- Division of Infectious Diseases, Rhode Island Hospital, Alpert Medical School and Brown University, Providence, RI, United States
There is a drought of new antibacterial compounds that exploit novel targets. Thioredoxin reductase (TrxR) from the Gram-positive bacterial antioxidant thioredoxin system has emerged from multiple screening efforts as a potential target for auranofin, ebselen, shikonin, and allicin. Auranofin serves as the most encouraging proof of concept drug, demonstrating TrxR inhibition can result in bactericidal effects and inhibit Gram-positive bacteria in both planktonic and biofilm states. Minimal inhibitory concentrations are on par or lower than gold standard medications, even among drug resistant isolates. Importantly, existing drug resistance mechanisms that challenge treatment of infections like Staphylococcus aureus do not confer resistance to TrxR targeting compounds. The observed inhibition by multiple compounds and inability to generate a bacterial genetic mutant demonstrate TrxR appears to play an essential role in Gram-positive bacteria. These findings suggest TrxR can be exploited further for drug development. Examining the interaction between TrxR and these proof of concept compounds illustrates that compounds representing a new antimicrobial class can be developed to directly interact and inhibit the validated target.
Introduction
Years of antibiotic misuse and over prescription has taken a toll on the current drug arsenal, resulting in the emergence and expansion of drug resistant microbes. Even with improved hygiene practices and the implementation of antibiotic stewardship, the pharmacopeia is insufficient to remedy the evolving problem. There is a definitive need to develop new antimicrobial agents and define novel bacterial targets. Measures have been taken to engage in high throughput screens to identify previously unrecognized antimicrobial compounds and their respective targets (Moy et al., 2006; Hu et al., 2014; Katzianer et al., 2014; Rajamuthiah et al., 2014; Kim et al., 2015; Bageshwar et al., 2016; Ollinger et al., 2019). Here we pose that thioredoxin reductase (TrxR), part of the antioxidant thioredoxin system (TS), can serve as a new antimicrobial target in Gram-positive and a limited number of Gram-negative bacteria. Cells are constantly bombarded by reactive oxygen species, coming from environmental niches, hostile hosts in the form of immune responses, or even from the common task of metabolism and cellular respiration. Failure to maintain redox homeostasis leads to apoptosis.
Several compounds are recognized for inhibiting TrxR and provide proof of concept for validating this TrxR as an antimicrobial target. Within this review, we explore auranofin, shikonin, ebselen, and allicin as compounds that inhibit TrxR and remark on the antimicrobial activity profiles. The collective perspective is that TrxR can be targeted through new drug development with the aim of impacting predominately Gram-positive and glutathione independent bacteria.
Thioredoxin System
The TS is present in all living cells, inclusive of bacteria and fungi. Foremost, it serves as an antioxidant system through the conversion of thiol and disulfide bonds (Arnér and Holmgren, 2000). The TS is comprised of two antioxidant oxidoreductase proteins: thioredoxin (Trx) and TrxR, and an electron donor in the form of NADPH (Karlenius and Tonissen, 2010; Figure 1A; Table 1).
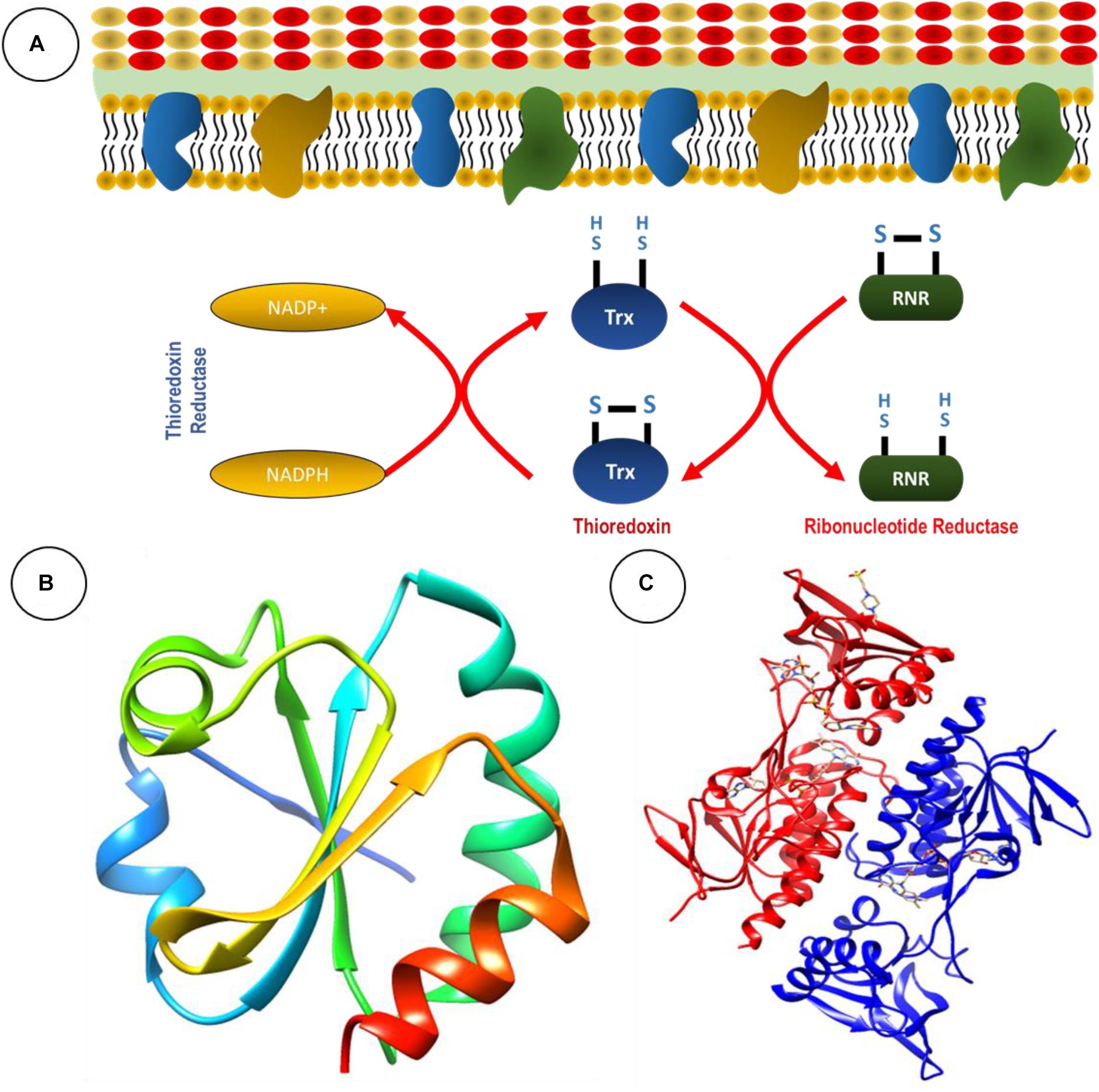
Figure 1. The thioredoxin system serves as an antioxidant system. (A) Basic chemical reaction of thioredoxin system in bacteria. Ribbon structure of (B) thioredoxin and (C) thioredoxin reductase from Staphyloococcus aureus generated through UCSF Chimera 1,14 software.
