- Jiangsu Collaborative Innovation Center of Chinese Medicinal Resources Industrialization, National and Local Collaborative Engineering Center of Chinese Medicinal Resources Industrialization and Formulae Innovative Medicine, Jiangsu Key Laboratory for High Technology Research of TCM Formulae, Nanjing University of Chinese Medicine, Nanjing, China
Plant-microorganism interaction in the rhizosphere is thought to play an important role in the formation of soil fertility, transformation and absorption of nutrients, growth and development of medicinal plants, and accumulation of medicinal ingredients. Yet, the role that they play in the phthalides accumulation of Angelica sinensis (Oliv.) Diels remains unclear. In the present study, we report a correlative analysis between rhizosphere microorganisms and phthalides accumulation in A. sinensis from Gansu, China where was the major production areas. Meanwhile, Bacillus was explored the potential functions in the plant growth and phthalide accumulation. Results revealed that the common bacterial species detected in six samples comprised 1150 OTUs which were involved in 368 genera, and predominant taxa include Actinobacteria, Acidobacteria, and Proteobacteria. The average contents of the six phthalides were 4.0329 mg/g. The correlation analysis indicated that 20 high abundance strains showed positive or negative correlations with phthalides accumulation. Flavobacterium, Nitrospira, Gaiella, Bradyrhizobium, Mycobacterium, Bacillus, RB41, Blastococcus, Nocardioides, and Solirubrobacter may be the key strains that affect phthalides accumulation on the genus level. By the plant-bacterial co-culture and fermentation, Bacillus which were isolated from rhizosphere soils can promote the plant growth, biomass accumulation and increased the contents of the butylidenephthalide (36∼415%) while the ligustilide (12∼67%) was decreased. Altogether, there is an interaction between rhizosphere microorganisms and phthalides accumulation in A. sinensis, Bacillus could promote butylidenephthalide accumulation while inhibiting ligustilide accumulation.
Introduction
The Chinese medicine producing areas are affected by many factors, such as light, soil, and water. Of the soil environmental factors, the rhizosphere as a micro-environment between the root system and soil interface plays an important role in the soil-root system-microbes interact with each other (Butler et al., 2003). The rhizosphere harbors diverse microbes, community composition and change of rhizosphere microorganisms are important indicators used for measuring soil quality, maintaining soil fertility, and affecting the quality of medicinal materials (Zhou et al., 2015; Xu et al., 2018). Some bacteria can conduce to improve the bioavailability of important mineral elements, such as N, P, and K (Meena et al., 2018); some bacteria can increase the ability of plants to resist stress and suppress soil diseases (Nihorimbere et al., 2012; Haney et al., 2015). Furthermore, it has been found that rhizosphere bacteria also affect the synthesis of officinal effective ingredients. In a previous study on Baphicacanthus cusia (NeeS) Bremek, Burkholderia was reported to play a major role in the synthesis of indigo (Zeng et al., 2018). In a separate study, the combination of Piriformospora indica and Azotobacter chroococcum increased the artemisinin content in Artemisia annua L (Arora et al., 2016). In another study, the rhizobacterial strain, N5.18, was found to significantly increased hypericin and pseudohypericin in Hypericum perforatum (Manero et al., 2012). Research on harnessing rhizosphere microorganisms for medicinal ingredients accumulation was meaningful in sustainable development of Chinese medicine resources.
The roots of A. sinensis are commonly used as an herbal drug and have a long officinal history in Chinese, Korean, and Japanese medicines (Sowndhararajan et al., 2017). A. sinensis is mainly distributed in Gansu, Yunnan and Sichuan Province, China. Due to the excellent quality, superior clinical effects and good market reputation, the Min County, Gansu Province is the traditional top-geoherb region of A. sinensis (Tan et al., 2015). In traditional Chinese medicine, it has functions of enriching blood, promoting blood circulation, relieving pain, and stimulating bowel evacuation, which is famous for its treatment of various gynecological diseases, known as “female ginseng” (Chen et al., 2013; Fang et al., 2014). Since the 1970s, 165 chemical compounds have been separated or identified in A. sinensis. Its chemical composition has also been connected to biological activities and clinical applications (Tan et al., 2015). Phthalides are one type of important active ingredient found in A. sinensis oil. Common phthalides, including ligustilide, butylphthalide, butylidenephthalide, senkyunolide H, senkyunolide I, and senkyunolide A, are usually used as biomarkers for quality evaluation (Wei and Huang, 2015).
Currently, research on the phthalides of A. sinensis mainly focuses on chemical constituent isolation and identification (Lv et al., 2018), quality control (Wei and Huang, 2015), pharmacological activity (Chen et al., 2017), catalytic reaction (Kattela et al., 2018), and practical application (Farias et al., 2018). Meanwhile, rhizosphere microorganism studies of A. sinensis mainly focus on investigating community changes and the effect of continuous cropping soil (Jiang et al., 2009; Zhang et al., 2015). However, our understanding and systematic research on the correlation between rhizosphere microorganisms and phthalides accumulation remain insufficient.
In this study, the main purpose was to reveal the correlation between rhizosphere microorganisms and phthalides accumulation from Gansu province. The ultra-high performance liquid chromatography-tandem mass spectrometry (UHPLC-MS/MS) method was utilized to determine the content of six phthalides in A. sinensis which were collected from Gansu. Meanwhile, Illumina MiSeq high-throughput sequencing technology was used to investigate the diversity and composition of rhizosphere microorganisms. A relationship analysis was conducted in order to reveal a correlation between rhizosphere microorganisms and phthalides accumulation. Then, the evaluation of the effect on the phthalides accumulation of two strains of Bacillus isolated from rhizosphere soils by tissue culture seedling co-culture and fermentation experiment. Thus, it could provide clues to further explore the influence of microorganisms on the medicinal materials quality.
Materials and Methods
Plant Materials and Reagents
The fresh roots of biennial A. sinensis were collected from Min Country, Dingxi city, Gansu Province, China (E 103.8571, N 34.5190, H 2943 m). The roots were authenticated by the corresponding author, Dr. Hui Yan. Roots were packed in sterile plastic bags and stored at −20°C for further analyses. Soil samples were collected from six random locations within the same origin. All collected soil samples were cleared of plant roots, leaf debris, and others, then stored at −80°C after flash freezing with liquid nitrogen for further analyses (Table 1).
Sample Preparation
Fresh samples were cut into small pieces (particle size < 0.5 cm), and 0.5 g fresh root was accurately weighed. Then, 20 mL methanol was added to a 50 mL conical flask with sonication at 100 Hz and 25°C for 45 min. After extraction when the samples were weighed, methanol was added if any weight loss was observed (Zhu et al., 2017). The supernatant on the sample plate was obtained by centrifugation at 13,000 r/min for 10 min and filtered through a 0.22 μm membrane filter then stored at 4°C before ultra-high performance liquid chromatography (UHPLC) analyses were conducted. The standard solution was prepared by mixing the six standard stock solutions, except clarithromycin, in equal amounts. Then, the solution was diluted with methanol to a suitable concentration for determining and constructing a standard curve. A detailed description of the six standard stock solutions was available in the Supplemental Experimental Procedures.
