- 1State Key Laboratory of Infectious Disease Prevention and Control, Collaborative Innovation Center for Diagnosis and Treatment of Infectious Diseases, National Institute for Communicable Disease Control and Prevention, Chinese Center for Disease Control and Prevention, Beijing, China
- 2Department of Clinical Diagnosis, China-Japan Friendship Hospital, Beijing, China
- 3Institute of Clinical Pharmacology, Peking University First Hospital, Beijing, China
lsa(E) is a pleuromutilin, lincosamide, and streptogramin A (PLSA phenotype) resistance gene that was first described in S. aureus and was thought to have been transferred from Enterococcus sp. This study aimed to elucidate the prevalence of the lsa(E) gene among E. faecium isolates at a tertiary teaching hospital and to evaluate the transferability of the lsa(E) gene from E. faecium to S. aureus in vitro. A total of 96 E. faecium strains isolated from one hospital in Beijing in 2013 were analysed for quinupristin-dalfopristin (QDA) resistance genes, and multilocus sequence typing (MLST) was performed. The transferability of QDA resistance between ten E. faecium strains and four S. aureus strains was determined by filter mating. Genome sequencing of the transconjugant was performed. A total of 46 E. faecium isolates (46/96, 47.92%) tested positive for lsa(E), while two isolates (2/96, 2.08%) tested positive for lsa(A). Thirty-six lsa(E)-positive strains (36/46, 78.3%) belonged to ST78. Among 40 mating tests, lsa(E) was successfully transferred through one conjugation at a frequency of 1.125 × 10–7 transconjugants per donor. The QDA resistance of the transconjugant N7435-R3645 was expressed at a higher level (MIC = 16 mg/L) than that of the parent S. aureus strain (MIC = 0.38 mg/L). Next-generation sequencing (NGS) analysis of the transconjugant N7435-R3645 showed that the complete sequence of the lsa(E)-carrying plasmid pN7435-R3645 had a size of 92,396 bp and a G + C content of 33% (accession no. MT022086). The genetic map of pN7435-R3645 had high nucleotide similarity and shared the main open reading frame (ORF) features with two plasmids: E. faecium pMG1 (AB206333.1) and E. faecium LS170308 (CP025078.1). The rep gene of pN7435-R3645 showed 100% identity with that of pMG1, although it did not belong to the rep1-19 family but instead a unique rep family. Multiple antibiotic resistance genes, including lsa(E), aadE and lnu(B), erm(B), ant6-Ia, and lnu(B), were present on the plasmid. In conclusion, an lsa(E)-carrying plasmid that can be transferred by conjugation from E. faecium to S. aureus in vitro was identified. This multidrug resistance (MDR) pMG1-like plasmid may act as a vector in the dissemination of antimicrobial resistance among species.
Introduction
Enterococci and Staphylococcus aureus are well-documented opportunistic pathogens. Due to the emergence of antimicrobial resistance as a result of antibiotic overuse, a great concern is infection by methicillin-resistant Staphylococcus aureus (MRSA) and vancomycin-resistant Enterococcus species (VRE), which can lead to increased treatment failure and higher mortality rates (Blot et al., 2002; Yaw et al., 2014). Antibiotic resistance in S. aureus can emerge through point mutations or horizontal transfer of mobile genetic elements (MGEs). Genetic exchange of genes coding for antibiotic resistance between enterococci and S. aureus has been reported for genes such as the vancomycin resistance gene vanA (Weigel, 2003), the tetracycline resistance gene tetM (Leon-Sampedro et al., 2016), the trimethoprim resistance gene dfrK (Lopez et al., 2012), the multiresistance gene cfr (Liu et al., 2012) and the macrolide resistance gene erm(B) (Wan et al., 2016).
Quinupristin-dalfopristin (QDA) is a semisynthetic 70:30 mixture of streptogramin A and B and is used mainly for the treatment of glycopeptide-resistant Enterococcus faecium (GRE) and MRSA infections. The two mixture components act synergistically on the bacterial 50S ribosomal subunit, inhibiting protein synthesis. Resistance to streptogramin B does not confer resistance to QDA, while resistance to streptogramin A does (Hancock, 2005). Resistance to streptogramin A-type antibiotics can be caused by different mechanisms, such as the acetyltransferase Vat (Allignet et al., 1993), the ABC transporters Vga (Allignet et al., 1992, 1998; Kadlec and Schwarz, 2009; Schwendener and Perreten, 2011) and Lsa (Wendlandt et al., 2013b), and the methyltransferase Cfr (Long et al., 2006).
The lsa(E) gene was first described in three S. aureus strains of human origin, namely, one MRSA ST398-t011 strain and two methicillin-susceptible S. aureus (MSSA) ST9-t337 strains, and encodes an ABC transporter of unknown function (Wendlandt et al., 2013b). The lsa(E) gene was identified as a macrolide-lincosamide-streptogramin (MLS) resistance gene and was speculated to have been transferred from Enterococcus (Wendlandt et al., 2013b). The lsa(E) gene has been described not only in S. aureus but also in coagulase-negative staphylococci (CoNS) and other species, such as Erysipelothrix rhusiopathiae, Streptococcus suis, and Streptococcus agalactiae (Montilla et al., 2014; Wendlandt et al., 2015; Zhang et al., 2015; Huang et al., 2016). It is most often located in a multiresistance region in chromosomal DNA (Wendlandt et al., 2013b, 2014, 2015; Sarrou et al., 2016; Deng et al., 2017) and is sometimes detected on plasmids (Li et al., 2013; Wendlandt et al., 2013a).
We previously demonstrated that 98% (44/45) of QDA-resistant S. aureus isolates sampled from slaughter pigs in northeastern China harboured lsa(E) (Yan et al., 2014). Genome sequencing of the lsa(E)-positive strains revealed that the transposon with the lsa(E) gene cluster showed similarity to the plasmid pEF418 of E. faecalis and the plasmid pXD4 of E. faecium (Yan et al., 2016). However, limited information is known about the presence of the lsa(E) gene in E. faecium strains isolated from inpatients in China and the transferability of the lsa(E) gene between E. faecium and S. aureus.
The objective of this study was to elucidate the prevalence of the lsa(E) gene among E. faecium strains isolated at a tertiary teaching hospital and to evaluate the transferability of the lsa(E) gene from E. faecium to S. aureus in vitro.
Materials and Methods
Bacterial Isolates
A total of 96 E. faecium strains isolated from one hospital in Beijing in 2013 were analysed in the present study (Supplementary Table 1). The isolates were identified as E. faecium using a Vitek-2 microbiology analyser (bioMérieux, Marcy l’Etoile, France).
Antimicrobial Susceptibility Testing and QDA Resistance Gene Detection
The susceptibility to 13 antimicrobial agents—ampicillin, penicillin, erythromycin, ciprofloxacin, levofloxacin, nitrofurantoin, tetracycline, vancomycin, linezolid, quinupristin/dalfopristin, tigecycline, high-level gentamicin and streptomycin—was tested with a Vitek-2 microbiology analyser according to the manufacturer’s instructions. QDA resistance was reconfirmed by Etest (bioMérieux SA, Marcy l’Etoile, France). The minimum inhibitory concentrations (MICs) for all the antimicrobials were interpreted using Clinical and Laboratory Standards Institute (CLSI) criteria [Clinical and Laboratory Standards Institute (CLSI), 2021].
