- 1State Key Laboratory of Marine Resource Utilization in the South China Sea, Hainan University, Haikou, China
- 2Ministry of Education Key Laboratory of Cell Activities and Stress Adaptations, School of Life Sciences, Lanzhou University, Lanzhou, China
- 3Hainan Provincial Key Laboratory for Tropical Hydrobiology and Biotechnology, Hainan University, Haikou, China
- 4College of Marine Sciences, Hainan University, Haikou, China
Protease-producing bacteria play vital roles in degrading organic matter of aquaculture system, while the knowledge of diversity and bacterial community structure of protease-producing bacteria is limited in this system, especially in the tropical region. Herein, 1,179 cultivable protease-producing bacterial strains that belonged to Actinobacteria, Firmicutes, and Proteobacteria were isolated from tropical aquaculture systems, of which the most abundant genus was Bacillus, followed by Vibrio. The diversity and relative abundance of protease-producing bacteria in sediment were generally higher than those in water. Twenty-one genera from sediment and 16 genera from water were identified, of which Bacillus dominated by Bacillus hwajinpoensis in both and Vibrio dominated by Vibrio owensii in water were the dominant genera. The unique genera in sediment or water accounted for tiny percentage may play important roles in the stability of community structure. Eighty V. owensii isolates were clustered into four clusters (ET-1–ET-4) at 58% of similarity by ERIC-PCR (enterobacterial repetitive intergenic consensus-polymerase chain reaction), which was identified as a novel branch of V. owensii. Additionally, V. owensii strains belonged to ET-3 and ET-4 were detected in most aquaculture ponds without outbreak of epidemics, indicating that these protease-producing bacteria may be used as potential beneficial bacteria for wastewater purification. Environmental variables played important roles in shaping protease-producing bacterial diversity and community structure in aquaculture systems. In sediment, dissolved oxygen (DO), chemical oxygen demand (COD), and salinity as the main factors positively affected the distributions of dominant genus (Vibrio) and unique genera (Planococcus and Psychrobacter), whereas temperature negatively affected that of Bacillus (except B. hwajinpoensis). In water, Alteromonas as unique genus and Photobacterium were negatively affected by NO3−-N and NO2−-N, respectively, whereas pH as the main factor positively affected the distribution of Photobacterium. These findings will lay a foundation for the development of protease-producing bacterial agents for wastewater purification and the construction of an environment-friendly tropical aquaculture model.
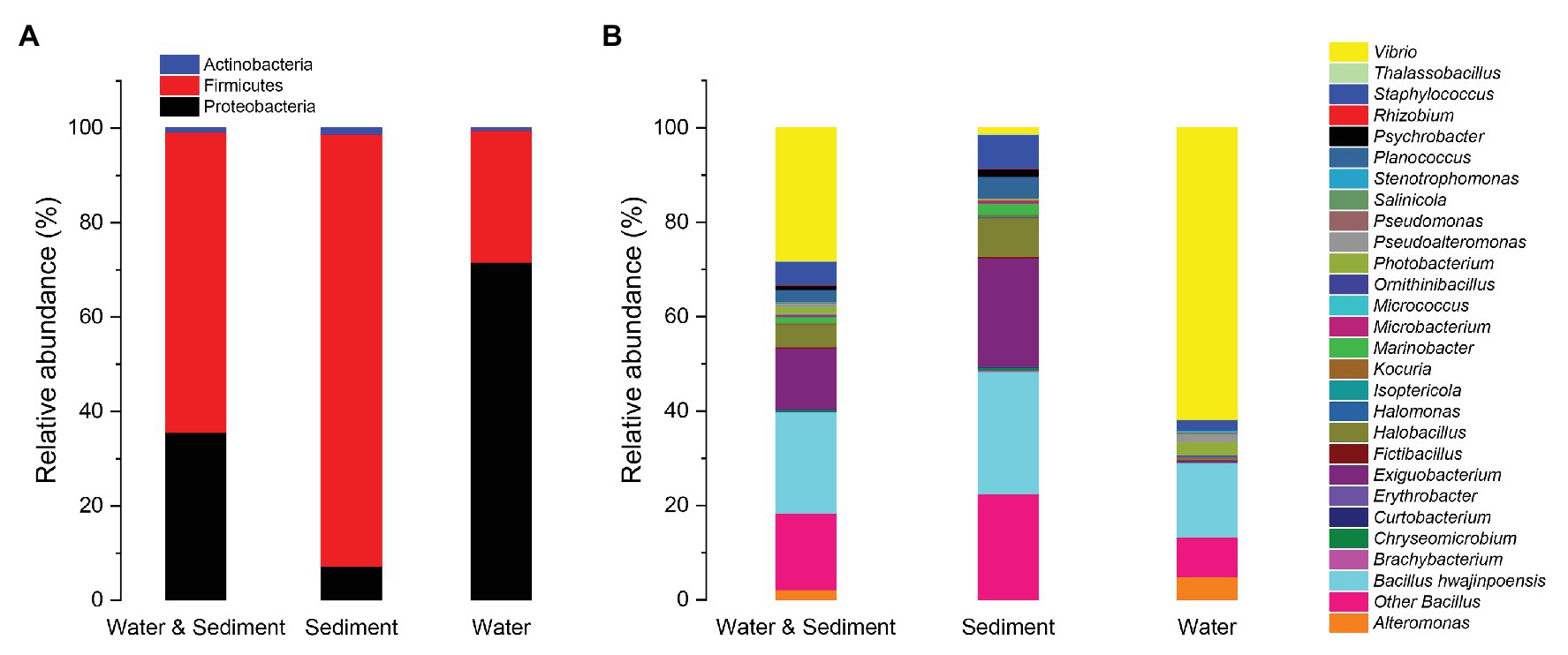
Graphical Abstract. Community structure of protease-producing bacteria cultivated from aquaculture systems: potential impact of a tropical environment, in the case of Wenchang, Hainan, China.
Introduction
Aquaculture as an important food production has become an important economic activity in many countries (Hamza et al., 2017; Santos and Ramos, 2018). However, with the rapid development of aquaculture industry, a large number of animal residues, residual feed, and excrement have been produced, thereby aggravating the accumulation of organic matter in aquaculture (Lin and Chen, 2001, 2003; Kuhn et al., 2010; Guo et al., 2016; Li et al., 2020; Mariane de Morais et al., 2020). The main sources of nitrogen and carbon of aquaculture are from the particulate organic matters containing large amounts of proteins and amino acids. Generally, particulate organic matter is difficult to be dissolved, which should be first decomposed into dissolved form. In turn, the dissolved organic matter can be transformed into nitrogen gas by ammonification, nitrification, and denitrification. Bacteria play key roles in these processes by secreting degradation enzymes (Olson and Lesser, 2013; Su et al., 2020).
Protease-producing bacteria as the main degraders of organic matter can effectively hydrolyze the organic matter into peptides and amino acids by secreting extracellular proteases (Su et al., 2020). As we all know, peptides and amino acids are essential for subsequent catabolism of organisms. Reportedly, protease-producing bacteria could not only improve protein digestibility and growth of the host, but also reduce organic pollutants in aquaculture (Shi et al., 2016; Amin, 2018; Su et al., 2020). Protease-producing bacteria that belong to four major phyla, Proteobacteria, Firmicutes, Actinobacteria, and Bacteroidetes, have been identified, which are dominated by Bacillus, Pseudomonas, and Pseudoalteromonas genera (Su et al., 2020).
Many methods have been developed to enhance protein digestibility of aquafeed in aquaculture. However, the application of exogenous enzymes has been limited because of expensive and poor stability in various processing conditions. During the feed processing, dietary enzymes may lose their activities under high temperature and pressure conditions. Fortunately, an increasingly accepted method by supplementing protease-producing bacteria considered as an environment-friendly method was good for growth enhancement (Shi et al., 2016; Amin, 2018), which has been confirmed to not only enhance protein digestibility, but also reduce organic pollutants produced by undigested feed (Suzer et al., 2008; Ambas et al., 2015; Reda and Selim, 2015). Reportedly, feed by supplementing 1.0 × 1010CFU/g protease-producing bacteria could improve growth and nonspecific immune system of Nile tilapia (Selim and Reda, 2015).
Protease-producing bacteria play a vital role in degrading organic matter. However, the diversity and community of protease-producing bacteria have seldom been addressed in aquaculture. As a consequence, in this article, 1,179 cultivable protease-producing bacterial strains were isolated and screened from sediment and water samples in Wenchang (Hainan, China), and then their diversity and community structure were investigated. Additionally, the physicochemical factors related to diversity and distribution of protease-producing bacterial communities in sediment and water were comprehensively analyzed, respectively. The dominant protease-producing Vibrio owensii strains in aquaculture water were classified using enterobacterial repetitive intergenic consensus-polymerase chain reaction (ERIC-PCR).
Materials and Methods
Study Site Description and Sample Collection
Surface sediment (2- to 3-cm thickness) and aquaculture water (1-m water depth) samples were collected from six prawn ponds (19°27'28-32''N, 110°45'13''E) and one nature sea pool (closed to the prawn ponds) located at Huiwen Town, Wenchang City, China. The special geographical position is shown in Supplementary Figure S1. The experimental ponds are summarized in Supplementary Table S1.