UHPLC-QqQ-MS Analysis
Chromatographic analyses were performed using a Waters Acquity UPLC system (Waters, Corp., MA, United States). Mass spectrometry (MS) was carried out using an AB SCIEX Triple Quad 6500 plus (AB SCIEX Corp., MA, United States) with electrospray ionization (ESI). The methodology validation and detailed description of chromatographic condition were showed in the Supplemental Experimental Procedures. All samples that removed moisture interference were prepared according to section 2.2; identification of the target peaks was performed by comparing their UHPLC retention times and mass/charge ratios (m/z) with the standards (Zhang et al., 2019). The concentration of each sample was calculated by an internal standard comparison method.
PCR Amplification and Illumina MiSeq Sequencing
Microbial DNA was extracted from soil samples by using an E.Z.N.A.® soil DNA Kit (Omega Bio-tek, GA, United States) following the manufacturer’s instructions. DNA concentration and purification were determined by using a NanoDrop 2000 UV-vis spectrophotometer (Thermo Scientific, DE, United States). DNA quality was checked by 1% agarose gel electrophoresis. An Illumina Miseq platform (Illumina, CA, United States) was used to measure the diversity and composition of the bacterial community. An equimolar amount of the purified PCR products were submitted to amplicon library on an Illumina MiSeq platform (Illumina, CA, United States) following the Shanghai Majorbio Bio-Pharm Technology Co. Ltd. (China) standard protocols (Yang and Wang, 2018). Sequencing was performed using Illumina Miseq PE300 platform (Shanghai Majorbio Bio-Pharm Technology Co., Ltd.). The datasets generated during the current study were deposited and are available at the National Center for Biotechnology Information (NCBI), Sequence Read Archive (SRA), under the accession number PRJNA5556711.
Amplicon Data Analysis
Raw fastq files were demultiplexed and quality-filtered by Trimmomatic, then merged by Flash v1.2.112. Operational taxonomic units (OTUs) were clustered with 97% similarity cutoff using UPARSE v7.03. Chimeric sequences were identified and removed using UCHIME. The taxonomy of each 16S rDNA sequence was analyzed using the RDP Classifier v2.24 algorithm against the Silva 16S rDNA database (Release1285) using a confidence threshold of 70% (Qu et al., 2018; Zhang et al., 2018). These sequences were clustered to OTUs with 97% sequence identity using Mothur software. OTUs were used for alpha diversity and beta diversity by QIIME v1.9.16. Alpha-diversity analysis is mainly for intra-sample comparison, including species dilution curve, richness and diversity index (Ace, Chao1, Simpson, Shannon index), etc., calculated by the Mothur component based on the OTUs level. Beta-diversity analysis is mainly a comparison between samples or groups. Correspondence diagrams between samples and bacteria are made by Circos7 which reflects the proportion of dominant bacteria in each sample. The PCoA analysis steps are as follows: first calculate the diversity matrix in the QIIME software according to the sample OTUs file and the evolutionary tree file, and the distance between the samples uses Bray-Curtis and weighted-unifrac, respectively, distance algorithm; then according to the distance matrix in the R software (V3.2.3) output graphics. All data analyses were conducted using the cloud platform8 provided by Shanghai Majorbio Bio-Pharm Technology Co., Ltd.
Co-culture of Different Bacterial Solutions With Tissue Culture Seedlings of A. sinensis
Two stains bacterial, XG-2 and XG-3, isolated from the A. sinensis soils of Min Country were identified as the Bacillus subtilis and Bacillus velezensis, based on 16S rDNA sequence. Seeds of A. sinensis which were collected from Min Country, Dingxi city, Gansu Province, China, were used in experiments. 20 μL sterile distilled water, 20 μL of XG-2-JY/XG-3-JY and XG-2-J/XG-3-J was added to each plant rhizosphere and cultivation was continued in the same condition when 30 to 40 days which the cotyledons were flattened and true leaf had grown were after planting. Each treatment was performed on 12 plants. In the colonization experiment, A. sinensis were collected at 7 days. Two plants as one sample was replicated in the experiment. Plant height were measured by using a precision straight edge (Deli, China). A whole plant was determined the fresh weight. Reference the method of “UHPLC-QqQ-MS Analysis” determined the phthalides contents. The culture conditions of bacterial and tissue culture seedling were available in the Supplemental Experimental Procedures.
Fermentation
Powders of A. sinensis were the organic nitrogen and carbohydrate source in the fermentation medium. The solid-state fermentation (XG-2-G/XG-3-G) was composed of 4 g A. sinensis powders, 1 g wheat bran, 6 mL sterile distilled water, and 1 mL bacterium liquid culture (Xia et al., 2017). The liquid fermentation (XG-2-Y/XG-3-Y) was composed of 5 g A. sinensis powders, 95 mL sterile distilled water, and 5 mL bacterium liquid culture. 1/5 mL sterile distilled water were added into fermentation medium as control-G/control-Y. All mixtures except bacterium liquid culture were sterilized at 121°C for 15 min. The cooled samples were incubated at 30°C with constant shaking at 160 rpm for 7 days after inoculating bacterium liquid culture. We set up control group with sterile distilled water. The mediums were dried to constant weight before content analysis. All experiments were divided six groups and three replications per treatment. Reference the method of “UHPLC-QqQ-MS Analysis” determined the phthalides contents.
Data Processing and Statistical Analyses
Student’s t-test of the phthalides content analysis and growth parameters was performed with SPSS v21.0. Differences between groups were considered significant when p < 0.05. The top 50 abundant genera and samples and the Spearman correlation coefficients of the top 50 abundant genera and phthalides accumulation were calculated and displayed on a heat map. Spearman correlation coefficients were calculated using R v3.5.1. The correlation coefficient is always between −1 and 1; the closer the correlation to ±1, the closer it is to a perfect linear relationship (Liu et al., 2017). Results was built and optimized using GraphPad Prism 7.0, which were presented as the mean ± standard deviation (SD).
Results
Diversity of the Soil Bacterial Community
The alpha bacterial community diversity indices, such as Sobs, Shannon, Simpson, Chao, Ace and Coverage were provided (Table 2). The Sobs means the observed richness in the samples. The Chao and Ace were the indexes to estimate the number of OTUs contained in the sample. The Shannon and Simpson were the indexes to estimate the community diversity in the sample. The larger Shannon index means higher community diversity, but the larger Simpson index means the lower community diversity. The M-ZC2-G-4 samples exhibited lower community diversity compared to other samples. The rarefaction curve (sobs index) showed the richness of species and plausibility of sequencing data in samples; as the rarefaction curves tended to approach saturation, it indicated the rationality of the data (Figure 1A). The Shannon-Wiener curve tended to be flat, and the amount of sequencing data was large enough to reflect the vast majority of microbial information in the samples (Figure 1B). Good’s coverage estimates for all soil samples were >0.95 and represented good sampling depth.
