- Department of Clinical Analyses, Toxicology and Food Science, School of Pharmaceutical Sciences of Ribeirão Preto, University of São Paulo, Ribeirão Preto, Brazil
The pandemic Escherichia coli sequence type 131 (ST131) carrying plasmid-mediated colistin resistance mcr genes has emerged worldwide causing extraintestinal infections, with lineages belonging to three major clades (A, B, and C). Clade B is the most prevalent in animals, contaminating associated meat products, and can be transmitted zoonotically. However, the blaCTX–M–15 gene has only been associated with C2 subclade so far. In this study, we performed a genomic investigation of an E. coli (strain S802) isolated from a kale crop in Brazil, which exhibited a multidrug-resistant (MDR) profile to clinically significant antimicrobials (i.e., polymyxin, broad-spectrum cephalosporins, aminoglycosides, and fluoroquinolones). Whole-genome sequencing analysis revealed that the S802 strain belonged to serotype O25:H4, ST131/CC131, phylogenetic group B2, and virotype D5. Furthermore, S802 carried the clade B-associated fimH22 allele, genes encoding resistance to clinically important antimicrobials, metals, and biocides, and was phylogenetically related to human, avian, and swine ST131-H22 strains. Additionally, IncHI2-IncQ1, IncF [F2:A-:B1], and ColE1-like plasmids were identified harboring mcr-1.1, blaCTX–M–15, and qnrB19, respectively. The emergence of the E. coli ST131-H22 sublineage carrying mcr-1.1, blaCTX–M–15, and qnrB19 in agricultural soil represents a threat to food and environmental safety. Therefore, a One Health approach to genomic surveillance studies is required to effectively detect and limit the spread of antimicrobial-resistant bacteria and their resistance genes.
Introduction
The rapid spread of plasmid-mediated colistin resistance mcr genes has gained worldwide attention as a critical public health issue, since colistin is a last resort antimicrobial used to treat severe infections caused by multidrug-resistant (MDR) and extensively drug-resistant (XDR) bacteria (Perez et al., 2016; Tsuji et al., 2019).
Currently, epidemiological studies have shown that the spread of colistin-resistant mcr-positive bacteria is not a concern restricted to hospitals, but also represents a growing problem involving environmental and food safety. In this regard, various factors such as environmental sources, food-producing animals, international travel, and food trade, have accelerated the worldwide spread of mcr-type genes at the human-animal-environment interface (Liu et al., 2016; Hassan and Kassem, 2020; Johura et al., 2020).
In this context, the pandemic Escherichia coli sequence type 131 (ST131) carrying mcr-type genes has emerged causing extraintestinal infections (Liu et al., 2018; Mamani et al., 2019; Reid et al., 2019; Li et al., 2021). The complex subclonal structure of ST131 elucidated three major clades, each associated with a specific allele of the type 1 fimbrial adhesin gene (fimH), namely clade A with fimH41, clade B with fimH22, and clade C with fimH30 (Petty et al., 2014; Stoesser et al., 2016).
Most studies have focused on the ST131-H30 sublineage, which is one of the leading causes of extraintestinal infections in humans, including C2 subclade associated with the blaCTX–M–15 gene (Dahbi et al., 2014; Matsumura et al., 2015; Mamani et al., 2019). In contrast, the most prevalent animal ST131 strains belong to the ST131-H22 sublineage and can be transmitted zoonotically, presenting a public health challenge (Liu et al., 2018; Roer et al., 2019; Saidenberg et al., 2020).
Specifically, contamination of crops by critical priority pathogens is of great concern, since these pathogens can also contaminate vegetables for consumption (Cantas et al., 2013; Araújo et al., 2017; Lopes et al., 2017; Reid et al., 2020; Lopes et al., 2021), increasing the risk of human exposure to antimicrobial-resistant bacteria, including mcr-positive strains. Despite this, little is known about the occurrence of bacteria carrying mcr-type genes in soils. Therefore, in this study, we performed a genomic investigation of an mcr-1-positive E. coli strain exhibiting an MDR profile to clinically significant antimicrobials and isolated from agricultural soil in the light of the One Health context that integrates human, animal, and environmental health.
Materials and Methods
Soil Sampling and Bacterial Isolation
During a surveillance study conducted between October and December 2019 to monitor the presence of clinically significant MDR Gram-negative bacteria in crops, 15 soil samples with a history of cow manure use were collected at a depth of ∼5 cm from chicory (n = 3), kale (n = 3), mustard (n = 3), parsley (n = 3), and chive (n = 3) crops on a farm in the state of São Paulo (21°00′36.0″ S; 47°27′00.0″ W), Brazil. All samples were stored at 4 °C and processed within 24 h. For bacterial isolation, 1 g of soil was inoculated in Luria-Bertani broth (Oxoid Ltd., United Kingdom) and incubated at 37 °C for 24 h. Subsequently, the cultures were streaked onto MacConkey agar plates (Oxoid Ltd., United Kingdom) supplemented with ceftriaxone (2 μg/ml) or colistin (2 μg/ml). Colonies were picked from the selective plates, subcultured, and streaked to obtain pure cultures. Bacterial identification was initially performed using 16S rRNA gene sequencing (Weisburg et al., 1991).
Antimicrobial Susceptibility Testing and Detection of Resistance Genes
Antimicrobial susceptibility testing was performed by disk diffusion, VITEK 2 (biomérieux, France), and/or agar dilution methods with interpretative criteria from CLSI guidelines [CLSI (Clinical and Laboratory Standards Institute), 2020]. Colistin minimum inhibitory concentration (MIC) was determined by broth microdilution according to EUCAST1. Extended-spectrum β-lactamase (ESBL) production was screened by the double-disk synergy test (Jarlier et al., 1988). Additionally, mcr-type (mcr-1 to mcr-9) and blaCTX–M-type (blaCTX–M–1, blaCTX–M–2, blaCTX–M–8, and blaCTX–M–9 groups) genes were investigated by PCR (Dallenne et al., 2010; Liu et al., 2016; Xavier et al., 2016; Borowiak et al., 2017; Carattoli et al., 2017; Yin et al., 2017; Yang et al., 2018; Wang et al., 2019).
DNA Isolation and Whole-Genome Sequencing
For whole-genome sequencing (WGS), total DNA was extracted from an overnight culture using the GenEluteTM Bacterial Genomic DNA Kit (Sigma-Aldrich, United States) according to the manufacturer’s instructions. Sequencing was performed using the Illumina HiSeq 4000 (2 × 150 bp) platform (Illumina, United States).
Data Processing, Assembly, and Genome Analysis
A quality check of the raw sequencing data was performed using the FastQC v.0.11.9 program2 and the reads were trimmed with Trimmomatic v.0.39 (Bolger et al., 2014). The quality value used for the base-calling program was Q = 20. In the next step, de novo genome assembly was carried out with SPAdes v.3.15.0 (Bankevich et al., 2012) and annotation was performed with Prokka v.1.14.5 (Seemann, 2014). Sequence type, serotype, FimH type, and clonotype were identified using MLST v2.0 (Larsen et al., 2012), SerotypeFinder v.2.0 (Joensen et al., 2015), FimTyper v.1.0 (Roer et al., 2017), and CHTyper v.1.0 (Roer et al., 2018), respectively. Antimicrobial resistance genes were detected using ResFinder v.4.1 (Zankari et al., 2012) and Antibiotic Resistance Gene-ANNOTation (ARG-ANNOT) v.4 (Gupta et al., 2014). Metals and biocides resistance genes were analyzed by BacMet v.2.0 (Pal et al., 2014). VirulenceFinder v.2.0 (Joensen et al., 2014) and the Virulence Factor Database (VFDB) v.R5 (Chen et al., 2005) were used to detect virulence genes, whereas virulence phylogroup was determined using the online Clermont typing tool3.
Phylogenetic Analysis
For phylogenetic analysis, we selected the E. coli strain reported in this study and 849 other strains representative of all clades (A, B, and C) of E. coli ST131. A minimum spanning tree was constructed based on the MSTree v.2 algorithm and the wgMLST scheme in Enterobase4. The tree was visualized with iTOL v.5.7 (Letunic and Bork, 2019).
Plasmid Assembly, Annotation, and Typing
Putative plasmid contigs were assembled using plasmidSPAdes v.3.15.0 (Antipov et al., 2016) and subjected to BLASTn analysis followed by gap closure. Annotation was performed by the Rapid Annotations using Subsystems Technology (RAST) server (Aziz et al., 2008) and manually curated with Geneious v.11.1.5 (Biomatters Ltd., Auckland, New Zealand). Plasmid replicon types and multilocus sequence typing were determined using PlasmidFinder v.2.1 and pMLST v.2.0 (Carattoli et al., 2014), respectively.
Conjugation Assays
Conjugation assays were conducted using azide-resistant E. coli C600 as recipient strain. Overnight cultures of donor and recipient strains were mixed (ratio 1:1) and incubated for 18 h at 37 °C without shaking as previously described (Furlan et al., 2020a). Transconjugants were selected using MacConkey agar (Oxoid Ltd., United Kingdom) supplemented with sodium azide (200 μg/ml) and ceftriaxone (2 μg/ml), or sodium azide (200 μg/ml) and colistin (2 μg/ml), and confirmed by PCR for the detection of mcr- and blaCTX–M-type genes as described above.
