- 1Jena University Hospital, Institute of General Practice and Family Medicine, Jena, Germany
- 2Unit 37: Nosocomial Infections, Surveillance of Antimicrobial Resistance and Consumption, Robert Koch Institute, Berlin, Germany
- 3Division Nosocomial Pathogens and Antibiotic Resistances, Department of Infectious Diseases, National Reference Centre for Staphylococci and Enterococci, Robert Koch Institute, Wernigerode, Germany
Vancomycin-resistant Enterococcus faecium (VREF) and methicillin-resistant Staphylococcus aureus (MRSA) are associated with significant health burden. We investigated linezolid and daptomycin resistance among VREF and MRSA in the EU/EEA between 2014 and 2018. Descriptive statistics and multivariable logistic regression were used to analyze 6,949 VREF and 35,131 MRSA blood isolates from patients with bloodstream infection. The population-weighted mean proportion of linezolid resistance in VREF and MRSA between 2014 and 2018 was 1.6% (95% CI 1.33–2.03%) and 0.28% (95% CI 0.32–0.38%), respectively. Daptomycin resistance in MRSA isolates was similarly low [1.1% (95% CI 0.75–1.6%)]. On the European level, there was no temporal change of daptomycin and linezolid resistance in MRSA and VREF. Multivariable regression analyses showed that there was a higher likelihood of linezolid and daptomycin resistance in MRSA (aOR: 2.74, p < 0.001; aOR: 2.25, p < 0.001) and linezolid in VREF (aOR: 1.99, p < 0.001) compared to their sensitive isolates. The low proportion of linezolid and daptomycin resistance in VREF and MRSA suggests that these last-resort antibiotics remain effective and will continue to play an important role in the clinical management of these infections in Europe. However, regional and national efforts to contain antimicrobial resistance should continue to monitor the trend through strengthened surveillance that includes genomic surveillance for early warning and action.
Introduction
Staphylococcus aureus and Enterococcus faecium are Gram-positive pathogens that frequently colonize the skin, nostrils, and gut of humans with potential for invasive infections in humans (Fisher and Phillips, 2009). They are the most important Gram-positive organisms causing nosocomial infections especially bloodstream and other invasive infections (Suetens et al., 2018). These pathogens have shown combined resistance to multiple antimicrobial classes including beta-lactams, cephalosporins, fluoroquinolones etc. making their treatment increasingly difficult (Hollenbeck and Rice, 2012; O’Driscoll and Crank, 2015; Lakhundi and Zhang, 2018). Consequently, they are responsible for significant health and economic burden on hospitalized patients, families and society (Zhen et al., 2019).
In Europe and many other countries across the world, Enterococcus spp. and S. aureus infections are commonly observed among hospital patients with high vancomycin and methicillin resistance proportions, respectively (Lakhundi and Zhang, 2018; García-Solache and Rice, 2019). Moreover, these multidrug-resistant pathogens have been associated with significant mortality and morbidity in several healthcare settings (Caballero-Granado et al., 2001; Pinholt et al., 2014; Boncagni et al., 2015; Brady et al., 2017; Nelson et al., 2017; Kramer et al., 2018; Cassini et al., 2019). Across Europe, the population-weighted proportion of methicillin resistance among S. aureus is 16.4%, while vancomycin resistance among E. faecium isolates was estimated to be 18.3% in 2019 (Ayobami et al., 2020a; European Centre for Disease Prevention Control, 2020). Recent studies have demonstrated the rising trend of vancomycin resistance in Enterococcus spp., especially in E. faecium, and its pervasiveness across Europe (Markwart et al., 2019; Ayobami et al., 2020a).
While a declining trend of MRSA is reported in Europe, the incidence is increasing among the extremes of age groups, and its resistance proportion is still high in many countries of the European Union (Cassini et al., 2019; European Centre for Disease Prevention Control, 2020). The diminishing antibiotic arsenal, widespread resistance to several antibiotics, and other pharmacological concerns, renders antimicrobials such as linezolid and daptomycin, the antibiotics of last-resort to manage VREF and MRSA infection especially among critically ill hospitalized patients who are at the greatest risk of mortality (Rodvold and McConeghy, 2014; Hashemian et al., 2018; Heidary et al., 2018). Despite the therapeutic utility of these drugs, there is an increasing report of linezolid, and daptomycin resistance among patients infected with VREF and MRSA worldwide (Miller et al., 2016; Bender et al., 2018a; Heidary et al., 2018).
Ensuring the effectiveness of these last resort antibiotics is a priority globally, considering the severity of multi-drug resistant Gram-positive bacterial infections, broken antibiotic market and the prevailing insufficient public health actions to address antimicrobial resistance. It is on this premise that this study sought to characterize the magnitude and trend of resistance of VREF and MRSA to linezolid and daptomycin using European-wide surveillance data (2014-2018) from patients with bloodstream infections.
Materials and Methods
Outcomes, Study Design, and the European Antimicrobial Resistance Surveillance Database
The primary outcome was the population-weighted proportion of linezolid resistance among vancomycin-resistant E. faecium, and resistance to linezolid and daptomycin among methicillin-resistant S. aureus isolates. We conducted a retrospective observational study on E. faecium and S. aureus (2014–2018) using data retrieved from the European Antimicrobial Resistance Surveillance Network (EARS-Net) TESSy database with the approval of the European Centre for Disease Prevention and Control. EARS-Net is a network of European surveillance systems that collects routine clinical antimicrobial susceptibility (AST) data on invasive isolates [blood and cerebrospinal fluid (CSF)] from the 27 countries in the European Union as well as Norway, Iceland, and the United Kingdom (European Centre for Disease Prevention Control, 2018a).
Selection of Isolates
The TESSy database of EARS-Net only includes the first isolate from a given patient in the respective year. To identify unique isolates, we created a composite identifier for each isolate using “R” (v. 3.6.1). The composite identifier is composed of the (i) reporting country, (ii) unique laboratory identifier, (iii) hospital identifier, (iv) patient identifier, (v) date of sample collection, and (vi) the identified pathogen. These variables are part of the original dataset from EARS-Net and are provided by the participating countries. Isolates with duplicate composite identifiers, more than one AST against the same antibiotic, and those not assigned a hospital ID were excluded. Only E. faecium isolates that were tested for vancomycin susceptibility were included. For S. aureus, only isolates that were tested for “methicillin resistance” (see the section on Variables and Definitions below) were included for analysis.
Variables and Definitions
Patient age was categorized into four age categories (<1, 1–19, 20–64, ≥65 years). Patient gender was classified into a female, male or unknown. The country of origin of the isolate was grouped into four major regions of Europe (North: Denmark, Finland, Iceland, Ireland, Norway, Sweden, United Kingdom; West: Austria, Belgium, France, Germany, Luxembourg, Netherlands; South: Croatia, Cyprus, Greece, Hungary, Italy, Malta, Portugal, Slovenia, Spain; East: Bulgaria, Czech Republic, Estonia, Latvia, Lithuania, Poland, Romania, Slovakia). Hospital unit types were categorized into intensive care units (ICU) (including pediatric ICUs) and non-intensive care units, such as internal medicine, surgery, oncology, etc.
In the TESSy database, results on antimicrobial susceptibility testing are classified as sensitive (S), intermediate (I), or resistant (R) based on the standards used in the participating laboratories, e.g., guidelines and annual tables of clinical breakpoints of the European Committee on Antimicrobial Susceptibility Testing [EUCAST vs. 4.0 (2014) to 8.1 (2018)], Clinical and Laboratory Standards Institute (CLSI) or other national guidelines. An Enterococcus faecium isolate was defined as vancomycin-resistant if it was tested resistant or intermediate against vancomycin based on the MIC cut-offs in the respective AST guideline used by the laboratories and validated by EARS-net. Even though EARS-Net prioritized detection of the mecA gene by PCR or positive PBP2A-agglutination test over phenotypic susceptibility results, S. aureus isolates were defined as methicillin-resistant if it was tested resistant or intermediate against oxacillin or cefoxitin. An isolate was defined as resistant to linezolid and daptomycin if it was tested as resistant or intermediate against those antibiotics, respectively.
Outcomes and Statistical Analyses
The population-weighted proportion of antibiotic-resistant E. faecium and S. aureus among all tested isolates was expressed as a percentage with its 95% confidence intervals (95% CI). To assess the potential association of last-resort antibiotic resistance with ward type (i.e., ICU vs. non-ICU) and year of sampling (temporal trend), respectively, multivariable logistic regression analyses were performed including the following independent variables: Year of sampling, gender, age group, European region, and ward type. These variables were selected before the analysis based on the availability of data and our prior hypotheses about variables that may be associated with linezolid and daptomycin resistance in VREF and MRSA. All variables were treated as categorical variables, except year of sampling which was treated as a continuous variable. All statistical analyses were performed using R version 3.6.1 (R Core Team, 2019) and the “survey” package (version 3.37) (Lumley, 2020). For all analyses in all strata (i.e., descriptive analyses of resistance proportions, logistic regression analyses, and Chi2-tests) we accounted for clustering at hospital level and applied European region population-based weighting (Ayobami et al., 2020b). The population of the four European regions was calculated from the individual populations of the region’s countries. Population data of individual countries were obtained from the Eurostat database (Eurostat, 2020). European regions population weighting was used to ensure that the data from each major European region contributed proportionally (in relation to their respective population size) to the calculation of resistance proportions. This was done to minimize bias from significant differences in isolate numbers from various European regions.