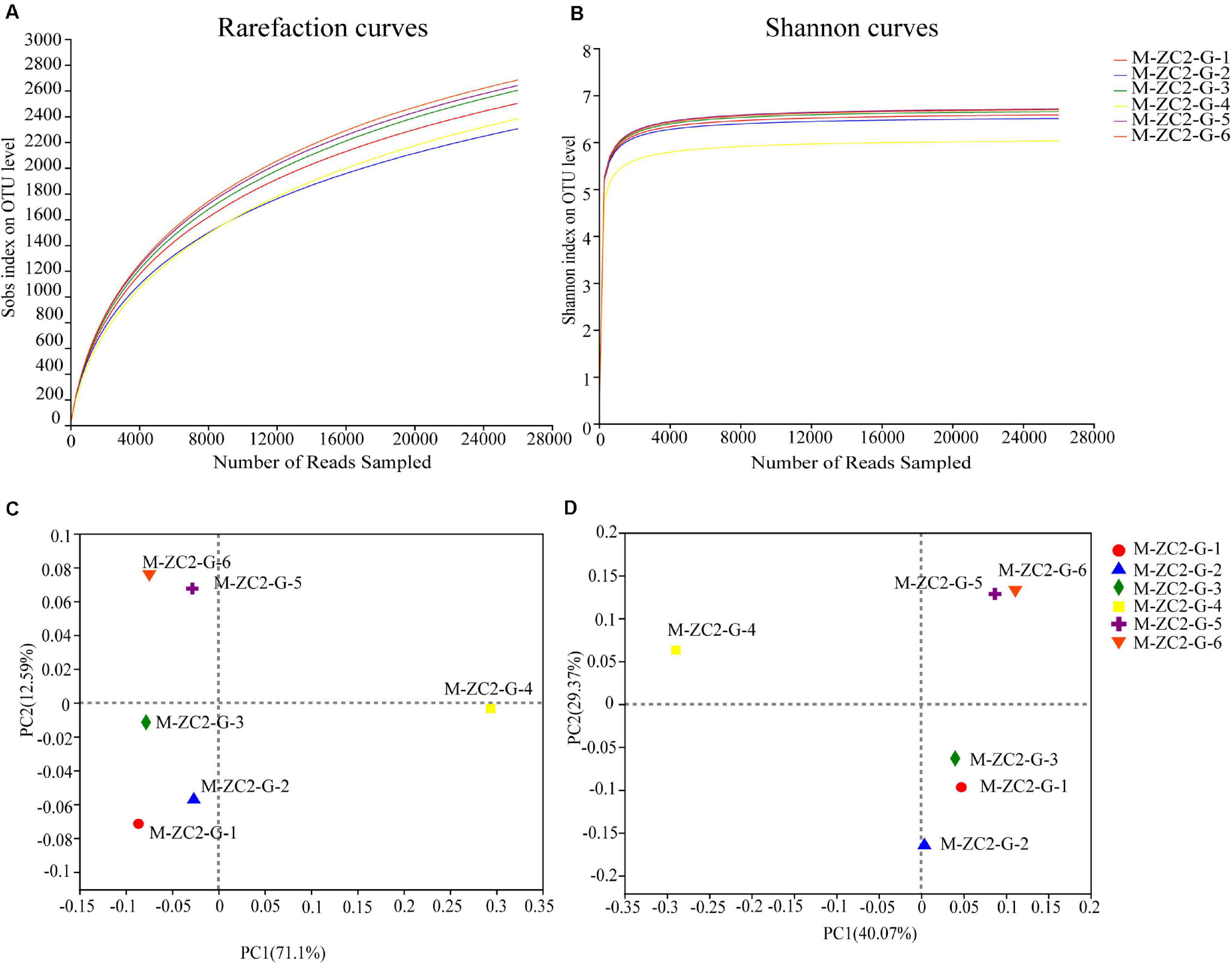
Figure 1. Alpha and Beta diversity analyses of sample soils. (A) Rarefaction curve from sobs index, (B) Shannon-Wiener curve, (C) PCoA analysis based on Bray-Curtis, and (D) PCoA analysis based on weighted-unifrac.
In order to analyze the differences in six soils, a principal coordinate analysis (PCoA) was conducted (Zhou et al., 2017, 2018). All data were normalized using SPSS. The PCoA analyses were based on Bray-Curtis and weighted-unifrac (Figures 1C,D). Similarity distances revealed that M-ZC2-G-4 was widely separated from the other Gansu samples (Bray-Curtis PC1 = 71.1%, PC2 = 12.59%; weighed-unifrac PC1 = 40.07%, and PC2 = 29.37%). The alpha bacterial community diversity indices were showed that the M-ZC2-G-4 sample exhibited lower community diversity compared to other samples. This also has an expression in beta bacterial community diversity which M-ZC2-G-4 was widely separated from the other Gansu samples.
Soil Bacterial Community Composition
A total of 302613 high-quality 16S rDNA sequences were obtained from 6 soil samples. These sequences were distributed among 4107 different OTUs at 97% similarity (Supplementary Table 1). Bacterial species detected in these samples comprised 36 phyla, 87 classes, 168 orders, 333 families, 648 genera, and 1431 species.
The bacterial composition was analyzed at the phylum and class level (Supplementary Figure 1). Results revealed that the soils were dominated by Actinobacteria, Proteobacteria, Chloroflexi, Acidobacteria, and Bacteroidetes. On the class level, the dominant strains were Actinobacteria, Alphaproteobacteria, Acidobacteria, Betaproteobacteria, and KD4-96. The Venn diagram revealed that 1150 OTUs of 368 genera were common in Gansu soils (Supplementary Figure 2). Results indicated that the diversity of bacterial composition was similar in the six rhizosphere soils. The common bacteria mainly concentrated on 27 phyla, Actinobacteria, Proteobacteria, Chloroflexi, Acidobacteria and Bacteroidetes, etc. Gracilibacteria has only existed in the M-ZC2-G-6 sample. No specific strain exists in other samples on phylum level. The relative abundance of bacteria in the rhizosphere soil was then analyzed on the genus level; the top 50 relatively abundant bacteria are mainly presented (Supplementary Figure 3). Analyze the dominant bacteria in each sample by Circos (Figure 2), the core top 10 bacteria with a relatively high abundance were Flavobacterium, Nitrospira, Gaiella, Bradyrhizobium, Mycobacterium, Bacillus, RB41, Blastococcus, Nocardioides, and Solirubrobacter.
