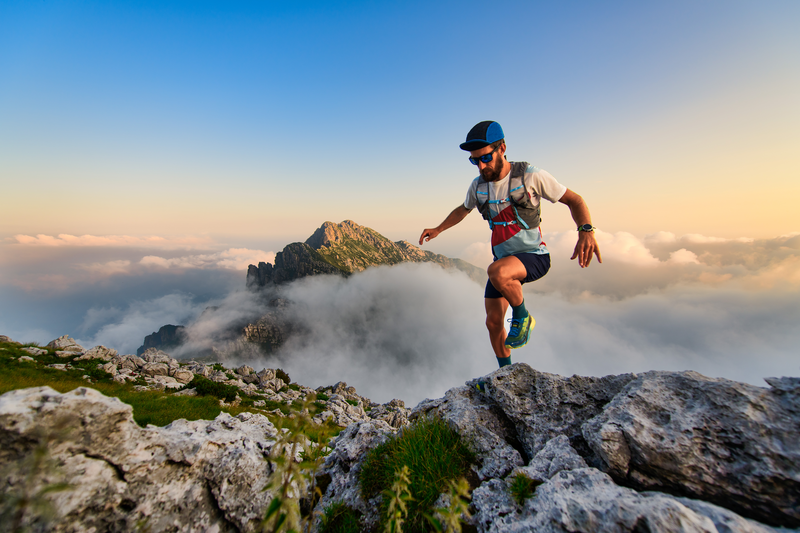
94% of researchers rate our articles as excellent or good
Learn more about the work of our research integrity team to safeguard the quality of each article we publish.
Find out more
REVIEW article
Front. Microbiol. , 07 May 2021
Sec. Microbial Immunology
Volume 12 - 2021 | https://doi.org/10.3389/fmicb.2021.660901
Viruses have evolved diverse strategies to manipulate cellular signaling pathways in order to promote infection and/or persistence. Human cytomegalovirus (HCMV) possesses a number of unique properties that allow the virus to alter cellular events required for infection of a diverse array of host cell types and long-term persistence. Of specific importance is infection of bone marrow derived and myeloid lineage cells, such as peripheral blood monocytes and CD34+ hematopoietic progenitor cells (HPCs) because of their essential role in dissemination of the virus and for the establishment of latency. Viral induced signaling through the Epidermal Growth Factor Receptor (EGFR) and other receptors such as integrins are key control points for viral-induced cellular changes and productive and latent infection in host organ systems. This review will explore the current understanding of HCMV strategies utilized to hijack cellular signaling pathways, such as EGFR, to promote the wide-spread dissemination and the classic life-long herpesvirus persistence.
Human cytomegalovirus (HCMV) infection is a significant public health threat worldwide (Stagno et al., 1986; Bentz et al., 2006; Arvin, 2007; Nogalski et al., 2014; Fulkerson et al., 2021). HCMV is a leading cause of morbidity and mortality in developing fetuses and the immunocompromised population (Stagno et al., 1986; Bentz et al., 2006; Arvin, 2007; Nogalski et al., 2014; Fulkerson et al., 2021). HCMV infection in neonates is the leading cause of congenital central nervous system damage and deafness (Boppana et al., 2011). HCMV is also a leading infectious agent negatively affecting the outcome of solid organ and bone marrow transplants. In healthy individuals, HCMV infection can cause mononucleosis and is associated with some vascular diseases and cancers (Soderberg-Naucler, 2008; Pass et al., 2009; Wang et al., 2017; Styczynski, 2018; Gerna and Lilleri, 2020).
A hallmark of HCMV infection is wide-spread dissemination of the virus from the blood in monocytes to most organs and then the establishment of latency in the bone marrow in progenitor cells that in turn, during reactivation, differentiate into monocytes to again disseminate the virus to host organ tissue (Sinzger et al., 1995; Smith et al., 2004a; Stevenson et al., 2014; Collins-McMillen et al., 2018b). Therefore, one of the first essential steps for hematogenous dissemination is the infection of monocytes (Smith et al., 2004b; Yurochko, 2008; Chan et al., 2010, 2012a; Nogalski et al., 2011). The virus, in turn, hijacks infected monocyte signaling pathways in order to promote efficient viral spread to most peripheral organs and the bone marrow (Smith et al., 2004b; Yurochko, 2008; Chan et al., 2010, 2012a; Nogalski et al., 2011). Furthermore, infiltration of infected monocytes into host organ systems allows for viral spread to additional hosts through release of new virus in a variety of body fluids (such as in saliva and urine) and spread to the bone marrow where latency is established in CD34+ hematopoietic progenitor cells (HPCs). The CD34+ HPCs during reactivation differentiate along myeloid lineage development pathways to become monocytes allowing organ dissemination and additional host to host spread (Goodrum et al., 2002; Kim et al., 2017; Collins-McMillen et al., 2018a). This review examines the processes of HCMV infection of these two critical cells and specifically how viral signaling through cellular receptors is a key molecular trigger for HCMV infection and persistence.
Human cytomegalovirus must first attach to the cell via an interaction between viral membrane glycoproteins and specific cell surface receptor-binding molecules (Chan et al., 2009b, 2012a; Collins-McMillen et al., 2017a). Several viral glycoprotein complexes are crucial for viral entry/internalization of monocytes and other cell types, two of which (gB and the gH complexes) will be focused on extensively in this report. gB and the gH/gL complexes help dictate infection tropism and serve as central ligands for viral attachment to clinically relevant cell types (Nogalski et al., 2013). The gB and the gH/gL complexes engage the cell through the epidermal growth factor receptor (EGFR) and cellular integrins, respectively (Chan et al., 2009b; Nogalski et al., 2011, 2013; Kim et al., 2016). This receptor-ligand engagement in turn activates cellular signaling and the functional changes in the target monocytes to promote both productive infection and persistence in host organ systems.
To contact a target host cell, the viral ligands in the envelope, gB (UL55), gH (UL75)/gL (UL115)/gO (ULl74), which make up the gH/gL/gO complex or the trimer, and the gH/gL/UL128-131 complex or the pentamer, and gM (UL100)/gN (UL73) interact with cellular receptors that in turn lead to the activation of various signal molecules in the infected host cells (Mach et al., 2005; Shimamura et al., 2006; Shen et al., 2007; Fields et al., 2013; Stegmann et al., 2017a; Liu et al., 2018; Nguyen et al., 2018; Gerna et al., 2019; Nishimura and Mori, 2019). There are some receptors that seem to be present on most infected cell types and these include heparan sulfate proteoglycans, cellular integrins (α2β1, α6β1, and αvβ3) (Feire et al., 2004; Wang et al., 2005) and Toll-like receptors (Compton et al., 2003; Boehme et al., 2006), while other receptors such as EGFR (Wang et al., 2003, 2005), are only found on some cell types and may help dictate tropism. Other potential tropism receptors include the Platelet-Derived Growth Factor Receptor [PDGFR (Wu et al., 2017; Nguyen and Kamil, 2018; Wu et al., 2018; Nishimura and Mori, 2019)], Neuropilin-2 [Nrp-2 (Martinez-Martin et al., 2018)], and Olfactory Receptor Family 14 Subfamily I Member 1 [OR14I1 (Xiaofei et al., 2019)]. In general, whether these receptors are specific for a specific cell type or are more conserved across a variety of cell types, they are important for viral attachment, internalization, and fusion and entry into the cytoplasm of the infected cell (Nowlin et al., 1991; Navarro et al., 1993; Boyle and Compton, 1998; Compton, 2000; Isaacson and Compton, 2009). They also all seem to initiate multiple downstream cellular signaling pathways during infection (Nogalski et al., 2007; Smith et al., 2007; Yurochko, 2008; Reeves et al., 2012).
As an extended introduction, we would like to emphasize why we chose to focus primarily on monocytes and CD34+ HPCs in this review. Both cell types are important targets for HCMV infection and are critical to life-long persistence and dissemination within an individual infected host and the general human population. Monocytes are essential for hematogenous dissemination of HCMV to multiple organ systems following primary infection (Nogalski et al., 2011). That is, HCMV uses the biological features of monocytes: motility, ability to migrate to all tissue types and differentiation into long-lived macrophages (Nogalski et al., 2012; Stevenson et al., 2014; Collins-McMillen et al., 2017b, 2018b) to promote initial dissemination to the multiple host organs that serve as the source of viral spread from host to host and for the eventual establishment of the viral persistence/latency seen in the bone marrow in CD34+ HPCs. Monocytes also seem to play an essential role in the establishment of viral latency through this initial spread and then in the organ dissemination following reactivation in CD34+ HPCs and the differentiation of these CD34+ HPCs into monocytes (Stevenson et al., 2014; Collins-McMillen et al., 2017b, 2018a; Kim et al., 2017). Like infection of monocytes, initial infection of CD34+ HPCs relies on receptor-ligand engagement with EGFR engagement being key to early infection (Kim et al., 2016). Although we did not focus on the receptor-ligand engagement for different cell types, distinct downstream signaling pathways, and functional consequences in this review, it also occurs during the infection of epithelial, endothelial cells, fibroblasts, and others (Sinzger et al., 1995; Chan et al., 2008b; Yurochko, 2008).
For HCMV to initially infect monocytes, the virus must deal with several biological barriers, and one such barrier is the short lifespan of blood monocytes and the initial lack of viral gene expression and replication in these cells. HCMV-infected monocytes are initially short lived and are non-permissive for productive infection Yurochko et al., 1989, 1990, 1992, 1995, 1997a,b, 1999; Taylor-Wiedeman et al., 1991; Mendelson et al., 1996; Hahn et al., 1998; Yurochko and Huang, 2000; Chan et al., 2010). To regulate these processes, HCMV utilizes a unique signalosome generated initially by the signaling downstream of gB/EGFR and pentamer/β1 and β3 integrin engagement at the cell surface and then activation of EGFR and integrin dependent events post entry (Collins-McMillen et al., 2017b; Fulkerson et al., 2020). This signalosome, as discussed in more detail below, alters key biological steps in infected monocyte motility (Smith et al., 2004b; Chan et al., 2009a) and survival through manipulation of Bcl-2 family members (Chan et al., 2010; Collins-McMillen et al., 2016). In addition, this viral ligand-induced signalosome promotes monocyte-to-macrophage differentiation and polarization of these differentiated macrophages (Yurochko et al., 1989; Stevenson et al., 2014; Collins-McMillen et al., 2017a, b, 2018b). Because macrophages are naturally long-lived cells and once differentiated can produce and shed virus for weeks to months (Smith et al., 2004b; Stevenson et al., 2014), they can serve as a source of organ persistence for the virus and shedding in bodily fluids to other hosts. Although distinct, latent infection of CD34+ HPCs also produces a unique signalosome that is essential for the establishment of, maintenance of, and reactivation from latency and the associated differentiation of these progenitor cells into monocytes (Goodrum et al., 2002; Streblow and Nelson, 2003). We hope to shed light on HCMV signaling through key cellular receptors, and how that signaling initiates productive infection and persistence in this review.
Human cytomegalovirus is a species-specific β-herpesvirus that infects an extensive range of cell types, including monocytes, macrophages, endothelial cells, epithelial cells, vascular smooth muscle cells, stromal cells, neuronal cells, fibroblasts, CD34+ HPCs, and hepatocytes (Sinzger et al., 1995; Jarvis and Nelson, 2002). Although HCMV can infect many different cell and tissue types, the virus has a set number of viral entry complexes that can be used, which means the virus has adapted to utilize these complexes in a way that promotes efficient transmission and infection of different cell types in the new host to allow for lifelong persistence (see Figure 1 for a cartoon of viral glycoproteins and their cognate ligands on monocytes/CD34+ HPCs and other cell types). HCMV gB, like all herpesvirus gBs, is essential for viral membrane fusion with cellular surface or vesicular membranes (Compton et al., 1992; Navarro et al., 1993; Tugizov et al., 1994; Vanarsdall et al., 2008; Isaacson and Compton, 2009; Zhou et al., 2015) and in an HCMV-specific manner, HCMV gB is a receptor-ligand binding protein for EGFR (Wang et al., 2003, 2005; Chan et al., 2012a; Fulkerson et al., 2020). HCMV gB is synthesized as a 160 kDa precursor that is cleaved by furin in the Golgi, resulting in 116 and 55 kDa fragments that become disulfide-linked to make the mature gB (Britt and Auger, 1986). This mature gB is what engages several cell-surface proteins, including the primary receptor, EGFR (Wang et al., 2003; Chan et al., 2012a; Fulkerson et al., 2020), as well as perhaps PDGFR-α (Soroceanu et al., 2008) and some integrins (Bentz and Yurochko, 2008; Feire et al., 2010). In monocytes, this receptor likely helps determine tropism for blood leukocytes, as B cells and T cells do not express EGFR, correlating with the lack of infection of these cells (Chan and Yurochko, 2014). In addition, PDGFR is not reported to be expressed on freshly-isolated blood monocytes (Chan et al., 2009b) or freshly-isolated CD34+ HPCs (Su et al., 2002) suggesting that the gB/EGFR interaction plays a key role in the infection of these cell types. For this review and for infection of monocytes and CD34+ HPCs, gB engagement of EGFR is essential for entry and the post entry events related to nuclear translocation as well as for the associated downstream signaling (Chan et al., 2009b; Kim et al., 2017; Fulkerson et al., 2020).
Figure 1. Schematic of the HCMV glycoprotein complexes and their cognate receptors. HCMV attachment and entry into monocytes depends on several envelope glycoprotein complexes including gM/gN, gB, and the gH complexes. gM/gN mediates initial attachment via glycosaminoglycans. gB is known to interact with the epidermal growth factor receptor (EGFR) on monocytes. gB may also interact with integrins on monocytes, although this is unresolved. The trimeric gH/gL/gO complex interacts with integrins and the platelet-derived growth factor receptor alpha (PDGFRα) on fibroblasts; at present the trimer only has been reported to interact with integrins on monocytes. The pentameric gH/gL/UL128-131 complex interacts with β1- and β3-integrins on monocytes. Several other receptors, such as OR14I1 and Nrp2, have been reported to bind to the pentamer; this engagement remains unresolved during infection of monocytes. The membrane of fibroblasts is illustrated on the right and the membrane of epithelial cells is illustrated on the left and they include the multiple receptors that have been reported to engage the HCMV glycoprotein complexes during infection of fibroblasts and epithelial cells, respectively.
