- 1Department of Microbiology and Immunology, Seoul National University College of Medicine, Seoul, South Korea
- 2Department of Biomedical Sciences, Seoul National University College of Medicine, Seoul, South Korea
- 3Institute of Endemic Diseases, Seoul National University Medical Research Center and Bundang Hospital, Seoul, South Korea
Autotransporter proteins are widely present in Gram-negative bacteria. They play a pivotal role in processes related to bacterial pathogenesis, including adhesion, invasion, colonization, biofilm formation, and cellular toxicity. Bioinformatics analysis revealed that Orientia tsutsugamushi, the causative agent of scrub typhus, encodes six different autotransporter genes (scaA–scaF). Although four of these genes (scaA, scaC, scaD, and scaE) are present in diverse strains, scaB and scaF have been detected in only a limited number of strains. Previous studies have demonstrated that ScaA and ScaC are involved in the adherence of host cells. However, the putative function of other O. tsutsugamushi Sca proteins has not been studied yet. In this study, we show that scaB is transcribed and expressed on the surface of O. tsutsugamushi Boryong strain. Using a heterologous Escherichia coli expression system, we demonstrated that ScaB-expressing E. coli can successfully mediate adherence to and invasion into non-phagocytic cells, including epithelial and endothelial cells. In addition, pretreatment with a recombinant ScaB polypeptide inhibits the entry of O. tsutsugamushi into cultured mammalian cells. Finally, we also identified the scaB gene in the Kuroki and TA686 strains and observed high levels of sequence variation in the passenger domains. Here, we propose that the ScaB protein of O. tsutsugamushi can mediate both adhesion to and invasion into host cells in the absence of other O. tsutsugamushi genes and may play important roles in bacterial pathogenesis.
Introduction
Scrub typhus is a re-emerging vector-borne disease prevalent in the Asia-Pacific region (Kelly et al., 2009; Kala et al., 2020; Richards and Jiang, 2020). It is caused by an obligate intracellular Gram-negative bacterium, namely Orientia tsutsugamushi, which is transmitted to mammalian hosts, including rodents and humans, via Leptotrombidium mites at their larval stage (Seong et al., 2001). The clinical symptoms of scrub typhus are highly variable, ranging from mild to fatal infections. Approximately 1–2 weeks after being bitten by the infected vector, patients present with fever, headache, rash, nausea, lymphadenopathy, and eschar at the bite site (Ponnusamy et al., 2018; Kala et al., 2020). Delayed treatment with appropriate antibiotics, such as tetracycline, chloramphenicol, and macrolides, often results in pneumonitis, meningitis, myocarditis, acute renal failure, and acute respiratory distress syndrome. The mortality rate of scrub typhus in the pre-antibiotic era reached up to 40–50% (Taylor et al., 2015).
More than a million cases are estimated to occur annually and a billion individuals are under risk of suffering this disease (Kelly et al., 2009, 2015). In addition, accumulating reports have shown confirmed or suspected scrub typhus cases occurring outside the traditional endemic regions, such as in South America, the Arabian Peninsula, and Africa (Weitzel et al., 2016). Moreover, rodents infected with bacterial strains closely related to O. tsutsugamushi have also been found in Africa and Southern Europe (Izzard et al., 2010; Cosson et al., 2015; Kolo et al., 2016; Masakhwe et al., 2018). These reports suggest that the disease is no longer restricted to its endemic territory and is widely distributed. These findings also indicate the existence of unidentified antigenically different strains may exist (Ghorbani et al., 1997; Izzard et al., 2010; Cosson et al., 2015; Weitzel et al., 2016; Jiang and Richards, 2018).
Despite the growing risk and wide spread of the disease, there is no vaccine available to prevent it. Although various types of vaccine candidates have been extensively studied, most of them induce only short-term immunity to their homologous strain (Chattopadhyay and Richards, 2007; Valbuena and Walker, 2012). Therefore, further identification of potential targets as vaccine antigens is required to develop effective vaccines.
Orientia tsutsugamushi is an obligate intracellular bacterium that must be internalized into host cells for propagation of infection. Although the precise mechanism of host cell invasion is poorly characterized, several bacterial proteins have been shown to be necessary for attachment and entry into host cells. Previously, we reported that one of the major bacterial outer membrane proteins, a 56 kDa type-specific antigen (TSA56), mediates cellular adherence to or invasion into non-phagocytic cells by interacting with fibronectin (Lee et al., 2008; Cho et al., 2010b). Additionally, we found that there were five different autotransporter proteins (ScaA–ScaE) encoded in the genomes of various strains and defined as Va subclass based on protein 3D structure prediction (Ha et al., 2013). Recently, another group identified a sixth autotransporter gene, scaF, in some strains; however, its structure has not yet been classified (Ha et al., 2012; Koralur et al., 2018).
Bacterial autotransporter proteins have been demonstrated to play a role during infection. In general, autotransporter proteins are widely found in Gram-negative bacteria and possess unique structural properties, with their structure consisting of a signal peptide, an N-terminal passenger domain, and a C-terminal autotransporter domain. The passenger domain is translocated through the autotransporter domain and exposed to the extracellular environment, conferring multiple virulence functions, including adhesion, invasion, colonization, biofilm formation, and cellular toxicity (Henderson and Nataro, 2001; Nishimura et al., 2010; Meuskens et al., 2019). Autotransporter genes, including scaA, scaC, scaD, and scaE genes, have been detected in most strains of O. tsutsugamushi, whereas scaB and scaF have only been found in a few strains. Previously, we showed that scaA and scaC are transcribed and actively expressed on the bacterial surface (Ha et al., 2011, 2015). ScaA functions as an adhesion molecule and induces an antibody response that can neutralize the bacterial entry into the host cell, whereas ScaC mediates only adherence, but not invasion into the host cells. Preincubation of host cells with recombinant ScaC significantly inhibited their interaction with O. tsutsugamushi (Ha et al., 2011, 2015). Considering the functional role of these autotransporters in O. tsutsugamushi and their genetic distribution among various strains, further investigation of the biological effects of other Sca proteins needs to be performed.
In this study, we investigated the function of the ScaB protein of O. tsutsugamushi. We found that ScaB expression in Escherichia coli was sufficient to mediate both bacterial adherence to and invasion into non-phagocytic host cells, and preincubation of mammalian cell cultures with the ScaB protein inhibited bacterial invasion. In addition, we newly detected the presence of the scaB gene in the Kuroki and TA686 strains and observed high levels of sequence variation in the passenger domains. Taken together, these results demonstrate that O. tsutsugamushi utilizes ScaB as a ligand protein for the adhesion and invasion of host cells and suggest that ScaB may play an important role in bacterial pathogenesis.
Materials and Methods
Ethics Statement
Ethical approval for this work was granted by the Institutional Review Boards of Seoul National University Hospital (IRB 1308-058-513). Animal experiments were approved by the Seoul National University Hospital Institutional Animal Care and Use Committee (SNU-180727-6-7) and performed in strict accordance with the recommendations in the National Guide Line for the care and use of laboratory animals.
Cell Lines
L929 cells (ATCC NCTC929, American Type Culture Collection), HeLa cells (ATCC CCL-2), A549 cells (ATCC CCL-185), and ECV304 (ATCC CRL-1998), an endothelial cell-like cell line, were maintained in DMEM (Welgene, Daegu, Korea) supplemented with 10% heat-inactivated fetal bovine serum (FBS; Welgene), 100 U/ml penicillin, and 100 μg/ml streptomycin (Gibco BRL) at 37°C in 5% CO2.
Preparation of O. tsutsugamushi
Orientia tsutsugamushi strains Boryong (AM494475) and Kuroki (M63380) were semi-purified as previously described (Ha et al., 2011). Briefly, when more than 90% of the cells were infected, as determined by an indirect immunofluorescence antibody technique, the cells were collected, homogenized using a glass Dounce homogenizer (Wheaton, Inc., Millville, NJ, United States), and centrifuged at 500 × g for 5 min (Kim et al., 2019). The supernatant was stored in liquid nitrogen until use.
Reverse Transcription-PCR
To detect O. tsutsugamushi scaB gene expression, total RNA was isolated from O. tsutsugamushi-infected L929 cells by using an RNeasy minikit (Qiagen, Hilden, Germany). The RNA samples were then digested with RNase-free DNase (Qiagen, Hilden, Germany) at room temperature to remove any contaminating DNA. Reverse transcriptase PCR (RT-PCR) was performed using a reverse transcription system (iNtRON Biotechnology, Seongnam, South Korea) and an AccuPower® Taq PCR PreMix kit (Bioneer, Daejon, South Korea). The reactions were performed according to the manufacturers’ instructions by using 5 μg total RNA and 5 μM concentrations of the relevant primers listed in Table 1.
Cloning and Expression of ScaB
The full-length of scaB gene (nucleotide 1–1,950) was amplified from the genomic DNA of the O. tsutsugamushi Boryong and Kuroki genotype by using the primer pairs listed in Table 1. The PCR product from Kuroki genotype is directly cloned into pCR™2.1 vector for sequencing. To generate the recombinant ScaB protein, a gene fragment corresponding to the ScaB passenger domain (amino acids 23–372) was amplified from the Boryong genomic DNA by using the primer pair listed in Table 1. The amplified fragment was then directionally cloned into pET28a or pGEX4T-1 vector (Novagen, Gibbstown, NJ, United States).