Results
Baseline Characteristics
In total, 55,074 E. faecium and 211,379 S. aureus isolates from patients with bloodstream infections were included in the analysis. The characteristics of the included isolates are outlined in Table 1. The population-weighted mean proportion (2014–2018) of vancomycin resistance among E. faecium isolates was 13.1% (95% CI 11.5–14.7%) and 17.3% (95% CI 16.1–18.5%) of the S. aureus isolates were methicillin-resistant. The proportional distribution of the isolates from the regions roughly corresponded with the population size of the four European regions, although isolates from Eastern Europe were somewhat underrepresented in the dataset. For both pathogens, isolates were mainly derived from elderly patients [E. faecium: median = 70 years, interquartile range (IQR) = 60–79 years; S. aureus: median = 70 years, IQR = 57–80 years] and from male patients (E. faecium: female/male ratio: 0.64; S. aureus: female/male ratio: 0.61). Among the E. faecium and S. aureus isolates, 24.7% and 12.8% were collected in ICUs, respectively.
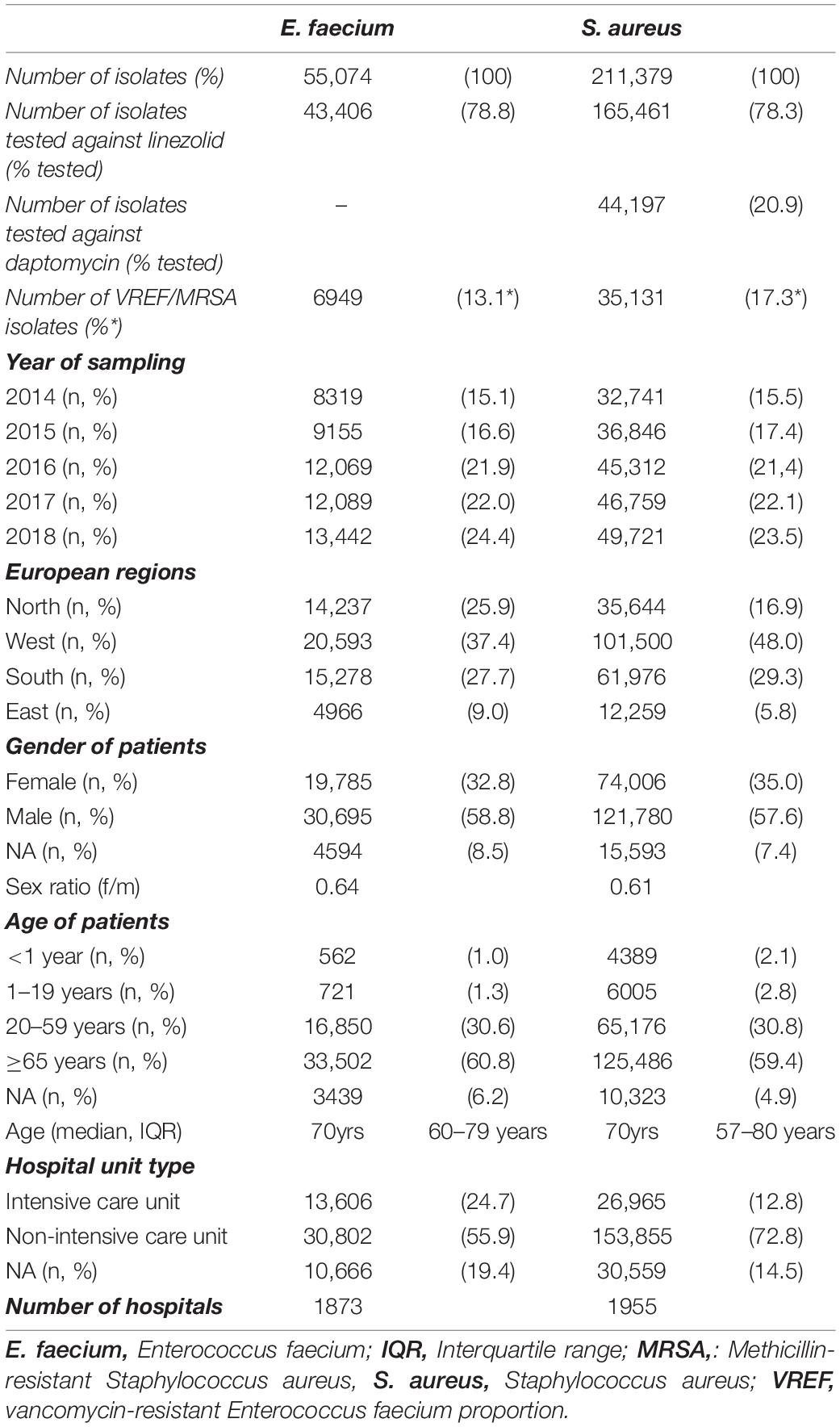
Table 1. Baseline characteristics of analyzed isolates of Enterococcus faecium and Staphylococcus aureus from patients with bloodstream infections.
Linezolid Resistance in Vancomycin-Resistant Enterococcus faecium
Between 2014 and 2018, the linezolid resistance proportion among vancomycin-resistant E. faecium isolates from patients with bloodstream infections was 1.64% (95% CI 1.33–2.03%). Although descriptive analyses of linezolid resistance proportions in VREF isolates suggested a decreasing trend between 2014 and 2018, a multivariable analysis adjusting for factors that might be associated with linezolid resistance did not confirm this declining trend [Odds Ratio (OR) = 0.86 (95%CI 0.73–1.02), p = 0.088] (Figure 1A, Table 2). Across all hospital wards, linezolid resistance was lower in vancomycin-sensitive E. faecium isolates compared to VREF isolates [0.86% (95% CI 0.72–1.01%) vs. 1.64% (95% CI 1.34–2.03%), p < 0.001] (Figure 1B). This finding is supported by the multivariable regression analysis that showed that linezolid resistance more likely occurred in VREF isolates compared to vancomycin-sensitive E. faecium isolates [adjusted OR: 1.99 (95% CI 1.56–2.54), p < 0.001] (Supplementary Table 1). We found that there was a trend of higher linezolid resistance proportions among VREF isolates in ICUs compared to non-ICUs, although this difference was not statistically significant [1.94% (95% CI 1.31–2.86%) vs. 1.36%, (95% CI 1.03–1.80%), p = 0.107; adjusted OR: 1.36 (95% CI 0.87–2.12), p = 0.174] (Table 2).
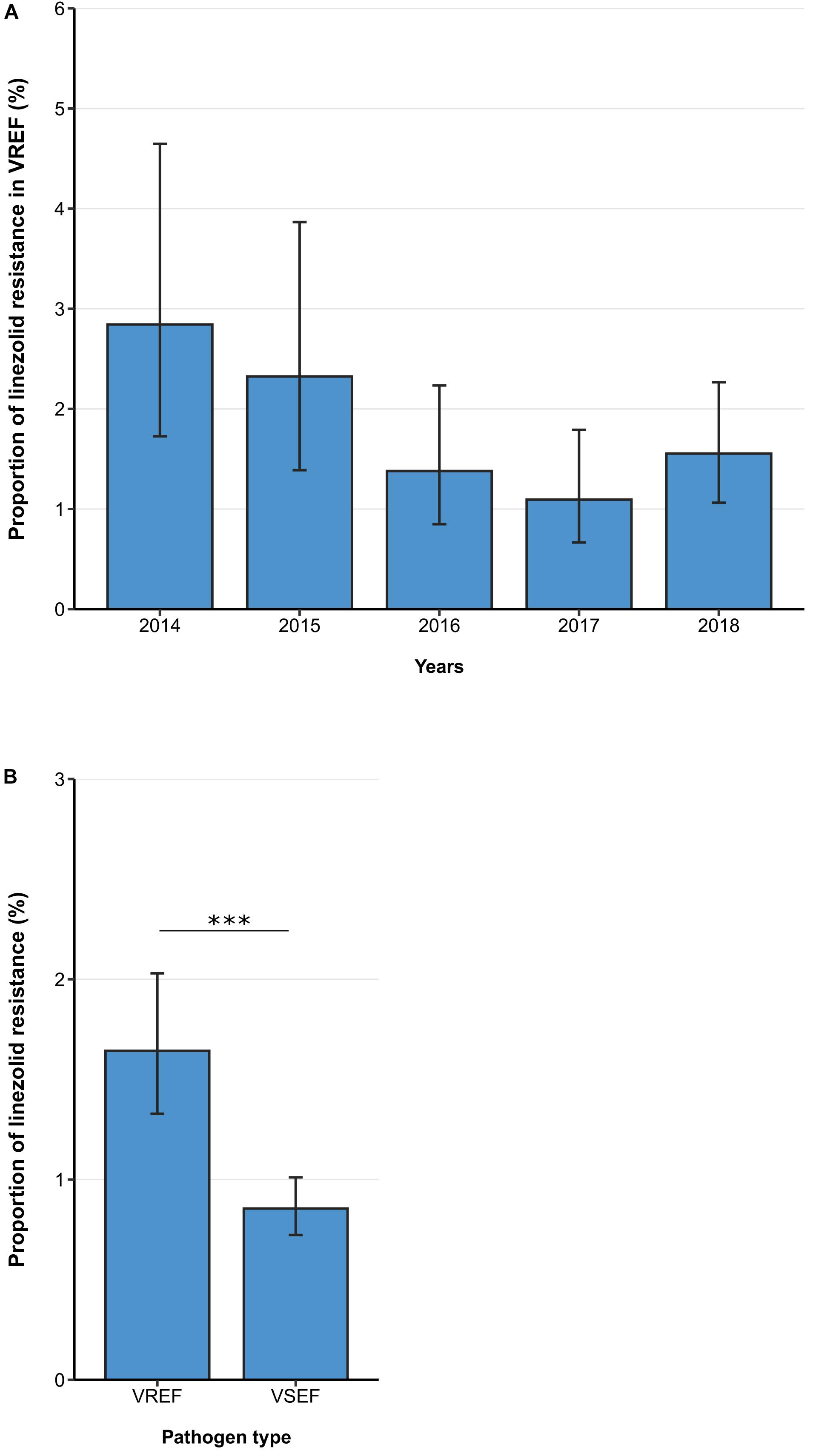
Figure 1. Linezolid resistance in vancomycin-resistant and -sensitive E. faecium isolates from patients with bloodstream infections. (A) Linezolid resistance proportions in vancomycin-resistant E. faecium (VREF) between 2014 and 2018 and (B) linezolid resistance in VREF and vancomycin-sensitive E. faecium (VSEF) Linezolid resistance proportions are expressed as population-weighted mean proportions of resistant isolates among all tested isolates with corresponding 95% confidence intervals. The difference in linezolid resistance proportions between VREF and VSEF isolates (B) was analyzed using the Chi square test. *** p < 0.001.
