- 1Dpto. de Microbiología y Parasitología, Instituto de Salud Tropical (ISTUN), Instituto de Investigación Sanitaria de Navarra, Universidad de Navarra, Pamplona, Spain
- 2Unidad de Producción y Sanidad Animal, Centro de Investigación y Tecnología Agroalimentaria de Aragón, Zaragoza, Spain
- 3Instituto Agroalimentario de Aragón-IA2, CITA-Universidad de Zaragoza, Zaragoza, Spain
- 4Centro de Ciencias Genómicas, Universidad Nacional Autónoma de México, Cuernavaca, Mexico
The brucellae are facultative intracellular bacteria with a cell envelope rich in phosphatidylcholine (PC). PC is abundant in eukaryotes but rare in prokaryotes, and it has been proposed that Brucella uses PC to mimic eukaryotic-like features and avoid innate immune responses in the host. Two PC synthesis pathways are known in prokaryotes: the PmtA-catalyzed trimethylation of phosphatidylethanolamine and the direct linkage of choline to CDP-diacylglycerol catalyzed by the PC synthase Pcs. Previous studies have reported that B. abortus and B. melitensis possess non-functional PmtAs and that PC is synthesized exclusively via Pcs in these strains. A putative choline transporter ChoXWV has also been linked to PC synthesis in B. abortus. Here, we report that Pcs and Pmt pathways are active in B. suis biovar 2 and that a bioinformatics analysis of Brucella genomes suggests that PmtA is only inactivated in B. abortus and B. melitensis strains. We also show that ChoXWV is active in B. suis biovar 2 and conserved in all brucellae except B. canis and B. inopinata. Unexpectedly, the experimentally verified ChoXWV dysfunction in B. canis did not abrogate PC synthesis in a PmtA-deficient mutant, which suggests the presence of an unknown mechanism for obtaining choline for the Pcs pathway in Brucella. We also found that ChoXWV dysfunction did not cause attenuation in B. suis biovar 2. The results of these studies are discussed with respect to the proposed role of PC in Brucella virulence and how differential use of the Pmt and Pcs pathways may influence the interactions of these bacteria with their mammalian hosts.
Introduction
Brucellosis is a worldwide extended zoonosis caused by bacteria of the genus Brucella, a group of facultative intracellular pathogens belonging to the α-2 class of Proteobacteria. B. melitensis, B. abortus, and B. suis are the best-known Brucella species and they were divided long ago into biovars according to phenotypic criteria (Alton et al., 1988; Moreno, 2014). The B. abortus and B. melitensis biovars are genetically and phenotypically homogeneous and they predominantly infect cattle and small ruminants, respectively. The B. suis biovars, in contrast, exhibit greater phylogenomic diversity and broader host ranges (Moreno et al., 2002; Scholz et al., 2008; Whatmore, 2009; Al Dahouk et al., 2012). B. suis biovars 1 and 3, for instance, are endemic in America and Asia where they cause serious reproductive problems in pigs and severe disease in humans (EFSA, 2009). B. suis biovar 2 poses an important problem for pig farming in Europe (EFSA, 2009) where wild boars and hares act as reservoirs (Garin-Bastuji et al., 2000, 2006; Muñoz et al., 2010). B. suis biovar 4 infects reindeer in Eurasia and Alaska, and B. suis biovar 5 has been isolated from wild rodents in Transcaucasia (Zheludkov and Tsirelson, 2010). Other brucellae infect marine mammals (B. pinnipedialis and B. ceti), the American wood rat (B. neotomae), the European common vole (B. microti), domestic dogs (B. canis), and sheep (B. ovis) (Zheludkov and Tsirelson, 2010); in the case of B. neotomae and B. canis, human infections have also been reported (Suárez-Esquivel et al., 2017; Moreno, 2021). B. inopinata, B. vulpis, and B. papionis are species proposed for a few isolates respectively obtained from a breast implant (one strain), fox mandibular lymph nodes (2 strains), and abortion-related materials in captive baboons (two strains) (Scholz et al., 2010, 2016; Whatmore et al., 2014). All these species except B. inopinata form a core group separated from early diverging clades closer to other α-2 Proteobacteria. Early diverging brucellae include B. inopinata and unnamed Brucella isolates from Australian rodents and frogs (Wattam et al., 2014; Soler-Lloréns et al., 2016; Al Dahouk et al., 2017).
Investigations carried out mostly with strains representative of biovars 1 of B. melitensis, B. abortus, and B. suis show that their pathogenicity is largely linked to deficient detection by the host innate immune system during the early stages of infection. The subsequent delay in induction of the inflammatory response allows these bacteria to reach safe intracellular niches where they multiply and establish long-lasting infections (Barquero-Calvo et al., 2007, 2009; Martirosyan et al., 2011). Brucella cell envelope (CE) components such as the lipopolysaccharide (LPS) and lipoproteins as well as the overall CE architecture do not bear the typical pathogen-associated molecular patterns (PAMPs) that in other bacteria are recognized by Toll-like receptors (TLRs) and targeted by bactericidal peptides and the antibody-independent complement cascade. Instead, the CE of brucellae has an atypical LPS, peculiar amino lipids, and large amounts of phosphatidylcholine (PC) (Gamazo and Moriyon, 1987; Parent et al., 2007; Martirosyan et al., 2011; Palacios-Chaves et al., 2011). This typically eukaryotic phospholipid, while scarcely present in most bacterial groups (Aktas et al., 2010), is common in the α-2 Proteobacteria. This phylogenetic group includes Brucella and the plant pathogens and symbionts Agrobacterium and Sinorhizobium, which live in close association with eukaryotic cells (Moreno, 1992). The observation that Brucella and Agrobacterium mutants lacking PC in their cell envelopes are defective in their interactions with their respective eukaryotic hosts has led to the proposition that this phospholipid plays an important role in allowing these bacteria to avoid recognition by host defenses (Comerci et al., 2006; Conde-Álvarez et al., 2006; Aktas et al., 2010).
Whereas in eukaryotes PC synthesis occurs through the CDP-choline pathway (also called the Kennedy pathway), PC can be synthesized in bacteria by either the phospholipid N-methylation (Pmt) pathway or the phosphatidylcholine synthase (Pcs) pathway (Sohlenkamp et al., 2000, 2003; Aktas et al., 2010) (Figure 1). The Pmt pathway consists of the stepwise methylation of phosphatidylethanolamine (PE) sequentially generating monomethyl-PE (MMPE), dimethyl-PE (DMPE), and PC (Sohlenkamp et al., 2003; Aktas et al., 2010; Geiger et al., 2013). These three steps are catalyzed by a single cytosolic phospholipid N-methyltransferase (PmtA) that uses S-adenosylmethionine (SAM) as the methyl donor (Sohlenkamp et al., 2003), although other methyltransferases (PmtX) capable of catalyzing this reaction have been described in Bradyrhizobium (Aktas et al., 2010). The choline-dependent Pcs pathway consists of the condensation of this quaternary amine with CDP-diacylglycerol (CDP-DAG) catalyzed by Pcs (Sohlenkamp et al., 2000). Both pathways are active in Agrobacterium and Sinorhizobium (Aktas et al., 2010), but previous studies have reported that only the Pcs pathway is functional in B. abortus and B. melitensis due to the presence of mutated pmtA alleles in these strains (Martínez-Morales et al., 2003; Comerci et al., 2006; Aktas et al., 2010). Accordingly, the choline transporter ChoXWV1 is also required for PC biosynthesis in B. abortus (Herrmann et al., 2013).
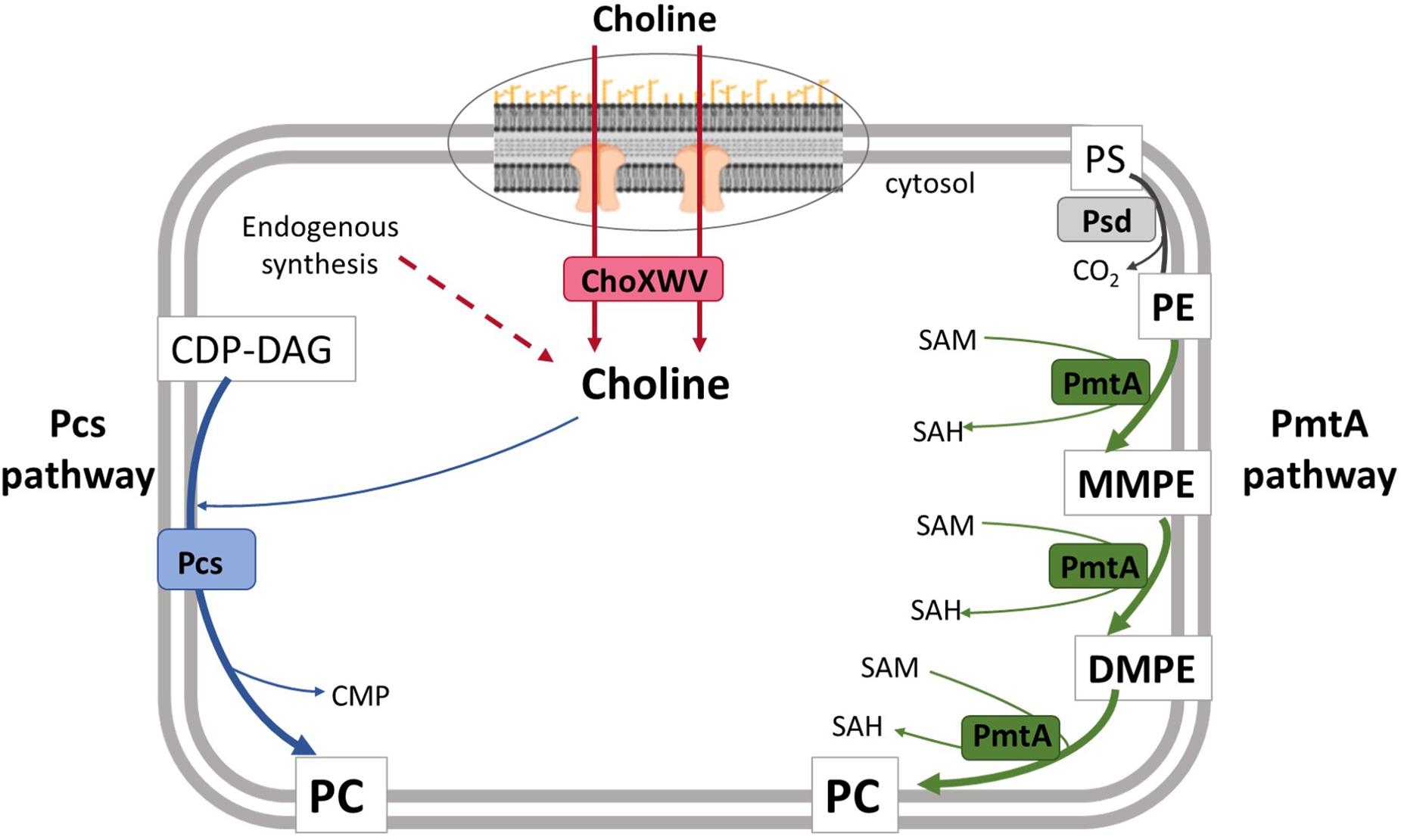
Figure 1. Model for phosphatidylcholine biosynthesis in B. suis biovar 2. In the Pcs pathway, the membrane protein Pcs condenses CDP-DAG and choline to generate PC. This choline is taken up by the ChoXWV transporter. Dashed arrows indicate steps for which putative genes have not been identified in Brucella. In the PmtA pathway, three successive methylations catalyzed by PmtA convert PE to PC. PmtA, phospholipid N-methyltransferase; Pcs, phosphatidylcholine synthase; ChoXWV, high-affinity choline transport system; SAM, S-adenosylmethionine; SAH, S-adenosylhomocysteine, CMP; cytidine monophosphate; CDP-DAG, cytidine diphosphate diacylglycerol; PS, phosphatidylserine; PE, phosphatidylethanolamine; MMPE, monomethylphosphatidylethanolamine; DMPE, dimethylphosphatidylethanolamine; PC, phosphatidylcholine.