For the expression and purification of ScaB proteins, E. coli BL21 (DE3; Novagen) was transformed with ScaB passenger domain then following induction with isopropyl β-D-thiogalactoside (IPTG; 0.1 mM, Duchefa, Zwijndrecht, Netherlands) at 16°C for 16 h, the proteins were purified using Ni-nitrilotriacetic acid His-resin (Qiagen, Carlsbad, CA, United States) or glutathione-Sepharose 4Bcolumns (GE Healthcare, Piscataway, NJ, United States) according to manufacturer’s instructions. The purified proteins were dialyzed against phosphate-buffered saline (PBS) in a Slide-A-Lyzer Dialysis Cassette (Thermo scientific, Rockford, IL, United States) at 4°C for overnight.
Sequence Analysis
Nucleotide sequences of scaB genes amplified from Kuroki genotype were deposited to GenBank under accession no. MW216678. Sequences of scaB genes from various genotypes (AM494475.1 for Boryong, LAOM01000054.1 for Sido, LAOA01000033.1 for TA716 genotype, and SPR13699.1 for TA686) were also used for comparative analysis. Nucleotide sequence alignments for constructing phylogenetic trees were processed by Clustal W with maximum likelihood method implemented in MEGA6 software (Tamura et al., 2013). The similarity and identity of those nucleotides and amino acids were calculated through Matrix Global Alignment Tool (MatGAT) version 2.03 (Campanella et al., 2003). The aligned nucleotide sequences were evaluated in SimPlot version 3.5.1 with Kimura (2-parameter) and Empiric Ts/Tv ratio settings (Lole et al., 1999). The aligned amino acid sequences were analyzed through the BLOSUM62-referenced 100 amino acid sliding window analysis. The output values were calculated from R-Project1 (Ha et al., 2015; Kim et al., 2017). Line graphs were visualized by GraphPad Prism software version (Graph-Pad Software Inc., La Jolla, CA, United States). For protein structural prediction, HHPred,2 PSIPRED,3 and I-TASSER4 were used (Söding et al., 2005; Buchan et al., 2013; Yang and Zhang, 2015; Buchan and Jones 2019).
Antibodies and Reagents
Both preimmune and anti-ScaB polyclonal mouse serum (produced by immunization with purified ScaB24–372 protein; Koma Biotech, Seoul, South Korea) were used for the experiments. Six week old female C57BL/6 mice (Orientt Bio Inc., Seongnam, South Korea; n=5) were immunized subcutaneously three times with 2 weeks interval. Ten microgram of purified ScaB protein in PBS emulsified 1:1 with 2% alhydrogel adjuvant (Invitrogen, Brenntag Biosector, Denmark) was used for immunization and blood were collected at 1 week after the last immunization.
Scrub typhus patient sera were used for staining O. tsutusgamushi in this study. Human sera were prepared from scrub typhus patients, control patients with an acute febrile illness not diagnosed as scrub typhus, or healthy volunteers, following institutional review board approval and the receipt of informed consent from all subjects. Horseradish peroxidase (HRP)-conjugated anti-mouse or anti-human IgG secondary antibodies (Thermo Fisher Scientific, MA, United States) were used for immunostaining. The anti-E. coli antibody (Abcam, Cambridge, MA, United States) was used for visualizing the association of E. coli with cells. The Alexa Fluor 488‐ or Alexa Fluor 594-conjugated anti-mouse, -human antibodies and Alexa Fluor 488 Phalloidin and TO-PRO3 used in the immunofluorescence assays were purchased from Molecular Probes (Thermo Fisher Scientific, MA, United States).
Protein Binding Assay
HeLa cells (2 × 105 cells in a 24-well plate) were incubated with glutathione S-transferase (GST) or GST-ScaB proteins for 1 h, washed extensively with PBS, and fixed with 4% paraformaldehyde for 15 min. Cells were subsequently incubated with ToPro-3 (Thermo Fisher Scientific, MA, United States) for nuclear staining and observed under a confocal microscope or analyzed using a BD LSRFortessa (Becton Dickinson, Mountain View, CA, United States).
Membrane Fractionation of E. coli
The outer membrane of recombinant E. coli was fractionated as previously described (Cardwell and Martinez, 2009). Briefly, 10 ml of induced E. coli that harboring an empty vector or a vector encoding scaB gene (nucleotide 118 to 1947) cultures was pelleted and resuspended in 1 ml of lysis buffer (PBS plus protease inhibitor cocktail). Cells were lysed by sonication for 3 s and incubated further for 10 s on ice. Unbroken cells were removed by centrifugation for 10 min at 4°C at 1,000 × g. The supernatant was transferred to a new tube, and inner membrane protein was extracted with Sarkosyl (final concentration, 0.5%) at room temperature for 5 min. Outer membrane fractions were pelleted by centrifugation at 13,000 × g for 30 min at 4°C, resuspended in 2 X sample buffer, resolved by sodium dodecyl sulfate-polyacrylamide gel electrophoresis (SDS-PAGE), and subsequently analyzed by immunoblotting with anti-ScaB mouse serum and goat anti-mouse IgG-HRP conjugate.
Immunofluorescence Microscopy
Immunofluorescence microscopy was used to visualize O. tsutsugamushi. L929 cells infected with O. tsutsugamushi were washed with PBS and fixed with 4% paraformaldehyde and then permeabilized with 0.2% Triton X-100. Pooled scrub typhus human serum or anti-ScaB immune serum for 1 h, followed by incubation with Alexa Fluor 488-conjugated goat anti-mouse IgG and Alexa Fluor 594-conjugated goat anti-human IgG (Thermo Fisher Scientific). In some experiments, recombinant E. coli was stained with preimmune mouse serum, anti-ScaB serum, or anti-E. coli followed by incubation with Alexa Fluor 488-conjugated goat anti-mouse IgG (Thermo Fisher Scientific). Alexa Fluor 488 Phalloidin and TO-PRO3 were used for staining the cell actin and nucleus. Cells were examined under an Olympus FV1000 laser scanning confocal microscope (Olympus, Tokyo, Japan). Images of cell sections were analyzed and processed using the Olympus Fluoview software (Olympus).
Cellular Adhesion and Invasion Assay
Bacterial adhesion and invasion assays were performed as previously described (Cardwell and Martinez, 2009; Riley et al., 2010). Briefly, E. coli strains harboring an empty vector or a vector encoding scaB gene were induced with IPTG and added to confluent monolayers of HeLa, A549, and ECV304 cells in serum-free media. Portions of the bacterium-containing media were plated to determine the number of colony-forming unit (CFU) added to each host cell monolayer. Contact between bacteria and the cultured cells was synchronized by centrifugation at 200 × g, and the preparations were incubated at 37°C for either 20 or 60 min for the adherence and invasion assays, respectively. For the invasion assays, infected cells were washed extensively with PBS and incubated for 2 h with complete medium supplemented with 100 μg/ml of gentamicin to kill any extracellular bacteria. For all E. coli assays, infected cells were washed extensively with PBS and the bacteria liberated by incubation with 0.1% Triton X-100 in sterile water. The lysate was then plated on LB agar to enumerate the cell-associated bacteria. The results were expressed as the percentages of bacteria recovered relative to the number of bacteria in the initial inoculum.
For the inhibition assays, cells were preincubated with 50 μg/ml of GST or GST-ScaB24–372 at 37°C for 1 h. Thirty minutes after bacterial inoculation, infected cells were washed three times with PBS, fixed with 4% paraformaldehyde, and permeabilized in a 0.2% Triton X-100 solution for use in immunofluorescence assays incorporating scrub typhus patient serum followed by Alexa Fluor 488-conjugated goat anti-human IgG to stain host cell-associated bacteria. One hundred cells were randomly selected by using an Olympus FV1000 laser scanning confocal microscope and analyzed using the Fluoview software.
Statistical Analysis
The data were analyzed using the Graph Pad Prism 5.01 software. Statistical analysis was performed using the two-tailed Student’s t-test with 95% confidence interval. Data were expressed as means ± SDs.
Results
ScaB Is Expressed in O. tsutsugamushi
To determine whether scaB is actively transcribed in O. tsutsugamushi Boryong during interaction with host cells, we purified total RNA from bacteria-infected L929 cells, removed any contaminating bacterial or host genomic DNA, and performed RT-PCR to amplify the scaB transcripts. As shown in Figure 1A, the expression of specific scaB transcripts (1950 bp) was confirmed in O. tsutsugamushi. Next, to identify endogenous ScaB protein in O. tsutsugamushi, we generated a ScaB antiserum by immunization of mice with the soluble ScaB passenger domain (amino acids 36–373; Figure 1B). The scaB gene is predicted to encode a protein with a molecular mass of ~73 kDa. Western blot analysis of whole-cell lysates of O. tsutsugamushi using anti-ScaB serum showed a reactive band at ~80 kDa, which was not observed in non-immunized mouse serum (Figure 1C). To further confirm the cellular localization of ScaB in O. tsutsugamushi, bacteria were immunostained with pooled sera of scrub typhus patients together with anti-ScaB serum or preimmune mouse serum. As shown in Figure 1D, ScaB was observed on the surface of O. tsutsugamushi cells, but not when stained with the preimmune mouse serum control. Taken together, these results confirm that the scaB gene is actively transcribed and translated in O. tsutsugamushi and that the protein might be expressed on the bacterial outer membrane surface.