Isolation and Screening of Protease-Producing Bacteria
Sediment (0.5g) and water (100μl) samples were serially diluted with sterile normal saline solution (0.85% NaCl) within 24h of collection to obtain 1:10, 1:100, and 1:1,000 dilutions. One hundred microliters of each diluted sample was spread-plated on marine 2216E agar and incubated at 30°C for 18h, which was finally ascertained by preliminary selection for the condition of cultural bacteria. For screening of protease-producing bacteria, 2μl of overnight growth culture in the marine 2216E of each bacterial isolate was spot plated on casein-gelatin agar [containing 0.2% yeast extract, 0.3% xasein, sodium salt, 5% gelatin, 0.13% (NH4)2SO4, 0.05% MgSO4·7H2O, and 1.5% agar and seawater; pH 7.5–8.0]. The protease-producing bacterial isolates were further used for DNA extraction.
Quantification of Protease Activity
Protease activity was elevated in terms of hydrolytic capacity (HC); the pure bacterial isolates were cultured in 2166E broth. A solution with OD at 600 nm of 0.2ml was set, of which 1μl was pipetted out and dropped on casein-gelatin agar plate in duplicates. Diameters of bacterial colonies and related-clearance zones were measured after 24-h incubation at 28°C ± 2°C. The HC value determined the protease activity was calculated from the ratio between the diameter of the caseinolytic zone and the diameter of the bacterial colony. In this study, we selected only colonies with a ratio greater than three.
DNA Extraction and PCR Amplification
Bacterial strain with the highest protease activity was identified using genotypic assay. Genomic DNAs of culturable protease-producing bacteria were extracted using a commercial DNA extraction kit (Tiangen Biotech, Beijing, China; Sun et al., 2008; Marquez-Santacruz et al., 2010), after bacterial growth on 2216E medium and incubation at 30°C for 18h. The 16S rDNA genes were amplified from genomic DNA by PCR using the universal primers 27F (5'-AGAGTTTGATCCTGGCTCAG-3') and 1492R (5'-GGCTACCTTGTTACGACTT-3'). PCR amplifications were performed using the following conditions: initial denaturation of template DNA (95°C for 2 min), then 1cycle consisting of denaturation (30 s at 95°C), annealing (30 s at 60°C), extension (1min at 72°C), 25cycles, and a final extension at 72°C for 5 min. Bacteria sequences obtained by culture-dependent approach were compared with 16S rDNA reference gene sequences by BLAST.1
Genetic Diversity of Dominant V. owensii Using ERIC-PCR
All the dominant isolates identified and confirmed as V. owensii were chosen for further molecular typing by ERIC-PCR. One microliter of the extracted DNA was added to 12.5μl of PCR amplification mixture, containing 9.5μl ddH2O, and 2μl primers (forward and reverse). Amplification reactions were carried out in a thermal cycler (Bio-Rad, United States) using the primer set ERIC-1R 5'-AAGTAAGTGACTGGGGTGAGCG-3', and ERIC-2F 5'-ATGTAAGCTCCTGGGGATTCAC-3'. The amplification program was as follows: an initial denaturation step at 95°C for 7min, denaturation 90°C for 30min; annealing at 52°C for 1min; extension at 65°C for 8min; and after 35cycles, a final extension at 65°C for 16min. Six microliters of each amplified product was electrophoresed on 1% agarose gel with DNA safe stain (Greenview Plus, Andy Gold™, United States) 1 × TAE buffer along with 1 Kb DNA Ladder (Thermo Fischer Scientific, United States) and M2-DL2000 marker (Takara, Japan), at 180V for 50min. The amplification was repeated three times.
Gels were viewed by Gel Imaging System (Tanon3500R, Shanghai, China). The images were captured for further analysis. In turn, “1” and “0” were assigned for the presence or lack of the banding pattern and recorded in sequence according to the reading direction. The genetic patterns were calculated according to the molecular weights and quantification of the bands in each sample. Simpson’s Index of Diversity was used to calculated the discriminatory index of ERIC-PCR (Pishbin and Mehrabian, 2020), and the “unweighted pair group method with dice similarity coefficient” option in NTSYS v. 12 program (Bakhshi et al., 2018) was used to cluster the V. owensii isolates.
Results
Analysis of Diversity and Distribution of Cultivable Protease-Producing Bacterial Strains
There were 1,179 cultivable protease-producing bacterial strains isolated and screened from sediment (654 strains) and water (525 strains) samples of aquaculture systems, and the bacterial counts of sediment and water varied from 2 × 104 to 2.6 × 105 CFU/g and 658 to 2.1 × 103CFU/ml, respectively (Table 1). All of the isolates belonged to three phyla, Actinobacteria, Firmicutes, and Proteobacteria, and classified into 27 genera dominated by Bacillus (37.7%) and Vibrio (28.1%) genera (Figure 1, “Water & Sediment” bars), of which Firmicutes accounted for the highest proportion. Additionally, Bacillus hwajinpoensis in Bacillus was dominant species in whole tropical aquaculture system (Figure 1B). Twenty-one genera from sediment belonged to Actinobacteria, Firmicutes, and Proteobacteria, and bacterial diversity was found to be maximum (up to about 91.6%) in the phylum of Firmicutes. The largest genus was Bacillus (B. hwajinpoensis as the dominant species, 26%), followed by Exiguobacterium in sediment (Figure 1, “Sediment” bars). Additionally, 12 unique genera were isolated only from sediment samples, namely Brachybacterium, Chryseomicrobium, Curtobacterium, Erythrobacter, Halomonas, Isoptericola, Kocuria, Marinobacter, Planococcus, Psychrobacter, Rhizobium, and Thalassobacillus. Sixteen genera from aquaculture water samples also belonged to Actinobacteria, Firmicutes, and Proteobacteria, whereas their relative abundance displayed significant differences with those in sediment samples. The relative abundance of Proteobacteria was up to about 71.6%, followed by Firmicutes (28.0%), and the largest genus was Vibrio (61.7%) as the dominant group in aquaculture water samples (Figure 1, “Water” bars). Meanwhile, there were seven unique genera from water samples, namely, Alteromonas, Micrococcus, Ornithinibacillus, Pseudoalteromonas, Pseudomonas, Salinicola, and Stenotrophomonas. Previous studies have reported that protease-producing bacteria were extremely diverse, including main genera such as Acinetobacter, Aeromonas, Enterobacter, Enterococcus, Microbacterium, Micrococcus, Pseudomonas, Staphylococcus, Stenotrophomonas, and Streptomyces, as well as other unclassified bacteria especially Bacillus and Vibrio, which were similar to our results (Dat et al., 2019). In this work, 80 V. owensii strains among the dominant Vibrio genus were isolated. It is worth noting that cultivable protease-producing bacterial diversity of sediment was generally higher than that of water (Figure 1B and Table 1).
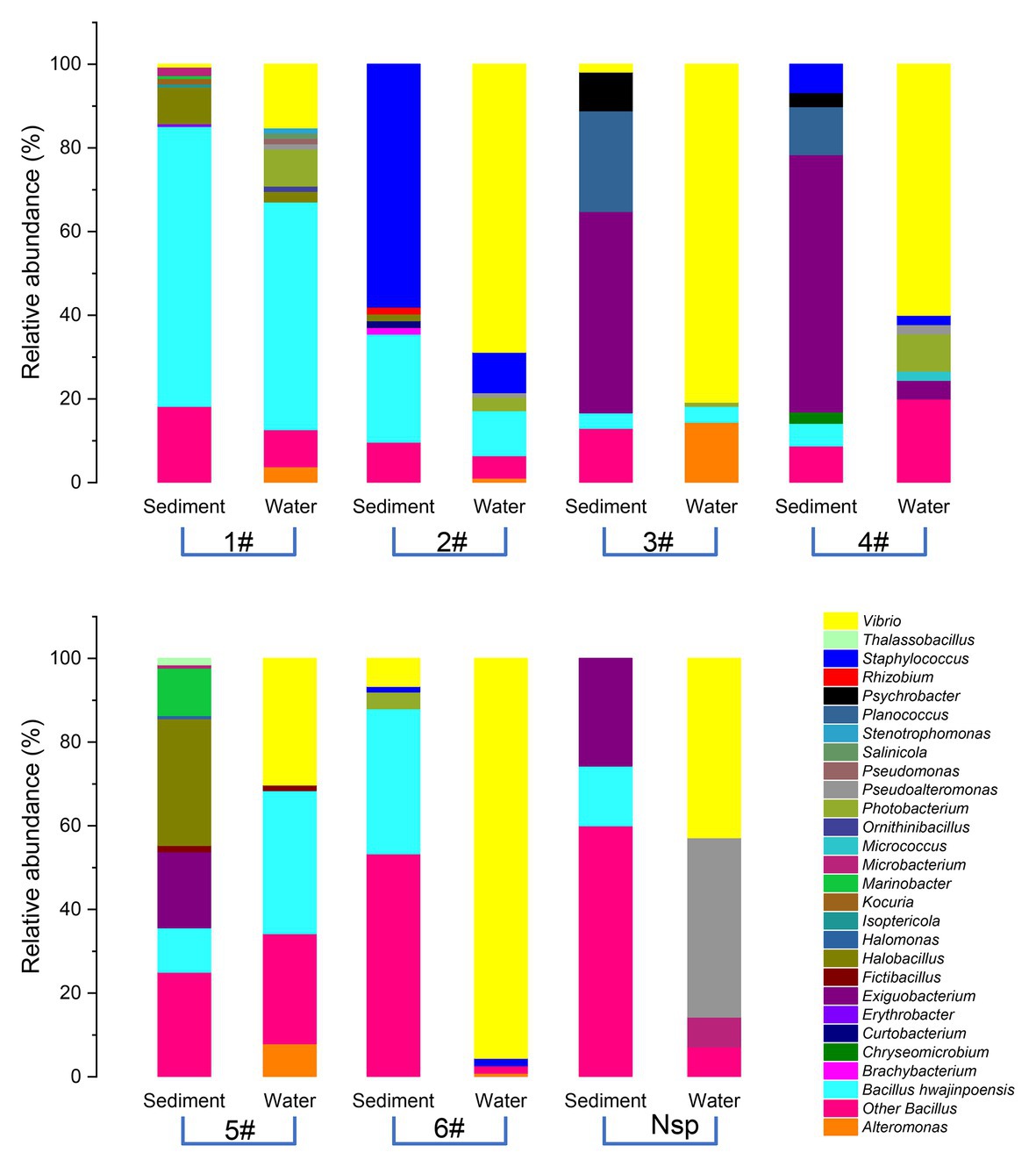
Figure 1. Relative abundances of cultivable protease-producing bacterial strains in water and sediment, sediment, and water of aquaculture systems at the level of phylum (A) and genus (B), respectively.
