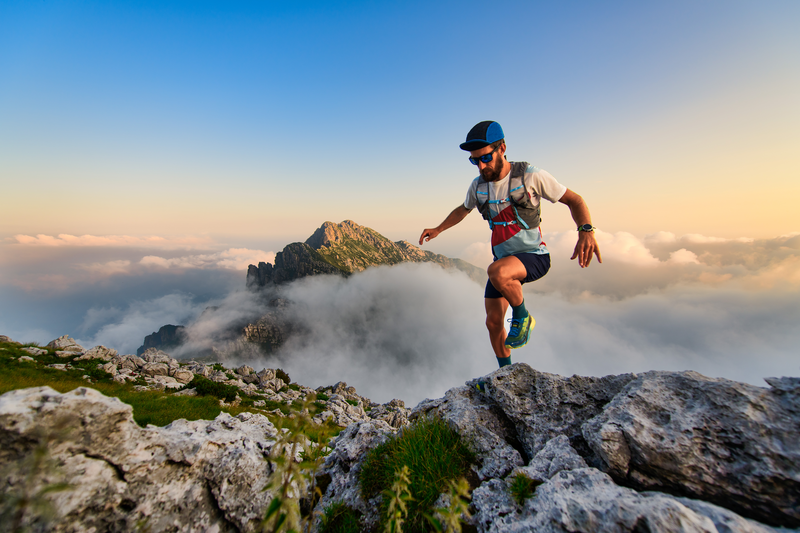
95% of researchers rate our articles as excellent or good
Learn more about the work of our research integrity team to safeguard the quality of each article we publish.
Find out more
ORIGINAL RESEARCH article
Front. Microbiol. , 29 April 2021
Sec. Antimicrobials, Resistance and Chemotherapy
Volume 12 - 2021 | https://doi.org/10.3389/fmicb.2021.655242
This article is part of the Research Topic Worldwide Emergence of Drug Resistant Fungi: from Basic to Clinic View all 24 articles
Resistance caused by the formation of the Candida albicans (C. albicans) biofilm is one of the main reasons for antifungal therapy failure. Thus, it is important to find indicators that predict C. albicans biofilm formation to provide evidence for the early prevention and treatment of the C. albicans biofilms. In this study, C. albicans samples were selected from C. albicans septicemia that were sensitive to common antifungal agents. It was found that the agglutinin-like sequence 3 (ALS3) gene was differentially expressed in free, antifungal, drug-sensitive C. albicans. The average ALS3 gene expression was higher in the C. albicans strains with biofilm formation than that in the C. albicans strains without biofilm formation. Then, it was further confirmed that the rate of biofilm formation was higher in the high ALS3 gene expression group than that in the low ALS3 gene expression group. It was found that C. albicans with biofilm formation was more resistant to fluconazole, voriconazole, and itraconazole. However, it maintained its sensitivity to caspofungin and micafungin in vitro and in mice. Further experiments regarding the prevention of C. albicans biofilm formation were performed in mice, in which only caspofungin and micafungin prevented C. albicans biofilm formation. These results suggest that the expression level of ALS3 in C. albicans may be used as an indicator to determine whether C. albicans will form biofilms. The results also show that the biofilm formation of C. albicans remained sensitive to caspofungin and micafungin, which may help to guide the selection of clinical antifungal agents for prevention and therapy.
Infection is one of the most common serious complications associated with the use of medical devices retained in the body, particularly central venous catheters. As a major cause of catheter-related bloodstream infections, Candida albicans (C. albicans) has the propensity to form biofilms. Compared with its planktonic form, the biofilm formation of C. albicans is up to 1,000 times more resistant to azole antifungal agents (Lamfon, 2004) and up to 20 times more resistant to echinocandins (Nett et al., 2010; Tobudic et al., 2010; Taff et al., 2013). Because of its ability to form biofilms, the treatment of C. albicans catheter-related infections is challenging (Walraven and Lee, 2013). Recent evidence suggested that even when the minimal inhibitory concentration (MIC50) increases, C. albicans biofilm formation remains sensitive to echinocandins (Ramage et al., 2005; Choi et al., 2007; Katragkou et al., 2008).
It is important to identify which C. albicans strains have the tendency to form biofilms. A goal of this study was to find some indicators that could predict the biofilm formation of C. albicans, which may provide a basis for the early prevention and treatment of C. albicans biofilm formation. In previous studies (Deng et al., 2016, 2017), C. albicans was resistant to fluconazole, voriconazole, and itraconazole after biofilm formation. The MIC50 of caspofungin and micafungin to 50% of the tested C. albicans biofilm increased to different degrees, but they did not reach drug resistance. Further studies found that there were differences in the expression levels of genes in the ALS gene family, particularly ALS3, between C. albicans strains before biofilm formation. C. albicans with higher ALS3 expression levels tended to form biofilms.
