- 1Athens Veterinary Diagnostic Laboratory, College of Veterinary Medicine, University of Georgia, Athens, GA, United States
- 2Department of Infectious Diseases, College of Veterinary Medicine, University of Georgia, Athens, GA, United States
- 3Department of Pathobiology, Ontario Veterinary College, University of Guelph, Guelph, ON, Canada
- 4Institute of Bioinformatics, University of Georgia, Athens, GA, United States
- 5Center for Tropical and Emerging Global Diseases, University of Georgia, Athens, GA, United States
- 6Southeastern Cooperative Wildlife Disease Study, Departments of Pathology and Population Health, College of Veterinary Medicine, University of Georgia, Athens, GA, United States
- 7Veterinary Medical Teaching Hospital, University of California, Davis, Davis, CA, United States
- 8Center for Genome Sciences, United States Army Medical Research Institute of Infectious Diseases, Frederick, MD, United States
- 9U.S. Geological Survey, National Wildlife Health Center, Madison, WI, United States
The Pasteurellaceae family has been associated with fatal diseases in numerous avian species. Several new taxa within this family, including Bisgaard taxon 40, have been recently described in wild birds, but their genomic characteristics and pathogenicity are not well understood. We isolated Bisgaard taxon 40 from four species of seabirds, including one sampled during a mass, multi-species mortality event in Florida, United States. Here, we present a comprehensive phenotypic and genetic characterization of Bisgaard taxon 40 and comparative genomic analysis with reference strains from the Pasteurellaceae family, aiming at determining its phylogenetic position, antimicrobial susceptibility profile, and identifying putative virulence factors. In silico multilocus sequence-based and whole-genome-based phylogenetic analysis clustered all Bisgaard taxon 40 strains together on a distinct branch separated from the other members of the Pasteurellaceae family, indicating that Bisgaard taxon 40 could represent a new genus. These findings were further supported by protein similarity analyses using the concatenation of 31 conserved proteins and other taxonomic approaches such as the percentage of conserved protein test. Additionally, several putative virulence factors were identified, including those associated with adhesion (capsule, ompA, ompH) and colonization (exbD, fur, galU, galE, lpxA, lpxC, and kdsA) of the host and a cytolethal distending toxin (cdt), which may have played a role in disease development leading to the mortality event. Considerably low minimum inhibitory concentrations (MICs) were found for all the drugs tested, in concordance with the absence of antimicrobial resistance genes in these genomes. The novel findings of this study highlight genomic and phenotypic characteristics of this bacterium, providing insights into genome evolution and pathogenicity. We propose a reclassification of these organisms within the Pasteurellaceae family, designated as Mergibacter gen. nov., with Mergibacter septicus sp. nov. as the type species. The type strain is Mergibacter septicus A25201T (=DSM 112696).
Introduction
The family Pasteurellaceae is the only member of the order Pasteurellales in the class Gammaproteobacteria (Christensen et al., 2014). Members of the family Pasteurellaceae have been isolated from mucosal membranes of the alimentary, respiratory, and reproductive tracts of healthy and diseased vertebrates (Christensen et al., 2003b). Although most Pasteurellaceae species are considered opportunistic and secondary invaders, some serve as primary pathogens and are responsible for various diseases in humans and animals (Eid et al., 2019).
Important avian pathogens are included in the Pasteurellaceae family with a broad clinical presentation that ranges from acute septicemia to chronic and localized infections, leading to significant morbidity and mortality in domestic and wild birds (Christensen et al., 2014). Pasteurella multocida subspecies multocida is the most common cause of fowl cholera in birds, although P. multocida subspecies septica and P. multocida subspecies gallicida may also cause fowl cholera-like disease (Christensen and Bisgaard, 2000; Christensen et al., 2014). The genus Gallibacterium has been reported worldwide from a broad host range among farmed and wild birds, mainly associated with reproductive disorders (Bojesen et al., 2008; Narasinakuppe Krishnegowda et al., 2020). Avibacterium paragallinarum is the causative agent of infectious coryza, a severe disease of the upper respiratory tract of chickens (Blackall et al., 2005; Chen et al., 2014). Other Pasteurellaceae species and taxa, including Bisgaard taxa, have been described in avian hosts (Christensen et al., 2003b; Blackall et al., 2005; Gregersen et al., 2009). The Bisgaard taxa represent a group of unclassified members of the Pasteurellaceae family isolated from several orders of birds and rodents with and without the disease (Christensen et al., 2003b, 2014). Bisgaard taxa were confirmed to belong to the Pasteurellaceae family by integrating comprehensive phenotypic characterization and 16S rRNA gene sequence analysis (Christensen et al., 2003b). With the advent of following next-generation sequencing (NGS) technologies, further taxonomic efforts established more definite classification for numerous Bisgaard taxa, resulting in the establishment of five new genera (Volucribacter, Frederiksenia, Caviibacterium, Conservatibacter, and Spirabiliibacterium) within the Pasteurellaceae family (Christensen et al., 2014; Korczak et al., 2014; Adhikary et al., 2018; Bisgaard and Christensen, 2019). However, the classification of some Bisgaard taxa, including Bisgaard taxon 40, is still not fully resolved, and this taxon remained unnamed (Christensen et al., 2003b).
Bisgaard taxon 40 was first isolated from the respiratory tract of a common gull (Larus canus) in Denmark (Christensen et al., 2003b). More recently, Bisgaard taxon 40 has been reported in rhinoceros auklets (Cerorhinca monocerata) with pneumonia and septicemia in Washington, United States (Knowles et al., 2019). Phylogenetic analysis based on 16S rRNA sequence revealed that Bisgaard taxon 40 was closely related to Bisgaard taxon 14 (reclassified as Spirabiliibacter mucosae), which was isolated from the respiratory tract of ducks, pigeons, turkeys, and pheasants with septicemia (Bisgaard and Mutters, 1986) and Bisgaard taxon 32 (reclassified as Spirabiliibacter pneumonia) isolated from hawks and pigeons with pneumonia and conjunctivitis (Boot and Bisgaard, 1995). These taxa (40, 14, and 32) formed a clade with testudinistestudinis and Chelonobacter oris and were classified in the Testudinis 16S rRNA group (Christensen et al., 2003b). Aside from their 16S relationships, little information is available on the genomic diversity, virulence factors, antimicrobial resistance, and evolutionary relationship between Bisgaard taxon 40 and other Pasteurellaceae members.
Here, we describe the first whole-genome sequence characterization for five Bisgaard taxon 40 strains, including the one isolated in a mass mortality event involving common terns (Sterna hirundo) and sandwich terns (Thalasseus sandvicensis) on the coast of Marco Island, Florida (United States) in October 2018 (Niedringhaus et al., 2021). Comparative genomic and proteomic analyses were conducted between Bisgaard taxon 40 and fifty-eight representative members belonging to thirty-three genera within the Pasteurellaceae family. This included the investigation of virulence genes and sequence analysis and phylogenetic relationships using core genome and targeted gene sequences. This study also aimed to investigate the presence of antimicrobial resistance genes and the susceptibility of Bisgaard taxon 40 to eighteen antimicrobial agents.
Materials and Methods
Bacterial Isolation, Species Identification, and Phenotypical Characterization
Brain, liver, heart, and spleen samples from three common terns were submitted to the Athens Veterinary Diagnostic Laboratory for aerobic and anaerobic bacterial culture at the University of Georgia (UGA). Tissues were rinsed with sterile phosphate-buffered saline (PBS) and processed in 3ml of brain heart infusion (BHI) enrichment broth (BD, Franklin Lakes, New Jersey, United States) using bead beater technology (Next Advance, Troy, New York, United States). After homogenization, the specimens were cultured on 5% sheep blood (BD, Franklin Lakes, New Jersey, United States), MacConkey, and Chocolate agar (Remel, San Diego, California, United States) and incubated at 42°C for 24–48h in aerobic conditions. Brucella agar (Remel, San Diego, California, United States) was also used to evaluate growth in anaerobic conditions. Matrix-assisted laser desorption ionization time-of-flight (MALDI-TOF) mass spectrometry analysis (VITEK MS - bioMérieux, Marcy-lʹÉtoile, France) was used following the manufacturer’s instructions to attempt bacterial genus and species identification. Additionally, specific bacterial traits were tested, including catalase, oxidase, urease, and indole production. As per the manufacturer’s instructions, carbon source utilization testing was performed using a GEN III MicroPlate (Biolog, Inc., Hayward, California, United States).
Four additional isolates were obtained by the U.S. Geological Survey, National Wildlife Health Center (NWHC; Madison, Wisconsin) during routine disease investigations across the United States. Information on these isolates is presented in Table 1. Strains from these four birds were isolated by touching the tissue directly onto 5% sheep blood agar plates and streaking the inoculated area of the plate for isolation. Plates were incubated at 37°C for 24–48h under aerobic conditions. Isolates originating from NWHC were identified by sequencing the V1-V3 regions of the 16S rRNA gene as described previously (Shearn-Bochsler et al., 2018). Carbon source utilization testing on NWHC isolates was performed with commercially available kits and assays, including GEN III MicroPlates (Biolog, Inc., Hayward, California, United States), Analytical Profile Index (API) 20E test strips (bioMérieux, Inc., Durham, North Carolina, United States), and 4-MU discs (Key Scientific Products, Stamford, Texas, United States).
Whole-Genome-Sequencing and Assembly Approach
At UGA, DNA was extracted using the DNeasy Extraction Kit (Qiagen, Hilden, Germany) following the manufacturer’s protocol. The concentration of total DNA extracted was determined using the Qubit double-stranded DNA assay kit (Thermo Fisher Scientific, Waltham, Massachusetts, United States). For the short-read sequencing using Illumina technologies (Illumina Inc., San Diego, California, United States), approximately 50ng of DNA was used for library preparation using Nextera DNA Library Prep kit (Illumina). Paired-end 300bp length reads were generated using the Illumina iSeq 100 System (Illumina Inc., San Diego, California, United States). Approximately 400ng of DNA was used for library preparation using Oxford Nanopore Technologies (ONT) library preparation SQK-RBK004 Rapid Barcoding Kit for the long-read sequencing. According to the manufacturer’s instructions, sequencing was performed on MinION with the FLOW-MIN 106 (R9.4 SpotOn) flow cell (Nanopore, Oxford, United Kingdom). Barcoded ONT sequencing reads were demultiplexed using Porechop v0.2.41 and used as input into Flye v2.6 assembler (Lin et al., 2016; Kolmogorov et al., 2019) with the expected genome size parameters set at 2Mb. Illumina paired-end reads were first qualitatively checked using FastQC v0.11.8 (Wingett and Andrews, 2018) and then submitted to Trimmomatic v0.36 (Bolger et al., 2014) to remove adapters and select reads with Phred scores >30. Illumina reads were then aligned to the ONT-based resulting genome assembly using BWA v0.7.17 (Li and Durbin, 2009). Both draft ONT assembly and alignment results were then submitted to Pilon v1.23 (Walker et al., 2014) for error correction and assembly base call polishing. Assembly metrics were evaluated using the Quality Assessment Tool for Genome Assemblies v5.0.2 (QUAST; Gurevich et al., 2013). The polished assembly was then submitted to the Circlator v1.5.3 pipeline (Hunt et al., 2015) to attempt automatic assembly circularization. The final genome sequence was annotated using the NCBI Prokaryotic Genome Annotation Pipeline (PGAP; Tatusova et al., 2016; Haft et al., 2018) and deposited in GenBank under the accession number CP054053.
At NWHC, DNA was extracted from overnight cultures using a phenol: chloroform extraction method (Barbier et al., 2019). This DNA was sequenced on a Pacific Biosciences RSII at the Center for Genome Sciences, U.S. Army Medical Research Institute of Infectious Diseases (USAMRIID-CGS). Following the manufacturer’s protocol, sequencing libraries were prepared using the SMRTbell™ Template Prep Kit (Pacific Biosciences, Menlo Park, California, United States). DNA (~5μg) was fragmented using gTUBE (Covaris Inc., Woburn, Massachusetts, United States) to ~20kb. After DNA damage and ends repair, blunt hairpin adapters were ligated to the template, and failed ligation products were digested with ExoIII and ExoVII exonucleases. The resulting SMRTbell templates were size selected on a BluePippin system (Sage Science, Beverly, Massachusetts, United States) using 0.75% dye-free agarose cassette with 4–10kb Hi-Pass protocol and low-cut set on 4kb. Size selected templates were cleaned and concentrated with AMPure PB beads. P6 polymerase was used in combination with the C4 sequencing kit, and 360-min movies were collected. HGAP3 (Chin et al., 2013) was used for de novo genome assembly. Assembled genomes were visualized using Gepard v1.30 (Krumsiek et al., 2007). When evidence that a contig/chromosome was circularly complete was observed, the redundant sequence was trimmed from the ends. The breakpoint was adjusted so that the linear representation of the circular sequence began at the first base of the dnaA gene. Partial sequences were left unmodified. All assembled sequences were then error-corrected using Quiver v0.90 (Chin et al., 2013). Finally, all assemblies were submitted to Genbank for annotation with PGAP (Tatusova et al., 2016; Haft et al., 2018) and deposited in GenBank under the accession numbers CP022010–CP022013.
