- State Key Laboratory of Veterinary Etiological Biology, Lanzhou Veterinary Research Institute, Chinese Academy of Agricultural Sciences, Lanzhou, China
Although it has been reported that deletion of the response regulator, CpxR, in the CpxRA system confers sensitivity to aminoglycosides (AGAs) and β-lactams in Salmonella enterica serovar Typhimurium, the regulatory effects of CpxA on multidrug resistance (MDR) are yet to be fully investigated in this organism. Here, to explore the role of CpxA in MDR, various cpxA mutants including a null mutant (JSΔcpxA), a site-directed mutant (JSΔcpxA38) and an internal in-frame deletion mutant (JSΔcpxA92–104) of the S. enterica serovar Typhimurium strain JS, were constructed. It was revealed that cpxA and cpxR deletion mutants have opposing roles in the regulation of resistance to AGAs and β-lactams. Amikacin and cefuroxime can activate the CpxRA system, which results in increased resistance of the wild-type compared with the cpxR deletion mutant. All the cpxA mutations significantly increased resistance to AGAs and β-lactams due to CpxRA system activation via the phosphorylation of CpxR. Moreover, AckA-Pta-dependent activation of CpxR increased the antibiotic resistance of cpxA deletion mutants. Further research revealed that the AcrAB-TolC conferred resistance to some AGAs and β-lactams but does not influence the regulation of resistance by CpxRA against these antibiotics. The detection of candidate MDR-related CpxR regulons revealed that the mRNA expression levels of spy, ycca, ppia, htpX, stm3031, and acrD were upregulated and that of ompW was downregulated in various cpxA mutants. Furthermore, the expression levels of nuoA and sdhC mRNAs were downregulated only in JSΔcpxA92–104. These results suggested that cpxA mutations contribute to AGAs and β-lactams resistance, which is dependent on CpxR.
Introduction
With the overuse and misuse of antibacterial agents, the emergence and spread of multidrug resistant (MDR) Salmonella enterica serovar Typhimurium (S. Typhimurium) has become a clinical and public health threat worldwide (Levy, 2002; Quinn et al., 2006). To adapt to adverse environmental conditions, bacteria have developed many sophisticated signal transduction systems, referred to as two-component systems (TCSs). These systems sense changes in environmental pH, ion concentrations, nutrient levels, quorum signals, and antibiotic concentrations and, in response, regulate the expression of genes involved in processes such as cell growth, virulence, biofilm formation, quorum sensing, and antibiotic resistance (De la Cruz et al., 2015; Padilla-Vaca et al., 2017; Tiwari et al., 2017; Fujimoto et al., 2018). TCSs have been identified as potential drug targets. Unlike conventional antibiotics, these drugs would likely be effective against various drug-resistant bacteria and avoid the emergence of resistant strains (Gotoh et al., 2010; Tiwari et al., 2017). Understanding the regulation mechanisms of TCSs to conventional antibiotic resistance would be beneficial for the reasonable application and low occurrence of drug resistance to these TCSs-targeted antibacterial agents.
The CpxRA system is an important TCS in Escherichia coli and Salmonella. It consists of an inner membrane-bound histidine sensor kinase, CpxA, and a cytoplasmic response regulator, CpxR (De Wulf et al., 2002). CpxP is a periplasmic chaperone that binds to CpxA and inhibits its kinase activity. The periplasmic protease DegP can disrupt CpxP binding to misfolded proteins (Isaac et al., 2005). Upon sensing extracytoplasmic stresses, CpxP binds to misfolded proteins, dissociates from CpxA, and is degraded by DegP, and then CpxA autophosphorylates and donates its phosphate group to CpxR at D51 (D51). This allows phosphorylated CpxR (CpxR-P) containing a specific DNA-binding site (M199) to bind to the promoter regions of target genes at the consensus sequence (GTAAAN5GTAAA) (De Wulf et al., 2002; DiGiuseppe and Silhavy, 2003), and to regulate a series of target genes associated with antibiotic resistance and pathogenesis (Nishino et al., 2010; Dbeibo et al., 2018). cpxA knock-out mutation can result in constitutive activation of the CpxRA system. This is because cpxA deletion abolishes its phosphatase activity for CpxR-P, CpxR then accepts a phosphoryl group from acetyl phosphate, the product of the phosphotransacetylase (Pta)-acetate kinase (AckA) pathway (Wolfe et al., 2008). Similarly, a strain containing the mutant cpxA gene (cpxA∗) encoding a CpxA variant lacking several amino acids in the periplasmic loop, exhibits constitutive CpxRA system activity. This occurs because the mutant CpxA∗ protein still functions as an autokinase and CpxR kinase but lacks phosphatase activity, resulting in accumulation of CpxR-P and hyperactivation of the CpxRA response (Raivio and Silhavy, 1997).
The CpxRA envelope stress response system is closely related to drug resistance. In E. coli, cpxA deletion or cpxA∗ mutations will confer the resistance to hydroxyurea, β-lactams, aminoglycosides, and fosfomycin (Mahoney and Silhavy, 2013; Masi et al., 2020). Moreover, overexpression of cpxR can confer the resistance to kanamycin, amikacin, deoxycholate, novobiocin, and β-lactams (Hirakawa et al., 2003a,b). In S. Typhimurium, the cpxRA deletion confers susceptibility to ceftriaxone by influencing the expression of stm1530 and ompD (Hu et al., 2011), and overexpression of cpxR in the cpxR deletion mutant increases resistance to AGAs and β-lactams as a result of the downregulation of outer membrane protein gene expression (Huang et al., 2016). However, the regulatory effect of CpxA on MDR has not been fully investigated. One study reported that both the cpxR and cpxA mutants were more sensitive to amikacin than the wild-type strain, but the cpxA∗ mutation renders S. Typhimurium more resistant to the antibiotic amikacin (Humphreys et al., 2004), which suggests that different mutant versions of the CpxA sensor protein exhibit unexpected resistance regulation in S. Typhimurium. Another study reported that the various activation pathways of the CpxRA system determine different sensitivities to β-lactams (Delhaye et al., 2016). Therefore, the various mutations of cpxA should be analyzed in concert to understand the full extent of the effects of CpxA on MDR. In this study, we constructed different mutants of the cpxA gene to systematically analyze the regulatory effects of CpxA on AGAs and β-lactams resistance and explored the related molecular mechanism. The results suggested that various cpxA mutations can activate the CpxRA system and confer resistance to AGAs and β-lactams.
Materials and Methods
Bacterial Strains, Plasmids, and Culture Conditions
The bacterial strains and plasmids used in this study are listed in Table 1. All bacterial strains were mutant derivatives of S. enterica serovar Typhimurium strain JS. All strains were cultured aerobically in Luria–Bertani (LB) medium [1% (w/v) tryptone, 0.5% (w/v) yeast extract, and 1% (w/v) NaCl, pH = 7.0] (Takara Bio., Kusatsu, Japan) at 37°C. When necessary, LB medium were supplemented with ampicillin (100 μg/ml), kanamycin (50 μg/ml), chloramphenicol (25 μg/ml), or sucrose (8%, w/v).
Construction of Various Mutants of S. Typhimurium
All deletion mutants were generated via the Red recombinase system, as reported previously (Datsenko and Wanner, 2000). The respective primers are shown in Supplementary Table 1. The genes cpxA, cpxR, cpxRA, ackA-pta, acrB, and tolC were replaced with a kanamycin (kan) resistance cassette in the S. Typhimurium strain CVCC541, generating mutant strains JSΔcpxA::kan, JSΔcpxR::kan, JSΔcpxRA:kan, JSΔackA-pta::kan, JSΔacrB::kan, and JSΔtolC::kan, respectively. Using helper plasmid pCP20, expressing the FLP recombinase, the kan resistance cassette was excised from the JSΔcpxA::kan, JSΔcpxR::kan, JSΔcpxRA::kan, JSΔackA-pta::kan, JSΔacrB::kan, and JSΔtolC::kan mutants, generating mutant strains JSΔcpxA, JSΔcpxR, JSΔcpxRA, JSΔackA-pta, JSΔacrB, and JSΔtolC, respectively. Multiple additional mutants were then constructed from these mutants using the Red recombinase system. From the JSΔcpxA mutant, additional mutant strains JSΔcpxAΔackA-pta, JSΔcpxAΔacrB, and JSΔcpxAΔtolC were constructed. From the JSΔcpxR strain, additional mutants JSΔcpxRΔacrB and JSΔcpxRΔtolC were constructed. The main regulon member deletion mutants JSΔstm3031::Kan, JSΔhtpX::Kan, and JSΔspy::Kan were constructed from the wild-strain JS, and JSΔcpxAΔstm3031::Kan, JSΔcpxAΔhtpX::Kan, and JSΔcpxAΔspy::Kan were constructed from the JSΔcpxA mutant. The mutants JSΔacrD::Kan, JSΔcpxAΔacrD::Kan, JSΔacrBΔacrD::Kan, and JSΔcpxAΔacrBΔacrD::Kan were constructed from the corresponding strain JS, JSΔcpxA, JSΔacrB, and JSΔcpxAΔacrB.