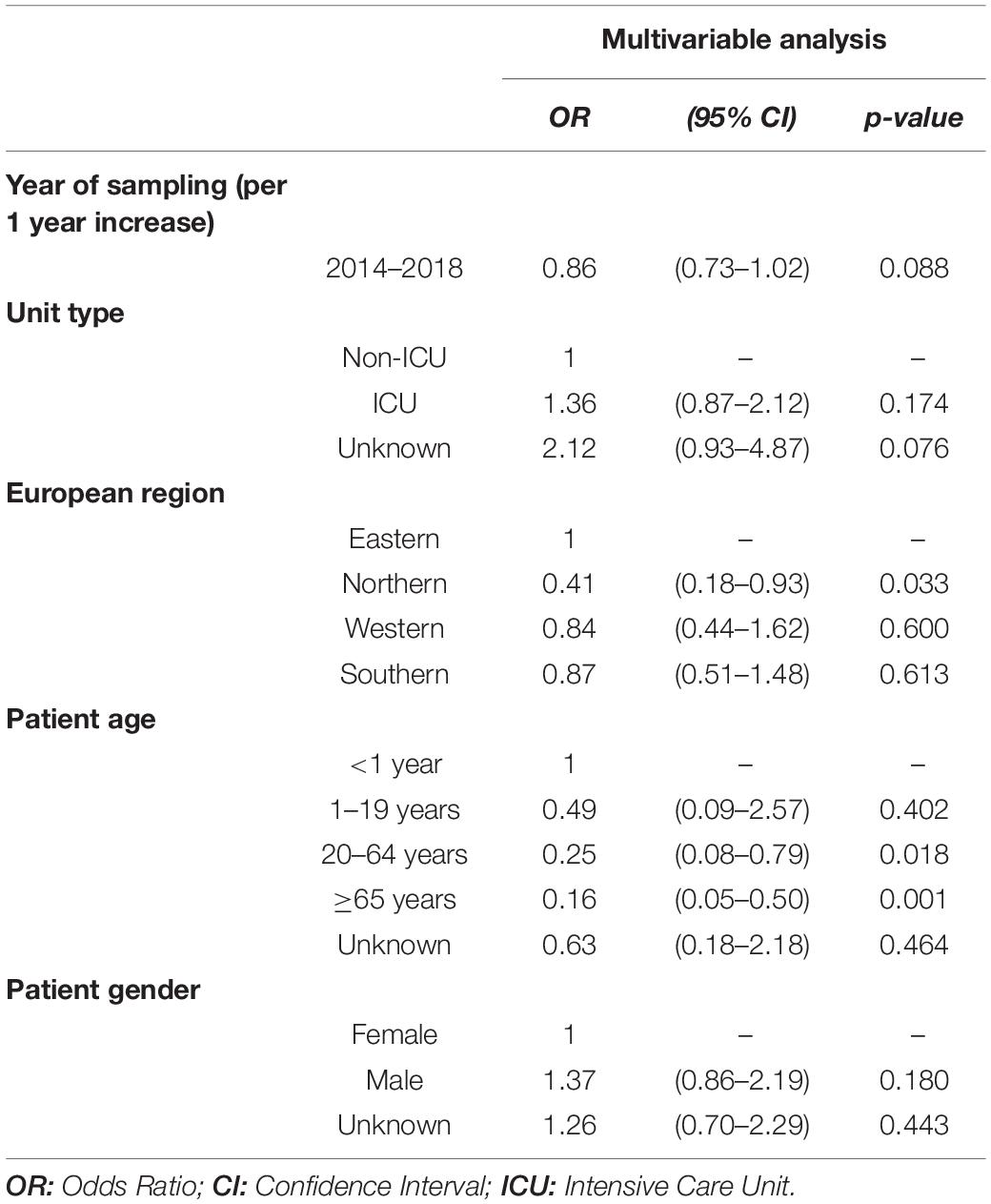
Table 2. Multivariable logistic regression analysis of factors associated with linezolid resistance in vancomycin-resistant E. faecium blood isolates.
Linezolid Resistance in Methicillin-Resistant Staphylococcus aureus
The linezolid resistance proportion among MRSA isolates from patients with bloodstream infections was 1ow [0.28% (95% CI 0.21–0.38%)] and no temporal change was observed between 2014 and 2018 [adjusted OR: 0.90 (95% CI 0.70–1.16), p = 0.410] (Figure 2A). Similar to findings in VREF isolates, hospital-wide linezolid resistance proportion is higher in MRSA isolates compared to methicillin-sensitive S. aureus isolates [0.28% (95% CI 0.21–0.38%) vs. 0.10 (95%CI 0.08–0.13%), p < 0.001] (Figure 2B). In line with this, the multivariable analysis also showed that the likelihood of linezolid resistance was higher in MRSA isolates than in methicillin-sensitive S. aureus isolates [adjusted OR: 2.74 (95% CI 1.98–3.78), p < 0.001] (Supplementary Table 2). Our data also indicate that linezolid resistance rates in MRSA isolates were higher in isolates from ICUs compared to isolates from non-ICUs [0.56% (95% CI 0.36–0.87%) vs. 0.24% (95%CI 0.14–0.38%), p < 0.01], which was also confirmed by the multivariable analysis [adjusted OR: 2.86 (95% CI 1.58–5.19), p < 0.001] (Figure 2C, Table 3).
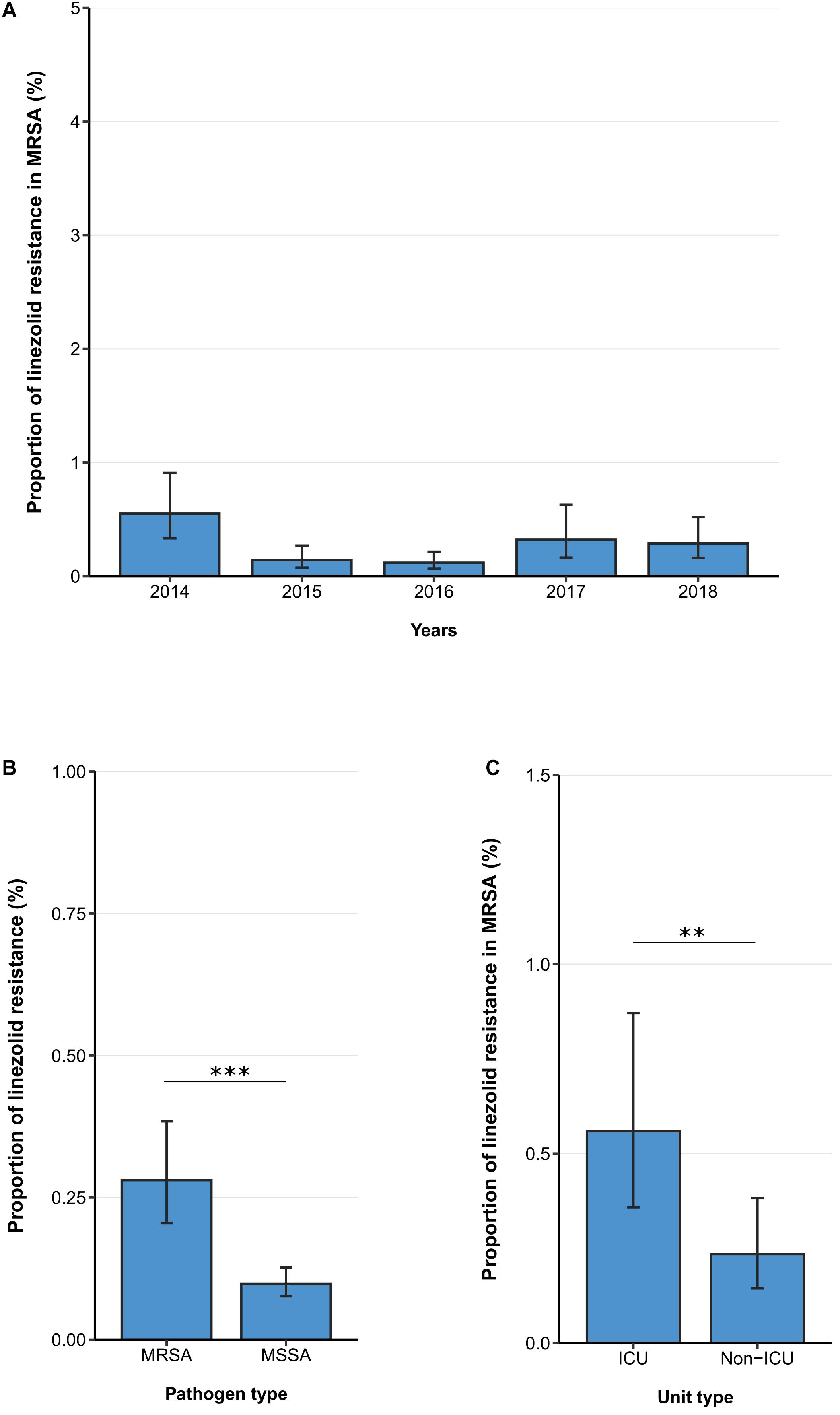
Figure 2. Linezolid resistance in methicillin-resistant and -sensitive S. aureus isolates from patients with bloodstream infections. (A) Linezolid resistance proportions in methicillin-resistant S. aureus (MRSA) between 2014 and 2018, (B) linezolid resistance in MRSA and methicillin-sensitive S. aureus (MSSA) and (C) linezolid resistance in MRSA stratified by unit type (ICU vs. non-ICU). Linezolid resistance proportions are expressed as population-weighted mean proportions of resistant isolates among all tested isolates with corresponding 95% confidence intervals. (B) The difference in linezolid resistance proportions between MRSA and MSSA isolates and (C) between ICU vs. non-ICU MRSA isolates was analyzed using the Chi square test. ** p < 0.01; *** p < 0.001.