Results
MDR mcr-1-Positive ESBL-Producing E. coli Isolated From Agricultural Soil
In this study, the presence of a mcr-1-positive ESBL-producing E. coli strain (named S802), identified by 16S rRNA gene sequence analysis and pairwise genome comparison of average nucleotide identity, was confirmed in one soil sample from the kale crop. In addition, the E. coli strain S802 displayed an MDR profile, defined as resistant to at least one antimicrobial of three or more different categories (Magiorakos et al., 2012). The MDR profile of E. coli S802 included resistance to colistin, penicillin, cephalosporins, aztreonam, aminoglycosides, quinolones, tetracycline, and chloramphenicol. In contrast, the strain displayed an intermediary resistance profile to ampicillin/sulbactam, remaining susceptible to piperacillin/tazobactam, amikacin, and carbapenems (Table 1).
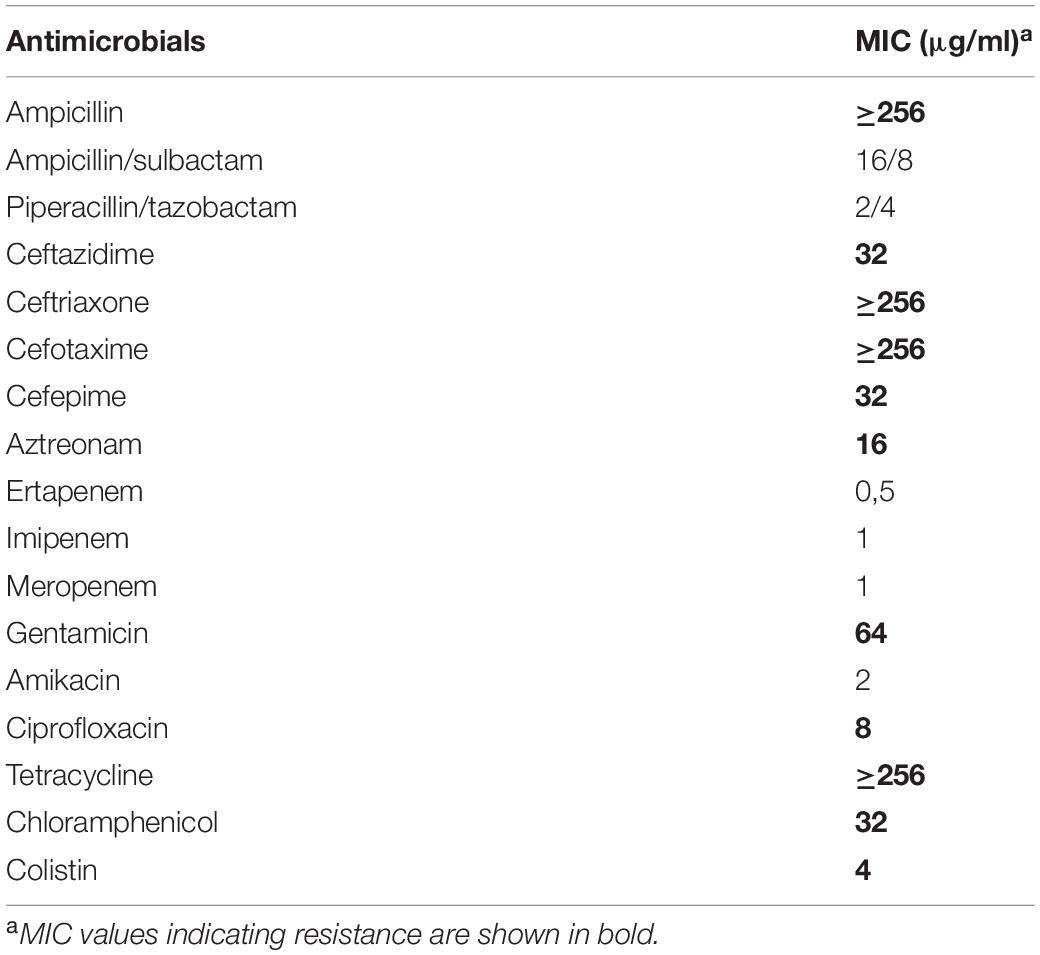
Table 1. MICs of antimicrobials for mcr-1-positive ESBL-producing E. coli strain S802 from agricultural soil.
Identification of the Pandemic Escherichia coli ST131 Lineage and Phylogenetic Analysis
WGS revealed that E. coli strain S802 belonged to serotype O25:H4 and phylogroup B2, known for including highly virulent extraintestinal lineages. Strain S802 carried fimH22 allele and was assigned to the clade B pandemic ST131/CC131 lineage (Figure 1). In addition, the clonotype CH40-22 was determined.
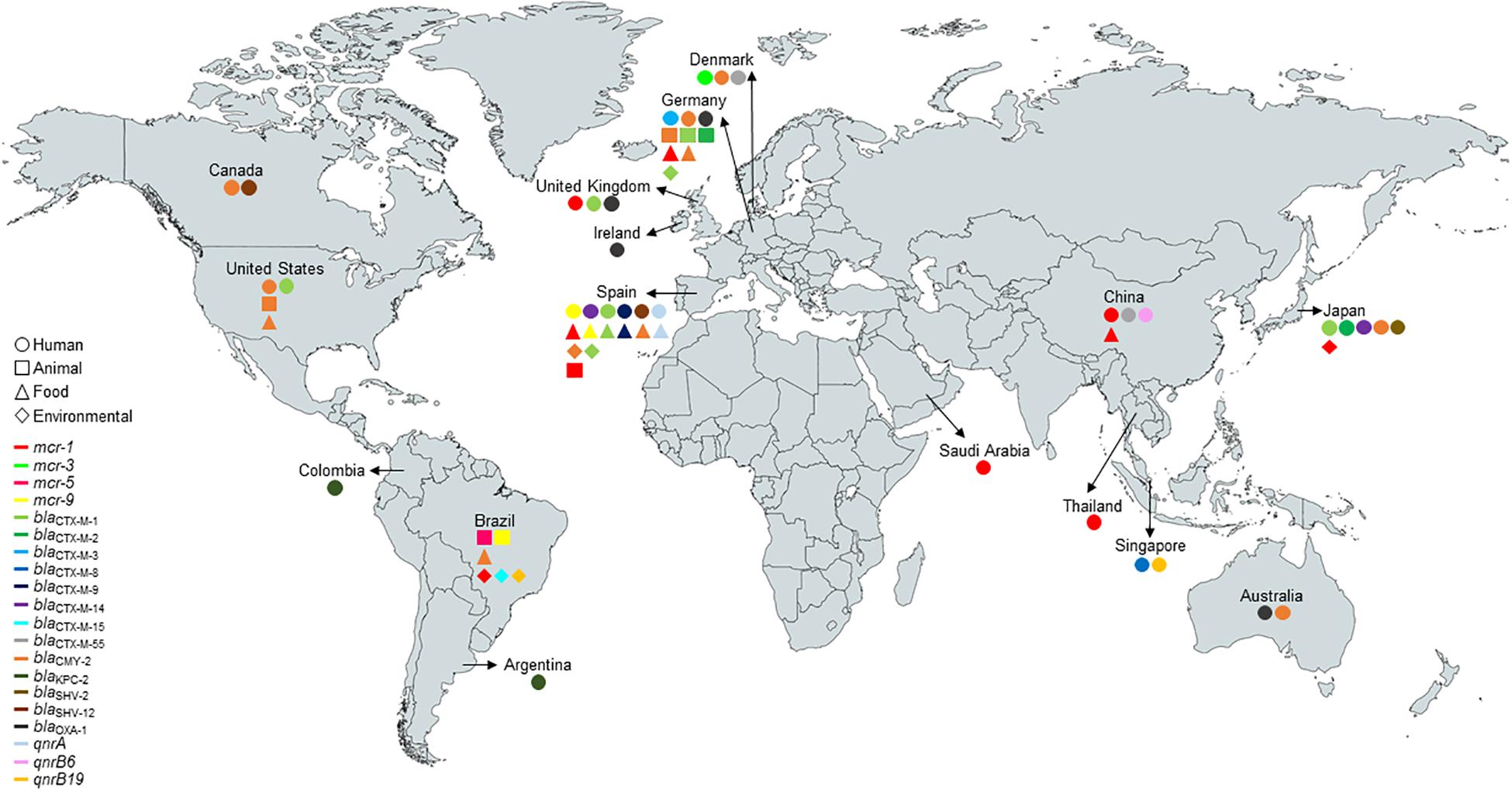
Figure 1. Worldwide distribution and sources of E. coli ST131-H22 carrying mcr-type, β-lactamase, and qnr-type genes at the human-animal-environment interface. The figure was produced using data retrieved from Enterobase (http://enterobase.warwick.ac.uk/species/index/ecoli) and Pubmed (https://pubmed.ncbi.nlm.nih.gov/) (blaTEM-types were not included in the figure).
Phylogenetic relatedness among 850 genomes of globally reported E. coli ST131 strains (Figure 2A) assigned S802 to a cluster comprising human E. coli ST131-H22 strains from Spain, Netherlands, Germany, and Belgium; one avian strain from Germany; and one swine strain from Spain. E. coli strain S802 was most related to two strains isolated from humans in Spain in 2010 (Figure 2B).
