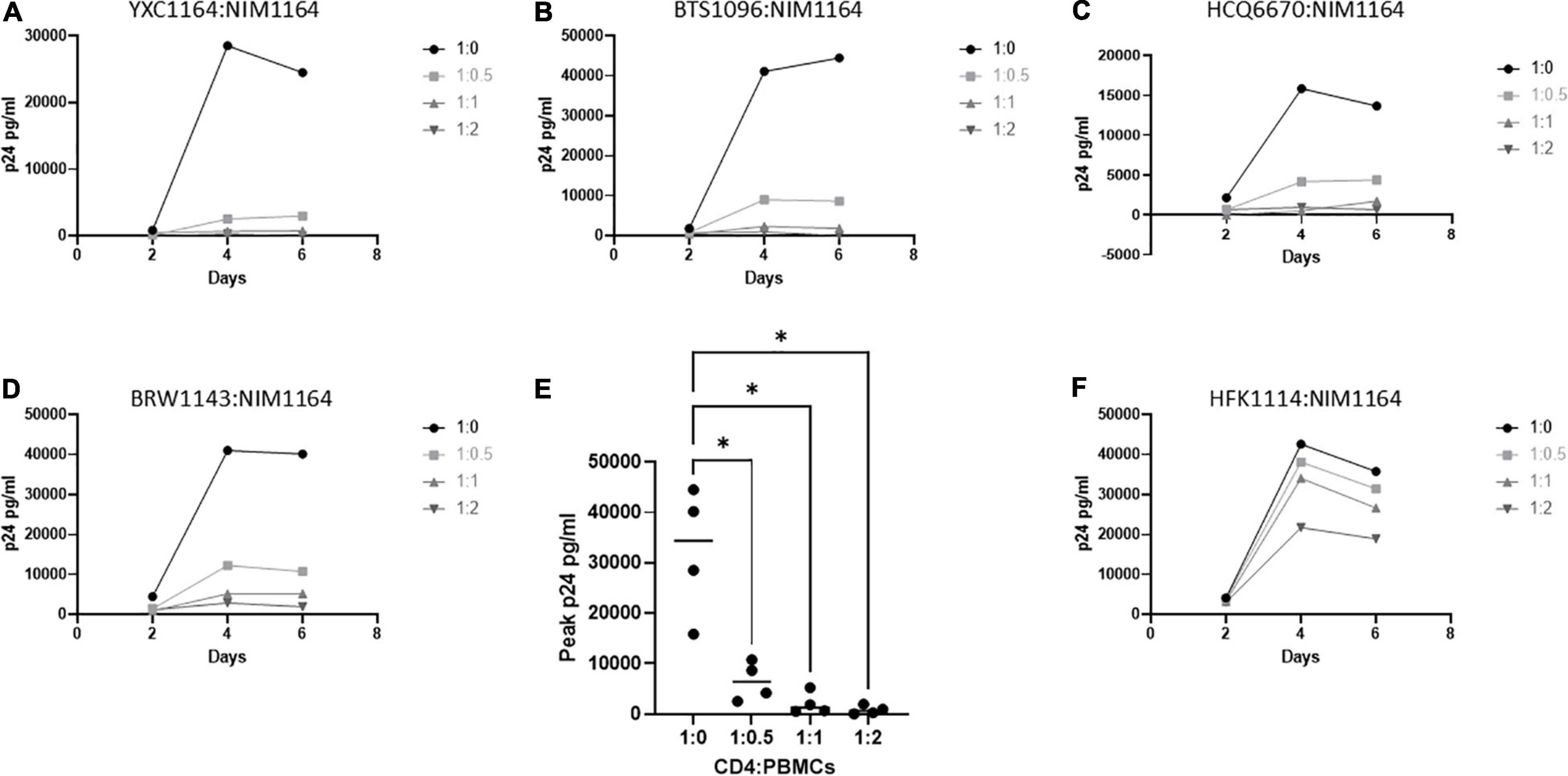
Figure 4. HLA compatibility is a requirement for LVL mediated HIV-1 suppression in heterologous CD4+ T-cells. PBMCs from LVL NIM1164 and isolated CD4+ T-cells from donors with varying degrees of HLA match were induced with PHA and IL-2. Heterologous CD4+ T-cell isolates from HLA-matched and mismatched donors and PBMCs from a LVL (NIM1164) were co-cultured at the indicated ratio of CD4:PBMCs and infected with NL4-3. Supernatant was collected every 48 h post infection and virus levels were determined by p24 ELISA. (A) YXC1164 is an HLA-matched normal donor primary relative of NIM1164. (B) BTS1096 is an HLA-matched normal donor. (C) HCQ6670 is a HLA-matched LVL. (D) BRW1143 is a HLA-matched HVL. (E) Measurement of peak p24 production from LVL HLA-matched donors at the indicated CD4:PBMC ratio. One-way ANOVA was performed. P-value: ∗ < 0.05. (F) HFK1114 is a HLA-mismatch normal donor.
Concurrent HIV Infection Is Required for CD8+ T-Cell Anti-HIV Function in LVL Donors
During the duration of this study, several donors classified as LVLs were placed on ART (Table 3). To determine if the ability to control viral replication in vitro was related to viremia, we followed these individuals over time after initiation of therapy. PBMCs isolated from pre-therapy (black lines) and post-therapy (blue lines) were evaluated for their ability to support HIV-1 replication in vitro (Figure 5). For the LVL NIM1164, we observed suppression of viral replication in PBMCs for over a year prior to the start of therapy. ART successfully reduced viral load to undetectable levels (Table 3) and with this we observed low levels of viral replication in vitro (Figure 4A). A similar trend was observed with the LVL MPY1313 wherein therapy quickly suppressed viral load and a subsequent increase in the susceptibility of PBMCs to viral replication was observed. At 273 days post initiation of therapy, robust viral replication was measured in vitro (Figure 5B). These findings suggest that a detectable viral load is required for the observed control in vitro. In LVL VQY4910 a different pattern was observed. Following the start of ART an increase in the susceptibility of PBMCs to viral infection was observed over time (Figure 5C). For all three subjects PBMCs from the last time point sampled post-ART were incapable of supporting viral replication (Figure 5D). However, several pre-therapy samples for VQY4910 showed the ability to permit low level viral replication in vitro. This susceptibility fluctuated over time. Where data was available, we observed that time points that demonstrated viral control had a CD4:CD8 ratio in the blood of far <1 (Table 3). The samples with the greatest observed replication had a ratio close to one. Looking back at our other LVL samples that exhibited control, we also saw that these individuals had a CD4:CD8 ratio less than one, despite maintaining healthy CD4+ T-cell counts. This observation suggested that a subject must have both a detectable viral load and a CD4:CD8 ratio <1 in order to exhibit control in vitro.
