- 1Food and Pharmaceutical Engineering Institute, Guiyang University, Guiyang, China
- 2Guizhou Fruit Processing Engineering Technology Research Center, Guiyang, China
- 3College of Food and Bioengineering, Henan University of Science and Technology, Luoyang, China
- 4College of Food Science and Engineering, Changchun University, Changchun, China
Cronobacter sakazakii is an opportunistic food-borne pathogen that endangers the health of neonates and infants. This study aims to elucidate the antibacterial activity and mechanism of Chrysanthemum buds crude extract (CBCE) against C. sakazakii and its application as a natural disinfectant. The antibacterial activity was evaluated by the determination of the diameter of inhibition zone (DIZ), minimum inhibitory concentration (MIC), and minimum bactericide concentration (MBC). The antibacterial mechanism was explored based on the changes of growth curve assay, intracellular ATP concentration, membrane potential, intracellular pH (pHin), content of soluble protein and nucleic acid, and cell morphology. Finally, the inactivation effects of CBCE against C. sakazakii in biofilm on stainless steel tube, tinplate, glass, and polystyrene were evaluated. The results showed that the DIZ, MIC, and MBC of CBCE against C. sakazakii were 14.55 ± 0.44–14.84 ± 0.38 mm, 10 mg/mL, and 20 mg/mL, respectively. In the process of CBCE acting on C. sakazakii, the logarithmic growth phase of the tested bacteria disappeared, and the concentrations of intracellular ATP, pHin, bacterial protein, and nucleic acid were reduced. Meanwhile, CBCE caused the cell membrane depolarization and leakage of cytoplasm of C. sakazakii. In addition, about 6.5 log CFU/mL of viable C. sakazakii in biofilm on stainless steel tube, tinplate, glass, and polystyrene could be inactivated after treatment with 1 MIC of CBCE for 30 min at 25°C. These findings reveal the antibacterial activity and mechanism of CBCE against C. sakazakii and provide a possibility of using a natural disinfectant to kill C. sakazakii in the production environment, packaging materials, and utensils.
Introduction
Cronobacter sakazakii, a gram-negative, facultatively anaerobic, motile emerging food-borne pathogen, can adhere tightly to the surface of equipment, packaging materials, and utensils due to its strong ability for biofilm formation, which is believed to be one of the important reasons for the contamination of food by this pathogen (Friedemann, 2007; Amalaradjou and Venkitanarayanan, 2011). Importantly, C. sakazakii can cause some serious life-threatening diseases including sepsis, meningitis, and necrotizing enterocolitis in infants and adults with immunodeficiency, with a 40–80% mortality rate (Hunter and Bean, 2013). In 2002, the International Commission on Microbiological Standards for Foods (ICMSF) defined C. sakazakii as “a pathogen that leads to serious damage, endangers life or causes long-term, chronic and substantial sequelae to the specific population” (Iversen and Forsythe, 2003). Therefore, it is significant to prevent and control C. sakazakii for the guarantee of food safety and public health.
Previous studies have shown that C. sakazakii mainly leads to food safety risks by contaminating processing equipment, packaging materials, and utensils (Kusumaningrum et al., 2003; Brandl et al., 2014). Currently, the most common way to eliminate C. sakazakii from the environment in which food is exposed is still the use of chemical disinfectants (Meireles et al., 2016). However, C. sakazakii strains have strong efficient biofilm formation ability, which helps weaken the bactericidal effect of chemical disinfectants (Lehner et al., 2005; Kim et al., 2006). Meanwhile, due to the long and unscientific use of chemical disinfectants, some C. sakazakii strains resistant to chemical disinfectants have been found (Park et al., 2015). In addition, the potential toxicity and environmental unfriendliness of chemical disinfectants cannot be ignored, which make consumers unwilling to accept them (Meireles et al., 2016). Therefore, a natural disinfectant with good antibacterial effect is urgently needed.
In recent years, a growing number of researchers are focusing their attention on screening natural antibacterial extracts to inhibit food-borne pathogens, because of their safety and nature (Fei et al., 2018a; Guo L. et al., 2020). Chrysanthemum morifolium is a traditional medicated and edible cash crop and contains polyphenols, flavones, essential oils, and other chemical components, which have been shown to have strong antioxidant, anti-inflammatory, and antibacterial effects (Kim and Lee, 2005; Cui et al., 2014; Kuang et al., 2018). Chrysanthemum buds is made by drying the C. morifolium flowers when they are not fully opened and is widely used in tea drinks and traditional Chinese medicine for its stronger anti-inflammatory effect in China, suggesting that C. buds extract should be used in the development of natural disinfectants.
With this background, the purpose of this study was to assess the antibacterial activity of C. buds crude extract (CBCE) against C. sakazakii and explore the possible action approach by revealing the changes in the growth curves, intracellular ATP concentration, cell membrane potential, intracellular pH (pHin), levels of bacterial protein and nucleic acid, and cell morphology. Moreover, its application as a natural disinfectant was implemented and evaluated.
Materials and Methods
Experimental Materials
All growth media were obtained from Qingdao Hopebio Biotechnology Co. Ltd. (Qingdao, China). All reagents were purchased from Shanghai MacLean Biochemical Technology Co., Ltd. (Shanghai, China). The stainless steel tube, tinplate, glass, and polystyrene were supplied by Luoyang Zhenguo Packaging Material Co. Ltd. (Luoyang, China).
Bacterial Strains
A total of 8 C. sakazakii strains were used in the study; among them, C. sakazakii ATCC 29544 was provided from the American Type Culture Collection (ATCC, Manassas, VA, United States), and C. sakazakii PD2, IN3, CFP6, FP1, V1, F2, and C1 were isolated from prepared dishes, instant noodles, chilled fresh pork, PIF, vegetables, fruits, and cookies, respectively, which has been reported in our previous study (Fei et al., 2018b). All strains were used to evaluate the antibacterial activity of CBCE, and C. sakazakii ATCC 29544 was used to analyze the antibacterial mechanism. Before the experiment was carried out, all strains were stored in Luria–Bertani (LB) broth with 20% glycerol (v/v) at −80°C. The above cultures were streaked on tryptone soya agar (TSA) and incubated at 37°C for 24 h. A single colony of each strain was inoculated into LB broth, followed by incubation at 37°C for 12 h for subsequent study.
Preparation of CBCE
The C. buds were crushed and passed through an 82-mesh sieve to obtain their powders. These powders were dissolved in sterile distilled water (SDW) with a ratio of 1:30 (m:v) and extracted at 70°C for 80 min. Then, the filtrate was collected and the filter residues were further extracted twice by the same method. All the filtrate was concentrated using a rotary evaporator (Zhejiang Nader Scientific Instrument Co. Ltd., Hangzhou, China) at 40°C for 10 h to obtain the paste of CBCE. Finally, the paste was dried into powder by a vacuum freeze-drying machine (Tokyo Riken Co., Ltd., Tokyo, Japan). Specific parameters are as follows: paste thickness is 3 mm, pre-cooling temperature is −55°C, vacuum pressure is 45 Pa, sublimation temperature is 40°C, and drying time is 16 h. The images of C. buds and CBCE powders are shown in Supplementary Figure 1, and the main chemical components of CBCE are shown in Supplementary Table 1.
Determination of the DIZ
The diameter of inhibition zone (DIZ) of CBCE against C. sakazakii used the Oxford cup method according to the previous report (Xu et al., 2019). Briefly, 100 μL of C. sakazakii bacterial suspensions (about 107 CFU/mL) was uniformly plated on the sterilized TSA plates. Three sterile oxford cups with a diameter of 8 mm (Shanghai Precision Instrument Co., Ltd., Shanghai, China) were evenly placed on the TSA surface and gently pressed with a tweezer. Then, 200 μL of CBCE (10 mg/mL) was added in the oxford cups, followed by incubation at 37°C for 24 h. Finally, the DIZ value was determined by taking an average of the diameters of three transparent inhibition zones on the same plate.
Determination of MIC and MBC
The minimum inhibitory concentration (MIC) and minimum bactericide concentration (MBC) of CBCE against eight C. sakazakii strains were determined using the agar dilution method as previously described (Fei et al., 2018a). In brief, 500 μL of TSA media containing different concentrations of CBCE (0, 0.3125, 0.625, 1.25, 2.5, 5, 10, and 20 mg/mL) was added into sterile 24-well plates (Shanghai Precision Instrument Co., Ltd., Shanghai, China), respectively. After the TSA medium was solidified, 2 μL (106 CFU/mL) of bacterial suspension was uniformly coated on the surface of agar block followed by incubation at 37°C for 24 h. MIC was defined as the lowest concentration at which CBCE completely prevented C. sakazakii from growing under visual observation. Further, 100 μL (106 CFU/mL) of C. sakazakii was treated with 10, 20, 40, and 80 mg/mL of CBCE for 30 min, respectively, then was spread onto TSA plates and incubated at 37°C for 24 h. The lowest concentration at which no colonies were observed was considered as the MBC of CBCE against C. sakazakii. In addition, the TSA medium without CBCE was used as negative control, and the TSA medium containing 0.1 mg/mL ampicillin was used as positive control.
