- 1Laboratório de Microbiologia Molecular e Clínica, Programa de Pós-Graduação em Ciências da Saúde, Universidade São Francisco, Braganca Paulista, Brazil
- 2Departamento de Bioquímica, Instituto de Química, Universidade de São Paulo, São Paulo, Brazil
- 3Hospital das Clínicas, Divisão Laboratório Central, Faculdade de Medicina, Universidade de São Paulo, São Paulo, Brazil
- 4Laboratório de Técnicas Especiais, Hospital Israelita Albert Einstein, São Paulo, Brazil
Polymyxins are one of most important antibiotics available for multidrug-resistant Gram-negative infections. Diverse chromosomal resistance mechanisms have been described, but the polymyxin resistance phenotype is not yet completely understood. The objective of this study was to characterize colistin resistant mcr-1-producing strains isolated from human infections over one year in a hospital setting (Hospital das Clínicas, São Paulo, Brazil). We isolated 490 colistin-resistant Gram-negative rods, of which eight were mcr-1.1-positive Escherichia coli, the only species with this result, indicating a low incidence of the mcr-1 production mechanism among colistin-resistant isolates. All mcr-1.1 positive isolates showed similarly low MICs for colistin and were susceptible to most antibiotics tested. The isolates showed diversity of MLST classification. The eight mcr-1.1-positive E. coli genomes were sequenced. In seven of eight isolates the mcr-1.1 gene is located in a contig that is presumed to be a part of an IncX4 plasmid; in one isolate, it is located in a contig that is presumed to be part of an IncHI2A plasmid. Three different genomic contexts for mcr-1.1 were observed, including a genomic cassette mcr-1.1-pap2 disrupting a DUF2806 domain-containing gene in six isolates. In addition, an IS1-family transposase was found inserted next to the mcr-1.1 cassette in one isolate. An mcr-1.1-pap2 genomic cassette not disrupting any gene was identified in another isolate. Our results suggest that plasmid dissemination of hospital-resident strains took place during the study period and highlight the need for continued genomic surveillance.
Introduction
Multidrug-resistant Gram-negative bacterial clinical isolates are responsible for high mortality rates worldwide (Agência Nacional de Vigilância Sanitária, 2016; De Waele et al., 2020), and constitute a challenge for healthcare professionals with respect to therapy choice, because few options are currently available. Isolates that are resistant to antibiotics that have been recently produced by the pharmaceutical industry have already been reported (Wang et al., 2020). Moreover, these new antibiotics have no activity against carbapenem-resistant Acinetobacter baumannii (Zhanel et al., 2013; Nelson et al., 2017; Shields et al., 2017; Rodriguez et al., 2018; Noval et al., 2020). Thus, polymyxins remain the antimicrobial of last resort for treatment of infections caused by these microorganisms.
The increase of clinical use of polymyxins has been associated with elevation of the resistance rates for antibiotics of this class (Lee et al., 2015; Girardello et al., 2017; Rossi et al., 2017a; Braun et al., 2018; Zavascki et al., 2018). Briefly, the molecular mechanism of polymyxins resistance involves two component systems, which regulate modifications in the bacterial membranes, reducing the negative charge of bacterial surface, and consequently, decreasing the interaction between polymyxins and bacterial cell (Moffatt et al., 2019).
In 2015, the plasmidial mechanism of colistin resistance mcr-1 was described by Liu et al. (2016). This report left the clinical community alarmed, due to the possibility of further dissemination of this kind of resistance. The mcr-1 mechanism acts by adding phosphoethanolamine in the lipid A of the outer membrane lipopolysaccharide, decreasing the negative charge of the bacterial surface (Gao et al., 2016). In follow-up studies, mcr genes have been found in many other isolates, in different parts of the world (Li et al., 2016; García et al., 2018). After the first description of this gene, several studies have shown that it was also present in strains isolated over three decades ago (Arcilla et al., 2016; Hu et al., 2016; Kluytmans-van den Bergh et al., 2016; Olaitan et al., 2016; Suzuki et al., 2016; Tse and Yuen, 2016; Elbediwi et al., 2019).
In 2016, mcr-1 was detected in our institution for the first time, in a colistin-resistant Escherichia coli isolate from blood culture site (Rossi et al., 2017b). The present study aimed to evaluate the further dissemination in our institution of resistance to colistin caused by the mcr plasmid-located gene after the 2016 detection, and to characterize de genome of the mcr producing isolates.
Materials and Methods
Colistin Resistant Isolates
All colistin resistant Gram-negative strains, isolated between April, 2016 and March, 2017 in the Hospital das Clínicas, a hospital complex of São Paulo, Brazil, were screened by PCR, for the presence of mcr genes, using specific primers, previously described (Liu et al., 2016, 2020; Rebelo et al., 2018). The isolates identification was performed using Vitek MS (bioMeriéux, France).
Antimicrobial Susceptibility Tests
The antimicrobial susceptibility test was performed by using Vitek-2 (bioMeriéux, France), and the colistin resistance was prospectively confirmed using CLSI broth microdilution (CLSI, 2020).
Whole Genome Sequencing
The mcr-1 positive Gram-negative isolates were submitted to whole genome sequencing using the MiSeq Platform (Illumina, Inc., United States), after DNA extraction using QiaAmp DNA Mini Kit (Qiagen, Germany). The DNA quantification was performed using QubitTM dsDNA HS Assay Kit, according to manufacture recommendations (ThermoFisher, MA, United States). The sequencing libraries were prepared from 1 ng of total DNA using the Illumina Nextera XT DNA library preparation kit (Illumina, Inc., United States), according to the manufacture recommendations, and the sequencing run was performed in the Illumina MiSeq to generate 250 bp paired end reads.
Bioinformatics
Genome assembly was performed using A5-miseq (Coil et al., 2015), ABySS 2.2.4 (Jackman et al., 2017), DISCOVAR (Weisenfeld et al., 2014), MaSuRCA 3.3.3 (Zimin et al., 2013), MIRA 4.9.6 (Chevreux et al., 1999), and SPAdes 3.13.1 (Bankevich et al., 2012). These assemblies were then combined into a single superior assembly using Metassembler 1.5 (Wences and Schatz, 2015). The merging of Metassembler is iterative, using the locally best sequences of the next assembly to improve the last, so it was convenient to rank the six assemblies by N50 beforehand. The next step was a scaffolding with MeDuSa 1.6 (Bosi et al., 2015) using five of the closest genomes found on NCBI as reference. The all-against-all genome comparison was done with fastANI (Jain et al., 2018). The phylogenetic tree was inferred using the program parsnp (Treangen et al., 2014).
Multi-locus sequence typing was done using the server available at https://pubmlst.org. Identification of plasmids was done with PlasmidFinder 2.0.1 (Carattoli et al., 2014), and antibiotic resistance genes were found with ResFinder 3.2 (Zankari et al., 2012).