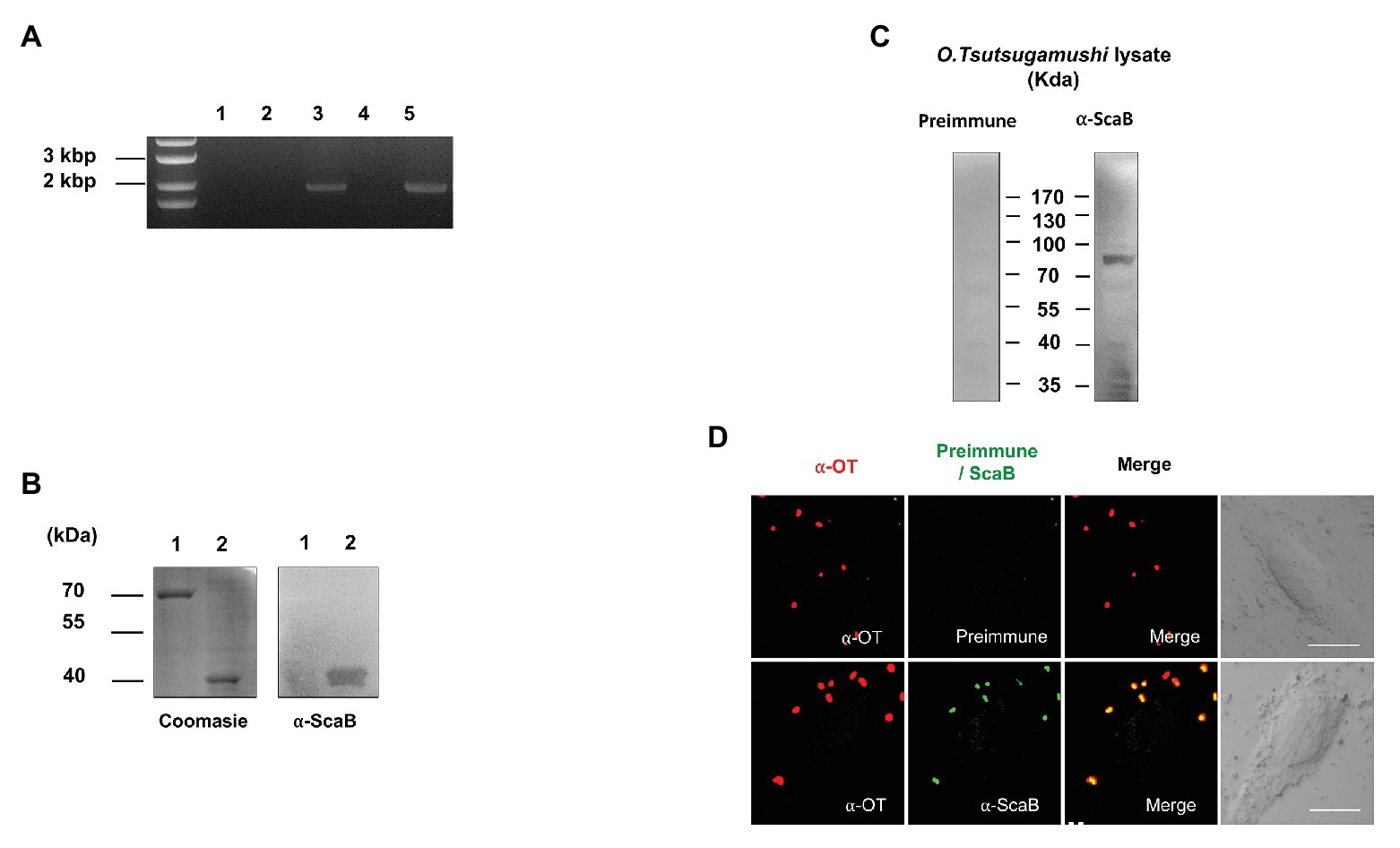
Figure 1. Expression of ScaB by Orientia tsutsugamushi. (A) Reverse transcriptase PCR (RT-PCR) of scaB mRNA from L929 cells infected with O. tsutsugamushi. Primer only without template (lane 1), total RNA (without reverse transcription) isolated from infected cells (lane 2), from genomic DNA (lane 3), from cDNA from uninfected cells (lane 4), and from cDNA from infected cells (lane 5). PCR product is 1950 bp in size. (B) Specificity of anti-ScaB antisera. BSA (lane 1) and recombinant ScaB protein (amino acids 23–1,372; lane 2) were stained with Coomassie blue (left panel) and immunoblotted with the anti-ScaB antisera. Recombinant ScaB protein is 36 kDa in size. (C) Western immunoblot analysis of O. tsutsugamushi whole-cell lysate probed with mouse preimmune sera (left panel) and anti-ScaB serum (right panel). Full-length ScaB protein is 77 kDa in size. (D) Immunofluorescence confocal microscopy using preimmune serum or anti-ScaB serum in the O. tsutsugamushi-infected L929 cells. The left-hand panels show bacteria stained with pooled serum from scrub typhus patients (∝-OT). DIC, differential interference contrast. Scale bars, 10 μm.
ScaB Protein Mediates Adherence to and Invasion Into Host Cells
In previous studies (Ha et al., 2011, 2015), we reported that the passenger domain of ScaA and ScaC proteins from O. tsutsugamushi could mediate bacterial adherence to host cells by using the heterologous E. coli expression system. Here, we examined whether the O. tsutsugamushi ScaB protein is also involved in bacterial adherence or invasion. First, we performed a protein-binding assay using either purified GST or GST-ScaB soluble protein. Incubation of HeLa cells with GST-ScaB protein resulted in increased binding to the cells and punctate distribution in the cell membrane (Figure 2A). Binding efficiency was quantified by flow cytometry, and the mean fluorescence intensity (MFI) of the HeLa cells incubated with GST-ScaB was significantly increased (MFI = 183.7) compared to that of cells incubated with GST (MFI = 20.7) or that of untreated cells (MFI = 3.35; Figure 2B).
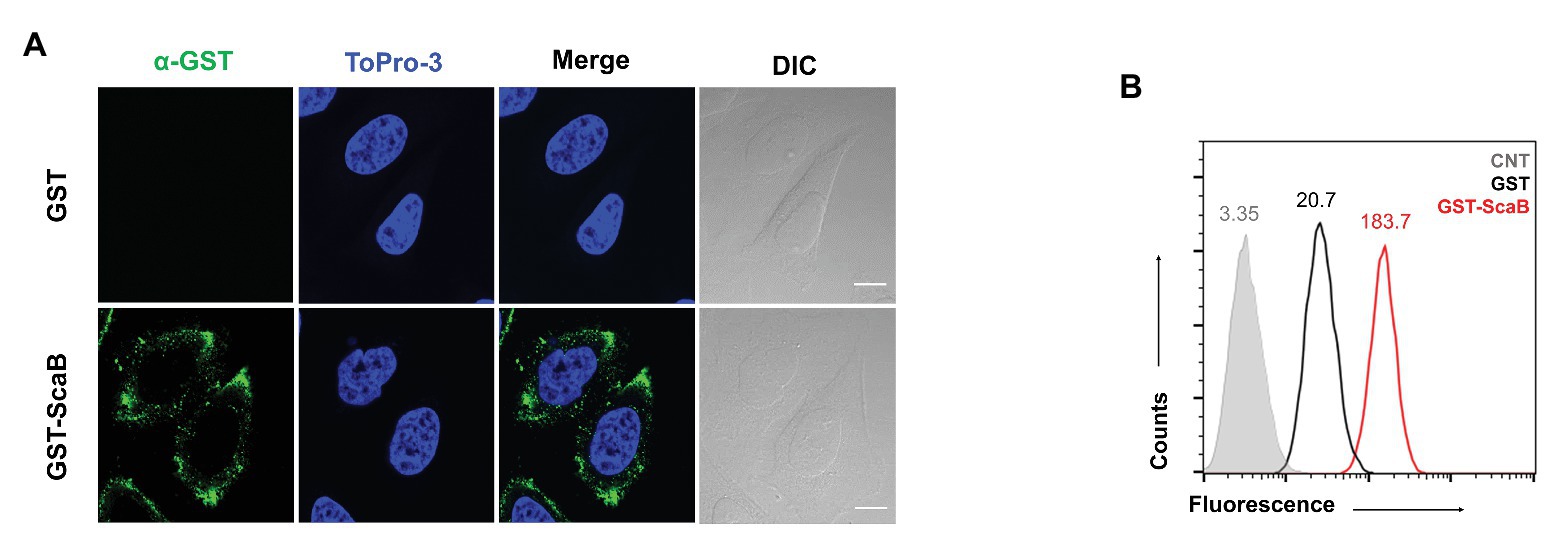
Figure 2. Recombinant ScaB protein-binding assay to HeLa cells. (A) Recombinant glutathione S-transferase (GST) or GST-ScaB23–372 protein was incubated with HeLa cells for 1 h. Cells were visualized by fluorescence microscopy after incubated with anti-GST followed by Alexa488-conjugated goat anti-mouse IgG antibody (green) and ToPro-3 (blue). Scale bars, 10 μm. (B) Flow cytometric analysis of the GST (black) and GST-ScaB (red) protein binding to HeLa cells. The gray histogram represents untreated cells
To further confirm the putative role of ScaB, the full length of the scaB open reading frame was cloned into an E. coli IPTG-inducible expression vector with a pelB signal sequence. Cellular localization and expression of ScaB were confirmed by confocal microscopy and immunoblotting with the anti-ScaB antibody. ScaB was observed on the surface of all recombinant E. coli cells, and a ~72 kDa product was confirmed in the isolated outer membrane fraction from ScaB-expressing E. coli (Figures 3A,B). Next, we assessed the ability of ScaB-expressing E. coli to adhere to cultured mammalian cells, including epithelial (HeLa and A549) and endothelial (ECV304) cells. Confluent monolayered cultured cells were incubated with IPTG-induced E. coli harboring either an empty vector or the ScaB-containing vector. Immunofluorescence analysis revealed an increase in the number of adherent ScaB-expressing E. coli bacteria (Figure 3C). This ScaB-mediated enhanced adhesion was verified by removing the adherent bacteria from the live host cells and counting them by CFU-based quantification assays. As a result, ScaB-expressing E. coli showed a significant increase in the adherence to both epithelial and endothelial cells (Figure 3D).