Growth Curves
The growth curves of C. sakazakii ATCC 29544 strains with different treatments were determined according to the method described by Shi et al. (2016a), with minor modifications. One milliliter of tested bacteria cultures (OD600 = 0.1) was added into the 96-well microtiter plates (Shanghai Precision Instrument Co., Ltd., Shanghai, China), then CBCE was added to the above solution and mixed well, and the final concentration was adjusted to 0 (control), 0.5, 1, 1.5, and 2 MIC. All samples were cultured at 37°C for 24 h, the optical density (OD)600 values of which were measured by a multimode plate reader (Tecan, Infinite M200 PRO, Männedorf, Switzerland) every 2 h to assess bacterial growth.
Measurement of Intracellular ATP Concentrations
After 107 CFU/mL of C. sakazakii ATCC 29544 strains were treated by 0, 1, and 2 MIC of CBCE at 37°C for 30 min, the intracellular ATP concentrations of tested bacteria were determined on the basis of the previous report (Sánchez et al., 2010). The treated strains were cracked using the ultrasound treatment, and then a treatment of 100°C for 3 min was performed to inactivate the ATP in the system. The above samples were centrifuged at 8,000 × g for 3 min at 37°C to obtain the detected supernatant. Finally, the relative luminescence value representing the intracellular ATP concentration of the tested bacteria was determined using an ATP kit (AmyJet Scientific Inc., Wuhan, China) according to the product instructions.
Membrane Potential Determination
The method reported by Guo et al. (2019) was followed to determine the membrane potential of the tested bacteria. Briefly, 125 μL of bacterial suspension (107 CFU/mL) and 0.5 μL of bis-(1,3-dibutylbarbituriis-(1,3-dibutylbarbituric acid) trimethine oxonol (Beijing Solarbio Science and Technology Co. Ltd., Beijing, China) fluorescent probe were added into a black and opaque 96-well plate and cultured at 37°C for 30 min. Then, CBCE was added to the 96-well plate, and the final concentrations were adjusted to 1 and 2 MIC, respectively. The untreated bacterial suspension was used as a control. After incubating at 37°C for 30 min, the fluorescence intensities of all samples at the excitation wavelength and emission wavelength of 492 and 515 nm, respectively were measured using a multifunctional microplate reader (Beijing Potenov Technology Co. Ltd., Beijing, China).
Determination of pHin
The effects of CBCE on pHin of C. sakazakii ATCC 29544 strains were analyzed based on the method as described by Guo L. et al. (2020). In brief, 1.0 μM carboxyfluorescein diacetate succinimidyl ester dye as the fluorescent probe was added to 108 CFU/mL of bacterial suspensions, then incubated at 37°C for 30 min. After a centrifugation at 11200 × g for 5 min, the cell pellets were obtained and suspended into sterilized normal saline (NS) followed by addition of glucose (10 mM). The mixed system was incubated at 37°C for 30 min and centrifuged at 11,200 × g for 5 min to get the cell pellets. Then, these cell pellets were washed twice and resuspended in NS. One hundred and twenty-five microliters of bacterial suspension was treated by 0, 1, and 2 MIC of CBCE in a black and opaque 96-well plate at 37°C for 20 min. The fluorescence intensities were detected using a multimode plate reader (Tecan, Infinite M200 PRO, Männedorf, Switzerland) at 25°C under the excitation wavelength of 440 nm and emission wavelength of 490 nm. The ratio of fluorescence intensities at 490/440 nm was considered as the pHin of cells. In addition, the fluorescence intensity of the cell-free control was deducted to eliminate the background error.
Measurement of C. sakazakii Protein Levels
About 107 CFU/mL of C. sakazakii ATCC 29544 suspension was treated with 1 MIC of CBCE at 37°C for 3, 6, 9, and 12 h, respectively. The untreated C. sakazakii ATCC 29544 was used as a control. All samples were centrifuged at 11,200 × g for 5 min to obtain the cell pellets and supernatants. The pellets were resuspended in NS and analyzed by sodium dodecyl sulfate-polyacrylamide gel electrophoresis (SDS-PAGE) to determine the effect of CBCE on protein in bacteria according to our previous study (Fei et al., 2018a). The protein contents in supernatants were analyzed to determine the amount of protein leaking by the Coomassie bright blue method as described by He et al. (2014).
Measurement of Nucleic Acid Leakage
The method reported by Ma et al. (2016) was performed to determine the nucleic acid content of C. sakazakii ATCC 29544. Approximately 109 CFU/mL of bacterial suspensions was treated with 0, 0.5, 1, 1.5, and 2 MIC of CBCE, respectively, then incubated at 37°C for 12 h. Every 2 h during incubation, 200 μL of cultures was absorbed and centrifuged at 11,200 × g for 10 min to obtain the supernatants. The OD260 values of supernatants were measured by a multimode plate reader (Tecan, Infinite M200 PRO, Männedorf, Switzerland).
Transmission Electron Microscope Observation
The effects of CBCE on the cell morphology of C. sakazakii ATCC 29544 were observed using a transmission electron microscope (TEM) according to the previous report (Fei et al., 2019). Briefly, C. sakazakii strains were treated by 0 (control), 1, and 2 MIC of CBCE for 4 h followed by centrifuging at 3,000 × g for 5 min to collect the cell pellets. The cell pellets were fixed with 0.1 M sodium phosphate buffer containing 2.5% glutaraldehyde for 12 h, then washed three times with NS. Further, the cells were stored in 1% osmium tetroxide at room temperature for 6 h followed by washing three times with NS again. After the above treatments, the cells were dehydrated with 50, 70, 90, and 100% ethanol for 10 min and embedded in Epon Lx-112 (Ladd Research, Williston, VT, United States). Finally, the samples were stained with uranyl acetate and lead citrate, then observed under TEM (Hitachi, Tokyo, Japan).
Application of CBCE as a Natural Disinfectant
The inhibitory abilities of CBCE against C. sakazakii ATCC 29544 in biofilm on stainless steel tube, tinplate, glass, and polystyrene were assessed according to the report of Fei et al. (2020). In brief, all the materials were sterilized and cut into 50 mm × 20 mm coupons then placed into 50-mL tubes containing 30 mL of bacterial suspensions (107 CFU/mL) followed by incubation at 4°C for 24 h. The coupons were washed twice with SDW for 10 s and cultured in 30 mL of LB broth at 25°C for 24 h then washed twice with SDW for 10 s. The samples were treated with 0, 1, and 2 MIC of CBCE and collected at 0, 10, 20, and 30 min, respectively, then washed twice with 300 mL of SDW for 10 s. Then, 30 mL of sterile phosphate-buffered saline and 3 g glass bead (300–600 μm) were added into the tubes containing the coupon, followed by swirling for 5 min. Finally, the plate count method was used to determine the viable counts of C. sakazakii in phosphate-buffered saline (PBS, pH 7.2). The PBS suspension was serially diluted and plated onto TSA plates, followed by incubation at 37°C for 24 h to enumerate the viable count of C. sakazakii.
Statistical Analysis
All experiments except SDS-PAGE and TEM analysis were repeated three times. The data were expressed as mean values ± standard deviation (SD). The significance test was performed by analysis of variance (ANOVA) in the SPSS 20.0 software (SPSS Inc., Chicago, IL, United States). P < 0.05 was considered as significant difference.
Results
DIZ, MIC, and MBC of CBCE Against C. sakazakii
The DIZs, MICs, and MBCs of CBCE against eight C. sakazakii strains are given in Table 1. The results showed that the DIZs ranged from 14.55 ± 0.44 to 14.84 ± 0.38 mm, and no significant difference (P > 0.05) was found between these strains. The MICs and MBCs of CBCE against all C. sakazakii strains were 10 and 20 mg/mL, respectively.
Growth Curves
As shown in Figure 1, compared with the control group, the growth rates of C. sakazakii ATCC 29544 treated by CBCE were obviously reduced and slowed down with the increase of CBCE concentration. When C. sakazakii was treated by 1 MIC of CBCE, the logarithmic phase of C. sakazakii disappeared. When treating by 2 MIC, C. sakazakii stagnated in the delayed stage and stopped growing.