The diversity and distribution of cultivable protease-producing bacterial strains in different sampling stations were investigated (Figure 2). The results showed obviously different protease-producing bacterial community structures displayed between each aquaculture pond at the genus level. Interestingly, Vibrio from water samples was found in all sampling stations (Supplementary Table S2) and accounted for the relatively higher proportion (Figure 2). Bacillus also displayed high abundance in most sampling stations.
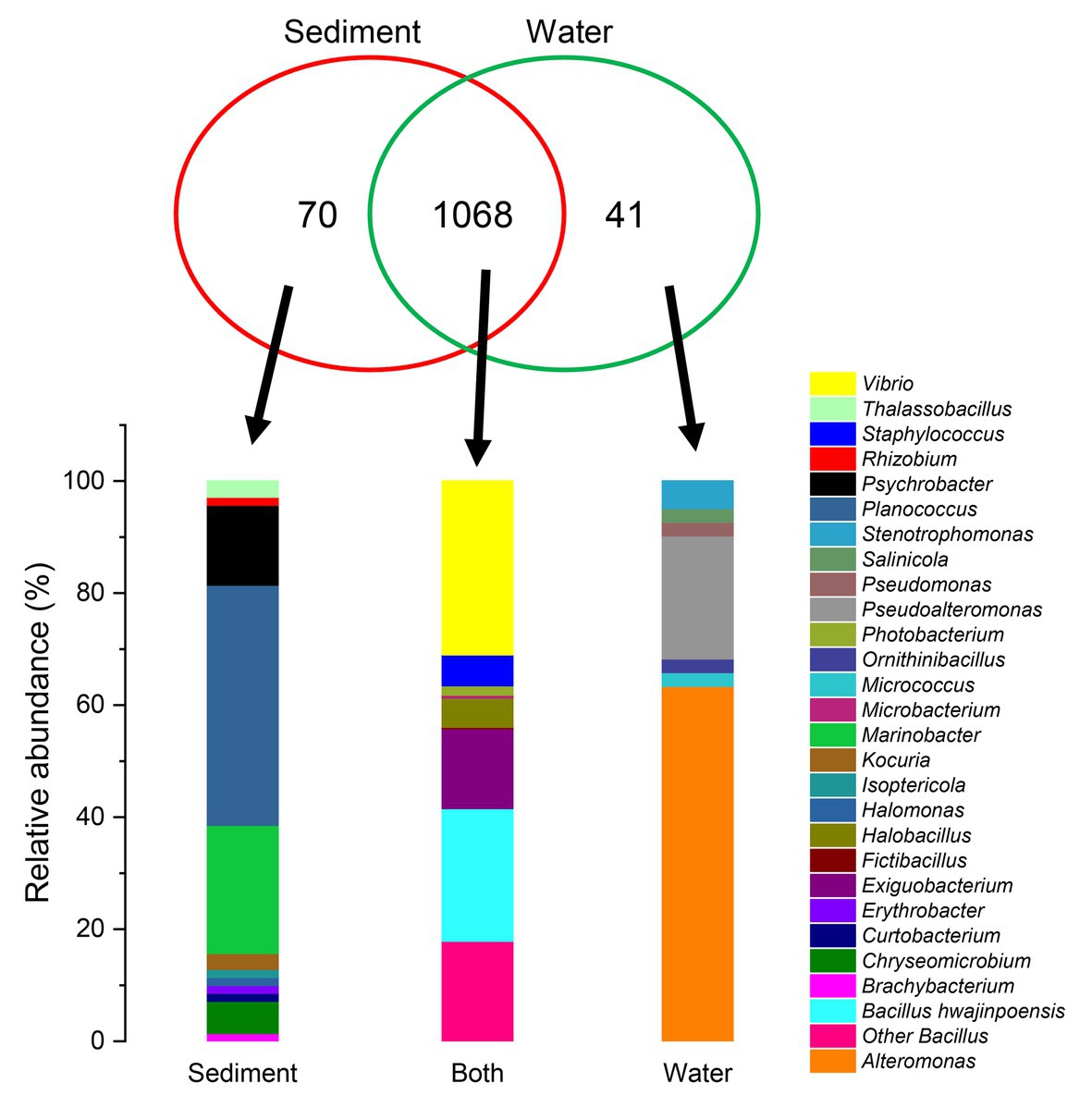
Figure 2. Relative abundances of cultivable protease-producing bacterial strains in sediment and water of aquaculture ponds on the level of genus. The aquaculture ponds are coded 1#–6#, and Nsp represents nature seawater pool.
Comparison of Cultivable Protease-Producing Bacterial Distribution of Sediments and Water in Aquaculture Systems
As shown in Figure 3, of the 1,179 total cultivable protease-producing bacterial strains from different genera, most strains were found in both sediment and water samples, whereas the sediment and water samples harbored only 70 and 41 unique strains, respectively. Planococcus (42.9%) and Marinobacter (22.9%) were notably abundant in the unique genus of sediment samples, whereas Alteromonas (63.4%) displayed the highest relative abundance in the unique genus of water samples. Among the genera in both locations, Vibrio (31.0%) as the dominant group accounted for the highest proportion.
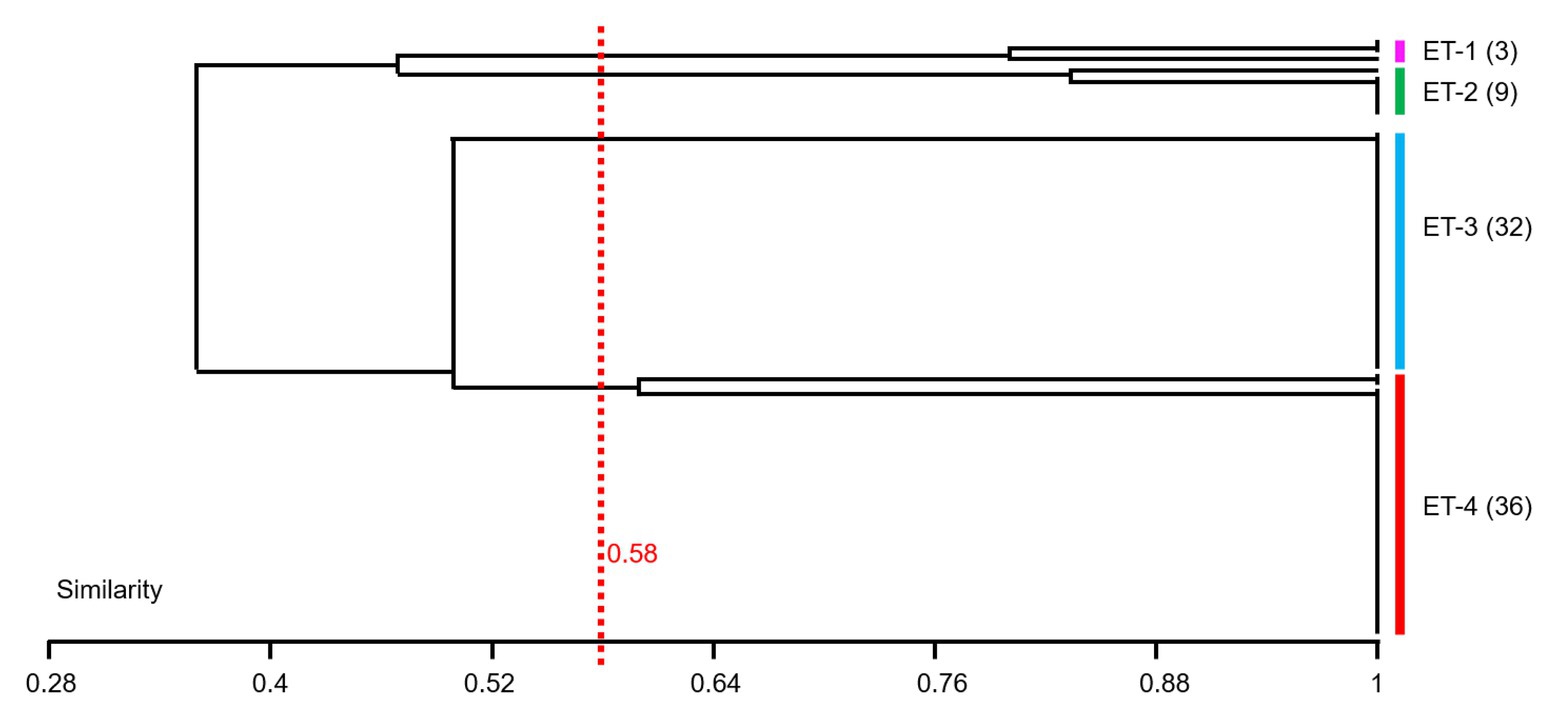
Figure 3. Comparison of the cultivable protease-producing bacteria distribution of sediment and water samples at the genus level. Proportional Venn diagram showing the number of strains found only in sediment samples, only in water samples, and in both. These strains were used to create the relative abundance bar graphs at the genus level under the panel Venn.