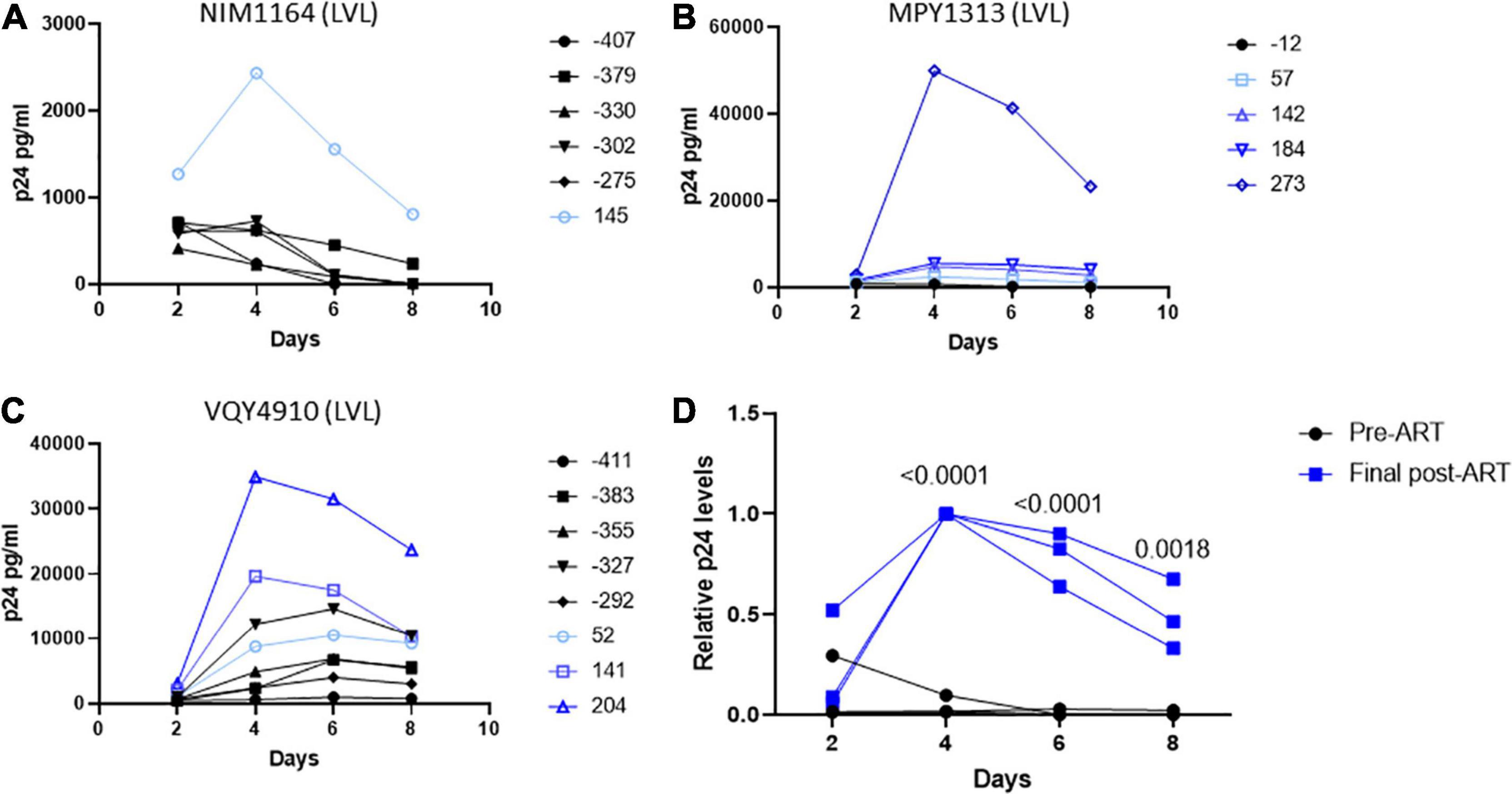
Figure 5. Suppression of viral load in LVLs by ART results in loss of in vitro control of viral replication. Longitudinal PBMC samples from LVLs (A–C) placed on ART. PBMCs were induced with PHA and IL-2 then infected with NL4-3 48 h later. Supernatant was collected every 48 h post infection and virus levels were determined by p24 ELISA. (D) Comparison of relative p24 levels pre- vs final post-ART for LVL donors. One-way ANOVA was performed. (A–D) Numbers indicate days post-ART. Black lines are pre-ART PBMC cultures and blue lines are post-ART.
Pre-therapy CD8+ T-Cells From LVLs Are Capable of Suppressing Viral Replication in Post-therapy CD4+ T-Cells
We wanted to determine if CD8+ T-cells from pre-therapy, in vitro controlling, LVL donors could suppress viral replication in post-therapy samples that have lost the ability to control HIV-1 replication in vitro. To test this we activated and infected mixed PBMC cultures of MPY1313 from pre- and post-therapy time points with NL4-3 (post:pre; Figure 6A). As expected, post-therapy PBMC cultures alone supported and pre-therapy cultures alone suppressed viral replication. A mixture of post- and pre- therapy samples at 1:1 and 1:2 showed a complete suppression of viral replication. To confirm that this effect was specific to CD8+ T-cells as described above, we co-cultured activated, isolated CD4+ T-cells from post-therapy samples with activated, isolated CD8+ T-cells from pre-therapy samples and infected with NL4-3 (Donor MPY1313 and VQY4910; Figures 6B,C). CD8+ T-cells from pre-therapy samples successfully suppressed viral replication in CD4+ T-cells from post-therapy samples.

Figure 6. Pre-therapy CD8+ T-cells suppress replication in post-therapy CD4+ T-cells. Samples from before and after initiation of therapy were used to determine if pre-therapy samples could control viral replication in post-therapy samples. (A) PBMCs from MPY1313 and isolated CD4+ and CD8+ T-cells from (B) MPY1313 and (C) VQY4910 pre and post-therapy were mixed at the indicated ratios (post:pre) and infected with NL4-3. Supernatant was collected every 48 h and virus levels were determined by p24 ELISA.
To expand upon these findings and examine the need for cell to cell contact, we performed a similar experiment using a transwell setup to separate the isolated CD4+ and CD8+ T-cells (Figure 7). T-cell populations were isolated from MPY1313 and VQY4910 PBMCs. CD4+ T-cells from post-therapy time points were placed in a well and CD8+ T-cells from pre-therapy time points were either co-cultured directly with CD4+ T-cells or placed in transwell. Cultures were infected with NL4-3 and viral replication followed by measuring p24 levels in the supernatant over 6 days. Direct contact of CD8+ and CD4+ T-cells prevented viral replication. CD8+ T-cells in transwell were unable to mediate this effect, suggesting a requirement for cell to cell contact.
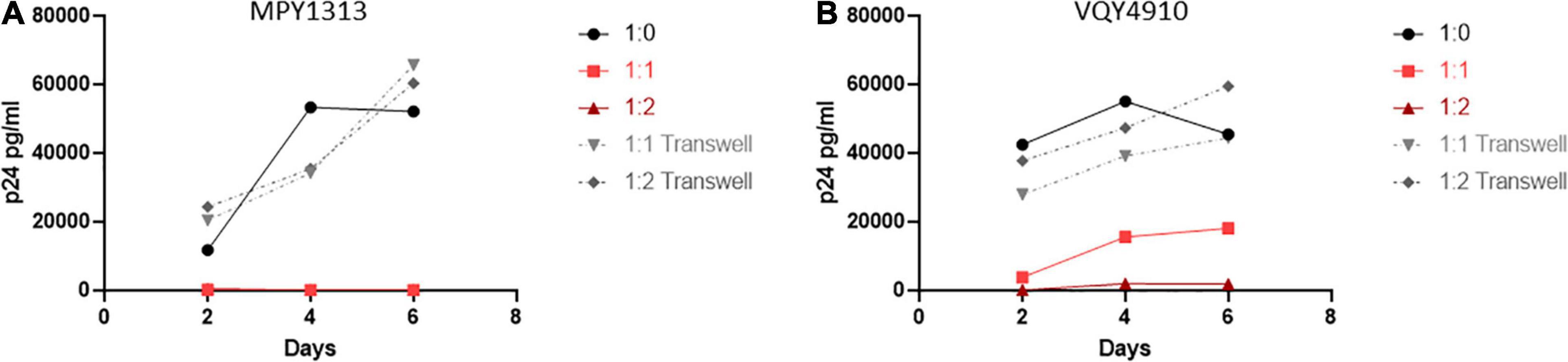
Figure 7. Cell contact is necessary to mediate viral suppression in LVLs. CD4+ T-cells were isolated from post-therapy samples from (A) MPY1313 and (B) VQY4910 and cultured with isolated CD8+ T-cells from the same donor pre-therapy. CD4+ and CD8+ T-cells were stimulated with PHA and IL-2 for 48 h before being combined in the given ratios (CD4:CD8) in direct contact (red solid lines) or in transwell (gray dashed lines). Cultures were infected with NL4-3, supernatant was collected every 48 h post infection and virus levels were determined by p24 ELISA.