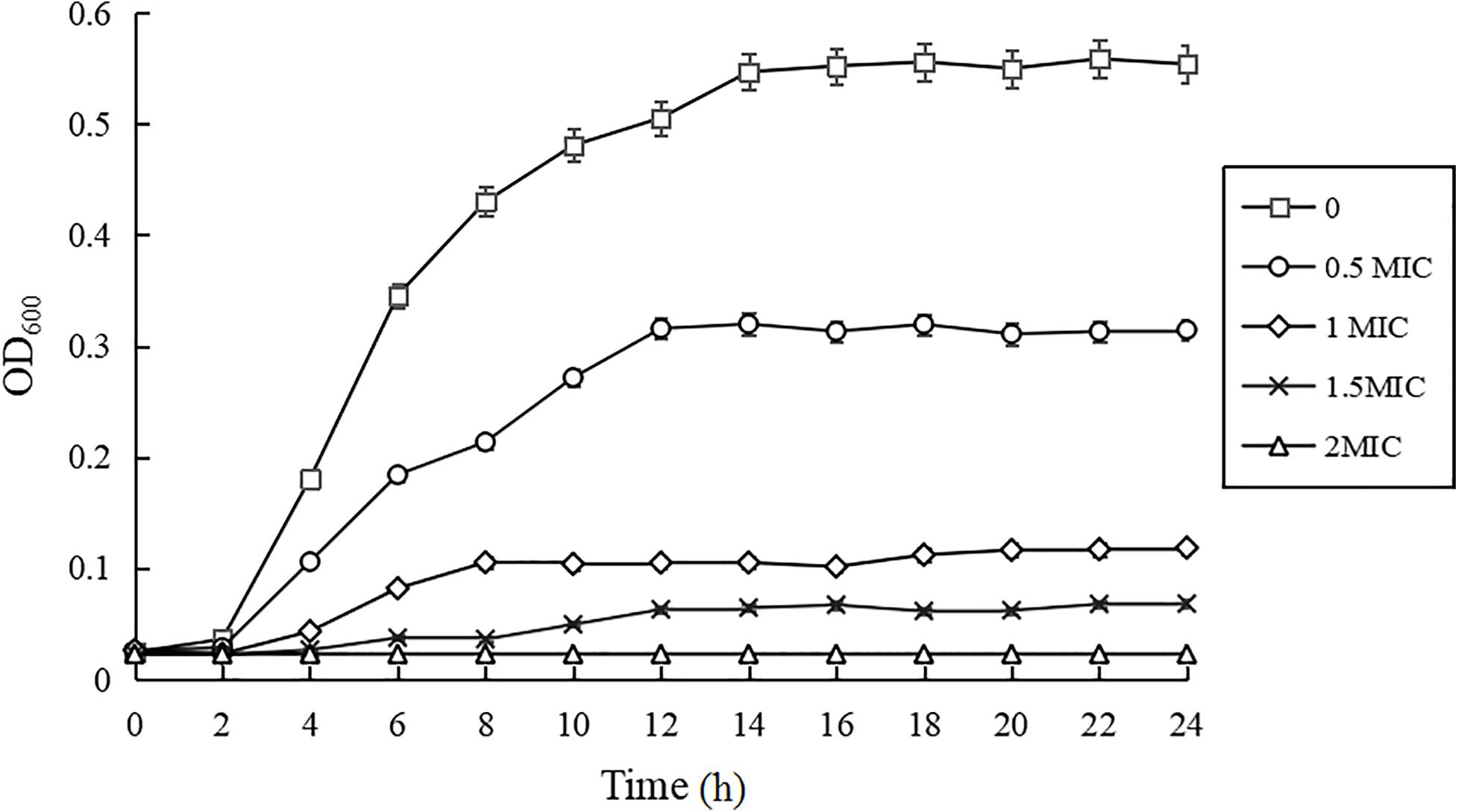
Figure 1. Growth curves of C. sakazakii ATCC 29544 treated with various concentrations of CBCE. Bars represent the SD (n = 3).
Changes in Intracellular ATP Concentration
The effect of CBCE on the intracellular ATP concentration of C. sakazakii ATCC 29544 is shown in Figure 2. Compared to the control group, the intracellular ATP concentration of C. sakazakii ATCC after treatments with 1 and 2 MIC of CBCE decreased significantly (P < 0.05) with the increase of concentration.
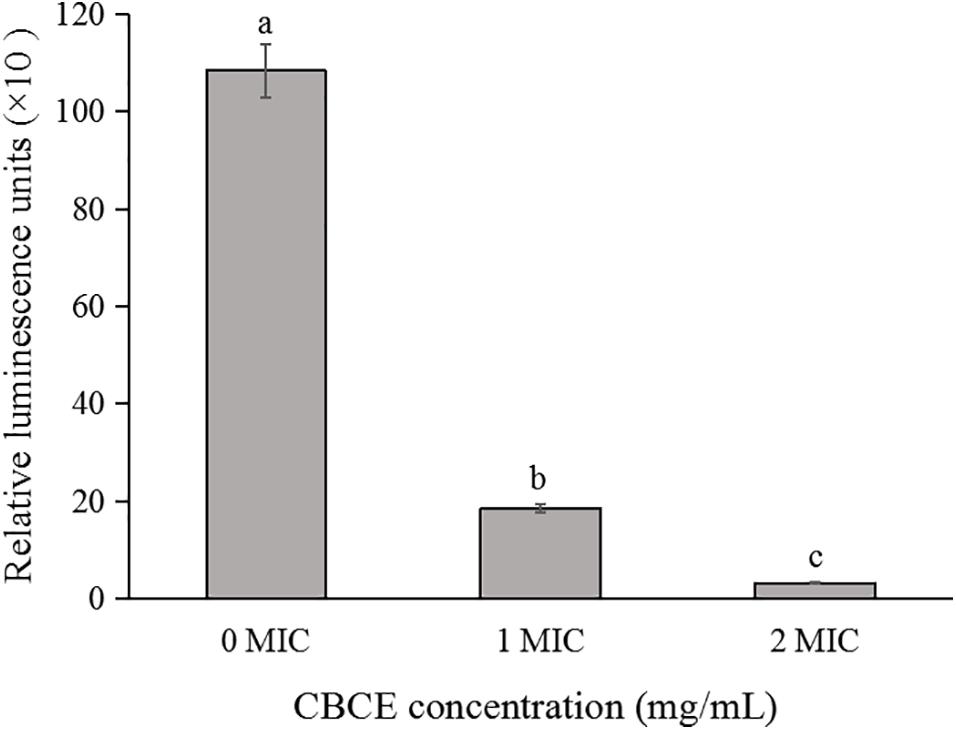
Figure 2. Effects of CBCE on the intracellular ATP concentrations of C. sakazakii ATCC 29544. The mean values of the three independent experiments were used for statistical analysis. Bars represent the SD (n = 3). Different letters represent significant differences (P < 0.05).
Changes in Membrane Potential
Figure 3 illustrates the changes in membrane potential of C. sakazakii ATCC 29544 after treatments with 0, 1, and 2 MIC of CBCE. It can be seen that the cell membrane potential of experimental groups was significantly increased in comparison with the control (P < 0.05), indicating that the membrane potential depolarization occurred in cells treated with CBCE.
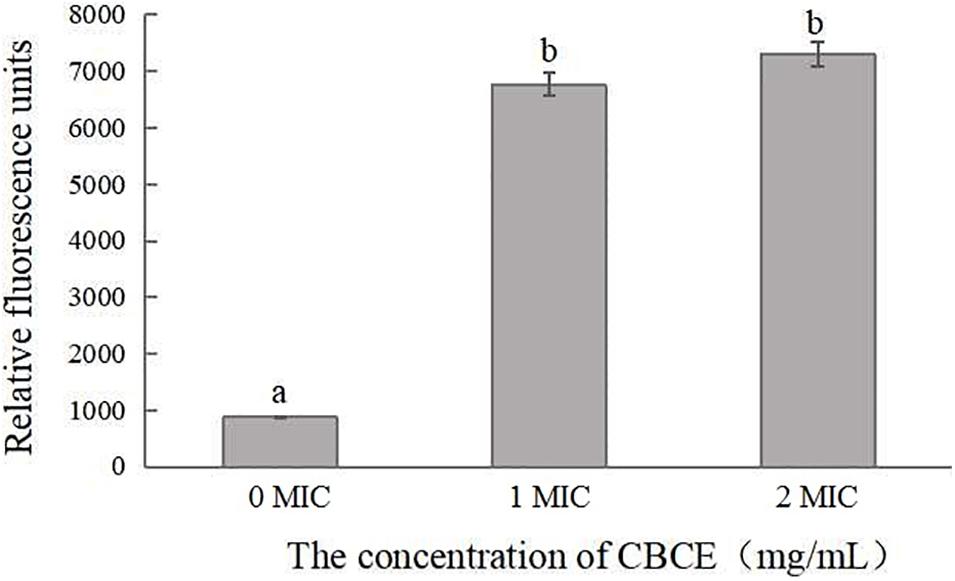
Figure 3. Effects of CBCE on the membrane potential of C. sakazakii ATCC 29544. The mean values of the three independent experiments were used for statistical analysis. Bars represent the SD (n = 3). Different letters represent significant differences (P < 0.05).
Changes in pHin
As shown in Figure 4A, the pHin value had a good linear relationship with the fluorescence ratio of 490 nm and 440 nm (y = −1.1701x + 12.917, R2 = 0.9929). Figure 4B shows that with the addition of CBCE, the pHin value of C. sakazakii ATCC 29544 decreased significantly, and there were significant differences among the three groups (P < 0.05). Specifically, after treatments with 1 and 2 MIC of CBCE, the pHin value dropped from 7.68 ± 0.52 to 4.21 ± 0.19 and 3.01 ± 0.10, respectively.