Genome Diversity Analysis
Average nucleotide identity (ANI) and tetranucleotide signature correlation index (TETRA) scores (Richter and Rossello-Mora, 2009) were calculated using JSpecies (Richter et al., 2016) on the Progressive Mauve (Darling et al., 2004) alignment of the five Mergibacter whole-genome sequences obtained in this study (Table 1). Additionally, visualization of the Mergibacter alignment (Figure 1) was obtained using Blast Ring Image Generator (Alikhan et al., 2011).
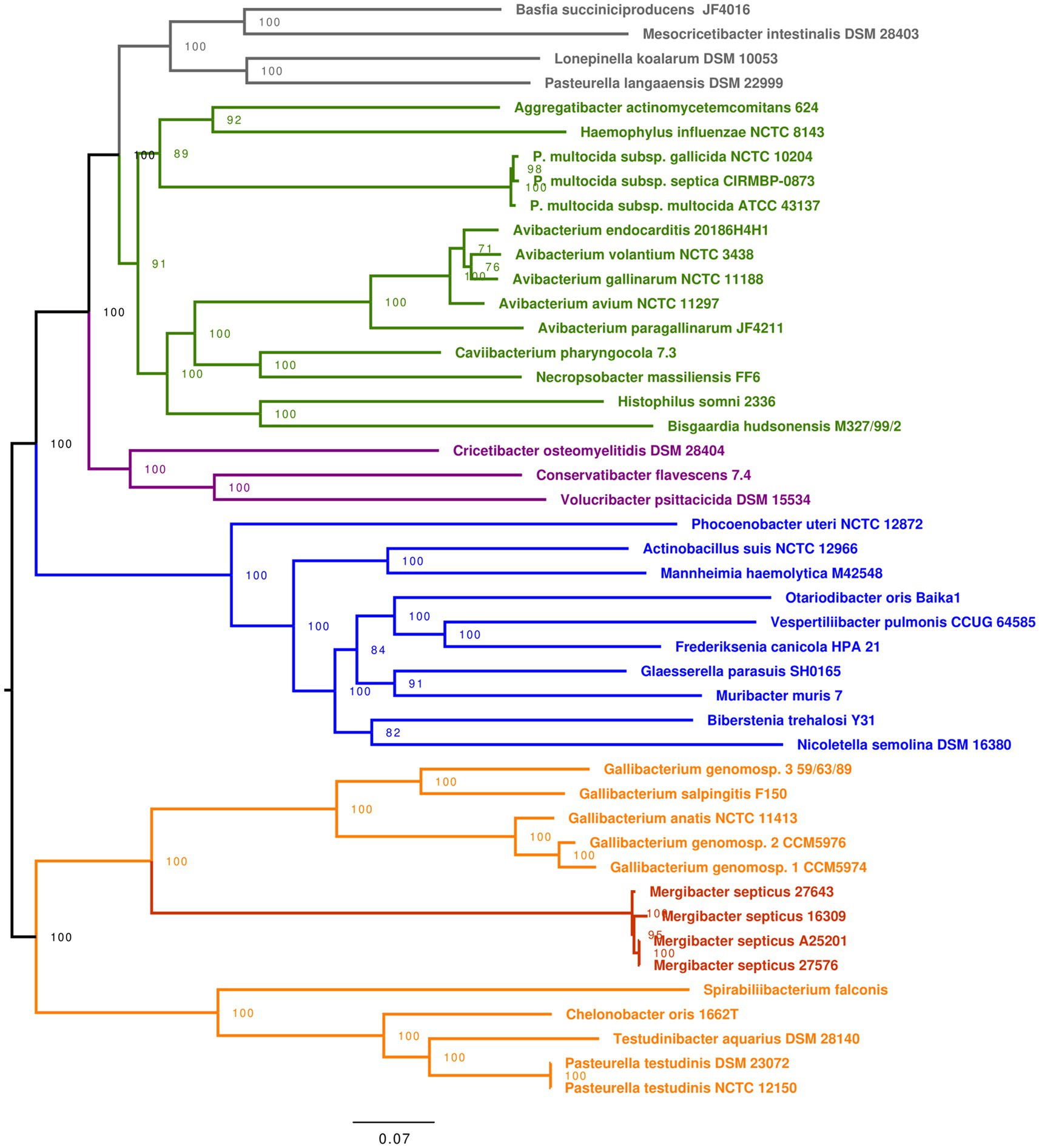
Figure 1. M. septicus whole-genome synteny based on five strains. Circular map of the comparative genome analysis performed using and the Blast Ring Image Generator software. The rings depict the percent identity between M. septicus genomes, represented by different colors. The second ring represents the GC content (in black).
Phylogenomic Analyses
Three phylogenetic analyses were performed in this study based on (i) Pasteurellaceae bacterial core genomes (ii) 16S rRNA gene, and (iii) on a Pasteurellaceae housekeeping multilocus sequence (MLS). First, the complete genomes or assemblies of 58 Pasteurellaceae strains were downloaded from NCBI (Supplementary Table S1). The sequence alignment for the core genome-based phylogenetic analysis included 41 out of 58 Pasteurellaceae strains and five Mergibacter genomes, and it was performed using Progressive Mauve (Darling et al., 2004), followed by phylogenetic tree estimation with RAxML (Stamatakis, 2014). Then, the nucleotide sequences of the 16S rRNA and four additional housekeeping genes were manually extracted from whole genomes of these 58 Pasteurellaceae representatives and from Mergibacter strains (Supplementary Table S1). The 16S rRNA gene [1,261bp in length; from nucleotide 51 to 1,312 of Pasteurella multocida 20N (GenBank: CP028926)] core portion was used to construct the 16S rRNA gene tree. The Pasteurellaceae housekeeping multilocus sequence-based tree was constructed using the concatenated core sequences of four Pasteurellaceae housekeeping genes used in previous taxonomic approaches for this family (Christensen et al., 2007, 2014): atpD [1,152bp in length; from nucleotide 137 to 1,289 of Pasteurella multocida 20N (GenBank: CP028926)], infB [1,511bp in length; from nucleotide 714 to 2,225 of Pasteurella multocida 20N (GenBank: CP028926)], recN [1,347bp in length; from nucleotide 35 to 1,382 of Pasteurella multocida 20N (GenBank: CP028926)], and rpoB [3,918bp in length; from nucleotide 88 to 4,006 of Pasteurella multocida 20N (GenBank: CP028926)]. The sequence alignment for the two gene trees (16S rRNA gene, housekeeping multilocus sequence) was performed with MAFFT (Katoh and Standley, 2013) in Geneious software (version 2020.0.4), and phylogenetic trees were estimated using RAxML (Stamatakis, 2014). All trees were visualized and edited in FigTree (v1.4.4).2
Taxonomic Analyses
Average amino acid identity (AAI) was calculated using MAFFT (Katoh and Standley, 2013) on the Progressive Mauve (Darling et al., 2004) alignment of the 5 Mergibacter sequences and 27 representatives of the Pasteurellaceae family, including the most genetically closed related species: C. oris, Pasteurella testudines, and Gallibacterium species.
The taxonomic approach known as percentage of conserved protein (POCP) was applied to the M. septicus type strain A25201T (=DSM 112696; CP054053) and its three most closely genetically related species (C. oris, P. testudines, and Gallibacterium genomosp. 3) as previously described (Qin et al., 2014; Barco et al., 2020). Briefly, the predicted CDS (coding DNA sequences) for the four mentioned species were extracted using Artemis (Carver et al., 2012). BLASTp algorithm3 was used to find conserved proteins with an E value of less than 1e−5, sequence identity >40%, and comparable region of the query protein sequence >50%. Then, the POCP between two genomes was calculated as [(C1+ C2)/(T1+ T2)]×100%, where C1 and C2 represent the conserved number of proteins in the two genomes being compared, respectively, and T1 and T2 represent the total number of proteins in the two genomes being compared, respectively (Qin et al., 2014).
Finally, the taxonomic strategy for genus demarcation within the Pasteurellaceae family, which was previously described (Christensen and Bisgaard, 2018), was used in this study to support the reclassification of Bisgaard taxon 40 as the new genus Mergibacter. Briefly, the sequences of 31 conserved CDS selected (Christensen and Bisgaard, 2018) were manually extracted from M. septicus A25201, C. oris, Pasteurella testudines, and Gallibacterium species and concatenated using Geneious software (version 2020.0.4). Then, multisequence amino acid alignments were performed, and inter genus pairwise similarities were estimated with MUSCLE (Edgar, 2004).
Identification of Virulence Factors
BLASTN v 2.2.9 (Altschul et al., 1997) was used to align the whole-genome sequences of the five Mergibacter isolates presented in this study against the Virulence Factors Database (VFDB; Chen et al., 2005). The parameters used for the BLAST search were ≥70% gene identity and ≥0% sequence length. Additionally, virulence factors previously described in other Pasteurellaceae members (Chen et al., 2014; Christensen et al., 2014; Khamesipour et al., 2014; Furian et al., 2016), including those related to capsule, outer membrane proteins (ompA, ompH, oma87, plpB, psl), adhesins (ptfA, pfhA, tadD, hsf-1, hsf-1, hsf-2, ptfA, fimA, pfhA, tadD) iron metabolism (fur, exbD, tonB, hgbA, hgbB), superoxide dismutase (sodA, sodC, tbpA), sialidase (nanH, nanB), toxin (toxA, cdt), and hyaluronic acid synthetase (pmHAS), were manually searched in all the annotated genomes using Geneious software (version 2020.0.4). The corresponding DNA sequences of virulence factors of interest were then manually extracted, and comparative analyses with those of the reference strains most closely related to Margibacter and/or associated with avian disease insurgence in the Pasterellaceae family were performed. A multisequence alignment for each virulence factor was performed with MAFFT (Katoh and Standley, 2013), and phylogenetic relatedness was estimated with RAxML (Stamatakis, 2014). Alignments and phylogenetic trees were visualized in Gingr (v 1.1.1) from the Harvest Package (Treangen et al., 2014). As a result of the comparison, heatmaps containing the percentage similarity of each virulence factor were generated using GraphPad Prism version 8.4.3 (GraphPad Software San Diego, California United States).
Antimicrobial Susceptibility Testing and Identification of Antimicrobial Resistance Genes
Antimicrobial susceptibility testing was performed on Mergibacter type strain M. septicus A25201T (DSM 112696) by broth microdilution using the Trek Sensititre system (Trek Diagnostic Systems, Cleveland, OH), followed by strip testing using Etest (bioMérieux). For the Trek Sensititre testing, individual colonies were selected from a blood agar plate and used to prepare a 0.5 McFarland suspension for inoculation of AVIAN1F plates (Thermo Fisher Scientific, Waltham, MA, United States), according to the manufacturer’s instructions. The minimum inhibitory concentration (MIC) values were visually confirmed using an inverted mirror after 24 and 48h of incubation at 42°C. The following antimicrobial compounds are included in the AVIAN1F plates: amoxicillin, ceftiofur, clindamycin, enrofloxacin, erythromycin, florfenicol, gentamicin, neomycin, novobiocin, oxytetracycline, penicillin G, spectinomycin, streptomycin, sulfadimethoxine, sulphathiazole, tetracycline, trimethoprim/sulfamethoxazole, and tylosin. Additionally, we used E-strips on Mueller-Hinton agar plates (bioMérieux) to evaluate the susceptibility for amoxicillin, amoxicillin-clavulanate, imipenem, and doxycycline, according to the manufacturer’s instructions.
We then searched for antimicrobial resistance genes (AMR) in all five whole-genome sequences analyzed, using three different databases: (i) Comprehensive Antibiotic Resistance Database (CARD) with criteria selected as perfect and strict, excluding nudge of loose hits to strict and high quality/coverage sequences4; (ii) ResFinder for acquired antimicrobial resistance genes, with default settings of 90% nucleotide similarity and a 60% of minimum length5 (Zankari et al., 2012); and (iii) AMRFinderPlus for acquired antimicrobial resistance genes, with default settings of −1.0 as the minimum identity for a blast-based hit and 0.5 as minimum coverage of the reference protein.6 Additionally, Plasmid Finder7 and COPLA plasmid taxonomic classifier8 databases were used to identify plasmids in all the five whole-genome sequences. Further, for the A25201 strain, three different assembly approaches were used as databases input: The Nanopore-based assembly, the Illumina reads-based assembly, and the polished assembly to investigate to which extent the assembly approach could influence the detection of plasmids.
Results
Growth Characteristics and Phenotype of Bisgaard Taxon 40 Strains
Growth and phenotypic analysis were performed in parallel at the University of Georgia (UGA, n=1) and at the National Wildlife Health Center (NWHC, n=4) on five bacterial isolates. In addition, a preliminary taxonomic identification of the isolates was performed by amplification and sequencing of a portion of bacterial housekeeping gene the 16S rRNA, indicating an exact match of these five isolates with sequences of the Bisgaard taxon 40 from the Pasteurellaceae family [i.e., AY172732.1 (Christensen et al., 2003b); MG735704.1 (Knowles et al., 2019)].