Control of Infection Is Associated With Low Expression of the PD-1 Exhaustion Marker on CD8+ T-Cells
We next sought to determine if control in LVLs was the result of measurable changes in CD8+ T-cells. Chronic infection has been associated with alteration of the activation of state of T-cells and the expression of markers of immune exhaustion (Wherry et al., 2007; Wherry and Kurachi, 2015; Saeidi et al., 2018). Increased expression of exhaustion markers such as PD-1 are associated with decreased CD8+ T-cell function (Day et al., 2006; Petrovas et al., 2006; Trautmann et al., 2006) and these markers have been seen in HIV infected individuals (Day et al., 2006; Petrovas et al., 2006; Trautmann et al., 2006; Sauce et al., 2011). We performed flow cytometry to assess the levels of PD-1 expressed on CD8+ T-cells from normal donors, HVL and LVL donors (Figure 8). In keeping with previously published studies, CD8+ T-cells from HIV + subjects with high viral loads had increased levels of PD-1. Our LVL subjects had PD-1 levels indistinguishable from normal donors (Figures 8B,C). To determine if expression of PD-1 correlates with control we examined the level of PD-1 expression on CD8+ T-cells from LVLs before the start of ART and the same subjects after ART when their PBMCs were able to support replication of HIV-1 in culture (Figure 8D). PD-1 levels were significantly higher in LVLs after the initiation of ART. We also performed flow cytometry to assess changes in immune activation by measuring the co-expression of HLA-DR and CD38 on CD8+ T cells from normal donors, LVLs, and HVLs (Figures 8E–G). Similar to what was observed with exhaustion, CD8+ T cells from HVLs with high viral loads had increased immune activation in comparison to both normal donors and LVLs (Figure 8F). To determine if immune activation also correlates with control, we evaluated the longitudinal co-expression of CD38/HLA-DR on CD8+ T cells both pre- and post-ART in our cohort of LVLs and determined that there was no significant difference in immune activation during times of in vitro control (pre-ART) in comparison to when PBMCs were susceptible to infection in vitro (post-ART) (Figure 8G).
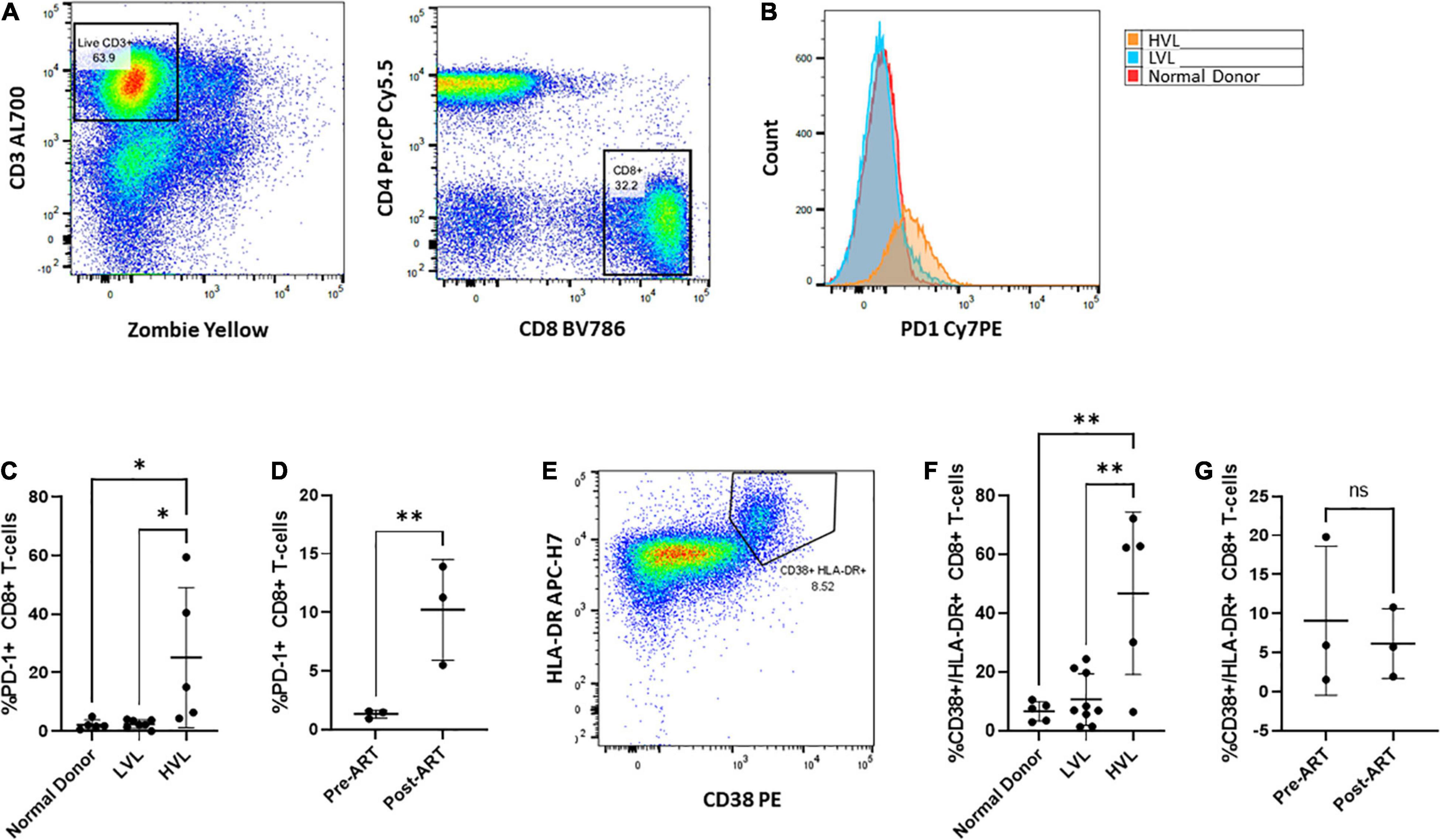
Figure 8. Phenotypic characterization of CD8+ T cells Pre- and Post-therapy. PBMCs from normal, LVL or HVL donors were stained for both T-cell exhaustion using PD-1 and immune activation using HLA-DR and CD38 and analyzed by flow cytometry. (A) Gating strategy for determining the exhausted population of CD8+ T-cells. Following gating to exclude debris and doublets, gates were drawn around live CD3 + T-cells and this population used to identify CD4-/ CD8+ T-cell populations. (B) Comparison between normal donor (red), ART naïve LVL donor (blue), and ART naïve HVL donor (orange) expression of PD-1. (C) Comparison of CD8+ T-cell expression of PD-1 between normal donors (n = 5), ART naïve LVLs (n = 7), and ART naïve HVLs (n = 5) statistical significance was determined by one-way ANOVA, ∗P < 0.05. (D) A longitudinal evaluation of CD8+ T-cell exhaustion pre- and post- therapy. A comparison of the expression of PD-1 was evaluated for 3 LVL donors both pre-ART and post-ART, ∗∗P < 0.01. (E) Immune activation was determined by gating CD8+ T-cells that expressed both HLA-DR and CD38. (F) Comparison of CD8+ T-cell expression of HLA-DR and CD38 between normal donors (n = 5), ART naïve LVLs (n = 7), and ART naïve HVLs (n = 5) statistical significance was determined by one-way ANOVA, ∗∗P < 0.01. (G) A longitudinal evaluation of CD8+ T-cell activation pre- and post-therapy.
Discussion
Despite years of study we still do not completely understand what mediates natural control of HIV-1 infection in some individuals. Although studies have implicated multiple mechanisms that underlie many observed types of control, some mechanisms have not yet been elucidated. Our studies indicate that infected individuals with constantly low viral loads also demonstrate control of viral replication in vitro (Figure 2). This finding in itself provides another assay that could be performed to help further stratify types of control beyond available clinical observations.
