- 1Department of Medical Microbiology, NUTRIM School of Nutrition and Translational Research in Metabolism, Maastricht University Medical Centre+, Maastricht, Netherlands
- 2Division of Gastroenterology/Hepatology, Department of Internal Medicine, NUTRIM School of Nutrition and Translational Research in Metabolism, Maastricht University Medical Centre+, Maastricht, Netherlands
- 3Department of Medical Microbiology, Caphri School for Public Health and Primary Care, Maastricht University Medical Centre+, Maastricht, Netherlands
- 4Department of Pathology, Radboud Institute for Molecular Life Sciences (RIMLS), Radboud University Medical Center, Nijmegen, Netherlands
- 5Department of Medical Microbiology and Infection Control, Amsterdam University Medical Center, Location VUMC, Amsterdam, Netherlands
Bacteroides fragilis has previously been linked to Crohn’s disease (CD) exacerbations, but results are inconsistent and underlying mechanisms unknown. This study investigates the epidemiology of B. fragilis and its virulence factors bft (enterotoxin) and ubiquitin among 181 CD patients and the impact on the intestinal epithelial barrier in vitro. The prevalence of B. fragilis was significantly higher in active (n = 69/88, 78.4%) as compared to remissive (n = 58/93, 62.4%, p = 0.018) CD patients. Moreover, B. fragilis was associated with intestinal strictures. Interestingly, the intestinal barrier function, as examined by transepithelial electrical resistance (TEER) measurements of Caco-2 monolayers, increased when exposed to secretomes of bft-positive (bft-1 and bft-2 isotype; increased TEER ∼160%, p < 0.001) but not when exposed to bft-negative strains. Whole metagenome sequencing and metabolomics, respectively, identified nine coding sequences and two metabolites that discriminated TEER-increasing from non-TEER-increasing strains. This study revealed a higher B. fragilis prevalence during exacerbation. Surprisingly, bft-positive secretomes increased epithelial resistance, but we excluded Bft as the likely causative factor.
Introduction
Crohn’s disease (CD) is a chronic inflammatory disease, characterized by patchy inflammation of the intestinal mucosa with or without extra-intestinal manifestations (Baumgart and Sandborn, 2012). The disease course varies largely among patients with alternating periods of remission and exacerbations. Insufficient control of the recurrent mucosal inflammation results in phenotype progression and complications, such as strictures or fistulas, contributing to a high disease and economic burden (Solberg et al., 2007; Floyd et al., 2015).
CD onset is considered to involve genetic predisposition, environmental factors and an adverse immune reaction to the host microbiota (Baumgart and Sandborn, 2012). However, the factors influencing the occurrence of exacerbations, complications and disease phenotype remain largely unclear.
In recent years, microbial dysbiosis gained increasing attention as a factor contributing to exacerbations (Baumgart and Sandborn, 2012; Tedjo et al., 2016). Several studies reported a decreased microbial diversity and altered microbial composition in active CD patients compared to remission (Prosberg et al., 2016; Tedjo et al., 2016; Galazzo et al., 2019). As a consequence of compositional changes, alterations in overall microbial functionality are conceivable (Li et al., 2020). Since the intestinal epithelium limits bacterial attachment by a mucus layer, bacteria often interact with the host via their secretome, consisting of metabolites, proteins and bacterial membrane vesicles (MVs) (Kaparakis-Liaskos and Ferrero, 2015; Sicard et al., 2017; Parker et al., 2018; Caruana and Walper, 2020). On the one hand, secreted glycosidases and mucinases have been shown to degrade the mucus layer and allow microbes to directly interact with epithelial cells (van Passel et al., 2011; Sicard et al., 2017). On the other hand, bacterial metabolites, such as the short chain fatty acid butyrate, have been reported to promote mucus production and the sealing capacity of the intercellular junctional complex (Sun et al., 2016; Zheng et al., 2017). In CD, an impaired intestinal epithelial barrier function has increasingly been recognized as a hallmark of exacerbations (Baumgart and Sandborn, 2012). The impaired barrier function has been associated with alterations in the epithelial junctional complex (Zeissig et al., 2007; Lameris et al., 2013). It remains unknown whether the observed altered microbiota composition and functionality during CD exacerbations can contribute to this impaired epithelial barrier.
In addition to alterations in microbial diversity, specific microbial taxa were detected in CD exacerbations (Eeckhaut et al., 2013; Schäffler et al., 2016; Tedjo et al., 2016) of which Bacteroides fragilis is a prominent example (Wills et al., 2014; Schäffler et al., 2016; Tedjo et al., 2016). Several studies investigated the colonization of B. fragilis during exacerbation and remission in fecal samples and biopsies. Together, the results are inconclusive and based on rather low sample sizes (Scanlan et al., 2006; Wills et al., 2014; Schäffler et al., 2016; Tedjo et al., 2016). So far, it is still unclear whether the prevalence of B. fragilis differs in different disease stages of CD and how it may affect disease activity.
B. fragilis is a Gram-negative commensal of the phylum Bacteroidetes and some strains secrete various virulence factors, such as B. fragilis toxin (Bft; fragilysin) and an eukaryotic-like ubiquitin (Ubb) (Sears, 2009; Stewart et al., 2018). It is yet unclear whether these virulence factors contribute to CD exacerbations.
To gain more insights into the interaction between the intestinal microbiota and the intestinal barrier in CD, especially during dysbiosis, research essentially needs to focus on microbial functionality, such as reflected by the secretome. In this study, we therefore aim to investigate firstly the prevalence and relative abundance of B. fragilis and virulence-factor positive strains in a large, well-defined cross-sectional CD patient cohort and secondly the impact of the B. fragilis secretome on the intestinal epithelial barrier as first site of interaction and pathophysiological factor in CD exacerbations. We hypothesize that B. fragilis and its virulence factors bft and ubb are involved in exacerbations and by disrupting the intestinal epithelial barrier.
Materials and Methods
Patient Inclusion
Fecal samples of CD patients participating in the IBD South Limburg (IBDSL) biobank project (van den Heuvel et al., 2015) were available to evaluate the colonization of B. fragilis and relevant strains in CD patients and the relation with disease activity. Baseline characteristics, including medication use and demographic information, were extracted from the IBDSL database (van den Heuvel et al., 2015).
Patients with a fecal calprotectin level >200 μg/g were defined as active (n = 88) and patients with a fecal calprotectin level <100 μg/g as remissive (n = 93). Remissive patients without previously reported calprotectin level >200 μg/g were excluded to avoid misclassification unless the previously elevated calprotectin level was combined with a CRP value <10 mg/l.
In addition, from two patients having a colonoscopy scheduled for clinical reasons, biopsies were collected from the macroscopically non-inflamed tissue of the ascending colon for crypt isolation and subsequent organoid culture (Xu et al., 2018).
All patients participated in the IBDSL cohort and gave written informed consent prior to sample collection. The study protocol was approved by the Medical Ethics Committee of the Maastricht University Medical Centre+ (NL31636.068.10) and registered on www.clinicaltrials.gov (NCT02130349).
Fecal DNA Isolation
Fecal samples stored at –80°C within 24 h after collection were obtained from the IBDSL biobank (van den Heuvel et al., 2015) and cut frozen to obtain ∼0.5 g feces. DNA isolation was conducted using Qiagen QIAamp DNA mini kit (Qiagen, ref.: 51306) according to protocol Q of the International Human Microbiome Standards consortium (Costea et al., 2017) with minor adjustments in FastprepTM cycles (three series of 1 min of beating at 5.5 ms and 1 min resting) and vacuum drying at 37°C for 7 min instead of 3 min. Eluted DNA was stored in Buffer AE (Qiagen) at –20°C until further analysis.
Enumeration of Fecal B. fragilis
Metagenomic DNA obtained from the fecal samples was analyzed for the presence and relative abundance of B. fragilis as well as the virulence factors bft and ubb by means of real-time quantitative PCR. Samples containing B. fragilis specific gyrB were subsequently analyzed for the presence of bft and ubb.
The PCR mix for gyrB and bft contained 5 μl DNA, 12.5 μl Absolute quantitative PCR mix (Abgene, Epsom, United Kingdom), 500 mM forward and reverse primer (Sigma-Aldrich; Supplementary Table 1), 250 nM probe and was supplemented with DNAse-free water to a final volume of 25 μl. qPCR and analysis were conducted with 7900HT Fast Real-Time PCR System (Applied Biosystems) and SDS 2.3 software (Applied Biosystems). PCR cycles for gyrB and bft were 2 min at 50°C, 10 min at 95°C, followed by 42 amplification cycles of 15 s at 95°C and 60 s at 60°C.
PCR mix for ubb and 16S rRNA contained 2 μl DNA, 12.5 μl Absolute qPCR SYBR Green supermix (Bio-Rad, Hercules, CA), 300 nM forward and reverse primer (Sigma-Aldrich; Supplementary Table 1) and was supplemented with DNAse-free water to a final volume of 25 μl. qPCR and analysis were conducted with MyIQ Single Color Real-Time PCR Detection System (BioRad) and iQ5 software (BioRad). PCR cycles for ubb and 16S rRNA were 3 min at 95°C, followed by 40, respectively, 35 amplification cycles of 95°C, 20 s at 63°C, respectively, 55°C, and 30 s at 72°C. The melting curve was assessed in 60 cycles of 0.5°C for 10 s each.
B. fragilis Isolation From Fecal Samples
To retrieve toxigenic and non-toxigenic B. fragilis strains for in vitro experiments, a random selection of fecal samples of three CD patients and two samples of healthy controls (HC) from a previous study that were positive for B. fragilis specific gyrB were cultured. A portion of ∼1 g was dissolved in Brain Heart Infusion broth (BHI; Sigma Millipore, ref.: 53285, Darmstadt, Germany) containing 4 μg/ml Vancomycin hydrochloride (No 15327, Cayman Chemical, Michigan, United States) and incubated in an anaerobic jar (80% N2, 10% CO2, 20% H2) at 37°C for 24 h. Next, 1:100 dilutions were inoculated on Bacteroides Bile Esculin Agar with Amikacin (Becton Dickinson, ref.: 254480, Landsmeer, Netherlands) and incubated for 48 h under the same conditions. Medium to large sized single colonies were subsequently inoculated on Columbia Agar with 5% Sheep Blood (Becton Dickinson, ref.: 254005, Landsmeer, Netherlands) and incubated for 48 h, as described before. This was repeated to guarantee pure cultures. A single colony was then collected in DNAse-free water and checked for gyrB, bft and ubb using qPCR as described above. The PCR products of bft and ubb were confirmed using Sanger sequencing to avoid false positive results. Amplicons were purified from remaining nucleotides using the MSB Spin PCRapace-kit (Stratec molecular, ref.: 1020220400) according to manufacturer’s descriptions. One microliter of DNA was then added to 5.5 μl DNAse-free water, 1 μl forward or reverse primer (2 pmol/l), 1.5 μl BDT 1.1 buffer and 1 μl BDT v1.1 enzyme mix. The DNA was amplified with one cycle of 1 min at 96°C and 22 cycles of 10 s at 96°C, 5 s at 58°C and 2–3 min at 60°C. Subsequently, the amplicons were sequenced using an ABI 3730 DNA Analyzer (Thermo Fisher Scientific) and were compared to the Nucleotide Basic Local Alignment Search Tool (BLAST; NCBI) database for sequence similarity with previously sequenced B. fragilis derived bft and ubb.