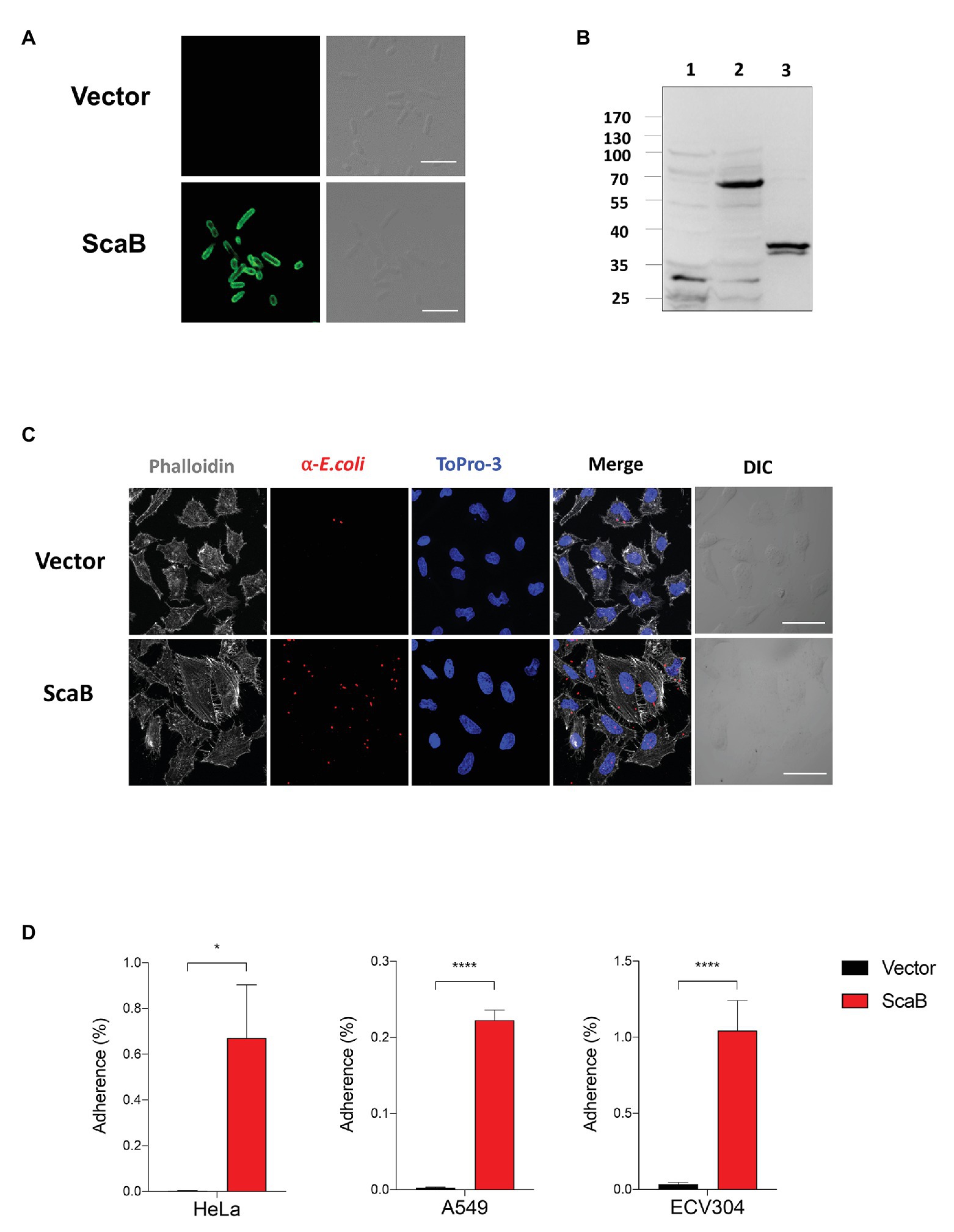
Figure 3. Expression of ScaB in Escherichia coli is sufficient to mediate adherence to mammalian cells. (A) Immunofluorescence microscopy using an anti-scaB antibody revealed the presence of ScaB on the surface of the recombinant E. coli (lower panel). Preimmune serum did not detect the recombinant protein (upper panel). Scale bars, 5 μm. (B) Expression of O. tsutsugamushi ScaB on the surface of E. coli. Immunoblot analysis of outer membrane fractions of induced E. coli harboring the empty vector (lane 1), ScaB (lane 2), and recombinant ScaB23–372 protein was performed using an anti-ScaB serum. (C) E. coli transformed with the pET28a vector or with ScaB domain was induced with isopropyl β-D-thiogalactoside (IPTG) and incubated with HeLa cells. Confluent monolayers of HeLa cells were infected for 30 min at 37°C, washed repeatedly with phosphate-buffered saline (PBS), and then stained with an anti-E. coli antibody (red), Phalloidin (gray), and ToPro-3 for nuclear staining (blue). Scale bars, 50 μm. (D) Colony-forming unit (CFU)-based quantification of adherent E. coli transformed with the vector (black bars) or ScaB (red bars) was performed for different host cells (HeLa, A549, and ECV304 cell lines). *p < 0.05 and ****p < 0.0001. The data presented are representative of at least three independent assays for each cell line. Error bars represent the SD of each data set.
In a member of the nearest relative genus, Rickettsia conorii, OmpA (Sca0), OmpB (Sca5), and Sca2 have been shown to mediate not only adherence, but also invasion of host cells. To further confirm the ability of ScaB to mediate invasion of host cells, we utilized a gentamicin protection assay. Extracellular bacteria were killed by gentamicin, and internalized bacteria were quantified by CFU assays. As shown in Figure 4, in the absence of any O. tsutsugamushi surface antigens, ScaB is sufficient to mediate the invasion of either epithelial or endothelial cells. Taken together, these results demonstrate that unlike other O. tsutsugamushi Sca proteins (Ha et al., 2011, 2015), ScaB is involved in both adherence and invasion of host cells.