Although absolute CD4+ T-cell count was initially used prognostically to assess progression to AIDS, it was observed that the rate of CD4+ T-cell decline varied significantly. Some individuals lost > 100 cells/μl per year, while others remained immunologically stable with normal CD4+ T-cell counts that sustained little to no loss over several years (Phair et al., 1992; Levy, 1993; Schwartländer et al., 1993; Buchbinder et al., 1994; Keet et al., 1994; Muñoz et al., 1995; Patrikar et al., 2014; Parsa et al., 2020). Before viral loads could be routinely measured, Dr. Ho et al. described a cohort of LTNPs who were HIV positive yet remained clinically asymptomatic without prolonged use of ART (Huang et al., 1995). These LTNPs, who were defined by CD4+ T-cell measurements and clinical outcomes, maintained normal CD4+ T-cell counts and had not developed opportunistic disease over an extended period of time. With the development of new technologies, focus shifted to quantifying plasma HIV-1 RNA as an independent reproducible marker of disease progression (Saksela et al., 1994; Mellors et al., 1995; Mellors et al., 1996). In 1997 Mellors et al., evaluated the rate of HIV disease progression in respect to both CD4+ T-cell count and plasma viremia. It was demonstrated that the rate of CD4+ T-cell loss was directly correlated to the level of HIV-1 viremia and together these parameters constituted the most accurate predictors of HIV-1 progression (O’Brien W. A. et al., 1996; Mellors et al., 1997; de Wolf et al., 1997). Those with high “viral loads” lost CD4+ T-cells more quickly than those with low or undetectable viral loads, who had little to no loss of CD4+ T-cells. Effective HIV-1 therapy confirmed that control of viremia prevented CD4+ T-cell loss and even allowed some CD4+ T-cell recovery in most cases. In support of this, it was found that the viral loads in LTNP cohorts varied from below average to undetectable (Pinto et al., 1995; García et al., 1997).
The measurement of viral load allowed further delineation of levels of control. Subjects who maintained low, but still detectable viral load remained LTNPs. Infected individuals with undetectable viral loads (<50 RNA copies/ml) began to be appreciated as a separate group (Clerici et al., 1992; Kelker et al., 1992; Cao et al., 1995; Pinto et al., 1995; Rowland-Jones et al., 1995; Fowke et al., 1996; Rowland-Jones et al., 1998; Lefrère et al., 1999; Pereyra et al., 2008). In the 2000’s, researchers began focusing on these subjects. This cohort, called elite controllers (EC) by some, has been studied by several groups (Shacklett, 2006; Dyer et al., 2008; Pereyra et al., 2008; Gonzalo-Gil et al., 2017; Pernas et al., 2018; Lopez-Galindez et al., 2019; Nguyen et al., 2019). Each showed that EC CD4+ T-cells supported HIV-1 in vitro growth as well as non-EC. Work on understanding the mechanism by which ECs suppress the virus has focused on CD8+ T-cells and the cytotoxic T-lymphocyte (CTL) response. Genetic analysis has found partially “protective” MHC alleles (Migueles et al., 2000). In these studies, isolated CD4+ T-cell were used exclusively (Rabi et al., 2011).
Previous studies, especially those examining ECs, have focused on the role of the CD8+ CTL response in controlling infection (Koup et al., 1994; Borrow et al., 1994; Barker et al., 1998; Wilkinson et al., 1999; Betts et al., 2001; Migueles and Connors, 2001; Sáez-Cirión et al., 2007; Betts et al., 2006; Yan et al., 2013; Cartwright et al., 2016; Nishimura et al., 2017; Nguyen et al., 2019; McBrien et al., 2020a,b). The PBMCs from our LVLs were resistant to infection, but isolated CD4+ T-cells were capable of supporting low level viral replication (Figure 2). Further experiments with isolated CD4+ and CD8+ T-cells revealed that CD8+ T-cells were both necessary and sufficient to mediate control of infection in vitro (Figure 3). The ability of LVL PBMCs to mediate suppression of viral replication in HLA-matched allogeneic CD4+ T-cells (Figure 4) and the requirement for cell to cell contact (Figure 7) strongly support that the observed control is due to classical CTL function. What remains to be determined is how LVLs maintain effective CD8+ T-cell functionality during HIV infection. That a detectable viral load remains in these individuals and the lack of known protective HLA alleles suggests this mechanism is different from what has been described for ECs (Migueles et al., 2000; Brener et al., 2015; Adland et al., 2018).
Unique to this study is the realization that the observed control in vitro is dependent upon the presence of detectable viral load in the donor and correlates with a low CD4:CD8 ratio (Figures 4, 5). This observation lead us to discover that HVL donors who had these characteristics (detectable plasma viral load and CD4:CD8 ratio < 1) also had PBMCs that were resistant to viral replication in vitro (Figures 5D,E). Our data does not indicate why this control in tissue culture correlates with successful clinical control in some donors but not others. The observation that LVLs can lose control over time (Figure 5) and that CD8+ T-cells from time points before the loss of control can suppress replication in post-control CD4+ T-cells (Figure 6) implies a change not in the target T-cell, but the functionality of the CD8+ T-cells.
The elucidation of the CD8+ T-cell driven mechanism of control in our LVL donors strongly supports the examination of viral replication in vitro as a way to further delineate types of clinical control. While it is possible that the lack of control in HVLs may be due to CTL escape of the virus, our examination of loss of control in LVLs suggests that the failure to control may be related to the subject’s immune system and not the virus. Our studies specifically highlight a role for changes in the level of CD8+ T-cell exhaustion and not immune activation. Although examination of changes over a greater period of time may reveal a role for T-cell activation. Extensive studies in the field have linked chronic immune activation to disease progress (Ascher and Sheppard, 1988; Fuchs et al., 1988; Fahey et al., 1990; Sheppard et al., 1991; Giorgi et al., 1993, 1999; Liu et al., 1997; Zajac et al., 1998; Hazenberg et al., 2003; Deeks et al., 2004; Hunt et al., 2008; Liovat et al., 2009) and more recent studies have examined T-cell exhaustion in chronic HIV infection (Day et al., 2006; Petrovas et al., 2006; Trautmann et al., 2006; Wherry et al., 2007; Sauce et al., 2011; Wherry and Kurachi, 2015; Saeidi et al., 2018). These two mechanisms go hand in hand and are strong candidates for explaining loss of control in LVLs over time as suggested by our evaluation of PD-1. Understanding this mechanism may lead to the development of interventions that could be used to induce a functional cure in people living with HIV.
Materials and Methods
Ethics Statement
The Smith Center for Urban Health and Infectious Disease, East Orange, NJ obtained written informed consent for the collection of blood donations from participating subjects. Samples were collected by trained medical staff under approved University of the Sciences’ protocol (IRB protocol 900702-3 and 797649-3).
Human Subjects
All donors were recruited by the Smith Center for Urban Health and Infectious Disease. Two cohorts of HIV-1 infected donors were recruited, Low Viral Load (LVL) and High Viral Load (HVL) (characteristics at recruitment—Table 1). For the LVL cohort, 8 HIV-1 seropositive ART naïve donors were recruited, who were able to suppress HIV-1 infection independent of known protective HLA alleles. Four of these LVL donors were placed on combination ART during the course of this study. The HVL cohort included HIV-1 seropositive HVL donors pre- and post-therapy. Additionally, we recruited HIV-1 seronegative healthy control donors, Normal Donors that matched the age and characteristics of the two HIV-1 positive groups. For this study, classification as an HIV-1 controller required viremic control for a duration of at least 12 consecutive months in the absence of ART (O’Brien T. R. et al., 1996; Quinn et al., 2000; Gurdasani et al., 2014). HIV-1 controllers are defined as seropositive donors with viral loads of < 2,000 copies/mL and a CD4+ T-cell count > 500 cells/mm3 (O’Brien T. R. et al., 1996; Quinn et al., 2000; Gurdasani et al., 2014). HIV-1 seropositive donors that had low CD4+ T-cell counts, below 200 and had high viral load, above 5,000 copies/mL were designated as HVL donors.