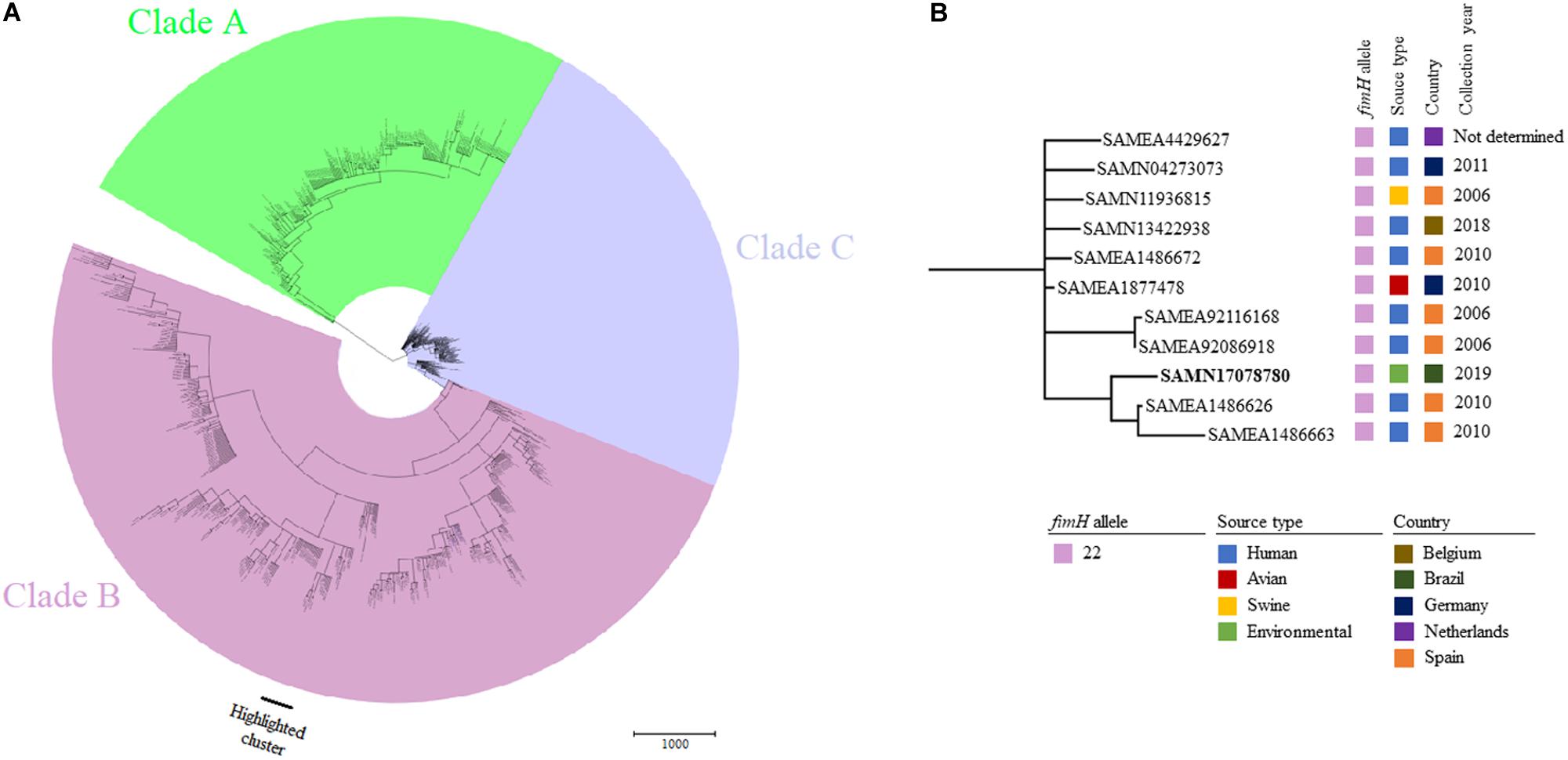
Figure 2. (A) Minimum spanning tree based on wgMLST of 850 worldwide distributed E. coli strains belonging to ST131. (B) Highlighted cluster containing E. coli S802 (BioSample accession indicated in bold) and showing the fimH allele, source of origin, country, and collection year of closely related strains.
Wide Resistome Against Multiple Antimicrobial Categories
In addition to the colistin resistance gene mcr-1.1, WGS analysis showed that ESBL production in the S802 strain was associated with the presence of the blaCTX–M–15 gene. Furthermore, a wide resistome was detected encoding other resistance determinants to β-lactams (blaTEM–1A, blaTEM–1B), aminoglycosides [aac(3)-IIa, aadA1, aadA2b, aph(3′)-Ia, aph(3″)-Ib, aph(6)-Id], fluoroquinolones (qnrB19), sulphonamides (sul1, sul2, sul3), trimethoprim (dfrA1, dfrA5), macrolides (mdfA), phenicols (catA1, cmlA1), and tetracyclines (tetA), as well as mutations in the quinolone resistance-determining region of gyrA (Ser83Leu, Asp87Asn) and parC (Ser80Ile) (Table 2).
Genes predicted to confer tolerance to metals, including copper (cueOR, cutACEF, pcoEABCDRSE), silver (silESRCBAP), copper/silver (cusSRCFBA), mercury (merRTPCADE), tellurium (tehAB, terY3Y2XY1W, terZABCDE), tellurium/selenium/chromium (ruvB), nickel (nikABCDE), nickel/cobalt/iron (rcnABR), cobalt/magnesium/manganese (corAB), and zinc (zraP) were also identified.
Regarding biocides resistance, genes encoding efflux pumps, transport modulators, and other proteins associated with resistance to acridines (acrAEFS, tehAB, tolC), chlorhexidine (cpxA), crystal violet (mdtABCEFGHKNOP, tehAB), ethidium bromide (acrAEFS, sugE, tehAB, tolC), hydrochloric acid (gadCEWX), hydrogen peroxide (cpxA, fetAB, sitABCD), organic solvents (marRAB), quaternary ammonium compounds (acrAEFS, cpxA, emrABEKRY, mdtABCEFGHKNOP sugE, tolC), sodium deoxycholate (evgAS), and sodium dodecyl sulfate (acrAEFS, emrABEKRY, mdtABCEFGHKNOP sugE, tolC) were detected (Table 2).
Virulome
Virulome analysis of E. coli S802 revealed a diversity of virulence factors, including chuA (outer membrane hemin receptor), cia (colicin Ia), cnf1 (cytotoxic necrotizing factor), cvaABC/cvi [colicin (microcin) V operon], etsC (putative type I secretion outer membrane protein), fyuA (yersiniabactin receptor), gad (glutamate decarboxylase), hlyCABD (α-hemolysin operon), hlyF (hemolysin F), hra (heat-resistant agglutinin), ibeA (invasin of brain endothelial cells), iroBCDEN (salmochelin operon), irp1-2/ybtAEPQSTUX (yersiniabactin synthesis), iss (increased serum survival lipoprotein), iucABCD/iutA (aerobactin operon), kpsE (capsule polysaccharide export inner-membrane protein), kpsM II-K5 (polysialic acid transport protein; group II capsule), mchF (ABC transporter protein), ompT [outer membrane protease (protease 7)], papIBAHCDJKEFG (P fimbriae operon), sitABCD (iron and manganese transport system), traT (complement resistance protein), usp (uropathogenic specific protein), and yfcV (fimbrial protein) (Table 2). In this regard, E. coli S802 was assigned to the virotype D5, based on the presence of the cnf1, hlyA, ibeA, kpsM II-K5, and papGIII genes (Dahbi et al., 2014).
Plasmids and Horizontal Transfer
Three plasmids, named pS802-MCR, pS802-CTX-M, and pS802-QnrB, were harbored by the S802 strain and carried mcr-1.1, blaCTX–M–15, and qnrB19, respectively (Figure 3).
