- 1Instituto de Medicina Preventiva Veterinaria, Facultad de Ciencias Veterinarias, Universidad Austral de Chile, Valdivia, Chile
- 2Escuela de Graduados, Facultad de Ciencias Veterinarias, Universidad Austral de Chile, Valdivia, Chile
- 3Instituto de Ciencias Biomédicas, Facultad de Ciencias de la Salud, Universidad Autónoma de Chile, Santiago, Chile
- 4Department of Animal Pathology, Instituto Universitario de Investigación Mixto Agroalimentario de Aragón (IA2), Universidad de Zaragoza, Zaragoza, Spain
- 5Sección Microbiología de Alimentos, Instituto de Salud Pública de Chile, Santiago, Chile
Vibrio parahaemolyticus is the leading cause of seafood-associated bacterial gastroenteritis worldwide. Although different studies have focused on its pattern of variation over time, knowledge about the environmental factors driving the dynamics of this pathogen, within the Chilean territory, is still lacking. This study determined the prevalence of total and pathogenic V. parahaemolyticus strains (tdh and/or trh genes) in mussels (Mytilus chilensis) collected from two natural growing areas between 2017 and 2018, using selective agar and PCR analysis. V. parahaemolyticus was detected in 45.6% (93/204) of pooled samples from the Valdivia River Estuary. The pathogenic strains carrying the tdh and/or trh gene were detected in 11.8% (24/204): tdh in 9.8% (20/204), trh in 0.5% (1/204), and 1.5% (3/204) presented both genes. In Reloncaví Fjord, V. parahaemolyticus was detected in 14.4% (30/209) of the samples, pathogenic V. parahaemolyticus carrying the trh gene was detected in 0.5% (1/209) of the samples, while the tdh gene was not detected in the samples from this area. The total count of mauve-purple colonies typical of V. parahaemolyticus on CHROMagar was positively associated by multivariate analysis with area, water temperature, and salinity. Similarly, V. parahaemolyticus detection rates by PCR had a positive correlation with the area and water temperature. The chances of detecting total V. parahaemolyticus in the Valdivia River Estuary are significantly higher than in the Reloncaví Fjord, but inversely, during spring-summer months, the interaction factor between the area and temperature indicated that the chances of detecting V. parahaemolyticus are higher in the Reloncaví Fjord. Interestingly, this period coincides with the season when commercial and natural-growing shellfish are harvested. On the other hand, pathogenic V. parahaemolyticus tdh+ was significantly correlated with an increase of water temperature. These environmental parameters could be used to trigger a warning on potential hazard, which would influence human health and economic losses in aquaculture systems.
Introduction
Vibrio parahaemolyticus is a Gram-negative halophilic bacterium, ubiquitous in marine and estuarine environments (Joseph et al., 1982; Su and Liu, 2007; Raszl et al., 2016). Gastroenteritis caused by V. parahaemolyticus has been reported worldwide. This bacterium is the principal foodborne pathogen associated with the consumption of raw or undercooked seafood in Asia and the United States (Martinez-Urtaza et al., 2004; Su and Liu, 2007; Newton et al., 2012; Wu et al., 2014). In Chile, V. parahaemolyticus is considered an important human pathogen, which in 1997 was associated with a large outbreak in Antofagasta, northern Chile, causing more than 300 cases. It was also responsible for several outbreaks between 2004 and 2010 in Puerto Montt, southern Chile, with more than 5,000 cases reported. This region is one of the main shellfish-producing areas, mainly of Mytilus chilensis (González-Escalona et al., 2005; Fuenzalida et al., 2006; García et al., 2013).
Particularly, pathogenic V. parahaemolyticus isolates capable of inducing gastroenteritis are rare in environmental samples (2–3%) (Nair et al., 2007; Blanco-Abad et al., 2009; Terzi and Martinez-Urtaza, 2016) and are often undetected (Takeda, 1982; Shirai et al., 1990; Honda and Iida, 1993). Furthermore, the thermostable direct hemolysin (tdh) and tdh-related hemolysin (trh) genes are virulence factors commonly associated with pathogenic strains (Broberg et al., 2011; Zhang and Orth, 2013; Raghunath, 2015). However, little is known of their variation pattern over time, as well as environmental factors affecting such variation under Chilean conditions.
The objective of this work is to perform a longitudinal field study to assess temporal and spatial distributions of total V. parahaemolyticus and potential pathogenic variants (tdh+ and/or trh+) associated with M. chilensis and, in addition, to address a knowledge gap on the prevalence of V. parahaemolyticus strains carrying tdh and/or trh in natural mussels grown in southern Chile, an area with a strong producer and tourist component. Moreover, this study will also evaluate the association between V. parahaemolyticus and environmental parameters, such as temperature and salinity. The information generated by the study will provide new knowledge of the distribution and ecology of V. parahaemolyticus across Chile’s southern seawaters. Additionally, the government could use it to manage shellfish harvesting within safe limits and with appropriate post-harvest measures to control and prevent problems for the seafood industry and consumers.
Materials and Methods
Sampling Sites and Samples Collection
A longitudinal study was realized between January 2017 and May 2018 from two natural extraction areas of mussels (Mytilus chilensis) in the southeastern Pacific coast of Chile (Valdivia River Estuary in the Los Ríos Region and the Reloncaví Fjord in the Los Lagos Region) (Figure 1). Briefly, the Valdivia River Estuary is considered a local natural extraction zone of mussels with important tourism as it is near Valdivia city. This area receives the influence of fresh water and has a tidal range less than 2 m where mussels are in the subtidal zone; therefore, they have had little exposure to direct solar radiation, which implies minor internal temperature variations. Monthly, 25 samples were collected at each of three points, point 1 (39°52′33″S, 73°23′08″W), point 2 (39°53′39″S, 73°23′10″W), and point 3 (39°52′10″S, 73°21′19″W).
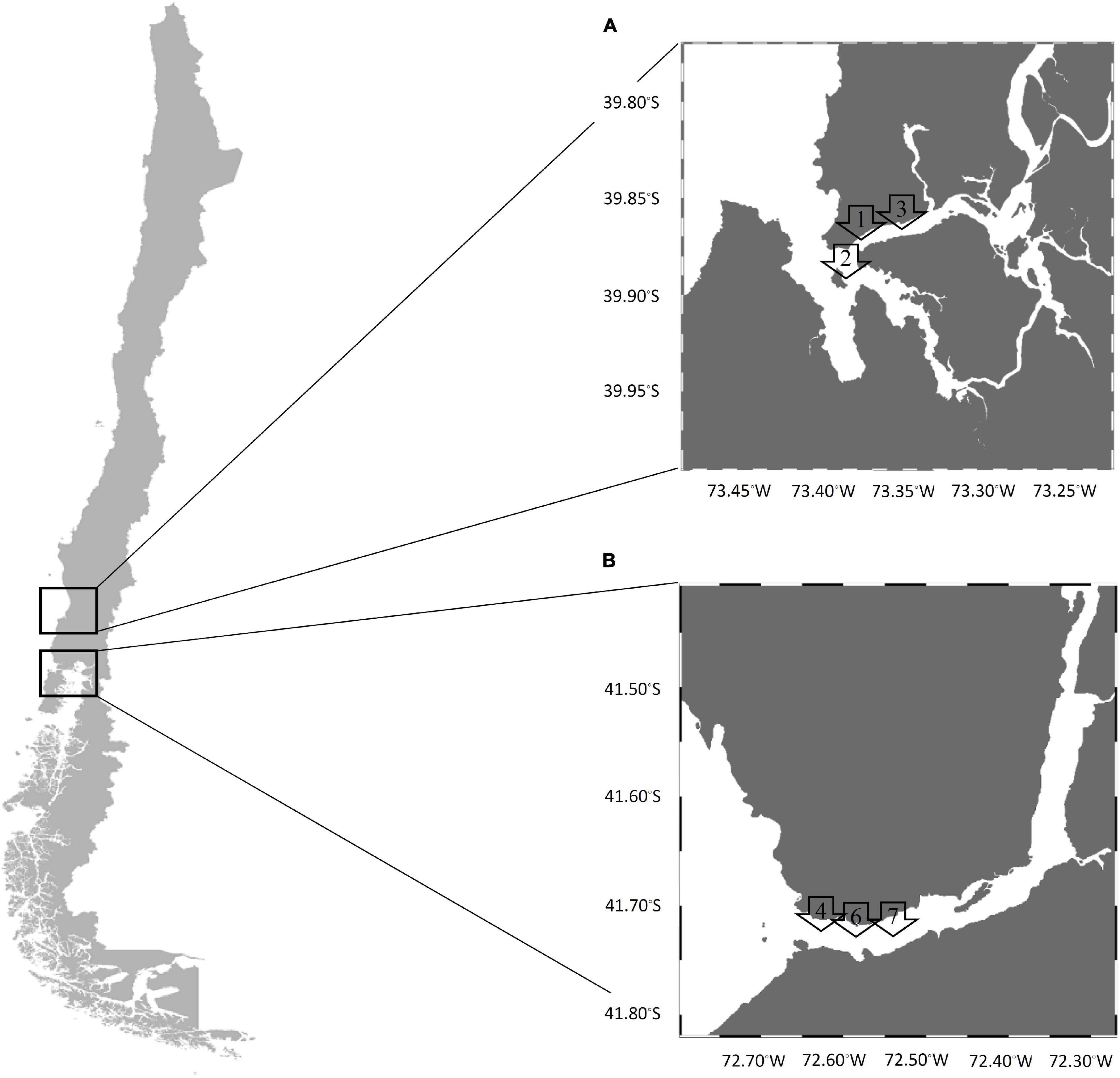
Figure 1. Sampling map in the southern Chile coast (A) Valdivia River Estuary and (B) Reloncaví Fjord. Arrows denote the sites of each sampling area.