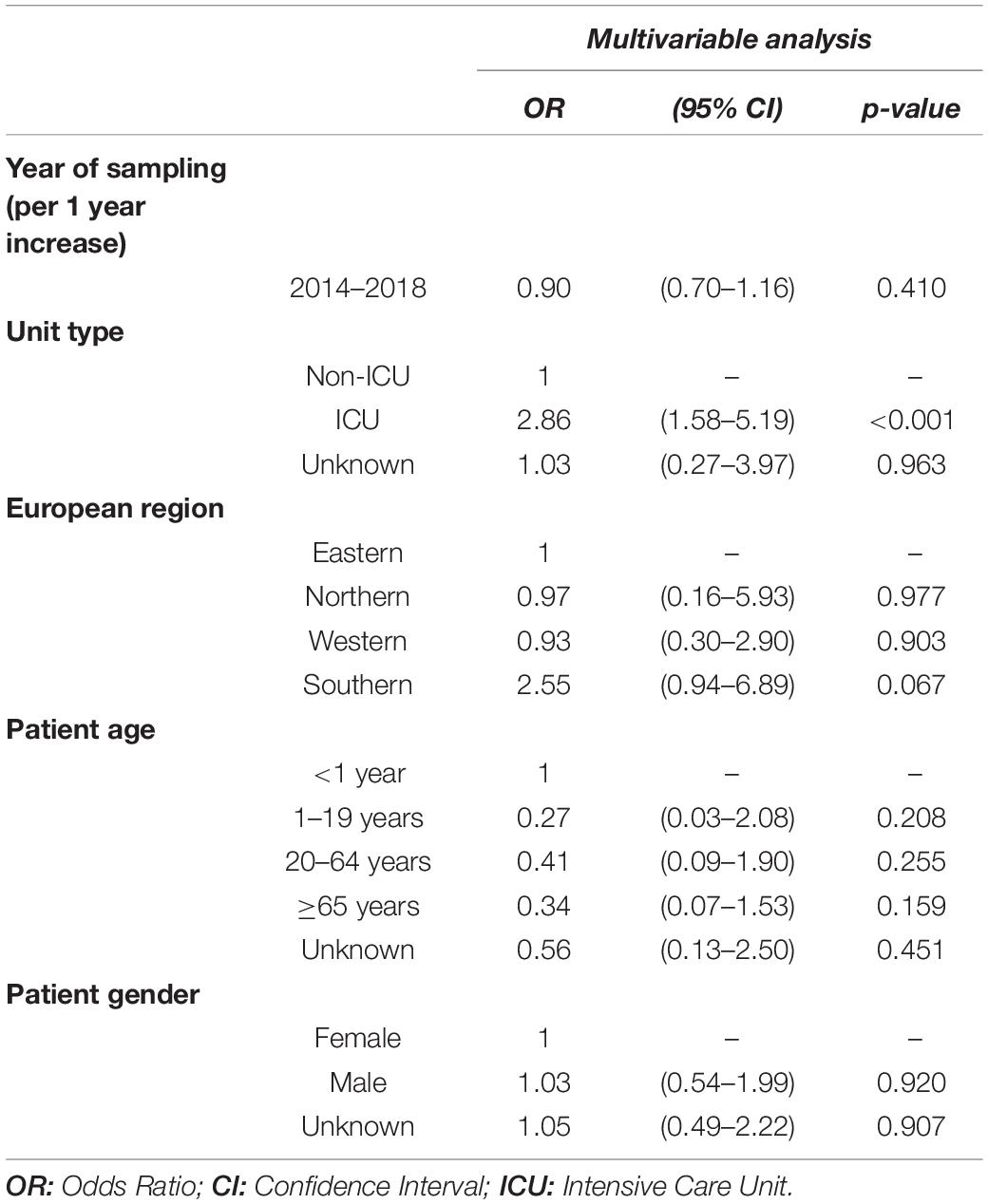
Table 3. Multivariable logistic regression analysis of factors associated with linezolid resistance in methicillin-resistant S. aureus blood isolates.
Daptomycin Resistance in MRSA
Between 2014 and 2018, the daptomycin resistance proportion among MRSA isolates from patients with bloodstream infections was 1.11% (95% CI 0.75–1.63%) (Figure 3A) and no clear temporal trend was observed. [adjusted OR: 1.38 (95% CI 0.94–2.05%), p = 0.104]. Similar to the findings for linezolid, daptomycin resistance was higher among MRSA isolates than among methicillin-sensitive S. aureus isolates [1.11% (95% CI 0.75–1.63%) vs. 0.48% (95% CI 0.34–0.67%), p < 0.001] (Figure 3B), which was also confirmed by the multivariable regression analysis [adjusted OR: 2.25 (95% CI 1.45–3.49), p < 0.001]. There was a statistically significant higher daptomycin resistance proportion in MRSA isolates from ICUs compared to isolates from non-ICUs [1.96% (95% CI 0.93–4.08%) vs. 1.03% (95% CI 0.60–1.78%), p = 0.039] (Figure 3C). This finding was also observed after adjusting for other potential predictors in a multivariable regression analysis, that showed that there was a higher likelihood of daptomycin resistance in MRSA blood isolates from ICUs compared to non-ICU wards [adjusted OR: 2.65 (95% CI 1.13–6.26), p = 0.0245] (Table 4).
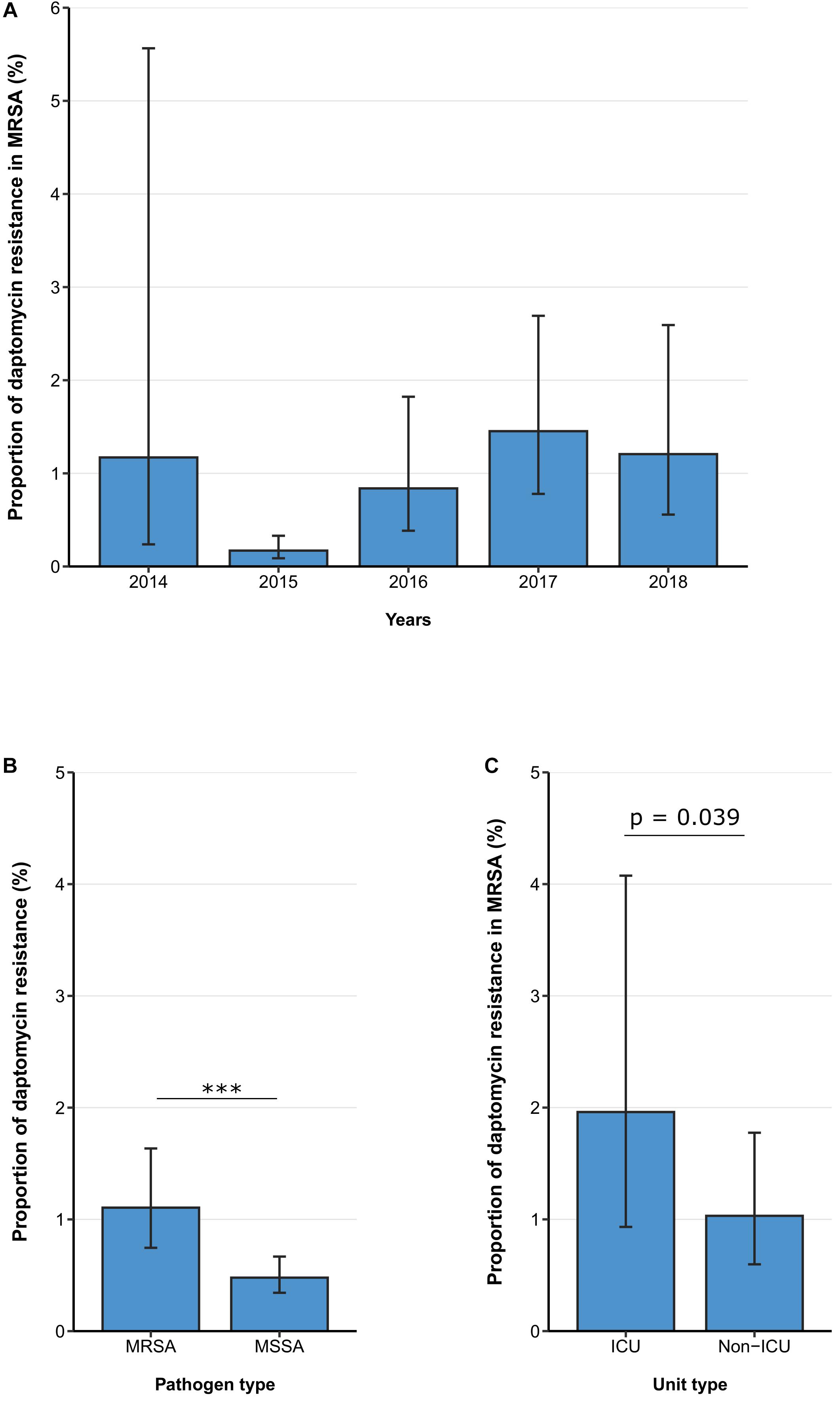
Figure 3. Daptomycin resistance in methicillin-resistant and -sensitive S. aureus isolates from patients with bloodstream infections. (A) Daptomycin resistance proportions in methicillin-resistant S. aureus (MRSA) between 2014 and 2018, (B) daptomycin resistance in MRSA and methicillin-sensitive S. aureus (MSSA), and (C) daptomycin resistance in MRSA stratified by unit type (ICU vs. non-ICU). Daptomycin resistance proportions are expressed as population-weighted mean proportions of resistant isolates among all tested isolates with corresponding 95% confidence intervals. (B) The difference in daptomycin resistance proportions between MRSA and MSSA isolates, and (C) between ICU vs. non-ICU MRSA isolates was analyzed using the Chi square test. *** p < 0.001.
