- 1Institute for Medical Microbiology and Infection Control, University Hospital, Goethe University Frankfurt am Main, Frankfurt, Germany
- 2Faculty of Biological Sciences of the Goethe University Frankfurt am Main, Frankfurt, Germany
- 3University Center of Competence for Infection Control of the State of Hesse, Frankfurt, Germany
- 4Institute for Medical Microbiology, Immunology and Hygiene, German Center for Infection Research (DZIF Partner Site Cologne-Bonn), University Hospital of Cologne, Cologne, Germany
- 5Institute for Medical Microbiology, University Hospital of Oldenburg, Oldenburg, Germany
OXA-48-like carbapenemases are among the most frequent carbapenemases in Gram-negative Enterobacterales worldwide with the highest prevalence in the Middle East, North Africa and Europe. Here, we investigated the so far uncharacterized carbapenemase OXA-484 from a clinical E. coli isolate belonging to the high-risk clone ST410 regarding antibiotic resistance pattern, horizontal gene transfer (HGT) and genetic support. OXA-484 differs by the amino acid substitution 214G compared to the most closely related variants OXA-181 (214R) and OXA-232 (214S). The blaOXA–484 was carried on a self-transmissible 51.5 kb IncX3 plasmid (pOXA-484) showing high sequence similarity with plasmids harboring blaOXA–181. Intraspecies and intergenus HGT of pOXA-484 to different recipients occurred at low frequencies of 1.4 × 10–7 to 2.1 × 10–6. OXA-484 increased MICs of temocillin and carbapenems similar to OXA-232 and OXA-244, but lower compared with OXA-48 and OXA-181. Hence, OXA-484 combines properties of OXA-181-like plasmid support and transferability as well as β-lactamase activity of OXA-232.
Introduction
Carbapenem resistance in Enterobacterales has dramatically increased worldwide in recent years and poses a major threat to public health. Antibiotic treatment options for infections caused by carbapenem-resistant Enterobacterales (CPE) are severely limited, with often very few or even no antibiotic agents remaining effective. Resistance to carbapenems is primarily caused by carbapenemases, i.e., bacterial enzymes that hydrolyze carbapenems and most other β-lactam antibiotics. OXA-48 is one of the most prevalent carbapenemases worldwide with the highest prevalence in the Middle East, North Africa and Europe (Cantón et al., 2012). OXA-48 enzymes lead to carbapenem resistance and are most often found in Escherichia coli and Klebsiella pneumoniae (Pitout et al., 2019).
The rapid dissemination of OXA-48 has been attributed to efficient horizontal gene transfer (HGT) of plasmids harboring blaOXA–48 and a low fitness burden by carriage of these plasmids (Hamprecht et al., 2019). At least 35 variants of OXA-48, commonly referred to as OXA-48-like β-lactamases (e.g., OXA-181, OXA-232, OXA-244), have been reported (Naas et al., 2017). Even though these variants differ only by single or few amino acid substitutions, a high degree of diversity is observed regarding the β-lactam hydrolysis pattern, genetic support of OXA-48-like encoding genes and HGT (Potron et al., 2011, 2013). The gene encoding OXA-48, blaOXA–48, is mostly present on an epidemic 63.6 kb IncL plasmid and bracketed by two identical insertion sequences (IS), IS1999, forming the composite transposon Tn1999 (Poirel et al., 2012). In contrast, blaOXA–181 is primarily found on a 51.5 kb IncX3 plasmid and has been associated with ISEcp1 in a Tn2013 transposon structure, whereas blaOXA–232 is commonly located on a non-conjugative 6.1 kb ColE-type plasmid (Qin et al., 2018; Li et al., 2019). In case of blaOXA–244, the gene is mostly identified on the chromosome of E. coli and has been increasingly identified in the European Union in recent years (European Centre for Disease Prevention and Control, 2020; Kremer et al., 2020; Chudejova et al., 2021).
OXA-484, a variant of the OXA-48-like family, has been previously reported from a collection of OXA-48-like carbapenemase in the United Kingdom, but so far only the sequence is known (Findlay et al., 2017). Here we report on the genetic background, antibiotic resistance phenotype and HGT of this carbapenemase.
Materials and Methods
Bacterial Isolates and Antimicrobial Susceptibility Testing
Enterobacterales clinical isolates carrying the plasmid-borne OXA-48-like carbapenemases OXA-48, OXA-181, OXA-232, OXA-244, and OXA-484 were recovered from patients of the University Hospital Frankfurt, Germany (Table 1). All isolates were phenotypically characterized and tested for blaOXA–48–like by PCR and Sanger sequencing as previously described (Göttig et al., 2015). Antimicrobial susceptibility was determined using antibiotic gradient strips (Liofilchem, Roseto degli Abruzzi, Italy), broth micro dilution for colistin and agar dilution for fosfomycin as recommended by EUCAST. Minimal inhibitory concentrations (MIC) were interpreted according to EUCAST guidelines v10.0. For detection of carbapenemases, chromID® CARBA SMART plates (bioMérieux, Nürtingen, Germany) and the immunochromatographic CARBA 5 lateral flow test (NG-Biotech, Guipry, France) were used (Baeza et al., 2019; Göttig et al., 2020).
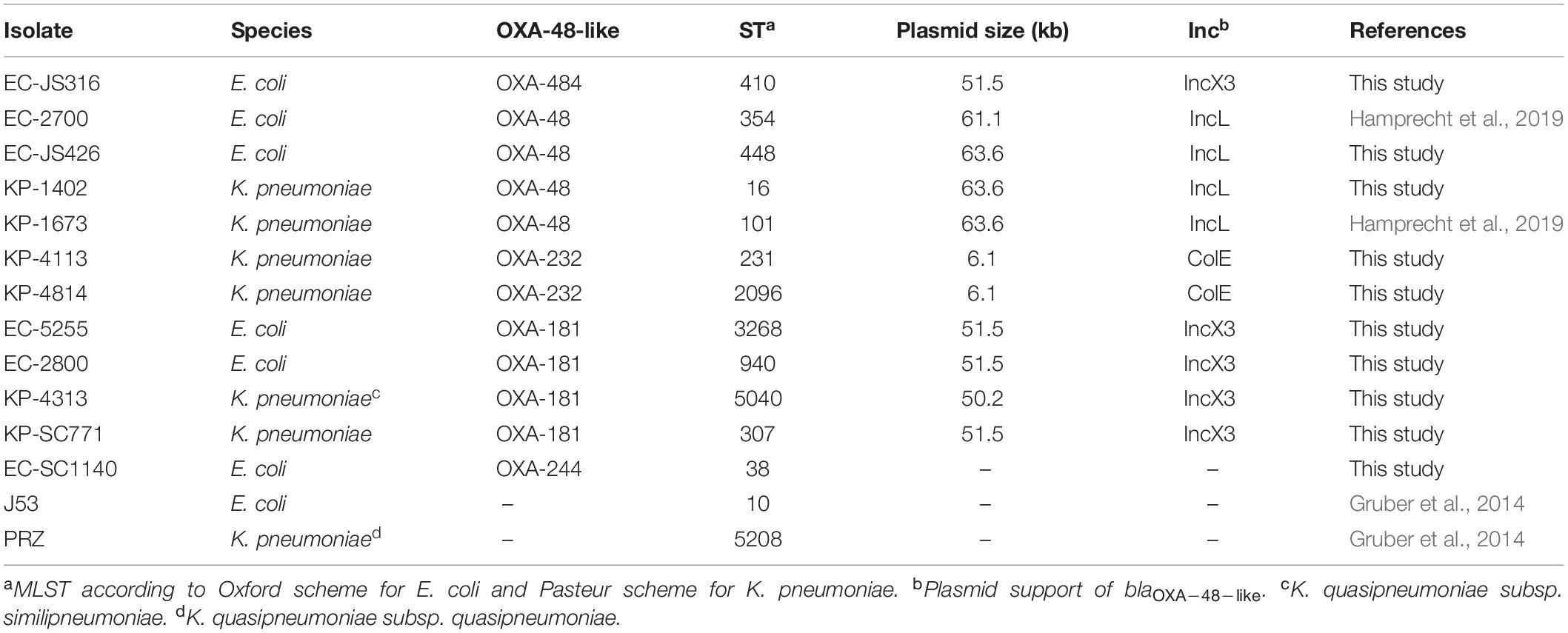
Table 1. Characteristics of clinical isolates harboring OXA-48-like carbapenemases and recipient strains E.coli J53 and K. quasipneumoniae subsp. quasipneumoniae PRZ.