In total, five B. fragilis strains were isolated. As no ubb-positive, bft-negative genotype was identified, a reference strain from the American Type Culture Collection (ATCC® 25285TM) was obtained. Two further reference strains were kindly provided by prof. Sears (John Hopkins University, Baltimore, United States), namely bft-1-positive VPI 18786 (VPI) and bft-2-positive 86-5443-2-2 (086) and their respective bft deletion mutants (VPI Δbft, 086 Δbft) (Myers et al., 1984; Myers and Schoop, 1987; Rhee et al., 2009; Chung et al., 2018). Finally, the following strains were available for further analysis: one ubb-positive strain (ATCC® 25285TM), two bft-positive strains (CD-2, CD-3), one strain positive for both bft and ubb (HC-1), two strains negative for both virulence factors (HC-2, CD-1; Supplementary Table 2), one bft-1-positive strain (VPI) with corresponding deletion mutant (VPI Δbft), and one bft-2-positive strain (086) with corresponding deletion mutant (086 Δbft).
B. fragilis Supernatant
To study the impact of excreted B. fragilis products on the intestinal barrier in vitro, bacterial culture supernatant was used. Therefore, three to five colonies of B. fragilis strains were inoculated in BHI (Sigma Millipore, ref.: 53285) for 24 h at 37°C in anaerobic jars. Subsequently, bacterial cultures were centrifuged at 4,500 × g for 15 min at 4°C and supernatant was filtered through 0.2 μm pore size syringe filters (Pall Life Sciences, ref: 4652). To increase the sensitivity, filtered supernatant was concentrated 20× using Amicon® Centrifugal Units 10 K (Merck Millipore, ref.: UFC8010) at 4,000 × g for 20 min. Cell-free supernatant was stored at 4°C and used for transepithelial electrical resistance (TEER) experiments within 24 h.
B. fragilis MVs
To investigate the impact of MVs on intestinal epithelial barrier function we separated the MVs from the B. fragilis cell-free supernatant. First, 30 ml of supernatant (see above) was concentrated to ∼250 μl during several centrifugation steps at 4,000 × g at 4°C using Ultracentrifugal Filters of 100 kDa (Merck Millipore, ref: UFC9100). After collection of the concentrate, the filter was washed with 250 μl PBS to recover the remaining particles. MVs were isolated by Size Exclusion Chromatography using CL-2B Sepharose (GE healthcare, Little Chalfont, United Kingdom). As described by Benedikter et al. (2017) vesicle-rich fractions were pooled and the concentration was determined by tunable resistive pulse sensing using qNano Gold (Izon Science Ltd., Oxford, United Kingdom) and Izon Control Suite software v3.2.
Bft Secretion and Activity
To examine the presence of Bft in the cell-free B. fragilis supernatant, we isolated the total protein fraction following the protocol of Casterline et al. (2017) with minor modifications. One milliliter of culture supernatant was precipitated with 10% (v/v) trichloric acid in acetone containing 20 mM 1,4-dithiothreitol (DTT) for 1 h on ice and centrifuged at 15,000 × g for 15 min. The pellet was washed twice with acetone, carefully resuspended and centrifuged at full speed for 15 min. The pellet was then recovered in 2× Laemmli buffer, containing 4% SDS, 10% 2-mercaptoethanol, 20% glycerol, 0.004% bromophenol blue and 0.125 M Tris-HCl, and stored at –20°C. For subsequent western blot analysis, the samples were further denatured at 95°C for 10 min and loaded on a 13% SDS gel according to standard protocol. After separation and wet blotting on an AmershamTM ProtranTM 0.2 μm nitrocellulose membrane (GE Healthcare), the membrane was blocked with skim milk in TBST for 1 h at RT. The membrane was then incubated with anti-Bft antibody (Cusabio, ref.: CSB-PA346537LA01BDP) diluted 1:2,000 for 36 h at 4°C, followed by goat-anti-rabbit antibody conjugated with horseradish peroxidase (Dako p0448) diluted 1:2,000 for 30 min at RT and developed with 0.1 M Tris pH 7.5, 0.2 mg/ml DAB, 0.01% NiCl2 and 0.03% H2O2. Pictures were taken with Mini HD 9 (Uvitec, Cambridge, United Kingdom).
Bft activity was confirmed by a slightly adapted HT-29 cell rounding assay (Weikel et al., 1992; Mundy and Sears, 1996). In brief, HT-29 cells were cultured for 5 days in a 96-well plate at a density of 2,000 cells/well with Dulbecco’s Modified Eagle Medium (DMEM; Sigma Aldrich, ref.: D6429) supplemented with 10% heat-inactivated fetal bovine serum (FBS; Gibco, ref. 10500) and 1% antibiotic-antimycotic (100×; anti-anti; Gibco, ref.: 15240-062). Cells were then washed thrice with PBS (Gibco, Life Technologies, ref.: 10010-031) and incubated overnight with 20× concentrated B. fragilis cell-free supernatant diluted 1:10 in HT-29 culture medium without FBS at 37°C. Cell rounding was examined by confocal light microscopy (Leica Microsystems GmbH, Mannheim, Germany), images were taken using LAS-AF software (Leica Microsystems) and analyzed using ImageJ (Abràmoff et al., 2004).
Barrier Function Analysis
Caco-2 cell monolayers (passage number 47–57), as a well-established in vitro model for intestinal epithelial barrier function (Srinivasan et al., 2015), were seeded on Millicell Hanging Cell Culture Inserts (Merck Millipore, ref.: MCHT24H48) at a density of 100,000 cells/insert in DMEM supplemented with 1% FBS, 0.1% non-essential amino acids (Gibco, ref.: 11140050) and 0.1% anti-anti. Culture medium was refreshed every 2–3 days in both compartments. Monolayers were allowed to differentiate during 14–21 days at 37°C and 5% CO2 and the TEER was evaluated using the EVOM2 Epithelial Volt/Ohm Meter (World Precision Instruments, Sarasota, FL, United States). Mature monolayers (TEER > 600 Ω∗cm2) were luminally exposed to BHI or bacterial-free concentrated culture supernatant, each diluted 1:10 in Caco-2 culture medium, or to PBS or a final concentration of 5 × 107 MVs in PBS, each diluted 1:2 in Caco-2 culture medium, and incubated for 24 h. To investigate whether B. fragilis supernatant is able to prevent cytokine induced barrier disruption, experimental conditions were pre-incubated basally with 100 ng/ml tumor necrosis factor α (TNF-α; Sigma, ref.: T6674) in combination with 100 ng/ml interferon γ (IFN-γ; Sigma, ref.: I3265) for 1 h, before B. fragilis supernatant was added luminally. After another 24 h of co-incubation, TEER values were assessed and expressed as percentage of the TEER value prior to incubation.
To confirm that relevant findings were not restricted to Caco-2 cells, concentrated B. fragilis culture supernatant was also applied to the more physiological model of colonic patient-derived organoids. Therefore, colonic biopsies were collected in cold PBS, washed thrice with 1% anti-anti in PBS, thrice with 10 mM DTT in PBS and incubated with 2 mM EDTA in PBS for 1 h at 4°C and 5 rpm. Biopsies were transferred to PBS and crypts were separated by several rounds of mechanical shaking. The pooled crypt supernatant was then supplemented with 5% FBS and centrifuged at 400 × g for 8 min at 4°C. The pellet was washed twice with 2 ml cold basal medium (DMEM/F12; Gibco, ref.: 12634-010) supplemented with 1% GlutaMaxTM (Life Technologies), 1% Hepes buffer (Life Technologies) and 5% FBS. After centrifugation at 400 × g for 3 min, crypts were plated in GelTrexTM (Gibco, ref: A1413201) and IntestiCultTM Organoid Growth Medium (StemCell Technology GmbH, Germany, ref.: SC-06010) was added 15 min after incubation at 37°C, 5% CO2. Medium was refreshed every 3 days and passaged when the morphology appeared complex, according to previous descriptions (Sato et al., 2011). In brief, GelTrexTM with enclosed organoids was solubilized on ice for 15 min. Culture medium was replaced by ice cold basal medium. Organoids were disrupted mechanically using a 1000P pipette and centrifuged at 150 × g for 5 min. The supernatant was replaced by TrypLETM Express (Thermofisher Scientific, ref: 12605010) containing 10 μM y27632 (Tebu-bio BV) and incubated at 37°C for 2 min. Organoids were then dissociated mechanically using a firepolished Pasteur pipetted, washed and centrifuged twice with basal culture medium at 400 × g for 5 min. Finally, the pellet was resuspended with GelTrexTM and cultured as described above.
Single lumen organoids (passage numbers 10 and 11) were incubated basolaterally with BHI or B. fragilis culture supernatant, diluted 1:10 in organoid medium, or organoid medium only at 37°C for 24 h. Epithelial barrier function was evaluated by 1 mg/ml fluorescein isothothiocyanate-labeled dextran of 4 kDa (FITC-d4; Sigma) added 6–8 h before confocal light microscopy (Leica Microsystems GmbH, Mannheim, Germany). Images were taken using LAS-AF software and processed using ImageJ. The experiment was conducted twice and at least eight organoids were evaluated per condition and experiment.
Analysis of Tight- and Adherens Junction Expression
After TEER measurements of Caco-2 monolayers, RNA was isolated using RNeasy Mini Kit (Qiagen) according to manufacturer’s instructions, including DNAse treatment for 15 min. RNA quantity and purity were determined using a Nanodrop spectrophotometer (NanoDrop Technologies, Wilmington, United States). cDNA was synthesized using 75% RNA template, 20% iScript reaction mix and 5% reverse transcriptase (by iScript cDNA Syntgeses Kit BioRad) and the recommended cycle of 5 min 25°C, 30 min 42°C and 5 min 85°C.
