- 1Department of Microbiology and Parasitology, Facultad de Medicina, ISTUN Instituto de Salud Tropical, University of Navarra, Pamplona, Spain
- 2Navarra Institute for Health Research (IdiSNA), Pamplona, Spain
- 3Unidad de Producción y Sanidad Animal, Centro de Investigación y Tecnología Agroalimentaria de Aragón (CITA), Zaragoza, Spain
- 4Instituto Agroalimentario de Aragón-IA2, CITA-Universidad de Zaragoza, Zaragoza, Spain
- 5Research Unit in Biology of Microorganisms (URBM), NARILIS, University of Namur, Namur, Belgium
Brucella species cause brucellosis, a worldwide extended zoonosis. The brucellae are related to free-living and plant-associated α2-Proteobacteria and, since they multiply within host cells, their metabolism probably reflects this adaptation. To investigate this, we used the rodent-associated Brucella suis biovar 5, which in contrast to the ruminant-associated Brucella abortus and Brucella melitensis and other B. suis biovars, is fast-growing and conserves the ancestral Entner-Doudoroff pathway (EDP) present in the plant-associated relatives. We constructed mutants in Edd (glucose-6-phosphate dehydratase; first EDP step), PpdK (pyruvate phosphate dikinase; phosphoenolpyruvate ⇌ pyruvate), and Pyk (pyruvate kinase; phosphoenolpyruvate → pyruvate). In a chemically defined medium with glucose as the only C source, the Edd mutant showed reduced growth rates and the triple Edd-PpdK-Pyk mutant did not grow. Moreover, the triple mutant was also unable to grow on ribose or xylose. Therefore, B. suis biovar 5 sugar catabolism proceeds through both the Pentose Phosphate shunt and EDP, and EDP absence and exclusive use of the shunt could explain at least in part the comparatively reduced growth rates of B. melitensis and B. abortus. The triple Edd-PpdK-Pyk mutant was not attenuated in mice. Thus, although an anabolic use is likely, this suggests that hexose/pentose catabolism to pyruvate is not essential for B. suis biovar 5 multiplication within host cells, a hypothesis consistent with the lack of classical glycolysis in all Brucella species and of EDP in B. melitensis and B. abortus. These results and those of previous works suggest that within cells, the brucellae use mostly 3 and 4 C substrates fed into anaplerotic pathways and only a limited supply of 5 and 6 C sugars, thus favoring the EDP loss observed in some species.
Introduction
Members of the genus Brucella are α2-Proteobacteria that infect a wide range of vertebrates causing brucellosis in mammals (Al Dahouk et al., 2008; Whatmore, 2009; Soler-Lloréns et al., 2016), a zoonosis with a high impact on developing countries worldwide (McDermott et al., 2013). Although the genus includes an increasing number of species (Moreno, 2020), Brucella abortus, Brucella melitensis, and Brucella suis (often referred to as the classical smooth spp.) are by far those having the more severe impact on both livestock and humans and they were divided long ago into biovars following phenotypic criteria (Alton, 1987). Although for a long time thought to be a very homogeneous group (Hoyer and McCullough, 1968; Verger et al., 1987), the identification of new spp. and phylogenomic studies show that B. abortus, B. melitensis, B. suis, Brucella neotomae, Brucella ovis, and Brucella canis plus isolates from sea mammals and the common vole form a relatively heterogeneous core group separated from several early diverging brucellae (Wattam et al., 2014; Soler-Lloréns et al., 2016; Moreno, 2020). These studies also show that, while all B. abortus and B. melitensis biovars group into two clades, the five B. suis biovars show a greater diversity that is inconsistent with their current taxonomic status as a single sp. (Moreno and Moriyón, 2002; Al Dahouk et al., 2008; Scholz et al., 2008a; Whatmore, 2009; Moreno, 2020).
The weight of the evidence shows that the brucellae have evolved from environmental α2-Proteobacteria (Moreno, 2020). Since they are facultative intracellular pathogens unable to persist in nature outside their hosts, this origin implies that they have probably adapted their metabolism to the peculiarities of the Brucella containing vacuoles (BCV) where they multiply. Undoubtedly because of their early identification and greater impact on domestic livestock and humans, metabolism has been investigated almost exclusively in B. abortus, B. melitensis, and biovars 1 and 3 of B. suis. These spp. and biovars, although auxotrophic only for a few vitamins and occasionally for a few amino acids (Gerhardt and Wilson, 1948; Plommet, 1991), are often described as fastidious because of their complex requirements for primary isolation (peptone-yeast extract media, often supplemented with serum) and slow growth. However, B. suis biovar 5 and Brucella microti, both rodent-associated brucellae, display much faster growth (Scholz et al., 2008b; Zúñiga-Ripa et al., 2018), which suggests a more ancestral metabolism. Consistent with this, we have recently found (Machelart et al., 2020) that, like the environmental α2-Proteobacteria neighbors, the Entner-Doudoroff pathway (EDP) is fully active in those rodent-associated spp. so that glucose is fueled into the tricarboxylic acid cycle (TCA) mostly through EDP with little contribution of the Pentose Phosphate Pathway (PPP). Thus, whereas B. suis biovar 5 mutants in edd (coding for the 6-phosphogluconate dehydratase involved in the first step of EDP) have a severe growth defect, mutation of gnd (6-phosphogluconate dehydrogenase of the first step of PPP) has only a reduced effect. On the other hand, B. abortus, B. melitensis, and B. suis other than biovar 5 rely exclusively on PPP because all carry a disabling mutation in edd. This shows the dispensability of EDD in the spp. that cause disease in livestock and, consistent with the lack of the phosphofructokinase of the Embden-Meyerhof-Parnas (classical glycolysis) in all brucellae (Barbier et al., 2018), supports the hypothesis that glucose fueling into TCA is not essential in BCVs and was thus lost in some clades. Although B. suis biovar 5 and B. microti represent a suitable mode to test this hypothesis, we have consistently failed to obtain a double edd-gnd mutant, in all likelihood because PPP becomes essential when EDD is not functional (Machelart et al., 2020). However, as that hypothesis can be tested by blocking pyruvate synthesis at other levels of the central C pathways, in this work we applied this approach by deleting edd in B. suis biovar 5 and, instead of gnd, the genes putatively coding for pyruvate phosphate dikinase (ppdK) and pyruvate kinase (pyk) (Figure 1). Here, we present experiments that confirm the corresponding predicted metabolic phenotypes as well as the results of an assessment of virulence in the mouse model of brucellosis.