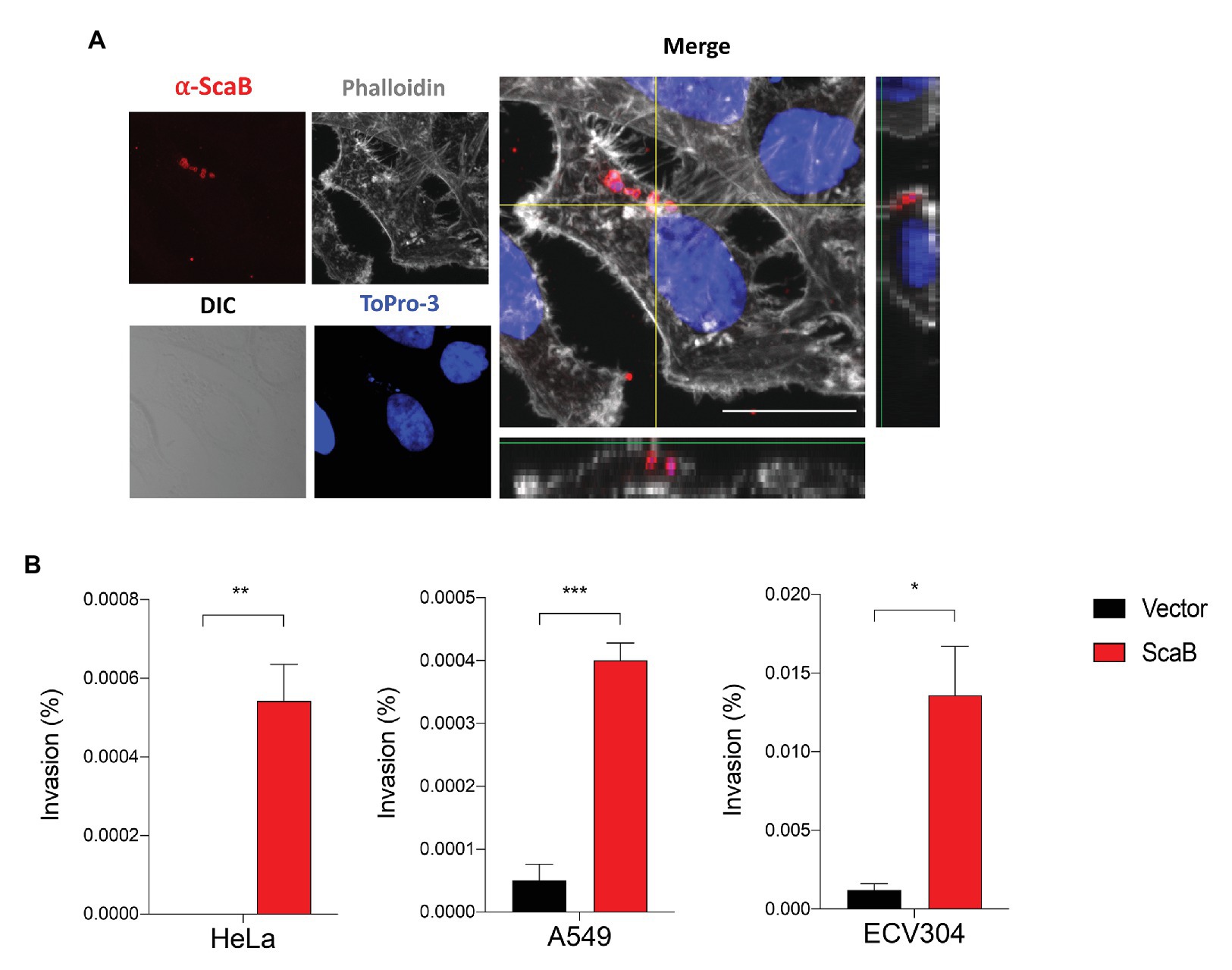
Figure 4. Expression of ScaB in E. coli is mediate invasion of mammalian cells. (A) Confluent cell monolayers of HeLa cells were infected for 1 h with E. coli transformed with the vector or ScaB and assessed for invasion by gentamicin protection assay. Cells were stained with an anti-scaB antisera (red), Phalloidin (gray), and ToPro-3 for nuclear (blue). The image is a projection of a 1 μm z-stack collected through x60 objective. Scale bars, 50 μm. (B) CFU-based quantification of bacterial invasion into mammalian host cells. Invasion is presented as the percentage of bacteria recovered after the gentamicin challenge out of the inoculums. The data presented are representative of at least two independent experiments for each individual cell line. Error bars represent the SD of each data set. *p < 0.05, **p < 0.01, and ***p < 0.001.
The Soluble ScaB Polypeptide Inhibits Invasion by O. Tsutsugamushi
In order to confirm the ScaB-mediated invasion of cultured mammalian cells, we further determined whether the soluble ScaB polypeptide could competitively inhibit O. tsutsugamushi-host cell interaction. HeLa cells were preincubated with 50 μg/ml of soluble GST or GST-ScaB polypeptide for 1 h, followed by incubation with O. tsutsugamushi for 30 min. Cells were extensively washed and the O. tsutsugamushi-host cell ratio was determined by confocal microscopy. As shown in Figure 5, preincubation with GST-ScaB induced a significant decrease in the total number of bacteria per cell [14 multiplicity of infection (MOI)/cell] compared to that of cells incubated with GST (9 MOI/cell). The number of bacteria per cell was reduced by approximately 35% when incubated with the GST-ScaB polypeptide compared to that of GST treated cells, suggesting that the soluble ScaB polypeptide competitively inhibits ScaB-mediated O. tsutsugamushi adherence to host cells by blocking its cognate mammalian ligand.
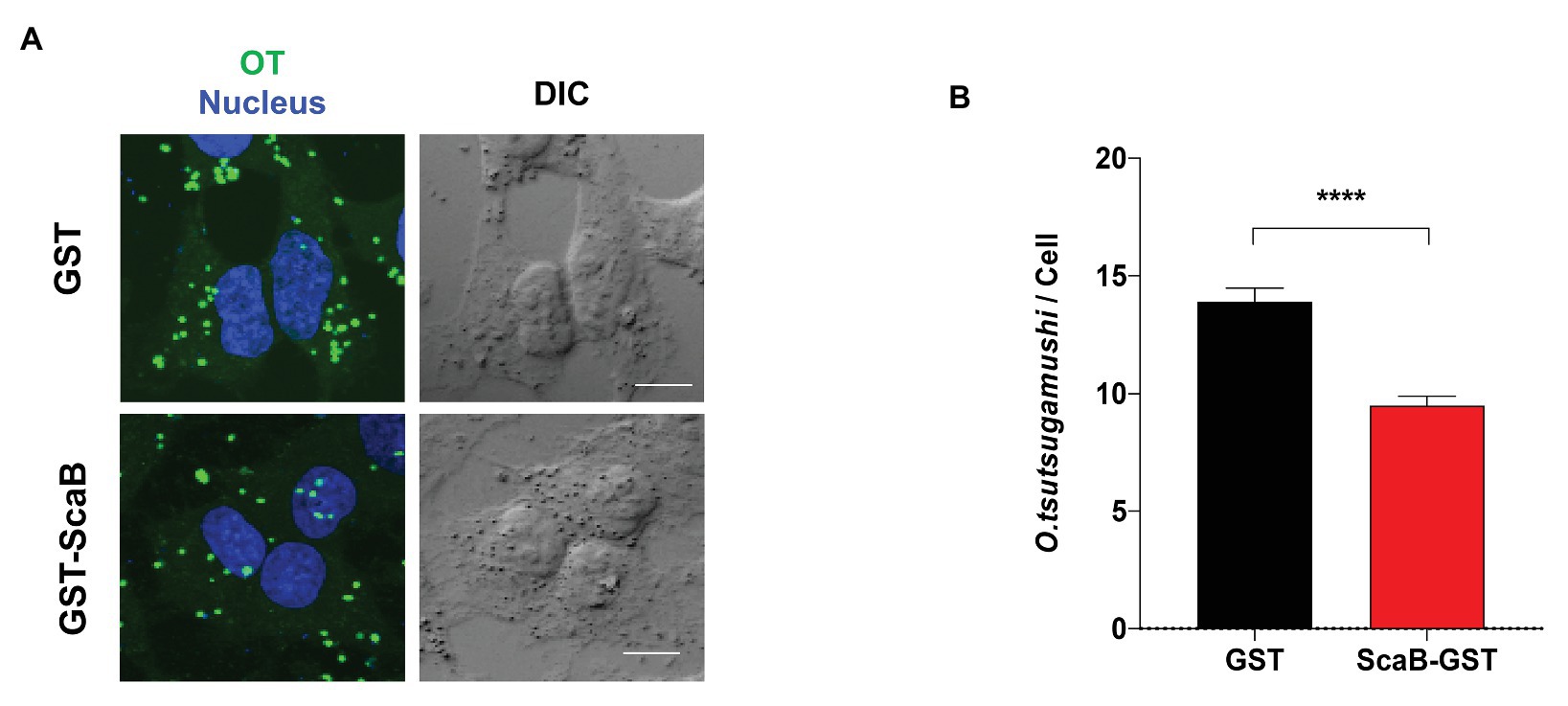
Figure 5. Inhibition of O. tsutsugamushi interactions with host cells by the ScaB polypeptide. (A) Preincubation of HeLa cells with GST-ScaB (lower panels) significantly inhibited O. tsutsugamushi interactions with host cells compared with preincubation with GST (upper panels). After incubation of the polypeptides with the host cells, O. tsutsugamushi was added, and incubation continued for a further 30 min. Cells were visualized by immunofluorescence microscopy. Orientia tsutsugamushi (green) and Nuclei (blue). (B) The numbers of bacteria associated with each of 100 randomly selected host cells. The bars indicate the means ± SDs of triplicate experiments. ****p < 0.0001.
Genetic Variation Among ScaB Genes From Different Strains of O. tsutsugamushi
In a previous study using bioinformatics analysis, we reported duplicated scaB genes (GenBank accession no. CAM79930.1 and CAM81232.1) were only found in the Boryong strain; however, Koralur et al. (2018) also detected scaB in Sido and TA716 strains by tBLASTn. This prompted us to examine the presence or absence of scaB in various strains and to compare the genetic variation among the identified genes. Based on the complete scaB sequence from the Boryong strain, we performed conventional PCR with different O. tsutsugamushi genomic DNA samples using primer sets that cover the full-length or the passenger domain (Table 1). In addition, using tBLASTn with the Boryong ScaB protein sequence, we analyzed the presence of scaB genes from different partial O. tsutsugamushi genome sequences available in the NCBI database. As a result, we detected for the first time the full-length scaB gene in the Kuroki and TA686 strains by conventional PCR and tBLASTn. Comparison of the size of scaB genes showed highly conserved sizes with no significant differences among Boryong, Kuroki (1950 bp), Sido (1998 bp), TA686 (1971 bp), and TA716 (1995 bp) strains. To further investigate the sequence differences among the strains, we used various software tools to analyze the phylogenetic distance and sequence similarity and identity. As shown in Figure 6A, genetic variation among the strains was quite high. In the phylogenetic tree, two gene clusters were generated by maximum likelihood and the similarity and identity between the translated amino acid sequences of full-length ScaB were 70.6–100.0 and 53.9–99.7%, respectively. The sequence variation observed in ScaB is primarily attributed to the high level of sequence variation in the passenger domain (Figures 6B,C). The similarity and identity of the translated amino acid sequences of the passenger (P) domain were 56.2–100.0 and 33.3–99.7%, respectively, and of the autotransporter (AT) domain were 87.9–97.4 and 80.1–97.1%, respectively (Figure 6B). In addition, using a structural prediction program, we compared the secondary structure of each passenger domain. As shown in Figure 6D, it was characterized by 11–14 helices, but their locations were different. Along with similarities in the gene cluster, the Boryong and Kuroki strains also showed almost identical location of the ∝-helix; however, the Sido, TA686, and TA716 strains showed different patterns. Taken together, these results suggest that scaB is present in specific strains of O. tsutsugamushi and is highly variable among different strains, although it supports adhesion to or invasion into host cells.