In view of the importance of PC biosynthesis for the wild-type virulence of B. abortus (Comerci et al., 2006; Conde-Álvarez et al., 2006), we thought it worthwhile to investigate the PC biosynthesis pathways and ChoXWV choline uptake systems in other Brucella spp. Our experimental findings indicate that most Brucella strains excluding B. abortus and B. melitensis have the genetic capacity to carry out PC synthesis using either the Pmt or Pcs pathway, and all but B. canis and B. inopinata strains encode functional choline transporters. Remarkably, our studies provide evidence that B. canis can utilize the choline-dependent Pcs pathway in the absence of a functional high-affinity choline transporter, which suggests the presence of an uncharacterized choline biosynthesis pathway in these bacteria.
Materials and Methods
Bacterial Strains and Growth Conditions
The characteristics of the bacterial strains and plasmids are shown in Supplementary Table 1. The virulent B. suis biovar 2 (bv2) strain CITA 198 (henceforth Bs2WT) was selected for this study because the reference bv2 strain B. suis Thomsen is attenuated in mice (Aragón-Aranda et al., 2020). The choline-containing media used were tryptic soy broth (TSB, Scharlau, Barcelona, Spain), the same medium with 15% agar (TSA) or Blood Agar Base (BAB; Oxoid, United Kingdom), supplemented when necessary with 50 μg/ml kanamycin (Km), or/and 5% sucrose or/and 0.2% activated charcoal (Sigma) (see below). The choline-free medium was the lactate-glutamate-glycerol-vitamins medium of Gerhardt (1958) supplemented with 1 mM methionine (a Bs2WT requirement; Zúñiga-Ripa, unpublished results) (henceforth mGSM). E. coli strains were cultured on TSA-Km (50 μg/ml), supplemented with 1 mM diaminopimelic acid (DAP [Sigma]) in the case of E. coli β2150. All strains were stored at −80°C either in skimmed milk (Scharlau, Barcelona, Spain) or in TSB containing 0.5% yeast extract and 7% dimethylsulfoxide. Work was performed under Biosafety Level 3 (BSL-3) conditions.
Sequence Analyses
Genomic sequences of B. suis bv2 Thomsen (ATCC 23445 or NCBI:txid470137), B. melitensis bv1 16M (ATCC 23456 or NCBI:txid224914), B. abortus bv1 2308 (NCBI:txid359391), and S. meliloti 1021 (NCBI:txid266834) were obtained from the databases at National Center for Biotechnology Information (NCBI) or Kyoto Encyclopedia of Genes and Genomes (KEGG). For Bs2WT (genomic sequence not available), the ORF of interest were PCR amplified and then sequenced at “Servicio de Secuenciación del Centro de Investigación Médica Aplicada” (CIMA, Pamplona, Spain). Sequence alignments were performed with Clustal Omega.
DNA Manipulations and Construction of Mutants
Plasmids and chromosomal DNA were extracted with QIAprep® Spin Miniprep Kit and QIAamp® DNA Miniprep Kit (Qiagen GmbH, Hilden, Germany). When needed, DNA was purified from agarose gels using a QIAquick Gel extraction Kit (Qiagen GmbH, Hilden, Germany). Primers were synthesized by Sigma-Genosys Ltd. (Haverhill, United Kingdom).
Bs2WT in-frame deletion mutants encompassing the consensus sequences of pcs and pmtA were constructed using plasmids pRCI-22 and pRCI-10, respectively (Conde-Álvarez et al., 2006). These suicide plasmids were extracted from E. coli TOP10F′ and transformed into the DAP auxotrophic E. coli β2150 donor strain (Allard et al., 2015). To obtain the Bs2Δpcs mutant, pRCI-22 was introduced into Bs2WT by tri-parental mating with E. coli β2150 (carrying the suicide plasmid) and E. coli β2150-pRK2013 (helper strain) (Figurski and Helinski, 1979). The first recombinant was selected by Km resistance and DAP independence on media with charcoal. Bacteria resulting from allelic exchange by double recombination were selected on sucrose, and the loss of the plasmid was confirmed by Km sensitivity. The same strategy was followed to construct Bs2ΔpmtA using suicide plasmid pRCI-10 and the double mutant Bs2ΔpcsΔpmtA by deletion of pmtA in Bs2Δpcs. The loss of the plasmid concomitant with the gene deletion in each of these mutants was confirmed by PCR (oligonucleotides in Supplementary Table 2). The Bs2ΔpcsΔpmtA_pcs and Bs2ΔpcsΔpmtA_pmtA complemented strains were constructed by introduction of pRCI-40 (Conde-Álvarez et al., 2006) and pBA-8 in Bs2ΔpcsΔpmtA, respectively. To generate the pBA-8 plasmid, pRCI-41 (Conde-Álvarez et al., 2006) was modified at position G62D by PCR site-directed mutagenesis (Supplementary Table 2) to restore the PmtA consensus motif [VL(E/D)XGXGXG] present in B. suis bv2.
The suicide plasmid used to generate in-frame deletions in choX1 was constructed by PCR overlap using B. abortus 2308 genomic DNA as the template. Oligonucleotides choX1-F1 and choX1-R2 (Supplementary Table 2) were used to amplify a 493-base pair (bp) fragment including codons 1 to 54 of the BAB1_1593 homolog and 330 bp upstream of its first putative start codon, and primers choX1-F3 and choX1-R4 (Supplementary Table 2) were used to amplify a second fragment of 770 bp including codons 253 to 322 of BAB1_1593 and 555 bp downstream of its stop codon. These two fragments were ligated by overlapping PCR using primers choX1-F1 and choX1-R4 for amplification and the complementary regions between choX2-R2 and choX1-F3 for overlapping. The new fragment (containing the deletion allele) was cloned into pCR2.1 (Invitrogen) to generate pLPI-1. Plasmid pLPI was sequenced to confirm that the reading frame was maintained and then subcloned into the XbaI and the BamHI sites of plasmid pJQK (Scupham and Triplett, 1997) to produce pLPI-2. This suicide plasmid was introduced into Bs2WT by conjugation as described above. The mutant generated by allelic exchange was selected by Km sensitivity and sucrose resistance and confirmed by PCR using the corresponding oligonucleotides (Supplementary Table 2).
Bs2ΔchoX was constructed using the same methodology. Oligonucleotides choX2-F1 and choX2-R2 (Supplementary Table 2) were used to amplify a 446-bp fragment including codons 1 to 3 of the BAB2_0502 homolog and 437 bp upstream of its start codon, and oligonucleotides choX2-F3 and choX2-R4 (Supplementary Table 2) were used to amplify a second 494-bp fragment including codons 268 to 288 of BAB2_0502 and 428 bp downstream of the stop codon. These two fragments were ligated using primers choX2-F1 and choX2-R4, and the new fragment containing the deletion allele was cloned into pCR2.1 to generate pLPI-8. After sequencing to confirm that the reading frame was maintained, pLPI-8 was subcloned in pJQK to produce the mutator plasmid pLPI-9. Plasmid pLPI-9 was used to construct Bs2ΔchoX2 and Bs2ΔchoX1ΔchoX2 using Bs2WT and Bs2ΔchoX1 as background, respectively. After conjugation with Bs2WT, the resulting colonies were screened by PCR (Supplementary Table 2) to identify the choX2-deleted mutants. Similarly, mutant Bs2ΔpmtAΔchoX1ΔchoX2 was constructed on Bs2ΔpmtA by sequential use of plasmids pLPI-2 and pLPI-9. The Bs2ΔchoX1_choX1 complemented strain was constructed by stable insertion of the miniTn7 transposon into the chromosome of Bs2ΔchoX1 (Choi et al., 2005). To generate the pBA-13 plasmid, we first generated a PCR product using oligonucleotides choX1_Fw_Tn7 and choX1_Rv_Tn7 (Supplementary Table 2). This PCR product was cloned into the corresponding sites of the linearized pUC18 R6KT miniTn7T KmR vector (Llobet et al., 2009). The plasmid was introduced into E. coli S17 and transferred to Bs2ΔchoX1 mutant by tetra-parental conjugation between E. coli S17 (pBA-13), E. coli SM10 λpir (pTNS2), and E. coli HB101 (pRK2013). The construct (Bs2ΔchoX1_choX1) was examined by PCR for the correct insertion and orientation of the transposon between the glmS and recG genes as previously described (Martínez-Gómez et al., 2018). Finally, BcΔpmtA was constructed by in-frame deletion of pmtA using pRCI-10 as described for Bs2WT.
Growth Curves
Growth curves were obtained in a Bioscreen C (Lab Systems) apparatus. When mGSM was used, the inocula were prepared in this choline-free medium as follows. First, bacteria were grown in 10 ml of TSB at 37°C with orbital shaking for 18 h, harvested by centrifugation, resuspended in 10 ml of mGSM up to an optical density at 600 nm (O.D.600 nm) of 0.1, and incubated at 37°C with orbital shaking. These exponentially growing bacteria were harvested by centrifugation, resuspended at an O.D.600 nm of 0.1 in the same medium and 200 μl/well aliquots dispensed as technical replicates in Bioscreen C multi-well plates. Plates were incubated with continuous shaking at 37°C for 5 days and absorbance values at 420–580 nm recorded every 30 min. Growth curves in TSB were obtained following a similar protocol but the exponential phase inoculum was prepared in TSB. All experiments were repeated at least three times. Controls with medium and no bacteria were included in all experiments.
Choline Transport Assay
Transport assays were performed as described by Dupont et al. (2004) with minor modifications. Cells were grown in 10 ml of TSB at 37°C with orbital shaking until they reached the exponential phase (O.D.600 nm = 0.7), harvested by centrifugation, resuspended in mGSM at an O.D.600 nm of 1.0, and 1 ml of this suspension was mixed with 9 ml of this choline-free medium. When bacteria reached the exponential phase (O.D.600 nm = 0.5), they were washed twice with fresh mGSM and adjusted to an O.D.600 nm of 0.8. Transport assays were carried out using 1 ml of this cell suspension, with [methyl-3H]-choline chloride (82 Ci/mmol; Perkin Elmer) at a final concentration of 0.04 μM (optimized in preliminary experiments). After 15 and 60 min with continuous shaking at 37°C, bacteria were inactivated with phenol (0.5% final concentration) for 30 min at 37°C, collected on 22-μm membrane MF-Millipore (Sigma) filters using a 25-mm Swinnex filter holder (Merck). The filters were washed twice with 1 ml of fresh mGSM and the radioactivity in bacteria retained on the filter was measured in 2 ml of AquaLight (Hidex; Tecnasa) scintillation cocktail by a Hidex 300 SL Automatic TDCR Liquid Scintillation Counter (Gammadata Instrument AB, Uppsala, Sweden). Accumulation was expressed as μM of choline taking advantage of the efficiency correction (i.e., measurements in disintegrations per minute [d.p.m.]) of the apparatus. As a negative control, 1% phenol inactivated Bs2WT cells were used. All experiments were repeated at least twice.