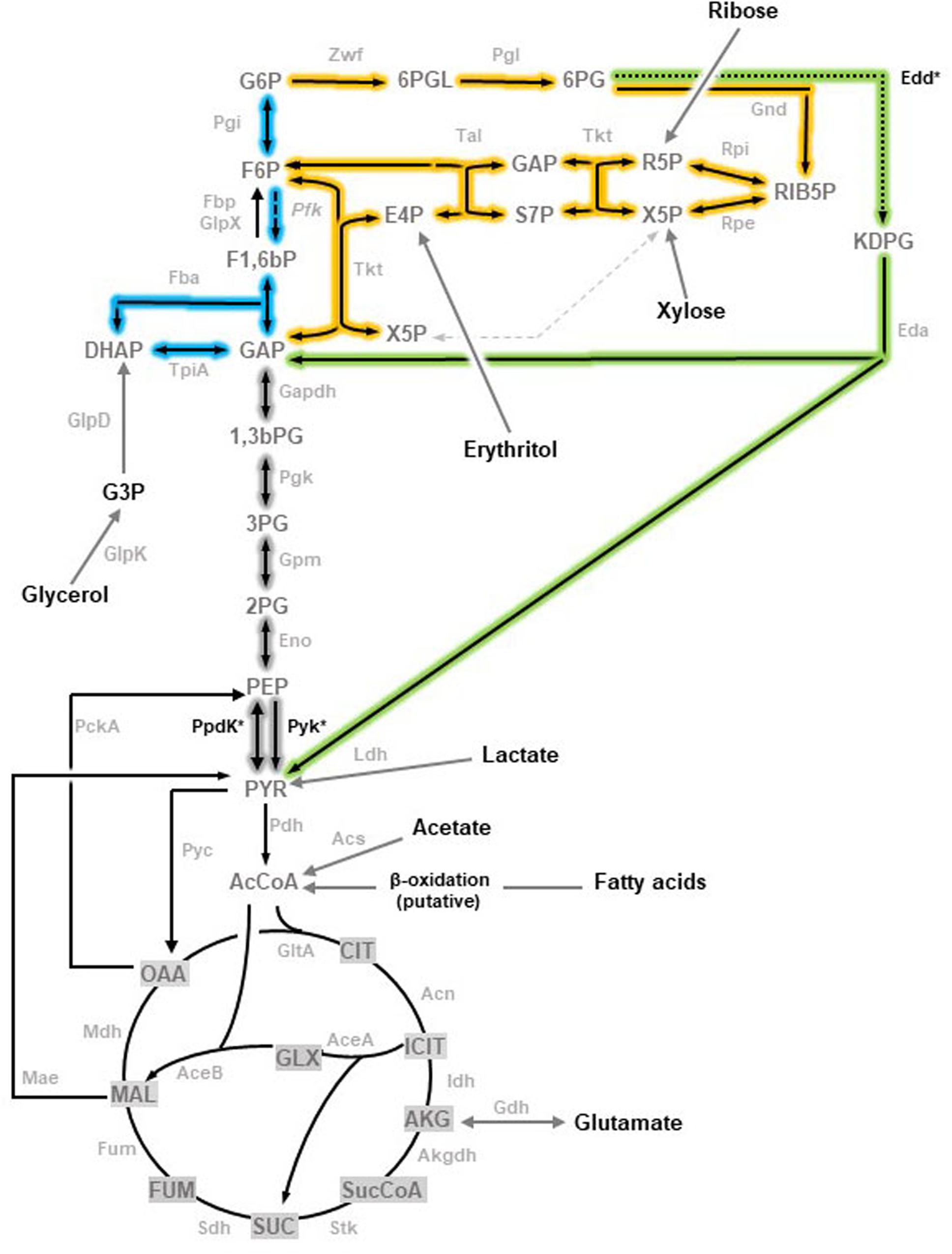
Figure 1. Topology of the three glycolytic routes in Brucella (adapted from Zúñiga-Ripa et al., 2018). Embden-Meyerhof-Parnas (EMP) pathway, often referred to as glycolysis, is shown in blue with a dashed arrow marking the reaction catalyzed by the key enzyme Pfk absent in brucellae. Pentose Phosphate (PP) pathway is complete and highlighted in yellow. The Entner-Doudoroff (ED) pathway is shown in green with a dotted arrow marking the step catalyzed by Edd. Reactions that are shared by the three pathways are highlighted in gray. The enzymes corresponding to the genes investigated are marked with and asterisk. Abbreviations used are Metabolites: 1,3,bPG, 1,3-bisphosphoglycerate; KDPG, 2-keto-3-deoxy-phosphogluconate; 2PG, 2-phosphoglycerate; 3PG, 3-phosphoglycerate; 6PGL, 6-P- (gluconolactone; 6PG, 6-phosphogluconate; AcCoA, acetyl-coenzyme A; AKG, alpha-ketoglutarate; CIT, citrate; ICIT, isocitrate; DHAP, dihydroxyacetone-P; E4P, erythrose-4-P; F1,6bP, fructose-1,6-bisphosphate; F6P, fructose-6-P; FUM, fumarate; G6P, glucose-6-P; GAP, glyceraldehyde-3-P; G3P, glycerol-3-P; GLX, glyoxylate; MAL, malate; OAA, oxaloacetate; PEP, phosphoenolpyruvate; PYR, pyruvate; R5P, ribose-5-P; RIB5P, ribulose-5-P; S7P, sedoheptulose-7-P; SUC, succinate; SucCoA, succinyl-coenzyme A; X5P, xylulose-5-P. Enzymes: Edd, 6-phospho-D-gluconate dehydratase; Gnd, 6-phosphogluconate dehydrogenase; Pgl,6-phosphogluconolactonase; Acs, acetyl-coenzyme A synthetase; Acn, aconitate hydratase; Akgdh, alpha-ketoglutarate dehydrogenase; GltA, citrate synthase; Eno, enolase; Fbp, GlpX, fructose-1,6-bisphosphatase; Fba, fructose bisphosphate aldolase; Fum, fumarase; Zwf, glucose-6-P dehydrogenase; Pgi, glucose-6-P isomerase; Gdh, glutamate dehydrogenase; Gapdh, glyceraldehyde-3-P dehydrogenase; GlpD, glycerol-3-P dehydrogenase; GlpK, glycerol kinase; Idh, isocitrate dehydrogenase; AceA, isocitrate lyase; Eda, 2-dehydro-3-deoxy-phosphogluconate aldolase; Ldh, lactate dehydrogenase; Mdh, malate dehydrogenase; AceB, malate synthase; Mae, malic enzyme; PckA, phosphoenolpyruvate carboxykinase; Pfk, phosphofructokinase; Pgk, phosphoglycerate kinase; Gpm, phosphoglycerate mutase; Pyc, pyruvate carboxylase; Pdh, pyruvate dehydrogenase; Pyk, pyruvate kinase; PpdK, pyruvate phosphate dikinase; Rpi, ribose-5-phosphate isomerase; Rpe, ribulose-5-P-3-epimerase; Sdh, succinate dehydrogenase; Stk, succinyl-coenzyme A synthetase; Tal, transaldolase; Tkt, transketolase; Tpi, triose P isomerase.)