Cloning of blaOXA–48–like
The open reading frames of blaOXA–484, blaOXA–244, blaOXA–232, blaOXA–181, and blaOXA–48, including their native promoters, were amplified using the primers OXA-TOPO-F1, OXA-244-TOPO-F1, OXA-48-TOPO-F1, and preOXA-48B (Supplementary Table 1) and cloned into the expression vector pCR-Blunt II-TOPO (Invitrogen, Darmstadt, Germany) as described before (Potron et al., 2011). The resulting vectors pTOPO_OXA-484, pTOPO_OXA-244, pTOPO_OXA-232, pTOPO_OXA-181, and pTOPO_OXA-48 were used to transform electrocompetent E. coli J53 and K. quasipneumoniae subsp. quasipneumoniae PRZ (Choi et al., 2006).
Horizontal Gene Transfer of blaOXA–48–like Harboring Plasmids
Transconjugation of blaOXA–48–like harboring plasmids was conducted by liquid mating as previously described (Gruber et al., 2014). Briefly, a mixture of donors and recipients in brain heart infusion broth were incubated over night at 37°C and subsequently plated on chromogenic agar plates containing 100 mg/L sodium azide and 10 mg/L amoxicillin-clavulanic acid to select for transconjugants (Tc). Clinical E. coli and K. pneumoniae isolates encoding blaOXA–48, blaOXA–181, blaOXA–232, or blaOXA–484 were employed as donors and sodium azide-resistant E. coli J53 and K. quasipneumoniae subsp. quasipneumoniae PRZ as recipients (Hamprecht et al., 2019). Plasmid DNA from clinical isolates carrying blaOXA–232 was extracted using the PureYieldTM Plasmid Midiprep System (Promega, Walldorf, Germany). Transformation of J53 and PRZ using blaOXA–232 encoding plasmids was done by electroporation and transformants (Tf) were selected as described above. Presence of OXA-48-like carbapenemases in Tc and Tf was verified by disk diffusion antibiotic testing and the CARBA 5 lateral flow test. All E. coli J53 Tc were analyzed using long-read genome sequencing and disk diffusion testing as recommended by EUCAST. Transconjugation frequency was determined by dividing the numbers of Tc colonies by the number of acceptor colonies.
Whole Genome Sequencing
Whole genome sequencing was carried out for all clinical isolates using short-read technology (MiSeq or NovaSeq platform, Illumina, San Diego, United States) and long-read technology (MinION platform, Oxford Nanopore Technologies, Oxford, United Kingdom). DNA was extracted from isolates using the DNeasy UltraClean Microbial Kit (Qiagen, Hilden, Germany). For Illumina sequencing, a v3 reagent kit was applied generating either 150 or 250 bp paired-end reads. Library preparation for Nanopore sequencing was done using the SQK-RBK004 rapid barcoding kit. Sequencing was performed on a MinION MK1B sequencer utilizing a R9.4.1 flow cell. Raw signal data was base called and demultiplexed using the high accuracy base calling model of guppy basecaller version 4.0.11.
Bioinformatic Analysis
Raw data was filtered using trimmomatic for short reads and NanoFilt for long reads resulting in datasets of reads with an average genome coverage of at least 100-fold for short-reads and at least 30-fold for long-reads (Bolger et al., 2014; de Coster et al., 2018). De novo hybrid assembly was conducted using Unicycler version 0.4.8 utilizing the bold assembly mode (Wick et al., 2017). The obtained assemblies were annotated using Prokka version 1.14.6 (Seemann, 2014). Sequence types were determined using the software mlst1 v2.19.0 (Carattoli et al., 2014). ABRicate2 v1.0.1 was applied using the databases PlasmidFinder and NCBI AMRFinderPlus for identification of plasmid incompatibility groups and antibiotic resistance genes, respectively, using thresholds of 100% gene coverage and ≥98% nucleotide sequence identity (Feldgarden et al., 2019). ISfinder was employed for annotation of insertion sequences (Siguier et al., 2006). Plasmid sequences were aligned using MAFFT 1.4.0 and visualized using Geneious3 11.1.5 (Katoh and Standley, 2013).
Statistical Analysis
For the comparison of transconjugation frequencies, continuous variables were assessed by Mann-Whitney U test. A P-value of < 0.05 was considered significant.
Results
Identification of a Clinical Isolate Harboring blaOXA–484
The E. coli isolate JS316 (EC-JS316) was obtained from a rectal swab of a patient admitted to the University Hospital Frankfurt in Germany. The patient was hospitalized due to acute dengue fever following a stay in India. The isolate EC-JS316 showed resistance to ertapenem with a MIC of 1 mg/L but was susceptible to imipenem (MIC 0.25 mg/L) and meropenem (MIC 0.125 mg/L) (Table 2). Furthermore, the isolate was resistant to piperacillin-tazobactam, cefotaxime, fluoroquinolones, gentamicin, tobramycin and trimethoprim-sulfamethoxazole, but remained susceptible to amikacin, fosfomycin, tigecycline and colistin (Supplementary Table 2). Strikingly, the isolate EC-JS316 did not grow on chromID® CARBA SMART screening agar plates used for detection of CPE.