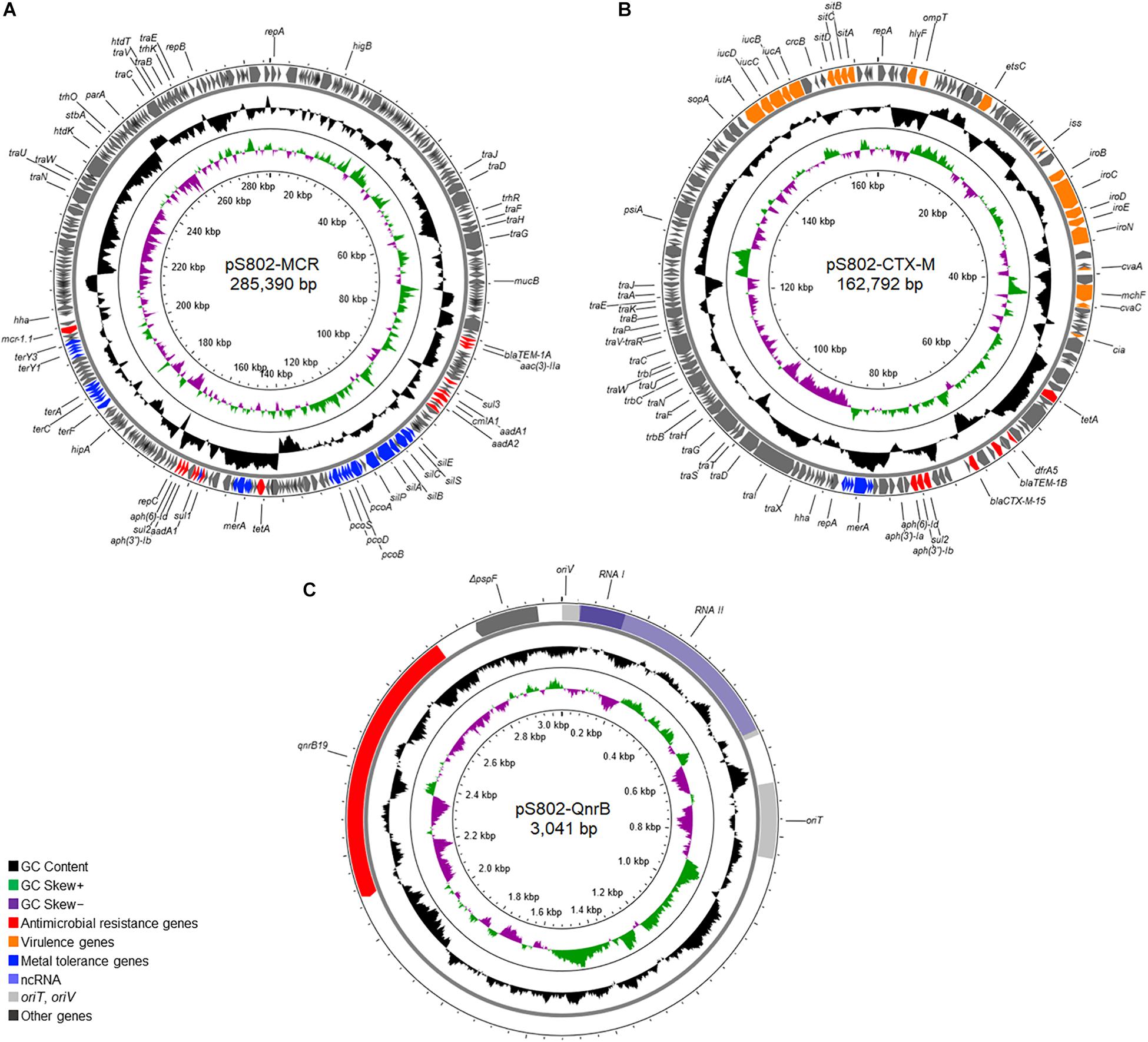
Figure 3. Overview of the plasmids (A) pS802-MCR, (B) pS802-CTX-M, and (C) pS802-QnrB carrying mcr-1.1, blaCTX–M–15, and qnrB19, respectively, in E. coli S802.
The pS802-MCR plasmid was 285,390 bp in length, containing 48.47% GC and 315 coding regions (CDSs), and belonged to the HI2 (ST4) and Q1 incompatibility groups. Besides mcr-1.1, this plasmid carried blaTEM–1A, aac(3)-IIa, aadA1, aadA2b, aph(3″)-Ib, aph(6)-Id, sul1, sul2, sul3, dfrA1, catA1, cmlA1, tetA, merRTPCADE, pcoEABCDRSE, silESRCBAP, terY3Y2XY1W, terZABCDEF, and qacEΔ1 resistance genes (Table 2). Analysis of the genetic context of mcr-1.1 revealed the presence of ISApl1 upstream and pap2 downstream of the gene. Moreover, pS802-MCR showed a high nucleotide identity with other IncHI2 plasmids of E. coli strains isolated from animal, human, and food in European and Asian countries, as well as with plasmids of Salmonella Schwarzengrund strains isolated from poultry in Brazil (Figure 4).
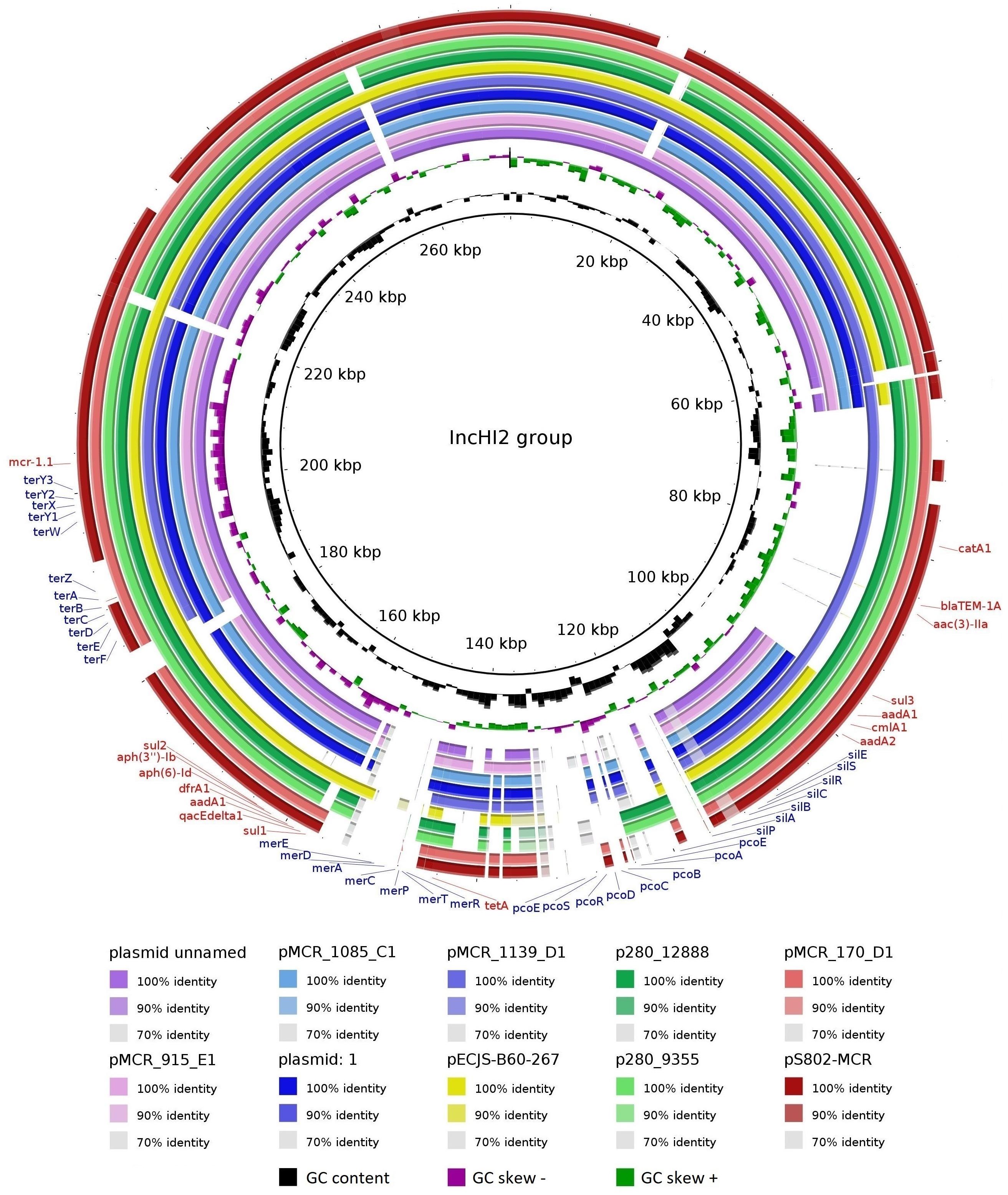
Figure 4. Comparison between IncHI2 plasmids. Plasmids of E. coli strains: plasmid unnamed (GenBank: CP015833.1) from a human in the United Kingdom; plasmid: 1 (GenBank: LR882919.1) from a human in the Netherlands; pECJS-B60-267 (GenBank: KX254341.1) from a pig in China; pS802-MCR (GenBank: MW495059.1) from soil in Brazil; pMCR_915_E1 (GenBank: MT929285.1), pMCR_1085_C1 (GenBank: MT929286.1), pMCR_1139_D1 (GenBank: MT929287.1), and pMCR_170_D1 (GenBank: MT929288.1) from turkey meat in the Czech Republic. Plasmids of Salmonella Schwarzengrund strains: p280_12888 (GenBank: CP045449.1) and p280_9355 (GenBank: CP045446.1) from poultry in Brazil. Matches with less than 70% identity and no matches appear as blank spaces. Resistance genes to antimicrobials/biocides and metals are indicated in red and blue, respectively.
The pS802-CTX-M plasmid was a 162,792 bp IncF [F2:A-:B1] plasmid, containing 52.48% GC and 174 CDSs. In addition to the blaTEM–1B, aph(3′)-Ia, aph(3″)-Ib, aph(6)-Id, sul2, dfrA5, tetA, merRTPCADE, and sitABCD resistance genes (Table 2), the ISEcp1-blaCTX–M–15-Δorf477 transposition unit was identified inserted in the transposon Tn2 truncated by IS26 in this plasmid. Virulence genes, namely cia, cvaABC/cvi, etsC, hlyF, iroBCDEN, iss, iucABCD, iutA, mchF, ompT, sitABCD, and traT, were also carried on pS802-CTX-M. The pS802-CTX-M plasmid exhibited a high nucleotide identity with IncF plasmids of E. coli strains isolated from animal, human, and food in North American, Asian, Australian, and European countries (Figure 5).
