- 1Department of Biological Safety, German Federal Institute for Risk Assessment, Berlin, Germany
- 2Department of Aquaculture, Korea National College of Agriculture and Fisheries, Jeonju, South Korea
- 3Department of Bacteriology and Immunology, Medicum, Human Microbiome Research Program, Faculty of Medicine, University of Helsinki, Helsinki, Finland
- 4Division of Clinical Microbiology, HUSLAB, Helsinki University Hospital, Helsinki, Finland
Yersinia pseudotuberculosis is an important animal pathogen, particularly for birds, rodents, and monkeys, which is also able to infect humans. Indeed, an increasing number of reports have been published on zoo animals that were killed by this species. One option to treat diseased animals is the application of strictly lytic (virulent) phages. However, thus far relatively few phages infecting Y. pseudotuberculosis have been isolated and characterized. To determine the prevalence of Y. pseudotuberculosis phages in zoo animals, fecal samples of birds and some primates, maras, and peccaries kept in the Tierpark Berlin were analyzed. Seventeen out of 74 samples taken in 2013 and 2017 contained virulent phages. The isolated phages were analyzed in detail and could be allocated to three groups. The first group is composed of 10 T4-like phages (PYps2T taxon group: Myoviridae; Tevenvirinae; Tequatrovirus), the second group (PYps23T taxon group: Chaseviridae; Carltongylesvirus; Escherichia virus ST32) consists of five phages encoding a podovirus-like RNA polymerase that is related to an uncommon genus of myoviruses (e.g., Escherichia coli phage phiEcoM-GJ1), while the third group is comprised of two podoviruses (PYps50T taxon group: Autographiviridae; Studiervirinae; Berlinvirus) which are closely related to T7. The host range of the isolated phages differed significantly. Between 5.5 and 86.7% of 128 Y. pseudotuberculosis strains belonging to 20 serotypes were lysed by each phage. All phages were additionally able to lyse Y. enterocolitica B4/O:3 strains, when incubated at 37°C. Some phages also infected Y. pestis strains and even strains belonging to other genera of Enterobacteriaceae. A cocktail containing two of these phages would be able to lyse almost 93% of the tested Y. pseudotuberculosis strains. The study indicates that Y. pseudotuberculosis phages exhibiting a broad-host range can be isolated quite easily from zoo animals, particularly birds.
Introduction
The genus Yersinia is currently composed of 28 species of which three are known to be pathogenic for humans.1 Yersinia pestis is the causative agent of Plague. Yersinia pseudotuberculosis and Y. enterocolitica are enteropathogenic species which may cause diseases termed yersiniosis (Carniel, 2001). Yersiniosis is the fourth most common bacterial enteritis in Europe, which is mainly caused by Y. enterocolitica (European Food Safety Authority, 2021). However, an increasing number of human yersiniosis outbreaks have also been reported for Y. pseudotuberculosis (Inoue et al., 1984; Tertti et al., 1984; Nakano et al., 1989; Press et al., 2001; Hallanvuo et al., 2003; Jalava et al., 2004, 2006; Nuorti et al., 2004; Rimhanen-Finne et al., 2009; Nakamura et al., 2013; Pärn et al., 2014; Vasala et al., 2014; Williamson et al., 2016). This species is very closely related to Y. pestis and therefore, it is not surprising that Y. pseudotuberculosis and Y. enterocolitica have different reservoirs. In Europe, the presence of Y. enterocolitica is clearly associated with pigs (Fredriksson-Ahomaa et al., 2006a,b). Infections by this species are mainly caused by the consumption of contaminated pork (Laukkanen et al., 2008). By contrast, the host spectrum of Y. pseudotuberculosis is more diverse, as this species is mainly an animal pathogen, which more rarely infects humans (Nikolova et al., 2001; Nakamura et al., 2015). Indeed, there is a growing number of reports describing the occurrence of Y. pseudotuberculosis in, e.g., wild boars and zoo animals (Iwata et al., 2008; Fredriksson-Ahomaa et al., 2011; Fogelson et al., 2015; Nakamura et al., 2015; Arrausi-Subiza et al., 2016; Reinhardt et al., 2018a,b). Y. pseudotuberculosis infections and outbreaks among zoo animals have been a problem for decades and therefore, vaccinations are in use in some zoos. Particularly birds quite often carry this pathogen, which can be lethal for these animals (Cork et al., 1999; Galosi et al., 2015; Nakamura et al., 2016; Shopland et al., 2020). Treatment with antibiotics might be an option but multidrug resistance encoded by conjugative plasmids has already been described for Y. pseudotuberculosis (Cabanel et al., 2017). To prevent further resistance development, one alternative approach to treat bacterial infections is the application of virulent (strictly lytic) phages. Phages are viruses which exclusively infect bacteria. They generally have a narrow host range and occur everywhere where their hosts are living. Moreover, virulent phages are also effective against multidrug-resistant bacteria and have a self-replicative mode of action (Wang et al., 2010). On the other hand, temperate phages may integrate their genome into the bacterial chromosome or may reside as a plasmid, which is then called a prophage. Since temperate phages sometimes contain virulence or antibiotic resistance genes, which can be transmitted by horizontal gene transfer to other bacteria, they are not suited for phage therapy (Owens et al., 2013). Compared to the two other human-pathogenic Yersinia species, there are only few reports on the isolation and characterization of Y. pseudotuberculosis phages, although some Y. pestis phages are able to lyse Y. pseudotuberculosis and even showed a lytic activity on certain Escherichia coli, Klebsiella pneumoniae, and Shigella sonnei strains (Filippov et al., 2012; Rashid et al., 2012). The broad-host range phages PY100, vB_YenP_Rambo (Rambo) and vB_YenM_P281 (P281) did not only infect the three pathogenic, but also some non-pathogenic Yersinia species (Schwudke et al., 2008; Hammerl et al., 2021). By contrast, eight phages exclusively infecting Y. pseudotuberculosis O:1a strains were isolated from pig stools (Salem et al., 2015). All stools from which these phages were recovered, were negative for Y. pseudotuberculosis O:1a suggesting that the phages might have replicated in another host (Salem et al., 2015). Recently, three other T4-like phages from pig stools infecting Y. pseudotuberculosis and Y. pestis using LPS and OmpF as receptors were reported (Salem et al., 2021).
In this work for the first-time, fecal samples from animals kept in a zoo in Berlin were screened for the presence of phages able to infect Y. pseudotuberculosis. We wanted to know whether zoo animals may carry virulent or temperate Y. pseudotuberculosis phages and which properties they have. The isolated phages were characterized in terms of their morphology, host range, and genome composition with special emphasis on tail fiber proteins that are important determinants for host specificity.
Materials and Methods
Bacterial Strains and Culture Conditions
Overall, 287 bacterial strains used in this study were selected from the strain collections of the Consiliary Laboratory for Yersinia at the German Federal Institute for Risk Assessment (BfR), Berlin, Germany and of the Skurnik laboratory at University of Helsinki, Finland. Information on the species and serotypes of strains is given in Tables 1 and 2. If not otherwise indicated, Yersinia sp. bacteria were cultivated in lysogeny broth (LB) at 28°C with continuous shaking at 200–225 rpm (Hammerl et al., 2008), while other Enterobacteriaceae were incubated in LB at 37°C.
Isolation, Propagation, and Purification of Phages
In total, 74 fecal samples taken from 56 birds and 18 mammals (Chacoan peccaries, Patagonian maras, and various monkeys) in the zoo Tierpark Berlin were analyzed in August 2013 and July 2017 (Supplementary Table S1). Samples collected from the ground were immediately suspended on site in approximately 5 ml 0.85% NaCl solution. After arrival in the laboratory, 45 ml of SM buffer (Jäckel et al., 2015) was added to further suspend the samples. Following this, 10 ml of the material was transferred to a centrifuge tube and spun for 20 min at 8,000 rpm and 10°C. The supernatants were passed through 0.45 μm pore-size filters (VWR International, Darmstadt, Germany) and stored at 4°C until they were used to determine lytic activity. This was done by spotting 10 μl of serial dilutions of each sample onto a lawn of 20 Y. pseudotuberculosis indicator strains belonging to the serotypes O:1a, b; O:2a, b, c; O:3, O:4a, b, c; O:5a, b; and O:6, O:7. After incubation overnight at 28°C, plaques were inspected. Individual phages were isolated by 3-fold recovery of single plaques. High-titer lysates of the phages were obtained by infecting 200 ml cultures of the indicator strain (OD588 = 0.5) with phages at a MOI of 0.01 to 0.1. After cell lysis, lysates were centrifuged for 20 min at 10,000 x g to remove debris and then filtered (see above). Phages were concentrated by ultracentrifugation and purified using CsCl step gradients (Jäckel et al., 2017).
Host Range Determination
The host range of the phages was determined twice by activity assays. 100 μl of the respective indicator strain was mixed with 6 ml prewarmed NZCYM soft agar (0.6%) and poured onto a LB agar plate (Jäckel et al., 2015). Ten μl of serial dilutions of each lysate (adjusted to ~1 × 107 pfu/ml) was spotted onto the overlay agar. Plates were incubated overnight at 28°C or 37°C. Lytic activity of the phages was identified by single plaques.