There are multiple gH complexes, from the newly discovered gH interaction with UL116 [gH (UL75)/UL116] (Caló et al., 2016) to the known gH complexes of gH/gL (UL115)/gO (UL174) or the trimer (Stegmann et al., 2017a) and gH/gL/UL128-131 or the pentamer (Nguyen and Kamil, 2018; Nishimura and Mori, 2019). These unique complexes directly affect cell-type-specific entry. For example, fibroblast entry processes are largely mediated by the glycoproteins/glycoprotein complexes gB, gH/gL/gO (the trimer), and gM (UL100)/gN (UL73), whereas entry into epithelial cells, endothelial cells, and monocytes/macrophages also require the gH/gL/UL128-131 pentameric complex (Hahn et al., 1998, 2004; Jarvis and Nelson, 2002; Ricciotti and Fitzgerald, 2011; Aoki and Narumiya, 2012; Stegmann et al., 2017b). The HCMV gM/gN complex (Mach et al., 2005) is the most abundant glycoprotein complex found on virions (Soroceanu et al., 2008; Feire et al., 2010) and has been shown to interact with heparin sulfate proteoglycans on the cell surface (Kari and Gehrz, 1992), and may also have some intracellular roles during viral replication (Pignatelli et al., 2003; Mach et al., 2005). HCMV UL116 has recently been shown to be required for the production of infectious virus and may help chaperone gH complexes into virions (Caló et al., 2016; Nguyen and Kamil, 2018; Gatault et al., 2021).
There are both shared and unique features of the gH/gL glycoprotein complexes among the different herpesviruses. For example, gH/gL (along with gB) comprise the central herpesvirus membrane fusion machinery (Weed and Nicola, 2017). However, other aspects of these complexes are unique to HCMV and/or the other β-herpesviruses. gO (and homologs) are found only in β-herpesviruses, and the gO-associated trimer is required for cellular infectivity of cell-free HCMV virions (Wille et al., 2010; Liu et al., 2018); however, its role in modulating signal transduction pathways in virus-infected host cells is not resolved. The pentameric complex is unique to HCMV (Wang and Shenk, 2005b; Ryckman et al., 2008; Ciferri et al., 2015; Chandramouli et al., 2017) and is required for infection of epithelial and endothelial cells, as well as leukocytes (Bentz et al., 2006; Kim et al., 2017). The complex is genetically unstable during HCMV passage in fibroblasts and many lab-adapted stains have lost this complex through mutation in one or more of the pentamer complex-specific glycoprotein coding sequences (Akter et al., 2003; Wille et al., 2010). Repairing the HCMV pentamer complex in a fibroblast-adapted laboratory strain restores infectivity of epithelial and endothelial cells, and monocytes (Wang and Shenk, 2005a, b; Nogalski et al., 2013). The pentamer binds to different cellular integrins and seems to have different integrin binding partners on different cell types (Nogalski et al., 2014; Collins-McMillen et al., 2017b; Vanarsdall et al., 2018). In addition, the pentamer was recently reported to bind to Nrp2 (Martinez-Martin et al., 2018) and OR14I1 (Xiaofei et al., 2019) on epithelial cells, perhaps providing epithelial cell tropism. The pentamer has also been documented to interact with thrombomodulin (Hsu et al., 2015; van den Boomen and Lehner, 2015). CD147 (Vanarsdall et al., 2018) and CD151 (tetraspanin) and THY-1 (CD90) have also been found to play a role during HCMV infection of some cell types (Hochdorfer et al., 2016). However, the biological relevance and importance of these receptors in infection monocytes or CD34+ HPCs and in cell signaling remain unresolved. Overall, viral glycoproteins are an indispensable cell type specific strategic weapon for successful viral infection via their receptor binding capabilities, their ability to induce cellular signaling in host cells and to allow viral entry into that target cell. Further studies are needed to determine the precise mechanisms by which the many HCMV glycoproteins and the many receptors together or independently alters host signaling pathways in the many cells that HCMV infects.
Figure 2. Human cytomegalovirus (HCMV) glycoprotein binding to cognate receptors controls viral signaling, trafficking and the key cellular functions required for productive infection of monocytes. The HCMV glycoproteins bind several cellular receptors, including EGFR and the β1- and β3-integrins. Following binding, these receptor engagements trigger a variety of signal transduction pathways in the infected monocyte. For example, gB binding to EGFR results in activation of the intrinsic EGFR tyrosine kinase and down stream signaling that is required for entry, for the unique multi-vesicular nuclear translocation process seen in monocytes, as well as for motility, and the survival of the monocytes and their differentiation into macrophages. The engagement of the pentameric complex, gH/gL/UL128-131 with β1- and β3-integrins on the surface of monocytes activates c-Src to drive the required signaling pathways needed for entry, nuclear translocation, motility, survival and differentiation. More specifically, this downstream signaling following EGFR and integrin engagement acts in concert to activate numerous down stream signaling pathways, including the NF-κB pathway, PI(3)K, and the MAPK pathway, which culminates in enhanced transactivation of many host cell promoters via increases in transcriptional regulators, altercations in actin regulatory proteins that modulate motility (such as N-WASP and paxillin) and changes in Mcl-1 and Bcl-2 to promote survival of short-lived monocytes.
The HCMV glycoproteins regulate the key intracellular signaling pathways in human primary monocytes required for productive infection and the establishment of persistence. Following the engagement of heparan sulfate proteoglycans, HCMV infection of monocytes begins via the interaction of gB and the gH/gL complexes with host cell surface EGFR and β1 and β3 integrins (Chan et al., 2009b, 2012a; Nogalski et al., 2011, 2013; Kim et al., 2016; Fulkerson et al., 2020). Epidermal growth factor receptor (EGFR or ErbB-1) is a member of the ErbB receptor tyrosine kinase family, which can bind epidermal growth factor (EGF) or transforming growth factor-alpha (TGF-α) (Wieduwilt and Moasser, 2008). Studies have revealed that downstream EGFR signaling can be unique and functionally distinct based on the ligand and the cell type expressing EGFR (Wieduwilt and Moasser, 2008). In support of this cell type specific EGFR biology during HCMV infection, one can note the differences between EGFR-dependent signaling seen in infected monocytes (Chan et al., 2009b, 2012a; Nogalski et al., 2011, 2013; Kim et al., 2016; Fulkerson et al., 2020) vs. that seen in infected endothelial cells (ECs) (Bentz and Yurochko, 2008) or infected trophoblasts (LaMarca et al., 2006). There are also reported differences in EGFR signaling in CD34+ HPCs (Kim et al., 2017) and that will be discussed in more detail below.
In monocytes, HCMV induces the phosphorylation of EGFR following viral binding and that engagement initiates the activation of PI(3)K and Akt. When considering other peripheral blood cell types, such as T cell and B cells, EGFR expression seems to be involved in the select myeloid tropism of the virus (Xiaofei et al., 2019). This HCMV-activated EGFR in monocytes promotes motility and transendothelial migration to allow virus-infected cells to gain entry into peripheral organ tissues (Smith et al., 2004a, b, 2007; Bentz et al., 2006; Chan et al., 2009b; Collins-McMillen et al., 2017a, b). Mechanistically, gB/EGFR engagement results in the upregulation and select usage of the Neural Wiskott–Aldrich Syndrome protein (N-WASP) as a mechanism to promote heightened motility (Chan et al., 2009b), as opposed to the use of the standard Wiskott–Aldrich Syndrome protein (WASP) that controls chemokine-induced motility in monocytes (Snapper and Rosen, 1999; Tsuboi, 2006; Chan et al., 2009b; Lee et al., 2017). That is, under normal circumstances, WASP usually helps modulate monocyte motility (Snapper and Rosen, 1999; Tsuboi, 2006; Chan et al., 2009b; Lee et al., 2017). WASP and N-WASP are actin nucleators that mechanistically drive changes in the actin cytoskeleton associated with cell movement. This use of N-WASP showcases some of the distinct properties of the gB/EGFR receptor-ligand engagement in monocytes. N-WASP is responsible for highly motile cancer cell movement (Yamaguchi and Condeelis, 2007; Kurisu and Takenawa, 2010; Frugtniet et al., 2015) and biochemically is more efficient at nucleating actin than WASP. Thus, we propose this switch to N-WASP during HCMV infection of monocytes promotes migration in the absence of a chemotactic gradient into organ tissues (Chan et al., 2009b). Rapid activation of the EGFR signaling pathway following HCMV infection also turns out to be essential for viral entry into monocytes [Figure 2 (Chan et al., 2009b; Fulkerson et al., 2020)]. Thus, EGFR plays an essential role in the pathobiology of HCMV by mediating viral entry into monocytes and stimulating the cellular signaling activity that promotes hematogenous dissemination of the virus.
The HCMV gB engages EGFR and then rapidly and directly activates downstream signaling via its intrinsic tyrosine kinase (Walker et al., 1998). gB mainly interacts with EGFR on monocytes, although in some cell types, it may also interact with cellular integrins (Yurochko et al., 1992; Bentz and Yurochko, 2008; Nogalski et al., 2011, 2013; Collins-McMillen et al., 2016; Kim et al., 2016). This receptor-ligand engagement stimulates the activation of transcription factors, NF-κB (discussed in more detail below) and Sp-1 (Yurochko et al., 1995, 1997a,b) and numerous signaling pathways (Chan et al., 2012a; Campadelli-Fiume et al., 2016; Eberhardt et al., 2016; Collins-McMillen et al., 2017b; Yurochko, 2017). In addition to activating signaling molecules and transcription factors, these functional changes initiated by HCMV gB binding to EGFR serve as a molecular convergence point linking the changes in molecular and biochemical pathways with the biological changes required for productive infection. One example is the viral activation of STAT1 (Collins-McMillen et al., 2017a, b). Not only is STAT-1 activated within minutes of viral binding, as well as chronically (3 plus weeks after infection) as documented by changes in the patterns of appropriate activating phosphorylated residues, its specific activation is required for the survival and differentiation of monocytes that ultimately is needed for productive infection and viral persistence in organ macrophages (Collins-McMillen et al., 2017b).
The HCMV infection elicits a robust immune response (Gerna et al., 2005; Sinzger et al., 2006; Mason et al., 2012). However, symptomatic infections largely only occur in immunocompromised patients (Stagno et al., 1986; Bentz et al., 2006; Arvin, 2007; Nogalski et al., 2014; Fulkerson et al., 2021). Thus, the immune response keeps the virus under tight control in healthy individuals. How viral infection and the immune response interacts during life-long persistence remains unresolved? With monocytes playing a central role during infection, understanding how the virus manipulates EGFR signaling pathways in monocytes (Yurochko, 2008; Stevenson et al., 2014), promotes reactivation (Streblow and Nelson, 2003; Nogalski et al., 2014; Collins-McMillen et al., 2018a, 2019), enhances production of suppressive cytokines (Chan et al., 2009a; Wu et al., 2017; Mikell et al., 2019) and alters differentiation and phenotypic polarization of monocytes and macrophages (Chan et al., 2008a, 2012a; Collins-McMillen et al., 2017a, b) could pave the way for a better understanding of the pathobiology of the virus.
We previously described that viral engagement of β1 and β3 integrins are essential for infection of human peripheral monocytes via the interaction of the HCMV gH/gL/UL128-131 complex with these two integrin families [Figure 2 (Nogalski et al., 2013; Kim et al., 2016)]. It is also known from other groups that HCMV binds to integrins and facilitates viral entry into human fibroblasts (Compton, 2000; Wang et al., 2005; Campadelli-Fiume et al., 2016). However, we have noted that there are functional changes that occur during monocytes infection, including the requirement for multiple integrins, that was not observed in fibroblasts (Nogalski et al., 2013; Campadelli-Fiume et al., 2016). That is, engagement of both β1 and β3 integrins are required during viral attachment to mediate entry and outside-in signaling in monocytes (Campadelli-Fiume et al., 2016). HCMV binding to integrins on monocytes rapidly activates the integrin/c-Src signaling pathway to induce PI(3)K and other essential signaling pathways.
The HCMV binding to host cell receptors induces signals in a distinct manner for each cell type. In monocytes, this receptor-ligand engagement results in cellular differentiation and long-term cellular survival (Smith et al., 2004b; Chan et al., 2008a; Yurochko, 2008; Campadelli-Fiume et al., 2016); but how are integrins involved? Integrins are a family of heterodimeric receptors composed of a single α-chain and a single β-chain that when engaged via their various cognate ligands leads to the induction of specific signaling pathways via activation of c-Src and other tyrosine kinases (Yamada and Miyamoto, 1995; Hynes, 2002). Based on transcriptome analysis, integrin-mediated signaling plays a central role as a convergent point linking the specific cellular molecules that have been activated by HCMV viral binding on monocytes. For example, the integrin-mediated rapid phosphorylation of paxillin identified that paxillin is both required for HCMV entry and for the hematogenous dissemination of the virus in monocytes through altered motility (Nogalski et al., 2011). Paxillin is an adaptor protein and an important signal transducer in the regulation of actin rearrangement during cellular adhesion and movement. HCMV-infected human primary monocytes showed heightened levels of paxillin mRNA and protein expression in an integrin-dependent manner (Nogalski et al., 2011). The use of siRNA knockdown in monocytes revealed that paxillin is essential for virus-induced motility and viral entry (Nogalski et al., 2011). Thus, the activation of the integrin/c-Src/paxillin signaling pathway is essential for triggering the cellular changes that allow for HCMV entry and hematogenous dissemination.
It is important to note that both the gB/EGFR and the pentamer/integrin signaling axes drive independent events, as well as cooperate to modify the infected cell. For example, as discussed briefly in this review, integrins activate paxillin through integrin/c-Src activation (Nogalski et al., 2011), and EGFR separately regulates the expression of the N-WASP (Chan et al., 2009b). Together, both are required for activation of motility, since inhibition of either pathway block infected monocyte motility and migration. From this data, HCMV-cell interactions emerge as an essential trigger for the cellular alterations that allow for HCMV entry and hematogenous dissemination.