Results
Colistin Resistant Clinical Isolates
During one year of investigation, 7,852 Gram-negative bacilli were isolated in the hospital complex; 490 (6.24%) Gram-negative bacilli were resistant to colistin, of which 399 were classified as Klebsiella pneumoniae (81.43%), 29 as A. baumannii complex (5.92%), 19 as E. coli (3.88%), 19 as Enterobacter cloacae (3.88%), 18 as Pseudomonas aeruginosa (3.67%), 3 as Citrobacter freundii (0.61%), 2 as Klebsiella aerogenes (0.41%), and 1 as Citrobacter youngae (0.20%). Among the 490 colistin-resistant isolates, 418 (85.3%) showed colistin Minimal Inhibitory Concentration (MIC) > 16 μg/mL, and 72 (14.7%) showed borderline MICs, from 4 to 8 μg/mL.
mcr-1-Producing Isolates
All 490 colistin-resistant isolates were submitted to PCR for mcr gene screening, and mcr-1 was detected in eight E. coli isolates, corresponding to 1.63% of all colistin resistant Gram-negative bacilli, and to 42% of all E. coli isolates recovered in the hospital during the study. mcr-1 was not detected in any other colistin-resistant species. None of the mcr-2 through mcr-10 variants were detected in the examined strains. The mcr-1-positive strains were recovered from urine (three isolates), blood culture (two isolates), and bone, soft-tissue, and peritoneal fluid (one isolate, each). The antimicrobial susceptibility profile from eight isolates is described in Table 1. All mcr-1-producing isolates showed similar antimicrobial susceptibility profiles, being susceptible to third-generation cephalosporins and carbapenems antibiotics and showing borderline colistin MICs (4 and 8 μg/mL, four isolates each). Five of eight mcr-1-producing E. coli isolates were simultaneously resistant to ciprofloxacin. Three strains (Ec482, Ec483, and Ec716) were isolated from the same patient (Patient 2; bone, soft tissue, and urine, respectively) (Table 2). All patients from whom the mcr-1-producing strains were isolated had their infections successfully treated.
Whole Genome Sequencing
We have sequenced the genomes of the eight mcr-1-positive E. coli isolates; genome sequencing data and accession numbers are given in Table 3; all genomes are in draft status. Genome size varies from 4,758,738 to 5,770,437 bp. CheckM (Parks et al., 2015) results showed that all genomes had at least 99.04% completeness and at most 0.55% contamination. The genome of isolate EcHC891 has been reported previously (Rossi et al., 2017b) and is included here because it was isolated during the same period as the others. The isolates showed diversity of sequence types (STs): ST156 (Patient 1); ST410 (3 isolates from Patient 2); ST648 (Patient 3), ST10 (Patient 4 and Patient 5), and ST744 (Patient 6) (Table 2). We also performed a whole genome comparison of all against all (Supplementary Table 1) and inferred a phylogenetic tree from single nucleotide polymorphisms (Figure 1).
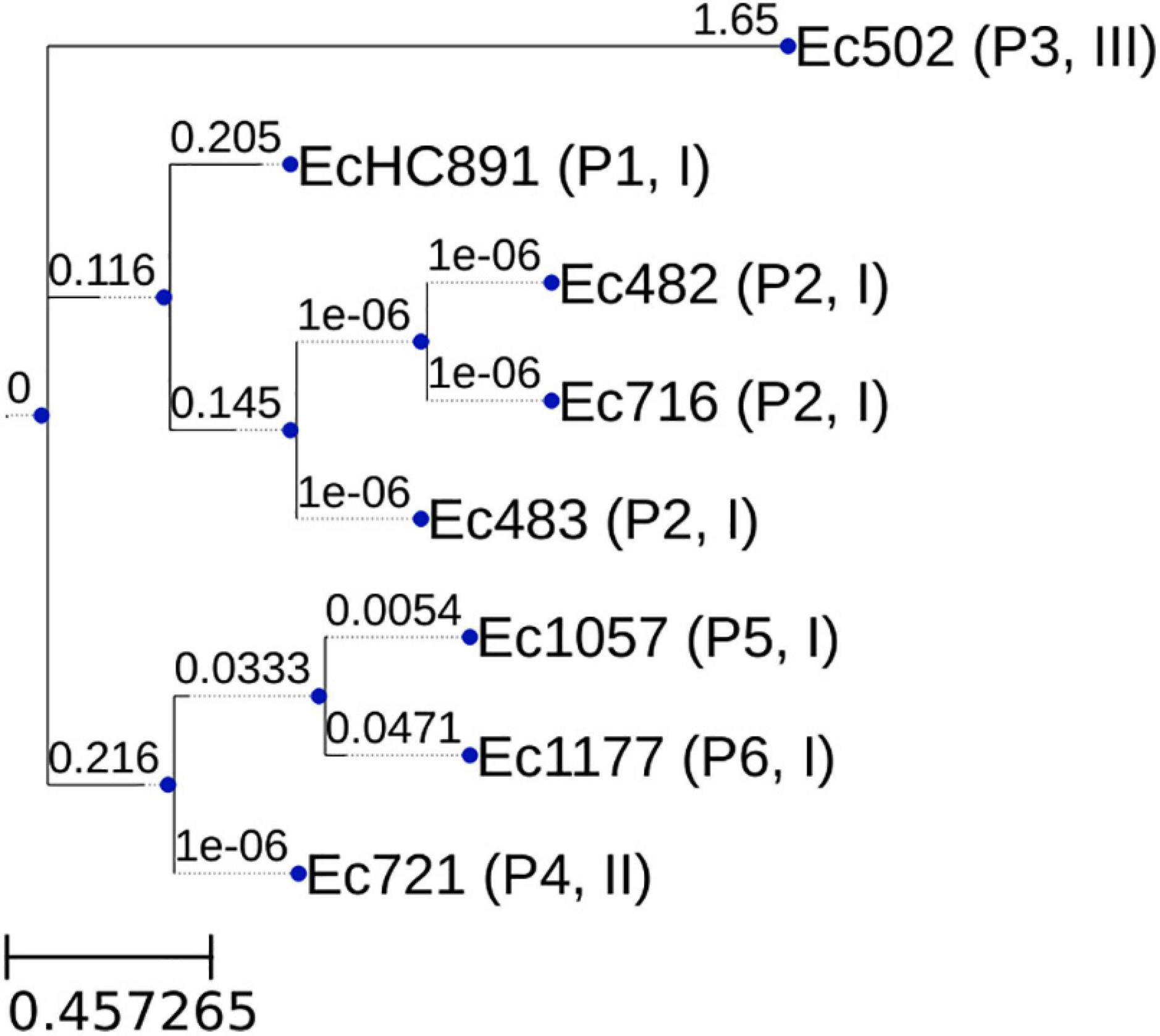
Figure 1. Phylogenetic tree generated by program parsnp (core genome SNP tree). The tree was inferred with FastTree 2.1 (Price et al., 2009). The numbers above branches are lengths, measured in number of substitutions per site. Leaf labels: Isolate identification (Patient number, Genomic Context type). The core genome size was 3,812,930 bp. This resulted from multiplying the individual genome cluster coverage value by its size, adding up these results, and dividing by the total number of genomes in the core genome computation.
We found the mcr-1.1 gene in each genome (Table 2). In seven isolates, mcr-1.1 is located in a contig that is presumed to be part of an IncX4 plasmid (EcHC891, Ec482, Ec483, Ec716, Ec721, Ec1057, and Ec1177). In the Ec502 isolate, mcr-1.1 is located in a contig that is presumed to be part of an IncHI2A plasmid (Table 2).