Real-time qPCR was performed according to manufacturer’s instructions using SYBR Green Supermix (BioRad, Veenendal, Netherlands, ref.: 1708885) and CFX 96 Real Time System C1000 Touch Thermal Cycler (Bio-Rad). Primer sequences are listed in Supplementary Table 3. Expression of mRNA was analyzed relative to GAPDH, using the 2–ΔCt method (Schmittgen and Livak, 2008).
Analysis of Tight Junction Localization
After TEER measurements of Caco-2 monolayers, the cells were washed with PBS, fixated with 4% (w/v) paraformaldehyde as described previously (Elamin et al., 2012). The protocol was adjusted by 30 min permeabilization with 0.5% (v/v) Triton X-100 and washing with PBS instead of Hank’s balanced salt solution. Monolayers inside the transwells were incubated with FITC-conjugated Zonulin-1 antibodies (1:200; Invitrogen, ref.: 339111) overnight at 4°C. Confocal images were obtained as described in section “Barrier Function Analysis.”
Whole Genome Sequencing
To characterize the isolated B. fragilis strains and to predict possible differences in secreted proteins, the genome of all six strains was sequenced. Genomic DNA was isolated from single colonies resuspended in PBS using MasterPureTM Complete DNA and RNA purification kit (Epicenter, MC 85200), following the manufacturer’s protocol. Library preparation sequencing and de novo assembly was performed as described previously (Flipse et al., 2019). Coding sequences were annotated using Prokka (v1.7) (Seemann, 2014). Gene presence/absence was determined using Roary (v3.11.2) (Page et al., 2015). SNP distances among isolates was determined using SKA (v1.0) (Harris, 2018). All bioinformatics tools were run in default settings. Sequencing data are deposited at European Nucelotide Archive (ENA) with accession number PRJEB41450.
Metabolite Identification Using NMR Spectroscopy
To further examine potential differences in excreted metabolites by the different B. fragilis strains, the culture supernatants were analyzed using proton (1H) NMR spectroscopy. Therefore, 150 μl of 20× concentrated B. fragilis culture supernatant was diluted in 420 μl 100 mM phosphate buffer (pH 7.4 at 25°C) and 30 μl Deuterium oxide (D2O, 99 atom%D, 100G, Sigma-Aldrich, Germany) containing 1 mM 3-(trimethylsilyl)propiomic-2,2,3,3-d4 acid (TSP, 98 atom%D TSP, 1G, Sigma-Aldrich, Germany). The samples were then transferred into a 5 mm NMR tube (Bruker samplejet, Sigma-Aldrich, Germany). All 1H NMR spectra were recorded on a 700 MHz Bruker Avance spectrometer (Bruker, Germany) at 300 K. Spectra were acquired using a one-dimensional NOESY-presat pulse sequence (RD-90°-t-90°-tm-90°-ACQ), an acquisition time of 2 s, a relaxation delay (D1) of 5 s, mixing time (D8) of 100 ms, receiver gain of 32, 64 scans, 45 K data points and a spectral width of 11,161 Hz (15.934 ppm). Spectral preprocessing and preliminary comparisons of the spectra were performed using the Bruker TopSpin 3.2 software.
Statistical Analyses
In order to compare the prevalence and relative abundance of B. fragilis (virulence factors) in active and remissive CD patients’ samples, the χ2-test with Yate’s correction and Wilcoxson rank sum test (for samples tested positive for the respective gene) were performed. To predict carriage of B. fragilis, based on the combination of patient baseline characteristics (age, gender, Montreal classification, and medication use), multivariate logistic regression was conducted using backward stepwise regression based on the likelihood ratio. Analyses were computed in IBM SPSS Statistics 25 and statistical significance was considered when p < 0.05.
Comparing the effects of B. fragilis supernatant and MVs on TEER of Caco-2 epithelial monolayers one-way ANOVA and Tukey’s post hoc test was performed using GraphPad Prism 5. For subsequent analysis of differences in tight junction expression, student’s t-test was applied. Statistical significance was considered when p < 0.05. Analyses were based on at least duplicate experiments with each three technical replicates.
Partial Least Squares Discriminant Analysis (PLS-DA) was conducted to identify biologically relevant metabolites that differ between TEER-increasing and non-TEER-increasing groups using MetaboAnalyst v2.0 (Chong et al., 2019). The selection of the most discriminating metabolites, that are responsible for the pattern of the PLS-DA score plot, was based on both the interpretation of PLS-loading plot and Variable Importance in Projection (VIP) scores. Metabolites were identified using HMDB 4.0 (The Human Metabolome Database) (Wishart et al., 2018).
Results
Patient Population
In total, 181 CD patients, 88 with active disease and 93 in remission, from the IBDSL (van den Heuvel et al., 2015) cohort fulfilling the inclusion criteria were available for the present study. Baseline characteristics were comparable between patient groups, although steroid use was slightly higher among patients with active disease (Table 1).
B. fragilis Prevalence and Relative Abundance
Based on the detection of the B. fragilis specific gyrB gene, the prevalence of B. fragilis was significantly higher among CD patients during an exacerbation (78.4%) when compared to those in remission (62.4%, p = 0.018, Figure 1A). We subsequently determined the prevalence of the B. fragilis virulence factors bft and ubb. Only 11.4 and 7.5% (p = 0.376) of the samples from the exacerbation and remission group, respectively, were positive for bft. When considering only samples that were tested positive for B. fragilis, this equals to a proportion of bft-positive samples of 14.5 and 12.1% (p = 0.689), respectively. For ubb, the prevalence was 19.3 and 11.8% (p = 0.164) in the entire exacerbation and remission group, respectively, and 24.6 and 19.0% (p = 0.442), when considering the gyrB positive samples only (Figure 1A). The relative abundance of gyrB, bft and ubb was not significantly different between remission and exacerbation (Figures 1B–D).
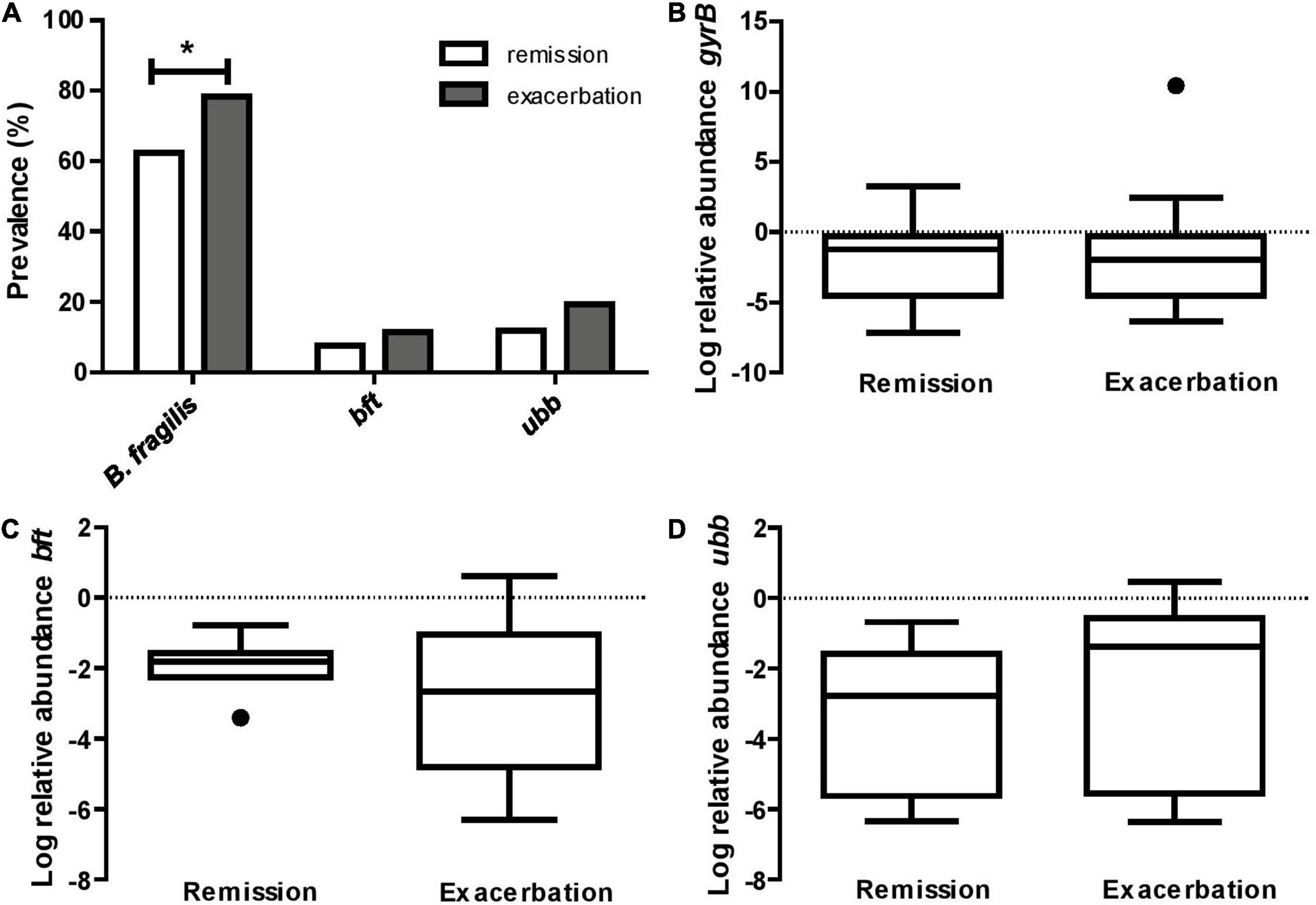
Figure 1. B. fragilis is more prevalent in CD exacerbation samples. (A) The prevalence of B. fragilis specific gyrB is 16.0% higher in active CD compared to remission (*p = 0.018), while the prevalence of the virulence factors bft and ubb does not differ significantly between groups (p = 0.376 and p = 0.164, respectively). The relative abundance of gyrB (B), bft (C), and ubb (D), based on 16S rRNA gene copy number, does not significantly differ between active CD and remission (p = 0.837, p = 0.283 and p = 0.196, respectively).
Based on multivariable logistic regression, we found the following patient characteristics to be correlated with overall B. fragilis colonization: a stricturing disease behavior (Odds ratio (OR) = 3.212, 95% CI = 1.218; 8.469, p = 0.018), active Crohn’s disease (OR = 2.048, 95% CI = 1.042; 4.027 p = 0.038), and previous intestinal resections (OR = 0.406, 95% CI = 0.194; 0.848, p = 0.016).