All the isolates were investigated for the QDA resistance genes lsa(A), lsa(C), lsa(E), vatD, vatE, vatH, and vgaD by PCR, and eat(A) mutations, which are designated eat(A)v, were checked by sequencing (Supplementary Table 2).
Multilocus Sequence Typing (MLST)
MLST of E. faecium isolates was performed by amplifying seven housekeeping genes—adk, atpA, ddl, gyd, gdh, purK and pstS—as described previously (Homan et al., 2002). The sequences were submitted to the MLST website for E. faecium1, and sequence types (STs) were assigned according to the allelic profiles. The clonal complex (CC) was analysed with goeBURST v1.2.1.
Mating Experiments
The transferability of QDA resistance was determined by performing filter mating. Ten rifampin-susceptible E. faecium strains (9200, P9772, 5118, 6354, 6474, 3240, 4103, N7435, P2505 and P3814) harbouring lsa(E) were randomly selected as donors for the mating experiments. The recipients were four clinical lsa(E)-negative, rifampin- and methicillin-resistant S. aureus isolates (109, R3645, R3680, and 121) that were plasmid-free after plasmid extraction (Table 1). A donor:recipient ratio of 1:9 was used for the mating experiments (Tomita et al., 2002). Selection was performed on brain-heart infusion agar (BHI, OXOID LTD., Basingstoke, Hampshire, England) supplemented with 4 or 8 mg/L virginiamycin and 128 mg/L rifampicin. Rifampicin- and virginiamycin-resistant colonies of putative S. aureus transconjugants were isolated and identified by lsa(E) PCR. QDA was determined by Etest for the lsa(E)-positive transconjugant. The microdilution broth method was used to determine the MICs of 18 antimicrobial agents, namely, penicillin, cefoxitin, tetracycline, chloramphenicol, ciprofloxacin, gentamicin, rifampicin, vancomycin, nitrofurantoin, trimethoprim-sulphamethoxazole, erythromycin, teicoplanin, clindamycin, linezolid, tigecycline, mupirocin, fusidic acid, and daptomycin. The transfer frequency was expressed as the number of transconjugants per donor.
SmaI- and S1 Nuclease (S1)-Pulsed-Field Gel Electrophoresis (PFGE), Southern Blotting and Hybridisation Assays
Transconjugants were further confirmed by Southern blotting. SmaI- and S1-PFGE analyses were performed as described previously (Tomita et al., 2002; Yan et al., 2011). Southern blotting was performed using a DIG High Prime DNA labelling and Detection Starter Kit (Roche, Basel, Switzerland) according to the manufacturer’s instructions. The digoxigenin-labelled lsa(E)-specific probe was prepared using primers (forward 5′-ACAGCGAGTTGTTTCCTGCT-3′; and reverse 5′-GCACGTTTCATCGCTTTTGC-3′) that amplified a 410-bp region of the lsa(E) gene. After S1-PFGE, the DNA was transferred to a nylon membrane (Hybond N, Amersham, United Kingdom) that was hybridised with the prepared lsa(E)-specific probe. Detection was performed using an NBT/BCIP colour detection kit (Roche, Switzerland).
Transconjugant Stability
The stability of the lsa(E)-carrying transconjugants was evaluated by daily serial passage on antibiotic-free blood agar. Colonies were tested daily for lsa(E) by PCR. The stability of the lsa(E)-carrying plasmid was also evaluated by growing on virginiamycin (4 and 8 mg/L) MH agar after storage at 4 and −80°C for 4 weeks.
Plasmid Sequencing, Assembly and Annotation
The transconjugant N7435-R3645 genome (named with donor and recipient strains) was extracted using a commercial kit (Promega, Madison, United States). Genome sequencing was performed by using the Illumina HiSeq 4000 platform and PacBio RS II platform (10 kb insert library; Pacific Biosciences, Menlo Park, CA, United States) at the Beijing Genomics Institute (BGI, Shenzhen, China).
De novo assemblies and contig assembly for the plasmid pN7435-R3645 of transconjugant N7435-R3645 were performed using Soapdenovo 2.0. Open reading frames (ORFs) were predicted with GeneMarkS.2 The overlapping regions were found by BLASTing the sequences of the beginning and the end of the final contig. The closed plasmid was confirmed by PCR (JH-F 5′-CTCTACCAGATGGTTGGAGCA-3′; JH-R 5′-CCTACGATCACGGCACCAAT-3′) and Sanger sequencing of the resulting amplicons. The plasmid nucleotide sequences were compared with sequences in the GenBank database using BLASTN.3
Nucleotide Sequence Accession Number
The sequence of the conjugated plasmid pN7435-R3645 was deposited in the DDBJ/EMBL/GenBank nucleotide sequence databases under accession number MT022086.
Results
Antimicrobial Susceptibility
For the E. faecium isolates, the resistance rates to ampicillin, penicillin, erythromycin, ciprofloxacin, levofloxacin, nitrofurantoin, gentamicin, streptomycin, tetracycline and vancomycin were 91.67, 92.71, 94.79, 92.71, 92.71, 75.00, 66.67 56.25, 31.25 and 20.83%, respectively. A low resistance rate was observed for linezolid (1.04%) and tigecycline (1.04%).
Antimicrobial Resistance Genotype and Phenotype of QDA Resistance in E. faecium Strains
A total of 46 E. faecium isolates (46/96; 47.92%) tested positive for lsa(E), while two isolates (2/96; 2.08%) tested positive for lsa(A). The eat(A)v mutation (C1349T) was found in 41 of 96 E. faecium isolates. The vatD, vatE, vatH, vgaD and lsa(C) genes were not detected in any of the isolates. Four antibiotic resistance gene profiles were observed, namely, lsa(E) (n = 27), eat(A)v (n = 22), lsa(E)-lsa(A)-eat(A)v (n = 2), and lsa(E)-eat(A)v (n = 17) (Table 2).
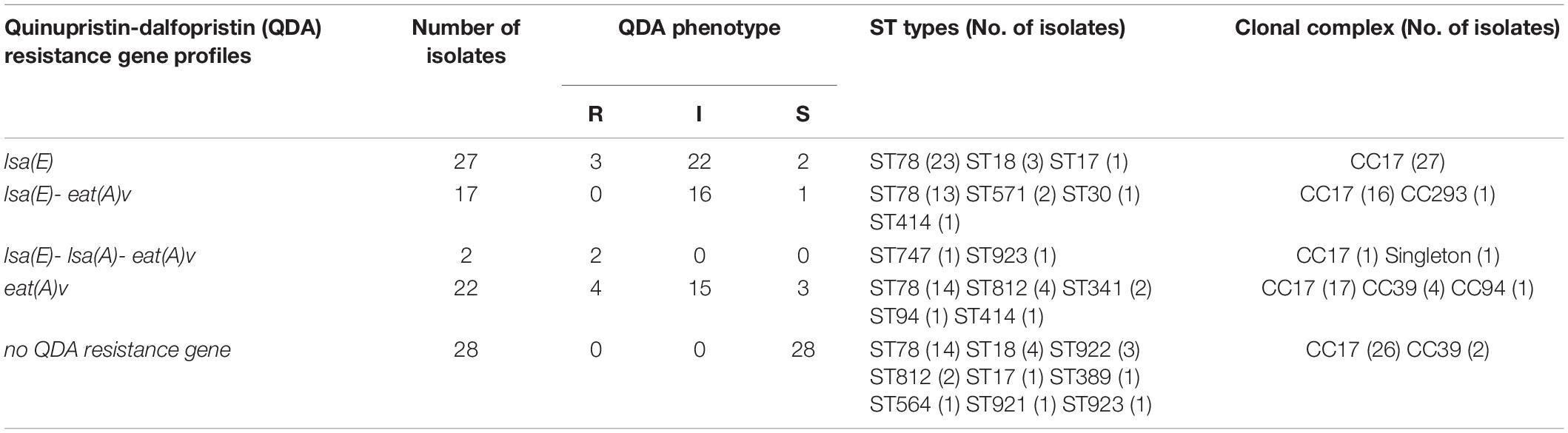
Table 2. Quinupristin-dalfopristin (QDA) resistance gene profiles and ST types in E. faecium strains isolated from patients.