The Reloncaví Fjord is one of the principal extraction zones of mussels in Chile. This area is near Puerto Montt city and receives the influence of fresh water from the Petrohué and Puelo rivers, and also the influence of ocean water. The tidal range is between 6 and 7 m, and the mussels are found in the intertidal zone exposed to solar radiation for several hours (especially during sunny days, where mussels can reach internal temperatures around 30°C) and adverse environmental conditions with a lower food supply. Monthly, 25 samples were taken at each of three points, point 4 (41°42′33″S, 72°36′60″W), point 6 (41°42′37″S, 72°36′32″W), and point 7 (41°42′37″S, 72°36′06″W).
In addition, environmental parameters, temperature (°C), and salinity water (ppt) were measured each month (YSI Model 30 Salinity, Conductivity and Temperature System, Xylem INC, United States) at all points, at water depths of 0–2 m (sub-surface zone) and 2–8 m (depth zone), in the Valdivia River Estuary and Reloncaví Fjord (Table 1). However, in the present study, the statistical analyses were performed using parameters from subtidal (depth zone) or intertidal zones (sub-surface zone), depending on mussel habitats. Samples were bagged with ice and transported to the laboratory for immediate analysis.
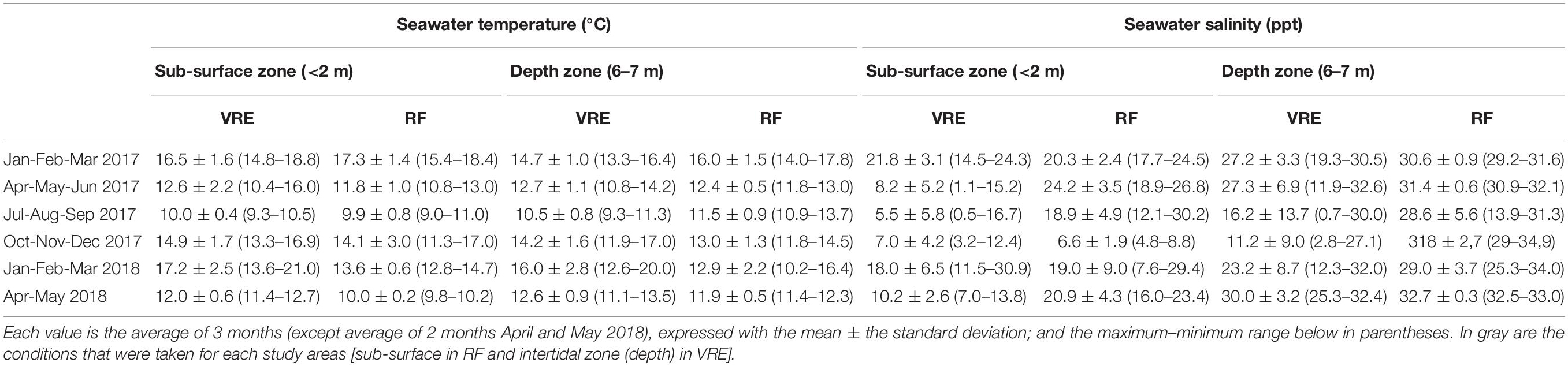
Table 1. Average temperature and salinity seawater conditions recorded during the different weather stations and for each study areas: Valdivia River Estuary (VRE) and Reloncaví Fjord (RF).
Microbiological Analysis
Samples were tested for numbers of total V. parahaemolyticus organisms using specific chromogenic medium for Vibrio growth. Briefly, each mussel was scrubbed with a sterile brush under running tap water to remove any mud. Five pooled samples with five mussels were then formed randomly from each point of the sample, with a total of 15 pooled samples for the area and month. Mussels were opened in sterilized conditions (meat and shell liquid were collected in a sterile laboratory bag) and homogenized (Stomacher® 400 Circulator, Seward Ltd., United Kingdom) at low speed for 1.5 min.
Decimal serial dilutions were prepared in Vibrio phosphate-buffered saline (Vibrio-PBS) composed of 7.650 g NaCl (Merck, Germany), 0.724 g Na2HPO4 (Merck, Germany), and 0.210 g KH2PO4 (Merck, Germany) per liter of distilled water, with the pH adjusted to 7.4 using a 1N NaOH solution according to the expected densities of V. parahaemolyticus in the sample.
An aliquot of 100 μl of each homogenate pool was streaked onto CHROMagar Vibrio plates (CHROMagar Microbiology, France) and incubated at 37°C for 18–24 h. The temperature of incubation was chosen to enumerate only Vibrio capable of growth in human tissues, as the purpose of this study is to determine the relative risks of encountering human pathogens in tissues of consumer food invertebrates. All tests were performed in duplicate. All Vibrio colonies and presumptive colonies of V. parahaemolyticus (the size and mauve-purple color of the colonies are the main differences with respect to other bacterial genera) were counted manually to determine the density of viable cells (CFU ml–1) for each pooled sample (Hara-Kudo et al., 2001; Lopez-Joven et al., 2011). Then, mauve-purple colonies were purified, and each purified isolate was cryopreserved at −80°C for further analysis.
DNA Extraction
Total DNA was extracted from each purified isolate. Briefly, each isolate was grown onto Tryptic Soy Agar (TSA; Merck, Germany) supplemented with 2% NaCl (Merck, Germany), incubated at 35°C for 18 to 24 h. One colony was then transferred into 10 ml of Tryptic Soy Broth (TSB; Merck, Germany) containing 2% NaCl (Merck, Germany) and incubated under the same conditions. Afterward, for DNA extraction, an aliquot of 1 ml was centrifuged at 4000 × g for 5 min. Then, the supernatant was discarded, and the resultant pellet was resuspended in 500 μl lysis buffer with 5 μl of K proteinase and heated at 56°C for 60 min. The suspension was boiled at 100°C for 10 min and immediately placed on ice for 10 min. The lysate was centrifuged at 4000 × g for 5 min, and the supernatant containing the DNA was transferred to a new tube. Afterward, 500 μl of ethanol at 100% was used for precipitating the DNA. The tube was centrifuged at 16.300 × g for 7 min, the supernatant was discarded, then 200 μl of ethanol at 70% was added, and later it was centrifuged under the same conditions. The supernatant was discarded again, and the tube was dried for at least 1 h at room temperature. Finally, nuclease free water (100 μl) was added, and the tube was heated at 100°C for 3 min. DNA was stored at −20°C until its use as a template for PCR analysis to confirm V. parahaemolyticus total [thermolabile hemolysin (tlh) gene] and pathogenic organisms carrying the tdh and/or trh genes.
For positive control, DNeasy® UltraClean® Microbial Kit (QIAGEN, Germany) was used for DNA extraction.
Molecular Analysis and Virulence Genes Detection
The DNA concentration was measured by spectrophotometry and it was adjusted to 40 ng μl–1. V. parahaemolyticus primers encoding the tlh gene were used to confirm species (Taniguchi et al., 1986), and primers for tdh or trh were employed to identify pathogenic strains (Bej et al., 1999). Final PCR amplification was performed in a 25 μl volume consisting of 12.5 μl GoTaq® Green Master Mix (Promega, United States), 1.25 μl forward and reverse primers (IDT, United States), 9 μl nuclease free water, and 1 μl of the DNA template.