The JScpxRD51A, JScpxRM199A, JScpxA38, and JScpxA92–104 mutants were constructed in our previous study (Jing et al., 2020). Subsequently, mutant strains JSΔcpxAcpxRD51A, JSΔcpxAcpxRM199A, JSΔcpxA38cpxR::kan, and JSΔcpxA92–104cpxR::kan were constructed from mutant strains JScpxRD51A, JScpxRM199A, JScpxA38, and JScpxA92–104, respectively, using the Red recombinase system.
Complementation of Various Mutants
To be able to construct the complemented strains of various cpxA mutants (JSΔcpxA, JScpxA38, and JScpxA92–104), a strategy to construct the complemented strain in the bacterial chromosome was used according to our previous study (Jing et al., 2020). In order to distinguish cpxA complemented strains from wild strains, we changed the terminate code “UAA” of the cpxA gene in the wild strain to “UAG” in the cpxA complemented strains. First, the sacBKan fragment was amplified from plasmid psacBKan using the primers CpxA-H1P1/H2P2, and then integrated into the corresponding cpxA mutations locus using the Red recombinase system to obtain the intermediate strain. Positive clones were screened by PCR using primer pairs CpxA-F/R, CpxA-F/sacBKan-k2, sacBKan-k1/CpxA-R, and sacBKan-k1/k2. Then, using primers CpxA-UF/CpxA-CL-UR and CpxA-CL-DF/CpxA-DR, the wild-type cpxA fragment with synonymous substitution of terminates codon was prepared on the BamHI-digested vector pSTV28. The recombinogenic cpxA fragment was amplified using primer pair CpxA∗-F/R. Finally, the recombinogenic cpxA fragment replaced the sacBKan cassette and the corresponding cpxA complemented strains (JSΔcpxA-CL, JScpxA38-CL, and JScpxA92–104-CL) were confirmed by PCR.
The acrB and tolC complemented strains in the chromosome were constructed using the λ Red recombination system (Datsenko and Wanner, 2000). The corresponding PCR products were obtained using primer pairs AcrB-CL-F/AcrB-CL-R and TolC-CL-F/TolC-CL-F. To distinguish acrB complemented strains from wild strains, we changed the 884th codon “GTC” of the acrB gene in the wild strain to “GTG” in the acrB complemented strains. To distinguish tolC complemented strains from wild strains, we changed the terminate code “UGA” of the tolC gene in the wild strain to “UAA” in the tolC complemented strains. The corresponding PCR products were then used to replace the deletion mutations using the λ Red recombinase system. The complemented strains (JSΔacrB-CL and JSΔtolC-CL) were selected on LB agar plates containing cefuroxime (1 μg/ml) and confirmed by Sanger sequencing.
Real-Time Quantitative PCR (qPCR) Analysis of Bacterial Gene Expression
Total RNA was isolated from bacterial cultures (OD600 = 1.0) using a TransZol UP Plus RNA Kit (TransGen Biotech, Beijing, China) according to the manufacturer’s instructions. Aliquots (1 μg) of the total RNA were then transcribed into cDNA using a PrimeScript RT Reagent Kit with gDNA Eraser (Takara Bio., Kusatsu, Japan). Quantitative PCR (qPCR) assays were performed using the CFX96 Real-Time System and a C1000 Touch Thermal Cycler (Bio-Rad, Hercules, CA, United States), as well as a QuantiNova SYBR Green PCR Kit (Qiagen, Hilden, Germany). The primers used for qPCR analysis are outlined in Supplementary Table 1. The expression levels of all genes were normalized against that of internal reference gene rpoD, and relative fold changes in expression were calculated using the 2–ΔΔCT method. The expression level of each mRNA in strain JS represents a one-fold change.
Antibiotic-Induced Activation Assays
Briefly, the test strains were grown overnight in LB medium, diluted 1:100, and subcultured to the mid-exponential phase (OD600 of 0.4 to 0.6). When we used LB medium for the antibiotic activation test, it was found that the MICs values of the JS strain against amikacin and cefuroxime was 16 and 8 μg/ml in LB, respectively. So, to observe the relationship between the concentration of amikacin and cefuroxime with the activation level of the CpxRA system, the S. Typhimurium wild-type strain was grown in LB medium or LB medium with 4, 8, or 16 μg/ml amikacin or 1, 2, or 4 μg/ml cefuroxime to a stationary growth phase (OD600 ≥ 1.0). To observe the influence of ΔcpxA or ΔcpxR on induction of amikacin and cefuroxime, the wild-type, ΔcpxA, and ΔcpxR cells were grown in LB medium with 16 μg/ml amikacin or 4 μg/ml cefuroxime to a stationary growth phase (OD600 ≥ 1.0). The mRNA expression levels of the target genes cpxP and degP were used to measure the activation level of the CpxRA system.
Antibiotic Susceptibility Testing
The MIC values of selected antibiotics against the various bacterial strains were measured in Mueller-Hinton (MH) medium (Hopebio, Qingdao, China) via the broth microdilution method as per the Clinical and Laboratory Standards Institute’s (CLSI) guidelines (Cockerill et al., 2012). The tested antibiotics included amikacin (AMK), netilmicin (NET), streptomycin (STR), kanamycin (KAN), cefalotin (CEP), cefuroxime (CXM), ceftriaxone (CRO), and cefotaxime (CTX). All assays were conducted in triplicate.
Statistical Analysis
Statistical analyses were performed using GraphPad Prism 6.0 Windows software and data were compared using the Student’s t-test (GraphPad Software, United States). A probability (p) value of <0.05 was considered statistically significant.
Results
cpxA and cpxR Deletion Mutants Have Opposing Roles in the Regulation of Resistance to AGAs and β-Lactams
As described in the introduction, cpxA deletion mutations sometimes show different resistance to amikacin. To investigate the effects of cpxA deletion mutations on the regulation of drug resistance, cpxA and cpxR deletion mutants – JSΔcpxA and JSΔcpxR – were generated from the S. Typhimurium strain JS. The complemented strain JSΔcpxA-CL was also prepared. As shown in Table 2, compared with parental strain JS, two- to eight-fold increases in the MICs of the tested AGA and β-lactam antibiotics against the cpxA null mutant were observed; whereas two- to eight-fold decreases in the MICs of the tested AGA and β-lactam antibiotics against the cpxR deletion mutant were observed. The MICs of AGA and β-lactam antibiotics decreased by two- to eight-fold for the complemented strain JSΔcpxA-CL, as compared to those for JSΔcpxA. These results indicated that deletions of cpxA and cpxR have opposing roles in the regulation of AGAs and β-lactams resistance.
AGAs and β-Lactams Activate the CpxRA System
To evaluate whether the CpxRA system is activated by AGAs and β-lactams, a series of concentrations of amikacin (4, 8, or 16 μg/ml) and cefuroxime (1, 2, or 4 μg/ml) were added to the culture medium. The expression levels of cpxP and degP, two target genes of the CpxRA system (Fujimoto et al., 2018), were measured using a qRT-PCR assay to evaluate the activation level of the CpxRA system of the wild-type strain JS in the presence or absence of the corresponding antimicrobial. As shown in Figures 1A,B, the transcription levels of both cpxP and degP increased with increasing amikacin and cefuroxime concentrations. Whereas deletion of cpxR resulted in significantly reduced expression levels of cpxP and degP in the presence of amikacin and cefuroxime, compared with the wild-type strain JS (Figures 1C,D). The deletion of cpxA resulted in significantly increased expression levels of cpxP in presence of cefuroxime and amikacin, increased expression levels of degP in presence of cefuroxime, and no significantly different expression levels of degP in presence of amikacin (Figures 1C,D), compared with the wild-type strain JS. These results suggest that AGAs and β-lactams activate the S. Typhimurium CpxRA system dependent on CpxR; however, cpxA deletion can activate the CpxRA system independently of amikacin and cefuroxime.