QDA resistance was observed in 9 isolates (9.37%; 9/96), while 53 isolates (55.2%; 53/96) showed intermediate susceptibility. The majority of the lsa(E)-carrying strains (43/46; 93.48%) showed QDA resistance or an intermediate susceptible phenotype. Among the lsa(E)-positive strains, two strains carrying lsa(E)-lsa(A)-eat(A)v showed high QDA MIC values of 24 and 6 mg/L.
Molecular Characterisation of E. faecium Isolates
MLST for all the isolates revealed fifteen ST types that belonged to four clonal complexes and one singleton (Table 2). ST78 (CC17) was the most frequent ST type and was identified in 64 of 96 isolates (64/96, 66.7%), followed by ST18 (CC17) (7/96, 7.3%) and ST812 (CC39) (6/96, 6.3%). Moreover, forty-four lsa(E)-positive strains (44/46, 95.6%) belonged to CC17.
Conjugative Transfer of lsa(E) From E. faecium to S. aureus
Among the 40 mating tests performed, QDA resistance was successfully transferred in one conjugation at a frequency of 1.125 × 10–7 transconjugants per donor. Transfer occurred from E. faecium N7435 to S. aureus R3645. The match of the conjugated N7435-R3645 with the recipient was confirmed by comparing their SmaI-PFGE profiles (Supplementary Figure 1). One extra ∼100 kb band was observed in the N7435-R3645 SmaI-PFGE profile. The QDA resistance of conjugated N7435-R3645 was expressed at a higher level (MIC = 16 mg/L) than that of the parent S. aureus strain (MIC = 0.38 mg/L) (Figure 1). The erythromycin resistance of conjugated N7435-R3645 was also expressed at a higher level (MIC > 2048 mg/L) than that of the parent S. aureus strain (MIC = 512 mg/L). There was no difference in the MIC values of the other 17 antibiotics.
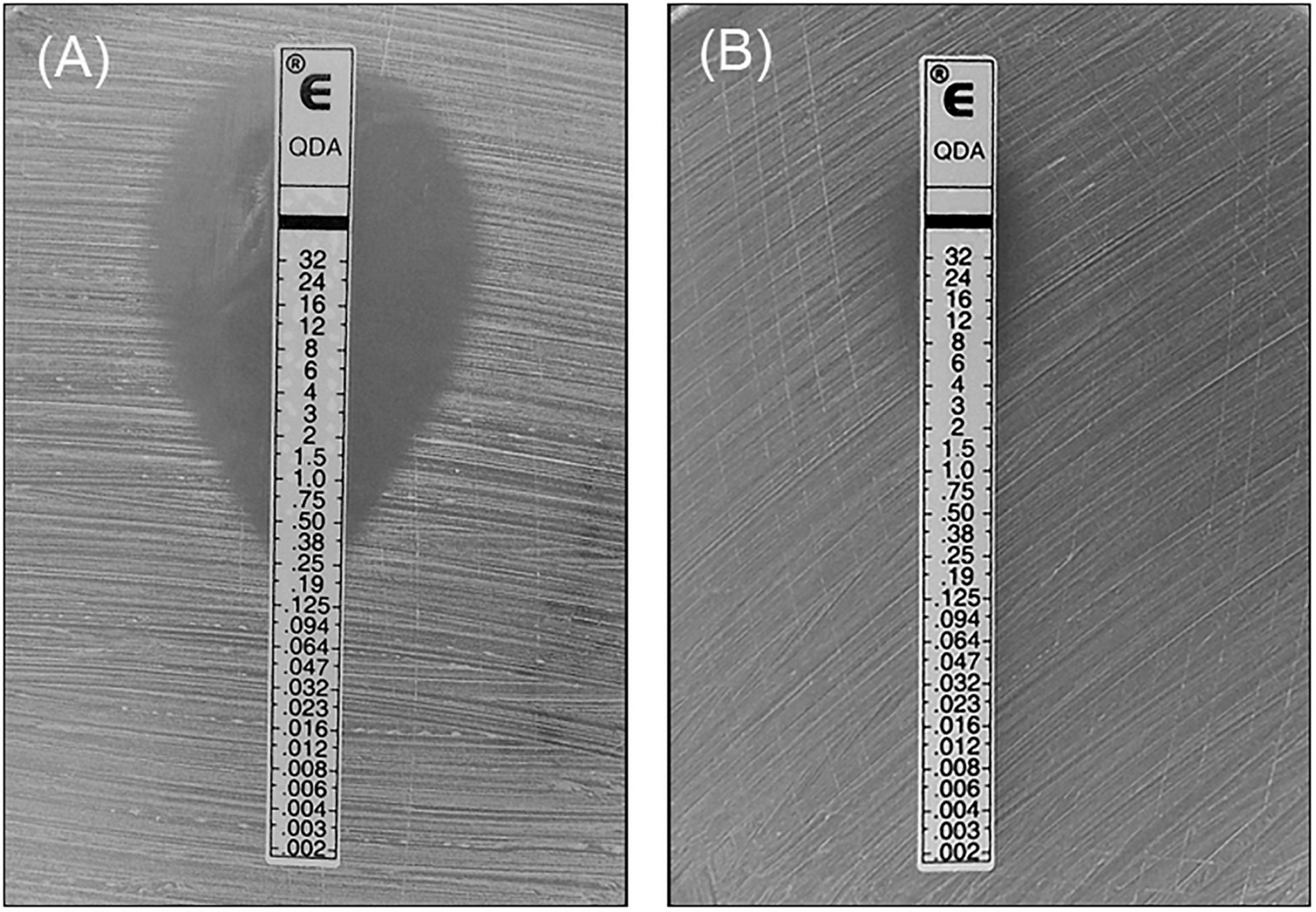
Figure 1. The MIC value of QDA against the recipient strain and the transconjugant strain. (A) Recipient strain, R3645; (B) Transconjugant strain, N7435/R3645.
Location and Stability of the lsa(E) Gene in the Transconjugant
The location of the lsa(E) gene in the transconjugant N7435-R3645 was investigated by S1-PFGE followed by Southern blotting (Figure 2). S1-PFGE revealed that recipient R3645 did not harbour plasmids, while transconjugant N7435-R3645 carried a single ∼100 kb plasmid. Hybridisation assays showed that lsa(E) was located on the ∼100 kb plasmid in the transconjugant N7435-R3645.