Thioredoxin is a 10- to 12-kDa ubiquitous protein with a conserved catalytic site (Cys-Pro-Gly-Cys) (Lu and Holmgren, 2014). The primary role of Trx is to serve as a disulfide oxidoreductase and interact with a broad range of proteins involved in electron transport during substrate reduction or regulate the activity by controlling thiol-redox (Lu and Holmgren, 2014). Trx exerts influence of the overall redox system by reducing ribonucleotide reductase during DNA and protein repair and regulating transcription factors, suggesting significant involvement in cellular redox homeostasis (Lu and Holmgren, 2014). The ribbon structures of thioredoxin and TrxR from Staphylococcus aureus are given in Figures 1B,C.
Thioredoxin reductase is part of the pyridine nucleotide-disulfide oxidoreductase family. The average subunit mass in bacteria, archaea, and lower eukaryotes is approximately 35 kDa (Williams et al., 2000). Some of the familiar examples are mercuric ion reductase, lipoamide dehydrogenase, glutathione reductase, and NADH oxidase (Lu and Holmgren, 2014). Prokaryotic TrxR have high substrate specificity (Becker et al., 2000; Gromer et al., 2004), while NADPH and FAD are the common binding sites present in TrxR of bacteria (Gromer et al., 2004). In the process of TrxR catalysis, NADPH reduces the FAD of TrxR, and then FAD reduces Trx by disulfide exchange. This mechanism provides reducing equivalents to other target proteins essential for maintaining cellular redox homeostasis (Becker et al., 2000).
Thioredoxin reductase contains the rare amino acid selenocysteine (Sec), making it a selenoprotein. Sec is a cysteine analog in which selenium replaces the sulfur present in the cysteine. It is a rare naturally occurring amino acid, known to be in 54 human proteins (Parsonage et al., 2016). The sequence UGA normally act as a stop codon; however, when the UGA codon is followed by an Sec insertion sequence (SecIS) in bacteria Sec is added through co-translational insertion (Parsonage et al., 2016).
Thioredoxin reductase importance in maintaining systemic redox homeostasis has been noted not only in Gram-positive bacteria but several parasites as well, where researchers have shown interruption of the enzyme presents a potential therapeutic target (Bonilla et al., 2008; Angelucci et al., 2009; Debnath et al., 2013; Andrade and Reed, 2015; Parsonage et al., 2016). Parsonage and colleagues examined TrxR structure from the parasite Entamoeba histolytica using crystallography and found auranofin interaction with Cys286 (Parsonage et al., 2016). However, a Cys286 TrxR mutant retained catalytic activity and auranofin susceptibility leading to the suggestion that it is a decoy site and binding is not fully dependent on this interaction. The authors also suggest that auranofin interaction alone with TrxR may not be sufficient to inhibit the E. histolytica, indicating the potential for additional targets. Angelucci et al. (2009) present alternative findings in the parasite Schistosoma mansoni where a glutaredoxin domain is fused with a TrxR domain to create thioredoxin-glutathione reductase. It is indicated by the data of this study, that auranofin interacts with thioredoxin-glutathione reductase through a selenocysteine mediated transfer of gold from auranofin to Cys sites and gold provides the inhibitory activity, essentially making auranofin the pro-drug for gold delivery (Angelucci et al., 2009). It is not yet be fully elucidated how auranofin interaction with TrxR in Gram-positive bacteria leads to inhibition but there is the potential that the CXXC motif could be a target. Another parasite, Leishmania infantum, offers insight into auranofin interference of the redox system by inhibiting trypanothione reductase, an enzyme that replaces many of the thioredoxin/TrxR and glutathione/glutathione reductase functions. Looking at the X-ray crystal structure of auranofin-trypanothione reductase-NADPH complex, gold was found bound to two cysteine residues (Cys52 and Cys57) while the sugar moiety bound to the docking site from another parasite, L. infantum (Ilari et al., 2012).
Where we have seen potential to target TrxR in Gram-positive bacteria and the functional orthologs in parasites, this opportunity is lacking for Gram-negative bacteria. In most Gram-negative bacteria, the TS is backed up by a glutathione-glutaredoxin (GSH) system that is also able to scavenge reactive oxygen and nitrogen species in order to maintain redox homeostasis (Couto et al., 2016). Therefore, TrxR lacks the essential nature in Gram-negative bacteria that it plays in Gram-positive bacteria. The GSH system is comprised of NADPH, glutathione reductase, glutathione, and glutaredoxin (Lu and Holmgren, 2014). The Gram-negative Helicobacter pylori proves to be an exception to the classification rule as a GSH-independent bacterium (Lu and Holmgren, 2014). Further, Mycobacterium tuberculosis, which does not fit within the Gram classification, is TrxR-dependent and GSH-independent (Lu and Holmgren, 2014). This suggests that compounds directly inhibiting the TS, especially through TrxR, hold the potential to inhibit medically important Gram-positive bacteria such as Staphylococcus spp., Streptococcus spp., Enterococcus spp., or the exceptional GSH independent bacteria H. pylori and M. tuberculosis.
Reliance on Trx and TrxR in GSH-Independent Bacteria
Helicobacter pylori is an interesting organism for TS studies as one of the only Gram-negative bacteria to be GSH-independent. The important antioxidants TS system in this bacterium is comprised of one TrxR and two Trx (Trx1 and Trx2) proteins (Table 1; Windle et al., 2000). Though most bacteria fit within the Gram classification system, M. tuberculosis is another bacterium that does not, and also represents a GSH-independent bacterium utilizing the TS. It has three Trx proteins, TrxA, TrxB, and TrxC, but only one TrxR (Table 1). However, M. tuberculosis TrxR does not transfer electrons to TrxA, making TrxB and TrxC the recipients and the active disulfide reductases (Trivedi et al., 2012). M. tuberculosis also has thiol peroxidase (Tpx) and alkyl hydroperoxide peroxidase (AhpC) that respond to oxidative and nitrosative stresses imposed by macrophages. Similarly, H. pylori also utilizes AhpC as an essential antioxidant for maintaining redox hemostasis (Baker et al., 2001). In M. tuberculosis, TrxB transfers electrons to Tpx, and TrxC can transfer to either Tpx or AhpC (Lu and Holmgren, 2014). As GSH-independent bacteria, H. pylori and M. tuberculosis are susceptible to TrxR targeting compounds, both demonstrating sensitivity to auranofin (Harbut et al., 2015; Owings et al., 2016). Thus, GSH designation as dependent or independent has thus far proven to influence bacterial susceptibility to TrxR targeting compounds and presents a new bacterial classification outside of the well-defined Gram system.
TrxR Inhibitors and the Impact to Microbes
There are several drugs and molecules that target TrxR. Two naturally occurring compounds, shikonin and allicin (Figure 2), are produced by plant roots, presumptively to combat bacteria in the rhizosphere, an indication that nature has already identified TrxR as an antimicrobial target site. Screening efforts to explore the pharmacopeia for antimicrobial compounds also identified auranofin and ebselen for antimicrobial properties that appear to also target TrxR (Figure 2). By understanding and repurposing these molecules, we can define a path toward building a new class of antimicrobial compounds that specifically target bacterial TrxR.
Among the four molecules, auranofin has the lowest minimal inhibitory concentration (MIC) against most of the Gram-positive bacteria. Auranofin has shown efficacy toward S. aureus (0.0625–1 μg/mL), Enteroccocus faecalis (0.125–0.5 μg/mL), Enterococcus faecium (0.125–0.25 μg/mL), Clostridium difficile (0.25–4 μg/mL), Bacillus subtilis (0.3–0.05 μg/mL), Streptococcus pneumoniae (0.025–0.25 μg/mL), and Streptococcus agalactiae (0.0625–0.0015 μg/mL) (Harbut et al., 2015; Thangamani et al., 2016a; AbdelKhalek et al., 2019). The MIC of shikonin against Gram-positive bacteria including drug resistant methicillin-resistant S. aureus (MRSA) ranged between 6.5 and 31.2 μg/mL, thus showing a propensity to inhibit bacteria but not with the same impact as auranofin (Vegara et al., 2011; Lee et al., 2015).