Lipid Analyses
The free-lipid fraction was extracted as described by Bligh and Dyer (1959) and analyzed by high-performance thin-layer chromatography (HPTLC) on silica gel 60 plates (Merck). The plates were pre-run with n-propyl alcohol:propionic acid:chloroform:water (3:2:2:1, vol/vol) and dried thoroughly before sample loading. Chromatography was performed in the same mixture of solvents (Higgins and Fieldsend, 1987) and plates were developed by charring at 180°C with 15% sulfuric acid in ethanol or with 10% CuSO4 in 8% phosphoric acid. L-β,γ-Dipalmitoyl-α cephalin (phosphatidylethanolamine) (Fluka A.G. Buch, Switzerland) and L-α-phosphatidylcholine distearoyl (Sigma Chemical Co., St. Louis, MO, United States) were used as standards.
The incorporation of choline into PC was studied in both TSB and mGSM. For TSB, a loopful of bacteria grown on TSA was inoculated in 20 ml of TSB, grown overnight, and an aliquot of the culture was resuspended in 20 ml of either TSB or mGSM to an O.D.600 nm of 0.2. This broth was supplemented with 6.6 μCi of [methyl-3H]-choline chloride (82 Ci/mmol; Perkin Elmer) and incubated at 37°C with stirring until the middle exponential phase (O.D.600 nm of 0.8). Then, cells were harvested by centrifugation and free lipids were analyzed as described above. Free lipids (extracted as described above) were chromatographed and the HPTLC plates were exposed to Biomax Kodak films at −80°C for 20 days. Moreover, the radioactivity in the appropriate phospholipid spots was quantified as follows. Plates were developed by charring with 10% CuSO4 in 8% phosphoric acid, the phospholipid spots were scraped, and the silica was collected. After washing the powder with methanol, the phospholipid was extracted with 1:2 chloroform:methanol and 2:1 chloroform:methanol (Guijas et al., 2016), the solvent was evaporated, and the extract was suspended in 2 ml of AquaLight (Hidex; Tecnasa) scintillation cocktail. Radioactivity was quantified as described above.
Radioactive labeling of free lipids with acetate was performed similarly using 6.6 μCi of sodium [1,2–14C] acetate (52 mCi/mmol; PerkinElmer) in 20 ml. Cells were grown, lipids were extracted, and autoradiography was performed as described above.
Mouse Experiments
Seven-week-old female BALB/c mice (ENVIGO, Harlan) were lodged in cages in a BSL-3 facility (ES/31-2010-000132) with water and food ad libitum for 2 weeks before and during the experiments. For virulence assessment, groups of 10 mice were inoculated with the mutants or Bs2WT as parental strain control. Inocula were prepared by harvesting BAB grown bacteria in sterile buffered saline (BSS; 0.85% NaCl, 0.1% KH2PO4, and 0.2% K2HPO4; pH 6.85) and adjusting the suspension to 1 × 106 CFU/ml. Mice were inoculated intraperitoneally with 1 × 105 CFU in 0.1 ml (the exact dose was determined retrospectively on triplicate BAB plates). Two and 8 weeks after inoculation, mice were euthanized; the spleens were aseptically removed, individually weighed, and homogenized in 9 volumes of BSS; and serial 10-fold dilutions were plated by triplicate on BAB plates. After 5 days at 37°C, CFU were counted and the identity was confirmed by PCR. The data (mean CFU/spleen) were normalized by logarithmic transformation and the mean log10 CFU/spleen values and standard deviations (SDs) were calculated. These values were used for plotting and for statistical comparisons by one-way ANOVA and Fisher’s Protected Least Significant Differences (PLSD) tests (statistical procedures established for virulence comparisons in the mouse model of brucellosis, Grilló et al., 2012).
The procedures were in accordance with the current European (directive 86/609/EEC) and Spanish (RD 53/2013) legislations, supervised by both Ethical Committee for Animal Experimentation of CITA and Animal Welfare Committee of the University of Navarra and authorized by Aragón (reports No. 2014-20 and 2014-21) and Navarra (CEEA 045/12) Governments.
Results
Phosphatidylcholine Synthesis in B. suis Biovar 2 Takes Place Through Both the Methylation (PmtA) Pathway and the PC Synthase (Pcs) Pathway
We first assessed whether the synthesis of PC in Bs2WT was dependent on the PmtA and/or the Pcs pathways (Figure 1). To this end, we constructed single (Bs2ΔpmtA and Bs2Δpcs) and double (Bs2ΔpcsΔpmtA) non-polar mutants by making extensive in-frame deletions encompassing the consensus motifs associated with PmtA function. We then obtained stationary phase bacteria in TSB, extracted the free-lipid fractions and compared their composition by HPTLC. As shown in Figure 2, whereas Bs2WT and the single mutants Bs2Δpcs and Bs2ΔpmtA produced similar amounts of PC, Bs2ΔpcsΔpmtA did not produce PC in TSB. Moreover, we observed MMPE and DMPE (the PC precursors that demonstrate the activity of PmtA) only for Bs2WT and Bs2Δpcs. When we complemented Bs2ΔpcsΔpmtA with a plasmid carrying pcs, PC production was restored (Supplementary Figure 1). Introduction of a plasmid carrying pmtA (with an intact consensus sequence) into Bs2ΔpcsΔpmtA resulted in MMPE and DMPE and PC production, although at lower levels than those in Bs2WT (Supplementary Figure 1). These observations show that PC synthesis in Bs2WT can take place through both the PmtA and Pcs pathways.
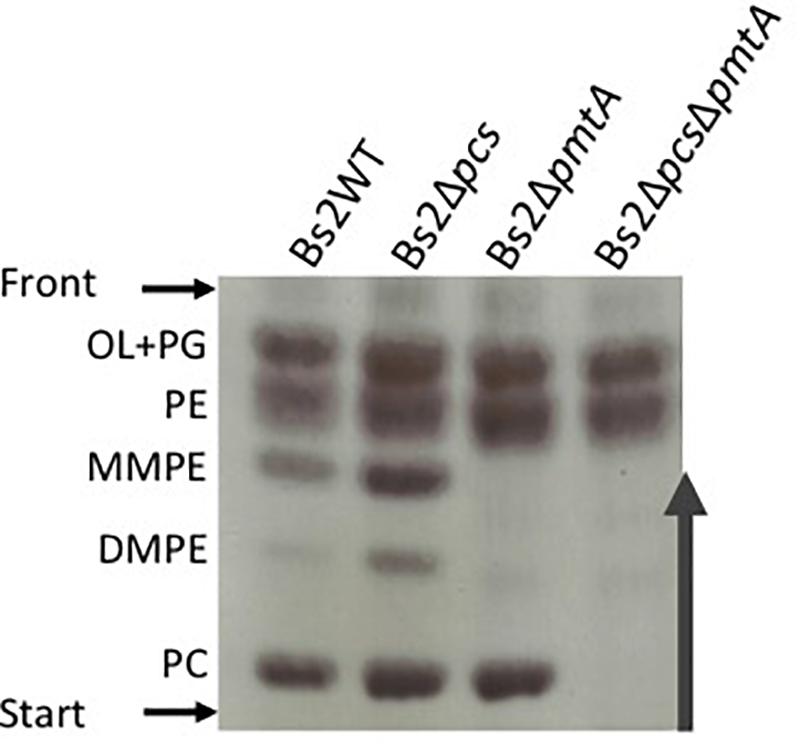
Figure 2. Phosphatidylcholine synthesis in Bs2WT takes place through the methylation (PmtA) and the phosphatidylcholine synthase pathway (Pcs). HPTLC analysis of the free lipids of Bs2WT, Bs2Δpcs, Bs2ΔpmtA, and Bs2ΔpcsΔpmtA grown in TSB. OL, ornithine lipids; PG, phosphatidylglycerol; PE, phosphatidylethanolamine; MMPE, mono-methyl-phosphatidylethanolamine; DMPE, dimethyl-phosphatidylethanolamine; PC, phosphatidylcholine.
An intact Pcs pathway is required for the wild-type growth of B. abortus 2308 in a rich medium (Comerci et al., 2006; Conde-Álvarez et al., 2006; Supplementary Figure 2). In contrast, both Bs2ΔpmtA and Bs2Δpcs grew normally in TSB, but the Bs2ΔpcsΔpmtA mutant displayed slightly retarded growth in this medium (Supplementary Figure 2). These results are consistent with the functionality of both the PmtA and Pcs pathways in Bs2WT.
Phospholipid N-Methyltransferase Is Conserved in Brucella Species Other Than B. abortus and B. melitensis
To better understand the differences in the PC synthesis pathways of Bs2WT and those reported for B. abortus 2308 and B. melitensis, and to explore whether these differences extend to other Brucella species, we carried out genomic comparisons. In the B. suis bv2 reference strain Thomsen genome sequence, BSUIS_A1967 is annotated as encoding the phospholipid N-methyltransferase PmtA, and we confirmed this annotation by sequencing the homologous ORF of Bs2WT. In the subsequent analyses, we found that the mutations identified previously in the SAM binding sites of the PmtAs encoded by B. abortus 2308 and B. melitensis 16M (Comerci et al., 2006; Conde-Álvarez et al., 2006) were not present in B. suis bv2 Thomsen or Bs2WT (Supplementary Figure 3). Moreover, when we examined the genome sequences of other Brucella strains representing different species and biovars, we found that all of the B. melitensis and B. abortus strains examined encode pmtA alleles carrying the same mutations described in B. abortus 2308 and B. melitensis 16M. In contrast, the genomes of type strains of B. canis, B. microti, B. vulpis, B. ovis, B. ceti, and B. pinnipedialis, and all of the genomes of the B. suis strains examined except B. suis bv1 1330 encode pmtA genes with conserved SAM binding motifs [VL(E/D)XGXGXG] (de Rudder et al., 2000; Sohlenkamp et al., 2003) (Supplementary Table 3). This polymorphism in the SAM binding motif of B. suis bv1 1330 was reported previously (Comerci et al., 2006) and, interestingly, is found in only one of the two available B. suis bv1 1330 genome sequences. No differences exist in the consensus motif (Sohlenkamp et al., 2000) of Pcs of all the Brucella spp. and biovars studied (Supplementary Table 4).
Choline Transport Is Active in B. suis Biovar 2
Since the ChoXWV system is important for choline-dependent PC synthesis in B. abortus 2308 (Herrmann et al., 2013), we studied its functionality and connection with PC synthesis in Bs2WT. To this end, we first searched for orthologs of the B. abortus 2308 ChoXWV genes in B. suis bv2 Thomsen. We identified three operons: ORFs BSUIS_A1635 to 1637 (henceforth ChoXWV1) on chromosome I, BSUIS_B0730 to 0732 (henceforth ChoXWV2) on chromosome II, and BSUIS_A0222 to 0225 on chromosome I. Each of these operons contained orthologs of choX, choW, and choV, respectively, annotated as encoding a choline-glycine betaine-proline binding protein, a permease, and an ATP-binding protein (Aktas et al., 2011; Supplementary Figure 4). S. meliloti ChoX has high affinity for choline (equilibrium dissociation constant KD 2.3–2.7 μM), only medium affinity for acetylcholine (KD 100–145 μM) (Dupont et al., 2004; Oswald et al., 2008), and binds no betaine (Dupont et al., 2004). Analogous studies in A. tumefaciens found KD values for choline, acetylcholine, and betaine of 2, 80, and 470 μM, respectively (Aktas et al., 2011). Similarly, the ChoX1 homolog in B. abortus shows dissociation constants of 7 ± 2 nM for choline and 350 ± 30 μM for betaine, and no specific L-carnitine or L-proline binding (Herrmann et al., 2013). While these types of experiments have not been conducted with B. abortus ChoX2, ligand binding assays performed with B. abortus YehZ (the protein encoded by the homolog of BSUIS_A0222) showed no interaction with glycine betaine, choline, ectoine, or carnitine at low ligand concentration, and only low affinity for glycine betaine (Herrou et al., 2017).