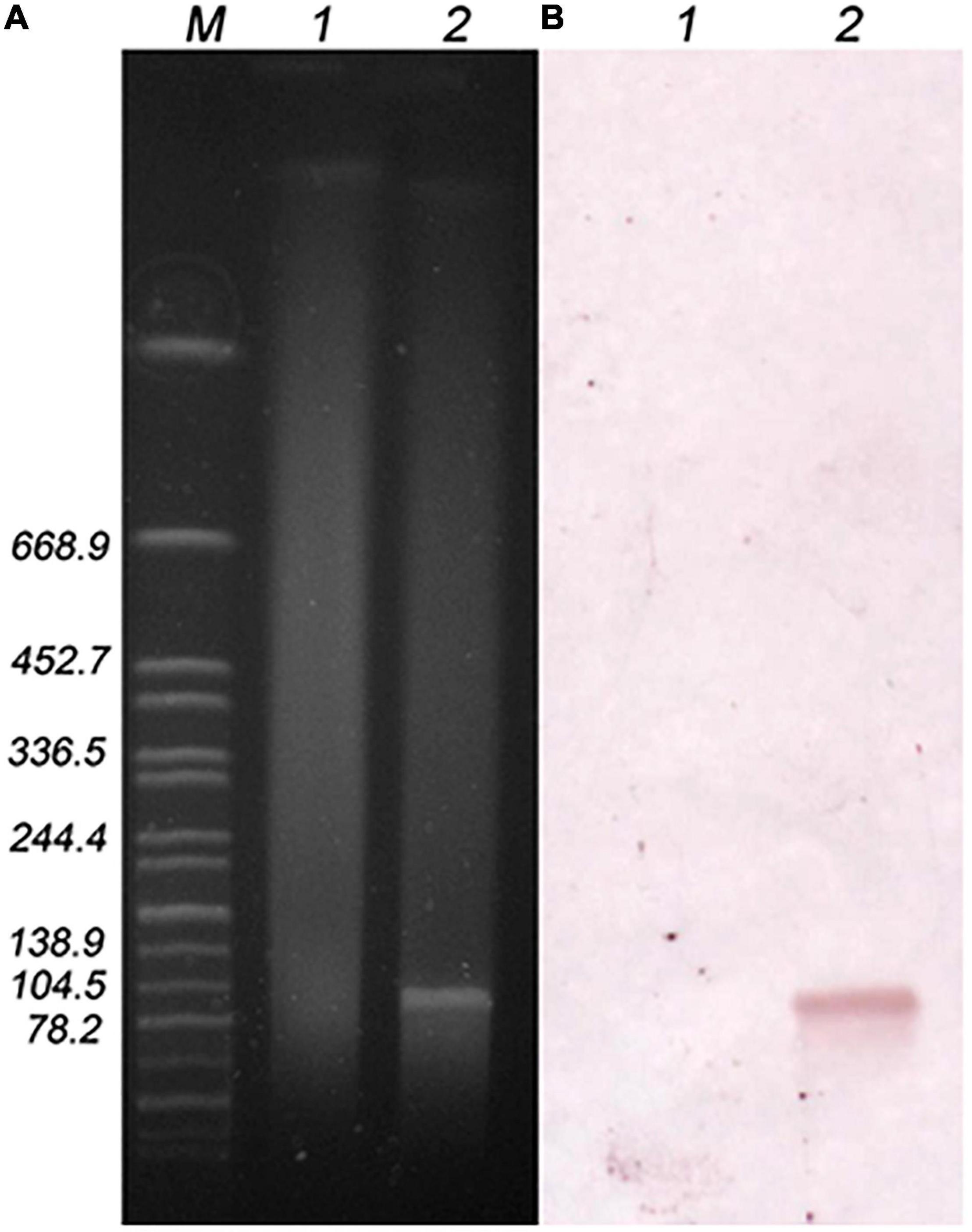
Figure 2. S1-PFGE plasmid profile of the recipient and transconjugant strains (A) and hybridisation with the lsa(E) probe (B). M, Global reference strain H9812 of the Salmonella Braenderup serotype digested with the XbaI enzyme; lane 1, recipient strain (R3645); lane 2, transconjugant strain (N7435/R3645).
lsa(E) was stable after ten overnight passages on antibiotic-free blood agar. The transconjugant continued to grow on MH agar supplemented with virginiamycin (4 and 8 mg/L) even after storage at 4 and −80°C for 4 weeks.
Characteristics of the Transconjugated Plasmid
Next-generation sequencing (NGS) analysis of the pN7435-R3645 transconjugant showed that the complete sequence of the lsa(E)-carrying plasmid pN7435-R3645 was 92,396 bp in size and had a G + C content of 33% (accession no. MT022086). Sequence analysis identified 119 ORFs. The genetic map of pN7435-R3645 is shown in Figure 3 along with the maps of elements showing high nucleotide similarity and the main ORF features with two plasmids, E. faecium pMG1 (AB206333.1) and E. faecium LS170308 plasmid (CP025078.1).
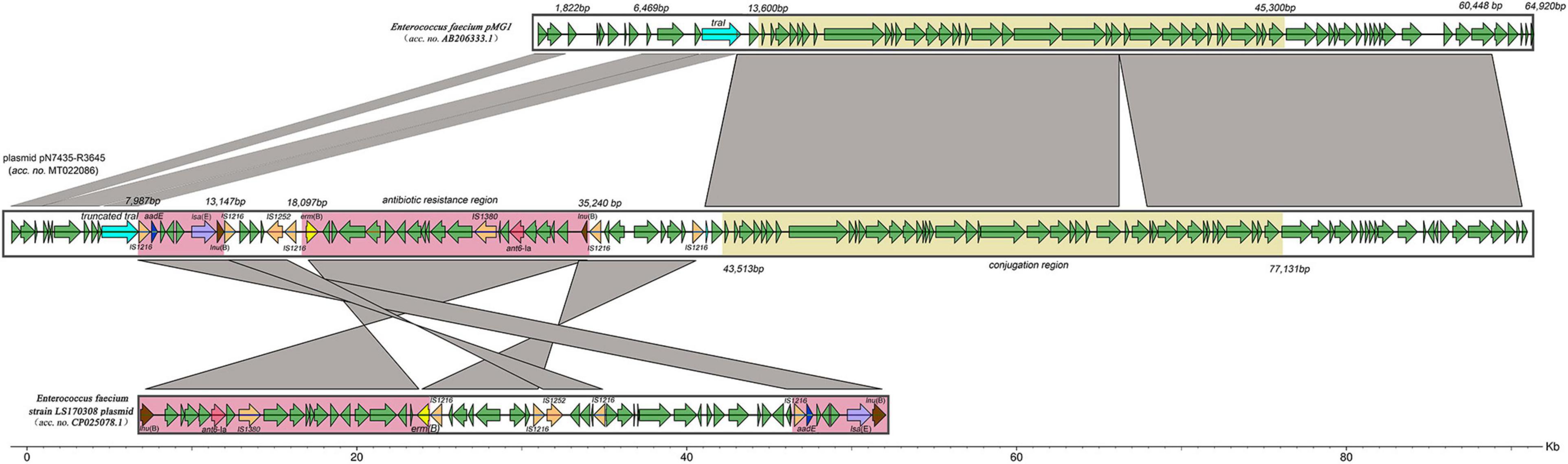
Figure 3. Structure of the lsa(E)-carrying plasmid pN7435-R3645 and similar regions in plasmid pMG1 and plasmid LS170308 of E. faecium. The structure of the plasmid pN7435-R3645 is illustrated based on the nucleotide sequences deposited in the GenBank database (MT022086). ORFs are indicated by arrows coloured as follows: orange, insertion sequences; purple, lsa(E); blue, aadE; pink, ant6-Ia; brown, lnu(B); yellow, erm(B); light blue, traI or truncated traI; and green, other genes. The similarities in the structures are indicated by grey shading. Antibiotic resistance regions and conjugation regions are shown in pink and yellow, respectively.
pN7435-R3645 is highly equivalent to the 1–60,447 bp region of E. faecium pMG1, and this region of similarity is divided into two parts by the insertion of the E. faecium LS170308 plasmid. The insertion site was in the ORF region of the TraI topoisomerase-encoding gene. The pN7435-R3645 plasmid retained most of the genes from the E. faecium pMG1 plasmid and lost mainly the 1,822–6,469 bp and 60,448–64,920 bp regions, corresponding to the aminoglycoside resistance gene (aac/aph) and insertion sequence (IS) elements, respectively.