Positive and negative controls were prepared for each PCR assay. The reference strain ATCC 17802 (carrying tlh) and pathogen strain ST64 (carrying tdh and trh) were used as positive controls and nuclease free water was used as the negative control. The presence of the tlh, tdh, and trh genes was analyzed according to the PCR cycling conditions described by Bej et al. (1999).
Strains without amplification of gene tlh by PCR were considered negative for V. parahaemolyticus and were not subject to further analysis for tdh and trh genes. Presence of virulence related genes tdh and/or trh was determined using independent PCR assays for each gene. The amplification product was separated by electrophoresis in a 1.5% agarose gel.
Statistical Analysis
A descriptive analysis was used to explore the statistical distribution of colony counts, water temperature (°C), and salinity (ppt) variables. A generalized linear mixed effect modeling (GLMM) approach was used to account for the hierarchical structure of the study design and the correlation present on the data set, due to the longitudinal collection of samples from the same points in each area.
Specifically, two Poisson-based GLMM were used to study the association between environmental factors with the total count of vibrio colonies (model 1) and the total count of mauve-purple colonies in the CHROMagar culture (model 2). Environmental factors (independent variables) such as area, time (sampling month), muscle-weight (gm), water temperature, and salinity were assessed.
Similarly, three logistic-based GLMM were used to assess the relationship between presence/absence of tlh, tdh, and/or trh genes (dependent variables) and the same independent variables mentioned before. Additionally, in all models, the statistical interaction between predictive factors was also carried out. To address the correlation present in the dataset, sampling and month were used as nested random effects. In the case of the logistic-based models, the odd ratios (OR) were computed to estimate the magnitude of the effect of independent variables. The best fitted model was selected based on the computation of the Akaike Information Criteria (AIC), the likelihood ratio test, and the graphical distribution of model residuals. All statistical analyses were performed using the R software version 3.6.1. (R Core Team, 2019), and GLMM was fitted using the lme4 package (Bates et al., 2015) for the R software.
Results
A total of 1,200 mussels, grouped into 240 pools, were analyzed from three points in the Valdivia River Estuary and 1,045 mussels (209 pools) from three points in the Reloncaví Fjord. Due to severe adverse weather conditions, no samples from the Valdivia River Estuary (October 2017), and neither from the Reloncaví Fjord (June 2017, November 2017, and May 2018) were collected. Additionally, a contaminated pool sample from the Reloncaví Fjord was discarded.
Environmental Parameters
Collected samples were divided in two areas and three sites per area. Results showed different patterns in temperature and water salinity through the study. Water temperature ranged from 9.3 to 20.0°C in the Valdivia River Estuary in the depth zone and from 9.0 to 18.4°C in the Reloncaví Fjord in the sub-surface zone. In warmer months (January to March) mean temperature was 14.7 ± 1.0°C in 2017 and 16.0 ± 2.8°C in 2018 at the Valdivia River Estuary, while in the Reloncaví Fjord it was 17.3 ± 1.4°C in 2017 and 13.6 ± 0.6°C in 2018 (Table 1). There was no statistical difference in water temperature between sample sites, but statistical differences were found between sampled months.
Water salinity ranged from 0.7 to 32.6 ppt in the Valdivia River Estuary in the depth zone and from 4.8 to 30.2 ppt in the Reloncaví Fjord in sub-surface zone. Water salinity of the Valdivia River Estuary was significantly higher than the Reloncaví Fjord. The highest salinity mean was recorded from April to June in both areas, the Valdivia River Estuary with 27.3 ± 6.9 ppt in 2017 and 30.0 ± 3.2 ppt in 2018, and the Reloncaví Fjord with 24.2 ± 3.5 ppt in 2017 and 20.9 ± 4.3 ppt in 2018. The lowest salinity mean was recorded from October to December 2017, 11.2 ± 9.0 ppt in the Valdivia River Estuary, while a mean of 6.6 ± 1.9 ppt was recorded in the Reloncaví Fjord. Between the sampling point in each area, point 3 in the Valdivia River Estuary was statistically different from the other two points, with a lower salinity. Specifically, this point presented a salinity mean of 2.4 ± 1.5 ppt from August to December 2017.
Total Vibrios and Presumptive V. parahaemolyticus Abundance and Distribution in CHROMagar Vibrio
There was a seasonal distribution of Vibrio colonies and presumptive colonies of V. parahaemolyticus (indicated by purple-mauve coloration of colonies) incubated at 37°C in CHROMagar Vibrio, to enumerate only Vibrio capable of growth in human tissues. In general, both total vibrios and the presumptive V. parahaemolyticus were more abundant in summer than in winter.
During the 17 months survey, it was revealed that Vibrio species counted in the CHROMagar Vibrio were permanently present in all mussel samples from both areas except, in May 2017 (point 4) and Sept 2017 (points 4 and 6) in the Reloncaví Fjord (Figure 2). The best fitted GLMM for the association between the total count of vibrio colonies in the CHROMagar culture and predictive factors (Table 2) showed a statistical difference in the sites, where the Valdivia River Estuary presented a significant higher total count than the Reloncaví area. When the analysis was stratified by sampling areas, point 1 and point 3 presented a significantly higher total count than other points, indicating statistical differences inside the Valdivia River Estuary (P < 0.001), but not in the Reloncaví Fjord points (P = 0.191). And the total count of vibrio colonies was statistically associated with the water temperature (Table 2). Conversely, other factors such as total weight, muscle weight, salinity, and the interaction between factors were not associated to this response variable.
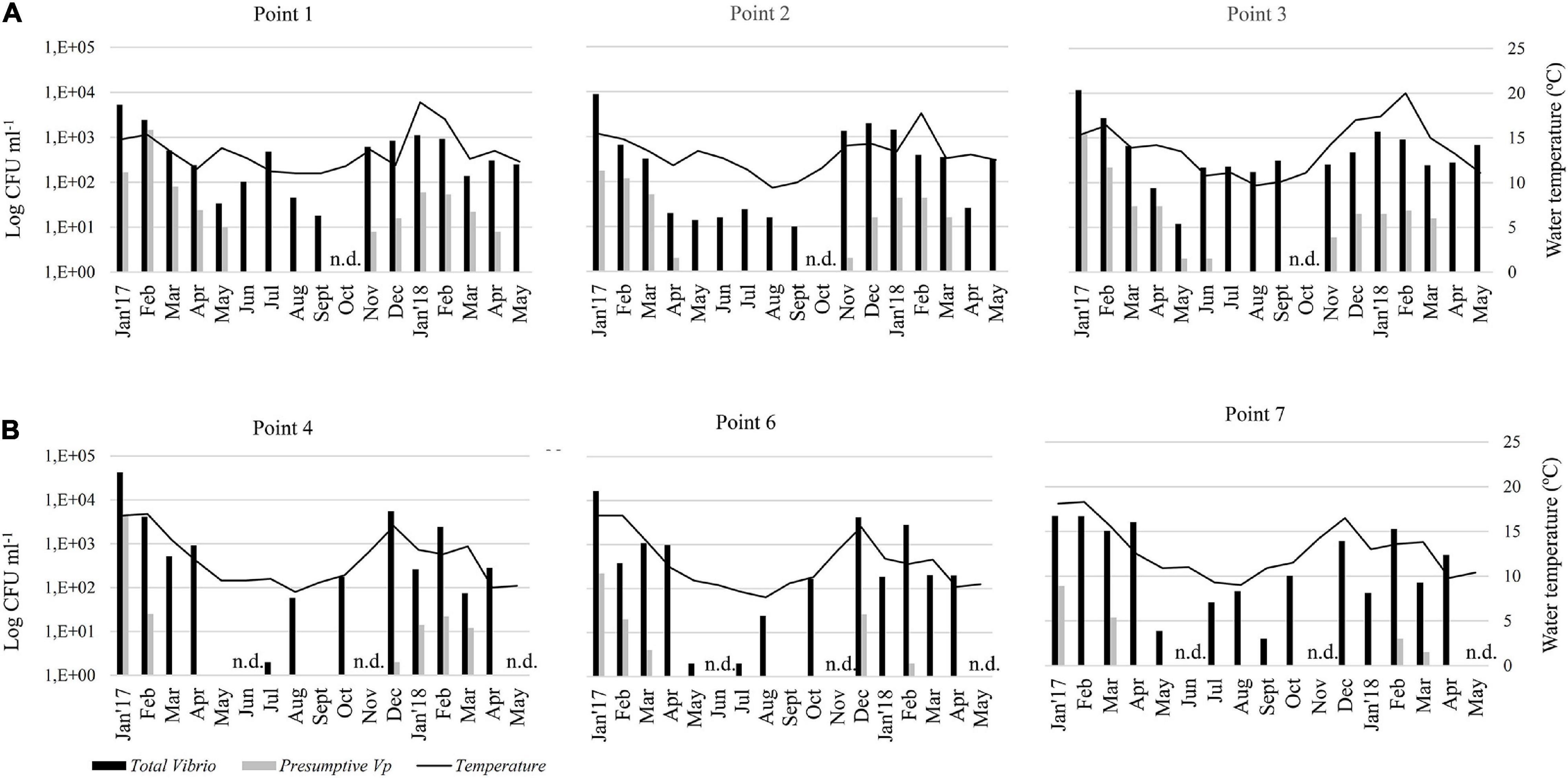
Figure 2. Monthly abundance of total vibrios and presumptive V. parahaemolyticus in the pools of mussels’ samples in CHROMagar medium. Histograms show apparent concentrations (UFC ml–1) based on CHROMagar plates that represents the arithmetic mean of 5 pools of each point. Samples were collected in two areas: (A) Valdivia River Estuary (Points 1, 2, and 3), and (B) Reloncaví Fjord (Points 4, 6, and 7). Seawater temperature (°C) is represented by a black line. n.d., not determined.