Materials and Methods
Bacterial Strains and Growth Conditions
The bacterial strains and plasmids used are listed in Supplementary Table 1. At the University of Navarra, bacteria were routinely grown in peptone-glucose [TSB, bioMerieux; bio-Trypcase (17 g/L), bio-Soyase (3 g/L), glucose (2.5 g/L), NaCl (5 g/L), and K2HPO4 (2.5 g/L)], or on this medium supplemented with agar (TSA). For the animal experiments at CITA (see below), the inocula were grown on Blood Agar Base No. 2 [BAB2, Oxoid; proteose peptone (15 g/L), liver digest (2.5 g/L), yeast extract (5 g/L), NaCl (5 g/L), and agar (12 g/L)] a basal medium previously shown to be equivalent to TSA with a large number of strains (in preliminary experiments, it was confirmed that the strains used in this study grew similarly on both media, as expected) (De Miguel et al., 2011). To study the phenotype of the metabolic mutants, a base medium [modified Plommet’s medium; (Plommet, 1991; Barbier, 2014)] was used: 9.2 g/L K2HPO4; 3.0 g/L KH2PO4; 0.1 g/L Na2S2O3; 5.0 g/L NaCl; 0.2 g/L nicotinic acid; 0.2 g/L thiamine; 0.07 g/L pantothenic acid; 0.5 g/L (NH4)2SO4; 0.01 g/L MgSO4; 0.1 mg/L MnSO4; 0.1 mg/L FeSO4; 0.1 mg/L biotin. For metabolic studies, this base medium was supplemented with the appropriate C source at 1 g/L. When necessary, peptone-glucose was supplemented with kanamycin (50 μg/ml), polymyxin B (2 μg/ml), or sucrose (5%). All strains were stored in skimmed milk (Scharlau) at −80°C.
Growth Curves
The following protocol was used to avoid any nutrient carry over. Bacteria were first grown in 10 ml of peptone-glucose in a 50 ml flask at 37°C for 18 h with orbital agitation, harvested by centrifugation, and then resuspended in 10 ml of base medium supplemented with the appropriate C source at an optical density of 0.1 at 600 nm (O.D.600nm). This broth was incubated with orbital agitation at 37°C for 18 h, cells harvested by centrifugation, resuspended to an O.D.600nm of 0.1 in 1 ml of the same medium, and transferred to Bioscreen plates (200 μl/well) in technical triplicates. Growth was monitored every 0.5 h at 420−580 nm with continuous shaking at 37°C in a Bioscreen C incubator (Lab Systems) using wells with sterile medium as the blank. All experiments were repeated at least three times.
DNA Manipulations
Genomic sequences of B. suis 513 (the reference strain of the B. suis biovar 5) were obtained from the Kyoto Encyclopedia of Genes and Genomes (KEGG) database1. Searches for DNA and protein homologies were carried out using the National Center for Biotechnology Information (NCBI)2, the European Molecular Biology Laboratory (EMBL)-European Bioinformatics Institute server3, and The Broad Institute of Harvard and MIT-Brucella group databases. Primers were synthesized by the Sigma-Genosys (Haverhill, United Kingdom). Restriction-modification enzymes were used as recommended by the manufacturers. Plasmid DNAs were extracted with the QIAprep Spin Miniprep (Qiagen GmbH, Hilden, Germany) and genomic DNAs from individual colonies by boiling in water. When needed, DNA was purified from agarose gels using the QIAquick Gel Extraction Kit (Qiagen).
Mutagenesis
The B. suis 513 Bs5ΔppdK in-frame mutant in ppdK [described in a previous work (Zúñiga-Ripa et al., 2018)] carried a deletion encompassing 86% of the corresponding gene. To obtain Bs5Δpyk, a first plasmid (pAZI-36; Supplementary Table 1) was prepared as follows. First, two PCR fragments were generated using oligonucleotides Pyk-F1 (5′- GCTGACGTCGCGCTATTATT-3′) and Pyk-R2 (5′-CGTGGCGAGAATCTTGACC-3′), which amplified a 282 bp fragment including codons 1–12 of pyk, as well as 246 bp upstream of the pyk start codon; and oligonucleotides Pyk-F3 (5′-GGTGCAAGATTCTCGCCACGGGTGCAACCAATATGCTGC-3′) and Pyk-R4 (5′-CGCTCTGAATTCGCATTTG-3′), which amplified a 296 bp fragment including the last 60 bp of pyk. To join the two fragments, a third PCR used oligonucleotides Pyk-F1 and Pyk-R4 for amplification and the complementary regions between Pyk-R2 and Pyk-F3 for overlapping. The resulting fragment, containing the pyk deletion lacking 96% of the wild-type ORF, was cloned into pCR2.1 (Invitrogen) to obtain pAZI-36. After sequence verification, the deletion allele was excised using BamHI–NotI and cloned into a pJQKm suicide vector (Scupham and Triplett, 1997). The resulting pLZI-1 mutator plasmid (Supplementary Table 1) was transformed into E. coli strains TOP10F’ and S17λpir and transferred to B. suis 513 by conjugation. Integration of the suicide vector in the chromosome was selected by polymyxin (B. suis 513 is intrinsically resistant) and kanamycin resistance, and excision of pLZI-1 (producing Bs5Δpyk by allelic exchange) by polymyxin and sucrose resistance and kanamycin sensitivity. The resulting colonies were screened by PCR with primers Pyk-F1 and Pyk-R4, which amplified a fragment of 578 bp and 1917 bp in the mutant and parental strain, respectively.
Bs5ΔppdKΔpyk was constructed introducing pLZI-1 into Bs5ΔppdK by conjugation and selection by polymyxin and sucrose resistance and kanamycin sensitivity, and confirmed by PCR using oligonucleotides Pyk-F1 and Pyk-R4. To check for both mutations, the internal primer Pyk-R5 (5′- TTTTCCGTCATCGATCAACA -3′) hybridizing in the deleted region was used.
To construct mutant Bs5Δedd, the suicide mutator plasmid pNPTSΔedd (Supplementary Table 1), carrying the edd deleted allele (Machelart et al., 2020) was introduced into E. coli S17λpir by transformation. Then, pNPTSΔedd was introduced into B. suis 513 by conjugation. Following the protocol described above, colonies from the second recombination were screened by PCR with primers Edd-F1 (5′-GGCACGATTTCATCAGCGCA-3′) and Edd-R4 (5′-CCGCCATTCATGGCATTCTGG-3′), which amplified a fragment of 1,447 bp in the mutant and a fragment of 3,271 bp in the parental strain. The deletion removed 56% of the ORF and was identified using the internal primer Edd-R5 (5′-TCCTGAATGCGTTTATGTGC-3′) which hybridized in the deleted region.
To construct Bs5ΔpykΔedd, Bs5ΔppdKΔedd, and Bs5ΔppdKΔpykΔedd, pNPTSΔedd was introduced into Bs5Δpyk, Bs5ΔppdK, and Bs5ΔppdKΔpyk by conjugation and primers Edd-F1, Edd-R4, and Edd-R5 were used to screen the resulting colonies.
Virulence Assays in Mice
Seven-week-old female BALB/c mice (Envigo-Harlan Laboratories, Barcelona, Spain) were accommodated under BSL-3 biosafety containment conditions in the facility of Centro de Investigación y Tecnología Agroalimentaria de Aragón (CITA; Registration code ES502970012025) with water and food ad libitum. The animal handling and procedures were in accordance with the current European (directive 86/609/EEC) and Spanish (RD/53/2013) legislation and authorized by the Animal Welfare Committee of the institution. For each strain, inoculum was prepared from a 24 h culture on Blood Agar Base No. 2 (see above “Bacterial Strains and Growth Conditions”) at 37°C. Bacterial suspensions in sterile phosphate buffered saline (0.85% NaCl, 0.1% KH2PO4, 0.2% K2HPO4; pH 6.85) were spectrophotometrically adjusted to 1 × 109 colony forming units (CFU) and diluted to the required concentration. Mice (n = 5) were inoculated intraperitoneally with approximately 1 × 105 CFU in 0.1 mL of the corresponding inoculum (exact doses were retrospectively assessed by CFU accounts on BAB plates) and then euthanized 2 and 8 weeks after inoculation. Spleens were aseptically removed, individually weighed, homogenized in nine volumes of sterile saline buffer and serial 10-fold dilutions plated by triplicate on BAB plates for CFU accounts. The identity of the isolates was confirmed by PCR. Individual data (mean CFU/spleen) were normalized by logarithmic transformation and the mean log CFU/spleen values and the standard deviation (n = 5) calculated for statistical comparisons by one-way ANOVA followed by the Dunnett’s test.