PBMC Isolation and Activation
PBMCs were purified from whole blood samples using Ficoll (GE Healthcare) gradient centrifugation and cryopreserved in 90% Fetal Bovine Serum (FBS; HyClone) containing 10% dimethyl sulfoxide (DMSO; Fisher). Frozen PBMCs were thawed and cultured in Roswell Park Memorial Institute (RPMI)-1640 complete media (GenClone) supplemented with 20% heat inactivated FBS, 1x penicillin-streptomycin-glutamine (PSG; Thermo Fisher Scientific), and 5% (5 U/mL) human rIL-2 (NIH AIDS Reagent Program). Cells were induced with 5 μg/mL phytohaemagglutinin-P (PHA-P) (Sigma) for 48 h at 37°C and 5% CO2.
CD4+ and CD8+ T-Cell Isolation and Activation
CD4+ and CD8+ T-cells were purified from frozen PBMCs using MACS Miltenyi negative isolation kits (cat# 130-096-533 and cat# 130-096-495, respectively) according to manufacturer’s protocol. Enriched CD4+ and CD8+ T-cell populations were independently activated in RPMI complete media with 5μg/mL PHA for 48 h at 37°C and 5% CO2.
HIV-1 Virus Stock
The HIV-1 stock used in this study was generated by transfecting pNL4-3 (NIH AIDS Reagent Program, ARP-2852, contributed by Dr. M. Martin) into HEK293T cells [American Type Culture Collection (ATCC, CRL-11268)] using TransFectin Lipid Reagent (BioRad, Cat# 1703351) following manufacturer’s instructions. Transfected cells were cultured in Dulbecco’s Modified Eagle’s Medium (DMEM) supplemented with 10% FBS and 1x PSG for 48 h at 37°C and 5% CO2. Virus containing supernatants were aspirated from the cells, filtered, and frozen in 1 ml aliquots. Frozen stocks were quantified by p24 Gag ELISA.
Spreading Infection Assay
Activated total PBMCs, isolated CD4+ T-cells, or mixed CD4+ and CD8+ T-cells were infected with 17 ng/ml NL4-3 virus at 37°C and 5% CO2. For experiments where isolates were collected and recombined, cells were co-cultured for 3–6 h prior to infection. After 24 h, the virus containing media was removed and replaced. Virus production was evaluated by measuring the p24 levels in the supernatant using ELISA (Zeptometrix). Time points were collected every 48 h post infection for six to 8 days.
Flow Cytometry
For phenotypic analysis of cell populations, all cells were washed with Phosphate Buffered Saline (PBS) without Ca2+ and Mg2+ (GenClone) and stained using fluorescently conjugated antibodies against the following cell surface markers following manufacturer’s instructions: CD3 (BD Biosciences (BD), Alexa700, clone SP34-2), CD4 (BD, PerCP-Cy5.5), CD8 (BD, BV786, clone RPA-T8) and CD279 (PD-1) (Invitrogen, clone eBioJ105), HLA-DR (BD, APC-H7, clone L243), and CD38 (BD, PE, clone HIT2). Cells were also stained for viability using the live/dead stain Zombie Yellow (BioLegend). Cell enumeration was carried out using Cytek FACSort DxP12 flow cytometer and data analysis using FlowJo v10.6.1 software.
Data Availability Statement
The original contributions presented in the study are included in the article/supplementary material, further inquiries can be directed to the corresponding author/s.
Ethics Statement
The Smith Center for Urban Health and Infectious Disease, East Orange, NJ obtained written informed consent for the collection of blood donations from participating subjects. Samples were collected by trained medical staff under approved University of the Sciences’ protocol (IRB protocol 900702-3 and 797649-3).
Author Contributions
ZK and SS conceptualized this project. SK and AJ designed research, performed experiments, and analyzed and interpreted data. TJ assisted in experiments. AJ, SK, SS, and ZK wrote the manuscript. ZK aided in the design of the experiments and the analysis and interpretation of data. ES recruited patients, handled patient education and organization of clinical data. All authors contributed to the article and approved the submitted version.
Funding
This work was supported by an NIDA/NIH grant (DP2 DA044550-01) to ZK, Drexel University College of Medicine internal funds to ZK, and contract funds from the Smith Center for Urban Health and Infectious Diseases.
Conflict of Interest
The authors declare that the research was conducted in the absence of any commercial or financial relationships that could be construed as a potential conflict of interest.
Acknowledgments
We would like to thank both the participating donors and The Smith Center for Urban Health and Infectious Disease for providing human blood samples. We would also like to thank Dr. Rachel Van Duyne and Angel Lin for critical review of the manuscript.
References
Adland, E., Hill, M., Lavandier, N., Csala, A., Edwards, A., Chen, F., et al. (2018). Differential Immunodominance Hierarchy of CD8+ T-Cell Responses in HLA-B∗27:05- and -B∗27:02-Mediated Control of HIV-1 Infection. J. Virol. 92:e1685-17. doi: 10.1128/JVI.01685-17
Ascher, M. S., and Sheppard, H. W. (1988). AIDS as immune system activation: a model for pathogenesis. Clin. Exp. Immunol. 73, 165–167.
Barker, E., Mackewicz, C. E., Reyes-Terán, G., Sato, A., Stranford, S. A., Fujimura, S. H., et al. (1998). Virological and immunological features of long-term human immunodeficiency virus-infected individuals who have remained asymptomatic compared with those who have progressed to acquired immunodeficiency syndrome. Blood 92, 3105–3114.