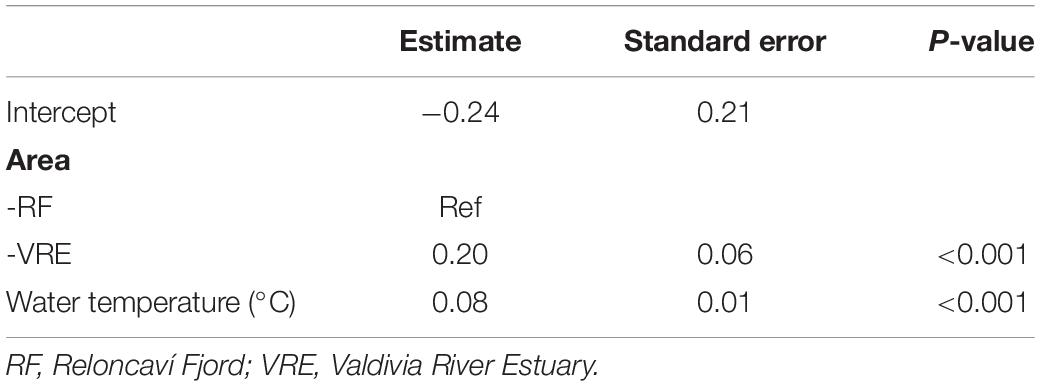
Table 2. Generalized linear mixed effect model results of the association of the total count of vibrio colonies in the CHROMagar culture and predictive factors.
On the other hand, the 17-months survey revealed that presumptive V. parahaemolyticus in CHROMagar Vibrio were absent in almost all mussel samples from June to Sept 2017, and April and May 2018 in the Valdivia River Estuary, and from April to Oct 2017 and also April and May 2018 in the Reloncaví Fjord (Figure 2). The best fitted GLMM for the association between the total count of mauve-purple colonies in the CHROMagar culture and predictive factors (Table 3) showed a similar pattern than the total count of the vibrio colonies model. The Valdivia River Estuary area presented a significantly higher count than the Reloncaví Fjord, and water temperature was positively associated with this response variable. However, salinity was negatively associated with the count of mauve-purple colonies. In addition, when a stratified analysis of the presumptive V. parahaemolyticus by area was performed, statistical differences were observed between points (P < 0.001), where in the Valdivia River Estuary point 1 presented a significantly higher presumptive V. parahaemolyticus count than points 2 and 3. Points 6 and 7 in the Reloncaví Fjord had a significantly lower presumptive V. parahaemolyticus count than point 4.
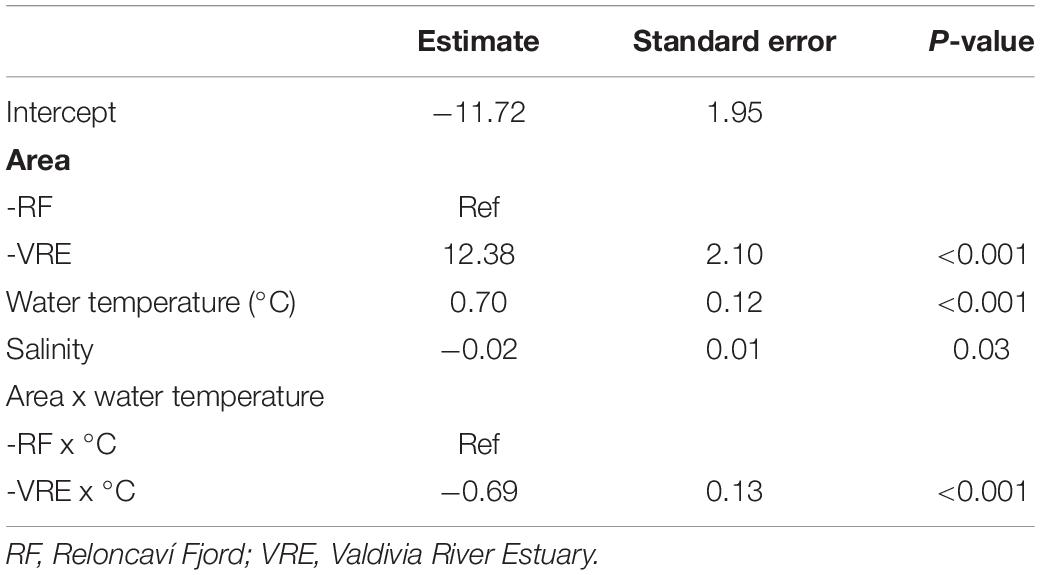
Table 3. Generalized linear mixed effect model results of the association of the total count of mauve-purple colonies in the CHROMagar culture and predictive factors.
Additionally, the interaction between area and temperature was significant, showing that during warm months the area effect is reversed, because the Valdivia River Estuary presented lower counts than the Reloncaví Fjord. This means that a higher count of mauve-purple colonies is expected in the Valdivia River Estuary over the year, but during the summer the risk of finding mauve-purple colonies in mussels increases significantly in the Reloncaví Fjord area. Other factors and interactions assessed were not significant.
Prevalence of Total and Potentially Pathogenic V. parahaemolyticus Abundance and Distribution
A total of 204 pools (from 240 pools grouped to presumptive culture) from the Valdivia River Estuary and 209 from the Reloncaví Fjord (all presumptive culture pools) were assessed. The prevalence of total V. parahaemolyticus by targeting the tlh gene was 45.6% in the Valdivia River Estuary (93/204 pools) and 14.4% in the Reloncaví Fjord (30/209 pooled samples) (Table 4). There were statistical differences between the sampled sites (P < 0.001), but not between the sampled points within each area. A seasonal distribution of V. parahaemolyticus was observed over time, increasing in prevalence during warm months, and decreasing in cold months. Total V. parahaemolyticus was detected from January to June 2017 and from December 2017 to April 2018 in the Valdivia River Estuary (Figure 3A). On the other hand, a lower prevalence was observed in the Reloncaví Fjord, with total V. parahaemolyticus detected from January to February 2017 and from December 2017 to March 2018 (Figure 3B).