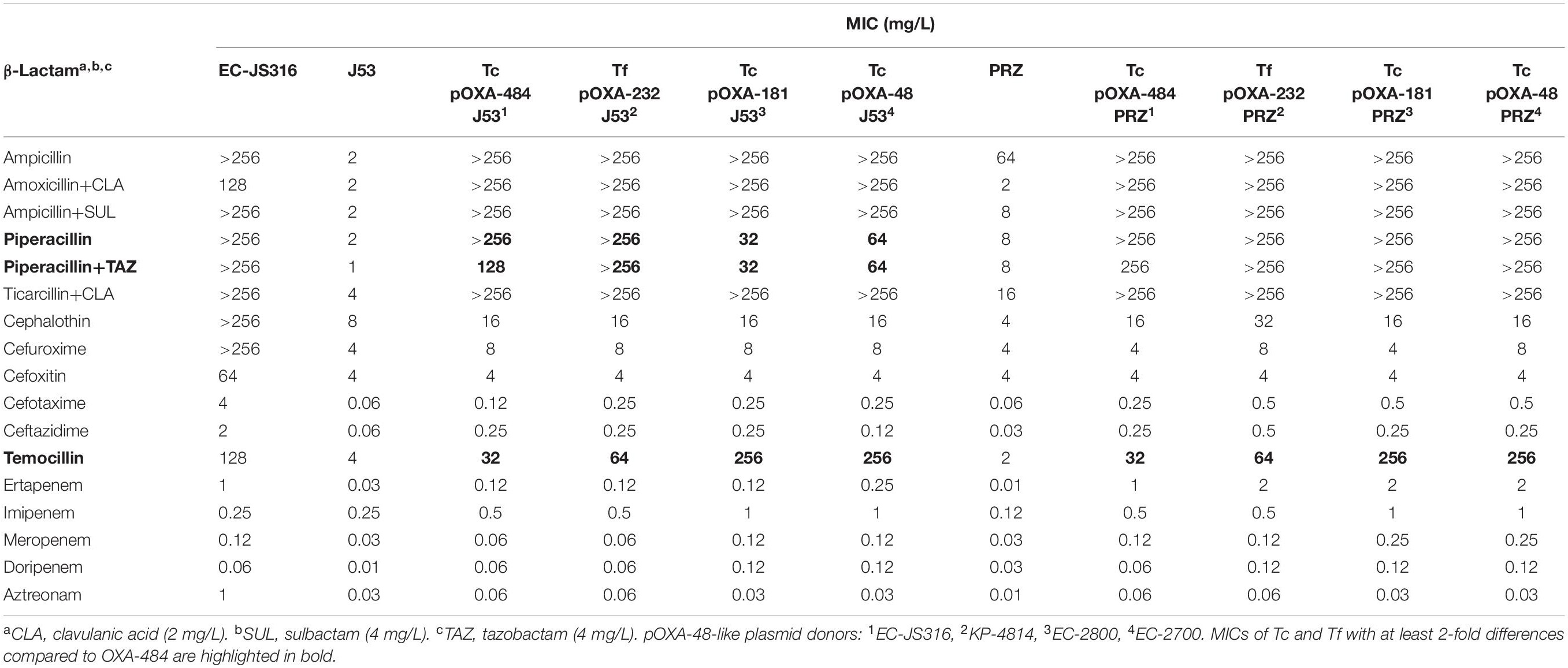
Table 2. MICs of the clinical isolate EC-JS316 and transformants (Tf) or transconjugants (Tc) of the recipients E. coli J53 and K. quasipneumoniae subsp. quasipneumoniae PRZ harboring plasmids encoding blaOXA–484, blaOXA–181, blaOXA–232, and blaOXA–48.
The CARBA 5 lateral flow test revealed the presence of an OXA-48-like β-lactamase. PCR and subsequent Sanger sequencing of the blaOXA–48–like open reading frame identified blaOXA–484 (accession no. NG_049766.1). The gene blaOXA–484 has a single nucleotide substitution at position 640 (A→G) compared to the most closely related gene blaOXA–181 and two substitutions at position 639 (A→G) and 641 (T→A) compared to blaOXA–232. These substitutions result in different amino acids at position 214 which is essential for enzyme activity: 214G for OXA-484, 214R for OXA-181, and 214S for OXA-232. The amino acid change R214S is also present in OXA-244 (Supplementary Figure 1).
Genome Analysis of E. coli EC-JS316
The genome of EC-JS316 was sequenced and assembled using a combined short- and long-read sequencing approach, resulting in the nucleotide sequences of a circular chromosome and five circular plasmids (Supplementary Table 3). The chromosome had a size of 4,718,403 bp coding for 4,398 predicted proteins and a GC-content of 50.8%. The ST of EC-JS316 was 410, which has been associated with international high-risk clones and acquisition of ESBL as well as different carbapenemases including OXA-181 (Roer et al., 2018; Patiño-Navarrete et al., 2020).
The resistome of EC-JS316 comprised the β-lactamase genes blaOXA–484, blaTEM–1, and blaEC–15. BlaOXA–484 was localized together with the fluoroquinolone resistance gene qnrS1 on the IncX3 plasmid pOXA-484 with a size of 51,480 bp (Figure 1). Additional antibiotic resistance genes were localized on the chromosome (blaEC–15), on a 67 kb IncF plasmid and a non-typeable 9 kb plasmid, conferring resistance to trimethoprim-sulfamethoxazole (dfrA17, sul2), aminoglycosides [aph(6)-Id, aac(3)-IId, aadA5], tetracyclines [tet(B)], and macrolides [mph(A)] (Supplementary Table 3).
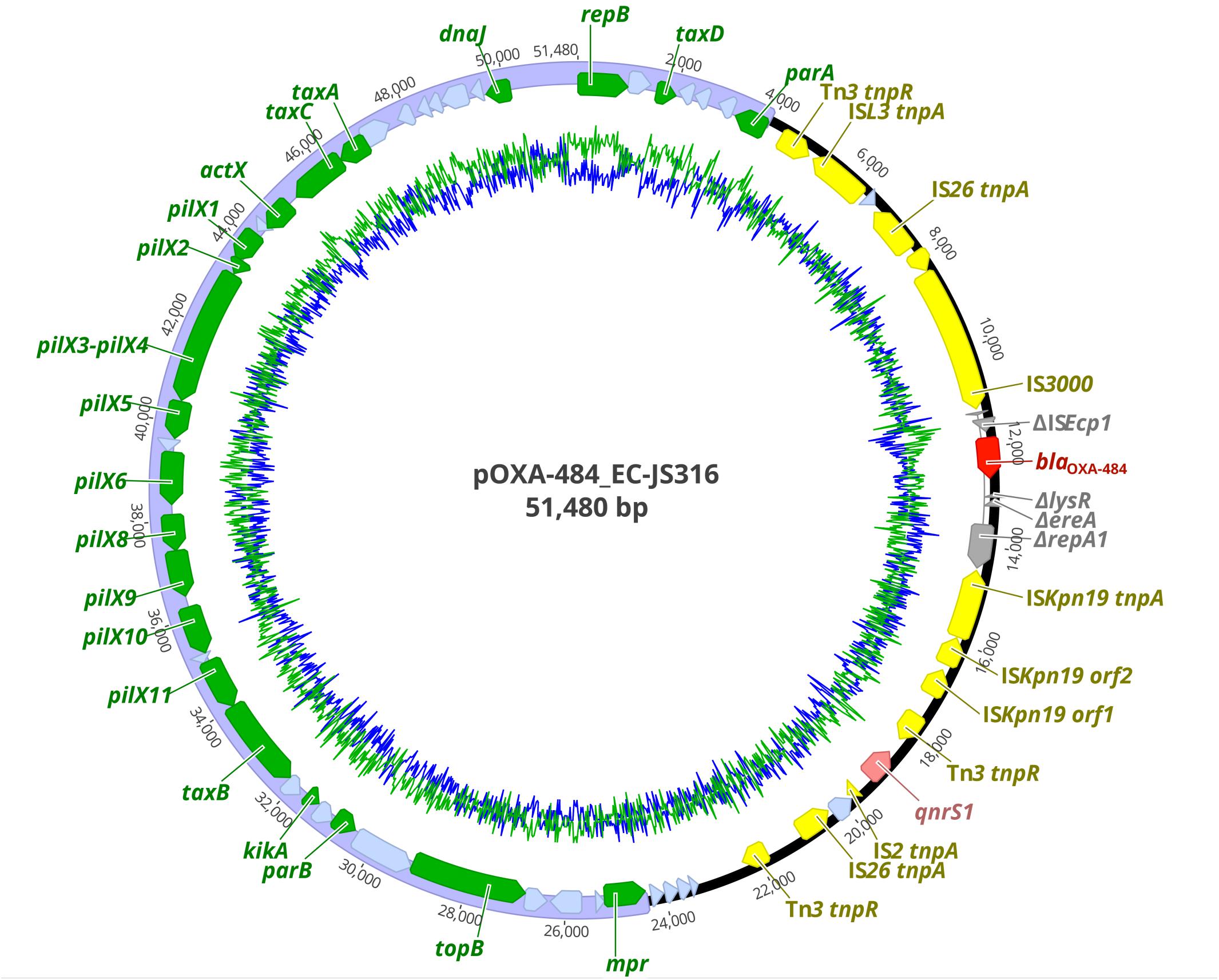
Figure 1. Plasmid map of pOXA-484 harboring blaOXA–484. Colored arrows indicate antibiotic resistance genes blaOXA–484 (dark red) and qnrS1 (light red), insertion elements (yellow), disrupted genes (gray), replication, partitioning, plasmid stabilization, and transfer genes of the IncX3 plasmid backbone (green) and hypothetical proteins (light blue). The IncX3 plasmid backbone is marked in purple. GC content is represented by the inner green line and AT content by the inner blue line.