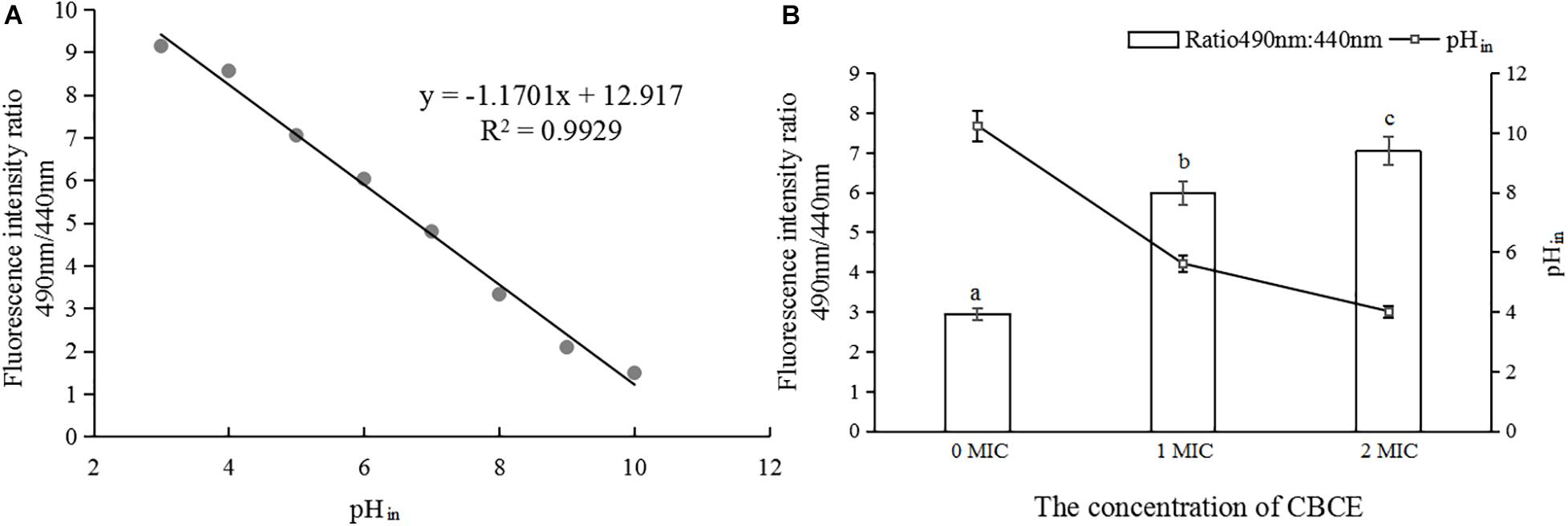
Figure 4. Effects of CBCE on the pHin of C. sakazakii ATCC 29544. (A) The linear relationship between pHin and the ratio of fluorescence intensity at 490 and 440 nm. (B) Changes in pHin of C. sakazakii ATCC 29544 after treatments with 1 and 2 MIC of ATCE. The mean values of the three independent experiments were used for statistical analysis. Bars represent the SD (n = 3). Different letters represent significant differences (P < 0.05).
Changes in C. sakazakii Protein Levels
The changes in protein levels of C. sakazakii ATCC 29544 after treatment with 1 MIC of CBCE are presented in Figure 5. The SDS-PAGE image (Figure 5A) showed the changes in the bacterial protein where the protein bands were obviously weaker and almost disappeared when 1 MIC of CBCE was applied for 12 h. The Coomassie bright blue test result (Figure 5B) showed that the amount of protein leaking from the bacteria significantly increased at different treatment times.
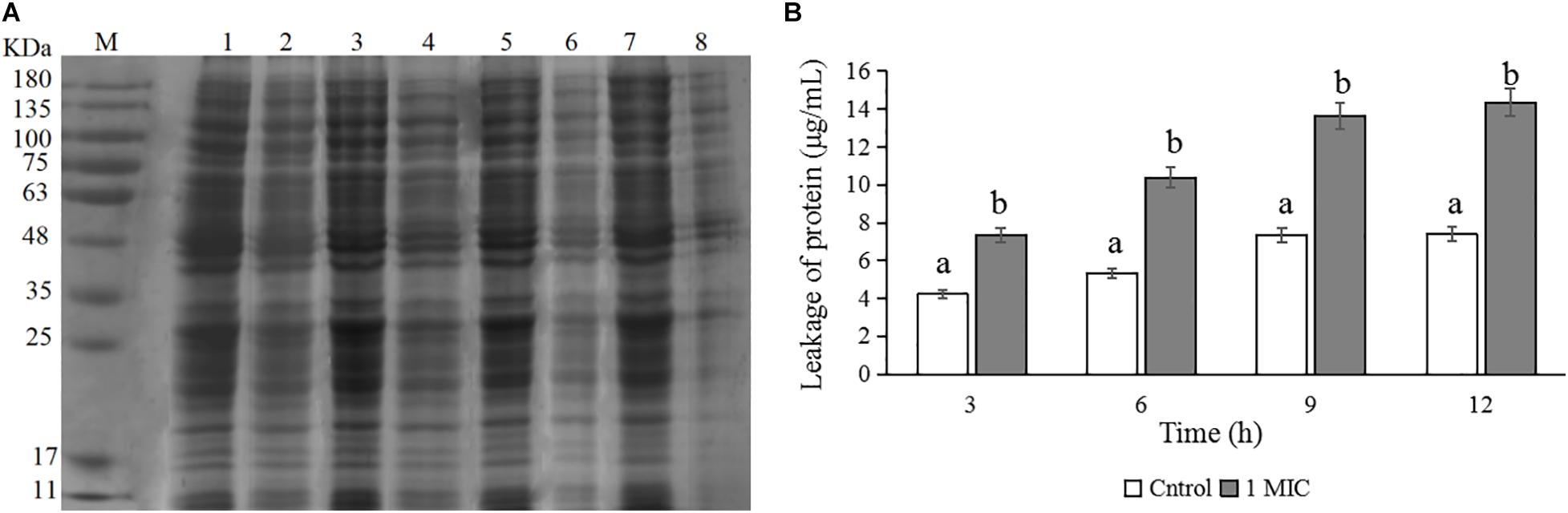
Figure 5. Effects of CBCE on the protein level of C. sakazakii ATCC 29544. (A) SDS-PAGE analysis of the protein in cells treated with 0 and 1 MIC of CBCE. Lane M: marker; Lanes 1, 3, 5, and 7: untreated for 3, 6, 9, and 12 h; Lanes 2, 4, 6, and 8: treated with 1 MIC of CBCE for 3, 6, 9, and 12 h. (B) The amount of protein leaking from cells treated with 0 and 1 MIC of CBCE for 3, 6, 9, and 12 h. The mean values of the three independent experiments were used for statistical analysis. Bars represent the SD (n = 3). Different letters represent significant differences (P < 0.05).
Leakage of the Nucleic Acid
The amounts of nucleic acid leakage from C. sakazakii ATCC 29544 cells were expressed as the absorbance value at 260 nm and given in Figure 6. After treatments with CBCE, the OD260 nm values of supernatants increased significantly with the increase of concentrations, suggesting that CBCE could cause a large amount of nucleic acid leakage from the tested cells.