An investigation into receptor-ligand mediated signaling and the associated regulation of survival and differentiation led us to a finding that cellular signaling during viral binding also controls unique post entry events; these include the intracellular trafficking and nuclear translocation of the virus during infection of monocytes (Figure 2). All herpesviruses replicate their DNA in the nucleus, and thus the steps of intracellular trafficking and nuclear translocation are essential steps following viral entry for productive infection. As this brief discussion will show, the virus has evolved to utilize the same signaling pathways required for entry to modulate, in a cell specific manner, these key post entry events. Briefly, we observed in monocytes that the gH/gL/UL128-131 complex through binding to β1 and β3 integrins and activation of c-Src signaling drives a pattern of intracellular trafficking and nuclear translocation not seen in other cell types. That is, HCMV nuclear translocation in monocytes is distinct from that seen in fibroblasts, endothelial cells, and CD34+ cells (Kim et al., 2016; Fulkerson et al., 2020). Nuclear translocation occurs roughly 30 min post infection in fibroblasts and endothelial cells (Kim et al., 2016). In contrast, post entry of viral DNA into the nucleus of monocytes is extended until 3 days post infection (dpi) (Kim et al., 2016; Fulkerson et al., 2020). Furthermore, we showed that mature HCMV particles are initially retained in early endosomes, they then move to the trans-Golgi network (TGN) and then finally to recycling endosomes in monocytes, where de-envelopment occurs (Kim et al., 2016). We recently added to this work and showed that gB/EGFR binding continues past the entry process and that this chronic gB/EGFR engagement was essential for nuclear translocation following the integrin/pentamer/c-Src decision point (Fulkerson et al., 2020). That is, viral engagement of EGFR continues from the attachment step through the post entry phase in order to promote correct viral tracking and nuclear translocation in monocytes (Fulkerson et al., 2020). Although studied in less detail, it seems to be a similar, although expedited, process occurs in CD34+ HPCs (Kim et al., 2017). This unique signaling pathway suggests that HCMV-infected monocytes use a distinctive mechanism in infected monocytes to promote productive infection and that this process is controlled by pentamer/integrin and gB/EGFR engagement.
Monocyte-to-macrophage differentiation in HCMV-infected monocytes is essential for long-term viral persistence. Monocytes have a short lifespan of 1–3 days in circulation and are not initially permissive for viral gene expression and replication (Min et al., 2020). Thus, HCMV must extend the life span of the infected monocytes and promote differentiation of these cells into long lived macrophages that are permissive for viral replication (Min et al., 2020). Briefly, as described in the mentioned review and associated references (Min et al., 2020), HCMV binds to and enters monocytes and initiates the process of nuclear translocation; simultaneously to the viral manipulated events that are essential to getting the genome into the nucleus, the virus must promote survival and differentiation of that infected monocyte. Only in the differentiated cell (the macrophage) does viral gene expression initiate along with all of the other steps associated with productive infection. This process is essential for organ infection following primary infection (Min et al., 2020) and following reactivation of virus from latency. Figures 2, 3 show the signaling associated with these discussed biological changes. Mcl-1, a member of the Bcl-2 gene family, serves to regulate monocyte survival (Yang et al., 1996; Zhou et al., 1997). HCMV uses the EGFR/PI3K signaling pathway to upregulate this anti-apoptotic protein after infection; thus, overcoming the intrinsic apoptotic fate of monocytes (Chan et al., 2010). Mcl-1 was upregulated/maintained early in HCMV-infected monocytes (Chan et al., 2010) and then begins to diminish to near background levels around 48 h post infection (hpi). Reeves et al. (2012) have also shown the induced expression of Mcl-1 during monocyte infection. siRNA-mediated knockdown of Mcl-1 revealed the strong anti-apoptotic role that Mcl-1 plays in HCMV-infected monocytes during the first 48 hpi (Chan et al., 2010). Additional studies showed that Bcl-2 then takes over for Mcl-1 in monocytes after the first 48 hpi (Chan et al., 2010; Stevenson et al., 2014; Collins-McMillen et al., 2016). Bcl-2 is known to be an anti-apoptotic factor in the survival of myeloid cells (Lin et al., 1996; Lagasse and Weissman, 1997; Sanz et al., 1997; Adams and Cory, 1998; Perlman et al., 2000; Liu et al., 2001; Zhang et al., 2003; Reeves et al., 2012; Zhu et al., 2018; Shnayder et al., 2020). Molecularly, known to be anti-apoptotic, their regulation and linear regulation (Mcl-1 and then Bcl-2) is not seen in mock infected cells and monocytes treated with other stimuli (Zhou et al., 1997; Collins-McMillen et al., 2016). Both of these factors are induced by cellular signaling with Mcl-1 early regulation being controlled by the gB/EGFR axis and the later Bcl-2 upregulation being largely controlled by the pentamer/integrin axis (Zhou et al., 1997; Chan et al., 2010; Reeves et al., 2012; Collins-McMillen et al., 2016). To add to the potential complexities of the control of monocyte survival, HCMV seems to block full caspase-3 activation, while inducing partial activation of caspase-3, which in turn is important for monocyte-to-macrophage differentiation (Chan et al., 2012b). Thus, it seems that HCMV modulation of monocyte survival is related to monocyte-to-macrophage differentiation (discussed below) and these combined major biological changes, usurped during infection of monocytes, are essential for productive organ infection and long-term persistence of the virus in a variety of organ macrophages (Min et al., 2020).
Figure 3. Human cytomegalovirus infection of monocytes drives a number of key molecular mechanisms required to enhance survival of normally short-lived monocytes and for the promotion of the differentiation of these infected monocytes into long-lived productively infected macrophaqes. It is important to note that monocytes, although they can be infected, they are not permissive for viral replication; only upon differentiation is de novo viral gene expression observed. HCMV-infected monocytes show enhanced expression of Mcl-1 early after infection (<48 h post infection) to prolong survival through the key 48-h viability gate. Mcl-1 levels then gradually decrease through this 48 h viability gate. Enhanced expression of Bcl-2 is then observed, as well as partial activation of caspase-3, which together seem to promote both long term survival and differentiation of these infected monocytes toward viral replication-permissive tissue macrophages that begin to produce mature virus around 3 weeks after infection.
Because infected monocyte-to-macrophage differentiation is required for viral replication and long-term viral persistence, we have focused on the control of differentiation of monocytes into macrophages and how cellular signaling via gB-EGFR engagement controls this process. In general, macrophage phenotypes have been classified as M1 and M2 (Chan et al., 2009a; Italiani and Boraschi, 2014). M2 macrophages have been further classified into M2a, M2b, and M2c (Mantovani et al., 2004). M1 macrophages are usually considered to be classically activated cells and produce proinflammatory cytokines and these cells help initiate immune responses (Mantovani et al., 2004; Sica and Mantovani, 2012). M2 macrophages are usually considered to be alternatively activated cells and generally produce anti-inflammatory cytokines or participate in events such as wound healing (Mantovani et al., 2004; Lawrence and Natoli, 2011; Murray and Wynn, 2011; Tugal et al., 2013). HCMV infection of monocytes stimulates the differentiation of infected cells toward macrophages (Figure 3) that are polarized toward an M1 proinflammatory phenotype that we argue is a critical step for viral persistence (Smith et al., 2004a; Min et al., 2020). It is important to note that these infected macrophages, although biased toward M1 (based on ‘omics profiling), also show aspects of M2 polarization, especially the production of some anti-inflammatory and immune inhibitory cytokines (Smith et al., 2004a; Chan et al., 2008a; Min et al., 2020). For example, analysis of chemokine production by transcriptome and secretome analysis revealed that HCMV-infected monocytes exhibit a distinct reprogramming of their differentiation and polarization with more M1-associated gene transcripts being upregulated than M2-associated gene transcripts. Similarly, M1-associated chemokines were also more dominantly secreted compared to M2-associated chemokines in HCMV-infected monocytes (Chan et al., 2008a, 2009a). In both of these ‘omics analyses, we saw a bias toward an M1 phenotype, but not an absolute, as the infected cells appeared to be unique M1/M2 polarized macrophages expressing key elements of both polarization potentials. It seems that the virus favors the aspects of both M1 and M2 that promote persistence (Stevenson et al., 2014). That is, the proinflammatory aspects are useful to allow monocytes to move from blood to tissue and differentiate in macrophages that can be productively infected and that can recruit additional monocytes while retaining the ability to secrete immune inhibitory products and prevent T and B cell infiltration (Chan et al., 2008a, 2009a). Flow cytometry analysis has also shown that both M1- and M2-associated macrophage markers were increased in HCMV-infected monocytes when compared to mock-infected control monocytes (Chan et al., 2008a, 2009a; Stevenson et al., 2014; Collins-McMillen et al., 2017a, b). Viral receptor-ligand events dictate these changes - that is the gB/EGFR and pentamer/integrin signaling are key to dictating and initiating this process of differentiation and polarization (Chan et al., 2012a, b; Min et al., 2020).
The above-mentioned changes occur during primary or direct infection of freshly isolated blood monocytes. Because monocytes are also an essential player in the process of reactivation from latency, where infected CD34+ HPCs differentiate during reactivation into monocytes, there is also an intense interest in understanding the phenotypic and molecular underpinnings of these cells during reactivation. Differences appear to exist in the nature of these monocytes during reactivation and this is likely due to the variable nature of these cells. It has been argued that reactivating monocytes express a proinflammatory biology (Goodrum et al., 2002; Streblow and Nelson, 2003; Sinclair, 2008) and/or an immunosuppressive or anergic state as described by Zhu et al. (2018) and Shnayder et al. (2020). Together, this combined biological data suggest that HCMV directs the nature of the differentiation and the M1- and M2-polarized phenotypes (Stevenson et al., 2014), and that this end polarization phenotype does not fall into a strict M1- or M2-like phenotype or perhaps does not fall into a strict proinflammatory or immunosuppressive state.
NF-κB is essential for initial HCMV gene expression since the HCMV major immediate early promoter requires NF-κB (Fields and Knipe, 2013; Fields et al., 2013). This early identification of the impact of NF-κB on the initiation of HCMV gene expression served as the impetus to drive early studies into the regulation of NF-κB. It has been shown that HCMV activates both the canonical and non-canonical NF-κB signaling pathways (Figure 2) to aid in viral gene expression, viral replication, and productive infection (Andreoni et al., 2002; Chan et al., 2008b, 2012a; Yurochko, 2008; Collins-McMillen et al., 2017b). From a regulatory standpoint, it was shown in both fibroblasts and monocytes that gB- and gH-mediated signaling induces rapid activation of NF-κB signaling pathways (and degradation of IκBα); only in monocytes, however, did this rapid activation of NF-κB lead to increased inflammatory cytokine production, motility, endothelial cell adhesion, and transendothelial migration (Yurochko et al., 1997a, 1999; Smith et al., 2004b, 2007; Yurochko, 2008; Chan et al., 2009b), suggesting cell type-specific effects. Because viral-induced changes in NF-κB seem to control cellular function in monocytes separate from the direct role on viral gene expression, NF-κB activation and its role in changes in infected monocyte function remain a topic of interest. Furthermore, because NF-κB controls multiple aspects of hematopoiesis and cellular development (Hancock et al., 2017; Zhang et al., 2017), it is likely the receptor-ligand changes in this transcription factor may help explain some of the broad biological changes described in the sections above. ‘Omics profiling is consistent with this idea, where inhibition of NF-κB signaling blocked or down-regulated over 500 genes at 4 hpi, including multiple gene products associated with apoptosis, motility, and differentiation (Chan et al., 2008b). These observations imply that modulation of NF-κB signaling serves as a cell-specific convergence point where viral activation mediated by receptor-ligand engagement through EGFR and integrins stimulates a cell in a specific manner to promote the required changes for infection of that cell. It is important to point out that the viral miRNAs miR-US5-1 and miR-UL112-3p target the IκB kinases IKKa and IKKb for downregulation and can negatively influence proinflammatory cytokine expression suggesting additional mechanisms by which HCMV may specifically control this key pathway or specific parts of this pathway during lytic and/or latent infection (Hancock et al., 2017). Other transcription factors have also been reported to be regulated by receptor-ligand signaling and during key points of latent or lytic infection cycle (Fields and Knipe, 2013; Fields et al., 2013). The entirety of this process is beyond the scope of the current review.
The HCMV latency and reactivation are crucial for long-term persistence and stable spread within the human population (Jarvis and Nelson, 2002; Streblow and Nelson, 2003; Petrucelli et al., 2009; Buehler et al., 2016; Collins-McMillen and Goodrum, 2017; Kim et al., 2017; Collins-McMillen et al., 2018a, 2019; Hancock et al., 2020a). HCMV latency also exploits cellular signaling pathways to help establish and maintain latency and then upon recognition of appropriate signals, to manipulate the cellular environment and allow successful viral reactivation and differentiation of the infected CD34+ HPCs to blood monocytes and then to tissue macrophages (Jarvis and Nelson, 2002; Petrucelli et al., 2009; Collins-McMillen et al., 2018a, 2019; Hancock et al., 2020a). As with primary infection, EGFR signaling is also an important cellular receptor controlling this aspect of the viral infection process (Figure 4). HCMV activation of EGFR and downstream PI3K signaling is important for viral entry into CD34+ HPCs (Kim et al., 2017). Furthermore, through the use of the EGFR inhibitor, AG1478, it was shown that inhibition of EGFR signaling post entry resulted in increased transcription of IE1/IE2, while curbing the transcription of the latency-associated UL138 transcript (Kim et al., 2017). EGFR is also essential for the maintenance of latency, with the virus controlling a low level of constant signaling to maintain stable latency (Buehler et al., 2019; Mikell et al., 2019); this will be discussed in more detail below. Thus, viral signaling during attachment and entry is important in a distinct manner in numerous cell types essential for viral persistence; while long-term regulation of EGFR signaling and possibly integrin signaling post entry is critical for latency and persistence. Cellular differentiation (Min et al., 2020) is also closely related to both latency and reactivation and one expects multiple aspects of altered signaling during reactivation and latency to be central to the process in much the same way that signaling is essential for the establishment of persistence during primary infection.