Genetic Diversity of Dominant V. owensii Using ERIC-PCR
In this study, 80 V. owensii strains as the dominant cultivable protease-producing bacteria were isolated from aquaculture systems. In view of the high-importance Vibrio and their increased prevalence in aquaculture, the genetic linkage of these isolates was investigated by ERIC-PCR. In the extragenic regions of V. owensii genome, the sequences of enterobacterial repetitive intergenic consensus consist of highly conserved central inverted repeats. In this study, 80V. owensii isolates were fingerprinted by ERIC-PCR. This technique generated two to six amplification bands ranging in size from 800 to 7,500bp (Supplementary Figure S2). Adopting 58% of similarity, 80 V. owensii isolates were clustered into four clusters (Figure 4 and Supplementary Table S3), ET-1, ET-2, ET-3, and ET-4. The predominant clusters were ET-3 and ET-4, as most isolates had a common banding pattern (7,000bp; Supplementary Figure S2). Only three strains were clustered into ET-1, and nine strains were clustered into ET-2. The Simpson’s Index of Diversity was used to elevate the discriminatory power of this typing method.
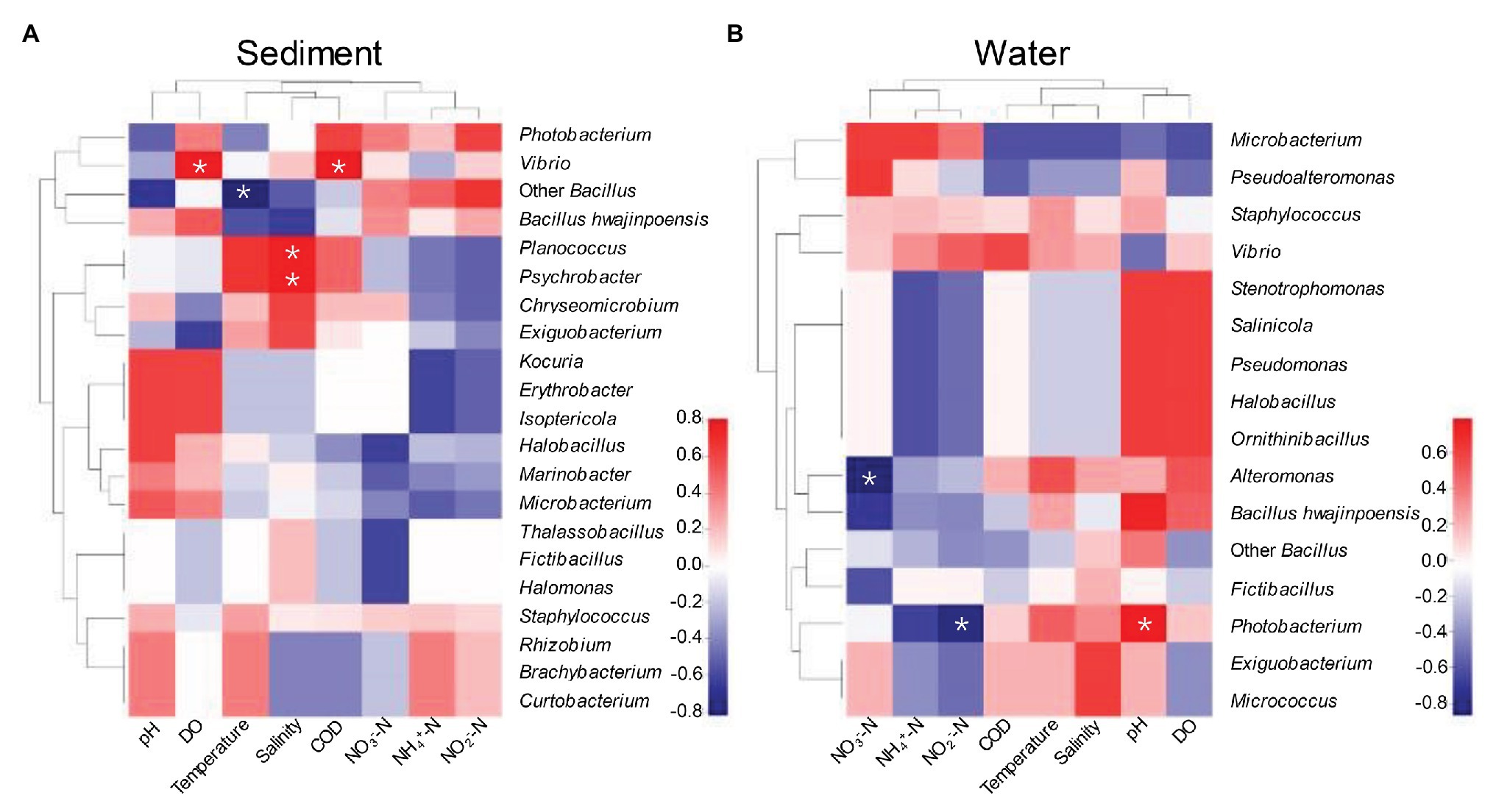
Figure 4. Dendrogram obtained from ERIC-PCR fingerprinting of 80 Vibrio owensii strains. Adopting 58% of similarity, isolates were clustered into four clusters, ET-1, ET-2, ET-3, and ET-4.
Overview of Physicochemical Parameters in the Survey Area
Temperature varied among different sampling aquaculture ponds, ranging from 28.1 to 29.7°C. pH was relatively stable and varied slightly among the different sampling ponds. Salinity ranged from 20.29‰ to 29.98‰. The differences in dissolved inorganic N, such as NH4+-N, NO2—N, and NO3−-N, were obvious. Physical-chemical parameters of the survey areas were summarized in Table 2.
Discussion
With the rapid development of aquaculture industry, aquaculture system is loaded with abundant organic matter, ammonia-nitrogen, and phosphorus due to high concentration of organic and inorganic pollutants from uneaten feed and aquaculture animal excreta (Dat et al., 2019), and long-term use of antibiotics and chemicals to prevent diseases in aquaculture has led to a series of environmental problems, such as water eutrophication and atmospheric pollution (caused by volatilization of ammonia and hydrogen sulfide; Hai, 2015), which greatly hinders the sustainable development of aquaculture industry. Therefore, exploring a harmless and recyclable technology for reducing excessive organic matter in aquaculture system is necessary to ensure the sustainable development of aquaculture and for environmental protection. Microorganisms such as potential probiotic biocontrol candidates play important roles in diseases resistance and improvement of water quality (Blancheton et al., 2013; Hucheng et al., 2020). Abundant enzymes produced by microorganisms, such as protease, lipase, and amylase, can effectively decompose the excessive bait and other organic matter, which play a key role in material transformation and energy metabolism in aquaculture system. Intensive aquaculture system is a closed or semiclosed material circulation system. Protein and other high-molecular substances from bait are the main sources of pollution in aquaculture system. Therefore, aquaculture system is highly dependent on functional bacteria that can promote the decomposition of high-molecular substances, such as protease-producing bacteria. Meanwhile, tropical marine region with unique climate and environmental condition harbors diverse bacterial communities with unique metabolic and physiological capabilities (Hamza et al., 2017; Ren et al., 2018, 2019). Wenchang in Hainan Island belonging to a tropical marine region is one of the largest aquaculture production areas in China, but the knowledge of protease-producing bacterial community structure in this area, especially in aquaculture system, is extremely limited.