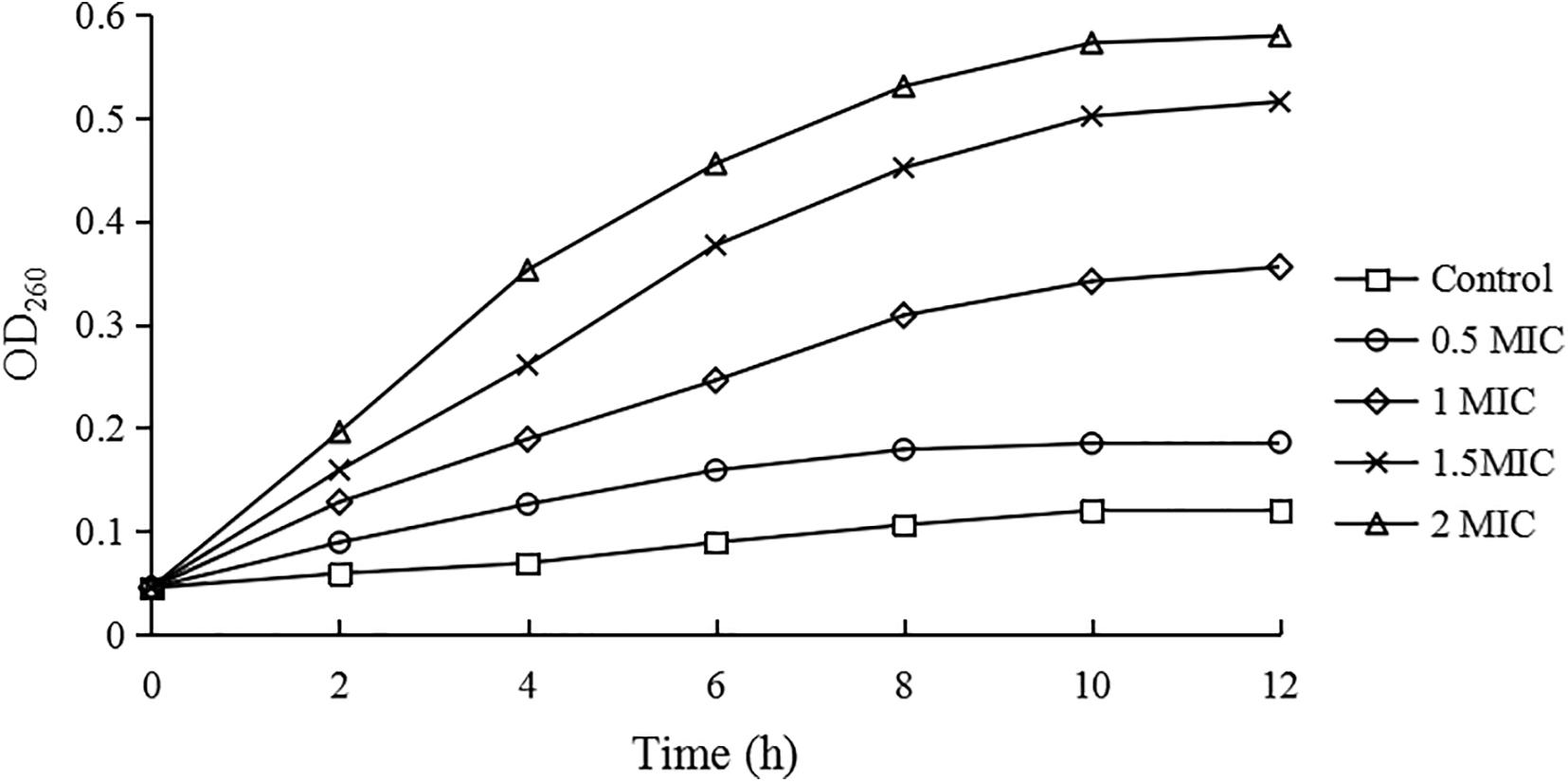
Figure 6. The nucleic acid leakage of C. sakazakii ATCC 29544 after treatments with different concentrations of CBCE. Bars represent the SD (n = 3).
TEM Observation
After treatments with 1 and 2 MIC of CBCE for 4 h, TEM was used to observe the changes in C. sakazakii ATCC 29544 cell morphology and is shown in Figure 7. The untreated cell was used as the control (Figure 7A), which showed regular morphology, smooth surface, and uniform cytoplasm. After treatments with CBCE, the deformation, collapse, and leakage of cell fluid occurred in the tested cells, and the damage degree of cell morphology was more serious with the increase of CBCE concentration (Figures 7B,C).
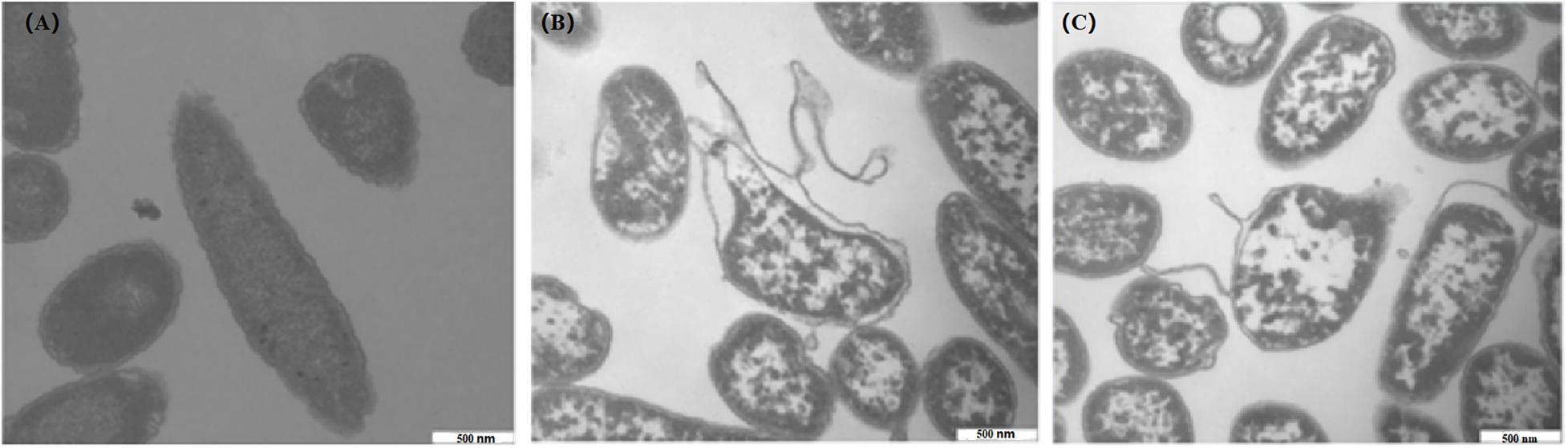
Figure 7. TEM observations (40,000 × magnification) of C. sakazakii ATCC 29544 cells after different treatments. (A) Untreated for 4 h, (B) treated with 1 MIC of CBCE for 4 h, and (C) treated with 2 MIC of CBCE for 4 h.
Inactivation Activity of CBCE
The inactivation activities of CBCE against C. sakazakii ATCC 29544 in biofilm on stainless steel tube (Figure 8A), glass (Figure 8B), tinplate (Figure 8C), and polystyrene (Figure 8D) were evaluated. After treatments with CBCE, the number of viable bacteria in biofilm on the surfaces of the four materials was significantly reduced as compared to the control group (P < 0.05), and about 6.5 log CFU/mL of strains could be completely inactivated after a 1 MIC-30 min treatment or 2 MIC-20 min treatment. In addition, among the four materials, CBCE has the best antibacterial ability against C. sakazakii in biofilm on the polyetherone resin, followed by the stainless steel tube, tinplate, and glass.
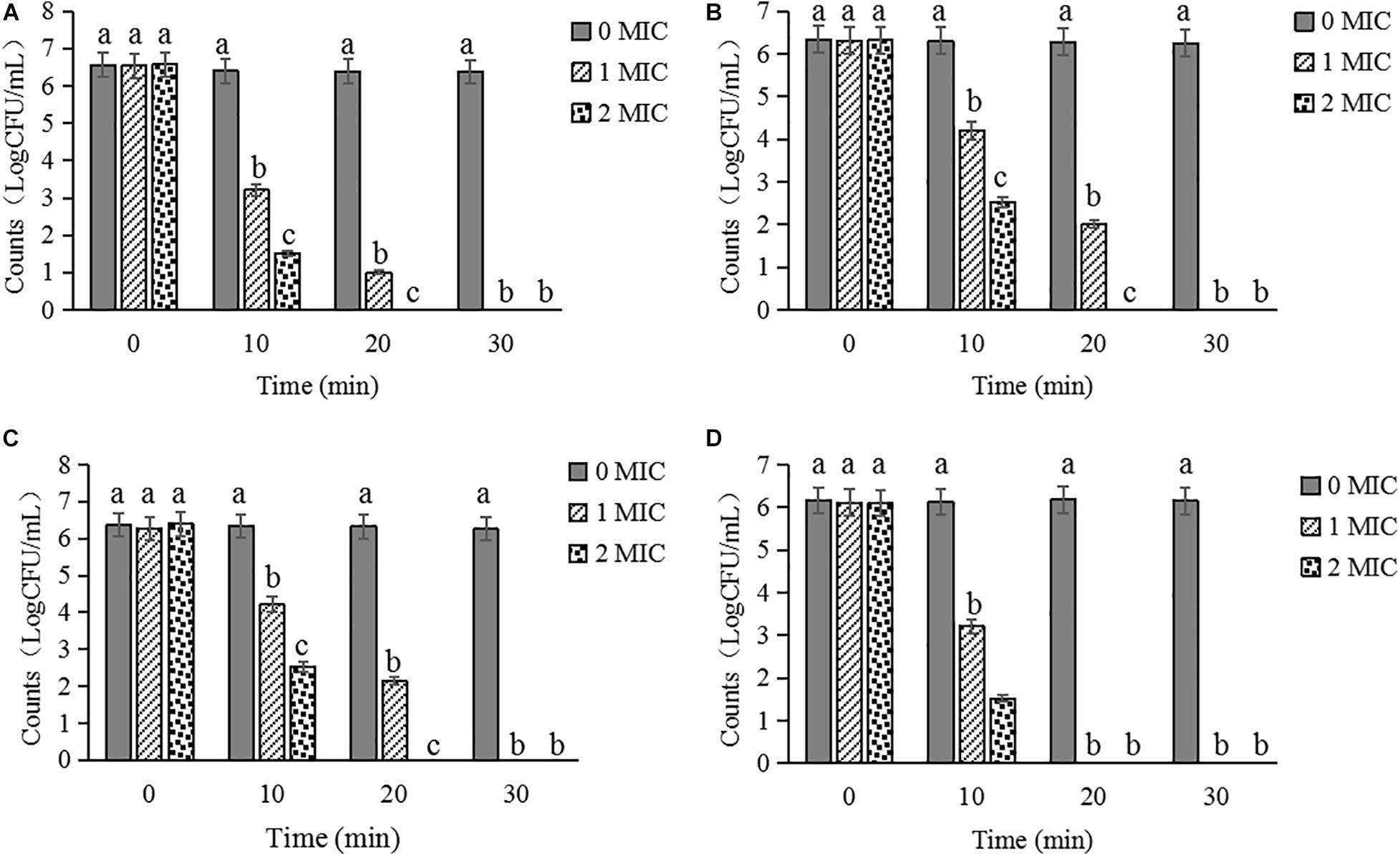
Figure 8. Inactivated effects of CBCE against C. sakazakii strains in biofilm on the (A) stainless steel tube, (B) tinplate, (C) glass, and (D) polystyrene. All strains on coupons were treated by 0, 1, and 2 MIC of CBCE for 30 min at 25°C. Bars represent the SD (n = 3). Different letters denote significant differences (P < 0.05).