This study selected sensitive C. albicans that were isolated from blood samples of C. albicans septicemia to investigate the correlation between ALS3 expression and C. albicans biofilm formation and resistance. Then, an in vivo experiment to study the prevention of C. albicans biofilm formation in mice was performed.
A total of 55 strains of C. albicans isolated from the blood samples of 55 patients with C. albicans septicemia who were admitted to the hospital from January 2017 to December 2018 were collected. The patients had not received antifungal therapy before the diagnosis of C. albicans septicemia and had not received catheter therapy. All C. albicans strains were sensitive to common antifungal agents.
A small number of clinically isolated C. albicans was selected via inoculation ring. C. albicans were inoculated on Sabouraud dextrose agar (SDA) using a three-zone scribing method and placed into a temperature box at 37°C overnight. The following day, one of the growing strains was transplanted into 5 mL of yeast peptone glucose (YPG) medium and cultured overnight in a shaking table at a speed of 200 rpm at 35°C. On the third day, the medium was removed from the shaker and centrifuged for 5 min at 3,000 rpm to collect the thalli. The obtained thalli were rinsed with saline solution three times and diluted with RPMI-1640 medium. C. albicans were then subcultured in yeast peptone and glucose medium at 150 rpm and 35°C in a shaking table overnight (Deng et al., 2016, 2017). The concentration of C. albicans solution was adjusted to 1 × 107 cells/mL.
The susceptibilities of C. albicans strains to antifungal agents, including fluconazole (Diflucan, Pfizer Manufacturing Deut.), voriconazole (Vfend, Pfizer Manufacturing Deut.), itraconazole (Sporanox, Xian-Janssen Pharmaceutical Ltd.), caspofungin (Concidas, Merck & Co., Inc.), and micafungin (Mycamine, Astellas Pharma Tech Co., Ltd), were assayed in vitro using a microliquid-based dilution method M27-A3 [Clinical and Laboratory Standards Institute (CLSI), 2008]. The ATCC control used in this study was AT0CC10231.
To detect ALS3 expression levels, total RNA extracted from grinded fungi with TRIzol reagent (Invitrogen, Carlsbad, CA, United States) was used as the template for all reverse transcriptase reactions. The cDNA was synthesized with random priming using 10 μL of total RNA and RevertAid First Strand cDNA Synthesis Kit (Fermentas, CA, United States) following the manufacturer’s protocol. The upstream primer of ALS3 was 5′-CCGGTTTCATCTGAATCATTTAGTT-3′. The downstream primer of ALS3 was 5′-ACGACAAGGTGTACGAATTAACATCT-3′. The upstream primer of the internal gene (ACT1) was 5′-TGGGCCAAAAGGATTCTTATG-3′. The downstream primer of the internal gene was 5′-AGATCTTTTCCATATCATCCCAG-3′. The housekeeping gene fragments of C. albicans consisted of AAT1A, ACC1, ADP1, MPIB, SYA1, VPS13, and ZWF1B (Bougnoux et al., 2003). Quantitative expression of ALS3 levels was conducted by real-time RT-PCR with a LightCycler 96 system (Roche, Switzerland). The amplification consisted of denaturation at 95°C for 30 s (s), annealing at 95°C for 3 s, and extension at 60°C for 30 s (40 cycles). Each reaction was run in triplicate. ALS3 expression was normalized to ACT1. Expression levels of the regulatory gene and ALS3 were determined using the delta-delta Ct (2–△△Ct) method. Ct indicates the average threshold period of the genes obtained in three independent experiments (Escribano et al., 2017). Data are presented as mRNA transcripts (arbitrary units) relative to ACT1 (Hosseini et al., 2019).
All 55 strains C. albicans were added to 12-well culture plates at 2 mL/well. A 10 mm sterile indwelling catheter was placed in each well. The culture plates were incubated in a 150 rpm shaking table at 35°C for 90 min (min). Then, culture plates and catheters were washed three times using aseptic saline and cultured in YPG medium for 72 h (h) at 37°C. Biofilm formation in vitro was completed in the 12-well culture plates (Deng et al., 2016, 2017).
Six-week-old male C57 mice weighing 20.24 g ± 1.78 g (n = 50, Beijing Vitonlihua Experimental Animal Technology Co., Ltd., Beijing, China) were randomly divided into three groups. On the basis of ALS3 expression in C. albicans, mice were grouped into either the high ALS3 expression group (20 mice), low ALS3 expression group (20 mice), or blank control group (10 mice). A 20 mm long sterile indwelling catheter was placed into the abdominal cavity of each mouse. Mice in the two experimental groups received 1 × 107 C. albicans with either high or low ALS3 expression by intraperitoneal injection 48 h later. Mice in the control group did not receive C. albicans injection. In all three groups, catheters were kept in the abdominal cavity.
