- 1Institute of Medical Engineering and Mechatronics, Ulm University of Applied Sciences, Ulm, Germany
- 2Institute of Medical Microbiology and Hygiene, University Hospital Ulm, Ulm, Germany
Due to the globally observed increase in antibiotic resistance of bacterial pathogens and the simultaneous decline in new antibiotic developments, the need for alternative inactivation approaches is growing. This is especially true for the treatment of infections with the problematic ESKAPE pathogens, which include Enterococcus faecium, Staphylococcus aureus, Klebsiella pneumoniae, Acinetobacter baumannii, Pseudomonas aeruginosa, and Enterobacter species, and often exhibit multiple antibiotic resistances. Irradiation with visible light from the violet and blue spectral range is an inactivation approach that does not require any additional supplements. Multiple bacterial and fungal species were demonstrated to be sensitive to this disinfection technique. In the present study, pathogenic ESKAPE organisms and non-pathogenic relatives are irradiated with visible blue and violet light with wavelengths of 450 and 405 nm, respectively. The irradiation experiments are performed at 37°C to test a potential application for medical treatment. For all investigated microorganisms and both wavelengths, a decrease in colony forming units is observed with increasing irradiation dose, although there are differences between the examined bacterial species. A pronounced difference can be observed between Acinetobacter, which prove to be particularly light sensitive, and enterococci, which need higher irradiation doses for inactivation. Differences between pathogenic and non-pathogenic bacteria of one genus are comparatively small, with the tendency of non-pathogenic representatives being less susceptible. Visible light irradiation is therefore a promising approach to inactivate ESKAPE pathogens with future fields of application in prevention and therapy.
Introduction
Hospital acquired infections (HAIs) challenge the health care sector (World Health Organization, 2017) and affect millions of patients each year. The increasing development of antibiotic resistances is worsening the situation creating an urgent need for establishing new anti-infective strategies. Consequently, to expedite the utilization of new technologies is critical, as the increasing prevalence of multidrug resistant microorganisms in hospitals became a serious threat for public health (Boucher et al., 2009). Most of the ESKAPE pathogens appear on the World Health Organization (WHO) list of the most problematic microbial species resulting in an appeal to concentrate research efforts on this topic. For 2007, the European Centre for Disease and Control (ECDC) estimated the death of 25,000 patients in the European Union, Norway and Iceland and an additional 900 million Euros hospital costs due to five selected antibiotic-resistant species (European center for disease prevention and control, 2009), completely intersecting with the ESKAPE pathogens. In 2016, HAI developed in 8.4% of patients staying in an intensive care unit for more than 2 days (European center for disease prevention and control, 2018).
Among research efforts to find alternative antimicrobial approaches, light-based technologies experience an intensified investigation (Mulani et al., 2019). Especially irradiation treatment with wavelengths in the range of visible light is often indicated as a possible antibiotic alternative (Wang et al., 2017; Hamblin and Abrahamse, 2019). Indeed, there seem to be multiple advantages. Due to current progress in the development of light emitting diodes (LED) this method is easily available, durable, sustainable and cost-effective. Several implementations in hospital-associated applications have been suggested. The equipment of endotracheal tubes with blue LEDs might intervene, where other antimicrobial approaches are struggling for many years to prevent ventilator associated pneumonia (VAP; Sicks et al., 2020). Endoscopically delivered light reduced gastric Helicobacter pylori infection (Ganz et al., 2005), urinary tract infection could successfully be treated in a rat model (Huang et al., 2018) and biofilm growth on catheter material was demonstrated to be reduced by the influence of irradiation (Vollmerhausen et al., 2017). The development of a light diffusing fiber delivery system (Shehatou et al., 2019), a minimally invasive ingestible capsule (Romano et al., 2016), the investigation of a continuous surface disinfection of hospital rooms during the patient’s presence (Maclean et al., 2013) and a whole range of different patents (Ahmed et al., 2018), demonstrate simultaneously the emerging significance of light-based technologies and their applicability.
The mechanisms for visible light inactivation is based on endogenous photosensitizers present in bacterial cells, which absorb photons of a certain wavelength converting the photosensitizers into an excited state. Those can interact with ambient oxygen to form reactive oxygen species (ROS) (Hamblin and Abrahamse, 2020), which cause damage to several intracellular targets (Dai et al., 2013; Adair and Drum, 2016; Kim and Yuk, 2017; Djouiai et al., 2018; Chu et al., 2019; Hyun and Lee, 2020). The violet wavelength of 405 nm was proven to be especially effective (Maclean et al., 2008; Endarko et al., 2012) tracing back to endogenous porphyrins, which are recognized as responsible photosensitizers (Ashkenazi et al., 2003; Hamblin et al., 2005; Lipovsky et al., 2009). The blue wavelength of 450 nm also has a considerable inactivation impact, albeit weaker than for 405 nm (Hessling et al., 2017; Plavskii et al., 2018; Tomb et al., 2018) but may be preferable for clinical applications due to higher tissue penetration depth and lower absorption by blood. For 450 nm flavins are considered as responsible photosensitizer (Cieplik et al., 2014; Plavskii et al., 2018; Hoenes et al., 2019).
It has been noticed that differences exist in the way, in which microbial genera react to the exposure to visible light of the same wavelength (Hessling et al., 2017; Tomb et al., 2018). However, the underlying causes are not totally resolved. Varying compositions and concentrations of endogenous photosensitizers might play a role, as those are the responsible mediators between light and bacterial damage. Due to differing investigation methods, the comparison of test results for different strains is problematic. It is hard to determine if variables arise from biological factors or the different treatment and environmental properties. Parameters like pH of the culture medium (Kjeldstad et al., 1984), specific culture conditions and growth phase (Keshishyan et al., 2015; Abana et al., 2017; Biener et al., 2017; Fyrestam et al., 2017) as well as pH, salt concentration and temperature during irradiation (Ghate et al., 2015; Keshishyan et al., 2015; McKenzie et al., 2016), have been demonstrated to influence bacterial inactivation. Barneck et al. (2016) found that the irradiation effect is dose-dependent and that different irradiation intensities did not play a role concerning the inactivation result at a specific dose, independent of the irradiation time. Nevertheless, this was only a single study, so it might be risky to rely on the dose-dependency when comparability is desired.
Our aim was therefore to perform all our investigations on pathogenic and non-pathogenic representatives of different genera with the same test protocol. In this study, we wanted to examine whether pathogenic bacterial species and closely related non-pathogenic species respond in the same manner to visible light irradiation at wavelengths of 405 and 450 nm. Therefore, a pathogenic strain and a non-pathogenic relative were investigated in comparison. To come up with new methods capable to cope with ESKAPE pathogens we chose those 6 species for our experiments. In total, we thus investigated 11 strains, as no non-pathogenic Klebsiella strain is available.
Materials and Methods
Bacterial Strains and Cultivation
Enterococcus moraviensis (DSM 15919), Staphylococcus carnosus (DSM 20501), Acinetobacter kookii (DSM 29071), Pseudomonas stutzeri (DSM 5190), Escherichia coli (DSM 1607), and Enterococcus faecium (DSM 17050) were obtained from DSMZ (Deutsche Sammlung für Mikroorganismen und Zellkulturen, Braunschweig, Germany). Staphylococcus aureus (ATCC 43300), Klebsiella pneumoniae (ATCC 700603), Acinetobacter baumannii (ATCC 19606), and Pseudomonas aeruginosa (ATCC 27853) were obtained from American Type Culture Collection (ATCC, Manassas, VA, United States). Escherichia coli BSU1286 was obtained from the Institute of Medical Microbiology and Hygiene (University Hospital Ulm, Germany). All strains were cultivated to mid-exponential phase and then centrifuged at 7,000 g for 5 min. The resultant pellet was resuspended in phosphate buffered saline (PBS) and washed in PBS, before the suspension was diluted to the desired population density between 5 × 107 and 108 colony forming units per ml (CFU/ml) for experimental use. All experiments were conducted in PBS to avoid the possible photosensitizing impact and absorbance of medium ingredients. Media that were used for cultivation are listed in Table 1.