Isolate A25201 was obtained at UGA from heart and liver samples of one common tern after 48h of aerobic incubation at 42°C. Isolate A25201 grew on blood agar presenting β-hemolysis and a shiny, circular, whitish-cream colony morphology (Supplementary Figure S1). Additionally, isolate A25201 was catalase and oxidase-positive, while urease and indole tests were negative. No bacterial growth was obtained on MacConkey and Brucella agar, and Gram-negative staining rods were identified when observing A25201 isolate under the microscope (data not shown). No bacterial identification was obtained from MALDI-TOF analysis. In contrast, GEN III Microbial ID analysis revealed a mixed identification of the isolates with a low percentage of probability, including Pasteurella canis/stomatis (51.6%), Histophilus somni (15.5%), Nicoletella semolina (9%), and Pasteurella pneumotropica (9%). Acid was formed from D-glucose, D-mannose, D-maltose, D-fructose, D-galactose, D-cellobiose, methyl pyruvate, inosine, and acetoacetic acid.
Similar growth characteristics and phenotypes were observed in the four isolates (27643, 27576, 16309, and 275176) processed at NWHC (β-hemolytic, shiny, circular, whitish colonies after 48h incubation, catalase-positive, oxidase-positive, urease negative, indole negative, and no growth of on MacConkey). Surprisingly, three of the four NWHC isolates differ from isolate A25201 in their ability to utilize various carbon sources. All the phenotypic characteristics comparable between the five strains are reported in Table 2, but overall, within the characters that separate the Bisgaard taxon 40 strains from the other Pasteurellaceae genera are the presence of β-hemolysis and acid formation from D-mannitol and sucrose (Table 2).
Bisgaard Taxon 40 Genomic Features and Genome Diversity
For UGA strain A25201, 229 ONT reads were assembled, resulting in one single contig of 1.900Mb length with no extra leftover contigs. A total of 1.760,502 Illumina paired-end reads were aligned to the ONT resultant draft genome, and the polished assembly consisted of one single contig with a total length of 1. 887Mb. No signs of circularization and overlapping ends were identified in silico. For the four NWHC strains, an estimated 590-731x coverage of the genome was obtained with single-molecule real-time (PacBio) reads, and assembly resulted in a single contig. In silico evidence for these contigs being circularly complete was observed in strains 27517 and 27576, presenting a genome size of ~1.887Mb in length. The de novo assembled genomes for the other two strains, 16309 and 26643, were 1.908Mb and 1.861Mb in length, respectively. The genomic features for the five strains are summarized in Table 3.
The genomes of the five Bisgaard taxon 40 strains identified and studied in this project showed high genome similarity, despite missing regions, as depicted in Figure 1. The ANI scores between the five genomes were 98.29–100%, well above the species boundary (Richter et al., 2016). Similarly, the TETRA analysis yielded correlation coefficients of 0.9985, confirming they were all isolates of the same species (Richter et al., 2016). The genomic alignment of the five isolates produced a 1,647,356bp consensus sequence with 1630; 1680; 1678; 1697; and 1677 annotated regions for strains A25201, 27643, 27576, 16309, and 27517, respectively. Of the annotated regions, 1468; 1462; 1460; 1479 and 1459 were, respectively, confirmed as protein-coding sequences (CDS).
Bisgaard Taxon 40, the New Pasteurellaceae Genus Mergibacter
The genetic relatedness of the five strains of this study with 41 representative members of the Pasteurellaceae family was assessed by whole-genome sequence alignments. The highest inter-genus nucleotide similarity percentages were 69.2–72.9% with Pasteurella, 70% with Frederiksenia, 70–70.4% with Mannheimia, 70.4–70.5% with Bibersteinia, 70.6–70.7% with Actinobacillus, 70.6–72.4% with Avibacterium, 70.9–71.1% with Caviibacterium, 70.9–71.1% with Chelonobacter, 71% with Glaesserella, 72.4–72.6% with Haemophilus, 72.7–73.0% with Histophilus, 72.8–72.9% with Conservatibacter, 73.3–73.4% with Volucribacter, and 73.6–75.4% with members belonging to Gallibacterium genus. A core genome-based phylogenetic analysis divided Pasteurellaceae into five main clades and consistently placed the five Bisgaard taxon 40 strains as a distinct, well-defined terminal monophyletic group (Figure 2). Although closely related, Bisgaard taxon 40 and genera Gallibacterium, Pasteurella, and Chelonobacter form differentiated clades under a common node indicating the identity of Bisgaard taxon 40 as a new genus.
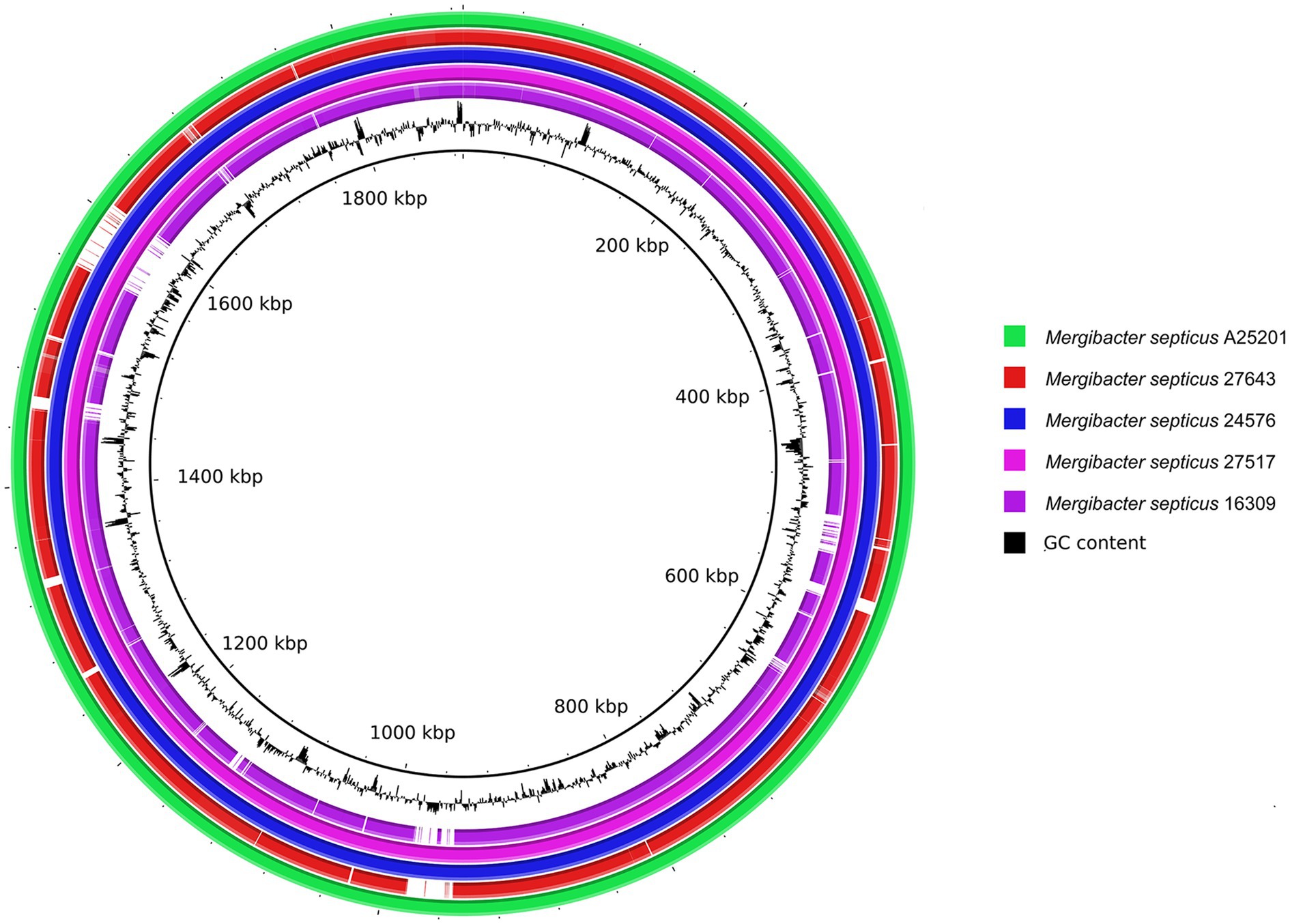
Figure 2. Core genome-based phylogeny of Mergibacter within the Pasteurellaceae family. The sequence alignment for the core genome-based phylogenetic analysis was obtained using Progressive Mauve (Darling et al., 2004), and phylogenetic tree estimation was done with RAxML (Stamatakis, 2014). The tree was visualized and edited in FigTree (v1.4.4; http://tree.bio.ed.ac.uk/software/figtree). Scale bar represents average number of nucleotide substitutions per site.
Three different taxonomic tests were performed to determine the integrity of this finding. First, an AAI analysis using 764 CDS conserved across Pasteurellaceae showed that the five Bisgaard taxon 40 isolates shared the highest amino acid similarity percentages with Gallibacterium, ranging from 67.78–68% with G. genomosp. 2 to 69.8–70% with G. genomosp. 3. The AAI scores observed here are far below the AAI thresholds for separating potential genera in other bacterial families (Konstantinidis and Tiedje, 2007; Sangal et al., 2016), further supporting the belonging of the five subject strains to a new genus. Second, a POCP taxonomic analysis was performed on Bisgaard taxon 40 and its three most closely genetically related species (according to core genome phylogeny). Surprisingly, the results obtained by testing the taxonomy of the Bisgaard taxon 40 strains with this popular approach were inconclusive. The current POCP value proposed as a genus boundary for most prokaryotic groups is <50% (Qin et al., 2014). However, all the POCP values obtained in this study were above the 50% threshold even between members of supposedly genetically distant genera, such as Chelonobacter and Pasteurella, that shared the highest percentage (~79%) of conserved proteins in this study. Indeed, POCP values of 68.34, 63.28, and 60.81% were obtained between Bisgaard taxon 40 strain A25201 and G. genomosp. 3, C. oris, and P. testudinis, respectively (Supplementary Table S3). In addition, strain A25201 had a lower shared POCPs with the representative strains of its closest genera (Gallibacterium, Chelonobacter, and Pasteurella) than those genera members between each other. Finally, we performed a Pasteurellaceae-specific taxonomic approach based on the AAI of 31 randomly selected conserved protein sequences (Christensen and Bisgaard, 2018). Our results showed that the similarity percentages calculated between Bisgaard taxon 40 A25201 and the closest Pasteurellaceae members ranged from 68.2% with P. testudinis to 69.59% with G. genomosp. 3, well below the highest inter genus pairwise similarity (88%) identified between Pasteurellaceae members (Christensen and Bisgaard, 2018; Supplementary Table S4). These results recognize once again Bisgaard taxon 40 as a new genus within the Pasteurellaceae family.
Hence, based on this taxonomic evidence and the core genome phylogeny described above, we renamed Bisgaard taxon 40 as genus Mergibacter, with M. septicus type strain A25201 (DSM 112696; CP054053) as a type strain.
16SrRNA-Based Phylogeny Is Not Accurate for Genera Classification in Pasteurellaceae
We tested the utility of 16S-based taxonomy to differentiate Mergibacter from other Pasteurellaceae genera. New sequence similarity and phylogenetic analyses were performed by using the 16S sequences extracted from the 58 Pasteurellaceae species and the five Mergibacter strains (Supplementary Table S1). In addition, 19 16S rRNA sequences downloaded from NCBI were included, to represent also some Pasteurellaceae species for which a whole-genome sequence was not publicly available (Supplementary Table S2). The five M. septicus strains showed high sequence similarities among each other, with nucleotide identities of 99.5–100% for the 16S rRNA gene. The highest nucleotide identities between Mergibacter and other Pasteurellaceae species were found with P. testudinis (92.2%), Spirabiliibacter pneumoniae (91.3–91.4%), Spirabiliibacter mucosae (91.8–92%), and members belonging to the genus Gallibacterium (91–92.3%). In our 16S rRNA-based phylogenetic tree, the five Mergibacter strains appeared on a distinct terminal group separated from its closest relatives by good bootstrap support values (Figure 3). This finding further supports Mergibacter as a new genus and the capacity of 16S-based taxonomy to identify Mergibacter representatives among other Pasteurellaceae. Still, some differences were observed between the core genome- and the 16S-based phylogeny estimated in this study. First, we observed that the support values for most of the nodes of the 16S were very low (in many cases <50%), even when 19 additional sequences were included in the study. In fact, the only terminal nodes that showed bootstrap values of 100% were found in the clade that separates Mergibacter from the other Pasteurellaceae genera (Figure 3). Second, the core genome-based phylogeny classified the Pasteurellaceae into five main clades. This topology is not as clear in the 16S tree. Additionally, the core genome phylogenetic analysis placed Gallibacterium as Mergibacter closest genus (Figure 2), while 16S-based phylogeny placed Gallibacterium in a very distant clade (Figure 3). This indicates that although the amplification and subsequent sequencing of the 16S rRNA gene are adequate to identify Mergibacter, this gene may not be the best representative to estimate phylogenetic relatedness among the members of the Pasteurellaceae family.