Ebselen exhibited efficacy at inhibiting Gram-positive bacteria including: B. subtilis, Bacillus cereus, S. aureus [MRSA, Linezolid-resistant S. aureus, Mupirocin-resistant S. aureus, vancomycin resistant S. aureus (VRSA), and vancomycin intermediate S. aureus (VISA)] (MIC 0.125–0.64 μg/mL), Staphylococcus epidermidis (MIC 0.5 μg/mL), E. faecium (MIC 0.25–4 μg/mL), E. faecalis (MIC 0.125–2 μg/mL), Streptococcus pyogenes (MIC 0.5 μg/mL), and S. agalactiae (MIC 0.5 μg/mL) (Thangamani et al., 2015a, b; Gustafsson et al., 2016; AbdelKhalek et al., 2019). Allicin is the least potent among the group with activity against Gram-positive bacteria like S. aureus at an MIC of 32–64 μg/mL (Leng et al., 2011).
Auranofin
Auranofin is an FDA approved gold containing compound used to treatment rheumatoid arthritis. Since auranofin has been used since the mid 1980s and was even provided in studies to children ages 1–17, information is available related to drug safety at therapeutic dosages pertinent to arthritis (Kean et al., 1997). When provided orally at 6 mg/day, 25% of the administered compound is detected in the plasma bound to albumin with a half-life of 15–25 days. Approximately 55–80 days is required to fully eliminate the drug with excretion routes being urine and feces. Bioavailability is 40 and 60% protein binding (Finkelstein et al., 1976; Kean et al., 1997). The most common side effect encountered when using auranofin to treat arthritis was diarrhea and skin rash (Felson et al., 1990). Diarrhea was experienced by 40% of patients and rash occurred in 20% of patients (Kean et al., 1997). Proteinuria was also reported in 5% of treated patients and is hypothesized to be a result of gold or gold complexes from auranofin damaging the renal tubule. Auranofin manifest reduction in arthritis stiff joints, reduces the number of swollen joints, and improves grip strength through eliciting a reduction in blood IgG, decreasing α2-globulin, increasing albumin ratios, and decreasing rheumatoid factor titers (Finkelstein et al., 1976).
Auranofin Bacterial Inhibitory Mechanism
A high throughput screen identified auranofin for prolonging the survival of the invertebrate model Caenorhabditis elegans infected with S. aureus. The results demonstrated that auranofin could clear bacteria from the host at low concentrations (0.78 mg/mL), but the inhibitory impact was reduced by secondary oxidative scavenger glutathione (Fuchs et al., 2016). Indeed, auranofin has been identified by other screening efforts. In 2016, auranofin emerged as a hit when searching for compounds that disrupt preformed S. aureus biofilm (Torres et al., 2016).
The S. aureus target for auranofin was confirmed when it was shown to provide dose dependent inhibition of bacterial TrxR. Enzymatic activity was evaluated through a colorimetric assay where DTNB is reduced with NADPH to become TNB in an assay catalyzed by TrxR. The reaction produces a yellow color but the color is reduced by the presence of a TrxR inhibitor (Tharmalingam et al., 2019).
This was not the first implication that auranofin could inhibit TrxR. Using the colorimetric assay where DTNB is reduced with NADPH to become TNB in the presence of TrxR, Harbut and colleagues showed auranofin appears to directly reduce the enzymatic activity of M. tuberculosis and S. aureus TrxR in a dose-dependent manner (Harbut et al., 2015). Lin et al. (2016) used a conditional mutant to show TrxR targeting reduced bacterial burden in a mouse infection model, concluding that auranofin targets TrxB2 but acknowledge this may not be the only enzyme affected.
There are possibly other targets or influences asserted by auranofin. Thangamani et al. (2016a) suggested that auranofin has antibacterial activity against Gram-positive bacteria by inhibiting several biosynthesis mechanisms or pathways like protein, DNA, and cell wall synthesis. The authors observed suppressed protein synthesis in S. aureus leading to reduced MRSA toxin productivity.
Auranofin Inhibits Bacterial Pathogens
Auranofin is able to inhibit a significant cache of bacteria and exhibited antibacterial activity against non-replicating and replicating strains of M. tuberculosis under nutrient deprivation. After 5 days, 1.3 and 3.7 log reductions in bacterial viability were recorded when treated with 100 nM and 1.0 μM of auranofin, respectively (Harbut et al., 2015). The MIC of auranofin against M. tuberculosis (ΔpanCD,ΔRD1) and M. tuberculosis H37Ra was 0.5 μg/mL, while the MIC of B. subtilis was between 0.3 and 0.05 μg/mL (Harbut et al., 2015). Clinical isolates of C. difficile were inhibited up to 50% by 1 μg/mL of auranofin. At this concentration, the toxin productivity and spore formation of C. difficile were also inhibited. Clinical and reference strains of C. difficile exhibited an MIC between 0.25 and 4 μg/mL. Reference strains of E. faecalis and E. faecium had an MICs ranging between 0.125–1 and 0.25–4 μg/mL, respectively (Harbut et al., 2015; Thangamani et al., 2016a; AbdelKhalek et al., 2019).
Staphylococcus aureus has demonstrated susceptible to auranofin, inclusive of drug resistant isolates. Reference strains, clinical isolates, MRSA, VISA, VRSA, linezolid resistant S. aureus, and glycopeptide intermediate S. aureus exhibited MICs ranging between 0.0625 and 1 μg/mL (Harbut et al., 2015; Thangamani et al., 2016a; AbdelKhalek et al., 2019). In 2019, auranofin was tested to have an MIC ranging between 0.125 and 1 μg/mL against 503 clinical isolates of S. aureus (Tharmalingam et al., 2019). S. agalactiae also demonstrated susceptibility with MICs ranging between 0.0625 and 0.0015 μg/mL. Thus, showing susceptibility of Gram-positive bacteria and lack of resistance, even among drug resistant isolates.
Antimicrobial Efficacy in Animal Models
Auranofin antimicrobial efficacy extends beyond in vitro data and was found to have an in vivo impact by prolonging survival of mice infected with Gram-positive bacteria in several reports (Table 2). Provision of auranofin in systemic, peritonitis, and topical infection mouse models inhibited MRSA, demonstrated through bacterial load reduction (Thangamani et al., 2016b).
Oral administration of auranofin (0.125 or 0.25 mg/kg) promoted survival in 80% of mice post MRSA infection in a lethal septicemic mouse model. Similar survival rates (80%) were observed in mice treated with linezolid (25 mg/kg) showing comparable results with a standard of care medication. Further, auranofin reduced mean bacterial load by 90% in the liver compared to a 70% reduction in animals treated with linezolid. Auranofin given to mice infected with a non-lethal dose of MRSA USA300 reduced the bacterial load up to 95% in the spleen (Thangamani et al., 2016b). In another infection model, Harbut et al. (2015) tested auranofin in CD1 female mice using a MRSA peritonitis model. The animals were injected with 0.12 or 0.012 mg/kg of auranofin daily. After treatment, four out of eight mice (50%) experienced prolonged survival by day 7 when provided with 0.12 mg/kg auranofin compared to three out of eight mice (37.5%) survived to day 7 of the study when provided with 0.012 mg/kg (Harbut et al., 2015).