The conjugation region (43,513–77,131 bp; G + C content, 32.07%) of the pN7435-R3645 plasmid is approximately 33.6 kb. This region showed 99% identity with the conjugation region of pMG1 (13,600–45,300 bp). The rep gene of pN7435-R3645 showed 100% identity with that of pMG1, which did not belong to the rep1-19 family but belonged to a unique rep family. The IS elements in the pN7435-R3645 plasmid involved in possible recombination processes included mainly the IS1216 transposase.
The resistance genes on the pN7435-R3645 plasmid were located mainly in the region of similarity with the E. faecium LS170308 plasmid. In addition, the plasmid structure was rearranged in these regions. Different AR elements were found in the following two pN7435-R3645 regions:
lsa(E) region (7,987–13,147 bp; G + C content, 35.69%). This region included three AR genes, lsa(E), aadE and lnu(B), which confer resistance to lincosamides/streptogramin A/pleuromutilins, aminoglycosides, and lincosamide, respectively. This region exhibited more than 99% nucleotide identity with multiple plasmids of E. faecium (plasmids of E. faecium strain LS170308, pEF37BA, pXD5, pY13, etc.), E. faecalis (pEF418, pE15, p11-27, etc.), E. gallinarum (pY15), and S. aureus (pV7037) as well as the chromosome region of Streptococcus agalactiae. This region was flanked by two identical IS1216 transposase genes with the same orientation.
erm(B), ant6-Ia, and lnu(B) regions (18,097–35,240 bp; G + C content, 35.72%). This segment contained three AR genes, erm(B), ant6-Ia, and lnu(B), which confer resistance to macrolides, aminoglycosides and lincosamide, respectively. This region exhibited 99% nucleotide identity with part of the E. faecium strain LS170308 plasmid and was flanked by two identical IS1216 transposase genes.
Discussion
Plasmids harbour a number of antibiotic genes and are widely found in enterococci, mainly E. faecalis and E. faecium, which are currently leading causes of multiresistant hospital-acquired infections. Conjugation is a primary means of intercellular DNA transfer in enterococci. Moreover, enterococci are reservoirs for antibiotic resistance genes, which can spread to other important pathogens, most notably S. aureus.
The present study provided the first evidence of the ability of the lsa(E) gene to undergo plasmid-mediated transfer and of the ability of an E. faecium plasmid carrying a lsa(E) gene to replicate in a clinical MRSA strain. To date, only the van(A) gene has been shown to be transferred from E. faecalis to S. aureus by conjugation in vitro (de Niederhausern et al., 2011). Peptide sex pheromones secreted by S. aureus induce conjugation-related mating functions and may play an important role in Tn1546-containing pheromone-responding plasmid transfer in E. faecalis (Showsh et al., 2001). To our knowledge, lsa (E) is the first gene that has been confirmed to be transferred from E. faecium to S. aureus in vitro and probably has a different transfer mechanism than the van(A) gene. Plasmid pN7435-R3645 in this study retained most of the genes in the E. faecium pMG1 plasmid. pMG1, which has been completely sequenced, is a 65 kb conjugative plasmid from E. faecium containing a Tn4001-like element and is a non-pheromone-responding plasmid (Ike et al., 1998; Tanimoto and Ike, 2008). It can transfer relatively well to other E. faecium strains in broth as well as to E. faecalis and E. hirae. pMG1 family elements have significantly contributed to the spread of vancomycin and gentamicin resistance among enterococci, particularly within E. faecium (Tomita et al., 2003). Although insertion of the plasmid pLS170308 region resulted in partial deletion of the TraI topoisomerase-encoding gene, pN7435-R3645 still retained the complete conjugation region of pMG1 (13,600–45,300 bp) (Tanimoto and Ike, 2008). Therefore, we speculated that the horizontal transfer of lsa(E) between E. faecium and S. aureus was dependent mainly on a non-pheromone-responding pMG1-like plasmid through conjugation.
Another lsa(E)-carrying non-conjugative plasmid, pY13 (28,489 bp), from a porcine linezolid-resistant E. faecium isolate has been reported (Si et al., 2015). The conjugative plasmid pN7435-R3645, approximately 92,396 bp, in this study is much larger than pY13 and has a structure different from that of pY13. Rearrangement and inversion regions were observed on the plasmid pN7435-R3645. Since all of these segments were flanked by ISs, pN7435-R3645 may have derived from interplasmidic recombination events in which ISs, such as IS1216 and IS1252, were involved.
In the present study, four clinical ST239 MRSA strains were selected as recipients, and only one strain (R3645) was successfully transferred. Mutations in genes of the SauI type I restriction-modification (RM) system and deficiency in the type IV RM system have been shown to increase a strain’s ability to accept foreign DNA (Waldron and Lindsay, 2006; Corvaglia et al., 2010). Intact type I and IV RM systems were found in R3645 (data not shown). Other characteristics, such as mutations of CRISPR loci, that may contribute to the ability to acquire lsa(E)-carrying non-conjugative plasmids need to be further investigated.
E. faecalis is intrinsically resistant to QDA as a result of the presence of the lsa determinant, while E. faecium always acquires QDA resistance (Singh et al., 2002). To date, the prevalence of QDA resistance among E. faecium clinical isolates in many countries has been low, but relatively high resistance rates have occasionally been reported, such as 6.7% (9/135) in Poland (Sadowy et al., 2013), 10% (25/249) in Korea (Oh et al., 2005), and 60% in northwest Iran (6/10) (Haghi et al., 2019). The rate of intermediate resistance to QDA is relatively high in some countries, such as 17.6% (28/159) in Japan (Isogai et al., 2013), 26.7% (36/135) in Poland (Sadowy et al., 2013) and 28.9% (250/865) in Greece (Karanika et al., 2008). An investigation in a Chinese hospital in Wenzhou reported that 9 of 911 (1.0%) E. faecium isolates were resistant to QDA (Wang et al., 2016). In this study, QDA resistance was observed in 9 isolates (9.37%; 9/96), while 53 isolates (55.2%; 53/96) showed intermediate susceptibility. This finding indicated that QDA resistance differed among hospitals and regions in China. Although QDA has not been marketed in China, virginiamycin, which belongs to the same antibiotic class as QDA, has been widely used as an animal growth promoter in poultry, cattle and swine. The resistance of E. faecium strains isolated from animals to QDA ranged from 2.2 to 33.6%, and 38.5–83.2% of the strains were classified as not sensitive in European countries from 2004 to 2014 (Wang et al., 2016; de Jong et al., 2019). However, virginiamycin has been banned for use as a growth promoter in Europe since 1999. This may be explained by the possible co-selection of resistance genes by compounds currently approved to treat clinical diseases.