We define the genomic context of the mcr-1.1-pap2 cassette as including the flanking upstream and flanking downstream regions of lengths between 1000 and 2000 kbp (these lengths vary according to isolate, as will be seen). The genome characterization of the eight mcr-1.1-producing E. coli shows three different genomic contexts. The first group, including EcHC891, Ec482, Ec483, Ec716, Ec1057, and Ec1177 isolates, shows genomic context I. For these isolates, the genomic cassette mcr-1.1-pap2 disrupts a pre-existing DUF2806-domain-containing gene (Figure 2). This genomic cassette has been described in diverse studies from samples of retail poultry meats and chicken meat from Korea, Japan, and Brazil (Accession numbers: MK875286.1; LC227558). In addition, this genomic context has also been identified in isolates from animals, such as migratory Magellanic penguins, and in human and chicken gut microbiota (Accession numbers: CP021419.1; CP017246.1, KY689633, and KY689632) (Donà et al., 2017; Sellera et al., 2017; Kim et al., 2020). The nucleotide sequence identity between the mcr-1.1-containing regions in all genomes of genomic context I is 100%.
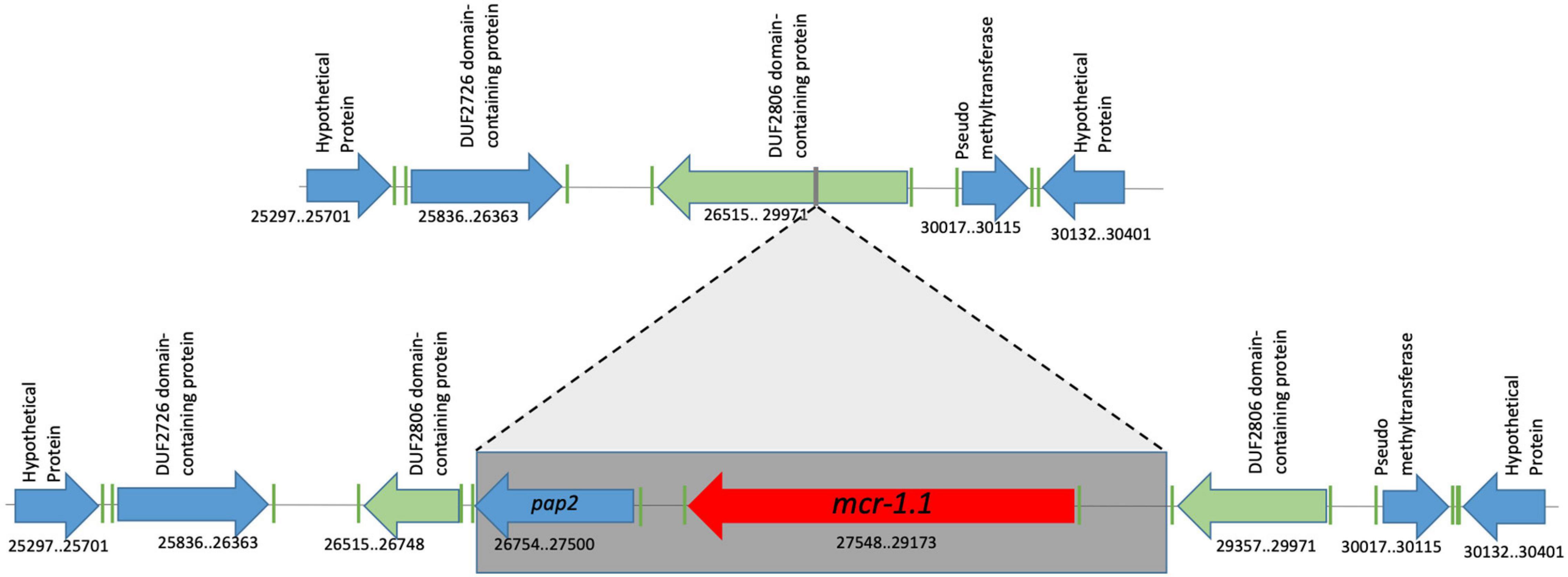
Figure 2. Genomic context #1 identified in EcHC891, Ec482, Ec483, Ec716, Ec1057 and Ec1177 isolates. The genomic cassette mcr-1.1-pap2 was found disrupting a pre-existing DUF2806-domain-containing gene, without any associated insertion sequence. Genomic coordinates refer to scaffold 3 of the Ec482 genome.
Genomic context II is represented by the Ec721 isolate and is identical to genomic context I, except for the presence of a 698-bp IS1-family transposase (which contains a frameshift, and has therefore been annotated as a pseudogene) inserted upstream of the mcr-1.1 gene on the opposite strand (Figure 3). The mcr-1.1 containing region in the Ec721 genome also has 100% nucleotide identity to the corresponding regions of context I genomes, excepting the IS1 transposase insertion.
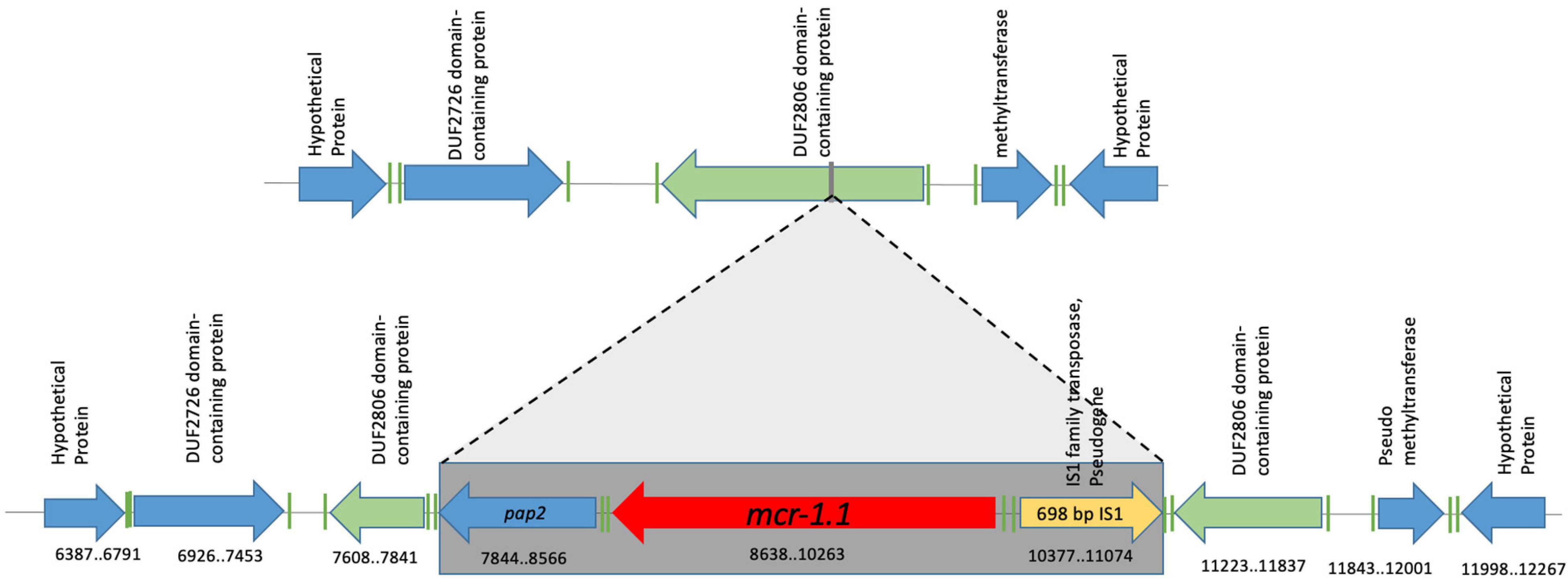
Figure 3. Genomic context #2 identified in the Ec721 isolate. A 698 bp Family 1 transposase was found upstream of the mcr-1.1 gene, on the opposite strand; otherwise, the genomic context is the same as the one shown in Figure 2. Genomic coordinates refer to scaffold 2 of the Ec721 genome.