Figure 4. Many HCMV-expressed proteins and miRNAs regulate the signaling pathways known to be important for the establishment, maintanence and reactivation from latency. The initial infection of CD34+ HPCs lays the foundation for the establishment of latency. Initially, gB engagement of EGFR and the ensuing signaling is required for HCMV entry into CD34+ HPCs. This initial ligand-dependent event prepares the cell for the establishment of latency through the manipulation of host signaling pathways and regulation of viral latent gene expression. These latency gene products in turn are critical for the establishment, the maintenance of and/or reactivation from latency. For example, gene products such as UL138 and UL135 regulate viral latency and host interactions by regulating various signaling pathways [such as Egr-1 activity or PI(3)K signaling] during the establishment of and exit from latency. Other products such as the viral miRNAs miR-US5-1 and miR-UL112-3p target and down regulate IKKα and IKKβ to control inflammatory cytokine regulation (such as IL-6 and TNFα) and likely to mitigate NF-KB dependent signaling that might interfere with latency programming. Other products such as soluble UL7 are released during reactivation and through binding to the Flt-3R induces activation of the downstream PI3K/AKT and MAPK/ERK signaling pathways that in turn triggers the HPC and monocyte differentiation required for complete viral reactivation. US28, another critical latency modulator, is involved in the MEK/ERK and EGFR/PI3K signaling required for HCMV reactivation from latency, as well as playing a key role in monocyte to macrophage differentiation. In addition, a variety of viral miRNAs (such as miR-US5-1, miR-US5-2, miR-US22, and miR-UL112-3p) target cellular processes and regulate virus-mediated signaling. As examples, miR-US5-2 blocks NAB1 activity, a represser of TGFβ resulting in increased production of the TGFβ that suppresses hematopoietic events; miR-US5-2 also down regulates GAB1, thus lowering MEK/ERK signaling, as well as regulates EGR1 and UL138 expression supporting a role for miR US5-2 during reactivation. Overall these viral miRNAs, UL7, US28, UL138/135 control vital signaling pathways during the establishment, maintenance of and during reactivation from latency and are intimately involved in the differentiation of the infected CD34+ HPCs into monocytes and then into productive tissue macrophages.
UL135 and UL138 are two key viral genes in the ULb’ region important in latency and reactivation. They have been shown to have opposing roles in regulating EGFR’s function in viral replication/reactivation (Umashankar et al., 2014; Buehler et al., 2016). The ULb’ region encodes the UL133-UL138 locus, which is required for infection and persistence in the host. The UL133, UL135, UL136, and UL138 genes have been shown to have important functions in vascular endothelial cells (Bughio et al., 2013, 2015) and to differentially regulate latency and reactivation in CD34+ HPCs (Petrucelli et al., 2009, 2012; Umashankar et al., 2011, 2014). pUL135 appears to promote replication, via inhibition of EGFR signaling through the turnover of EGFR from the cell surface. UL135 promotes endocytic trafficking of EGFR for lysosomal degradation through its interaction with the adapter proteins, Abelson interactor 1 (ABI-1) and SH3 domain-containing kinase-binding protein 1 (CIN85) (Rak et al., 2018). Loss of UL135 or disruption of its interaction with Abi-1 or CIN85 results in increased cellular and surface levels of EGFR and a failure to reactivate from latency (Buehler et al., 2016; Rak et al., 2018). In contrast, pUL138 activity is required for continued surface expression of EGFR (Buehler et al., 2016). Thus, in this model, pUL135 and pUL138, along with EGFR signaling, comprise a molecular convergence point to control latency and reactivation.
Viruses encode miRNAs that regulate viral and host gene expression to generate a more favorable cellular environment and/or inhibit host immune responses. Several herpesvirus family members express miRNAs that regulate host cell signaling pathways (Gottwein and Cullen, 2008; Hook et al., 2014; Hancock et al., 2020a). The 14 HCMV miRNAs (Stark et al., 2012) are unique among human herpesviruses because they are not clustered within defined latency-associated genomic regions and thus are differentially expressed during lytic and latent infection (Dunn et al., 2005; Pfeffer et al., 2005; Buck et al., 2007; Dolken et al., 2007). Overall, herpesvirus miRNAs target broadly similar cellular functions, including secretory pathways, immune evasion, survival, and proliferation of infected cells. A number of the HCMV miRNAs control the signaling pathways associated with long-term viral persistence; some of these key miRNAs are briefly discussed below.
HCMV miR-US5-1, miR-US5-2, and miR-UL112-3p down-modulate secretion of the inflammatory cytokines tumor necrosis factor-alpha (TNF-α) and interleukin-6 (IL-6), by targeting multiple members of the endocytic secretory pathway (Hook et al., 2014). In addition, miR-US5-1 and miR-UL112-3p block NF-κB-dependent induction of proinflammatory cytokines through direct targeting of the IκB kinase (IKK) complex components IKKα and IKKβ (Hancock et al., 2017). It has also been determined that miR-UL112-3p can modulate the Toll-Like Receptor (TLR) innate immune pathway through direct targeting of TLR2. Because TLRs play a critical role in controlling viral infections (Akira et al., 2006), this altered signaling could also be involved in viral persistence. Specifically, it was shown that miR-UL112-3p efficiently down-regulated the TLR2/IRAK1/NF-κB signaling axis (Landais et al., 2015).
The HCMV reactivation from latency can cause significant disease in transplant recipients (Safdar et al., 2002; Ljungman et al., 2011; Hancock et al., 2020a). Clinical manifestations include myelosuppression and graft rejection as a result of infection of CD34+ HPCs (Fries et al., 1993). Mechanistically, little had been uncovered to account for the global suppression caused by infection of only a small number of CD34+ HPCs, until a recent study examining the regulation of TGF-β. TGF-β is a member of a multifunctional cytokine family responsible for many cellular processes (Marshall et al., 2000; Capron et al., 2010; Blank and Karlsson, 2015), including potent inhibition of early progenitor cell proliferation (Sing et al., 1988). Recently it was shown that latently expressed HCMV miR-US5-2 downregulates NAB1, a repressor of the transcriptional factor NGFI-A (Egr-1), which in turn results in increased TGF-β production that causes myelosuppression in CD34+ HPCs. These results implicate viral miRNAs in regulating HCMV-induced myelosuppression during solid organ and hematopoietic stem cell transplantation (Hancock et al., 2020a). Viral miRNAs can also influence viral gene expression. For example, miR-US5-1 and miR-US5-2 can both regulate HCMV US7 expression (Tirabassi et al., 2011) while miR-UL112-3p targets the immediate early protein IE1 (IE72) (Grey et al., 2007; Murphy et al., 2008), which has implications in latency establishment and immune cell recognition (Lau et al., 2016). Together, these examples show how the viral miRNAs can influence cell signaling and cytokine secretion that in turn promotes key elements of viral persistence.
So far, several studies have addressed how HCMV miRNAs influence EGFR and EGFR-dependent downstream signaling. HCMV miR-US5-2 targeting of the EGFR adaptor protein GAB1 results in a block in MEK/ERK signaling downstream of EGFR activation that affects both cell proliferation and expression of pUL138, thus interfering with the feed-forward loop of EGFR activation of pUL138 expression (Hancock et al., 2020b). pUL138 expression is also regulated by the HCMV miRNA miR-US22, which directly targets the transcription factor Egr-1 downstream of EGF-mediated MEK/ERK signaling. Through targeting Egr-1, miR-US22 regulates pUL138 expression and CD34+ HPC proliferation and plays an important role in viral reactivation (Mikell et al., 2019). With the role chronic EGFR signaling plays in the maintenance of latency (Buehler et al., 2016), it seems probable that these miRNAs will strongly tune the EGFR response by modulating signaling pathways to promote latency or, when appropriate, viral reactivation.
UL7 is a secreted HCMV-encoded signaling molecule known to play a robust role during lytic and latent infection (Engel et al., 2011; Crawford et al., 2018; Perez-Carmona et al., 2018; Min et al., 2020). UL7 belongs to the HCMV RL11 gene family (RL11-13, UL1, UL4-11, RL6, and RL5A) and signals through Fms like tyrosine kinase 3 receptor (Flt-3R), a Class III receptor tyrosine kinase predominantly expressed in HCMV infection of myeloid lineage cells, such as CD34+ HPCs or monocytes (Beaudin et al., 2014).
Flk2/Flt3 promotes both myeloid and lymphoid development by expanding non-self-renewing multipotent hematopoietic progenitor cells (Beaudin et al., 2014). The binding of HCMV UL7 to the Flt-3R triggers HPC/monocyte differentiation via PI3K and MAPK/ERK1/2 signaling (MacManiman et al., 2014; Crawford et al., 2018). UL7 has also been shown to promote angiogenesis (MacManiman et al., 2014) and alter cytokine production (Engel et al., 2011). The differences in UL7’s effect on monocytes vs. other cell types suggest its effects on signaling may vary from cell type to cell type. Deletion of UL7 from the virus results in loss of reactivation from latency in vitro and in humanized mice, further highlighting the functional role of UL7 during viral reactivation (Crawford et al., 2018; Hancock et al., 2020a). Finally, it was recently published that HCMV utilizes secreted protein pUL7, miR-US5-1, and miR-UL112-3p to reduce the proapoptotic transcription factor FOXO3a, which in turn reduces expression of proapoptotic gene BCL2L11 and prevents virus-induced apoptosis after infection of CD34+ HPCs (Hancock et al., 2021).
US28, one of the 4 HCMV encoded G-protein coupled receptors (GPCRs), has been documented to signal through a number of pathways and this chemokine receptor has a number of biological functions (Streblow et al., 1999; Miller et al., 2003, 2012; Vischer et al., 2006; Elder and Sinclair, 2019; Elder et al., 2019). US28 binds to both members of the CC and CX3C chemokine families making it unique among GPCRs; and furthermore, the receptor elicits ligand-specific signaling and functions that are also cell type specific (Vomaske et al., 2009). US28 can also signal in a ligand-dependent and -independent manner (Streblow et al., 2003; Miller et al., 2012). US28 has been shown to alter smooth muscle cell migration (Streblow et al., 1999) and to be important in vascular disease, as well as play a role during latency where US28 is argued to be key in both maintenance of and reactivation from latency (Crawford et al., 2019). US28 is expressed in naturally infected human peripheral blood cells during periods of latency (Krishna et al., 2017), reactivation in lung transplant recipients (Boomker et al., 2006), and in the model of HCMV latency using CD34+ HPCs, monocytes, and in monocyte-derived macrophages during active infection (Zipeto et al., 1999; Beisser et al., 2001; Goodrum et al., 2002; Cheung et al., 2006; Humby and O’Connor, 2015; Crawford et al., 2019). Overall, US28 regulates host cell signaling, which in many cases is through changes in PI3K/Akt and MAPK signaling and alterations in EGFR and downstream processes. Together these varied mechanisms briefly discussed along with others not discussed (Fields et al., 1996, 2013; Fields and Knipe, 2013) demonstrate the complex mechanisms controlling HCMV reactivation from latency and the intimate linking of these various viral gene products in controlling the cellular signaling required for reactivation.
The HCMV has evolved a complicated strategy to disrupt biological pathways in infected host cells. The understanding of the relationship between viral infection and cellular signaling helps provide clues for how viral proteins and other regulators (whether they control primary infection or latency) hijack host cellular signaling pathways and control the pathways to the virus’ advantage. Deciphering the impact of cellular receptor engagement during viral entry and the corresponding exploitation of downstream host cellular signaling is vital to understanding viral dissemination and life-long viral persistence in individual hosts and in the human population in general. HCMV gB and gH complexes are critical for initiating these events through their binding to cognate host cell receptors during primary infection, while a number of viral proteins and miRNAs are critical to controlling the signaling associated with maintenance and reactivation from latency. Because each signaling event in each cell type is likely different, it is important to study the multiple cell types and how each event dictates the unique biology and infection characteristics within each cell. We argue this last point is evident during infection of monocytes and CD34+ HPCs, two critical cell types required for persistence and dissemination, where data emphasizes that what is seen in other cell types such as fibroblasts and epithelial and endothelial cells is not seen during infection of these two cell types and vice versa. Overall, we believe that by defining the HCMV signaling pathway activated during infection, it will be possible to develop new treatment options for viral diseases.
All authors listed have made a substantial, direct and intellectual contribution to the work, and approved it for publication.
AY was supported by grants from the National Institutes of Health (NIH) (AI056077, AI127335, P30-GM110703, P20-GM121307, P20-GM121288, and P20-GM134974). FG was supported by grants from NIH (AI079059 and AI127335). PC was supported by a grant from NIH (AI127335). MH was supported by a grant from NIH (AI127335). DS was supported by grants from the NIH (AI127335 and AI116633).
The authors declare that the research was conducted in the absence of any commercial or financial relationships that could be construed as a potential conflict of interest.
Adams, J. M., and Cory, S. (1998). The Bcl-2 protein family: arbiters of cell survival. Science 281, 1322–1326. doi: 10.1126/science.281.5381.1322
Akira, S., Uematsu, S., and Takeuchi, O. (2006). Pathogen recognition and innate immunity. Cell 124, 783–801. doi: 10.1016/j.cell.2006.02.015
Akter, P., Cunningham, C., McSharry, B. P., Dolan, A., Addison, C., Dargan, D. J., et al. (2003). Two novel spliced genes in human cytomegalovirus. J. Gen. Virol. 84, 1117–1122. doi: 10.1099/vir.0.18952-0
Andreoni, K. A., Wang, X., Huang, S. M., and Huang, E. S. (2002). Human cytomegalovirus hyperimmune globulin not only neutralizes HCMV infectivity, but also inhibits HCMV-induced intracellular NF-kappaB, Sp1, and PI3-K signaling pathways. J. Med. Virol. 67, 33–40. doi: 10.1002/jmv.2189
Aoki, T., and Narumiya, S. (2012). Prostaglandins and chronic inflammation. Trends Pharmacol. Sci. 33, 304–311. doi: 10.1016/j.tips.2012.02.004
Arvin, A. M. (2007). Human Herpesviruses : Biology, Therapy, and Immunoprophylaxis. Cambridge: Cambridge University Press.
Beaudin, A. E., Boyer, S. W., and Forsberg, E. C. (2014). Flk2/Flt3 promotes both myeloid and lymphoid development by expanding non-self-renewing multipotent hematopoietic progenitor cells. Exp. Hematol. 42, 218–229.e4.