Betts, M. R., Ambrozak, D. R., Douek, D. C., Bonhoeffer, S., Brenchley, J. M., Casazza, J. P., et al. (2001). Analysis of total human immunodeficiency virus (HIV)-specific CD4(+) and CD8(+) T-cell responses: relationship to viral load in untreated HIV infection. J. Virol. 75, 11983–11991. doi: 10.1128/JVI.75.24.11983-11991.2001
Betts, M. R., Nason, M. C., West, S. M., De Rosa, S. C., Migueles, S. A., Abraham, J., et al. (2006). HIV nonprogressors preferentially maintain highly functional HIV-specific CD8+ T-cells. Blood 107, 4781–4789. doi: 10.1182/blood-2005-12-4818
Borrow, P., Lewicki, H., Hahn, B. H., Shaw, G. M., and Oldstone, M. B. (1994). Virus-specific CD8+ cytotoxic T-lymphocyte activity associated with control of viremia in primary human immunodeficiency virus type 1 infection. J. Virol. 68, 6103–6110. doi: 10.1128/JVI.68.9.6103-6110.1994
Brener, J., Gall, A., Batorsky, R., Riddell, L., Buus, S., Leitman, E., et al. (2015). Disease progression despite protective HLA expression in an HIV-infected transmission pair. Retrovirology 12:55. doi: 10.1186/s12977-015-0179-z
Buchbinder, S. P., Katz, M. H., Hessol, N. A., O’Malley, P. M., and Holmberg, S. D. (1994). Long-term HIV-1 infection without immunologic progression. AIDS 8, 1123–1128. doi: 10.1097/00002030-199408000-00014
Cao, Y., Qin, L., Zhang, L., Safrit, J., and Ho, D. D. (1995). Virologic and immunologic characterization of long-term survivors of human immunodeficiency virus type 1 infection. N. Engl. J. Med. 332, 201–208. doi: 10.1056/NEJM199501263320401
Cartwright, E. K., Spicer, L., Smith, S. A., Lee, D., Fast, R., Paganini, S., et al. (2016). CD8(+) lymphocytes are required for maintaining viral suppression in SIV-infected macaques treated with short-term antiretroviral therapy. Immunity 45, 656–668. doi: 10.1016/j.immuni.2016.08.018
Clerici, M., Giorgi, J. V., Chou, C. C., Gudeman, V. K., Zack, J. A., Gupta, P., et al. (1992). Cell-mediated immune response to human immunodeficiency virus (HIV) type 1 in seronegative homosexual men with recent sexual exposure to HIV-1. J. Infect. Dis. 165, 1012–1019. doi: 10.1093/infdis/165.6.1012
Day, C. L., Kaufmann, D. E., Kiepiela, P., Brown, J. A., Moodley, E. S., Reddy, S., et al. (2006). PD-1 expression on HIV-specific T-cells is associated with T-cell exhaustion and disease progression. Nature 443, 350–354. doi: 10.1038/nature05115
de Wolf, F., Spijkerman, I., Schellekens, P. T., Langendam, M., Kuiken, C., Bakker, M., et al. (1997). AIDS prognosis based on HIV-1 RNA, CD4+ T-cell count and function: markers with reciprocal predictive value over time after seroconversion. AIDS 11, 1799–1806. doi: 10.1097/00002030-199715000-00003
Deeks, S. G., Kitchen, C. M., Liu, L., Guo, H., Gascon, R., Narváez, A. B., et al. (2004). Immune activation set point during early HIV infection predicts subsequent CD4+ T-cell changes independent of viral load. Blood 104, 942–947. doi: 10.1182/blood-2003-09-3333
Dyer, W. B., Zaunders, J. J., Yuan, F. F., Wang, B., Learmont, J. C., Geczy, A. F., et al. (2008). Mechanisms of HIV non-progression; robust and sustained CD4+ T-cell proliferative responses to p24 antigen correlate with control of viraemia and lack of disease progression after long-term transfusion-acquired HIV-1 infection. Retrovirology 5:112. doi: 10.1186/1742-4690-5-112
Egger, M., May, M., Chêne, G., Phillips, A. N., Ledergerber, B., Dabis, F., et al. (2002). Prognosis of HIV-1-infected patients starting highly active antiretroviral therapy: a collaborative analysis of prospective studies. Lancet 360, 119–129. doi: 10.1016/s0140-6736(02)09411-4
Fahey, J. L., Taylor, J. M., Detels, R., Hofmann, B., Melmed, R., Nishanian, P., et al. (1990). The prognostic value of cellular and serologic markers in infection with human immunodeficiency virus type 1. N. Engl. J. Med. 322, 166–172. doi: 10.1056/NEJM199001183220305
Fowke, K. R., Nagelkerke, N. J., Kimani, J., Simonsen, J. N., Anzala, A. O., Bwayo, J. J., et al. (1996). Resistance to HIV-1 infection among persistently seronegative prostitutes in Nairobi, Kenya. Lancet 348, 1347–1351. doi: 10.1016/S0140-6736(95)12269-2
Fuchs, D., Hausen, A., Reibnegger, G., Werner, E. R., Dierich, M. P., and Wachter, H. (1988). Neopterin as a marker for activated cell-mediated immunity: application in HIV infection. Immunol. Today 9, 150–155. doi: 10.1016/0167-5699(88)91203-0
García, F., Vidal, C., Gatell, J. M., Miró, J. M., Soriano, A., and Pumarola, T. (1997). Viral load in asymptomatic patients with CD4+ lymphocyte counts above 500 x 10(6)/l. AIDS 11, 53–57. doi: 10.1097/00002030-199701000-00008
Giorgi, J. V., Hultin, L. E., McKeating, J. A., Johnson, T. D., Owens, B., Jacobson, L. P., et al. (1999). Shorter survival in advanced human immunodeficiency virus type 1 infection is more closely associated with T lymphocyte activation than with plasma virus burden or virus chemokine coreceptor usage. J. Infect. Dis. 179, 859–870. doi: 10.1086/314660
Giorgi, J. V., Liu, Z., Hultin, L. E., Cumberland, W. G., Hennessey, K., and Detels, R. (1993). Elevated levels of CD38+ CD8+ T cells in HIV infection add to the prognostic value of low CD4+ T cell levels: results of 6 years of follow-up. The Los Angeles Center, Multicenter AIDS Cohort Study. J. Acquir. Immune Defic. Syndr. 6, 904–912.
Gonzalo-Gil, E., Ikediobi, U., and Sutton, R. E. (2017). Mechanisms of Virologic Control and Clinical Characteristics of HIV+ Elite/Viremic Controllers. Yale J. Biol. Med. 90, 245–259.
Gurdasani, D., Iles, L., Dillon, D. G., Young, E. H., Olson, A. D., Naranbhai, V., et al. (2014). A systematic review of definitions of extreme phenotypes of HIV control and progression. AIDS 28, 149–162. doi: 10.1097/QAD.0000000000000049
Hazenberg, M. D., Otto, S. A., van Benthem, B. H., Roos, M. T., Coutinho, R. A., Lange, J. M., et al. (2003). Persistent immune activation in HIV-1 infection is associated with progression to AIDS. AIDS 17, 1881–1888. doi: 10.1097/00002030-200309050-00006
Huang, Y., Zhang, L., and Ho, D. D. (1995). Characterization of nef sequences in long-term survivors of human immunodeficiency virus type 1 infection. J. Virol. 69, 93–100. doi: 10.1128/JVI.69.1.93-100.1995
Hunt, P. W., Brenchley, J., Sinclair, E., McCune, J. M., Roland, M., Page-Shafer, K., et al. (2008). Relationship between T-cell activation and CD4+ T-cell count in HIV-seropositive individuals with undetectable plasma HIV RNA levels in the absence of therapy. J. Infect. Dis. 197, 126–133. doi: 10.1086/524143
Ingole, N. A., Kukreja, S. M., and Mehta, P. R. (2011). Role of HIV-1 viral load in initiating antiretroviral therapy. World J. AIDS 1, 149–154.
Institute of Medicine (US) Committee on Social Security HIV Disability Criteria (2010). HIV and Disability: Updating the Social Security Listings. Washington, DC: National Academies Press.