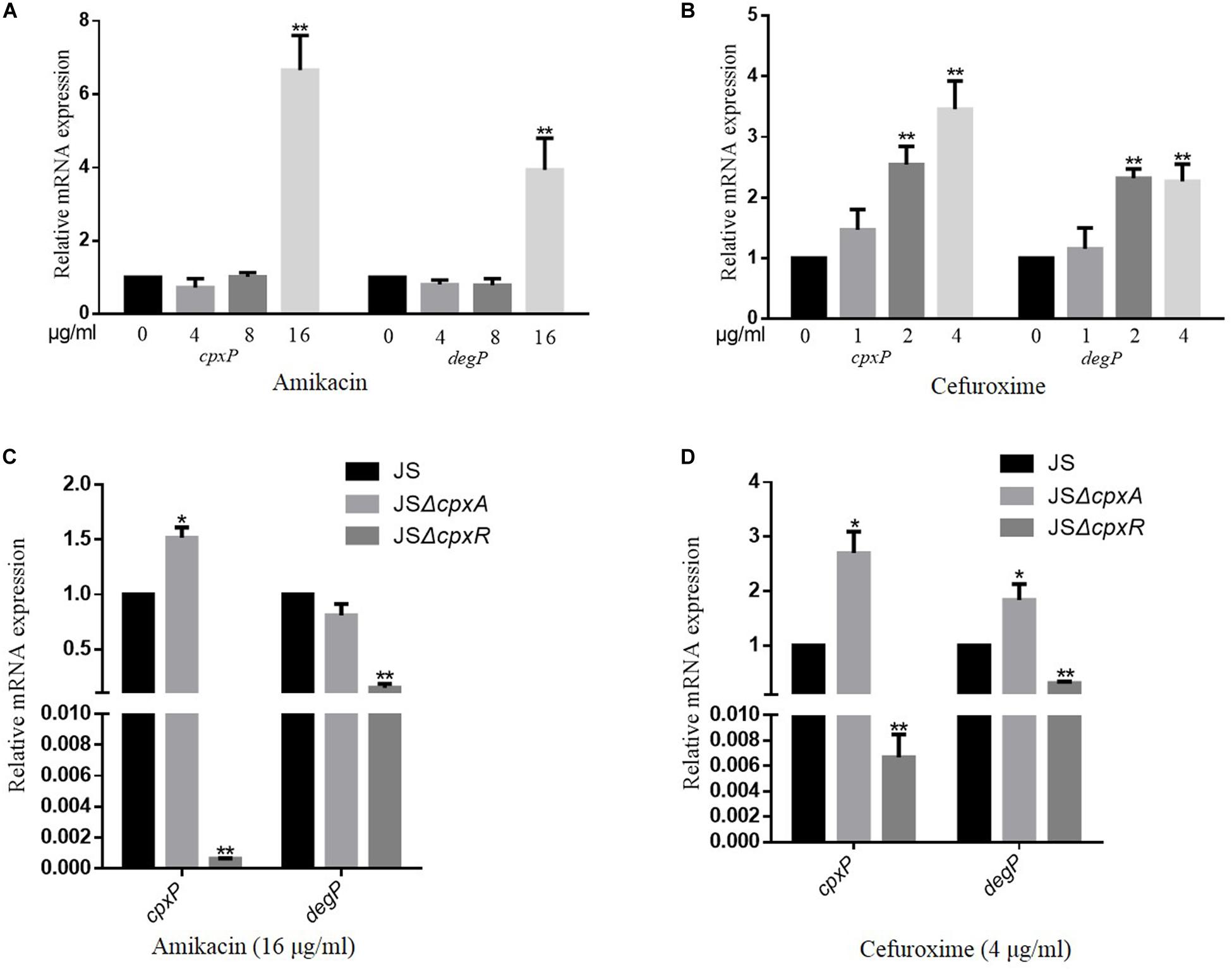
Figure 1. CpxRA is activated by amikacin and cefuroxime. (A,B) S. Typhimurium wild-type strains were grown in LB medium or LB medium with 4, 8, and 16 μg/ml amikacin or 1, 2, and 4 μg/ml cefuroxime to the stationary growth phase (OD600 ≥ 1.0), and mRNA levels of CpxRA-regulated genes (cpxP and degP) were measured by reverse transcription-qPCR. (C,D) S. Typhimurium wild-type strains, the JSΔcpxA, or the JSΔcpxR mutant strain were grown in LB medium with 16 μg/ml amikacin or 4 μg/ml cefuroxime to the stationary growth phase (OD600 ≥ 1.0), and mRNA levels cpxP and degP were measured by reverse transcription-qPCR. Data were normalized to rpoD expression levels. Bars represent means ± standard deviations. *, P < 0.05; **, P < 0.01.
AckA-Pta-Dependent Activation of CpxR Increases Antibiotic Resistance
To investigate the underlying genetic basis of the increased resistance of the cpxA deletion mutant to AGAs and β-lactams, the first step was to confirm whether the increased resistance of the cpxA null mutant was caused by pleiotropic effects, given that CpxA can regulate downstream target genes by interacting with other regulators rather than CpxR (Nakayama et al., 2003). For this reason, mutant strain JSΔcpxRA, lacking both cpxA and cpxR, was constructed. The antibiotic sensitivity of the double mutant was equivalent to that of strain JSΔcpxR (Table 2). We also constructed point mutations in cpxR (cpxRD51A, cpxRM199A) and corresponding double mutations based on the cpxA deletion mutations (ΔcpxAcpxRD51A, ΔcpxAcpxRM199A). Compared with strain JS, two- to four-fold decreases in the MICs of the tested AGA and β-lactam antibiotics for JS mutant cpxRD51A were observed, and there was no significant change in JScpxRM199A. Compared with strain JSΔcpxA, 4- to 16-fold decreases in the MICs of the tested AGA and β-lactam antibiotics for mutant strains JSΔcpxAcpxRD51A and JSΔcpxAcpxRM199A were observed (Table 2). These results demonstrated that the observed resistance of the cpxA deletion mutant to AGAs and β-lactams is dependent on CpxR and is not caused by pleiotropic effects.
CpxA acts as an autokinase, a kinase that activates CpxR, and a phosphatase (7,8), and cpxA null mutations would therefore presumably disrupt all three of these activities. The lack of phosphatase activity leads to the phosphorylation of CpxR via alternative sources, such as acetyl phosphate produced by the AckA and Pta enzymes (14,28). To investigate whether AckA and Pta participate in the regulation of AGAs and β-lactams resistance in the cpxA deletion mutant, double deletion mutant JSΔackA-pta and triple deletion mutant JSΔcpxAΔackA-pta were constructed. Compared with JSΔackA-pta, no significant differences in the MICs of any of the tested antibiotics against strain JSΔcpxAΔackA-pta were observed. However, 2- to 16-fold decreases in the MICs of the tested antibiotics against JSΔcpxAΔackA-pta were observed compared with the MICs of JSΔcpxA (Table 2). This result suggests that the increased resistance of the cpxA null mutant to AGA and β-lactam antibiotics is associated with AckA-Pta. We then evaluated the activation of the CpxRA system by measuring the expression levels of cpxP and degP. As shown in Figure 2A, compared with the wild-type strain, significant increases in mRNA levels of both genes were observed in strain JSΔcpxA. Compared with strain JSΔcpxA, significant decreases in the expression of both genes were observed in strains JSΔcpxRA, JSΔcpxAcpxRD51A, JSΔcpxAcpxRM199A, and JSΔcpxAΔackA-pta. It has been suggested that the knock-out of cpxA could confer the resistance to the AGA and β-lactam antibiotics. This regulatory mechanism is involved in the activation of the CpxRA system via phosphorylation of CpxR by the AckA-Pta pathway.