Quantification of the Phthalides From Gansu Samples
Ultra-high performance liquid chromatography-QqQ-MS was applied to simultaneously determine six markers in 12 samples of A. sinensis (Figure 3 and Supplementary Table 2). The most selective and specific transition was chosen for MRM determination, and all MRM parameters are provided (Supplementary Table 3). The UHPLC method was validated by assessing linearity, precision, stability, LOD, LOQ, and recovery (Supplementary Table 4).
The content of ligustilide was highest among the six components in all samples (2.1857–4.4892 mg/g). The contents of the six phthalides were 4.0329 mg/g. The average contents of ligustilide, butylphthalide, butylidenephthalide, senkyunolide H, senkyunolide I and senkyunolide A were 3.5130, 0.0940, 0.0593, 0.0064, 0.1996, and 0.1607 mg/g, respectively.
Correlation Between Rhizosphere Microorganisms and Phthalides Accumulation
The Spearman correlation coefficient of phthalides accumulation and the top 50 abundant bacteria on genus level were calculated. Results revealed that 20 strains (Figure 4) which were concentrated in Proteobacteria, Actinobacteria and Chloroflexi exhibited the positive/negative correlations with phthalides accumulation.
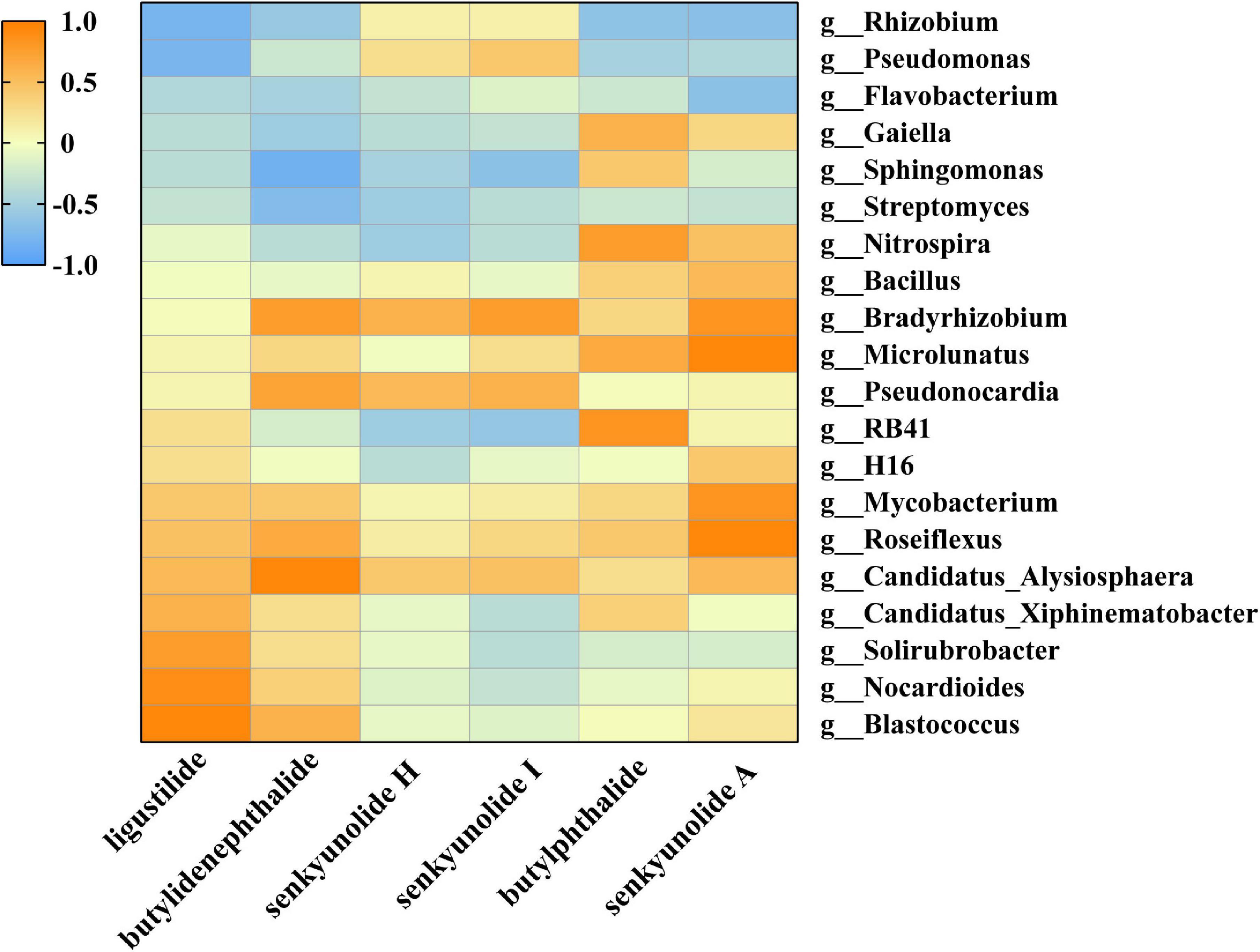
Figure 4. The 20 strains that exhibited a significant positive/negative correlation with phthalides accumulation.
Candidatus_Alysiosphaera, Roseiflexus, Mycobacterium, Pseudonocardia, and Bradyrhizobium exhibited a positive correlation with six phthalides. On the contrary, Streptomyces and Flavobacterium exhibited a negative correlation with six phthalides. Of course, some strains show different effects on the six phthalides. Several bacteria, including Rhizobium and Pseudomonas exhibited a negative correlation with ligustilide, butylidenephthalide, senkyunolide A and butylphthalide while they were showed a positive correlation with senkyunolide H, senkyunolide I. Some strains, such as Nitrospira and Bacillus exhibited a negative correlation with ligustilide, butylidenephthalide and senkyunolide I, but they also showed a positive correlation with senkyunolide A and butylphthalide. These results suggest that the rhizosphere bacteria were involved in six phthalides accumulation (Supplementary Table 5), which indicates that these can be used as important biomarkers when the correlation between the rhizosphere microorganisms and phthalides accumulation is considered.
Plant Growth Parameters and Phthalides Contents
Bacillus subtilis (XG-2) and Bacillus velezensis (XG-3) bacterial cell suspension addition all significantly increased the plant heights and fresh weights (Figure 5). The average plant heights and fresh weights in the co-culture with XG-2-JY (2.73 cm, 36.56 mg)/XG-3-JY (2.28 cm, 34.87 mg) and XG-2-J (2.57 cm, 35.17 mg)/XG-3-J (2.40 cm, 28.46 mg) was significantly higher than the control samples (1.75 cm, 27.55 mg) (p < 0.05). The result proved that Bacillus strains had a function in growth promoting of A. sinensis.