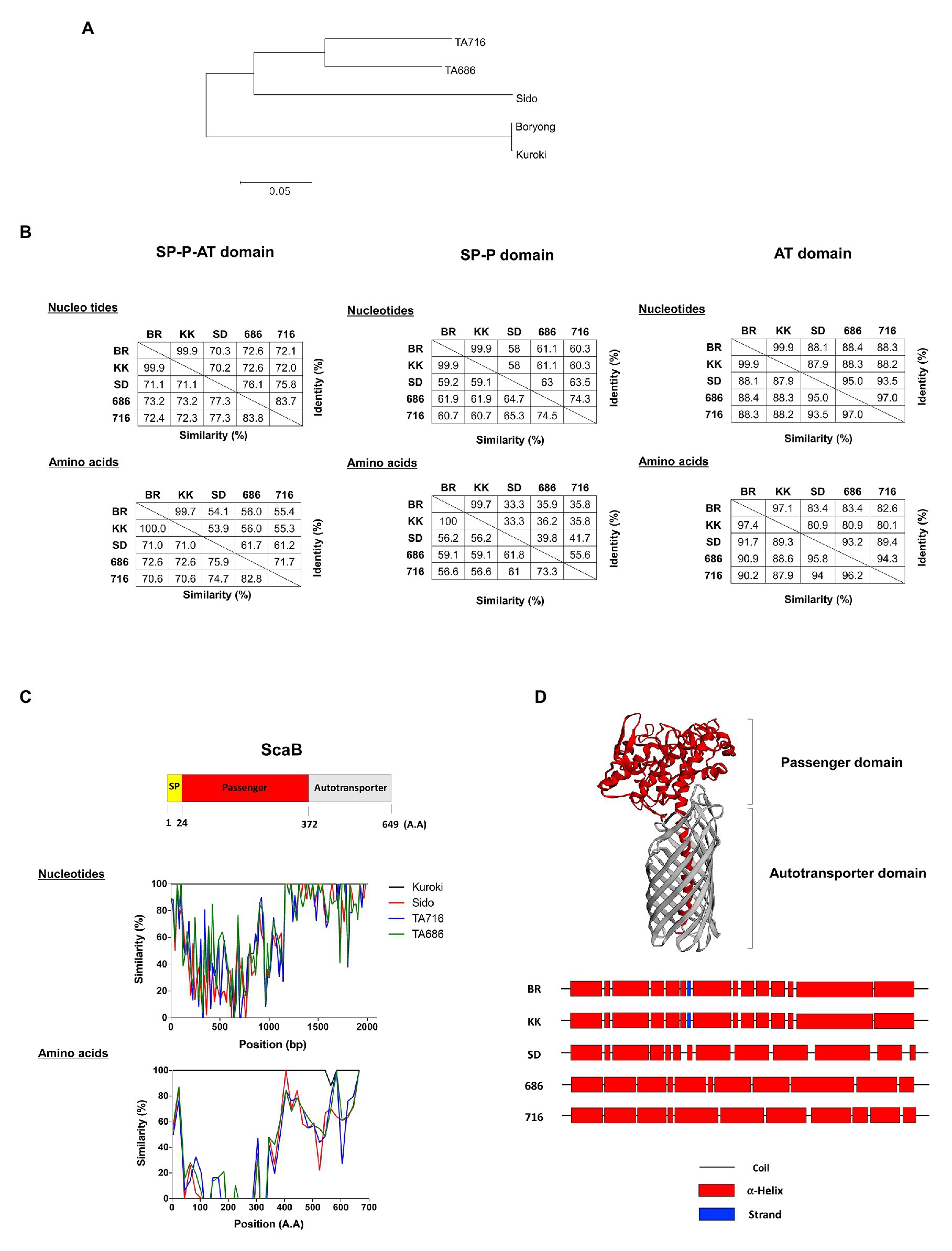
Figure 6. Comparison of scaB gene from indicated O. tsutsugamushi strains. (A) Amino acids sequence alignments for constructing phylogenetic trees were processed by Clustal W with the maximum likelihood method. (B) The similarity and identity of those nucleotides and amino acids were calculated through Matrix Global Alignment Tool [MatGAT; left: full-length of ScaB, middle: signal peptide(SP)-passenger(P) domain, and right: autotransporter (AT) domain]. (C) Similarity plot comparing ScaB sequence among the indicated O. tsutsugamushi strains and schematic representation above the graph indicates the relative sizes of translated ScaB amino acids sequence from Boryong strain and their sequence motiffs. (D) Secondary/tertiary structure of ScaB protein was predicted by HHPred, PSIPRED, and I-TASSER program. Predicted tertiary of ScaB protein (upper) and predicted secondary structure of passenger domain (Lower). BR, Boryong; KK, Kuroki; SD, Sido; 686, TA686; 716, TA716.
Discussion
Adherence to and invasion into host cells are essential steps of obligate intracellular bacteria and facilitate their persistence and survival in the host. These processes are mediated by multivalent ligand-receptor interactions (Pizarro-Cerda and Cossart, 2006). In this regard, O. tsutsugamushi must enter host cells to exploit their cellular machinery for intracellular replication. Although a precise understanding of host cell invasion mechanisms remains to be elucidated, several bacterial ligands for bacterial adhesion have been identified. Based on the genomic analysis of two O. tsutsugamushi strains, there are more than hundred O. tsutsugamushi-specific genes that are absent from the sequenced genomes of other species belonging to the family Rickettsiaceae (Cho et al., 2010a). Among the O. tsutsugamushi-specific genes, several outer membrane proteins, including those encoded by the tsa56 and autotransporter genes, were shown to be expressed (Cho et al., 2007, 2010a; Nakayama et al., 2008). Previously, we reported that O. tsutsugamushi can bind to host fibronectin and utilize it for adherence or invasion via interactions with the outer membrane protein TSA56 (Lee et al., 2008). In addition, ScaA and ScaC were demonstrated to mediate adherence to mammalian host cells and could be used for diagnosis and applied as potential vaccine antigens. However, the biological role and immunogenicity of other Sca proteins have not been elucidated yet.
In this study, we investigated the role of ScaB during bacterial infection and analyzed the sequence conservation among various strains. Due to the challenges in the genetic manipulation of O. tsutsugamushi, we utilized the surrogate E. coli expression system that has been successfully used to characterize the rOmpB, Sca1, and Sca2 functions of R. conorii (Cardwell and Martinez, 2009; Chan et al., 2009; Riley et al., 2010). Using ScaB-expressing E. coli, we demonstrated that ScaB is sufficient to mediate adherence to and invasion into cultured epithelial and endothelial cells (Figures 3A,B). During the early stages of infection, O. tsutsugamushi exploits integrin-mediated signaling that induces local actin rearrangement at the site of infection (Cho et al., 2010b). It has also been shown that the Sca2 autotransporter protein of Rickettsia conorii mimics eukaryotic formins, generating long and unbranched actin filaments by combining nucleation and progressive barbed-end elongation activities (Cardwell and Martinez, 2012). As indicated by our immunofluorescence microscopy data (Figure 3C), ScaB-expressing E. coli did not induce actin rearrangement at the adhesion site, suggesting that ScaB may simply increase bacterial affinity to host cells. Nevertheless, preincubation of mammalian cells with recombinant ScaB polypeptide reduced O. tsutsugamushi invasion (Figure 5), suggesting that ScaB may function in the initial stage of O. tsutsugamushi adhesion and support the invasion process mediated by other bacterial virulence factors. The mammalian receptor involved in ScaB-mediated adhesion/invasion needs to be identified in future studies.
Here, we also detected the scaB gene in Kuroki and TA686 strains by searching the ScaB protein sequence and confirmed its presence by conventional PCR. The sequence analysis of full-length scab and the genetic variation among the five strains, Boryong, Kuroki, Sido, TA716, and TA686, indicated that the protein encoded by this gene possesses high levels of amino acid sequence variation in the passenger domains, showing variable similarity and identity of 70.6–100.0 and 53.9–99.7%, respectively (Figure 6). Considering the variation in the passenger domains potentially involved in the interaction with the environment, ScaB proteins in different strains may endow differential ability to adhere to and invade host cells. For example, UpaH, a virulence factor of uropathogenic E. coli (UPEC), is an autotransporter protein that mediates adherence to human extracellular matrix protein. Amino acid sequences of UpaH are highly variable among different UPEC strains, and their sequence variation is associated with differences in the abilities of different strains to mediate host adherence and biofilm formation (Allsopp et al., 2012). Therefore, functional differences in ScaB-mediated host cell adhesion and invasion among different strains could be an interesting subject for future studies.