A high prevalence of lsa(E) (47.92%, 46/96) was found among clinical E. faecium isolates, and the majority of lsa(E)-carrying strains (43/46; 93.48%) showed QDA resistance or an intermediate susceptible phenotype in this study. Acetyltransferases encoded by vatD and vatE have been found in enterococci from various sources, including humans, animals and the environment in Europe, the United States and Asia (Soltani et al., 2000; Werner et al., 2000; Jackson et al., 2007; Hwang et al., 2010); however, the vatD and vatE genes were not detected in this study. To date, only two papers have reported the distribution of lsa(E) in enterococcus, and both papers are from China. The lsa(E) gene was found in 30.3% (10/33) of human enterococcal strains and 53.6% (37/69) of swine enterococcal strains in Henan Province, China. Most of them were clonally unrelated, with the exception of E. faecium ST29 (n = 4) and ST362 (n = 4) (Li et al., 2014). The lsa(E) gene was also detected in five E. faecalis strains, one E. faecium strain and one E. gallinarum strain among thirty-five enterococcal strains isolated from a pig farm in Guangxi Province, China (Si et al., 2015). In this study, thirty-six lsa(E)-positive strains (36/46, 78.3%) belonged to ST78, which is an epidemic clone in hospitals in China (Sun et al., 2019). This result suggested that the high lsa(E) detection rate in clinical strains may be due to the spread of E. faecium-resistant clones.
In conclusion, a high prevalence of lsa(E) was found in clinical E. faecium strains. An lsa(E)-carrying plasmid that can be transferred from E. faecium to S. aureus in vitro by conjugation was identified. This MDR pMG1-like plasmid may act as a vector in the dissemination of antimicrobial resistance among species.
Data Availability Statement
The datasets presented in this study can be found in online repositories. The names of the repository/repositories and accession number(s) can be found below: https://www.ncbi.nlm.nih.gov/genbank/, MT022086.
Author Contributions
X-MY, JW, and J-ZZ conceived the study. X-MY wrote the manuscript and performed the Southern blotting experiment. JW, H-BJ, HY, and Y-HY collected the strains and performed the antibiotic resistance experiments. X-XT, F-LM, BZ, and YH carried out the molecular typing, mating, and QDA resistance gene detection. X-MY and Y-HY analysed the genome sequencing data. J-ZZ revised the manuscript. All authors read and approved the final manuscript.
Funding
This work was supported by the National Key R&D Programme of China (2018YFC1603800) and the National Natural Science Foundation of China (Grant No. 81873959).
Conflict of Interest
The authors declare that the research was conducted in the absence of any commercial or financial relationships that could be construed as a potential conflict of interest.
Supplementary Material
The Supplementary Material for this article can be found online at: https://www.frontiersin.org/articles/10.3389/fmicb.2021.667415/full#supplementary-material
Footnotes
- ^ https://pubmlst.org/bigsdb?db=pubmlst_efaecium_seqdef
- ^ http://topaz.gatech.edu/
- ^ http://blast.ncbi.nlm.nih.gov/blast
References
Allignet, J., Liassine, N., and El, S. N. (1998). Characterization of a staphylococcal plasmid related to pUB110 and carrying two novel genes, vatC and vgbB, encoding resistance to streptogramins A and B and similar antibiotics. Antimicrob. Agents. Chemother. 42, 1794–1798. doi: 10.1128/AAC.42.7.1794
Allignet, J., Loncle, V., and El, S. N. (1992). Sequence of a staphylococcal plasmid gene, vga, encoding a putative ATP-binding protein involved in resistance to virginiamycin A-like antibiotics. Gene 117, 45–51. doi: 10.1016/0378-1119(92)90488-b
Allignet, J., Loncle, V., Simenel, C., Delepierre, M., and El, S. N. (1993). Sequence of a staphylococcal gene, vat, encoding an acetyltransferase inactivating the A-type compounds of virginiamycin-like antibiotics. Gene 130, 91–98. doi: 10.1016/0378-1119(93)90350-c
Blot, S. I., Vandewoude, K. H., Hoste, E. A., and Colardyn, F. A. (2002). Outcome and attributable mortality in critically Ill patients with bacteremia involving methicillin-susceptible and methicillin-resistant Staphylococcus aureus. Arch. Intern. Med. 162, 2229–2235. doi: 10.1001/archinte.162.19.2229
Clinical and Laboratory Standards Institute (CLSI). (2021). Performance Standards for Antimicrobial Susceptibility Testing, 31st Edn, CLSI supplement M100 (ISBN 978-1-68440-104-8 [Print]; ISBN 978-1-68440-105-5 [Electronic]). Wayne, PA: Clinical and Laboratory Standards Institute.
Corvaglia, A. R., Francois, P., Hernandez, D., Perron, K., Linder, P., and Schrenzel, J. (2010). A type III-like restriction endonuclease functions as a major barrier to horizontal gene transfer in clinical Staphylococcus aureus strains. Proc. Natl. Acad. Sci. U.S.A. 107, 11954–11958. doi: 10.1073/pnas.1000489107
de Jong, A., Simjee, S., Rose, M., Moyaert, H., El, G. F., and Youala, M. (2019). Antimicrobial resistance monitoring in commensal enterococci from healthy cattle, pigs and chickens across Europe during 2004-14 (EASSA Study). J. Antimicrob. Chemother. 74, 921–930. doi: 10.1093/jac/dky537
de Niederhausern, S., Bondi, M., Messi, P., Iseppi, R., Sabia, C., Manicardi, G., et al. (2011). Vancomycin-resistance transferability from VanA enterococci to Staphylococcus aureus. Curr. Microbiol. 62, 1363–1367. doi: 10.1007/s00284-011-9868-6
Deng, F., Wang, H., Liao, Y., Li, J., Fessler, A. T., Michael, G. B., et al. (2017). Detection and genetic environment of pleuromutilin-lincosamide-streptogramin a resistance genes in staphylococci isolated from Pets. Front. Microbiol. 8:234. doi: 10.3389/fmicb.2017.00234
Haghi, F., Lohrasbi, V., and Zeighami, H. (2019). High incidence of virulence determinants, aminoglycoside and vancomycin resistance in enterococci isolated from hospitalized patients in Northwest Iran. BMC Infect. Dis. 19:744. doi: 10.1186/s12879-019-4395-3
Hancock, R. E. (2005). Mechanisms of action of newer antibiotics for Gram-positive pathogens. Lancet. Infect. Dis. 5, 209–218. doi: 10.1016/S1473-3099(05)70051-7
Homan, W. L., Tribe, D., Poznanski, S., Li, M., Hogg, G., Spalburg, E., et al. (2002). Multilocus sequence typing scheme for Enterococcus faecium. J. Clin. Microbiol. 40, 1963–1971. doi: 10.1128/jcm.40.6.1963-1971.2002