After feeding under the same conditions for 2 weeks, all the three groups of mice were sacrificed by cervical dislocation. Catheters were taken out, washed three times using saline solution, then cultured in YPG medium for 48 h at 37°C. After catheters were cultured, the growing colonies of C. albicans were isolated. The randomly amplified polymorphic DNA (RAPD) method (Perrone et al., 2009) was used to determine whether C. albicans isolated in vitro were the same as those inoculated in abdominal cavities of the mice. Four arbitrary promoters (LEG2, CDL6s, Leptopatho, and CDL6as) were selected for the RAPD method (Vrioni and Matsiota-Bemard, 2001). Finally, ALS3 expression in C. albicans strains was detected, also we performed a drug sensitivity test.
Six-week-old male C57 mice weighing 21.02 ± 1.12 g (n = 40, Beijing Vitonlihua Experimental Animal Technology Co., Ltd, Beijing, China) were divided into four groups in the prevention of biofilm formation experiment. The mice were divided into the voriconazole group, caspofungin group, micafungin group, and control group, with 10 mice in each group. Ten C. albicans strains with high ALS3 expression were selected for the prevention experiment. A 20 mm long sterile indwelling catheter was placed into the abdominal cavity of each mouse. Ten mice in each group received a dose of 1 × 107 C. albicans by intraperitoneal injection 48 h later. All mice in the experiment groups received preventive therapy consisting of different antifungal agents, which included voriconazole (10 mg/kg per day), caspofungin (1.5 mg/kg per day), and micafungin (1.5 mg/kg per day) in each group. After feeding under the same conditions for 2 weeks, C. albicans were extracted from the in vitro cultures.
SPSS 17.0 (SPSS, Inc., Chicago, IL, United States) statistical software was used for statistical analysis. Data were expressed as the mean ± standard error and analyzed by t-tests and F-tests. Susceptibility tests for antifungal agents were compared using non-parametric tests for independent samples. P < 0.05 was considered a statistically significant difference.
In the biofilm formation experiment, 29 C. albicans strains out of the 55 total strains formed biofilms in vitro. The C. albicans budded and began to form mycelium after 6 h of cell culture in vitro under observation with an inverted microscope. C. albicans colonies fused with each other and arranged into a network after 12 h of culture. C. albicans then clumped along the mycelium formation after 24 h of culture. Finally, the mycelium of C. albicans interlaced and formed a membrane network structure inside the entire catheter after 48 h of culture in vitro.
Cell walls of C. albicans were broken in the 26 strains that did not form biofilms. The thickness of most cell walls was about 110–220 nm. Electron density in the strains was higher in structures with spores that sprouted (Figure 1A). In the 29 strains that formed biofilms in vitro, cell walls were about 200–350 nm. Electron density in these strains and in structures with spores were the same as those that did not form biofilms. However, the strains that formed biofilms were rich in mitochondria (Figure 1B).
Figure 1. Ultrastructure of biofilms. (A) The cell wall of strain that did not form biofilms was about 220 nm. (B) The biofilm formation strains were rich in mitochondria.
There were obvious differences among ALS3 expression in the 55 C. albicans strains after they were collected from patients. The ratio of ALS3 expression to the median of the 55 C. albicans strains is shown in Figure 2.
Figure 2. The expression of ALS3 gene was obvious differences in the 55 strains. There were obvious differences of the ALS3 gene expression level in the 55 C. albicans when they were collected from patients.
Objective stripes were presented at 100–200 bp after electrophoresis of gene amplification products with 2% sepharose gel. The average ALS3 expression was higher in the 29 C. albicans with biofilm formation than that in the 26 C. albicans without biofilm formation (P = 0.000). The average ALS3 expression declined after culture in vitro in the 29 strains with biofilm formation (P = 0.013). However, there were no differences in the 26 strains without biofilm formation before and after culturing (P = 0.167; Figure 3).
Figure 3. The changes of the ALS3 gene expression. The average of ALS3 gene expression was higher in the 29 C. albicans with biofilm formation than that of in the 26 C. albicans without biofilm formation. The average of ALS3 gene expression declined after culture in the 29 C. albicans with biofilm formation. But there was no different in the 26 C. albicans without biofilm formation before and after culture.