Transmission Electron Microscopy
CsCl-purified phages were investigated by TEM using the negative staining procedure with uranyl acetate. Briefly, drops of phage preparations were applied to pioloform-carbon-coated, 400-mesh copper grids (Plano GmbH, Wetzlar, Germany), incubated for 10 min, and fixed with 2.5% aqueous glutaraldehyde (Taap Laboratories, Aldermaston, United Kingdom) for 1 min. Thereafter, phages were stained with a 2% aqueous uranyl acetate (Merck, Darmstadt, Germany) solution (pH 7.0) for 3 min. Specimens were examined by TEM using a JEM-1010 (JEOL, Tokyo, Japan) at 80 kV acceleration voltage.
Phage DNA Preparation, Sequencing, and Genome Annotation
For short-read, paired end whole-genome sequencing, phage DNA was extracted from concentrated virions by proteinase K/SDS treatment at 56°C for 2 h as previously described (Jäckel et al., 2015). The phage DNA was used for the preparation of DNA sequencing libraries using the Illumina DNA Flex Library Preparation kit (Illumina, CA, United States). MiSeq sequencing was conducted as previously described (Osieka et al., 2017). Raw reads were subjected to de novo assembly using the spades algorithm of the PATRIC database (version 3.6.2). For initial prediction of putative coding sequences, the annotation tool of the PATRIC database was used. Further bioinformatics analysis was conducted using the blast-suite2 (BLASTn, BLASTx BLASTp) of the National Center for Biotechnology Information (NCBI). Prediction of potential transcription terminators was conducted using ARNold3 (Hofacker et al., 1994; Macke et al., 2001).
Phylogenetic analyses were performed using the CSI Phylogeny tool (version 1.4; default parameters),4 of the Center for Genomic Epidemiology (Kaas et al., 2014). The resulting Newick files were further processed with FigTree (version 1.4.4).5 Dot Plot analyses were conducted using Accelrys DS Gene (version 2.5; Accelrys Inc.) with in text specified parameters.
Nucleotide Sequence Accession
The genomes of the Y. pseudotuberculosis phages described in this study were deposited in GenBank under the specified accession numbers: PYps2T (MT828551), PYps3T (MW147599), PYps4T (MW147600), PYps5T (MT828552), PYps10T (MT828553), PYps11T (MT515751), PYps14T (MT526905), PYps15T (MT515752), PYps16N (MW147601), PYps16T (MW147602), PYps23T (MW147598), PYps32T (MT515753), PYps35T (MT515754), PYps47T (MT515755), PYps49T (MW147603), PYps50T (MT515757), and PYps55T (MT515756).
Results
Isolation, Host Range, and Morphology of 17 Y. pseudotuberculosis Phages
The phages described in this study were isolated from fecal samples of animals kept in the Tierpark Friedrichsfelde, Berlin (Germany). In 2013, only samples of birds were investigated, whereas in 2017, some samples of other animals were additionally examined (Supplementary Table S1). After purification of the samples (see “Materials and Methods”), preparations were spotted onto 20 Y. pseudotuberculosis strains belonging to various serotypes (e.g., O:1a, O:2a, O:3, O:4a, O:5a, O:6, and O:7). In total, seven (out of 20) and 10 (out of 54) samples collected in 2013 and 2017, respectively, showed lytic activity on at least one indicator strain. With the exception of one phage (PYps23T) isolated from a capuchin monkey (Cebus), all positive samples had been taken from birds (Table 3). By contrast, altogether 57 samples did not show lytic activity on any tested indicator strain.
After isolation of single plaques, phages were propagated, purified, and analyzed by electron microscopy. Fifteen phages are myoviruses. However, while 10 phages (PYps2T, 5T, 10T, 11T, 14T, 15T, 32T, 35T, 47T, and 55T) exhibited a prolate head (110 × 90 nm) and a rather short tail (length: ~100 nm), the head of five phages (PYps3T, 4T, 16T, 16N and 23T) was isometric (diameter: ~105 nm), and their tail significantly longer (length: ~200 nm, Figure 1). Contracted tails and tail fibers were discernible in most preparations. Unlike the aforementioned phages, two phages (PYps49T and 50T) are podoviruses (Figure 1). They showed a small isometric head (diameter: ~58 nm) and a very short tail. Thus, on the basis of morphology, the investigated phages could be allocated to three groups, the type phages of which are PYps2T, PYps23T, and PYps50T.
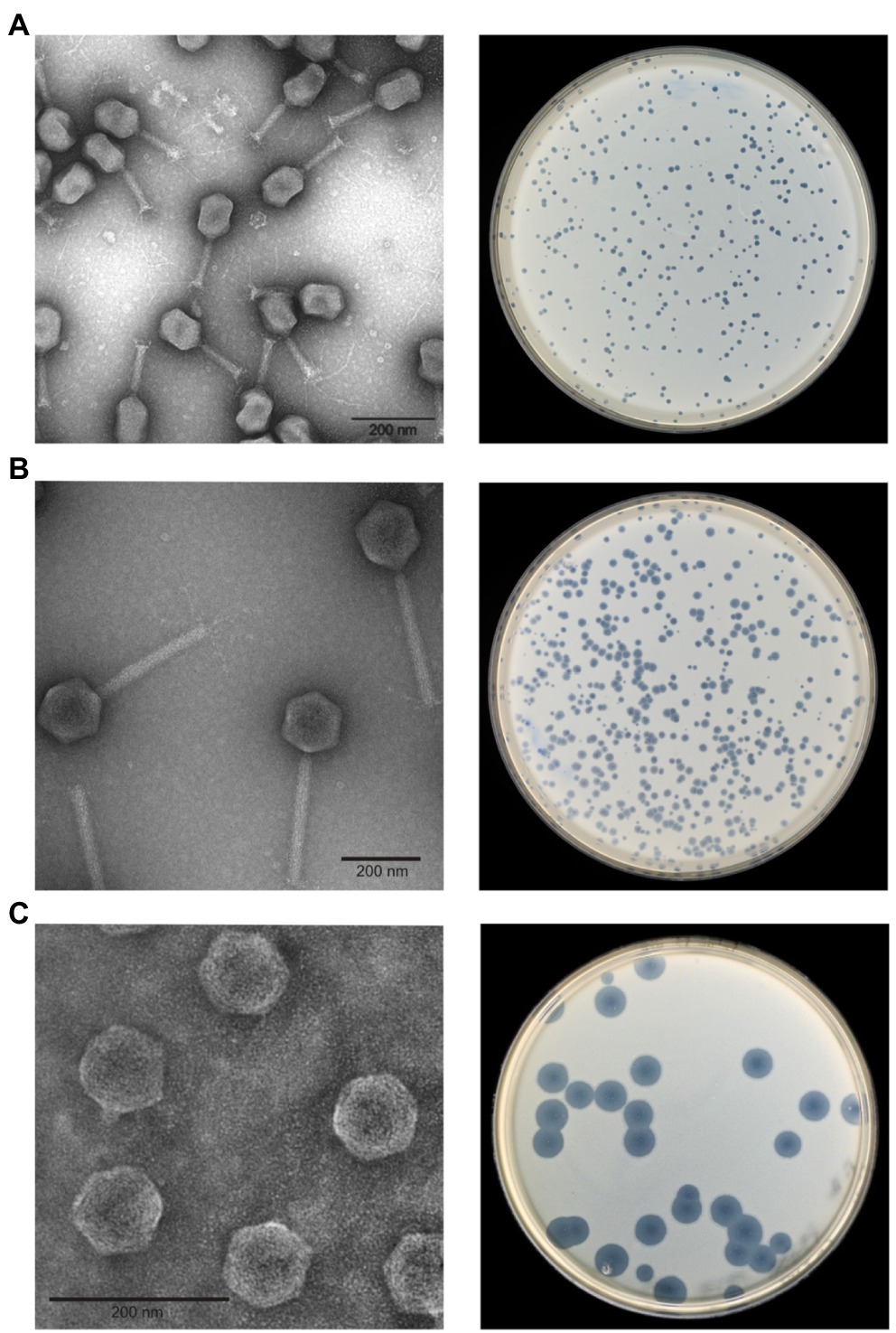
Figure 1. Morphology and plaque formation of Yersinia pseudotuberculosis phages. (A) PYps2T group, (B) PYps23T group, and (C) PYps50T group.
The host ranges of the phages were determined by testing 128 Y. pseudotuberculosis strains belonging to 20 serotypes and strains belonging to other species. The study showed that all phages lysed several Y. pseudotuberculosis serotypes but that the host specificity diverged in part significantly. Between 5.5 and 86.7% of the tested strains were infected by each phage (Table 1). The five myoviruses possessing an isometric head and a long tail (PYps23T group) lysed only few strains which, however, belonged to various serotypes. Most of the remaining myoviruses (PYps2T group) infected many more Y. pseudotuberculosis strains. All tested serotypes were lysed by at least one phage. Among the myoviruses, PYps35T exhibited the broadest host range, as it lysed 106 out of 128 (82.8%) strains. More strains (86.7%) were only lysed by the two podoviruses PYps49T and 50T. Plaques produced by the phages revealed different morphologies ranging from very small (diameter less than 1 mm) to very large (approximately 1 cm in size) produced by the podoviruses (Figure 1).