Irradiation Setup
Two different wavelengths were applied for irradiation. Due to safety regulations the strains were investigated in two groups and in two laboratories with test setups slightly adapted to laboratory conditions, but with the same parameters. A truncated hollow pyramid with a high reflective inside, already described before in Hoenes et al., 2019, ensured that the sample area was irradiated homogenously. LEDs were mounted to a heat sink, which was actively cooled with a fan during experiments to avoid heating the sample (Figure 1). Experiments were performed in separate vessels with 11 mm diameter. For irradiation at the violet wavelength, LEDs with a peak wavelength of 405 nm were applied. The second wavelength was chosen from the blue wavelength range at 450 nm. For both wavelengths, an intensity of 20 mW/cm2 was selected. Irradiation intensity was measured by means of an optical power meter OPM150 (Qioptiq, Göttingen, Germany).
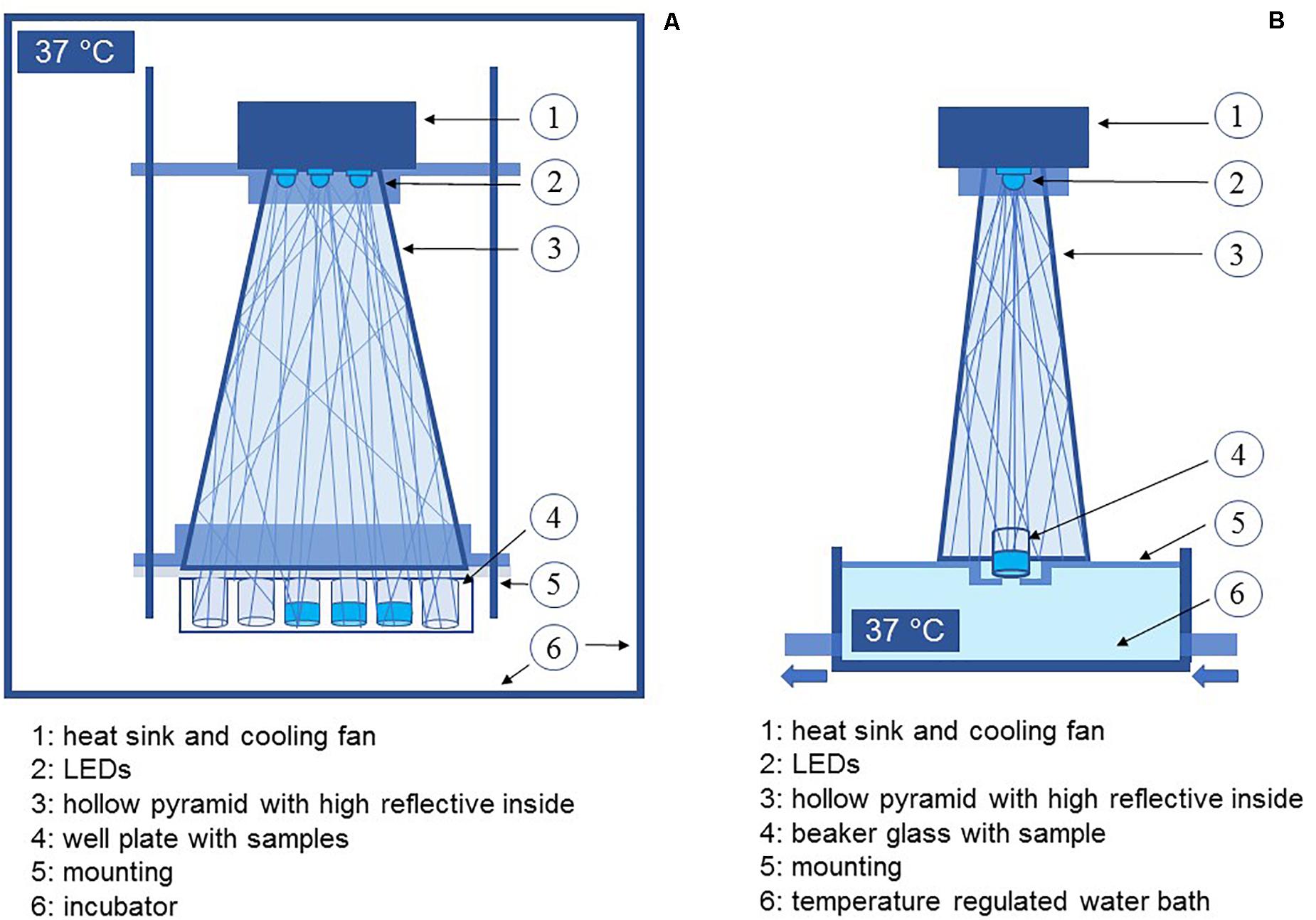
Figure 1. Schematic depiction of the experimental setup for experiments with pathogenic bacteria (A) and non-pathogenic bacteria (B).
As we wanted to refer to therapeutic applications taking place at or in the human body the experiments were conducted at 37°C. For pathogenic microorganisms, the whole setup was placed inside an incubator. Samples of non-pathogenic strains were heated by a temperature regulated water bath ICC basic (IKA, Staufen, Germany), with tempered water passing the lower third of the vessel continuously. The sample temperature was checked at each sampling interval with an infrared thermometer (Raytek Fluke Process Instruments GmbH, Berlin, Germany).
Samples were drawn in certain time intervals with increasing doses and plated on nutrient agar. The media used for agar plates have been the same as the cultivation media for non-pathogenic and sheep blood agar plates (TSA + SB, Oxoid, Basingstoke, United Kingdom) for pathogenic strains. After incubation at 37°C for 24–48 h grown colonies were enumerated manually. The resultant count was converted to CFU/ml and expressed as log reduction in comparison to the starting concentration. Each experiment was performed in triplicates and repeated at least three times.
Results
In this study we wanted to evaluate the irradiation susceptibility of different microbial genera and potential differences between closely related pathogenic and non-pathogenic bacterial species. In Figure 2 the inactivation results for the wavelengths 405 and 450 nm are depicted for two different species in each illustration - one pathogenic and one non-pathogenic of a certain genus, to compare whether there are differences in their inactivation profile. The dotted lines portray the non-pathogenic representative, while the solid lines represent the pathogenic species. There was no considerable decrease in most non-irradiated controls. However, the E. moraviensis control was reduced by 1.38 log at the end of the longer 450 nm experiment at 48 h. Therefore, the log decrease in the control at this strain was subtracted from the irradiation value at each sampling point to demonstrate the sole light impact. Likewise, A. kookii results were corrected in this manner. For all other strains, the decrease in the control was so low to not perceptibly affect the irradiation results.