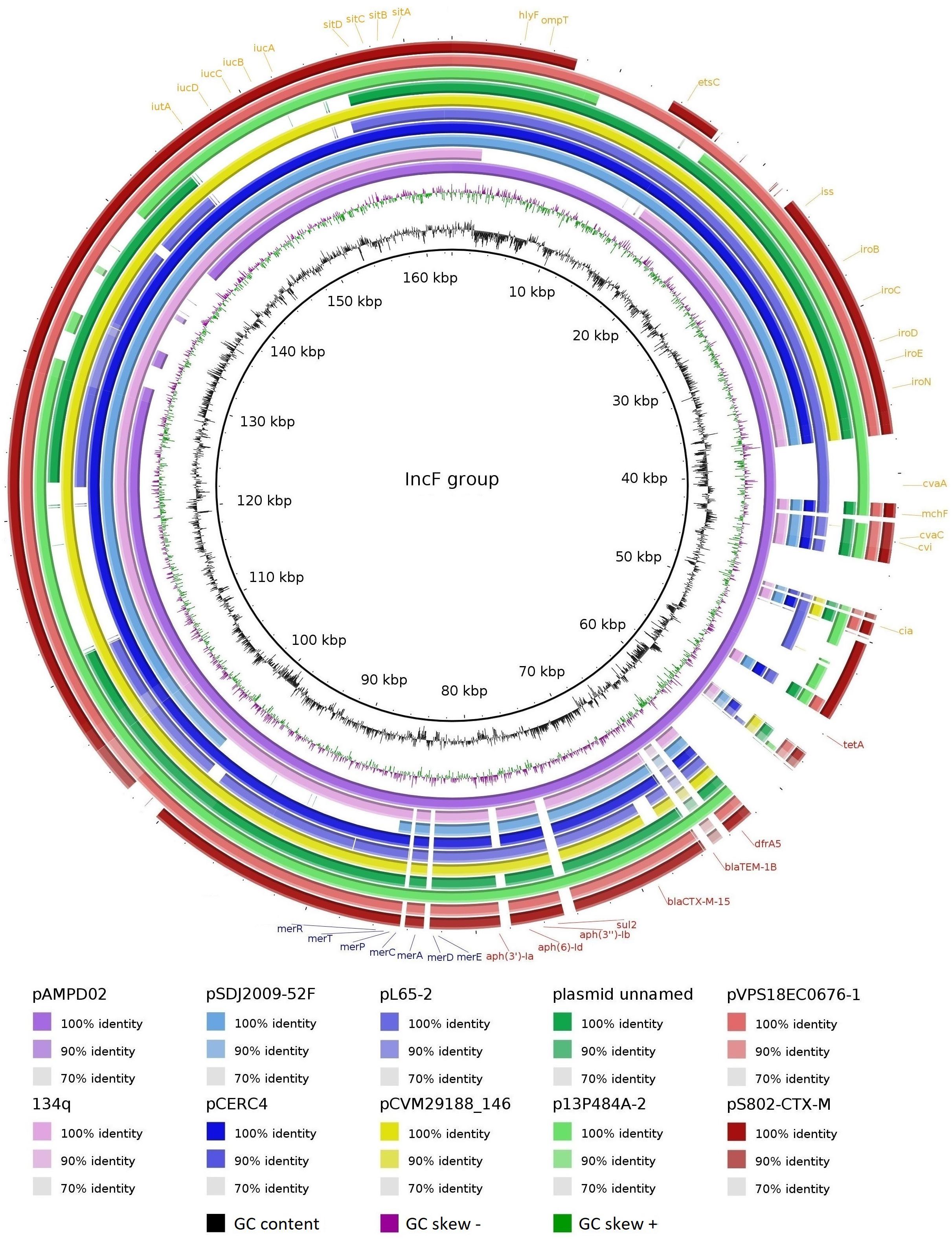
Figure 5. Comparison between IncF plasmids. Plasmids of E. coli strains: pAMPD02 (GenBank: CP058310.1) from a giant panda in China; 134q (GenBank: CP023363.1) from a canine in Scotland; pSDJ2009-52F (GenBank: MH195200.1) and pCERC4 (GenBank: KU578032.1) from a human in Australia; plasmid unnamed (GenBank: CP027485.1) from a human in the United States; pL65-2 (GenBank: CP034739.1) from a goose in China; p13P484A-2 (GenBank: CP019282.1) from a pig in China; pVPS18EC0676-1 (GenBank: CP063726.1) from veal in the United States; pS802-CTX-M (GenBank: MW495061.1) from soil in Brazil. Plasmid of Salmonella Kentucky: pCVM29188_146 (GenBank: CP001122.1) from poultry in the United States. Matches with less than 70% identity and no matches appear as blank spaces. Resistance genes to antimicrobials and metals are indicated in red and blue, respectively, whereas virulence genes are indicated in orange.
Additionally, pS802-MCR and pS802-CTX-M harbored genes involved in the replication (rep genes), partition/maintenance (par genes), conjugation (tra, trb, trh operons), toxin-antitoxin systems (higB/higA, hipB/hipA, hok/sok, relE/parE), and inhibition of SOS response (psiAB). Conjugation assays confirmed transfer of pS802-MCR and pS802-CTX-M from E. coli S802 at frequencies of 4.25 × 10–6 and 5.32 × 10–3 transconjugants/receptor, respectively.
The pS802-QnrB was a small 3,041 bp ColE1-like plasmid, containing 52.09% GC and only the qnrB19 and ΔpspF (truncated transcription activator) genes (Table 2). The qnrB19 gene was located in the conserved genetic context between the sequence encoding the regulatory RNAII and the Xer-mediated recombination site. The pS802-QnrB plasmid was related to other ColE1-like plasmids of Enterobacterales isolated worldwide at the human-animal-environment interface and shared 70% query coverage and ∼99.5% nucleotide identity with plasmids of the same incompatibility group of E. coli strains isolated from poultry in Brazil (GenBank accession numbers: KX452393.1 and KX452394.1), similarly to the IncHI2-IncQ1 plasmid in this study.
Discussion
The emergence of clinically relevant bacterial strains in soils is an underestimated public and environmental health problem that requires attention. In this regard, mcr-positive E. coli lineages from farming soil and agricultural soil have been previously reported in China (Zheng et al., 2017) and Algeria (Touati et al., 2020), respectively. In Brazil, mcr-type genes from soil samples have only been detected in total DNA or cultivable microbiota so far (Oliveira et al., 2019; Furlan et al., 2020b; Dos Santos et al., 2020). In this study, we report for the first time the presence of an mcr-1-positive E. coli isolated from the soil ecosystem in American countries, representing a potential risk of human exposure to antimicrobial-resistant bacteria.
E. coli belonging to the ST131 pandemic high-risk clone has been identified in human, animal, environmental, and food samples (Figure 1). In addition, E. coli ST131 has been frequently reported carrying clinically significant antimicrobial resistance genes, such as mcr-types and/or ESBL genes (Rodrigues et al., 2017; Reid et al., 2019), and associated with extraintestinal diseases, mainly bloodstream and urinary tract infections (Liu et al., 2018; Mamani et al., 2019; Reid et al., 2019).
Whereas E. coli ST131-H30 is the most prevalent sublineage causing extraintestinal infections in humans (Dahbi et al., 2014; Matsumura et al., 2015; Mamani et al., 2019), ST131-H22 predominates in animals, contaminating associated meat products, and can be transmitted zoonotically (Liu et al., 2018; Roer et al., 2019; Saidenberg et al., 2020). Findings from our phylogenetic analysis showed that avian, swine, and human ST131-H22 strains were closely related, supporting results from previous studies (Liu et al., 2018; Reid et al., 2019; Roer et al., 2019; Saidenberg et al., 2020), and also included our environmental strain in the same cluster as those strains (Figure 2), highlighting their transmission at the human-animal-environment interface.
Notably, IncF [F1:A2:B20] plasmids without blaCTX–M–15, the most clinically relevant ESBL gene worldwide (Bevan et al., 2017), and IncF [F2:A1:B-] plasmids with this gene are the most frequently associated with the C1 and C2 subclades of ST131, respectively (Johnson et al., 2016; Pitout and DeVinney, 2017). In contrast, IncF [F2:A-:B1] without blaCTX–M–15 is commonly detected in clade B (Reid et al., 2019; Flament-Simon et al., 2020). Interestingly, we reported the presence of blaCTX–M–15 in clade B of ST131 in this study (Figure 1). Analysis of pS802-CTX-M, an IncF [F2:A-:B1] plasmid, revealed that the ISEcp1-blaCTX–M–15-Δorf477 transposition unit was inserted in a truncated Tn2 transposon, highlighting the role of the insertion sequence ISEcp1 for the mobilization of blaCTX–M–15 onto plasmids (Dhanji et al., 2011; Zong et al., 2015).