Discussion
So far, a variety of natural extracts, such as polyphenols, organic acids, anthocyanins, essential oils, and plant crude extracts, have been shown to have antibacterial effects against C. sakazakii (Amalaradjou et al., 2009; Li et al., 2016). Among these, plant crude extracts were more suitable as the natural disinfectants due to their low cost and simple processing technique. Yemis et al. (2019) found that the MIC of polyphenol-rich pomegranate peel extract against C. sakazakii was 20 mg/mL. Fei et al. (2020) explored the possibility of Amaranthus tricolor crude extract (ATCE) as a potential disinfectant and found that the MIC and MBC of ATCE against C. sakazakii were 20 and 40 mg/mL, respectively. In this study, MIC and MBC were 10 and 20 mg/mL, respectively, which indicated that CBCE had a better antibacterial effect against C. sakazakii.
ATP was closely related to the release, storage, and utilization of energy in the metabolism of substances, so it was considered as an important parameter that could reflect the survival status of cells (Guo et al., 2019). Our findings showed that the CBCE significantly reduced the intracellular ATP concentration of C. sakazakii cells, which is consistent with the changes of ATP concentration after olive oil polyphenol extract (OOPE), ATCE, citral, and syringic acid acted on C. sakazakii cells (Shi et al., 2016a,b; Fei et al., 2018a, 2020). Fei et al. (2018a) believed that the decrease of intracellular ATP concentration was related to the improvement of membrane permeability and excessive hydrolysis of ATP after treatment with natural extracts. Besides, some studies showed that the reduction of ATP synthase activity, loss of K+ inos, and increase in cell hydrolysis rate caused the decrease of ATP content in the cells treated by natural extracts (Pasqua et al., 2010; Sánchez et al., 2010; Khan et al., 2017).
Membrane potential, the potential difference between the two sides of the cell membrane in the resting state of the cell, is an important indicator to characterize the vitality of bacteria and play a pivotal role in the absorption of antibiotics and bactericidal action (Yun and Lee, 2017). When the cell is destroyed, the migration of Na+ can cause the cell membrane depolarization, while the leakage of K+ ions and changes of pH in cells can lead to the hyperpolarization (Bot and Prodan, 2009; Gries et al., 2013). In the current study, the cell membrane depolarization was found in the C. sakazakii strains after treatment with CBCE. Similar results have been reported in previous studies on the bacteriostatic process on nopal cactus methanolic extracts against Vibrio cholerae, lipoic acid against C. sakazakii, and ATCE against Staphylococcus aureus (Sánchez et al., 2010; Shi et al., 2016c; Guo L. et al., 2020). As a comparison, the cell membrane hyperpolarization was observed in the C. sakazakii cells treated by citral and syringic acid (Shi et al., 2016a,b).
Maintenance of pHin homeostasis can ensure the normal operation of bacterial enzymatic reactions, DNA transcription, and protein synthesis (Bracey et al., 1998). In this study, after treatments with CBCE, the pHin of the C. sakazakii cell dropped significantly, which suggested that the DNA and protein synthesis in the tested cell should be disrupted, leading to the growth of bacteria to be inhibited. Similarly, a significant pHin reduction was also found in the tested bacteria during the inhibition of lipoic acid, ATCE, and citral against C. sakazakii, and mustard essential oil against Escherichia coli O157:H7 and Salmonella typhi (Turgis et al., 2009; Shi et al., 2016a,c; Fei et al., 2020). In addition, Fitzgerald et al. (2004) found that once the integrity of the cell membrane was destroyed, bacteria could not maintain a stable pHin through ion exchange inside and outside of the cell membrane. Interestingly, the TEM results in our study showed that CBCE destroyed the cell morphology of C. sakazakii, which is consistent with the above idea.
Previous studies have taken the change in bacterial protein as one of the important ways to reveal the antibacterial mechanism of natural products and found that the bacterial protein of the tested bacteria significantly decreased after the treatments with natural products (Pasqua et al., 2010; Wang et al., 2015; Fei et al., 2018a; Guo L. et al., 2020). Chen et al. (2017) reported that the reduction of bacterial protein was associated with the increase of membrane permeability and obstruction in protein expression and synthesis. Similarly, after treatments with CBCE, the protein bands of C. sakazakii became much weaker, suggesting that the bacterial protein level decreased. In addition, we also found that the protein content in the supernatant increased significantly, which suggested that CBCE enhanced the membrane permeability of C. sakazakii, resulting in a large amount of intracellular protein leakage.
In this study, the amount of nucleic acid leaking from C. sakazakii cells treated by CBCE were significantly increased compared with untreated cells, which was consistent with the previous studies (Ulanowska et al., 2006; Sperotto et al., 2013; Cui et al., 2018). Ulanowska et al. (2006) found that the synthesis of DNA and RNA was disturbed when polyphenols entered the test cells, thereby reducing the expression of genetic information and resulting in antibacterial effects. Sperotto et al. (2013) reported that the natural products can lead to the decrease of the DNA gyrase and ribonucleic acid synthetase activity, thus hindering synthesis and restoration of nucleic acid substance in cell. Furthermore, the study reported by Cui et al. (2018) indicated that the reduction of DNA content under the action of natural products was associated with the destruction of cellular integrity and the damage to the original double helix structure of DNA.
A large number of researches indicated that one of the most important reasons that natural products effectively inhibit the food-borne pathogens is that they can cause the cellular morphology destruction and intracellular component leakage (Rhayour et al., 2003; Borges et al., 2013; Joshi et al., 2014; Fei et al., 2018a, 2020). In the current study, CBCE led to the irreversible changes of C. sakazakii, including cell deformation, collapse and damage, and cell fluid leakage, which was consistent with the previous findings of Fei et al. (2018a, 2020) who revealed the effects of OOPE and ATCE on the morphology of C. sakazakii cells. Meanwhile, the effects of natural products on the cell morphology of food-borne pathogenic bacteria are diverse, due to the differences in the antibacterial mechanism of natural products and the tolerance of the tested bacteria. Rhayour et al. (2003) found that after treatments with clove essential oil, the holes appeared on the cell surface of E. coli, while morphological changes occurred in Bacillus subtilis. Joshi et al. (2014) reported that blueberry proanthocyanidin can only lead to the appearance of pores and bubbles on the surface of C. sakazakii cells, resulting in the reversible antibacterial effect of blueberry proanthocyanidin.
It has been reported that C. sakazakii can attach to the surface of various materials to form the biofilm, thereby providing a certain physical protective barrier for the bacteria to resist a variety of external pressures, such as heat, osmotic pressure, disinfectants, and antibiotics (Borucki et al., 2003). At present, the common way to kill C. sakazakii on equipment and material surfaces in the food industry is to use the chemical disinfectants. However, the excessive and long-term use of chemical disinfectants was liable to cause drug dependence on bacteria to make it less effective, and the potential impact of its also cannot be ignored (Carlie et al., 2020). For example, sodium hypochlorite is so unfriendly to the environment that it has been banned in some European countries (Meireles et al., 2016). Meanwhile, as the common chemical disinfectants, benzalkonium chloride, oxyacetic acid, and chlorine dioxide had lower killing efficacy to C. sakazakii on the polystyrene surface and only removed 18% of the biofilm biomass (Torlak et al., 2015). In the present study, as a natural disinfectant, CBCE has the obvious bactericidal effects against C. sakazakii in biofilm on stainless steel tube, tinplate, glass, and polystyrene; among them, the antibacterial effect against this pathogen on the polystyrene surface was the greatest. Further, after treatments with 1 MIC of CBCE for 30 min at 25°C, approximately 6.5 log CFU/mL of C. sakazakii strains were fully inactivated, which was better than the antibacterial activity of coenzyme Q0 reported by Guo D. et al. (2020) who found that 1 MIC of coenzyme Q0 can significantly decrease but not completely inactivate the C. sakazakii strains on a stainless steel tube under the same treatment time and temperature.