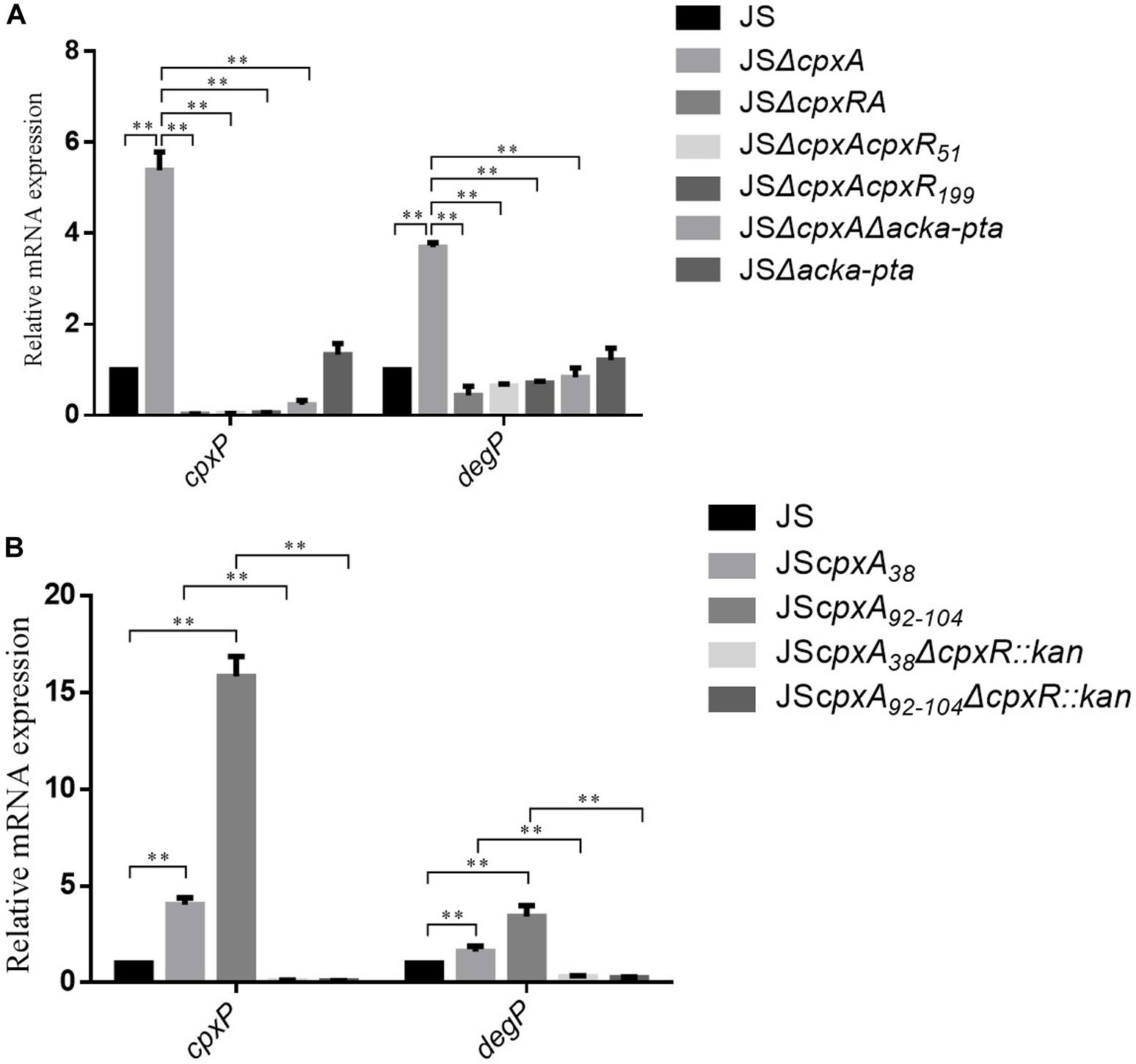
Figure 2. Relative mRNA levels of the CpxRA-regulated genes (cpxP and degP) were measured by real-time PCR. (A) Relative mRNA levels of the were measured in the WT S. Typhimurium JS strain and its isogenic JSΔcpxA, JSΔcpxRA, JSΔcpxAcpxR51, JSΔcpxAcpxR199, JSΔcpxAΔacka-pta, and JSΔacka-pta. (B) Relative mRNA levels of the were measured in the WT S. Typhimurium JS strain and its isogenic JScpxA38, JScpxA92–104, JScpxA38ΔcpxR::kan, JSΔcpxA92–104ΔcpxR::kan. The expression level of each mRNA in strain JS represents one-fold. Data were normalized to rpoD expression levels. Bars represent means ± standard deviations. **, P < 0.01.
AGAs and β-Lactams Resistance Can Be Conferred by CpxA-Mediated Ativation of CpxR
It has been confirmed that an in-frame deletion mutation that removed amino acids 92–104, or a site-directed mutation resulting in a Leu38Phe substitution in the periplasmic domain of CpxA, both constitutively activated the CpxRA system (collectively referred to as a cpx∗ mutation) (Pogliano et al., 1998; Humphreys et al., 2004). Here, to determine whether the CpxA-mediated activation of CpxR also confers AGAs and β-lactams resistance in S. Typhimurium, constitutively active mutants of cpxA (JScpxA38 and JScpxA92–104) were constructed and the MICs of AGAs and β-lactams were measured. Moreover, the corresponding complemented strains JScpxA38-CL and JScpxA92–104-CL were constructed. As shown in Table 2, the MICs of JScpxA38 and JScpxA92–104 were the same as that of the cpxA null mutant. Compared with strain JScpxA38 and JScpxA92–104, two- to eight-fold decreases in the MICs of the tested AGA and β-lactam antibiotics against the complemented strain JScpxA38-CL and JScpxA92–104-CL were observed. In the strains that do not produce cpxR (JSΔcpxA38ΔcpxR:kan, JSΔcpxA92–104ΔcpxR:kan), the MICs of all of the drugs except for kanamycin were the same as that of the cpxR deletion mutant (JSΔcpxR). As shown in Figure 2B, compared with the wild-type strain, significant increases in mRNA levels of both target genes cpxP and degP were observed in strains JScpxA38 and JScpxA92–104, whereas significant decreases in the expression of both genes were observed in strains JScpxA38ΔcpxR:kan and JScpxA92–104ΔcpxR:kan compared with strains JScpxA38 and JScpxA92–104. These results demonstrate that resistance to AGAs and β-lactams can be conferred by activated CpxA, which is dependent on the phosphorylation of CpxR.
CpxRA System Can Modulate Resistance to AGAs and β-Lactams Independent of the AcrAB-TolC Efflux Pump
Drug efflux mechanisms are ubiquitous among Gram-negative bacteria and contribute significantly to MDR. Among the mechanisms reported to date, the AcrAB-TolC efflux pump plays a significant role in resistance to various antibiotics (Baucheron et al., 2004; Du et al., 2014; Li et al., 2015). To investigate whether AcrAB-TolC affects CpxRA-mediated regulation of drug resistance, we constructed several double and triple deletion mutant strains from single mutants JSΔcpxA, JScpxA38, JScpxA92–104, JSΔcpxR, JSΔacrB, and JSΔtolC, as shown in Table 2. Compared with strain JS, 2- to 32-fold decreases in the MICs of all of the drugs except for netilmicin, kanamycin and ceftriaxone against strain JSΔacrB were observed. Meanwhile, the MICs of all the tested antibiotics against complemented strain JSΔacrB-CL were the same as strain JS. Compared with strain JSΔacrB, two- to four-fold increases in the MICs of all of the drugs against strain JSΔcpxAΔacrB, JScpxA38ΔacrB, and JSCpxA92–104ΔacrB were observed, whereas two- to four-fold decreases in the MICs of all of the drugs except for cefalotin against strain JSΔcpxRΔacrB were observed. Compared with strain JS, 2- to 32-fold decreases in the MICs of all of the drugs except for netilmicin and ceftriaxone against strain JSΔtolC were observed. Meanwhile, the MICs of all the tested antibiotics against complemented strain JSΔtolC-CL were the same as strain JS. Compared with strain JSΔtolC, two- to eight-fold increases in the MICs of all of the drugs against strains JSΔcpxAΔtolC, JScpxA38ΔtolC, and JScpxA92–104ΔtolC were observed, whereas two- to eight-fold decreases in the MICs of all of the drugs except for cefalotin against strain JSΔcpxRΔtolC were observed (Table 2). As shown in Figure 3, the expression levels of acrB, tolC, and the transcription factor genes marA, soxS, and ramA were measured in strains JS, JSΔcpxA, JScpxA38, JScpxA92–104, and JSΔcpxR. In all cpxA mutants, a significant decrease in the expression levels of marA and soxS was observed, along with a significant increase in the expression level of ramA, and no significant difference in the expression levels of acrB and tolC were observed compared with strain JS. We then constructed mutants JSΔramA::kan and JSΔcpxAΔramA::kan. Compared with strain JSΔramA::kan, two- to four-fold increases in the MICs of all of the drugs except for kanamycin against strain JSΔcpxAΔramA::kan were observed. These results demonstrate that AcrB and TolC confer resistance to some AGAs and β-lactams, but both activation and inactivation of the CpxRA system can modulate resistance to AGAs and β-lactams in both an acrB or tolC background and a ΔacrB or ΔtolC background.