Recently, a survey on sca prevalence was performed on 178 O. tsutsugamushi DNA samples collected from 12 endemic countries from diverse geographical areas; only scaA, scaC, scaD, and scaE were widely detected (93.8–100.0%). In contrast, scaB and scaF were detected less frequently (33.7–43.3%) and in samples from a limited number of countries. Interestingly, most DNA samples from Australia were positive for scaB. Bioinformatic analysis of species of Rickettsia, which forms the sister clade of Orientia, showed that at least 17 autotransporter genes, denoted as surface cell antigen (sca) genes, are present in the bacterial genome, and five of the sca genes, including ompA (sca0), sca1, sca2, sca4, and ompB (sca 5), have evolved under positive selection and are present in the genomes of most rickettsial species (Blanc et al., 2005). Among the autotransporter genes from O. tsutsugamushi, scaA and scaE have evolved under positive selection (Koralur et al., 2018). Except for the Boryong and Ikeda strains, the genome sequences of other O. tsutsugamushi strains in the databases were incomplete and comprised many contigs. Therefore, it is not possible to confirm whether the scaB gene is actually absent from their genomes or is only absent in the available incomplete assemblies. Since a limited number of scaB gene sequences are available, evolutionary pressure on the scaB gene can only be assessed after a sufficient number of sequences are available. Furthermore, the presence of scaB in diverse strains and its correlation with disease severity need to be investigated further.
Currently, the selection of the proper antigens is one of the critical barriers to generating cross-protective immunity against antigenically diverse strains of O. tsutsugamushi (Kelly et al., 2009). Thus far, TSA56 has been the best antigen to provide protective immunity in mouse infection models, but only against homologous strains. Previously, we demonstrated that immunization with combined TSA56 and ScaA autotransporter proteins significantly enhanced protective immunity against heterologous strains (Ha et al., 2015). The potential applicability of the ScaB antigen as a vaccine candidate can be also studied in animal infection models.
To our knowledge, similar to NadA from Neisseria meningitidis (Capecchi et al., 2005) and YadA (Heise and Dersch, 2006) from enteropathogenic Yersinia species, the ScaB protein of O. tsutsugamushi may mediate both adhesion and invasion of host cells and play important roles in bacterial pathogenesis. ScaB-expressing E. coli can adhere to and invade host cells in the absence of other O. tsutsugamushi genes. Moreover, the presence of a recombinant ScaB polypeptide in infection media specifically inhibits the association of O. tsutsugamushi with mammalian host cells. Functional characterization of the scaB gene in various strains and the use of potential vaccine targets with TSA56 should also be performed to facilitate the development of effective vaccines against scrub typhus.
Data Availability Statement
The datasets presented in this study can be found in online repositories. The names of the repository/repositories and accession number(s) can be found in the article/supplementary material.
Ethics Statement
The studies involving human participants were reviewed and approved by Institutional Review Boards of Seoul National University Hospital. The patients/participants provided their written informed consent to participate in this study. The animal study was reviewed and approved by Seoul National University Hospital Institutional.
Author Contributions
YN and CK with the assistance of H-iK performed the majority of the experiments and analyzed the data. KJ generated bioinformatics data. YK, N-YH, and N-HC confirmed the data and wrote the manuscript. YN, CK, and YK contributed equally to this manuscript and should be considered equally contributing first authors. All authors contributed to the article and approved the submitted version.
Funding
This work was supported by a National Research Foundation of Korea (NRF) grant funded by the Korean government (MSIT; no. 2020R1C1C1014224).
Conflict of Interest
The authors declare that the research was conducted in the absence of any commercial or financial relationships that could be construed as a potential conflict of interest.
Footnotes
References
Allsopp, L. P., Beloin, C., Moriel, D. G., Totsika, M., Ghigo, J. M., and Schembri, M. A. (2012). Functional heterogeneity of the UpaH autotransporter protein from uropathogenic Escherichia coli. J. Bacteriol. 194, 5769–5782. doi: 10.1128/JB.01264-12
Blanc, G., Ngwamidiba, M., Ogata, H., Fournier, P. E., Claverie, J. M., and Raoult, D. (2005). Molecular evolution of rickettsia surface antigens: evidence of positive selection. Mol. Biol. Evol. 22, 2073–2083. doi: 10.1093/molbev/msi199
Buchan, D. W. A., and Jones, D. T. (2019). The PSIPRED protein analysis workbench: 20 years on. Nucleic Acids Res. 47, W402–W407. doi: 10.1093/nar/gkz297
Buchan, D. W. A., Minneci, F., Nugent, T. C. O., Bryson, K., and Jones, D. T. (2013). Scalable web services for the PSIPRED protein analysis workbench. Nucleic Acids Res. 41, W349–W357. doi: 10.1093/nar/gkt381
Campanella, J. J., Bitincka, L., and Smalley, J. (2003). MatGAT: an application that generates similarity/identity matrices using protein or DNA sequences. BMC Bioinformatics 4:29. doi: 10.1186/1471-2105-4-29
Capecchi, B., Adu-Bobie, J., Di Marcello, F., Ciucchi, L., Masignani, V., Taddei, A., et al. (2005). Neisseria meningitidis NadA is a new invasin which promotes bacterial adhesion to and penetration into human epithelial cells. Mol. Microbiol. 55, 687–698. doi: 10.1111/j.1365-2958.2004.04423.x
Cardwell, M. M., and Martinez, J. J. (2009). The Sca2 autotransporter protein from Rickettsia conorii is sufficient to mediate adherence to and invasion of cultured mammalian cells. Infect. Immun. 77, 5272–5280. doi: 10.1128/IAI.00201-09
Cardwell, M. M., and Martinez, J. J. (2012). Identification and characterization of the mammalian association and actin-nucleating domains in the Rickettsia conorii autotransporter protein, Sca2. Cell. Microbiol. 14, 1485–1495. doi: 10.1111/j.1462-5822.2012.01815.x
Chan, Y. G., Cardwell, M. M., Hermanas, T. M., Uchiyama, T., and Martinez, J. J. (2009). Rickettsial outer-membrane protein B (rOmpB) mediates bacterial invasion through Ku70 in an actin, c-Cbl, clathrin and caveolin 2-dependent manner. Cell. Microbiol. 11, 629–644. doi: 10.1111/j.1462-5822.2008.01279.x
Chattopadhyay, S., and Richards, A. L. (2007). Scrub typhus vaccines: past history and recent developments. Hum. Vaccin. 3, 73–80. doi: 10.4161/hv.3.3.4009
Cho, B. A., Cho, N. H., Min, C. K., Kim, S. Y., Yang, J. S., Lee, J. R., et al. (2010a). Global gene expression profile of Orientia tsutsugamushi. Proteomics 10, 1699–1715. doi: 10.1002/pmic.200900633
Cho, B. A., Cho, N. H., Seong, S. Y., Choi, M. S., and Kim, I. S. (2010b). Intracellular invasion by Orientia tsutsugamushi is mediated by integrin signaling and actin cytoskeleton rearrangements. Infect. Immun. 78, 1915–1923. doi: 10.1128/IAI.01316-09
Cho, N. H., Kim, H. R., Lee, J. H., Kim, S. Y., Kim, J., Cha, S., et al. (2007). The Orientia tsutsugamushi genome reveals massive proliferation of conjugative type IV secretion system and host-cell interaction genes. Proc. Natl. Acad. Sci. U. S. A. 104, 7981–7986. doi: 10.1073/pnas.0611553104
Cosson, J. F., Galan, M., Bard, E., Razzauti, M., Bernard, M., Morand, S., et al. (2015). Detection of Orientia sp. DNA in rodents from Asia, West Africa and Europe. Parasit. Vectors 8:172. doi: 10.1186/s13071-015-0784-7
Ghorbani, R. P., Ghorbani, A. J., Jain, M. K., and Walker, D. H. (1997). A case of scrub typhus probably acquired in Africa. Clin. Infect. Dis. 25, 1473–1474. doi: 10.1086/516990
Ha, N. Y., Cho, N. H., Kim, Y. S., Choi, M. S., and Kim, I. S. (2011). An autotransporter protein from Orientia tsutsugamushi mediates adherence to nonphagocytic host cells. Infect. Immun. 79, 1718–1727. doi: 10.1128/IAI.01239-10