Conclusion
In summary, compared with the reported plant crude extracts, CBCE showed superior antibacterial activity against C. sakazakii and it exerts antibacterial effects by reducing the content of ATP, causing cell depolarization, reducing pHin, promoting the release of protein and nucleic acid substances, and destroying cell morphology. Moreover, CBCE can inactivate C. sakazakii in biofilm on processing equipment, packaging materials, and utensils. If only as a disinfectant, the safety of natural products and their effect on food sensory quality can be ignored; however, the dosage of CBCE needs to be optimized before application. In this study, we prepared the crude extracts of C. buds; therefore, CBCE should be purified in the following study in order to improve its antibacterial effect. In future studies, the focus of research may be on the determination of antibacterial components, combination of natural antibacterial substances and electrolytic water, and development of natural preservatives.
Data Availability Statement
The original contributions presented in the study are included in the article/Supplementary Material, further inquiries can be directed to the corresponding author/s.
Author Contributions
PF and YC conceived and designed the experiments. YC, MX, HF, XH, and MS performed the experiments. PF, YC, and HF supervised the project. YC, MX, XH, and LM analyzed the data. PF, YC, and MX wrote the manuscript. YW and BG contributed to the revision of the manuscript. All authors contributed to the article and approved the submitted version.
Funding
This research was supported by the Key Scientific Research Projects of Institutions of Higher Learning in Henan (21A550007), the Student Research Training Program of College of Food and Bioengineering, Henan University of Science and Technology (SP202006), the Discipline and Master’s Site Construction Project of Guiyang University by Guiyang City Financial Support Guiyang University (SY-2020), the Science and Technology Support Plan Project of Guizhou Provincial Department of Science and Technology [Qian Ke He Support (2020) No. 1Y149], Project of Scientific and Technological Top Notch Talents Support Program of Guizhou Education Department [Qian Jiao He KY (2018) 061], the Science and Technology Public Relations of Henan Province (202102110290), and the Youth Talent Support Project of Henan Province (2020HYTP029).
Conflict of Interest
The authors declare that the research was conducted in the absence of any commercial or financial relationships that could be construed as a potential conflict of interest.
Supplementary Material
The Supplementary Material for this article can be found online at: https://www.frontiersin.org/articles/10.3389/fmicb.2020.632177/full#supplementary-material
Supplementary Figure 1 | The images of C. buds and CBCE powder. (A) C. buds image, (B) CBCE powders image.
References
Amalaradjou, M. A. R., Hoagland, T. A., and Venkitanarayanan, K. (2009). Inactivation of Enterobacter sakazakii in reconstituted infant formula by trans-cinnamaldehyde. Int. J. Food Microbiol. 129, 146–149. doi: 10.1016/j.ijfoodmicro.2008.11.016
Amalaradjou, M. A. R., and Venkitanarayanan, K. (2011). Effect of trans-cinnamaldehyde on inhibition and inactivation of Cronobacter sakazakii biofilm on abiotic surfaces. J. Food Protect. 74, 200–208.
Borges, A., Ferreira, C. M., Saavedra, M. J., and Simoes, M. (2013). Antibacterial activity and mode of action of ferulic and gallic acids against pathogenic bacteria. Microb. Drug Resist. 19, 256–265. doi: 10.1089/mdr.2012.0244
Borucki, M. K., Peppin, J. D., White, D., Loge, F., and Call, D. R. (2003). Variation in biofilm formation among strains of Listeria monocytogenes. Appl. Environ. Microb. 69, 7336–7342. doi: 10.1016/j.fm.2006.06.006
Bot, C., and Prodan, C. (2009). Probing the membrane potential of living cells by dielectric spectroscopy. Eur. Biophysics J. Biophy. 38, 1049–1059. doi: 10.1007/s00249-009-0507-0
Bracey, D., Holyoak, C. D., Nebe-Von Caron, G., and Coote, P. J. (1998). Determination of the intracellular pH (pH(i)) of growing cells of Saccharomyces cerevisiae: the effect of reduced-expression of the membrane H+-ATPase. J. Microbiol. Meth. 31, 113–125. doi: 10.1016/S0167-7012(97)00095-X
Brandl, H., Fricker-Feer, C., Ziegler, D., Mandal, J., Stephan, R., and Lehner, A. (2014). Distribution and identification of culturable airborne microorganisms in a Swiss milk processing facility. J. Dairy Sci. 97, 240–246. doi: 10.3168/jds.2013-7028
Carlie, S. M., Boucher, C. E., and Bragg, R. R. (2020). Molecular basis of bacterial disinfectant resistance. Drug Resist. Update 48:100672. doi: 10.1016/j.drup.2019.100672
Chen, M., Zhao, Z., Meng, H., and Yu, S. (2017). The antibiotic activity and mechanisms of sugar beet (Beta vulgaris) molasses polyphenols against selected food-borne pathogens. LWT Food Sci. Technol. 82, 760–773. doi: 10.1016/j.lwt.2017.04.063
Cui, H., Zhang, C., Li, C., and Lin, L. (2018). Antimicrobial mechanism of clove oil on Listeria monocytogenes. Food Control 94, 140–146. doi: 10.1016/j.foodcont.2018.07.007
Cui, Y., Wang, X., Xue, J., Liu, J., and Xie, M. (2014). Chrysanthemum morifolium extract attenuates high-fat milk-induced fatty liver through peroxisome proliferator-activated receptor α–mediated mechanism in mice. Nutr. Res. 34, 268–275. doi: 10.1016/j.nutres.2013.12.010
Fei, P., Ali, M. A., Gong, S. Y., Sun, Q., Bi, X., Liu, S., et al. (2018a). Antimicrobial activity and mechanism of action of olive oil polyphenols extract against Cronobacter sakazakii. Food Control 94, 289–294. doi: 10.1016/j.foodcont.2018.07.022
Fei, P., Jiang, Y., Gong, S., Li, R., Jiang, Y., Yuan, X., et al. (2018b). Occurrence, genotyping, and antibiotic susceptibility of Cronobacter spp. in drinking water and food samples from Northeast China. J. Food Protect. 81, 456–460. doi: 10.4315/0362-028X.JFP-17-326
Fei, P., Feng, H. X., Wang, Y. Y., Kang, H. B., Xing, M., Chang, Y. H., et al. (2020). Amaranthus tricolor crude extract inhibits Cronobacter sakazakii isolated from powdered infant formula. J. Dairy Sci. 103, 9969–9979. doi: 10.3168/jds.2020-18480
Fei, P., Xu, Y. F., Zhao, S. J., Gong, S. Y., and Guo, L. (2019). Olive oil polyphenol extract inhibits vegetative cells of Bacillus cereus isolated from raw milk. J. Dairy Sci. 102, 3894–3902. doi: 10.3168/jds.2018-15184
Fitzgerald, D. J., Stratford, M., Gasson, M. J., Ueckert, J., Bos, A., and Narbad, A. (2004). Mode of antimicrobial action of vanillin against Escherichia coli, Lactobacillus plantarum and Listeria nnocua. J. Appl. Microbiol. 97, 104–113. doi: 10.1111/j.1365-2672.2004.02275.x
Friedemann, M. (2007). Enterobacter sakazakii in food and beverages (other than infant formula and milk powder). Int. J. Food Microbiol. 116, 1–10. doi: 10.1016/j.ijfoodmicro.2006.12.018
Gries, C. M., Bose, J. L., Nuxoll, A. S., Fey, P. D., and Bayles, K. W. (2013). The Ktr potassium transport system in Staphylococcus aureus and its role in cell physiology, antimicrobial resistance and pathogenesis. Molecul. Microbiol. 89, 760–773. doi: 10.1111/mmi.12312
Guo, D., Wang, S., Li, J., Bai, F., Yang, Y., Xu, Y., et al. (2020). The antimicrobial activity of coenzyme Q0 against planktonic and biofilm forms of Cronobacter sakazakii. Food Microbiol. 86:103337. doi: 10.1016/j.fm.2019.103337
Guo, L., Wang, Y., Bi, X., Duo, K., Sun, Q., Yun, X., et al. (2020). Antimicrobial activity and mechanism of action of the Amaranthus tricolor crude extract against Staphylococcus aureus and potential application in cooked meat. Foods 9:359. doi: 10.3390/foods9030359
Guo, L., Sun, Q., Gong, S., Bi, X., Jiang, W., Xue, W., et al. (2019). Antimicrobial Activity and action approach of the olive oil polyphenol extract against Listeria monocytogenes. Front. Microbiol. 10:1586. doi: 10.3389/fmicb.2019.01586