Finally, genomic context III is represented by the Ec502 isolate. The genomic cassette mcr-1.1-pap-2 was also found, but not disrupting a pre-existing gene. For this isolate we did not identify any IS in the genomic cassette (Figure 4). This same context has been described in accession numbers CP026933.2 and CP022165.1 (Gilrane et al., 2017). It is worth noting here that, besides being the only strain to present this mcr-1 cassette composition, the genome of the Ec502 isolate is the most different from the others we sequenced (Supplementary Table 1).

Figure 4. Genomic context #3 identified in the Ec502 isolate. Genomic coordinates refer to contig Qabyss.discovar.masurca.spades.a5_22 of the Ec502 genome.
In seven genomes (EcHC891, Ec482, Ec483, Ec716, Ec721, Ec1057, and Ec1177 isolates) the pap-2 protein product has 247 aa, whereas in Ec502 it has 297 aa. BLAST alignments showed a GenBank record of this same protein product (EFD4953355.1) with 327 aa. The different lengths occur at the 3’ end of the protein sequence, and are therefore not due to different choices of the translation start codon. Based solely on genomic information (and given that the nucleotide similarity is very high between these E. coli strains) it is not possible to determine which of these lengths is the “correct” one: the shorter ones may have had a mutation that created a premature stop with respect to the longer one; or the longer ones could have had mutations that changed the stop codon to a non-stop codon; or all these variants may be different but nevertheless functional (i.e., these are not pseudogenes). The question seems to require experimental data to be clarified.
Discussion
Colistin became the first-line drug for Gram-negative infections in patients admitted to the ICU in our institution in the last years, since the carbapenem resistance rates among Acinetobacter spp. increased from 30 to 70%, between 2010 and 2014 (Rossi et al., 2017a). The polymyxins resistance can be induced during the therapy with the drug, so, despite the polymyxin susceptibility still showing relatively high rates for most bacterial species, the resistance rates have been increasing worldwide in the last few years (Braun et al., 2018; Zavascki et al., 2018; de Lastours et al., 2020).
It has been reported that most colistin resistant isolates presenting the mcr-1 mechanism have polymyxin MICs around the breakpoint for resistance (Principe et al., 2018; Lee et al., 2019). In some cases, these genes are found among susceptible isolates (Fernandes et al., 2016; Aires et al., 2017). In this study, we evaluated clinical isolates with MICs classifying them as resistant, according to CLSI guidelines (≥4 μg/mL); and also found this gene among isolates with borderline MICs (4 and 8 μg/mL); however, differently from what was reported in a multicentric study (Principe et al., 2018), among all of the 11 examined mcr-1-negative colistin-resistant E. coli, we observed colistin MIC > 16 μg/mL (Supplementary Table 2). Because of this observation, in our institution, β-lactam susceptibility plus colistin resistance or borderline MIC values are phenotypes that trigger mcr-1 screening.
After the first detection of mcr-1 in our hospital in 2016 (Rossi et al., 2017b), this mechanism was sporadically detected. The mcr-1 plasmid resistance mechanism appears to be less clinically important when compared to other mechanisms in our institution. In this study, the most common colistin-resistant species recovered was K. pneumoniae, representing more than 80% of all colistin-resistant isolates. Differently from what was observed among E. coli isolates, K. pneumoniae showed high resistant rates to all antimicrobials tested and elevated MICs for colistin (>16 μg/mL); however, mcr-1 was not detected in these isolates (Supplementary File 3). The main colistin resistance mechanism among K. pneumoniae in our hospital appears to be the mgrB mutation (data not shown), as already reported in the literature (Cannatelli et al., 2014). On the other hand, mcr-1 appears to be an important colistin resistance mechanism in E. coli strains.
Interestingly, in our study we isolated three mcr-1.1-producing E. coli from one patient (Patient 2) over two months. It is not clear what is the origin of these mcr-1.1 isolates. We speculate that an mcr-1.1-producing E. coli strain was naturally present in the patient’s gut microbiota and, after selective pressure caused by the antimicrobials used over the long hospital stay, this same strain was responsible for the urinary infection. The urine infection site is suggestive of a gut microbiota source after colonization with mcr-1-positive E. coli due to previous infection episodes. We base this hypothesis on the fact that all three isolates were classified as ST410, and the all-against-all genome comparison shows that these three isolates are the most similar to one another compared to the other five isolates (Table 2 and Supplementary Table 1). The genomic similarities observed between these three strains are high (minimum value 99.93%), and this needs to take into account that genome assembly was only at the draft level (meaning that the similarities could be even closer to 100% if the genomes had been finished). The inferred phylogenetic tree shows that the isolates from patient P2 cluster together.
In our study we observed two different mcr-1-carrying contig types, which can be described by the plasmid marker found in these contigs: IncX4 (seven isolates) and IncHI2A (one isolate). Wang et al. (2018) found 13 different plasmids carrying mcr-1 worldwide, and IncI2 and IncX4 were the dominant plasmid types found.
mcr-1 has been sporadically described among human clinical isolates with multidrug resistance profiles (MDR; Fernandes et al., 2016; Aires et al., 2017; Conceição-Neto et al., 2017; Dalmolin et al., 2017, 2018; Rocha et al., 2017; Lorenzoni et al., 2018; Oliveira et al., 2018; Tada et al., 2018; Tonini et al., 2018; Farzana et al., 2019). However, the most frequently reported detection of mcr-1 is in environmental and food-animal strains. In addition, the environmental strains appear to be more pathogenic than those recovered from human infections, including ESβL and carbapenemase-producing isolates (Fernandes et al., 2016, 2017; Monte et al., 2017; Drali et al., 2018; Tuo et al., 2018; Wu et al., 2018; Barlaam et al., 2019; Rumi et al., 2019; Yang et al., 2019; Dandachi et al., 2020; Loayza-Villa et al., 2020). In a global systematic review, Elbediwi et al. (2019) state that the estimated prevalence of mcr-1 pathogenic E. coli was higher in food-animals than in humans and food products, which suggests a role for foodborne transmission. Brazil is a major producer and exporter of chicken, pig and poultry meat, and colistin sulfate, until recently, was used as a growth promoter (Morales et al., 2012). According to Fernandes et al. (2016) this can support a link between the use of colistin in livestock and the appearance of colistin resistance in E. coli isolates. Chiba et al. (2019) have reported mcr-1 in chicken meat imported from Brazil. Yang et al. (2019) state that the multidrug resistance associated with livestock not only affects animal production, but also human health, by contaminating the food chain. However, the low mcr-1 detection rate in human infections, observed in our study, allows us to hypothesize that this mechanism is not frequent inside a hospital environment, as observed with KPC-producing K. pneumoniae and OXA-producing A. baumannii strains. Additional investigation is required in order to determine the real origin and relevance of the polymyxins plasmid-mediated mcr-1 mechanism.