The crystal structure of S. meliloti ChoX shows two domains connected by two β-strands that conform a single functional linker in which a glycine residue (Gly116) flanked by alanine is thought to play an important role in substrate binding (Oswald et al., 2008). The amino acid sequence of the B. suis bv2 Thomsen ChoX1 and ChoX2 homologs showed that, although both have a Gly120, only ChoX1 has a flanking Ala121. Also, whereas both B. suis bv2 Thomsen ChoX1 and ChoX2 contain the Asp45 residue that forms a salt bridge with the choline head group in S. meliloti, only ChoX1 contains the Asn156–Asp157 residues that form hydrogen bonds with the tail of choline in S. meliloti ChoX (Supplementary Figure 5). Other amino acids involved in creating the ChoX–choline complex in S. meliloti are Cys33–Cys247, which form a disulfide bridge, and Met91–Pro92 and Glu206–Pro207, which form two cis-peptide bonds. In B. suis bv2 Thomsen, a disulfide bridge in a similar position (Cys37–Cys251) was presented only in ChoX1 (Supplementary Figure 5). Concerning the two cis-peptide bonds, the Brucella ChoX1 has Asn95–Pro96 and Glu210–Pro211, while ChoX2 presents Leu87–Pro88 and Ser200–Pro201. Amino acids Trp43, Trp90, Tyr119, and Trp205 in S. meliloti and Trp40 and Trp87 in A. tumefaciens are also important for the binding of choline (Oswald et al., 2008; Aktas et al., 2011), and the B. suis bv2 Thomsen homologs presented similar characteristics (Trp47, Trp94, Tyr123, and Trp209 for ChoX1; Trp38, Trp86, Tyr109, and Trp199 for ChoX2). Sequencing of the choX1 and choX2 orthologs showed that all the above-summarized characteristics of B. suis bv2 Thomsen were reproduced in Bs2WT. In contrast with ChoX1 and ChoX2, the BSUIS_A0222 encoded protein does not show any of the relevant features described in ChoX of S. meliloti and A. tumefaciens, in accordance with the results presented by Herrou et al. (2017) describing the B. abortus BSUIS_A0222 ortholog.
We used the above-summarized data to investigate the importance of choline uptake in pcs-dependent PC synthesis in Bs2WT. First, we constructed a Bs2ΔchoX1 non-polar mutant. Also, since despite the absence of the Gly120–Ala121 and Asn156–Asp157 residues (described as relevant in B. abortus, Herrmann et al., 2013) ChoX2 still keeps features relevant in choline binding, we ensured removal of any residual activity by constructing a double Bs2ΔchoX1ΔchoX2 mutant. Then, we grew the bacteria in mGSM supplemented with a low amount (0.04 μM) of methyl-3H-choline to avoid any interference of a potential low-affinity choline uptake system such as that described in B. abortus (Herrmann et al., 2013). Whereas viable Bs2WT accumulated [methyl-3H]-choline, neither Bs2ΔchoX1 nor Bs2ΔchoX1ΔchoX2 accumulated the probe either after 15 (Figure 3) or 60 min (not shown). Moreover, introduction of choX1 into Bs2ΔchoX1 restored the ability to accumulate [methyl-3H]-choline to the levels of Bs2WT (Supplementary Figure 6). These results are in keeping with the observations in B. abortus (Herrmann et al., 2013) and indicate that ChoXWV1 also provides the major pathway for choline import in Bs2WT.
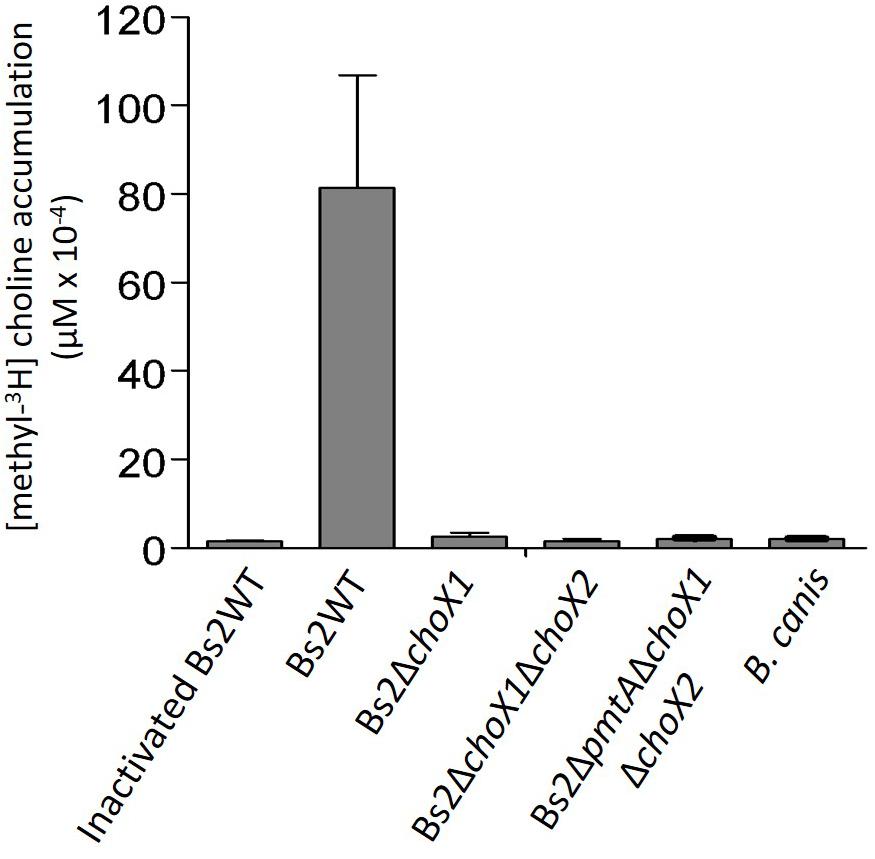
Figure 3. Choline accumulation in Bs2WT, Bs2ΔchoX1, Bs2ΔchoX1ΔchoX2, Bs2ΔpmtAΔchoX1ΔchoX2, and B. canis grown in mGSM. Values are the mean ± standard error of technical duplicates of a representative experiment, repeated at least two times with similar results.
ChoX Is Not Conserved in All Brucella Species
Consistent with the postulated import of choline from the host Comerci et al. (2006); Herrmann et al. (2013) noted that the characteristics of a functional ChoX1 described in the previous paragraph are conserved in all B. abortus strains sequenced. However, when we extended the genomic analyses to other brucellae to confirm the association of ChoX1 conservation with parasitism, we observed two conspicuous exceptions: B. canis and B. inopinata. In all sequenced strains of B. canis, the choX1 ortholog carries a deletion in the position equivalent to nucleotide 67 that creates a frameshift that extends to most of the gene (Supplementary Table 5). On the other hand, choX2, which is not related to choline uptake, is conserved (Supplementary Table 6). Strikingly, the same choX1 frameshift observed in B. canis is also present in B. inopinata BO1. We did not find other remarkable mutations in ChoW1 and ChoV1 in either of these species (Supplementary Tables 7, 8). The presence of this choX1 frameshift strongly suggests that wild-type B. canis and B. inopinata are unable to take up choline, at least under the experimental conditions used here. When we tested this prediction using B. canis, we found no accumulation of [methyl-3H]-choline (Figure 3).
Phosphatidylcholine Synthesis in ChoX-Deficient Brucellae
Brucella inopinata is known to produce PC (Scholz et al., 2010), and according to one early report, B. canis also produces PC (Kulikov and Dranovskaia, 1988). Since both species are naturally ChoX deficient, it seems highly likely that these bacteria produce PC exclusively through the PmtA pathway and that Pcs, although conserved, no longer plays a role in PC synthesis. We tested this hypothesis by comparing the lipid profiles of wild-type B. canis and an isogenic pmtA mutant. We found that the parental strain produced PC in both TSB and mGSM (Figure 4), a result that shows that B. canis does not depend on the ChoXWV system to produce PC regardless of the complexity of the medium. However, we also observed that disabling pmtA in B. canis (BcΔpmtA mutant) did not abrogate its capacity to synthesize PC in TSB (Figure 4).
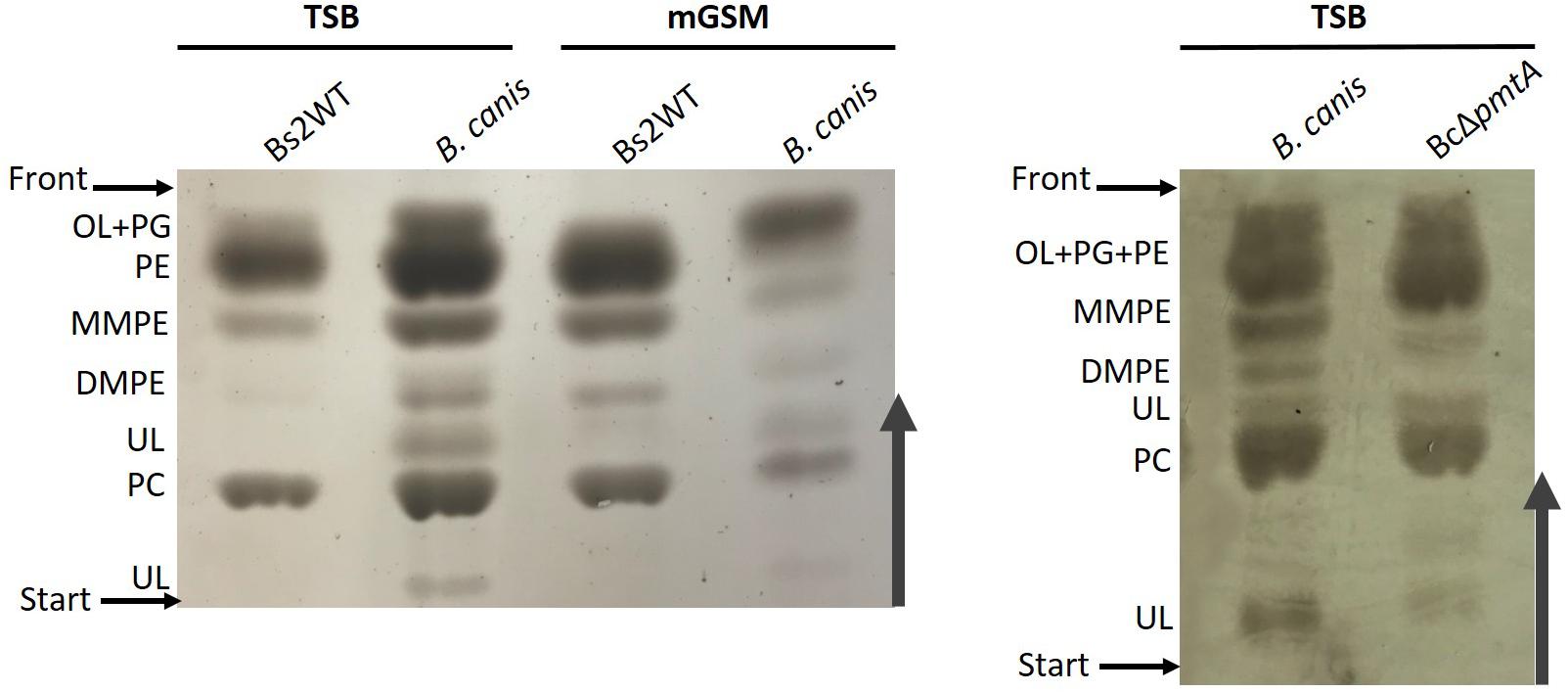
Figure 4. High-performance thin-layer chromatography analysis of the free lipids of B. canis and BcΔpmtA grown in TSB or mGSM. OL, ornithine lipids; PG, phosphatidylglycerol; PE, phosphatidylethanolamine; MMPE, monomethyl-phosphatidylethanolamine; DMPE, dimethyl-phosphatidylethanolamine; PC, phosphatidylcholine; UL, unknown lipid.