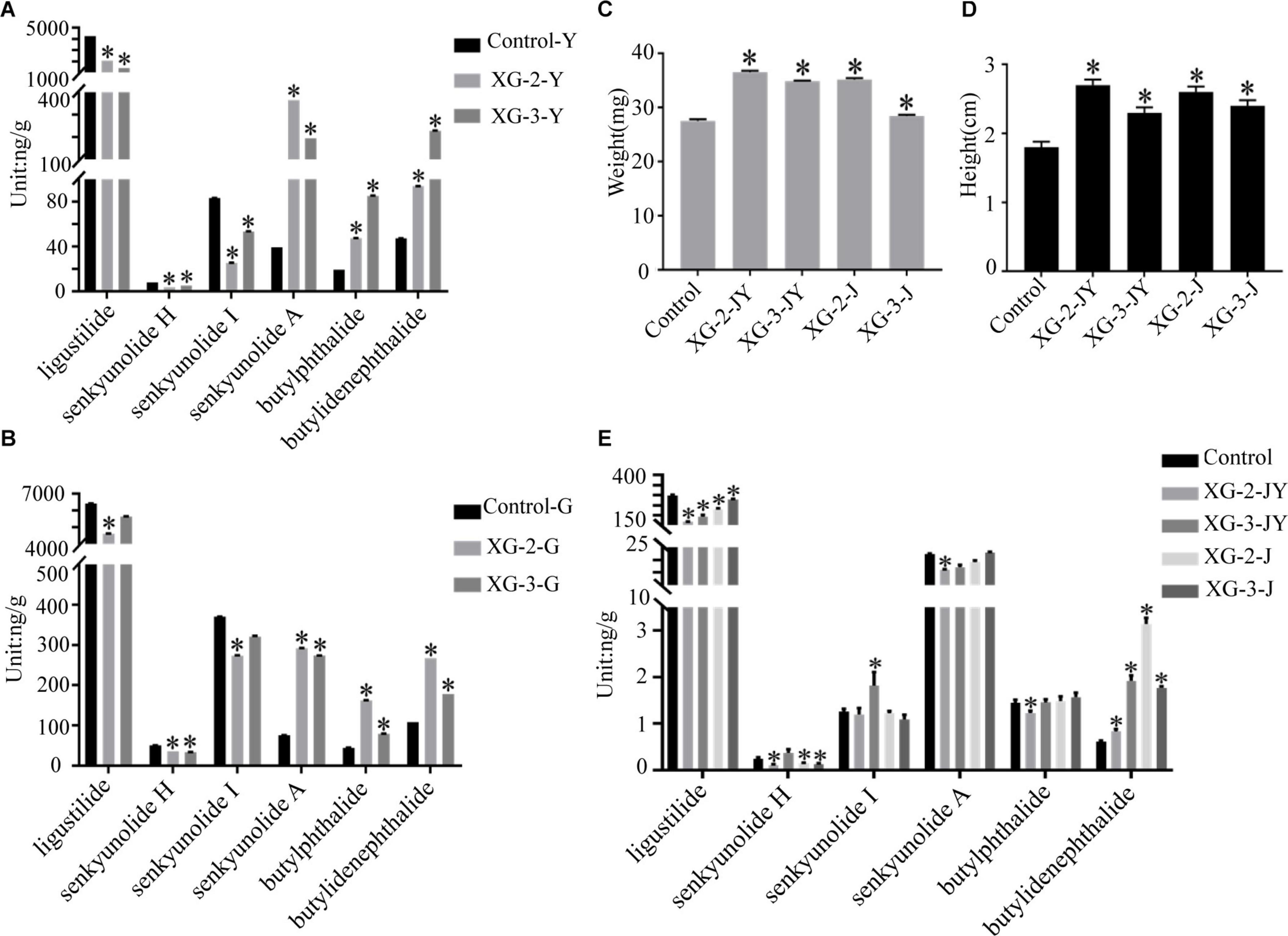
Figure 5. The plant growth parameters and phthalides contents. (A) Phthalides contents of liquid fermentation, (B) phthalides contents of solid-state fermentation, (C) plant heights of tissue culture seedlings, (D) plant fresh weights of tissue culture seedlings, (E) phthalides contents of issue culture seedlings. Asterisks indicate significant differences relative to control (∗p < 0.05, Student’s t-test).
The effect of phthalides accumulation by co-culture and fermentation showed Bacillus subtilis and Bacillus velezensis could increase the contents of the butylidenephthalide while the ligustilide was decreased. The average content of ligustilide in the XG-2-JY/J/Y/G (169.9205, 230.7876, 2083.6412, 4634.7486 μg/g, respectively) and XG-3-JY/J/Y/G (193.8898, 44.9352, 1400.9418, 5661.0393 μg/g, respectively) groups was significantly lower than the control/Y/G (298.52, 4268.0747, 6420.3539 μg/g) (p < 0.05 except XG-3-G). The average content of butylidenephthalide in the XG-2-JY/J/Y/G (0.8290, 3.1409, 94.0077, 267.2646 μg/g, respectively) and XG-3-JY/J/Y/G (1.9195, 0.8703, 229.3146, 178.1602 μg/g, respectively) groups was significantly higher than the control/Y/G (0.6087, 47.2929, 108.3795 μg/g) (p < 0.05). The results revealed that Bacillus subtilis and Bacillus velezensis play an important role in plant growth and different phthalides accumulation of A. sinensis.
Discussion
For the soil bacteria community and diversity of rhizosphere microorganisms from Gansu soils in this study, core bacterial of the A. sinensis rhizosphere microbiota by Circos analysis were Flavobacterium, Nitrospira, Gaiella, Bradyrhizobium, Mycobacterium, Bacillus, RB41, Blastococcus, Nocardioides, and Solirubrobacter. Actinobacteria which were a group of important plant-associated spore-forming bacteria were well-known as dominant phyla in soil (Novello et al., 2017). In our result, half of the core bacterial, including Gaiella, Mycobacterium, Blastococcus, Nocardioides, and Solirubrobacter, belong to Actinobacteria. Many researches have reported for their biocontrol, plant growth promotion, and interaction with plant (Palaniyandi et al., 2013). The study of the biosynthesis of phthalides, which began with the structural determination of mycophenolic acid, was showed the phthalide fragment derived from the polyketide pathway (Leon et al., 2017). Specially, Mycobacterium, and Nocardioides which show a positive correlation with phthalides accumulation were found to be with the potential for producing polyketides (Saxena et al., 2003; Sun et al., 2012). Moreover, Nitrospira play an important role in Nitrification which is a central nitrogen (N) cycling process, the sequential aerobic oxidation of ammonia to nitrate via nitrite (Koch et al., 2019). Bacillus are known to contain plant growth promoting bacteria with being able to produce ammonia, siderophores, catalase, indole acetic acid (Khan et al., 2019) and antibiotics against soilborne pathogenic fungi (Cazorla et al., 2007). Flavobacterium had the function of degradation of organophosphorus compounds (Singh and Walker, 2006). The various functions of the strains may be the reason why they become core bacteria in A. sinensis soils.