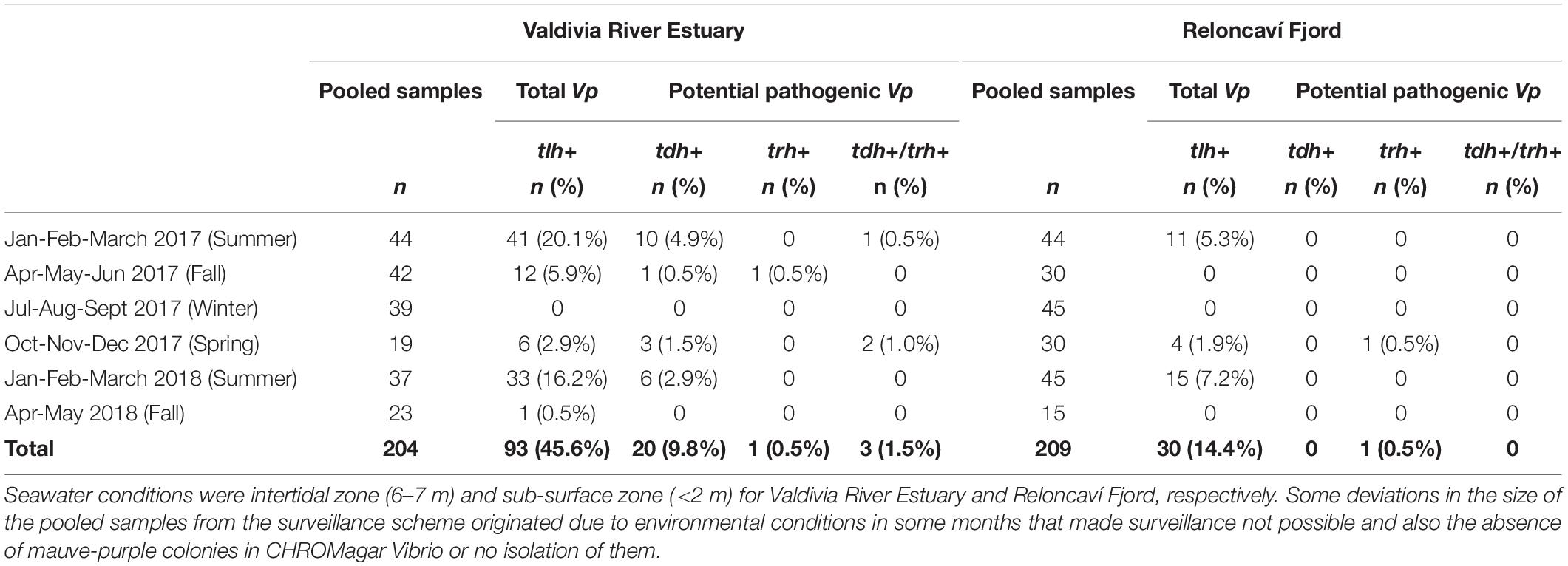
Table 4. Prevalence of total V. parahaemolyticus (tlh+) and potential pathogenic V. parahaemolyticus (tdh+ and/or trh+) in pooled samples of M. chilensis from Valdivia River Estuary and Reloncaví Fjord stratified by weather season (summer, fall, winter and spring).
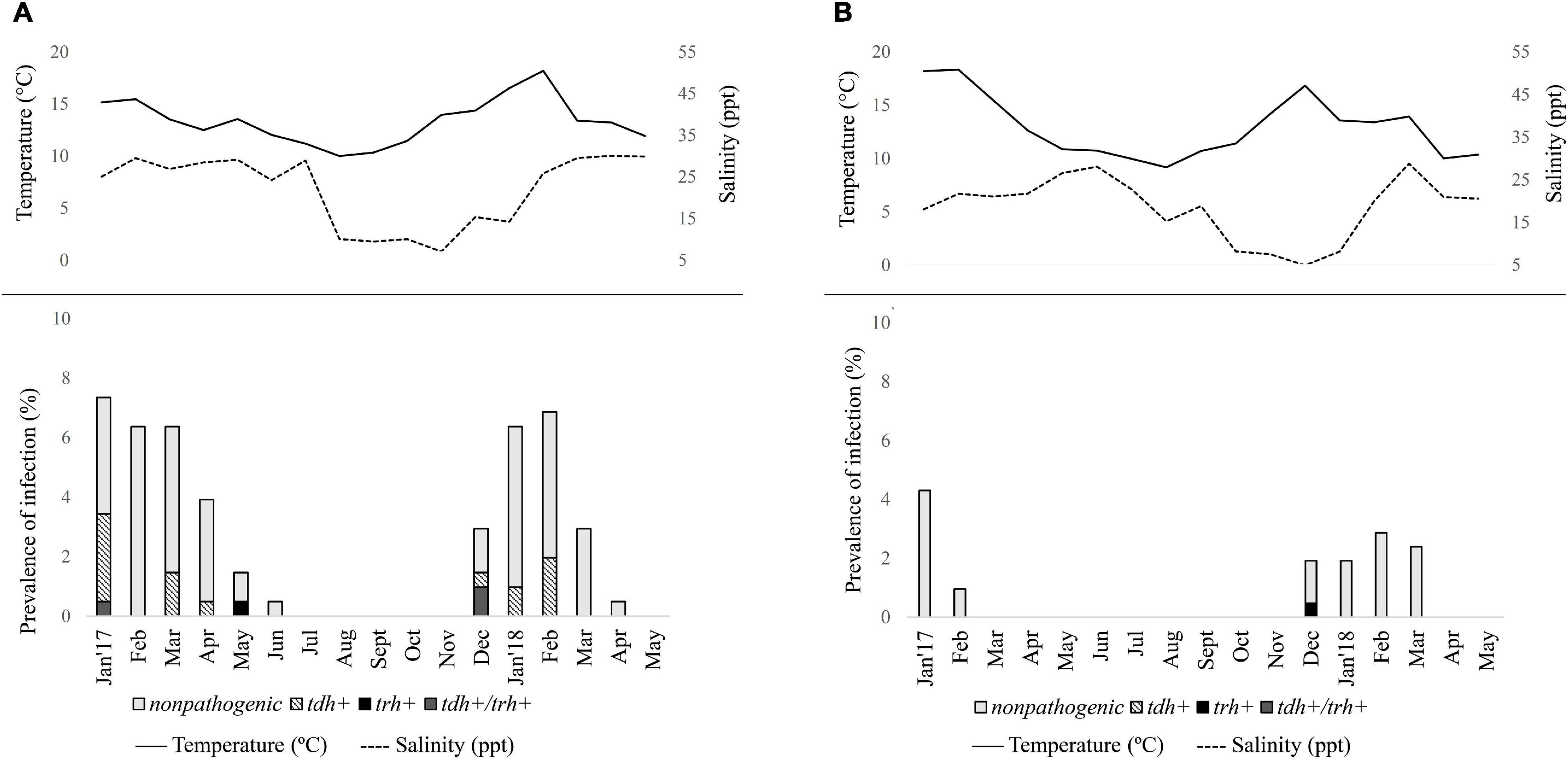
Figure 3. Seasonal distribution of total and pathogenic Vibrio parahaemolyticus in Mytilus chilensis from January 2017 to May 2018 in (A) Valdivia River Estuary and (B) Reloncaví Fjord of southern Chile. Each line or bar represents the arithmetic mean of three points. Solid line represents seawater temperature (°C); dashed line represents salinity (ppt) during each campaign.
Significant differences were observed in the presence of pathogenic variants between areas (P < 0.001). Prevalence of pathogenic V. parahaemolyticus (tdh+ and/or trh+) was 11.8% (24/204) in the Valdivia River Estuary, with the presence of the tdh gene in 9.8% (20/204) of samples, while the trh gene was present in 0.5% (1/204) of samples, and 1.5% (3/204) presented both genes (tdh/trh) (Table 4 and Figure 3A). In the case of the Reloncaví Fjord, prevalence of pathogenic V. parahaemolyticus (tdh+ and/or trh+) was 0.5% (1/209 pools), with the detection of only the trh gene (1/209) in December 2017 (Table 4 and Figure 3B).
Multivariate Analysis to Determine the Influence of Environmental Factors in the Presence of V. parahaemolyticus
A GLMM was used to determine the presence or absence of total V. parahaemolyticus (tlh gene). The best fitted model included area, water temperature, salinity, and pool sample weight (without shell), and also the interaction between area and water temperature. In the case of salinity and muscle-weight, borderline significance was observed (P < 0.10), while the rest of the fixed effect parameters showed P-values lower than 0.001 (Table 5).