Julg, B., Pereyra, F., Buzón, M. J., Piechocka-Trocha, A., Clark, M. J., Baker, B. M., et al. (2010). Infrequent recovery of HIV from but robust exogenous infection of activated CD4(+) T cells in HIV elite controllers. Clin. Infect. Dis. 51, 233–238. doi: 10.1086/653677
Keet, I. P., Krol, A., Klein, M. R., Veugelers, P., de Wit, J., Roos, M., et al. (1994). Characteristics of long-term asymptomatic infection with human immunodeficiency virus type 1 in men with normal and low CD4+ cell counts. J. Infect. Dis. 169, 1236–1243. doi: 10.1093/infdis/169.6.1236
Kelker, H. C., Seidlin, M., Vogler, M., and Valentine, F. T. (1992). Lymphocytes from some long-term seronegative heterosexual partners of HIV-infected individuals proliferate in response to HIV antigens. AIDS Res. Hum. Retroviruses 8, 1355–1359. doi: 10.1089/aid.1992.8.1355
Killian, M. S., Teque, F., and Sudhagoni, R. (2018). Analysis of the CD8+ T-cell anti-HIV activity in heterologous cell co-cultures reveals the benefit of multiple HLA class I matches. Immunogenetics 70, 99–113. doi: 10.1007/s00251-017-1021-7
Koup, R. A., Safrit, J. T., Cao, Y., Andrews, C. A., McLeod, G., Borkowsky, W., et al. (1994). Temporal association of cellular immune responses with the initial control of viremia in primary human immunodeficiency virus type 1 syndrome. J. Virol. 68, 4650–4655. doi: 10.1128/JVI.68.7.4650-4655.1994
Lefrère, J. J., Mariotti, M., Morand-Joubert, L., Thauvin, M., and Roudot-Thoraval, F. (1999). Plasma human immunodeficiency virus RNA below 40 Copies/mL is rare in untreated persons even in the first years of infection. J. Infect. Dis. 180, 526–529. doi: 10.1086/314906
Levy, J. A. (1993). HIV pathogenesis and long-term survival. AIDS 7, 1401–1410. doi: 10.1097/00002030-199311000-00001
Liovat, A. S., Jacquelin, B., Ploquin, M. J., Barré-Sinoussi, F., and Müller-Trutwin, M. C. (2009). African non human primates infected by SIV - Why don’t they get sick? Lessons from studies on the early phase of non-pathogenic SIV infection. Curr. HIV Res. 7, 39–50. doi: 10.2174/157016209787048546
Liu, Z., Cumberland, W. G., Hultin, L. E., Prince, H. E., Detels, R., and Giorgi, J. V. (1997). Elevated CD38 antigen expression on CD8+ T cells is a stronger marker for the risk of chronic HIV disease progression to AIDS and death in the Multicenter AIDS Cohort Study than CD4+ cell count, soluble immune activation markers, or combinations of HLA-DR and CD38 expression. J. Acquir. Immune Defic. Syndr. Hum. Retrovirol. 16, 83–92. doi: 10.1097/00042560-199710010-00003
Lopez-Galindez, C., Pernas, M., Casado, C., Olivares, I., and Lorenzo-Redondo, R. (2019). Elite controllers and lessons learned for HIV-1 cure. Curr. Opin. Virol. 38, 31–36. doi: 10.1016/j.coviro.2019.05.010
Mackewicz, C. E., Garovoy, M. R., and Levy, J. A. (1998). HLA compatibility requirements for CD8(+)-T-cell-mediated suppression of human immunodeficiency virus replication. J. Virol. 72, 10165–10170. doi: 10.1128/JVI.72.12.10165-10170.1998
McBrien, J. B., Mavigner, M., Franchitti, L., Smith, S. A., White, E., Tharp, G. K., et al. (2020a). Robust and persistent reactivation of SIV and HIV by N-803 and depletion of CD8+ cells. Nature 578, 154–159. doi: 10.1038/s41586-020-1946-0
McBrien, J. B., Wong, A., White, E., Carnathan, D. G., Lee, J. H., Safrit, J. T., et al. (2020b). Combination of CD8β Depletion and Interleukin-15 Superagonist N-803 Induces Virus Reactivation in Simian-Human Immunodeficiency Virus-Infected. Long-Term ART-Treated Rhesus Macaques. J. Virol. 94:e00755-20. doi: 10.1128/JVI.00755-20
Mellors, J. W., Kingsley, L. A., Rinaldo, C. R. Jr., Todd, J. A., Hoo, B. S., Kokka, R. P., et al. (1995). Quantitation of HIV-1 RNA in plasma predicts outcome after seroconversion. Ann. Intern. Med. 122, 573–579. doi: 10.7326/0003-4819-122-8-199504150-00003
Mellors, J. W., Muñoz, A., Giorgi, J. V., Margolick, J. B., Tassoni, C. J., Gupta, P., et al. (1997). Plasma viral load and CD4+ lymphocytes as prognostic markers of HIV-1 infection. Ann. Intern. Med. 126, 946–954. doi: 10.7326/0003-4819-126-12-199706150-00003
Mellors, J. W., Rinaldo, C. R. Jr., Gupta, P., White, R. M., Todd, J. A., and Kingsley, L. A. (1996). Prognosis in HIV-1 infection predicted by the quantity of virus in plasma. Science 272, 1167–1170. doi: 10.1126/science.272.5265.1167
Merci, N. M., Emerence, U., Augustin, N., Habtu, M., Julie, I., Angelique, T., et al. (2017). CD4+ cells recovery in HIV positive patients with severe immunosuppression at HAART initiation at Centre Medico-Social Cor-Unum, Kigali. Pan Afr. Med. J. 26:14. doi: 10.11604/pamj.2017.26.14.10488
Migueles, S. A., and Connors, M. (2001). Frequency and function of HIV-specific CD8(+) T-cells. Immunol. Lett. 79, 141–150. doi: 10.1016/s0165-2478(01)00276-0
Migueles, S. A., Sabbaghian, M. S., Shupert, W. L., Bettinotti, M. P., Marincola, F. M., Martino, L., et al. (2000). HLA B∗5701 is highly associated with restriction of virus replication in a subgroup of HIV-infected long term nonprogressors. Proc. Natl. Acad. Sci. U.S.A. 97, 2709–2714. doi: 10.1073/pnas.050567397
Muñoz, A., Kirby, A. J., He, Y. D., Margolick, J. B., Visscher, B. R., Rinaldo, C. R., et al. (1995). Long-term survivors with HIV-1 infection: incubation period and longitudinal patterns of CD4+ lymphocytes. J. Acquir. Immune Defic. Syndr. Hum. Retrovirol. 8, 496–505. doi: 10.1097/00042560-199504120-00010
Nguyen, S., Deleage, C., Darko, S., Ransier, A., Truong, D. P., Agarwal, D., et al. (2019). Elite control of HIV is associated with distinct functional and transcriptional signatures in lymphoid tissue CD8+ T-cells. Sci. Transl. Med. 11:eaax4077. doi: 10.1126/scitranslmed.aax4077
Nishimura, Y., Gautam, R., Chun, T. W., Sadjadpour, R., Foulds, K. E., Shingai, M., et al. (2017). Early antibody therapy can induce long-lasting immunity to SHIV. Nature 543, 559–563. doi: 10.1038/nature21435
O’Brien, T. R., Blattner, W. A., Waters, D., Eyster, E., Hilgartner, M. W., Cohen, A. R., et al. (1996). Serum HIV-1 RNA levels and time to development of AIDS in the Multicenter Hemophilia Cohort Study. JAMA 276, 105–110.
O’Brien, W. A., Hartigan, P. M., Martin, D., Esinhart, J., Hill, A., Benoit, S., et al. (1996). Changes in plasma HIV-1 RNA and CD4+ lymphocyte counts and the risk of progression to AIDS. Veterans Affairs Cooperative Study Group on AIDS. N. Engl. J. Med. 334, 426–431. doi: 10.1056/NEJM199602153340703
O’Connell, K. A., Rabi, S. A., Siliciano, R. F., and Blankson, J. N. (2011). CD4+ T cells from elite suppressors are more susceptible to HIV-1 but produce fewer virions than cells from chronic progressors. Proc. Natl. Acad. Sci. U.S.A. 108, E689–E698. doi: 10.1073/pnas.1108866108
Opportunistic Infections Project Team of the Collaboration of Observational Hiv Epidemiological Research in Europe (Cohere) in EuroCoord, Young, J., Psichogiou, M., Meyer, L., Ayayi, S., Grabar, S., et al. (2012). CD4 cell count and the risk of AIDS or death in HIV-Infected adults on combination antiretroviral therapy with a suppressed viral load: a longitudinal cohort study from COHERE. PLoS Med. 9:e1001194. doi: 10.1371/journal.pmed.1001194
Parsa, N., Zaheri, P. M., Hewitt, R. G., Karimi Akhormeh, A., Taravatmanesh, S., and Wallin, L. (2020). The rapid CD4+T-lymphocyte decline and human immunodeficiency virus progression in females compared to males. Sci. Rep. 10:16816. doi: 10.1038/s41598-020-73852-0
Patrikar, S., Basannar, D. R., Bhatti, V. K., Kotwal, A., and Gupta, R. M. (2014). Rate of decline in CD4 count in HIV patients not on antiretroviral therapy. Med. J. Armed Forces India 70, 134–138. doi: 10.1016/j.mjafi.2013.08.005
Pereyra, F., Addo, M. M., Kaufmann, D. E., Liu, Y., Miura, T., Rathod, A., et al. (2008). Genetic and immunologic heterogeneity among persons who control HIV infection in the absence of therapy. J. Infect. Dis. 197, 563–571. doi: 10.1086/526786
Pernas, M., Tarancón-Diez, L., Rodríguez-Gallego, E., Gómez, J., Prado, J. G., Casado, C., et al. (2018). Factors leading to the loss of natural elite control of HIV-1 infection. J. Virol. 92:e1805-17.