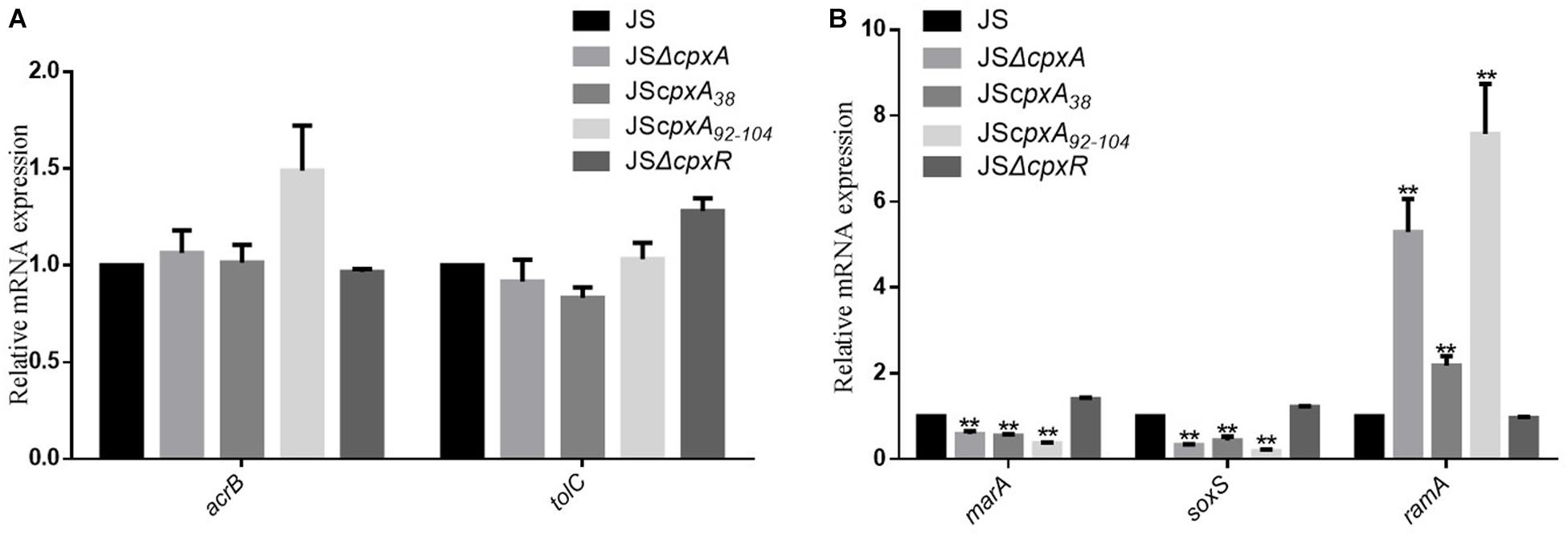
Figure 3. The influence of various cpxA mutations on the expression levels of the efflux pump AcrAB-TolC. (A) Relative mRNA expression levels of the efflux pump genes acrB and tolC. (B) Relative mRNA expression levels of the transcription factor genes marA, soxS, and ramA. The expression level of each mRNA in strain JS represents one-fold. Data were normalized to rpoD expression levels. Bars represent means ± standard deviations. **, P < 0.01.
Effects of Different CpxA Mutations on the Expression Levels of Cpx Regulons
The CpxRA system can alter the expression levels of a series of published Cpx regulon members to mediate drug resistance, such as those encoding outer membrane proteins OmpF, OmpC, OmpD, OmpW, and STM3031; efflux pump AcrD; proteases PpiA and HtpX; protein folding factors Spy and YccA, and respiration-related proteins CyoA, NuoA, and SdhC (Hu et al., 2011; Mahoney and Silhavy, 2013; Raivio et al., 2013; Raivio, 2014; Huang et al., 2016). To identify whether different cpxA mutations have a consistent effect on the expression levels of these Cpx regulons, we detected the relative mRNA expression levels of these genes. As shown in Figure 4, compared with strain JS, the mRNA expression levels of ompW, acrD, spy, ycca, ppia, htpX, and stm3031 in all three cpxA mutants changed significantly (P < 0.01 or P < 0.05). In detail, the mRNA expression level of ompW was decreased, whereas the expression levels of acrD, spy, ycca, ppia, htpX, and stm3031 were increased. The mRNA expression levels of ompD, cyoA, and acrF showed no significant differences in these three cpxA mutants. The mRNA expression levels of ompF and ompC were increased in JSΔcpxA and JScpxA92–104, but showed no significant difference in JScpxA38. The mRNA expression levels of nuoA and sdhC were decreased in JScpxA92–104, but showed no significant difference in JSΔcpxA and JScpxA38. In mutant strain JSΔcpxR, significant decreases (P < 0.01 or P < 0.05) in mRNA expression were only seen for spy, ycca, ppia, htpX, and stm3031. Then, we individually constructed null mutations in sharply upregulated genes stm3031, htpX, and spy in a cpxA-deficient background and WT (JSΔstm3031::kan, JSΔhtpX::kan, JSΔspy::kan, JSΔcpxAΔstm3031::kan, JSΔcpxAΔhtpX::kan, and JSΔcpxAΔspy::kan). However, compared with JSΔcpxA, none of these genes showed a decrease in the resistance conferred by the cpxA deletion mutation (Table 2). To further study the impact of efflux pumps on the resistance conferred by the cpxA deletion mutation, we also constructed some corresponding acrD null mutations (JSΔacrD::Kan, JSΔcpxAΔacrD::Kan, JSΔacrBΔacrD::Kan, and JSΔcpxAΔacrBΔacrD::Kan). As shown in Table 2, the MICs of JSΔacrD::Kan were the same as that of the JS. Compared with JSΔcpxA, the MICs of JSΔcpxAΔacrD::Kan were the same as that of the cpxA null mutant. Compared with JSΔacrBΔacrD::Kan, two- to four-fold increases in the MICs of all of the drugs except for kanamycin against strain JSΔcpxAΔacrBΔacrD::Kan.