During the biofilm formation experiment in mice, two mice in the high ALS3 expression group died on the third and fourth days after the sterile indwelling catheter was placed into the abdominal cavity. No mice died in the low ALS3 expression group or control group. After 2 weeks, the catheters were removed. A total of 14 C. albicans strains (14/18, 77.8%) in the high ALS3 expression group formed biofilms with membrane structures attached to the catheters walls when observed under a microscope.
Three C. albicans strains (3/20, 15%) in the low ALS3 expression group formed biofilms. The rate of biofilm formation in the high ALS3 expression group was higher than that in the low ALS3 expression group (P = 0.000; Figure 4A). After the catheters were cultured, growing colonies of C. albicans were extracted. There were 12 C. albicans strains from catheters of the high ALS3 expression group and eight C. albicans strains from catheters of the low ALS3 expression group that were extracted from culture in vitro (Figure 4B). All C. albicans strains that were isolated from culture in vitro were identified by RAPD as having the same origins as C. albicans inoculated in the abdominal cavities of the mice.
Figure 4. Biofilm formation in mice. (A) A total of 14 C. albicans (14/18, 77.8%) in the high ALS3 gene expression group formed biofilms. Three C. albicans strains (3/20, 15%) in the low ALS3 gene expression group formed biofilms (P = 0.000). (B) After the catheters were cultured, there were 12 C. albicans strains from the catheters of the high ALS3 expression group and the eight C. albicans strains from the catheters of the low ALS3 expression group were isolated from culture in vitro. (C) The average of ALS3 gene expression in 12 C. albicans from the catheters of the high ALS3 expression group declined from 13710 ± 1839 to 8828 ± 1680 (P < 0.001). (D) The average of ALS3 gene expression in eight C. albicans from the catheters of the low ALS3 expression group was 1860 ± 706. While before the biofilm formation experiment, it was 1607 ± 324 in this group (P = 0.189).
In the prevention of biofilm formation experiment, eight C. albicans strains (8/10, 80%) in the voriconazole group, two C. albicans strains (2/10, 20%) in the caspofungin group, and three C. albicans strains (3/10, 30%) in the micafungin group formed biofilms with membrane structures attached to the catheter walls when observed under a microscope (Figure 5). The rates of biofilm formation in the caspofungin and micafungin groups were lower than that in voriconazole group (Pcaspofungin = 0.007 and Pmicafungin = 0.025). However, there were no differences in the biofilm formation rate between the caspofungin and micafungin groups (P = 0.606).
Figure 5. Prevention of biofilm formation in mice. Eight C. albicans (8/10, 80%) in voriconazole group, two C. albicans (2/10, 20%) in caspofungin group and three C. albicans strains (3/10, 30%) in micafungin group formed biofilms with membrane structure attached in the wall of catheters under microscope. The rate of biofilm formation in the caspofungin group and in the micafungin group were lower than that of in the voriconazole group, respectively (Pcaspofungin = 0.007 and Pmicafungin = 0.025). But there was no different of the biofilm formation rate in the caspofungin and micafungin group (P = 0.606).
After the catheters removed from the mice were cultured in vitro, growing colonies of C. albicans were isolated. A total of 12 C. albicans strains in the high ALS3 expression group were isolated from the culture system, while eight C. albicans strains in the low ALS3 expression group were isolated. All 12 C. albicans strains in the high ALS3 expression group formed biofilms, while only one C. albicans strain formed biofilm in the low ALS3 expression group. The average ALS3 expression of 12 C. albicans strains in the high ALS3 gene expression group declined from 13,710 ± 1,839 to 8,828 ± 1,680. The average ALS3 expression of eight C. albicans in the low ALS3 expression group was 1,860 ± 706, while it was 1,607 ± 324 in the same group before the biofilm formation experiment. There were no differences in average ALS3 expression before and after the biofilm formation experiment in the low ALS3 expression group (Figures 4C,D).
The susceptibility of C. albicans to antifungal agents (e.g., fluconazole, voriconazole, itraconazole, caspofungin, and micafungin) in vitro was assayed. The activities of antifungal agents against the planktonic form of 55 C. albicans strains isolated from blood samples of patients with candidaemia were examined. All antifungal agents showed high antifungal activity against the 55 C. albicans strains (Table 1). After the biofilm formation experiment in vitro, activities of antifungal agents against the 26 C. albicans strains without biofilm formation and 29 C. albicans strains with biofilm formation were compared. The C. albicans strains with biofilm formation were more resistant to fluconazole, voriconazole, and itraconazole. However, these strains remained sensitive to caspofungin and micafungin. The C. albicans strains without biofilm formation were sensitive to all antifungal agents (Table 2).
Table 1. The number of strains with MIC50 value of different drugs in 55 planktonic Candida albicans.