Strains of Y. pestis, Y. similis, and Y. wautersii, which are closely related to Y. pseudotuberculosis, revealed susceptibility as well. All nine tested Y. pestis strains were lysed by the myoviruses PYps14T and PYps35T and the podovirus PYps49T. In addition, the myovirus PYps35T and the podoviruses PYps49T and PYps50T lysed all five Y. similis strains and one of two Y. wautersii strains belonging to serotype O:4a. Interestingly, this strain was susceptible also to the PYps3T group. As phages of this group were able to lyse several O:4a strains of Y. pseudotuberculosis, they might have a preference for this serotype (Table 1). We additionally tested Y. enterocolitica strains belonging to the bio/serotypes B4/O:3 (Pärn et al., 2014), B2/0:9 (Press et al., 2001), B2/O:5,27 (Pärn et al., 2014), and 1B:O:8 (Nakano et al., 1989), which cause most human infections in Europe. Surprisingly, all B4/O:3 strains but no strains of the other bio/serotypes were susceptible to the phages. Though, some phages of the PYps2T and PYps23T group only lysed B4/O:3 strains at a temperature of 37°C while the remaining phages were lytic at both temperatures, 37°C and 28°C (data not shown).
It has previously been reported that some Y. pseudotuberculosis phages infected species outside the genus Yersinia. We therefore examined a number of other enterobacteria. As shown in Table 2, some E. coli (16/44), Morganella morganii (1/37), Shigella (2/3), and Salmonella (2/3) strains were lysed by several phages, whereas the species Citrobacter freundii (n = 3), Enterobacter cloacae (n = 8), Klebsiella spp. (n = 34), Proteus mirabilis (n = 5), and Providencia rettgeri (n = 6) were not susceptible.
Members of Each Group Possess Highly Conserved Genomes but Are Not Related to Phages of Other Groups
To study the 17 phages in detail, they were subjected to nucleic acid isolation followed by whole-genome sequencing (see “Materials and Methods”). Sequence analyses indicated that phages within each group are very similar (91.3 to 99.9% identity over 92 to 100% of the genomes), whereas no similarities were detected between the groups. Members of the PYps2T group contain large genomes of approximately 166 to 170 kb and are related to T4-like phages. The strongest similarities exist to the Escherichia phages YUEEL01 and vB_EcoM_JB75 (Acc. Nos. KY290975.2 and MH355584.1) with an identity of 99% over 94 and 93% of the covered genome, respectively. PYps23T group members contain smaller genomes (ca. 53 to 56 kb). The closest relatives are Escherichia phage ST32 (Liu et al., 2018) and Enterobacteria phage phiEcoM-GJ1 (Jamalludeen et al., 2008), to which PYps23T is approximately 92% identical over 70% of the covered genomes. The podoviruses of the PYps50T group have a size of 39.6 kb and are very similar to Salmonella phage BSP161 (Acc. no. MG471392.1), to the Enterobacteria phages 285P (Xu et al., 2014) and BA14 (Mertens and Hausmann, 1982) and to Escherichia phage vB_EcoP_S523 (Acc. no. MH031343.1), which all are T7-related phages. They exhibit nucleotide identity values of more than 90% over 94% of the covered genomes.
The PYps2T Group Is Closely Related to T4-like Phages of the Taxid: Caudovirales; Myoviridae; Tevenvirinae; Tequatrovirus
The dot plot alignment of PYps2T with T4 demonstrates that both phages are very similar along their collinear genomes (97.24% identity over 88% of the genome, Figure 2A). As many T4 proteins have previously been characterized in detail, a functional prediction of 202 out of 269 PYps2T gene products could be made (Figure 3; Supplementary Table S2). Thus, a large number of genes probably involved in virion assembly, replication, or transcription were identified. In addition, 10 tRNA genes and eight homing nuclease genes were detected, which are common “molecular parasites” of T4 phage genomes that can promote their own horizontal transfer by inserting into a cognate site not containing the endonuclease gene (Sandegren and Sjoberg, 2004; Edgell et al., 2010). All PYps2T group members have a similar-sized circularly permuted genome ranging from 165,733 bp (PYps32T) to 169,493 bp (PYps10T) with a GC content of ~35.4% (Table 4). Moreover, the genome composition of the phages is very similar. Thus, the question arises why some members of this group differed so clearly in their host range lysing between 19 (PYps5T) and 106 (PYps35T) out of 128 Y. pseudotuberculosis strains. The gene products gp37 and gp38 of T4-related phages, which encode the long tail fiber (LTF) distal half fiber including the receptor-recognizing tip and an adhesin, respectively, have been shown to be important for phage adsorption and host specificity (Yoichi et al., 2005; Trojet et al., 2011; Storms and Sauvageau, 2014). Comparison of the large distal half fiber proteins of PYps2T group phages disclosed two subgroups, each possessing almost identical proteins (except for PYps55T that showed some amino acid exchanges), whereas phages belonging to different subgroups exhibited a sequence identity of only 80% (Supplementary Figure S1). However, there was no discernible link between the gp37 sequences and the host ranges of the phages, since the phages with the narrowest (PYps5T) and broadest (PYps35T) host range possess very similar distal half fibers. As with the gp37 products, the same subgroups could be formed by comparison of the tail fiber adhesins (gp38). Though, here, each subgroup revealed more prominent differences. The adhesins of phage PYps35T and PYps55T, e.g., contain a deletion of four and an insertion of three amino acid residues, respectively, whereas the PYps5T protein revealed a number of single amino acid exchanges (Figure 4). In the other subgroup, PYps47T differs from PYps14T, PYps15T, and PYps32T by an insertion of 15 amino acid residues. It is conceivable that these deviations are important for the host specificity of the phages.
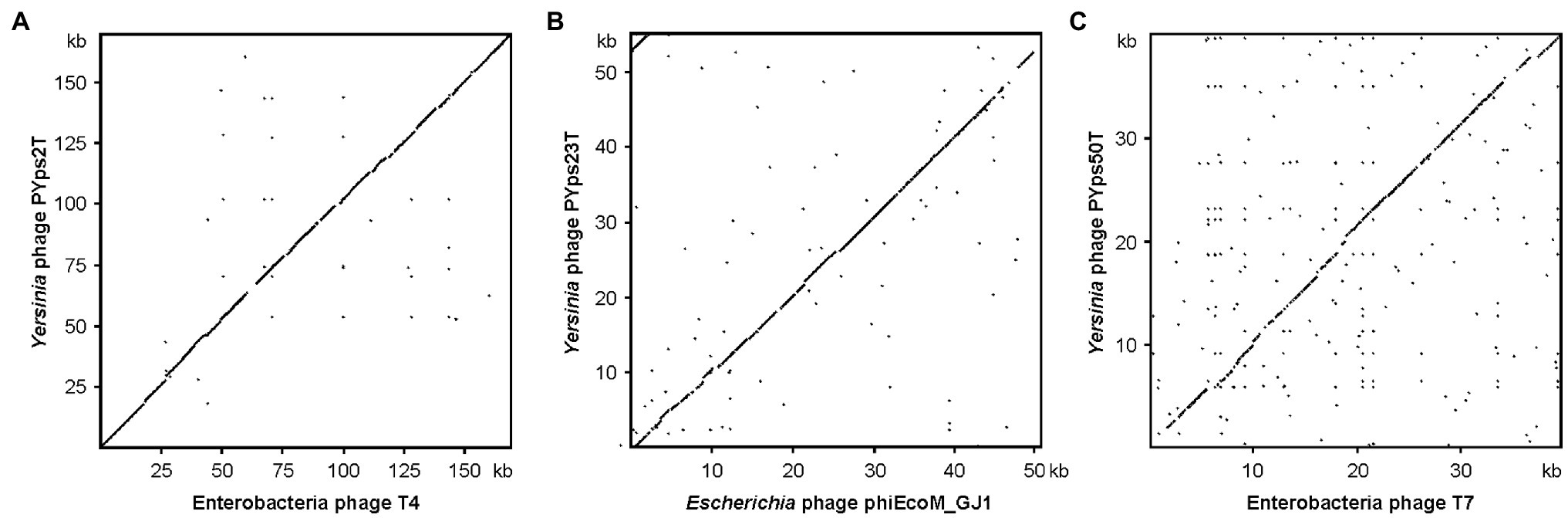
Figure 2. Dot plot analyses of the three Y. pseudotuberculosis type phages with closely related phages. (A) PYps2T, (B) PYps23T, and (C) PYps50T. Dot plot analyses were conducted using Accelrys DS Gene (version 2.5; Accelrys Inc.) with the following settings: minimum sequence identity: 65%; window size: 25; and hash value: 6.