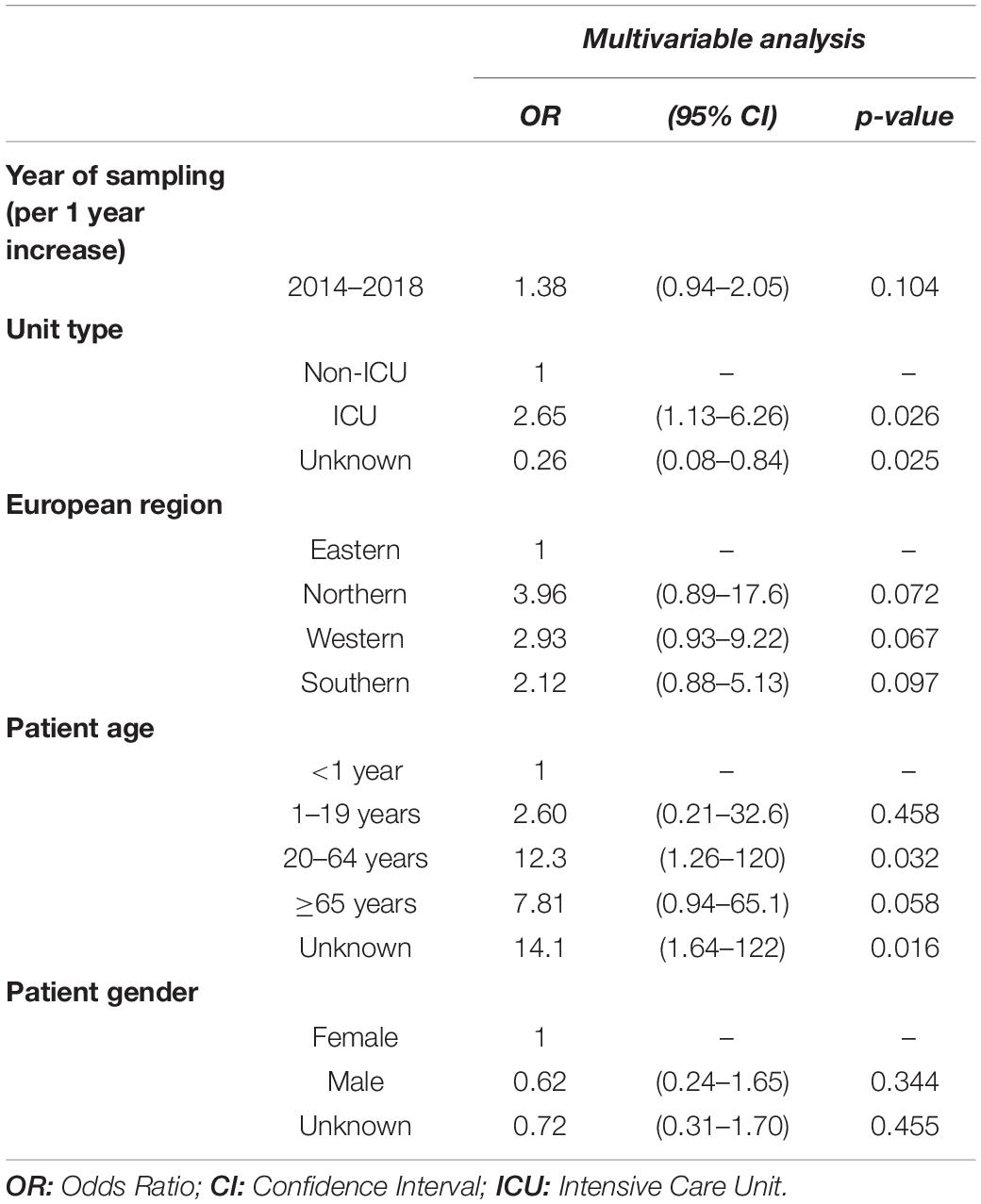
Table 4. Multivariable logistic regression analysis of factors associated with daptomycin resistance in methicillin-resistant S. aureus blood isolates.
Discussion
In this study, we examined linezolid and daptomycin resistance patterns among VREF and MRSA isolates isolated from patients with bloodstream infections in Europe. In sum, resistance proportions of the analyzed last line antibiotics in VREF and MRSA are relatively low in Europe. The population-weighted mean proportion of linezolid resistance among VREF isolates was 1.6% (95% CI 1.3–2.0%). While the in-country proportion varies across several local settings within Europe (Gawryszewska et al., 2016; Sassi et al., 2019; Xanthopoulou et al., 2020), this European mean proportion is comparably similar to what is seen in many within-country studies from other regions of the world such as South Korea, China, India, Iran, and United States where it remains less than 2% (Flamm et al., 2015; Houri et al., 2017; Yadav et al., 2017; Bi et al., 2018; Cho et al., 2018; Zhang et al., 2018; Huang et al., 2019).
Also, the European mean proportion of linezolid resistance among MRSA blood isolates included in this study was found to be very 1ow [0.29% (95% CI 0.21–0.40%)], attesting to the overall susceptibility of MRSA isolates to linezolid in Europe. This is also similar to local studies in Germany and Spain (Sierra et al., 2013; Yayan et al., 2015), Russia (Gostev et al., 2015), China (Huang et al., 2019), and Latin America (Vega and Dowzicky, 2017), but remarkably lower compared to results from Pakistan (48.1%) (Azhar et al., 2017) and India (2.8–7.0%) (Kaur and Chate, 2015; Kumar, 2016). In comparison to other multi-country surveillance studies, such as ZAAPS and SENTRY, linezolid activity against VREF and MRSA remains similarly very high (>98%) in Europe (Mendes et al., 2016; Deshpande et al., 2018).
Our results suggested no significant change in the linezolid resistance proportions among MRSA and VREF isolates over the study period in Europe despite the reported increasing trend of linezolid consumption between 2009 and 2018 in Europe (European Centre for Disease Prevention Control, 2019a; Kramer et al., 2019). We also found that VREF and MRSA isolates are more likely to be linezolid-resistant than the vancomycin-sensitive and methicillin-sensitive isolates, respectively. While previous studies have shown linezolid resistance can be seen in both VREF and vancomycin-sensitive isolates (Allen and Bierman, 2009), the increased likelihood among VREF and MRSA isolates suggests the higher chance of co-resistance to linezolid among VREF and MRSA isolates under selective pressure such as linezolid exposure (Cantón and Ruiz-Garbajosa, 2011). Whether this is driven mainly by de novo mutations and/or horizontal transfer of resistance genes is for future studies to clarify. However, this observation reinforces the need to preserve the effectiveness of vancomycin and penicillinase-resistant β-lactams, to reduce the use of linezolid and safeguard its efficacy as an important last-line antibiotic.
In line with the findings for linezolid resistance, the European population-weighted mean proportion of daptomycin resistance among MRSA isolates was similarly low [1.0% (95% CI 0.82–1.28%)]. The low proportion is comparable to the non-susceptibility proportion recorded in a multicenter ICU surveillance studies from Canada (Denisuik et al., 2018), China (Qin et al., 2016), and a global surveillance that reported daptomycin resistance in MRSA isolates of bone and joint infections (Jones et al., 2017). Similar to linezolid resistance, our results showed daptomycin resistance among MRSA isolates is substantially more likely compared to MSSA isolates. Our data showed MRSA blood isolates from the ICU are more likely to be linezolid and daptomycin resistant compared to isolates from non-ICU wards of the hospital. This might be due to the prominence of MRSA in the European ICUs (Vincent et al., 1995; Pujol et al., 1996; Boncagni et al., 2015; European Centre for Disease Prevention Control, 2018c) where linezolid and daptomycin are frequently prescribed antibiotic (Davis et al., 2005; Rodríguez et al., 2009; Hashemian et al., 2018; Kramer et al., 2019). These results can’t overstate the need for early initiation of effective antibiotics to treat MRSA infections especially among vulnerable patients that usually populate ICU wards, as a matter of patient safety.
Various mechanisms underlie the resistance of gram-positive pathogens to linezolid including mutation of 23S rRNA, ribosomal proteins (L3, L4) but very few studies have reported the transmissible optrA, cfr, and poxtA mediated linezolid resistance in Europe. The recent report of the highest prevalence (22.7%) of optrA, and poxtA genes ever in human linezolid-resistant enterococci isolates in Ireland (Egan et al., 2020), highlights its potential clinical and surveillance challenge in Europe (Feßler et al., 2013; Brenciani et al., 2015; Antonelli et al., 2016; de Dios Caballero et al., 2016; Gawryszewska et al., 2017; Lazaris et al., 2017; Bender et al., 2018a, b; Ruiz-Ripa et al., 2020, 2021).