Results
The Simultaneous Deletion of edd, ppdK, and pyk Abolishes B. suis 513 Growth on 5 and 6 C Sugars
Whereas the oxidative PPP yields phosphoenolpyruvate (PEP) that is then converted into pyruvate, the EDP produces PEP and directly pyruvate (Figure 1). Since pyruvate can be converted directly into acetyl-CoA to feed the TCA, when glucose is the only C source it can be predicted: (i) that the steps connecting PEP and pyruvate should be dispensable for growth if EDP is active; and (ii) that an edd mutant defective in these steps should not grow on glucose.
According to genomic predictions, the B. suis 513 (the reference strain of the B. suis biovar 5) enzymes involved in PEP-pyruvate conversions would be a (putative) pyruvate phosphate dikinase (PpdK) and a (putative) pyruvate kinase (Pyk) (Figure 1). Therefore, as a first test for those predictions, we examined Bs5ΔppdK and Bs5Δpyk for growth on glucose and on peptone-glucose as a control. As can be seen in the upper left panel of Figure 2, Bs5ΔppdK grew on glucose reaching the level of the parental strain with a short delay and similar generation times (about 8 h during the exponential phase). On glucose, although Bs5Δpyk displayed a longer generation time (about 11 h), it also reached a stationary phase level like that of the parental strain (Figure 2, upper left panel). Both mutants grew normally in peptone-glucose (Figure 2, lower left panel). As a control, we included a mutant in edd. Whereas this Bs5Δedd mutant grew normally on peptone-glucose (Figure 2, lower left panel), we observed that it grew less and more slowly than its parental B. suis 513 strain on glucose (Figure 2, upper left panel). This result confirms the functionally of the EDP and, since growth was not abrogated, it also shows a minor activity of a complementary glucose oxidative route, which should be the oxidative PPP because of the lack of phosphofructokinase and hence classical glycolysis in all brucellae.
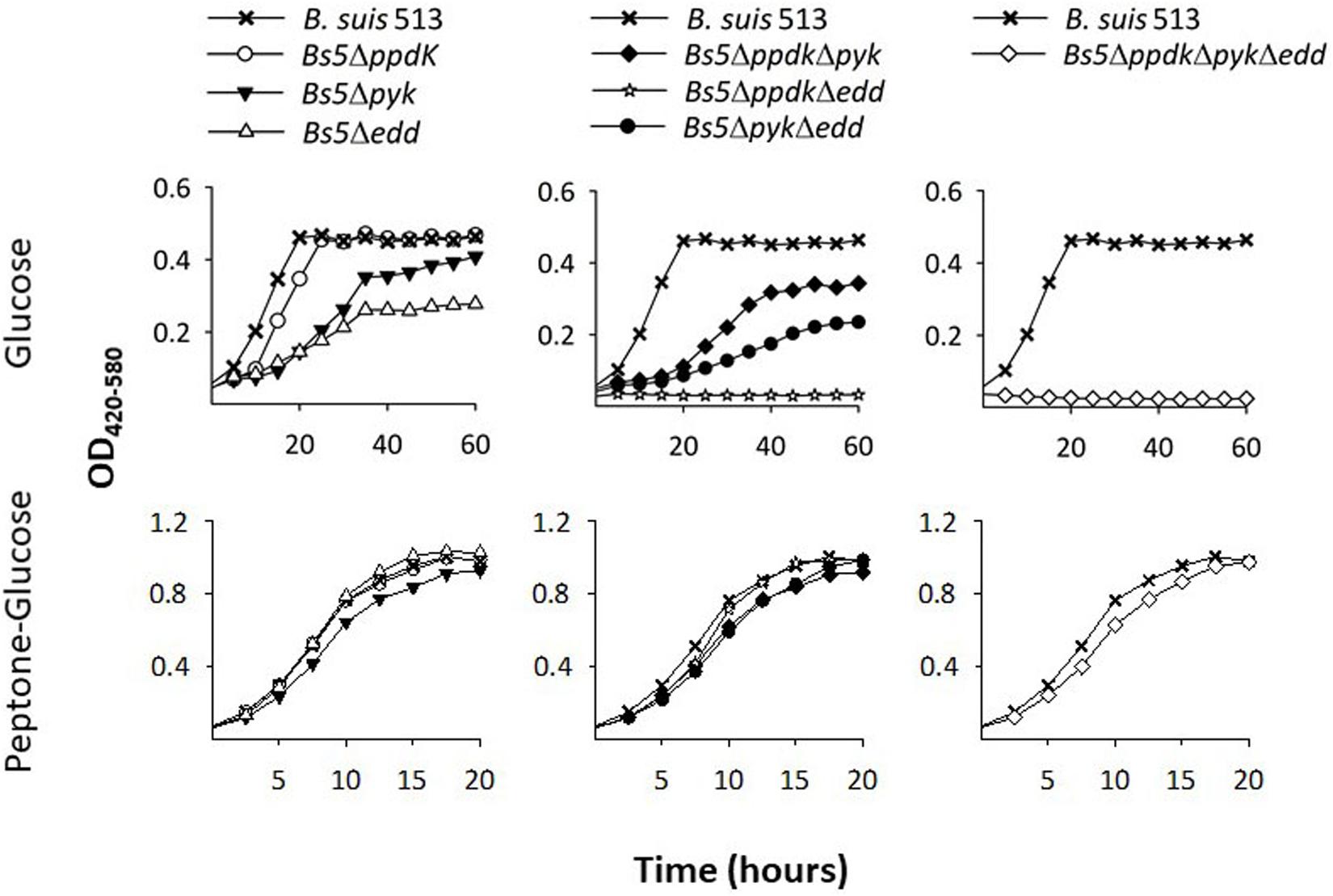
Figure 2. Deletion of ppdk, pyk, and edd abrogates growth of B. suis 513 on glucose as sole C source. Growth curves were obtained in modified base Plommet’s medium supplemented with glucose (upper panels) or in peptone-glucose broths (lower panels). Each point represents the mean ± standard error of an experiment performed in technical triplicates (error bars are within the size of the symbols). The experiments were repeated at least three times with similar results.
Based on these results, we then constructed and tested the double Bs5ΔppdKΔpyk and triple Bs5ΔppdKΔpykΔedd mutants. We found that, while growth of the double mutant Bs5ΔppdKΔpyk was delayed but not arrested on glucose (Figure 2, upper central panel), edd became essential for growth when both ppdK and pyk were mutated (Figure 2, upper right panel). On the other hand, these mutants grew normally in complex medium (Figure 2, lower central right panels). These results strongly suggest that PpdK and Pyk are functional, confirm that B. suis 513 has an operative ED route and are consistent with our predictions.