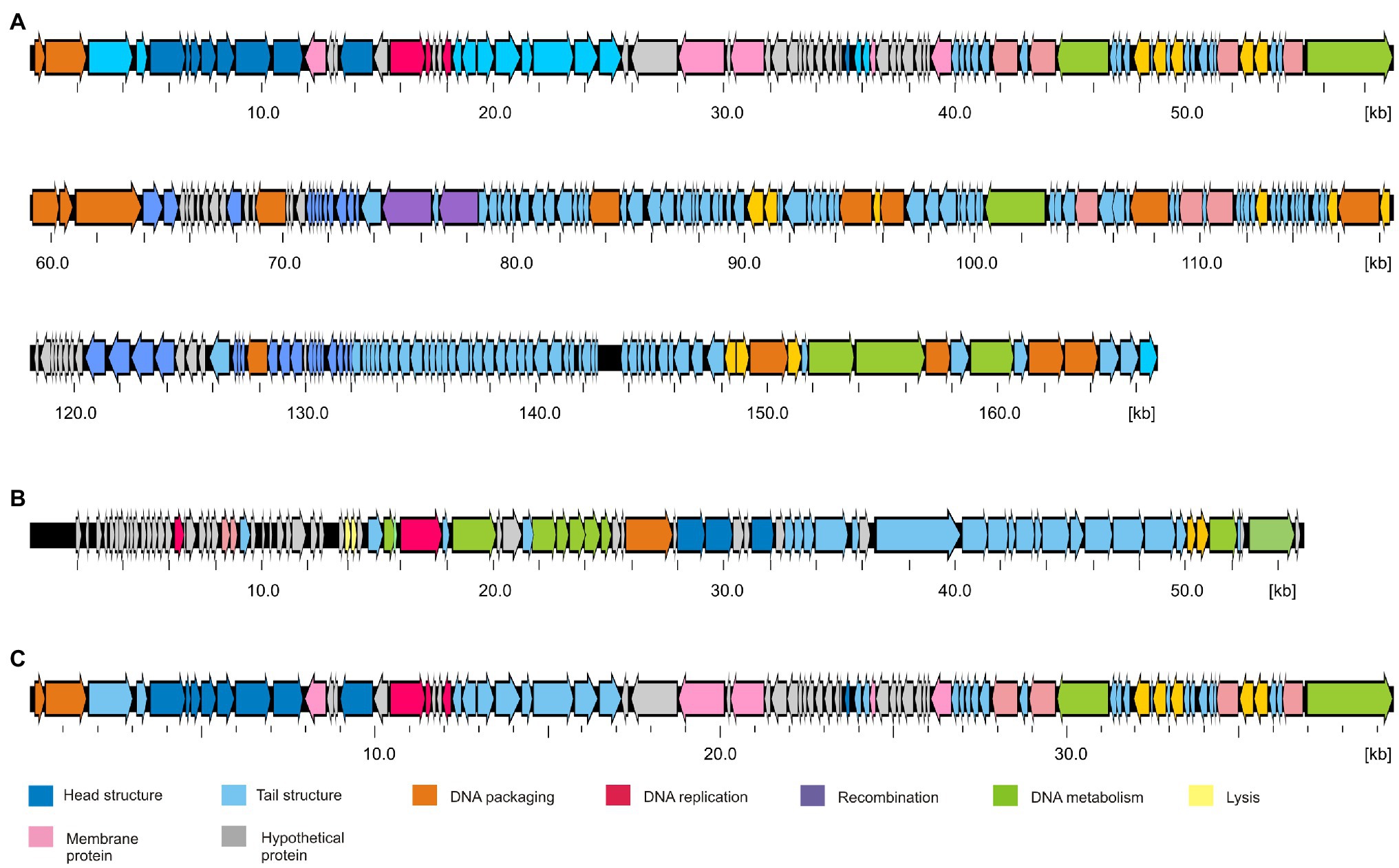
Figure 3. Gene maps of the Y. pseudotuberculosis type phages. (A) PYps2T, (B) PYps23T, and (C) PYps50T. Predicted functions of gene products are indicated by different colors.
PYps23T-like Phages Belong to an Unusual Group of Myoviruses (Taxid: Caudovirales; Chaseviridae; Carltongylesvirus; Escherichia Virus ST32)
Members of the PYps23T group revealed strong similarities to a number of myoviruses possessing some proteins of podoviruses, e.g., Escherichia phage ST32, Escherichia phage phiEcoM-GJ1, Pectobacterium carotovorum phage PM1, and Erwinia amylovora phage vB_EamM-Y2 (Figure 2B). In type phage, PYps23T 83 predicted genes were identified and annotated, and all the predicted genes, except for one, are located on the same DNA strand. Only 43 predicted gene products could be functionally assigned, while similar to phage phiEcoM-GJ1, the predicted functions of most of the small gene products encoded by genes at the beginning of the genome are still unknown (Figure 3; Supplementary Table S3). Most PYps23T genes including a gene for a T7-RNA polymerase also exist in the same order in the other phages of this group. However, a gene encoding tRNA for arginine or leucine identified in some of these phages was only detected in two phages (PYps16N and 23T) of the PYps23T group. Interestingly, the phages PYps3T, 4T, and 16T, which were isolated 4 years earlier, lack exactly the tRNA gene, whereas adjacent DNA-sequences are almost identical to those of the other phages suggesting that the tRNA gene can be acquired or lost by horizontal gene transfer. As with most other proteins, the terminase large subunit is very similar in the PYps23T group and also in the related phages infecting other species indicating the same packaging mechanism (Liu et al., 2018). The terminases of these phages resemble proteins of phages with a T1-like headful packaging mechanism. However, while for phage phiEcoM-GJ1 a circularly permuted genome has been suggested (Jamalludeen et al., 2008), direct terminal repeats of approximately 2.6 kb have been described for the phages vB_EamM-Y2 and PM1 (Born et al., 2011; Lim et al., 2014). We therefore compared MbiI and NdeI restriction patterns of digested PYps23T DNA with patterns predicted by in silico analysis of the sequenced phage. For this comparison, the zero point of the PYps23T genomic sequence was determined in accordance with those of the related phages vB_EamM-Y2 and PM1. The MbiI digest showed a 4 kb fragment and an additional band of approximately 1.3 kb that were absent in the in silico analysis, whereas a predicted fragment of 2.8 kb was missing in the digested DNA (Figure 5A). Thus, the digested phage genome was approximately 2.5 kb larger than that determined by in silico analysis. This result was supported by digestion of the DNA with NdeI. Here, an additional fragment of approximately 4.8 kb appeared in the gel, while an approximately 2.3 kb fragment of a double-band predicted by in silico analysis was missing. To ascertain whether the additional sequence represents a direct terminal repeat, the PYps23T phage DNA was treated with T4 ligase and used for PCR analysis applying a reverse primer deduced from the predicted left end of the sequenced genome and a forward primer deduced from the predicted repeat sequence at the right end (Figure 5B). We indeed obtained a PCR product of the expected size. Sequencing of the product indicated a repeat of 2.339 bp. The repetitive sequence was confirmed by direct sequencing of phage DNA using primers deduced from the upstream region of the repetitive sequence (Figure 5B).