Experience with the evolution of antibiotic resistance warns that the present effectiveness comes with a price of triggered treatment failure among future patients. With the treatment difficulties associated with VREF and MRSA, an increasing reliance on linezolid and daptomycin is expected since both have been shown to be equally efficacious in the treatment of VREF and MRSA (Crank et al., 2010; Gonzalez-Ruiz et al., 2011; Twilla et al., 2012; Heidary et al., 2018). Prevention of resistance to linezolid and daptomycin will require multi-pronged approaches including genomic surveillance, screening of high-risk patients where beneficial, combination antibiotic therapy, and enhanced infection prevention and control strategies (Gonzales et al., 2015; Yim et al., 2017). Therefore, the low proportion of resistance to linezolid and daptomycin among VREF and MRSA isolates in Europe should be seen as a window of opportunity to strengthen existing AMR prevention and control measures, and tackle the difficulties plaguing the research and development of new antibiotics (McKenna, 2020).
Strengths and Limitations
With 7,000 and 35,000 VREF and MRSA isolates from patients with bloodstream infections, respectively, this study is the largest and most comprehensive analysis of last line antibiotic resistance profiles among these difficult to treat multidrug resistant pathogens in the EU/EEA. The analyzed dataset shows a high level of representativeness for the EU/EEA region, since the isolates were derived from routine clinical microbiological sampling and antimicrobial susceptibility testing and were transmitted to EARS-Net from 29 EU/EEA countries and the United Kingdom. Notably, population, hospital, and isolate sample representativeness was assessed as “high” in 25 countries (European Centre for Disease Prevention Control, 2019b). In addition, the validity of these AMR data is regularly assessed in external quality assessments of participating laboratories (European Centre for Disease Prevention Control, 2018b) ensuring high data quality.
A potential limitation is the variation in population coverage among reporting countries, although half of the participating countries reported a national coverage greater than 80% (European Centre for Disease Prevention Control, 2019b). To minimize possible bias from differences in population size and isolate numbers from various countries, EU/EEA-wide resistance proportions and multivariable regression analyses were population-weighted based on the population sizes of the individual regions. Moreover, differences in sampling routines, admission characteristics (e.g., stay duration, bed space density) and healthcare resources across EU/EEA countries can result in biased estimates of resistance proportions. However, to reduce the extent of this potential bias, in EARS-Net only isolates from bloodstream infections are collected, since microbiological sampling routines for these infections are generally similar across countries of the EU/EEA, even though some variations (such as frequency of sampling) between hospitals and countries cannot fully excluded.
Also, despite the off-label use of linezolid as a therapy option in some settings, it is questionable whether blood isolates are the most appropriate to determine linezolid resistance among VREF isolates from patient with nosocomial bloodstream infections because of its non-bactericidal effects. Therefore, our results should be interpreted with these limitations in mind.
Conclusion
Proportions of linezolid and daptomycin resistance among VREF and MRSA blood isolates remain low in the EU/EEA throughout the study period. However, VREF and MRSA were consistently more resistant to linezolid and daptomycin compared to their sensitive isolates. MRSA blood isolates from the ICU were more likely to be linezolid and daptomycin resistant compared to isolates from non-ICU wards of the hospital. In addition to existing antibiotics stewardship programs, it is necessary to strengthen surveillance in Europe, especially the genomic characterization of the resistance genes that compromise the efficacy of these last line antibiotics in VREF and MRSA.
Data Availability Statement
The data analyzed in this study is subject to the following licenses/restrictions: The datasets will be made available with the express permission of the European Centre for Disease Control (ECDC) Stockholm Sweden. The ECDC on behalf of the EU/EEA countries have the exclusive control over the sharing of the data. Requests to access these datasets should be directed to DATA ACCESS REQUEST European Centre for Disease Prevention and Control (ECDC) Gustav III:s boulevard, 40 16973 Solna Sweden. Further inquiries should be directed to the corresponding author.
Author Contributions
OA, RM, and TE: conceptualization. RM and OA: original draft. NW, RM, and OA: statistical analysis. RM, OA, GW, and TE: critical review, revision, and Editing. RM, OA, NW, GW, and TE: final approval. All authors contributed to the article and approved the submitted version.
Funding
The study was funded with the internal funds of the Robert Koch Institute, a federal health agency of the German Ministry of Health.
Conflict of Interest
The authors declare that the research was conducted in the absence of any commercial or financial relationships that could be construed as a potential conflict of interest.
Acknowledgments
We thank our colleague Angelina Taylor at the Robert Koch Institute for her contribution to this study. We would like to also thank all EARS-Net participating laboratories and hospitals in the EU/EEA countries for providing data. We wish to thank the European Centre for Disease Prevention and Control for providing the dataset for our paper. “The views and opinions of the authors expressed herein do not necessarily state or reflect those of ECDC. The accuracy of the authors’ statistical analysis and the findings they report are not the responsibility of ECDC. ECDC is not responsible for conclusions or opinions drawn from the data provided. ECDC is not responsible for the correctness of the data and for data management, data merging and data collation after provision of the data. ECDC shall not be held liable for improper or incorrect use of the data”.
Supplementary Material
The Supplementary Material for this article can be found online at: https://www.frontiersin.org/articles/10.3389/fmicb.2021.664199/full#supplementary-material
Abbreviations
BSI, bloodstream infections; CDC, Center for Disease Control and Prevention; ECDC, European Centre for Disease Prevention and Control; HAIs, hospital-acquired infections; E, Enterococcus faecium; VREF, vancomycin-resistant Enterococcus faecium; MRSA, Methicillin-resistant Staphylococcus aureus; LRVREF, Linezolid-Vancomycin resistant Enterococcus faecium; ICU, intensive care unit; NHSN, National Healthcare Safety Network; WHO, World Health Organization; 95% CI, 95% confidence interval; ZAAPS, Zyvox§ Annual Appraisal of Potency and Spectrum programme; SENTRY, SENTRY Antimicrobial Surveillance Program; PCR, Polymerase Chain Reaction.
References
Allen, G. P., and Bierman, B. C. (2009). In vitro analysis of resistance selection by linezolid in vancomycin-susceptible and -resistant Enterococcus faecalis and Enterococcus faecium. Int. J. Antimicrob. Agents 34, 21–24. doi: 10.1016/j.ijantimicag.2008.12.011
Antonelli, A., D’Andrea, M. M., Galano, A., Borchi, B., Brenciani, A., Vaggelli, G., et al. (2016). Linezolid-resistant cfr-positive MRSA, Italy. J. Antimicrob. Chemother. 71, 2349–2351.
Ayobami, O., Willrich, N., Reuss, A., Eckmanns, T., and Markwart, R. (2020a). The ongoing challenge of vancomycin-resistant Enterococcus faecium and Enterococcus faecalis in Europe: an epidemiological analysis of bloodstream infections. Emerging Microb. Infect. 9, 1180–1193. doi: 10.1080/22221751.2020.1769500
Ayobami, O., Willrich, N., Suwono, B., Eckmanns, T., and Markwart, R. (2020b). The epidemiology of carbapenem-non-susceptible Acinetobacter species in Europe: analysis of EARS-Net data from 2013 to 2017. Antimicrob. Resist. Infect. Contr. 9:89.
Azhar, A., Rasool, S., Haque, A., Shan, S., Saeed, M., Ehsan, B., et al. (2017). Detection of high levels of resistance to linezolid and vancomycin in Staphylococcus aureus. J. Med. Microbiol. 66, 1328–1331. doi: 10.1099/jmm.0.000566
Bender, J. K., Cattoir, V., Hegstad, K., Sadowy, E., Coque, T. M., Westh, H., et al. (2018a). Update on prevalence and mechanisms of resistance to linezolid, tigecycline and daptomycin in enterococci in Europe: Towards a common nomenclature. Drug Resist. Updates 40, 25–39. doi: 10.1016/j.drup.2018.10.002
Bender, J. K., Fleige, C., Lange, D., Klare, I., and Werner, G. (2018b). Rapid emergence of highly variable and transferable oxazolidinone and phenicol resistance gene optrA in German Enterococcus spp. clinical isolates. Int. J. Antimicrob. Agents 52, 819–827. doi: 10.1016/j.ijantimicag.2018.09.009
Bi, R., Qin, T., Fan, W., Ma, P., and Gu, B. (2018). The emerging problem of linezolid-resistant enterococci. J. Glob. Antimicrob. Resist. 13, 11–19. doi: 10.1016/j.jgar.2017.10.018
Boncagni, F., Francolini, R., Nataloni, S., Skrami, E., Gesuita, R., Donati, A., et al. (2015). Epidemiology and clinical outcome of Healthcare-Associated Infections: a 4-year experience of an Italian ICU. Minerva Anestesiol. 81, 765–775.
Brady, M., Oza, A., Cunney, R., and Burns, K. (2017). Attributable mortality of hospital-acquired bloodstream infections in Ireland. J. Hospit. Infect. 96, 35–41. doi: 10.1016/j.jhin.2017.02.006
Brenciani, A., Morroni, G., Vincenzi, C., Manso, E., Mingoia, M., Giovanetti, E., et al. (2015). Detection in Italy of two clinical Enterococcus faecium isolates carrying both the oxazolidinone and phenicol resistance gene optrA and a silent multiresistance gene cfr. J. Antimicrob. Chemother. 71, 1118–1119. doi: 10.1093/jac/dkv438
Caballero-Granado, F. J., Becerril, B., Cuberos, L., Bernabeu, M., Cisneros, J. M., and Pachón, J. (2001). Attributable Mortality Rate and Duration of Hospital Stay Associated with Enterococcal Bacteremia. Clin. Infect. Dis. 32, 587–594. doi: 10.1086/318717
Cantón, R., and Ruiz-Garbajosa, P. (2011). Co-resistance: an opportunity for the bacteria and resistance genes. Curr. Opin. Pharmacol. 11, 477–485. doi: 10.1016/j.coph.2011.07.007
Cassini, A., Högberg, L. D., Plachouras, D., Quattrocchi, A., Hoxha, A., Simonsen, G. S., et al. (2019). Attributable deaths and disability-adjusted life-years caused by infections with antibiotic-resistant bacteria in the EU and the European Economic Area in 2015: a population-level modelling analysis. Lancet Infect. Dis. 19, 56–66.