B. fragilis Strain Isolation and Characteristics
In order to examine the impact of B. fragilis and its virulence factors on barrier function, we selected five B. fragilis strains cultured from samples of two healthy controls (HC-1, HC-2) and three CD patients (CD-1, CD-2, CD-3) and additionally included one reference strain (ATCC® 25285TM). Based on whole genome sequencing, pairwise genetic distance revealed only 160 SNPs between the CD-2 (bft-positive) and the CD-1 (bft-negative) strains, whereas the median distance was 16960 SNPs among all strains (Figure 2A). The genomic similarity was 89.7%, based on shared split kmers between these two strains using Jaccard dissimilarity (mean Jaccard index 59.9%). This indicates a large shared genomic backbone in these two strains. Sanger sequencing confirmed the presence of bft-1 in HC-1, CD-2, and CD-3 and the presence of ubb in HC-1 and ATCC® 25285TM with 93–99 and 96–99% sequence similarity, respectively. HC-2, ATCC® 25285TM, and CD-1 were confirmed negative for bft, and HC-2, CD-1, CD-2, and CD-3 for ubb. Cell rounding of subconfluent HT-29 cells could be detected in response to the supernatants of all bft-positive strains, which was interpreted as Bft activity (Figure 2B and Supplementary Figure 1). No cell rounding was detected in bft-negative strains, including both bft deletion mutants, which supported the assay specificity. However, the concentration of Bft was found to be below the detection limit of western blot analysis (Figure 2C). In addition, no cell rounding was detected when applying concentrated culture supernatant of bft-1 positive strains (HC-1, CD-2, CD-3) on Caco-2 cells (data not shown) or colonic organoids (CD-3; Figure 3E), which suggests a cell type-dependent response to Bft. No cell rounding was detected on bft-1 positive strains derived MVs, suggesting that the isolated MVs do not contain or release relevant amounts of (active) Bft (Figure 2D).
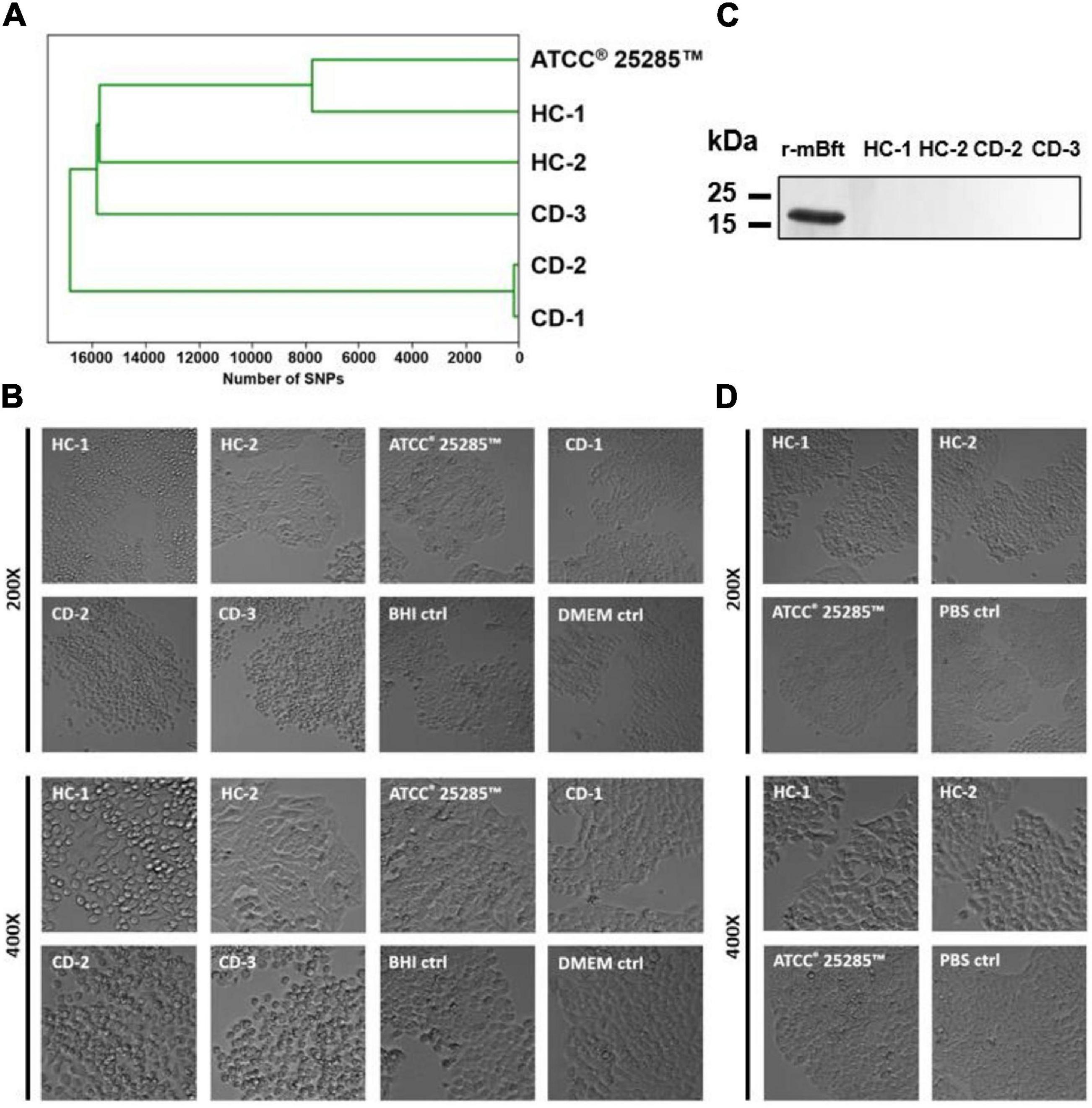
Figure 2. Characteristics of cultured B. fragilis strains. (A) Based on whole genome sequencing, pairwise genetic distance revealed 160 SNPs between CD-1 and CD-2, whereas the median distance was 16960 SNPs. (B) Cell rounding of subconfluent HT-29 cells could be detected in the supernatants of all bft-positive strains (HC-1, CD-2, CD-3). (C) Mature Bft could not be detected in the supernatant of bft-positive strains when compared to 200 ng of recombinant mature Bft (r-mBFT). (D) Cell rounding of subconfluent HT-29 cells could not be detected in response to bft-positive strain-derived MVs.
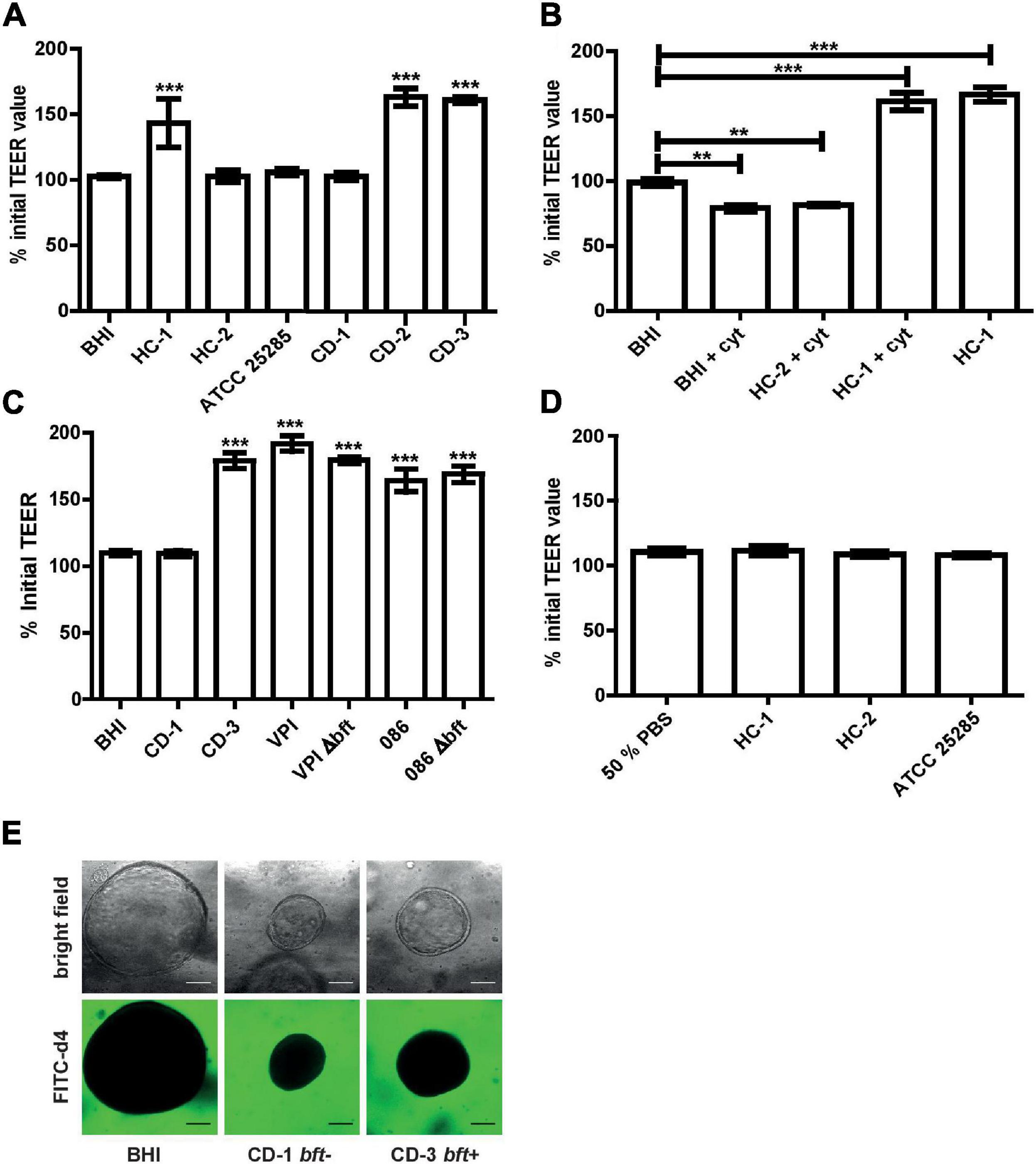
Figure 3. Barrier function examination of Caco-2 monolayer and colonic organoids exposed to B. fragilis culture supernatant and MVs. (A) Luminally applied B. fragilis concentrated culture supernatant of HC-1, CD-2, and CD-3 led to increased TEER after 24 h. (B) Pre-incubation with 100 ng/ml TNF-α and IFN-γ could not impair the TEER enhancing effect of HC-1. (C) Luminally applied B. fragilis concentrated culture supernatant of 086, 086 Δbft-2, VPI, and VPI Δbft-1 led to increased TEER after for 24 h. (D) Luminally applied MVs, isolated from different B. fragilis strains, did not lead to TEER alterations in Caco-2 cell monolayers after 24 h. (E) CD patient-derived colonic organoids treated basally with B. fragilis CD-1 and CD-3 concentrated supernatant and FITC d4 (green) remain a stable barrier after 24 h incubation. Means ± SD was based on triplicates of one representative experiment. cyt, cytokines; BHI, brain heart infusion broth. **p < 0.01, ***p < 0.001.