In the experiments presented thus far, we noticed that Bs5ΔppdK grew faster than Bs5Δpyk, which implies that ppdK cannot fully replace pyk when the bacteria are growing on glucose (Figure 2, upper left panel). This prompted us to investigate whether the reactions catalyzed by these two enzymes are similarly effective when combined with the EDP. For this, we compared mutants Bs5ΔppdKΔedd and Bs5ΔpykΔedd on glucose. Unexpectedly, the results (Figure 2, upper central panel) showed no growth for Bs5ΔppdKΔedd and generation times for Bs5ΔpykΔedd not very different from those of the Bs5Δedd single mutant, suggesting a major and not dispensable role for PpdK. No growth defect was apparent on peptone-glucose (Figure 2, lower central panel).
We also noticed that Bs5Δedd showed longer generation times and stationary phase yields lower than those of Bs5ΔppdK or Bs5Δpyk (Figure 2, left panel). As indicated above, growth of Bs5Δedd under these conditions should occur only through the oxidative PPP, being in this regard similar to the three classical smooth Brucella spp. The PPP yields PEP through glyceraldehyde-3-P (GAP), and then PEP yields pyruvate through the Pyk and PpdK catalyzed reactions (Figure 1). Therefore, a direct proof of PPP as the only sugar catabolic route remaining in mutant Bs5Δedd would be that Pyk and Ppdk become essential for growth on pentoses when EDP is not functional. We confirmed this prediction (Figure 3) by taking advantage of the ability of B. suis 513 to grow on xylose and ribose as the only C source (Zúñiga-Ripa et al., 2018).
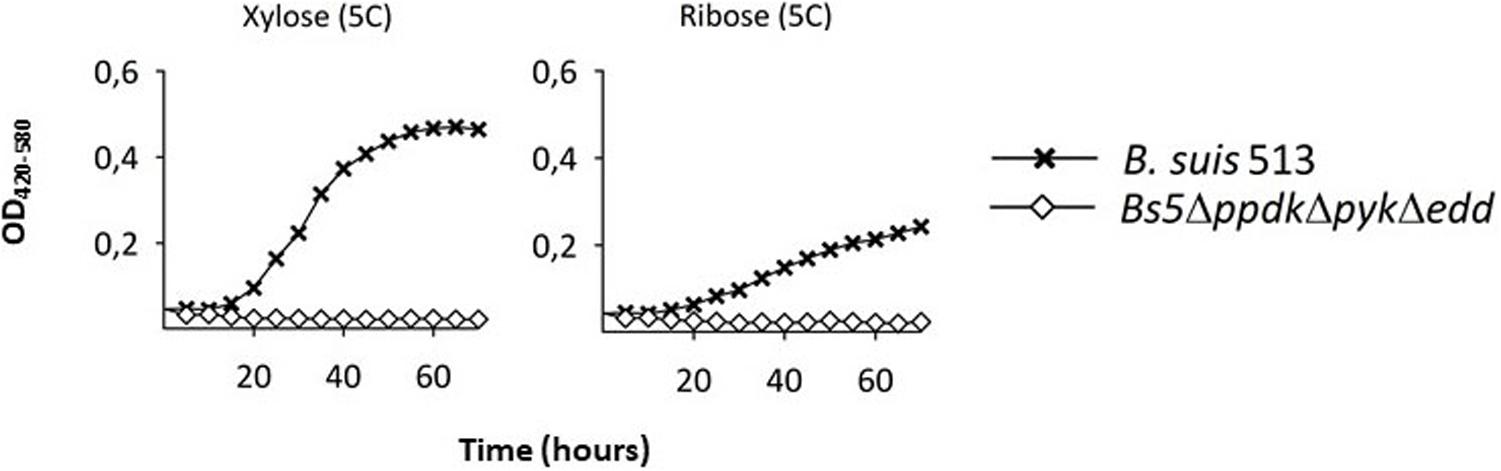
Figure 3. A triple Bs5ΔppdkΔpykΔedd mutant cannot grow on xylose or ribose. Each point represents the mean ± standard error of an experiment performed in technical triplicates (error bars are within the size of the symbols). The experiment was repeated at least three times with similar results.
The Simultaneous Deletion of edd, ppdK, and pyk Does Not Affect B. suis 513 Virulence in Mice
The phenotype of the Bs5ΔppdKΔpykΔedd provided a tool to investigate whether the catabolism of 6 (and 5 C) sugars was essential during infection. To investigate this, we inoculated BALB/c mice with Bs5ΔppdKΔpykΔedd and, as controls, Bs5ΔppdK and B. suis 513, and determined the CFU/spleen after 2 or 8 weeks (acute and chronic phase of infection, respectively). We found that the triple mutant Bs5ΔppdKΔpykΔedd was not attenuated in this virulence model (Figure 4).
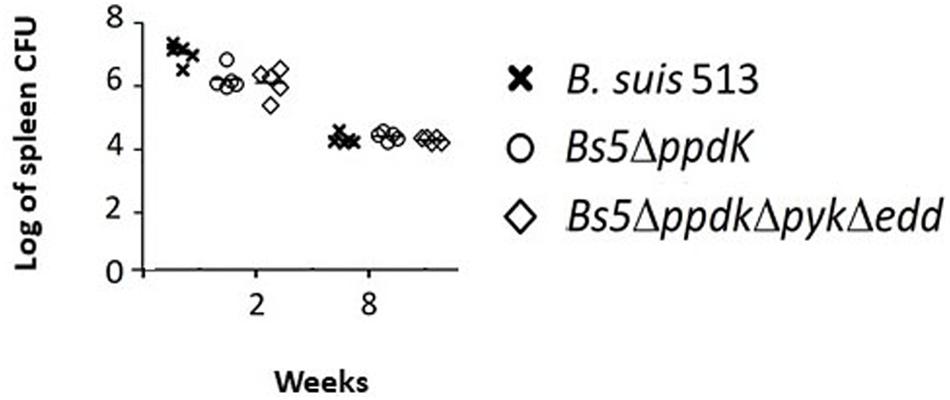
Figure 4. The triple mutant Bs5ΔppdkΔpykΔedd is not attenuated in the mouse model. Each point is the mean ± standard deviation (n = 5) of the logs of CFUs per spleen in technical triplicates. There were no statistical differences at any of the two times tested (one-way ANOVA followed by Dunnett’s test; p > 0.5).