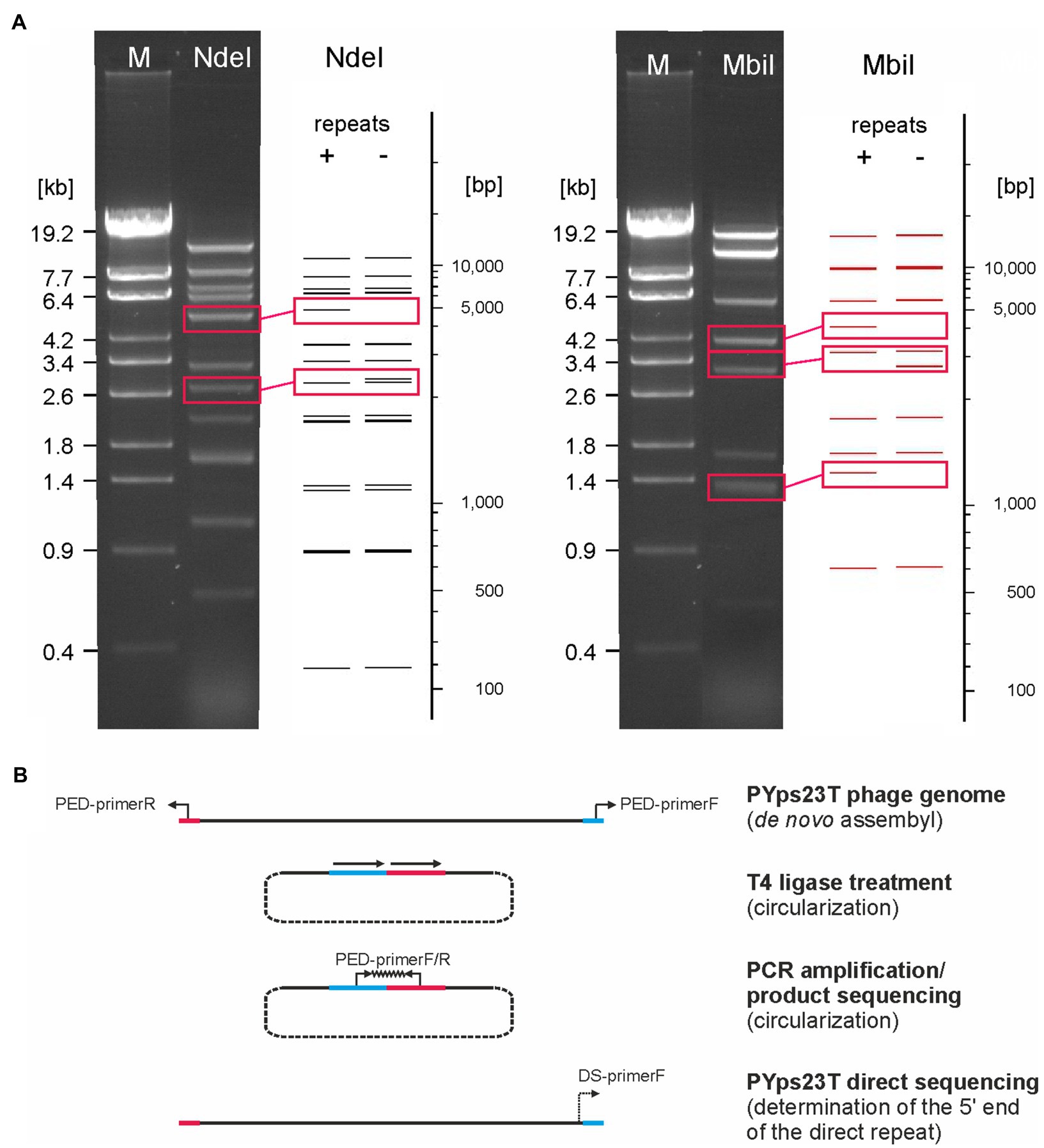
Figure 5. The PYps23T genome contains long terminal direct repeats. (A) MbiI and NdeI restriction patterns of the phage DNA obtained by agarose gel electrophoresis and by in silico analysis. Fragments that were present only in the gel or in the in silico analysis are boxed. In silico restriction pattern predictions of PYps23T were obtained using NEBcutter v2.0 (Vincze et al., 2003). Shown are patterns of the PYps23T genome without and with terminal repeats of 2.3 kb. M, Lambda Eco32I marker (fragment sizes are given). (B). Determination of the start and end of the direct repeat. PED, PCR End Determination primer; DS, Direct Sequencing primer. See the text for details.
What phages of the PYps23T group have in common is a very similar, narrow host range. They lysed only few Y. pseudotuberculosis strains, but also individual strains of other species, e.g., E. coli (Table 2). This raises the question whether the tail fiber proteins of these phages are related to those of the E. coli phages ST32 and phiEcoM-GJ1. There are several proteins probably involved in the synthesis of the tail fibers. In PYps23T, Gp76 is likely a large main tail fiber protein of 1,224 amino acids, while a Gp84 may be a smaller tail fiber protein of 463 amino acids. In addition, Gp85 (tail fiber repeat protein) and Gp86 (tail fiber assembly protein) may be important for tail fiber synthesis. Though, while the main tail fiber proteins of phages belonging to the PYps23T group are 90 to 100% identical, they showed only identities of 67 and 64% to the corresponding proteins of phiEcoM-GJ1 and ST32, respectively. Moreover, the other PYps23T tail fiber-associated products are much more dissimilar to their counterparts in the E. coli phages with identities of 44% (Gp84) and 37% (Gp85). For Gp86, no counterpart could be detected in these phages. It is therefore unclear, why the PYps32T group can lyse E. coli and doubtful whether the mentioned E. coli phages are able to infect Y. pseudotuberculosis as well.
PYps49T and PYps50T Are Phages of the Taxid: Autographiviridae; Studiervirinae; Berlinvirus
These phages are closely related to phage T7 (Figure 2C). Like most other T7-like phages, the genomes of PYps49T and PYps50T contain direct terminal repeats, which have a length of 184 bp. Thirty-four of the 45 predicted PYps50T gene products could be functionally assigned. Besides a number of genes for virion assembly (head and tail proteins), some genes encoding proteins involved in DNA metabolism (e.g., for a RNA polymerase), host cell lysis, and DNA packaging were identified (Figure 3; Supplementary Table S2). The gene order is almost identical to that of other T7-related phages. Among the closest relatives of PYps50T and PYps49T are the Y. pestis phages Yepe2, YpP-G, Yep-phi, and Berlin, which are almost 92% identical over 85 to 86% of their covered genomes (Zhao et al., 2011). For Yep-phi, it has been reported that the phage does not infect species other than Y. pestis (Zhao et al., 2013). At first glance, this seems to be surprising since PYps49T and 50 T are able to lyse not only Y. pseudotuberculosis and Y. pestis but also some strains of E. coli, Salmonella and Shigella. A closer look at the tail fiber proteins of the phages, however, revealed that the proteins of PYps49T and 50 T are only 55% identical to those of the aforementioned Y. pestis phages. While the first 320 amino acids of the proteins are very similar, the next approximately 180 amino acids diverge significantly (Figure 6). Interestingly, close to the transition point from the related to the unrelated region a number of repetitive sequences are present which might have caused this break. The C-terminal part of the proteins containing the receptor binding domain is slightly similar (Zhao et al., 2013). By contrast, the PYps49T and 50T tail fiber proteins are 91% identical to that of the Salmonella phage BSP161. Due to the very high overall similarity to BSP161, the question arises whether also this phage is able to lyse both Salmonella and Y. pseudotuberculosis.
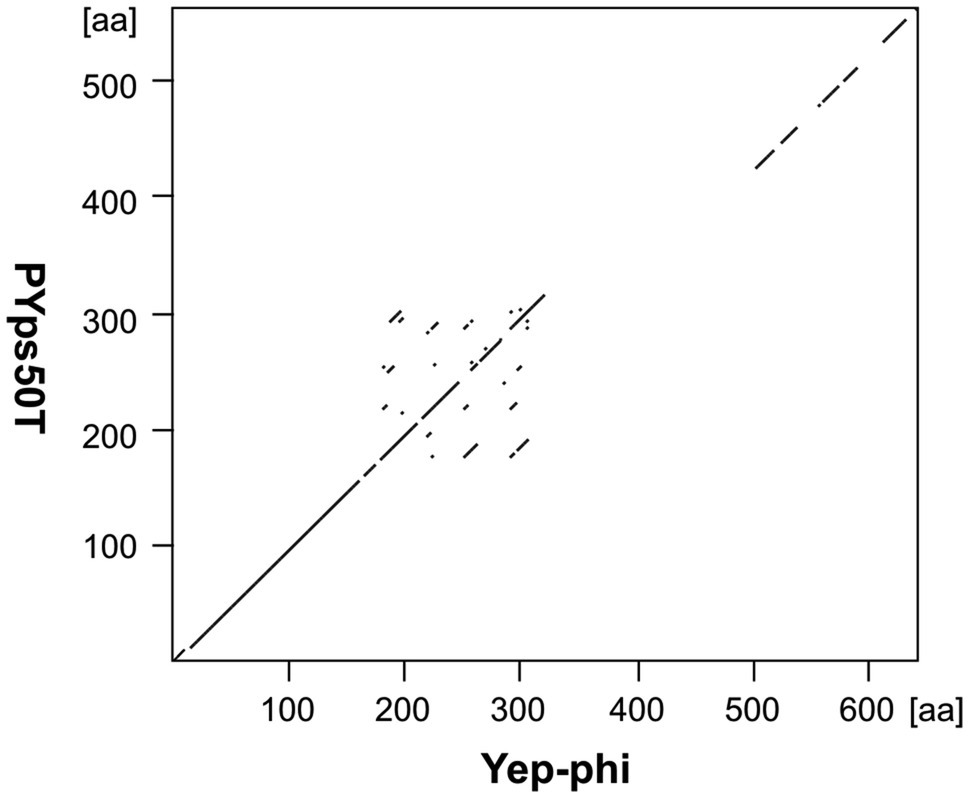
Figure 6. Dot plot of the tail fiber proteins of PYps50T and Yep-phi. Dot plot analyses were conducted using Accelrys DS Gene (version 2.5; Accelrys Inc.) with the following settings: minimum sequence identity: 65%; window size: 15; and hash value: 2.