Barrier Modulation by B. fragilis Supernatant and MVs
After 24 h exposure to concentrated supernatant of bft-positive strains, no decrease in TEER was detected. Instead, TEER values increased up to 160% (p < 0.001) when compared to BHI control and to the concentrated culture supernatant of naturally bft-negative strains (Figure 3A). A similar increase by bft-positive supernatant was also observed after 1 h basal pre-incubation with 100 ng/ml TNF-α and 100 ng/ml IFN-γ (+cyt), while bft-negative culture supernatant did not alter TEER (Figure 3B). To examine the potential role of bft-1 in the observed TEER increase, we applied the concentrated culture supernatant of a bft-1-positive reference strain and its respective deletion mutant. In addition, we examined whether the effect is isotype specific by the additional inclusion of a bft-2 reference strain and its respective deletion mutant.
For bft-1-positive VPI and bft-2-positive 086, as well as their respective deletion mutants, a significant TEER increase was detected (Figure 3C).
Besides culture supernatant, we also examined the effect of MVs, which are vesicles released by Gram positive and Gram negative bacteria and can deliver a variety of products, including toxins, to host cells or neighboring bacteria (Brown et al., 2015; Kaparakis-Liaskos and Ferrero, 2015; Caruana and Walper, 2020). After 24 h incubation with 5 × 107 MVs/ml, no alterations in TEER were observed in differentiated Caco-2 cell monolayers (Figure 3D).
Using a more physiological patient-derived colonic organoid model and a functional FITC-d4 permeation assay, we confirmed that the supernatant of different B. fragilis strains did not disrupt the epithelial barrier nor altered the morphology upon 24 h basal incubation (Figure 3E).
Junctional Alterations in Response to B. fragilis Supernatant
To further elucidate the mechanisms underlying the TEER enhancing effect of Bft-positive culture supernatant, we performed qPCR to analyze the mRNA expression of various junctional genes and the proto-oncogene c-myc in Caco-2 cells. When comparing one bft-positive (CD-3) and one bft-negative (CD-1) strain in quadruplicate, we could not detect any significant alterations in gene expression levels of junctional genes or c-myc (Figure 4 and Supplementary Figure 2). When evaluating ZO-1 protein localization, no alterations were observed comparing exposure to CD-1 and CD-3 supernatants (Supplementary Figure 3).
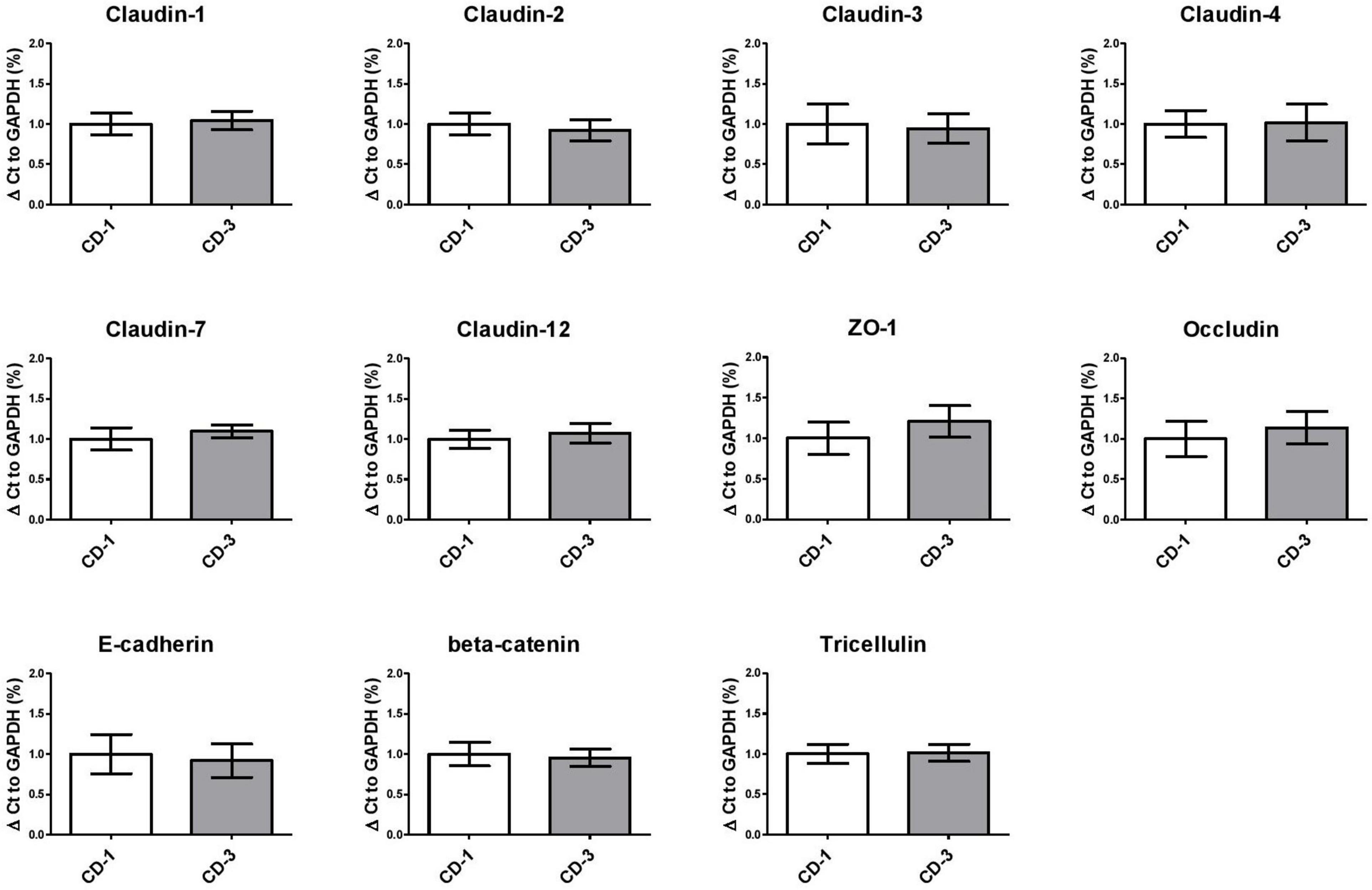
Figure 4. Paracellular junction gene expression in Caco-2 cells exposed to B. fragilis culture supernatants remains unaltered. Tight junction (Claudins, ZO-1, Occludin), adherens junction (E-cadherin, beta-catenin) and tricellulin gene expression were unaltered in B. fragilis bft-positive CD-3 compared to bft-negative CD-1 culture supernatant. Means ± SD were based on two independent experiments. ZO-1, Zonulin 1.
Identification of B. fragilis Genome and Metabolome
To identify potential bacterial proteins that may strengthen the epithelial barrier, coding sequences of TEER-elevating strains were compared to those of non-TEER-elevating strains. Herein, nine proteins were identified which were only present in TEER-elevating strains: Bft1, Metalloprotease II (MPII), putative amidoligase, transposase like protein, putative transposase/insertion sequence protein, and four hypothetical proteins (Table 2).
To further identify metabolites produced by the different B. fragilis strains that might contribute to the observed barrier enhancing effect, the concentrated culture supernatants were analyzed using 1H Nuclear Magnetic Resonance (NMR) spectroscopy. All strains show comparable metabolic profiles (Figure 5A). Based on PLS-DA, the TEER-enhancing and non-TEER-enhancing groups could be discriminated based on a decreased relative concentration of acetate and lactate in the TEER-enhancing group (Figure 5B).
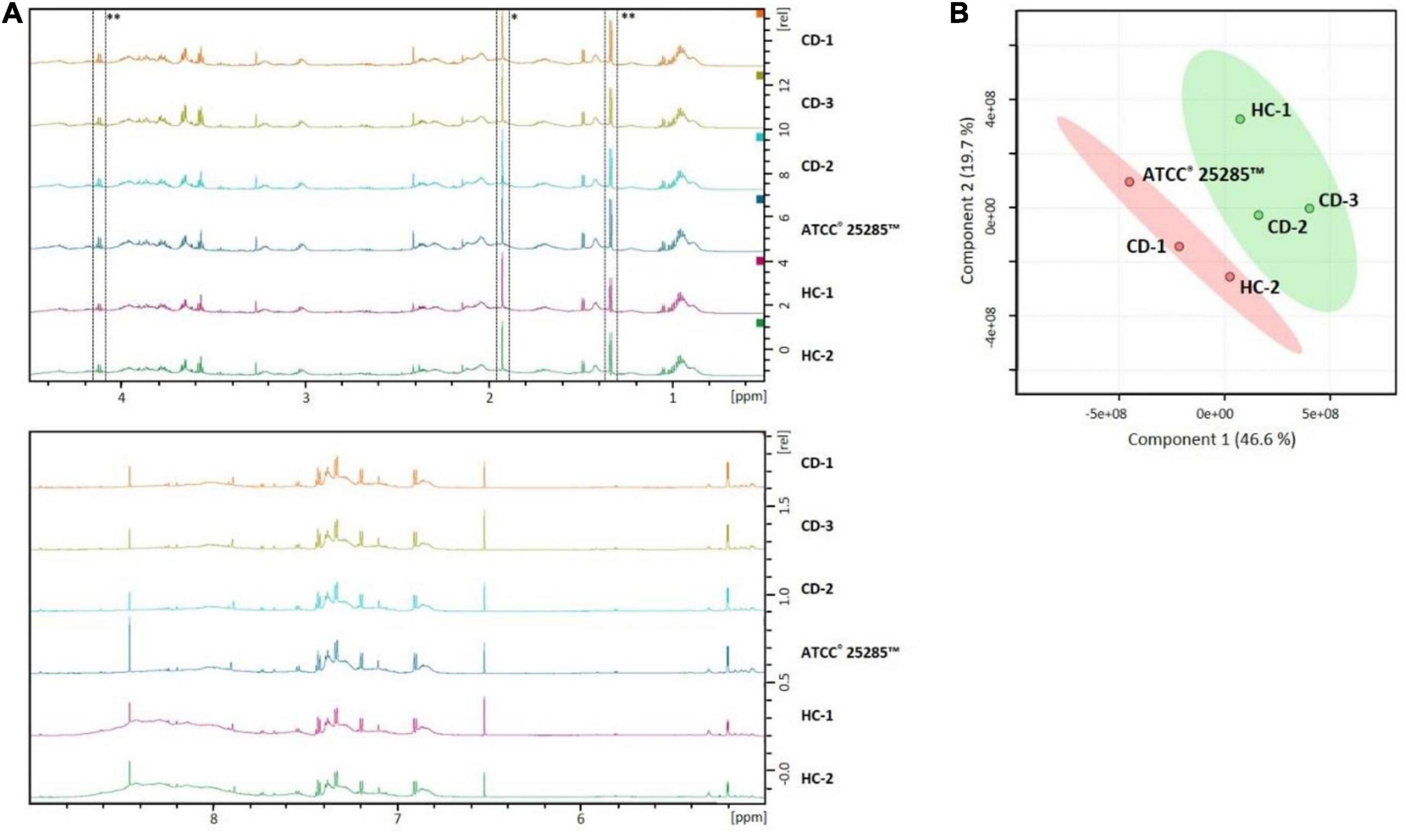
Figure 5. NMR profiles of B. fragilis supernatant can distinguish between TEER-enhancing and non-TEER-enhancing strains. (A) The NMR spectra of all strains are largely comparable. Acetate (*) and lactate (**) is relatively decreased in the TEER-enhancing B. fragilis strains HC-1, CD-1, and CD-3 compared to all other strains. (B) PLS-DA plot demonstrates that the TEER-enhancing group (green) can be clearly discriminated from the non-TEER-enhancing (red) group.