Sequence Analysis of pOXA-484
The plasmid pOXA-484 consisted of a conserved IncX3 backbone region of ∼31 kb length encoding 21 genes for plasmid replication, mobilization and stabilization as well as 19 hypothetical proteins (Figure 1). The second ∼20 kb region of the plasmid contained the two antibiotic resistance genes blaOXA–484 and qnrS1 inside a mosaic region of 12 insertion sequences, 6 genes coding for hypothetical proteins, and 4 disrupted genes (Figure 1). Two IS26 sequences form a functional composite transposon including the gene blaOXA–484 (Qin et al., 2018). The blaOXA–484 was flanked by a truncated ISEcp1 element and two AT-rich direct target repeats (ATCTT), which has been associated with mobilization of blaOXA–181 from ColE2 to IncX3 plasmids (Liu et al., 2015).
For sequence comparison of pOXA-484 to plasmids encoding related OXA-48-like variants, we conducted whole genome sequencing (WGS) using short- and long-read sequencing of the clinical isolates E. coli 2800 (EC-2800) and K. pneumoniae 4814 (KP-4814). EC-2800 carried blaOXA–181 on an IncX3 plasmid of 51.5 kb and KP-4814 blaOXA–232 on a ColE plasmid of 6.1 kb. Both plasmids share high sequence similarity of >99.9% with previously described plasmids encoding blaOXA–181 and blaOXA–232 (Qin et al., 2018; Li et al., 2019).
Comparison of pOXA-484 to the nucleotide sequence of pOXA-181 from EC-2800 revealed a sequence identity of >99.9% with only four bases difference (Figure 2). Sequence comparison of pOXA-484 to the NCBI database revealed numerous IncX3 plasmids harboring blaOXA–181 from different Enterobacterales with a nucleotide sequence similarity >99.9% (accession no. KX523903.1, MG893567.1, and MK412916.1) indicating a common origin (Skalova et al., 2017; Qin et al., 2018; Mouftah et al., 2019). The sequence comparison of pOXA-484 to pOXA-232 from isolate KP-4814 revealed a fragment of only 2,964 bp shared between the two plasmids with >99.9% sequence identity (Figure 2).
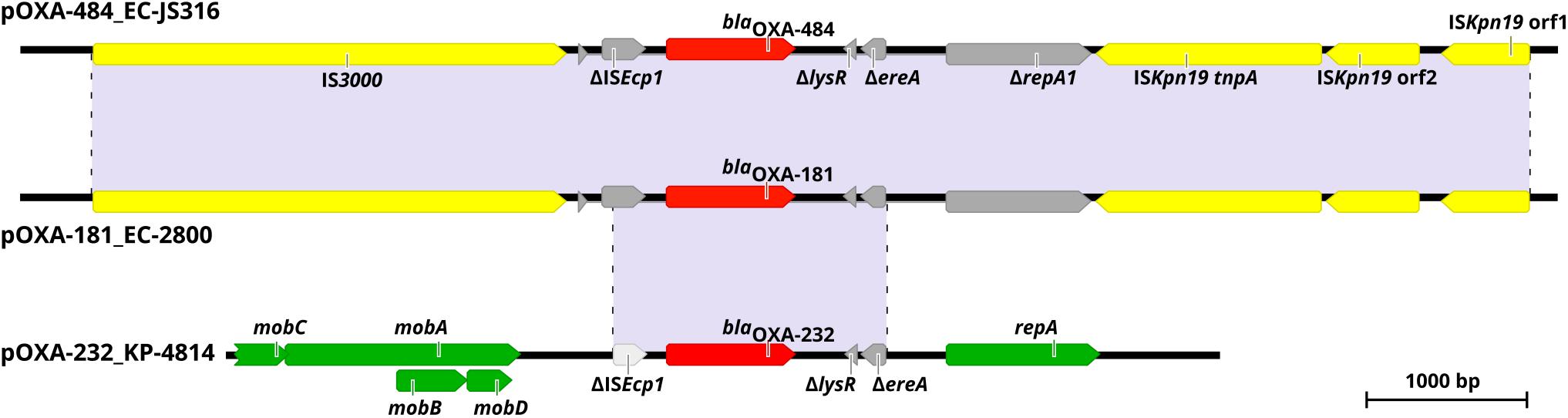
Figure 2. Genetic environment of blaOXA–484, blaOXA–181, and blaOXA–232 within pOXA-484_EC-JS316, pOXA-181_EC-2800, and pOXA-232_KP-4814. Colored arrows indicate insertion elements (yellow), fragmented genes (gray), antibiotic resistance genes (red) and genes of the plasmid backbone (green). Shaded regions between the plasmids share >99% nucleotide sequence identity.
Horizontal Gene Transfer of pOXA-484
To evaluate the HGT efficiency of pOXA-484, we compared the transconjugation frequencies of plasmid pOXA-484 carrying blaOXA–484 to the frequencies of plasmids encoding blaOXA–48, blaOXA–181, and blaOXA–232, using the recipient strains E. coli J53 and K. quasipneumoniae subsp. quasipneumoniae PRZ. Four representative clinical isolates carrying blaOXA–48 or blaOXA–181 were chosen from a collection of clinical isolates based on species (E. coli and K. pneumoniae) and different STs. Since OXA-232 is rarely found in E. coli, only two clinical K. pneumoniae isolates harboring blaOXA–232 were selected (Table 1 and Supplementary Table 4).
Transconjugation of plasmids harboring blaOXA–48, blaOXA–181, and blaOXA–484 from all clinical isolates to E. coli J53 and K. quasipneumoniae subsp. quasipneumoniae PRZ was observed, thereby showing intraspecies and intergenus HGT (Figure 3). Transconjugation of plasmid pOXA-484 to J53 and PRZ occurred at mean frequencies of 1.4 × 10–7 and 2.1 × 10–6, respectively (Figure 3). Similar frequencies were determined for blaOXA–181, ranging from 2.7 × 10–7 to 3.6 × 10–5 for J53 and 5.2 × 10–8 to 6.7 × 10–7 for PRZ. In contrast, transconjugation frequencies of blaOXA–48 from E. coli and K. pneumoniae donors to J53 and PRZ were significantly higher, ranging from 8.6 × 10–5 to 8.0 × 10–4 for J53 and 1.3 × 10–5 to 3.6 × 10–5 for PRZ (P < 0.001).