He, M. Y., Wu, T., Pan, S. Y., and Xu, X. Y. (2014). Antimicrobial mechanism of flavonoids against Escherichia coli ATCC 25922 by model membrane study. Appl. Surf. Sci. 305, 515–521. doi: 10.1016/j.apsusc.2014.03.125
Hunter, C. J., and Bean, J. F. (2013). Cronobacter: an emerging opportunistic pathogen associated with neonatal meningitis, sepsis and necrotizing enterocolitis. J. Perinatol. 33, 581–585. doi: 10.1038/jp.2013.26
Iversen, C., and Forsythe, S. J. (2003). Risk profile of Enterobacter sakazakii, an emergent pathogen associated with infant milk formula. Trends Food Sci. Tech. 14, 443–454. doi: 10.1016/S0924-2244(03)00155-9
Joshi, S. S., Howell, A. B., and D’Souza, D. H. (2014). Cronobacter sakazakii reduction by blueberry proanthocyanidins. Food Microbiol. 39, 127–131. doi: 10.1016/j.fm.2013.11.002
Khan, I., Bahuguna, A., Kumar, P., Bajpai, V. K., and Kang, S. C. (2017). Antimicrobial potential of carvacrol against uropathogenic Escherichia coli via membrane disruption, depolarization, and reactive oxygen species generation. Front. Microbiol. 8:2421. doi: 10.3389/fmicb.2017.02421
Kim, H., Ryu, J., and Beuchat, L. R. (2006). Attachment of and biofilm formation by Enterobacter sakazakii on stainless steel and enteral feeding tubes. Appl. Environ. Microb. 72, 5846–5856. doi: 10.1128/AEM.00654-06
Kim, H. J., and Lee, Y. S. (2005). Identification of new dicaffeoylquinic acids from Chrysanthemum morifolium and their antioxidant activities. Planta Med. 71, 871–876. doi: 10.1055/s-2005-873115
Kuang, C., Lv, D., Shen, G., Li, S., Luo, Q., and Zhang, Z. (2018). Chemical composition and antimicrobial activities of volatile oil extracted from Chrysanthemum morifolium Ramat. J. Food Sci. Tech. Mys. 55, 2786–2794. doi: 10.1007/s13197-018-3203-1
Kusumaningrum, H. D., Riboldi, G., Hazeleger, W. C., and Beumer, R. R. (2003). Survival of foodborne pathogens on stainless steel surfaces and cross-contamination to foods. Int. J. Food Microbiol. 85, 227–236. doi: 10.1016/S0168-1605(02)00540-8
Lehner, A., Riedel, K., Eberl, L., Breeuwer, P., Diep, B., and Stephan, R. (2005). Biofilm formation, extracellular polysaccharide production, and cell-to-cell signaling in various Enterobacter sakazakii strains: aspects promoting environmental persistence. J. Food Protect. 68, 2287–2294. doi: 10.4315/0362-028X-68.11.2287
Li, R., Fei, P., Man, C. X., Lou, B. B., Niu, J. T., Feng, J., et al. (2016). Tea polyphenols inactivate Cronobacter sakazakii isolated from powdered infant formula. J. Dairy Sci. 99, 1019–1028. doi: 10.1016/10.3168/jds.2015-10039
Ma, Q., Davidson, M. P., Critzer, F., and Zhong, Q. (2016). Antimicrobial activities of lauric arginate and cinnamon oil combination against foodborne pathogens: improvement by ethylenediaminetetraacetate and possible mechanisms. LWT Food Sci. Technol. 72, 9–18. doi: 10.1016/j.lwt.2016.04.021
Meireles, A., Giaouris, E., and Simoes, M. (2016). Alternative disinfection methods to chlorine for use in the fresh-cut industry. Food Res. Int. 82, 71–85. doi: 10.1016/j.foodres.2016.01.021
Park, S. Y., Mizan, M. F. R., and Ha, S. D. (2015). Inactivation of Cronobacter sakazakii in head lettuce by using a combination of ultrasound and sodium hypochlorite. Food Control 60, 582–587. doi: 10.1016/j.foodcont.2015.08.041
Pasqua, R. D., Mamone, G., Ferranti, P., Ercolini, D., and Mauriello, G. (2010). Changes in the proteome of salmonella enterica serovar thompson as stress adaptation to sublethal concentrations of thymol. Proteomics 10, 1040–1049. doi: 10.1002/pmic.200900568
Rhayour, K., Bouchikhi, T., Tantaoui-Elaraki, A., Sendide, K., and Remmal, A. (2003). The mechanism of bactericidal action of oregano and clove essential oils and of their phenolic major components on Escherichia coli and Bacillus subtilis. J. Essent. Oil. Res. 15, 286–292. doi: 10.1080/10412905.2003.9698611
Sánchez, E., García, S., and Heredia, N. (2010). Extracts of edible and medicinal plants damage membranes of Vibrio cholerae. Appl. Environ. Microb. 76, 6888–6894. doi: 10.1128/AEM.03052-09
Shi, C., Song, K., Zhang, X., Sun, Y., Sui, Y., Chen, Y., et al. (2016a). Antimicrobial activity and possible mechanism of action of citral against Cronobacter sakazakii. PLoS One 11:e0159006. doi: 10.1371/journal.pone.0159006
Shi, C., Sun, Y., Zheng, Z., Zhang, X., Song, K., Jia, Z., et al. (2016b). Antimicrobial activity of syringic acid against Cronobacter sakazakii and its effect on cell membrane. Food Chem. 197, 100–106. doi: 10.1016/j.foodchem.2015.10.100
Shi, C., Sun, Y., Zhang, X., Zheng, Z., Yang, M., Ben, H., et al. (2016c). Antimicrobial effect of lipoic acid against Cronobacter sakazakii. Food Control 59, 352–358. doi: 10.1016/j.foodcont.2015.05.041
Sperotto, A. R. M., Moura, D. J., Peres, V. F., Damasceno, F. C., Caramão, E. B., Henriques, J. A. P., et al. (2013). Cytotoxic mechanism of piper gaudichaudianum kunth essential oil and its major compound nerolidol. Food Chem. Toxicol. 57, 57–68. doi: 10.1016/j.fct.2013.03.013
Torlak, E., Gokmen, M., and Aydemir, S. (2015). Efficacy of disinfectants against Cronobacter biofilm on plastic surfaces. Qual. Assur. Saf. Crop. 1, 1–5. doi: 10.3920/QAS2013.0423
Turgis, M., Han, J., Caillet, S., and Lacroix, M. (2009). Antimicrobial activity of mustard essential oil against Escherichia coli O157:H7 and Salmonella typhi. Food Control 20, 1073–1079. doi: 10.1016/j.foodcont.2009.02.001
Ulanowska, K., Tkaczyk, A., Konopa, G., and Wegrzyn, G. (2006). Differential antibacterial activity of genistein arising from global inhibition of DNA, RNA and protein synthesis in some bacterial strains. Arch. Microbiol. 184, 271–278. doi: 10.1007/s00203-005-0063-7
Wang, C., Chang, T., Yang, H., and Cui, M. (2015). Antibacterial mechanism of lactic acid on physiological and morphological properties of Salmonella Enteritidis, Escherichia coli and Listeria monocytogenes. Food Control 47, 231–236. doi: 10.1016/j.foodcont.2014.06.034
Xu, P., Ding, L., Wei, J., Li, Q., Gui, M., He, X., et al. (2019). A new aquatic pathogen inhibitor produced by the marine fungus Aspergillus sp. LS116. Aquaculture 520:734670. doi: 10.1016/j.aquaculture.2019.734670
Yemis, G. P., Bach, S., and Delaquis, P. (2019). Antibacterial activity of polyphenol-rich pomegranate peel extract against Cronobacter sakazakii. Int. J. Food Prop. 22, 985–993. doi: 10.1080/10942912.2019.1622564
Keywords: Cronobacter sakazakii, Chrysanthemum buds crude extract, antibacterial activity, mechanism, application
Citation: Chang Y, Xing M, Hu X, Feng H, Wang Y, Guo B, Sun M, Ma L and Fei P (2021) Antibacterial Activity of Chrysanthemum buds Crude Extract Against Cronobacter sakazakii and Its Application as a Natural Disinfectant. Front. Microbiol. 11:632177. doi: 10.3389/fmicb.2020.632177
Received: 22 November 2020; Accepted: 29 December 2020;
Published: 03 February 2021.
Edited by:
Mutamed Ayyash, United Arab Emirates University, United Arab EmiratesReviewed by:
Salam A. Ibrahim, North Carolina Agricultural and Technical State University, United StatesAmin N. Olaimat, Hashemite University, Jordan
Copyright © 2021 Chang, Xing, Hu, Feng, Wang, Guo, Sun, Ma and Fei. This is an open-access article distributed under the terms of the Creative Commons Attribution License (CC BY). The use, distribution or reproduction in other forums is permitted, provided the original author(s) and the copyright owner(s) are credited and that the original publication in this journal is cited, in accordance with accepted academic practice. No use, distribution or reproduction is permitted which does not comply with these terms.
*Correspondence: Peng Fei, feipeng0220@126.com; 736422337@qq.com
†These authors have contributed equally to this work