Discussion
Previously, B. fragilis colonization has been investigated in CD exacerbations, together providing inconclusive results (Prindiville et al., 2000; Wills et al., 2014; Schäffler et al., 2016; Tedjo et al., 2016). Based on previous research, we hypothesized that B. fragilis and its virulence factors bft and ubb are associated with exacerbations and may contribute to exacerbations by disruption of the intestinal epithelial barrier (Wells et al., 1996; Chambers et al., 1997; Obiso et al., 1997; Wu et al., 1998; Riegler et al., 1999).
In the present study, we indeed observed a higher B. fragilis prevalence among active CD patients when compared to patients in remission, but not in virulence factor-positive strains. Furthermore, disruption of the intestinal epithelial barrier by supernatant of various (non)virulent B. fragilis strains could not be observed. Intriguingly, Bft-positive culture supernatant even led to a significant increase of intestinal epithelial barrier resistance in Caco-2 monolayers.
The cross-sectional investigation of B. fragilis in our large CD cohort demonstrated a higher prevalence in active CD patients. However, no differences in relative abundance were detected when analyzing B. fragilis positive samples. Logistic regression analysis confirmed the association between B. fragilis colonization and disease activity and additionally highlighted an increased likelihood of B. fragilis carriage among patients with a stricturing disease behavior. To our knowledge, an association with disease behavior has previously not been reported. Previous research has shown that B. fragilis lipopolysaccharide can activate Toll-like receptor 4, which in turn has been associated with liver fibrosis and was suggested as a potential mechanism in stricturing CD (Mancuso et al., 2005; Li et al., 2019). This potential mechanism needs further investigation to clarify whether B. fragilis can induce fibrosis and may thereby directly contribute to the development or persistence of strictures.
To study the relevance of B. fragilis virulence factors, we next compared the prevalence and relative abundance of bft and ubb positive strains. Bft and Ubb are two B. fragilis proteins, which have previously been shown to affect the host via direct and indirect interactions, respectively (Wu et al., 1998; Stewart et al., 2018). The metalloprotease Bft has been shown to disrupt the epithelial barrier by cleaving the adherens junctions protein E-cadherin in vitro (Wu et al., 1998), whereas Ubb was found to mimic human Ubb and led to antibody formation in vivo (Stewart et al., 2018). These two compounds were therefore considered of interest and detected in the fecal metagenomes of CD patients.
In our cohort, no difference was found in virulence factor positive B. fragilis strains when comparing active and remissive CD. Further analyses on associations between virulence factor carriage and CD related parameters were not conclusive due to the overall low prevalence. Although previous rather small scale studies on bft in CD patients could also not demonstrate a link with disease exacerbations (Prindiville et al., 2000; Zitomersky et al., 2013) and studies on ubb among CD patients are lacking, their gene products might still play a role in intestinal barrier dysfunction and thereby contribute to intestinal inflammation. We therefore subsequently investigated representative bacterial strains for their effect on intestinal epithelial barrier function using a validated Caco-2 barrier function model (Srinivasan et al., 2015). To investigate the functional impact, we chose to apply bacterial culture supernatant as it contains all secreted products, including proteins, metabolites and MVs (Kaparakis-Liaskos and Ferrero, 2015; Sicard et al., 2017; Parker et al., 2018). In vivo, these products can interact with the host by directly targeting the epithelial lining or indirectly via the immune system (Kaparakis-Liaskos and Ferrero, 2015; Parker et al., 2018; Badi et al., 2019).
In contrast to our hypothesis, none of the investigated supernatants led to a disrupted epithelial barrier when examined with TEER analysis. On the contrary, bft-positive culture supernatant significantly increased epithelial barrier resistance. The observed TEER increase was comparable for both, bft-1- and bft-2-positive isotypes, which include the most prevalent isotypes in the human gut (Sears, 2009). Even in the presence of the inflammatory cytokines TNF-α and IFN-γ, which are known to disrupt tight junctions (Eeckhaut et al., 2013; Sturgeon and Fasano, 2016), bft-positive culture supernatant evoked an equivalent TEER increase. As previous studies have described a barrier disrupting effect induced by Bft in some other colonic (adeno)carcinoma cell lines, such as HT-29 or T84 (Chambers et al., 1997; Obiso et al., 1997), but not in others, for instance Caco-2, NCI-H508 and LS174T (Van Tassell et al., 1992), we also tested the effect of bft-positive culture supernatant on a more physiological CD patient-derived colonic organoid model of epithelial barrier function (Xu et al., 2018). This likely confirmed that exposure to bft-positive and bft-negative B. fragilis culture supernatant did not lead to barrier disruption or any conspicuous morphological changes, such as cell rounding, although it remains possible that organoids from different hosts might respond differently.
To further elucidate mechanisms underlying the pronounced TEER elevation, junctional gene expression was examined. The qPCR-based approach showed that altered gene expression levels of junctional proteins are unlikely to contribute to the TEER elevation evoked by Bft-positive culture supernatant. Furthermore, induction of proliferation via the previously described Bft-induced upregulation of the proto-oncogene c-myc (Wu et al., 2003) was found to be unlikely. Other cellular mechanisms might rather be involved in the detected TEER increase, such as alterations in ion flux or post-translational modifications of junctional proteins (Srinivasan et al., 2015; Shigetomi and Ikenouchi, 2018). We further excluded a medium-based effect, since the bacterial culture medium was the same for all strains and was also used in the negative control.
Altogether, the above findings are not in line with previous research on the mechanisms of Bft. Several studies showed that isolated Bft could significantly decrease TEER via the cleavage of the adherens junction protein E-cadherin (Wells et al., 1996; Chambers et al., 1997; Obiso et al., 1997; Wu et al., 1998). In those studies, Bft was purified and titrated to approximate physiological concentrations (Van Tassell et al., 1992; Wells et al., 1996; Chambers et al., 1997; Obiso et al., 1997; Wu et al., 1998). In the present study, we chose to apply the complete culture supernatant of 24 h bacterial cultures instead of purified Bft, to include other secreted molecules and mimic a more physiological situation. Although we succeeded to detect secreted Bft in the supernatant, using a previously established HT-29 cell rounding assay (Weikel et al., 1992), Bft concentrations were below the detection limit of western blot. Furthermore, for barrier function analysis Caco-2 cells were used instead of HT-29 or T84 cells, which might vary in Bft susceptibility (Van Tassell et al., 1992; Chambers et al., 1997; Obiso et al., 1997).
Next to a lack of epithelial barrier disruption in our Caco-2 model, we showed that bft-positive culture supernatant also did not disrupt the epithelial barrier in more physiological, human colonic organoids as mentioned above. This suggests that our findings are not merely the result of a potentially more resistant Caco-2 phenotype. However, as it has been previously described that the response to Bft differs between cell lines, we cannot completely rule out that the lack of response in both, the Caco-2 model and human colonic organoids from a CD patient, might be because of a more resistant phenotype. Based on the previously reported E-cadherin cleavage and subsequent barrier disruption (Obiso et al., 1997; Wu et al., 1998), it seemed rather unlikely that Bft is contributing to the observed increased TEER values in Caco-2 monolayers. We could exclude Bft as causing factor, using additional bft-1- and bft-2-positive reference strains with their respective bft-deletion mutants. Both, wild type and mutants-derived supernatants showed similar TEER elevation as observed in the original bft-positive strains. Therefore, further research was conducted on identifying the potential factor that might induce the observed TEER elevation. As part of the bacterial secretome, B. fragilis MVs were isolated from the supernatant and applied on the Caco-2 monolayers. Since TEER values did not change after 24 h incubation, MVs were excluded as TEER elevating factors. Next, whole genome sequencing was applied to identify strain specific coding sequences, indicating differences in potentially secreted proteins. The predicted proteins were related to the pathogenicity island, including bft and metalloprotease II (mpII), the transposon machinery, including transposase like protein and putative transposase/insertion sequence protein, and a putative amidoligase was predicted. So far, there is no reported evidence on interaction with amidoligases and tight or adherens junctions. Considering the related functions of the other predicted proteins, they seem rather unlikely to induce TEER elevation, except for MPII, which has earlier been shown to bind to E-cadherin (Remacle et al., 2014). Since the close phylogeny of one TEER-elevating and one non-TEER-elevating strain combined with our observed TEER elevation in the bft deletion mutants offer limited possibilities for the involvement of other genes, we hypothesize that MPII likely contributed to the TEER elevation. Finally, relative concentrations of acetate and lactate were lower in TEER-increasing strains. However, short chain fatty acids, including acetate, and lactate are reported to rather strengthen the epithelial barrier (Bruininx et al., 2010; Zheng et al., 2017). The lower acetate and lactate concentrations found in the supernatant of TEER-enhancing strains are therefore unlikely to explain the TEER-enhancing effect. Altogether, we could not elucidate the mechanism underlying the significant increase in TEER by bft-positive B. fragilis strains.
Besides the effect of Bft-containing supernatant, we further evaluated the impact of ubb-positive strains. As mentioned above, we could not observe epithelial disruption in this condition. So far, little is known about the pathophysiological impact of Ubb, but it has been associated with chronic auto-immune diseases, including ulcerative colitis (Stewart et al., 2018). Since we could not find an impact on barrier function, future studies should focus on the interaction of Ubb with other pathophysiological factors, especially pro-inflammatory immune mediators, in Crohn’s disease. A combined clinical and in vitro study could explore the presence of anti-Ubb antibodies in CD patients and the subsequent immune response.