The in vivo efficacy of auranofin was also tested against the drug resistant strain S. pneumoniae 48 (serotype) in a mouse bacteremia model. After auranofin treatment at the concentrations of 1, 5, or 10 mg/kg mouse survival was observed at 50, 30, and 20%, respectively within a 96-h period. The same time interval results in 100% mortality of infected mice in the placebo group. Dosing with 1 mg/kg auranofin was determined to significantly prolong survival and no trace of bacteria was reported in tail vein collected blood samples 24 h after of infection (Aguinagalde et al., 2015). The greater efficacy at lower doses of 1 and 5 mg/kg suggested in this study by Aguinagalde et al. (2015) implicate potential influence by the paradoxical Eagle effect as seen with other antibiotics, a phenomenon where a decrease in net cell death occurs when drug concentrations exceed optimal bactericidal levels (Eagle, 1948; Prasetyoputro et al., 2019).
The same study interrogated MRSA strain 132 susceptibility to auranofin in an intramuscular infection model. At 24 h post infection, bacteria were enumerated revealing a significant reduction when provided with 5 mg/kg auranofin compared to untreated mice (Aguinagalde et al., 2015). Using the same 5 mg/kg auranofin concentration, it was also reported to reduce MRSA biofilm seeded onto mesh implanted intraperitoneally. After 6 days, auranofin daily therapy significant reduced the number of S. aureus CFUs recovered from the recovered mesh compared to untreated mice (Aguinagalde et al., 2015).
Broad Scope Efficacy
There is evidence that auranofin impact on infectious diseases extends beyond Gram-positive bacteria and includes parasites. Auranofin inhibits E. histolytica, a parasite that causes diarrhea, by targeting TrxR (Andrade and Reed, 2015; Parsonage et al., 2016). It also affects Giardia lamblia and S. mansoni (Andrade and Reed, 2015). It is from these parasitic interactions that we gain valuable insight into the physical interaction of auranofin with the TrxR target. The ability to inhibit both bacteria and parasites lend to the potential of elevating auranofin or other effective TrxR targeting compounds to become broad-spectrum anti-infectious therapeutic agents.
Ebselen
Like auranofin, the antimicrobial activity for ebselen was also revealed in a high throughput screening effort to find compounds that inhibit S. aureus and was found to have greater impact on planktonic cells than biofilm (Torres et al., 2016). Ebselen, chemically known as 2-phenyl-1,2-benzisoselenazol-3(2H)-one, is a seleno-organic, non-toxic drug reported to have antiatherosclerotic, anti-inflammatory, and cytoprotective properties (Schewe, 1995). It was initially developed to mimic the structure of glutathione peroxidase (Müller et al., 1984), but also reacts with peroxynitrite and can inhibit enzymes such as lipoxygenases, NO synthases, NADPH oxidase, protein kinase C, and H+/K+-ATPase (Parnham and Sies, 2000). In mammalian cells, ebselen is a favorable substrate for TrxR dependent detoxification of hydroperoxides (Müller et al., 1984).
Under in vitro analysis, ebselen exhibited antibacterial activity against MRSA strains USA300, USA100, USA200, USA500, USA1000, USA1100, linezolid resistant, VRSA and mupirocin resistant strains of S. aureus (King et al., 2006; Thangamani et al., 2015a), helping to bolster the idea that there is S. aureus broad scope susceptibility regardless of strain or drug resistance characteristics. Indeed, the inhibitory activity against S. aureus was robust with an MIC at 0.5 mg/mL (Thangamani et al., 2015a). The observed antimicrobial activity extends to other Gram-positive bacteria such as Streptococcus and Enterococcus. Once again, H. pylori proved to be an exceptional Gram-negative bacteria, exhibiting sensitivity to ebselen with an MBC at 3.13–12.5 mg/mL and an MIC at 3.13 mg/mL for various isolates (Lu et al., 2013). Additionally, M. tuberculosis demonstrated ebselen sensitivity with an MIC at 20 μg/mL, congruent to findings with auranofin (Lu et al., 2013). Susceptible bacteria did not easily develop resistance to ebselen as demonstrated by Gustafsson et al. (2016) who were unable to induce S. aureus or B. subtilis resistance after extended exposure. This could be due to the essential nature of the currently identified TrxR target or suggest the compound has a larger impact and affects other cellular systems.
By measuring Trx reduction in a colorimetric assay with DTNB, Lu et al. (2013) demonstrate that ebselen acts as a competitive inhibitor to E. coli TrxR. When ebselen was removed from the reaction through membrane filtration, 80% of TrxR activity was restored (Lu et al., 2013). However, unlike auranofin, which was effective at reducing bacterial growth and lysed M. tuberculosis, ebselen did not work via TrxB inhibition and likely affects alternative targets in this bacterium (Lin et al., 2016).
Shikonin
The napthoquinone shikonin is a Chinese herbal medicine, also known as Zicao, isolated from Lithospermum erythrorhizon roots, a plant known for wound healing and anti-inflammatory effects (Andujar et al., 2013; Duan et al., 2014; Lee et al., 2015). There are several Gram-positive bacteria with demonstrated sensitivity to shikonin: S. aureus, E. faecium, and B. subtilis, with MICs ranging from 0.3 to 6.25 mg/mL (Andujar et al., 2013). Shikonin also prevents H. pylori growth in a dose dependent manner, reaching 86% inhibition at 40 μM (Kuo et al., 2004).
Duan and colleagues report that shikonin is yet another compound that interacts with cytosolic TrxR. The compound targets the Sec residue of the Gly-Cys-Sec-Gly active site motif of the human TrxR and irreversibly inhibits the enzyme (Duan et al., 2014). This interaction inhibits TrxR activity without depleting the cellular accumulation of the enzyme (Duan et al., 2014). Shikonin activity can be inhibited by the inclusion of N-acetyl-L-cysteine, a known antioxidant and a precursor to GSH, which neutralizes ROS as part of the alternate glutathione synthase pathway, or through the over expression of TrxR itself (Duan et al., 2014). Thus, shikonin appears to target the TS antioxidant activity as asserted by antagonistic effect when alternate cellular antioxidants are included. Although shikonin inhibits S. aureus, it does not reach the same impact as auranofin which exhibited lower MICs. The lesser inhibitory activity is likely due to impeded cellular entry due to factors that restrict shikonin passive entry. It is likely impeded by the cell membrane but inclusion of membrane permeabilization agents such as Triton X-100 enhances antimicrobial activity against S. aureus (Lee et al., 2015). Entry challenges are affirmed when peptidoglycans is added and shows competitive inhibition (Lee et al., 2015).
Although it has been used in an animal models, there are distinct challenges that impede shikonin moving forward as a widely antimicrobial therapeutic agent such as poor solubility. Also, shikonin can elicit adverse toxic effects. Although shikonin presents challenges of advancing as an antimicrobial agent in a clinical setting, it does highlight TrxR as a viable antibacterial target and present scaffolding structure that can be built upon for improved activity. And, importantly, it provides an example of a natural product that targets TrxR.