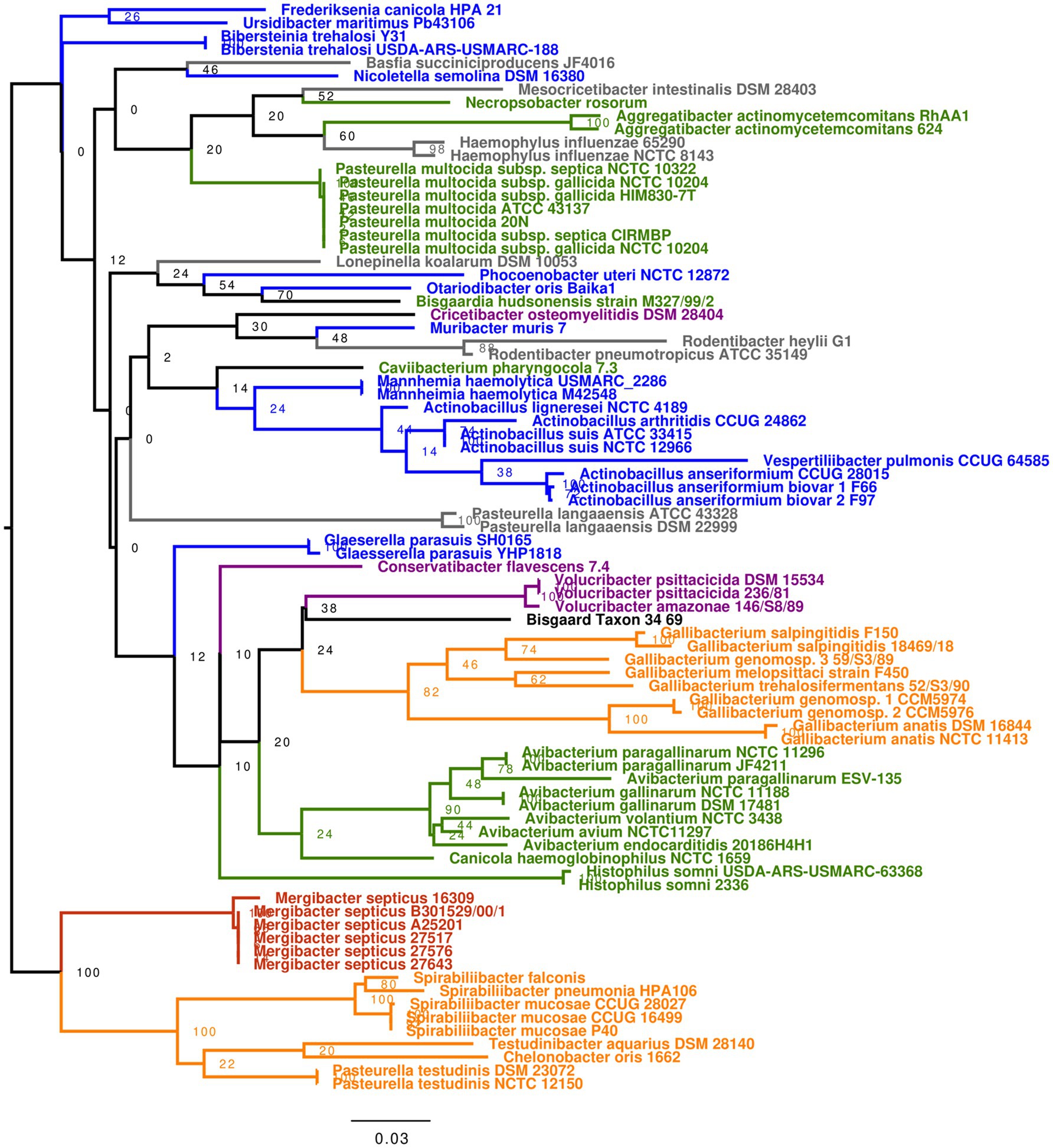
Figure 3. 16S rRNA gene-based phylogeny of 82 Pasteurellaceae members including five M. septicus strains. The sequence alignment for the 16S rRNA-based phylogenetic analysis was performed using MAFFT (Katoh and Standley, 2013) in Geneious software (version 2020.0.4), and phylogenetic trees were estimated using RAxML (Stamatakis, 2014). All trees were visualized and edited in FigTree (v1.4.4; http://tree.bio.ed.ac.uk/software/figtree).
Considering the findings mentioned above, we decided to investigate a cost-effective alternative method for accurately identifying Mergibacter and classifying Pasteurellaceae. Housekeeping genes infB, recN, atpD, and rpoB have been used for this purpose in a previous study (Christensen et al., 2004). We extracted these four genes from the same 63 genomes (Supplementary Table S1) used in the 16S rRNA-based phylogeny, and for each of the genomes, we concatenated the cores of the four genes to create a representative multilocus sequence (MLS). Once again, the five M. septicus showed high similarities among each other, with nucleotide identities of 99.2–99.9% for the MLS, and the closest relatedness was found with members of the Gallibacterium genus, with nucleotide identity values of 80.2–83.3%, 76.6–82.7%, 62.8–64.8%, 82.2–83.5%, and 79.5–80.7%, respectively. The MLS-based phylogenetic tree showed a similar topology to the core genome-based tree with five distinct clades, and Mergibacer as a terminal monophyletic group in a cluster containing closest genera Gallibacterium, Chelonobacer, Spirilibacterium, and Testudinibacter (Figure 4). Although higher values are appreciated in the MLS tree, these are still below those observed in the core genome tree and genera Mesocribacter and Basfia appear misplaced (Figure 4). This suggests that our MLS approach is better than conventional 16S rRNA-based taxonomic methods but still inferior to core genome-based phylogeny.
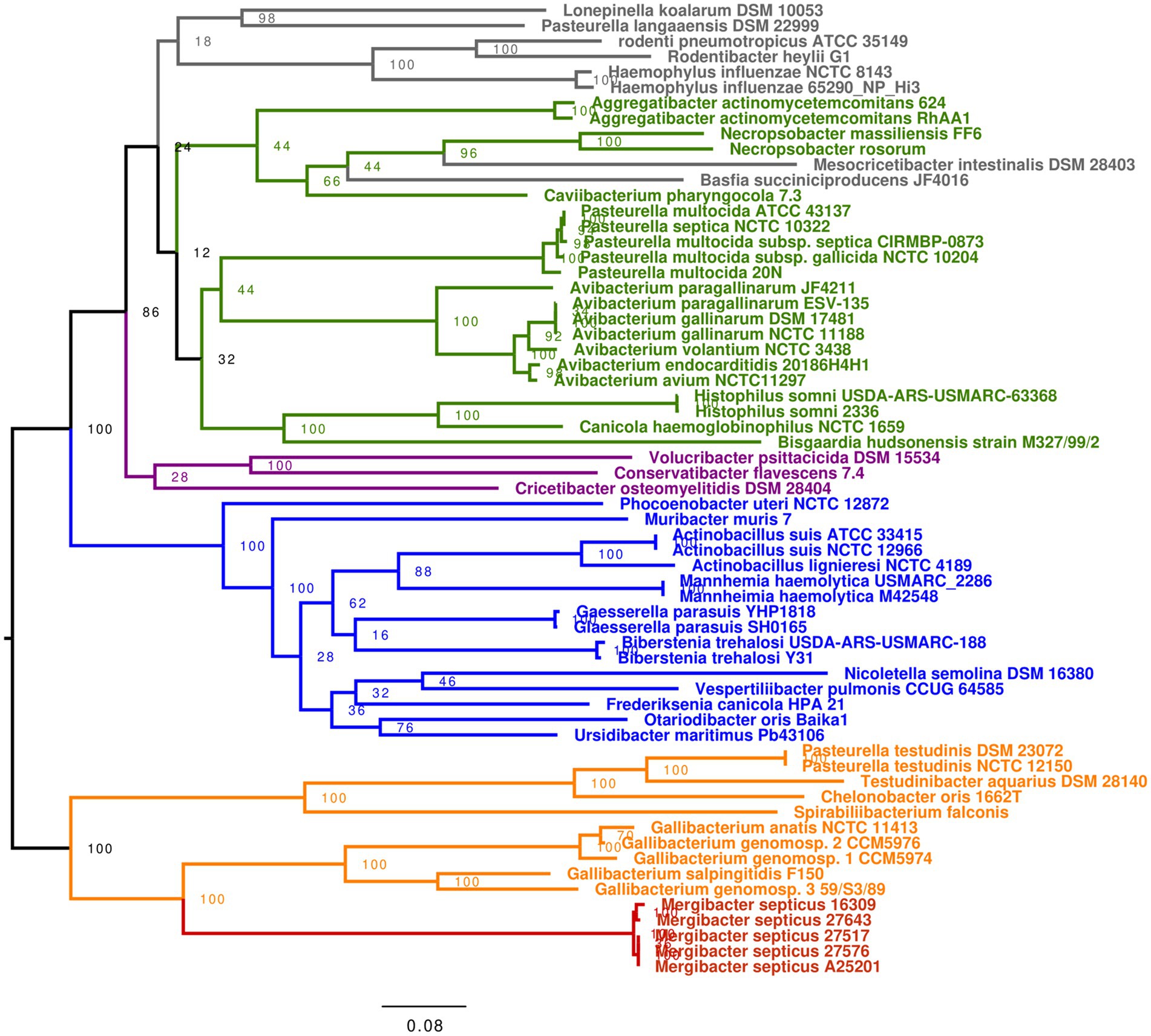
Figure 4. MLS-based phylogeny of 63 Pasteurellaceae members including five M. septicus strains. For each strain, core sequences of atdP, infB, rpoB, and recN genes were selected and concatenated to produce a multilocus sequence or MLS. The MLSs alignment was performed using MAFFT (Katoh and Standley, 2013) in Geneious software (version 2020.0.4), and phylogenetic trees were estimated using RAxML (Stamatakis, 2014). All trees were visualized and edited in FigTree (v1.4.4; http://tree.bio.ed.ac.uk/software/figtree).
Comparative Analysis of Mergibacter Virulence Genes
Twelve putative virulence factors were identified in the genome of M. septicus type strain A25201 (Table 4), including genes encoding: a putative capsule (capsule), outer membrane proteins (ompA, ompH), a cytolethal distending toxin (cdt), iron metabolic proteins (exbD, fur), a superoxide dismutases (sodA), and proteins involved in lipooligosaccharide (LOS) synthesis (galU, galE, lpxA, lpxC, and kdsA). Because virulence factors are essential for establishing an infected host and provide insight into the evolutionary characteristic of bacteria (Parkhill et al., 2003), we performed a comparative analysis of each virulence factor identified in M. septicus A25201 with those of the other Mergibacer and other Pasteurellaceae avian pathogens. M. septicus A25201 presented 1 virulence factor (LOS synthesis lpxA gene) missing in the other isolates of this new genus but present in other Pasteurellaceae (Figures 5, 6). Nine virulence genes (galU, galE, lpxC, lpxA, kdsA, ompA, sodA, kdsA, and capsule) were shared by all Mergibacter members and most of the other Pasteurellaceae genera, and two putative virulence genes rarely found in common Pasteurellaceae avian pathogens, cdt and ompH, were found in all Mergibacer. For most of the shared virulence genes, Mergibacter showed the highest sequence similarity with members of the genus Gallibacterium (Figure 5), and in all resultant phylogenetic trees, it clustered on a different branch, which descended from the same node of Gallibacterium members (Figure 6).
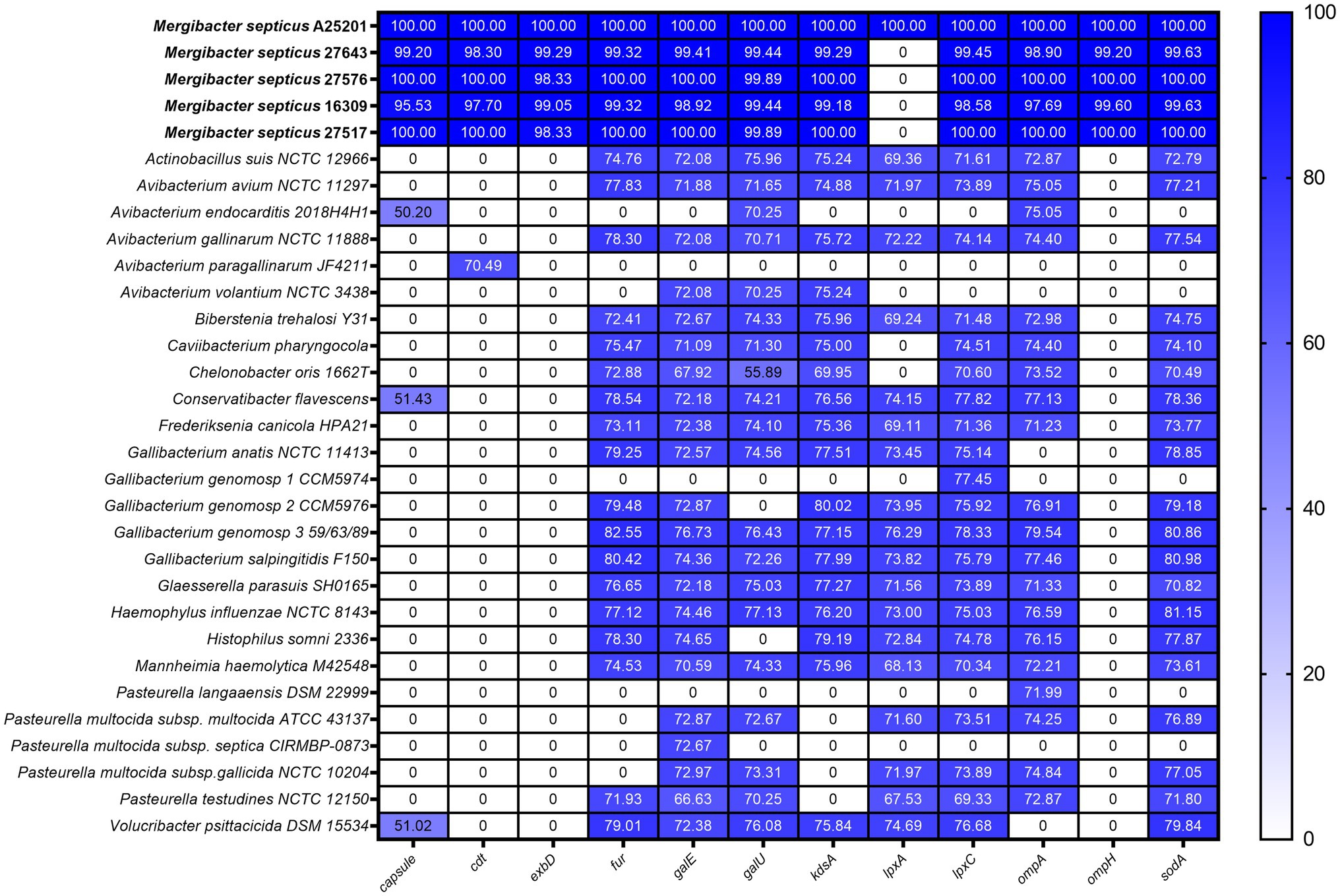
Figure 5. Presence and absence of virulence factors in Mergibacter septicus and other Pasteurellaceae avian pathogens. The heatmap was generated based on the similarity of each virulence gene using GraphPad Prism version 8.4.3. The color key on the right indicates percent gene similarity. Cells in white correspond to absent genes.