For this purpose, we herein communicate that protease-producing bacteria displayed high diversity and complex community structure. The diversity and relative abundance of protease-producing bacteria in aquaculture sediments were generally higher than those in aquaculture water. Similar results were also found in Guangdong (China) aquaculture system (Wenguang et al., 2015). All of the protease-producing bacteria isolates from the survey aquaculture systems belonged to three phyla, Actinobacteria, Firmicutes, and Proteobacteria, of which Firmicutes accounted for the highest proportion, and the dominant genera were Bacillus (37.7%) and Vibrio (28.1%) in aquaculture system, which were similar with that in aquacultured yellowtail (Ramírez and Romero, 2017). However, the relative abundances of these phyla in sediment and water of aquaculture had significant differences. Firmicutes (91.6%) in sediment and Proteobacteria (71.6%) in water accounted for the highest proportion, respectively. Reportedly, some cultivable members of these phyla from aquaculture systems belonged to antibiotic resistance bacteria with (fluoro)quinolone-resistant, sulfamethoxazole-resistant, and oxytetracycline-resistant and were most abundant in fish ponds (Akinbowale et al., 2010; Hoa et al., 2011; Takasu et al., 2011; Wenguang et al., 2015). Firmicutes was described as the most predominant in the intestinal content of aquacultured animals (Ramírez and Romero, 2017). Some researchers reported that the dominant phyla of aquaculture system in Guangdong, China, were Proteobacteria, Bacteroidetes, and Firmicutes in sediment and Proteobacteria, Actinobacteria, and Bacteroidetes in water (Wenguang et al., 2015). Although only less than 1% bacteria of the microbial community could be detected by culture-dependent approach, the living bacteria can be obtained (Ramírez and Romero, 2017), which is an essential method for various researches. We only focused on culturable protease-producing bacterial community structure of tropical aquaculture system in this study; it still indirectly implied significant differences in bacterial community structure between tropical aquaculture system and other regional aquaculture systems. Reportedly, the microbiome of aquaculture systems could be more similar to structure of aquatic animal microbiomes (Ramírez and Romero, 2017).
Additionally, the composition and relative abundance of protease-producing bacteria at the genus level in sediment and water also displayed significantly different in aquaculture systems. Reportedly, Bacillus, Lactobacillus, and Enterococcus as protease-producing bacteria positively affected the bait digestibility and growth of aquaculture animals (Yanbo and Zirong, 2006; Ghosh et al., 2008; Suzer et al., 2008; Iehata et al., 2009; Askarian et al., 2011). In this study, Bacillus dominated by B. hwajinpoensis displayed high relative abundance in both sediment and aquaculture water. Bacillus as probiotic has been commonly selected to improve water quality by reducing organic matter, ammonia-nitrogen, and phosphorus accumulation and inhibit certain pathogenic bacteria of fishery by producing antimicrobial peptides, thereby making them more suitable candidates compared to other probiotics (Rengpipat et al., 1998; Verschuere et al., 2000; Hai, 2015; Kumari et al., 2016; Saravanan et al., 2018; Sonune and Garode, 2018; Yi et al., 2018; Kuebutornye et al., 2019; Wang et al., 2019). SW-72T from a tidal flat of the Yellow Sea in Korea was the first reported B. hwajinpoensis (Yoon et al., 2003). The function of heterotrophic nitrification-aerobic denitrification of B. hwajinpoensis has been reported (Cheng et al., 2016), which can effectively improve water quality by removing inorganic nitrogen and total nitrogen.
The unique genera of sediment or water were also isolated in this work. Twelve unique genera were only isolated from sediment samples, Brachybacterium, Chryseomicrobium, Curtobacterium, Erythrobacter, Halomonas, Isoptericola, Kocuria, Marinobacter, Planococcus, Psychrobacter, Rhizobium, and Thalassobacillus. Meanwhile, there were seven unique genera from water samples, Alteromonas, Micrococcus, Ornithinibacillus, Pseudoalteromonas, Pseudomonas, Salinicola, and Stenotrophomonas. Although these unique genera accounted for tiny percentage in sediment or water, we reason that they played important roles in the stability of community structure. For example, Brachybacterium from Lates calcarifer showed inhibitory activities against Lysinibacillus, Paenibacillus, Pseudomonas, Escherichia coli, and Mesorhizobium (Orsod et al., 2012); Halomonas could improve the survival, growth, water quality, and robustness and modifies the gut microbial composition of shrimp (Gao et al., 2019); Kocuria as probiotic from the intestinal microbiota of rainbow trout showed resistance to sulphatriad and had health benefits in aquaculture (Sharifuzzaman et al., 2018); Marinobacter from a recycling aquaculture system could perform only aerobic denitrification but not nitrification (Liu et al., 2016); Pseudomonas showed antibiofilm properties and reduced the risk of pathogenic infection to aquaculture animals by their exopolysaccharides (Ang et al., 2020).
It is worth to note that Vibrio as the dominant genus was isolated from most aquaculture ponds (Figure 2 and Supplementary Table S2), which were dominated by V. owensii species (80 strains). The DY05T and 47,666-1 belonging to the Harveyi clade of the genus were the first reported V. owensii strains from diseased cultured crustaceans (Cano-Gomez et al., 2010). So far, most of Vibrio from aquaculture systems in southern China belonged to V. harveyi, V. alginolyticus, V. parahaemolyticus, V. splendidus, and V. fischeri, whereas no V. owensii was detected. In this study, V. owensii as dominate species in tropical aquaculture systems could be isolated from most sampling stations, indicating that V. owensii in tropical region may be a normal species and played important roles in material circulation. Reportedly, V. owensii were potential bacterial pathogens in mariculture systems (Yu et al., 2013; Liu et al., 2018), necessitating the designation of an appropriate typing approach to completely fathom their transmission tactics and control infection strategies. So far, there were many reports on the molecular types of V. alginolyticus (Kahla-Nakbi et al., 2006), V. tapetis (Rodríguez et al., 2006), and V. parahaemolyticus (Chen et al., 2012), but no reports on that of V. owensii. ERIC-PCR as an easy and cost-effective genotyping approach for discriminating different strains types (Bakhshi et al., 2018) has urged us to use this technique to inspect phylogenetic closeness of V. owensii isolates. All 80V. owensii isolates produced banding patterns after amplification by ERIC-PCR (Supplementary Figure S2), indicating the complete type ability of V. owensii using this technology, which were clustered into four clusters (ET-1–ET-4) at 58% of similarity (Figure 4). We found that all V. owensii had a common band around 7,000bp (Supplementary Figure S2), indicating that they had a similar genetic background. We reason that the common band may be an effective molecular marker for V. owensii. The predominant clusters, ET-3 and ET-4, contained most isolates, indicating that these two genotypes were representative strains in tropical aquaculture. Additionally, the V. owensii strains belonging to ET-3 and ET-4 were detected in most ponds without outbreak of epidemics in this region (Supplementary Table S1) and significantly different from V. owensii DY05T from other regions on the basis of 16S rDNA (Supplementary Figure S3), implying that V. owensii strains in this area may be potential beneficial bacteria for the wastewater purification and the construction of an environment-friendly aquaculture model in tropical region, especially in Hainan Island.
Our experimental design aimed to determine the relative influence of environmental variables of aquaculture on protease-producing microbial communities in aquaculture system. Environmental variables must play important roles in shaping protease-producing bacterial diversity and community structure in aquaculture systems (Holmström and Kjelleberg, 1999; Li et al., 2018). Therefore, Spearman rank correlation coefficient between relative abundance of protease-producing bacterial communities and environmental variables at the genus level was investigated (Figure 5). As shown in Figure 5A, DO and COD were the main factors positively affecting the distributions of dominant Vibrio in sediment, and salinity as a main factor also positively affected the distributions of unique genera of sediment, Planococcus and Psychrobacter. Planococcus spp. were associated with biological sulfamethoxazole degradation pathway, which can respond more quickly and adapt to environmental changes in aquaculture system (Kong et al., 2020). It is worth mentioning that the antagonistic effect against pathogenic species of Psychrobacter has been demonstrated and reported (Sun et al., 2009), and Psychrobacter could be commonly found in intestinal microbiota of several fish (Ringo et al., 2006; Sun et al., 2009; Yang et al., 2011; Ramirez and Romero, 2017). Reportedly, Psychrobacter could also improve immunological parameters and growth performance in tilapia (Makled et al., 2017), whereas temperature was the main factor negatively affecting the distribution of dominant Bacillus (except B. hwajinpoensis) in sediment, which was agreed with some previous studies in different regional oceans (Pomeroy and Wiebe, 2001; Fuhrman et al., 2008; Li et al., 2018). In this study, DO, COD, salinity, and temperature played important roles in shaping bacterial diversity of aquaculture sediment by affecting the key species. The conditions with low concentration of NO3−-N and NO2−-N were more suitable for the growth of Alteromonas and Photobacterium, and pH as a main factor positively affecting the distribution of Photobacterium in water (Figure 5B). Photobacterium genus was detected worldwide in aquaculture systems and identified as pathogens causing wound infections and hemorrhagic septicemia (Terceti et al., 2018; Labella et al., 2020). It is worth noticing that Alteromonas was only isolated from aquaculture water, which was identified as probiotic bacteria in aquaculture system (Kesarcodi-Watson et al., 2010; Torres et al., 2016). In conclusion, DO, temperature, salinity, and COD as the main factor could affect the protease-producing bacterial community structure of sediment, whereas pH, NO2—N, and NO3−-N were the main factors affecting that of aquaculture water.