Discussion
This study showed that phages able to infect Y. pseudotuberculosis strains are widespread in birds kept in the zoo Tierpark Berlin. From 35 to 19% of the samples collected in 2013 and 2017, respectively, phages could be isolated. All isolated phages are myoviruses or podoviruses. Though, while 10 and nine myoviruses belonging to the PYps2T and PYps23T group, respectively, were predominantly occurring, only two podoviruses were isolated, both of which in the same year. As far as we know siphoviruses (phages with long non-contractile tails) infecting Y. pseudotuberculosis have yet not been described, even though this group is predominant within the order Caudovirales. The different frequencies of positive samples may be caused by the fact that in August 2013 exclusively birds of prey and owls were studied, whereas in July 2017, also a rather large number (n = 18) of samples from mammals were analyzed. Thus, in that year, 28% of the samples of birds were positive for Y. pseudotuberculosis phages. We did not try to isolate Y. pseudotuberculosis from fecal samples because the animals did not show any clinical symptoms and there is no standardized protocol for such a procedure (Reinhardt et al., 2018). Nevertheless, the facts that Y. pseudotuberculosis phages could almost exclusively be isolated from birds and that the presence of phages is strongly associated with bacteria, in which they propagate suggests that birds are an important reservoir of hosts of those phages. In Finland, a comprehensive study on the prevalence of Y. enterocolitica and Y. pseudotuberculosis phages in pig stool was performed (Salem et al., 2015). In 90 out of 793 (11%) pig stool samples, Yersinia phages were detected, of which, however, only eight samples contained phages infecting Y. pseudotuberculosis. As Y. pseudotuberculosis could not be isolated in that study, this finding together with our results underlines the assumption that the enteropathogenic Yersinia species have different reservoirs. Though, since we did not screen the birds for Y. pseudotuberculosis and due to the ability of all phages to infect also non-Yersinia strains, it cannot be excluded that they may have replicated in other bacteria. This does not particularly concern the PYps2T group and PYps49T/PYps50T, most of them infected many Y. pseudotuberculosis strains. By contrast, PYps23T-like phages lysed very few Y. pseudotuberculosis strains but, e.g., the E. coli strain ATCC 35218 (Tables 1 and 2). The ability of some Yersinia phages to infect other Enterobacteriaceae has already been reported (Filippov et al., 2012; Rashid et al., 2012). However, PYps23T-related phages have yet only been described for E. coli, Erwinia, and Pectobacterium (Jamalludeen et al., 2008;Born et al., 2011; Lim et al., 2014), but not for Y. pseudotuberculosis. Therefore, it remains open, which is the natural host of PYps23T-like phages, but it is noteworthy that members of this group were isolated in 2013 and 2017 suggesting that their host is prevalent in the birds gut. Similar to closely related phages like phiEcoM-GJ1, the PYps23T group is unusual myoviruses, because they encode some podoviral proteins, particularly a T7-like single-subunit RNA polymerase, even though tRNA genes were only detected in phages isolated in 2017. Another striking difference to phiEcoM-GJ1 pertains to the proposed genome ends of the phages. Although the PYPs23T terminase large subunit is 97% identical over 99% of the protein to its counterpart in phiEcoM-GJ1, the PYps23T genome clearly contains direct terminal repeats and is not circularly permuted. Direct terminal repeats have already been reported for the phages vB_EamM-Y2 and PM1 whose terminase large subunits are also very similar to the proteins of PYps23T and phiEcoM-GJ1 (Born et al., 2011; Lim et al., 2014). We therefore speculate that all phages of this group possess terminal direct repeats at their genome ends. Compared to the PYps23T group, many more Y. pseudotuberculosis strains were lysed by phages belonging to the PYps2T group, isolated from 18% of the fecal samples of birds, even though the host specificity of individual members of this group diverged in part significantly (Table 1). This group is closely related to T4-like phages, particularly Escherichia and Shigella phages, but also to some Yersinia phages, e.g., YpsP-PST, which has an extended host range, as it lyses not only strains of Y. pestis and Y. pseudotuberculosis, but also other Enterobacteriaceae (Rashid et al., 2012). The wide spectrum of host specificity that we determined for members of the PYps2T group might be caused by different gp38 adhesins which are primary determinants of host range in T4-type bacteriophages (Trojet et al., 2011). Indeed, as with gp37 (LTF distal half fiber), the alignments of the gp38 adhesins of the PYps2T group revealed two subgroups. Though, there was no clear link discernable between the membership of a group and the host range. One subgroup composed of the phages PYps14T, 15T, 32T, and 47T whose gp37 and gp38 are very similar to that of YpsP-PST lysed on the average more Y. pseudotuberculosis strains than the other subgroup, in which, however, two members (PYps35T and PYps55T) also showed a broad-host range. It still has to be resolved, whether the insertion and deletion observed in the gp38 adhesins of PYps35T and PYps55T may affect their host specificity. In 2017, the two very similar podoviruses PYps49T and 50 T were isolated, which showed a very broad-host range and a strong lytic activity. They are related to T7-like phages, which also include some Y. pestis and Y. enterocolitica phages (Zhao et al., 2011; Salem and Skurnik, 2018). However, the tail fiber proteins of PYps49T and 50T differ significantly from those of other Yersinia podoviruses, which may explain why they could lyse so many strains. This property is rather unusual for podoviruses, whose host ranges are generally narrower than those of myoviruses (Wichels et al., 1998). Indeed, a cocktail containing one podovirus (PYps49T or 50T) and one myovirus (e.g., PYps35T) would be suitable to lyse 93% of the tested 128 Y. pseudotuberculosis strains belonging to 20 different serotypes. In addition, these two phages also lysed all tested Y. enterocolitica B4/O:3 strains at 37°C, whereas a significantly lower proportion (23%) of E. coli strains were susceptible.
Conclusion
This study showed that zoo animals can be a promising source to isolate phages that can be used for applications to combat Y. pseudotuberculosis. The fact that very similar phages were isolated at an interval of 4 years indicates that they belong to the normal intestinal flora of birds. We are planning to repeat a sampling in 2022 to verify this notion and will then try to isolate Y. pseudotuberculosis from the samples to elucidate, whether this species may be the natural host of the phages.
Data Availability Statement
The datasets presented in this study can be found in online repositories. The names of the repository/repositories and accession number(s) can be found in the article/Supplementary Material.
Author Contributions
JH and SH designed the study. JH, ABa, ABi, AD, ND, CJ, NM, JJ, MS, JU, and SH performed the experiments. JH, ABa, CJ, MS, and SH analyzed the data. JH, CJ, MS, and SH prepared the tables and figures and wrote the manuscript. All authors edited the manuscript and contributed to the article and approved the submitted version.
Funding
The work was financially supported by the Bundesinstitut für Risikobewertung (grant no. 45-002).
Conflict of Interest
The authors declare that the research was conducted in the absence of any commercial or financial relationships that could be construed as a potential conflict of interest.
Publisher’s Note
All claims expressed in this article are solely those of the authors and do not necessarily represent those of their affiliated organizations, or those of the publisher, the editors and the reviewers. Any product that may be evaluated in this article, or claim that may be made by its manufacturer, is not guaranteed or endorsed by the publisher.
Acknowledgments
The authors are greatly indebted to the curator Andreas Pauli (Zoo Tierpark Friedrichsfelde, Berlin) to take samples.