In summary, this study shows a higher B. fragilis, but not bft or ubb, prevalence in CD exacerbations and an association with a stricturing disease course. Surprisingly, the direct effect of B. fragilis products on colonic epithelial cells led to a significant TEER increase in bft-positive strains, which might indicate a barrier stabilizing effect. This was, however, not due to Bft. More detailed pathophysiological mechanisms and a potential clinical relevance need further investigation. Additionally, this study clearly stresses the need to investigate functional host-microbe interactions to pursue on taxonomic and functional associations based on microbiome research.
Data Availability Statement
The original contributions presented in the study are publicly available. This data can be found here: https://www.ncbi.nlm.nih.gov/PRJEB41450.
Ethics Statement
The studies involving human participants were reviewed and approved by Medical Ethics Committee of the Maastricht University Medical Centre+. The patients/participants provided their written informed consent to participate in this study.
Author Contributions
PS, HB, DJ, and JP conceived this study. HB, FS, LB, AB, DJ, and JP designed the experiments. HB and MP carried out the patient inclusion and sampling. HB, CJ, LB, and PX conducted the experiments (HB: qPCR, TEER, WB, cell rounding, organoids; CJ: whole genome sequencing; LB: NMR spectroscopy; PX: organoids). HB, DJ, and JP drafted the manuscript. All authors revised the article and approved the final version for submission.
Funding
HB was funded by the Nutrim Graduate Program.
Conflict of Interest
The authors declare that the research was conducted in the absence of any commercial or financial relationships that could be construed as a potential conflict of interest.
Acknowledgments
We would like to thank Prof. Cynthia Sears for kindly providing the B. fragilis strains VPI 13784 and 86-5443-2-2, and the respective deletion mutants. This manuscript has been released as a pre-print at bioRxiv, Becker et al. (2020).
Supplementary Material
The Supplementary Material for this article can be found online at: https://www.frontiersin.org/articles/10.3389/fmicb.2021.598232/full#supplementary-material
References
Abràmoff, M. D., Magalhães, P. J., and Ram, S. J. (2004). Image processing with imageJ. Biophotonics Int. 11, 36–41. doi: 10.1201/9781420005615.ax4
Badi, S. A., Khatami, S., Irani, S., and Siadat, S. D. (2019). Induction effects of Bacteroides fragilis derived outer membrane vesicles on toll like receptor 2, toll like receptor 4 genes expression and cytokines concentration in human intestinal epithelial cells. Cell J. 21, 57–61. doi: 10.22074/CELLJ.2019.5750
Becker, H. E. F., Jamin, C., Bervoets, L., Xu, P., Pierik, M. J., Stassen, F. R. M., et al. (2020). Higher prevalence of Bacteroides fragilis in Crohn’s disease exacerbations and strain-dependent increase of epithelial resistance. bioRxiv [Preprint]. doi: 10.1101/2020.05.16.099358
Benedikter, B. J., Bouwman, F. G., Vajen, T., Heinzmann, A. C. A., Grauls, G., Mariman, E. C., et al. (2017). Ultrafiltration combined with size exclusion chromatography efficiently isolates extracellular vesicles from cell culture media for compositional and functional studies. Sci. Rep. 7:15297. doi: 10.1038/s41598-017-15717-7
Brown, L., Wolf, J. M., Prados-Rosales, R., and Casadevall, A. (2015). Through the wall: extracellular vesicles in gram-positive bacteria, mycobacteria and fungi. Nat. Rev. Microbiol. 13, 620–630. doi: 10.1016/j.physbeh.2017.03.040
Bruininx, E. M. A. M., Koninkx, J. F. J. G., Binnendijk, G. P., Zandstra, T., Heetkamp, M. J. W., van der Peet-Schwering, C. M. C., et al. (2010). Effects of prefermented cereals or the end products of fermentation on growth and metabolism of enterocyte-like Caco-2 cells and on intestinal health of restrictedly fed weanling pigs. Animal 4, 40–51. doi: 10.1017/S175173110999084X
Caruana, J. C., and Walper, S. A. (2020). Bacterial membrane vesicles as mediators of microbe – microbe and microbe – host community interactions. Front. Microbiol. 11:432. doi: 10.3389/fmicb.2020.00432
Casterline, B. W., Hecht, A. L., Choi, V. M., and Bubeck Wardenburg, J. (2017). The Bacteroides fragilis pathogenicity island links virulence and strain competition. Gut Microbes 8, 374–383. doi: 10.1080/19490976.2017.1290758
Chambers, F. G., Koshy, S. S., Saidi, R. F., Clark, D. P., Moore, R. D., and Sears, C. L. (1997). Bacteroides fragilis toxin exhibits polar activity on monolayers of human intestinal epithelial cells (T84 cells) in vitro. Infect. Immun. 65, 3561–3570.
Chong, J., Yamamoto, M., and Xia, J. (2019). MetaboAnalyst 2.0: from raw spectra to biological insights. Metabolites 9:57. doi: 10.3390/metabo9030057
Chung, L., Thiele Orberg, E., Geis, A. L., Chan, J. L., Fu, K., DeStefano Shields, C. E., et al. (2018). Bacteroides fragilis toxin coordinates a pro-carcinogenic inflammatory cascade via targeting of colonic epithelial cells. Cell Host. Microbe 23, 203–214. doi: 10.1016/j.chom.2018.01.007
Costea, P. I., Zeller, G., Sunagawa, S., Pelletier, E., Alberti, A., Levenez, F., et al. (2017). Towards standards for human fecal sample processing in metagenomic studies. Nat. Biotechnol. 35:1069. doi: 10.1038/nbt.3960
Eeckhaut, V., Machiels, K., Perrier, C., Romero, C., Maes, S., Flahou, B., et al. (2013). Butyricicoccus pullicaecorum in inflammatory bowel disease. Gut 62, 1745–1752. doi: 10.1136/gutjnl-2012-303611
Elamin, E., Jonkers, D., Juuti-Uusitalo, K., van Ijzendoorn, S., Troost, F., Duimel, H., et al. (2012). Effects of ethanol and acetaldehyde on tight junction integrity: in vitro study in a three dimensional intestinal epithelial cell culture model. PLoS One 7:e35008. doi: 10.1371/journal.pone.0035008
Flipse, J., von Wintersdorff, C. J. H., van Niekerk, J. M., Jamin, C., van Tiel, F. H., Hasman, H., et al. (2019). Appearance of vanD-positive Enterococcus faecium in a tertiary hospital in the Netherlands: prevalence of vanC and vanD in hospitalized patients. Sci. Rep. 9:6949. doi: 10.1038/s41598-019-42824-4
Floyd, D. N., Langham, S., Séverac, H. C., and Levesque, B. G. (2015). The economic and quality-of-life burden of crohn’s disease in Europe and the United States, 2000 to 2013: a systematic review. Dig. Dis. Sci. 60, 299–312. doi: 10.1007/s10620-014-3368-z
Galazzo, G., Tedjo, D. I., Wintjens, D. S. J., Savelkoul, P. H. M., Masclee, A. A. M., Bodelier, A. G. L., et al. (2019). Faecal microbiota dynamics and their relation to disease course in Crohn’s Disease. J. Crohns Colitis 13, 1273–1282. doi: 10.1093/ecco-jcc/jjz049
Harris, S. R. (2018). Split kmer analysis toolkit for bacterial genomic epidemiology. Biorxiv [Preprint]. bioRxiv: 453142,Google Scholar
Kaparakis-Liaskos, M., and Ferrero, R. L. (2015). Immune modulation by bacterial outer membrane vesicles. Nat. Rev. Immunol. 15, 375–387. doi: 10.1038/nri3837
Lameris, A. L., Huybers, S., Kaukinen, K., Mäkelä, T. H., Bindels, R. J., Hoenderop, J. G., et al. (2013). Expression profiling of claudins in the human gastrointestinal tract in health and during inflammatory bowel disease. Scand. J. Gastroenterol. 48, 58–69. doi: 10.3109/00365521.2012.741616
Li, J., Mao, R., Kurada, S., Wang, J., Lin, S., Chandra, J., et al. (2019). Pathogenesis of fibrostenosing Crohn’s disease. Transl. Res. 209, 39–54. doi: 10.1016/j.trsl.2019.03.005
Li, L., Ning, Z., Zhang, X., Mayne, J., Cheng, K., Stintzi, A., et al. (2020). RapidAIM: a culture- and metaproteomics-based rapid assay of individual microbiome responses to drugs. Microbiome 8:33. doi: 10.1186/s40168-020-00806-z
Mancuso, G., Midiri, A., Biondo, C., Beninati, C., Gambuzza, M., Macrì, D., et al. (2005). Bacteroides fragilis-derived lipopolysaccharide produces cell activation and lethal toxicity via toll-like receptor 4. Infect. Immun. 73, 5620–5627. doi: 10.1128/IAI.73.9.5620-5627.2005
Mundy, L. M., and Sears, C. L. (1996). Detection of toxin production by Bacteroides fragilis: assay development and screening of extraintestinal clinical isolates. Clin. Infect. Dis. 23, 269–276. doi: 10.1093/clinids/23.2.269
Myers, L. L., Firehammer, B. D., Shoop, D. S., and Border, M. M. (1984). Bacteroides fragilis: a possible cause of acute diarrheal disease in newborn lambs. Infect. Immun. 44, 241–244.
Myers, L. L., and Schoop, D. (1987). Association of enterotoxigenic Bacteroides fragilis with diarrheal disease in young pigs. Am. J. Vet. Res. 48, 774–775.