In this study, two strains, Bacillus subtilis and Bacillus velezensis, were isolated from the rhizosphere soil of A. sinensis. Bacillus was one of the core top 10 abundant strains on genus level which was exhibited correlations with phthalides accumulation. In the treatment experiments, the results indicated Bacillus has a significant positive effect on the plant heights and fresh weights. The previous study has reported that Bacillus were found to possess nitrogen fixing, phosphorous solubility and IAA biosynthesis activities to promote the growth of black pepper (Lau et al., 2020). These characteristics of Bacillus were its possible way of promoting the growth of A. sinensis tissue culture seedling. Hence, Bacillus can act as an alternative to plant growth enhancement agrochemicals in the field cultivation of A. sinensis. However, as a Chinese medicinal material, it was also necessary to consider the accumulation of its medicinal active ingredients during the growth process. In present study, Bacillus subtilis and Bacillus velezensis could increase the contents of the butylidenephthalide while the ligustilide was decreased. Although the result was consistent with the correlation analysis, it had some certain questions in practical applications. For the influence of phthalides accumulation suggested that we should pay attention to the addition quantity when using Bacillus as a bio-fertilizer of A. sinensis.
Many researches proved the plant growth promoting rhizobacteria (PGPR) as a biological fertilizer could stimulate plant growth and component accumulation (Nawaz et al., 2020). Based on the correlation analysis and results of co-culture and fermentation, the interaction between rhizosphere microorganisms and phthalides accumulation may depend on two possible pathways, direct and indirect mechanisms. In terms of direct mechanisms, bacteria colonize the plant and produced the components, such as some enzymes, that were involved in the synthesis of ligustilide and other components. In terms of indirect mechanisms, bacteria produced some components in rhizosphere, but only when the components were absorbed and utilized by plants, it could participate in phthalide accumulation. For example, the mechanism of biological control reduced the impact of diseases by the rhizobacteria production of siderophores and protection enzymes, such as chitinase, glucanase, and ACC-deaminase (Ongena and Jacques, 2008; Vejan et al., 2016). Notably, a plant’s own secretions will affect rhizosphere microorganisms. A previous report was showed that ligustilide at a 100 μg/mL concentration was confirmed against Bacillus (Chou et al., 2006). Moreover, Bacillus exhibited high degradation efficiency of a polycyclic aromatic hydrocarbons (PAHs) mixture of naphthalene, fluorene, phenanthrene, anthracene, and fluoranthene in soils heavily contaminated with crude oil (Ma et al., 2018). It may be the high degradation and moderate inhibition ability exhibited by Bacillus that contributes to its negative correlation with ligustilide. Due to the lack of research on phthalides biosynthetic pathways, the mechanism of Bacillus effect on phthalides accumulation was still unclear which should need a further exploration.
Conclusion
Overall, the rhizosphere microorganisms and phthalides accumulation indicated that rhizosphere microorganisms may play an important role in phthalides accumulation, meanwhile, phthalides also was showed an effect on bacteria growth. These findings will serve as supplemental information regarding the rhizosphere microorganisms of A. sinensis and are of great scientific significance with a potential applicable value in the rhizosphere of A. sinensis. Although the result provided some insight of the rhizosphere microorganisms as the bio-fertilizer in order to the sustainable development of A. sinensis, the interaction between rhizosphere microorganisms and phthalides accumulation will only be understood when we have better comprehension in the potential functions of rhizosphere microorganisms and the information of phthalides biosynthesis. In the long run, this information could assist the screening of beneficial probiotics in the rhizosphere as the bio-fertilizer in order to improve the yield and phthalides accumulation and promote sustainable development of the A. sinensis.
Data Availability Statement
The datasets presented in this study can be found in online repositories. The names of the repository/repositories and accession number(s) can be found below: https://www.ncbi.nlm.nih.gov/, PRJNA555671.
Author Contributions
W-MF, PL, HY, and J-AD conceived and designed the experiments. W-MF and J-WM performed the experiments. W-MF, PL, E-XS, and D-WQ analyzed the data. J-AD, D-WQ, SZ, GY, and SJ contributed reagents, materials, and analysis tools. W-MF and PL wrote the manuscript. All authors have read and approved the final manuscript.
Funding
This research was supported financially by National Natural Science Foundation of China (81773848), Special FundsProject for the Construction of Modern Agricultural Technology System (CARS-21), and Natural Science Foundation of Jiangsu Province, China (BK20201403). This work was also partly sponsored by Six talents peaks project in Jiangsu Province (JNHB-066), Qing Lan Project, the Major Projects of Natural Science Foundation of Universities in Jiangsu Province (19KJA320002) and College Students’ Innovative Entrepreneurial Training Plan Program of Jiangsu Province (201910315037Z).
Conflict of Interest
The authors declare that the research was conducted in the absence of any commercial or financial relationships that could be construed as a potential conflict of interest.
Acknowledgments
We thank Shanghai Majorbio Bio-Pharm Technology Co., Ltd. for providing a sequencing platform. We also thank LetPub (www.letpub.com) for its linguistic assistance during the preparation of this manuscript.
Supplementary Material
The Supplementary Material for this article can be found online at: https://www.frontiersin.org/articles/10.3389/fmicb.2020.611143/full#supplementary-material
Footnotes
- ^ https://www.ncbi.nlm.nih.gov/bioproject/PRJNA555671
- ^ https://ccb.jhu.edu/software/FLASH/index.shtml
- ^ http://drive5.com/uparse/
- ^ http://sourceforge.net/projects/rdp-classifier/
- ^ http://www.arb-silva.de
- ^ http://qiime.org/install/index.html
- ^ http://circos.ca/
- ^ https://www.i-sanger.com/
References
Arora, M., Saxena, P., Choudhary, D. K., Abdin, M. Z., and Varma, A. (2016). Dual symbiosis between Piriformospora indica and Azotobacter chroococcum enhances the artemisinin content in Artemisia annua L. World J. Microbiol. Biotechnol. 32:19. doi: 10.1007/s11274-015-1972-5
Butler, J. L., Williams, M. A., Bottomley, P. J., and Myrold, D. D. (2003). Microbial community dynamics associated with rhizosphere carbon flow. Appl. Environ. Microbiol. 69, 6793–6800. doi: 10.1128/aem.69.11.6793-6800.2003
Cazorla, F. M., Romero, D., Perez-Garcia, A., Lugtenberg, B. J. J., de Vicente, A., and Bloemberg, G. (2007). Isolation and characterization of antagonistic Bacillus subtilis strains from the avocado rhizoplane displaying biocontrol activity. J. Appl. Microbiol. 103, 1950–1959. doi: 10.1111/j.1365-2672.2007.03433.x
Chen, M. C., Hsu, W. L., Chang, W. L., and Chou, T. C. (2017). Antiangiogenic activity of phthalides-enriched Angelica Sinensis extract by suppressing WSB-1/pVHL/HIF-1alpha/VEGF signaling in bladder cancer. Sci. Rep. 7:5376. doi: 10.1038/s41598-017-05512-9
Chen, X.-P., Li, W., Xiao, X.-F., Zhang, L.-L., and Liu, C.-X. (2013). Phytochemical and pharmacological studies on Radix Angelica sinensis. Chin. J. Nat. Med. 11, 577–587. doi: 10.1016/s1875-5364(13)60067-9
Chou, S. C., Everngam, M. C., Sturtz, G., and Beck, J. J. (2006). Antibacterial activity of components from Lomatium californicum. Phytother. Res. 20, 153–156. doi: 10.1002/ptr.1828
Fang, L., Xiao, X. F., Liu, C. X., and He, X. (2012). Recent advance in studies on Angelica sinensis. Chin. Herbal Med. 4:14. doi: 10.3969/j.issn.1674-6384.2012.01.004.