Petrovas, C., Casazza, J. P., Brenchley, J. M., Price, D. A., Gostick, E., Adams, W. C., et al. (2006). PD-1 is a regulator of virus-specific CD8+ T-cell survival in HIV infection. J. Exp. Med. 203, 2281–2292. doi: 10.1084/jem.20061496
Phair, J., Jacobson, L., Detels, R., Rinaldo, C., Saah, A., Schrager, L., et al. (1992). Acquired immune deficiency syndrome occurring within 5 years of infection with human immunodeficiency virus type-1: the Multicenter AIDS Cohort Study. J. Acquir. Immune Defic. Syndr. 5, 490–496.
Pinto, L. A., Sullivan, J., Berzofsky, J. A., Clerici, M., Kessler, H. A., Landay, A. L., et al. (1995). ENV-specific cytotoxic T lymphocyte responses in HIV seronegative health care workers occupationally exposed to HIV-contaminated body fluids. J. Clin. Invest. 96, 867–876. doi: 10.1172/JCI118133
Quinn, T. C., Wawer, M. J., Sewankambo, N., Serwadda, D., Li, C., Wabwire-Mangen, F., et al. (2000). Viral load and heterosexual transmission of human immunodeficiency virus type 1. Rakai Project Study Group. N. Engl. J. Med. 342, 921–929. doi: 10.1056/NEJM200003303421303
Rabi, S. A., O’Connell, K. A., Nikolaeva, D., Bailey, J. R., Jilek, B. L., Shen, L., et al. (2011). Unstimulated primary CD4+ T-cells from HIV-1-positive elite suppressors are fully susceptible to HIV-1 entry and productive infection. J. Virol. 85, 979–986. doi: 10.1128/JVI.01721-10
Rowland-Jones, S., Sutton, J., Ariyoshi, K., Dong, T., Gotch, F., McAdam, S., et al. (1995). HIV-specific cytotoxic T-cells in HIV-exposed but uninfected Gambian women. Nat. Med. 1, 59–64. doi: 10.1038/nm0195-59
Rowland-Jones, S. L., Dong, T., Fowke, K. R., Kimani, J., Krausa, P., Newell, H., et al. (1998). Cytotoxic T-cell responses to multiple conserved HIV epitopes in HIV-resistant prostitutes in Nairobi. J. Clin. Invest. 102, 1758–1765. doi: 10.1172/JCI4314
Saeidi, A., Zandi, K., Cheok, Y. Y., Saeidi, H., Wong, W. F., Lee, C., et al. (2018). T-Cell Exhaustion in chronic infections: reversing the state of exhaustion and reinvigorating optimal protective immune responses. Front. Immunol. 9:2569. doi: 10.3389/fimmu.2018.02569
Sáez-Cirión, A., Lacabaratz, C., Lambotte, O., Versmisse, P., Urrutia, A., Boufassa, F., et al. (2007). HIV controllers exhibit potent CD8 T cell capacity to suppress HIV infection ex vivo and peculiar cytotoxic T lymphocyte activation phenotype. Proc. Natl. Acad. Sci. U.S.A. 104, 6776–6781. doi: 10.1073/pnas.0611244104
Saksela, K., Stevens, C., Rubinstein, P., and Baltimore, D. (1994). Human immunodeficiency virus type 1 mRNA expression in peripheral blood cells predicts disease progression independently of the numbers of CD4+ lymphocytes. Proc. Natl. Acad. Sci. U.S.A. 91, 1104–1108. doi: 10.1073/pnas.91.3.1104
Sauce, D., Larsen, M., Fastenackels, S., Pauchard, M., Ait-Mohand, H., Schneider, L., et al. (2011). HIV disease progression despite suppression of viral replication is associated with exhaustion of lymphopoiesis. Blood 117, 5142–5151. doi: 10.1182/blood-2011-01-331306
Schwartländer, B., Bek, B., Skarabis, H., Koch, J., Burkowitz, J., and Koch, M. A. (1993). Improvement of the predictive value of CD4+ lymphocyte count by beta 2-microglobulin, immunoglobulin A and erythrocyte sedimentation rate. The Multicentre Cohort Study Group. AIDS 7, 813–821.
Shacklett, B. L. (2006). Understanding the “lucky few”: the conundrum of HIV-exposed, seronegative individuals. Curr. HIV/AIDS Rep. 3, 26–31. doi: 10.1007/s11904-006-0005-2
Shearer, G. M. (1998). HIV-induced immunopathogenesis. Immunity 9, 587–593. doi: 10.1016/s1074-7613(00)80656-1
Sheppard, H. W., Ascher, M. S., McRae, B., Anderson, R. E., Lang, W., and Allain, J. P. (1991). The initial immune response to HIV and immune system activation determine the outcome of HIV disease. J. Acquir. Immune Defic. Syndr. 4, 704–712.
Trautmann, L., Janbazian, L., Chomont, N., Said, E. A., Gimmig, S., Bessette, B., et al. (2006). Upregulation of PD-1 expression on HIV-specific CD8+ T-cells leads to reversible immune dysfunction. Nat. Med. 12, 1198–1202. doi: 10.1038/nm1482
Wherry, E. J., Ha, S. J., Kaech, S. M., Haining, W. N., Sarkar, S., Kalia, V., et al. (2007). Molecular signature of CD8+ T cell exhaustion during chronic viral infection. Immunity 27, 670–684. doi: 10.1016/j.immuni.2007.09.006
Wherry, E. J., and Kurachi, M. (2015). Molecular and cellular insights into T cell exhaustion. Nat. Rev. Immunol. 15, 486–499. doi: 10.1038/nri3862
Wilkinson, J., Zaunders, J. J., Carr, A., and Cooper, D. A. (1999). CD8+ anti-human immunodeficiency virus suppressor activity (CASA) in response to antiretroviral therapy: loss of CASA is associated with loss of viremia. J. Infect. Dis. 180, 68–75. doi: 10.1086/314833
Yan, J., Sabbaj, S., Bansal, A., Amatya, N., Shacka, J. J., Goepfert, P. A., et al. (2013). HIV-specific CD8+ T cells from elite controllers are primed for survival. J. Virol. 87, 5170–5181. doi: 10.1128/JVI.02379-12
Keywords: HIV-1, HIV controller, Low Viral Load, CD8+ T cell, HLA compatibility, CD4:CD8 ratio
Citation: Jones AD, Khakhina S, Jaison T, Santos E, Smith S and Klase ZA (2021) CD8+ T-Cell Mediated Control of HIV-1 in a Unique Cohort With Low Viral Loads. Front. Microbiol. 12:670016. doi: 10.3389/fmicb.2021.670016
Received: 19 February 2021; Accepted: 27 April 2021;
Published: 28 May 2021.
Edited by:
Cristina Parolin, University of Padua, ItalyReviewed by:
Pradeep D. Uchil, Yale University, United StatesJohn Zaunders, St Vincent’s Hospital Sydney, Australia
Copyright © 2021 Jones, Khakhina, Jaison, Santos, Smith and Klase. This is an open-access article distributed under the terms of the Creative Commons Attribution License (CC BY). The use, distribution or reproduction in other forums is permitted, provided the original author(s) and the copyright owner(s) are credited and that the original publication in this journal is cited, in accordance with accepted academic practice. No use, distribution or reproduction is permitted which does not comply with these terms.
*Correspondence: Zachary A. Klase, ems3NkBkcmV4ZWwuZWR1
†These authors have contributed equally to this work