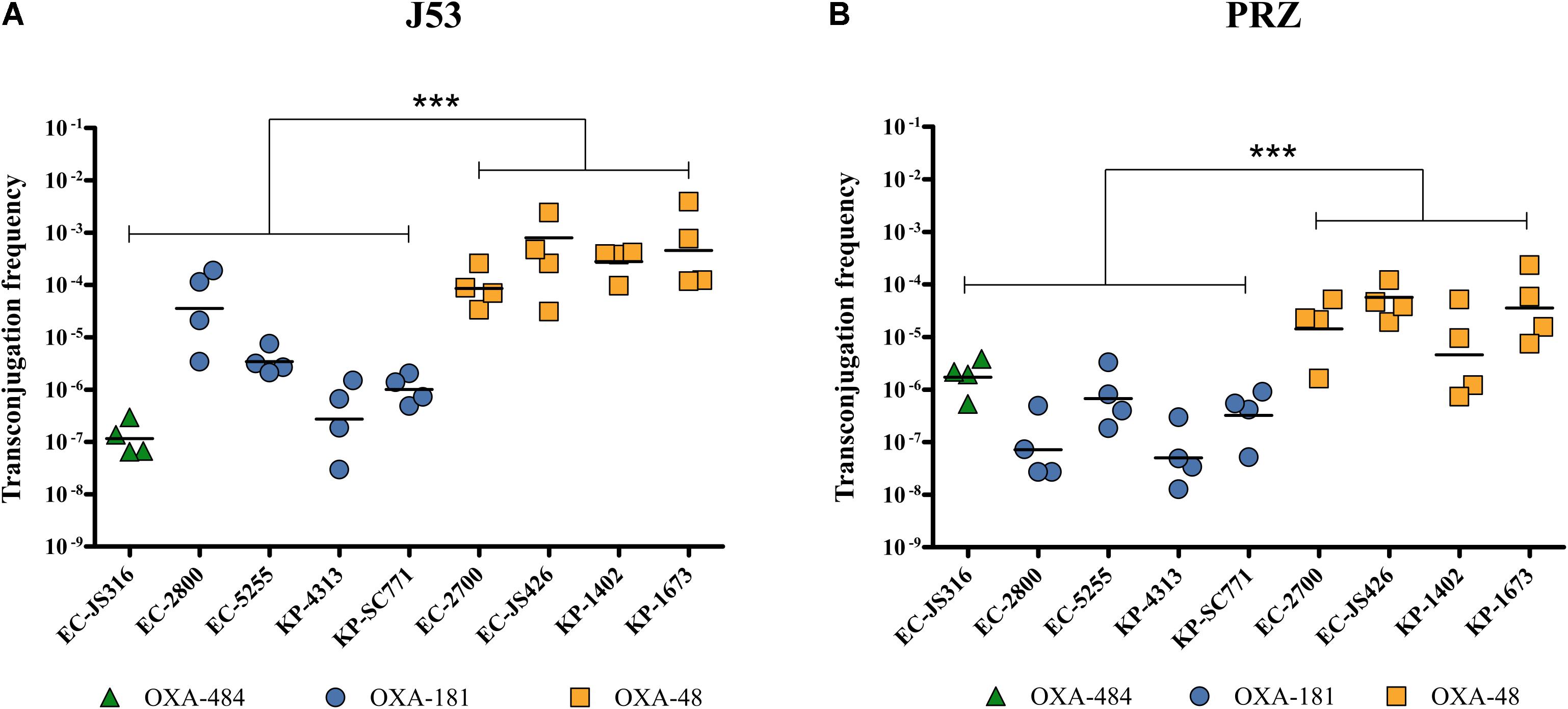
Figure 3. Transconjugation frequencies during horizontal transfer of plasmids harboring blaOXA–484, blaOXA–181, and blaOXA–48 from E. coli and K. pneumoniae clinical isolates to E. coli J53 and K. quasipneumoniae subsp. quasipneumoniae PRZ. Each dot represents the frequency of a single transconjugation experiment, whereas the bars indicate the mean of all experiments. ***P < 0.0001 (Mann-Whitney U test). Transconjugation frequencies to (A) E. coli J53 and (B) K. quasipneumoniae subsp. quasipneumoniae PRZ.
E. coli J53 Tc were further investigated by long-read genome sequencing and disk diffusion which verified the presence of the respective blaOXA–48–like as well as qnrS1 in blaOXA–181 and the blaOXA–484 Tc, respectively, as anticipated (Supplementary Tables 5, 6). For blaOXA–181 and blaOXA–484 Tc only the blaOXA–48-like harboring IncX3 plasmids were transferred. Notably, in three out of four blaOXA–48 Tc additional plasmids were identified: a 61.1 kb IncFII plasmid in Tc-EC-2700, a 5.2 kb Col440II plasmid in Tc-EC-1402 and a 3.5 kb Col(pHAD28) as well as a 10.7 kb Col440II plasmid in Tc-EC-1673. However, these plasmids did not harbor any antibiotic resistance genes nor was an impact on HGT frequency or antibiotic resistance phenotype observed when comparing all blaOXA–48 Tc to each other (Figure 3 and Supplementary Table 6).
Beta-Lactam Resistance Mediated by OXA-484
To analyze the resistance phenotype caused by OXA-484, we evaluated MICs of Tc or Tf harboring the natural plasmids as well as Tf carrying pTOPO expression vectors encoding the genes for OXA-484, OXA-181, OXA-232, OXA-244, and OXA-48, respectively. Since blaOXA–244 is generally localized on the chromosome, only MICs of Tf carrying pTOPO expression vector encoding blaOXA–244 were analyzed.
The presence of pOXA-48-like plasmids resulted in increased MICs for penicillins and carbapenems compared to J53 and PRZ parental strains, whereas only minor differences were observed for cephalosporins and aztreonam (Table 2). Carbapenem MICs of pOXA-484 were either unchanged or slightly lower compared to other OXA-48 variants. In contrast, differences in MICs of at least 2-fold between the OXA-48-like variants were detected for the antibiotics piperacillin ± tazobactam and temocillin. Tc with plasmids encoding blaOXA–48 and blaOXA–181 presented higher MICs for temocillin compared to recipients harboring blaOXA–484 and blaOXA–232 but lower MICs for piperacillin ± tazobactam in case of J53.
In J53 and PRZ Tf carrying the high copy pTOPO expression vector, the lower MICs for temocillin caused by OXA-484 and OXA-232 compared to OXA-181 and OXA-48 were also observed. Transformants carrying TOPO encoding blaOXA–244 showed almost identical MICs for all antibiotics tested compared to Tf pTOPO OXA-484. Furthermore, pTOPO OXA-48 Tf displayed higher carbapenem MICs compared to the other Tf.
Taken together, the β-lactam resistance patterns of Tc and Tf harboring blaOXA–484 revealed similar MICs to OXA-232 and OXA-244. Compared to OXA-181 and OXA-48, piperacillin ± tazobactam MICs were higher for Tc pOXA-484 J53, whereas temocillin MICs were lower for both Tc and Tf harboring either the natural plasmid or pTOPO expression vector.