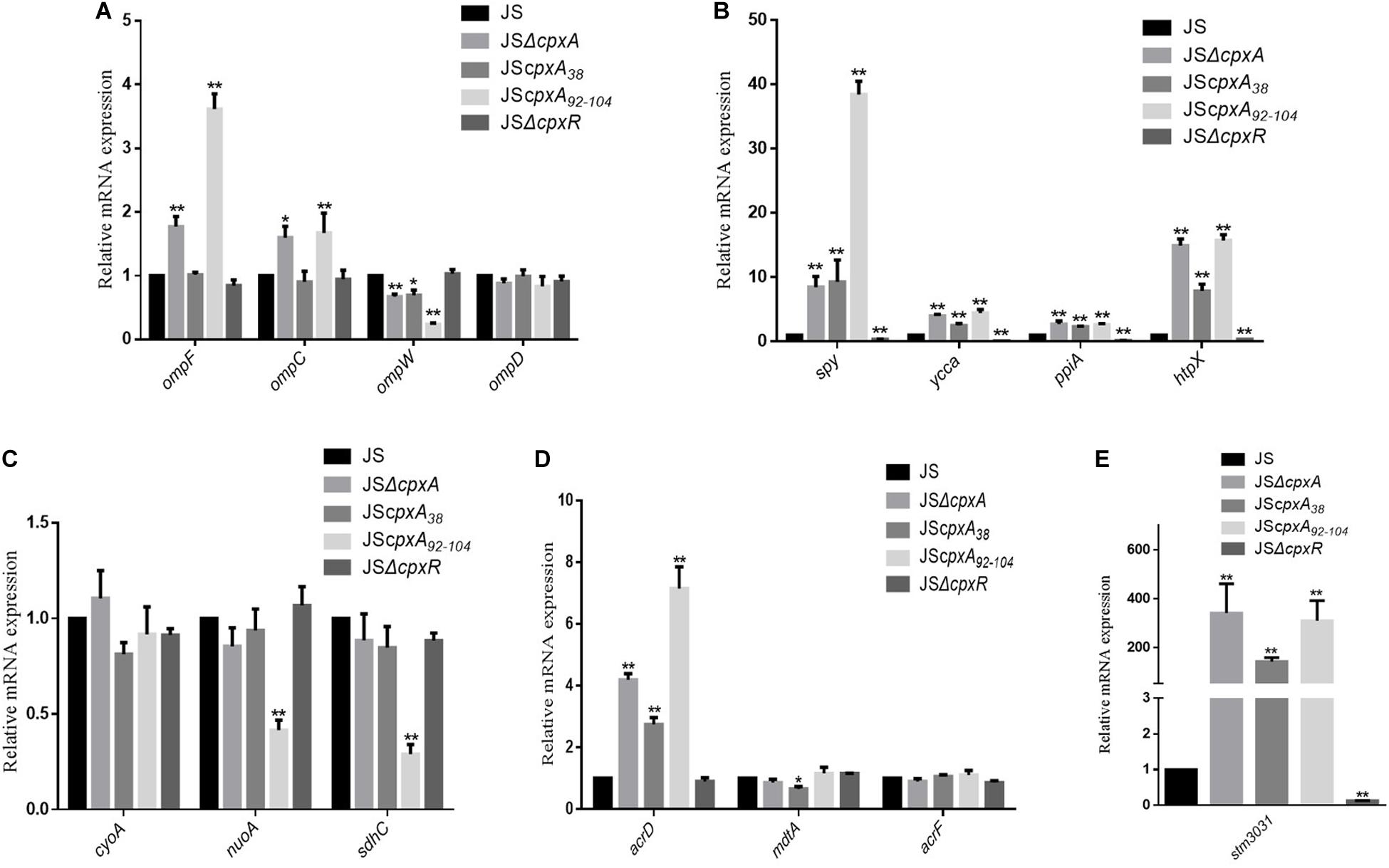
Figure 4. Relative mRNA levels of a series of MDR-related genes were measured by real-time PCR. (A) Relative mRNA expression levels of the outer membrane proteins genes ompF, ompC, ompW, and ompD; (B) Relative mRNA expression levels of protease genes ppiA and htpX and protein folding factors genes spy and yccA; (C) Relative mRNA expression levels of the genes involved in respiration cyoA, nuoA, and sdhC; (D) Relative mRNA expression levels of the efflux pumps genes acrD, mdtA, and acrF; (E) Relative mRNA expression levels of the outer membrane proteins genes STM3031; The expression level of each mRNA in strain JS represents one-fold. Data were normalized to rpoD expression levels. Bars represent means ± standard deviations. *, P < 0.05; **, P < 0.01.
Discussion
In this study, all of the tested cpxA mutants of S. Typhimurium were found to confer resistance to AGAs and β-lactams. The previous studies showed the strain cpxA∗ (JScpxA92–104) was more resistant to amikacin than its wild-type strain SL1344, but the cpxA deletion mutant was more sensitive to amikacin than its parent strain (Humphreys et al., 2004). Such contradictory findings suggested that although cpxA deletion can active the CpxRA system and display numerous phenotypes, whether null mutations of cpxA regulating resistance are caused solely by hyperphosphorylation of CpxR in S. Typhimurium still needs to be determined. On the basis of studies involving cpxR deletion and site-directed mutagenesis, we ruled out the pleiotropic effects of CpxA and provided evidence that the increased resistance of cpxA deletion mutants to AGAs and β-lactams is directly mediated by CpxR-P, which is activated via the acetyl phosphate pathway. This finding is consistent with previous reports showing that the deletion of cpxA eliminates its phosphatase activity, causing CpxR to accept phosphate groups from acetyl phosphate generated by Acka and Pta, activating the CpxRA system and contributing to some phenotypic changes (Danese and Silhavy, 1998; Buelow and Raivio, 2005; Lima et al., 2012).
JSΔcpxR showed no significant differences in the mRNA expression levels of the major tested genes in the absence of antibiotics, which is consistent with a previous report in S. Typhimurium (Huang et al., 2016). It is also consistent with a previous study in E. coli that showed that a cpxR mutant had few differentially regulated genes compared with wild-type uropathogenic E. coli, which indicated that the CpxRA system is only minimally active in the wild-type strain under the experimental growth conditions (Dbeibo et al., 2018). This raises the question of why the wild-type strain shows more resistance to AGAs and β-lactams. Here, we confirmed that amikacin and cefuroxime can activate the CpxRA system and that the activated CpxRA system increases the resistance to amikacin and cefuroxime. Therefore, it is proposed that the presence of antibiotics activates the CpxRA response system in wild-type strains, which in turn, leads to an increase in drug resistance compared with strain JSΔcpxR. The results also suggest that the phenotypic changes caused by cpxR deletion mainly depend on whether the CpxRA pathway can be activated by a corresponding external stimulation.
In Gram-negative bacteria, RND-family multidrug efflux pumps play the most prominent roles in drug resistance (Poole, 2001; Becker and Cooper, 2013; Du et al., 2018). Among the RND efflux pumps, AcrD is mainly responsible for resistance to the hydrophilic class of drugs such as AGAs in E. coli (Rosenberg et al., 2000). Moreover, some reports suggest that this efflux pump confers resistance to some other compounds, such as tetracycline, novobiocin, norfloxacin, Fosfomycin, and some β-lactams in E. coli (Nishino and Yamaguchi, 2001; Nishino et al., 2003). By contrast, AcrB confers resistance to practically all types of antibacterial agents, except aminoglycosides in E. coli (Elkins and Nikaido, 2002; Li et al., 2015). In the current study, a significant increase in the expression level of acrD was observed in the cpxA mutants, which is consistent with previous reports and supports the important role of the AcrAD-TolC efflux pump in resistance regulation of the CpxRA system (Hu et al., 2011; Huang et al., 2016). However, the acrD deletion mutation did not decrease the resistance conferred by the cpxA deletion mutation, which is consistent with previous research and suggests that no single factor is necessary of the resistance to β-lactams and AGAs conferred by the cpxA mutation (Mahoney and Silhavy, 2013). AcrAB-TolC contributes to the resistance to some aminoglycosides (amikacin and streptomycin) in S. enterica serovar Typhimurium JS, which is consistent with the previous result where the deletion of acrB decreased resistance to aminoglycosides (amikacin and neomycin) (Huang et al., 2016). To our knowledge, it is unclear why the mutant strain lacking acrB is more susceptible to some aminoglycosides in S. enterica serovar Typhimurium JS and a further study is needed. We did not find any significant change in the mRNA expression levels of acrB and tolC, this differed from a previous report showing that overexpression of cpxR results in a significant decrease in the mRNA expression levels of acrB and tolC compared with the wild-type strain JS (Huang et al., 2016). This difference may be explained by the paradigm that, for the tested global regulatory factors, the three cpxA mutations all led to a significant reduction in the levels of marA and soxS, thereby downregulating the expression levels of the AcrAB-TolC efflux pump, but led to a significant increase in the levels of ramA, thereby upregulating the expression of the AcrAB-TolC efflux pump. Moreover, in the acrB and tolC deletion mutants, which showed decreased resistance to AGAs and β-lactams, both activation and inactivation of the CpxRA system could modulate resistance. To investigate further, we constructed strain JSΔcpxAΔramA::kan and JSΔramA::kan. Compared with strain JSΔramA::kan, the MICs of the tested antibiotics also showed an increase. These results suggest that the efflux pump AcrAB-TolC does not play a decisive role in the CpxRA-mediated AGAs and β-lactams resistance of S. Typhimurium.