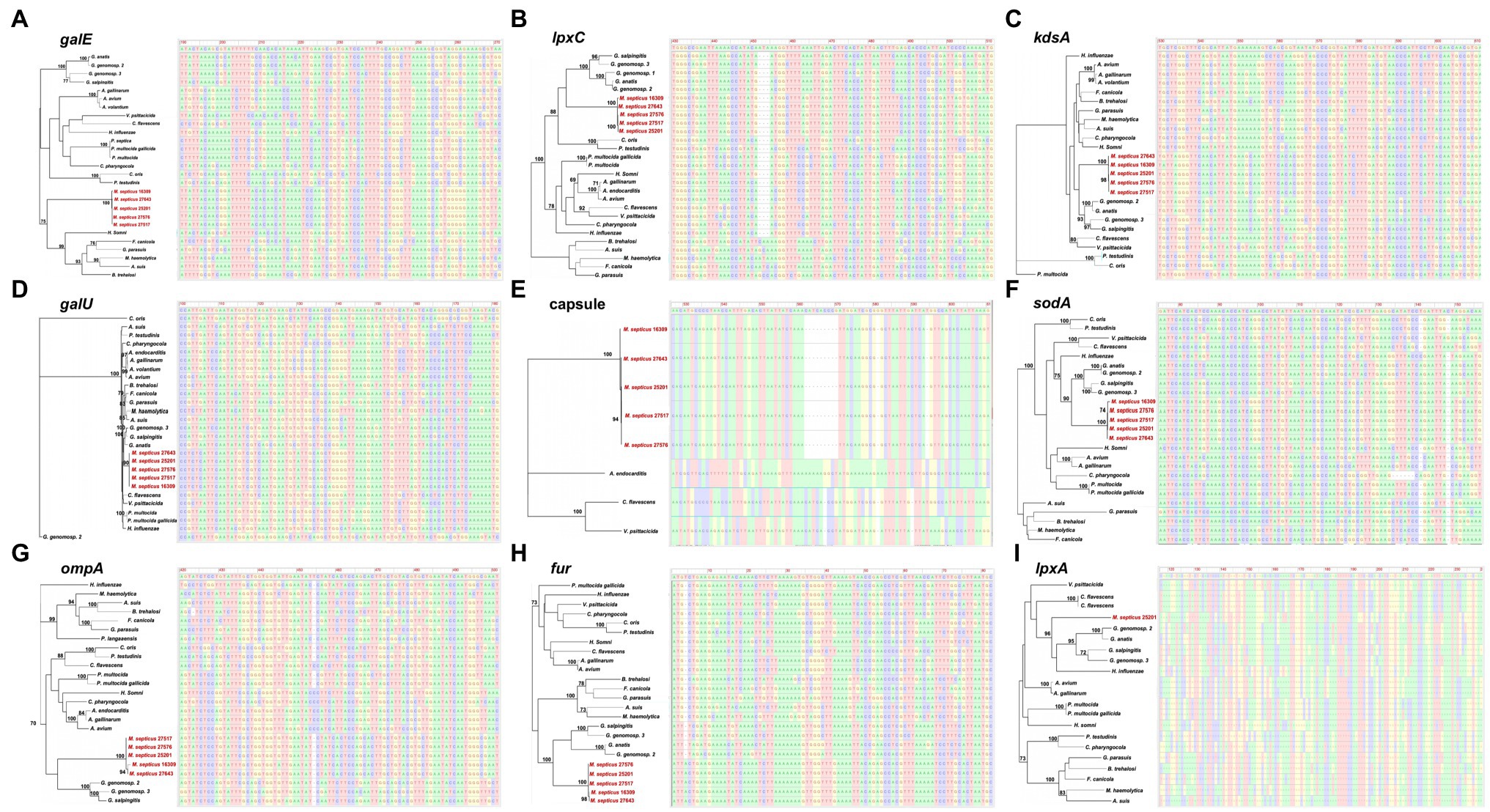
Figure 6. Phylogenetic relationship of virulence factors; galE (A), lpxC (B), kdsA (C), galU (D), capsule (E), sodA (F), ompA (G), fur (H) and lpxA (I); between Mergibacter septicus (in red) and other Pasteurellaceae avian pathogens (in black). A multisequence alignment for each virulence factor was performed with MAFFT (Katoh and Standley, 2013), and phylogenetic relatedness was estimated with RAxML (Stamatakis, 2014). Alignments and phylogenetic trees were visualized in Gingr (v 1.1.1) from the Harvest Package (Treangen et al., 2014).
Mergibacter Antimicrobial Resistance Phenotype and Potential ARGs and ARGs-carrying Plasmids
The antimicrobial resistance phenotype of M. septicus type strain A25201 (DSM 112696; CP054053) was tested in this study. Isolate A25201 presented considerably low MICs for all the drugs tested, including penicillin, amoxicillin, and tetracycline (Table 5). Furthermore, no antimicrobial resistance genes were found in all the five Mergibacter genomes after searching on the ResFinder and ARMFinder databases. Of note, CARD database analysis identified sequence variants (92.6% of nucleotide identity) described in Escherichia coli elongation factor Tu (EF-Tu), conferring resistance to pulvomycin (Zeef et al., 1994). Comparison with phenotype for pulvomycin was not possible as it is not included in commercially available antimicrobial susceptibility panels. No plasmids were identified using Plasmid Finder and COPLA plasmid taxonomic classifier databases.
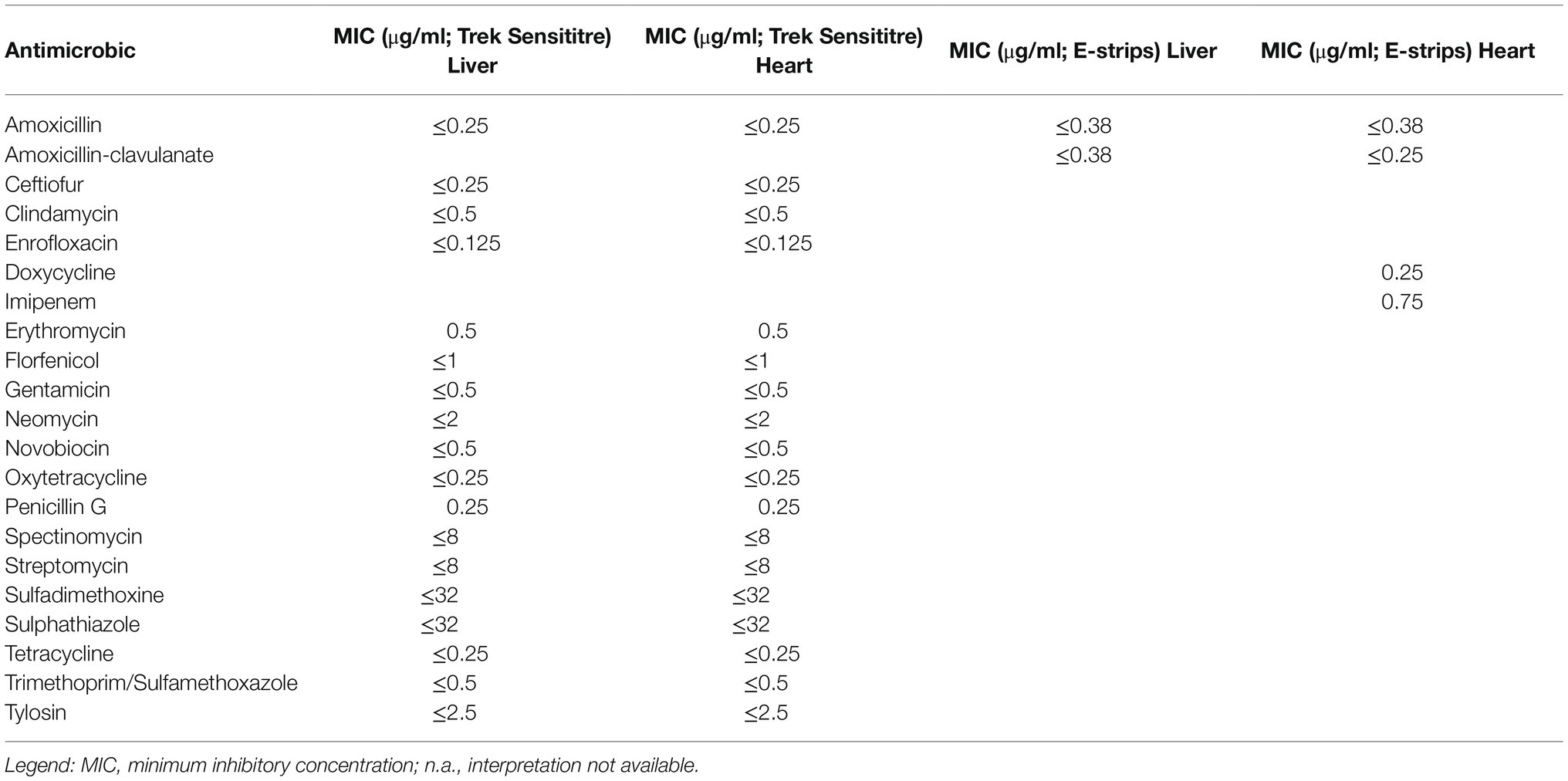
Table 5. Antimicrobial susceptibility profile of Mergibacter septicus strain A25201 against 21 antimicrobials agents using Trek Sensititre and E-strips.
Discussion
Bisgaard taxon 40, initially isolated from respiratory tract lesions of a gull and classified in the Pasteurellaceae family, represents a recently discovered bacterium causing disease in wild birds (Christensen et al., 2003b). In recent years, increasing evidence indicates that Bisgaard taxon 40 is associated with a wide range of pathological changes leading to mass mortality events in wild birds (Knowles et al., 2019). The present study presents a comprehensive phenotypic and genetic characterization of 5 isolates of Bisgaard taxon 40 and a comparative genomic analysis between them and 58 reference members belonging to 31 genera within the Pasteurellaceae family. Our data demonstrated that Bisgaard taxon 40 represents a new genus that we nominated as Mergibacter, with the type species M. septicus and type strain M. septicus A25201 (DSM 112696; CP054053). Additionally, this project identified twelve potential virulence factors putatively involved in Mergibacter pathogenesis.
A new genus of bacteria is primarily recognized by a distinct phenotype that separates it from its neighbors (Christensen et al., 2014). Phenotypical and biochemical characteristics, including catalase, urease, indole, ornithine decarboxylase, phosphatase tests, growth on MacConkey, and carbon source utilization tests, are frequently the most frequently used for genera differentiation within the Pasteurellaceae family (Blackall et al., 2005; Christensen et al., 2007). The five Mergibacter strains studied here shared similar phenotypic characteristics, such as β-hemolysis and a shiny, circular, whitish-cream colony morphology on blood agar. Within the features which separate Mergibacter strains from other Pasteurellaceae genera were the presence of β-hemolysis and acid formation from D-mannitol and sucrose (Christensen et al., 2003a). However, this limited number of phenotypic differences and the high phenotypic divergence presented by the different species of the family made Mergibacter phenotype-based classification very difficult. Furthermore, misidentification based upon phenotypic characterization represents a common problem among taxa of the Pasteurellaceae (Christensen et al., 2007).