Allicin
Another natural plant derived compound that exerts antimicrobial effects is allicin, a biologically active oxygenated sulfur compound present in freshly crushed garlic extract that is chemically known as thio-2-propene-1-sulfinic acid S-allyl ester (Ankri and Mirelman, 1999). In 1944, Cavallito and Bailey (1944), first identified allicin and reported the antibacterial activity of diluted allicin against Gram-positive and Gram-negative bacteria. Later, in 1970, the antifungal activity of allicin was revealed (Feldberg et al., 1988). It was further identified to have antiparasitic activity against E. histolytica and G. lamblia (Ankri and Mirelman, 1999).
Allicin exerts antibacterial activity against S. aureus in combination or synergistically with cefazolin/oxacillin and cefoperazone (Cai et al., 2007). When allicin is provided at 100 μg/mL it prevents S. aureus biofilm formation on implant materials like reticular polypropylene meshes used in hernia repair (Pérez-Köhler et al., 2015a, b). When biofilm inhibitory activity was tested in an in vivo rabbit model, allicin (delivered at 4 mg/L) significantly inhibited the formation of S. epidermidis biofilm formation (Zhai et al., 2014).
Like auranofin, allicin is also able to inhibit H. pylori, possibly due to the GSH-independent status of the microbes (Jonkers et al., 1999; Aydin et al., 2000; Koçkar et al., 2001). The MIC and MBC were both measured at 6 μg/mL against H. pylori (O’Gara et al., 2000). In a cohort consisting of 210 H. pylori positive patients, a group (n = 30) treated with standard care medications lansaprasol, clarithromycin, and amoxicillin experienced eradication in 66.6% of the group. When allicin was added to the treatment regimen at 4200 μg/day eradication reached 90% within the group (Koçkar et al., 2001).
Mycobacterium tuberculosis was also sensitive to allicin, exhibiting MICs ranging from 2.58 to 33.33 μg/mL among drug resistant and drug susceptible isolates (Dwivedi et al., 2019). With multiple types of microbes inhibited by allicin, it is not surprising that its mode action is promiscuous. Allicin undergoes a chemical reaction with thiol groups present in several enzymes including TrxR, RNA polymerase, and alcohol dehydrogenase (Ankri and Mirelman, 1999). Muller et al. (2016) measured total sulfhydryl concentrations and demonstrated a reduction using crude extracts from cells treated with allicin. Sulfhydryl levels were quantified with DTNB (described above) and showed that TrxR is one of the targeted enzymes.
Cidal and Static Activity
Natural and synthetic drugs exhibit two types of inhibitory effects on bacteria: bacteriostatic and bactericidal. The MIC is the concentration at which a compound inhibits the visible growth of bacterial at 24 h under optimum condition. While the minimum bactericidal concentration (MBC) is defined as the point at which a compound causes 1,000 fold bacterial density reduction at 24 h under standardized growth conditions (Wald-Dickler et al., 2018). Bactericidal activity is defined when the ratio of MBC to MIC is ≤4 and bacteriostatic activity is defined as the MBC to MIC ratio is >4. Therefore, a drug which achieves reduction of >1,000 fold in density of bacteria at concentration eight-fold above the MIC is considered bacteriostatic (Pankey and Sabath, 2004; French, 2006; Nemeth et al., 2015; Wald-Dickler et al., 2018). Thus, effective bactericidal compound needs to have an MBC close to the MIC.
Auranofin has been reported to exhibit bactericidal activity against Gram-positive bacteria like B. subtilis, C. difficile, E. faecalis, E. faecium, S. aureus (including MRSA and VISA strains), S. epidermidis, S. pneumoniae, and S. agalactiae. Reports also suggest bactericidal activity of auranofin against M. tuberculosis (Harbut et al., 2015; Thangamani et al., 2016b; AbdelKhalek et al., 2019). The MBC to MIC ratio of shikonin is reported above >4 against various strains of S. aureus. This suggest that shikonin exhibits bacteriostatic activity (Vegara et al., 2011; Lee et al., 2015). Ebselen shows MBC to MIC ratio is <4 against Gram-positive bacteria like B. subtilis, Enterococcus spp. (including VRE), S. epidermidis, S. aureus (including MRSA, VRSA, and VISA strains), S. pyogenes, and S. agalactiae (Thangamani et al., 2015b; Gustafsson et al., 2016; AbdelKhalek et al., 2018). Apart from these three molecules, allicin is reported to inhibit S. aureus at >32 μg/mL. This suggest that allicin possess bacteriostatic activity. Thus, among these four molecules (auranofin, shikonin, ebselen, and allicin), auranofin is potentially the most active at inhibiting bacteria in a bactericidal capacity.
Conclusion
Although there are differences between auranofin, ebselen, shikonin, and allicin, together, these compounds strongly suggest TrxR is a valid antimicrobial target. Although all of the compounds commonly impact TrxR there is debate as to whether it is the sole target. Indeed, other cellular systems may be augmented.
Only auranofin has been used clinically (with FDA approval) and found to be non-toxic. Therefore, holding the greatest potential to be repositioned for antimicrobial therapy. Interestingly, studies suggest TrxR inhibitors could also have anti-cancer efficacy due to increased growth rates and elevated cellular ROS from increased mitochondrial respiration that accumulate during accelerated growth resulting in high levels of Trx and TrxR to reduce oxidative stressors (reviewed in Mohammadi et al., 2019). Clinical trials are ongoing using a TrxR inhibitor ethaselen to treat non-small cell lung cancers.
Focusing on the common target, each of these compounds have been shown to reduce TrxR enzymatic activity and the inhibition of bacterial growth has been demonstrated with in vitro and in vivo assays. Antimicrobial impact is best reflected in Gram-positive bacteria with the exception of a couple of GSH-independent microbes, namely H. pylori and M. tuberculosis. Multiple studies demonstrate that drug resistant microbes are susceptible to TrxR targeting compounds and resistance is not developed upon exposure. Among GSH-independent bacteria, the essential nature of TrxR makes it a very attractive target. Although this review is restricted to bacterial inhibition, TrxR also poses a potential target in fungi. Indeed, Missall and Lodge (2005) and Ianiri and Idnrum (2015) report the essential nature of fungal TrxR in Cryptococcus neoformans with failure to produce a mutant strain.
The quickest path forward to introducing TrxR as a novel antimicrobial target not yet tapped by current antibiotics is to progress one of the existing compounds as a repurposed drug. With low toxicity and good efficacy at low concentrations, the best candidate for this ascension is auranofin. However, the creation of new compounds specifically designed to target Gram-positive TrxR can initiate a new antimicrobial class.
Author Contributions
BF and LF prepared the initial draft. EM provided insightful discussions and contributed to the writing. All authors contributed to the article and approved the submitted version.
Funding
LF and BF were supported by NIH grant P20 GM121344.
Conflict of Interest
The authors declare that the research was conducted in the absence of any commercial or financial relationships that could be construed as a potential conflict of interest.