Obiso, R. J., Azghani, A. O., and Wilkins, T. D. (1997). The Bacteroides fragilis toxin fragilysin disrupts the paracellular barrier of epithelial cells. Infect. Immun. 65, 1431–1439. doi: 10.1128/IAI.65.4.1431-1439.199
Page, A. J., Cummins, C. A., Hunt, M., Wong, V. K., Reuter, S., Holden, M. T., et al. (2015). Roary: rapid large-scale prokaryote pan genome analysis. Bioinformatics 31, 3691–3693. doi: 10.1093/bioinformatics/btv421
Parker, A., Lawson, M. A. E., Vaux, L., and Pin, C. (2018). Host-microbe interaction in the gastrointestinal tract. Environ. Microbiol. 20, 2337–2353. doi: 10.1111/1462-2920.13926
Prindiville, T. P., Sheikh, R. A., Cohen, S. H., Tang, Y. J., Cantrell, M. C., and Silva, J Jr (2000). Bacteroides fragilis enterotoxin gene sequences in patients with inflammatory bowel disease. Emerg. Infect. Dis. 6:171. doi: 10.3201/EID0602.000210
Prosberg, M., Bendtsen, F., Vind, I., Petersen, A. M., and Gluud, L. L. (2016). The association between the gut microbiota and the inflammatory bowel disease activity: a systematic review and meta-analysis. Scand. J. Gastroenterol. 51, 1407–1415. doi: 10.1080/00365521.2016.1216587
Remacle, A. G., Shiryaev, S. A., and Strongin, A. Y. (2014). Distinct interactions with cellular e-cadherin of the two virulent metalloproteinases encoded by a bacteroides fragilis pathogenicity island. PLoS One 9:e113896. doi: 10.1371/journal.pone.0113896
Rhee, K. J., Wu, S., Wu, X., Huso, D. L., Karim, B., Franco, A. A., et al. (2009). Induction of persistent colitis by a human commensal, enterotoxigenic Bacteroides fragilis, in wild-type C57BL/6 mice. Infect. Immun. 77, 1708–1718. doi: 10.1128/IAI.00814-08
Riegler, M., Lotz, M., Sears, C., Pothoulakis, C., Castagliuolo, I., Wang, C. C., et al. (1999). Bacteroides fragilis toxin 2 damages human colonic mucosa in vitro. Gut 44, 504–510. doi: 10.1136/gut.44.4.504
Sato, T., Stange, D. E., Ferrante, M., Vries, R. G., Van Es, J. H., Van den Brink, S., et al. (2011). Long-term expansion of epithelial organoids from human colon, adenoma, adenocarcinoma, and barrett’s epithelium. Gastroenterology 141, 1539–1541. doi: 10.1053/j.gastro.2011.07.050
Scanlan, P. D., Shanahan, F., Mahony, C. O., and Marchesi, J. R. (2006). Culture-independent analyses of temporal variation of the dominant fecal microbiota and targeted bacterial subgroups in Crohn’s disease. J. Clin. Microbiol. 44, 3980–3988. doi: 10.1128/JCM.00312-06
Schäffler, H., Kaschitzki, A., Alberts, C., Bodammer, P., Bannert, K., Köller, T., et al. (2016). Alterations in the mucosa-associated bacterial composition in Crohn’s disease: a pilot study. Int. J. Colorectal. Dis. 31, 961–971. doi: 10.1007/s00384-016-2548-z
Schmittgen, T. D., and Livak, K. J. (2008). Analyzing real-time PCR data by the comparative CT method. Nat. Protoc. 3, 1101–1108. doi: 10.1038/nprot.2008.73
Sears, C. L. (2009). Enterotoxigenic Bacteroides fragilis: a rogue among Symbiotes. Clin. Microbiol. Rev. 22, 349–369. doi: 10.1128/CMR.00053-08
Seemann, T. (2014). Prokka: rapid prokaryotic genome annotation. Bioinformatics 30, 2068–2069. doi: 10.1093/bioinformatics/btu153
Shigetomi, K., and Ikenouchi, J. (2018). Regulation of the epithelial barrier by post-translational modifications of tight junction membrane proteins. J. Biochem. 163, 265–272. doi: 10.1093/jb/mvx077
Sicard, J.-F., Le Bihan, G., Vogeleer, P., Jacques, M., and Harel, J. (2017). Interactions of intestinal bacteria with components of the intestinal mucus. Front. Cell Infect. Microbiol. 7:387. doi: 10.3389/fcimb.2017.00387
Solberg, I. C., Vatn, M. H., Høie, O., Sauar, J., Jahnsen, J., Moum, B., et al. (2007). Clinical course in Crohn’s disease: results of a Norwegian population-based ten-year follow-up study. Clin. Gastroenterol. Hepatol. 5, 1430–1438. doi: 10.1016/j.cgh.2007.09.002
Srinivasan, B., Kolli, A. R., Esch, M. B., Abaci, H. E., Shuler, M. L., and Hickman, J. J. (2015). TEER measurement techniques for in vitro barrier model systems. J. Lab. Autom. 20, 107–126. doi: 10.1177/2211068214561025
Stewart, L., Edgar, J. D. M., Blakely, G., and Patrick, S. (2018). Antigenic mimicry of ubiquitin by the gut bacterium Bacteroides fragilis: a potential link with autoimmune disease. Clin. Exp. Immunol. 194, 153–165. doi: 10.1111/cei.13195
Sturgeon, C., and Fasano, A. (2016). Zonulin, a regulator of epithelial and endothelial barrier functions, and its involvement in chronic inflammatory diseases. Tissue Barriers 4:e1251384. doi: 10.1080/21688370.2016.1251384
Sun, J., Shen, X., Li, Y., Guo, Z., Zhu, W., Zuo, L., et al. (2016). Therapeutic potential to modify the mucus barrier in inflammatory bowel disease. Nutrients 8:44. doi: 10.3390/nu8010044
Tedjo, D. I., Smolinska, A., Savelkoul, P. H., Masclee, A. A., van Schooten, F. J., Pierik, M. J., et al. (2016). The fecal microbiota as a biomarker for disease activity in Crohn’s disease. Sci. Rep. 6:35216. doi: 10.1038/srep35216
van den Heuvel, T. R., Jonkers, D. M., Jeuring, S. F., Romberg-Camps, M. J., Oostenbrug, L. E., Zeegers, M. P., et al. (2015). Cohort profile: the inflammatory bowel disease south limburg cohort (IBDSL). Int. J. Epidemiol. 46:e7. doi: 10.1093/ije/dyv088
van Passel, M. W. J., Kant, R., Zoetendal, E. G., Plugge, C. M., Derrien, M., Malfatti, S. A., et al. (2011). The genome of Akkermansia muciniphila, a dedicated intestinal mucin degrader, and its use in exploring intestinal metagenomes. PLoS One. 6:e16876. doi: 10.1371/journal.pone.0016876
Van Tassell, R. L., Lyerly, D. M., and Wilkins, T. D. (1992). Purification and characterization of an enterotoxin from Bacteroides fragilis. Infect. Immun. 60, 1343–1350.
Weikel, C. S., Grieco, F. D., Reuben, J., Myers, L. L., and Sack, R. B. (1992). Human colonic epithelial cells, HT29/C1, treated with crude Bacteroides fragilis enterotoxin dramatically alter their morphology. Infect. Immun. 60, 321–327. doi: 10.1128/IAI.60.2.321-327.1992
Wells, C. L., Van de Westerlo, E. M. A., Jechorek, R. P., Feltis, B. A., Wilkins, T. D., and Erlandsen, S. L. (1996). Bacteroides fragilis enterotoxin modulates epithelial permeability and bacterial internalization by HT-29 enterocytes. Gastroenterology 110, 1429–1437. doi: 10.1053/gast.1996.v110.pm8613048
Wills, E. S., Jonkers, D. M. A. E., Savelkoul, P. H., Masclee, A. A., Pierik, M. J., and Penders, J. (2014). Fecal microbial composition of ulcerative colitis and Crohn’s disease patients in remission and subsequent exacerbation. PLoS One 9:e90981. doi: 10.1371/journal.pone.0090981
Wishart, D., Feunang, Y., Macu, A., Guo, A., Liang, K., Vázquez-Fresno, R., et al. (2018). HMDB 4.0 - the human metabolome database for 2018. Nucleic Acids Res. 46, D608–D617. doi: 10.1093/nar/gkx1089
Wu, S., Lim, K. C., Huang, J., Saidi, R. F., and Sears, C. L. (1998). Bacteroides fragilis enterotoxin cleaves the zonula adherens protein, E-cadherin. Proc. Natl. Acad. Sci. U.S.A. 95, 14979–14984. doi: 10.1073/pnas.95.25.14979
Wu, S., Morin, P. J., Maouyo, D., and Sears, C. L. (2003). Bacteroides fragilis enterotoxin induces c-Myc expression and cellular proliferation. Gastroenterology 124, 392–400. doi: 10.1053/gast.2003.50047
Xu, P., Becker, H., Elizalde, M., Masclee, A., and Jonkers, D. (2018). Intestinal organoid culture model is a valuable system to study epithelial barrier function in IBD. Gut 67, 1905–1906. doi: 10.1136/gutjnl-2017-315685
Zeissig, S., Bürgel, N., Günzel, D., Richter, J., Mankertz, J., Wahnschaffe, U., et al. (2007). Changes in expression and distribution of claudin 2, 5 and 8 lead to discontinuous tight junctions and barrier dysfunction in active Crohn’s disease. Gut 56, 61–72. doi: 10.1136/gut.2006.094375
Zheng, L., Kelly, C. J., Battista, K. D., Schaefer, R., Lanis, J. M., Alexeev, E. E., et al. (2017). Microbial-derived butyrate promotes epithelial barrier function through IL-10 receptor-dependent repression of Claudin-2. J. Immunol. 199, 2976–2984. doi: 10.4049/jimmunol.1700105
Zitomersky, N. L., Atkinson, B. J., Franklin, S. W., Mitchell, P. D., Snapper, S. B., Comstock, L. E., et al. (2013). Characterization of adherent bacteroidales from intestinal biopsies of children and young adults with inflammatory bowel disease. PLoS One. 8:e63686. doi: 10.1371/journal.pone.0063686
Keywords: Bacteroides fragilis, Crohn’s disease, barrier function, prevalence, metabolomics, whole genome sequencing, organoids
Citation: Becker HEF, Jamin C, Bervoets L, Boleij A, Xu P, Pierik MJ, Stassen FRM, Savelkoul PHM, Penders J and Jonkers DMAE (2021) Higher Prevalence of Bacteroides fragilis in Crohn’s Disease Exacerbations and Strain-Dependent Increase of Epithelial Resistance. Front. Microbiol. 12:598232. doi: 10.3389/fmicb.2021.598232
Received: 24 August 2020; Accepted: 30 April 2021;
Published: 08 June 2021.
Edited by:
Markus M. Heimesaat, Charité – Universitätsmedizin Berlin, GermanyReviewed by:
Jozsef Soki, University of Szeged, HungaryJuliane Bubeck Wardenburg, Washington University in St. Louis, United States
Copyright © 2021 Becker, Jamin, Bervoets, Boleij, Xu, Pierik, Stassen, Savelkoul, Penders and Jonkers. This is an open-access article distributed under the terms of the Creative Commons Attribution License (CC BY). The use, distribution or reproduction in other forums is permitted, provided the original author(s) and the copyright owner(s) are credited and that the original publication in this journal is cited, in accordance with accepted academic practice. No use, distribution or reproduction is permitted which does not comply with these terms.
*Correspondence: John Penders, j.penders@maastrichtuniversity.nl; Daisy M. A. E. Jonkers, d.jonkers@maastrichtuniversity.nl