Supplementary Material
The Supplementary Material for this article can be found online at: https://www.frontiersin.org/articles/10.3389/fmicb.2021.634289/full#supplementary-material
Footnotes
1. ^https://lpsn.dsmz.de/genus/yersinia
2. ^https://blast.ncbi.nlm.nih.gov/Blast.cgi
3. ^http://rssf.i2bc.paris-saclay.fr/toolbox/arnold/
References
Arrausi-Subiza, M., Gerrikagoitia, X., Alvarez, V., Ibabe, J. C., and Barral, M. (2016). Prevalence of Yersinia enterocolitica and Yersinia pseudotuberculosis in wild boars in the Basque Country, northern Spain. Acta Vet. Scand. 58, 1–7. doi: 10.1186/s13028-016-0184-9
Born, Y., Fieseler, L., Marazzi, J., Lurz, R., Duffy, B., and Loessner, M. J. (2011). Novel virulent and broad-host-range Erwinia amylovora bacteriophages reveal a high degree of mosaicism and a relationship to Enterobacteriaceae phages. Appl. Environ. Microbiol. 77, 5945–5954. doi: 10.1128/AEM.03022-10
Cabanel, N., Galimand, M., Bouchier, C., Chesnokova, M., Klimov, V., and Carniel, E. (2017). Molecular bases for multidrug resistance in Yersinia pseudotuberculosis. Int. J. Med. Microbiol. 307, 371–381. doi: 10.1016/j.ijmm.2017.08.005
Carniel, E. (2001). The Yersinia high-pathogenicity island: an iron-uptake island. Microbes Infect. 3, 561–569. doi: 10.1016/S1286-4579(01)01412-5
Cork, S. C., Collins-Emerson, J. M., Alley, M. R., and Fenwick, S. G. (1999). Visceral lesions caused by Yersinia pseudotuberculosis, serotype II, in different species of bird. Avian Pathol. 28, 393–399. doi: 10.1080/03079459994669
Edgell, D. R., Gibb, E. A., and Belfort, M. (2010). Mobile DNA elements in T4 and related phages. Virol. J. 7:290. doi: 10.1186/1743-422X-7-290
European Food Safety Authority (2021). The European Union One Health 2019 Zoonoses Report. EFSA J. 19:e06406. doi: 10.2903/j.efsa.2021.6406
Filippov, A. A., Sergueev, K. V., He, Y., and Nikolich, M. P. (2012). Bacteriophages capable of lysing Yersinia pestis and Yersinia pseudotuberculosis: efficiency of plating tests and identification of receptors in Escherichia coli K-12. Adv. Exp. Med. Biol. 954, 123–134. doi: 10.1007/978-1-4614-3561-7_16
Fogelson, S. B., Yau, W., and Rissi, D. R. (2015). Disseminated Yersinia pseudotuberculosis infection in a paca (Cuniculus paca). J. Zoo Wildl. Med. 46, 130–134. doi: 10.1638/2014-0150R.1
Fredriksson-Ahomaa, M., Stolle, A., and Korkeala, H. (2006a). Molecular epidemiology of Yersinia enterocolitica infections. FEMS Immunol. Med. Microbiol. 47, 315–329. doi: 10.1111/j.1574-695X.2006.00095.x
Fredriksson-Ahomaa, M., Stolle, A., Siitonen, A., and Korkeala, H. (2006b). Sporadic human Yersinia enterocolitica infections caused by bioserotype 4/O: 3 originate mainly from pigs. J. Med. Microbiol. 55, 747–749. doi: 10.1099/jmm.0.46523-0
Fredriksson-Ahomaa, M., Wacheck, S., Bonke, R., and Stephan, R. (2011). Different enteropathogenic Yersinia strains found in wild boars and domestic pigs. Foodborne Pathog. Dis. 8, 733–737. doi: 10.1089/fpd.2010.0711
Galosi, L., Farneti, S., Rossi, G., Cork, S. C., Ferraro, S., Magi, G. E., et al. (2015). Yersinia pseudotuberculosis, serogroup O:1a, infection in two amazon parrots (Amazona aestiva and Amazona oratrix) with hepatic hemosiderosis. J. Zoo Wildl. Med. 46, 588–591. doi: 10.1638/2014-0140.1
Hallanvuo, S., Nuorti, P., Nakari, U. M., and Siitonen, A. (2003). Molecular epidemiology of the five recent outbreaks of Yersinia pseudotuberculosis in Finland. Adv. Exp. Med. Biol. 529, 309–312. doi: 10.1007/0-306-48416-1_58.pdf
Hammerl, J. A., Barac, A., Erben, P., Fuhrmann, J., Gadicherla, A., Kumsteller, F., et al. (2021). Properties of Two Broad Host Range Phages of Yersinia enterocolitica Isolated from Wild Animals. Int. J. Mol. Sci. 22:11381. doi: 10.3390/ijms222111381
Hammerl, J. A., Klein, I., Lanka, E., Appel, B., and Hertwig, S. (2008). Genetic and functional properties of the self-transmissible Yersinia enterocolitica plasmid pYE854, which mobilizes the virulence plasmid pYV. J. Bacteriol. 190, 991–1010. doi: 10.1128/JB.01467-07
Hofacker, I. L., Fontana, W., Stadler, P. F., Bonhoeffer, S., Tacker, M., and Schuster, P. (1994). Fast folding and comparison of RNA secondary structures. Monatsh. Chem. 125, 167–188. doi: 10.1007/BF00818163
Inoue, M., Nakashima, H., Ueba, O., Ishida, T., Date, H., Kobashi, S., et al. (1984). Community outbreak of Yersinia pseudotuberculosis. Microbiol. Immunol. 28, 883–891. doi: 10.1111/j.1348-0421.1984.tb00744.x
Iwata, T., Une, Y., Okatani, A. T., Kato, Y., Nakadai, A., Lee, K., et al. (2008). Virulence characteristics of Yersinia pseudotuberculosis isolated from breeding monkeys in Japan. Vet. Microbiol. 129, 404–409. doi: 10.1016/j.vetmic.2007.11.029
Jäckel, C., Hammerl, J. A., Reetz, J., Kropinski, A. M., and Hertwig, S. (2015). Campylobacter group II phage CP21 is the prototype of a new subgroup revealing a distinct modular genome organization and host specificity. BMC Genomics 16:629. doi: 10.1186/s12864-015-1837-1
Jäckel, C., Hertwig, S., Scholz, H. C., Nöckler, K., Reetz, J., and Hammerl, J. A. (2017). Prevalence, host range, and comparative genomic analysis of temperate Ochrobactrum phages. Front. Microbiol. 8:1207. doi: 10.3389/fmicb.2017.01207
Jalava, K., Hakkinen, M., Valkonen, M., Nakari, U. M., Palo, T., Hallanvuo, S., et al. (2006). An outbreak of gastrointestinal illness and erythema nodosum from grated carrots contaminated with Yersinia pseudotuberculosis. J. Infect. Dis. 194, 1209–1216. doi: 10.1086/508191
Jalava, K., Hallanvuo, S., Nakari, U. M., Ruutu, P., Kela, E., Heinasmaki, T., et al. (2004). Multiple outbreaks of Yersinia pseudotuberculosis infections in Finland. J. Clin. Microbiol. 42, 2789–2791. doi: 10.1128/JCM.42.6.2789-2791.2004
Jamalludeen, N., Kropinski, A. M., Johnson, R. P., Lingohr, E., Harel, J., and Gyles, C. L. (2008). Complete genomic sequence of bacteriophage phiEcoM-GJ1, a novel phage that has myovirus morphology and a podovirus-like RNA polymerase. Appl. Environ. Microbiol. 74, 516–525. doi: 10.1128/AEM.00990-07
Kaas, R. S., Leekitcharoenphon, P., Aarestrup, F. M., and Lund, O. (2014). Solving the problem of comparing whole bacterial genomes across different sequencing platforms. PLoS One 9:e104984. doi: 10.1371/journal.pone.0104984
Laukkanen, R., Martinez, P. O., Siekkinen, K. M., Ranta, J., Maijala, R., and Korkeala, H. (2008). Transmission of Yersinia pseudotuberculosis in the pork production chain from farm to slaughterhouse. Appl. Environ. Microbiol. 74, 5444–5450. doi: 10.1128/AEM.02664-07
Lim, J. A., Shin, H., Lee, D. H., Han, S. W., Lee, J. H., Ryu, S., et al. (2014). Complete genome sequence of the Pectobacterium carotovorum subsp. carotovorum virulent bacteriophage PM1. Arch. Virol. 159, 2185–2187. doi: 10.1007/s00705-014-2005-7
Liu, H., Geagea, H., Rousseau, G. M., Labrie, S. J., Tremblay, D. M., Liu, X., et al. (2018). Characterization of the Escherichia coli virulent myophage ST32. Viruses 10:616. doi: 10.3390/v10110616
Macke, T. J., Ecker, D. J., Gutell, R. R., Gautheret, D., Case, D. A., and Sampath, R. (2001). RNAMotif, an RNA secondary structure definition and search algorithm. Nucleic Acids Res. 29, 4724–4735. doi: 10.1093/nar/29.22.4724
Mertens, H., and Hausmann, R. (1982). Coliphage BA14: A new relative of phage T7. J. Gen. Virol. 62, 331–341. doi: 10.1099/0022-1317-62-2-331
Nakamura, S., Hayashidani, H., Sotohira, Y., and Une, Y. (2016). Yersiniosis caused by Yersinia pseudotuberculosis in captive toucans (Ramphastidae) and a Japanese squirrel (Sciurus lis) in zoological gardens in Japan. J. Vet. Med. Sci. 78, 297–299. doi: 10.1292/jvms.15-0298
Nakamura, S., Hayashidani, H., Yonezawa, A., Suzuki, I., and Une, Y. (2015). Yersiniosis due to infection by Yersinia pseudotuberculosis 4b in captive meerkats (Suricata suricatta) in Japan. J. Vet. Diagn. Investig. 27, 641–644. doi: 10.1177/1040638715596035
Nakamura, S., Settai, S., Hayashidani, H., Urabe, T., Namai, S., and Une, Y. (2013). Outbreak of yersiniosis in Egyptian rousette bats (Rousettus aegyptiacus) caused by Yersinia pseudotuberculosis serotype 4b. J. Comp. Pathol. 148, 410–413. doi: 10.1016/j.jcpa.2012.07.007