To further investigate PC synthesis by Pcs in ChoX-deficient brucellae, we carried out experiments in Bs2WT taking advantage of the mutants developed during this work. First, we combined the non-polar pmtA and choX1choX2 mutations in a triple Bs2ΔpmtAΔchoX1ΔchoX2 mutant and, after confirming its inability to transport choline (Figure 3 and Supplementary Figure 7), we compared the free lipids produced by the parental strain and this mutant in TSB. While Bs2WT synthetized PC and the MMPE and DMPE precursors that confirm the activity of PmtA, Bs2ΔpmtAΔchoX1ΔchoX2 showed PC but not MMPE and DMPE (Figure 5A), a result that parallels that obtained with the BcΔpmtA mutant. We then examined the free lipids of bacteria grown in mGSM. We first confirmed the functionality of the pathways in mGSM using Bs2Δpcs, Bs2ΔpmtA, and a double Bs2ΔpcsΔpmtA as controls. As expected, these mutants respectively showed MMPE, DMPE, and PC, reduced amounts of PC (as expected in a nutrient-limited medium), and no PC (Figure 5B). In mGSM, we detected traces of PC in Bs2ΔpmtAΔchoX1ΔchoX2 (Figure 5B; see also Figure 6A). When we extended these analyses to Bs2WT, Bs2ΔchoX1ΔchoX2, and Bs2ΔpmtAΔchoX1ΔchoX2 grown in [1,2–14C] acetate-mGSM, we also observed PC in Bs2WT and Bs2ΔchoX1ΔchoX2 and traces of PC in Bs2ΔpmtAΔchoX1ΔchoX2 (Figure 6).
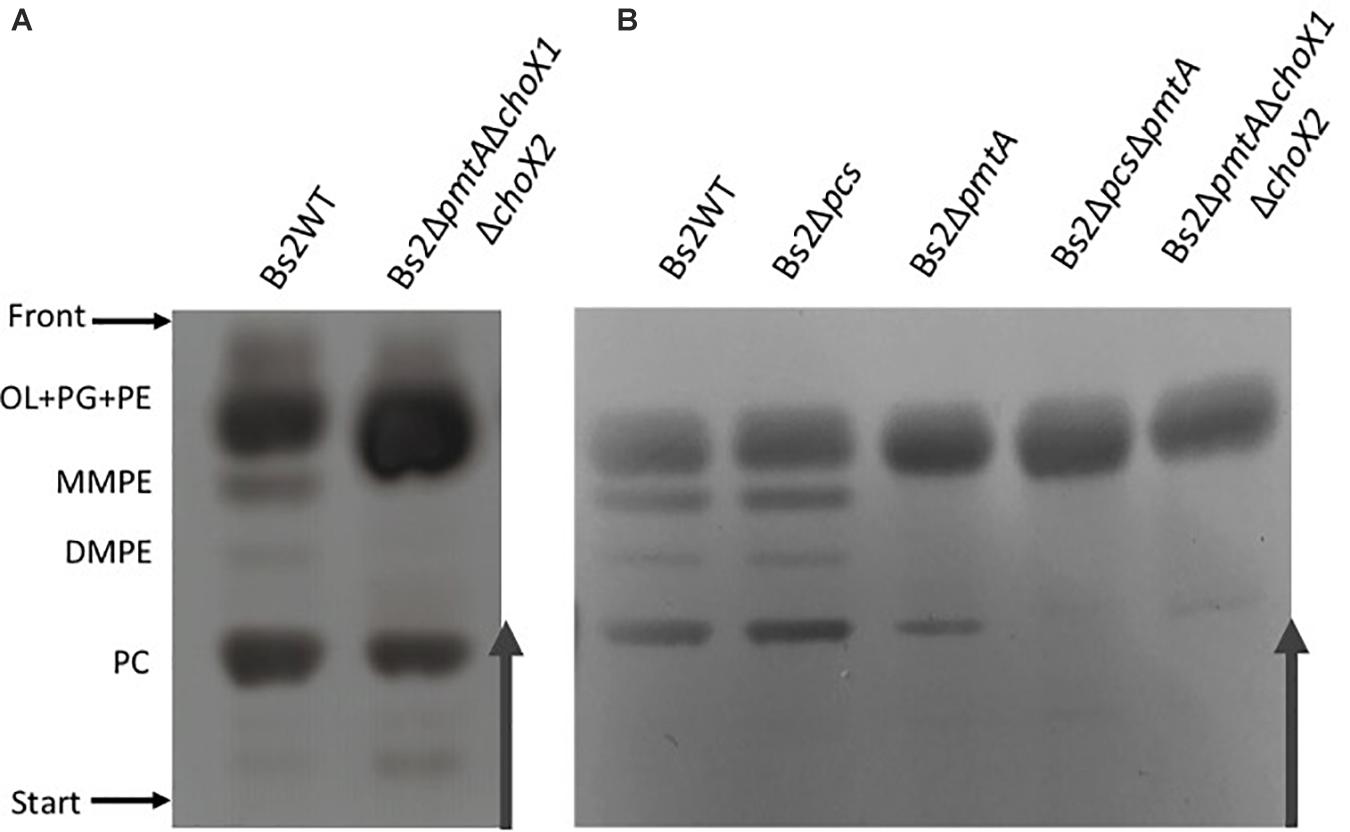
Figure 5. High-performance thin-layer chromatography analysis of the free lipids of Bs2WT- and Bs2-derived (A) mutants grown in TSB and (B) mutants grown in mGSM. OL, ornithine lipids; PG, phosphatidylglycerol; PE, phosphatidylethanolamine; MMPE, monomethyl-phosphatidylethanolamine; DMPE, dimethyl-phosphatidylethanolamine; PC, phosphatidylcholine; UL, unknown lipid.
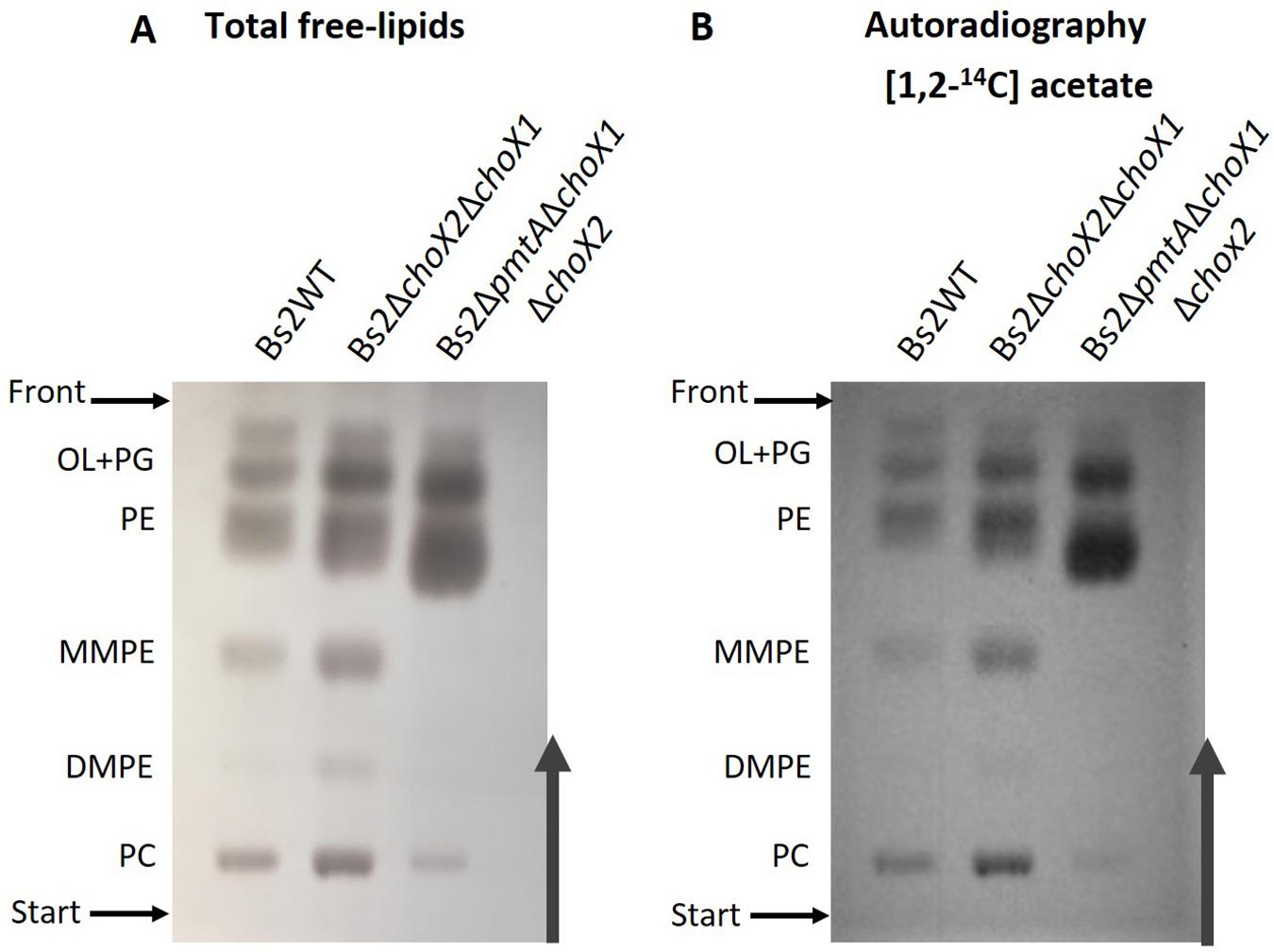
Figure 6. High-performance thin-layer chromatography analysis of Bs2WT, Bs2ΔchoX1ΔchoX2, and Bs2ΔpmtAΔchoX1ΔchoX2 after in vivo labeling with [1,2− 14C] acetate in mGSM. Total free lipids were revealed by charring (A) or autoradiography (B). OL, ornithine lipids; PG, phosphatidylglycerol; PE, phosphatidylethanolamine; MMPE, monomethyl-phosphatidylethanolamine; DMPE, dimethyl-phosphatidylethanolamine; PC, phosphatidylcholine.
Phosphatidylcholine Synthesis Is Required for Bs2WT Full Virulence in Mouse Model
We used the mouse model (Grilló et al., 2012; Aragón-Aranda et al., 2020) to examine the contribution of PC to B. suis bv2 virulence. We observed that impairment of PC synthesis in Bs2WT by deletion of both pcs and pmtA resulted in a low degree of attenuation that only became significant at late stages of the infection (Figure 7). This observation is reminiscent of the attenuation of B. abortus 2308 PC-deficient mutants, which is also more marked at later stages of infection (Comerci et al., 2006; Conde-Álvarez et al., 2006). This profile was more clearly observed for the double mutant, suggesting that in Bs2WT, both pathways are active in this virulence model. However, when we tested Bs2ΔchoX1ΔchoX2, we did not observe an effect on virulence at any time (Figure 7), suggesting that PC synthesis in B. suis biovar 2 proceeds independently of ChoX in vivo.