Cho, S. Y., Kim, H. M., Chung, D. R., Kim, S. H., Huh, H. J., Kang, C. I., et al. (2018). Resistance mechanisms and clinical characteristics of linezolid-resistant Enterococcus faecium isolates: A single-centre study in South Korea. J. Glob. Antimicrob. Resist. 12, 44–47. doi: 10.1016/j.jgar.2017.09.009
Crank, C. W., Scheetz, M. H., Brielmaier, B., Rose, W. E., Patel, G. P., Ritchie, D. J., et al. (2010). Comparison of outcomes from daptomycin or linezolid treatment for vancomycin-resistant enterococcal bloodstream infection: A retrospective, multicenter, cohort study. Clin. Therapeut. 32, 1713–1719. doi: 10.1016/j.clinthera.2010.09.008
Davis, K. A., Moran, K. A., McAllister, C. K., and Gray, P. J. (2005). Multidrug-resistant Acinetobacter extremity infections in soldiers. Emerging Infect. Dis. 11, 1218–1224.
de Dios Caballero, J., Pastor, M. D., Vindel, A., Máiz, L., Yagüe, G., Salvador, C., et al. (2016). Emergence of cfr-Mediated Linezolid Resistance in a Methicillin-Resistant Staphylococcus aureus Epidemic Clone Isolated from Patients with Cystic Fibrosis. Antimicrob. Agents Chemother. 60:1878. doi: 10.1128/aac.02067-15
Denisuik, A. J., Garbutt, L. A., Golden, A. R., Adam, H. J., Baxter, M., Nichol, K. A., et al. (2018). Antimicrobial-resistant pathogens in Canadian ICUs: results of the CANWARD 2007 to 2016 study. J. Antimicrob. Chemother. 74, 645–653. doi: 10.1093/jac/dky477
Deshpande, L. M., Castanheira, M., Flamm, R. K., and Mendes, R. E. (2018). Evolving oxazolidinone resistance mechanisms in a worldwide collection of enterococcal clinical isolates: results from the SENTRY Antimicrobial Surveillance Program. J. Antimicrob. Chemother. 73, 2314–2322. doi: 10.1093/jac/dky188
Egan, S. A., Shore, A. C., O’Connell, B., Brennan, G. I., and Coleman, D. C. (2020). Linezolid resistance in Enterococcus faecium and Enterococcus faecalis from hospitalized patients in Ireland: high prevalence of the MDR genes optrA and poxtA in isolates with diverse genetic backgrounds. J. Antimicrob. Chemother. 75, 1704–1711. doi: 10.1093/jac/dkaa075
European Centre for Disease Prevention Control (2018a). Antimicrobial Resistance: Tessy Reporting Protocol (2018). Solna Municipality: European Centre for Disease Prevention Control.
European Centre for Disease Prevention Control (2018b). External quality assessment of laboratory performance - European Antimicrobial Resistance Surveillance Network (EARS-Net), 2017. Stockholm: European Centre for Disease Prevention Control.
European Centre for Disease Prevention Control (2018c). Incidence and attributable mortality of healthcare-associated infections in intensive care units in Europe, 2008–2012 Stockholm. Solna Municipality: European Centre for Disease Prevention Control.
European Centre for Disease Prevention Control (2019a). Antimicrobial consumption in the EU/EEA: Annual epidemiological report for 2018. Stockholm: European Centre for Disease Prevention Control.
European Centre for Disease Prevention Control (2019b). Surveillance of antimicrobial resistance in Europe 2018. Stockholm: European Centre for Disease Prevention Control.
European Centre for Disease Prevention Control (2020). Surveillance of antimicrobial resistance in Europe 2019. Solna Municipality: European Centre for Disease Prevention Control.
Feßler, A. T., Calvo, N., Gutiérrez, N., Muñoz Bellido, J. L., Fajardo, M., Garduño, E., et al. (2013). Cfr-mediated linezolid resistance in methicillin-resistant Staphylococcus aureus and Staphylococcus haemolyticus associated with clinical infections in humans: two case reports. J. Antimicrob. Chemother. 69, 268–270. doi: 10.1093/jac/dkt331
Fisher, K., and Phillips, C. (2009). The ecology, epidemiology and virulence of Enterococcus. Microbiol 155, 1749–1757. doi: 10.1099/mic.0.026385-0
Flamm, R. K., Mendes, R. E., Hogan, P. A., Ross, J. E., Farrell, D. J., and Jones, R. N. (2015). In vitro activity of linezolid as assessed through the 2013 LEADER surveillance program. Diagnost. Microbiol. Infect. Dis. 81, 283–289. doi: 10.1016/j.diagmicrobio.2014.12.009
García-Solache, M., and Rice, L. B. (2019). The Enterococcus: a Model of Adaptability to Its Environment. Clin. Microbiol. Rev. 32, e58–e18.
Gawryszewska, I., Żabicka, D., Bojarska, K., Malinowska, K., Hryniewicz, W., and Sadowy, E. (2016). Invasive enterococcal infections in Poland: the current epidemiological situation. Eur. J. Clin. Microbiol. Infect. Dis. 35, 847–856. doi: 10.1007/s10096-016-2607-y
Gawryszewska, I., Żabicka, D., Hryniewicz, W., and Sadowy, E. (2017). Linezolid-resistant enterococci in Polish hospitals: species, clonality and determinants of linezolid resistance. Eur. J. Clin. Microbiol. Infect. Dis. 36, 1279–1286. doi: 10.1007/s10096-017-2934-7
Gonzales, P. R., Pesesky, M. W., Bouley, R., Ballard, A., Biddy, B. A., Suckow, M. A., et al. (2015). Synergistic, collaterally sensitive β-lactam combinations suppress resistance in MRSA. Nat. Chem. Biol. 11, 855–861. doi: 10.1038/nchembio.1911
Gonzalez-Ruiz, A., Beiras-Fernandez, A., Lehmkuhl, H., Seaton, R. A., Loeffler, J., and Chaves, R. L. (2011). Clinical experience with daptomycin in Europe: the first 2.5 years. J. Antimicrob. Chemother. 66, 912–919. doi: 10.1093/jac/dkq528
Gostev, V. V., Kalinogorskaya, O. S., Popenko, L. N., Chernenkaya, T. V., Naumenko, Z. S., Voroshilova, T. M., et al. (2015). [Antibiotic Resistance of MRSA in the Russian Federation]. Antibiot Khimioter 60, 3–9.
Hashemian, S. M. R., Farhadi, T., and Ganjparvar, M. (2018). Linezolid: a review of its properties, function, and use in critical care. Drug Des. Devel. Ther. 12, 1759–1767. doi: 10.2147/dddt.s164515
Heidary, M., Khosravi, A. D., Khoshnood, S., Nasiri, M. J., Soleimani, S., and Goudarzi, M. (2018). Daptomycin. J. Antimicrob. Chemother. 73, 1–11.
Hollenbeck, B. L., and Rice, L. B. (2012). Intrinsic and acquired resistance mechanisms in Enterococcus. Virulence 3, 421–433. doi: 10.4161/viru.21282
Houri, H., Kazemian, H., Sedigh Ebrahim-Saraie, H., Taji, A., Tayebi, Z., and Heidari, H. (2017). Linezolid activity against clinical Gram-positive cocci with advanced antimicrobial drug resistance in Iran. J. Glob. Antimicrob. Resist. 10, 200–203. doi: 10.1016/j.jgar.2017.06.002
Huang, L., Zhang, R., Hu, Y., Zhou, H., Cao, J., Lv, H., et al. (2019). Epidemiology and risk factors of methicillin-resistant Staphylococcus aureus and vancomycin-resistant enterococci infections in Zhejiang China from 2015 to 2017. Antimicrob. Resist. Infect. Contr. 8:90.
Jones, R. N., Flamm, R. K., Castanheira, M., Sader, H. S., Smart, J. I., and Mendes, R. E. (2017). Activity of telavancin against Gram-positive pathogens isolated from bone and joint infections in North American, Latin American, European and Asia-Pacific nations. Diagnost. Microbiol. Infect. Dis. 88, 184–187. doi: 10.1016/j.diagmicrobio.2017.03.003
Kaur, D. C., and Chate, S. S. (2015). Study of Antibiotic Resistance Pattern in Methicillin Resistant Staphylococcus aureus with Special Reference to Newer Antibiotic. J. Glob. Infect. Dis. 7, 78–84. doi: 10.4103/0974-777x.157245
Kramer, T. S., Remschmidt, C., Werner, S., Behnke, M., Schwab, F., Werner, G., et al. (2018). The importance of adjusting for enterococcus species when assessing the burden of vancomycin resistance: a cohort study including over 1000 cases of enterococcal bloodstream infections. Antimicrob. Resist. Infect. Control 7:133.
Kramer, T. S., Schwab, F., Behnke, M., Hansen, S., Gastmeier, P., and Aghdassi, S. J. S. (2019). Linezolid use in German acute care hospitals: results from two consecutive national point prevalence surveys. Antimicrob. Resist. Infect. Contr. 8:159.
Kumar, M. (2016). Multidrug-Resistant Staphylococcus aureus, India, 2013–2015. Emerging Infect. Dis. J. 22:1666.