We observed three different genomic contexts for the mcr1.1-pap2 cassette in the genomes here presented. This diversity suggests a capacity of mcr-1 to be mobile, using different cassette compositions, and to insert itself in variable contexts, as observed in other studies. Snesrud et al. (2016) state that, after transposition of the mcr-1 cassette, fragments of the transposon may be lost. Furthermore, the mcr-1 cassette may be inserted in various locations in the genome, including the chromosome; Wang et al. (2018) have presented similar results.
The presence of Insertion Sequences (ISs) in the mcr-1 genomic context has been described in diverse studies, and Snesrud et al. (2016) proposed a model of transposition of the genomic cassette mcr-1 – pap2 flanked by two copies of ISApl1 in the same orientation, and suggest that the mcr-1 cassette is mobilized as a composite transposon. These authors have also described a single copy of ISApl1 upstream of mcr-1. Different from observed in our study, ISApl1 is an IS30-family sequence. Furthermore, in isolate Ec721, the IS1-family transposase is inserted upstream of the mcr-1 cassette, on the opposite strand, and may therefore not play a role in the cassette mobility. Wang et al. (2018) observed higher presence of ISApl1 transposon background from China isolates compared to other countries, and suggest a single mobilization of ISApl1-mcr-1 – pap2 – ISApl1 fragment with subsequent diversification during global spread. However, these authors state that the origin of mcr-1 prior to its mobilization remains unclear.
Conclusion
Our results suggest that, in the setting and period investigated, intra-hospital E. coli mcr-1.1-carrying plasmid dissemination took place. The evidence are the six isolates for which we found the identical mcr-1-pap2 cassette associated with a IncX4-plasmid, which were isolated from four different patients. The case of the Ec502 isolate, which is the most different from the others, both in the genomic sequence as a whole, as well as in the mcr-1-pap2 cassette and plasmid incompatibility complex type, is more difficult to interpret with available data. It might have acquired its mcr1.1 gene in the hospital, or it might have come from the outside already with the mcr-1.1 gene, possibly as part of the patient’s microbiota. The genome sequencing of new isolates, coupled with detailed patients’ histories, may help clarify this issue. Such additional sequencing will also help with the much-needed continued surveillance for the mcr-1.1 gene.
Data Availability Statement
The datasets presented in this study can be found in online repositories. The names of the repository/repositories and accession number(s) can be found in Table 3.
Author Contributions
RG contributed with study design, conduction of experiments, data analysis, and writing and revision of the manuscript. CP and JM participated in the conduction of experiments and data analysis. MM, AC, MF, FM, and NR contributed with conduction of experiments. JP and FR contributed with critical reading and supervision. AD contributed with funding and supervision. JS contributed with conduction of experiments, data analysis, writing, and revision of the manuscript. All authors contributed to the article and approved the submitted version.
Funding
This study was supported in part by the National Council for Scientific and Technological Development (CNPq), Brazil (grant 305961/2019-5 to JS) and by The São Paulo State Research Foundation (FAPESP).
Conflict of Interest
The authors declare that the research was conducted in the absence of any commercial or financial relationships that could be construed as a potential conflict of interest.
Acknowledgments
We would like to thank the Microbiology Laboratory Group of HC-FMUSP for its contribution to the clinical routine.
Supplementary Material
The Supplementary Material for this article can be found online at: https://www.frontiersin.org/articles/10.3389/fmicb.2021.663414/full#supplementary-material
References
Agência Nacional de Vigilância Sanitária (2016). Boletim de Segurança do Paciente e Qualidade em Serviços de Saúde N° 14: Avaliação dos Indicadores Nacionais das Infecções Relacionadas à Assistência à Saúde (IRAS) e Resistência Microbiana do Ano de 2015. Brasília: Agência Nacional de Vigilância Sanitária.
Aires, C. A. M., da Conceição-Neto, O. C., Tavares, E., Oliveira, T. R., Dias, C. F., Montezzi, L. F., et al. (2017). Emergence of the plasmid-mediated MCR-1 gene in clinical KPC-2-producing Klebsiella Pneumoniae sequence type 392 in brazil. Antimicrob. Agents Chemother. 61, e317–e317. doi: 10.1128/AAC.00317-17
Arcilla, M. S., van Hattem, J. M., Matamoros, S., Melles, D. C., Penders, J., de Jong, M. D., et al. (2016). Combat consortium. dissemination of the MCR-1 colistin resistance gene. Lancet Infect Dis. 16, 147–149. doi: 10.1016/S1473-3099(15)00541-1
Bankevich, A., Nurk, S., Antipov, D., Gurevich, A. A., Dvorkin, M., Kulikov, A. S., et al. (2012). Spades: a new genome assembly algorithm and its applications to single-cell sequencing. J. Comput. Biol. 19, 455–477. doi: 10.1089/cmb.2012.0021
Barlaam, A., Parisi, A., Spinelli, E., Caruso, M., Taranto, P. D., and Normanno, G. (2019). Global emergence of colistin-resistant Escherichia Coli in food chains and associated food safety implications: a review. J. Food Prot. 82, 1440–1448. doi: 10.4315/0362-028X.JFP-19-116
Bosi, E., Donati, B., Galardini, M., Brunetti, S., Sagot, M. F., Lió, P., et al. (2015). Medusa: a multi-draft based scaffolder. Bioinformatics 31, 2443–2451. doi: 10.1093/bioinformatics/btv171
Braun, G., Cayô, R., Matos, A. P., de Mello Fonseca, J., and Gales, A. C. (2018). Temporal evolution of polymyxin b-resistant Klebsiella Pneumoniae clones recovered from blood cultures in a teaching hospital during a 7-year period. Int. J. Antimicrob. Agents 51, 522–527. doi: 10.1016/j.ijantimicag.2017.08.031
Cannatelli, A., Giani, T., D’Andrea, M. M., Di Pilato, V., Arena, F., Conte, V., et al. (2014). Colgrit study group. mGRB inactivation is a common mechanism of colistin resistance in kpc-producing Klebsiella Pneumoniae of clinical origin. Antimicrob. Agents Chemother. 58, 5696–5703. doi: 10.1128/AAC.03110-14
Carattoli, A., Zankari, E., García-Fernández, A., Voldby Larsen, M., Lund, O., Villa, L., et al. (2014). In silico detection and typing of plasmids using plasmidfinder and plasmid multilocus sequence typing. Antimicrob. Agents Chemother. 58, 3895–3903. doi: 10.1128/AAC.02412-14
Chevreux, B., Wetter, T., and Suhai, S. (1999). “Genome Sequence Assembly Using Trace Signals and Additional Sequence Information. Computer Science and Biology,” in Proceedings of the German Conference on Bioinformatics (GCB), (Hannover: GCB), 45–56.
Chiba, N., Tanimoto, K., Hisatsune, J., Sugai, M., Shibayama, K., Watanabe, H., et al. (2019). Detection of MCR-1-mediated colistin resistance in e. Coli isolate from imported chicken meat from brazil. J. Glob. Antimicrob. Resist. 16, 249–250. doi: 10.1016/j.jgar.2019.02.004
CLSI (2020). Performance Standards for Antimicrobial Susceptibility Testing, M100, 30th Edn. Pennsylvania: CLSI.