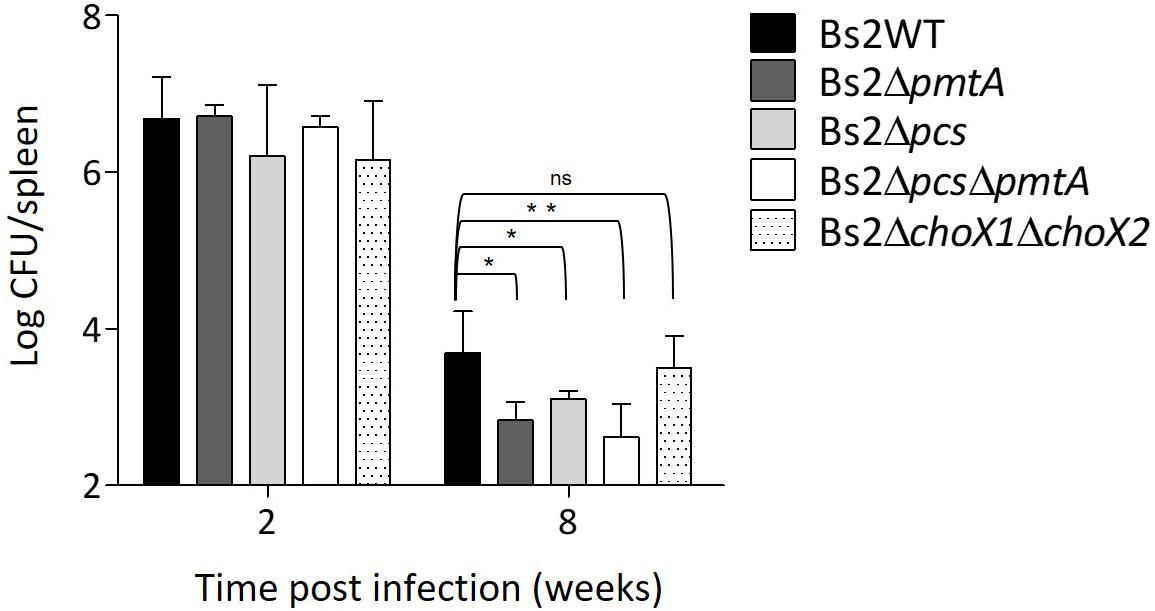
Figure 7. Phosphatidylcholine synthesis is required for Bs2WT full virulence. CFUs in spleen of infected BALB/c mice were counted after intraperitoneal inoculation with 105 CFU/mouse of Bs2WT (parental strain), Bs2Δpcs, Bs2ΔpmtA, Bs2ΔpcsΔpmtA, or Bs2ΔchoX1ΔchoX2. *p ≤ 0.05, **p ≤ 0.01, n.s. not significant.
Discussion
Phosphatidylcholine has been shown to be required for the fitness of many α-2 Proteobacteria including Brucella and the successful interactions of these bacteria with their respective eukaryotic hosts (Comerci et al., 2006; Conde-Álvarez et al., 2006). Studies employing B. abortus 2308 and B. melitensis 16M suggested that the brucellae may rely exclusively on the Pcs pathway for PC biosynthesis based on the presence of dysfunctional pmtA genes (Comerci et al., 2006). However, considering the recently recognized physiologic and metabolic diversity that exists between Brucella strains (Scholz et al., 2008, 2010; Vizcaíno and Cloeckaert, 2012; Wattam et al., 2012; Whatmore et al., 2014; Soler-Lloréns et al., 2016; Barbier et al., 2017; Conde-Álvarez et al., 2018; Zúñiga-Ripa et al., 2018; Machelart et al., 2020; Lázaro-Antón et al., 2021), we thought it worthwhile to re-examine PC synthesis in these bacteria. We first investigated the functionality of the Pcs and PmtA pathways in a virulent strain of B. suis bv2, a choice based on both its position within the core brucellae (Whatmore, 2009) and biosafety considerations (this biovar is highly virulent in pigs but not in humans; Welfare, 2009). We found both pathways to be functional in this strain, and a subsequent genomic comparison across the genus showed that, in Brucella, there are polymorphisms in the metabolism of PC via PmtA. It is remarkable that the converse situation, e.g., Pcs dysfunction and PmtA functionality, does not occur in wild-type Brucella or in their close phylogenetic neighbors. For the latter, although the PmtA pathway may be more energetically demanding because it requires S-adenosyl-homocysteine recycling, it may be beneficial in the soil where competition for choline can occur. However, the Pcs pathway may be sufficient during the interactions of these bacteria with their eukaryotic hosts where choline should be available, which could explain why both pathways have been retained (Aktas et al., 2014a,b). Notably, the zoonotic brucellae do not multiply in nature outside their mammalian hosts, and the availability of host-derived choline has been proposed as the basis for the loss of functionality of the Pmt pathway in B. abortus and B. melitensis (Comerci et al., 2006). Why this has happened in these ruminant-associated strains but not in the other zoonotic Brucella species may reflect unknown characteristics of the nutritional environment in different animal hosts or the randomness that is at the basis of bacterial evolution.
Despite the proposed advantage of the Pcs pathway for Brucella strains in mammalian hosts, the corresponding choline transporter ChoXWV1 is not conserved in B. canis or B. inopinata BO1, and the results presented here show that the frameshift mutation present in the B. canis choX1 gene inactivate high-affinity choline import in this strain. These studies also support those reported by Herrmann et al. (2013), which indicate that ChoXWV2 (unharmed in B. canis) is not involved in choline uptake, even though its conservation throughout the genus suggests that it may serve for the uptake of a substrate structurally akin to choline (Aragón-Aranda, unpublished observations). The ChoXWV1 dysfunction in B. canis was unexpected due to the close relatedness of this species to B. suis biovar 3 and 4 strains (Scholz et al., 2008; Whatmore, 2009; Wattam et al., 2014), which carry a canonical ChoX1. Finding the same mutation in the B. inopinata BO1 choX1 gene was even more unexpected since this strain is phylogenetically very distant from B. canis and B. suis biovar 3 and 4 strains. While we do not know the reasons for this coincidence, these observations are not trivial because they pose questions on the origin of the choline used by the otherwise highly conserved Pcs pathway and on how it is obtained from the mammalian host.
Although unable to take up choline, B. canis keeps Pcs as well as PmtA and a BcΔpmtA mutant maintains the ability to synthesize PC in TSB, a medium that contains choline (Figure 4). Similarly, Bs2ΔpmtAΔchoX1ΔchoX2 produced PC in this medium (Figure 5A). Moreover, even though definite proof of its identity requires a more refined analysis than HPTLC, Bs2ΔpmtA showed low amounts of a component compatible with PC but not MMPE or DMPE in nutrient-limited mGSM (Figure 5B). Low amounts of PC can also be observed in [1,2]14C-acetate autoradiograms of B. abortus 2308 and the isogenic BAB2_0502 (choX2)-BAB1_0226 (third choX) double mutant grown in Gerhardt’s synthetic medium (similar to mGSM) (Comerci et al., 2006; Herrmann et al., 2013). One possibility is that choline could be synthesized from ethanolamine by the action of non-previously described methylases and, although mGSM does not contain ethanolamine, ethanolamine could be derived from the action of phospholipases on PE via PLA-1 (Kerrinnes et al., 2015). However, while the results with radioisotopes and Bs2ΔpmtAΔchoX1ΔchoX2 studies (Figure 6 and Supplementary Figure 7) do not rigorously rule out this possibility, they show that such an endogenous source of choline is minimal in a nutrient-limited medium. An alternative explanation would be that Pmt-like enzymes produce PC in low amounts, thus making detection of MMPE and DMPE exceedingly difficult. In Bradyrhizobium japonicum, a PmtA homolog is required for efficient PC synthesis but PmtA mutants still produce minor amounts of PC (Minder et al., 2004). B. japonicum and Bradyrhizobium SEMIA 6144 R carry four and two additional Pmt enzymes, respectively (Aktas et al., 2010), and several pmt candidates exist in Rhodopseudomonas palustris, Rhizobium etli, and R. leguminosarum (Hacker et al., 2008). Brucella genomes also contain up to 11 additional genes encoding putative methyltransferases but cloning and expression of these genes in E. coli has failed to detect enzymatic activity consistent with a role in PC biosynthesis (Vences-Guzmán, unpublished observations). Finally, as TSB contains low amounts of choline, a plausible explanation for the presence of PC in Bs2ΔpmtAΔchoX1ΔchoX2 grown in this medium would be the activity of an alternative choline low-affinity uptake system, as proposed by Herrmann et al. (2013), and we favor this hypothesis. If such a system were relevant in vivo, e.g., in an environment with abundant choline, it could explain why the pcs gene is conserved in Brucella. However, what is clear is that the ChoXWV-Pcs and PmtA pathways are by far the major routes for PC biosynthesis in the genus Brucella in vitro. Nevertheless, there are dysfunctions that affect one pathway or the other in some Brucella species, and the differential use of these pathways may reflect important differences that exist between Brucella strains and/or the natural hosts they inhabit and whether they have an environmental niche outside of these natural hosts.
Previous evidence on the need for PC in fitness and virulence was obtained with B. abortus Pcs mutants in the same mouse model used here, and consistent with this, we observed that PC-lacking B. suis biovar 2 (i.e., BsΔpcsΔpmtA) displays similar defects. This effect was much less intense than that caused by mutations in the immunodominant lipopolysaccharide of Brucella, a molecule playing a chief role in escaping detection by innate immunity (Martirosyan et al., 2011). Therefore, it seems that the lack of PC has a comparatively small impact on the stealthiness of this parasite, which explains why it is manifested only in the chronic phase of infection in mice. It is also noteworthy that abrogation of high-affinity choline import in B. suis biovar 2 did not affect virulence under the same experimental conditions, suggesting that PC synthesis in B. suis biovar 2 in vivo proceeds independently of ChoX. This could also explain the existence of naturally ChoX-deficient virulent brucellae like B. canis and B. inopinata. Although B. canis presented low amounts of unknown lipids (Figure 4), we did not find that they could vary significantly upon growth in the two media or after pmtA mutation, evidence that could suggest membrane compensatory effects associated with PC deficiencies. It is worth noting that B. inopinata and other Brucella species produce low amounts of polar lipids that vary depending on the strain or species and whose identities and roles remain unknown (reviewed in Scholz et al., 2010). Interestingly, Herrmann et al. (2013) found that a B. abortus ChoX mutant was not attenuated 30 days after intragastric inoculation with 1010 CFU, which contrasts with the attenuation of an isogenic B. abortus pcs mutant using the same protocol. Indeed, it may be that choline availability during infection varies from the early to the late stages and depending on the route of inoculation and the laboratory model (Herrmann et al., 2013). In addition, Herrmann et al. (2013) also made the interesting observation that attenuation in macrophages was detected when Gerhardt’s synthetic medium but not when choline-rich TSB was used to prepare the inoculum. Therefore, whereas all authors conclude that PC is necessary for full virulence, it may be that different media for inoculum preparation and CFU counting, and different infection routes, yield similar but not identical results. The demonstration that most Brucella spp. keep the Pcs and the PmtA pathways and a functional ChoXWV, and that virulent B. canis also possesses both pathways but lacks a functional ChoXWV, opens the possibility of gaining further insight into the role of these three systems in Brucella virulence in natural hosts.