Discussion
This study is the first characterization of the carbapenemase OXA-484, a variant of the growing family of OXA-48-like enzymes. The dissemination of OXA-48-like carbapenemases has been attributed to highly efficient HGT to different species, low fitness burden of plasmids encoding blaOXA–48–like, association with epidemiological successful lineages and difficult to detect carbapenemase phenotypes (Hamprecht et al., 2019; Pitout et al., 2019). The plasmid pOXA-484 from isolate EC-JS316 was transferable to E. coli and K. quasipneumoniae subsp. quasipneumoniae, revealing efficient intraspecies and intergenus transfer (Figure 3). The transconjugation frequencies of this plasmid are very similar to those of the widely distributed IncX3 plasmid harboring blaOXA–181. Sequence analysis of pOXA-484 revealed the close relationship to IncX3 plasmids containing blaOXA–181 suggesting that pOXA-484 might has evolved from pOXA-181 (Figure 2). Sequence analysis of IncX3 plasmids harboring blaOXA–181 has revealed the potential mobilization of blaOXA–181 by action of an ISEcp1 from ColE-plasmids to IncX3 plasmids (Liu et al., 2015). The highly similar sequences between the IncX3 plasmids harboring blaOXA–484 and blaOXA–181 including the ISEcp1 elements and two AT-rich direct target repeats bracketing blaOXA–484 suggest the same mechanism of mobilization for blaOXA–484. The spread of OXA-181 and OXA-232 has additionally been associated with specific high-risk clones like ST101, ST307 and ST15 for K. pneumoniae and ST38 and ST410 for E. coli (Cubero et al., 2015; Li et al., 2019; Pitout et al., 2019; Chudejova et al., 2021). Notably, the E. coli isolate JS316 belongs to the high-risk ST410, which has been identified as a worldwide distributed extraintestinal pathogenic E. coli lineage causing nosocomial outbreaks (Roer et al., 2018; Patiño-Navarrete et al., 2020).
The β-lactam resistance pattern caused by OXA-484 was comparable to the resistance phenotypes mediated by OXA-232 and OXA-244. However, carbapenem and temocillin MICs in OXA-484 expressing E. coli and K. quasipneumoniae subsp. quasipneumoniae were lower compared to OXA-48 and OXA-181 producers (Tables 2, 3). In a recent study, analyses of kinetic parameters and structural models of OXA-181 variants with mutations at amino acid position 214 in the β5-β6 loop revealed the critical impact of this amino acid on β-lactamase activity (Supplementary Figure 1) (Oueslati et al., 2020). Interestingly, an OXA-181 variant with a R214G mutation resembling the exact amino acid sequence of OXA-484 was included in this study. Enzyme kinetics of OXA-181 214G (or OXA-484) revealed low catalytic efficiencies for temocillin [0.15 ± 0.07 kcat/Km (mM–1/s–1)] and imipenem [20 ± 6.9 kcat/Km (mM–1/s–1)], which are similar to OXA-232 and lower than for OXA-181 and OXA-48 (Oueslati et al., 2014, 2020). These results are in line with our antimicrobial susceptibility results (Tables 2, 3).
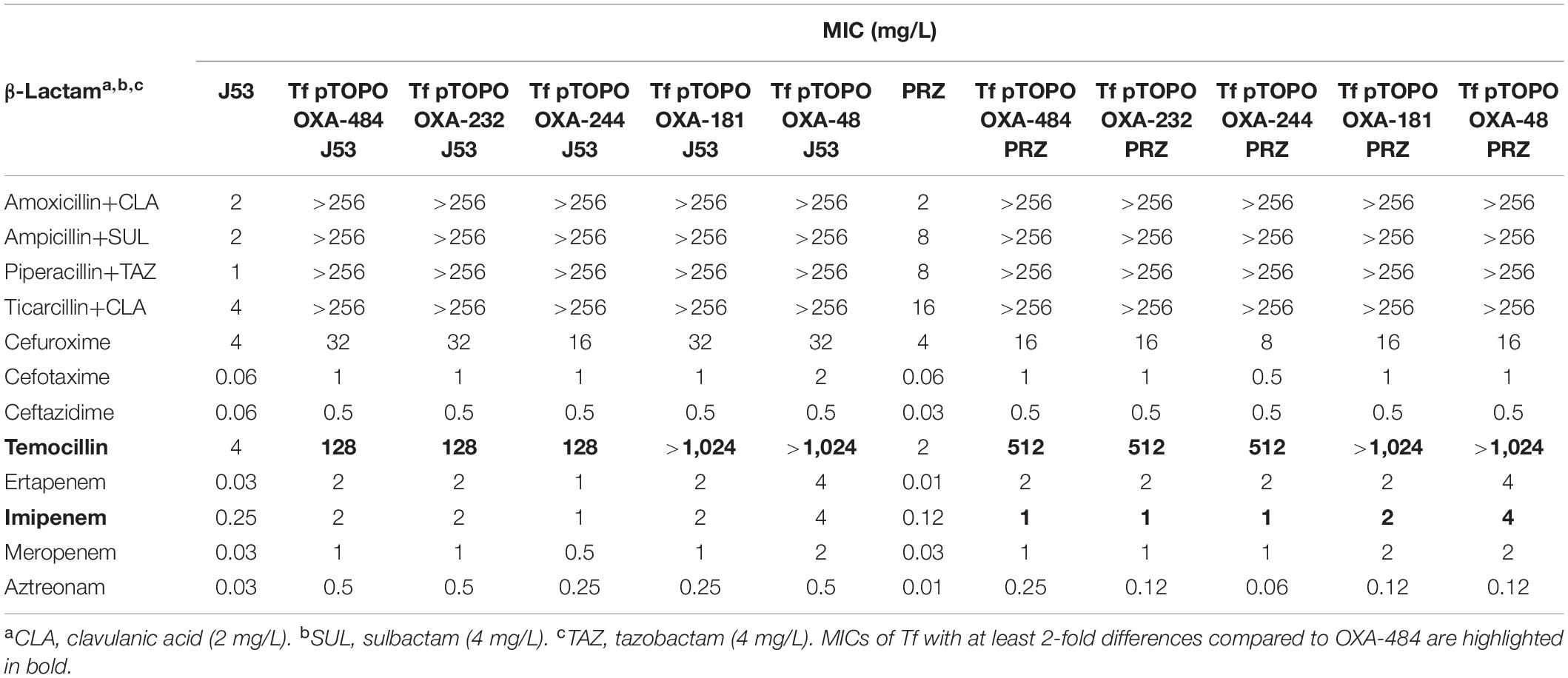
Table 3. MICs of E. coli J53 and K. quasipneumoniae subsp. quasipneumoniae PRZ transformants (Tf) harboring pTOPO plasmids encoding blaOXA–484, blaOXA–232, blaOXA–244, blaOXA–181, and blaOXA–48.
Substitution of arginine at amino acid 214 in OXA-48 to glycine in OXA-244 (R214G), which is also found in OXA-484, led to a reduced hydrolysis activity and MICs for temocillin and carbapenems (Hoyos-Mallecot et al., 2017). Reduced temocillin hydrolysis will most likely affect the sensitivity of temocillin-based screening plates and tests (Hopkins et al., 2019). Recently, increasing numbers of isolates expressing OXA-244 in the European Union have been linked with difficult detection because of only moderately elevated MICs for carbapenems of these isolates (European Centre for Disease Prevention and Control, 2020; Kremer et al., 2020). Likewise, the isolate EC-JS316 harboring blaOXA–484 did not grow on selective agar plates used for detection of CPE, suggesting that OXA-484 might be more easily missed and hence be more prevalent than currently known.