Farias, E. S., Silva, E. M. P., Teixeira, M. G., Ferreira, J. S., Alvarenga, E. S., and Marcelo, C. P. (2018). Phthalides as promising insecticides against Tuta absoluta (Lepidoptera: Gelechiidae). J. Environ. Sci. Health B 53, 49–56. doi: 10.1080/03601234.2017.1371556
Haney, C. H., Samuel, B. S., Bush, J., and Ausubel, F. M. (2015). Associations with rhizosphere bacteria can confer an adaptive advantage to plants. Nat. Plants 1:15051. doi: 10.1038/nplants.2015.51
Jiang, S., Duan, J., Yan, H., and Yu, G. (2009). Population structure and ecological distribution of rhizospheric microorganisms of Angelica sinensis. Zhongguo Zhong Yao Za Zhi 34, 1483–1488. doi: 10.3724/sp.j.1011.2008.01483
Kattela, S., De Lucca, E. C. Jr., and Correia, C. R. D. (2018). Enantioselective Synthesis of Phthalides and Isochromanones via Heck-Matsuda Arylation of Dihydrofurans. Chemistry 24, 17691–17696. doi: 10.1002/chem.201804958
Khan, N., Bano, A., Rahman, M. A., Jia, G., Zhiyu, K., and Ali, B. (2019). Comparative Physiological and Metabolic Analysis Reveals a Complex Mechanism Involved in Drought Tolerance in Chickpea (Cicer arietinum L.) Induced by PGPR and PGRs. Sci. Rep. 9:2097. doi: 10.1038/s41598-019-38702-8
Koch, H., van Kessel, M. A. H. J., and Lucker, S. (2019). Complete nitrification: insights into the ecophysiology of comammox Nitrospira. Appl. Microbiol. Biotechnol. 103, 177–189. doi: 10.1007/s00253-018-9486-3
Lau, E. T., Tani, A., Khew, C. Y., Chua, Y. Q., and Hwang, S. S. (2020). Plant growth-promoting bacteria as potential bio-inoculants and biocontrol agents to promote black pepper plant cultivation. Microbiol Res. 8:126549. doi: 10.1016/j.micres.2020.126549
Leon, A., Del-Angel, M., Avila, J. L., and Delgado, G. (2017). Phthalides: distribution in nature, chemical reactivity, synthesis, and biological activity. J. Prog. Chem. Org. Nat. Prod. 2017, 127–246. doi: 10.1007/978-3-319-45618-8_2
Liu, P., Shang, E. X., Zhu, Y., Yu, J. G., Qian, D. W., and Duan, J. A. (2017). Comparative analysis of compatibility effects on invigorating blood circulation for cyperi rhizoma series of herb pairs using untargeted metabolomics. Front. Pharmacol. 8:677. doi: 10.3389/fphar.2017.00677
Lv, J. L., Zhang, L. B., and Guo, L. M. (2018). Phthalide dimers from Angelica sinensis and their COX-2 inhibition activity. Fitoterapia 129, 102–107. doi: 10.1016/j.fitote.2018.06.016
Ma, X. K., Li, T. T., Fam, H., Charles Peterson, E., Zhao, W. W., and Bo, Z. (2018). The influence of heavy metals on the bioremediation of polycyclic aromatic hydrocarbons in aquatic system by a bacterial-fungal consortium. Environ. Technol. 39, 2128–2137. doi: 10.1080/09593330.2017.1351492
Manero, F. J., Algar, E., Martin Gomez, M. S., Saco Sierra, M. D., and Solano, B. R. (2012). Elicitation of secondary metabolism in Hypericum perforatum by rhizosphere bacteria and derived elicitors in seedlings and shoot cultures. Pharm. Biol. 50, 1201–1209. doi: 10.3109/13880209.2012.664150
Meena, V. S., Zaid, A., Maurya, B. R., Meena, S. K., Bahadur, I., and Saha, M. (2018). Evaluation of potassium solubilizing rhizobacteria (KSR): enhancing K-bioavailability and optimizing K-fertilization of maize plants under Indo-Gangetic Plains of India. Environ. Sci. Pollut. Res. Int. 25, 36412–36424. doi: 10.1007/s11356-018-3571-0
Nawaz, A., Shahbaz, M., Asadullah, Imran, A., Marghoob, M. U., Imtiaz, M., et al. (2020). Potential of salt tolerant PGPR in growth and yield augmentation of wheat (Triticum aestivum L.) Under Saline Conditions. Front. Microbiol. 2:2019. doi: 10.3389/fmicb.2020.02019
Nihorimbere, V., Cawoy, H., Seyer, A., Alain, B., Philippe, T., and Marc, O. (2012). Impact of rhizosphere factors on cyclic lipopeptide signature from the plant beneficial strain Bacillus amyloliquefaciens S499. FEMS Microbiol. Ecol. 79, 176–191. doi: 10.1111/j.1574-6941.2011.01208.x
Novello, G., Gamalero, E., Bona, E., Lara, B., Flavio, M., and Nadia, M. (2017). The Rhizosphere Bacterial Microbiota of Vitis vinifera cv. pinot noir in an integrated pest management vineyard. Front. Microbiol. 14:1528. doi: 10.3389/fmicb.2017.01528
Ongena, M., and Jacques, P. (2008). Bacillus lipopeptides: versatile weapons for plant disease biocontrol. Trends Microbiol. 16, 115–125. doi: 10.1016/j.tim.2007.12.009
Palaniyandi, S. A., Yang, S. H., Zhang, L., and Suh, J. W. (2013). Effects of actinobacteria on plant disease suppression and growth promotion. Appl. Microbiol. Biotechnol. 97, 9621–9636. doi: 10.1007/s00253-013-5206-1
Qu, M., Li, N., Li, H., Yang, T., Liu, W., Yan, Y., et al. (2018). Phytoextraction and biodegradation of atrazine by Myriophyllum spicatum and evaluation of bacterial communities involved in atrazine degradation in lake sediment. Chemosphere 209, 439–448. doi: 10.1016/j.chemosphere.2018.06.055
Saxena, P., Yadav, G., Mohanty, D., and Gokhale, R. S. (2003). A new family of type III polyketide synthases in Mycobacterium tuberculosis. J. Biol. Chem. 278, 44780–44790. doi: 10.1074/jbc.M306714200
Singh, B. K., and Walker, A. (2006). Microbial degradation of organophosphorus compounds. FEMS Microbiol. Rev. 30, 428–471. doi: 10.1111/j.1574-6976.2006.00018.x