The arrival of new genetic-based classification methods for the Pasteurellaceae family (Christensen et al., 2003a) has resulted in the discovery of additional genera and the reclassification of some of the original members in recent years, including Pasteurella, Haemophilus, and Actinobacillus. The availability of these genomes provided the opportunity to complete comprehensive phylogenetic and comparative analyses, which identified molecular signatures, commonly shared by closely related species within the family Pasteurellaceae (Naushad et al., 2015). Here, in addition to the phenotypic characterization, core genome and targeted gene analyses were used to investigate the genetic variation between different Mergibacter isolates and also its classification within the Pasteurellaceae family. High sequence similarities were observed across the five Mergibacter isolates in this study collected from four different bird species, indicating that although genetically similar, Mergibacter can infect a broad range of wild bird species within the Aequorlitornithes clade. Additionally, the five Mergibacter strains consistently clustered together forming a well-separated terminal monophyletic group in all phylogenetic trees built in this study (16S, MLS, core genome, and virulence genes) despite not known common geographic or environmental associations (e.g., eastern and western United States vs. Scotland), reflects the potential spread of this bacteria via bird migration patterns as observed for known viral and bacterial pathogens such as West Nile Disease virus and Salmonella (Jourdain et al., 2007).
Classic molecular taxonomic approaches corroborated the phylogenetic evidence that Bisgaard taxon 40 is a new genus within the Pasteurellaceae family. One of the proposed molecular methods to differentiate bacterial genera is based on the average amino acid identity (AAI) of the conserved genes between subject species (Konstantinidis and Tiedje, 2007), with a range of AAI values (65 to 72%) as the threshold to define a different genus. Mergibacter strains shared 96.7–100% of amino acid identity with each other, placing all the isolates as members of the same genus. The highest AAI values, when Mergibacter was compared with other representative members of the Pasteurellaceae family, were observed with species of the genus Gallibacterium (ranging 67–70%). Thus, the AAI values were below the genus threshold, further supporting the genus identity of Mergibacter. Along with AAI, the percentage of conserved protein (POCP)-based taxonomic test has been demonstrated to be a robust tool for establishing the genus boundary, set to below 50% for most prokaryotic groups (Qin et al., 2014; Barco et al., 2020). POCP values between Mergibacter and its closest genetic species (based on our core genome phylogeny) were above the 50% threshold (i.e., 68.34% with nearest neighbor G. genomosp. 3). However, the POCP values observed between the other Pasteurellaceae members included in the analysis were never lower than 63%, as observed in the POCP study between Chelonobacter and Gallibacterium, suggesting that the 50% threshold may not be reliably applied to the Pasteurellaceae family. An additional investigation involving different genera belonging to the Pasteurellaceae family may be helpful to assess a reliable POCP-based genus boundary value for this specific group of bacterial pathogens. A new taxonomic approach to defining a genus boundary within the Pasteurellaceae family based on an AAI method using only 31 randomly selected CDS was described (Christensen and Bisgaard, 2018). The authors concluded that Pasteurellaceae isolates sharing 88% amino acid pairwise similarity belonged to the same genus, establishing a 88% genus boundary within the Pasteurellaceae family (Christensen and Bisgaard, 2018). In this study, the highest similarity percentage observed between Mergibacter and the other Pasteurellaceae strains was 69.59%, well below the 88% genus boundary value (Christensen and Bisgaard, 2018). Similar to (Christensen and Bisgaard, 2018), an exception was found between Chelonobacter and P. testudinis, which shared values above the 88% threshold. However, they are currently classified into two different genera within the Pasteurellaceae family. These findings indicate that a reclassification for some Pasteurellaceae members may be warranted, as similarly stated by (Christensen and Bisgaard, 2018).
Sequence comparison based on 16S rRNA and housekeeping genes has been deemed suitable for phylogenetic inference due to these genes’ evolutionary conservation and low selection pressure (Christensen et al., 2004). Indeed, within the Pasteurellaceae family, published minimal standards for describing a new genus require a monophyletic grouping based on 16S rRNA gene sequences and a 16S rRNA gene sequence divergence greater than ~5% from other recognized genera (Christensen et al., 2007). Based on the 16S rRNA gene sequence analysis, the five M. septicus strains showed sequence similarities among each other greater than 99.5%, while the highest nucleotide identities that were found between Mergibacer and other Pasteurellaceeae species never went above 92.2%. Additionally, the monophyly of genera within the Pasteurellaceae has been confirmed by sequence comparison of infB and rpoB genes with members of the same genus showing similarity values of 85–88% or higher at the nucleotide sequence level (Christensen et al., 2007). Here, we further investigated the sequence similarity of the Merigbacer strains with an MLS that comprised the core portion of housekeeping genes atpD, infB, recN, and rpoB. Once again, M. septicus showed sequence similarities among each other above 99%, while the highest nucleotide identities found between Mergibacer strains and other Pasteurellaceeae species never reached 85%. This further evidence the correct classification of Mergibacer as a new genus.
The tree’s topology resulting from the MLS-based phylogeny was very similar to the topology of the tree estimated using Pasteurellaceae core genomes, placing genera Mergibacter Gallibacterium Pasteurella, Chelonobacter, Testudinibacter, and Spirabiliibacterium as well-differentiated terminal groups of a more extensive common clade. Surprisingly, this was not observed in the 16S tree where Mergibacter appeared at a considerable genetic distance from the Gallibacterium species. Additionally, the unreliability of the 16S-based taxonomy for this family is also confirmed by the inconsistent clustering of members belonging to the Pasteurella genus observed in the 16S phylogenetic tree and the lack of bootstrap support. These two factors highly improved in the MLS-based phylogenetic analysis; nevertheless, two genera appeared to be misclassified. This indicates that, although not perfect, the four housekeeping genes employed in this study better represent the Pasteurellaceae whole genomes, and their phylogenetic relatedness is more accurately described using our MLS approach than when exclusively using the 16S rRNA gene. Overall, our study highlights that a reclassification for some Pasteurellaceae members is needed using new and more robust taxonomical approaches.
Pathogenicity in Pasteurellaceae species and subspecies is associated with various virulence factors that critically contribute to colonization and invasion of the host (Ewers et al., 2006). Most of the M. septicus strains characterized in this study originated from mortality events of wild birds with evidence of septicemia (Knowles et al., 2019; Niedringhaus et al., 2021). Our in silico genetic characterization revealed that M. septicus carries up to twelve major virulence factors associated with disease in other avian pathogens of the family Pasteurellaceae, including genes that encode a putative capsule (capsule), outer membrane proteins (ompA, ompH), a cytolethal distending toxin (cdt), iron metabolic proteins (exbD, fur), a superoxide dismutases (sodA), and proteins involved in lipooligosaccharide (LOS) synthesis (galU, galE, lpxA, lpxC, kdsA). Indeed, the presence of a capsule gene homolog to the one identified in M. septicus has been correlated to increased virulence in Pasteurella multocida because encapsulated organisms are more resistant to phagocytosis and to the bactericidal activity of complement (Boyce et al., 2000). Similarly, the putative virulence genes found in Margibacter are homologs to outer membrane protein encoding genes ompA and ompH. It has been described that ompA and ompH mediate P. multocida-host cells interaction via heparin and/or fibronectin binding and therefore act as an important invasive factors which might determine the outcome of initial infection (Katoch et al., 2014). Furthermore, the iron metabolism-related genes homologs to the ones found in Mergibacter are associated with the iron uptake from transferrin proteins (Magarinos et al., 1994), while the superoxide dismutase enzymes neutralize toxic levels of reactive oxygen species generated by the host cell, thus contributing to the virulence (Cox et al., 2003). Contrary to previous studies, which have widely demonstrated that adhesins are frequently among pathogenic isolates of Pasteurella multocida, no adhesin genes were identified in Mergibacter strains. Instead, several genes involved in lipooligosaccharide production were detected. Intriguingly, all the strains of this study carried a cdt gene encoding the cytolethal distending toxin proven to cause cell cycle arrest and death in eukaryotic cells (Elwell and Dreyfus, 2000; Lara-Tejero and Galan, 2000). Except for Avibacterium paragallinarum (Chen et al., 2014), M. septicus represents the only Pasteurellaceae member known to carry this virulence factor, indicating a role in disease outbreak. For most of the shared virulence genes, the phylogenetic relationships of Mergibacter with Pasteurellaceae members were investigated, and all the resultant phylogenetic trees performed showed its taxonomic position in a distinct branch within the Pasteurellaceae family (Figure 6), supporting the reclassification of Mergibacter as a novel genus of this family.
Antibiotic therapy is an effective tool in treating infections caused by Pasteurellaceae in birds; however, an increased occurrence of multidrug-resistant strains has been widely described in the past decade (Furian et al., 2016). In this study, the Mergibacter type strain M. septicus A25201 demonstrated low MICs for all the antibiotics tested, and no ARGs or ARGs-carrying plasmids were observed in the Mergibacter genome. Additional antimicrobial susceptibility data and clinical outcomes from treatments of seabirds would be helpful to implement specific antimicrobial susceptibility recommendations, as no specific clinical breakpoints are currently in place.
The major limitation of this study is the small number of isolates investigated. Evaluation of a more significant number of isolates may be helpful to examine whether diversity in virulence factors is associated with disease prevalence and severity and ascertain the taxonomic position of this bacterium. Additionally, for the type strain A25201 (DSM 112696; CP054053), we used a combination of short and long-read sequencing as an assembly approach, which is considered the method of choice for assembling plasmids (Margos et al., 2017). However, the step of lysing bacterial cells represents a critical phase in DNA extraction, and the quality of plasmid DNA recovered is primarily determined in this step (Zhu et al., 2006). Because we did not use a specific plasmid extraction kit, the loss and/or fragmentation of plasmids carrying antimicrobial resistance genes and/or virulence factors during the DNA extraction process cannot be ruled out.
Conclusion
We describe the first whole-genome sequence characterization for Bisgaard taxon 40 strains implicated in avian disease and mortality events. Our results provide a better understanding of the phylogenetic relationships among the Pasteurellaceae. They indicate that Bisgaard taxon 40, although closely related to the Gallibacterium genus, warrants classification as a new genus within the Pasteurellaceae family, and it was renamed as Mergibacter with the type species M. septicus A25201 (DSM 112696; CP054053). A25201 demonstrated considerably low MICs for all the drugs tested, including penicillin, amoxicillin, and tetracycline, which may represent potential therapeutic suggestions. Furthermore, this study provides insights into the pathogenic potential of M. septicus, highlighting that virulence factors involved in the adhesion and colonization of the host, as well as the cdt toxin, may have played a significant role in the disease development in several seabird species and contributed to mass mortality events. This study provides a genomic characterization and comparative analysis of this bacterium, highlighting the importance of advanced biotechnologies, such as whole-genome sequencing, as an essential tool to characterize and unravel the complex phylogeny of the Pasteurellaceae members. We also demonstrated the usefulness of MLS-based phylogenetic analysis as a reliable approach to distinguish Mergibacter from other genera of the Pasteurellaceae family. Although 16S RNA-based taxonomy is sufficient to separate Mergibacter from other Pasteurellaceae genera, this study provides insights into the limitations of this method to accurately classify the Pasteurellaceae. Our novel MLS method appears to be a cost-effective alternative to 16S rRNA, especially in case of limited access to whole-genome sequencing resources. Given Mergibacter ability to infect avian species and its association with large-scale mortality events, we posit that the public health community should maintain vigilance against and awareness of the unusual clinical, pathological, and microbiological presentation of this microorganism.
Protologue
Description of Mergibacter gen. nov.
Mergibacter (Mer.gi.bac’ter. L. masc. n. mergus a seabird; N.L. masc. n. bacter a rod; N.L. masc. n. Mergibacter a rod of a seabird). Mergibacter is a new genus within the Pasteurellaceae family. The cells are Gram-negative staining rods, and no flagella or pili have been observed. Mergibacter was isolated on 5% sheep blood agar after 48h of aerobic incubation at 42°C, while no growth was observed on MacConkey. The colonies were β-hemolytic, shiny, circular, and whitish-cream. Positive reactions were observed for oxidase, catalase; negative tests were observed for urease and indole. Acid was formed from maltose and dextrin but not from D-mannitol, L-fucose, sucrose, and trehalose. A comparison of phenotypic characters separating the genus Mergibacter from the other Pasteurellaceae is given in Table 2. Mergibacter septicus is the type species of the genus.
Description of Mergibacter septicum sp.nov.
Mergibacter septicus (sep’ti.cus. L. masc. Adj. septicus septic). In addition to characteristics included in the genus description, acid is formed from D-sorbitol and D-galactose. Most of the strains, including the type strain, are able to produce acid from D-mannose. Isolates have mainly been obtained from internal organs, such as the heart, lung, spleen, and brain.
The type strain is Mergibacter septicus A25201T (DSM 112696), isolated from the heart and liver of one common tern (Sterna hirundo) from a mortality event in Florida, United States. In addition to the characteristics described above, using GEN III Microbial ID acid was formed from D-maltose, D-cellobiose, N-acetyl-D-Glucosamine, N-acetyl-β-D-Mannosamine, N-Acetyl-Neuraminic acid, D-Glucose, D-Mannose, D-Sorbitol, D-Glucose6-PO4, D-Fructose6-PO4, and acetoacetic acid. The genome of the type strain is 1,887,770bp long with a G+C content of 36.4%. The genome sequence is deposited in GenBank under the accession number CP054053.1.
Deposit in Culture Collections
The type strain A25201T (Mergibacter septicus sp.; nov.) has been deposited in the DSMZ (German collection of microorganisms and cell cultures) under the number DSM 112696. In addition deposit at ATCC is in progress, AcqID-003043.