Membrane proteases is one of the important mechanisms of AGAs resistance (Becker and Cooper, 2013). When bacteria are exposed to AGAs, there is an increase in the abundance of misfolded and mistargeted proteins within cells. Whereafter, genes that are involved in protein turnover are upregulated, as well as those encoding membrane proteases. If the membrane proteases cannot keep up with the increasing number of faulty proteins, their surplus accumulation eventually destroys the integrity of the membrane and kills the bacteria (Magnet and Blanchard, 2005; Becker and Cooper, 2013). In this study, the selected protease genes, ppiA and htpX, and the protein folding factor genes, spy and yccA, were all upregulated in various cpxA mutants, suggesting that the function of maintaining membrane integrity of the CpxRA envelope stress response system, is important for the resistance to AGAs.
One theory proposes that all bactericidal antibiotics may act through a common mechanism involving the production of reactive oxygen species (ROS), which is dependent on metabolism-related NADH depletion and the electron transport chain (Van Acker and Coenye, 2017). It has been shown that cpxA or cpxR deletion mutations confer antibiotic resistance by reducing the production of ROS (Kohanski et al., 2008). A study also demonstrated that compared with the parent strain, the expression levels of genes encoding succinate dehydrogenase (sdh), NADH dehydrogenase (nuo), and cytochrome oxidase (cyo) were significantly downregulated under multiple conditions in the transient NlpE overexpression strain, and the elimination of these genes (cyoA, nuoA, and sdhC) conferred resistance to amikacin (Raivio et al., 2013). However, we found that this common factor was inconsistent among the tested cpxA mutants. The mRNA expression levels of nuoA and sdhC were only found to be decreased in strain JScpxA92–104, with no significant differences detected in strains JSΔcpxA and JScpxA38. These results suggested that the decrease in ROS may explain the accompanied tolerance to aminoglycosides of the activated CpxRA system in certain cpxA mutants, particularly JScpxA92–104. Furthermore, the factors involved in drug resistance regulation appear to differ between the tested cpxA mutants.
Conclusion
In conclusion, this study analyzed the mechanism involved in the CpxA-mediated regulation of resistance to AGAs and β-lactams in S. Typhimurium. It was revealed that various cpxA mutations show the same resistance phenotype relying on phosphorylated CpxR. These findings broaden our understanding of the complex regulatory network governing CpxRA-mediated antibiotic resistance.
Data Availability Statement
The original contributions presented in the study are included in the article/Supplementary Material, further inquiries can be directed to the corresponding author/s.
Author Contributions
WJ, JL, and SW designed the experiment. YL was responsible for funding acquisition and project supervision. WJ, XL, and YL contributed to manuscript writing. All authors contributed to the article and approved the submitted version.
Funding
This work was supported by the Innovative Engineering of Bacterial Disease in Grazing Animal Team, Lanzhou, China.
Conflict of Interest
The authors declare that the research was conducted in the absence of any commercial or financial relationships that could be construed as a potential conflict of interest.
Acknowledgments
We thank Dr. Ganwu Li for providing the plasmids used in this study. We also thank the editor and the reviewers for their helpful feedback.
Supplementary Material
The Supplementary Material for this article can be found online at: https://www.frontiersin.org/articles/10.3389/fmicb.2021.604079/full#supplementary-material
Supplementary Table 1 | Primers used in this study.
References
Baucheron, S., Tyler, S., Boyd, D., Mulvey, M. R., Chaslus-Dancla, E., and Cloeckaert, A. (2004). AcrAB-TolC directs efflux-mediated multidrug resistance in Salmonella enterica serovar typhimurium DT104. Antimicrob. Agents Chemother. 48, 3729–3735. doi: 10.1128/AAC.48.10.3729-3735.2004
Becker, B., and Cooper, M. A. (2013). Aminoglycoside antibiotics in the 21st century. ACS Chem. Biol. 8, 105–115. doi: 10.1021/cb3005116
Buelow, D. R., and Raivio, T. L. (2005). Cpx signal transduction is influenced by a conserved N-terminal domain in the novel inhibitor CpxP and the periplasmic protease DegP. J. Bacteriol. 187, 6622–6630. doi: 10.1128/JB.187.19.6622-6630.2005
Cockerill, F., Wikler, M., Alder, J., Dudley, M., Eliopoulos, G., Ferraro, M., et al. (2012). Methods for dilution antimicrobial susceptibility tests for bacteria that grow aerobically: approved standard. Clin. Lab. Stand. Inst. 32, M07–M09.
Danese, P. N., and Silhavy, T. J. (1998). CpxP, a stress-combative member of the Cpx regulon. J. Bacteriol. 180, 831–839.
Datsenko, K. A., and Wanner, B. L. (2000). One-step inactivation of chromosomal genes in Escherichia coli K-12 using PCR products. Proc. Natl. Acad. Sci. U. S. A. 97, 6640–6645. doi: 10.1073/pnas.120163297
Dbeibo, L., van Rensburg, J. J., Smith, S. N., Fortney, K. R., Gangaiah, D., Gao, H., et al. (2018). Evaluation of CpxRA as a Therapeutic Target for Uropathogenic Escherichia coli Infections. Infect. Immun. 86, 798–717e. doi: 10.1128/IAI.00798-717
De la Cruz, M. A., Perez-Morales, D., Palacios, I. J., Fernandez-Mora, M., Calva, E., and Bustamante, V. H. (2015). The two-component system CpxR/A represses the expression of Salmonella virulence genes by affecting the stability of the transcriptional regulator HilD. Front. Microbiol. 6:807. doi: 10.3389/fmicb.2015.00807
De Wulf, P., McGuire, A. M., Liu, X., and Lin, E. C. (2002). Genome-wide profiling of promoter recognition by the two-component response regulator CpxR-P in Escherichia coli. J. Biol. Chem. 277, 26652–26661. doi: 10.1074/jbc.M203487200
Delhaye, A., Collet, J. F., and Laloux, G. (2016). Fine-Tuning of the Cpx Envelope Stress Response Is Required for Cell Wall Homeostasis in Escherichia coli. mBio 7, 47–16e. doi: 10.1128/mBio.00047-16
DiGiuseppe, P. A., and Silhavy, T. J. (2003). Signal detection and target gene induction by the CpxRA two-component system. J. Bacteriol. 185, 2432–2440. doi: 10.1128/jb.185.8.2432-2440.2003
Du, D., Wang-Kan, X., Neuberger, A., van Veen, H. W., Pos, K. M., Piddock, L. J. V., et al. (2018). Multidrug efflux pumps: structure, function and regulation. Nat. Rev. Microbiol. 16, 523–539. doi: 10.1038/s41579-018-0048-46
Du, D., Wang, Z., James, N. R., Voss, J. E., Klimont, E., Ohene-Agyei, T., et al. (2014). Structure of the AcrAB-TolC multidrug efflux pump. Nature 509, 512–515. doi: 10.1038/nature13205
Elkins, C. A., and Nikaido, H. (2002). Substrate specificity of the RND-type multidrug efflux pumps AcrB and AcrD of Escherichia coli is determined predominantly by two large periplasmic loops. J. Bacteriol. 184, 6490–6498. doi: 10.1128/jb.184.23.6490-6499.2002
Fujimoto, M., Goto, R., Haneda, T., Okada, N., and Miki, T. (2018). Salmonella enterica Serovar Typhimurium CpxRA Two-Component System Contributes to Gut Colonization in Salmonella-Induced Colitis. Infect. Immun. 86, 280–218e. doi: 10.1128/IAI.00280-218
Gotoh, Y., Eguchi, Y., Watanabe, T., Okamoto, S., Doi, A., and Utsumi, R. (2010). Two-component signal transduction as potential drug targets in pathogenic bacteria. Curr. Opin. Microbiol. 13, 232–239. doi: 10.1016/j.mib.2010.01.008
Hirakawa, H., Nishino, K., Hirata, T., and Yamaguchi, A. (2003a). Comprehensive studies of drug resistance mediated by overexpression of response regulators of two-component signal transduction systems in Escherichia coli. J. Bacteriol. 185, 1851–1856.