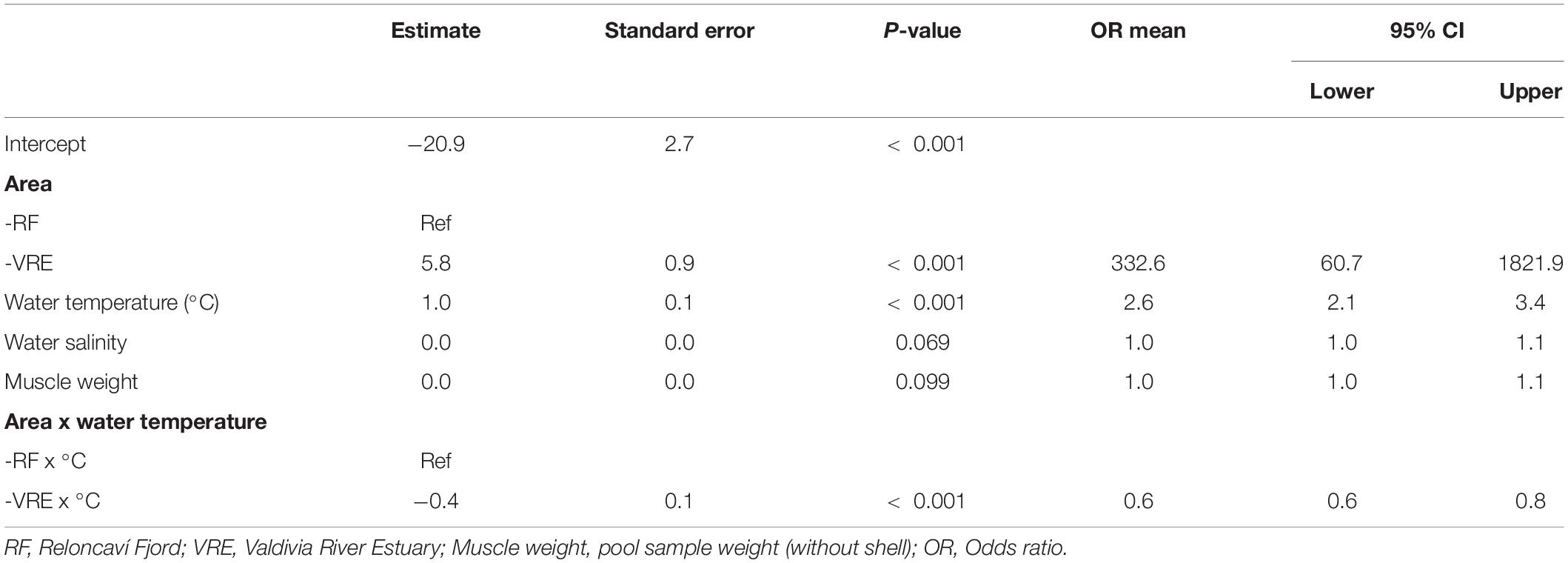
Table 5. Generalized linear mixed effects model results of the association between the presence of total V. parahaemolyticus and predictive factors.
The presence of V. parahaemolyticus (tlh+) was positively associated with the area and water temperature, where the OR value estimated that the chances of finding V. parahaemolyticus in the Valdivia River Estuary were 332.6 times higher than the Reloncaví Fjord, additionally with every increase in the temperature unit (°C), the chances of detecting V. parahaemolyticus raised 2.6 times.
Conversely, the interaction factor between area and water temperature had a negative slope, with an OR equal to 0.60, which indicated that during the warming months the chances of finding V. parahaemolyticus were higher in the Reloncaví Fjord than in the Valdivia River Estuary. This pattern suggests that the latter area presents a higher endemic prevalence than the Reloncaví Fjord. However, the presence of V. parahaemolyticus in the Reloncaví Fjord is more sensitive to changes in temperature.
Although salinity and muscle-weight were also positively associated with the presence of V. parahaemolyticus, the evidence supporting this association is not as robust as with the other predictors (Table 5). Additionally, a GLMM was used to determinate the presence or absence of pathogenic V. parahaemolyticus with independent variables such as area, time (sampling month), muscle-weight (gm), water temperature, and salinity. However, the presence of pathogenic V. parahaemolyticus (tdh gene) was statistically associated only with the water temperature, where the chances of detecting the tdh gene increased 1.56 times for each degree increase in water temperature (P < 0.001). On the other hand, no predictor was associated with the presence of the trh gene.
Discussion
The present study reports on the environmental dynamics of Vibrio species in two natural extraction areas used for mussel harvesting in the southeastern Pacific coast of Chile. Parameters influencing their ecology were identified by monitoring total vibrios and potential pathogenic V. parahaemolyticus for 17 months (2017–2018).
Overall, the annual dynamics of presumptive V. parahaemolyticus (mauve-purple colonies) correlated with temperature and salinity, in contrast with recent findings by Vezzulli et al. (2015) and Lopez-Joven et al. (2018) in Mediterranean lagoons.
Vibrio parahaemolyticus has been the object of laboratory surveillance in Chile, since 2000, because of the outbreaks of foodborne diseases that have occurred in the past years (Heitmann et al., 2005; García et al., 2009; Raszl et al., 2016). However, regulatory limits are not established for V. parahaemolyticus in the internal consumption of seafood in Chile (Raszl et al., 2016).
Numbers of total V. parahaemolyticus organisms increased when seawater temperatures were high (Kaneko and Colwell, 1973; DePaola et al., 1990; Duan and Su, 2005; Parveen et al., 2008; Cruz et al., 2015). A seasonal trend of increasing numbers of V. parahaemolyticus was observed when seawater temperatures increased. The outbreak of foodborne diseases in 2004 and 2005 in Puerto Montt, in the Los Lagos Region, was related to the increase of water temperatures above 16°C in the summer months (González-Escalona et al., 2005; Fuenzalida et al., 2006; García et al., 2013).
Growth rates of pathogenic Vibrio strains (tdh-positive) may be higher than growth rates of confirmed V. parahaemolyticus (tlh-positive), and therefore we may be underestimating tlh-positive cells due to the high incubation temperature (Alarcón Elvira et al., 2020). However, in this study, the incubation temperature to enumerate culturable Vibrio was chosen to select for human pathogens that would optimally grow at 37°C. Surprisingly, the present study showed that during warm months there are increased chances of finding V. parahaemolyticus in the Reloncaví Fjord than in Valdivia River Estuary, supporting the fact that the temperature was an important factor associated with total V. parahaemolyticus. In fact, the two areas have different characteristics that may affect the prevalence of V. parahaemolyticus. Mussels in the Reloncaví Fjord were in the intertidal zone, exposed to solar radiation for several hours (especially during sunny days), and adverse environmental conditions with a lower food supply. During summer, exposed mussels can reach internal temperatures around 30°C which largely favors the V. parahaemolyticus growth (García et al., 2013). On the other hand, surface seawater temperatures are higher in the Valdivia River Estuary (Garcés-Vargas et al., 2013), and mussels are in the subtidal zone thus spending more time underwater to filter bacteria, which favor an endemic prevalence compared with the Reloncaví Fjord. Additionally, subtidal located mussels are less exposed to direct solar radiation, which implies minor internal temperature variations.
To our knowledge the Valdivia River Estuary does not have a prevalence of V. parahaemolyticus in mussels. Prevalence of V. parahaemolyticus carrying the tdh gene in the Valdivia River Estuary agrees with other worldwide studies; DePaola et al. (2003) found that 13% of oysters carry the tdh gene, Duan and Su (2005) found samples with less than 10%, and Lopez-Joven et al. (2015) found 6.7%. It was possible to detect strains of V. parahaemolyticus with both genes (tdh and trh) in environmental samples.
The prevalence of potential pathogenic V. parahaemolyticus (tdh and/or trh) in the Valdivia River Estuary is higher than in the Reloncaví Fjord, and these results coincide with those obtained by Aranda et al. (2015), who did not detect pathogenic V. parahaemolyticus in mussels from the Reloncaví Fjord. We show that the presence of pathogenic V. parahaemolyticus carrying the tdh gene was statistically associated with the water temperature, since the probability to detect the tdh gene increased 1.56 times for each increase in this factor.
However, when the interaction factor between area/time and consequently area/temperature was considered, it was suggested that during the warming months the chances of finding V. parahaemolyticus are higher in the Reloncaví Fjord than in the Valdivia River Estuary, locating Reloncaví Fjord as a high-risk zone of V. parahaemolyticus. Although surface seawater temperatures are higher in the Valdivia River Estuary, it seems that differences are not enough on their own to favor the growth of pathogenic variants, and consequently, the risk could be the same in both locations. This interaction could explain the outbreaks in Puerto Montt, in the Los Lagos Region related to the consumptions of uncooked or raw bivalves, with more than 1,500 and 3,725 cases reported in 2004 and 2005, respectively, both in warm months (González-Escalona et al., 2005; Fuenzalida et al., 2006; García et al., 2013).
The influence of water salinity as an environmental parameter is not as well defined as water temperature. Different studies have report negative, but also positive correlations between salinity and the abundance of potential V. parahaemolyticus. A negative correlation was reported by Lopez-Joven et al. (2015) and Davis et al. (2017). Moreover, a positive correlation was detected by DePaola et al. (2003), Zimmerman et al. (2007), and Martinez-Urtaza et al. (2008). Although water salinity and muscle-weight were positively associated with the presence of V. parahaemolyticus (tlh gene) in our study, it was not statistically associated (P > 0.05) with these parameters. However, in the case of the weight of the meat (without shell), it is necessary to consider that larger mussels have a greater chance of harboring total bacteria, but not pathogenic bacteria, which would indicate that the presence of pathogenic bacteria is something typical of the environment and not of the mollusk.