Ha, N. Y., Choi, M. S., and Cho, N. H. (2013). Molecular characterization of sca genes found in Orientia tsutsugamushi genome. J. Bacteriol. Virol. 43, 152–158. doi: 10.4167/jbv.2013.43.2.155
Ha, N. Y., Kim, Y., Choi, J. H., Choi, M. S., Kim, I. S., Kim, Y. S., et al. (2012). Detection of antibodies against Orientia tsutsugamushi Sca proteins in scrub typhus patients and genetic variation of sca genes of different strains. Clin. Vaccine Immunol. 19, 1442–1451. doi: 10.1128/CVI.00285-12
Ha, N. Y., Sharma, P., Kim, G., Kim, Y., Min, C. K., Choi, M. S., et al. (2015). Immunization with an autotransporter protein of Orientia tsutsugamushi provides protective immunity against scrub typhus. PLoS Negl. Trop. Dis. 9:e0003585. doi: 10.1371/journal.pntd.0003585
Heise, T., and Dersch, P. (2006). Identification of a domain in Yersinia virulence factor YadA that is crucial for extracellular matrix-specific cell adhesion and uptake. Proc. Natl. Acad. Sci. U. S. A. 103, 3375–3380. doi: 10.1073/pnas.0507749103
Henderson, I. R., and Nataro, J. P. (2001). Virulence functions of autotransporter proteins. Infect. Immun. 69, 1231–1243. doi: 10.1128/IAI.69.3.1231-1243.2001
Izzard, L., Fuller, A., Blacksell, S. D., Paris, D. H., Richards, A. L., Aukkanit, N., et al. (2010). Isolation of a novel Orientia species (O. chuto sp. nov.) from a patient infected in Dubai. J. Clin. Microbiol. 48, 4404–4409. doi: 10.1128/JCM.01526-10
Jiang, J., and Richards, A. L. (2018). Scrub typhus: no longer restricted to the tsutsugamushi triangle. Trop. Med. Infect. Dis. 3:11. doi: 10.3390/tropicalmed3010011
Kala, D., Gupta, S., Nagraik, R., Verma, V., Thakur, A., and Kaushal, A. (2020). Diagnosis of scrub typhus: recent advancements and challenges. 3 Biotech 10:396. doi: 10.1007/s13205-020-02389-w
Kelly, D. J., Foley, D. H., and Richards, A. L. (2015). A spatiotemporal database to track human scrub typhus using the vectormap application. PLoS Negl. Trop. Dis. 9:e0004161. doi: 10.1371/journal.pntd.0004161
Kelly, D. J., Fuerst, P. A., Ching, W. M., and Richards, A. L. (2009). Scrub typhus: the geographic distribution of phenotypic and genotypic variants of Orientia tsutsugamushi. Clin. Infect. Dis. 48(Suppl. 3), S203–S230. doi: 10.1086/596576
Kim, H. I., Ha, N. Y., Kim, G., Min, C. K., Kim, Y., Yen, N. T. H., et al. (2019). Immunization with a recombinant antigen composed of conserved blocks from TSA56 provides broad genotype protection against scrub typhus. Emerg. Microbes Infect. 8, 946–958. doi: 10.1080/22221751.2019.1632676
Kim, G., Ha, N. Y., Min, C. K., Kim, H. I., Yen, N. T., Lee, K. H., et al. (2017). Diversification of Orientia tsutsugamushi genotypes by intragenic recombination and their potential expansion in endemic areas. PLoS Negl. Trop. Dis. 11:e0005408. doi: 10.1371/journal.pntd.0005408
Kolo, A. O., Sibeko-Matjila, K. P., Maina, A. N., Richards, A. L., Knobel, D. L., and Matjila, P. T. (2016). Molecular detection of zoonotic Rickettsiae and Anaplasma spp. in domestic dogs and their ectoparasites in Bushbuckridge, South Africa. Vector Borne Zoonotic Dis. 16, 245–252. doi: 10.1089/vbz.2015.1849
Koralur, M. C., Ramaiah, A., and Dasch, G. A. (2018). Detection and distribution of Sca autotransporter protein antigens in diverse isolates of Orientia tsutsugamushi. PLoS Negl. Trop. Dis. 12:e0006784. doi: 10.1371/journal.pntd.0006784
Lee, J. H., Cho, N. H., Kim, S. Y., Bang, S. Y., Chu, H., Choi, M. S., et al. (2008). Fibronectin facilitates the invasion of Orientia tsutsugamushi into host cells through interaction with a 56-kDa type-specific antigen. J. Infect. Dis. 198, 250–257. doi: 10.1086/589284
Lole, K. S., Bollinger, R. C., Paranjape, R. S., Gadkari, D., Kulkarni, S. S., Novak, N. G., et al. (1999). Full-length human immunodeficiency virus type 1 genomes from subtype C-infected seroconverters in India, with evidence of intersubtype recombination. J. Virol. 73, 152–160. doi: 10.1128/JVI.73.1.152-160.1999
Masakhwe, C., Linsuwanon, P., Kimita, G., Mutai, B., Leepitakrat, S., Yalwala, S., et al. (2018). Identification and characterization of Orientia chuto in trombiculid chigger mites collected from wild rodents in Kenya. J. Clin. Microbiol. 56:e01124–18. doi: 10.1128/JCM.01124-18
Meuskens, I., Saragliadis, A., Leo, J. C., and Linke, D. (2019). Type V secretion systems: an overview of passenger domain functions. Front. Microbiol. 10:1163. doi: 10.3389/fmicb.2019.01163
Nakayama, K., Yamashita, A., Kurokawa, K., Morimoto, T., Ogawa, M., Fukuhara, M., et al. (2008). The whole-genome sequencing of the obligate intracellular bacterium Orientia tsutsugamushi revealed massive gene amplification during reductive genome evolution. DNA Res. 15, 185–199. doi: 10.1093/dnares/dsn011
Nishimura, K., Tajima, N., Yoon, Y. H., Park, S. Y., and Tame, J. R. (2010). Autotransporter passenger proteins: virulence factors with common structural themes. J. Mol. Med. 88, 451–458. doi: 10.1007/s00109-010-0600-y
Pizarro-Cerda, J., and Cossart, P. (2006). Bacterial adhesion and entry into host cells. Cell 124, 715–727. doi: 10.1016/j.cell.2006.02.012
Ponnusamy, L., Willcox, A. C., Roe, R. M., Davidson, S. A., Linsuwanon, P., Schuster, A. L., et al. (2018). Bacterial microbiome of the chigger mite Leptotrombidium imphalum varies by life stage and infection with the scrub typhus pathogen Orientia tsutsugamushi. PLoS One 13:e0208327. doi: 10.1371/journal.pone.0208327
Richards, A. L., and Jiang, J. (2020). Scrub typhus: historic perspective and current status of the worldwide presence of Orientia species. Trop. Med. Infect. Dis. 5:49. doi: 10.3390/tropicalmed5020049
Riley, S. P., Goh, K. C., Hermanas, T. M., Cardwell, M. M., Chan, Y. G., and Martinez, J. J. (2010). The Rickettsia conorii autotransporter protein Sca1 promotes adherence to nonphagocytic mammalian cells. Infect. Immun. 78, 1895–1904. doi: 10.1128/IAI.01165-09
Seong, S. Y., Choi, M. S., and Kim, I. S. (2001). Orientia tsutsugamushi infection: overview and immune responses. Microbes Infect. 3, 11–21. doi: 10.1016/S1286-4579(00)01352-6
Söding, J., Biegert, A., and Lupas, A. N. (2005). The HHpred interactive server for protein homology detection and structure prediction. Nucleic Acids Res. 33, W244–W248. doi: 10.1093/nar/gki408
Tamura, K., Stecher, G., Peterson, D., Filipski, A., and Kumar, S. (2013). MEGA6: molecular evolutionary genetics analysis version 6.0. Mol. Biol. Evol. 30, 2725–2729. doi: 10.1093/molbev/mst197
Taylor, A. J., Paris, D. H., and Newton, P. N. (2015). A systematic review of mortality from untreated scrub typhus (Orientia tsutsugamushi). PLoS Negl. Trop. Dis. 9:e0003971. doi: 10.1371/journal.pntd.0003971
Valbuena, G., and Walker, D. H. (2012). Approaches to vaccines against Orientia tsutsugamushi. Front. Cell. Infect. Microbiol. 2:170. doi: 10.3389/fcimb.2012.00170
Weitzel, T. S., Dittrich, J., Lopez, W., Phuklia, C., Martinez-Valdebenito, K., Velasquez, S. D., et al. (2016). Endemic scrub typhus in South America. N. Engl. J. Med. 375, 954–961. doi: 10.1056/NEJMoa1603657
Keywords: scrub typhus, autotransporter, adhesion, invasion, vaccine
Citation: Nguyen YTH, Kim C, Kim Y, Jeon K, Kim H-i, Ha N-Y and Cho N-H (2021) The Orientia tsutsugamushi ScaB Autotransporter Protein Is Required for Adhesion and Invasion of Mammalian Cells. Front. Microbiol. 12:626298. doi: 10.3389/fmicb.2021.626298
Edited by:
Lígia M. Saraiva, New University of Lisbon, PortugalReviewed by:
Dirk Linke, University of Oslo, NorwayJack Christopher Leo, Nottingham Trent University, United Kingdom
Copyright © 2021 Nguyen, Kim, Kim, Jeon, Kim, Ha and Cho. This is an open-access article distributed under the terms of the Creative Commons Attribution License (CC BY). The use, distribution or reproduction in other forums is permitted, provided the original author(s) and the copyright owner(s) are credited and that the original publication in this journal is cited, in accordance with accepted academic practice. No use, distribution or reproduction is permitted which does not comply with these terms.
*Correspondence: Na-Young Ha, nyha6803@snu.ac.kr; Nam-Hyuk Cho, chonh@snu.ac.kr
†These authors have contributed equally to this work and share first authorship