References
AbdelKhalek, A., Abutaleb, N. S., Mohammad, H., and Seleem, M. N. (2018). Repurposing ebselen for decolonization of vancomycin-resistant enterococci (VRE). PLoS One 13:e0199710. doi: 10.1371/journal.pone.0199710
AbdelKhalek, A., Abutaleb, N. S., Mohammad, H., and Seleem, M. N. (2019). Antibacterial and antivirulence activities of auranofin against Clostridium difficile. Int. J. Antimicrob. Agents 53, 54–62. doi: 10.1016/j.ijantimicag.2018.09.018
Abutaleb, N. S., and Seleem, M. N. (2020). Auranofin, at clinically achievable dose, protects mice and prevents recurrence from Clostridioides difficile infection. Sci. Rep. 10:7701. doi: 10.1038/s41598-020-64882-9
Aguinagalde, L., Díez-Martínez, R., Yuste, J., Royo, I., Gil, C., and Lasa, Í, et al. (2015). Auranofin efficacy against MDR Streptococcus pneumoniae and Staphylococcus aureus infections. J. Antimicrob. Chemother. 70, 2608–2617. doi: 10.1093/jac/dkv163
Andrade, R. M., and Reed, S. L. (2015). New drug target in protozoan parasites: the tole of thioredoxin reductase. Front. Microbiol. 6:975. doi: 10.3389/fmicb.2015.00975
Andujar, I., Recio, M., Giner, R., and Ríos, J. (2013). Traditional chinese medicine remedy to jury: the pharmacological basis for the use of shikonin as an anticancer therapy. Curr. Med. Chem. 20, 2892–2898. doi: 10.2174/09298673113209990008
Angelucci, F., Sayed, A. A., Williams, D. L., Boumis, G., Brunori, M., Dimastrogiovanni, D., et al. (2009). Inhibition of Schistosoma mansoni thioredoxin-glutathione reductase by auranofin: structural and kinetic aspects. J. Biol. Chem. 284, 28977–28985. doi: 10.1074/jbc.M109.020701
Ankri, S., and Mirelman, D. (1999). Antimicrobial properties of allicin from garlic. Microbes Infect. 1, 125–129.
Arnér, E. S. J., and Holmgren, A. (2000). Physiological functions of thioredoxin and thioredoxin reductase. Eur. J. Biochem. 267, 6102–6109. doi: 10.1046/j.1432-1327.2000.01701.x
Aydin, A., Ersöz, G., Tekesin, O., Akçiçek, E., and Tuncyürek, M. (2000). Garlic oil and Helicobacter pylori infection. Am. J. Gastroenterol. 95, 563–564. doi: 10.1016/S0002-9270(99)00871-0
Bageshwar, U., VerPlank, L., Baker, D., Dong, W., Hamsanathan, S., Whitaker, N., et al. (2016). High throughput scree for Escherichia coli twin arginine translocation (Tat) inhibitors. PLoS One 11:e0149659. doi: 10.1371/journal.pone.0149659
Baker, L. M. S., Raudonikiene, A., Hoffman, P. S., and Poole, L. B. (2001). Essential thioredoxin-dependent peroxiredoxin system from Helicobacter pylori: genetic and kinetic characterization. J. Bacteriol. 183, 1961–1973. doi: 10.1128/JB.183.6.1961-1973.2001
Becker, K., Gromer, S., Heiner Schirmer, R., and Müller, S. (2000). Thioredoxin reductase as a pathophysiological factor and drug target. Eur. J. Biochem. 267, 6118–6125. doi: 10.1046/j.1432-1327.2000.01703.x
Bonilla, M., Denicola, A., Novoselov, S. V., Turanov, A. A., Protasio, A., Izmendi, D., et al. (2008). Platyhelminth mitochondrial and cytosolic redox homeostasis is controlled by a single thioredoxin glutathione reductase and dependent on selenium and glutathione. J. Biol. Chem. 283, 17898–17907. doi: 10.1074/jbc.M710609200
Cai, Y., Wang, R., Pei, F., and Liang, B. B. (2007). Antibacterial activity of allicin alone and in combination with β-lactams against Staphylococcus spp. and Pseudomonas aeruginosa. J. Antibiot. 60, 335–338. doi: 10.1038/ja.2007.45
Cavallito, C. J., and Bailey, J. H. (1944). Allicin, the antibacterial principle of allium sativum. I. Isolation, physical properties and antibacterial action. J. Am. Chem. Soc. 66, 1950–1951. doi: 10.1021/ja01239a048
Couto, N., Wood, J., and Barber, J. (2016). The role of glutathione reductase and related enzymes on cellular redox homoeostasis network. Free Radic. Biol. Med. 95, 27–42. doi: 10.1016/j.freeradbiomed.2016.02.028
Debnath, A., Ndao, M., and Reed, S. (2013). Reprofiled drug targets ancient protozoans. Gut Microbes 4, 66–71. doi: 10.4161/gmic.22596
Duan, D., Zhang, B., Yao, J., Liu, Y., and Fang, J. (2014). Shikonin targets cytosolic thioredoxin reductase to induce ROS-mediated apoptosis in human promyelocytic leukemia HL-60 cells. Free Radic. Biol. Med. 70, 182–193. doi: 10.1016/j.freeradbiomed.2014.02.016
Dwivedi, V. P., Bhattacharya, D., Singh, M., Bhaskar, A., Kumar, S., Fatima, S., et al. (2019). Allicin enhances antimicrobial activity of macrophages during Mycobacterium tuberculosis infection. J. Ethnopharmacol. 243:111634. doi: 10.1016/j.jep.2018.12.008
Eagle, H. (1948). A paradoxical zone phenomenon in the bactericidal action of penicillin in vitro. Science 107, 44–45.
Feldberg, R. S., Chang, S. C., Kotik, A. N., Nadler, M., Neuwirth, Z., Sundstrom, D. C., et al. (1988). In vitro mechanism of inhibition of bacterial cell growth by allicin. Antimicrob. Agents Chemother. 32, 1763–1768. doi: 10.1128/AAC.32.12.1763
Felson, D., Anderson, J., and Meenan, R. (1990). The comparative efficacy and toxicity of second-line drugs in rheumatoid. Arthritis Rheum. 33, 1449–1461.
Finkelstein, A. E., Walz, D. T., Batista, V., Mizraji, M., Roisman, F., and Misher, A. (1976). Auranofin. New oral gold compound for treatment of rheumatoid arthritis. Ann. Rheum. Dis. 35, 251–257. doi: 10.1136/ard.35.3.251
French, G. L. (2006). Bactericidal agents in the treatment of MRSA infections - The potential role of daptomycin. J. Antimicrob. Chemother. 58, 1107–1117. doi: 10.1093/jac/dkl393
Fuchs, B. B., Rajamuthiah, R., Souza, A. C. R., Eatemadpor, S., Rossoni, R. D., Santos, D. A., et al. (2016). Inhibition of bacterial and fungal pathogens by the orphaned drug auranofin. Future Med. Chem. 8, 117–132.
Gromer, S., Urig, S., and Becker, K. (2004). The thioredoxin system - from science to clinic. Med. Res. Rev. 24, 40–89. doi: 10.1002/med.10051
Gustafsson, T. N., Osman, H., Werngren, J., Hoffner, S., Engman, L., and Holmgren, A. (2016). Ebselen and analogs as inhibitors of Bacillus anthracis thioredoxin reductase and bactericidal antibacterials targeting Bacillus species, Staphylococcus aureus and Mycobacterium tuberculosis. Biochim. Biophys. Acta Gen. Subj. 1860, 1265–1271. doi: 10.1016/j.bbagen.2016.03.013
Harbut, M. B., Vilchèze, C., Luo, X., Hensler, M. E., Guo, H., Yang, B., et al. (2015). Auranofin exerts broad-spectrum bactericidal activities by targeting thiol-redox homeostasis. Proc. Natl. Acad. Sci. U.S.A. 112, 4453–4458. doi: 10.1073/pnas.1504022112
Hu, Y., Palmer, S., Munoz, H., and Bullard, J. (2014). High throughput screen identified natural product inhibitor of phenylalanyl-tRNA synthetase from Pseudomonas aeruginosa and Streptococcus pneumoniae. Curr. Drug Discov. Technol. 11, 279–292.