Sowndhararajan, K., Deepa, P., Kim, M., Park, S. J., and Kim, S. (2017). A review of the composition of the essential oils and biological activities of angelica species. Sci. Pharm. 85:33. doi: 10.3390/scipharm85030033
Sun, W., Peng, C., Zhao, Y., and Li, Z. (2012). Functional gene-guided discovery of type II polyketides from culturable actinomycetes associated with soft coral Scleronephthya sp. PLoS One 7:e42847. doi: 10.1371/journal.pone.0042847
Tan, H. S., Hu, D. D., Song, J. Z., Xu, Y., Cai, S. F., and Chen, Q. L. (2015). Distinguishing Radix Angelica sinensis from different regions by HS-SFME/GC-MS. Food Chem. 186, 200–206. doi: 10.1016/j.foodchem.2014.05.152
Vejan, P., Abdullah, R., Khadiran, T., Ismail, S., and Nasrulhaq Boyce, A. (2016). Role of plant growth promoting rhizobacteria in agricultural sustainability-a review. Molecules 21:573. doi: 10.3390/molecules21050573
Wei, W. L., and Huang, L. F. (2015). Simultaneous determination of ferulic acid and phthalides of Angelica sinensis based on UPLC-Q-TOF/MS. Molecules 20, 4681–4694. doi: 10.3390/molecules20034681
Xia, J., Li, R., He, A., Xu, J., Liu, X.\, Li, X., et al. (2017). Production of poly (β-l-malic acid) by Aureobasidium pullulans HA-4D under solid-state fermentation. Bioresour Technol. 244, 289–295. doi: 10.1016/j.biortech.2017.07.148
Xu, J., Zhang, Y., Zhang, P., Pankaj, T., Nadia, R., and Yayu, W. (2018). The structure and function of the global citrus rhizosphere microbiome. Nat. Commun. 9:4894. doi: 10.1038/s41467-018-07343-2
Yang, G., and Wang, J. (2018). Kinetics and microbial community analysis for hydrogen production using raw grass inoculated with different pretreated mixed culture. Bioresour. Technol. 247, 954–962. doi: 10.1016/j.biortech.2017.09.041
Zeng, M., Zhong, Y., Cai, S., and Diao, Y. (2018). Deciphering the bacterial composition in the rhizosphere of Baphicacanthus cusia (NeeS) Bremek. Sci. Rep. 8:15831. doi: 10.1038/s41598-018-34177-1
Zhang, C., Zhang, W., Zhang, J., Jing, Y., Yang, M., and Du, L. (2018). Gut microbiota dysbiosis in male patients with chronic traumatic complete spinal cord injury. J. Transl. Med. 16:353. doi: 10.1186/s12967-018-1735-9
Zhang, H. Q., Liu, P., Duan, J. A., Dong, L., Shang, E. X., and Ping, X. (2019). Hierarchical extraction and simultaneous determination of flavones and triterpenes in different parts of Trichosanthes kirilowii Maxim. by ultra-high-performance liquid chromatography coupled with tandem mass spectrometry. J. Pharm. Biomed. Anal. 167, 114–122. doi: 10.1016/j.jpba.2019.02.003
Zhang, X. H., Lang, D. Y., Zhang, E. H., and Wang, Z. S. (2015). Effect of autotoxicity and soil microbes in continuous cropping soil on angelica sinensis seedling growth and rhizosphere soil microbial population. Chin. Herbal Med. 7:7.
Zhou, L., Wang, Y., Xie, Z., Zhang, Y., Malhi, S. S., and Guo, Z. (2018). Effects of lily/maize intercropping on rhizosphere microbial community and yield of Lilium davidii var. unicolor. J. Basic Microbiol. 58, 892–901. doi: 10.1002/jobm.201800163
Zhou, N., Qi, W. H., Xiao, G. S., Ding, B., Zhang, H., and Shen, W. (2015). Correlation between distribution of rhizospheric microorganisms and contents of steroidal saponins of Paris polyphylla var. yunnanensis. Zhongguo Zhong Yao Za Zhi 40, 1055–1060.
Zhou, Y. J., Li, J. H., Ross Friedman, C., and Wang, H. F. (2017). Variation of Soil Bacterial Communities in a Chronosequence of Rubber Tree (Hevea brasiliensis) Plantations. Front. Plant Sci. 8:849. doi: 10.3389/fpls.2017.00849
Zhu, S., Guo, S., Duan, J. A., Qian, D., Yan, H., Sha, X., et al. (2017). UHPLC-TQ-MS coupled with multivariate statistical analysis to characterize nucleosides, nucleobases and amino acids in angelicae sinensis radix obtained by different drying methods. Molecules 22:918. doi: 10.3390/molecules22060918
Keywords: Angelica sinensis, rhizosphere microorganism, phthalides, bacterial community, Bacillus
Citation: Feng W-M, Liu P, Yan H, Zhang S, Shang E-X, Yu G, Jiang S, Qian D-W, Ma J-W and Duan J-A (2021) Impact of Bacillus on Phthalides Accumulation in Angelica sinensis (Oliv.) by Stoichiometry and Microbial Diversity Analysis. Front. Microbiol. 11:611143. doi: 10.3389/fmicb.2020.611143
Received: 28 September 2020; Accepted: 10 December 2020;
Published: 08 January 2021.
Edited by:
Valentina Fiorilli, University of Turin, ItalyReviewed by:
Guangxiu Liu, Chinese Academy of Sciences (CAS), ChinaXingang Zhou, Northeast Agricultural University, China
Copyright © 2021 Feng, Liu, Yan, Zhang, Shang, Yu, Jiang, Qian, Ma and Duan. This is an open-access article distributed under the terms of the Creative Commons Attribution License (CC BY). The use, distribution or reproduction in other forums is permitted, provided the original author(s) and the copyright owner(s) are credited and that the original publication in this journal is cited, in accordance with accepted academic practice. No use, distribution or reproduction is permitted which does not comply with these terms.
*Correspondence: Jin-Ao Duan, ZGphQG5qdWNtLmVkdS5jbg==; Hui Yan, Z2xvcnkteWFuQDE2My5jb20=
†These authors have contributed equally to this work