Data Availability Statement
The datasets presented in this study can be found in online repositories. The names of the repository/repositories and accession number(s) can be found in the article/Supplementary Material.
Author Contributions
EL: formal analysis, investigation, methodology, bioinformatic analysis, writing-original draft, and writing-review and editing. SÁ-N: formal analysis, investigation, bioinformatic analysis, and writing-review and editing. GM: formal analysis, investigation, methodology, and writing-review and editing. RB: bioinformatic analysis and writing-review and editing. NN: writing-review and editing. KN: writing-review and editing. JLa: formal analysis, methodology, supervision, and writing-review and editing. JLo: methodology and writing-review and editing. GK: investigation and writing-review and editing. SL: formal analysis and writing-review and editing. GP: funding acquisition, supervision and writing-review and editing. SS: conceptualization, methodology, funding acquisition, investigation, resources, supervision, and writing-review and editing. All authors contributed to the article and approved the submitted version.
Funding
This work was funded by the Athens Veterinary Diagnostic Laboratory, Department of Infectious Diseases, University of Georgia, Georgia, United States of America and a grant from the FDA to SS 5U18FD006157. EL was supported by the College of Veterinary Medicine at the University of Georgia and Boehringer Ingelheim. The use of trade, firm, or product names is for descriptive purposes only and does not imply endorsement by the U.S. Government. The work was funded in part by the Defense Threat Reduction Agency.
Conflict of Interest
The authors declare that the research was conducted in the absence of any commercial or financial relationships that could be construed as a potential conflict of interest.
Publisher’s Note
All claims expressed in this article are solely those of the authors and do not necessarily represent those of their affiliated organizations, or those of the publisher, the editors and the reviewers. Any product that may be evaluated in this article, or claim that may be made by its manufacturer, is not guaranteed or endorsed by the publisher.
Supplementary Material
The Supplementary Material for this article can be found online at: https://www.frontiersin.org/articles/10.3389/fmicb.2021.667356/full#supplementary-material
Acknowledgments
We would like to acknowledge the Bacteriology Section of the Athens Veterinary Diagnostic Laboratory (AVDL), especially Amy McKinney, for her outstanding bacteriology skills. Paula Bartlett is recognized for her exceptional guidance throughout the next-generation sequencing and constructive criticism of the manuscript. We would like to thank Roberta Di Paolo (Södertörn University, Stockholm, Sweden) and Vittorio Dell’Aquila (University of Milan, Italy) for their excellent assistance in the bacterial nomenclature. The content of this publication does not necessarily reflect the views or policies of the U.S. Department of Defense or U.S. Department of the Army. Still, it does represent the views of the U.S. geological survey.
Footnotes
1. ^https://github.com/rrwick/Porechop
2. ^http://tree.bio.ed.ac.uk/software/figtree
3. ^https://www.ncbi.nlm.nih.gov/
4. ^https://card.mcmaster.ca/analyze/rgi
5. ^https://cge.cbs.dtu.dk/services/ResFinder/
6. ^https://www.ncbi.nlm.nih.gov/pathogens/antimicrobial-resistance/AMRFinder/
References
Adhikary, S., Bisgaard, M., Nicklas, W., and Christensen, H. (2018). Reclassification of Bisgaard taxon 5 as Caviibacterium pharyngocola gen. nov., sp. nov. and Bisgaard taxon 7 as Conservatibacter flavescens gen. nov., sp. nov. Int. J. Syst. Evol. Microbiol. 68, 643–650. doi: 10.1099/ijsem.0.002558
Alikhan, N. F., Petty, N. K., Ben Zakour, N. L., and Beatson, S. A. (2011). BLAST ring image generator (BRIG): simple prokaryote genome comparisons. BMC Genomics 12:402. doi: 10.1186/1471-2164-12-402
Altschul, S. F., Madden, T. L., Schaffer, A. A., Zhang, J., Zhang, Z., Miller, W., et al. (1997). Gapped BLAST and PSI-BLAST: a new generation of protein database search programs. Nucleic Acids Res. 25, 3389–3402. doi: 10.1093/nar/25.17.3389
Angen, O., Ahrens, P., Kuhnert, P., Christensen, H., and Mutters, R. (2003). Proposal of Histophilus somni gen. nov., sp. nov. for the three species incertae sedis ‘Haemophilus somnus’, ‘Haemophilus agni’ and ‘Histophilus ovis’. Int. J. Syst. Evol. Microbiol. 53, 1449–1456. doi: 10.1099/ijs.0.02637-0
Angen, O., Mutters, R., Caugant, D. A., Olsen, J. E., and Bisgaard, M. (1999). Taxonomic relationships of the [Pasteurella] haemolytica complex as evaluated by DNA-DNA hybridizations and 16S rRNA sequencing with proposal of Mannheimia haemolytica gen. nov., comb. nov., Mannheimia granulomatis comb. nov., Mannheimia glucosida sp. nov., Mannheimia ruminalis sp. nov. and Mannheimia varigena sp. nov. Int. J. Syst. Bacteriol. 49, 67–86. doi: 10.1099/00207713-49-1-67
Barbier, F. F., Chabikwa, T. G., Ahsan, M. U., Cook, S. E., Powell, R., Tanurdzic, M., et al. (2019). A phenol/chloroform-free method to extract nucleic acids from recalcitrant, woody tropical species for gene expression and sequencing. Plant Meth. 15:62. doi: 10.1186/s13007-019-0447-3
Barco, R. A., Garrity, G. M., Scott, J. J., Amend, J. P., Nealson, K. H., and Emerson, D. (2020). A genus definition for bacteria and archaea based on a standard genome relatedness index. MBio 11:e02475-19. doi: 10.1128/mBio.02475-19
Bisgaard, M., and Christensen, H. (2019). Classification of Bisgaard’s taxa 14 and 32 and a taxon from kestrels demonstrating satellitic growth and proposal of Spirabiliibacterium gen. nov., including the description of three species: Spirabiliibacterium mucosae sp. nov., Spirabiliibacterium pneumoniae sp. nov. and Spirabiliibacterium falconis sp. nov. Int. J. Syst. Evol. Microbiol. 71. doi: 10.1099/ijsem.0.004758
Bisgaard, M., and Mutters, R. (1986). A new facultatively anaerobic gram-negative fermentative rod obtained from different pathological lesions in poultry and tentatively designated taxon 14. Avian Pathol. 15, 117–127. doi: 10.1080/03079458608436271
Blackall, P. J., Bojesen, A. M., Christensen, H., and Bisgaard, M. (2007). Reclassification of [Pasteurella] trehalosi as Bibersteinia trehalosi gen. nov., comb. nov. Int. J. Syst. Evol. Microbiol. 57, 666–674. doi: 10.1099/ijs.0.64521-0
Blackall, P. J., Christensen, H., Beckenham, T., Blackall, L. L., and Bisgaard, M. (2005). Reclassification of Pasteurella gallinarum, [Haemophilus] paragallinarum, Pasteurella avium and Pasteurella volantium as Avibacterium gallinarum gen. nov., comb. nov., Avibacterium paragallinarum comb. nov., Avibacterium avium comb. nov. and Avibacterium volantium comb. nov. Int. J. Syst. Evol. Microbiol. 55, 353–362. doi: 10.1099/ijs.0.63357-0
Bojesen, M., Christensen, J. P., and Bisgaard, M. (2008). “Gallibacterium infections and other avian Pasteurellaceae,” in Poultry Diseases. 6th Edn. eds. M. Pattison, P. F. McMullin, J. M. Bradbury, and D. J. Alexander (London: Elsevier).
Bolger, A. M., Lohse, M., and Usadel, B. (2014). Trimmomatic: a flexible trimmer for Illumina sequence data. Bioinformatics 30, 2114–2120. doi: 10.1093/bioinformatics/btu170
Boot, R., and Bisgaard, M. (1995). Reclassification of 30 Pasteurellaceae strains isolated from rodents. Lab. Anim. 29, 314–319. doi: 10.1258/002367795781088342
Boyce, J. D., Chung, J. Y., and Adler, B. (2000). Pasteurella multocida capsule: composition, function and genetics. J. Biotechnol. 83, 153–160. doi: 10.1016/S0168-1656(00)00309-6
Carver, T., Harris, S. R., Berriman, M., Parkhill, J., and Mcquillan, J. A. (2012). Artemis: an integrated platform for visualization and analysis of high-throughput sequence-based experimental data. Bioinformatics 28, 464–469. doi: 10.1093/bioinformatics/btr703
Chen, Y. C., Tan, D. H., Shien, J. H., Hsieh, M. K., Yen, T. Y., and Chang, P. C. (2014). Identification and functional analysis of the cytolethal distending toxin gene from Avibacterium paragallinarum. Avian Pathol. 43, 43–50. doi: 10.1080/03079457.2013.861895
Chen, L., Yang, J., Yu, J., Yao, Z., Sun, L., Shen, Y., et al. (2005). VFDB: a reference database for bacterial virulence factors. Nucleic Acids Res. 33, D325–D328. doi: 10.1093/nar/gki008
Chin, C. S., Alexander, D. H., Marks, P., Klammer, A. A., Drake, J., Heiner, C., et al. (2013). Nonhybrid, finished microbial genome assemblies from long-read SMRT sequencing data. Nat. Methods 10, 563–569. doi: 10.1038/nmeth.2474
Christensen, J. P., and Bisgaard, M. (2000). Fowl cholera. Rev. Sci. Tech. 19, 626–637. doi: 10.20506/rst.19.2.1236
Christensen, H., and Bisgaard, M. (2018). Classification of genera of Pasteurellaceae using conserved predicted protein sequences. Int. J. Syst. Evol. Microbiol. 68, 2692–2696. doi: 10.1099/ijsem.0.002860
Christensen, H., Bisgaard, M., Bojesen, A. M., Mutters, R., and Olsen, J. E. (2003a). Genetic relationships among avian isolates classified as Pasteurella haemolytica, ‘Actinobacillus salpingitidis’ or Pasteurella anatis with proposal of Gallibacterium anatis gen. nov., comb. nov. and description of additional genomospecies within Gallibacterium gen. nov. Int. J. Syst. Evol. Microbiol. 53, 275–287. doi: 10.1099/ijs.0.02330-0
Christensen, H., Foster, G., Christensen, J. P., Pennycott, T., Olsen, J. E., and Bisgaard, M. (2003b). Phylogenetic analysis by 16S rDNA gene sequence comparison of avian taxa of Bisgaard and characterization and description of two new taxa of Pasteurellaceae. J. Appl. Microbiol. 95, 354–363. doi: 10.1046/j.1365-2672.2003.01986.x
Christensen, H., Kuhnert, P., Busse, H. J., Frederiksen, W. C., and Bisgaard, M. (2007). Proposed minimal standards for the description of genera, species and subspecies of the Pasteurellaceae. Int. J. Syst. Evol. Microbiol. 57, 166–178. doi: 10.1099/ijs.0.64838-0
Christensen, H., Kuhnert, P., Nørskov-Lauritsen, N., Planet, P., and Bisgaard, M. (2014). “The family Pasteurellaceae,” in The Prokaryotes. eds. E. F. DeLong, S. Lory, E. Stackebrandt, and F. Thompson (Germany, Berlin: Springer), 535–564.