Lakhundi, S., and Zhang, K. (2018). Methicillin-Resistant Staphylococcus aureus: Molecular Characterization, Evolution, and Epidemiology. Clin. Microbiol. Rev. 31, e20–e18.
Lazaris, A., Coleman, D. C., Kearns, A. M., Pichon, B., Kinnevey, P. M., Earls, M. R., et al. (2017). Novel multiresistance cfr plasmids in linezolid-resistant methicillin-resistant Staphylococcus epidermidis and vancomycin-resistant Enterococcus faecium (VRE) from a hospital outbreak: co-location of cfr and optrA in VRE. J. Antimicrob. Chemother. 72, 3252–3257. doi: 10.1093/jac/dkx292
Markwart, R., Willrich, N., Haller, S., Noll, I., Koppe, U., Werner, G., et al. (2019). The rise in vancomycin-resistant Enterococcus faecium in Germany: data from the German Antimicrobial Resistance Surveillance (ARS). Antimicrob. Resist. Infect. Contr. 8:147.
McKenna, M. (2020). The antibiotic paradox: why companies can’t afford to create life-saving drugs. Nature 584, 338–341. doi: 10.1038/d41586-020-02418-x
Mendes, R. E., Hogan, P. A., Jones, R. N., Sader, H. S., and Flamm, R. K. (2016). Surveillance for linezolid resistance via the Zyvox§Annual Appraisal of Potency and Spectrum (ZAAPS) programme (2014): evolving resistance mechanisms with stable susceptibility rates. J. Antimicrob. Chemother. 71, 1860–1865. doi: 10.1093/jac/dkw052
Miller, W. R., Bayer, A. S., and Arias, C. A. (2016). Mechanism of Action and Resistance to Daptomycin in Staphylococcus aureus and Enterococci. Cold Spring Harb. Perspect. Med. 6:a026997. doi: 10.1101/cshperspect.a026997
Nelson, R. E., Slayton, R. B., Stevens, V. W., Jones, M. M., Khader, K., Rubin, M. A., et al. (2017). Attributable Mortality of Healthcare-Associated Infections Due to Multidrug-Resistant Gram-Negative Bacteria and Methicillin-Resistant Staphylococcus aureus. Infect. Control. Hosp. Epidemiol. 38, 848–856. doi: 10.1017/ice.2017.83
O’Driscoll, T., and Crank, C. W. (2015). Vancomycin-resistant enterococcal infections: epidemiology, clinical manifestations, and optimal management. Infect. Drug Resist. 8, 217–230. doi: 10.2147/idr.s54125
Pinholt, M., Østergaard, C., Arpi, M., Bruun, N. E., Schønheyder, H. C., Gradel, K. O., et al. (2014). Incidence, clinical characteristics and 30-day mortality of enterococcal bacteraemia in Denmark 2006–2009: a population-based cohort study. Clin. Microbiol. Infect. 20, 145–151. doi: 10.1111/1469-0691.12236
Pujol, M., Peña, C., Pallares, R., Ariza, J., Ayats, J., Dominguez, M. A., et al. (1996). Nosocomial Staphylococcus aureus bacteremia among nasal carriers of methicillin-resistant and methicillin-susceptible strains. Am. J. Med. 100, 509–516. doi: 10.1016/s0002-9343(96)00014-9
Qin, Y. X., Li, Q. D., Wan, X. Y., Bi, L. Y., and Wang, J. (2016). [Investigation of molecular epidemiology of methicillin-resistant Staphylococcus aureus in department of critical care medicine]. Zhonghua Yi Xue Za Zhi 96, 3324–3328.
Rodríguez, O., Alvarez, F., Oltra, R., Cereijo, E., Latorre, M. M., and Martínez, H. (2009). Use of linezolid in critically ill patients admitted to intensive care units. Rev. Esp. Quimioter. 22, 68–75.
Rodvold, K. A., and McConeghy, K. W. (2014). Methicillin-Resistant Staphylococcus aureus Therapy: Past, Present, and Future. Clin. Infect. Dis. 58(Suppl._1), S20–S27.
Ruiz-Ripa, L., Bellés, A., García, M., and Torres, C. (2021). Detection of a cfr-positive MRSA CC398 strain in a pig farmer in Spain. Enfermedades Infecciosas Microbiol. Clín. 39, 139–141. doi: 10.1016/j.eimce.2020.03.007
Ruiz-Ripa, L., Feßler, A. T., Hanke, D., Eichhorn, I., Azcona-Gutiérrez, J. M., Pérez-Moreno, M. O., et al. (2020). Mechanisms of Linezolid Resistance Among Enterococci of Clinical Origin in Spain-Detection of optrA- and cfr(D)-Carrying E. faecalis. Microorganisms 8:1155. doi: 10.3390/microorganisms8081155
Sassi, M., Guérin, F., Zouari, A., Beyrouthy, R., Auzou, M., Fines-Guyon, M., et al. (2019). Emergence of optrA-mediated linezolid resistance in enterococci from France, 2006–16. J. Antimicrob. Chemother. 74, 1469–1472. doi: 10.1093/jac/dkz097
Sierra, J. M., Camoez, M., Tubau, F., Gasch, O., Pujol, M., Martin, R., et al. (2013). Low Prevalence of Cfr-Mediated Linezolid Resistance among Methicillin-Resistant Staphylococcus aureus in a Spanish Hospital: Case Report on Linezolid Resistance Acquired during Linezolid Therapy. PLoS One 8:e59215. doi: 10.1371/journal.pone.0059215
Suetens, C., Latour, K., Kärki, T., Ricchizzi, E., Kinross, P., Moro, M. L., et al. (2018). Prevalence of healthcare-associated infections, estimated incidence and composite antimicrobial resistance index in acute care hospitals and long-term care facilities: results from two European point prevalence surveys, 2016 to 2017. Eurosurveillance 23:1800516.
Twilla, J. D., Finch, C. K., Usery, J. B., Gelfand, M. S., Hudson, J. Q., and Broyles, J. E. (2012). Vancomycin-resistant Enterococcus bacteremia: An evaluation of treatment with linezolid or daptomycin. J. Hospit. Med. 7, 243–248. doi: 10.1002/jhm.994
Vega, S., and Dowzicky, M. J. (2017). Antimicrobial susceptibility among Gram-positive and Gram-negative organisms collected from the Latin American region between 2004 and 2015 as part of the Tigecycline Evaluation and Surveillance Trial. Ann. Clin. Microbiol. Antimicrob. 16:50.
Vincent, J.-L., Bihari, D. J., Suter, P. M., Bruining, H. A., White, J., Nicolas-Chanoin, M.-H., et al. (1995). The Prevalence of Nosocomial Infection in Intensive Care Units in Europe: Results of the European Prevalence of Infection in Intensive Care (EPIC) Study. JAMA 274, 639–644. doi: 10.1001/jama.1995.03530080055041
Xanthopoulou, K., Peter, S., Tobys, D., Behnke, M., Dinkelacker, A. G., Eisenbeis, S., et al. (2020). Vancomycin-resistant Enterococcus faecium colonizing patients on hospital admission in Germany: prevalence and molecular epidemiology. J. Antimicrob. Chemother. 75, 2743–2751.
Yadav, G., Thakuria, B., Madan, M., Agwan, V., and Pandey, A. (2017). Linezolid and Vancomycin Resistant Enterococci: A Therapeutic Problem. J. Clin. Diagn. Res. 11, GC07–GC11.
Yayan, J., Ghebremedhin, B., and Rasche, K. (2015). No Outbreak of Vancomycin and Linezolid Resistance in Staphylococcal Pneumonia over a 10-Year Period. PLoS One 10:e0138895. doi: 10.1371/journal.pone.0138895
Yim, J., Smith, J. R., and Rybak, M. J. (2017). Role of Combination Antimicrobial Therapy for Vancomycin-Resistant Enterococcus faecium Infections: Review of the Current Evidence. Pharmacother. J. Hum. Pharmacol. Drug Ther. 37, 579–592. doi: 10.1002/phar.1922
Zhang, Y., Dong, G., Li, J., Chen, L., Liu, H., Bi, W., et al. (2018). A high incidence and coexistence of multiresistance genes cfr and optrA among linezolid-resistant enterococci isolated from a teaching hospital in Wenzhou, China. Eur. J. Clin. Microbiol. Infect. Dis. 37, 1441–1448. doi: 10.1007/s10096-018-3269-8
Keywords: vancomycin-resistant Enterococcus faecium, methicillin-resistant Staphylococcus aureus, daptomycin, linezolid, last-resort antibiotics, antimicrobial resistance
Citation: Markwart R, Willrich N, Eckmanns T, Werner G and Ayobami O (2021) Low Proportion of Linezolid and Daptomycin Resistance Among Bloodborne Vancomycin-Resistant Enterococcus faecium and Methicillin-Resistant Staphylococcus aureus Infections in Europe. Front. Microbiol. 12:664199. doi: 10.3389/fmicb.2021.664199
Received: 04 February 2021; Accepted: 06 May 2021;
Published: 31 May 2021.
Edited by:
Elisabeth Grohmann, Beuth Hochschule für Technik Berlin, GermanyReviewed by:
Carmen Torres, University of La Rioja, SpainAnkita Vaishampayan, Beuth Hochschule für Technik Berlin, Germany
Copyright © 2021 Markwart, Willrich, Eckmanns, Werner and Ayobami. This is an open-access article distributed under the terms of the Creative Commons Attribution License (CC BY). The use, distribution or reproduction in other forums is permitted, provided the original author(s) and the copyright owner(s) are credited and that the original publication in this journal is cited, in accordance with accepted academic practice. No use, distribution or reproduction is permitted which does not comply with these terms.
*Correspondence: Olaniyi Ayobami, ayobamio@rki.de
†These authors have contributed equally to this work