Coil, D., Jospin, G., and Darling, A. E. (2015). A5-miseq: an updated pipeline to assemble microbial genomes from illumina miseq data. Bioinformatics 31, 587–589. doi: 10.1093/bioinformatics/btu661
Conceição-Neto, O. C., Aires, C. A. M., Pereira, N. F., da Silva, L. H. J., Picão, R. C., Siqueira, B. N., et al. (2017). Detection of the plasmid-mediated MCR-1 gene in clinical KPC-2-producing Escherichia Coli isolates in brazil. Int. J. Antimicrob. Agents 50, 282–284. doi: 10.1016/j.ijantimicag.2017.05.003
Dalmolin, T. V., Castro, L., Mayer, F. Q., Zavascki, A. P., Martins, A. F., Lima-Morales, D., et al. (2017). Co-occurrence of MCR-1 and blakpc–2 in a clinical isolate of Escherichia Coli in brazil. J. Antimicrob. Chemother. 72, 2404–2406. doi: 10.1093/jac/dkx142
Dalmolin, T. V., Martins, A. F., Zavascki, A. P., de Lima-Morales, D., and Barth, A. L. (2018). Acquisition of the mcr-1 gene by a high-risk clone of KPC-2-producing klebsiella pneumoniae st437/cc258. BRAZIL. Diagn. Microbiol. Infect. Dis. 90, 132–133. doi: 10.1016/j.diagmicrobio.2017.09.016
Dandachi, I., Fayad, E., Sleiman, A., Daoud, Z., and Rolain, J. M. (2020). Dissemination of multidrug-resistant and MCR-1 gram-negative bacilli in broilers, farm workers, and the surrounding environment in lebanon. Microb. Drug Resist. 26, 368–377. doi: 10.1089/mdr.2019.0137
de Lastours, V., Poirel, L., Huttner, B., Harbarth, S., Denamur, E., and Nordmann, P. (2020). Emergence of colistin-resistant gram-negative enterobacterales in the gut of patients receiving oral colistin and neomycin decontamination. J. Infect. 80, 578–606. doi: 10.1016/j.jinf.2020.01.003
De Waele, J. J., Boelens, J., and Leroux-Roels, I. (2020). Multidrug-resistant bacteria in icu: fact or myth. Curr. Opin. Anaesthesiol. 33, 156–161. doi: 10.1097/ACO.0000000000000830
Donà, V., Bernasconi, O. J., Pires, J., Collaud, A., Overesch, G., Ramette, A., et al. (2017). Heterogeneous genetic location of MCR-1 in colistin-resistant Escherichia Coli isolates from humans and retail chicken meat in switzerland: emergence of MCR-1-carrying inck2 plasmids. Antimicrob. Agents Chemother. 61, e1245–e1217. doi: 10.1128/AAC.01245-17
Drali, R., Berrazeg, M., Zidouni, L. L., Hamitouche, F., Abbas, A. A., Deriet, A., et al. (2018). Emergence of mcr-1 plasmid-mediated colistin-resistant Escherichia Coli isolates from seawater. Sci. Total Environ. 642, 90–94. doi: 10.1016/j.scitotenv.2018.05.387
Elbediwi, M., Li, Y., Paudyal, N., Pan, H., Li, X., Xie, S., et al. (2019). Global burden of colistin-resistant bacteria: mobilized colistin resistance genes study (1980-2018). Microorganisms 7:461. doi: 10.3390/microorganisms7100461
Farzana, R., Jones, L. S., Rahman, M. A., Toleman, M. A., Sands, K., Portal, E., et al. (2019). Emergence of mcr-1 mediated colistin resistant Escherichia Coli from a hospitalized patient in bangladesh. J. Infect. Dev. Ctries 13, 773–776. doi: 10.3855/jidc.11541
Fernandes, M. R., McCulloch, J. A., Vianello, M. A., Moura, Q., Pérez-Chaparro, P. J., Esposito, F., et al. (2016). First report of the globally disseminated incx4 plasmid carrying the MCR-1 gene in a colistin-resistant Escherichia Coli sequence type 101 isolate from a human infection in brazil. Antimicrob. Agents Chemother. 60, 6415–6417. doi: 10.1128/AAC.01325-16
Fernandes, M. R., Sellera, F. P., Esposito, F., Sabino, C. P., Cerdeira, L., and Lincopan, N. (2017). Colistin-resistant MCR-1-positive Escherichia Coli on public beaches, an infectious threat emerging in recreational waters. Antimicrob. Agents Chemother. 61, e234–e217. doi: 10.1128/AAC.00234-17
Gao, R., Hu, Y., Li, Z., Sun, J., Wang, Q., Lin, J., et al. (2016). Dissemination and mechanism for the mcr-1 colistin resistance. PLoS Pathog. 12:e1005957. doi: 10.1371/journal.ppat.1005957
García, V., García-Meniño, I., Mora, A., Flament-Simon, S. C., Díaz-Jiménez, D., Blanco, J. E., et al. (2018). Co-occurrence of MCR-1, MCR-4 and MCR-5 genes in multidrug-resistant st10 enterotoxigenic and shiga toxin-producing Escherichia Coli in spain (2006-2017). Int. J. Antimicrob. Agents 52, 104–108. doi: 10.1016/j.ijantimicag.2018.03.022
Gilrane, V. L., Lobo, S., Huang, W., Zhuge, J., Yin, C., Chen, D., et al. (2017). Complete genome sequence of a colistin-resistant Escherichia Coli strain harboring MCR-1 on an inchi2 plasmid in the united states. Genome Announc. 5, e1095–e1017. doi: 10.1128/genomeA.01095-17
Girardello, R., Visconde, M., Cayô, R., Figueiredo, R. C., Mori, M. A., Lincopan, N., et al. (2017). Diversity of polymyxin resistance mechanisms among Acinetobacter Baumannii clinical isolates. Diagn. Microbiol. Infect. Dis. 87, 37–44. doi: 10.1016/j.diagmicrobio.2016.10.011
Hu, Y., Liu, F., Lin, I. Y., Gao, G. F., and Zhu, B. (2016). Dissemination of the MCR-1 colistin resistance gene. Lancet Infect. Dis. 16, 146–147. doi: 10.1016/S1473-3099(15)00533-2
Jackman, S. D., Vandervalk, B. P., Mohamadi, H., Chu, J., Yeo, S., Hammond, S. A., et al. (2017). Abyss 2.0: resource-efficient assembly of large genomes using a bloom filter. Genome Res. 27, 768–777. doi: 10.1101/gr.214346.116
Jain, C., Rodriguez-R, L. M., Phillippy, A. M., Konstantinidis, K. T., and Aluru, S. (2018). High throughput ani analysis of 90k prokaryotic genomes reveals clear species boundaries. Nat. Commun. 9:5114. doi: 10.1038/s41467-018-07641-9
Kim, S., Kim, H., Kim, Y., Kim, M., Kwak, H., and Ryu, S. (2020). Antimicrobial resistance of Escherichia Coli from retail poultry meats in korea. J. Food Prot. 83, 1673–1678. doi: 10.4315/JFP-20-150
Kluytmans-van den Bergh, M. F., Huizinga, P., Bonten, M. J., Bos, M., De Bruyne, K., Friedrich, A. W., et al. (2016). Presence of mcr-1-positive Enterobacteriaceae in retail chicken meat but not in humans in the netherlands since 2009. Euro Surveill. 21:30149. doi: 10.2807/1560-7917.ES.2016.21.9.30149