IncX3 plasmids have no significant negative effect on fitness of Enterobacterales isolates, which has also been shown for highly successful plasmids of the IncL group harboring blaOXA–48 and which is a predictor for efficient dissemination (Kassis-Chikhani et al., 2013; Hamprecht et al., 2019). These properties of the IncX3 plasmids might promote the further distribution of OXA-484, as already shown for other carbapenemases like NDM or KPC as well as CTX-M type ESBLs (Kassis-Chikhani et al., 2013; Wu et al., 2019).
Data Availability Statement
The datasets presented in this study can be found in online repositories. The names of the repository/repositories and accession number(s) can be found below: https://www.ncbi.nlm.nih.gov/bioproject/PRJNA644256 and https://www.ncbi.nlm.nih.gov/bioproject/PRJNA644257.
Ethics Statement
All bacterial strains were isolated as part of routine microbiological diagnostics and stored in an anonymized database. According to the Ethics Committee of the Hospital of Johann Wolfgang Goethe-University, Frankfurt am Main, no informed consent or ethical approval of the study is necessary.
Author Contributions
JSo and SG designed the study, analyzed the data, and drafted the manuscript. JSo, FK, KG, FW, MT, and SR-C performed the experiments. JSo carried out the sequencing and bioinformatic analyses. JSa, AH, and VK edited the manuscript. All authors reviewed the manuscript.
Funding
This study was supported by a scholarship of the Frankfurter Promotionsförderung (FPF) of the Faculty of Medicine of the Goethe University, Frankfurt am Main, Germany to JSo.
Conflict of Interest
The authors declare that the research was conducted in the absence of any commercial or financial relationships that could be construed as a potential conflict of interest.
Acknowledgments
We thank the team of curators of the Institute Pasteur MLST and whole genome MLST databases for curating the data and making them publicly available. We are indebted to Tobias Herther for outstanding technical assistance.
Supplementary Material
The Supplementary Material for this article can be found online at: https://www.frontiersin.org/articles/10.3389/fmicb.2021.660094/full#supplementary-material
Footnotes
References
Baeza, L. L., Pfennigwerth, N., Greissl, C., Göttig, S., Saleh, A., Stelzer, Y., et al. (2019). Comparison of five methods for detection of carbapenemases in Enterobacterales with proposal of a new algorithm. Clin. Microbiol. Infect. 25, 1286.e9–1286.e15. doi: 10.1016/j.cmi.2019.03.003
Bolger, A. M., Lohse, M., and Usadel, B. (2014). Trimmomatic: a flexible trimmer for Illumina sequence data. Bioinformatics 30, 2114–2120. doi: 10.1093/bioinformatics/btu170
Cantón, R., Akóva, M., Carmeli, Y., Giske, C. G., Glupczynski, Y., Gniadkowski, M., et al. (2012). Rapid evolution and spread of carbapenemases among Enterobacteriaceae in Europe. Clin. Microbiol. Infect. 18, 413–431. doi: 10.1111/j.1469-0691.2012.03821.x
Carattoli, A., Zankari, E., Garciá-Fernández, A., Larsen, M. V., Lund, O., Villa, L., et al. (2014). In silico detection and typing of plasmids using plasmidfinder and plasmid multilocus sequence typing. Antimicrob. Agents Chemother. 58, 3895–3903. doi: 10.1128/AAC.02412-14
Choi, K.-H. H., Kumar, A., and Schweizer, H. P. (2006). A 10-min method for preparation of highly electrocompetent Pseudomonas aeruginosa cells: application for DNA fragment transfer between chromosomes and plasmid transformation. J. Microbiol. Methods 64, 391–397. doi: 10.1016/J.MIMET.2005.06.001
Chudejova, K., Kraftova, L., Mattioni Marchetti, V., Hrabak, J., Papagiannitsis, C. C., and Bitar, I. (2021). Genetic plurality of OXA/NDM-encoding features characterized from Enterobacterales recovered from Czech Hospitals. Front. Microbiol. 12:641415. doi: 10.3389/fmicb.2021.641415
Cubero, M., Cuervo, G., Dominguez, M. Á, Tubau, F., Martí, S., Sevillano, E., et al. (2015). Carbapenem-resistant and carbapenem-susceptible isogenic isolates of Klebsiella pneumoniae ST101 causing infection in a tertiary hospital. BMC Microbiol. 15:177. doi: 10.1186/s12866-015-0510-9
de Coster, W., D’Hert, S., Schultz, D. T., Cruts, M., and van Broeckhoven, C. (2018). NanoPack: visualizing and processing long-read sequencing data. Bioinformatics 34, 2666–2669. doi: 10.1093/bioinformatics/bty149
European Centre for Disease Prevention and Control (2020). Increase in OXA-244-Producing Escherichia coli in the European Union/European Economic Area and the UK since 2013. Stockholm: ECDC.
Feldgarden, M., Brover, V., Haft, D. H., Prasad, A. B., Slotta, D. J., Tolstoy, I., et al. (2019). Validating the AMRFINder tool and resistance gene database by using antimicrobial resistance genotype-phenotype correlations in a collection of isolates. Antimicrob. Agents Chemother. 63:e00483-19. doi: 10.1128/AAC.00483-19
Findlay, J., Hopkins, K. L., Loy, R., Doumith, M., Meunier, D., Hill, R., et al. (2017). OXA-48-like carbapenemases in the UK: an analysis of isolates and cases from 2007 to 2014. J. Antimicrob. Chemother. 72, 1340–1349. doi: 10.1093/jac/dkx012
Göttig, S., Gruber, T. M., Stecher, B., Wichelhaus, T. A., and Kempf, V. A. J. (2015). In vivo horizontal gene transfer of the carbapenemase OXA-48 during a nosocomial outbreak. Clin. Infect. Dis. 60, 1808–1815. doi: 10.1093/cid/civ191
Göttig, S., Walker, S. V., Saleh, A., Koroska, F., Sommer, J., Stelzer, Y., et al. (2020). Comparison of nine different selective agars for the detection of carbapenemase-producing Enterobacterales (CPE). Eur. J. Clin. Microbiol. Infect. Dis. 39, 923–927. doi: 10.1007/s10096-019-03786-7
Gruber, T. M., Göttig, S., Mark, L., Christ, S., Kempf, V. A. J., Wichelhaus, T. A., et al. (2014). Pathogenicity of pan-drug-resistant Serratia marcescens harbouring blaNDM-1. J. Antimicrob. Chemother. 70, 1026–1030. doi: 10.1093/jac/dku482
Hamprecht, A., Sommer, J., Willmann, M., Brender, C., Stelzer, Y., Krause, F. F., et al. (2019). Pathogenicity of clinical OXA-48 isolates and impact of the OXA-48 IncL plasmid on virulence and bacterial fitness. Front. Microbiol. 10:2509. doi: 10.3389/fmicb.2019.02509
Hopkins, K. L., Meunier, D., Mustafa, N., Pike, R., and Woodford, N. (2019). Evaluation of temocillin and meropenem MICs as diagnostic markers for OXA-48-like carbapenemases. J. Antimicrob. Chemother. 74, 3641–3643. doi: 10.1093/jac/dkz383