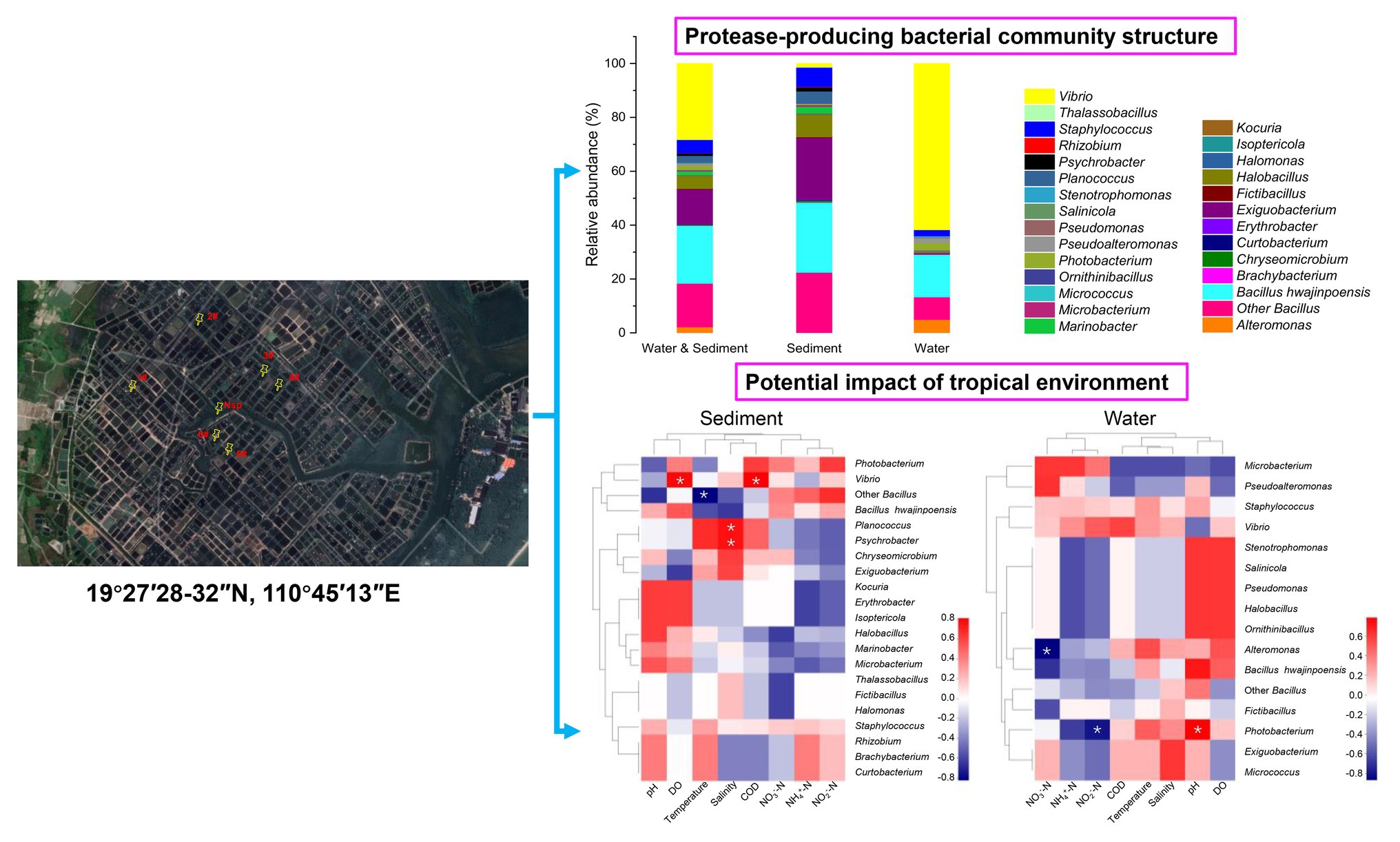
Figure 5. Spearman rank correlation coefficient between relative abundance of bacterial communities in sediment (A) and aquaculture water (B) and environmental variables at the genus. *p < 0.05 indicates significant correlation level.
Conclusion
In this study, cultivable protease-producing bacterial community structures in sediment and water of tropical aquaculture systems were completely surveyed. The protease-producing bacteria displayed high diversity and complex community structure. Bacillus genera in sediment and Vibrio in aquaculture water were the dominant genera. V. owensii strains as the dominant Vibrio species were clustered into four clusters, which was identified as a novel branch of V. owensii. Additionally, temperature, DO, COD, salinity, NO3−-N, NO2—N, and pH of aquaculture system played important roles in shaping protease-producing bacterial diversity and community structure in aquaculture system. These results will help the development useful and practical strategies for the improvement of water quality in the marine recirculating aquaculture system.
Data Availability Statement
The original contributions presented in the study are included in the article/Supplementary Material, further inquiries can be directed to the corresponding authors.
Author Contributions
YW, JB, WR, and ZX designed the study and wrote the manuscript. YW, JB, HL, XZ, XC, and AH performed the experiments. YW, WR, and ZX analyzed the data. YW and JB contributed equally to this work. All authors contributed to the article and approved the submitted version.
Funding
This research was financially supported by the Key Research and Development Project of Hainan Province (ZDYF2020088), the National Key Research and Development Project of China (2020YFD0901100), Marine Economic and Innovative Demonstration City Project of State Oceanic Administration (HHCL201802 and HHCL201813), the Foundation of Hainan Agricultural and Rural Department (NY-2019-819), the National Natural Science Foundation of China (31660744), and the Scientific Research Foundation of Hainan University (KYQD(ZR)1967, KYQD(ZR)1819 and KYQD(ZR)20060).
Conflict of Interest
The authors declare that the research was conducted in the absence of any commercial or financial relationships that could be construed as a potential conflict of interest.
Acknowledgments
We are thankful to the reviewers who helped improve this paper. We are also thankful to Mengjie Cheng from Hainan University for her support in data analysis.
Supplementary Material
The Supplementary Material for this article can be found online at: https://www.frontiersin.org/articles/10.3389/fmicb.2021.638129/full#supplementary-material
Footnotes
References
Akinbowale, O. L., Peng, H., and Barton, M. D. (2010). Diversity of tetracycline resistance genes in bacteria from aquaculture sources in Australia. J. Appl. Microbiol. 103, 2016–2025. doi: 10.1111/j.1365-2672.2007.03445.x
Ambas, I., Buller, N., and Fotedar, R. (2015). Isolation and screening of probiotic candidates from marron, C herax cainii (Austin, 2002) gastrointestinal tract (GIT) and commercial probiotic products for the use in marron culture. J. Fish Dis. 38, 467–476. doi: 10.1111/jfd.12257
Amin, M. (2018). Marine protease-producing bacterium and its potential use as an abalone probiont. Aquacult. Rep. 12, 30–35. doi: 10.1016/j.aqrep.2018.09.004
Ang, C. Y., Sano, M., Dan, S., Leelakriangsak, M., and Lal, T. M. (2020). Postbiotics applications as infectious disease control agent in aquaculture. Biocontrol Sci. 25, 1–7. doi: 10.4265/bio.25.1
Askarian, F., Kousha, A., Salma, W., and Ringø, E. (2011). The effect of lactic acid bacteria administration on growth, digestive enzyme activity and gut microbiota in Persian sturgeon (Acipenser persicus) and beluga (Huso huso) fry. Aquac. Nutr. 17, 488–497. doi: 10.1111/j.1365-2095.2010.00826.x
Bakhshi, B., Afshari, N., and Fallah, F. (2018). Enterobacterial repetitive intergenic consensus (ERIC)-PCR analysis as a reliable evidence for suspected Shigella spp. outbreaks. Braz. J. Microbiol. 49, 529–533. doi: 10.1016/j.bjm.2017.01.014
Blancheton, J., Attramadal, K., Michaud, L., D’orbcastel, E. R., and Vadstein, O. (2013). Insight into bacterial population in aquaculture systems and its implication. Aquac. Eng. 53, 30–39. doi: 10.1016/j.aquaeng.2012.11.009
Cano-Gomez, A., Goulden, E. F., Owens, L., and Høj, L. (2010). Vibrio owensii sp. nov., isolated from cultured crustaceans in Australia. FEMS Microbiol. Lett. 302, 175–181. doi: 10.1111/j.1574-6968.2009.01850.x
Chen, W., Xie, Y., Xu, J., Wang, Q., Gu, M., Yang, J., et al. (2012). Molecular typing of Vibrio parahaemolyticus isolates from the middle-east coastline of China. Int. J. Food Microbiol. 153, 402–412. doi: 10.1016/j.ijfoodmicro.2011.12.001
Cheng, Y., Li, Q. -F., Fei, Y. -T., and Zhang, Y. (2016). Screening and nitrogen removing characteristics of heterotrophic nitrification-aerobic denitrification bacteria SLWX2 from sea water. Huanjing Kexue. 37, 2681–2688. doi: 10.13227/j.hjkx.2016.07.035
Dat, T., Tam, V., Dung, T., Bui, L., Anh, H., and Oanh, P. (2019). Isolation and screening of cellulose and organic matter degrading bacteria from aquaculture ponds for improving water quality in aquaculture. IOP Conf. Se.: Earth and Environ. Sci. 266:012002. doi: 10.1088/1755-1315/266/1/012002
Fuhrman, J. A., Steele, J. A., Hewson, I., Schwalbach, M. S., Brown, M. V., Green, J. L., et al. (2008). A latitudinal diversity gradient in planktonic marine bacteria. Proc. Natl. Acad. Sci. U. S. A. 105, 7774–7778. doi: 10.1073/pnas.0803070105
Gao, M., Du, D., Bo, Z., and Sui, L. (2019). Poly-beta-hydroxybutyrate (PHB)-accumulating Halomonas improves the survival, growth, robustness and modifies the gut microbial composition of Litopenaeus vannamei postlarvae. Aquaculture 500, 607–612. doi: 10.1016/j.aquaculture.2018.10.032
Ghosh, S., Sinha, A., and Sahu, C. (2008). Dietary probiotic supplementation in growth and health of live-bearing ornamental fishes. Aquac. Nutr. 14, 289–299. doi: 10.1111/j.1365-2095.2007.00529.x
Guo, H., Xian, J. -A., and Wang, A. L. (2016). Analysis of digital gene expression profiling in hemocytes of white shrimp Litopenaeus vannamei under nitrite stress. Fish Shellfish Immunol. 56, 1–11. doi: 10.1016/j.fsi.2016.06.059
Hai, N. (2015). The use of probiotics in aquaculture. J. Appl. Microbiol. 119, 917–935. doi: 10.1111/jam.12886
Hamza, F., Satpute, S., Banpurkar, A., Kumar, A. R., and Zinjarde, S. (2017). Biosurfactant from a marine bacterium disrupts biofilms of pathogenic bacteria in a tropical aquaculture system. FEMS Microbiol. Ecol. 93:fix140. doi: 10.1093/femsec/fix140
Hoa, P. T. P., Managaki, S., Nakada, N., Takada, H., Shimizu, A., Anh, D. H., et al. (2011). Antibiotic contamination and occurrence of antibiotic-resistant bacteria in aquatic environments of northern Vietnam. Sci. Total Environ. 409, 2894–2901. doi: 10.1016/j.scitotenv.2011.04.030