Lee, J. Y., Choi, M. J., Choi, H. J., and Ko, K. S. (2015). Preservation of acquired colistin resistance in gram-negative bacteria. Antimicrob. Agents Chemother. 60, 609–612. doi: 10.1128/AAC.01574-15
Lee, Y. L., Lu, M. C., Shao, P. L., Lu, P. L., Chen, Y. H., Cheng, S. H., et al. (2019). Nationwide surveillance of antimicrobial resistance among clinically important gram-negative bacteria, with an emphasis on carbapenems and colistin: results from the surveillance of multicenter antimicrobial resistance in taiwan (smart) in 2018. Int. J. Antimicrob. Agents 54, 318–328. doi: 10.1016/j.ijantimicag.2019.06.009
Li, Z., Tan, C., Lin, J., and Feng, Y. (2016). Diversified variants of the MCR-1-carrying plasmid reservoir in the swine lung microbiota. Sci. China Life Sci. 59, 971–973. doi: 10.1007/s11427-016-5111-9
Liu, J., Zhang, Z., Feng, Y., Hu, H., Yu, Y., Qiu, L., et al. (2020). Molecular detection of the MCR genes by multiplex pcr. Infect. Drug Resist. 13, 3463–3468. doi: 10.2147/IDR.S256320
Liu, Y. Y., Wang, Y., Walsh, T. R., Yi, L. X., Zhang, R., Spencer, J., et al. (2016). Emergence of plasmid-mediated colistin resistance mechanism MCR-1 in animals and human beings in china: a microbiological and molecular biological study. Lancet Infect. Dis. 16, 161–168. doi: 10.1016/S1473-3099(15)00424-7
Loayza-Villa, F., Salinas, L., Tijet, N., Villavicencio, F., Tamayo, R., Salas, S., et al. (2020). Diverse Escherichia Coli lineages from domestic animals carrying colistin resistance gene MCR-1 in an ecuadorian household. J. Glob. Antimicrob. Resist. 22, 63–67. doi: 10.1016/j.jgar.2019.12.002
Lorenzoni, V. V., Dalmolin, T. V., Franco, L. N., Barth, A. L., and Hörner, R. (2018). Bloodstream infection by mcr-1-harboring Escherichia Coli in a cancer patient in southern brazil. Braz. J. Infect. Dis. 22, 356–357. doi: 10.1016/j.bjid.2018.07.001
Moffatt, J. H., Harper, M., and Boyce, J. D. (2019). Mechanisms of polymyxin resistance. Adv. Exp. Med. Biol. 1145, 55–71. doi: 10.1007/978-3-030-16373-0_5
Monte, D. F., Mem, A., Fernandes, M. R., Cerdeira, L., Esposito, F., Galvão, J. A., et al. (2017). Chicken meat as a reservoir of colistin-resistant Escherichia Coli strains carrying MCR-1 genes in south america. Antimicrob. Agents Chemother. 61, e2718–e2716. doi: 10.1128/AAC.02718-16
Morales, A. S., Fragoso de Araújo, J., de Moura Gomes, V. T., Reis Costa, A. T., dos Prazeres Rodrigues, D., and Porfida Ferreira, T. S. (2012). Colistin resistance in Escherichia Coli and Salmonella Enterica strains isolated from swine in brazil. ScientificWorldJournal 2012:109795. doi: 10.1100/2012/109795
Nelson, K., Hemarajata, P., Sun, D., Rubio-Aparicio, D., Tsivkovski, R., Yang, S., et al. (2017). Resistance to ceftazidime-avibactam is due to transposition of kpc in a porin-deficient strain of Klebsiella Pneumoniae with increased efflux activity. Antimicrob. Agents Chemother. 61, e989–e917. doi: 10.1128/AAC.00989-17
Noval, M., Banoub, M., Claeys, K. C., and Heil, E. (2020). The battle is on: new beta-lactams for the treatment of multidrug-resistant gram-negative organisms. Curr. Infect. Dis. Rep. 22:1. doi: 10.1007/s11908-020-0710-9
Olaitan, A. O., Chabou, S., Okdah, L., Morand, S., and Rolain, J. M. (2016). Dissemination of the MCR-1 colistin resistance gene. Lancet Infect. Dis. 16:147. doi: 10.1016/S1473-3099(15)00540-X
Oliveira, F. A., Zaccariotto, T. R., Piveta, C., Hofling, C. C., Resende, M. R., Levy, C. E., et al. (2018). MCR-1-positive colistin-resistant Escherichia Coli in immunocompromised hospitalised patients. Int. J. Antimicrob. Agents 52, 438–440. doi: 10.1016/j.ijantimicag.2018.06.011
Parks, D. H., Imelfort, M., Skennerton, C. T., Hugenholtz, P., and Tyson, G. W. (2015). Checkm: assessing the quality of microbial genomes recovered from isolates, single cells, and metagenomes. Genome Res. 25, 1043–1055. doi: 10.1101/gr.186072.114
Price, M. N., Dehal, P. S., and Arkin, A. P. (2009). Fasttree: computing large minimum-evolution trees with profiles instead of a distance matrix. Mol. Biol. Evolut. 26, 1641–1650. doi: 10.1093/molbev/msp077
Principe, L., Piazza, A., Mauri, C., Anesi, A., Bracco, S., Brigante, G., et al. (2018). Multicenter prospective study on the prevalence of colistin resistance in Escherichia Coli: relevance of MCR-1-positive clinical isolates in lombardy, northern italy. Infect. Drug Resist. 11, 377–385. doi: 10.2147/IDR.S160489
Rebelo, A. R., Bortolaia, V., Kjeldgaard, J. S., Pedersen, S. K., Leekitcharoenphon, P., Hansen, I. M., et al. (2018). Multiplex pcr for detection of plasmid-mediated colistin resistance determinants, MCR-1, MCR-2, MCR-3, MCR-4 and MCR-5 for surveillance purposes. Euro Surveill. 23, 17–00672. doi: 10.2807/1560-7917.ES.2018.23.6.17-00672
Rocha, I. V., Andrade, C. A. D. N., Campos, T. L., Rezende, A. M., Leal, N. C., Vidal, C. F. L., et al. (2017). Ciprofloxacin-resistant and extended-spectrum β-lactamase-producing Escherichia Coli st410 strain carrying the MCR-1 gene associated with bloodstream infection. Int. J. Antimicrob. Agents 49, 655–656. doi: 10.1016/j.ijantimicag.2017.03.001
Rodriguez, B. A., Girotto, J. E., and Nicolau, D. P. (2018). Ceftazidime/avibactam and ceftolozane/tazobactam: novel therapy for multidrug resistant gram negative infections in children. Curr. Pediatr. Rev. 14, 97–109. doi: 10.2174/1573396314666180308150908
Rossi, F., Girardello, R., Cury, A. P., Di Gioia, T. S., Almeida, J. N. Jr., and Duarte, A. J. (2017a). Emergence of colistin resistance in the largest university hospital complex of são paulo, brazil, over five years. Braz. J. Infect. Dis. 21, 98–101. doi: 10.1016/j.bjid.2016.09.011
Rossi, F., Girardello, R., Morais, C., Cury, A. P., Martins, L. F., da Silva, A. M., et al. (2017b). Plasmid-mediated MCR-1 in carbapenem-susceptible Escherichia Coli st156 causing a blood infection: an unnoticeable spread of colistin resistance in brazil? Clinics 72, 642–644. doi: 10.6061/clinics/2017(10)09