Discussion
In this work, we confirm and extend our previous results supporting the existence of an active ED route in B. suis 513 (biovar 5) that together with the oxidative PPP sustains growth of this biovar on glucose as the only C source in vitro (Machelart et al., 2020). In keeping with the prediction that these routes produce PEP/pyruvate, we also found that growth requires PpdK and Pyk, the former apparently playing a major and non-dispensable role in vitro. Indeed, simultaneous dysfunction of Edd, PpdK, and Pyk also abolished the ability of B. suis 513 to grow on xylose or ribose. Indirectly, the data also confirm the lack of an active EMP pathway, consistent with the absence of phosphofructokinase in all brucellae (Barbier et al., 2018). It has to be noted that but for the ppdK one (Zúñiga-Ripa et al., 2018), the mutants investigated were not complemented despite several attempts (Lázaro-Antón, Moriyón and Zúñiga-Ripa; unpublished results). We have already detected this experimental difficulty with some Brucella mutants affected in intermediary C pathways, and this could be due to the intricacies of metabolic regulatory loops, plasmid stability, and other factors (Zúñiga-Ripa et al., 2014). However, while strict proof would require such experiments, it has to be stressed that the phenotype of the mutants studied here fully correspond with the predicted ones, which strongly suggests that the conclusions that can be drawn are valid. Also worth commenting is that, while further research is necessary to ascertain the metabolic peculiarities of the slow- and fast-growing brucellae, the observation that deletion of edd considerably reduces the growth rates of B. suis 513 suggests that the shift from EDD to PPP as the major route for sugar metabolism could be one of the reasons for these different phenotypes.
On the connection virulence-metabolism in brucellae, here we examined whether glucose oxidation beyond pyruvate is necessary for B. suis 513 virulence in the mouse model, and we obtained a negative answer. Considering the in vitro phenotypes of B. suis 513 and its Bs5ΔppdKΔpykΔedd mutant, this conclusion can be extended to at least xylose and ribose, two pentoses feeding into the PPP. As discussed below, these conclusions do not exclude other uses of hexoses and/or pentoses by the brucellae.
Several works offer insight on the role of hexose/pentose metabolism in Brucella virulence. A signature-tagged mutagenesis screening in mice identified a gluP [glucose/galactose transporter; (Essenberg et al., 1997)] mutant of B. abortus 2308 among those attenuated 8 weeks after infection but not among those identified as attenuated at 2 weeks post-infection (Hong et al., 2000). This mutant, however, was not critically compromised [virulent/gluP mutant co-infection competitive index at week 8 23.4 as compared to 72.4 for a gltD (glutamate synthase) mutant tested in parallel]. In subsequent work with gluP, Xavier et al. (2013) proposed that an increased glucose availability mediated by peroxisome proliferator-activated receptor γ (PPARγ) facilitates B. abortus 2308 survival during the chronic phase in alternative activated macrophages. Although other authors have interpreted these results to mean that glycolysis may play an important role in metabolism and virulence of intracellular Brucella (Gao et al., 2016), the multiplication of B. abortus in mouse spleens occurs early during infection [when no role for gluP was observed (Hong et al., 2000)] before the numbers of bacteria reach a short plateau after which it decreases progressively (Grilló et al., 2012). Therefore, the gluP studies suggest that glucose or galactose are used after the acute phase of infection for purposes other than major C/energy sources for multiplication in at least those laboratory models. Similar considerations can explain the mild attenuation of B. suis 1330 ribose kinase (rbsk) and 6-phosphogluconate dehydrogenase (gnd) Tn5 mutants observed in macrophage-like human THP-1 cells 48 h after infection (log CFU reduction for both mutants of 1.8 vs. 5 for genes involved in amino acid synthesis) (Köhler et al., 2002). Moreover, in our hands a B. suis 1330 mutant in gnd is severely attenuated (Machelart et al., 2020). On the other hand, other works that provide results on how virulence is affected by mutation of enzymes of hexose/pentose metabolism cannot be unequivocally interpreted in terms of metabolism. For example, a 3 log CFU attenuation was found for a B. suis 1330 P-glucose isomerase (pgi) Tn5 mutant (Foulongne et al., 2000) but, indeed, this mutation has pleiotropic effects, including that on the synthesis of mannose and hexosamine, two sugars required for lipopolysaccharide building. Similarly, a glucose-6-P dehydrogenase (zwf) mutant of B. abortus 544 was described as completely unable to multiply in Hela cells but surprisingly the mutant was severely hampered in invasiveness (Kim et al., 2003), a phenotype that strongly suggests defects not related to its ability to multiply within cells.
Whereas our results are not in open conflict with those summarized in the previous paragraph, the lack of a role in virulence of pyk and ppdK manifested in the phenotype in mice of Bs5ΔppdKΔpykΔedd apparently contradicts conclusions obtained in other studies. Gao et al. (2016) constructed a B. abortus S2308 pyk mutant that, in contrast to the parental strain, was impaired for growth on glucose but not on pyruvate. This B. abortus pyk mutant did not multiply in RAW 264.7 macrophages and was attenuated in BALB/c mice (approximately 2.5 log CFU less than the parental strain 1 and 5 weeks after infection). More recently, Pitzer et al. (2018) reported that a B. abortus 2308 pyk mutant proved to be defective in the activity of Pyk displayed reduced ability to metabolize glucose, fructose, and galactose but not ribose, xylose, arabinose or erythritol, and was attenuated in C57BL/6 mice. The reasons for the discrepancies in both attenuation and the range of substrates used in vitro are not obvious. For the attenuation, a plausible explanation would be that these studies have been conducted in B. abortus 2308 and ours with B. suis 513. As emphasized in the Introduction, B. suis 513 (but not B. abortus 2308) is fast-growing and can use a wider menu of substrates as the only source of C and energy including lactate and glutamate, which by themselves do not support growth of B. abortus 2308W (Zúñiga-Ripa et al., 2018). In the host, these abilities of B. suis 513 could provide a way to circumvent the PEP → pyruvate conversion as lactate can provide pyruvate, and the TCA cycle can also be fed by glutamate (Zúñiga-Ripa et al., 2018). Discrepancies in the use of C sources in vitro by B. abortus in different works could be explained by subtle differences between strains 2308 and 2308W, as they are not genetically identical (Suárez-Esquivel et al., 2016) and/or by the experimental conditions. Gao et al. (2016) tested 2308 growth in a medium containing glucose or pyruvate and mineral salts but also 0.1% yeast extract, which makes the medium non-minimal and could thus account for the differences in growth on glucose of 2308 and 2308W. Also, the utilization of hexoses and pentoses by 2308 in the work of Pitzer et al. (2018) was tested in a Biolog system, which implies an undefined medium and, therefore, provides no information on the use of those substrates as the only C/energy sources. Like in the study of Gao et al. (2016) the minimal medium used by Pitzer et al. (2018) contained 0.1% yeast extract.
Regarding ppdK, we reported in a previous work that this gene is necessary for B. abortus 2308W virulence (Zúñiga-Ripa et al., 2014), and this was confirmed in the study of Pitzer et al. (2018). Recently, we showed that the homologous ppdK mutant in B. suis 513 was not attenuated in mice and we elucidated the reasons for this discrepancy: this B. suis biovar 5 strain can use PpdK and PEP carboxykinase (PckA) for PEP synthesis in vitro (Figure 1), PckA catalyzing oxaloacetate conversion into PEP, while B. abortus 2308W uses only PpdK, that catalyzes the PEP-pyruvate interconversion (Zúñiga-Ripa et al., 2018). Indeed, we showed that B. suis 513 attenuation occurs in the double PckA-PpdK mutant (Zúñiga-Ripa et al., 2018).