Beisser, P. S., Laurent, L., Virelizier, J. L., and Michelson, S. (2001). Human cytomegalovirus chemokine receptor gene US28 is transcribed in latently infected THP-1 monocytes. J. Virol. 75, 5949–5957. doi: 10.1128/jvi.75.13.5949-5957.2001
Bentz, G. L., Jarquin-Pardo, M., Chan, G., Smith, M. S., Sinzger, C., and Yurochko, A. D. (2006). Human cytomegalovirus (HCMV) infection of endothelial cells promotes naive monocyte extravasation and transfer of productive virus to enhance hematogenous dissemination of HCMV. J. Virol. 80, 11539–11555. doi: 10.1128/JVI.01016-06
Bentz, G. L., and Yurochko, A. D. (2008). Human CMV infection of endothelial cells induces an angiogenic response through viral binding to EGF receptor and beta1 and beta3 integrins. Proc. Natl. Acad. Sci. U.S.A. 105, 5531–5536. doi: 10.1073/pnas.0800037105
Blank, U., and Karlsson, S. (2015). TGF-beta signaling in the control of hematopoietic stem cells. Blood 125, 3542–3550. doi: 10.1182/blood-2014-12-618090
Boehme, K. W., Guerrero, M., and Compton, T. (2006). Human cytomegalovirus envelope glycoproteins B and H are necessary for TLR2 activation in permissive cells. J. Immunol. 177, 7094–7102. doi: 10.4049/jimmunol.177.10.7094
Boomker, J. M., Verschuuren, E. A., Brinker, M. G., de Leij, L. F., The, T. H., and Harmsen, M. C. (2006). Kinetics of US28 gene expression during active human cytomegalovirus infection in lung-transplant recipients. J. Infect. Dis. 193, 1552–1556. doi: 10.1086/503779
Boppana, S. B., Ross, S. A., Shimamura, M., Palmer, A. L., Ahmed, A., Michaels, M. G., et al. (2011). Saliva polymerase-chain-reaction assay for cytomegalovirus screening in newborns. N. Engl. J. Med. 364, 2111–2118. doi: 10.1056/nejmoa1006561
Boyle, K. A., and Compton, T. (1998). Receptor-binding properties of a soluble form of human cytomegalovirus glycoprotein B. J. Virol. 72, 1826–1833. doi: 10.1128/jvi.72.3.1826-1833.1998
Britt, W. J., and Auger, D. (1986). Synthesis and processing of the envelope gp55-116 complex of human cytomegalovirus. J. Virol. 58, 185–191. doi: 10.1128/jvi.58.1.185-191.1986
Buck, A. H., Santoyo-Lopez, J., Robertson, K. A., Kumar, D. S., Reczko, M., and Ghazal, P. (2007). Discrete clusters of virus-encoded micrornas are associated with complementary strands of the genome and the 7.2-kilobase stable intron in murine cytomegalovirus. J. Virol. 81, 13761–13770. doi: 10.1128/jvi.01290-07
Buehler, J., Carpenter, E., Zeltzer, S., Igarashi, S., Rak, M., Mikell, I., et al. (2019). Host signaling and EGR1 transcriptional control of human cytomegalovirus replication and latency. PLoS Pathog. 15:e1008037. doi: 10.1371/journal.ppat.1008037
Buehler, J., Zeltzer, S., Reitsma, J., Petrucelli, A., Umashankar, M., Rak, M., et al. (2016). Opposing regulation of the EGF receptor: a molecular switch controlling cytomegalovirus latency and replication. PLoS Pathog. 12:e1005655. doi: 10.1371/journal.ppat.1005655
Bughio, F., Elliott, D. A., and Goodrum, F. (2013). An endothelial cell-specific requirement for the UL133-UL138 locus of human cytomegalovirus for efficient virus maturation. J. Virol. 87, 3062–3075. doi: 10.1128/jvi.02510-12
Bughio, F., Umashankar, M., Wilson, J., and Goodrum, F. (2015). Human cytomegalovirus UL135 and UL136 genes are required for postentry tropism in endothelial cells. J. Virol. 89, 6536–6550. doi: 10.1128/jvi.00284-15
Caló, S., Cortese, M., Ciferri, C., Bruno, L., Gerrein, R., Benucci, B., et al. (2016). The human cytomegalovirus UL116 gene encodes an envelope glycoprotein forming a complex with gH independently from gL. J. Virol. 90, 4926–4938. doi: 10.1128/JVI.02517-15
Campadelli-Fiume, G., Collins-McMillen, D., Gianni, T., and Yurochko, A. D. (2016). Integrins as herpesvirus receptors and mediators of the host signalosome. Annu. Rev. Virol. 3, 215–236. doi: 10.1146/annurev-virology-110615-035618
Capron, C., Lacout, C., Lecluse, Y., Jalbert, V., Chagraoui, H., Charrier, S., et al. (2010). A major role of TGF-beta1 in the homing capacities of murine hematopoietic stem cell/progenitors. Blood 116, 1244–1253. doi: 10.1182/blood-2009-05-221093
Chan, G., Bivins-Smith, E. R., Smith, M. S., Smith, P. M., and Yurochko, A. D. (2008a). Transcriptome analysis reveals human cytomegalovirus reprograms monocyte differentiation toward an M1 macrophage. J. Immunol. 181, 698–711. doi: 10.4049/jimmunol.181.1.698
Chan, G., Bivins-Smith, E. R., Smith, M. S., and Yurochko, A. D. (2008b). Transcriptome analysis of NF- B- and phosphatidylinositol 3-kinase-regulated genes in human cytomegalovirus-infected monocytes. J. Virol. 82, 1040–1046. doi: 10.1128/JVI.00864-07
Chan, G., Bivins-Smith, E. R., Smith, M. S., and Yurochko, A. D. (2009a). NF-κB and phosphatidylinositol 3-kinase activity mediates the HCMV-induced atypical M1/M2 polarization of monocytes. Virus Res. 144, 329–333. doi: 10.1016/j.virusres.2009.04.026
Chan, G., Nogalski, M. T., Bentz, G. L., Smith, M. S., Parmater, A., and Yurochko, A. D. (2010). PI3K-dependent upregulation of Mcl-1 by human cytomegalovirus is mediated by epidermal growth factor receptor and inhibits Apoptosis in short-lived monocytes. J. Immunol. 184, 3213–3222. doi: 10.4049/jimmunol.0903025
Chan, G., Nogalski, M. T., Stevenson, E. V., and Yurochko, A. D. (2012a). Human cytomegalovirus induction of a unique signalsome during viral entry into monocytes mediates distinct functional changes: a strategy for viral dissemination. J. Leukocyte Biol. 92, 743–752. doi: 10.1189/jlb.0112040
Chan, G., Nogalski, M. T., and Yurochko, A. D. (2009b). Activation of EGFR on monocytes is required for human cytomegalovirus entry and mediates cellular motility. Proc. Natl. Acad. Sci. U.S.A. 106, 22369–22374. doi: 10.1073/pnas.0908787106
Chan, G., Nogalski, M. T., and Yurochko, A. D. (2012b). Human cytomegalovirus stimulates monocyte-to-macrophage differentiation via the temporal regulation of caspase 3. J. Virol. 86, 10714–10723. doi: 10.1128/JVI.07129-11
Chan, G. C., and Yurochko, A. D. (2014). Analysis of cytomegalovirus binding/entry-mediated events. Methods Mol. Biol. 1119, 113–121. doi: 10.1007/978-1-62703-788-4_8
Chandramouli, S., Malito, E., Nguyen, T., Luisi, K., Donnarumma, D., Xing, Y., et al. (2017). Structural basis for potent antibody-mediated neutralization of human cytomegalovirus. Sci. Immunol. 2:eaan1457. doi: 10.1126/sciimmunol.aan1457
Cheung, A. K., Abendroth, A., Cunningham, A. L., and Slobedman, B. (2006). Viral gene expression during the establishment of human cytomegalovirus latent infection in myeloid progenitor cells. Blood 108, 3691–3699. doi: 10.1182/blood-2005-12-026682
Ciferri, C., Chandramouli, S., Donnarumma, D., Nikitin, P. A., Cianfrocco, M. A., Gerrein, R., et al. (2015). Structural and biochemical studies of HCMV gH/gL/gO and Pentamer reveal mutually exclusive cell entry complexes. Proc. Natl. Acad. Sci. U.S.A. 112, 1767–1772. doi: 10.1073/pnas.1424818112
Collins-McMillen, D., Buehler, J., Peppenelli, M., and Goodrum, F. (2018a). Molecular determinants and the regulation of human cytomegalovirus latency and reactivation. Viruses 10:444. doi: 10.3390/v10080444
Collins-McMillen, D., Chesnokova, L., Lee, B. J., Fulkerson, H. L., Brooks, R., Mosher, B. S., et al. (2018b). HCMV infection and apoptosis: how do monocytes survive HCMV infection? Viruses 10:533. doi: 10.3390/v10100533
Collins-McMillen, D., and Goodrum, F. D. (2017). The loss of binary: Pushing the herpesvirus latency paradigm. Curr. Clin. Microbiol. Rep. 4, 124–131. doi: 10.1007/s40588-017-0072-8
Collins-McMillen, D., Kim, J. H., Nogalski, M. T., Stevenson, E. V., Chan, G. C., Caskey, J. R., et al. (2016). Human cytomegalovirus promotes survival of infected monocytes via a distinct temporal regulation of cellular Bcl-2 family proteins. J. Virol. 90, 2356–2371. doi: 10.1128/JVI.01994-15
Collins-McMillen, D., Rak, M., Buehler, J. C., Igarashi-Hayes, S., Kamil, J. P., Moorman, N. J., et al. (2019). Alternative promoters drive human cytomegalovirus reactivation from latency. Proc. Natl. Acad. Sci. U.S.A. 116, 17492–17497. doi: 10.1073/pnas.1900783116
Collins-McMillen, D., Stevenson, E. V., Kim, J. H., Lee, B.-J., Cieply, S. J., Nogalski, M. T., et al. (2017a). HCMV utilizes a non-traditional STAT1 activation cascade via signaling through EGFR and integrins to efficiently promote the motility, differentiation, and polarization of infected monocytes. J. Virol. 91:e00622-17. doi: 10.1128/JVI.00622-17
Collins-McMillen, D., Stevenson, E. V., Kim, J. H., Lee, B. J., Cieply, S. J., Nogalski, M. T., et al. (2017b). Human cytomegalovirus utilizes a nontraditional signal transducer and activator of transcription 1 activation cascade via signaling through epidermal growth factor receptor and integrins to efficiently promote the motility, differentiation, and polarization of infected monocytes. J. Virol. 91:e00622-17.
Compton, T. (2000). Analysis of cytomegalovirus ligands, receptors, and the entry pathway. Methods Mol. Med. 33, 53–65. doi: 10.1385/1-59259-244-9:53
Compton, T., Kurt-Jones, E. A., Boehme, K. W., Belko, J., Latz, E., Golenbock, D. T., et al. (2003). Human cytomegalovirus activates inflammatory cytokine responses via CD14 and Toll-like receptor 2. J. Virol. 77, 4588–4596. doi: 10.1128/jvi.77.8.4588-4596.2003
Compton, T., Nepomuceno, R. R., and Nowlin, D. M. (1992). Human cytomegalovirus penetrates host cells by pH-independent fusion at the cell surface. Virology 191, 387–395. doi: 10.1016/0042-6822(92)90200-9
Crawford, L. B., Caposio, P., Kreklywich, C., Pham, A. H., Hancock, M. H., Jones, T. A., et al. (2019). Human cytomegalovirus US28 ligand binding activity is required for latency in CD34(+) hematopoietic progenitor cells and humanized NSG mice. mBio 10:e01889-19.
Crawford, L. B., Kim, J. H., Collins-McMillen, D., Lee, B. J., Landais, I., Held, C., et al. (2018). Human cytomegalovirus encodes a novel FLT3 receptor ligand necessary for hematopoietic cell differentiation and viral reactivation. mBio 9:e00682-18.
Dolken, L., Perot, J., Cognat, V., Alioua, A., John, M., Soutschek, J., et al. (2007). Mouse cytomegalovirus microRNAs dominate the cellular small RNA profile during lytic infection and show features of posttranscriptional regulation. J. Virol. 81, 13771–13782. doi: 10.1128/jvi.01313-07
Dunn, W., Trang, P., Zhong, Q., Yang, E., van Belle, C., and Liu, F. (2005). Human cytomegalovirus expresses novel microRNAs during productive viral infection. Cell Microbiol. 7, 1684–1695. doi: 10.1111/j.1462-5822.2005.00598.x
Eberhardt, M. K., Deshpande, A., Fike, J., Short, R., Schmidt, K. A., Blozis, S. A., et al. (2016). Exploitation of interleukin-10 (IL-10) signaling pathways: alternate roles of viral and cellular IL-10 in rhesus cytomegalovirus infection. J. Virol. 90, 9920–9930. doi: 10.1128/jvi.00635-16
Elder, E., and Sinclair, J. (2019). HCMV latency: what regulates the regulators? Med. Microbiol. Immunol. 208, 431–438. doi: 10.1007/s00430-019-00581-1
Elder, E. G., Krishna, B. A., Williamson, J., Lim, E. Y., Poole, E., Sedikides, G. X., et al. (2019). Interferon-responsive genes are targeted during the establishment of human cytomegalovirus latency. mBio 10:e02574-19.
Engel, P., Perez-Carmona, N., Alba, M. M., Robertson, K., Ghazal, P., and Angulo, A. (2011). Human cytomegalovirus UL7, a homologue of the SLAM-family receptor CD229, impairs cytokine production. Immunol. Cell Biol. 89, 753–766. doi: 10.1038/icb.2011.55
Feire, A. L., Koss, H., and Compton, T. (2004). Cellular integrins function as entry receptors for human cytomegalovirus via a highly conserved disintegrin-like domain. Proc. Natl. Acad. Sci. U.S.A. 101, 15470–15475. doi: 10.1073/pnas.0406821101
Feire, A. L., Roy, R. M., Manley, K., and Compton, T. (2010). Theglycoprotein B disintegrin-like domain binds Beta 1 integrin tomediate cytomegalovirus entry. J. Virol. 84, 10026–10037. doi: 10.1128/JVI.00710-10
Fields, B. N., and Knipe, D. M. (2013). Fields Virology Volume 2, Vol. 2. Philadelphia, PA: Wolters Kluwer Health, Lippincott Williams & Wilkins. doi: 10.1128/jvi.00710-10
Fields, B. N., and Knipe, D. M. and Howley, P. M. (1996). FieldsVirology. Philadelphia, PA: Lippincott-Raven.