Ianiri, G., and Idnrum, A. L. (2015). Essential gene discovery in the Basidiomycete Cryptococcus neoformans for antifungal drug target prioritization. mBio 6:e02334-14. doi: 10.1128/mBio.02334-14
Ilari, A., Baiocco, P., Messori, L., Fiorillo, A., Boffi, A., Gramiccia, M., et al. (2012). A gold-containing drug against parasitic polyamine metabolism: the X-ray structure of trypanothione reductase from Leishmania infantum in complex with auranofin reveals a dual mechanism of enzyme inhibition. Amino Acids 42, 803–811. doi: 10.1007/s00726-011-0997-9
Jonkers, D., Van Den Broek, E., Van Dooren, I., Thijs, C., Dorant, E., Hageman, G., et al. (1999). Antibacterial effect of garlic and omeprazole on Helicobacter pylori. J. Antimicrob. Chemother. 43, 837–839. doi: 10.1093/jac/43.6.837
Karlenius, T. C., and Tonissen, K. F. (2010). Thioredoxin and cancer: a role for thioredoxin in all states of tumor oxygenation. Cancers 2, 209–232. doi: 10.3390/cancers2020209
Katzianer, D., Yano, T., Rubin, H., and Zhu, J. (2014). A high-throughput small-molecule screen to identify a novel chemical inhibitor of Clostridium difficile. Int. J. Antimicrob. Agents 44, 69–73.
Kim, W., Conery, A. L., Rajamuthiah, R., Fuchs, B. B., Ausubel, F. M., and Mylonakis, E. (2015). Identification of an antimicrobial agent effective against methicillin-resistant Staphylococcus aureus persisters using a fluorescence-based screening strategy. PLoS One 10:e0127640. doi: 10.1371/journal.pone.0127640
King, M. D., Humphrey, B. J., Wang, Y. F., Kourbatova, E. V., Ray, S. M., and Blumberg, H. M. (2006). Emergence of community-acquired methicillin-resistant Staphylococcus aureus USA 300 clone as the predominant cause of skin and soft-tissue infections. Ann. Intern. Med. 144, 309–317. doi: 10.7326/0003-4819-144-5-200603070-00005
Koçkar, C., Oztürk, M., and Bavbek, N. (2001). Helicobacter pylori eradication with beta carotene, ascorbic acid and allicin. Acta Medica 44, 97–100. doi: 10.14712/18059694.2019.92
Kuo, H.-M., Hsia, T.-C., Chuang, Y.-C., Lu, H.-F., Lin, S.-Y., and Chung, J.-G. (2004). Shikonin inhibits the growth and N-acetylation of 2-aminofluorene in Helicobacter pylori from ulcer patients. Anticancer Res. 24, 1587–1592.
Lee, Y. S., Lee, D. Y., Kim, Y. B., Lee, S. W., Cha, S. W., Park, H. W., et al. (2015). The mechanism underlying the antibacterial activity of shikonin against methicillin-resistant Staphylococus aureus. Evid. Based Complement. Altern. Med. 2015:520578. doi: 10.1155/2015/520578
Leng, B. F., Qiu, J. Z., Dai, X. H., Dong, J., Wang, J. F., Luo, M. J., et al. (2011). Allicin reduces the production of α-toxin by Staphylococcus aureus. Molecules 16, 7958–7968. doi: 10.3390/molecules16097958
Lin, K., O’Brien, K. M., Trujillo, C., Wang, R., Wallach, J. B., Schnappinger, D., et al. (2016). Mycobacterium tuberculosis Thioredoxin Reductase is rssential for thiol redox homeostasis but plays a minor role in antioxidant defense. PLoS Pathog. 12:e1005675. doi: 10.1371/journal.ppat.1005675
Lu, J., and Holmgren, A. (2014). The thioredoxin antioxidant system. Free Radic. Biol. Med. 66, 75–87. doi: 10.1016/j.freeradbiomed.2013.07.036
Lu, J., Vlamis-Gardikas, A., Kandasamy, K., Zhao, R., Gustafsson, T. N., Engstrand, L., et al. (2013). Inhibition of bacterial thioredoxin reductase: an antibiotic mechanism targeting bacteria lacking glutathione. FASEB J. 27, 1394–1403. doi: 10.1096/fj.12-223305
Missall, T. A., and Lodge, J. K. (2005). Thioredoxin reductase is essential for viability in the fungal pathogen Cryptococcus neoformans. Eukaryot. Cell 4, 487–489. doi: 10.1128/EC.4.2.487-489.2005
Mohammad, H., Abutaleb, N. S., and Seleem, M. N. (2020). Auranofin rapidly eradicates methicillin-resistant Staphylococcus aureus (MRSA) in an infected pressure ulcer mouse model. Sci. Rep. 10:7251. doi: 10.1038/s41598-020-64352-2
Mohammadi, F., Soltani, A., Ghahremanloo, A., Javid, H., and Hashemy, S. I. (2019). The thioredoxin system and cancer therapy: a review. Cancer Chemother. Pharmacol. 84, 925–935. doi: 10.1007/s00280-019-03912-4
Moy, T. I, Ball, A. R., Anklesaria, Z., Casadei, G., Lewis, K., and Ausubel, F. M. (2006). Identification of novel antimicrobials using a live-animal infection model. Proc. Natl. Acad. Sci. U.S.A. 103, 10414–10419. doi: 10.1073/pnas.0604055103
Müller, A., Cadenas, E., Graf, P., and Sies, H. (1984). A novel biologically active seleno-organic compound-I. Glutathione peroxidase-like activity in vitro and antioxidant capacity of PZ 51 (Ebselen). Biochem. Pharmacol. 33, 3235–3239. doi: 10.1016/0006-2952(84)90083-2
Muller, A., Eller, J., Albrecht, F., Prochnow, P., Kuhlmann, K., Bandow, J. E., et al. (2016). Allicin induces thiol stress in bacteria through S -allylmercapto modification of protein cysteines. J. Biol. Chem. 291, 11477–11490. doi: 10.1074/jbc.M115.702308
Nemeth, J., Oesch, G., and Kuster, S. P. (2015). Bacteriostatic versus bactericidal antibiotics for patients with serious bacterial infections: systematic review and meta-analysis. J. Antimicrob. Chemother. 70, 382–395. doi: 10.1093/jac/dku379
O’Gara, E., Hill, D., and Maslin, D. (2000). Activities of garlic oil, garlic powder, and their diallyl constituents against Helicobacter pylori. Appl. Environ. Microbiol. 66, 2269–2273.