Consistent with the absence of phosphofructokinase (Pfk) in all brucellae and the dispensability of the EDP, our results are in line with the hypothesis that hexose/pentose catabolism through the TCA is not necessary for growth in BCVs, which may account for the loss of EDP in B. abortus, B. melitensis, and some B. suis biovars. As PpdK catalyzes an amphibolic reaction that can support the synthesis of 3 to 6 C biosynthetic precursors and Pyk is catabolic, this could explain why the former seems to have a more important role for growth of at least B. suis 513. The data presented here together with those of previous works are consistent with a model (Zúñiga-Ripa et al., 2014, 2018) in which the brucellae thrive intracellularly by using 3 and 4 C substrates with a limited supply of 5 and 6 C sugars that are devoted to biosynthesis.
Data Availability Statement
The raw data supporting the conclusions of this article will be made available by the authors, without undue reservation.
Ethics Statement
The animal study was reviewed and approved by the Animal Welfare Committee of CITA.
Author Contributions
AZ-R, JL, MI, and IM conceived and coordinated the study. LL-A and AZ-R carried out the genomic analyses, mutants construction, and metabolic tests. MM, TB, RC-Á, and PM contributed in mutant construction, growth measurements, and experiments in mice. AZ-R, LL-A, and IM wrote the manuscript. All authors analyzed the results and approved the manuscript content.
Conflict of Interest
The authors declare that the research was conducted in the absence of any commercial or financial relationships that could be construed as a potential conflict of interest.
Funding
Research at the University of Navarra was supported by the MINECO (grants AGL2014-58795-C4-1-R and PID2019-107601RA-C32) and the Institute for Tropical Health funders (Obra Social la CAIXA -LCF/PR/PR13/11080005- and Fundación Caja Navarra, Fundación María Francisca de Roviralta, Ubesol and Inversiones Garcilaso de la Vega S.L). Research at URBM was supported by grants from the “Fonds National de la Recherche Scientifique” (FNRS) (Convention No. n° 2.4521.10. from Fonds de la Recherche Scientifique Médicale–FNRS, Belgium), and by the Interuniversity Attraction Poles Programme initiated by the Belgian Science Policy Office. Work at CITA was supported by MINECO (grants AGL2014-58795-C4-1-R and PID2019-107601RA-C32) and “Gobierno de Aragón” (Consolidated Group A14).
Acknowledgments
We thank Sara Serrano for her excellent technical assistance.
Supplementary Material
The Supplementary Material for this article can be found online at: https://www.frontiersin.org/articles/10.3389/fmicb.2020.620049/full#supplementary-material
Footnotes
References
Al Dahouk, S., Jubier-Maurin, V., Scholz, H. C., Tomaso, H., Karges, W., Neubauer, H., et al. (2008). Quantitative analysis of the intramacrophagic Brucella suis proteome reveals metabolic adaptation to late stage of cellular infection. Proteomics 8, 3862–3870. doi: 10.1002/pmic.200800026
Alton, G. G. (1987). Control of Brucella melitensis infection in sheep and goats: a review. Trop. Anim. Health Prod. 19, 65–74. doi: 10.1007/BF02297320
Barbier, T. (2014). Revision of the erythritol catabolic pathway and of the central metabolism of the pathogen Brucella. 1–241. Proc. Natl. Acad. Sci. 111, 17815–17820. doi: 10.1073/pnas.1414622111
Barbier, T., Zúñiga-Ripa, A., Moussa, S., Plovier, H., Sternon, J. F., Lázaro-Antón, L., et al. (2018). Brucella central carbon metabolism: an update. Crit. Rev. Microbiol. 44, 182–211. doi: 10.1080/1040841X.2017.1332002
De Miguel, M. J., Marín, C. M., Muñoz, P. M., Dieste, L., Grilló, M. J., Blasco, J. M., et al. (2011). Development of a selective culture medium for primary isolation of the main Brucella species. J. Clin. Microbiol. 4 9, 1458–1463. doi: 10.1128/jcm.02301-10
Essenberg, R. C., Candler, C., and Nida, S. K. (1997). Brucella abortus strain 2308 putative glucose and galactose transporter gene: cloning and characterization. Microbiology 143, 1549–1555. doi: 10.1099/00221287-143-5-1549
Foulongne, V., Bourg, G., Cazevieille, C., Michaux-Charachon, S., and O’Callaghan, D. (2000). Identification of Brucella suis genes affecting intracellular survival in an in vitro human macrophage infection model by signature-tagged transposon mutagenesis. Infect. Immun. 68, 1297–1303. doi: 10.1128/IAI.68.3.1297-1303.2000
Gao, J., Tian, M., Bao, Y., Li, P., Liu, J., Ding, C., et al. (2016). Pyruvate kinase is necessary for Brucella abortus full virulence in BALB/c mouse. Vet. Res. 47:87. doi: 10.1186/s13567-016-0372-7
Gerhardt, P., and Wilson, J. B. (1948). The nutrition of Brucellae: growth in simple chemically defined media. J. Bacteriol. 56, 17–24. doi: 10.1128/jb.56.1.17-24.1948
Grilló, M. J., Blasco, J. M., Gorvel, J.-P. P., Moriyón, I., and Moreno, E. (2012). What have we learned from brucellosis in the mouse model? Vet. Res. 43, 1–35. doi: 10.1186/1297-9716-43-29
Hong, P. C., Tsolis, R. M., and Ficht, T. A. (2000). Identification of genes required for chronic persistence of Brucella abortus in mice. Infect. Immun. 68, 4102–4107. doi: 10.1128/iai.68.7.4102-4107.2000
Hoyer, B. H., and McCullough, N. B. (1968). Polynucleotide homologies of Brucella deoxyribonucleic acids. J. Bacteriol. 95, 444–448. doi: 10.1128/jb.95.2.444-448.1968
Kim, S., Watarai, M., Kondo, Y., Erdenebaatar, J., Makino, S., and Shirahata, T. (2003). Isolation and characterization of Mini-Tn5Km2 insertion mutants of Brucella abortus deficient in internalization and intracellular growth in HeLa cells. Infect. Immun. 71, 3020–3027. doi: 10.1128/iai.71.6.3020-3027.2003
Köhler, S., Foulongne, V., Ouahrani-Bettache, S., Bourg, G., Teyssier, J., Ramuz, M., et al. (2002). The analysis of the intramacrophagic virulome of Brucella suis deciphers the environment encountered by the pathogen inside the macrophage host cell. Proc. Natl. Acad. Sci. U S A. 99, 15711–6. doi: 10.1073/pnas.232454299
Machelart, A., Willemart, K., Zúñiga-Ripa, A., Godard, T., Plovier, H., Conde-Álvarez, R., et al. (2020). Convergent evolution of zoonotic Brucella species towards the selective use of the pentose phosphate pathway. Proc. Natl. Acad. Sci. 117, 26374–26381. doi: 10.1073/PNAS.2008939117