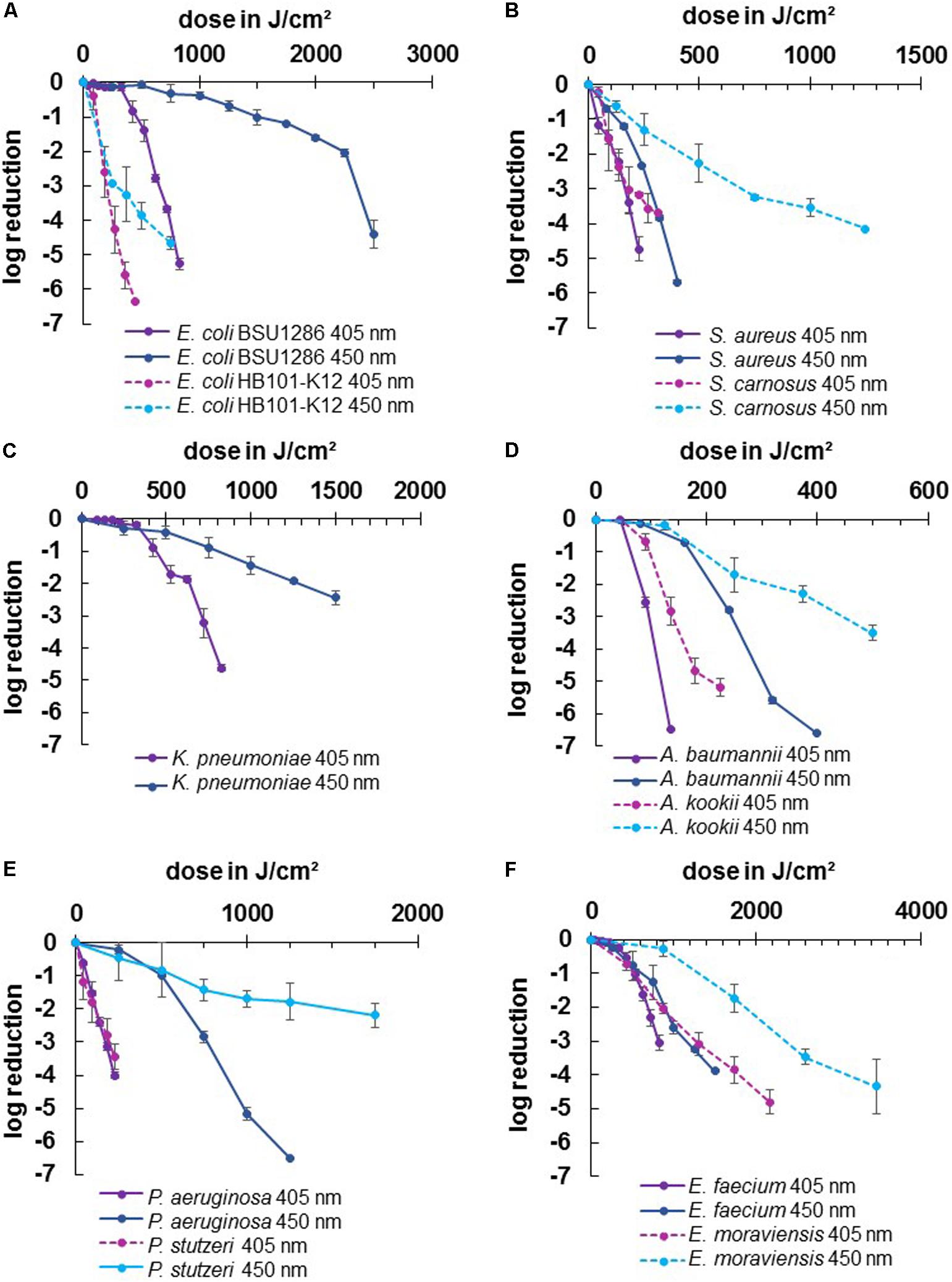
Figure 2. Inactivation results for pathogenic ESKAPE-strains and non-pathogenic relatives for 405 and 450 nm: E. coli BSU1286/E. coli HB101 K12 (A), S. aureus/S. carnosus (B), K. pneumoniae (C), A. baumannii/A. kookii (D), P. aeruginosa/P. stutzeri (E), E. faecium/E. moraviensis (F). Error bars represent standard deviation of three independent experiments.
For all tested strains, the violet wavelength of 405 nm exhibited a stronger inactivation effect compared to the blue wavelength of 450 nm (Figure 2). However, comparing the two wavelengths between pathogenic and non-pathogenic species, there were two cases in which 450 nm on the pathogenic strain was as effective as 405 nm on the non-pathogenic strain at a similar dose. For S. aureus we achieved 3.84 log reduction at 320 J/cm2 with 450 nm while S. carnosus required 315 J/cm2 of 405 nm to be inactivated by 3.70 log (Figure 2B). Likewise, for E. faecium 3.26 log at 1250 J/cm2 was achieved with 450 nm, while E. moraviensis was reduced by 3.08 log when exposed to 1296 J/cm2 of 405 nm (Figure 2F).
All strains exhibited the typical behavior for visible light inactivation with a non-monoexponential shape, showing a linear relationship of inactivation and dose in a semi-logarithmic representation. In some cases, a so called “shoulder” occured at lower doses indicating the ability of bacteria to overcome small damages. This slow initial reaction particularly became apparent for 450 nm irradiation of E. coli BSU1286, showing a slow decrease up to a dose of 2250 J/cm2, followed by a steeper slope (Figure 2A). In addition, at 450 nm P. aeruginosa illustrated a distinct development of this behavior (Figure 2E).
Both applied wavelengths were successful at inactivating the strains investigated here. However, the doses necessary to achieve a certain log reduction were varying, especially between different genera. The highest dose of 450 nm irradiation for a reduction of 3 or more log levels, had to be applied to E. coli BSU1286, with 2500 J/cm2 for 4.4 log reduction (Figure 2A). Meanwhile, only 320 J/cm2 of 450 nm had to be applied to A. baumannii for a 5.60 log reduction. Concerning 405 nm Acinetobacter likewise appeared to be the most susceptible genus with only 225 J/cm2 exposure that was necessary to reduce the non-pathogenic representative A. kookii by 5.19 log (Figure 2D). The microorganisms most difficult to diminish with 405 nm have been enterococci with E. faecium being inactivated by 3.06 log at 825 J/cm2 and E. moraviensis requiring 1296 J/cm2 for 3.08 log reduction (Figure 2F).
The difference in the effectiveness of the two wavelengths compared has been most apparent for pseudomonads (Figure 2E). For P. aeruginosa in addition the extensive development of a shoulder at 450 nm was observable, whereas the 405 nm inactivation progress illustrated an almost straight line, representing an exponential decline. Furthermore, the pathogen E. coli representative showed huge susceptibility differences between the two wavelengths, which is essentially caused by the low inactivation efficiency at 450 nm in this case (Figure 2A).
For 405 nm the non-pathogenic and pathogenic representatives of the same species exhibited similar behavior. Non-pathogenic strains tended to be rather less susceptible to 405 nm irradiation, becoming apparent when directly opposing log reductions. Indicating the non-pathogenic strain following by the pathogenic, reductions of 3.16/4.74 log at 225 J/cm2 for staphylococci, 0.69/2.55 log at 90 J/cm2 for Acinetobacter, 2.06/3.05 at 864/825 J/cm2 for enterococci, and 3.43/4.01 log at 225 J/cm2 for pseudomonads respectively, have been achieved. Only for E. coli the proportions are inverted with 6.36/0.84 log at 450/425 J/cm2, with the disparity being especially vast here. For the pathogenic E. coli strain BSU1286 a dose of 825 J/cm2 was necessary to reach a reduction of 5.26 log, which means that an approximately doubled dose was needed for the same reduction compared to the non-pathogenic relative (Figure 2A).
The same trend as for 405 nm became apparent for 450 nm. Likewise, the non-pathogenic strain was less susceptible than its pathogenic relative. Here we achieved log reductions of 2.27/5.69 at 500/400 J/cm2 for staphylococci, 2.30/6.61 at 375/400 J/cm2 for Acinetobacter, 1.73/3.89 log at 1728/1500 J/cm2 for enterococci, and 1.78/5.17 log at 1250 J/cm2 for pseudomonads, again first naming the non-pathogenic followed by the pathogenic strain. Again, the pathogenic E. coli strain was in contrary much less susceptible than the non-pathogenic strain with 4.66/0.33 log reduction at 750 J/cm2 of 450 nm. To reach an inactivation of 4.41 log the suspension had to be exposed to 2500 J/cm2 of 450 nm (Figure 2A).
Altogether, the least differences between pathogenic and non-pathogenic relative occurred for pseudomonads referring to the wavelength of 405 nm (Figure 2E). However, concerning 450 nm irradiation Pseudomonas together with Escherichia are the genera at which the differences between pathogenic and non-pathogenic strains investigated became most evident, albeit in opposite direction. Contemplating the general strain differences across all microorganisms, the differences at 450 nm irradiation had been more evident than for 405 nm.