Fields, B. N., and Knipe, D. M. andHowley, P. M. (2013). FieldsVirology Volume 1, Vol. 1. Philadelphia, PA: Wolters Kluwer Health, Lippincott Williams & Wilkins.
Fries, B. C., Khaira, D., Pepe, M. S., and Torok-Storb, B. (1993). Declining lymphocyte counts following cytomegalovirus (CMV) infection are associated with fatal CMV disease in bone marrow transplant patients. Exp. Hematol. 21, 1387–1392.
Frugtniet, B., Jiang, W. G., and Martin, T. A. (2015). Role of the WASP and WAVE family proteins in breast cancer invasion and metastasis. Breast Cancer (Dove Med Press) 7, 99–109. doi: 10.2147/bctt.s59006
Fulkerson, H. L., Chesnokova, L. S., Kim, J. H., Mahmud, J., Frazier, L. E., Chan, G. C., et al. (2020). HCMV-induced signaling through gB-EGFR engagement is required for viral trafficking and nuclear translocation in primary human monocytes. Proc. Natl. Acad. Sci. U.S.A. 117, 19507–19516. doi: 10.1073/pnas.2003549117
Fulkerson, H. L., Nogalski, M. T., Collins-McMillen, D., and Yurochko, A. D. (2021). Overview of human cytomegalovirus pathogenesis. Methods Mol. Biol. 2244, 1–18. doi: 10.1007/978-1-0716-1111-1_1
Gatault, P., Jones, I. K. A., Meyer, C., Kreklywich, C., Alexander, T., Smith, P. P., et al. (2021). Rat and human cytomegalovirus ORF116 encodes a virion envelope glycoprotein required for infectivity. Virology 557, 23–33. doi: 10.1016/j.virol.2020.12.014
Gerna, G., Kabanova, A., and Lilleri, D. (2019). Human cytomegalovirus cell tropism and host cell receptors. Vaccines (Basel) 7:70. doi: 10.3390/vaccines7030070
Gerna, G., and Lilleri, D. (2020). Human cytomegalovirus congenital (cCMV) infection following primary and nonprimary maternal infection: perspectives of prevention through vaccine development. Vaccines (Basel) 8:194. doi: 10.3390/vaccines8020194
Gerna, G., Percivalle, E., Lilleri, D., Lozza, L., Fornara, C., Hahn, G., et al. (2005). Dendritic-cell infection by human cytomegalovirus is restricted to strains carrying functional UL131-128 genes and mediates efficient viral antigen presentation to CD8+T cells. J. Gen. Virol. 86(Pt 2), 275–284. doi: 10.1099/vir.0.80474-0
Goodrum, F. D., Jordan, C. T., High, K., and Shenk, T. (2002). Human cytomegalovirus gene expression during infection of primary hematopoietic progenitor cells: a model for latency. Proc. Natl. Acad. Sci. U.S.A. 99, 16255–16260. doi: 10.1073/pnas.252630899
Gottwein, E., and Cullen, B. R. (2008). Viral and cellular microRNAs as determinants of viral pathogenesis and immunity. Cell Host Microbe 3, 375–387. doi: 10.1016/j.chom.2008.05.002
Grey, F., Meyers, H., White, E. A., Spector, D. H., and Nelson, J. (2007). A human cytomegalovirus-encoded microRNA regulates expression of multiple viral genes involved in replication. PLoS Pathog. 3:e163. doi: 10.1371/journal.ppat.0030163
Hahn, G., Jores, R., and Mocarski, E. S. (1998). Cytomegalovirus remains latent in a common precursor of dendritic and myeloid cells. Proc. Natl. Acad. Sci. U.S.A. 95, 3937–3942. doi: 10.1073/pnas.95.7.3937
Hahn, G., Revello, M. G., Patrone, M., Percivalle, E., Campanini, G., Sarasini, A., et al. (2004). Humancytomegalovirus UL131-128 genes are indispensable for virus growthin endothelial cells and virus transfer to leukocytes. J. Virol. 78, 10023–10033. doi: 10.1128/JVI.78.18.10023-10033.2004
Hancock, M. H., Crawford, L. B., Perez, W., Struthers, H. M., Mitchell, J., and Caposio, P. (2021). Humancytomegalovirus UL7, miR-US5-1, and miR-UL112-3p inactivation ofFOXO3a protects CD34(+) hematopoietic progenitor cells from Apoptosis. mSphere 6:e00986-20. doi: 10.1128/mSphere.00986-20
Hancock, M. H., Crawford, L. B., Pham, A. H., Mitchell, J., Struthers, H. M., Yurochko, A. D., et al. (2020a). Humancytomegalovirus miRNAs regulate TGF-beta to mediate myelosuppressionwhile maintaining viral latency in CD34(+) hematopoietic progenitorcells. Cell Host Microbe 27 104–114.e4.
Hancock, M. H., Hook, L. M., Mitchell, J., and Nelson, J. A. (2017). Humancytomegalovirus MicroRNAs miR-US5-1 and miR-UL112-3p blockproinflammatory cytokine production in response toNF-kappaB-activating factors through direct downregulation ofIKKalpha and IKKbeta. mBio 8:e00109-17.
Hancock, M. H., Mitchell, J., Goodrum, F. D., and Nelson, J. A. (2020b). Human cytomegalovirus miR-US5-2 downregulation of GAB1 regulates cellular proliferation and UL138 expression through modulation of epidermal growth factor receptor signaling pathways. mSphere 5:e00582-20. doi: 10.1128/mSphere.00582-20
Hochdorfer, D., Florin, L., Sinzger, C., and Lieber, D. (2016). Tetraspanin CD151 promotes initial events in human cytomegalovirus infection. J. Virol. 90, 6430–6442. doi: 10.1128/JVI.00145-16
Hook, L. M., Grey, F., Grabski, R., Tirabassi, R., Doyle, T., Hancock, M., et al. (2014). Cytomegalovirus miRNAs target secretory pathway genes to facilitate formation of the virion assembly compartment and reduce cytokine secretion. Cell Host Microbe 15, 363–373. doi: 10.1016/j.chom.2014.02.004
Hsu, J. L., van den Boomen, D. J., Tomasec, P., Weekes, M. P., Antrobus, R., Stanton, R. J., et al. (2015). Plasma membrane profiling defines an expanded class of cell surface proteins selectively targeted for degradation by HCMV US2 in cooperation with UL141. PLoS Pathog. 11:e1004811. doi: 10.1371/journal.ppat.1004811
Humby, M. S., and O’Connor, C. M. (2015). Human cytomegalovirus US28 is important for latent infection of hematopoietic progenitor cells. J. Virol. 90, 2959–2970. doi: 10.1128/jvi.02507-15
Isaacson, M. K., and Compton, T. (2009). Human cytomegalovirus glycoprotein B is required for virus entry and cell-to-cell spread but not for virion attachment, assembly, or egress. J. Virol. 83, 3891–3903. doi: 10.1128/jvi.01251-08
Italiani, P., and Boraschi, D. (2014). From monocytes to M1/M2 macrophages: phenotypical vs. functional differentiation. Front. Immunol. 5:514.
Jarvis, M. A., and Nelson, J. A. (2002). Human cytomegalovirus persistence and latency in endothelial cells and macrophages. Curr. Opin. Microbiol. 5, 403–407. doi: 10.1016/S1369-5274(02)00334-X
Kari, B., and Gehrz, R. (1992). A human cytomegalovirus glycoprotein complex designated gC-II is a major heparin-binding component of the envelope. J. Virol. 66, 1761–1764. doi: 10.1597/04-196R.1
Kim, J. H., Collins-McMillen, D., Buehler, J. C., Goodrum, F. D., and Yurochko, A. D. (2017). Human cytomegalovirus requires epidermal growth factor receptor signaling to enter and initiate the early steps in the establishment of latency in CD34 + human progenitor cells. J. Virol. 78, 4444–4453. doi: 10.1128/JVI.01206-16
Kim, J. H., Collins-McMillen, D., Caposio, P., and Yurochko, A. D. (2016). Viral binding-induced signaling drives a unique and extended intracellular trafficking pattern during infection of primary monocytes. Proc. Natl. Acad. Sci. U.S.A. 113, 8819–8824. doi: 10.1073/pnas.1604317113
Krishna, B. A., Spiess, K., Poole, E. L., Lau, B., Voigt, S., Kledal, T. N., et al. (2017). Targeting the latent cytomegalovirus reservoir with an antiviral fusion toxin protein. Nat. Commun. 8:14321.
Kurisu, S., and Takenawa, T. (2010). WASP and WAVE family proteins: friends or foes in cancer invasion? Cancer Sci. 101, 2093–2104. doi: 10.1111/j.1349-7006.2010.01654.x
Lagasse, E., and Weissman, I. L. (1997). Enforced expression of Bcl-2 in monocytes rescues macrophages and partially reverses osteopetrosis in op/op mice. Cell 89, 1021–1031. doi: 10.1016/s0092-8674(00)80290-1
LaMarca, H. L., Nelson, A. B., Scandurro, A. B., Whitley, G. S., and Morris, C. A. (2006). Human cytomegalovirus-induced inhibition of cytotrophoblast invasion in a first trimester extravillous cytotrophoblast cell line. Placenta 27, 137–147. doi: 10.1016/j.placenta.2005.03.003
Landais, I., Pelton, C., Streblow, D., DeFilippis, V., McWeeney, S., and Nelson, J. A. (2015). Human cytomegalovirus miR-UL112-3p targets TLR2 and modulates the TLR2/IRAK1/NFkappaB signaling pathway. PLoS Pathog. 11:e1004881. doi: 10.1371/journal.ppat.1004881
Lau, B., Poole, E., Van Damme, E., Bunkens, L., Sowash, M., King, H., et al. (2016). Human cytomegalovirus miR-UL112-1 promotes the down-regulation of viral immediate early-gene expression during latency to prevent T-cell recognition of latently infected cells. J. Gen. Virol. 97, 2387–2398. doi: 10.1099/jgv.0.000546
Lawrence, T., and Natoli, G. (2011). Transcriptional regulation of macrophage polarization: enabling diversity with identity. Nat. Rev. Immunol. 11, 750–761. doi: 10.1038/nri3088
Lee, P. P., Lobato-Marquez, D., Pramanik, N., Sirianni, A., Daza-Cajigal, V., Rivers, E., et al. (2017). Wiskott-Aldrich syndrome protein regulates autophagy and inflammasome activity in innate immune cells. Nat. Commun. 8:1576.
Lin, E. Y., Orlofsky, A., Wang, H. G., Reed, J. C., and Prystowsky, M. B. (1996). A1, a Bcl-2 family member, prolongs cell survival and permits myeloid differentiation. Blood 87, 983–992. doi: 10.1182/blood.v87.3.983.bloodjournal873983
Liu, H., Perlman, H., Pagliari, L. J., and Pope, R. M. (2001). Constitutively activated Akt-1 is vital for the survival of human monocyte-differentiated macrophages. Role of Mcl-1, independent of nuclear factor (NF)-kappaB, Bad, or caspase activation. J. Exp. Med. 194, 113–126. doi: 10.1084/jem.194.2.113
Liu, J., Jardetzky, T. S., Chin, A. L., Johnson, D. C., and Vanarsdall, A. L. (2018). The human cytomegalovirus trimer and pentamer promote sequential steps in entry into epithelial and endothelial cells at cell surfaces and endosomes. J. Virol. 92:e01336-18.
Ljungman, P., Hakki, M., and Boeckh, M. (2011). Cytomegalovirus in hematopoietic stem cell transplant recipients. Hematol. Oncol. Clin. North Am. 25, 151–169.
Mach, M., Kropff, B., Kryzaniak, M., and Britt, W. (2005). Complex formation by glycoproteins M and N of human cytomegalovirus: structural and functional aspects. J. Virol. 79, 2160–2170. doi: 10.1128/JVI.79.4.2160
MacManiman, J. D., Meuser, A., Botto, S., Smith, P. P., Liu, F., Jarvis, M. A., et al. (2014). Human cytomegalovirus-encoded pUL7 is a novel CEACAM1-like molecule responsible for promotion of angiogenesis. mBio 5:e02035.
Mantovani, A., Sica, A., Sozzani, S., Allavena, P., Vecchi, A., and Locati, M. (2004). The chemokine system in diverse forms of macrophage activation and polarization. Trends Immunol. 25, 677–686. doi: 10.1016/j.it.2004.09.015
Marshall, C. J., Kinnon, C., and Thrasher, A. J. (2000). Polarized expression of bone morphogenetic protein-4 in the human aorta-gonad-mesonephros region. Blood 96, 1591–1593. doi: 10.1182/blood.v96.4.1591.h8001591_1591_1593
Martinez-Martin, N., Marcandalli, J., Huang, C. S., Arthur, C. P., Perotti, M., Foglierini, M., et al. (2018). An unbiased screen for human cytomegalovirus identifies neuropilin-2 as a central viral receptor. Cell 174, 1158–1171.e19.
Mason, G. M., Poole, E., Sissons, J. G., Wills, M. R., and Sinclair, J. H. (2012). Human cytomegalovirus latency alters the cellular secretome, inducing cluster of differentiation (CD)4+ T-cell migration and suppression of effector function. Proc. Natl. Acad. Sci. U.S.A. 109, 14538–14543. doi: 10.1073/pnas.1204836109
Mendelson, M., Monard, S., Sissons, P., and Sinclair, J. (1996). Detection of endogenous human cytomegalovirus in CD34+ bone marrow progenitors. J. Gen. Virol. 77(Pt 12), 3099–3102. doi: 10.1099/0022-1317-77-12-3099
Mikell, I., Crawford, L. B., Hancock, M. H., Mitchell, J., Buehler, J., Goodrum, F., et al. (2019). HCMV miR-US22 down-regulation of EGR-1 regulates CD34+ hematopoietic progenitor cell proliferation and viral reactivation. PLoS Pathog. 15:e1007854. doi: 10.1371/journal.ppat.1007854
Miller, W. E., Houtz, D. A., Nelson, C. D., Kolattukudy, P. E., and Lefkowitz, R. J. (2003). G-protein-coupled receptor (GPCR) kinase phosphorylation and beta-arrestin recruitment regulate the constitutive signaling activity of the human cytomegalovirus US28 GPCR. J. Biol. Chem. 278, 21663–21671. doi: 10.1074/jbc.m303219200
Miller, W. E., Zagorski, W. A., Brenneman, J. D., Avery, D., Miller, J. L., and O’Connor, C. M. (2012). US28 is a potent activator of phospholipase C during HCMV infection of clinically relevant target cells. PLoS One 7:e50524. doi: 10.1371/journal.pone.0050524
Min, C. K., Shakya, A. K., Lee, B. J., Streblow, D. N., Caposio, P., and Yurochko, A. D. (2020). The differentiation of human cytomegalovirus infected-monocytes is required for viral replication. Front. Cell Infect. Microbiol. 10:368.