Table 2. The number of strains with MIC50 value of different drugs in the 55 Candida albicans after biofilm formation experiment in vitro.
After the biofilm formation experiment in mice, activities of antifungal agents against the 12 C. albicans strains from the catheters in high ALS3 expression group and the eight C. albicans strains from the catheters in low ALS3 expression group were compared. The C. albicans from the catheters in the low ALS3 expression group were sensitive to all antifungal agents. The C. albicans strains from the catheters in high ALS3 expression group were more resistant to fluconazole, voriconazole, and itraconazole. These strains remained sensitive to caspofungin and micafungin (Table 3).
Table 3. The number of strains with MIC50 value of different drugs in the Candida albicans after biofilm formation experiment in mice.
Candida albicans biofilms are composed of a membranous multifungal complex adsorbed on the surface of biomaterials or a body cavity (Kojic and Darouiche, 2004; Gbelska et al., 2006; Uppuluri et al., 2010). C. albicans from biofilms are more invasive and resistant to azole antifungal agents than biofilms originating from planktonic C. albicans (Nett et al., 2010; Tobudic et al., 2010; Taff et al., 2013). Central venous catheter therapy is widely used in patients with malignant tumors. When C. albicans attaches to the surface of indwells in vivo (e.g., in catheters), it is easy to cause catheter-related candidiasis (Høiby et al., 2015). On this basis, C. albicans tends to form biofilms (Walraven and Lee, 2013). Biofilm-associated infections are difficult to eradicate because they are a self-perpetuating source of infection and are resistant to a variety of antifungal agents, particularly azole antifungal agents (Taff et al., 2013).
This study selected sensitive C. albicans isolated from blood samples from patients with candidiasis. The study intended to verify the results obtained from previous studies that ALS3 is a predictor of C. albicans biofilm formation (Deng et al., 2016, 2017). Further studies plan to provide a theoretical basis for the prevention and treatment of C. albicans biofilm formation.
In this study, there was no significant thickening of C. albicans cell walls after biofilm formation. This result is different from previous reports (Hamza et al., 2006), in which cell walls after C. albicans biofilm formation are twice as thick as those before biofilm formation. However, it was found that after biofilm formation, the electron density and mitochondrial richness of C. albicans are high. Another study showed that the sensitivity of C. albicans during biofilm formation depends on the metabolic activity of C. albicans (Marcos-Zambrano et al., 2014). C. albicans biofilm with low metabolic activity was more sensitive to micafungin than that with high metabolic activity. In this study, the electron density and mitochondrial richness of C. albicans may have been related to the increased drug resistance after biofilm formation.
It has been reported (Tawara et al., 2000) that C. albicans is resistant to fluconazole, voriconazole, and itraconazole after biofilm formation. Although the MIC50 value of caspofungin and micafungin increased, C. albicans after biofilm formation remained sensitive to these two antifungal agents. Caspofungin and micafungin overcame the resistance of C. albicans after biofilm formation, which is consistent with prior results.
Independent predictors of biofilm formation candida bloodstream infections (CBSIs) were the presence of central venous catheters (CVCs) and urinary catheters (Tumbarello et al., 2012). However, not all C. albicans bloodstream infections form biofilms. This study showed that 29 C. albicans strains out of the 55 total strains (52.7%) formed biofilms in vitro. This result indicates that C. albicans had the heterogeneity of biofilm formation. A future goal is to find out the characteristics of C. albicans that can form biofilms easily.
The detection and analysis of gene expression is an important factor related to the study of biofilm formation (Sherry et al., 2014). ALS is the main gene family that controls the adhesion of biofilms (Klotz et al., 2007). It has been reported that the expression of ALS3 is related to biofilm formation and that its expression gradually decreases after biofilm formation (Liu and Filler, 2011). In this experiment, the same results were obtained. This raised the issue of whether there is any difference in the ALS3 expression before the biofilm formation of C. albicans, and whether this difference in ALS3 expression can predict C. albicans biofilm formation? This study found a difference in the expression of ALS3 in free, antifungal, drug-sensitive C. albicans. In vitro results showed that the average ALS3 expression was higher in the 29 C. albicans strains with biofilm formation than that in the 26 C. albicans strains without biofilm formation. In the following experiments on biofilm formation in mice, similar results were obtained. The rate of biofilm formation was higher in the high ALS3 expression group than that in the low ALS3 expression group.