In Figure 3 where data have been categorized concerning better comparability of different genera, it becomes apparent that 3 strains especially stand out regarding their insusceptibility. K. pneumoniae, E. coli BSU1286, and E. faecium (Figure 3A) provide a strong contrast compared to the other pathogen representatives at 405 nm and clearly divide the microorganisms in two different susceptibility groups. To achieve a 3 log reduction, the dose ranges of 100–175 J/cm2 compared to 650–800 J/cm2 had to be applied, respectively. Non-pathogenic strains (Figure 3B) lie closer together with a range of 125–200 J/cm2 with only E. moraviensis as outlier with around 1200 J/cm2 of 405 nm. Yet it is necessary to mention here, that a non-pathogenic Klebsiella strain was not available for testing.
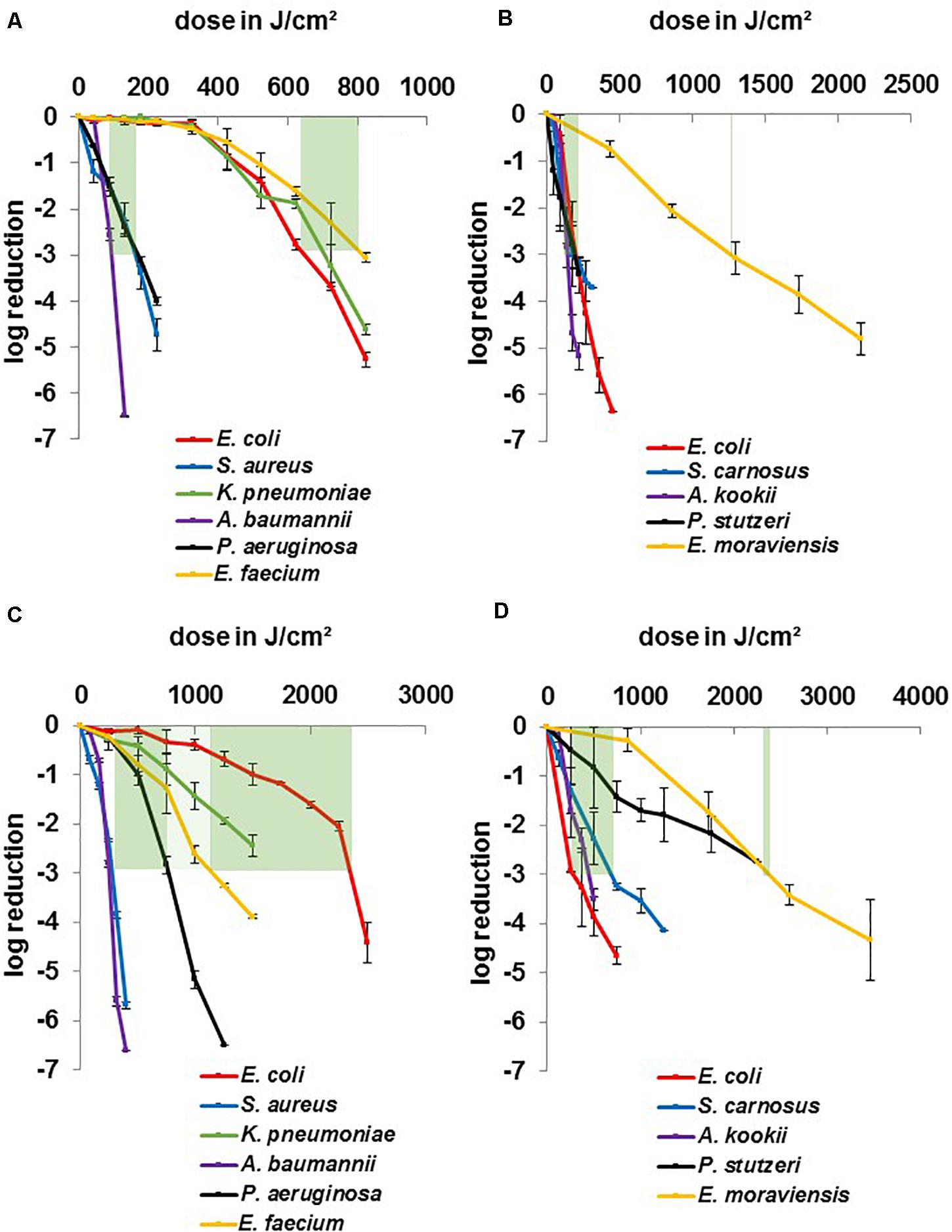
Figure 3. Inactivation results for pathogenic ESKAPE-strains and non-pathogenic relatives for 405 and 450 nm grouped by wavelength: pathogenic strains at 405 nm (A), non-pathogenic strains at405 nm (B), pathogenic strains at 450 nm (C), non-pathogenic strains at 450 nm (D). Green bars mark a dose range for similar behaving microorganisms for a 3 log reduction. Error bars represent standard deviation of three independent experiments.
A similar distribution is observable for the non-pathogenic strains at 450 nm (Figure 3D) with a dose range of 350–750 J/cm2 and E. moraviensis as well as P. stutzeri at around 2400 J/cm2, though it has to be noted that the field had widened at this wavelength. While E. coli and E. moraviensis required a doubled dose comparing 405 to 450 nm, S. carnosus and A. kookii exhibited even more reduced susceptibility at 450 nm, while still lying in the range of 2 to 5-fold dose increase, which is found in the literature comparing 405 and 450 nm (Hessling et al., 2017; Tomb et al., 2018). P. stutzeri required even the 12-fold dose at 450 nm compared to 405 nm, which is an unusual finding regarding the literature and was likewise not observed for the pathogenic P. aeruginosa.
The 450 nm dose range of the more susceptible pathogenic group (Figure 3C) was 250–750 J/cm2, indicating a 2 to 5-fold increase at the blue wavelength. Similarly, as for the non-pathogenic strains, it is observable that P. aeruginosa especially required higher doses at 450 nm, as mentioned before. E. faecium had not pulled apart extremely, but K. pneumoniae and E. coli BSU1286 spread the field to a dose range of 1,200–2,400 J/cm2. Overall, for pathogenic strains at 450 nm the dose distribution for different genera is evenly distributed. While the susceptibility tendencies of different genera are still visible, no similar clear separable categories as for 405 nm could be drawn.
Discussion
Photoinactivation with visible light has recently received increased interest due to the demand for the development of new disinfection technologies. Multiple studies investigating single bacterial species with a certain test setup at a specific wavelength have been published. However, extensive differences were observed in these data collections probably caused by a lack of uniform methodologies (Hessling et al., 2017; Tomb et al., 2018). Only few studies compare multiple strains with the same test protocol. Therefore, we aimed to investigate all ESKAPE pathogens under comparable conditions, including the comparison of related pathogenic and non-pathogenic species. As literature data about non-pathogenic microorganisms are still scarce we mainly concentrated on the pathogenic representatives for a comparison with the literature data to evaluate the achieved results.
Maclean et al. (2009) investigated 405 nm irradiation at 10 mW/cm2 against a broad range of microorganisms including all ESKAPE-pathogens. Their findings concerning the sensitivity of different genera is comparable to the results obtained here. A. baumannii, S. aureus, and P. aeruginosa are more susceptible to visible light, while for E. coli, enterococci and K. pneumoniae higher doses are necessary for 1 log reduction (Figure 2). Compared to our results there is a factor of approximately 2 in the dose for 1 log reduction of E. coli, enterococci and K. pneumoniae compared to Maclean et al. (2009), but the tendencies are similar, including the necessity of an exposure of about 300 J/cm2 for enterococci until inactivation progress is noticeable. In addition, the inactivation dose of 42.9 J/cm2 for 1 log reduction of P. aeruginosa is comparable to the 57.1 J/cm2 result achieved here.