Nakano, T., Kawaguchi, H., Nakao, K., Maruyama, T., Kamiya, H., and Sakurai, M. (1989). Two outbreaks of Yersinia pseudotuberculosis 5a infection in Japan. Scand. J. Infect. Dis. 21, 175–179. doi: 10.3109/00365548909039966
Nikolova, S., Wesselinova, D., and Vesselinova, A. (2001). Pretreatment of Guinea-pigs with iron or desferal influences the course of Yersinia enterocolitica infections. J. Vet. Med. B Infect. Dis Vet. Public Health 48, 167–178. doi: 10.1046/j.1439-0450.2001.00439.x
Nuorti, J. P., Niskanen, T., Hallanvuo, S., Mikkola, J., Kela, E., Hatakka, M., et al. (2004). A widespread outbreak of Yersinia pseudotuberculosis O:3 infection from iceberg lettuce. J. Infect. Dis. 189, 766–774. doi: 10.1086/381766
Osieka, V., Grobbel, M., Schmoger, S., Szentiks, C. A., Irrgang, A., Käsbohrer, A., et al. (2018). Complete draft genome sequence of an Extended-Spectrum beta-Lactamase-producing Citrobacter freundii strain recovered from the intestine of a house sparrow (Passer domesticus) in Germany, 2017. Genome Announc. 26:e00599-18. doi: 10.1128/genomeA.00599-18
Owens, J., Barton, M. D., and Heuzenroeder, M. W. (2013). The isolation and characterization of campylobacter jejuni bacteriophages from free range and indoor poultry. Vet. Microbiol. 162, 144–150. doi: 10.1016/j.vetmic.2012.08.017
Pärn, T., Hallanvuo, S., Salmenlinna, S., Pihlajasaari, A., Heikkinen, S., Telkki-Nykanen, H., et al. (2015). Outbreak of Yersinia pseudotuberculosis O:1 infection associated with raw milk consumption, Finland, spring 2014. Euro Surveill. 20:30033. doi: 10.2807/1560-7917.ES.2015.20.40.30033
Press, N., Fyfe, M., Bowie, W., and Kelly, M. (2001). Clinical and microbiological follow-up of an outbreak of Yersinia pseudotuberculosis serotype Ib. Scand. J. Infect. Dis. 33, 523–526. doi: 10.1080/00365540110026539
Rashid, M. H., Revazishvili, T., Dean, T., Butani, A., Verratti, K., Bishop-Lilly, K. A., et al. (2012). A Yersinia pestis-specific, lytic phage preparation significantly reduces viable Y. pestis on various hard surfaces experimentally contaminated with the bacterium. Bacteriophage 2, 168–177. doi: 10.4161/bact.22240
Reinhardt, M., Hammerl, J. A., and Hertwig, S. (2018a). Complete genome sequences of 10 Yersinia pseudotuberculosis isolates recovered from wild boars in Germany. Genome Announc. 6:e00266-18. doi: 10.1128/genomeA.00266-18
Reinhardt, M., Hammerl, J. A., Kunz, K., Barac, A., Nöckler, K., and Hertwig, S. (2018b). Yersinia pseudotuberculosis prevalence and diversity in wild boars in Northeast Germany. Appl. Environ. Microbiol. 84:e00675-18. doi: 10.1128/AEM.00675-18
Rimhanen-Finne, R., Niskanen, T., Hallanvuo, S., Makary, P., Haukka, K., Pajunen, S., et al. (2009). Yersinia pseudotuberculosis causing a large outbreak associated with carrots in Finland, 2006. Epidemiol. Infect. 137, 342–347. doi: 10.1017/S0950268807000155
Salem, M., Pajunen, M. I., Jun, J. W., and Skurnik, M. (2021). T4-like Bacteriophages IsolatedfromPig Stools Infect Yersiniapseudotuberculosis and Yersiniapestis Using LPSand OmpF asReceptors. Viruses 13:296. doi: 10.3390/v13020296
Salem, M., and Skurnik, M. (2018). Genomic characterization of sixteen Yersinia enterocolitica-infecting podoviruses of pig origin. Viruses 10:174. doi: 10.3390/v10040174
Salem, M., Virtanen, S., Korkeala, H., and Skurnik, M. (2015). Isolation and characterization of Yersinia-specific bacteriophages from pig stools in Finland. J. Appl. Microbiol. 118, 599–608. doi: 10.1111/jam.12722
Sandegren, L., and Sjoberg, B. M. (2004). Distribution, sequence homology, and homing of group I introns among T-even-like bacteriophages: evidence for recent transfer of old introns. J. Biol. Chem. 279, 22218–22227. doi: 10.1074/jbc.M400929200
Schwudke, D., Ergin, A., Michael, K., Volkmar, S., Appel, B., Knabner, D., et al. (2008). Broad-host-range Yersinia phage PY100: genome sequence, proteome analysis of virions, and DNA packaging strategy. J. Bacteriol. 190, 332–342. doi: 10.1128/JB.01402-07
Shopland, S., Barbon, A. R., Cotton, S., Whitford, H., and Barrows, M. (2020). Retrospective review of mortality in captive pink pigeons (Nesoenas mayeri) housed in European collections: 1977-2018. J. Zoo Wildl. Med. 51, 159–169. doi: 10.1638/2019-0121a
Storms, Z. J., and Sauvageau, D. (2014). Evidence that the heterogeneity of a T4 population is the result of heritable traits. PLoS One 9:e116235. doi: 10.1371/journal.pone.0116235
Tertti, R., Granfors, K., Lehtonen, O. P., Mertsola, J., Makela, A. L., Valimaki, I., et al. (1984). An outbreak of Yersinia pseudotuberculosis infection. J. Infect. Dis. 149, 245–250. doi: 10.1093/infdis/149.2.245
Trojet, S. N., Caumont-Sarcos, A., Perrody, E., Comeau, A. M., and Krisch, H. M. (2011). The gp38 adhesins of the T4 superfamily: a complex modular determinant of the phage's host specificity. Genome Biol. Evol. 3, 674–686. doi: 10.1093/gbe/evr059
Vasala, M., Hallanvuo, S., Ruuska, P., Suokas, R., Siitonen, A., and Hakala, M. (2014). High frequency of reactive arthritis in adults after Yersinia pseudotuberculosis O:1 outbreak caused by contaminated grated carrots. Ann. Rheum. Dis. 73, 1793–1796. doi: 10.1136/annrheumdis-2013-203431
Vincze, T., Posfai, J., and Roberts, R. J. (2003). NEBcutter: A program to cleave DNA with restriction enzymes. Nucleic Acids Res. 31, 3688–3691. doi: 10.1093/nar/gkg526
Wang, S., Zhao, W., Raza, A., Friendship, R., Johnson, R., Kostrzynska, M., et al. (2010). Prevalence of salmonella infecting bacteriophages associated with Ontario pig farms and the holding area of a high capacity pork processing facility. J. Sci. Food Agric. 90, 2318–2325. doi: 10.1002/jsfa.4090
Wichels, A., Biel, S. S., Gelderblom, H. R., Brinkhoff, T., Muyzer, G., and Schutt, C. (1998). Bacteriophage diversity in the North Sea. Appl. Environ. Microbiol. 64, 4128–4133. doi: 10.1128/AEM.64.11.4128-4133.1998
Williamson, D. A., Baines, S. L., Carter, G. P., da Silva, A. G., Ren, X., Sherwood, J., et al. (2016). Genomic insights into a sustained national outbreak of Yersinia pseudotuberculosis. Genome Biol. Evol. 8, 3806–3814. doi: 10.1093/gbe/evw285
Xu, B., Ma, X., Xiong, H., and Li, Y. (2014). Complete genome sequence of 285P, a novel T7-like polyvalent E. coli bacteriophage. Virus Genes 48, 528–533. doi: 10.1007/s11262-014-1059-7
Yoichi, M., Abe, M., Miyanaga, K., Unno, H., and Tanji, Y. (2005). Alteration of tail fiber protein gp38 enables T2 phage to infect Escherichia coli O157:H7. J. Biotechnol. 115, 101–107. doi: 10.1016/j.jbiotec.2004.08.003
Zhao, X., Cui, Y., Yan, Y., Du, Z., Tan, Y., Yang, H., et al. (2013). Outer membrane proteins ail and OmpF of Yersinia pestis are involved in the adsorption of T7-related bacteriophage yep-phi. J. Virol. 87, 12260–12269. doi: 10.1128/JVI.01948-13
Keywords: Yersinia pseudotuberculosis, phage, genome, diversity, Yersinia, zoo animals
Citation: Hammerl JA, Barac A, Bienert A, Demir A, Drüke N, Jäckel C, Matthies N, Jun JW, Skurnik M, Ulrich J and Hertwig S (2022) Birds Kept in the German Zoo “Tierpark Berlin” Are a Common Source for Polyvalent Yersinia pseudotuberculosis Phages. Front. Microbiol. 12:634289. doi: 10.3389/fmicb.2021.634289
Edited by:
Andrew Maitland Kropinski, University of Guelph, CanadaReviewed by:
Andrea Isabel Moreno Switt, Pontificia Universidad Católica de Chile, ChileMaria Fredriksson-Ahomaa, University of Helsinki, Finland
Copyright © 2022 Hammerl, Barac, Bienert, Demir, Drüke, Jäckel, Matthies, Jun, Skurnik, Ulrich and Hertwig. This is an open-access article distributed under the terms of the Creative Commons Attribution License (CC BY). The use, distribution or reproduction in other forums is permitted, provided the original author(s) and the copyright owner(s) are credited and that the original publication in this journal is cited, in accordance with accepted academic practice. No use, distribution or reproduction is permitted which does not comply with these terms.
*Correspondence: Stefan Hertwig, c3RlZmFuLmhlcnR3aWdAYmZyLmJ1bmQuZGU=