On the other hand, high expression of ALS3 in C. albicans plays an important role not only in biofilm formation, but also in pathogenicity (Mukherjee et al., 2005). This study found that the C. albicans strains with biofilm formation were more resistant to fluconazole, voriconazole, and itraconazole. These strains remained sensitive to caspofungin and micafungin. In vivo tests in mice showed that the activities of antifungal agents against the 12 C. albicans strains from catheters in the high ALS3 expression group were more resistant to fluconazole, voriconazole, and itraconazole than that in the eight strains from catheters in the low ALS3 expression group. However, these strains remained sensitive to caspofungin and micafungin. This result regarding the activities of antifungal agents against C. albicans with biofilm formation is consistent with previous reports (Jacobson et al., 2009; Simitsopoulou et al., 2013).
It has been shown that the expression of ALS3 is not significantly different among standard C. albicans strains, but there is a significant difference among clinical strains (Bruder-Nascimento et al., 2014), which was consistent with the results in this study. The C. albicans strains with high ALS3 expression were more likely to form biofilms in vitro and in mice, leading to increased resistance to azole antifungal drugs but maintenance of sensitivity to caspofungin and micafungin. In the further prevention experiments on biofilm formation in mice, only caspofungin and micafungin prevented C. albicans biofilm formation.
Whether the differential expression of ALS3 in C. albicans is a predictor of biofilm formation and whether its expression can further guide the selection of clinical antifungal agents remain to be further explored.
ALS3 is differentially expressed in C. albicans and C. albicans strains with high ALS3 expression are associated with biofilm formation in vitro and in mice. C. albicans strains with biofilm formation remain sensitive to caspofungin and micafungin. In the prevention of the biofilm formation experiment, only caspofungin and micafungin prevented formation biofilm formation.
The datasets presented in this study can be found in online repositories. The names of the repository/repositories and accession number(s) can be found below: https://www.ncbi.nlm.nih.gov/genbank/, XM_705343.2.
The patient gave their written informed consent in accordance with the Declaration of Helsinki. The patient agreed to the use of his specimens and data for our study. The animal study was reviewed and approved by the Tianjin First Central Hospital Medical Ethics Committee.
QD: concept and design. KD and WJ: drafting or revising the manuscript. YJ and JC: acquisition of data. WY and XZ: analysis and interpretation of data. All authors contributed to writing, review, and/or revision of the manuscript. QD, WY, and XZ: study supervision. All the authors listed have made a substantial, direct and intellectual contribution to this work, and approved it for publication.
This study was supported by grants from the Tianjin Municipal Health Bureau of Science and Technology Fund 2015 Project (No. 15KG135). The project title is: The establishment of the individualized programs to prevent invasive fungal disease in acute leukemia. This study was supported by the Science and Technology Fund of Tianjin Health and Family Planning Commission (Grant 2012KZ022). The project title is: Study on fungal bilfilm and antifungal drug resistance. This study was also supported by the Science and Technology Foud of Tianjin Health and Family Planning Commission (Grant 2014KZ029). The project title is: Molecular biology research of carbapenemase producing Klebsiella pneumoniae.
The authors declare that the research was conducted in the absence of any commercial or financial relationships that could be construed as a potential conflict of interest.
We thank the patients for the blood culture specimens and data provided in our experimental study. We also thank LetPub (www.letpub.com) for its linguistic assistance during the preparation of this manuscript.
Bougnoux, M. E., Tavanti, A., Bouchier, C., Gow, N. A. R., Magnier, A., Davidson, A. D., et al. (2003). Collaborative consensus for optimized multilocus sequence typing of Candida albicans[J]. J. Clin. Microbiol. 41, 5265–5266. doi: 10.1128/JCM.41.11.5265-5266.2003
Bruder-Nascimento, A., Camargo, C. H., Mondelli, A. L., Sugizaki, M. F., Sadatsune, T., and Bagagli, E. (2014). Candida species biofilm and Candida albicans als3 polymorphisms in clinical isolates. Braz. J. Microbiol. 45, 1371–1377. doi: 10.1590/S1517-83822014000400030
Choi, H. W., Shin, J. H., Jung, S. I., Kee, C. S., Cho, D., Kee, S. J., et al. (2007). Species-specific differences in the susceptibilities of biofilms formed by Candida bloodstream isolates to echinocandin antifungals. Antimicrob. Agents Chemother. 51, 1520–1523. doi: 10.1128/AAC.01141-06
Clinical and Laboratory Standards Institute (CLSI) (2008). Reference Method for Broth Dilution Antifungal Susceptibility Testing of Yeasts; Approved Standard. CLSI Document M27-A3. Wayne, IL: Clinical and Laboratory Standards Institute.