Gupta et al. (2015) investigated clinical isolates from infectious hip and knee arthroplasties including all ESKAPE species except A. baumannii at 123 mW/cm2 of 405 nm irradiation. The study indicated the dose needed to reach an approximate 5 log reduction, which was their measured maximum. With 441.9 J/cm2 for a calculated 1 log average inactivation their results fit well to the calculated 492.2 J/cm2 for 1 log E. coli BSU1286 inactivation achieved here. For K. pneumoniae and S. aureus the averaged 1 log exposures were lower than in this study, even though clinical isolates were used by Gupta et al. (2015), which are often considered to be more challenging to inactivate. For E. faecium Halstead et al. (2016) delivered a value of 393 J/cm2 for 1 log reduction for a clinical isolate at 400 nm irradiation, while the strain investigated here required 525 J/cm2.
Concluding, the results for the necessary dose at 405 nm irradiation in this study are relatively high, especially concerning the inactivation of Enterobacteriaceae - including Klebsiella and Escherichia – and enterococci. For S. aureus there are several studies coming to similar conclusions with doses between 50.2 and 61.6 for a 1 log reduction (Enwemeka et al., 2008; Guffey et al., 2013; McKenzie et al., 2016). Furthermore, our data is in agreement with the literature that Acinetobacter baumannii, declared as one of the most problematic species in the WHO priority list (World Health Organization, 2017), is the most susceptible ESKAPE pathogen at 405 nm irradiation.
Irradiation around 450 nm has not been tested as extensively as 405 nm, hence the literature data available to compare with the results obtained here are limited. As expected, the doses required for inactivation have been higher at 450 nm for all strains investigated compared to 405 nm. Interestingly, a linear fit applied to the 450 nm data of E. faecium and K. pneumoniae delivered coefficients of 0.9453 and 0.9534, respectively, while “shoulders” existed for 405 nm irradiation. In contrast, for S. aureus and P. aeruginosa a linear fit was suitable at 405 nm, whereas a distinct “shoulder” appeared at 450 nm treatment. It was noticed before (Schmid et al., 2019) that there seems to be a correlation between the length of the wavelength, the duration of the slow initial inactivation and the occurrence of a shoulder. Webb and Brown (1976) already described similar observations concerning the wavelength dependency of the shoulder-effect in 1976 for E. coli.
For P. aeruginosa two very diverse literature results for 450 nm irradiation exist. Tomb et al. (2018) reported 91.9 J/cm2 (Keshishyan et al., 2015) and 428.6 J/cm2 (Decarli et al., 2016) for a 1 log reduction at 450 and 460 nm, respectively. We measured 0.98 log at 500 J/cm2 and can therefore reproduce the value from the second study, although the first study was conducted at 37°C and the second at room temperature. Even though longer wavelengths were considered to have less impact on bacteria, the wavelength of 460 nm is probably not very different from 450 nm, as the flavin absorption peak is relatively broad. For S. carnosus we previously determined an increase of 12% for the required dose at 460 nm compared to 450 nm when conducting an action spectrum (Hoenes et al., 2019).
Lui et al. (2016) investigated E. faecalis at 455 nm irradiation for drinking water disinfection and found a required exposure dose of 410 J/cm2 for a 1 log reduction. With 0.78 log at 500 J/cm2 our results for E. faecium are comparable.
Some studies were conducted on pathogenic E. coli at wavelengths between 450 and 455 nm (Lipovsky et al., 2010; Keshishyan et al., 2015; Lui et al., 2016) ranging from approximately 100–300 J/cm2 for 1 log inactivation but none of them is close to our results with 1500 J/cm2 for 1.00 log. Within this study the E. coli strain stands out, although the majority of the data for all strains investigated here already rank at the upper end of visible light inactivation data.
The investigated S. aureus strain however was inactivated by 1.22 log at 160 J/cm2 of 450 nm, while literature data suggest a 1 log dose of around 300 J/cm2 at 450 nm at 37°C (Keshishyan et al., 2015), 400 J/cm2 at 455 nm (Lipovsky et al., 2010), or 300 J/cm2 at 460 nm (Decarli et al., 2016) for methicillin-sensitive strains.
To our knowledge, there are no data available for the inactivation of any Acinetobacter or Klebsiella strain with 450 nm light.
However, it is difficult to compare the data on bacterial inactivation of different research groups. Due to the lack of uniform test setups and protocols influences might occur that are not apparent at first sight. In this study, special attention was paid to a homogenous irradiation intensity by applying a reflective pyramid structure. Depending on the light source arrangement, without a homogenizing technique, great variations in intensity can occur due to the punctiform emission of LEDs, making it difficult to relate the achieved bacterial impact to a certain dose applied over time. A specificity for the results of this study is the investigation at 37°C, directing at achieving a benchmark for applications at human body temperature. As most literature data were obtained at room temperature (with varying degree of monitoring and regulation), this can make a direct comparison difficult. The research groups that obtained data at different temperatures tend to suggest that lower temperatures are favorable for visible light inactivation (McKenzie et al., 2014; Keshishyan et al., 2015; Kumar et al., 2015). This might partly explain the ranking of our data at the upper end compared to available reference values at room temperature.
This study is part of an ongoing development of an enhanced endotracheal tube. A potential protoype was presented earlier (Sicks et al., 2020). Utilizing the antimicrobial effects of photoinactivation and the applicability without adding external photosensitizers we aim to equip endotracheal tubes with a light source for prevention of VAP. The susceptibility investigation of ESKAPE microorganisms at the body core temperature of 37°C forms the foundation for further experiments under more realistic conditions. Data of this universal scientific state are nevertheless important and can likewise be used for multiple further medical developments. Catheter based approaches were already suggested (Vollmerhausen et al., 2017; Huang et al., 2018) as well as an ingestible capsule (Romano et al., 2016) against gastric ulcer, but also (burn) wound treatment is conceivable (Dai et al., 2013; Halstead et al., 2016).
It has been discussed before whether the Gram properties of microorganisms affect their susceptibility towards visible light (Maclean et al., 2009). We could not detect any tendency, as staphylococci and enterococci, the two Gram positive species investigated, exhibited very different inactivation behavior and belonged to different groups of susceptibility for both 405 and 450 nm (Figure 3). Confirmative, there was no distinction found between the entirety of Gram positive and Gram negative species by Tomb et al. (2018) when reviewing literature data, with both groups requiring around 200 J/cm2 of 405 nm irradiation in average for a 1 log reduction. The same absence of a clear difference between Gram properties was noticed in Hessling et al. (2017).
Except from the E. coli strains the irradiation at both wavelengths indicated a lower susceptibility for the non-pathogenic representatives investigated. Abana et al. (2017) investigated various E. coli strains, including a non-pathogenic strain, on their 455 nm light inactivation behavior. Comparable to our results the non-pathogenic strain was the most susceptible with approximately 2 log reduction at 120 J/cm2. Lacombe et al. (2016) however did not detect differences in response to 405 nm between pathogenic and non-pathogenic E. coli strains. Nevertheless, the majority of non-pathogenic strains have been less susceptible in our study. In the supplementary material of the review of Tomb et al. (2018) we found a reference to a P. stutzeri investigation, achieving a 1 log reduction at 60 J/cm2 of 405 nm. Our result with 3.43 log reduction at 225 J/cm2 of 405 nm can be converted to 67.4 J/cm2 necessary for 1 log reduction by a linear fit at log scale, which matches perfectly.