Huang, K., Zhang, Q., Song, Y., Zhang, Z., Zhang, A., Xiao, J., et al. (2016). Characterization of spectinomycin resistance in Streptococcus suis leads to two novel insights into drug resistance formation and dissemination mechanism. Antimicrob. Agents. Chemother. 60, 6390–6392. doi: 10.1128/AAC.01157-16
Hwang, I. Y., Ku, H. O., Lim, S. K., Lee, K. J., Park, C. K., Jung, G. S., et al. (2010). Distribution of streptogramin resistance genes and genetic relatedness among quinupristin/dalfopristin-resistant Enterococcus faecium recovered from pigs and chickens in Korea. Res. Vet. Sci. 89, 1–4. doi: 10.1016/j.rvsc.2010.01.011
Ike, Y., Tanimoto, K., Tomita, H., Takeuchi, K., and Fujimoto, S. (1998). Efficient transfer of the pheromone-independent Enterococcus faecium plasmid pMG1 (Gmr) (65.1 kilobases) to Enterococcus strains during broth mating. J. Bacteriol. 180, 4886–4892. doi: 10.1128/JB.180.18.4886-4892.1998
Isogai, N., Urushibara, N., Kawaguchiya, M., Ghosh, S., Suzaki, K., Watanabe, N., et al. (2013). Characterization of Enterococcus faecium with macrolide resistance and reduced susceptibility to quinupristin/dalfopristin in a Japanese hospital: detection of extensive diversity in erm(B)-regulator regions. Microb. Drug. Resist. 19, 298–307. doi: 10.1089/mdr.2012.0176
Jackson, C. R., Fedorka-Cray, P. J., Barrett, J. B., Hiott, L. M., and Woodley, T. A. (2007). Prevalence of streptogramin resistance in enterococci from animals: identification of vatD from animal sources in the USA. Int. J. Antimicrob. Agents. 30, 60–66. doi: 10.1016/j.ijantimicag.2007.03.010
Kadlec, K., and Schwarz, S. (2009). Novel ABC transporter gene, vga(C), located on a multiresistance plasmid from a porcine methicillin-resistant Staphylococcus aureus ST398 strain. Antimicrob. Agents. Chemother. 53, 3589–3591. doi: 10.1128/AAC.00570-09
Karanika, M., Prati, A., Kiritsi, M., Spiliopoulou, I., Neonakis, I., Anifantaki, M., et al. (2008). Reduced susceptibility to quinupristin/dalfopristin in Enterococcus faecium in Greece without prior exposure to the agent. Int. J. Antimicrob. Agents. 31, 55–57. doi: 10.1016/j.ijantimicag.2007.08.006
Leon-Sampedro, R., Novais, C., Peixe, L., Baquero, F., and Coque, T. M. (2016). Diversity and evolution of the Tn5801-tet(M)-Like integrative and conjugative elements among enterococcus, streptococcus, and staphylococcus. Antimicrob. Agents. Chemother. 60, 1736–1746. doi: 10.1128/AAC.01864-15
Li, B., Wendlandt, S., Yao, J., Liu, Y., Zhang, Q., Shi, Z., et al. (2013). Detection and new genetic environment of the pleuromutilin-lincosamide-streptogramin A resistance gene lsa(E) in methicillin-resistant Staphylococcus aureus of swine origin. J. Antimicrob. Chemother. 68, 1251–1255. doi: 10.1093/jac/dkt015
Li, X. S., Dong, W. C., Wang, X. M., Hu, G. Z., Wang, Y. B., Cai, B. Y., et al. (2014). Presence and genetic environment of pleuromutilin-lincosamide-streptogramin A resistance gene lsa(E) in enterococci of human and swine origin. J. Antimicrob. Chemother. 69, 1424–1426. doi: 10.1093/jac/dkt502
Liu, Y., Wang, Y., Wu, C., Shen, Z., Schwarz, S., Du, X., et al. (2012). First report of the multidrug resistance gene cfr in Enterococcus faecalis of animal origin. Antimicrob. Agents. Chemother. 56, 1650–1654. doi: 10.1128/AAC.06091-11
Long, K. S., Poehlsgaard, J., Kehrenberg, C., Schwarz, S., and Vester, B. (2006). The Cfr rRNA methyltransferase confers resistance to phenicols, lincosamides, oxazolidinones, pleuromutilins, and streptogramin a antibiotics. Antimicrob. Agents. Chemother. 50, 2500–2505. doi: 10.1128/AAC.00131-06
Lopez, M., Kadlec, K., Schwarz, S., and Torres, C. (2012). First detection of the staphylococcal trimethoprim resistance gene dfrK and the dfrK-carrying transposon Tn559 in enterococci. Microb. Drug. Resist. 18, 13–18. doi: 10.1089/mdr.2011.0073
Montilla, A., Zavala, A., Caceres, C. R., Cittadini, R., Vay, C., Gutkind, G., et al. (2014). Genetic environment of the lnu(B) gene in a Streptococcus agalactiae clinical isolate. Antimicrob. Agents. Chemother. 58, 5636–5637. doi: 10.1128/AAC.02630-14
Oh, W. S., Ko, K. S., Song, J. H., Lee, M. Y., Park, S., Peck, K. R., et al. (2005). High rate of resistance to quinupristin-dalfopristin in Enterococcus faecium clinical isolates from Korea. Antimicrob. Agents. Chemother. 49, 5176–5178. doi: 10.1128/AAC.49.12.5176-5178.2005
Sadowy, E., Sienko, A., Gawryszewska, I., Bojarska, A., Malinowska, K., and Hryniewicz, W. (2013). High abundance and diversity of antimicrobial resistance determinants among early vancomycin-resistant Enterococcus faecium in Poland. Eur. J. Clin. Microbiol. Infect. Dis. 32, 1193–1203. doi: 10.1007/s10096-013-1868-y
Sarrou, S., Liakopoulos, A., Tsoumani, K., Sagri, E., Mathiopoulos, K. D., Tzouvelekis, L. S., et al. (2016). Characterization of a novel lsa(E)- and lnu(B)-carrying structure located in the chromosome of a Staphylococcus aureus sequence Type 398 strain. Antimicrob. Agents. Chemother. 60, 1164–1166. doi: 10.1128/AAC.01178-15
Schwendener, S., and Perreten, V. (2011). New transposon Tn6133 in methicillin-resistant Staphylococcus aureus ST398 contains vga(E), a novel streptogramin A, pleuromutilin, and lincosamide resistance gene. Antimicrob. Agents. Chemother. 55, 4900–4904. doi: 10.1128/AAC.00528-11
Showsh, S. A., De Boever, E. H., and Clewell, D. B. (2001). Vancomycin resistance plasmid in Enterococcus faecalis that encodes sensitivity to a sex pheromone also produced by Staphylococcus aureus. Antimicrob. Agents. Chemother. 45, 2177–2178. doi: 10.1128/aac.45.7.2177-2178.2001
Si, H., Zhang, W. J., Chu, S., Wang, X. M., Dai, L., Hua, X., et al. (2015). Novel plasmid-borne multidrug resistance gene cluster including lsa(E) from a linezolid-resistant Enterococcus faecium isolate of swine origin. Antimicrob. Agents. Chemother. 59, 7113–7116. doi: 10.1128/AAC.01394-15
Singh, K. V., Weinstock, G. M., and Murray, B. E. (2002). An Enterococcus faecalis ABC homologue (Lsa) is required for the resistance of this species to clindamycin and quinupristin-dalfopristin. Antimicrob. Agents. Chemother. 46, 1845–1850. doi: 10.1128/aac.46.6.1845-1850.2002