McDermott, J. J., Grace, D., and Zinsstag, J. (2013). Economics of brucellosis impact and control in low-income countries. Sci. Tech. Rev. Off. Int. des Epizoot. 32, 249–261. doi: 10.20506/rst.32.1.2197
Miller, V. L., and Mekalanos, J. J. (1988). A novel suicide vector and its use in construction of insertion mutations: osmoregulation of outer membrane proteins and virulence determinants in Vibrio cholerae requires toxR. J. Bacteriol. 170, 2575–2583. doi: 10.1128/jb.170.6.2575-2583.1988
Moreno, E. (2020). The one hundred year journey of the genus Brucella (Meyer and Shaw 1920). FEMS Microbiol. Rev. 44:fuaa045. doi: 10.1093/femsre/fuaa045
Moreno, E., and Moriyón, I. (2002). Brucella melitensis: a nasty bug with hidden credentials for virulence. Proc. Natl. Acad. Sci. 99, 1–3. doi: 10.1073/pnas.022622699
Pitzer, J. E., Zeczycki, T. N., Baumgartner, J. E., Martin, D. W., and Roop, R. M. (2018). The manganese-dependent pyruvate kinase PykM is required for wild-type glucose utilization by Brucella abortus 2308 and its virulence in C57BL/6 Mice. J. Bacteriol. 200:e00471-18. doi: 10.1128/JB.00471-18
Plommet, M. (1991). Minimal requirements for growth of Brucella suis and other Brucella species. Zentralbl.Bakteriol. 275, 436–450. doi: 10.1016/s0934-8840(11)80165-9
Scholz, H. C., Al Dahouk, S., Tomaso, H., Neubauer, H., Witte, A., Schloter, M., et al. (2008a). Genetic diversity and phylogenetic relationships of bacteria belonging to the Ochrobactrum–Brucella group by recA and 16S rRNA gene-based comparative sequence analysis. Syst. Appl. Microbiol. 31, 1–16. doi: 10.1016/j.syapm.2007.10.004
Scholz, H. C., Hubalek, Z., Sedlacek, I., Vergnaud, G., Tomaso, H., Al Dahouk, S., et al. (2008b). Brucella microti sp. nov., isolated from the common vole Microtus arvalis. Int. J. Syst. Evol. Microbiol. 58, 375–382. doi: 10.1099/ijs.0.65356-0
Scupham, A. J., and Triplett, E. W. (1997). Isolation and characterization of the UDP-glucose 4’-epimerase-encoding gene, galE, from Brucella abortus 2308. Gene 202, 53–59. doi: 10.1016/s0378-1119(97)00453-8
Simon, L. D., Randolph, B., Irwin, N., and Binkowski, G. (1983). Stabilization of proteins by a bacteriophage T4 gene cloned in Escherichia coli. Proc. Natl. Acad. Sci. U S A. 80, 2059–62. doi: 10.1073/pnas.80.7.2059
Soler-Lloréns, P. F., Quance, C. R., Lawhon, S. D., Stuber, T. P., Edwards, J. F., Ficht, T. A., et al. (2016). A Brucella spp. isolate from a Pac-Man frog (Ceratophrys ornata) reveals characteristics departing from classical Brucellae. Front. Cell. Infect. Microbiol. 6:116. doi: 10.3389/fcimb.2016.00116
Spratt, B. G. J., Hedge, P., Heesen, S., Edelman, A., and Broome-Smith, J. K. (1986). Kanamycin-resistant vectors that are analogues of plasmids pUC8, pUC9, pEMBL8 and pEMBL9. Gene 41, 337–342. doi: 10.1016/0378-1119(86)90117-4
Suárez-Esquivel, M., Ruiz-Villalobos, N., Castillo-Zeledón, A., Jiménez-Rojas, C., Roop Ii, R. M., Comerci, D. J., et al. (2016). Brucella abortus Strain 2308 Wisconsin genome: importance of the definition of reference strains. Front. Microbiol. 7:1557. doi: 10.3389/fmicb.2016.01557
Verger, J. M., Grimont, F., Grimont, P. A., and Grayon, M. (1987). Taxonomy of the genus Brucella. Ann. Inst. Pasteur. Microbiol. 138, 235–238. doi: 10.1016/0769-2609(87)90199-2
Vershilova, P. A., Liamkin, G. I., and Malikov, V. E. (1983). Brucella strains from mouselike rodents in southwestern USSR. Int. J. Syst. Bacteriol. 33, 399–400. doi: 10.1099/00207713-33-2-399
Wattam, A. R., Foster, J. T., Mane, S. P., Beckstrom-Sternberg, S. M., Beckstrom-Sternberg, J. M., Dickerman, A. W., et al. (2014). Comparative phylogenomics and evolution of the Brucellae reveal a path to virulence. J. Bacteriol. 196, 920–930. doi: 10.1128/JB.01091-13
Whatmore, A. M. (2009). Current understanding of the genetic diversity of Brucella, an expanding genus of zoonotic pathogens. Infect. Genet. Evol. 9, 1168–1184. doi: 10.1016/j.meegid.2009.07.001
Xavier, M. N., Winter, M. G., Spees, A. M., den Hartigh, A. B., Nguyen, K., Roux, C. M., et al. (2013). PPARγ-mediated increase in glucose availability sustains chronic Brucella abortus infection in alternatively activated macrophages. Cell Host Microbe 14, 159–170. doi: 10.1016/j.chom.2013.07.009
Zúñiga-Ripa, A., Barbier, T., Conde-Álvarez, R., Martínez-Gómez, E., Palacios-Chaves, L., Gil-Ramírez, Y., et al. (2014). Brucella abortus depends on pyruvate phosphate dikinase and malic enzyme but not on Fbp and GlpX fructose-1,6-bisphosphatases for full virulence in laboratory models. J. Bacteriol. 196, 3045–3057. doi: 10.1128/JB.01663-14.
Zúñiga-Ripa, A., Barbier, T., Lázaro-Antón, L., de Miguel, M. J., Conde-Álvarez, R., Muñoz, P. M., et al. (2018). The fast-growing Brucella suis biovar 5 depends on phosphoenolpyruvate carboxykinase and pyruvate phosphate dikinase but not on Fbp and GlpX Fructose-1,6-bisphosphatases or isocitrate lyase for full virulence in laboratory models. Front. Microbiol. 9:641. doi: 10.3389/fmicb.2018.00641
Keywords: Brucella, metabolism, glucose, Entner-Doudoroff, virulence
Citation: Lázaro-Antón L, de Miguel MJ, Barbier T, Conde-Álvarez R, Muñoz PM, Letesson JJ, Iriarte M, Moriyón I and Zúñiga-Ripa A (2021) Glucose Oxidation to Pyruvate Is Not Essential for Brucella suis Biovar 5 Virulence in the Mouse Model. Front. Microbiol. 11:620049. doi: 10.3389/fmicb.2020.620049
Received: 21 October 2020; Accepted: 02 December 2020;
Published: 14 January 2021.
Edited by:
Roy Martin Roop II, East Carolina University, United StatesReviewed by:
Sean Crosson, Michigan State University, United StatesClayton Caswell, Virginia Tech, United States
Copyright © 2021 Lázaro-Antón, de Miguel, Barbier, Conde-Álvarez, Muñoz, Letesson, Iriarte, Moriyón and Zúñiga-Ripa. This is an open-access article distributed under the terms of the Creative Commons Attribution License (CC BY). The use, distribution or reproduction in other forums is permitted, provided the original author(s) and the copyright owner(s) are credited and that the original publication in this journal is cited, in accordance with accepted academic practice. No use, distribution or reproduction is permitted which does not comply with these terms.
*Correspondence: Amaia Zúñiga-Ripa, azuniga@unav.es