Virulence factors have been a recent topic of investigation within light-based approaches, as it was found that they will be destroyed by antimicrobial photodynamic inactivation (aPDI) using external photosensitizers for irradiation treatment (Kömerik et al., 2000; Tubby et al., 2009; Kato et al., 2013; Pourhajibagher et al., 2018). Bartolomeu et al. (2016) even found in their study investigating 6 S. aureus strains with varying properties, that those producing endotoxins were more susceptible to the aPDI treatment. Tang et al. (2007) noticed a stronger inactivation by irradiation for a MRSA strain compared with the corresponding ATCC strain, ascribed to the altered penicillin binding protein, which lead to a more permeable cell wall. A ROS induced impairment of factors for antimicrobial resistance or virulence, not only integral for the pathogenic properties but also fulfilling physiological performance in the microorganisms (Peterson, 1996), might therefore contribute to an additional reduction in viability. However, there might exist differences comparing aPDI and visible light irradiation, which is solely based on endogenous photosensitizers. In this study we therefore investigated whether similar tendencies are detectable for 405 and 450 nm irradiation without external dyes and if virulence plays a role for the behavior concerning irradiation.
The American Society for Microbiology (ASM) published a study, on important criteria for choosing appropriate surrogates for pathogens (Sinclair et al., 2012). For testing disinfection methods, it is recommended to choose a strain that is rather resistant compared to the target microorganisms, and thus testing a kind of worst case scenario. This criterion is fulfilled for all our non-pathogenic strains, besides E. coli.
The ASM further recommends to achieve a performance of 99.9% reduction in bactericidal tests (American Society for Microbiology, 2019). All investigated strains in this study reached this goal for irradiation with both 405 nm and 450 nm, besides K. pneumoniae and P. stutzeri at 450 nm for which the applied maximum dose of 1500 J/cm2 and 2250 J/cm2 led to a 2.44 log and a 2.75 log inactivation, respectively. The kinetics of the inactivation curves however suggest that 3 log can be reached at higher doses.
Conclusion
To our best knowledge, this has been the first time that all ESKAPE pathogens have been investigated within one study and therefore with the same test methodology, not only on their susceptibility to 405 nm but also to 450 nm. All strains in this study could be inactivated significantly with both wavelengths.
It has also been the first time that related pathogenic and non-pathogenic bacterial species have been directly compared. In our investigation, non-pathogenic strains tend to be less susceptible than the pathogenic representatives at both wavelengths, with the differences being more pronounced at 450 nm. As non-pathogenic relatives are rather more difficult to inactivate, it is considered valid to choose non-pathogenic surrogates for the investigation of visible light photoinactivation.
An exception was noticed for E. coli BSU1286, where the pathogenic strain was far less susceptible, not only compared to the non-pathogenic relative, but also compared to all other strains and to the available literature data.
Data Availability Statement
The raw data supporting the conclusions of this article will be made available by the authors, without undue reservation.
Author Contributions
KH co-designed the study, analyzed the effect of 405 and 450 nm on non-pathogenic microorganisms, and wrote the manuscript. RB analyzed the effect of 405 and 450 nm on pathogenic microorganisms and edited the manuscript. TM constructed the irradiation setup, helped with the experiments on non-pathogenic microorganisms and edited the manuscript. BS and MH designed the study, supervised the study, and edited the manuscript. All authors contributed to the article and approved the submitted version.
Funding
This work was supported by the German Federal Ministry of Education and Research (grant 13N15140) which is gratefully acknowledged.
Conflict of Interest
The authors declare that the research was conducted in the absence of any commercial or financial relationships that could be construed as a potential conflict of interest.
References
American Society for Microbiology. (2019). Antimicrobial Agents and Chemotherpy, Instructions to Authors. Available online at: https://aac.asm.org/sites/default/files/additional-assets/AAC-ITA.pdf (accessed September 07, 2020).
Abana, C. M., Brannon, J. R., Ebbott, R. A., Dunigan, T. L., Guckes, K. R., Fuseini, H., et al. (2017). Characterization of blue light irradiation effects on pathogenic and nonpathogenic Escherichia coli. Microbiologyopen 6:e00466. doi: 10.1002/mbo3.466
Adair, T. L., and Drum, B. E. (2016). RNA-Seq reveals changes in the Staphylococcus aureus transcriptome following blue light illumination. Genomics Data 9, 4–6. doi: 10.1016/j.gdata.2016.05.011
Ahmed, I., Fang, Y., Lu, M., Yan, Q., El-Hussein, A., Hamblin, M. R., et al. (2018). Recent patents on light-based anti-infective approaches. Recent Pat. Antiinfect. Drug Discov. 13, 70–88. doi: 10.2174/1872213X11666171108104104
Ashkenazi, H., Malik, Z., Harth, Y., and Nitzan, Y. (2003). Eradication of Propionibacterium acnes by its endogenic porphyrins after illumination with high intensity blue light. FEMS Immunol. Med. Microbiol. 35, 17–24. doi: 10.1111/j.1574-695X.2003.tb00644.x
Barneck, M. D., Rhodes, N. L., de La Presa, M., Allen, J. P., Poursaid, A. E., Nourian, M. M., et al. (2016). Violet 405-nm light: a novel therapeutic agent against common pathogenic bacteria. J. Surg. Res. 206, 316–324. doi: 10.1016/j.jss.2016.08.006
Bartolomeu, M., Rocha, S., Cunha, Â, Neves, M. G., Faustino, M. A., and Almeida, A. (2016). Effect of photodynamic therapy on the virulence factors of Staphylococcus aureus. Front. Microbiol. 7:267. doi: 10.3389/fmicb.2016.00267
Biener, G., Masson-Meyers, D. S., Bumah, V. V., Hussey, G., Stoneman, M. R., Enwemeka, C. S., et al. (2017). Blue/violet laser inactivates methicillin-resistant Staphylococcus aureus by altering its transmembrane potential. J. Photochem. Photobiol. B Biol. 170, 118–124. doi: 10.1016/j.jphotobiol.2017.04.002
Boucher, H. W., Talbot, G. H., Bradley, J. S., Edwards, J. E., Gilbert, D., Rice, L. B., et al. (2009). Bad bugs, no drugs: no ESKAPE! An update from the Infectious Diseases Society of America. Clin. Infect. Dis. 48, 1–12. doi: 10.1086/595011
Chu, Z., Hu, X., Wang, X., Wu, J., Dai, T., and Wang, X. (2019). Inactivation of Cronobacter sakazakii by blue light illumination and the resulting oxidative damage to fatty acids. Can. J. Microbiol. 65, 922–929. doi: 10.1139/cjm-2019-0054
Cieplik, F., Späth, A., Leibl, C., Gollmer, A., Regensburger, J., Tabenski, L., et al. (2014). Blue light kills Aggregatibacter actinomycetemcomitans due to its endogenous photosensitizers. Clin. Oral Investig. 18, 1763–1769. doi: 10.1007/s00784-013-1151-8
Dai, T., Gupta, A., Huang, Y.-Y., Yin, R., Murray, C. K., Vrahas, M. S., et al. (2013). Blue light rescues mice from potentially fatal Pseudomonas aeruginosa burn infection: efficacy, safety, and mechanism of action. Antimicrob. Agents Chemother. 57, 1238–1245. doi: 10.1128/AAC.01652-12
Decarli, M. C., Carvalho, M. T., Corrêa, T. Q., Bagnato, V. S., and de Souza, C. W. (2016). Different Photoresponses of Microorganisms: from Bioinhibition to Biostimulation. Curr. Microbiol. 72, 473–481. doi: 10.1007/s00284-015-0976-6
Djouiai, B., Thwaite, J. E., Laws, T. R., Commichau, F. M., Setlow, B., Setlow, P., et al. (2018). Role of DNA repair and protective components in Bacillus subtilis spore resistance to inactivation by 400-nm-Wavelength Blue Light∗. Appl. Environ. Microbiol. 84:e01604-18. doi: 10.1128/AEM.01604-18
Endarko, E., Maclean, M., Timoshkin, I. V., MacGregor, S. J., and Anderson, J. G. (2012). High-intensity 405 nm light inactivation of Listeria monocytogenes. Photochem. Photobiol. 88, 1280–1286. doi: 10.1111/j.1751-1097.2012.01173.x
Enwemeka, C. S., Williams, D., Hollosi, S., Yens, D., and Enwemeka, S. K. (2008). Visible 405 nm SLD light photo-destroys methicillin-resistant Staphylococcus aureus (MRSA) in vitro. Lasers Surg. Med. 40, 734–737. doi: 10.1002/lsm.20724
European center for disease prevention and control (2009). The Bacterial Challenge: Time to React a Call to Narrow the Gap Between Multidrug-Resistant bacteria in the EU and the Development of New Antibacterial Agents. Luxembourg: Publications Office.