Additionally, pS802-CTX-M harbored the ColV region, frequently identified in avian pathogenic E. coli (APEC) and associated with increased fitness and virulence of these strains (Johnson et al., 2006). The presence of ColV plasmid in E. coli strains isolated from humans can indicate evidence of zoonotic transmission (Rodriguez-Siek et al., 2005; Liu et al., 2018). As detected in the present study, ColV plasmids can also carry multiple antimicrobial resistance genes, which is clinically relevant due to the combination of virulence and resistance determinants in a single mobile genetic element (Flament-Simon et al., 2020).
Although the origin of the E. coli ST131-H22 high-risk sublineage carrying the mcr-1.1, blaCTX–M–15, and qnrB19 genes was not investigated, cow manure used for soil fertilization was the most likely source. In addition, other animal (e.g., wild animal feces), human (e.g., sewage), and environmental (e.g., contaminated irrigation water) sources could be involved in the dissemination of clinically relevant bacterial strains (Beuchat, 2002; Cantas et al., 2013; Araújo et al., 2017).
The range of hosts and sources of the E. coli ST131-H22 sublineage, including soil detected here, supports a genetic versatility and adaptation mediated by the gene content, which includes genes encoding resistance to antimicrobials, biocides, and heavy metals. In fact, the plasmids pS802-MCR and pS802-CTX-M co-harboring resistance genes to antimicrobials, biocides, and heavy metals were identified (Figures 3–5). In this regard, heavy metals could come from sources such as contaminated irrigation water, inorganic fertilizers, and pesticides commonly used in agricultural practices, remaining in the environment for long periods (Gimeno-Garcia et al., 1996; Sipter et al., 2008; Osaili et al., 2016; Bhilwadikar et al., 2019). Consequently, these compounds, as well as biocides, may act as selectors of strains resistant to antimicrobials.
Finally, the presence of MDR pathogens displaying a broad resistome in agricultural soil could lead to contamination of vegetables and, since these foods are usually consumed raw, the risk of human exposure to antimicrobial-resistant bacteria with clinical interest increases (Reid et al., 2020; Lopes et al., 2021). Although ingestion of these bacteria may not immediately have a direct impact on health, colonization by this pathway can contribute to the horizontal gene transfer of antimicrobial resistance to the gut microbiome (Maeusli et al., 2020). Thereafter, a potential threat to human health would be associated with future endogenous infections, mainly in immunosuppressed patients, in whom therapeutic failure could occur.
Conclusion
The emergence of zoonosis-associated E. coli ST131-H22 carrying a broad resistome, including mcr-1.1, blaCTX–M–15, and qnrB19, in agricultural soil represents a potential risk of human and animal exposure to antimicrobial-resistant bacteria and/or their resistance genes, posing a threat to public and environmental health. Also considering the possible contamination of vegetables for consumption from soil pathogens, appropriate measures, such as the improvement of agricultural practices, in addition to stricter regulations, need to be taken. Therefore, a One Health approach is required to effectively limit the spread of MDR bacteria and prevent their health impacts.
Data Availability Statement
The datasets presented in this study can be found in online repositories. The names of the repository/repositories and accession number(s) can be found below: https://www. ncbi.nlm.nih.gov/genbank/, JAENHI000000000.1, https://www. ncbi.nlm.nih.gov/genbank/, MW495059.1, https://www.ncbi.nlm.nih.gov/genbank/, MW495060.1, https://www.ncbi.nlm.nih.gov/genbank/, MW495061.1.
Author Contributions
RL, JF, LS, and IG carried out the research. RL and JF performed data curation and formal analysis. RL, JF, and ES conceived and designed the study, and reviewed and edited the manuscript. RL drafted the original manuscript. ES coordinated and acquired funding for the study. All authors read and approved the final manuscript.
Funding
This study was supported by the Coordenação de Aperfeiçoamento de Pessoal de Nível Superior (CAPES) (Grant Nos. 88887.464733/2019-00 and 88882.180855/2018-01, Finance code 001) and the Fundação de Amparo à Pesquisa do Estado de São Paulo (FAPESP) (Grant Nos. 2018/01890-3 and 2018/19539-0).
Conflict of Interest
The authors declare that the research was conducted in the absence of any commercial or financial relationships that could be construed as a potential conflict of interest.
The reviewer MD declared a shared affiliation with the authors to the handling editor at the time of review.
Footnotes
- ^ www.eucast.org
- ^ http://www.bioinformatics.babraham.ac.uk/projects/fastqc/
- ^ http://clermontyping.iame-research.center/
- ^ https://enterobase.warwick.ac.uk/species/index/ecoli
References
Antipov, D., Hartwick, N., Shen, M., Raiko, M., Lapidus, A., and Pevzner, P. A. (2016). plasmidSPAdes: assembling plasmids from whole genome sequencing data. Bioinformatics 32, 3380–3387. doi: 10.1093/bioinformatics/btw493
Araújo, S., Silva, I. A. T., Tacão, M., Patinha, C., Alves, A., and Henriques, I. (2017). Characterization of antibiotic resistant and pathogenic Escherichia coli in irrigation water and vegetables in household farms. Int. J. Food Microbiol. 257, 192–200. doi: 10.1016/j.ijfoodmicro.2017.06.020
Aziz, R. K., Bartels, D., Best, A. A., DeJongh, M., Disz, T., Edwards, R. A., et al. (2008). The RAST Server: rapid annotations using subsystems technology. BMC Genomics 9:75. doi: 10.1186/1471-2164-9-75
Bankevich, A., Nurk, S., Antipov, D., Gurevich, A. A., Dvorkin, M., Kulikov, A. S., et al. (2012). SPAdes: a new genome assembly algorithm and its applications to single-cell sequencing. J. Comput. Biol. 19, 455–477. doi: 10.1089/cmb.2012.0021
Beuchat, L. R. (2002). Ecological factors influencing survival and growth of human pathogens on raw fruits and vegetables. Microbes Infect. 4, 413–423. doi: 10.1016/s1286-4579(02)01555-1
Bevan, E. R., Jones, A. M., and Hawkey, P. M. (2017). Global epidemiology of CTX-M β-lactamases: temporal and geographical shifts in genotype. J. Antimicrob. Chemother. 72, 2145–2155. doi: 10.1093/jac/dkx146
Bhilwadikar, T., Pounraj, S., Manivannan, S., Rastogi, N. K., and Negi, P. S. (2019). Decontamination of microorganisms and pesticides from fresh fruits and vegetables: a comprehensive review from common household processes to modern techniques. Compr. Rev. Food Sci. Food Saf. 18, 1003–1038. doi: 10.1111/1541-4337.12453
Bolger, A. M., Lohse, M., and Usadel, B. (2014). Trimmomatic: a flexible trimmer for Illumina sequence data. Bioinformatics. 30, 2114–2120. doi: 10.1093/bioinformatics/btu170
Borowiak, M., Fischer, J., Hammerl, J. A., Hendriksen, R. S., Szabo, I., and Malorny, B. (2017). Identification of a novel transposon-associated phosphoethanolamine transferase gene, mcr-5, conferring colistin resistance in d-tartrate fermenting Salmonella enterica subsp. enterica serovar Paratyphi B. J. Antimicrob. Chemother. 72, 3317–3324. doi: 10.1093/jac/dkx327
Cantas, L., Shah, S. Q., Cavaco, L. M., Manaia, C. M., Walsh, F., Popowska, M., et al. (2013). A brief multi-disciplinary review on antimicrobial resistance in medicine and its linkage to the global environmental microbiota. Front. Microbiol. 4:96. doi: 10.3389/fmicb.2013.00096
Carattoli, A., Villa, L., Feudi, C., Curcio, L., Orsini, S., Luppi, A., et al. (2017). Novel plasmid-mediated colistin resistance mcr-4 gene in Salmonella and Escherichia coli, Italy 2013, Spain and Belgium, 2015 to 2016. Euro Surveill. 22, 30589. doi: 10.2807/1560-7917.ES.2017.22.31.30589
Carattoli, A., Zankari, E., Garcia-Fernandez, A., Voldby Larsen, M., Lund, O., Villa, L., et al. (2014). In silico detection and typing of plasmids using PlasmidFinder and plasmid multilocus sequence typing. Antimicrob. Agents Chemother. 58, 3895–3903. doi: 10.1128/AAC.02412-14
Chen, L., Yang, J., Yu, J., Yao, Z., Sun, L., Shen, Y., et al. (2005). VFDB: a reference database for bacterial virulence factors. Nucleic Acids Res. 33, D325–D328. doi: 10.1093/nar/gki008
CLSI (Clinical and Laboratory Standards Institute). (2020). Performance standards for antimicrobial susceptibility testing. CLSI Supplement M100, 30th Edn. Wayne, PA: Clinical and Laboratory Standards Institute.