Data Availability Statement
The original contributions presented in the study are included in the article/Supplementary Material, further inquiries can be directed to the corresponding author/s.
Ethics Statement
The animal study was reviewed and approved by the procedures were in accordance with the current European (directive 86/609/EEC) and Spanish (RD 53/2013) legislations, supervised by both Ethical Committee for Animal Experimentation of CITA and Animal Welfare Committee of the University of Navarra and authorized by Aragón (reports No. 2014-20 and 2014-21) and Navarra (CEEA 045/12) governments.
Author Contributions
RC-Á, MI, and IM conceived and coordinated the study. PM supervised the mouse studies. BA-A, LP-C, MS-B, MV-G, MM, LL-A, and AZ-R performed the experiments. RC-Á, BA-A, CS, and IM wrote the manuscript. All authors analyzed the results and approved the final version of the manuscript, and read and agreed to the published version of the manuscript.
Funding
This research at the University of Navarra was supported by the Institute for Tropical Health funders (Obra Social la CAIXA—LCF/PR/PR13/11080005—and Fundación Caja Navarra, Fundación María Francisca de Roviralta, Ubesol, and Inversiones Garcilaso de la Vega S.L) and MINECO (grants AGL2014-58795-C4-1-R and PID2019-107601RA-C32). Work at CITA was supported by the MINECO grant AGL2014-58795-C4-3-R and Aragón Government (Grupo de Investigación A13_17R). BA-A was the recipient of the Ph.D. Fellowship BES-2015-075609 funded by the MINECO.
Conflict of Interest
The authors declare that the research was conducted in the absence of any commercial or financial relationships that could be construed as a potential conflict of interest.
Publisher’s Note
All claims expressed in this article are solely those of the authors and do not necessarily represent those of their affiliated organizations, or those of the publisher, the editors and the reviewers. Any product that may be evaluated in this article, or claim that may be made by its manufacturer, is not guaranteed or endorsed by the publisher.
Acknowledgments
We are grateful to Marty Roop for a critical reading of the manuscript and to Christoph Dehio for the generous gift of the β2150 cells. The excellent technical assistance of Sara Serrano is also gratefully acknowledged.
Supplementary Material
The Supplementary Material for this article can be found online at: https://www.frontiersin.org/articles/10.3389/fmicb.2021.614243/full#supplementary-material
References
Aktas, M., Danne, L., Möller, P., and Narberhaus, F. (2014a). Membrane lipids in Agrobacterium tumefaciens: biosynthetic pathways and importance for pathogenesis. Front. Plant Sci. 5:109. doi: 10.3389/fpls.2014.00109
Aktas, M., Jost, K. A., Fritz, C., and Narberhaus, F. (2011). Choline uptake in Agrobacterium tumefaciens by the high-affinity ChoXWV transporter. J. Bacteriol. 193, 5119–5129. doi: 10.1128/JB.05421-11
Aktas, M., Köster, S., Kizilirmak, S., Casanova, J. C., Betz, H., Fritz, C., et al. (2014b). Enzymatic properties and substrate specificity of a bacterial phosphatidylcholine synthase. FEBS J. 281, 3523–3541. doi: 10.1111/febs.12877
Aktas, M., Wessel, M., Hacker, S., Klüsener, S., Gleichenhagen, J., and Narberhaus, F. (2010). Phosphatidylcholine biosynthesis and its significance in bacteria interacting with eukaryotic cells. Eur. J. Cell Biol. 89, 888–894. doi: 10.1016/j.ejcb.2010.06.013
Al Dahouk, S., Hofer, E., Tomaso, H., Vergnaud, G., Le Flèche, P., Cloeckaert, A., et al. (2012). Intraspecies biodiversity of the genetically homologous species Brucella microti. Appl. Environ. Microbiol. 78, 1534–1543. doi: 10.1128/AEM.06351-11
Al Dahouk, S., Köhler, S., Occhialini, A., Jiménez de Bagüés, M. P., Hammerl, J. A., Eisenberg, T., et al. (2017). Brucella spp. of amphibians comprise genomically diverse motile strains competent for replication in macrophages and survival in mammalian hosts. Sci. Rep. 7:44420. doi: 10.1038/srep44420
Allard, N., Garneau, D., Poulin-Laprade, D., Burrus, V., Brzezinski, R., and Roy, S. (2015). A diaminopimelic acid auxotrophic Escherichia coli donor provides improved counterselection following intergeneric conjugation with actinomycetes. Can. J. Microbiol. 61, 565–574. doi: 10.1139/cjm-2015-0041
Alton, G. G., Jones, L. M., Angus, R. D., and Verger, J.-M. (1988). Techniques for the Brucellosis Laboratory. Paris: INRA.
Aragón-Aranda, B., de Miguel, M. J., Lázaro-Antón, L., Salvador-Bescós, M., Zúñiga-Ripa, A., Moriyón, I., et al. (2020). Development of attenuated live vaccine candidates against swine brucellosis in a non-zoonotic B. suis biovar 2 background. Vet. Res. 51:92. doi: 10.1186/s13567-020-00815-8
Barbier, T., Zúñiga-Ripa, A., Moussa, S., Plovier, H., Sternon, J. F., Lázaro-Antón, L., et al. (2017). Brucella central carbon metabolism: an update. Crit. Rev. Microbiol. 44, 182–211.
Barquero-Calvo, E., Chaves-Olarte, E., Weiss, D. S., Guzmán-Verri, C., Chacón-Díaz, C., Rucavado, A., et al. (2007). Brucella abortus uses a stealthy strategy to avoid activation of the innate immune system during the onset of infection. PLoS One 2:e631. doi: 10.1371/journal.pone.0000631
Barquero-Calvo, E., Conde-Alvarez, R., Chacón-Díaz, C., Quesada-Lobo, L., Martirosyan, A., Guzmán-Verri, C., et al. (2009). The differential interaction of Brucella and Ochrobactrum with innate immunity reveals traits related to the evolution of stealthy pathogens. PLoS One 4:e5893. doi: 10.1371/journal.pone.0005893
Bligh, E. M., and Dyer, W. J. (1959). Canadian Journal of Biochemistry and Physiology. Can. J. Biochem. Physiol. 37, 911–917.
Choi, K.-H., Gaynor, J. B., White, K. G., Lopez, C., Bosio, C. M., Karkhoff-Schweizer, R. R., et al. (2005). A Tn7-based broad-range bacterial cloning and expression system. Nat. Methods 2, 443–448.
Comerci, D. J., Altabe, S., De Mendoza, D., and Ugalde, R. A. (2006). Brucella abortus synthesizes phosphatidylcholine from choline provided by the host. J. Bacteriol. 188, 1929–1934.
Conde-Álvarez, R., Grilló, M. J., Salcedo, S. P., de Miguel, M. J., Fugier, E., Gorvel, J. P., et al. (2006). Synthesis of phosphatidylcholine, a typical eukaryotic phospholipid, is necessary for full virulence of the intracellular bacterial parasite Brucella abortus. Cell Microbiol. 8, 1322–1335. doi: 10.1111/j.1462-5822.2006.00712.x
Conde-Álvarez, R., Palacios-Chaves, L., Gil-Ramírez, Y., Salvador-Bescós, M., Bárcena-Varela, M., Aragón-Aranda, B., et al. (2018). Identification of lptA, lpxE, and lpxO, three genes involved in the remodeling of Brucella cell envelope. Front. Microbiol. 8:2657. doi: 10.3389/fmicb.2017.02657
de Rudder, K. E. E., López-Lara, I. M., and Geiger, O. (2000). Inactivation of the gene for phospholipid N -methyltransferase in Sinorhizobium meliloti?: phosphatidylcholine is required for normal growth. Mol. Microbiol. 37, 763–772. doi: 10.1046/j.1365-2958.2000.02032.x
Dupont, L., Garcia, I., Poggi, M.-C., Alloing, G., Mandon, K., and Le Rudulier, D. (2004). The Sinorhizobium meliloti ABC transporter Cho is highly specific for choline and expressed in bacteroids from Medicago sativa nodules. J. Bacteriol. 186, 5988–5996. doi: 10.1128/JB.186.18.5988-5996.2004
EFSA (2009). Opinion of the panel of Animal Health and Welfare (AHAW) on a request from the commission on porcine brucellosis (Brucella suis). EFSA J. 1144, 1–12.
Figurski, D. H., and Helinski, D. R. (1979). Replication of an origin-containing derivative of plasmid RK2 dependent on a plasmid function provided in trans. Proc. Natl. Acad. Sci.U.S.A. 76, 1648–1652. doi: 10.1073/pnas.76.4.1648
Gamazo, C., and Moriyon, I. (1987). Release of outer membrane fragments by exponentially growing Brucella melitensis cells. Infect. Immun. 55, 609–615.
Garin-Bastuji, B., Hars, J., Calvez, D., Thiebaud, M., and Artois, M. (2000). Brucellosis of domestic pigs. Re-emergence of Brucella suis biovar 2 in France. Epidemiol. Sante Anim. 38, 1–5.
Garin-Bastuji, B., Vaillant, V., Albert, D., Tourrand, B., Danjean, M., Lagier, A., et al. (2006). “Is brucellosis due the biovar 2 of Brucella suis an emerging zoonosis in France? Two case reports in wild boar and hare hunters,” in Proceedings of the International Social Chemistry Disease Management Meeting, 1st International Meeting on Treatment of Human Brucellosis Loannina.
Geiger, O., López-Lara, I. M., and Sohlenkamp, C. (2013). Phosphatidylcholine biosynthesis and function in bacteria. Biochim. Biophys. Acta Mol. Cell Biol. Lipids 1831, 503–513. doi: 10.1016/j.bbalip.2012.08.009
Grilló, M. J., Blasco, J. M., Gorvel, J. P., Moriyón, I., and Moreno, E. (2012). What have we learned from brucellosis in the mouse model? Vet. Res. 43:29.
Guijas, C., Meana, C., Astudillo, A. M., Balboa, M. A., and Balsinde, J. (2016). Foamy monocytes are enriched in cis -7-hexadecenoic fatty acid (16:1n-9), a possible biomarker for early detection of cardiovascular disease. Cell Chem. Biol. 23, 689–699. doi: 10.1016/j.chembiol.2016.04.012
Hacker, S., Sohlenkamp, C., Aktas, M., Geiger, O., and Narberhaus, F. (2008). Multiple phospholipid N-methyltransferases with distinct substrate specificities are encoded in Bradyrhizobium japonicum. J. Bacteriol. 190, 571–580. doi: 10.1128/JB.01423-07
Herrmann, C. K., Bukata, L., Melli, L., Marchesini, M. I., Caramelo, J. J., and Comerci, D. J. (2013). Identification and characterization of a high-affinity choline uptake system of Brucella abortus. J. Bacteriol. 195, 493–501. doi: 10.1128/JB.01929-12
Herrou, J., Willett, J. W., Czyż, D. M., Babnigg, G., Kim, Y., and Crosson, S. (2017). Conserved ABC transport system regulated by the general stress response pathways of Alpha- and Gammaproteobacteria. J. Bacteriol. 199, 746–762. doi: 10.1128/JB.00746-16
Higgins, J. A., and Fieldsend, J. K. (1987). Phosphatidylcholine synthesis for incorporation into membranes or for secretion as plasma lipoproteins by Golgi membranes of rat liver. J. Lipid Res. 28, 268–278.