European center for disease prevention and control (2018). Healthcare-Associated Infections in Intensive Care Units: Annual Epidemiological Report for 2016. Stockholm: ECDC.
Fyrestam, J., Bjurshammar, N., Paulsson, E., Mansouri, N., Johannsen, A., and Östman, C. (2017). Influence of culture conditions on porphyrin production in Aggregatibacter actinomycetemcomitans and Porphyromonas gingivalis. Photodiagnosis Photodyn. Ther. 17, 115–123. doi: 10.1016/j.pdpdt.2016.11.001
Ganz, R. A., Viveiros, J., Ahmad, A., Ahmadi, A., Khalil, A., Tolkoff, M. J., et al. (2005). Helicobacter pylori in patients can be killed by visible light. Lasers Surg. Med. 36, 260–265. doi: 10.1002/lsm.20161
Ghate, V., Leong, A. L., Kumar, A., Bang, W. S., Zhou, W., and Yuk, H.-G. (2015). Enhancing the antibacterial effect of 461 and 521 nm light emitting diodes on selected foodborne pathogens in trypticase soy broth by acidic and alkaline pH conditions. Food Microbiol. 48, 49–57. doi: 10.1016/j.fm.2014.10.014
Guffey, J. S., Payne, W., Jones, T., and Martin, K. (2013). Evidence of resistance development by Staphylococcus aureus to an In Vitro, multiple stage application of 405 nm light from a Supraluminous Diode Array. Photomed. Laser Surg. 31, 179–182. doi: 10.1089/pho.2012.3450
Gupta, S., Maclean, M., Anderson, J. G., MacGregor, S. J., Meek, R. M., and Grant, M. H. (2015). Inactivation of micro-organisms isolated from infected lower limb arthroplasties using high-intensity narrow-spectrum (HINS) light. Bone Joint J. 97-B, 283–288. doi: 10.1302/0301-620X.97B2.35154
Halstead, F. D., Thwaite, J. E., Burt, R., Laws, T. R., Raguse, M., Moeller, R., et al. (2016). Antibacterial activity of blue light against nosocomial wound pathogens growing planktonically and as mature biofilms. Appl. Environ. Microbiol. 82, 4006–4016. doi: 10.1128/AEM.00756-16
Hamblin, M. R., and Abrahamse, H. (2019). Can light-based approaches overcome antimicrobial resistance? Drug Dev. Res. 80, 48–67. doi: 10.1002/ddr.21453
Hamblin, M. R., and Abrahamse, H. (2020). Oxygen-independent antimicrobial Photoinactivation: type III Photochemical Mechanism?. Antibiotics 9:53. doi: 10.3390/antibiotics9020053
Hamblin, M. R., Viveiros, J., Yang, C., Ahmadi, A., Ganz, R. A., and Tolkoff, M. J. (2005). Helicobacter pylori accumulates photoactive porphyrins and is killed by visible light. Antimicrob. Agents Chemother. 49, 2822–2827. doi: 10.1128/AAC.49.7.2822-2827.2005
Hessling, M., Spellerberg, B., and Hoenes, K. (2017). Photoinactivation of bacteria by endogenous photosensitizers and exposure to visible light of different wavelengths - a review on existing data. FEMS Microbiol. Lett. 364:fnw270. doi: 10.1093/femsle/fnw270
Hoenes, K., Wenzel, U., Spellerberg, B., and Hessling, M. (2019). Photoinactivation sensitivity of Staphylococcus carnosus to visible-light irradiation as a function of wavelength. Photochem. Photobiol. 96, 156–169. doi: 10.1111/php.13168
Huang, Y.-Y., Wintner, A., Seed, P. C., Brauns, T., Gelfand, J. A., and Hamblin, M. R. (2018). Antimicrobial photodynamic therapy mediated by methylene blue and potassium iodide to treat urinary tract infection in a female rat model. Sci. Rep. 8:7257. doi: 10.1038/s41598-018-25365-0
Hyun, J.-E., and Lee, S.-Y. (2020). Antibacterial effect and mechanisms of action of 460–470 nm light-emitting diode against Listeria monocytogenes and Pseudomonas fluorescens on the surface of packaged sliced cheese. Food Microbiol. 86:103314. doi: 10.1016/j.fm.2019.103314
Kato, I. T., Prates, R. A., Sabino, C. P., Fuchs, B. B., Tegos, G. P., Mylonakis, E., et al. (2013). Antimicrobial photodynamic inactivation inhibits Candida albicans virulence factors and reduces in vivo pathogenicity. Antimicrob. Agents Chemother. 57, 445–451. doi: 10.1128/AAC.01451-12
Keshishyan, E. S., Zaporozhtseva, Z. V., Zenina, O. M., and Zrodnikov, V. S. (2015). Photodynamic inactivation of bacteria in vitro under the effect of blue light. Bull. Exp. Biol. Med. 158, 475–477. doi: 10.1007/s10517-015-2788-x
Kim, M.-J., and Yuk, H.-G. (2017). Antibacterial Mechanism of 405-Nanometer Light-Emitting Diode against Salmonella at Refrigeration Temperature. Appl. Environ. Microbiol. 83:e02582-16. doi: 10.1128/AEM.02582-16
Kjeldstad, B., Johnsson, A., and Sandberg, S. (1984). Influence of pH on porphyrin production in Propionibacterium acnes. Arch. Dermatol. Res. 276, 396–400. doi: 10.1007/BF00413361
Kömerik, N., Wilson, M., and Poole, S. (2000). The effect of photodynamic action on two virulence factors of gram-negative bacteria. Photochem. Photobiol. 72, 676–680. doi: 10.1562/0031-865520000720676TEOPAO2.0.CO2
Kumar, A., Ghate, V., Kim, M.-J., Zhou, W., Khoo, G. H., and Yuk, H.-G. (2015). Kinetics of bacterial inactivation by 405nm and 520nm light emitting diodes and the role of endogenous coproporphyrin on bacterial susceptibility. J. Photochem. Photobiol. B Biol. 149, 37–44. doi: 10.1016/j.jphotobiol.2015.05.005
Lacombe, A., Niemira, B. A., Sites, J., Boyd, G., Gurtler, J. B., Tyrell, B., et al. (2016). Reduction of bacterial pathogens and potential surrogates on the surface of almonds using high-intensity 405-Nanometer Light. J. Food Prot. 79, 1840–1845. doi: 10.4315/0362-028X.JFP-15-418
Lipovsky, A., Nitzan, Y., Friedmann, H., and Lubart, R. (2009). Sensitivity of Staphylococcus aureus strains to broadband visible light. Photochem. Photobiol. 85, 255–260. doi: 10.1111/j.1751-1097.2008.00429.x
Lipovsky, A., Nitzan, Y., Gedanken, A., and Lubart, R. (2010). Visible light-induced killing of bacteria as a function of wavelength: implication for wound healing. Lasers Surg. Med. 42, 467–472. doi: 10.1002/lsm.20948
Lui, G. Y., Roser, D., Corkish, R., Ashbolt, N. J., and Stuetz, R. (2016). Point-of-use water disinfection using ultraviolet and visible light-emitting diodes. Sci. Total Environ. 553, 626–635. doi: 10.1016/j.scitotenv.2016.02.039
Maclean, M., Booth, M. G., Anderson, J. G., MacGregor, S. J., Woolsey, G. A., Coia, J. E., et al. (2013). Continuous decontamination of an intensive care isolation room during patient occupancy using 405 nm light technology. J. Infect. Prevent. 14, 176–181. doi: 10.1177/1757177413483646
Maclean, M., MacGregor, S. J., Anderson, J. G., and Woolsey, G. (2008). High-intensity narrow-spectrum light inactivation and wavelength sensitivity of Staphylococcus aureus. FEMS Microbiol. Lett. 285, 227–232. doi: 10.1111/j.1574-6968.2008.01233.x
Maclean, M., MacGregor, S. J., Anderson, J. G., and Woolsey, G. (2009). Inactivation of bacterial pathogens following exposure to light from a 405-nanometer light-emitting diode array. Appl. Environ. Microbiol. 75, 1932–1937. doi: 10.1128/AEM.01892-08
McKenzie, K., Maclean, M., Grant, M. H., Ramakrishnan, P., MacGregor, S. J., and Anderson, J. G. (2016). The effects of 405 nm light on bacterial membrane integrity determined by salt and bile tolerance assays, leakage of UV-absorbing material and SYTOX green labelling. Microbiology 162, 1680–1688. doi: 10.1099/mic.0.000350
McKenzie, K., Maclean, M., Timoshkin, I. V., MacGregor, S. J., and Anderson, J. G. (2014). Enhanced inactivation of Escherichia coli and Listeria monocytogenes by exposure to 405nm light under sub-lethal temperature, salt and acid stress conditions. Int. J. Food Microbiol. 170, 91–98. doi: 10.1016/j.ijfoodmicro.2013.10.016
Mulani, M. S., Kamble, E. E., Kumkar, S. N., Tawre, M. S., and Pardesi, K. R. (2019). Emerging strategies to combat ESKAPE Pathogens in the era of antimicrobial resistance: a review. Front. Microbiol. 10:539. doi: 10.3389/fmicb.2019.00539
Peterson, J. W. (1996). Bacterial Pathogenesis. Texas: University of Texas Medical Branch at Galveston.