Christensen, H., Kuhnert, P., Olsen, J. E., and Bisgaard, M. (2004). Comparative phylogenies of the housekeeping genes atpD, infB and rpoB and the 16S rRNA gene within the Pasteurellaceae. Int. J. Syst. Evol. Microbiol. 54, 1601–1609. doi: 10.1099/ijs.0.03018-0
Cox, G. M., Harrison, T. S., Mcdade, H. C., Taborda, C. P., Heinrich, G., Casadevall, A., et al. (2003). Superoxide dismutase influences the virulence of Cryptococcus neoformans by affecting growth within macrophages. Infect. Immun. 71, 173–180. doi: 10.1128/IAI.71.1.173-180.2003
Darling, A. C., Mau, B., Blattner, F. R., and Perna, N. T. (2004). Mauve: multiple alignment of conserved genomic sequence with rearrangements. Genome Res. 14, 1394–1403. doi: 10.1101/gr.2289704
Dickerman, A., Bandara, A. B., and Inzana, T. J. (2020). Phylogenomic analysis of Haemophilus parasuis and proposed reclassification to Glaesserella parasuis, gen. nov., comb. nov. Int. J. Syst. Evol. Microbiol. 70, 180–186. doi: 10.1099/ijsem.0.003730
Edgar, R. C. (2004). MUSCLE: multiple sequence alignment with high accuracy and high throughput. Nucleic Acids Res. 32, 1792–1797. doi: 10.1093/nar/gkh340
Eid, S., Marouf, S., Hefny, H. Y., and Al-Atfeehy, N. M. (2019). Pasteurellaceae members with similar morphological patterns associated with respiratory manifestations in ducks. Vet World 12, 2061–2069. doi: 10.14202/vetworld.2019.2061-2069
Elwell, C. A., and Dreyfus, L. A. (2000). DNase I homologous residues in CdtB are critical for cytolethal distending toxin-mediated cell cycle arrest. Mol. Microbiol. 37, 952–963. doi: 10.1046/j.1365-2958.2000.02070.x
Ewers, C., Lubke-Becker, A., Bethe, A., Kiebling, S., Filter, M., and Wieler, L. H. (2006). Virulence genotype of Pasteurella multocida strains isolated from different hosts with various disease status. Vet. Microbiol. 114, 304–317. doi: 10.1016/j.vetmic.2005.12.012
Furian, T. Q., Borges, K. A., Laviniki, V., Rocha, S. L., De Almeida, C. N., Do Nascimento, V. P., et al. (2016). Virulence genes and antimicrobial resistance of Pasteurella multocida isolated from poultry and swine. Braz. J. Microbiol. 47, 210–216. doi: 10.1016/j.bjm.2015.11.014
Gregersen, R. H., Neubauer, C., Christensen, H., Bojesen, A. M., Hess, M., and Bisgaard, M. (2009). Comparative studies on [Pasteurella] testudinis and [P.] testudinis-like bacteria and proposal of Chelonobacter oris gen. nov., sp. nov. as a new member of the family Pasteurellaceae. Int. J. Syst. Evol. Microbiol. 59, 1583–1588. doi: 10.1099/ijs.0.007203-0
Gurevich, A., Saveliev, V., Vyahhi, N., and Tesler, G. (2013). QUAST: quality assessment tool for genome assemblies. Bioinformatics 29, 1072–1075. doi: 10.1093/bioinformatics/btt086
Haft, D. H., Dicuccio, M., Badretdin, A., Brover, V., Chetvernin, V., O’neill, K., et al. (2018). RefSeq: an update on prokaryotic genome annotation and curation. Nucleic Acids Res. 46, D851–D860. doi: 10.1093/nar/gkx1068
Hunt, M., Silva, N. D., Otto, T. D., Parkhill, J., Keane, J. A., and Harris, S. R. (2015). Circlator: automated circularization of genome assemblies using long sequencing reads. Genome Biol. 16:294. doi: 10.1186/s13059-015-0849-0
Jourdain, E., Gauthier-Clerc, M., Bicout, D. J., and Sabatier, P. (2007). Bird migration routes and risk for pathogen dispersion into western Mediterranean wetlands. Emerg. Infect. Dis. 13, 365–372. doi: 10.3201/eid1303.060301
Kainz, A., Lubitz, W., and Busse, H. J. (2000). Genomic fingerprints, ARDRA profiles and quinone systems for classification of Pasteurella sensu stricto. Syst. Appl. Microbiol. 23, 494–503. doi: 10.1016/S0723-2020(00)80023-5
Katoch, S., Sharma, M., Patil, R. D., Kumar, S., and Verma, S. (2014). In vitro and in vivo pathogenicity studies of Pasteurella multocida strains harbouring different ompA. Vet. Res. Commun. 38, 183–191. doi: 10.1007/s11259-014-9601-6
Katoh, K., and Standley, D. M. (2013). MAFFT multiple sequence alignment software version 7: improvements in performance and usability. Mol. Biol. Evol. 30, 772–780. doi: 10.1093/molbev/mst010
Khamesipour, F., Momtaz, H., and Azhdary Mamoreh, M. (2014). Occurrence of virulence factors and antimicrobial resistance in Pasteurella multocida strains isolated from slaughter cattle in Iran. Front. Microbiol. 5:536. doi: 10.3389/fmicb.2014.00536
Knowles, S., Bodenstein, B. L., Berlowski-Zier, B. M., Thomas, S. M., Pearson, S. F., and Lorch, J. M. (2019). Detection of Bisgaard taxon 40 in rhinoceros auklets (Cerorhinca monocerata) with pneumonia and septicemia from a mortality event in Washington, USA. J. Wildl. Dis. 55, 246–249. doi: 10.7589/2017-12-309
Kolmogorov, M., Yuan, J., Lin, Y., and Pevzner, P. A. (2019). Assembly of long, error-prone reads using repeat graphs. Nat. Biotechnol. 37, 540–546. doi: 10.1038/s41587-019-0072-8
Konstantinidis, K. T., and Tiedje, J. M. (2007). Prokaryotic taxonomy and phylogeny in the genomic era: advancements and challenges ahead. Curr. Opin. Microbiol. 10, 504–509. doi: 10.1016/j.mib.2007.08.006
Korczak, B. M., Bisgaard, M., Christensen, H., and Kuhnert, P. (2014). Frederiksenia canicola gen. nov., sp. nov. isolated from dogs and human dog-bite wounds. Antonie Van Leeuwenhoek 105, 731–741. doi: 10.1007/s10482-014-0129-0
Krumsiek, J., Arnold, R., and Rattei, T. (2007). Gepard: a rapid and sensitive tool for creating dotplots on genome scale. Bioinformatics 23, 1026–1028. doi: 10.1093/bioinformatics/btm039
Lara-Tejero, M., and Galan, J. E. (2000). A bacterial toxin that controls cell cycle progression as a deoxyribonuclease I-like protein. Science 290, 354–357. doi: 10.1126/science.290.5490.354
Li, H., and Durbin, R. (2009). Fast and accurate short read alignment with burrows-wheeler transform. Bioinformatics 25, 1754–1760. doi: 10.1093/bioinformatics/btp324
Lin, Y., Yuan, J., Kolmogorov, M., Shen, M. W., Chaisson, M., and Pevzner, P. A. (2016). Assembly of long error-prone reads using de Bruijn graphs. Proc. Natl. Acad. Sci. U. S. A. 113, E8396–E8405. doi: 10.1073/pnas.1604560113
Magarinos, B., Romalde, J. L., Lemos, M. L., Barja, J. L., and Toranzo, A. E. (1994). Iron uptake by Pasteurella piscicida and its role in pathogenicity for fish. Appl. Environ. Microbiol. 60, 2990–2998. doi: 10.1128/aem.60.8.2990-2998.1994
Margos, G., Hepner, S., Mang, C., Marosevic, D., Reynolds, S. E., Krebs, S., et al. (2017). Lost in plasmids: next generation sequencing and the complex genome of the tick-borne pathogen Borrelia burgdorferi. BMC Genomics 18:422. doi: 10.1186/s12864-017-3804-5
Narasinakuppe Krishnegowda, D., Dhama, K., Kumar Mariappan, A., Munuswamy, P., Iqbal Yatoo, M., Tiwari, R., et al. (2020). Etiology, epidemiology, pathology, and advances in diagnosis, vaccine development, and treatment of Gallibacterium anatis infection in poultry: a review. Vet. Q. 40, 16–34. doi: 10.1080/01652176.2020.1712495
Naushad, S., Adeolu, M., Goel, N., Khadka, B., Al-Dahwi, A., and Gupta, R. S. (2015). Phylogenomic and molecular demarcation of the core members of the polyphyletic pasteurellaceae genera actinobacillus, haemophilus, and pasteurella. Int. J. Genom. 2015:198560. doi: 10.1155/2015/198560
Niedringhaus, K. D., Shender, L. A., Dinuovo, A., Flewelling, L. J., Maboni, G., Sanchez, S., et al. (2021). Mortality in common (Sterna hirundo) and Sandwich terns (Thalasseus sandvicensis) associated with Bisgaard taxon 40 infection on Marco Island, Florida, USA. J. Comp. Pathol. 184, 12–18. doi: 10.1016/j.jcpa.2021.01.009
Norskov-Lauritsen, N., Bruun, B., and Kilian, M. (2005). Multilocus sequence phylogenetic study of the genus Haemophilus with description of Haemophilus pittmaniae sp. nov. Int. J. Syst. Evol. Microbiol. 55, 449–456. doi: 10.1099/ijs.0.63325-0
Norskov-Lauritsen, N., and Kilian, M. (2006). Reclassification of Actinobacillus actinomycetemcomitans, Haemophilus aphrophilus, Haemophilus paraphrophilus and Haemophilus segnis as Aggregatibacter actinomycetemcomitans gen. nov., comb. nov., Aggregatibacter aphrophilus comb. nov. and Aggregatibacter segnis comb. nov., and emended description of Aggregatibacter aphrophilus to include V factor-dependent and V factor-independent isolates. Int. J. Syst. Evol. Microbiol. 56, 2135–2146. doi: 10.1099/ijs.0.64207-0
Parkhill, J., Sebaihia, M., Preston, A., Murphy, L. D., Thomson, N., Harris, D. E., et al. (2003). Comparative analysis of the genome sequences of Bordetella pertussis, Bordetella parapertussis and Bordetella bronchiseptica. Nat. Genet. 35, 32–40. doi: 10.1038/ng1227
Qin, Q. L., Xie, B. B., Zhang, X. Y., Chen, X. L., Zhou, B. C., Zhou, J., et al. (2014). A proposed genus boundary for the prokaryotes based on genomic insights. J. Bacteriol. 196, 2210–2215. doi: 10.1128/JB.01688-14
Richter, M., and Rossello-Mora, R. (2009). Shifting the genomic gold standard for the prokaryotic species definition. Proc. Natl. Acad. Sci. U. S. A. 106, 19126–19131. doi: 10.1073/pnas.0906412106
Richter, M., Rossello-Mora, R., Oliver Glockner, F., and Peplies, J. (2016). JSpeciesWS: a web server for prokaryotic species circumscription based on pairwise genome comparison. Bioinformatics 32, 929–931. doi: 10.1093/bioinformatics/btv681
Sangal, V., Goodfellow, M., Jones, A. L., Schwalbe, E. C., Blom, J., Hoskisson, P. A., et al. (2016). Next-generation systematics: An innovative approach to resolve the structure of complex prokaryotic taxa. Sci. Rep. 6:38392. doi: 10.1038/srep38392
Shearn-Bochsler, V., Schulz, J. L., Dobbs, R. C., Lorch, J. M., Waddle, J. H., and Grear, D. A. (2018). Novel Dermatophilosis and concurrent amyloidosis in sanderlings (Calidris Alba) from Louisiana, USA. J. Wildl. Dis. 54, 189–192. doi: 10.7589/2017-04-078
Stamatakis, A. (2014). RAxML version 8: a tool for phylogenetic analysis and post-analysis of large phylogenies. Bioinformatics 30, 1312–1313. doi: 10.1093/bioinformatics/btu033
Tatusova, T., Dicuccio, M., Badretdin, A., Chetvernin, V., Nawrocki, E. P., Zaslavsky, L., et al. (2016). NCBI prokaryotic genome annotation pipeline. Nucleic Acids Res. 44, 6614–6624. doi: 10.1093/nar/gkw569
Treangen, T. J., Ondov, B. D., Koren, S., and Phillippy, A. M. (2014). The harvest suite for rapid core-genome alignment and visualization of thousands of intraspecific microbial genomes. Genome Biol. 15:524. doi: 10.1186/s13059-014-0524-x
Walker, B. J., Abeel, T., Shea, T., Priest, M., Abouelliel, A., Sakthikumar, S., et al. (2014). Pilon: an integrated tool for comprehensive microbial variant detection and genome assembly improvement. PLoS One 9:e112963. doi: 10.1371/journal.pone.0112963
Wingett, S. W., and Andrews, S. (2018). FastQ screen: A tool for multi-genome mapping and quality control. F1000Res 7:1338. doi: 10.12688/f1000research.15931.1
Zankari, E., Hasman, H., Cosentino, S., Vestergaard, M., Rasmussen, S., Lund, O., et al. (2012). Identification of acquired antimicrobial resistance genes. J. Antimicrob. Chemother. 67, 2640–2644. doi: 10.1093/jac/dks261
Zeef, L. A., Bosch, L., Anborgh, P. H., Cetin, R., Parmeggiani, A., and Hilgenfeld, R. (1994). Pulvomycin-resistant mutants of E.coli elongation factor Tu. EMBO J. 13, 5113–5120. doi: 10.1002/j.1460-2075.1994.tb06840.x
Keywords: bisgaard taxon 40, Pasteurellaceae, genomic analysis, evolution, virulence factors, phylogeny, Mergibacter septicus, seabirds
Citation: De Luca E, Álvarez-Narváez S, Maboni G, Baptista RP, Nemeth NM, Niedringhaus KD, Ladner JT, Lorch JM, Koroleva G, Lovett S, Palacios GF and Sanchez S (2021) Comparative Genomics Analyses Support the Reclassification of Bisgaard Taxon 40 as Mergibacter gen. nov., With Mergibacter septicus sp. nov. as Type Species: Novel Insights Into the Phylogeny and Virulence Factors of a Pasteurellaceae Family Member Associated With Mortality Events in Seabirds. Front. Microbiol. 12:667356. doi: 10.3389/fmicb.2021.667356
Edited by:
Iain Sutcliffe, Northumbria University, United KingdomReviewed by:
Paul Alan Lawson, University of Oklahoma, United StatesMarike Palmer, University of Nevada, Las Vegas, United States
Copyright © 2021 De Luca, Álvarez-Narváez, Maboni, Baptista, Nemeth, Niedringhaus, Ladner, Lorch, Koroleva, Lovett, Palacios and Sanchez. This is an open-access article distributed under the terms of the Creative Commons Attribution License (CC BY). The use, distribution or reproduction in other forums is permitted, provided the original author(s) and the copyright owner(s) are credited and that the original publication in this journal is cited, in accordance with accepted academic practice. No use, distribution or reproduction is permitted which does not comply with these terms.
*Correspondence: Eliana De Luca, ZWxpYW5hZGVsdWNhOUBnbWFpbC5jb20=
†Present address: Jeffrey M. Lorch, The Pathogen and Microbiome Institute, Northern Arizona University, Flagstaff, AZ, United States