Hirakawa, H., Nishino, K., Yamada, J., Hirata, T., and Yamaguchi, A. (2003b). Beta-lactam resistance modulated by the overexpression of response regulators of two-component signal transduction systems in Escherichia coli. J. Antimicrob. Chemother. 52, 576–582. doi: 10.1093/jac/dkg406
Hu, W. S., Chen, H. W., Zhang, R. Y., Huang, C. Y., and Shen, C. F. (2011). The expression levels of outer membrane proteins STM1530 and OmpD, which are influenced by the CpxAR and BaeSR two-component systems, play important roles in the ceftriaxone resistance of Salmonella enterica serovar Typhimurium. Antimicrob. Agents Chemother. 55, 3829–3837. doi: 10.1128/AAC.00216-211
Huang, H., Sun, Y., Yuan, L., Pan, Y., Gao, Y., Ma, C., et al. (2016). Regulation of the Two-Component Regulator CpxR on Aminoglycosides and beta-lactams Resistance in Salmonella enterica serovar Typhimurium. Front. Microbiol. 7:604. doi: 10.3389/fmicb.2016.00604
Humphreys, S., Rowley, G., Stevenson, A., Anjum, M. F., Woodward, M. J., Gilbert, S., et al. (2004). Role of the two-component regulator CpxAR in the virulence of Salmonella enterica serotype Typhimurium. Infect. Immun. 72, 4654–4661. doi: 10.1128/IAI.72.8.4654-4661.2004
Isaac, D. D., Pinkner, J. S., Hultgren, S. J., and Silhavy, T. J. (2005). The extracytoplasmic adaptor protein CpxP is degraded with substrate by DegP. Proc. Natl. Acad. Sci. U. S. A. 102, 17775–17779. doi: 10.1073/pnas.0508936102
Jing, W., Liu, J., Wu, S., Chen, Q., Li, X., and Liu, Y. (2020). Development of a Method for Simultaneous Generation of Multiple Genetic Modification in Salmonella enterica Serovar Typhimurium. Front. Genet. 11:563491. doi: 10.3389/fgene.2020.563491
Kohanski, M. A., Dwyer, D. J., Wierzbowski, J., Cottarel, G., and Collins, J. J. (2008). Mistranslation of membrane proteins and two-component system activation trigger antibiotic-mediated cell death. Cell 135, 679–690. doi: 10.1016/j.cell.2008.09.038
Levy, S. B. (2002). The 2000 Garrod lecture. Factors impacting on the problem of antibiotic resistance. J. Antimicrob. Chemother. 49, 25–30. doi: 10.1093/jac/49.1.25
Li, X. Z., Plesiat, P., and Nikaido, H. (2015). The challenge of efflux-mediated antibiotic resistance in Gram-negative bacteria. Clin. Microbiol. Rev. 28, 337–418. doi: 10.1128/CMR.00117-114
Lima, B. P., Thanh Huyen, T. T., Basell, K., Becher, D., Antelmann, H., and Wolfe, A. J. (2012). Inhibition of acetyl phosphate-dependent transcription by an acetylatable lysine on RNA polymerase. J. Biol. Chem. 287, 32147–32160. doi: 10.1074/jbc.M112.365502
Magnet, S., and Blanchard, J. S. (2005). Molecular insights into aminoglycoside action and resistance. Chem. Rev. 105, 477–498. doi: 10.1021/cr0301088
Mahoney, T. F., and Silhavy, T. J. (2013). The Cpx stress response confers resistance to some, but not all, bactericidal antibiotics. J. Bacteriol. 195, 1869–1874. doi: 10.1128/JB.02197-2112
Masi, M., Pinet, E., and Pages, J. M. (2020). Complex Response of the CpxAR Two-Component System to beta-Lactams on Antibiotic Resistance and Envelope Homeostasis in Enterobacteriaceae. Antimicrob. Agents Chemother. 64:32229490. doi: 10.1128/AAC.00291-220
Nakayama, S., Kushiro, A., Asahara, T., Tanaka, R., Hu, L., Kopecko, D. J., et al. (2003). Activation of hilA expression at low pH requires the signal sensor CpxA, but not the cognate response regulator CpxR, in Salmonella enterica serovar Typhimurium. Microbiology 149, 2809–2817. doi: 10.1099/mic.0.26229-26220
Nishino, K., Yamada, J., Hirakawa, H., Hirata, T., and Yamaguchi, A. (2003). Roles of TolC-dependent multidrug transporters of Escherichia coli in resistance to beta-lactams. Antimicrob. Agents Chemother. 47, 3030–3033. doi: 10.1128/aac.47.9.3030-3033.2003
Nishino, K., and Yamaguchi, A. (2001). Analysis of a complete library of putative drug transporter genes in Escherichia coli. J. Bacteriol. 183, 5803–5812. doi: 10.1128/JB.183.20.5803-5812.2001
Nishino, K., Yamasaki, S., Hayashi-Nishino, M., and Yamaguchi, A. (2010). Effect of NlpE overproduction on multidrug resistance in Escherichia coli. Antimicrob. Agents Chemother. 54, 2239–2243. doi: 10.1128/AAC.01677-1679
Padilla-Vaca, F., Mondragon-Jaimes, V., and Franco, B. (2017). General Aspects of Two-Component Regulatory Circuits in Bacteria: Domains. Sign. Roles. Curr. Prot. Pept. Sci. 18, 990–1004. doi: 10.2174/1389203717666160809154809
Pogliano, J., Dong, J. M., De Wulf, P., Furlong, D., Boyd, D., Losick, R., et al. (1998). Aberrant cell division and random FtsZ ring positioning in Escherichia coli cpxA∗ mutants. J. Bacteriol. 180, 3486–3490. doi: 10.1128/JB.180.13.3486-3490.1998
Poole, K. (2001). Multidrug resistance in Gram-negative bacteria. Curr. Opin. Microbiol. 4, 500–508.
Quinn, T., O’Mahony, R., Baird, A. W., Drudy, D., Whyte, P., and Fanning, S. (2006). Multi-drug resistance in Salmonella enterica: efflux mechanisms and their relationships with the development of chromosomal resistance gene clusters. Curr. Drug Targets 7, 849–860.
Raivio, T. L. (2014). Everything old is new again: an update on current research on the Cpx envelope stress response. Biochim. Biophys. Acta 1843, 1529–1541. doi: 10.1016/j.bbamcr.2013.10.018
Raivio, T. L., Leblanc, S. K., and Price, N. L. (2013). The Escherichia coli Cpx envelope stress response regulates genes of diverse function that impact antibiotic resistance and membrane integrity. J. Bacteriol. 195, 2755–2767. doi: 10.1128/JB.00105-113
Raivio, T. L., and Silhavy, T. J. (1997). Transduction of envelope stress in Escherichia coli by the Cpx two-component system. J. Bacteriol. 179, 7724–7733.
Rosenberg, E. Y., Ma, D., and Nikaido, H. (2000). AcrD of Escherichia coli is an aminoglycoside efflux pump. J. Bacteriol. 182, 1754–1756.
Tiwari, S., Jamal, S. B., Hassan, S. S., Carvalho, P., Almeida, S., Barh, D., et al. (2017). Two-Component Signal Transduction Systems of Pathogenic Bacteria As Targets for Antimicrobial Therapy: An Overview. Front. Microbiol. 8:1878. doi: 10.3389/fmicb.2017.01878
Van Acker, H., and Coenye, T. (2017). The Role of Reactive Oxygen Species in Antibiotic-Mediated Killing of Bacteria. Trends Microbiol. 25, 456–466. doi: 10.1016/j.tim.2016.12.008
Keywords: S. enterica serovar Typhimurium, various mutants, resistance, AGAs, β-lactams, cpxA
Citation: Jing W, Liu J, Wu S, Li X and Liu Y (2021) Role of cpxA Mutations in the Resistance to Aminoglycosides and β-Lactams in Salmonella enterica serovar Typhimurium. Front. Microbiol. 12:604079. doi: 10.3389/fmicb.2021.604079
Received: 08 September 2020; Accepted: 12 January 2021;
Published: 04 February 2021.
Edited by:
Yuji Morita, Meiji Pharmaceutical University, JapanReviewed by:
Xiangmin Lin, Fujian Agriculture and Forestry University, ChinaJakob Frimodt-Møller, University of Copenhagen, Denmark
Copyright © 2021 Jing, Liu, Wu, Li and Liu. This is an open-access article distributed under the terms of the Creative Commons Attribution License (CC BY). The use, distribution or reproduction in other forums is permitted, provided the original author(s) and the copyright owner(s) are credited and that the original publication in this journal is cited, in accordance with accepted academic practice. No use, distribution or reproduction is permitted which does not comply with these terms.
*Correspondence: Xuerui Li, bGl4dWVydWlAY2Fhcy5jbg==; Yongsheng Liu, bGl1eW9uZ3NoZW5nQGNhYXMuY24=