Dahbi, G., Mora, A., Mamani, R., Lopez, C., Alonso, M. P., Marzoa, J., et al. (2014). Molecular epidemiology and virulence of Escherichia coli O16:H5-ST131: comparison with H30 and H30-Rx subclones of O25b:H4-ST131. Int. J. Med. Microbiol. 304, 1247–1257. doi: 10.1016/j.ijmm.2014.10.002
Dallenne, C., Da Costa, A., Decre, D., Favier, C., and Arlet, G. (2010). Development of a set of multiplex PCR assays for the detection of genes encoding important β-lactamases in Enterobacteriaceae. J. Antimicrob. Chemother. 65, 490–495. doi: 10.1093/jac/dkp498
Dhanji, H., Patel, R., Wall, R., Doumith, M., Patel, B., Hope, R., et al. (2011). Variation in the genetic environments of blaCTX–M–15 in Escherichia coli from the faeces of travellers returning to the United Kingdom. J. Antimicrob. Chemother. 66, 1005–1012. doi: 10.1093/jac/dkr041
Dos Santos, L. D. R., Furlan, J. P. R., Ramos, M. S., Gallo, I. F. L., de Freitas, L. V. P., and Stehling, E. G. (2020). Co-occurrence of mcr-1, mcr-3, mcr-7 and clinically relevant antimicrobial resistance genes in environmental and fecal samples. Arch. Microbiol. 202, 1795–1800. doi: 10.1007/s00203-020-01890-3
Flament-Simon, S. C., de Toro, M., Mora, A., García, V., García-Meniño, I., Díaz-Jiménez, D., et al. (2020). Whole genome sequencing and characteristics of mcr-1-harboring plasmids of porcine Escherichia coli isolates belonging to the high-risk clone O25b:H4-ST131 clade B. Front. Microbiol. 11:387. doi: 10.3389/fmicb.2020.00387
Furlan, J. P. R., dos Santos, L. D. R., Ramos, M. S., Gallo, I. F. L., and Stehling, E. G. (2020a). Presence of colistin resistance mcr-4 gene and clinically relevant antimicrobial resistance genes in sand samples from a public beach. Water Air Soil Pollut. 231, 321. doi: 10.1007/s11270-020-04707-7
Furlan, J. P. R., Lopes, R., Gonzalez, I. H. L., Ramos, P. L., von Zeska Kress, M. R., and Stehling, E. G. (2020b). Hypermucoviscous/hypervirulent and extensively drug-resistant QnrB2-, QnrS1-, and CTX-M-3-coproducing Klebsiella pneumoniae ST2121 isolated from an infected elephant (Loxodonta africana). Vet. Microbiol. 251, 108909. doi: 10.1016/j.vetmic.2020.108909
Gimeno-Garcia, E., Andreu, V., and Boluda, R. (1996). Heavy metals incidence in the application of inorganic fertilizers and pesticides to rice farming soils. Environ. Pollut. 92, 19–25. doi: 10.1016/0269-7491(95)00090-9
Gupta, S. K., Padmanabhan, B. R., Diene, S. M., Lopez-Rojas, R., Kempf, M., Landraud, L., et al. (2014). ARG-annot, a new bioinformatic tool to discover antibiotic resistance genes in bacterial genomes. Antimicrob. Agents Chemother. 58, 212–220. doi: 10.1128/AAC.01310-13
Hassan, J., and Kassem, I. I. (2020). Audacious hitchhikers: the role of travel and the international food trade in the global dissemination of mobile colistin-resistance (mcr) genes. Antibiotics (Basel) 9, 370. doi: 10.3390/antibiotics9070370
Jarlier, V., Nicolas, M. H., Fournier, G., and Philippon, A. (1988). Extended broad-spectrum beta-lactamases conferring transferable resistance to newer beta-lactam agents in Enterobacteriaceae: hospital prevalence and susceptibility patterns. Rev. Infect. Dis. 10, 867–878. doi: 10.1093/clinids/10.4.867
Joensen, K. G., Scheutz, F., Lund, O., Hasman, H., Kaas, R. S., Nielsen, E. M., et al. (2014). Real-time whole-genome sequencing for routine typing, surveillance, and outbreak detection of verotoxigenic Escherichia coli. J. Clin. Microbiol. 52, 1501–1510. doi: 10.1128/JCM.03617-13
Joensen, K. G., Tetzschner, A. M., Iguchi, A., Aarestrup, F. M., and Scheutz, F. (2015). Rapid and easy in silico serotyping of Escherichia coli isolates by use of whole-genome sequencing data. J. Clin. Microbiol. 53, 2410–2426. doi: 10.1128/JCM.00008-15
Johnson, T. J., Danzeisen, J. L., Youmans, B., Case, K., Llop, K., Munoz-Aguayo, J., et al. (2016). Separate F-Type plasmids have shaped the evolution of the H30 subclone of Escherichia coli sequence type 131. mSphere 1, e121–e116. doi: 10.1128/mSphere.00121-16
Johnson, T. J., Johnson, S. J., and Nolan, L. K. (2006). Complete DNA sequence of a ColBM plasmid from avian pathogenic Escherichia coli suggests that it evolved from closely related ColV virulence plasmids. J. Bacteriol. 188, 5975–5983. doi: 10.1128/JB.00204-06
Johura, F. T., Tasnim, J., Barman, I., Biswas, S. R., Jubyda, F. T., Sultana, M., et al. (2020). Colistin-resistant Escherichia coli carrying mcr-1 in food, water, hand rinse, and healthy human gut in Bangladesh. Gut Pathog. 12, 5. doi: 10.1186/s13099-020-0345-2
Larsen, M. V., Cosentino, S., Rasmussen, S., Friis, C., Hasman, H., Marvig, R. L., et al. (2012). Multilocus sequence typing of total-genome-sequenced bacteria. J. Clin. Microbiol. 50, 1355–1361. doi: 10.1128/JCM.06094-11
Letunic, I., and Bork, P. (2019). Interactive Tree Of Life (iTOL) v4: recent updates and new developments. Nucleic Acids Res. 47, W256–W259. doi: 10.1093/nar/gkz239
Li, Z., Shi, R., Wu, H., and Yan, P. (2021). First identification of a patient with prosthesis-related infection caused by an mcr-1.1-producing ST131 Escherichia coli after rhinoplasty. Infect. Drug Resist. 14, 249–257. doi: 10.2147/IDR.S295801
Liu, C. M., Stegger, M., Aziz, M., Johnson, T. J., Waits, K., Nordstrom, L., et al. (2018). Escherichia coli ST131-H22 as a foodborne uropathogen. mBio 9, e470–e418. doi: 10.1128/mBio.00470-18 ∗∗∗e00470-18
Liu, Y. Y., Wang, Y., Walsh, T. R., Yi, L. X., Zhang, R., Spencer, J., et al. (2016). Emergence of plasmid-mediated colistin resistance mechanism mcr-1 in animals and human beings in china: A microbiological and molecular biological study. Lancet Infect. Dis. 16, 161–168. doi: 10.1016/S1473-3099(15)00424-7
Lopes, R., Cerdeira, L. T., Fernandes, M. R., Perez-Chaparro, P. J., McCulloch, J. A., and Lincopan, N. (2017). Draft genome sequence of a CTX-M-15-producing endophytic Klebsiella pneumoniae ST198 isolate from commercial lettuce. J. Glob. Antimicrob. Resist. 10, 19–20. doi: 10.1016/j.jgar.2017.03.005
Lopes, R., Fuentes-Castillo, D., Fontana, H., Rodrigues, L., Dantas, K., Cerdeira, L., et al. (2021). Endophytic lifestyle of global clones of extended-spectrum β-lactamase producing priority pathogens in fresh vegetables: a Trojan horse strategy favoring human colonization? mSystems 6, e1125–e1120. doi: 10.1128/mSystems.01125-20
Maeusli, M., Lee, B., Miller, S., Reyna, Z., Lu, P., Yan, J., et al. (2020). Horizontal gene transfer of antibiotic resistance from Acinetobacter baylyi to Escherichia coli on lettuce and subsequent antibiotic resistance transmission to the gut microbiome. mSphere 5, e329–e320. doi: 10.1128/mSphere.00329-20
Magiorakos, A. P., Srinivasan, A., Carey, R. B., Carmeli, Y., Falagas, M. E., Giske, C. G., et al. (2012). Multidrug-resistant, extensively drug-resistant and pandrug-resistant bacteria: an international expert proposal for interim standard definitions for acquired resistance. Clin. Microbiol. Infect. 18, 268–281. doi: 10.1111/j.1469-0691.2011.03570.x
Mamani, R., Flament-Simon, S. C., Garcia, V., Mora, A., Alonso, M. P., Lopez, C., et al. (2019). Sequence types, clonotypes, serotypes, and virotypes of extended-spectrum beta-lactamase-producing Escherichia coli causing bacteraemia in a Spanish hospital over a 12-year period (2000 to 2011). Front. Microbiol. 10:1530. doi: 10.3389/fmicb.2019.01530
Matsumura, Y., Johnson, J. R., Yamamoto, M., Nagao, M., Tanaka, M., Takakura, S., et al. (2015). CTX-M-27- and CTX-M-14-producing, ciprofloxacin-resistant Escherichia coli of the H30 subclonal group within ST131 drive a Japanese regional ESBL epidemic. J. Antimicrob. Chemother. 70, 1639–1649. doi: 10.1093/jac/dkv017
Oliveira, C. C., Lopes, E. S., Barbosa, D. R., Pimenta, R. L., Sobrinho, N. M. B. A., Coelho, S. M. O., et al. (2019). Occurrence of the colistin resistance mcr-1 gene in soils from intensive vegetable production and native vegetation. Eur. J. Soil Sci. 70, 876–881. doi: 10.1111/ejss.12832