Deng, K. K., Deng, Q., Zhang, J. L., Chen, J. Y., and Jiang, Y. Y. (2016). The drug resistance of Candida albicans biofilm and the correlation of ALS3 gene expression and film forming. Chin. J. Infect. Dis. 34, 603–608. doi: 10.3760/cma.j.issn.1000-6680.2016.10.007
Deng, K. K., Deng, Q., Zhang, J. L., Chen, J. Y., Jiang, Y. Y., and Xing, Y. (2017). The als3 gene expression and the Candida albicans biofilm film forming in mice vivo. Chin. J. Infect. Dis. 34, 603–608. doi: 10.3760/cma.j.issn.1000-6680.2017.05.009
Escribano, P., Marcos-Zambrano, L. J., Gómez, A., Sánchez, C., Martínez-Jiménez, M. C., Bouza, E., et al. (2017). The etest performed directly on blood culture bottles is a reliable tool for detection of fluconazole-resistant Candida albicans isolates. Antimicrob. Agents Chemother. 61:e00400-17. doi: 10.1128/AAC.00400-17
Gbelska, Y., Krijger, J. J., and Breunig, K. D. (2006). Evolution of gene families: the multidrug resistance transporter genes in five related yeast species. FEMS Yeast Res. 6, 345–355. doi: 10.1111/j.1567-1364.2006.00058.x
Hamza, O. J., Van den Bout-van, C. J., Matee, M. I., Moshi, M. J., Mikx, F. H., Selemani, H. O., et al. (2006). Antifungal activity of some Tanzanian plants used traditionally for the treatment of fungal infections. J. Ethnopharmacol. 108, 124–132. doi: 10.1016/j.jep.2006.04.026
Høiby, N., Bjarnsholt, T., Moser, C., Bassi, G. L., Coenye, T., Donelli, G., et al. (2015). ESCMID guideline for the diagnosis and treatment of biofilm infections 2014. Clin. Microbiol. Infect. 21, S1–S25. doi: 10.1016/j.cmi.2014.10.024
Hosseini, S. S., Ghaemi, E., Noroozi, A., and Niknejad, F. (2019). Zinc oxide nanoparticles inhibition of initial adhesion and ALS1 and ALS3 gene expression in Candida albicans strains from urinary tract infections. Mycopathologia 184, 261–271. doi: 10.1007/s11046-019-00327-w
Jacobson, M. J., Steckelberg, K. E., Piper, K. E., Steckelberg, J. M., and Patel, R. (2009). In vitro activity of micafungin against planktonic and sessile Candida albicans isolates. Antimicrob. Agents Chemother. 53, 2638–2639. doi: 10.1128/AAC.01724-08
Katragkou, A., Chatzimoschou, A., Simitsopoulou, M., Dalakiouridou, M., Diza-Mataftsi, E., Tsantali, C., et al. (2008). Differential activities of newer antifungal agents against Candida albicans and Candida parapsilosis biofilms. Antimicrob. Agents Chemother. 52, 357–360. doi: 10.1128/AAC.00856-07
Klotz, S. A., Gaur, N. K., De Armond, R., Sheppard, D., Khardori, N., Edwards, J. E., et al. (2007). Candida albicans Als proteins mediate aggregation with bacteria and yeasts. Med. Mycol. 45, 363–370. doi: 10.1080/13693780701299333
Kojic, E. M., and Darouiche, R. O. (2004). Candida infections of medical devices. Clin. Microbiol. Rev. 17, 255–267. doi: 10.1128/CMR.17.2.255-267.2004
Lamfon, H. (2004). Susceptibility of Candida albicans biofilms grown in a constant depth film fermentor to chlorhexidine, fluconazole and miconazole: a longitudinal study. J. Antimicrob. Chemother. 53, 383–385. doi: 10.1093/jac/dkh071
Liu, Y., and Filler, S. G. (2011). Candida albicans Als3, a multifunctional adhesin and invasin. Eukaryot. Cell 10, 168–173. doi: 10.1128/EC.00279-10
Marcos-Zambrano, L. J., Escribano, P., González del Vecchio, M., and Guinea, J. (2014). Micafungin is more active against Candida albicans biofilms with high metabolic activity. J. Antimicrob. Chemother. 69, 2984–2987. doi: 10.1093/jac/dku222
Mukherjee, P. K., Zhou, G., Munyon, R., and Ghannoum, M. A. (2005). Candida biofilm: a well-designed protected environment. Med Mycol. 43, 191–208. doi: 10.1080/13693780500107554
Nett, J. E., Crawford, K., Marchillo, K., and Andes, D. R. (2010). Role of Fks1p and matrix glucan in Candida albicans biofilm resistance to an echinocandin, pyrimidine, and polyene. Antimicrob. Agents Chemother. 54, 3505–3508. doi: 10.1128/AAC.00227-10