Nonetheless, several other factors can influence the presence, abundance, and distribution of total and pathogenic V. parahaemolyticus in mussels, such as plankton composition (Turner et al., 2013), dissolve oxygen (Parveen et al., 2008; Davis et al., 2017), particulate organic matter availability (Thickman and Gobler, 2017), and chlorophyll (Zimmerman et al., 2007; Parveen et al., 2008). More studies considering these environmental factors and its interactions must be done.
Conclusion
In conclusion, these results demonstrate that the prevalence of total and pathogenic V. parahaemolyticus have a seasonal distribution over time, increasing in warm months and decreasing in cold months, in both areas of southern Chile. This fluctuation can be explained by the positive association with water temperature. Additionally, pathogenic V. parahaemolyticus strains with tdh or trh and both genes (tdh and trh) were detected in environmental samples. Considering that the Los Lagos Region is one of the main bivalve extraction areas, postharvest handling temperature should be considered, as inadequate temperatures can increase V. parahaemolyticus abundance and potential pathogenic V. parahaemolyticus (tdh and/or trh) in bivalves. These results provide information about the distribution of V. parahaemolyticus and could help to create measures to control future problems related to shellfish harvesting and consumption in the country.
Data Availability Statement
The raw data supporting the conclusions of this article will be made available by the authors, without undue reservation.
Ethics Statement
The animal study was reviewed and approved by the Cómite de Bioética “Uso de animales en la investigación”—Universidad Austral de Chile.
Author Contributions
CL-J conceived the study idea. CL-J, CV, KG, JP-L, and VC designed the sampling collections and experimental procedures. CB and JP-L performed the microbiology and molecular analyses. CL-J, CB, CV, and IB performed the statistical analysis and analyzed the data. All authors wrote, discussed, and approved the final version of this manuscript.
Funding
This work received financial support from ANID/CONICYT (FONDECYT Grant Number 11160642) and from Vicerrectoría de Investigación, Desarrollo y Creación Artística of Universidad Austral de Chile (DID-UACh Grant Number S-2016-42).
Conflict of Interest
The authors declare that the research was conducted in the absence of any commercial or financial relationships that could be construed as a potential conflict of interest.
Acknowledgments
We would like to thank Jose Martel and Juan Hernan for their support during sampling collections in the Valdivia River Estuary and Reloncaví Fjord, respectively. We are also thankful to the Laboratory of Food and Water, Universidad Austral de Chile, for technical assistance. Finally, the authors would like to thank Dr. Angelo DePaola and Beatriz Calzadilla for their contribution in the English editing of the final manuscript.
References
Alarcón Elvira, F., Pardío-Sedas, V. T., Martínez Herrera, D., Quintana Castro, R., Oliart Ros, R. M., López Hernández, K., et al. (2020). Comparative survival and the cold-induced gene expression of pathogenic and nonpathogenic Vibrio parahaemolyticus from tropical eastern oysters during cold storage. Int. J. Environ. Res. Public Health 17:1836. doi: 10.3390/ijerph17061836
Aranda, C. P., Yévenes, M., Rodriguez-Benito, C., Godoy, F. A., Ruiz, M., and Cachicas, V. (2015). Distribution and growth of Vibrio parahaemolyticus in southern chilean clams (Venus antiqua) and blue mussels (Mytilus chilensis). Foodborne Pathog. Dis. 12, 1–7. doi: 10.1089/fpd.2014.1819
Bates, D., Mächler, M., Bolker, B., and Walker, S. (2015). Fitting linear mixed-effects models using lme4. J. Stat. Softw. 67, 1–48. doi: 10.18637/jss.v067.i01
Bej, A. K., Patterson, D. P., Brasher, C. W., Vickery, M. C., Jones, D. D., and Kaysner, C. A. (1999). Detection of total and hemolysin-producing Vibrio parahaemolyticus in shellfish using multiplex PCR amplification of tl, tdh and trh. J. Microbiol. Methods 36, 215–225. doi: 10.1016/S0167-7012(99)00037-8
Blanco-Abad, V., Ansede-Bermejo, J., Rodriguez-Castro, A., and Martinez-Urtaza, J. (2009). Evaluation of different procedures for the optimized detection of Vibrio parahaemolyticus in mussels and environmental samples. Int. J. Food Microbiol. 129, 229–236.
Broberg, C. A., Calder, T. J., and Orth, K. (2011). Vibrio parahaemolyticus cell biology and pathogenicity determinants. Microbes Infect. 13, 992–1001. doi: 10.1016/j.micinf.2011.06.013
Cruz, C. D., Hedderley, D., and Fletcher, G. C. (2015). Long-term study of Vibrio parahaemolyticus prevalence and distribution in New Zealand shellfish. Appl. Environ. Microbiol. 81, 2320–2327. doi: 10.1128/AEM.04020-14
Davis, B. J. K., Jacobs, J. M., Davis, M. F., Schwab, K. J., DePaola, A., and Curriero, F. C. (2017). Environmental determinants of Vibrio parahaemolyticus in the Chesapeake Bay. Appl. Environ. Microbiol. 83, e1147–e1117. doi: 10.1128/AEM.01147-17
DePaola, A., Hopkins, L. H., Peeler, J. T., Wentz, B., and McPhearson, R. M. (1990). Incidence of Vibrio parahaemolyticus in US Coastal waters and oysters. Appl. Environ. Microbiol. 56, 2299–2302. doi: 10.1128/AEM.56.8.2299-2302.1990
DePaola, A., Ulaszek, J., Kaysner, C. A., Tenge, B. J., Nordstrom, J. L., Wells, J., et al. (2003). Molecular, serological, and virulence characteristics of Vibrio parahaemolyticus isolated from environmental, food, and clinical sources in North America and Asia. Appl. Environ. Microbiol. 69, 3999–4005. doi: 10.1128/AEM.69.7.3999-4005.2003
Duan, J., and Su, Y. C. (2005). Occurrence of Vibrio parahaemolyticus in two Oregon oyster-growing Bays. J. Food Sci. 70, M58–M63. doi: 10.1111/j.1365-2621.2005.tb09047.x
Fuenzalida, L., Hernandez, C., Toro, J., Rioseco, M. L., Romero, J., and Espejo, R. T. (2006). Vibrio parahaemolyticus in shellfish and clinical samples during two large epidemics of diarrhoea in southern Chile. Environ. Microbiol. 8, 675–683. doi: 10.1111/j.1462-2920.2005.00946.x
Garcés-Vargas, J., Ruiz, M., Pardo, L. M., Nuñez, S., and Pérez-Santos, I. (2013). Caracterización hidrográfica del estuario del río Valdivia, centro-sur de Chile. Lat. Am. J. Aquat. Res. 41, 113–125.