Soltani, M., Beighton, D., Philpott-Howard, J., and Woodford, N. (2000). Mechanisms of resistance to quinupristin-dalfopristin among isolates of Enterococcus faecium from animals, raw meat, and hospital patients in Western Europe. Antimicrob. Agents. Chemother. 44, 433–436. doi: 10.1128/aac.44.2.433-436.2000
Sun, H. L., Liu, C., Zhang, J. J., Zhou, Y. M., and Xu, Y. C. (2019). Molecular characterization of vancomycin-resistant enterococci isolated from a hospital in Beijing. China. J. Microbiol. Immunol. Infect. 52, 433–442. doi: 10.1016/j.jmii.2018.12.008
Tanimoto, K., and Ike, Y. (2008). Complete nucleotide sequencing and analysis of the 65-kb highly conjugative Enterococcus faecium plasmid pMG1: identification of the transfer-related region and the minimum region required for replication. Fems. Microbiol. Lett. 288, 186–195. doi: 10.1111/j.1574-6968.2008.01342.x
Tomita, H., Pierson, C., Lim, S. K., Clewell, D. B., and Ike, Y. (2002). Possible connection between a widely disseminated conjugative gentamicin resistance (pMG1-like) plasmid and the emergence of vancomycin resistance in Enterococcus faecium. J. Clin. Microbiol. 40, 3326–3333. doi: 10.1128/jcm.40.9.3326-3333.2002
Tomita, H., Tanimoto, K., Hayakawa, S., Morinaga, K., Ezaki, K., Oshima, H., et al. (2003). Highly conjugative pMG1-like plasmids carrying Tn1546-like transposons that encode vancomycin resistance in Enterococcus faecium. J. Bacteriol. 185, 7024–7028. doi: 10.1128/jb.185.23.7024-7028.2003
Waldron, D. E., and Lindsay, J. A. (2006). Sau1: a novel lineage-specific type I restriction-modification system that blocks horizontal gene transfer into Staphylococcus aureus and between S. aureus isolates of different lineages. J. Bacteriol. 188, 5578–5585. doi: 10.1128/JB.00418-06
Wan, T. W., Hung, W. C., Tsai, J. C., Lin, Y. T., Lee, H., Hsueh, P. R., et al. (2016). Novel structure of Enterococcus faecium-Originated ermB-Positive Tn1546-like element in Staphylococcus aureus. Antimicrob. Agents. Chemother. 60, 6108–6114. doi: 10.1128/AAC.01096-16
Wang, S., Guo, Y., Lv, J., Qi, X., Li, D., Chen, Z., et al. (2016). Characteristic of Enterococcus faecium clinical isolates with quinupristin/dalfopristin resistance in China. BMC Microbiol. 16:246. doi: 10.1186/s12866-016-0863-8
Weigel, L. M. (2003). Genetic Analysis of a High-Level vancomycin-resistant isolate of Staphylococcus aureus. Science 302, 1569–1571. doi: 10.1126/science.1090956
Wendlandt, S., Kadlec, K., Fessler, A. T., and Schwarz, S. (2015). Identification of ABC transporter genes conferring combined pleuromutilin-lincosamide-streptogramin a resistance in bovine methicillin-resistant Staphylococcus aureus and coagulase-negative staphylococci. Vet. Microbiol. 177, 353–358. doi: 10.1016/j.vetmic.2015.03.027
Wendlandt, S., Li, B., Ma, Z., and Schwarz, S. (2013a). Complete sequence of the multi-resistance plasmid pV7037 from a porcine methicillin-resistant Staphylococcus aureus. Vet. Microbiol. 166, 650–654. doi: 10.1016/j.vetmic.2013.07.017
Wendlandt, S., Li, J., Ho, J., Porta, M. A., Fessler, A. T., Wang, Y., et al. (2014). Enterococcal multiresistance gene cluster in methicillin-resistant Staphylococcus aureus from various origins and geographical locations. J. Antimicrob. Chemother. 69, 2573–2575. doi: 10.1093/jac/dku137
Wendlandt, S., Lozano, C., Kadlec, K., Gomez-Sanz, E., Zarazaga, M., Torres, C., et al. (2013b). The enterococcal ABC transporter gene lsa(E) confers combined resistance to lincosamides, pleuromutilins and streptogramin A antibiotics in methicillin-susceptible and methicillin-resistant Staphylococcus aureus. J. Antimicrob. Chemother. 68, 473–475. doi: 10.1093/jac/dks398
Werner, G., Klare, I., Heier, H., Hinz, K. H., Bohme, G., Wendt, M., et al. (2000). Quinupristin/dalfopristin-resistant enterococci of the satA (vatD) and satG (vatE) genotypes from different ecological origins in Germany. Microb. Drug Resist. 6, 37–47. doi: 10.1089/mdr.2000.6.37
Yan, X., Li, Z., Chlebowicz, M. A., Tao, X., Ni, M., Hu, Y., et al. (2016). Genetic features of livestock-associated Staphylococcus aureus ST9 isolates from Chinese pigs that carry the lsa(E) gene for quinupristin/dalfopristin resistance. Int. J. Med. Microbiol. 306, 722–729. doi: 10.1016/j.ijmm.2016.08.001
Yan, X., Tao, X., He, L., Cui, Z., and Zhang, J. (2011). Increasing resistance in multiresistant methicillin-resistant Staphylococcus aureus clones isolated from a Chinese hospital over a 5-year period. Microb. Drug. Resist. 17, 235–239. doi: 10.1089/mdr.2010.0029
Yan, X., Yu, X., Tao, X., Zhang, J., Zhang, B., Dong, R., et al. (2014). Staphylococcus aureus ST398 from slaughter pigs in northeast China. Int. J. Med. Microbiol. 304, 379–383. doi: 10.1016/j.ijmm.2013.12.003
Yaw, L. K., Robinson, J. O., and Ho, K. M. (2014). A comparison of long-term outcomes after meticillin-resistant and meticillin-sensitive Staphylococcus aureus bacteraemia: an observational cohort study. Lancet. Infect. Dis. 14, 967–975. doi: 10.1016/S1473-3099(14)70876-X
Keywords: Enterococcus faecium, conjugative plasmid, lsa(E), Staphylococcus aureus, quinupristin/dalfopristin
Citation: Yan X-M, Wang J, Tao X-X, Jia H-B, Meng F-L, Yang H, You Y-H, Zheng B, Hu Y, Bu X-X and Zhang J-Z (2021) A Conjugative MDR pMG1-Like Plasmid Carrying the lsa(E) Gene of Enterococcus faecium With Potential Transmission to Staphylococcus aureus. Front. Microbiol. 12:667415. doi: 10.3389/fmicb.2021.667415
Received: 13 February 2021; Accepted: 07 May 2021;
Published: 04 June 2021.
Edited by:
Jian-Hua Liu, South China Agricultural University, ChinaReviewed by:
Xiang-Dang Du, Henan Agricultural University, ChinaLiping Wang, Nanjing Agricultural University, China
Copyright © 2021 Yan, Wang, Tao, Jia, Meng, Yang, You, Zheng, Hu, Bu and Zhang. This is an open-access article distributed under the terms of the Creative Commons Attribution License (CC BY). The use, distribution or reproduction in other forums is permitted, provided the original author(s) and the copyright owner(s) are credited and that the original publication in this journal is cited, in accordance with accepted academic practice. No use, distribution or reproduction is permitted which does not comply with these terms.
*Correspondence: Jian-Zhong Zhang, emhhbmdqaWFuemhvbmdAaWNkYy5jbg==; Xiao-Mei Yan, eWFueGlhb21laUBpY2RjLmNu
†These authors have contributed equally to this work and share first authorship