Rumi, M. V., Mas, J., Elena, A., Cerdeira, L., Muñoz, M. E., Lincopan, N., et al. (2019). Co-occurrence of clinically relevant β-lactamases and mcr-1 encoding genes in Escherichia Coli from companion animals in argentina. Vet. Microbiol. 230, 228–234. doi: 10.1016/j.vetmic.2019.02.006
Sellera, F. P., Fernandes, M. R., Sartori, L., Carvalho, M. P., Esposito, F., Nascimento, C. L., et al. (2017). Escherichia Coli carrying incx4 plasmid-mediated MCR-1 and blactx–m genes in infected migratory Magellanic Penguins (Spheniscus Magellanicus). J. Antimicrob. Chemother. 72, 1255–1256. doi: 10.1093/jac/dkw543
Shields, R. K., Chen, L., Cheng, S., Chavda, K. D., Press, E. G., Snyder, A., et al. (2017). Emergence of ceftazidime-avibactam resistance due to plasmid-borne blakpc-3 mutations. Antimicrob. Agents Chemother. 61, e2097–e2016. doi: 10.1128/AAC.02097-16
Snesrud, E., He, S., Chandler, M., Dekker, J. P., Hickman, A. B., McGann, P., et al. (2016). A model for transposition of the colistin resistance gene MCR-1 by ISAPL1. Antimicrob. Agents Chemother. 60, 6973–6976. doi: 10.1128/AAC.01457-16
Suzuki, S., Ohnishi, M., Kawanishi, M., Akiba, M., and Kuroda, M. (2016). Investigation of a plasmid genome database for colistin-resistance gene MCR-1. Lancet Infect. Dis. 16, 284–285. doi: 10.1016/S1473-3099(16)00008-6
Tada, T., Uechi, K., Nakasone, I., Nakamatsu, M., Satou, K., Hirano, T., et al. (2018). Emergence of incx4 plasmids encoding MCR-1 in a clinical isolate of Klebsiella Pneumoniae in japan. Int. J. Infect. Dis. 75, 98–100. doi: 10.1016/j.ijid.2018.08.011
Tonini, M. A., Soares Jardim Lacerda Batista, J., Bueno, F. L., Borghi, M., Almeida, M. S., Spano, L. C., et al. (2018). Carbapenem-susceptible Escherichia Coli st3901 carrying MCR-1 and blactx–m genes isolated from a diabetic foot infection in brazil. J. Glob. Antimicrob. Resist. 13, 209–210. doi: 10.1016/j.jgar.2018.05.002
Treangen, T. J., Ondov, B. D., Koren, S., and Phillippy, A. M. (2014). The harvest suite for rapid core-genome alignment and visualization of thousands of intraspecific microbial genomes. Genome Biol. 15, 524. doi: 10.1186/s13059-014-0524-x
Tse, H., and Yuen, K. Y. (2016). Dissemination of the MCR-1 colistin resistance gene. Lancet Infect. Dis. 16, 145–146. doi: 10.1016/S1473-3099(15)00532-0
Tuo, H., Yang, Y., Tao, X., Liu, D., Li, Y., Xie, X., et al. (2018). The prevalence of colistin resistant strains and antibiotic resistance gene profiles in funan river, china. Front. Microbiol. 9:3094. doi: 10.3389/fmicb.2018.03094
Wang, R., van Dorp, L., Shaw, L. P., Bradley, P., Wang, Q., Wang, X., et al. (2018). The global distribution and spread of the mobilized colistin resistance gene MCR-1. Nat. Commun. 9:1179. doi: 10.1038/s41467-018-03205-z
Wang, Y., Wang, J., Wang, R., and Cai, Y. (2020). Resistance to ceftazidime-avibactam and underlying mechanisms. J. Glob. Antimicrob. Resist. 22, 18–27. doi: 10.1016/j.jgar.2019.12.009
Weisenfeld, N. I., Yin, S., Sharpe, T., Lau, B., Hegarty, R., Holmes, L., et al. (2014). Comprehensive variation discovery in single human genomes. Nat. Genet. 46, 1350–1355. doi: 10.1038/ng.3121
Wences, A. H., and Schatz, M. C. (2015). Metassembler: merging and optimizing de novo genome assemblies. Genome Biol. 16:207. doi: 10.1186/s13059-015-0764-4
Wu, J., Huang, Y., Rao, D., Zhang, Y., and Yang, K. (2018). Evidence for environmental dissemination of antibiotic resistance mediated by wild birds. Front. Microbiol. 9:745. doi: 10.3389/fmicb.2018.00745
Yang, F., Gu, Y., Zhou, J., and Zhang, K. (2019). Swine waste: a reservoir of high-risk blandm and MCR-1. Sci. Total Environ. 683, 308–316. doi: 10.1016/j.scitotenv.2019.05.251
Zankari, E., Hasman, H., Cosentino, S., Vestergaard, M., Rasmussen, S., Lund, O., et al. (2012). Identification of acquired antimicrobial resistance genes. J. Antimicrob. Chemother. 67, 2640–2644. doi: 10.1093/jac/dks261
Zavascki, A. P., Girardello, R., Magagnin, C. M., Antochevis, L. C., Maciel, R. A., Palmeiro, J. K., et al. (2018). Emergence of polymyxin b resistance in a polymyxin b-susceptible kpc-producing Klebsiella Pneumoniae causing bloodstream infection in a neutropenic patient during polymyxin b therapy. Diagn. Microbiol. Infect. Dis. 90, 134–138. doi: 10.1016/j.diagmicrobio.2017.10.006
Zhanel, G. G., Lawson, C. D., Adam, H., Schweizer, F., Zelenitsky, S., Lagacé-Wiens, P. R., et al. (2013). Ceftazidime-avibactam: a novel cephalosporin/β-lactamase inhibitor combination. Drugs 73, 159–177. doi: 10.1007/s40265-013-0013-7
Keywords: Polymyxin, colistin, plasmid, insertion sequence, hospital dissemination
Citation: Girardello R, Piroupo CM, Martins J Jr, Maffucci MH, Cury AP, Franco MRG, Malta FdM, Rocha NC, Pinho JRR, Rossi F, Duarte AJdS and Setubal JC (2021) Genomic Characterization of mcr-1.1-Producing Escherichia coli Recovered From Human Infections in São Paulo, Brazil. Front. Microbiol. 12:663414. doi: 10.3389/fmicb.2021.663414
Received: 02 February 2021; Accepted: 12 May 2021;
Published: 09 June 2021.
Edited by:
Kristina Kadlec, Independent Researcher, Wunstorf, GermanyReviewed by:
Aldert Zomer, Utrecht University, NetherlandsDiego Faccone, Administración Nacional de Laboratorios e Institutos de Salud (ANLIS), Argentina
Copyright © 2021 Girardello, Piroupo, Martins, Maffucci, Cury, Franco, Malta, Rocha, Pinho, Rossi, Duarte and Setubal. This is an open-access article distributed under the terms of the Creative Commons Attribution License (CC BY). The use, distribution or reproduction in other forums is permitted, provided the original author(s) and the copyright owner(s) are credited and that the original publication in this journal is cited, in accordance with accepted academic practice. No use, distribution or reproduction is permitted which does not comply with these terms.
*Correspondence: Raquel Girardello, raquel.girardello@usf.edu.br; João Carlos Setubal, setubal@iq.usp.br