García, K., Bastías, R., Higuera, G., Torres, R., Mellado, A., Uribe, P., et al. (2013). Rise and fall of pandemic Vibrio parahaemolyticus serotype O3:K6 in southern Chile. Environ. Microbiol. 15, 527–534. doi: 10.1111/j.1462-2920.2012.02883.x
García, K., Torres, R., Uribe, P., Hernández, C., Rioseco, M. L., Romero, J., et al. (2009). Dynamics of clinical and environmental Vibrio parahaemolyticus strains during seafood-related summer diarrhea outbreaks in southern Chile. Appl. Environ. Microbiol. 75, 7482–7487. doi: 10.1128/AEM.01662-09
González-Escalona, N., Cachicas, V., Acevedo, C., Rioseco, M. L., Vergara, J. A., and Cabello, F. (2005). Vibrio parahaemolyticus diarrhea, Chile, 1998 and 2004. Emerg. Infect. Dis. 11, 129–131. doi: 10.3201/eid1101.040762
Hara-Kudo, Y., Nishina, T., Nakagawa, H., Konuma, H., Hasegawa, J., and Kumagai, S. (2001). Improved method for detection of Vibrio parahaemolyticus in seafood. Appl. Environ. Microbiol. 67, 5819–5823. doi: 10.1128/AEM.67.12.5819-5823.2001
Heitmann, I. G., Jofré, L. M., Hormázabal, J. C. O., Olea, A. N., Vallebuona, C. S., and Valdés, C. H. (2005). Revisión y recomendaciones para el manejo de diarrea por Vibrio parahaemolyticus. Rev. Chil. Infect. 22, 131–140. doi: 10.4067/s0716-10182005000200003
Honda, T., and Iida, T. (1993). The pathogenicity of Vibrio parahaemolyticus and the role of the thermostable direct haemolysin and related haemolysins. Rev. Med. Microbiol. 4, 106–113. doi: 10.1097/00013542-199304000-00006
Joseph, S. W., Colwell, R. R., and Kaper, J. B. (1982). Vibrio parahaemolyticus and related halophilic Vibrios. Crit. Rev. Microbiol. 10, 77–124. doi: 10.3109/10408418209113506
Kaneko, T., and Colwell, R. R. (1973). Ecology of Vibrio parahaemolyticus in Chesapeake Bay. J. Bacteriol. 113, 24–32. doi: 10.1128/JB.113.1.24-32.1973
Lopez-Joven, C., de Blas, I., Furones, M. D., and Roque, A. (2015). Prevalences of pathogenic and non-pathogenic Vibrio parahaemolyticus in mollusks from the Spanish Mediterranean Coast. Front. Microbiol. 6:736. doi: 10.3389/fmicb.2015.00736
Lopez-Joven, C., de Blas, I., Ruiz-Zarzuela, I., Furones, M. D., and Roque, A. (2011). Experimental uptake and retention of pathogenic and nonpathogenic Vibrio parahaemolyticus in two species of clams: Ruditapes decussatus and Ruditapes philippinarum. J. Appl. Microbiol. 111, 197–208. doi: 10.1111/j.1365-2672.2011.05024.x
Lopez-Joven, C., Rolland, J.-L., Haffner, P., Caro, A., Roques, C., Carré, C., et al. (2018). Oyster farming, temperature, and plankton influence the dynamics of pathogenic Vibrios in the Thau Lagoon. Front. Microbiol. 9:2530. doi: 10.3389/fmicb.2018.02530
Martinez-Urtaza, J., Lozano-Leon, A., DePaola, A., Ishibashi, M., Shimada, K., Nishibuchi, M., et al. (2004). Characterization of pathogenic Vibrio parahaemolyticus isolates from clinical sources in Spain and comparison with Asian and North American pandemic isolates. J. Clin. Microbiol. 42, 4672–4678. doi: 10.1128/JCM.42.10.4672-4678.2004
Martinez-Urtaza, J., Lozano-Leon, A., Varela-Pet, J., Trinanes, J., Pazos, Y., and Garcia-Martin, O. (2008). Environmental determinants of the occurrence and distribution of Vibrio parahaemolyticus in the Rias of Galicia, Spain. Appl. Environ. Microbiol. 74, 265–274. doi: 10.1128/AEM.01307-07
Nair, G. B., Ramamurthy, T., Bhattacharya, S. K., Dutta, B., Takeda, Y., and Sack, D. A. (2007). Global dissemination of Vibrio parahaemolyticus serotype O3:K6 and its serovariants. Clin. Microbiol. Rev. 20, 39–48. doi: 10.1128/CMR.00025-06
Newton, A., Kendall, M., Vugia, D. J., Henao, O. L., and Mahon, B. E. (2012). Increasing rates of vibriosis in the United States, 1996–2010: review of surveillance data from 2 systems. Clin. Infect. Dis. 54, S391–S395. doi: 10.1093/cid/cis243
Parveen, S., Hettiarachchi, K. A., Browers, J. C., Jones, J. L., Tamplin, M. L., and Mckay, R. (2008). Seasonal distribution of total and pathogenic Vibrio parahaemolyticus in Chesapeake Bay oyster and waters. Int. J. Food Microbiol. 128, 354–361. doi: 10.1016/j.ijfoodmicro.2008.09.019
Raghunath, P. (2015). Roles of thermostable direct hemolysin (TDH) and TDH-related hemolysin (TRH) in Vibrio parahaemolyticus. Front. Microbiol. 5:805. doi: 10.3389/fmicb.2014.00805
Raszl, S. M., Froelich, B. A., Vieira, C. R. W., Blackwood, A. D., and Noble, R. T. (2016). Vibrio parahaemolyticus and Vibrio vulnificus in South America: water, seafood and human infections. J. Appl. Microbiol 121, 1201–1222. doi: 10.1111/jam.13246
Shirai, H., Ito, H., Hirayama, T., Nakamoto, Y., Nakabayashi, N., Kumagai, K., et al. (1990). Molecular epidemiologic evidence for association of thermostable direct hemolysin (TDH) and TDH-related hemolysin of Vibrio parahaemolyticus with gastroenteritis. Infect. Immun. 58, 3568–3573. doi: 10.1128/IAI.58.11.3568-3573.1990
Su, Y. C., and Liu, C. (2007). Vibrio parahaemolyticus: a concern of seafood safety. Food Microbiol. 24, 549–558. doi: 10.1016/j.fm.2007.01.005
Takeda, Y. (1982). Thermostable direct hemolysin of Vibrio parahaemolyticus. Pharmacol. Ther. 19, 123–146. doi: 10.1016/0163-7258(82)90044-4
Taniguchi, H., Hirano, H., Kubomura, S., Higashi, K., and Mizuguchi, Y. (1986). Comparison of the nucleotide sequences of the genes for the thermolabile hemolysin from Vibrio parahaemolyticus. Microb. Pathog. 1, 425–432. doi: 10.1016/0882-4010(86)90004-5
Terzi, G. G., and Martinez-Urtaza, J. (2016). Molecular characterizations of Vibrio parahaemolyticus in seafood from the Black Sea, Turkey. Lett. Appl. Microbiol. 62, 494–500. doi: 10.1111/lam.12579
Thickman, J. D., and Gobler, C. J. (2017). The ability of algal organic matter and surface runoff to promote the abundance of pathogenic and non-pathogenic strains of Vibrio parahaemolyticus in Long Island Sound, USA. PLoS One 12:e0185994. doi: 10.1371/journal.pone.0185994
Turner, J. W., Malayil, L., Guadagnoli, D., Cole, D., and Lipp, E. K. (2013). Detection of Vibrio parahaemolyticus, Vibrio vulnificus and Vibrio cholerae with respect to seasonal fluctuations in temperature and plankton abundance. Environ. Microbiol. 16, 1019–1028. doi: 10.1111/1462-2920.12246
Vezzulli, L., Pezzati, E., Stauder, M., Stagnaro, L., Venier, P., and Pruzzo, C. (2015). Aquatic ecology of the oyster pathogens Vibrio splendidus and Vibrio aestuarianus. Environ. Microbiol. 17, 1065–1080. doi: 10.1111/1462-2920.12484
Wu, Y., Wen, J., Ma, Y., Ma, X., and Chen, Y. (2014). Epidemiology of foodborne disease outbreaks caused by Vibrio parahaemolyticus, China, 2003–2008. Food Control 46, 197–202. doi: 10.1016/j.foodcont.2014.05.023
Zhang, L., and Orth, K. (2013). Virulence determinants for Vibrio parahaemolyticus infection. Curr. Opin. Microbiol. 16, 70–77. doi: 10.1016/j.mib.2013.02.002
Keywords: prevalence, Vibrio parahaemolyticus, tdh, trh, virulence genes, salinity, water temperature, mussels
Citation: Bacian C, Verdugo C, García K, Perez-Larruscain J, de Blas I, Cachicas V and Lopez-Joven C (2021) Longitudinal Study of Total and Pathogenic Vibrio parahaemolyticus (tdh+ and/or trh+) in Two Natural Extraction Areas of Mytilus chilensis in Southern Chile. Front. Microbiol. 12:621737. doi: 10.3389/fmicb.2021.621737
Received: 26 October 2020; Accepted: 22 February 2021;
Published: 18 March 2021.
Edited by:
Wade H. Jeffrey, University of West Florida, United StatesReviewed by:
Lisa Anne Waidner, University of West Florida, United StatesLigia Virginia Da Silva, University of Maryland Eastern Shore, United States
Copyright © 2021 Bacian, Verdugo, García, Perez-Larruscain, de Blas, Cachicas and Lopez-Joven. This is an open-access article distributed under the terms of the Creative Commons Attribution License (CC BY). The use, distribution or reproduction in other forums is permitted, provided the original author(s) and the copyright owner(s) are credited and that the original publication in this journal is cited, in accordance with accepted academic practice. No use, distribution or reproduction is permitted which does not comply with these terms.
*Correspondence: Carmen Lopez-Joven, carmen.lopez@uach.cl; cljoven@gmail.com