Osaili, T. M., Al Jamali, A. F., Makhadmeh, I. M., Taha, M., and Jarrar, S. K. (2016). Heavy metals in vegetables sold in the local market in Jordan. Food Addit. Contam. Part B Surveill. 9, 223–229. doi: 10.1080/19393210.2016.1181675
Pal, C., Bengtsson-Palme, J., Rensing, C., Kristiansson, E., and Larsson, D. G. J. (2014). BacMet: antibacterial biocide and metal resistance genes database. Nucleic Acids Res. 42, D737–D743. doi: 10.1093/nar/gkt1252
Perez, F., El Chakhtoura, N. G., Papp-Wallace, K. M., Wilson, B. M., and Bonomo, R. A. (2016). Treatment options for infections caused by carbapenem-resistant Enterobacteriaceae: Can we apply “precision medicine” to antimicrobial chemotherapy? Expert Opin. Pharmacother. 17, 761–781. doi: 10.1517/14656566.2016.1145658
Petty, N. K., Ben Zakour, N. L., Stanton-Cook, M., Skippington, E., Totsika, M., Forde, B. M., et al. (2014). Global dissemination of a multidrug resistant Escherichia coli clone. Proc. Natl. Acad. Sci. U.S.A 111, 5694–5699. doi: 10.1073/pnas.1322678111
Pitout, J. D., and DeVinney, R. (2017). Escherichia coli ST131: a multidrug-resistant clone primed for global domination. F1000Research 6, F1000FacultyRev–195. doi: 10.12688/f1000research.10609.1
Reid, C. J., Blau, K., Jechalke, S., Smalla, K., and Djordjevic, S. P. (2020). Whole genome sequencing of Escherichia coli from store-bought produce. Front. Microbiol. 10:3050. doi: 10.3389/fmicb.2019.03050
Reid, C. J., McKinnon, J., and Djordjevic, S. P. (2019). Clonal ST131-H22 Escherichia coli strains from a healthy pig and a human urinary tract infection carry highly similar resistance and virulence plasmids. Microb. Genom. 5, e000295. doi: 10.1099/mgen.0.000295
Rodrigues, C., Machado, E., Fernandes, S., Peixe, L., and Novais, Â (2017). Different Escherichia coli B2-ST131 clades (B and C) producing extended-spectrum β-lactamases (ESBL) colonizing residents of Portuguese nursing homes. Epidemiol, Infect 145, 3303–3306. doi: 10.1017/S0950268817002266
Rodriguez-Siek, K. E., Giddings, C. W., Doetkott, C., Johnson, T. J., Fakhr, M. K., and Nolan, L. K. (2005). Comparison of Escherichia coli isolates implicated in human urinary tract infection and avian colibacillosis. Microbiology 151, 2097–2110. doi: 10.1099/mic.0.27499-0
Roer, L., Johannesen, T. B., Hansen, F., Stegger, M., Tchesnokova, V., Sokurenko, E., et al. (2018). CHTyper, a web tool for subtyping of extraintestinal pathogenic Escherichia coli based on the fumC and fimH alleles. J. Clin. Microbiol. 56, e63–e18. doi: 10.1128/JCM.00063-18
Roer, L., Overballe-Petersen, S., Hansen, F., Johannesen, T. B., Stegger, M., Bortolaia, V., et al. (2019). ST131 fimH22 Escherichia coli isolate with a blaCMY–2/IncI1/ST12 plasmid obtained from a patient with bloodstream infection: highly similar to E. coli isolates of broiler origin. J. Antimicrob. Chemother. 74, 557–560. doi: 10.1093/jac/dky484
Roer, L., Tchesnokova, V., Allesøe, R., Muradova, M., Chattopadhyay, S., Ahrenfeldt, J., et al. (2017). Development of a web tool for Escherichia coli subtyping based on fimH alleles. J. Clin. Microbiol. 55, 2538–2543. doi: 10.1128/JCM.00737-17
Saidenberg, A. B. S., Stegger, M., Price, L. B., Johannesen, T. B., Aziz, M., Cunha, M. P. V., et al. (2020). mcr-positive Escherichia coli ST131-H22 from poultry in Brazil. Emerg. Infect. Dis. 26, 1951–1954. doi: 10.3201/eid2608.191724
Seemann, T. (2014). Prokka: rapid prokaryotic genome annotation. Bioinformatics 30, 2068–2069. doi: 10.1093/bioinformatics/btu153
Sipter, E., Rózsa, E., Gruiz, K., Tátrai, E., and Morvai, V. (2008). Site-specific risk assessment in contaminated vegetable gardens. Chemosphere 71, 1301–1307. doi: 10.1016/j.chemosphere.2007.11.039
Stoesser, N., Sheppard, A. E., Pankhurst, L., De Maio, N., Moore, C. E., Sebra, R., et al. (2016). Evolutionary history of the global emergence of the Escherichia coli epidemic clone ST131. mBio 7, e02162. doi: 10.1128/mBio.02162-15
Touati, M., Hadjadj, L., Berrazeg, M., Baron, S. A., and Rolain, J. M. (2020). Emergence of Escherichia coli harbouring mcr-1 and mcr-3 genes in North West Algerian farmlands. J. Glob. Antimicrob. Resist. 21, 132–137. doi: 10.1016/j.jgar.2019.10.001
Tsuji, B. T., Pogue, J. M., Zavascki, A. P., Paul, M., Daikos, G. L., Forrest, A., et al. (2019). International consensus guidelines for the optimal use of the polymyxins: Endorsed by the American College of Clinical Pharmacy (ACCP), European Society of Clinical Microbiology and Infectious Diseases (ESCMID), Infectious Diseases Society of America (IDSA), International Society for Anti-infective Pharmacology (ISAP), Society of Critical Care Medicine (SCCM), and Society of Infectious Diseases Pharmacists (SIDP). Pharmacotherapy 39, 10–39. doi: 10.1002/phar.2209
Wang, X., Wang, Y., Zhou, Y., Wang, Z., Wang, Y., Zhang, S., et al. (2019). Emergence of colistin resistance gene mcr-8 and its variant in Raoultella ornithinolytica. Front. Microbiol. 10:228. doi: 10.3389/fmicb.2019.00228
Weisburg, W. G., Barns, S. M., Pelletier, D. A., and Lane, D. J. (1991). 16S ribosomal DNA amplification for phylogenetic study. J. Bacteriol. 173, 697–703. doi: 10.1128/jb.173.2.697-703.1991
Xavier, B. B., Lammens, C., Ruhal, R., Kumar-Singh, S., Butaye, P., Goossens, H., et al. (2016). Identification of a novel plasmid-mediated colistin-resistance gene, mcr-2, in Escherichia coli, Belgium, June 2016. Euro Surveill. 21, 27. doi: 10.2807/1560-7917.ES.2016.21.27.30280
Yang, Y. Q., Li, Y. X., Lei, C. W., Zhang, A. Y., and Wang, H. N. (2018). Novel plasmid-mediated colistin resistance gene mcr-7.1 in Klebsiella pneumoniae. J. Antimicrob. Chemother. 73, 1791–1795. doi: 10.1093/jac/dky111
Yin, W., Li, H., Shen, Y., Liu, Z., Wang, S., Shen, Z., et al. (2017). Novel plasmid-mediated colistin resistance gene mcr-3 in Escherichia coli. mBio 8, e543–e517. doi: 10.1128/mBio.00543-17
Zankari, E., Hasman, H., Cosentino, S., Vestergaard, M., Rasmussen, S., Lund, O., et al. (2012). Identification of acquired antimicrobial resistance genes. J. Antimicrob. Chemother. 67, 2640–2644. doi: 10.1093/jac/dks261
Zheng, B., Huang, C., Xu, H., Guo, L., Zhang, J., Wang, X., et al. (2017). Occurrence and genomic characterization of ESBL-producing, mcr-1-harboring Escherichia coli in farming soil. Front. Microbiol. 8:8510. doi: 10.3389/fmicb.2017.02510
Keywords: acquired polymyxin resistance, emerging zoonotic E. coli, extended-spectrum β-lactamase, food and environmental safety, genomic surveillance, mcr-1, multidrug-resistant, One Health
Citation: Lopes R, Furlan JPR, dos Santos LDR, Gallo IFL and Stehling EG (2021) Colistin-Resistant mcr-1-Positive Escherichia coli ST131-H22 Carrying blaCTX–M–15 and qnrB19 in Agricultural Soil. Front. Microbiol. 12:659900. doi: 10.3389/fmicb.2021.659900
Received: 28 January 2021; Accepted: 15 March 2021;
Published: 09 April 2021.
Edited by:
Maria Jorge Campos, Polytechnic Institute of Leiria, PortugalReviewed by:
Torahiko Okubo, Hokkaido University, JapanSteven Philip Djordjevic, University of Technology Sydney, Australia
Milena Dropa, University of São Paulo, Brazil
Copyright © 2021 Lopes, Furlan, dos Santos, Gallo and Stehling. This is an open-access article distributed under the terms of the Creative Commons Attribution License (CC BY). The use, distribution or reproduction in other forums is permitted, provided the original author(s) and the copyright owner(s) are credited and that the original publication in this journal is cited, in accordance with accepted academic practice. No use, distribution or reproduction is permitted which does not comply with these terms.
*Correspondence: Eliana Guedes Stehling, ZWxpYW5hZ3NAdXNwLmJy