Holmström, C., and Kjelleberg, S. (1999). Marine Pseudoalteromonas species are associated with higher organisms and produce biologically active extracellular agents. FEMS Microbiol. Ecol. 30, 285–293. doi: 10.1111/j.1574-6941.1999.tb00656.x
Hucheng, J., Xiaohui, C., Wenji, B., Longlong, F., Qin, Q., Liqiang, Z., et al. (2020). Comparison of bacterial communities in channel catfish Ictalurus punctatus culture ponds of an industrial ecological purification recirculating aquaculture system. Aquac. Res. 51, 2432–2442. doi: 10.1111/are.14587
Iehata, S., Inagaki, T., Okunishi, S., Nakano, M., Tanaka, R., and Maeda, H. (2009). Colonization and probiotic effects of lactic acid bacteria in the gut of the abalone Haliotis gigantea. Fish. Sci. 75, 1285–1293. doi: 10.1007/s12562-009-0138-5
Kahla-Nakbi, A. B., Chaieb, K., Besbes, A., Zmantar, T., and Bakhrouf, A. (2006). Virulence and enterobacterial repetitive intergenic consensus PCR of Vibrio alginolyticus strains isolated from Tunisian cultured gilthead sea bream and sea bass outbreaks. Vet. Microbiol. 117, 321–327. doi: 10.1016/j.vetmic.2006.06.012
Kesarcodi-Watson, A., Kaspar, H., Lategan, M. J., and Gibson, L. (2010). Alteromonas macleodii 0444 and Neptunomonas sp. 0536, two novel probiotics for hatchery-reared Greenshell ™ mussel larvae, Perna canaliculus. Aquaculture 309, 49–55. doi: 10.1016/j.aquaculture.2010.09.019
Kong, S., Zhao, Y. -G., Guo, L., Gao, M., Jin, C., and She, Z. (2020). Transcriptomics of Planococcus kocurii O516 reveals the degrading metabolism of sulfamethoxazole in marine aquaculture wastewater. Environ. Pollut. 265:114939. doi: 10.1016/j.envpol.2020.114939
Kuebutornye, F. K., Abarike, E. D., and Lu, Y. (2019). A review on the application of Bacillus as probiotics in aquaculture. Fish Shellfish Immunol. 87, 820–828. doi: 10.1016/j.fsi.2019.02.010
Kuhn, D. D., Smith, S. A., Boardman, G. D., Angier, M. W., Marsh, L., and Flick, G. J. Jr. (2010). Chronic toxicity of nitrate to pacific white shrimp, Litopenaeus vannamei: impacts on survival, growth, antennae length, and pathology. Aquaculture 309, 109–114. doi: 10.1016/j.aquaculture.2010.09.014
Kumari, V., Yadav, A., Haq, I., Kumar, S., Bharagava, R. N., Singh, S. K., et al. (2016). Genotoxicity evaluation of tannery effluent treated with newly isolated hexavalent chromium reducing Bacillus cereus. J. Environ. Manag. 183, 204–211. doi: 10.1016/j.jenvman.2016.08.017
Labella, A. M., Rosado, J. J., Balado, M., Lemos, M. L., and Borrego, J. J. (2020). Virulence properties of three new Photobacterium species affecting cultured fish. J. Appl. Microbiol. 129, 37–50. doi: 10.1111/jam.14437
Li, Y. -Y., Chen, X. -H., Xie, Z. -X., Li, D. -X., Wu, P. -F., Kong, L. -F., et al. (2018). Bacterial diversity and nitrogen utilization strategies in the upper layer of the northwestern Pacific Ocean. Front. Microbiol. 9:797. doi: 10.3389/fmicb.2018.00797
Li, Y., Wang, L., Yan, Z., Chao, C., Yu, H., Yu, D., et al. (2020). Effectiveness of dredging on internal phosphorus loading in a typical aquacultural lake. Sci. Total Environ. 744:140883. doi: 10.1016/j.scitotenv.2020.140883
Lin, Y. C., and Chen, J. C. (2001). Acute toxicity of ammonia on Litopenaeus vannamei Boone juveniles at different salinity levels. J. Exp. Mar. Biol. Ecol. 259, 109–119. doi: 10.1016/S0022-0981(01)00227-1
Lin, Y. -C., and Chen, J. -C. (2003). Acute toxicity of nitrite on Litopenaeus vannamei (Boone) juveniles at different salinity levels. Aquaculture 224, 193–201. doi: 10.1016/S0044-8486(03)00220-5
Liu, Y., Ai, G. -M., Miao, L. -L., and Liu, Z. -P. (2016). Marinobacter strain NNA5, a newly isolated and highly efficient aerobic denitrifier with zero N2O emission. Bioresour. Technol. 206, 9–15. doi: 10.1016/j.biortech.2016.01.066
Liu, L., Xiao, J., Zhang, M., Zhu, W., Xia, X., Dai, X., et al. (2018). A Vibrio owensii strain as the causative agent of AHPND in cultured shrimp, Litopenaeus vannamei. J. Invertebr. Pathol. 153, 156–164. doi: 10.1016/j.jip.2018.02.005
Makled, S. O., Hamdan, A. M., El-Sayed, A. -F. M., and Hafez, E. E. (2017). Evaluation of marine psychrophile, Psychrobacter namhaensis SO89, as a probiotic in Nile tilapia (Oreochromis niloticus) diets. Fish Shellfish Immunol. 61, 194–200. doi: 10.1016/j.fsi.2017.01.001
Mariane De Morais, A. P., Abreu, P. C., Wasielesky, W., and Krummenauer, D. (2020). Effect of aeration intensity on the biofilm nitrification process during the production of the white shrimp Litopenaeus vannamei (Boone, 1931) in Biofloc and clear water systems. Aquaculture 514:734516. doi: 10.1016/j.aquaculture.2019.734516
Marquez-Santacruz, H. A., Hernandez-Leon, R., Orozco-Mosqueda, M. C., Velazquez-Sepulveda, I., and Santoyo, G. (2010). Diversity of bacterial endophytes in roots of Mexican husk tomato plants (Physalis ixocarpa) and their detection in the rhizosphere. Genet. Mol. Res. 9, 2372–2380. doi: 10.4238/vol9-4gmr921
Olson, N. D., and Lesser, M. P. (2013). Diazotrophic diversity in the Caribbean coral, Montastraea cavernosa. Arch. Microbiol. 195, 853–859. doi: 10.1007/s00203-013-0937-z
Orsod, M., Joseph, M., and Huyop, F. (2012). Characterization of exopolysaccharides produced by Bacillus cereus and Brachybacterium sp. isolated from Asian sea bass (Lates calcarifer). Mal. J. Microbiol. 8, 170–174. doi: 10.1006/geno.2001.6469
Pishbin, Z., and Mehrabian, S. (2020). Molecular analysis of Proteus mirabilis isolated from urine samples using ERIC-PCR. Gene Reports. 19:100637. doi: 10.1016/j.genrep.2020.100637
Pomeroy, L. R., and Wiebe, W. J. (2001). Temperature and substrates as interactive limiting factors for marine heterotrophic bacteria. Aquat. Microb. Ecol. 23, 187–204. doi: 10.3354/ame01431
Ramirez, C., and Romero, J. (2017). Fine flounder (Paralichthys adspersus) microbiome showed important differences between wild and reared specimens. Front. Microbiol. 8:271. doi: 10.3389/fmicb.2017.00271
Ramírez, C., and Romero, J. (2017). The microbiome of Seriola lalandi of wild and aquaculture origin reveals differences in composition and potential function. Front. Microbiol. 8:1844. doi: 10.3389/fmicb.2017.01844
Reda, R. M., and Selim, K. M. (2015). Evaluation of Bacillus amyloliquefaciens on the growth performance, intestinal morphology, hematology and body composition of Nile tilapia, Oreochromis niloticus. Aquac. Int. 23, 203–217. doi: 10.1007/s10499-014-9809-z
Ren, W., Cai, R., Yan, W., Lyu, M., Fang, Y., and Wang, S. (2018). Purification and characterization of a biofilm-degradable dextranase from a marine bacterium. Mar. Drugs 16:51. doi: 10.3390/md16020051
Ren, W., Liu, L., Gu, L., Yan, W., Feng, Y. L., Dong, D., et al. (2019). Crystal structure of GH49 dextranase from Arthrobacter oxidans KQ11: identification of catalytic base and improvement of thermostability using semirational design based on B-factors. J. Agric. Food Chem. 67, 4355–4366. doi: 10.1021/acs.jafc.9b01290
Rengpipat, S., Phianphak, W., Piyatiratitivorakul, S., and Menasveta, P. (1998). Effects of a probiotic bacterium on black tiger shrimp Penaeus monodon survival and growth. Aquaculture 167, 301–313. doi: 10.1016/S0044-8486(98)00305-6