Murphy, E., Vanicek, J., Robins, H., Shenk, T., and Levine, A. J. (2008). Suppression of immediate-early viral gene expression by herpesvirus-coded microRNAs: implications for latency. Proc. Natl. Acad. Sci. U.S.A. 105, 5453–5458. doi: 10.1073/pnas.0711910105
Murray, P. J., and Wynn, T. A. (2011). Protective and pathogenic functions of macrophage subsets. Nat. Rev. Immunol. 11, 723–737. doi: 10.1038/nri3073
Navarro, D., Paz, P., Tugizov, S., Topp, K., La Vail, J., and Pereira, L. (1993). Glycoprotein B of human cytomegalovirus promotes virion penetration into cells, transmission of infection from cell to cell, and fusion of infected cells. Virology 197, 143–158. doi: 10.1006/viro.1993.1575
Nguyen, C. C., and Kamil, J. P. (2018). Pathogen at the gates: human cytomegalovirus entry and cell tropism. Viruses 10:704. doi: 10.3390/v10120704
Nguyen, C. C., Siddiquey, M. N. A., Zhang, H., Li, G., and Kamil, J. P. (2018). Human cytomegalovirus tropism modulator UL148 interacts with SEL1L, a cellular factor that governs endoplasmic reticulum-associated degradation of the viral envelope glycoprotein gO. J. Virol. 92:e00688-18.
Nishimura, M., and Mori, Y. (2019). Entry of betaherpesviruses. Adv. Virus Res. 104, 283–312. doi: 10.1016/bs.aivir.2019.05.005
Nogalski, M. T., Chan, G., Stevenson, E. V., Gray, S., and Yurochko, A. D. (2011). Human cytomegalovirus-regulated paxillin in monocytes links cellular pathogenic motility to the process of viral entry. J. Virol. 85, 1360–1369. doi: 10.1128/jvi.02090-10
Nogalski, M. T., Chan, G. C., Stevenson, E. V., and Collins-McMillen, D. K. and Yurochko, A. D. (2012). Aquantitative evaluation of cell migration by the phagokinetic track motility assay. J. Vis. Exp. 70:e4165. doi: 10.3791/4165
Nogalski, M. T., Chan, G. C., Stevenson, E. V., Collins-McMillen, D. K., and Yurochko, A. D. (2013). The HCMV gH/gL/UL128-131 complex triggers the specific cellular activation required for efficient viral internalization into target monocytes. PLoS Pathog. 9:e1003463. doi: 10.1371/journal.ppat.1003463
Nogalski, M. T., Collins-McMillen, D., and Yurochko, A. D. (2014). Overview of human cytomegalovirus pathogenesis. Methods Mol. Biol. 1119, 15–28. doi: 10.1007/978-1-62703-788-4_2
Nogalski, M. T., Podduturi, J. P., DeMeritt, I. B., Milford, L. E., and Yurochko, A. D. (2007). The human cytomegalovirus virion possesses an activated casein kinase II that allows for the rapid phosphorylation of the inhibitor of NF-kappaB, IkappaBalpha. J. Virol. 81, 5305–5314. doi: 10.1128/jvi.02382-06
Nowlin, D. M., Cooper, N. R., and Compton, T. (1991). Expression of a human cytomegalovirus receptor correlates with infectibility of cells. J. Virol. 65, 3114–3121. doi: 10.1128/jvi.65.6.3114-3121.1991
Pass, R. F., Zhang, C., Evans, A., Simpson, T., Andrews, W., Huang, M. L., et al. (2009). Vaccine prevention of maternal cytomegalovirus infection. N. Engl. J. Med. 360, 1191–1199.
Perez-Carmona, N., Martinez-Vicente, P., Farre, D., Gabaev, I., Messerle, M., Engel, P., et al. (2018). A prominent role of the human cytomegalovirus UL8 glycoprotein in restraining proinflammatory cytokine production by myeloid cells at late times during infection. J. Virol. 92:e02229-17.
Perlman, H., Georganas, C., Pagliari, L. J., Koch, A. E., Haines, K. III, and Pope, R. M. (2000). Bcl-2 expression in synovial fibroblasts is essential for maintaining mitochondrial homeostasis and cell viability. J. Immunol. 164, 5227–5235. doi: 10.4049/jimmunol.164.10.5227
Petrucelli, A., Rak, M., Grainger, L., and Goodrum, F. (2009). Characterization of a novel Golgi apparatus-localized latency determinant encoded by human cytomegalovirus. J. Virol. 83, 5615–5629. doi: 10.1128/jvi.01989-08
Petrucelli, A., Umashankar, M., Zagallo, P., Rak, M., and Goodrum, F. (2012). Interactions between proteins encoded within the human cytomegalovirus UL133-UL138 locus. J. Virol. 86, 8653–8662. doi: 10.1128/jvi.00465-12
Pfeffer, S., Sewer, A., Lagos-Quintana, M., Sheridan, R., Sander, C., Grasser, F. A., et al. (2005). Identification of microRNAs of the herpesvirus family. Nat. Methods 2, 269–276.
Pignatelli, S., Dal Monte, P., Rossini, G., Chou, S., Gojobori, T., Hanada, K., et al. (2003). Human cytomegalovirus glycoprotein N (gpUL73-gN) genomic variants: identification of a novel subgroup, geographical distribution and evidence of positive selective pressure. J. Gen. Virol. 84(Pt 3), 647–655. doi: 10.1099/vir.0.18704-0
Rak, M. A., Buehler, J., Zeltzer, S., Reitsma, J., Molina, B., Terhune, S., et al. (2018). Human cytomegalovirus UL135 interacts with host adaptor proteins to regulate epidermal growth factor receptor and reactivation from latency. J. Virol. 92:e00919-18.
Reeves, M. B., Breidenstein, A., and Compton, T. (2012). Human cytomegalovirus activation of ERK and myeloid cell leukemia-1 protein correlates with survival of latently infected cells. Proc. Natl. Acad. Sci. U.S.A. 109, 588–593. doi: 10.1073/pnas.1114966108
Ricciotti, E., and Fitzgerald, G. A. (2011). Prostaglandins and inflammation. Arteriosclerosis Thromb. Vasc. Biol. 31, 986–1000. doi: 10.1161/ATVBAHA.110.207449
Ryckman, B. J., Rainish, B. L., Chase, M. C., Borton, J. A., Nelson, J. A., Jarvis, M. A., et al. (2008). Characterization of the human cytomegalovirus gH/gL/UL128-131 complex that mediates entry into epithelial and endothelial cells. J. Virol. 82, 60–70. doi: 10.1128/JVI.01910-07
Safdar, A., Papadopoulous, E. B., and Armstrong, D. (2002). Listeriosis in recipients of allogeneic blood and marrow transplantation: thirteen year review of disease characteristics, treatment outcomes and a new association with human cytomegalovirus infection. Bone Marrow Transplant 29, 913–916. doi: 10.1038/sj.bmt.1703562
Sanz, C., Benito, A., Silva, M., Albella, B., Richard, C., Segovia, J. C., et al. (1997). The expression of Bcl-x is downregulated during differentiation of human hematopoietic progenitor cells along the granulocyte but not the monocyte/macrophage lineage. Blood 89, 3199–3204. doi: 10.1182/blood.v89.9.3199
Shen, S., Wang, S., Britt, W. J., and Lu, S. (2007). DNA vaccines expressing glycoprotein complex II antigens gM and gN elicited neutralizing antibodies against multiple human cytomegalovirus (HCMV) isolates. Vaccine 25, 3319–3327. doi: 10.1016/j.vaccine.2007.01.011
Shimamura, M., Mach, M., and Britt, W. J. (2006). Human cytomegalovirus infection elicits a glycoprotein M (gM)/gN-specific virus-neutralizing antibody response. J. Virol. 80, 4591–4600. doi: 10.1128/jvi.80.9.4591-4600.2006
Shnayder, M., Nachshon, A., Rozman, B., Bernshtein, B., Lavi, M., Fein, N., et al. (2020). Single cell analysis reveals human cytomegalovirus drives latently infected cells towards an anergic-like monocyte state. eLife 9:e52168.
Sica, A., and Mantovani, A. (2012). Macrophage plasticity and polarization: in vivo veritas. J. Clin. Invest. 122, 787–795. doi: 10.1172/jci59643
Sinclair, J. (2008). Human cytomegalovirus: latency and reactivation in the myeloid lineage. J. Clin. Virol. 41, 180–185. doi: 10.1016/j.jcv.2007.11.014
Sing, G. K., Keller, J. R., Ellingsworth, L. R., and Ruscetti, F. W. (1988). Transforming growth factor beta selectively inhibits normal and leukemic human bone marrow cell growth in vitro. Blood 72, 1504–1511. doi: 10.1182/blood.v72.5.1504.bloodjournal7251504
Sinzger, C., Eberhardt, K., Cavignac, Y., Weinstock, C., Kessler, T., Jahn, G., et al. (2006). Macrophage cultures are susceptible to lytic productive infection by endothelial-cell-propagated human cytomegalovirus strains and present viral IE1 protein to CD4+ T cells despite late downregulation of MHC class II molecules. J. Gen. Virol. 87, 1853–1862. doi: 10.1099/vir.0.81595-0
Sinzger, C., Grefte, A., Plachter, B., Gouw, A. S., The, T. H., and Jahn, G. (1995). Fibroblasts, epithelial cells, endothelial cells and smooth muscle cells are major targets of human cytomegalovirus infection in lung and gastrointestinal tissues. J. Gen. Virol. 76(Pt 4), 741–750. doi: 10.1099/0022-1317-76-4-741
Smith, M. S., Bentz, G. L., Alexander, J. S., and Yurochko, A. D. (2004a). Human cytomegalovirus induces monocyte differentiation and migration as a strategy for dissemination and persistence. J. Virol. 78, 4444–4453. doi: 10.1128/JVI.78.9.4444-4453.2004
Smith, M. S., Bentz, G. L., Smith, P. M., Bivins, E. R., and Yurochko, A. D. (2004b). HCMV activates PI(3)K in monocytes and promotes monocyte motility and transendothelial migration in a PI(3)K-dependent manner. J. Leukocyte Biol. 76, 65–76. doi: 10.1189/jlb.1203621
Smith, M. S., Bivins-Smith, E. R., Tilley, A. M., Bentz, G. L., Chan, G., Minard, J., et al. (2007). Roles of phosphatidylinositol 3-kinase and NF-kappaB in human cytomegalovirus-mediated monocyte diapedesis and adhesion: strategy for viral persistence. J. Virol. 81, 7683–7694. doi: 10.1128/jvi.02839-06
Snapper, S. B., and Rosen, F. S. (1999). The Wiskott-Aldrich syndrome protein (WASP): roles in signaling and cytoskeletal organization. Annu. Rev. Immunol. 17, 905–929. doi: 10.1146/annurev.immunol.17.1.905
Soderberg-Naucler, C. (2008). HCMV microinfections in inflammatory diseases and cancer. J. Clin. Virol. 41, 218–223. doi: 10.1016/j.jcv.2007.11.009
Soroceanu, L., Akhavan, A., and Cobbs, C. S. (2008). Platelet-derived growth factor-α receptor activation is required for human cytomegalovirus infection. Nature 455, 391–395. doi: 10.1038/nature07209
Stagno, S., Pass, R. F., Cloud, G., Britt, W. J., Henderson, R. E., Walton, P. D., et al. (1986). Primary cytomegalovirus infection in pregnancy. Incidence, transmission to fetus, and clinical outcome. JAMA 256, 1904–1908. doi: 10.1001/jama.1986.03380140074025
Stark, T. J., Arnold, J. D., Spector, D. H., and Yeo, G. W. (2012). High-resolution profiling and analysis of viral and host small RNAs during human cytomegalovirus infection. J. Virol. 86, 226–235. doi: 10.1128/jvi.05903-11
Stegmann, C., Abdellatif, M. E. A., Laib Sampaio, K., Walther, P., and Sinzger, C. (2017a). Importance of highly conserved peptide sites of HCMV gO for the formation of the gH/gL/gO complex. J. Virol. 91:e01339-16. doi: 10.1128/JVI.01339-16
Stegmann, C., Hochdorfer, D., Lieber, D., Subramanian, N., Stöhr, D., Laib Sampaio, K., et al. (2017b). A derivative of platelet-derived growth factor receptor alpha binds to the trimer of human cytomegalovirus and inhibits entry into fibroblasts and endothelial cells. PLoS Pathog. 13:e1006273. doi: 10.1371/journal.ppat.1006273
Stevenson, E. V., Collins-McMillen, D., Kim, J. H., Cieply, S. J., Bentz, G. L., and Yurochko, A. D. (2014). HCMV reprogramming of infected monocyte survival and differentiation: a goldilocks phenomenon. Viruses 6, 782–807. doi: 10.3390/v6020782
Streblow, D. N., and Nelson, J. A. (2003). Models of HCMV latency and reactivation. Trends Microbiol. 11, 293–295. doi: 10.1016/s0966-842x(03)00149-5
Streblow, D. N., Soderberg-Naucler, C., Vieira, J., Smith, P., Wakabayashi, E., Ruchti, F., et al. (1999). The human cytomegalovirus chemokine receptor US28 mediates vascular smooth muscle cell migration. Cell 99, 511–520. doi: 10.1016/s0092-8674(00)81539-1
Streblow, D. N., Vomaske, J., Smith, P., Melnychuk, R., Hall, L., Pancheva, D., et al. (2003). Human cytomegalovirus chemokine receptor US28-induced smooth muscle cell migration is mediated by focal adhesion kinase and Src. J. Biol. Chem. 278, 50456–50465. doi: 10.1074/jbc.m307936200
Styczynski, J. (2018). Who is the patient at risk of CMV recurrence: a review of the current scientific evidence with a focus on hematopoietic cell transplantation. Infect Dis. Ther. 7, 1–16. doi: 10.1007/s40121-017-0180-z
Su, R. J., Zhang, X. B., Li, K., Yang, M., Li, C. K., Fok, T. F., et al. (2002). Platelet-derived growth factor promotes ex vivo expansion of CD34+ cells from human cord blood and enhances long-term culture-initiating cells, non-obese diabetic/severe combined immunodeficient repopulating cells and formation of adherent cells. Br. J. Haematol. 117, 735–746. doi: 10.1046/j.1365-2141.2002.03500.x
Taylor-Wiedeman, J., Sissons, J. G., Borysiewicz, L. K., and Sinclair, J. H. (1991). Monocytes are a major site of persistence of human cytomegalovirus in peripheral blood mononuclear cells. J. Gen. Virol. 72(Pt 9), 2059–2064. doi: 10.1099/0022-1317-72-9-2059
Tirabassi, R., Hook, L., Landais, I., Grey, F., Meyers, H., Hewitt, H., et al. (2011). Human cytomegalovirus US7 is regulated synergistically by two virally encoded microRNAs and by two distinct mechanisms. J. Virol. 85, 11938–11944. doi: 10.1128/jvi.05443-11
Tsuboi, S. (2006). A complex of Wiskott-Aldrich syndrome protein with mammalian verprolins plays an important role in monocyte chemotaxis. J. Immunol. 176, 6576–6585. doi: 10.4049/jimmunol.176.11.6576
Tugal, D., Liao, X., and Jain, M. K. (2013). Transcriptional control of macrophage polarization. Arterioscler. Thromb. Vasc. Biol. 33, 1135–1144. doi: 10.1161/atvbaha.113.301453
Tugizov, S., Navarro, D., Paz, P., Wang, Y., Qadri, I., and Pereira, L. (1994). Function of human cytomegalovirus glycoprotein B: syncytium formation in cells constitutively expressing gB is blocked by virus-neutralizing antibodies. Virology 201, 263–276. doi: 10.1006/viro.1994.1291
Umashankar, M., Petrucelli, A., Cicchini, L., Caposio, P., Kreklywich, C. N., Rak, M., et al. (2011). A novel human cytomegalovirus locus modulates cell type-specific outcomes of infection. PLoS Pathog. 7:e1002444. doi: 10.1371/journal.ppat.1002444
Umashankar, M., Rak, M., Bughio, F., Zagallo, P., Caviness, K., and Goodrum, F. D. (2014). Antagonistic determinants controlling replicative and latent states of human cytomegalovirus infection. J. Virol. 88, 5987–6002. doi: 10.1128/jvi.03506-13
van den Boomen, D. J., and Lehner, P. J. (2015). Identifying the ERAD ubiquitin E3 ligases for viral and cellular targeting of MHC class I. Mol. Immunol. 68, 106–111. doi: 10.1016/j.molimm.2015.07.005
Vanarsdall, A. L., Pritchard, S. R., Wisner, T. W., Liu, J., Jardetzky, T. S., and Johnson, D. C. (2018). CD147 promotes entry of pentamer-expressing human cytomegalovirus into epithelial and endothelial cells. mBio 9:e00781-18.