Ollinger, J., Kumar, A., Roberts, D., Bailey, M., Casey, A., and Parish, T. (2019). A high-throughput whole cell screen to identify inhibitors of Mycobacterium tuberculosis. PLoS One 14:e0205479. doi: 10.1371/journal.pone.0205479
Owings, J. P., McNair, N. N., Mui, Y. F., Gustafsson, T. N., Holmgren, A., Contel, M., et al. (2016). Auranofin and N-heterocyclic carbene gold-analogs are potent inhibitors of the bacteria Helicobacter pylori. FEMS Microbiol. Lett. 363, 1–6. doi: 10.1093/femsle/fnw148
Pankey, G. A., and Sabath, L. D. (2004). Clinical relevance of bacteriostatic versus bactericidal mechanisms of action in the treatment of Gram-positive bacterial infections. Clin. Infect. Dis. 38, 864–870. doi: 10.1086/381972
Parnham, M., and Sies, H. (2000). Ebselen: prospective therapy for cerebral ischaemia. Expert Opin. Investig. Drugs 9, 607–619. doi: 10.1517/13543784.9.3.607
Parsonage, D., Sheng, F., Hirata, K., Debnath, A., Mckerrow, J. H., Reed, S. L., et al. (2016). X-ray structures of thioredoxin and thioredoxin reductase from Entamoeba histolytica and prevailing hypothesis of the mechanism of auranofin action. J. Struct. Biol. 194, 180–190. doi: 10.1016/j.jsb.2016.02.015.X-ray
Pérez-Köhler, B., García-Moreno, F., Bayon, Y., Pascual, G., and Bellón, J. M. (2015a). Inhibition of Staphylococcus aureus adhesion to the surface of a reticular heavyweight polypropylene mesh soaked in a combination of chlorhexidine and allicin: an in vitro study. PLoS One 10:e0126711. doi: 10.1371/journal.pone.0126711
Pérez-Köhler, B., García-Moreno, F., Brune, T., Pascual, G., and Bellón, J. M. (2015b). Preclinical bioassay of a polypropylene mesh for hernia repair pretreated with antibacterial solutions of chlorhexidine and allicin: an in vivo study. PLoS One 10:e0142768. doi: 10.1371/journal.pone.0142768
Potamitou, A., Holmgren, A., and Vlamis-Gardikas, A. (2002). Protein levels of Escherichia coli thioredoxins and glutaredoxins and their relation to null mutants, growth phase, and function. J. Biol. Chem. 277, 18561–18567. doi: 10.1074/jbc.M201225200
Prasetyoputro, A., Jarrrad, A. M., Cooper, M. A., and Blaskovich, M. A. T. (2019). The Eagle effect and antibiotic-induced persistence: two sides of teh same coin? Trends Microbiol. 27, 339–354.
Rajamuthiah, R., Fuchs, B. B., Jayamani, E., Kim, Y., Larkins-Ford, J., Conery, A., et al. (2014). Whole animal automated platform for drug discovery against multi-drug resistant Staphylococcus aureus. PLoS One 9:e89189. doi: 10.1371/journal.pone.0089189
Schewe, T. (1995). Molecular actions of ebselen-an antiinflammatory antioxidant. Gen. Pharmacol. 26, 1153–1169. doi: 10.1016/0306-3623(95)00003-J
St. John, G., Brot, N., Ruan, J., Erdjument-Bromage, H., Tempst, P., Weissbach, H., et al. (2001). Peptide methionine sulfoxide reductase from Escherichia coli and Mycobacterium tuberculosis protects bacteria against oxidative damage from reactive nitrogen intermediates. Proc. Natl. Acad. Sci. U.S.A. 98, 9901–9906. doi: 10.1073/pnas.161295398
Thangamani, S., Mohammad, H., Abushahba, M. F. N., Sobreira, T. J. P., Hedrick, V. E., Paul, L. N., et al. (2016a). Antibacterial activity and mechanism of action of auranofin against multi-drug resistant bacterial pathogens. Sci. Rep. 6:22571. doi: 10.1038/srep22571
Thangamani, S., Mohammad, H., Abushahba, M. F. N., Sobreira, T. J. P., and Seleem, M. N. (2016b). Repurposing auranofin for the treatment of cutaneous staphylococcal infections. Int. J. Antimicrob. Agents 47, 195–201. doi: 10.1016/j.ijantimicag.2015.12.016
Thangamani, S., Younis, W., and Seleem, M. N. (2015a). Repurposing clinical molecule ebselen to combat drug resistant pathogens. PLoS One 10:e0133877. doi: 10.1371/journal.pone.0133877
Thangamani, S., Younis, W., and Seleem, M. N. (2015b). Repurposing ebselen for treatment of multidrug-resistant staphylococcal infections. Sci. Rep. 5:11596. doi: 10.1038/srep11596
Tharmalingam, N., Ribeiro, N., da Silva, D., Naik, M., Cruz, L., Kim, W., et al. (2019). Auranofin is an effective agent against clinical isolates of Staphylococcus aureus. Future Med. Chem. 11, 1417–1425.
Torres, N. S., Abercrombie, J. J., Srinivasan, A., Lopez-Ribot, J. L., Ramasubramanian, A. K., and Leung, K. P. (2016). Screening a commercial library of pharmacologically active small molecules against Staphylococcus aureus biofilms. Antimicrob. Agents Chemother. 60, 5663–5672. doi: 10.1128/AAC.00377-16
Trivedi, A., Singh, N., Bhat, S. A., Gupta, P., and Kumar, A. (2012). Redox biology of tuberculosis pathogenesis. Adv. Microb. Physiol. 60, 263–324. doi: 10.1016/B978-0-12-398264-3.00004-8
Uziel, O., Borovok, I., Schreiber, R., Cohen, G., and Aharonowitz, Y. (2004). Transcriptional regulation of the Staphylococcus aureus thioredoxin and thioredoxin reductase genes in response to oxygen and disulfide stress. J. Bacteriol. 186, 326–334. doi: 10.1128/JB.186.2.326
Vegara, S., Funes, L., Martí, N., Saura, D., Micol, V., and Valero, M. (2011). Bactericidal activities against pathogenic bacteria by selected constituents of plant extracts in carrot broth. Food Chem. 128, 872–873. doi: 10.1016/j.foodchem.2011.03.109
Wald-Dickler, N., Holtom, P., and Spellberg, B. (2018). Busting the myth of “static vs cidal”: a systemic literature review. Clin. Infect. Dis. 66, 1470–1474. doi: 10.1093/cid/cix1127
Williams, C. H., Arscott, L., Muller, S., and Lennon, B. (2000). Thioredoxin reductase: two modes of catalysis have evolved. Eur. J. Biochem. 267, 6110–6117.
Windle, H. J., Fox, Á, Eidhin, D. N., and Kelleher, D. (2000). The thioredoxin system of Helicobacter pylori. J. Biol. Chem. 275, 5081–5089. doi: 10.1074/jbc.275.7.5081
Zhai, H., Pan, J., Pang, E., and Bai, B. (2014). Lavage with allicin in combination with vancomycin inhibits biofilm formation by Staphylococcus epidermidis in a rabbit model of prosthetic joint infection. PLoS One 9:e102760. doi: 10.1371/journal.pone.0102760
Keywords: allicin, antimicrobial, auranofin, drug resistance, ebselen, shikonin, thioredoxin system, thioredoxin reductase
Citation: Felix L, Mylonakis E and Fuchs BB (2021) Thioredoxin Reductase Is a Valid Target for Antimicrobial Therapeutic Development Against Gram-Positive Bacteria. Front. Microbiol. 12:663481. doi: 10.3389/fmicb.2021.663481
Received: 03 February 2021; Accepted: 29 March 2021;
Published: 16 April 2021.
Edited by:
Anjan Debnath, University of California, San Diego, United StatesReviewed by:
Kaushiki Mazudar, Kelly Government Solutions, United StatesMarc Maresca, Aix-Marseille Université, France
Copyright © 2021 Felix, Mylonakis and Fuchs. This is an open-access article distributed under the terms of the Creative Commons Attribution License (CC BY). The use, distribution or reproduction in other forums is permitted, provided the original author(s) and the copyright owner(s) are credited and that the original publication in this journal is cited, in accordance with accepted academic practice. No use, distribution or reproduction is permitted which does not comply with these terms.
*Correspondence: Beth Burgwyn Fuchs, helen_fuchs@brown.edu