Ringo, E., Sperstad, S., Myklebust, R., Refstie, S., and Krogdahl, A. (2006). Characterisation of the microbiota associated with intestine of Atlantic cod (Gadus morhua L.) - the effect of fish meal, standard soybean meal and a bioprocessed soybean meal. Aquaculture 261, 829–841. doi: 10.1016/j.aquaculture.2006.06.030
Rodríguez, J. M., López-Romalde, S., Beaz, R., Alonso, M. C., Castro, D., and Romalde, J. L. (2006). Molecular fingerprinting of Vibrio tapetis strains using three PCR-based methods: ERIC-PCR, REP-PCR and RAPD. Dis. Aquat. Org. 69, 175–183. doi: 10.3354/dao069175
Santos, L., and Ramos, F. (2018). Antimicrobial resistance in aquaculture: current knowledge and alternatives to tackle the problem. Int. J. Antimicrob. Agents 52, 135–143. doi: 10.1016/j.ijantimicag.2018.03.010
Saravanan, M., Barik, S. K., Mubarakali, D., Prakash, P., and Pugazhendhi, A. (2018). Synthesis of silver nanoparticles from Bacillus brevis (NCIM 2533) and their antibacterial activity against pathogenic bacteria. Microb. Pathog. 116, 221–226. doi: 10.1016/j.micpath.2018.01.038
Selim, K. M., and Reda, R. M. (2015). Improvement of immunity and disease resistance in the Nile tilapia, Oreochromis niloticus, by dietary supplementation with Bacillus amyloliquefaciens. Fish Shellfish Immunol. 44, 496–503. doi: 10.1016/j.fsi.2015.03.004
Sharifuzzaman, S. M., Rahman, H., Austin, D. A., and Austin, B. (2018). Properties of probiotics Kocuria SM1 and Rhodococcus SM2 isolated from fish guts. Probiotics Antimicrob. Proteins. 10, 534–542. doi: 10.1007/s12602-017-9290-x
Shi, Z., Li, X. -Q., Chowdhury, M. K., Chen, J. -N., and Leng, X. -J. (2016). Effects of protease supplementation in low fish meal pelleted and extruded diets on growth, nutrient retention and digestibility of gibel carp, Carassius auratus gibelio. Aquaculture 460, 37–44. doi: 10.1016/j.aquaculture.2016.03.049
Sonune, N., and Garode, A. (2018). Isolation, characterization and identification of extracellular enzyme producer Bacillus licheniformis from municipal wastewater and evaluation of their biodegradability. Biotechnol. Res. Inno. 2, 37–44. doi: 10.1016/j.biori.2018.03.001
Su, H., Xiao, Z., Yu, K., Huang, Q., Wang, G., Wang, Y., et al. (2020). Diversity of cultivable protease-producing bacteria and their extracellular proteases associated to scleractinian corals. PeerJ. 8:e9055. doi: 10.7717/peerj.9055
Sun, L., Qiu, F., Zhang, X., Dai, X., Dong, X., and Song, W. (2008). Endophytic bacterial diversity in rice (Oryza sativa L.) roots estimated by 16S rDNA sequence analysis. Microb. Ecol. 55, 415–424. doi: 10.1007/s00248-007-9287-1
Sun, Y., Yang, H., Ling, Z., Chang, J., and Ye, J. (2009). Gut microbiota of fast and slow growing grouper Epinephelus coioides. Afr. J. Microbiol. Res. 3, 637–640. doi: 10.5897/AJMR.9000353
Suzer, C., Çoban, D., Kamaci, H. O., Saka, Ş., Firat, K., Otgucuoğlu, Ö., et al. (2008). Lactobacillus spp. bacteria as probiotics in gilthead sea bream (Sparus aurata, L.) larvae: effects on growth performance and digestive enzyme activities. Aquaculture 280, 140–145. doi: 10.1016/j.aquaculture.2008.04.020
Takasu, H., Suzuki, S., Reungsang, A., and Viet, P. H. (2011). Fluoroquinolone (FQ) contamination does not correlate with occurrence of FQ-resistant bacteria in aquatic environments of Vietnam and Thailand. Microbes Environ. 26, 135–143. doi: 10.1264/jsme2.ME10204
Terceti, M. S., Vences, A., Matanza, X. M., Dalsgaard, I., Pedersen, K., and Osorio, C. R. (2018). Molecular epidemiology of Photobacterium damselae subsp damselae outbreaks in marine rainbow trout farms reveals extensive horizontal gene transfer and high genetic diversity. Front. Microbiol. 9:2155. doi: 10.3389/fmicb.2018.02155
Torres, M., Rubio-Portillo, E., Anton, J., Ramos-Espla, A. A., Quesada, E., and Llamas, I. (2016). Selection of the N-Acylhomoserine lactone-degrading bacterium Alteromonas stellipolaris PQQ-42 and of its potential for biocontrol in aquaculture. Front. Microbiol. 7:646. doi: 10.3389/fmicb.2016.00646
Verschuere, L., Rombaut, G., Sorgeloos, P., and Verstraete, W. (2000). Probiotic bacteria as biological control agents in aquaculture. Microbiol. Mol. Biol. Rev. 64, 655–671. doi: 10.1023/A:1024127100993
Wang, Y., Bi, L., Liao, Y., Lu, D., Zhang, H., Liao, X., et al. (2019). Influence and characteristics of Bacillus stearothermophilus in ammonia reduction during layer manure composting. Ecotoxicol. Environ. Saf. 180, 80–87. doi: 10.1016/j.ecoenv.2019.04.066
Wenguang, X., Yongxue, S., Tong, Z., Ding, X., Li, Y., Wang, M., et al. (2015). Antibiotics, antibiotic resistance genes, and bacterial community composition in fresh water aquaculture environment in China. Microb. Ecol. 70, 425–532. doi: 10.1007/s00248-015-0583-x
Yanbo, W., and Zirong, X. (2006). Effect of probiotics for common carp (Cyprinus carpio) based on growth performance and digestive enzyme activities. Anim. Feed Sci. Technol. 127, 283–292. doi: 10.1016/j.anifeedsci.2005.09.003
Yang, H. L., Sun, Y. Z., Ma, R. L., Li, J. S., and Huang, K. P. (2011). Probiotic Psychrobacter sp. improved the autochthonous microbial diversity along the gastrointestinal tract of grouper Epinephelus coioides. J. Aquac. Res. Dev. s1:001. doi: 10.4172/2155-9546.S1-001
Yi, Y., Zhang, Z., Zhao, F., Liu, H., Yu, L., Zha, J., et al. (2018). Probiotic potential of Bacillus velezensis JW: antimicrobial activity against fish pathogenic bacteria and immune enhancement effects on Carassius auratus. Fish Shellfish Immunol. 78, 322–330. doi: 10.1016/j.fsi.2018.04.055
Yoon, J. -H., Kim, I. -G., Kang, K. H., Oh, T. -K., and Park, Y. -H. (2003). Bacillus marisflavi sp. nov. and Bacillus aquimaris sp. nov., isolated from sea water of a tidal flat of the Yellow Sea in Korea. J. Med. Microbiol. 53, 1297–1303. doi: 10.1016/S0370-2693(97)01497-4
Keywords: protease-producing bacteria, Vibrio owensii , tropical aquaculture, enterobacterial repetitive intergenic consensus-polymerase chain reaction, environmental variables, Bacillus hwajinpoensis , bacterial community structure
Citation: Wei Y, Bu J, Long H, Zhang X, Cai X, Huang A, Ren W and Xie Z (2021) Community Structure of Protease-Producing Bacteria Cultivated From Aquaculture Systems: Potential Impact of a Tropical Environment. Front. Microbiol. 12:638129. doi: 10.3389/fmicb.2021.638129
Edited by:
Konstantinos Ar. Kormas, University of Thessaly, GreeceReviewed by:
Jiazhang Chen, Chinese Academy of Fishery Sciences, ChinaHailun He, Central South University, China
Copyright © 2021 Wei, Bu, Long, Zhang, Cai, Huang, Ren and Xie. This is an open-access article distributed under the terms of the Creative Commons Attribution License (CC BY). The use, distribution or reproduction in other forums is permitted, provided the original author(s) and the copyright owner(s) are credited and that the original publication in this journal is cited, in accordance with accepted academic practice. No use, distribution or reproduction is permitted which does not comply with these terms.
*Correspondence: Wei Ren, cmVud2VpZmx5QDEyNi5jb20=; Zhenyu Xie, eGllenlzY3V0YUAxNjMuY29t; OTk0Mzk0QGhhaW5hbnUuZWR1LmNvbQ==
†These authors have contributed equally to this work