Hoyos-Mallecot, Y., Naas, T., Bonnin, R. A., Patino, R., Glaser, P., Fortineau, N., et al. (2017). OXA-244-producing Escherichia coli Isolates, a challenge for clinical microbiology laboratories. Antimicrob. Agents Chemother. 61:e00818-17. doi: 10.1128/AAC.00818-17
Kassis-Chikhani, N., Frangeul, L., Drieux, L., Sengelin, C., Jarlier, V., Brisse, S., et al. (2013). Complete nucleotide sequence of the first KPC-2- and SHV-12-encoding IncX plasmid, pKpS90, from Klebsiella pneumoniae. Antimicrob. Agents Chemother. 57, 618–620. doi: 10.1128/AAC.01712-12
Katoh, K., and Standley, D. M. (2013). MAFFT multiple sequence alignment software version 7: improvements in performance and usability. Mol. Biol. Evol. 30, 772–780. doi: 10.1093/molbev/mst010
Kremer, K., Kramer, R., Neumann, B., Haller, S., Pfennigwerth, N., Werner, G., et al. (2020). Rapid spread of OXA-244-producing Escherichia coli ST38 in Germany: insights from an integrated molecular surveillance approach; 2017 to January 2020. Eurosurveillance 25:2000923. doi: 10.2807/1560-7917.ES.2020.25.25.2000923
Li, X., Ma, W., Qin, Q., Liu, S., Ye, L., Yang, J., et al. (2019). Nosocomial spread of OXA-232-producing Klebsiella pneumoniae ST15 in a teaching hospital, Shanghai, China. BMC Microbiol. 19:235. doi: 10.1186/s12866-019-1609-1
Liu, Y., Feng, Y., Wu, W., Xie, Y., Wang, X., Zhang, X., et al. (2015). First report of OXA-181-producing Escherichia coli in China and characterization of the isolate using whole-genome sequencing. Antimicrob. Agents Chemother. 59, 5022–5025. doi: 10.1128/AAC.00442-15
Mouftah, S. F., Pál, T., Darwish, D., Ghazawi, A., Villa, L., Carattoli, A., et al. (2019). Epidemic incx3 plasmids spreading carbapenemase genes in the united arab emirates and worldwide. Infect. Drug Resist. 12, 1729–1742. doi: 10.2147/IDR.S210554
Naas, T., Oueslati, S., Bonnin, R. A., Dabos, M. L., Zavala, A., Dortet, L., et al. (2017). Beta-lactamase database (BLDB)–structure and function. J. Enzyme Inhib. Med. Chem. 32, 917–919. doi: 10.1080/14756366.2017.1344235
Oueslati, S., Nordmann, P., and Poirel, L. (2014). Heterogeneous hydrolytic features for OXA-48-like β-lactamases. J. Antimicrob. Chemother. 70, 1059–1063. doi: 10.1093/jac/dku524
Oueslati, S., Retailleau, P., Marchini, L., Berthault, C., Dortet, L., Bonnin, R. A., et al. (2020). Role of arginine 214 in the substrate specificity of OXA-48. Antimicrob. Agents Chemother. 64:e02329-19. doi: 10.1128/AAC.02329-19
Patiño-Navarrete, R., Rosinski-Chupin, I., Cabanel, N., Gauthier, L., Takissian, J., Madec, J. Y., et al. (2020). Stepwise evolution and convergent recombination underlie the global dissemination of carbapenemase-producing Escherichia coli. Genome Med. 12:10. doi: 10.1186/s13073-019-0699-6
Pitout, J. D. D. D., Peirano, G., Kock, M. M., Strydom, K.-A. A., and Matsumura, Y. (2019). The global ascendency of OXA-48-type carbapenemases. Clin. Microbiol. Rev. 33:e00102-19. doi: 10.1128/CMR.00102-19
Poirel, L., Bonnin, R. A., and Nordmann, P. (2012). Genetic features of the widespread plasmid coding for the carbapenemase OXA-48. Antimicrob. Agents Chemother. 56, 559–562. doi: 10.1128/AAC.05289-11
Potron, A., Nordmann, P., Lafeuille, E., Al Maskari, Z., Al Rashdi, F., and Poirel, L. (2011). Characterization of OXA-181, a carbapenem-hydrolyzing class D beta-lactamase from Klebsiella pneumoniae. Antimicrob. Agents Chemother. 55, 4896–4899. doi: 10.1128/AAC.00481-11
Potron, A., Rondinaud, E., Poirel, L., Belmonte, O., Boyer, S., Camiade, S., et al. (2013). Genetic and biochemical characterisation of OXA-232, a carbapenem- hydrolysing class D β-lactamase from Enterobacteriaceae. Int. J. Antimicrob. Agents 41, 325–329. doi: 10.1016/j.ijantimicag.2012.11.007
Qin, S., Cheng, J., Wang, P., Feng, X., and Liu, H. M. (2018). Early emergence of OXA-181-producing Escherichia coli ST410 in China. J. Glob. Antimicrob. Resist. 15, 215–218. doi: 10.1016/j.jgar.2018.06.017
Roer, L., Overballe-Petersen, S., Hansen, F., Schønning, K., Wang, M., Røder, B. L., et al. (2018). Escherichia coli sequence type 410 is causing new international high-risk clones. mSphere 3:e00337-18. doi: 10.1128/msphere.00337-18
Seemann, T. (2014). Prokka: rapid prokaryotic genome annotation. Bioinformatics 30, 2068–2069. doi: 10.1093/bioinformatics/btu153
Siguier, P., Perochon, J., Lestrade, L., Mahillon, J., and Chandler, M. (2006). ISfinder: the reference centre for bacterial insertion sequences. Nucleic Acids Res. 34, D32–D36. doi: 10.1093/nar/gkj014
Skalova, A., Chudejova, K., Rotova, V., Medvecky, M., Studentova, V., Chudackova, E., et al. (2017). Molecular characterization of OXA-48-like-producing Enterobacteriaceae in the Czech Republic and evidence for horizontal transfer of pOXA-48-like plasmids. Antimicrob. Agents Chemother. 61:e01889-16. doi: 10.1128/AAC.01889-16
Wick, R. R., Judd, L. M., Gorrie, C. L., and Holt, K. E. (2017). Unicycler: resolving bacterial genome assemblies from short and long sequencing reads. PLoS Comput. Biol. 13:e1005595. doi: 10.1371/journal.pcbi.1005595
Keywords: OXA-48, OXA-484, IncX, carbapenemases, beta-lactamases, Enterobacterales, plasmid
Citation: Sommer J, Gerbracht KM, Krause FF, Wild F, Tietgen M, Riedel-Christ S, Sattler J, Hamprecht A, Kempf VAJ and Göttig S (2021) OXA-484, an OXA-48-Type Carbapenem-Hydrolyzing Class D β-Lactamase From Escherichia coli. Front. Microbiol. 12:660094. doi: 10.3389/fmicb.2021.660094
Received: 28 January 2021; Accepted: 14 April 2021;
Published: 12 May 2021.
Edited by:
Karsten Becker, University Medicine Greifswald, GermanyReviewed by:
Costas C. Papagiannitsis, University of Thessaly, GreeceMarco Maria D’Andrea, University of Rome Tor Vergata, Italy
Copyright © 2021 Sommer, Gerbracht, Krause, Wild, Tietgen, Riedel-Christ, Sattler, Hamprecht, Kempf and Göttig. This is an open-access article distributed under the terms of the Creative Commons Attribution License (CC BY). The use, distribution or reproduction in other forums is permitted, provided the original author(s) and the copyright owner(s) are credited and that the original publication in this journal is cited, in accordance with accepted academic practice. No use, distribution or reproduction is permitted which does not comply with these terms.
*Correspondence: Stephan Göttig, Stephan.Goettig@kgu.de