Kerrinnes, T., Young, B. M., Leon, C., Roux, C. M., Tran, L., Atluri, V. L., et al. (2015). A Phospholipase A1 modulates cell envelope phospholipid content of Brucella melitensis, contributing to polymyxin resistance and pathogenicity. Antimicrob. Agents Chemother. 59, 6717–6724. doi: 10.1128/AAC.00792-15
Kulikov, V. I., and Dranovskaia, E. A. (1988). Fosfolipidy bakterii roda Brucella. Mol. Gen..Mikrobiol. Virusol. 17–21. Available online at: https://pubmed.ncbi.nlm.nih.gov/3211184/
Lázaro-Antón, L., De Miguel, M. J., Barbier, T., Conde-Álvarez, R., Muñoz, P. M., Letesson, J.-J., et al. (2021). Glucose oxidation to pyruvate is not essential for Brucella suis biovar 5 virulence in the mouse model. Front. Microbiol. 11:620049. doi: 10.3389/fmicb.2020.620049
Llobet, E., March, C., Giménez, P., and Bengoechea, J. A. (2009). Klebsiella Pneumoniae OmpA confers resistance to antimicrobial peptides. Antimicrob. Agents Chemother. 53, 298–302.
Machelart, A., Willemart, K., Zúñiga-Ripa, A., Godard, T., Plovier, H., Wittmann, C., et al. (2020). Convergent evolution of zoonotic Brucella species toward the selective use of the pentose phosphate pathway. Proc. Natl. Acad. Sci.U.S.A. 117, 26374–26381. doi: 10.1073/PNAS.2008939117
Martínez-Gómez, E., Ståhle, J., Gil-Ramírez, Y., Zúñiga-Ripa, A., Zaccheus, M., Moriyón, I., et al. (2018). Genomic insertion of a heterologous acetyltransferase generates a new lipopolysaccharideantigenic structure in Brucella abortus and Brucella melitensis. Front. Microbiol. 9:1092.
Martínez-Morales, F., Schobert, M., López-Lara, I. M., and Geiger, O. (2003). Pathways for phosphatidylcholine biosynthesis in bacteria. Microbiology 149, 3461–3471. doi: 10.1099/mic.0.26522-0
Martirosyan, A., Moreno, E., and Gorvel, J.-P. (2011). An evolutionary strategy for a stealthy intracellular Brucella pathogen. Immunol. Rev. 240, 211–234. doi: 10.1111/j.1600-065X.2010.00982.x
Minder, A. C., De Rudder, K. E. E., Narberhaus, F., Fischer, H.-M., Hennecke, H., and Geiger, O. (2004). Phosphatidylcholine levels in Bradyrhizobium japonicum membranes are critical for an efficient symbiosis with the soybean host plant. Mol. Microbiol. 39, 1186–1198. doi: 10.1111/j.1365-2958.2001.02325.x
Moreno, E. (1992). “Evolution of Brucella,” in Prevention of Brucellosis in the Mediterranean Countries, ed. M. Plommet (Wageningen: Pudoc Science. Publishing), 198–218.
Moreno, E. (2014). Retrospective and prospective perspectives on zoonotic brucellosis. Front. Microbiol 5:213. doi: 10.3389/fmicb.2014.00213
Moreno, E. (2021). The one hundred year journey of the genus Brucella (Meyer and Shaw 1920). FEMS Microbiol. Rev. 45:fuaa045.
Moreno, E., Cloeckaert, A., and Moriyón, I. (2002). Brucella evolution and taxonomy. Vet. Microbiol. 90, 209–227. doi: 10.1016/S0378-1135(02)00210-9
Muñoz, P. M., Boadella, M., Arnal, M., de Miguel, M. J., Revilla, M., Martínez, D., et al. (2010). Spatial distribution and risk factors of Brucellosis in Iberian wild ungulates. BMC Infect. Dis. 10:46. doi: 10.1186/1471-2334-10-46
Oswald, C., Smits, S. H. J., Höing, M., Sohn-Bösser, L., Dupont, L., Le Rudulier, D., et al. (2008). Crystal structures of the choline/acetylcholine substrate-binding protein ChoX from Sinorhizobium meliloti in the liganded and unliganded-closed states. J. Biol. Chem. 283, 32848–32859. doi: 10.1074/jbc.M806021200
Palacios-Chaves, L., Conde-Álvarez, R., Gil-Ramírez, Y., Zúñiga-Ripa, A., Barquero-Calvo, E., Chacón-Díaz, C., et al. (2011). Brucella abortus ornithine lipids are dispensable outer membrane components devoid of a marked pathogen-associated molecular pattern. PLoS One 6:e16030. doi: 10.1371/journal.pone.0016030
Parent, M. A., Goenka, R., Murphy, E., LeVier, K., Carreiro, N., Golding, B., et al. (2007). Brucella abortus bacA mutant induces greater pro-inflammatory cytokines than the wild-type parent strain. Microb. Infect. 9, 55–62. doi: 10.1016/j.micinf.2006.10.008
Scholz, H. C., Hubalek, Z., Sedlacek, I., Vergnaud, G., Tomaso, H., Al Dahouk, S., et al. (2008). Brucella microti sp. nov., isolated from the common vole Microtus arvalis. Int. J. Syst. Evol. Microbiol. 58, 375–382. doi: 10.1099/ijs.0.65356-0
Scholz, H. C., Nö, K., Gö, C., Bahn, P., Vergnaud, G., Tomaso, H., et al. (2010). Brucella inopinata sp. nov., isolated from a breast implant infection. Int. J. Syst. Evol. Microbiol. 60, 801–808. doi: 10.1099/ijs.0.011148-0
Scholz, H. C., Revilla-Ferná, S., Al Dahouk, S., Hammerl, J. A., Zygmunt, M. S., Cloeckaert, A., et al. (2016). Brucella vulpis sp. nov., isolated from mandibular lymph nodes of red foxes (Vulpes vulpes). Int. J. Syst. Evol. Microbiol. 66, 2090–2098. doi: 10.1099/ijsem.0.000998
Scupham, A. J., and Triplett, E. W. (1997). Isolation and characterization of the UDP-glucose 4′-epimerase-encoding gene, galE, from Brucella abortus 2308. Gene 202, 53–59. doi: 10.1016/S0378-1119(97)00453-8
Sohlenkamp, C., de Rudder, K. E. E., Röhrs, V., López-Lara, I. M., and Geiger, O. (2000). Cloning and characterization of the gene for phosphatidylcholine synthase. J. Biol. Chem. 275, 18919–18925. doi: 10.1074/jbc.M000844200
Sohlenkamp, C., López-Lara, I. M., and Geiger, O. (2003). Biosynthesis of phosphatidylcholine in bacteria. Prog. Lipid Res. 42, 115–162. doi: 10.1016/S0163-7827(02)00050-4
Soler-Lloréns, P. F., Quance, C. R., Lawhon, S. D., Stuber, T. P., Edwards, J. F., Ficht, T. A., et al. (2016). A Brucella spp. isolate from a Pac-Man Frog (Ceratophrys ornata) reveals characteristics departing from classical Brucellae. Front. Cell. Infect. Microbiol. 6:116. doi: 10.3389/fcimb.2016.00116
Suárez-Esquivel, M., Ruiz-Villalobos, N., Jiménez-Rojas, C., Barquero-Calvo, E., Chacón-Díaz, C., Víquez-Ruiz, E., et al. (2017). Brucella neotomae infection in humans. Costa Rica. Emerg Infect. Dis. 23, 997–1000.
Vizcaíno, N., and Cloeckaert, A. (2012). “Biology and genetics of the Brucella outer membrane,” in Brucella. Molecular Microbiology and Genomics, eds D. López-Goñi and I. O’Callaghan (Norfolk: Caister Academic Press), 133–161.
Wattam, A. R., Foster, J. T., Mane, S. P., Beckstrom-Sternberg, S. M., Beckstrom-Sternberg, J. M., Dickerman, A. W., et al. (2014). Comparative phylogenomics and evolution of the brucellae reveal a path to virulence. J. Bacteriol. 196, 920–930. doi: 10.1128/JB.01091-13
Wattam, A. R., Inzana, T. J., Williams, K. P., Mane, S. P., Shukla, M., Almeida, N. F., et al. (2012). Comparative genomics of early-diverging Brucella strains reveals a novel lipopolysaccharide biosynthesis pathway. MBio 3, e246–e212. doi: 10.1128/mBio.00246-12
Whatmore, A. M. (2009). Current understanding of the genetic diversity of Brucella, an expanding genus of zoonotic pathogens. Infect. Genet. Evol. 9, 1168–1184. doi: 10.1016/j.meegid.2009.07.001
Whatmore, A. M., Davison, N., Cloeckaert, A., Al Dahouk, S., Zygmunt, M. S., Brew, S. D., et al. (2014). Brucella papionis sp. nov., isolated from baboons (Papio spp.). Int. J. Syst. Evol. Microbiol. 64, 4120–4128. doi: 10.1099/ijs.0.065482-0
Zheludkov, M. M., and Tsirelson, L. (2010). Reservoirs of Brucella infection in nature. Biol. Bull. 37, 709–715.
Zúñiga-Ripa, A., Barbier, T., Lázaro-Antón, L., de Miguel, M. J., Conde-Álvarez, R., Muñoz, P. M., et al. (2018). The fast-growing Brucella suis biovar 5 depends on phosphoenolpyruvate carboxykinase and pyruvate phosphate dikinase but not on Fbp and GlpX fructose-1,6-bisphosphatases or isocitrate lyase for full virulence in laboratory models. Front. Microbiol. 9:641. doi: 10.3389/fmicb.2018.00641
Keywords: brucella, phospholipid, phosphatidylcholine, choline, cell envelope
Citation: Aragón-Aranda B, Palacios-Chaves L, Salvador-Bescós M, de Miguel MJ, Muñoz PM, Vences-Guzmán MÁ, Zúñiga-Ripa A, Lázaro-Antón L, Sohlenkamp C, Moriyón I, Iriarte M and Conde-Álvarez R (2021) The Phospholipid N-Methyltransferase and Phosphatidylcholine Synthase Pathways and the ChoXWV Choline Uptake System Involved in Phosphatidylcholine Synthesis Are Widely Conserved in Most, but Not All Brucella Species. Front. Microbiol. 12:614243. doi: 10.3389/fmicb.2021.614243
Received: 05 October 2020; Accepted: 09 July 2021;
Published: 04 August 2021.
Edited by:
François J. M. A. Meurens, INRA Ecole Nationale Vétérinaire, Agroalimentaire et de l’Alimentation de Nantes-Atlantique (Oniris), FranceReviewed by:
Juan Ugalde, National University of General San Martín, ArgentinaDiego J. Comerci, CONICET Institute of Biotechnological Research (IIB-INTECH), Argentina
Clayton Caswell, Virginia Tech, United States
Neeraj Chauhan, Rutgers Biomedical and Health Sciences, United States
George Carman, Rutgers, The State University of New Jersey, United States
Copyright © 2021 Aragón-Aranda, Palacios-Chaves, Salvador-Bescós, de Miguel, Muñoz, Vences-Guzmán, Zúñiga-Ripa, Lázaro-Antón, Sohlenkamp, Moriyón, Iriarte and Conde-Álvarez. This is an open-access article distributed under the terms of the Creative Commons Attribution License (CC BY). The use, distribution or reproduction in other forums is permitted, provided the original author(s) and the copyright owner(s) are credited and that the original publication in this journal is cited, in accordance with accepted academic practice. No use, distribution or reproduction is permitted which does not comply with these terms.
*Correspondence: Raquel Conde-Álvarez, rconde@unav.es