Plavskii, V. Y., Mikulich, A. V., Tretyakova, A. I., Leusenka, I. A., Plavskaya, L. G., Kazyuchits, O. A., et al. (2018). Porphyrins and flavins as endogenous acceptors of optical radiation of blue spectral region determining photoinactivation of microbial cells. J. Photochem. Photobiol. B Biol. 183, 172–183. doi: 10.1016/j.jphotobiol.2018.04.021
Pourhajibagher, M., Rokn, A. R., Rostami-Rad, M., Barikani, H. R., and Bahador, A. (2018). Monitoring of virulence factors and metabolic activity in Aggregatibacter Actinomycetemcomitans cells surviving Antimicrobial Photodynamic Therapy via Nano-Chitosan Encapsulated Indocyanine Green. Front. Phys. 6:124. doi: 10.3389/fphy.2018.00124
Romano, G., Tortora, G., Calusi, S., Orsini, B., Gnerucci, A., and Fusi, F. (2016). Minimally invasive ingestible device to perform anti-bacterial phototherapy in the stomach. Phys. Med. 32:215. doi: 10.1016/j.ejmp.2016.07.724
Schmid, J., Hoenes, K., Vatter, P., and Hessling, M. (2019). Antimicrobial Effect of Visible Light-Photoinactivation of Legionella rubrilucens by Irradiation at 450, 470, and 620 nm. Antibiotics 8:187. doi: 10.3390/antibiotics8040187
Shehatou, C., Logunov, S. L., Dunman, P. M., Haidaris, C. G., and Klubben, W. S. (2019). Characterizing the Antimicrobial Properties of 405 nm Light and the Corning® Light-Diffusing Fiber Delivery System. Lasers Surg. Med. 51, 887–896. doi: 10.1002/lsm.23132
Sicks, B., Hönes, K., Spellerberg, B., and Hessling, M. (2020). Blue LEDs in endotracheal tubes may prevent ventilator-associated Pneumonia. Photobiomodul. Photomed. Laser Surg. 38, 571–576. doi: 10.1089/photob.2020.4842
Sinclair, R. G., Rose, J. B., Hashsham, S. A., Gerba, C. P., and Haas, C. N. (2012). Criteria for selection of surrogates used to study the fate and control of pathogens in the environment. Appl. Environ. Microbiol. 78, 1969–1977. doi: 10.1128/AEM.06582-11
Tang, H. M., Hamblin, M. R., and Yow, C. M. (2007). A comparative in vitro photoinactivation study of clinical isolates of multidrug-resistant pathogens. J. Infect. Chemother. 13, 87–91. doi: 10.1007/s10156-006-0501-8
Tomb, R. M., White, T. A., Coia, J. E., Anderson, J. G., MacGregor, S. J., and Maclean, M. (2018). Review of the comparative susceptibility of microbial species to Photoinactivation Using 380-480 nm Violet-Blue Light. Photochem. Photobiol. 94, 445–458. doi: 10.1111/php.12883
Tubby, S., Wilson, M., and Nair, S. P. (2009). Inactivation of staphylococcal virulence factors using a light-activated antimicrobial agent. BMC Microbiol. 9:211. doi: 10.1186/1471-2180-9-211
Vollmerhausen, T. L., Conneely, A., Bennett, C., Wagner, V. E., Victor, J. C., and O’Byrne, C. P. (2017). Visible and UVA light as a potential means of preventing Escherichia coli biofilm formation in urine and on materials used in urethral catheters. J. Photochem. Photobiol. B Biol. 170, 295–303. doi: 10.1016/j.jphotobiol.2017.04.018
Wang, Y., Wang, Y., Wang, Y., Murray, C. K., Hamblin, M. R., Hooper, D. C., et al. (2017). Antimicrobial blue light inactivation of pathogenic microbes: state of the art. Drug Resist. Updates 3, 1–22. doi: 10.1016/j.drup.2017.10.002
Webb, R. B., and Brown, M. S. (1976). Sensitivity of strains of Escherichia coli differing in repair capability to far UV, near UV and visible radiations. Photochem. Photobiol. 24, 425–432. doi: 10.1111/j.1751-1097.1976.tb06849.x
World Health Organization (2017). Global Priority List of Antibiotic-Resistant Bacteria to Guide Research, Discovery, and Development of New Antibiotics. Available online at: https://www.who.int/medicines/publications/global-priority-list-antibiotic-resistant-bacteria/en/ (accessed September 14, 2020).
Keywords: visible light irradiation, photoinactivation, antimicrobial blue light, ESKAPE pathogens, non-pathogen, 405 nm, 450 nm
Citation: Hoenes K, Bauer R, Meurle T, Spellerberg B and Hessling M (2021) Inactivation Effect of Violet and Blue Light on ESKAPE Pathogens and Closely Related Non-pathogenic Bacterial Species – A Promising Tool Against Antibiotic-Sensitive and Antibiotic-Resistant Microorganisms. Front. Microbiol. 11:612367. doi: 10.3389/fmicb.2020.612367
Received: 30 September 2020; Accepted: 11 December 2020;
Published: 13 January 2021.
Edited by:
Rodolfo García-Contreras, National Autonomous University of Mexico, MexicoReviewed by:
Brock Aaron Arivett, Middle Tennessee State University, United StatesBernardo Franco, University of Guanajuato, Mexico
Copyright © 2021 Hoenes, Bauer, Meurle, Spellerberg and Hessling. This is an open-access article distributed under the terms of the Creative Commons Attribution License (CC BY). The use, distribution or reproduction in other forums is permitted, provided the original author(s) and the copyright owner(s) are credited and that the original publication in this journal is cited, in accordance with accepted academic practice. No use, distribution or reproduction is permitted which does not comply with these terms.
*Correspondence: Katharina Hoenes, a2F0aGFyaW5hLmhvZW5lc0B0aHUuZGU=