Perrone, T. M., Gonzatti, M. I., Villamizar, G., Escalante, A., and Aso, P. M. (2009). Molecular profiles of Venezuelan isolates of Trypanosoma sp. by random amplified polymorphic DNA method. Vet. Parasitol. 161, 194–200. doi: 10.1016/j.vetpar.2009.01.034
Ramage, G., Saville, S. P., Thomas, D. P., and López-Ribot, J. L. (2005). Candida biofilms: an update. Eukaryot. Cell 4, 633–638. doi: 10.1128/EC.4.4.633-638.2005
Sherry, L., Rajendran, R., Lappin, D. F., Borghi, E., Perdoni, F., Falleni, M., et al. (2014). Biofilms formed by Candida albicans bloodstream isolates display phenotypic and transcriptional heterogeneity that are associated with resistance and pathogenicity. BMC Microbiol. 14:182. doi: 10.1186/1471-2180-14-182
Simitsopoulou, M., Peshkova, P., Tasina, E., Katragkou, A., Kyrpitzi, D., Velegraki, A., et al. (2013). Species-specific and drugspecific differences in susceptibility of Candida biofilms to echinocandins: characterization of less common bloodstream isolates. Antimicrob. Agents Chemother. 57, 2562–2570. doi: 10.1128/AAC.02541-12
Taff, H. T., Mitchell, K. F., Edward, J. A., and Andes, D. R. (2013). Mechanisms of Candida biofilm drug resistance. Future Microbiol. 8, 1325–1337. doi: 10.2217/fmb.13.101
Tawara, S., Ikeda, F., Maki, K., Morishita, Y., Otomo, K., Teratani, N., et al. (2000). In vitro activities of a new lipopeptide antifungal agent, FK463, against a variety of clinically important fungi. Antimicrob. Agents Chemother. 44, 57–62. doi: 10.1128/AAC.44.1.57-62.2000
Tobudic, S., Kratzer, C., Lassnigg, A., Graninger, W., and Presterl, E. (2010). In vitro activity of antifungal combinations against Candida albicans biofilms. J. Antimicrob. Chemother. 65, 271–274. doi: 10.1093/jac/dkp429
Tumbarello, M., Fiori, B., Trecarichi, E. M., Posteraro, P. P., Losito, A. R., Luca, A. D., et al. (2012). Risk factors and outcomes of candidemia caused by biofilm-forming isolates in a tertiary care hospital. PLoS One. 7:e33705. doi: 10.1371/journal.pone.0033705
Uppuluri, P., Chaturvedi, A. K., Srinivasan, A., Banerjee, M., Ramasubramaniam, A. K., Köhler, J. R., et al. (2010). Dispersion as an important step in the Candida albicans biofilm developmental cycle. PLoS Pathog. 6:e1000828. doi: 10.1371/journal.ppat.1000828
Vrioni, G., and Matsiota-Bemard, P. (2001). Molecular typing of Candida isolates from patients hospitalized in an intensive care unit. J. Infect. 42, 50–56. doi: 10.1053/jinf.2000.0778
Keywords: Candida albicans, biofilms, resistance, gene expression, ALS3 gene
Citation: Deng K, Jiang W, Jiang Y, Deng Q, Cao J, Yang W and Zhao X (2021) ALS3 Expression as an Indicator for Candida albicans Biofilm Formation and Drug Resistance. Front. Microbiol. 12:655242. doi: 10.3389/fmicb.2021.655242
Received: 18 January 2021; Accepted: 08 April 2021;
Published: 29 April 2021.
Edited by:
Keke Huo, Fudan University, ChinaReviewed by:
Laura Judith Marcos Zambrano, IMDEA Food Institute, SpainCopyright © 2021 Deng, Jiang, Jiang, Deng, Cao, Yang and Zhao. This is an open-access article distributed under the terms of the Creative Commons Attribution License (CC BY). The use, distribution or reproduction in other forums is permitted, provided the original author(s) and the copyright owner(s) are credited and that the original publication in this journal is cited, in accordance with accepted academic practice. No use, distribution or reproduction is permitted which does not comply with these terms.
*Correspondence: Qi Deng, a2FjaHlkZW5nQDEyNi5jb20=; Wenjie Yang, eWFuZ204MDA2QHNpbmEuY29t; Xuequn Zhao, MTU1MjIzMjE4OTZAMTYzLmNvbQ==
Disclaimer: All claims expressed in this article are solely those of the authors and do not necessarily represent those of their affiliated organizations, or those of the publisher, the editors and the reviewers. Any product that may be evaluated in this article or claim that may be made by its manufacturer is not guaranteed or endorsed by the publisher.
Research integrity at Frontiers
Learn more about the work of our research integrity team to safeguard the quality of each article we publish.