Vanarsdall, A. L., Ryckman, B. J., Chase, M. C., and Johnson, D. C. (2008). Human cytomegalovirus glycoproteins gB and gH/gL mediate epithelial cell-cell fusion when expressed either in cis or in trans. J. Virol. 82, 11837–11850. doi: 10.1128/jvi.01623-08
Vischer, H. F., Leurs, R., and Smit, M. J. (2006). HCMV-encoded G-protein-coupled receptors as constitutively active modulators of cellular signaling networks. Trends Pharmacol. Sci. 27, 56–63. doi: 10.1016/j.tips.2005.11.006
Vomaske, J., Melnychuk, R. M., Smith, P. P., Powell, J., Hall, L., DeFilippis, V., et al. (2009). Differential ligand binding to a human cytomegalovirus chemokine receptor determines cell type-specific motility. PLoS Pathog. 5:e1000304. doi: 10.1371/journal.ppat.1000304
Walker, F., Kato, A., Gonez, L. J., Hibbs, M. L., Pouliot, N., Levitzki, A., et al. (1998). Activation of the Ras/mitogen-activated protein kinase pathway by kinase-defective epidermal growth factor receptors results in cell survival but not proliferation. Mol. Cell. Biol. 18, 7192–7204. doi: 10.1128/MCB.18.12.7192
Wang, D., and Shenk, T. (2005b). Human cytomegalovirus virion protein complex required for epithelial and endothelial cell tropism. Proc. Natl. Acad. Sci. U.S.A. 102, 18153–18158. doi: 10.1073/pnas.0509201102
Wang, D., and Shenk, T. (2005a). Human cytomegalovirus UL131 open reading frame is required for epithelial cell tropism. J. Virol. 79, 10330–10338. doi: 10.1128/JVI.79.16.10330-10338.2005
Wang, H., Peng, G., Bai, J., He, B., Huang, K., Hu, X., et al. (2017). Cytomegalovirus infection and relative risk of cardiovascular disease (ischemic heart disease, stroke, and cardiovascular death): a meta-analysis of prospective studies up to 2016. J. Am. Heart Assoc. 6:e005025.
Wang, X., Huang, D. Y., Huong, S.-M., and Huang, E.-S. (2005). Integrin alphavbeta3 is a coreceptor for human cytomegalovirus. Nat. Med. 11, 515–521. doi: 10.1038/nm1236
Wang, X., Huong, S. M., Chiu, M. L., Raab-Traub, N., and Huang, E. S. (2003). Epidermal growth factor receptor is a cellular receptor for human cytomegalovirus. Nature 424, 456–461. doi: 10.1038/nature01818
Weed, D. J., and Nicola, A. V. (2017). Herpes simplex virus membrane fusion. Adv. Anat. Embryol. Cell Biol. 223, 29–47. doi: 10.1007/978-3-319-53168-7_2
Wieduwilt, M. J., and Moasser, M. M. (2008). The epidermal growth factor receptor family: biology driving targeted therapeutics. Cell Mol. Life Sci. 65, 1566–1584. doi: 10.1007/s00018-008-7440-8
Wille, P. T., Knoche, A. J., Nelson, J. A., Jarvis, M. A., and Johnson, D. C. (2010). A human cytomegalovirus gO-null mutant fails to incorporate gH/gL into the virion envelope and is unable to enter fibroblasts and epithelial and endothelial cells. J. Virol. 84, 2585–2596. doi: 10.1128/JVI.02249-09
Wu, K., Oberstein, A., Wang, W., and Shenk, T. (2018). Role of PDGF receptor-α during human cytomegalovirus entry into fibroblasts. Proc. Natl. Acad. Sci. U.S.A. 115, E9889–E9898. doi: 10.1073/pnas.1806305115
Wu, Y., Prager, A., Boos, S., Resch, M., Brizic, I., Mach, M., et al. (2017). Human cytomegalovirus glycoprotein complex gH/gL/gO uses PDGFR-alpha as a key for entry. PLoS Pathog. 13:e1006281. doi: 10.1371/journal.ppat.1006281
Xiaofei, E., Meraner, P., Lu, P., Perreira, J. M., Aker, A. M., McDougall, W. M., et al. (2019). OR14I1 is a receptor for the human cytomegalovirus pentameric complex and defines viral epithelial cell tropism. Proc. Natl. Acad. Sci. U.S.A. 116, 7043–7052. doi: 10.1073/pnas.1814850116
Yamada, K. M., and Miyamoto, S. (1995). Integrin transmembrane signaling and cytoskeletal control. Curr. Opin. Cell Biol. 7, 681–689. doi: 10.1016/0955-0674(95)80110-3
Yamaguchi, H., and Condeelis, J. (2007). Regulation of the actin cytoskeleton in cancer cell migration and invasion. Biochim. Biophys. Acta 1773, 642–652. doi: 10.1016/j.bbamcr.2006.07.001
Yang, T., Buchan, H. L., Townsend, K. J., and Craig, R. W. (1996). MCL-1, a member of the BLC-2 family, is induced rapidly in response to signals for cell differentiation or death, but not to signals for cell proliferation. J. Cell Physiol. 166, 523–536. doi: 10.1002/(sici)1097-4652(199603)166:3<523::aid-jcp7>3.0.co;2-r
Yurochko, A. D. (2008). Human cytomegalovirus modulation of signal transduction. Curr. Top. Microbiol. Immunol. 325, 205–220. doi: 10.1007/978-3-540-77349-8_12
Yurochko, A. D. (2017). New mechanism by which human cytomegalovirus microRNAs negate the proinflammatory response to infection. mBio 8:e00505-17. doi: 10.1128/mBio.00505-17
Yurochko, A. D., Burger, C. J., and Elgert, K. D. (1990). Tumor modulation of autoreactivity: decreased macrophage and autoreactive T cell interactions. Cell. Immunol. 127, 105–119. doi: 10.1016/0008-8749(90)90118-B
Yurochko, A. D., Haywood Pyle, R., and Elgert, K. D. (1989). Changes in macrophage populations: phenotypic differences between normal and tumor-bearing host macro phages. Immunobiology 178, 416–435. doi: 10.1016/S0171-2985(89)80063-4
Yurochko, A. D., and Huang, E. S. (2000). Immunological methods for the detection of human cytomegalovirus. Methods Mol. Med. 33, 1–19. doi: 10.1385/1-59259-244-9:1
Yurochko, A. D., Huang, E. S., Smith, M. S., Smith, P. M., and Yurochko, A. D. (1999). Human cytomegalovirus binding to human monocytes induces immunoregulatory gene expression. J. Immunol. 181, 698–711.
Yurochko, A. D., Hwang, E. S., Rasmussen, L., Keay, S., Pereira, L., and Huang, E. S. (1997a). The human cytomegalovirus UL55 (gB) and UL75 (gH) glycoprotein ligands initiate the rapid activation of Sp1 and NF-kappaB during infection. J. Virol. 71, 5051–5059. doi: 10.1128/jvi.71.7.5051-5059.1997
Yurochko, A. D., Kowalik, T. F., Huong, S. M., and Huang, E. S. (1995). Human cytomegalovirus upregulates NF-kappa B activity by transactivating the NF-kappa B p105/p50 and p65 promoters. J. Virol. 69, 5391–5400. doi: 10.1128/jvi.69.9.5391-5400.1995
Yurochko, A. D., Liu, D. Y., Eierman, D., and Haskill, S. (1992). Integrins as a primary signal transduction molecule regulating monocyte immediate-early gene induction. Proc. Natl. Acad. Sci. U.S.A. 89, 9034–9038. doi: 10.1073/pnas.89.19.9034
Yurochko, A. D., Mayo, M. W., Poma, E. E., Baldwin, A. S., and Huang, E.-S. (1997b). Induction of the transcription factor Sp1 during human cytomegalovirus infection mediates upregulation of the p65 and p105/p50 NF-kappaB promoters. J. Virol. 71, 4638–4648. doi: 10.1021/nl901373j
Zhang, J., Li, Y., Yu, M., Chen, B., and Shen, B. (2003). Lineage-dependent NF-kappaB activation contributes to the resistance of human macrophages to apoptosis. Hematol. J. 4, 277–284. doi: 10.1038/sj.thj.6200252
Zhang, Q., Lenardo, M. J., and Baltimore, D. (2017). 30 years of NF-κB: A blossoming of relevance to human pathobiology. Cell 168, 37–57. doi: 10.1016/j.cell.2016.12.012
Zhou, M., Lanchy, J.-M., and Ryckman, B. J. (2015). Human cytomegalovirus gH/gL/gO promotes the fusion step of entry into all cell types, whereas gH/gL/UL128-131 broadens virus tropism through a distinct mechanism. J. Virol. 89, 8999–9009. doi: 10.1128/JVI.01325-15
Zhou, P., Qian, L., Kozopas, K. M., and Craig, R. W. (1997). Mcl-1, a Bcl-2 family member, delays the death of hematopoietic cells under a variety of apoptosis-inducing conditions. Blood 89, 630–643. doi: 10.1182/blood.v89.2.630
Zhu, D., Pan, C., Sheng, J., Liang, H., Bian, Z., Liu, Y., et al. (2018). Human cytomegalovirus reprogrammes haematopoietic progenitor cells into immunosuppressive monocytes to achieve latency. Nat. Microbiol. 3, 503–513. doi: 10.1038/s41564-018-0131-9
Keywords: human cytomegalovirus (HCMV), monocytes, progenitor cells, epidermal growth factor receptor (EGFR), glycoproteins, cell signaling, differentiation, latency
Citation: Lee B-J, Min C-K, Hancock M, Streblow DN, Caposio P, Goodrum FD and Yurochko AD (2021) Human Cytomegalovirus Host Interactions: EGFR and Host Cell Signaling Is a Point of Convergence Between Viral Infection and Functional Changes in Infected Cells. Front. Microbiol. 12:660901. doi: 10.3389/fmicb.2021.660901
Received: 01 February 2021; Accepted: 07 April 2021;
Published: 07 May 2021.
Edited by:
Maria Isabel Colombo, Universidad Nacional de Cuyo, ArgentinaReviewed by:
John Sinclair, University of Cambridge, United KingdomCopyright © 2021 Lee, Min, Hancock, Streblow, Caposio, Goodrum and Yurochko. This is an open-access article distributed under the terms of the Creative Commons Attribution License (CC BY). The use, distribution or reproduction in other forums is permitted, provided the original author(s) and the copyright owner(s) are credited and that the original publication in this journal is cited, in accordance with accepted academic practice. No use, distribution or reproduction is permitted which does not comply with these terms.
*Correspondence: Andrew D. Yurochko, YXl1cm9jQGxzdWhzYy5lZHU=
Disclaimer: All claims expressed in this article are solely those of the authors and do not necessarily represent those of their affiliated organizations, or those of the publisher, the editors and the reviewers. Any product that may be evaluated in this article or claim that may be made by its manufacturer is not guaranteed or endorsed by the publisher.
Research integrity at Frontiers
Learn more about the work of our research integrity team to safeguard the quality of each article we publish.